The Robogut: A Bioreactor Model of the Human Colon for Evaluation of Gut Microbial Community Ecology and Function
Connor M. Gianetto-Hill, Connor M. Gianetto-Hill, Sarah J. Vancuren, Sarah J. Vancuren, Brendan Daisley, Brendan Daisley, Simone Renwick, Simone Renwick, Jacob Wilde, Jacob Wilde, Kathleen Schroeter, Kathleen Schroeter, Michelle C. Daigneault, Michelle C. Daigneault, Emma Allen-Vercoe, Emma Allen-Vercoe
Abstract
The human colon is inhabited by a complex community of microbes. These microbes are integral to host health and physiology. Understanding how and when the microbiome causally influences host health will require microbiome models that can be tightly controlled and manipulated. While in vivo models are unrivalled in their ability to study host-microbial interplay, in vitro models are gaining in popularity as methods to study the ecology and function of the gut microbiota, and benefit from tight controllability and reproducibility, as well as reduced ethical constraints. In this set of protocols, we describe the Robogut, a single-stage bioreactor system designed to replicate the conditions of the distal human colon, to culture whole microbial communities derived from stool and/or colonic biopsy samples, with consideration of methods to create culture medium formulations and to build, run, and sample the bioreactor apparatus. Cleaning and maintenance of the bioreactor system are also described. © 2023 The Authors. Current Protocols published by Wiley Periodicals LLC.
Basic Protocol 1 : Growth medium preparation
Support Protocol 1 : Preparing medium supplements
Basic Protocol 2 : Preparing the bioreactor vessels
Support Protocol 2 : Making acid and base bottles
Support Protocol 3 : Preparing the effluent bottles
Support Protocol 4 : Making acid solution
Support Protocol 5 : Making base solution
Basic Protocol 3 : Preparing inoculum and inoculating bioreactors
Alternate Protocol 1 : Preparing inoculum less than 0.5% (w/v) of vessel volume
Alternate Protocol 2 : Preparing synthetic community aliquots and inoculation via the septum
Alternate Protocol 3 : Preparing inoculum from a tissue sample
Basic Protocol 4 : Sampling the bioreactor vessel
Basic Protocol 5 : Harvesting bioreactor vessel contents at end of experiment
Support Protocol 6 : Cleaning and sterilizing sampling needles
Basic Protocol 6 : Cleaning the bioreactor vessel
Support Protocol 7 : Cleaning bioreactor support bottles
INTRODUCTION
The various communities of microorganisms that reside in the gastrointestinal tract are collectively referred to as the gut microbiota and are comprised of bacteria, archaea, fungi, protists, and viruses. One of the most microbially diverse and abundant regions of the gastrointestinal tract of humans is the colon (Human Microbiome Project Consortium, 2012). This region is frequently studied because of the relative ease and minimally invasive method of sampling stool as a proxy for the colonic microbiome. Many studies have examined the composition of stool microbial communities, mainly the bacterial components, in relation to health and disease through the use of various omics techniques. Such studies often yield interesting results that correlate alterations in the gut microbiome function and structure to disease types including autoimmune, metabolic, and neurological diseases (Vijay & Valdes, 2022).
The myriad of microbes found within the gut microbiome interact extensively with each other as well as the host. These complex interactions can make it challenging to assess the effects of perturbations (for example, diet change or xenobiotic addition) on microbial community composition and function. One way to reduce the complexity of the system is to study the microbial communities in isolation of the host, within an in vitro system which can be tightly controlled and manipulated. Under these conditions, specific elements of host interaction can be introduced (such as host mucins and immune system components) and there is a reduced requirement for ethical oversight. Microbial communities supported by in vitro models can thus be studied under very specific conditions, and over time series experiments, to enable deep insight into microbial ecology in this niche. As such, in vitro models are rising in popularity for studies of gut microbial community function.
Many different in vitro models of the gut microbiome have been described. Some systems focus on recreating the environment of distinct sections of the human gastrointestinal (GI) tract (for example, the ascending, transverse, and distal colon) through the use of a three-stage continuous culture system (Macfarlane et al., 1998). Other systems go further to replicate compartments of the GI tract from stomach to colon, for example the Simulator of the Human Intestinal Microbial Ecosystem (SHIME) reactor (Molly et al., 1993), which has been further modified to assess the mucosal associated microbiome through the incorporation of mucin (M-SHIME; Van den Abbeele et al., 2012). Further complexity can also be introduced, for example, the TNO Intestinal Model 2 (TIM-2) system replicates the absorption of microbial metabolites by the host through the use of dialysis membranes and peristaltic pumping (Minekus et al., 1999). However, while it is clear that complexity can be built into in vitro model systems of the gastrointestinal tract, there is still much to be learned from studying the colonic microbiome alone, and to do this, simple, single-stage continuous culture bioreactor systems have been shown to support reproducible and representative distal colonic communities (McDonald et al., 2013). The protocols described here provide details for culturing whole microbial communities from human stool samples in a single-stage continuous culture bioreactor model emulating the conditions of the human distal colon. Although these protocols have been developed specifically for the adult human colonic microbiome, they can be adapted to model other regions and different life stages of the gastrointestinal tract of humans and other animals.
Basic Protocol 1 describes the preparation of growth medium to be used in the model bioreactor to replicate the nutrients found in the human colon. Support Protocol 1 describes the process of preparing and adding medium supplements to the bioreactor system. Basic Protocol 2 describes building the bioreactor vessel. Support Protocols 2 through 5 describe the preparation of acid/base additions and effluent bottles. Basic Protocol 3 describes how to prepare human fecal material for addition to the bioreactor vessels, including details of vessel inoculation. Alternate Protocol 1 describes how to prepare and add inoculum when biomass of samples are limited. Alternate Protocol 2 describes how to prepare a synthetic community and inoculate via the bioreactor septum. Alternate Protocol 3 describes how to prepare and add an inoculum from a tissue sample. Basic Protocol 4 describes how to aseptically sample bioreactor vessels throughout a given experiment. Basic Protocol 5 describes how to harvest the entire contents of a vessel at the end of an experiment. Support Protocol 6 describes how to properly clean needles following sampling. Basic Protocol 6 describes thorough cleaning protocols for the bioreactor vessels and Support Protocol 7 describes cleaning the effluent, acid, and base bottles. Schematic overviews are provided for main materials (Fig. 1) and assembly steps (Fig. 2) described in Basic Protocols 1 and 2 and Support Protocols 1 through 5.
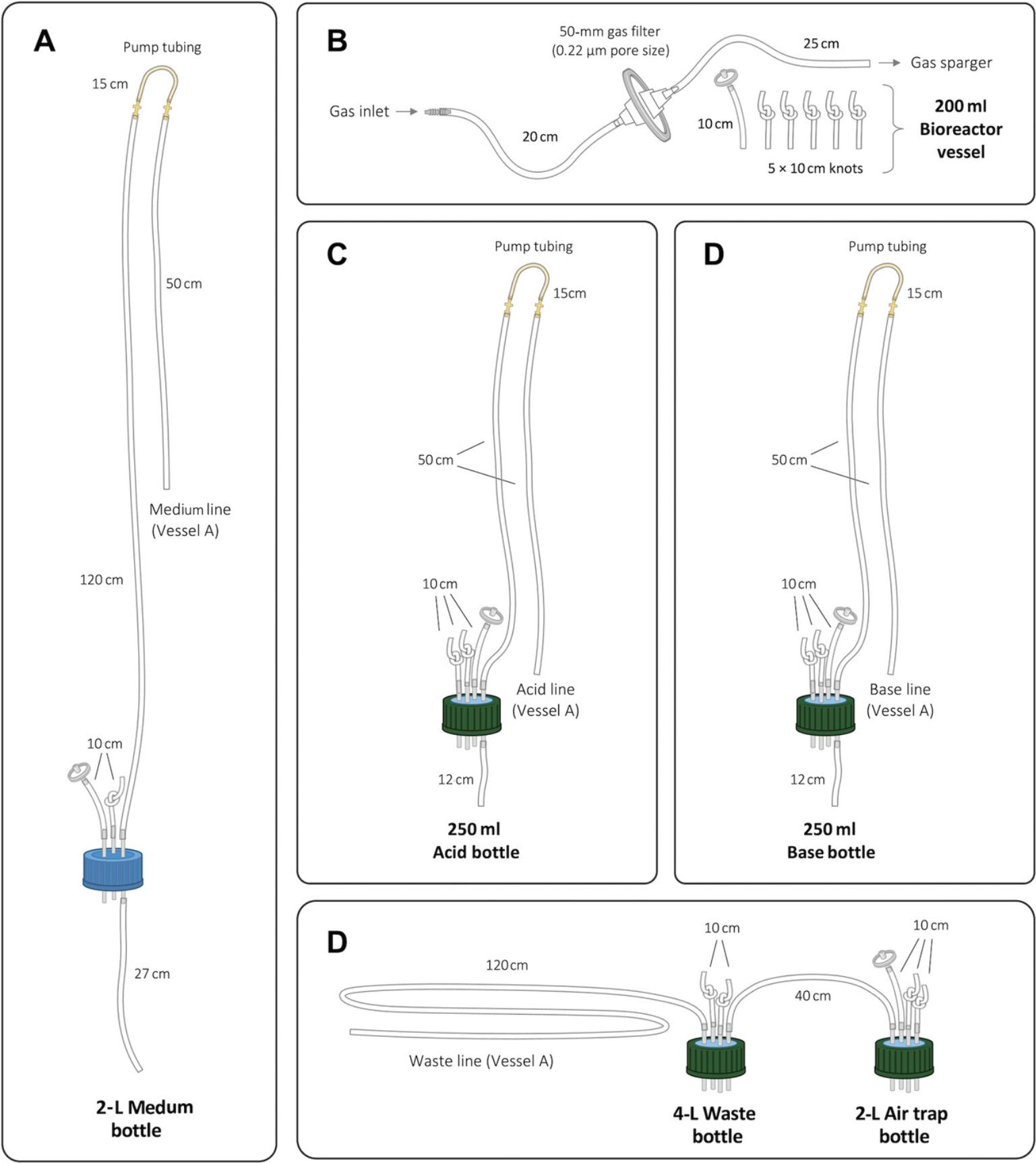
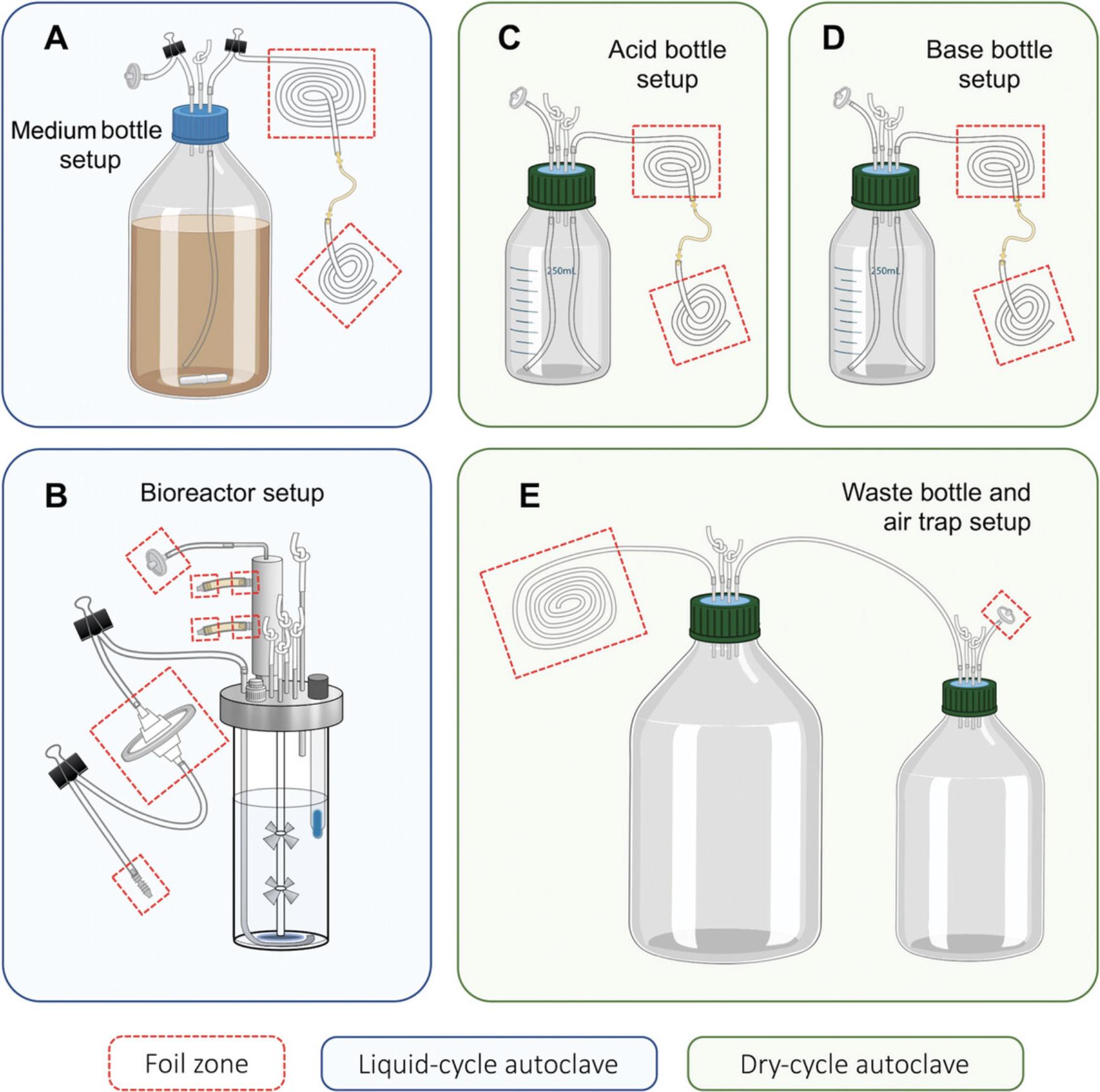
Biosafety Considerations
CAUTION : Human fecal specimens are potentially biohazardous. The appropriate Institutional Biosafety Review Board should be consulted before beginning these protocols. At the University of Guelph, research with human fecal specimens is conducted under Biosafety Level 2 (BSL-2). This includes use of lab coats, nitrile gloves, safety goggles, and steps to minimize the risk of injury involving sharps. Waste material should be placed in a biohazard bag and autoclaved or incinerated. Liquids should be decontaminated by autoclaving or by adding 10% (v/v) bleach as appropriate. Surfaces and equipment should be decontaminated with a freshly prepared bleach solution (10%, v/v, in water).
Human-Derived Materials
CAUTION : Research with human fecal samples requires approval of the appropriate Institutional Ethical Review Board and consent from donors. Follow all appropriate guidelines and regulations for the use and handling of human-derived materials.
Basic Protocol 1: GROWTH MEDIUM PREPARATION
The preparation of growth medium is a critical step to any experiment aiming to culture and model complex microbial communities. The medium formulation used in this protocol has evolved through successive iterations and originates from the formulation first described by Macfarlane et al. (1998) and modified by McDonald et al. (2013). There are, however, no standard media to grow microbial communities from human stool. It is important then, to consider the goals of an experiment before designing a medium type to use. (Supplemental Table S1 provides a comparison of medium components used in similar in vitro model systems from the published literature to help guide the reader in this aspect of experimental design.) Once a formulation is established, it is also important to remain consistent with its use throughout a given experiment, to avoid introducing confounders that would make experimental run comparisons challenging. As such, we recommend calculating the amounts of medium components required for an experimental series, and sourcing enough prior to starting work, to minimize any lot-to-lot variation from component manufacturers. Here we provide details of a complex medium that is designed to generally replicate nutrient conditions found in the adult human colon. The protocol is presented for the production of a 2-L volume, but depending on the experimental protocol planned, multiple bottles may be required. In this case, medium production can be scaled up to produce several 2-L bottles at a time, and prepared medium can be stored for ∼2 weeks at 4ºC.
This protocol describes creating culture medium with the intention of adding it steadily with a flow rate set to allow for a 24-hr retention in the bioreactor vessel. It is also possible to set the pumps to pulse medium into the system at set intervals, though these procedures will not be covered in depth here. Researchers are recommended to consult the user manual for the bioreactor system they intend to use.
This protocol uses reagents that are either soluble or, if insoluble, have particle sizes small enough to pass through Infors Multifors pump hoses with an interior diameter of 1 mm. Depending on the application, it may be important to include reagents with larger particle sizes that may not be suitable for the tubing sizes described here. In this situation, it is possible to use external pump systems to the built-in pump mechanisms on the Infors Multifors 2 system with larger tubing and pump hose sizes to overcome this limitation or to add such components as supplements pulsed into the system via an extraneous addition port that is not otherwise used by the system, as described in Support Protocol 2.
Materials
-
Oxoid peptone water (Thermo Fisher Scientific, cat. no. CM009)
-
Bacto™ yeast extract (Thermo Fisher Scientific, cat. no. 212750)
-
Sodium bicarbonate (NaHCO3), Powder/Certified ACS (Thermo Fisher Scientific, cat. no. S233-3)
-
Pectin from citrus peel (MilliporeSigma, cat. no. P9135)
-
Xylo-oligosaccharide (e.g., Bio Nutrition Pre-Biotic with Life Oligo Prebiotic Fiber XOS)
-
Arabinogalactan (e.g., ARA 6 - Pure Larch Powder; Genoma)
-
Starch from wheat, unmodified (MilliporeSigma, cat. no. S5127)
-
Casein (Alfa Aesar, cat. no. A13707)
-
Inulin (Glentham Life Sciences, cat. no. GC4090)
-
Sodium chloride (NaCl; Thermo Fisher Scientific, cat. no. BP358-1)
-
Porcine gastric mucin (Lee Biosolutions, cat. no. 435-11)
-
Bile salts (Millipore Sigma, cat. no. B8756)
-
L-Cysteine hydrochloride monohydrate (Thermo Fisher Scientific, cat. no. BP376)
-
Potassium phosphate dibasic anhydrous (K2HPO4; Thermo Fisher Scientific, cat. no. P290)
-
Potassium phosphate monobasic (KH2PO4; Thermo Fisher Scientific, cat. no. P285)
-
Magnesium sulfate (MgSO4; VWR, cat. no. 7487-88-9)
-
Calcium chloride (CaCl2; MilliporeSigma, cat. no. C4901)
-
Hemin solution (see recipe)
-
Menadione solution (see recipe)
-
Deionized water
-
2 M NaOH
-
Silicone Laboratory Tubing Internal Diameter (ID) 3.18 mm, Outer diameter (OD) 6.35 mm (Thermo Fisher Scientific, cat. no. 11-189-15L)
-
Aluminum foil
-
PharMed pump hose 1-mm diameter, 15 cm long (Masterflex, cat. no. RK-06508-13)
-
Polypropylene pump fittings, 1/8 in. × 3/32 in. (Cole-Parmer, cat. no. RK-06365-66)
-
GL45 screw cap with three fitted metal pipes, ID 2 mm, OD 4 mm, length 6 cm (BOLA, cat. no. D606-08)
-
Autoclavable 25-mm 0.22-µm filter (Thermo Fisher Scientific, cat. no. 09-719C)
-
2-L glass bottle with 30-mm wide neck (DWK Life Sciences, cat. no. KC143952000)
-
Analytical balance (e.g., OHAUS, cat. no. 30529239)
-
Reagent scoops
-
Weighing boats
-
2-L graduated cylinder (Thermo Fisher Scientific, cat. no. 03-007-45)
-
Large magnetic stir bar, 76-mm length × 12-mm diameter (VWR, cat. no. 58948-193)
-
Magnetic stir plate (Thermo Fisher Scientific, cat. no. 11676265)
-
Metal bulldog clips (32 mm)
-
Autoclavable bucket tray, 54.3-cm length × 43.5-cm width × 13-cm height (Thermo Fisher Scientific, cat. no. 6900-0020)
-
50-ml serological pipets (GeneBio Systems, cat. no. MSP5001)
-
Pipet filler
-
Sterile 1-L, glass bottle with 30-mm wide mouth and cap (DWK Life Sciences, cat. no. KC143951000)
-
0.22-µm bottle top filter (Thermo Fisher Scientific, cat. no. 595-3320)
-
Spray bottle containing 70% ethanol solution
-
Class II laminar flow hood equipped with UV light
-
2 × 500-ml glass beakers (VWR, cat. no. 13912-240)
-
Small magnetic stir bars, 38-mm length × 12-mm diameter (VWR, cat. no. 58947-138)
-
Disposable Pasteur pipets (Thermo Fisher Scientific, cat. no. 13-678-20C)
For each 2-L bottle of medium being prepared:
1.Prepare tubing by cutting two 10-cm, one 27-cm, one 50-cm, and one 120-cm pieces of silicone tubing. Tie a knot 1 cm from one end of one of the 10-cm pieces. Cut one 10-cm × 10-cm and two 25-cm × 15-cm pieces of aluminum foil.
2.Wrap one end of the 50-cm long tubing with the 10- × 10-cm piece of aluminum foil. Place one of the 25- × 15-cm pieces of aluminum foil on a flat surface and place flat onto the middle of the foil a coiled piece of the 50-cm tubing with foil covering one end, leaving the other end outside of the foil (Fig. 3). Fold the sides and bottom of the foil as shown by the dashed black lines in Figure 3A and 3B. Fold the last corner of the foil towards the center as shown in Figure 3C. The final prepared tubing (Fig. 3D) is referred to as the short tubing.
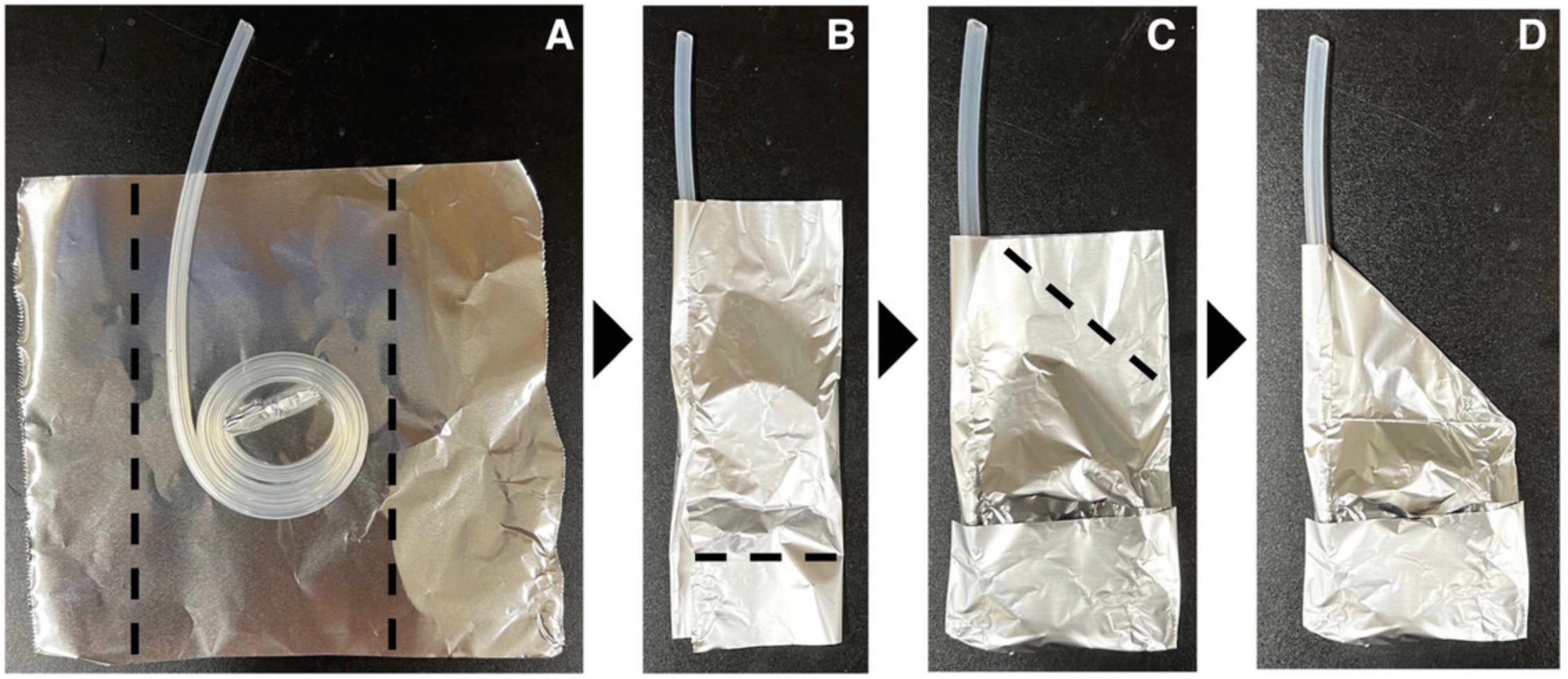
3.Place the other 25- × 15-cm piece of aluminum foil on a flat surface. Make a flat coil with the 120-cm length of tubing such that the ends of the tubing are not on the aluminum foil as shown in Figure 4A. Fold the foil to hold the tubing in place as shown by the black dashed lines in Figure 4B and 4C. The final prepared tubing (Fig. 4D) is referred to as the long tubing.
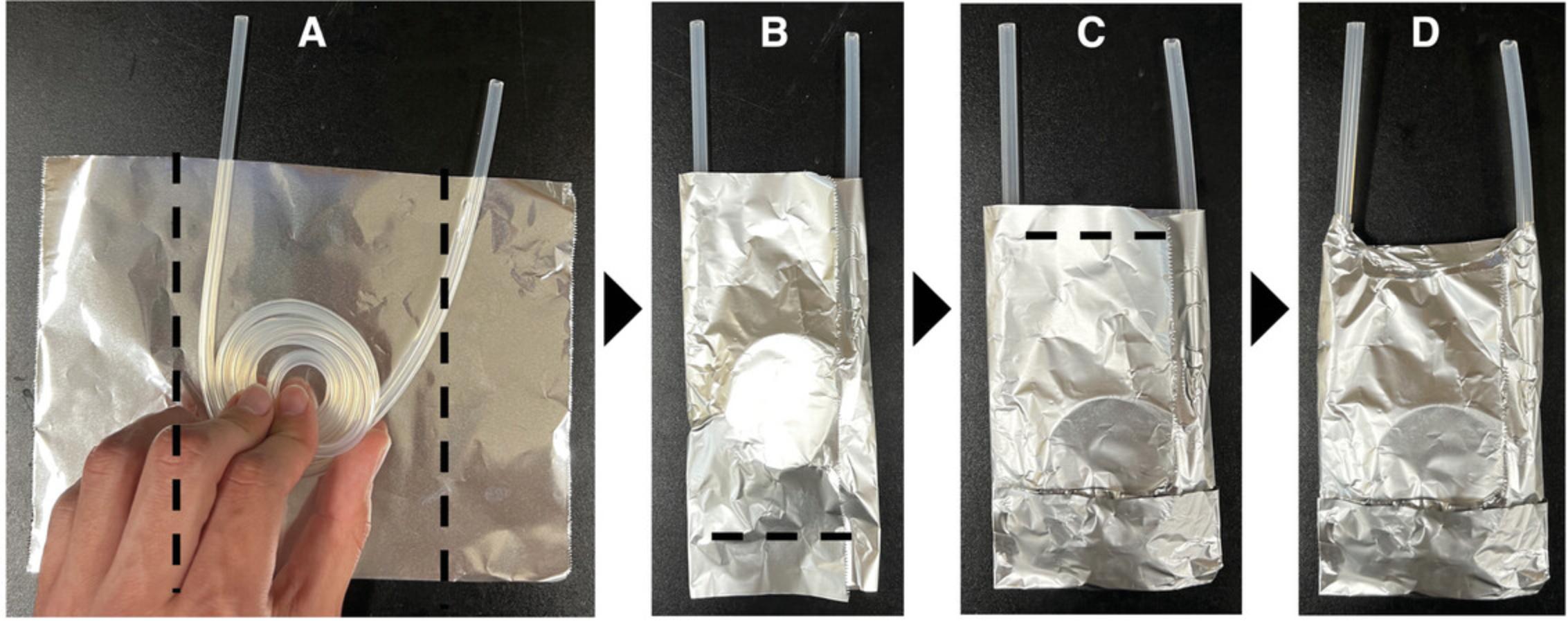
4.Attach pump fittings to the pump hose. Attach one end of the pump hose and fitting to the exposed end of the prepared short tubing and the other end of the pump hose to one end of the prepared long tubing via the pump fitting (Fig. 5A).
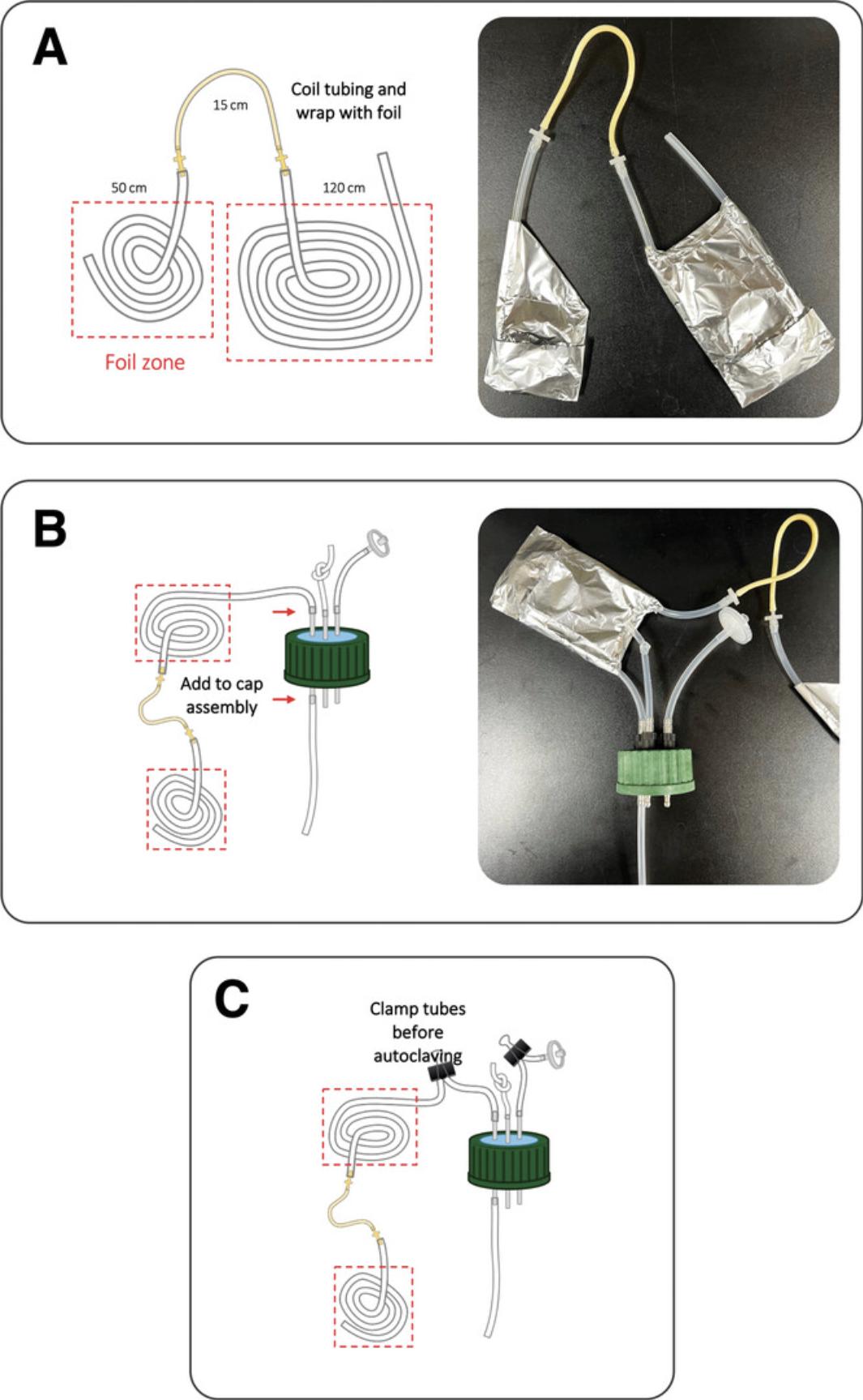
5.Connect the other end of the prepared long tubing to one of the ports of a three-port bottle cap (Fig. 5B). To this same metal port but on the opposite side (pointing into the bottle when attached) connect the 27-cm tubing (Fig. 5B).
6.Attach the knotted and unknotted 10-cm tubing lengths to the other two remaining ports on the bottle top so that they extend outside the bottle when the lid is on (Fig. 5B). Attach a 25-mm 0.22-µm filter to the end of the unknotted 10-cm tubing piece. Wrap filter and tubing with the prepared 10- × 10-cm piece of foil. Set all prepared tubing, filter, and bottle lid to one side as shown in the schematic diagram (Fig. 5C).
7.To a clean 2-L bottle, add 4 g peptone water, 4 g yeast extract, 4 g NaHCO3, 4 g pectin, 4 g xylo-oligosaccharide, 4 g arabinogalactan, 10 g starch, 6 g casein, 2 g inulin, 0.2 g NaCl, and 8 g porcine mucin powders. Weigh each medium component separately, using an analytical balance.
8.Add 1,800 ml deionized water to the medium bottle using a graduated cylinder.
9.Add the 76-mm stir bar into the bottle, place on a stir plate, and mix medium until a uniform suspension is obtained (∼30 min). During mixing, screw the prepared bottle cap with connected tubing onto the bottle, leaving it loose by one turn (to allow steam to escape during autoclaving).
10.Using three metal bulldog clips, fold over and clamp the tubing connected to the filter; the prepared long tubing; and the prepared short tubing (Fig. 5C).
11.Autoclave the prepared bottle at 121°C for 60 min (hereafter referred to as ‘liquid 60’; or as appropriate for the autoclave instrument used) to sterilize the medium.
12.Remove from the autoclave and allow sterilized medium to cool by placing at room temperature. Once cool, tighten the lid on the bottle.
13.Using the analytical balance, weigh 1 g bile salts into a 500-ml beaker (Beaker 1) and add 50 ml deionized water. Add a 38-mm magnetic stir bar to the beaker and mix on magnetic stir plate until fully dissolved.
14.Using the analytical balance, measure 1 g L-cysteine HCl, 0.08 g K2HPO4, 0.08 g KH2PO4, 0.02 g MgSO4, and 0.02 g CaCl2. Add these components to a second 500-ml beaker (Beaker 2).
15.Add 2 ml hemin solution and 2 ml menadione solution to Beaker 2.
16.Add 146 ml deionized water to Beaker 2.Add a 38-mm magnetic stir bar and stir on a stir plate until components are fully mixed.
17.Slowly add the bile salts solution from Beaker 1 into Beaker 2 while stirring.
18.Using a Pasteur pipet, add 2 M NaOH solution dropwise to the suspension until the precipitate is dissolved and a solution results.
19.Spray surfaces of a wrapped, sterile 50-ml glass pipet, a bottle top filter, a pipet filler or motorized pipet controller, and a sterilized 1-L bottle with 70% ethanol and place into a class II laminar flow cabinet. Irradiate under UV light for 15 min. Filter-sterilize the prepared medium addition solution from Beaker 2 into a sterilized 1-L bottle using the bottle-top filter.
20.Spray surface of the autoclaved and cooled 2-L medium bottle (from step 12) with 70% ethanol and bring into the class II laminar flow hood.
21.Unwrap serological pipet and aseptically transfer all 200 ml of the filter-sterilized solution to the 2-L bottle. Recap 2-L bottle and use prepared medium immediately or store at 4 ºC for up to 2 weeks.
Support Protocol 1: PREPARING MEDIUM SUPPLEMENTS
This protocol describes the process for creating and adding medium supplements to the bioreactor for components that are either volatile at room temperature for extended periods of time, are insoluble and have particulates too large to pass through pump fittings, or if there is an experimental interest in pulsing a reagent into the bioreactor system at specific time points. This method makes supplements in advance of a bioreactor experiment by storing in ready-to-use syringes, making use of the extra addition port on the vessel lid (Fig. 6) to pulse in supplements, followed by a water rinse to clear the port.
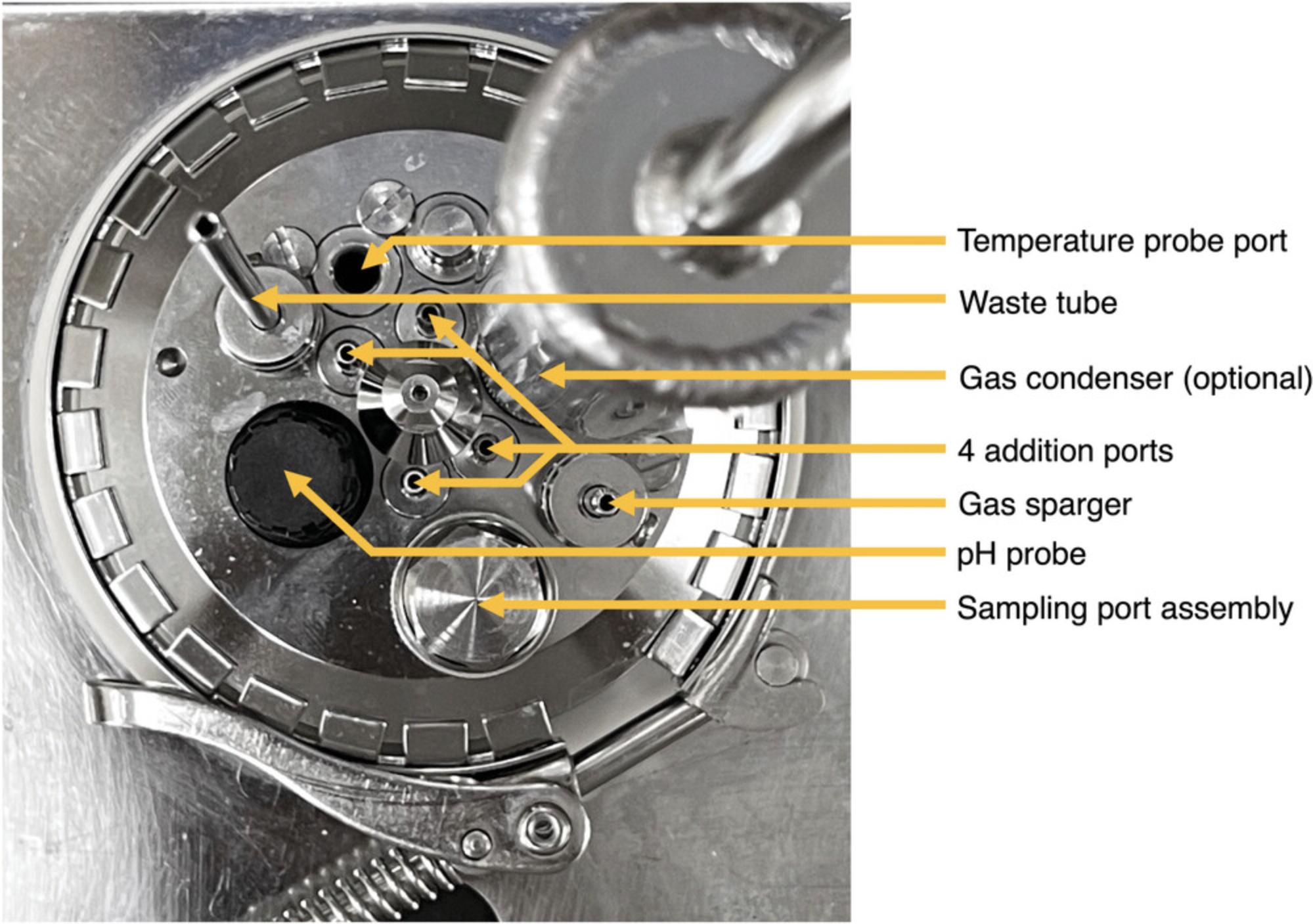
Materials
-
Supplements such as wheat bran (Bob's Red Mill) or cellulose (Thermo Fisher Scientific, cat. no. A17730)
-
Silicone Laboratory Tubing Internal Diameter (ID) 3.18 mm, Outer diameter (OD) 6.35 mm (Thermo Fisher Scientific, cat. no. 11-189-15 L)
-
Aluminum foil
-
Analytical balance (e.g., OHAUS, cat. no. 30529239)
-
Reagent scoops
-
Weighing boats
-
250-ml glass bottles with GL45 screw caps (Corning, cat. no. 1395-250)
-
GL45 screw cap with 1-3 fitted metal pipes (ID 2 mm, OD 4 mm, length 6 cm; BOLA, cat. no. D606-08)
-
Metal bulldog clips (32 mm)
-
Sterile, single-wrapped 5-ml Luer-Lok syringes (BD, cat. no. 309646)
-
Small magnetic stir bar, 38-mm length × 12-mm diameter (VWR, cat. no. 58947-138)
-
Spray bottle containing 70% ethanol solution
-
Class II laminar flow hood equipped with UV light
When preparing vessels that you know will require some form of medium supplementation through the excess port, plan ahead so that the spare addition port is the most easily accessible (Fig. 6), especially if additions are being done daily.
1.Prepare tubing by cutting two 10-cm pieces of tubing for each supplement being created to account for the supplementation syringe and the water rinse syringe. Knot one end of the tubing and sterilize in a dry 20 autoclave (or appropriate cycle for the autoclave instrument).
2.Prepare two GL45 bottle lids by cutting four 12-cm pieces of tubing and enough 10-cm pieces of tubing to account for excess ports on the lid. Knot the 10-cm pieces to block the extra ports. Place a 12-cm length of tubing on each side of one port. Place foil on the end of the 12-cm length of tubing on the top of the bottle lid to keep it sterile, clipping it 3 cm from the prong.
3.Prepare the medium component that is to be supplemented into the system and place in a 250-ml bottle (or appropriate size to accommodate dry weight and water volume). Weigh enough of the component to match how many supplement additions you will be making to the bioreactor system.
4.Add deionized water to a volume to match the desired concentration for each supplement addition you will be making.
5.Add a small magnetic stir bar to the bottle, screw on the prepared bottle lid from step 2 (leaving loose to a turn for steam to escape), and autoclave at 121°C for 30 min (hereafter referred to as ‘liquid 30’; or appropriate cycle for the autoclave instrument and volume) to sterilize.
6.Prepare a second bottle of sterile water with 3 ml water per supplement. Attach the second screw on the prepared bottle lid from step 2 (leaving loose to a turn for steam to escape) and sterilize by autoclaving in a liquid 30 cycle (or appropriate cycle for the autoclave instrument and volume) to sterilize.
7.Once cooled, spray the surfaces of the bottles containing the supplement mixture and sterilized water, the container with the sterilized knotted tubing, wrapped 5-ml Luer-Lok syringes, and a magnetic stir plate with 70% ethanol and move into a laminar flow hood. Expose to UV light for 15 min.
8.Place supplement mixture on the stir plate until a homogenous mixture is obtained.
9.Carefully remove foil and clip from the tubing on the lid of the bottle with the supplement mixture while it is still on the stir plate. Aliquot supplement mixture aseptically into the Luer-Lok syringes by unwrapping a sterile syringe and attaching it to the exposed end of tubing on the supplement bottle. Fill syringe to the desired volume, and while the syringe is still attached to the bottle tubing, invert it and push excess air out. Detach syringe from the bottle tubing and replace it with a sterile piece of knotted tubing as prepared in step 1.Supplement syringes should be stored in a clean container for no longer than 1 month to prevent chances of contamination.
To prepare the water syringes, follow the instructions from step 9.It can be useful to leave a small air pocket in the syringe prior to adding the knotted tubing on the water syringes to help rinse out the port when the supplement is being added to the bioreactor system.
10.To add the prepared supplement to the bioreactor systems, lay the supplement and water syringe horizontally on a clean piece of paper towel and spray it thoroughly with 70% ethanol to disinfect the surface.
11.Spray the remaining addition port (with the remaining piece of knotted tubing) and a pair of scissors with 70% ethanol. Remove clamp from the condenser so pressure can escape while adding the supplement to the bioreactor system.
12.Carefully cut the knotted tubing close to the knot that is on the addition port. Quickly remove the knotted tubing from the tip of the supplement syringe and affix it to the tubing left on the addition port. Slowly push syringe contents into the vessel.
13.To swap the supplement syringe for the water syringe, invert the supplement syringe that is still connected to the addition port and remove it. Quickly take the knotted tubing off the water syringe, placing the tubing back on the paper towel from step 10, and affix the syringe to the tubing on the addition port. Slowly push a small amount of water through the port (the entire 3 ml is not often required) to clear it of any remaining debris from the supplement and pull up on the plunger to fill it with gas.
14.Spray tubing that is still attached to the water syringe and addition port, and a razor blade with 70% ethanol.
15.Carefully cut tubing from the addition port with the razor blade to remove it. Quickly replace with the knotted tubing from the water syringe (set aside in step 13) to the addition port. Re-clamp the condenser.
Basic Protocol 2: PREPARING THE BIOREACTOR VESSELS
This protocol describes the steps taken to build the bioreactor vessel. It is specific to the Infors Multifors 2 bioreactor system but can easily be adapted to similar systems with modifications. The Multifors 2 system is controlled by a central processing unit which does not require any further software to control the system. However, if desired, additional software can be used to monitor a given run. The main purpose of this protocol is to describe a method to prepare a bioreactor vessel for use while maintaining sterility.
This protocol does not use antifoam; in our experience using the conditions described in this protocol, vessel contents do not create enough foam to become problematic during experimental runs.
The Infors Multifors 2 system can be run with an O2 sensor probe but for the anaerobic application described here, such a probe it is not required, and its use will not be described in this protocol.
Materials
-
pH 10.00 buffer solution (Thermo Fisher Scientific, cat. no. BPSB115500)
-
pH 4.00 buffer solution (Thermo Fisher Scientific, cat. no. SB101500)
-
Deionized water
-
Compressed nitrogen gas
-
70% ethanol solution
-
Silicone Laboratory Tubing Internal Diameter (ID) 3.18 mm, Outer diameter (OD) 6.35 mm (Thermo Fisher Scientific, cat. no. 11-189-15L)
-
Multifors 2 microbial system (0.4L TV, with 0.4L TV NW55/70 culture vessel; Infors HT)
-
Autoclave safe 50-mm 0.22-µm filter (Millipore Sigma, cat. no. SLFG05010)
-
EasyFerm Plus pH probe (Hamilton, cat. no. 238633-1513)
-
150-ml beakers (VWR, cat. no. 13912-182)
-
Self-healing silicon septum fitted into a septum collar (Infors HT)
-
Three metal bulldog clips (32 mm)
-
Aluminum foil (6- × 3- × 5-cm, 1- × 25- × 15-cm, and 1- × 10- × 10-cm sheets)
-
Autoclavable 25-mm, 0.22-µm filter (Thermo Fisher Scientific, cat. no. 09-719C)
-
Magnetic stir plate (Thermo Fisher Scientific, cat. no. 88880009)
1.Place glass bioreactor vessel into the vessel holder. Organize the layout of the vessel ports as depicted in Figure 6.The sampling port should be positioned such that it is furthest away from the center and will be closest to the user during operation (Fig. 6). The pH probe should be placed in one of the two ports that is closest to the center so that the probe is not touching the side of the vessel.
2.Configure vessel components below the lid as shown in Figure 7.The sparger ring should fit above the centering bearing and the magnetic coupling (Fig. 7). Adjust the position of the gas sparger by loosening the screw in the clamping adapter to allow the sparger to spin and move up and down. Once placed, tighten the screw to hold in place.
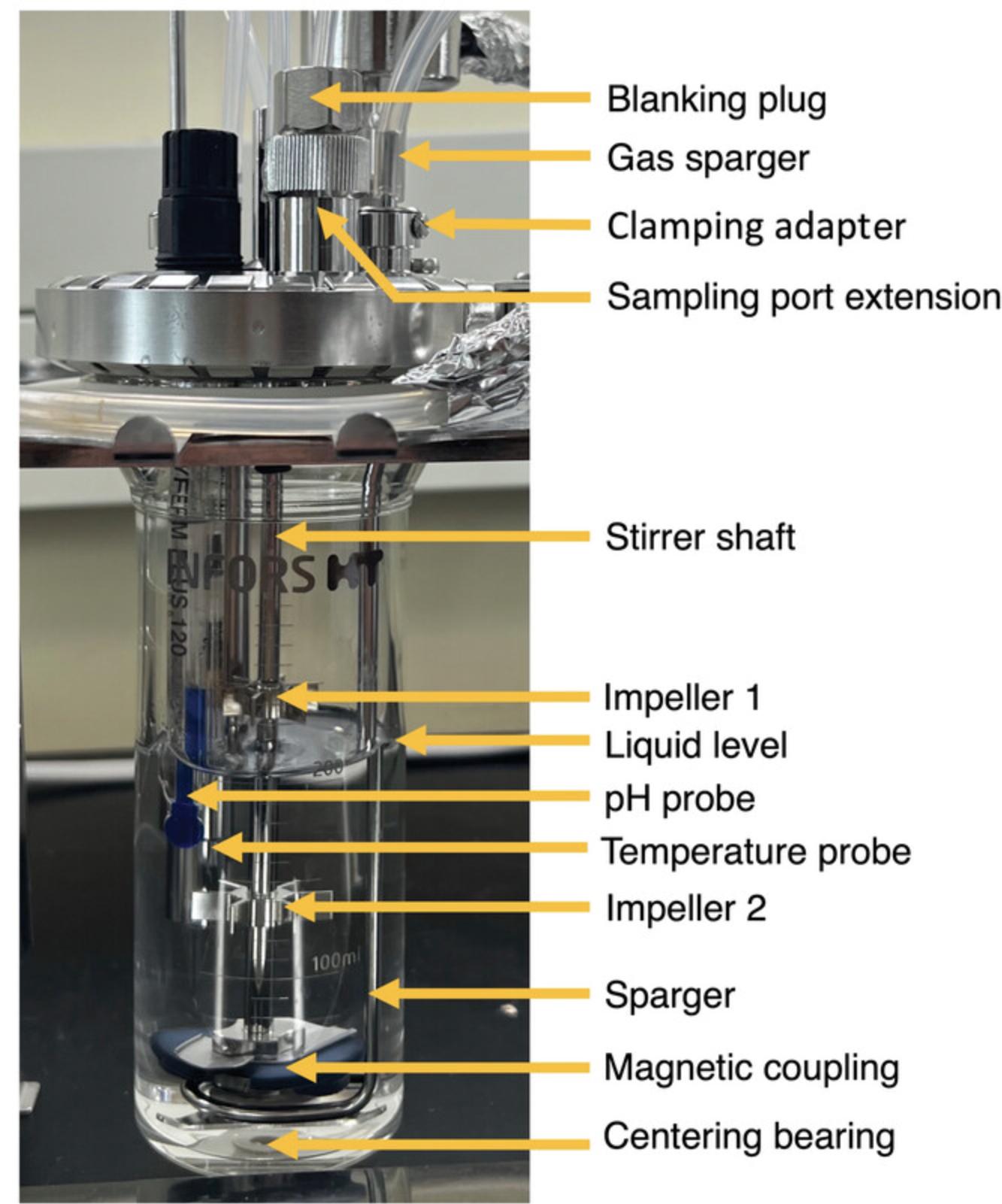
3.Adjust the two impellers on the stirrer shaft to the desired positions by loosening the screw located on each and tightening once in position (Fig. 7).
4.Place the lid on the glass vessel and close the quick-release clamp fastener.
5.Cut four 10-cm pieces of silicone tubing and tie a knot 3 cm from one end of each.
6.Place the untied ends of the tubing onto each of the four addition ports labeled in Figure 6; these will later be used for acid, base, medium, and as a spare.
7.Cut two 10-cm, one 20-cm, and one 25-cm pieces of silicone tubing.
8.Using scissors, cut aluminum foil into six 3-cm × 5-cm, one 25-cm × 15-cm, and one 10-cm × 10-cm strips.
9.Tie a knot 3 cm from one end of one of the 10-cm tubing pieces. Place the open end of this knotted tubing over the metal effluent pipe on the top surface of the lid (see diagram in Fig. 8A).
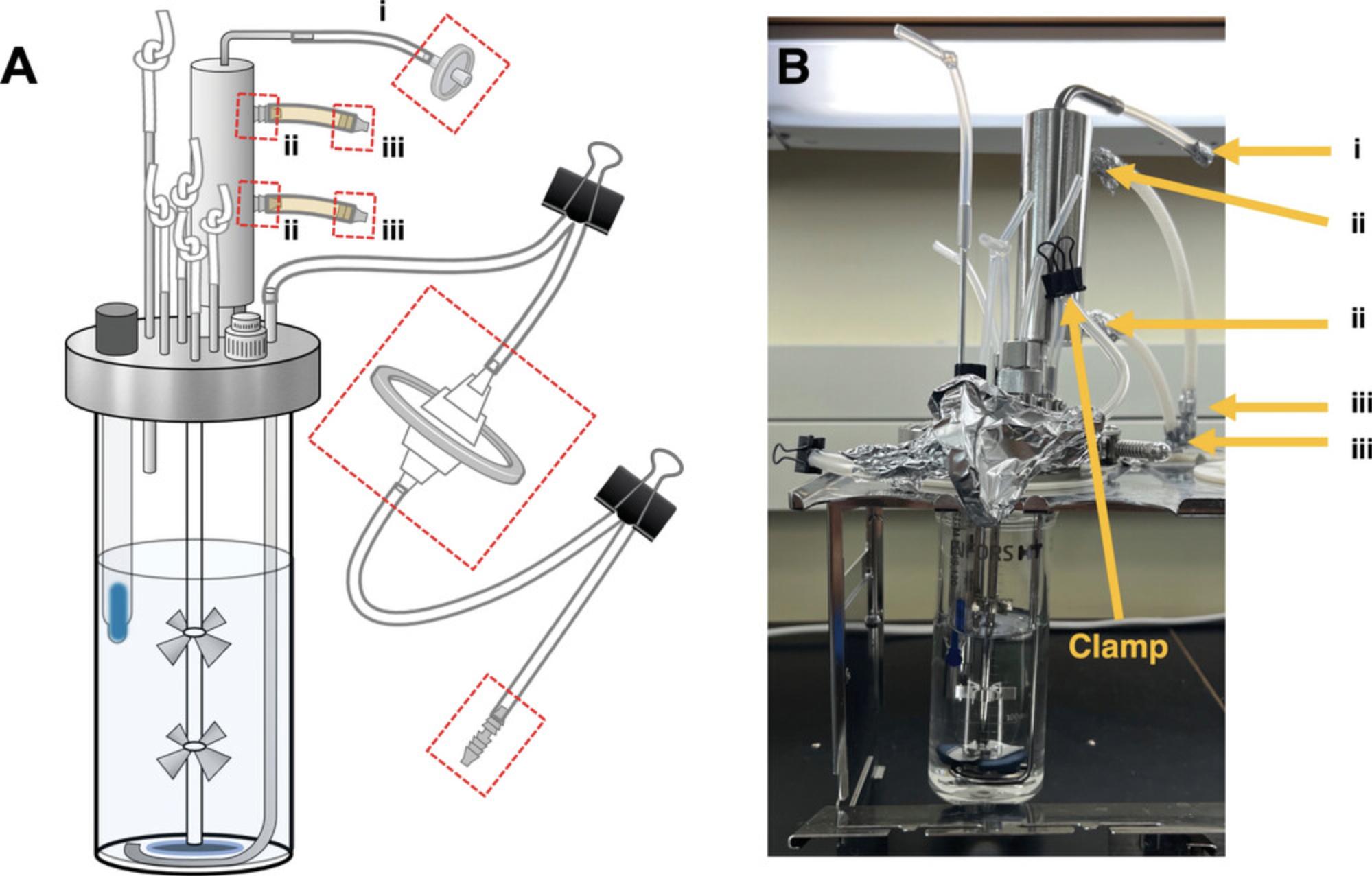
10.Lower the metal effluent tube by loosening the screw in the clamping adapter and position the bottom of the effluent pipe to the bottom of the vessel (Fig. 8). Tighten the screw once the waste pipe is in position.
11.Place one end of the other 10-cm tubing around the opening of the condenser vent. For autoclaving, wrap the end of the condenser tubing with a piece of the 3- × 5-cm aluminum foil.
12.Remove sampling port extension and blanking plug from the lid of the vessel and place a self-sealing septum inside a septum collar to seal the hole. Replace sampling port extension and tighten until just hand tight. Loosely screw the blanking plug closed.
13.To prepare the gas line, insert sparger tubing connector to one end of the 20-cm length of tubing (Fig. 9A) and the 50-mm 0.22-µm filter to the other end (Fig. 9B). Attach one end of the 25-cm length of tubing to the other side of the large filter (Fig. 9B) and the other end to the gas sparger (Fig. 6).
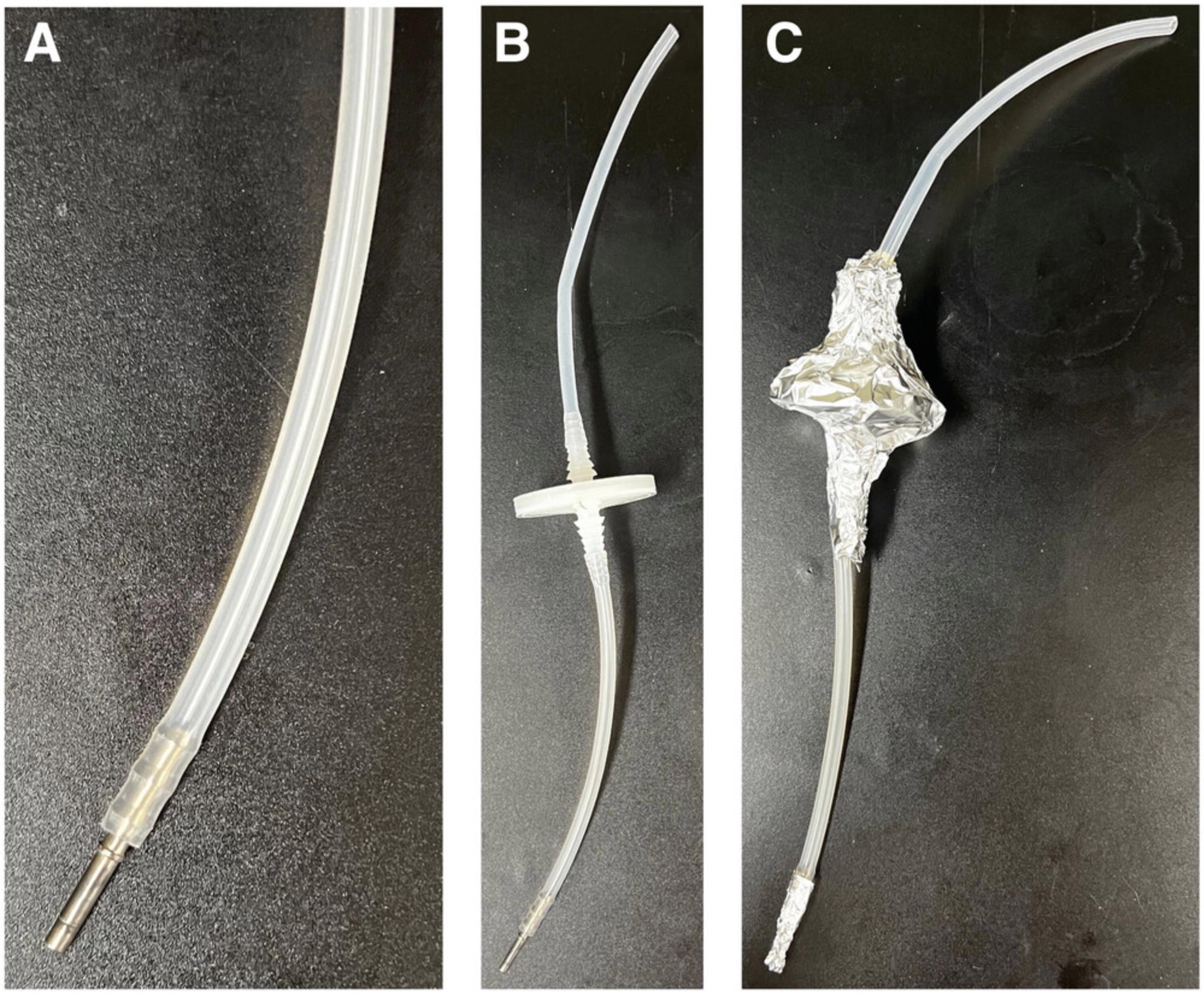
14.Fold over and clamp tubing between the sparger and 50-mm 0.22-µm filter and between the filter and the sparger tubing connector with bulldog clips (Fig. 8A).
15.Wrap gas line 50-mm 0.22-µm filter with the 25- × 15-cm rectangle of aluminum foil to prevent moisture leaching into the filter during autoclaving (Fig. 9C).
16.Using the 3- × 5-cm aluminum foil strips, wrap the ends of the tubing labeled i, ii, and iii in Figure 8B.
17.To calibrate the pH probe, add 100 ml of pH 4 buffer solution to one 150-ml beaker and 100 ml of pH 10 buffer solution to another 150-ml beaker. Calibrate pH probes according to manufacturer's directions. Brief directions for the use of indicated probes with the Infors Multifors 2 system are provided below.
-
For analogue Hamilton branded probes, navigate to the Batch menu. Make sure the previous run is ended under Bioreactor Operation and then select calibrate pH.
-
Attach pH probe to the pH probe sensor leads and rinse the storage buffer from the probe with deionized water.
-
First place pH probe in the pH 4 solution beaker and allow pH reading to equilibrate such that the mV reading does not change more than 0.1 over a 30-s period (this will take ∼5-10 min depending on the sensor quality).
-
Once the pH 4 calibration has been accepted, rinse probe with deionized water, and place into the pH 10 solution, repeating step 17c.
-
Once calibration is complete, be sure to save the calibrated values on the CPU. The pH probe can now be detached from the Infors system CPU leads and the probe cap can be replaced for autoclaving.
-
Screw calibrated pH probe into the pH probe port on the bioreactor vessel lid.
18.Fill bioreactor vessel with 200 ml deionized water to submerge the pH probe septum.
19.Wrap a 25-mm filter with the 10- × 10-cm aluminum foil sheet.
20.Place vessels with tubing and foil-wrapped filter in an autoclavable tray and run on a liquid 30 cycle (or as appropriate for autoclave used).
21.Remove sterilized vessels and foil-wrapped filter from the autoclave and place immediately into a class II laminar flow cabinet.
22.Unwrap autoclaved filter from the aluminum foil and remove foil from the end of the tubing connected to the top of the condenser. Aseptically attach filter to the end of this tubing.
23.Remove vessel from the laminar flow cabinet and transfer to the Multifors 2 instrument base.
24.Attach sparger tubing connector to the unit, insert temperature probe into the probe port (Fig. 6), and connect pH probe to the lead on the control unit.
25.From the Batch menu on the Infors system, start the run underneath the Bioreactor Operation section and select turn on stirring in the vessels to 50 rpm. Other controllers can be accessed via the Controller menu. Monitor the temperature reading from the Main or Controller menus and allow vessel contents to cool below 40°C before progressing.
26.Turn on the gas flow into the vessel and unclamp bulldog clips on the gas sparger tubing before and after the 50-mm 0.22-µm filter. Adjust gas flow so the float in the variable area flowmeter attached to the Multifors 2 is raised ∼1 mm. A stream of bubbles should be visible in the vessel.
27.Unwrap the long effluent tubing connected to the effluent bottle (prepared in Support Protocol 3; Fig. 2E), while ensuring the end of the tubing remains wrapped to maintain sterility.
28.Spray razor blade and knotted tubing on the metal effluent tube of the vessel lid (Fig. 8) with 70% ethanol and make a small slit in the knotted tubing where it meets the connector. Remove and discard knotted tubing. From the effluent tubing, remove aluminum foil strip covering the end and place it on the metal effluent tube; this will be the effluent tubing and is labeled A in Figure 10.
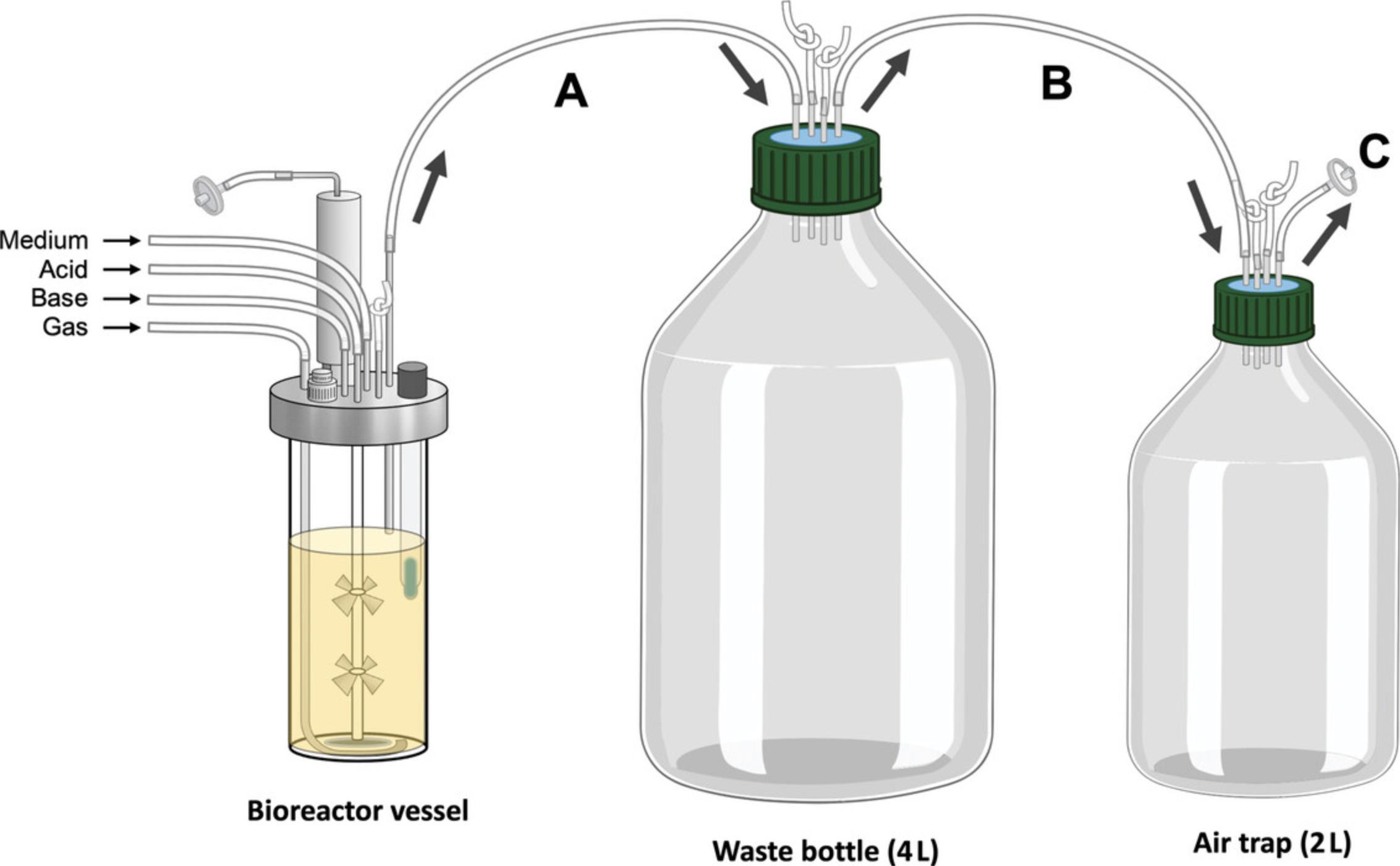
29.Unwrap foil from the length of tubing measured from the effluent bottle to the air trap bottle in Support Protocol 3 (Fig. 2E). Remove one of the knotted pieces of tubing from the effluent bottle by making a slit at the bottom with a razor blade. Attach the tubing from the air trap bottle to the effluent bottle; this is labeled B in Figure 10.Unwrap the other length of tubing connected to the air trap bottle (Fig. 2E) and run this tubing to a fume hood or other means of venting gas from the lab; this tubing is labeled C in Figure 10.
30.Pinch tube connected to the condenser outlet and clip with a 32-mm metal bulldog clip.
31.Place bottle of medium prepared in Basic Protocol 1 on a magnetic stir plate and set to stir to create a small vortex.
32.Connect the acid and base bottles to the vessel by unwrapping prepared tubing as shown in Figure 2C-D. Open the back of the pump head by pushing down on the clip (Fig. 11A). Push the PharMed pump tubing into the pump head as shown in Figure 11B. Ensure that the flow direction points towards the vessel. Replace the back of the pump head (Fig. 11C) and insert the drive shaft into the middle of the pump head (Fig. 11D).
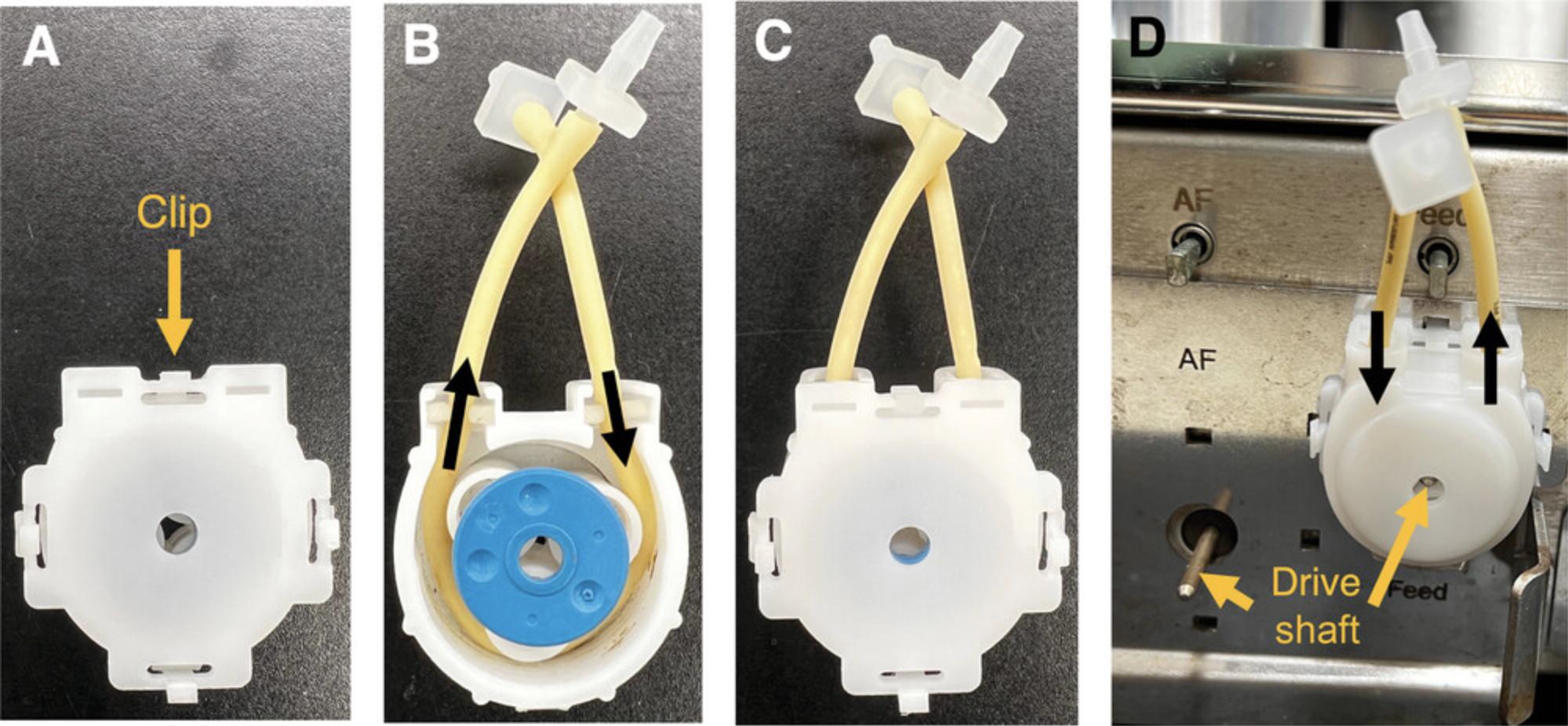
33.Spray the addition port area on the vessel lid (currently sealed with tubing with knotted ends; Fig. 6), a razor blade, and the wrapped end of the prepared tubing that will be connected to the vessel in (Fig. 1C-D, Fig. 2C-D) with 70% ethanol.
34.Using the razor blade to facilitate, as above, remove one of the knotted-end tubing pieces from one of the addition ports (Fig. 8B) and replace this with the prepared tubing attached to the 250-ml size bottle that will be used for containing acid. Repeat this step for the bottle intended for containing base and attach it to another addition port.
35.Remove lids from the acid and base bottles and fill as appropriate with the 5% acid or 5% base solutions prepared in Support Protocols 4 and 5.
36.Remove foil around the autoclaved 120-cm piece of silicone tubing attached to the medium bottle.
37.Feed the PharMed pump tubing through the pump head by first pushing down on the clip on the back of the pump head (Fig. 11A). Remove the back of the pump head and feed the tubing through (Fig. 11B). Ensure that the flow direction points towards the vessel. Replace the back of the pump head and insert the drive shaft into the middle of the pump head (Fig. 11C).
38.Unwrap the autoclaved, 50-cm length tubing and spray it, the razor blade, and the addition port area on the vessel lid with 70% ethanol.
39.As in step 34, use the razor blade to facilitate removal of one of the tubing pieces with a knotted end from the vessel lid and replace with the tubing running through the pump head attached to the medium bottle at the other end.
40.Prime medium, acid, and base feed lines by setting the pump speeds to 100% until liquid reaches within 1 cm of the addition ports.
41.Turn off the pumps for the medium, acid, and base feeds, and clip the acid and base lines with 32-mm metal bulldog clips 10 cm from the addition ports to prevent back-flow.
42.Once the water has drained from the vessel, raise the effluent pipe to the appropriate height according to the desired volume for the vessel (e.g., 200 ml for the 0.4-L vessel). Ensure that this volume of liquid in the vessel covers the pH probe above the probe septum (Fig. 7).
43.On the CPU controller, turn the feed control to 100% and allow medium pump to fill the vessel with medium to the height of the effluent pipe. Turn feed control back off.
44.Use 32-mm metal bulldog clips to clamp the tubing for the medium feed.
45.Set temperature of the system to 37°C.
46.Note the pH value of the medium (as read from the CPU screen) and leave vessel for 12-24 hr.
Support Protocol 2: MAKING ACID AND BASE BOTTLES
This protocol covers the preparation of the acid and base reservoir bottles while maintaining sterility. The reservoir bottles should be autoclaved separately from the prepared vessel and attached later.
Materials
- Silicone Laboratory Tubing Internal Diameter (ID) 3.18 mm, Outer diameter (OD) 6.35 mm (Thermo Fisher Scientific, cat. no. 11-189-15L)
- Polypropylene pump fittings, 1/8 in. × 3/32 in. (Cole-Parmer, cat. no. RK-06365-66)
- Aluminum foil
- PharMed pump hose 1-mm diameter, 15-cm long (Masterflex, cat. no. RK-06508-13)
- 250-ml glass bottles with GL45 neck (Corning, cat. no. 1395-250)
- GL45 screw cap with three fitted metal pipes (ID 2 mm, OD 4 mm, length 6 cm; BOLA, cat. no. D606-08)
- Autoclavable 25-mm 0.22-µm filter (Thermo Fisher Scientific, cat. no. 09-719C)
1.Cut one 12-cm, four 50-cm, and two 10-cm long pieces of silicone tubing.
2.Cut one 10-cm × 10-cm, two 3-cm × 5-cm, and four 15-cm × 25-cm strips of aluminum foil.
3.For two of the 50-cm lengths of tubing, use the 3-cm × 5-cm strips of foil to wrap the ends.
4.Working with the 50-cm lengths of tubing with one end wrapped in foil, start with the wrapped end and coil on top of aluminum square leaving the unwrapped end outside of the aluminum foil (Fig. 1).
5.Fold edges, bottom, and top according to Figure 1.
6.For the other two pieces of 50-cm silicone tubing, coil so that both ends are left extending off the aluminum foil as shown in Figure 4.Fold aluminum foil as shown in Figure 4.
7.Connect the unwrapped end of the tubing prepared in step 4 to the pump tubing and tubing prepared in step 6 as shown in Figure 5A.
8.Connect tubing assembly from step 7 to the three-connector bottle lid as shown in Figure 5B.
9.Connect the 12-cm piece of silicone tubing to the inside of the bottle lid on the same connector as the pump line assembly Figure 5B.
10.Tie a knot at one end for one of the 10-cm pieces of tubbing. Attach the knotted tubing to an open port of the bottle lid (Fig. 5B).
11.Connect a 25-mm 0.22-µm filter to one end of the 10-cm tubing and place the other end on one of the open ports to the bottle lid (Fig. 5C).
12.Clamp tube connected to the 0.22-µm filter and wrap it in the 10- × 10-cm foil piece.
13.Screw lid assembly to a 250-ml bottle, ensuring the lid is loose by at least one turn.
14.Repeat steps 1-14 to prepare a second bottle.
15.Sterilize bottles by autoclaving using a dry 20 cycle (or as appropriate for autoclave used). Tighten bottle lids following autoclave cycle.
Support Protocol 3: PREPARING THE EFFLUENT BOTTLES
The bioreactor vessels emulating the distal colonic environment produce a considerable volume of effluent depending on the retention time and size of vessel selected. This protocol describes the preparation of an effluent collection bottle to collect the effluent from a running bioreactor vessel. A secondary collection bottle (air trap) is connected to the effluent collection bottle, and helps to vent gas from the bioreactor headspace, as well as serving as a back-up container for any overspill.
Materials
- Silicone Laboratory Tubing Internal Diameter (ID) 3.18 mm, Outer diameter (OD) 6.35 mm (Thermo Fisher Scientific, cat. no. 11-189-15L)
- Aluminum foil
- GL45 screw cap with three fitted metal pipes ID 2 mm, OD 4 mm (BOLA, cat. no. D606-08)
- GL45 screw cap with four fitted metal pipes ID 2 mm, OD 4 mm (BOLA, cat. no. D606-08)
- 5-L glass bottle with GL45 neck (DWK Life Sciences, cat. no. SP14395-5000)
- 2-L glass bottle with GL45 neck (DWK Life Sciences, cat. no. KC143952000)
- Autoclavable 25-mm 0.2-µm syringe filter (Thermo Fisher Scientific, cat. no. 09-719C)
1.Cut a length of silicone tubing that can comfortably reach from the vessel's effluent output pipe to the desired position of the effluent bottle, with ∼20-30 cm of slack.
2.Wrap the end of the tubing with a 3- × 5-cm strip of aluminum foil. Make a flat coil with the rest of the tubing around the wrapped end and wrap the coil in a 15- × 25-cm strip of aluminum foil, leaving the other end of the tubing exposed.
3.Screw a three-port lid onto a 5-L glass bottle, leaving it loose by one turn.
4.Attach the prepared, coiled tubing to one of the ports.
5.Cut two 10-cm pieces of silicone tubing and knot one end of each piece ∼1 cm from one of the ends. Affix unknotted ends to the two remaining ports on the bottle lid.
6.Screw a four-port lid onto a 2-L bottle, leaving it loose by one turn.
7.Cut a length of silicone tubing that can reach from the effluent bottle to the trap bottle with ∼10-20 cm of slack. In general, a length of 40 cm is sufficient (Fig. 1D).
8.Wrap one end of this tubing length, make a flat coil as in step 2 of this protocol, and wrap in a 15- × 25-cm strip of aluminum foil leaving the unwrapped end exposed.
9.Attach exposed end of this tubing to one of the ports on the 2-L bottle lid.
10.Cut another length of silicone tubing to act as the gas vent tube.
11.Cut two 10-cm pieces of silicone tubing and knot one end of each piece ∼1 cm from one of the ends. Affix unknotted ends to the two remaining ports on the bottle lid.
12.Sterilize bottles by autoclaving using a dry 20 cycle (or as appropriate for instrument used). Once the cycle is finished, tighten the lids on the bottles.
Support Protocol 4: MAKING ACID SOLUTION
This protocol details preparation of a solution of 5% (v/v) hydrochloric acid for the acid reservoir of the bioreactor vessel. This solution should be prepared in a fume hood.
Materials
-
Concentrated hydrochloric acid (Thermo Fisher Scientific, cat. no. A149C-4)
-
Deionized water
-
250-ml glass graduated cylinder (DWK Life Sciences, cat. no. 20026-250)
-
1-L plastic graduated cylinder (Thermo Fisher Scientific, cat. no. 03-007-44)
-
1-L glass beaker (VWR, cat. no. 13912-284)
-
Small magnetic stir bars (38-mm length × 12-mm diameter; VWR, cat. no. 58947-138)
-
Magnetic stir plate (Thermo Fisher Scientific, cat. no. 88880009)
-
Sterile bottle top filter with 0.22-µm PES membrane and 45-mm neck (Thermo Fisher Scientific, cat. no. 595-4520)
-
Sterilized 1-L glass bottle with GL45 neck (Pyrex, cat. no. 13951LHTC)
1.Fill glass graduated cylinder with 135 ml HCl
2.Measure 1 L deionized water less the volume of HCl being added (in this case the amount of water required is 865 ml). Add water to beaker.
3.In the fume hood, slowly and carefully add HCl to the water.
4.Add stir bar and mix solution for 5 min
5.Using aseptic technique, place a 0.22-µm bottle top filter on the mouth of a 1-L bottle and connect to a vacuum system. Filter-sterilize the acid solution.
6.Remove bottle top filter and replace bottle cap. Clearly label bottle.
Support Protocol 5: MAKING BASE SOLUTION
This protocol details preparation of a solution of 5% (v/v) sodium hydroxide solution for the base reservoir of the bioreactor vessel This solution should be prepared in a fume hood.
In our experience, we have found that microbial communities derived from the human colon tend to use more base than acid during bioreactor operation. It is therefore wise to prepare up to twice the amount of base as acid solution for a given experiment.
Materials
-
Sodium hydroxide (Thermo Fisher Scientific, cat. no. S318-3)
-
Deionized water
-
Analytical balance (e.g., OHAUS, cat. no. 30529239)
-
Weighing boats
-
Scoopula
-
1-L plastic beaker (Thermo Fisher Scientific, cat. no. 1201-1000PK)
-
Small magnetic stir bars (38-mm length × 12-mm diameter; VWR, cat. no. 58947-138)
-
Magnetic stir plate (Thermo Fisher Scientific, cat. no. 88880009)
-
Sterile bottle top filter with 0.22-µm PES membrane and 45-mm neck (Thermo Fisher Scientific, cat. no. 595-4520)
-
Sterilized 1-L glass bottle with GL45 neck (Pyrex, cat. no. 13951LHTC)
1.Using an analytical balance, measure out 50 g NaOH pellets and add to a beaker large enough to hold a 1-L volume.
2.Add deionized water up to 1 L. Add a stir bar and mix until all pellets have dissolved.
3.Using aseptic technique, place a 0.22-µm bottle top filter on the mouth of a 1-L bottle and connect to a vacuum system. Filter-sterilize the base solution.
4.Remove bottle top filter and replace bottle cap. Clearly label the bottle.
Basic Protocol 3: PREPARING INOCULUM AND INOCULATING BIOREACTORS
The method of preparation of the inoculum for a given bioreactor vessel should be considered carefully to ensure that the microbes are given the best chance of survival. Stool samples can be maintained for long periods (several months) by freezing them at −80ºC without any need for added cryoprotectant, however, repetitive freeze and thaw cycles will dramatically reduce microbial viability. To prevent this problem, care should be taken to divide a fresh stool sample into smaller aliquots, or not to completely thaw the whole sample each time a portion of it is needed. A better approach is to prepare a large portion of a fresh stool sample for inoculation by homogenizing it in prepared medium at a ratio of 1:10 stool/medium, and aliquoting this material into tubes before freezing at −80ºC. This approach will improve vessel-to-vessel reproducibility across experimental series. However, it is also important to remember that most of the microbial species that reside in human stool are sensitive anaerobes and microaerophiles, and thus measures should be taken to prevent oxygen exposure when thawing and/or preparing stool samples for inoculation; carrying out stool inoculum preparation steps inside an anaerobic chamber with an atmosphere of 10% hydrogen, 10% carbon dioxide, and 80% nitrogen is ideal.
A starting inoculum volume of 0.5% to 1% of the total vessel running volume has, in our hands, proven sufficient for reproducible and representative bioreactor runs (McDonald et al., 2013, 2015; Yen et al., 2015), and here we describe a protocol for preparation and inoculation of bioreactor vessels with at least 0.5% (w/v) starting stool material.
Materials
-
Stool sample
-
10 ml bioreactor culture medium (as prepared in Basic Protocol 1), pre-reduced by placing into an anaerobic chamber in a container with a loosened lid for at least 6 hr
-
70% ethanol solution (in a spray bottle)
-
Silicone Laboratory Tubing Internal Diameter (ID) 3.18 mm, Outer diameter (OD) 6.35 mm (Thermo Fisher Scientific, cat. no. 11-189-15L)
-
Aluminum foil
-
Analytical balance (e.g., OHAUS, cat. no. 30529239)
-
Sterile sampling bag (Nasco, cat. no. B00679)
-
Sterile 50-ml conical tubes (Corning, cat. no. 352070)
-
Parafilm (Thermo Fisher Scientific, cat. no. 13-374-10)
-
Benchtop centrifuge (e.g., Sorvall Legend X1R Centrifuge, Thermo Fisher Scientific, cat. no. 75004261) and rotor with adapters for 50-ml conical tubes (e.g., TX-400 rotor adapters, Thermo Fisher Scientific, cat. no. 75003683)
-
BSL2 safety cabinet (e.g., Purifier Logic + Class 2 Biosafety Cabinet; Labconco, cat. no. 302311101)
-
Single-wrapped, sterile 20-ml Luer-Lok syringe (BD, cat. no. 302830)
-
Sterile 0.88-mm gauge needle (BD, cat. no. 305167)
1.Prepare a 10-cm piece of silicone tubing by knotting one end and wrapping the other end with a small piece of aluminum foil. Sterilize tubing in a dry 20 cycle (or as appropriate for autoclave instrument used).
2.If fecal sample is frozen, thaw slowly to room temperature.
3.Using an analytical balance, weigh out the desired amount of sample into a sterile bag.
4.Add 10 ml of pre-reduced bioreactor culture medium to the sample in the sterile bag, seal carefully, and mix thoroughly with firm manipulation between gloved hands in a BSL2 safety cabinet.
5.Carefully transfer homogenate from the bag into a sterile 50-ml conical tube and screw on the cap. If the sample is being prepared in an anaerobic chamber, seal tube using Parafilm before removing it from the chamber.
6.Spin at 1,000 × g for 10 min in a benchtop centrifuge.
7.Return sample to the anaerobe chamber (if using). Remove supernatant using a 20-ml disposable syringe. Use of a needle is optional for this step.
8.Remove needle from the syringe (if using) and dispose appropriately, then attach the pre-sterilized piece of knotted tubing from step 1 to the Luer lock.
9.Transport inoculum to the prepared bioreactor vessel. Ensure that the vessel content (prepared medium) is at 37ºC, at the desired pH (usually neutral for mimicking the distal colon), and has been sparged (bubbled through the medium) for at least 1 hr with nitrogen gas to displace excess oxygen.
10.Remove bulldog clip on the condenser vent tubing to allow gas to flow from the vessel headspace. Use the same clip to clamp the effluent tubing closed ∼15 cm from the effluent pipe.
11.Disinfect the blades of a pair of scissors and a region ∼10 cm (from the effluent pipe) of the outside surface of the effluent tubing by liberally spraying with 70% ethanol.
12.Cut effluent tube ∼5 cm from the end of the pipe with scissors. Quickly remove the knotted tubing from the end of the inoculating syringe and attach the Luer-Lok to the cut end of the effluent tubing connected to the effluent pipe of the vessel. Inject sample into the vessel with the syringe plunger.
13.Disinfect razor blade and effluent pipe with 70% ethanol. Use razor blade to make a small slit in the tubing connected to the waste pipe to facilitate its removal. Dispose of the syringe and tubing remnant appropriately.
14.Reattach waste tubing still connected to the waste bottle to the vessel at the freshly cut end. Unclamp effluent tubing and return the clip to the condenser vent.
15.Allow the microbial community to grow for 24 hr in batch culture (Fig. 12A and 12B).
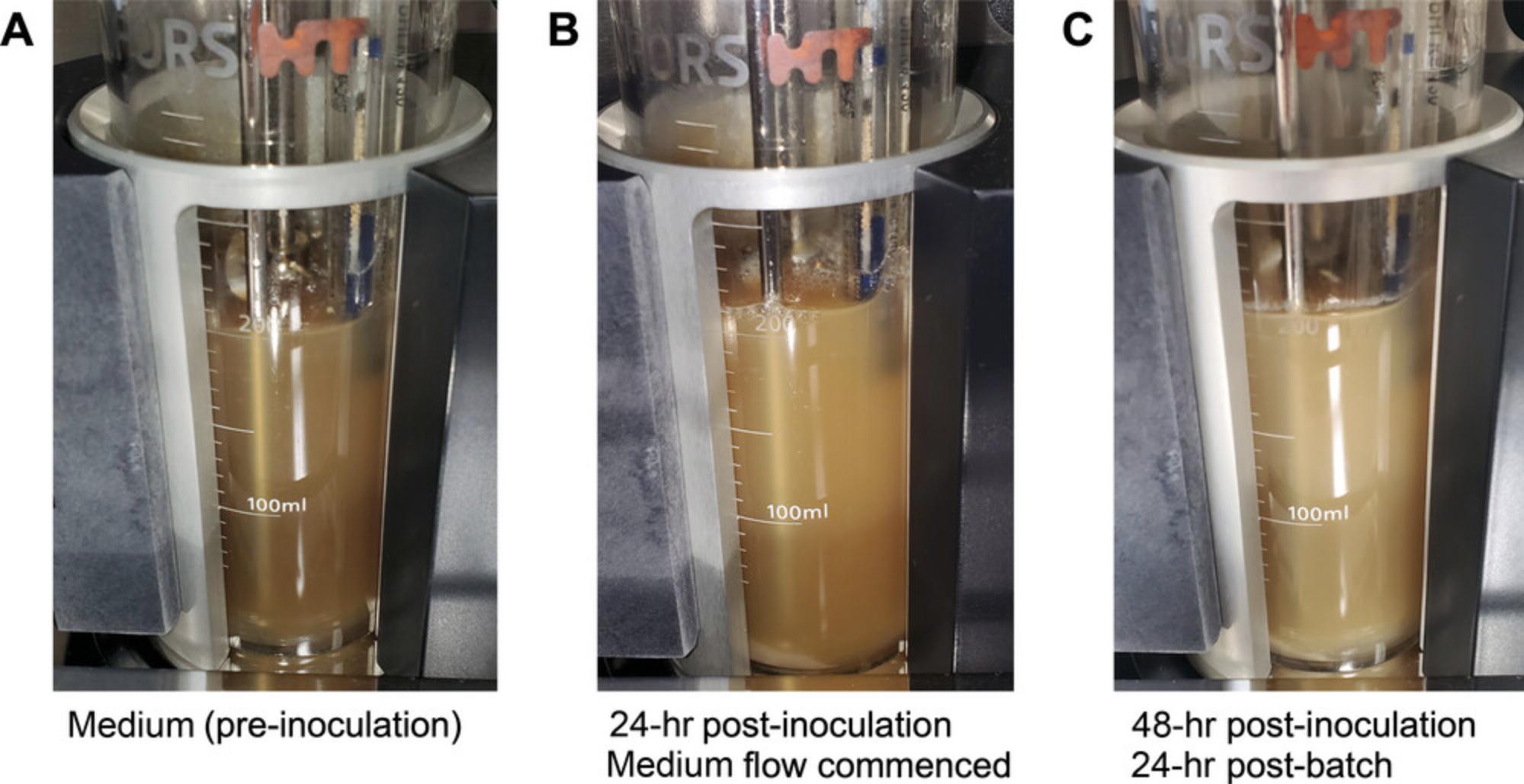
16.After 24 hr (Fig. 12C), turn on the medium flow at the desired rate (a retention time of 24 hr is often used to mimic transit time across the colon).
Alternate Protocol 1: PREPARING INOCULUM LESS THAN 0.5% (W/V) OF VESSEL VOLUME
Not all provided stool samples may be of appreciable volume, for example, some studies may have collected only milligram weights of stool samples. In our hands, it has been possible to work with even 100 mg samples of stool to create inocula and to effectively create complex communities from such samples (Ganobis, 2022). This alternate protocol is specific to inoculation volumes <0.5% (w/v) of the final vessel volume. The protocol is similar to Basic Protocol 3, with the main difference being the length of time the culture is left in batch. The batch culture period will depend on the size of the inoculum and is specific to each sample. Cell numbers can be estimated using quantification methods such as flow cytometry, should this be a concern (Vandeputte et al., 2017).
Additional Materials (see also Basic Protocol 1)
-
Stool sample
-
Pre-reduced bioreactor medium (prepared in Basic Protocol 1)
-
70% ethanol solution
-
Silicone Laboratory Tubing Internal Diameter (ID) 3.18 mm, Outer diameter (OD) 6.35 mm (Thermo Fisher Scientific, cat. no. 11-189-15L)
-
Aluminum foil
-
Analytical balance (e.g., OHAUS, cat. no. 30529239)
-
Sterile bag (Nasco, cat. no. B00679)
-
50-ml conical tubes (Corning, cat. no. 352070)
-
Parafilm (Thermo Fisher Scientific, cat. no. 13-374-10)
-
Benchtop centrifuge (e.g., Sorvall Legend X1R Centrifuge; Thermo Fisher Scientific, cat. no. 75004261) and rotor with adapters for 50-ml conical tubes (e.g., TX-400 rotor adapters; Thermo Fisher Scientific, cat. no. 75003683)
-
20-ml Luer-Lok sterilized syringe (BD, cat. no. 302830)
-
0.88-mm gauge needle (BD, cat. no. 305167) or equivalent
1.Prepare a 10-cm piece of silicone tubing by knotting one end and wrapping the other end with a small piece of aluminum foil. Sterilize tubing in a dry 20 cycle (or as appropriate for autoclave instrument used).
2.Obtain sample.
3.Using an analytical balance, weigh sample to obtain an estimate of biomass present, and place into a sterile bag.
4.Add 10 ml of pre-reduced bioreactor culture medium to the sample in the sterile bag, seal, and mix thoroughly with firm manipulation between gloved hands.
5.Return sample to the anaerobe chamber (if using). Remove supernatant (or homogenate) using a 20-ml disposable syringe. Use of a needle is optional for this step.
6.Remove needle from the syringe (if using) and dispose appropriately, then attach the pre-sterilized piece of knotted tubing from step 1 to the Luer lock.
7.Transport inoculum to the prepared bioreactor vessel. Ensure that the vessel content (prepared medium) is at 37ºC, at the desired pH (usually neutral for mimicking the distal colon), and has been sparged (bubbled through the medium) for at least 1 hr with nitrogen gas to displace excess oxygen.
8.Remove bulldog clip on the condenser vent tubing to allow gas flow directly from the vessel headspace. Use the same clip to clamp the effluent tubing closed ∼15 cm from the effluent pipe.
9.Disinfect blades of a pair of scissors and a region ∼10 cm (from the effluent pipe) of the outside surface of the effluent tubing with 70% ethanol.
10.Cut effluent tube ∼5 cm from the end of the pipe with scissors. Quickly remove the knotted tubing from the end of the inoculating syringe and attach the Luer-Lok to the cut end of the effluent tubing connected to the effluent pipe of the vessel. Inject sample into the vessel with the syringe plunger.
11.Disinfect razor blade and effluent pipe with 70% ethanol. Use razor blade to make a small slit in the tubing connected to the waste pipe to facilitate its removal. Dispose of the syringe and tubing remnant appropriately.
12.Reattach effluent tubing still connected to the waste bottle to the vessel at the freshly cut end. Unclamp effluent tubing and return clip to the condenser vent.
13.Allow the microbial community to grow for at least 48 hr in batch culture.
14.Turn on medium flow and clamp condenser vent tube.
Alternate Protocol 2: PREPARING SYNTHETIC COMMUNITY ALIQUOTS AND INOCULATION VIA THE SEPTUM
This protocol details the steps required to create aliquots of a synthetic community. These aliquots can be used to create replicate Roboguts within an experimental group or condition. A synthetic community (also known as a defined community) refers to any collection of cultured microbial isolates, where each isolate exists as a pure, monoclonal stock. To create a synthetic community, microbial strains must be isolated from a sample of interest and stored as individual stocks. These strains can be revived and pooled together to create a complex microbial consortium, where each constituent member is known and defined. A synthetic community thus sacrifices some degree of community diversity for a high degree of experimental control. In contrast, a fecal community may contain several hundred strains, but only a subset of those strains can be feasibly identified. Methods for culturing microbes from feces and creating a synthetic community have been reviewed elsewhere (Bayer et al., 2021; Renwick et al., 2021); this protocol will focus on how to pool microbial isolates to create experimentally equivalent aliquots.
Materials
-
Library of synthetic community microbial isolates (see introduction, Alternate Protocol 2; solid agar medium is preferred for each synthetic community isolate)
-
Supplemented Tryptic Soy Broth (sTSB), 5 ml and 1 ml (see recipe), pre-reduced by placing into an anaerobic chamber in a container with a loosened lid for at least 6 hr
-
Freezing medium, pre-reduced, 95 ml (see recipe)
-
10% (v/v) bleach
-
10-µl sterile inoculation loops (Globe Scientific, cat. no. 2815F)
-
5-ml Luer-Lok syringe (BD, cat. no. 309646)
-
BD 18-gauge PrecisionGlide Luer-Lok needle (Thermo Fisher Scientific, cat. no. 14-826-5D)
-
2-ml cryogenic storage vials (Thermo Fisher Scientific, cat. no. 02-912-728)
-
Pipet filler
-
25-ml serological pipet (GeneBio Systems, cat. no. MSP2501)
-
Sterilized cotton swabs (Thermo Fisher Scientific, cat. no. 14-959-92B)
-
Anaerobic chamber (or anaerobic jars for the cultivation of anaerobic microorganisms)
-
Ultralow temperature freezer
-
Vortex mixer (e.g., Thermo Fisher Scientific, cat. no. BV202)
1.Revive each member of the synthetic community on its preferred solid agar medium.
2.Streak each isolate to purity on a further agar plate.
3.For each isolate, pick an isolated colony and streak out an area large enough to yield ∼10 mg of biomass; incubate overnight or the required time for good growth yields.
4.Using a sterile 10-µl loop, transfer ∼10 mg or one full loop of biomass into 5 ml of pre-reduced sTSB in an anaerobic chamber. Repeat this step for each microbial isolate.
5.Use a sterile loop to gently blend the broth mixture until all lumps of microbial biomass have dispersed. Several sterile loops can be bunched together to agitate microbial biomass more efficiently; gentle vortexing and pipetting may also be used. Mix until the mixture appears homogenous and without lumps of biomass, then continue mixing gently for a further 5 min to ensure the mixture is completely homogeneous.
6.Transfer broth mixture into 95 ml of pre-reduced freezing medium.
7.Mix solution by gentle inversion and vortex for 5 min.
8.Aliquot solution into 2-ml sterile cryovials with the serological pipet.
9.Transfer aliquots to −80°C storage within 30 min.
10.To inoculate a prepared bioreactor vessel, thaw the community aliquot at room temperature, preferably in an anaerobic chamber.
11.Once the synthetic community aliquot is thawed, gently mix aliquot by inversion for 1 min.
12.Inside an anaerobic chamber, draw aliquot into a 5-ml Luer-Lok syringe fixed with a sterile metal hub Luer-Lok 18-gauge needle. Rinse cryovial with 1 ml sTSB and draw into the syringe.
13.Carefully invert syringe and replace needle with a new sterile, 18-gauge needle. Leave the new needle capped to facilitate transport to the bioreactor.
14.Remove syringe from the anaerobic chamber.
15.Prepare bioreactor for inoculation through the septum: Use a sterile cotton swab dipped in 10% bleach solution to liberally wipe the septum. Wait 2 min. Use another sterile cotton swab to blot the septum dry.
16.Carefully remove needle cap. Firmly pierce septum with the needle tip and inject the synthetic community aliquot directly into the prepared medium.
17.Carefully remove syringe with the needle from the vessel.
18.Allow the microbial community to grow for 24 hr in batch culture.
19.After 24 hr, turn on the medium flow at the desired rate (a retention time of 24 hr is often used to mimic transit time across the colon).
Alternate Protocol 3: PREPARING INOCULUM FROM A TISSUE SAMPLE
In cases where microbial communities associated with tumor presence are of interest, there may be an interest in cultivating gut microbial communities present from biopsy or tissue samples. This protocol will cover the process in preparing an inoculum from biopsies or samples of colorectal tissue. As for stool, appropriate biohazard and bioethical considerations for working with tissue samples are imperative.
Materials
-
Tumor sample or biopsy
-
70% ethanol solution
-
Supplemented Tryptic Soy Broth (sTSB; see recipe), pre-reduced by placing into an anaerobic chamber in a container with a loosened lid for at least 6 hr
-
Aluminum foil
-
Sterilization pouches (Thermo Fisher Scientific, cat. no. 15-234-13)
-
Forceps (Thermo Fisher Scientific, cat. no. 16-100-121)
-
Sterile scalpel holder (Thermo Fisher Scientific, cat. no. 08-917-5)
-
Sterile blade (Thermo Fisher Scientific, cat. no. 08-918-5A)
-
Sterile dissection tray or board (e.g., Thermo Fisher Scientific, cat. no. 73092)
-
Tissue grinder or mortar and pestle (e.g., Thermo Fisher Scientific, cat. no. NC0413484)
-
Sterile 15-ml conical tubes (Corning, cat. no. 352096)
-
1,000-µl wide-bore filter tips (VWR, cat. no. 53509-504)
-
Single-wrapped, sterile 20-ml Luer-Lok syringe (BD, cat. no. 302830)
-
Sterile 0.88-mm gauge needle (BD, cat. no. 305167)
-
Silicone Laboratory Tubing Internal Diameter (ID) 3.18 mm, Outer diameter (OD) 6.35 mm (Thermo Fisher Scientific, cat. no. 11-189-15L)
1.Prepare a 10-cm piece of silicone tubing by knotting one end and wrapping the other end with a small piece of aluminum foil. Sterilize tubing in a dry 20 autoclave cycle (or as appropriate for autoclave instrument used).
2.Prepare dissection tray, forceps (at least two pairs), and tissue grinder by wrapping in aluminum foil or sealing in sterilization pouches. Sterilize in a dry 20 autoclave cycle (or as appropriate for autoclave instrument used).
3.If the tissue sample is frozen, thaw slowly to room temperature.
4.Transfer tissue to the dissection tray. With the sterile blade affixed onto the scalpel holder, begin making cuts to break up the tissue. Two pairs of forceps may be used to tweeze apart particularly fibrous tissue. Once the sample is cut into smaller pieces for the appropriately sized tissue grinder or mortar and pestle being used, transfer to the mortar.
5.Add 1 ml sTSB to the mortar and begin grinding the sample until a slurry that could be passed through a pipet tip is achieved.
6.Transfer slurry to a sterile 15-ml conical tube. Rinse mortar and pestle with 1-2 ml sTSB and transfer it to the 15-ml conical tube as well. Top up the volume of the slurry to 10 ml with sTSB.
7.With a wide-bore filter tip, pipet up and down to mix and further break apart the tissue in the broth.
8.Once the tissue slurry is homogenized, gather it using a 20-ml disposable syringe. Use of a needle is optional for this step.
9.Remove needle from the syringe (if using) and dispose appropriately, then attach the pre-sterilized piece of knotted tubing from step 1 to the syringe.
10.Transport inoculum to the prepared bioreactor vessel. Ensure that the vessel content (prepared medium) is at 37ºC, at the desired pH (usually neutral for mimicking the distal colon), and has been sparged (bubbled through the medium) for at least 1 hr with nitrogen gas to displace excess oxygen.
11.Remove bulldog clip on the condenser vent tubing to allow gas flow directly from the vessel headspace. Use the same clip to clamp the effluent tubing closed ∼15 cm from the effluent pipe.
12.Disinfect blades of a pair of scissors and a region ∼10 cm (from the effluent pipe) of the outside surface of the effluent tubing with 70% ethanol.
13.Cut effluent tube ∼5 cm from the end of the pipe with scissors. Quickly remove the knotted tubing from the end of the inoculating syringe and attach the Luer-Lok to the cut end of the effluent tubing connected to the effluent pipe of the vessel. Inject sample into the vessel with the syringe plunger.
14.Disinfect razor blade and effluent pipe with 70% ethanol. Use razor blade to make a small slit in the tubing connected to the waste pipe to facilitate its removal. Dispose of the syringe and tubing remnant appropriately.
15.Reattach effluent tubing still connected to the waste bottle to the vessel at the freshly cut end. Unclamp effluent tubing and return clip to the condenser vent.
16.Allow the microbial community to grow for at least 48 hr in batch culture.
17.Turn on the medium flow and clamp the condenser vent tube.
Basic Protocol 4: SAMPLING THE BIOREACTOR VESSEL
This protocol details the sampling of the vessels throughout the experiment. It is important to determine before starting the types of downstream experiments that will be performed and the frequency of sampling. For example, ecosystem bacterial composition may be assessed using 16S rRNA gene sequence profiling, and metabolic output may be assessed using metabolomic techniques such as nuclear magnetic resonance (NMR; Current Protocols article: Ganobis et al., 2020). Regular (e.g., daily) sampling coupled with techniques such as moving window analysis allow assessment of equilibrium in the ecosystem, allowing the user to ascertain when the microbial community has reached a level of stability where experimental perturbation can be performed with minimal confounding effects (steady-state; Oliphant et al., 2019). Consideration should also be given to the volume of sample to be taken from a given vessel; this sample removal should not in itself disturb ecosystem equilibrium. In our hands, removal of no more than 10% of the total volume of a bioreactor supporting a human adult-derived gut microbial ecosystem at steady-state does not appreciably alter the equilibrium, though for other types of ecosystems the maximum sampling volume should be determined empirically before experimentation. A major benefit of the in vitro model system is the ability to rapidly retrieve and either preserve or directly analyze samples, a feature that lends itself well to the study of labile molecules such as RNA. Withdrawn samples can be directly frozen at −80ºC in the described bioreactor growth medium (Basic Protocol 1), which maintains viable cells and NMR-detectable intact metabolites for at least 1 year.
Materials
-
70% ethanol solution
-
Freshly prepared 10% (v/v) bleach solution in water
-
Sterilized deionized water
-
250-ml polypropylene graduated cylinder (Thermo Fisher Scientific, cat. no. 03-007-42)
-
2-ml sterile screw cap tubes with O-ring seals (Axygen, cat. no. SCT-200-SS-A-S)
-
Sterilized cotton swabs (Thermo Fisher Scientific, cat. no. 14-959-92B)
-
5-ml Luer-Lok syringe (BD, cat. no. 309646)
-
Sterilized (see Support Protocol 6) metal hub Luer-Lok sampling needle, 18 gauge, 12-in. length, AS style needle point (Hamilton, cat. no. 7751-09)
-
Ultralow temperature freezer (should samples require storage)
1.Label caps of as many sterile 2-ml screw cap tubes as are anticipated for the sampling effort.
2.Lay a Kimwipe nearby the vessel and spray with 70% ethanol.
3.Using 70% ethanol, spray the sampling port and unscrew the port leaving the septum collar in place. Place the sampling port cap onto the Kimwipe.
4.Use a sterile cotton swab dipped in 10% bleach solution to liberally wipe the septum. Wait 2 min. Use another sterile cotton swab to blot the septum dry.
5.Attach a 5-ml syringe to the end of a sampling needle, being careful to use aseptic technique. Firmly pierce septum with the needle tip and push carefully into the liquid.
6.Slowly draw up liquid into the syringe to the amount desired. This should be a volume of no more than 10% of the vessel content in any 24-hr period. Pull needle above the liquid surface in the vessel and withdraw remaining liquid in the needle into the syringe by gently pulling on the plunger until bubbles form in the syringe.
7.Carefully remove syringe with the needle from the vessel. Place needle, sharp end down, into a 250-ml graduated cylinder to protect it and to prevent accidental needlesticks.
8.Carefully detach syringe from the needle and use the syringe to fill the sample tubes with up to 2 ml liquid. Dispose of syringe appropriately after use.
9.If not used immediately, transfer sample tubes to a −80°C freezer.
10.Disinfect septum by wetting a cotton swab with 10% bleach solution and liberally wiping it on the septum. Wait 2 min and then use another cotton swab to dry the septum.
11.Clean any residual bleach from the port by dipping a sterilized cotton swab into sterile water and carefully cleaning any areas touched with bleach. Dry with a further sterile cotton swab.
12.Replace the blanking port in the septum collar.
Basic Protocol 5: HARVESTING BIOREACTOR VESSEL CONTENTS AT END OF EXPERIMENT
This protocol details harvesting the entire contents of the vessel once an experiment is complete. Maintaining an anaerobic environment for the vessel contents is an important consideration for microbial communities derived from the human gut, particularly if the downstream application includes microbial culture.
Materials
-
70% ethanol solution
-
250-ml glass bottles with GL45 screw caps (Corning, cat. no. 1395-250)
-
GL45 screw cap with four fitted metal pipes ID 2 mm, OD 4 mm (BOLA, cat. no. D606-08)
-
Silicone Laboratory Tubing Internal Diameter (ID) 3.18 mm, Outer diameter (OD) 6.35 mm (Thermo Fisher Scientific, cat. no. 11-189-15L)
-
50-ml sterile conical tubes (Thermo Fisher Scientific, cat. no. 06-443-18)
-
50-ml single-wrapped sterile serological pipets (GeneBio Systems, cat. no. MSP5001)
-
Parafilm (Thermo Fisher Scientific, cat. no. 13-374-10)
-
Pipet filler
-
Metal bulldog clips (32 mm)
1.Prepare a collection bottle: Screw a four-connector cap onto a 250-ml bottle. Cut four 10-cm lengths of silicone tubing and tie a knot in each, ∼1 cm from one end. Affix the open end of each tube onto each of the four connector ports on the bottle cap. Loosen cap by one turn and autoclave to sterilize.
2.Complete any desired small volume daily sampling of the vessel following Basic Protocol 4.
3.Standing within reach of the bioreactor, spray a razor blade and the top of the 250-ml bottle with 70% ethanol. Use the razor blade to make a small slit in the side of one of the knotted tubes to facilitate its removal.
4.Remove the knotted tube from the bottle cap. Quickly uncouple effluent tubing from the effluent bottle (tubing A in Fig. 10) and position it on the now empty port on the collection bottle cap. Allow to stand 2 min to permit gas to displace any oxygen in the bottle. Meanwhile, spray effluent port on the vessel with 70% ethanol and leave for 2 min.
5.Turn off the vessel pumps controlling pH and medium addition, and the impeller.
6.Slowly lower effluent pipe to the bottom of the vessel.
7.Once all vessel contents have been transferred, unclamp the bulldog clip on the tubing connected to the condenser.
8.Switch off the bioreactor unit and close the nitrogen gas valve. Use bulldog clips to clamp the effluent tubing ∼5 cm from the vessel and again 5 cm from the collection bottle. Use scissors to cut the tubing ∼5 cm beyond the clamp at the collection vessel end.
9.Cut effluent tubing ∼5 cm beyond the bulldog clip at the effluent collection bottle. Tie a knot in the end of this tubing and remove bulldog clip.
10.Remove pH probe from the vessel lid and use the pH porthole to fill the vessel with 200 ml deionized water. Replace probe. Proceed to Basic Protocol 6 for instructions of vessel cleaning and maintenance.
11.Use a 50-ml serological pipet to transfer material from the 250-ml bottle to conical tubes or other storage containers, label, and preserve at −80ºC or as desired.
Support Protocol 6: CLEANING AND STERILIZING SAMPLING NEEDLES
Cleaning the sampling needles promptly after use is important to prevent buildup of dried biomass in the needle bore.
Materials
-
Deionized water
-
100-ml glass bottle with GL45 neck (Kimble, cat. no. DWK14395-100)
-
5-ml Luer-Lok syringe (BD, cat. no. 309646)
-
Used metal hub Luer-Lok sampling needle, 18 gauge, 12-in. length, AS style needle point (Hamilton, cat. no. 7751-09)
-
200-ml beaker (PYREX, cat. no. 13912-207)
-
Aluminum foil
-
Autoclave
-
250-ml polypropylene graduated cylinder (Thermo Fisher Scientific, cat. no. 03-007-42)
1.Collect multiple 12-in. needles for autoclaving all at once in 250-ml graduated cylinders. Autoclave on a liquid 20 cycle (or as appropriate for the autoclave instrument used).
2.Fill a 100-ml glass bottle with ∼70 ml deionized water.
3.Attach the Luer lock of the autoclaved needle to the 5-ml syringe, carefully remove needle from the graduated cylinder and place it into the water. Use the syringe plunger to draw up ∼1 ml water.
4.Remove needle from the bottle and place into the beaker. Forcefully eject the water into the beaker through the needle using the syringe plunger.
5.Repeat steps 3-4 for a total of five times.
6.Remove syringe from the cleaned sampling needle and dispose of the syringe in the biohazard effluent.
7.Wet a clean piece of paper towel with deionized water and carefully wipe the length of the needle by wrapping it with the wetted paper towel with one downward stroke.
8.Place sampling needle sharp-side down into a clean 250-ml graduated cylinder. Air dry overnight.
9.Split sampling needles into groups of three to five needles per graduated cylinder. Cover the top of the graduated cylinder with aluminum foil and autoclave on a dry 20 cycle (or as appropriate for the autoclave instrument used).
Basic Protocol 6: CLEANING THE BIOREACTOR VESSEL
Proper cleaning of the bioreactor vessel after each use is imperative to maintain equipment functionality and prevent biofouling buildup. Vessels should never be left standing for longer than 2-3 days after the end of an experiment before cleaning.
Additional Materials (also see Basic Protocol 5)
-
pH probe storage solution, pH 4.00, Fisher Chemical™ (Thermo Fisher Scientific, cat. no. SB98-500)
-
Mild detergent (such as Versa-Clean; Thermo Fisher Scientific, cat. no. 18-200-700)
-
Medium bulldog clips (32 mm)
-
Aluminum foil
-
Non-abrasive sponge (e.g., Scotch-brite no scratch scrub sponge)
-
Autoclavable, high sided plastic tray (54.3-cm length × 43.5-cm width × 13-cm height; Thermo Fisher Scientific, cat. no. 6900-0020)
1.From the Infors system batch menu, end the run. Switch off the unit base and ensure that the gas flow has also been turned off. If using compressed gas, turn off gas flow at regulator as well.
2.Switch off stir plates and wipe down their surfaces with mild detergent diluted in warm water. Wipe dry with paper towel.
3.Unplug the leads connecting the pH and temperature sensor probes to base unit CPU from the vessel. Recap pH probe.
4.Using bulldog clips, clamp the ends of the medium feed, and acid and base tubing, ∼10 cm from the respective ports on the bioreactor lid. Cut each of these tubing lengths ∼6 cm from the vessel lid and tie a knot on the remnant tubing connected to the vessel.
5.Use bulldog clips to clamp the gas addition tubing both before and after the 50-mm filter, ∼6 cm from each end.
6.Cut a 25- × 15-cm piece of aluminum foil. Wrap the large filter with this foil along with the sparger tubing connector.
7.Cut a 10- × 10-cm strip of aluminum foil. Remove the 25-mm filter from the tube connected to the condenser port. Wrap the end of the tubing connected to the condenser with the foil strip.
8.Ensure the vessel is no longer connected to the base unit and unclip vessel holder in order to remove the vessel. Move to a vessel stand and place stand with vessel into an autoclavable high-sided tray. Autoclave on a liquid 30 cycle (or as appropriate for the instrument used).
9.Remove bulldog clip from the condenser tubing. Remove filter from the end of the condenser tubing. Cut a 3- × 5-cm piece of aluminum foil to wrap the end of the condenser tubing.
10.During the autoclave run, clean the base unit by wiping down all surfaces with soapy water, rinsing with deionized water, and wiping with paper towel to thoroughly dry. Carefully remove each pump head from its support pins and clean behind each before replacing them.
11.Remove vessel from the autoclave and cool for at least 1 hr, or until the glass vessels can be handled comfortably.
12.Use the razor blade to make slits in all of the tubing where it is connected to the vessel ports, to facilitate removal. Dispose of tubing appropriately.
13.Unscrew sampling port and remove septum and collar. Clean with soapy water, rinse in deionized water, and dry thoroughly. The septum can be reused if there are minimal punctures and none interfere with the barrier. Otherwise, dispose of septum appropriately and place a new septum in the collar.
14.Remove pH probe from the port. Rinse with water and gently scrub with a sponge to remove any debris on the outside of the probe. Store in recommended storage solution.
15.Unclamp vessel lid lock and carefully remove lid from the glass vessel.
16.Wash all areas of the lid assembly with soapy water, using the sponge to gently remove any biofouling. Rinse all surfaces and ports with deionized water and run deionized water through each of the ports to ensure they are clear.
17.Place lid assembly on a clean piece of paper towel to air dry overnight.
18.Dispose of the autoclaved vessel contents by pouring them down the sink with ∼1 L of water, or into a suitable liquid waste receptacle, as appropriate to local laboratory regulations.
19.Clean vessel using soapy water, using the sponge to thoroughly remove all debris from the vessel walls. Rinse with deionized water and invert onto a clean piece of paper towel. Leave to dry overnight.
20.Use soapy water to clean the vessel holder, rinse, and leave to dry overnight.
21.Once dry, loosely place lid assembly into the glass vessel, and return glass vessel to the vessel stand. Store in a dust-free area until next use.
Support Protocol 7: CLEANING BIOREACTOR SUPPORT BOTTLES
This protocol details the cleaning procedures for the effluent, acid, base, and air trap bottles.
Additional Materials (see also Basic Protocol 5)
-
Mild detergent (such as Versa-Clean; Thermo Fisher Scientific, cat. no. 18-200-700)
-
Autoclavable, high sided plastic tray (54.3-cm length × 43.5-cm width × 13-cm height; Thermo Fisher Scientific, cat. no. 6900-0020)
-
Non-abrasive sponge (e.g., Scotch-Brite no scratch scrub sponge)
-
Bottle brush (e.g., Thermo Fisher Scientific, cat. no. 03-570C)
1.Disconnect effluent bottle and air trap bottles from each other by removing the tubing. Tie a knot at the ends of the tubing connected to each of the bottles. If any ports on the tops of the bottles are left open, close them off by adding a 10-cm length of tubing, knotted 1 cm from the end.
2.Loosen the caps of the effluent and air trap bottles by one or two turns. Place into an autoclavable high sided tray and autoclave on a liquid 60 cycle (or as appropriate for the autoclave used).
3.Remove all the tubing from the effluent and trap bottles and discard appropriately. Dispose of any liquid in the bottles by pouring down the sink with plenty of water or by pouring into a suitable waste receptacle for disposal, according to local laboratory regulations.
4.Wash bottles with soapy water, using the bottle brush to remove as much biofouling as possible. Rinse thoroughly with deionized water.
5.Wash lids in soapy water and rinse in deionized water. Run deionized water through the ports of the lids to remove any debris.
6.Dry bottles upside down in a bottle drying rack to air dry overnight. Place lids on paper towel to air-dry overnight. Cap bottles and store in a dust-free area.
7.Remove pump tubing from the acid, base, and medium bottles. Wash in soapy water, rinse with deionized water, and set on paper towel to dry.
8.Dispose of any unused acid and base solutions appropriately, according to local regulations.
9.Remove all other tubing from the acid, base, and medium bottles and dispose of appropriately.
10.Dispose of any unused medium by pouring down the sink and flushing with a constant flow of water (∼1:1 volume of the medium being disposed), or by pouring into a suitable liquid waste receptacle, according to local laboratory regulations.
11.Clean all the bottles, caps, and stir bar with mild detergent, scrubbing with bottle brush and sponge as appropriate. Flush any ports on bottle caps with deionized water. Rinse all apparatus thoroughly with deionized water and air dry on a bottle drying rack overnight. Cap bottles and store in a dust-free area.
12.Wash pump tubing with soapy water and rinse thoroughly with deionized water, taking care to flush water through the tubing. Air dry on a piece of paper towel overnight. Store in a dust-free area.
REAGENTS AND SOLUTIONS
Freezing medium
- 12 g Carnation instant skim milk powder
- 98 ml deionized water
- 2 ml glycerol
- Sterilize by autoclaving in a liquid 30 cycle (or as appropriate for the given instrument).
- Store at 4°C for up to 1 month.
Hemin solution, 5 mg/ml
- 0.5 g hemin chloride (MilliporeSigma, cat. no. 3741)
- 5 ml 2 N sodium hydroxide (NaOH; Thermo Fisher Scientific, cat. no. S318-3)
- Deionized water to 100 ml
- Filter-sterilize solution using 0.22-µm filter.
- Store at 4°C protected from light for up to 1 month.
Menadione solution, 1 mg/ml
- 0.1 g menadione (MilliporeSigma, cat. no. M-9429)
- 100 ml of 100% ethanol (Greenfield Global, cat. no. P016EAAN)
- Store at 4°C protected from light for up to 1 month.
Supplemented tryptic soy broth (sTSB)
- 3 g BD Bacto tryptic soy broth (Thermo Fisher Scientific, cat. no. DF0370-17-3)
- 100 ml deionized water
- 100 µl menadione solution (see recipe)
- 100 µl hemin solution (see recipe)
- Filter-sterilize solution using 0.22-µm filter.
- Store at 4°C for up to 1 month.
COMMENTARY
Background Information
The use of bioreactors is an important tool for studying complex communities of microbes present in the human gut. Early attempts to cultivate communities of microbes from human stool used crude medium formulations, the basis of which were masticated food items such as carrots, lettuce, and celery (Miller & Wolin, 1981). Results from these early experiments showed evidence of fermentation, microbial growth, and the survival of anaerobic bacteria, all measures of some degree of success which, in turn, encouraged further development and refinement of these bioreactor systems. For example, carbon sources that would naturally resist digestion in the upper gastrointestinal tract were included as substrates in medium that mimicked the distal colonic environment (Macfarlane et al., 1998). In Table S1, we provide a historical overview of medium used in different in vitro models. As well as improvements in medium formulations, the model systems themselves evolved over time. Early experimentation was done using batch fermentation experiments, and later these systems were adapted to work under continuous flow, with some models using multi-stage reactors and/or elements to encourage biofilm growth (Allison et al., 1989; McDonald et al., 2015; Edwards et al., 1985). Using the single-stage bioreactor described here, our laboratory has been able to cultivate diverse microbes including bacteriophages (Attai et al., 2022; McDonald et al., 2013).
Regardless of the medium type or model used, the overall goal of the work presented here is to create an in vitro system capable of replicating the environment of the human gastrointestinal tract. These in vitro systems provide a greater level of control over the environment to which the microbiota is exposed than is possible with an in vivo model, and also easily allows for the simulation of dietary changes or other perturbational stresses as a result. A feature of the bioreactor system is that, by nature, the microbial communities are grown without host influence. Depending on the experimental goals, this can be seen as both an advantage or a disadvantage: on the one hand, the ecosystem under study can be examined in isolation of host factors, decoupling complex interactions to determine the importance of either microbes or host driven elements to a given system; on the other hand, this decoupling may be seen as detrimental to the model. However, it is possible to add host elements back to a model bioreactor system, for example by incorporating host components such as mucins or bile salts into the medium (Engevik et al., 2021; McDonald et al., 2013; McDonald et al., 2015), or by generating add-on flow systems across relevant tissue culture cell lines (Kim et al., 2012; Ulluwishewa et al., 2015). However experiments are performed, one of the more endearing aspects of model systems is that samples can be withdrawn regularly and processed rapidly; this is particularly useful for metabolomics (for example, for measurement of volatile metabolites that might otherwise be quickly absorbed by a host; Current Protocols article: Ganobis et al., 2020; Scheppach, 1994), or transcriptomics approaches.
The bioreactor model described in these protocols is designed to replicate the conditions found in the adult human colon. Specifically, the liquid content of the flow system as described most accurately models the proximal colon (Cremer et al., 2017), which is also the site of the majority of short chain fatty acid production, and the highest growth rates for the microbiota (Cremer et al., 2017; Martinez-Guryn et al., 2019). The microbial density of the proximal colon is ∼109 cells/ml (Marteau et al., 2001), which is similar to the maximal amount we observe in the model system described here.
Critical Parameters
The selection of growth medium type for bioreactor vessel operation is an important consideration for the experimental design. In this set of protocols, we have outlined the conditions for culture of the adult human colonic microbiome, and the medium we describe can be modified to represent a host of dietary inputs, including, for example, high protein or high fiber conditions. When considering medium feed components for addition to the bioreactor model, as well as the amount of substrate to add, it is important to consider the physical properties of each substrate, for example, whether it can withstand steam sterilization or should be made into a solution and filter-sterilized, or irradiated and made into a suspension, before adding to the medium base. Some dietary components, for example gums or carrageenan, form gels in solution which may clog tubing lines, and thus their concentrations should be taken into consideration when formulating medium recipes.
A further factor to consider is the type of gas used for maintaining bioreactor pressure (and displacing oxygen). Here we describe the use of nitrogen gas to maintain an anaerobic environment, and in our hands this is sufficient to support the environmental conditions of most complex fecal microbial ecosystems. However, in some cases more simple, synthetic communities of specific microbial species may be the target for experimentation. If these species are auxotrophic, e.g., carbon dioxide or hydrogen, the use of mixed anaerobic gas such as 80:10:10 N2/H2/CO2 should be considered instead of pure nitrogen.
Bioreactor vessels supporting human gut microbial ecosystems should always be run under Biosafety Level 2 (BSL-2) conditions or equivalent. If known pathogenic species are suspected to be present, or the experimental intent is to add them during the run, the bioreactors should be housed in a suitable secondary containment environment, for example a large-capacity BSL-2 containment hood.
Running multiple bioreactors
In our experience, a single operator can comfortably run two bioreactor vessels simultaneously. In this circumstance, acid and base bottles can be shared between both vessels, although daily monitoring (and refilling if necessary) of the acid and base itself is recommended. Maintaining more than four vessels is a considerable amount of work for a single operator and dedicated time, or technical assistance, would be an asset in this situation. However, there are many experimental scenarios in which it is desirable to perform bioreactor experiments simultaneously rather than sequentially. For example, a two vessel setup is a common approach in drug exposure experiments where identically inoculated vessels are differentially exposed to either a solvent (vehicle) control or the test substance of interest at the same point in time during a run. Using the Infors Multifors 2 system, a total of six bioreactor vessels—labeled vessels A-F under the Controller menu—can be run simultaneously per controller unit. To increase the number of bioreactor vessels, simply scale the materials listed (in the single bioreactor protocols described above) by the number of vessels desired.
There are, however, two exceptions with regard to materials that do not scale linearly. The first is the 2-L air trap bottle setup and associated tubing materials listed in Support Protocol 3. Each 2-L air trap as described with four fitted metal pipes can support a total of three bioreactor vessels (i.e., three waste line inputs with one gas escape output). Thus, one 2-L air trap is needed when running one to three bioreactors, whereas two 2-L air traps are needed when running four to six bioreactors, and so on.
The second exception is related to acid and base bottle setups. Each acid and base bottle can be adjusted to have two output lines, and thus can support two bioreactor vessels. Accordingly, one set of acid/base bottles are needed for one to two bioreactors, two sets for three to four bioreactors, three sets for five to six bioreactors, and so on.
Troubleshooting
There are several common problems that can be encountered when running the described bioreactor system. These and their potential solutions are summarized in Table 1.
Observation | Possible cause | Solution |
---|---|---|
Vessel is filled with culture surpassing intended volume where the effluent pipe is placed | There is a gas leak from one of the ports | To determine if there is a gas leak from a port, increase gas pressure and spray 70% ethanol around ports on the lid of the vessel. If bubbles are present around a port, then gas is leaking through there. Tighten loose ports or pH probe, taking care not to disturb other connections. |
The condenser tubing is unclamped | Check that a bulldog clip is secure on the pinched condenser tubing | |
The septum is compromised | Check that the septum is intact, free of cracks or holes. Water inside the sampling port is usually a sign of an improperly sealed or otherwise compromised septum. If the septum is damaged, sterilize a new septum set in a septum collar by wrapping in aluminum foil and autoclaving. To switch septa, turn up the gas to create positive pressure to lessen the chance of contamination. Remove the sampling port extension with cap and quickly swap the compromised septum with the new, sterile septum, placing over the sampling port with disinfected tweezers. Replace sampling port extension and cap. | |
Biofouling has clogged the effluent tubing | Massage tubing to free any biofilm buildup from the edges of the silicone tubing. Turn up the gas pressure to aid in removing any clogs. | |
Biofouling has clogged the effluent pipe | Following steps 9-13 during the inoculation procedure in Basic Protocol 3, attach a 5-ml Luer-Lok syringe containing sterile deionized water to the effluent pipe rather than a syringe containing an inoculum, and flush up and down until the clog is released. Replace effluent tubing. | |
Medium is not dripping into vessel | Medium components are clogged in the medium tubing |
Massage medium tubing line and pump hose to loosen any clogged medium components. Using the medium pump switch, move it back and forth to push medium through the pump hose to ensure it is clear. If your medium formulation contains reagents prone to clumping you may need to investigate alternative reagents or consider ways to add larger particulates as a supplement (see optional steps of Support Protocol 1) |
Biofouling is clogging the medium addition port | Biofouling back-growth from the bioreactor vessel will appear as light-colored material in the medium line near the addition port. Medium feed rate can be increased to 100% for a short amount of time (30 s) to loosen the blockage. If this is not successful, replace medium bottle with a freshly prepared one, or exchange medium tubing for autoclaved tubing aseptically in a laminar flow hood. | |
Medium, acid, or base are not being added to the system at the correct rate | Air is entering the tubing, reducing pump pressure |
Ensure that the medium, acid, or base line is sufficiently lowered onto the bioreactor vessel addition port and on the metal pipe on the bottle cap Check that the medium bottle is not being stirred so fast as to introduce air into the medium tubing. Stir rate may need to be reduced as the medium is consumed. Strong acid can corrode the metal piping on the bottle cap over time, leading to a poor seal. Wrap tubing and metal pipe with Parafilm to maintain the seal. |
The impellers are not stirring | The center bearing has come out of alignment | Insert a sterile needle through the sampling port and move the center bearing back below the magnetic coupling |
There has been a mechanical failure of the stir plate on that vessel position | If a neighboring position of the Multifors unit is available and the pH probe line can reach a neighboring position, prepare to move the vessel into that position. Place a vessel containing water in the new place and turn on the controls to warm up that position. Once the temperature is close to 37°C, clamp the heating jacket to release the vessel and gently lift the vessel up and out of its current place. Place the vessel in the metal holder over new position. Keep the pH probe attached from the previous position. The gas, medium, and acid/base lines can be left on in the previous position or changed to the new position. Change temperature probe to that of the new position. | |
pH is fluctuating beyond the expected scope | pH probe sensor is not correctly attached | Ensure that the pH probe sensor line is tightly fastened to the top of the pH probe. It can become loose when changing medium or sampling if care is not taken to leave it in place. |
Opacity of medium in vessel becomes noticeably and rapidly less opaque (during <36 hr), less dense | The vessel has washed-out by demanding excessive acid or base stocks | To determine if there is a faulty pH probe, navigate to the Trends menu on the Infors programming interface. Select pH and adjust the time range you would like to investigate. If the pH reading fluctuated more than expect for community fermentation, it is likely that the probe has had a mechanical failure and should not be trusted for future experiments. Vessel contents will no long be viable; proceed with bioreactor takedown and clean up in Basic Protocol 6. |
Medium in vessel turns darker (or even black) during run | No pH fluctuations noted | This requires no additional action. In our experience this is caused by blooms of certain bacteria and does not necessarily indicate a problem. |
Understanding Results
Interpretation of results obtained from running microbial ecosystems in vessels such as this will very much depend on the goal of the experiment performed. In general, it is important to understand the composition of the ecosystem being modeled. If the bacterial composition is of interest, 16S rRNA gene profiling can be performed using standard high throughput sequencing methods. Should a broader microbial analysis be desired, a metagenomic approach can be used. Samples should be taken across the entire time period of a run so that comparative analysis can be done; this is particularly helpful when trying to determine when equilibrium within an ecosystem is attained. For this, a 16S rRNA gene profiling approach combined with moving window analysis can be performed (Oliphant et al., 2019), and it is important to extract genomic DNA (gDNA) and run sequencing experiments in batches to avoid as much bias as possible; for this reason, storing collected samples at −80°C until enough for a batch have been collected is imperative. Metabolomic or transcriptomic analysis can also be used to understand the functional output of a given ecosystem. For metabolomic analysis, our laboratory uses an inexpensive one dimensional (1 D) proton nuclear magnetic resonance (1H NMR) approach to model metabolic output in a quantitative fashion, again using moving window analyses to infer equilibrium (Current Protocols article: Ganobis et al., 2020). Mass spectrometry approaches could also be used. Perturbational experiments (for example introducing a particular substrate or a dietary change) should only be performed when an ecosystem is at stable equilibrium, to avoid confounders. Following perturbation, the ecosystem should be left without further interference, under pre-perturbation conditions, for approximately a week to infer any potential for recovery.
Time Considerations
The preparation of bioreactor medium with sterilization time (Basic Protocol 1) typically takes 4 hr. The preparation of the autoclaved components of the medium can take place on a separate day from the filter-sterilized additions.
Preparing the bioreactor vessels (Basic Protocol 2) takes ∼4 hr. The time it takes the medium to be pumped into the vessel is variable depending on the size of the vessel. To fill a vessel to 200 ml takes approximately an hour. The vessel should be left for at least 24 hr and up to 48 hr as a sterility check prior to inoculation; no changes in the color of the medium that would indicate microbial growth should be seen during this time. This may be further checked by taking a small sample and inoculating a permissive broth culture such as tryptic soy broth.
Preparing daily medium supplements (Support Protocol 1) takes ∼4 hr including autoclave time, making additions to the bioreactor vessel (Support Protocol 1) takes ∼15 min.
Preparing the acid, base, effluent, and air trap bottles (Support Protocols 2 and 3 together) takes ∼2 hr including autoclave time.
The preparation of acid and base solutions (Support Protocols 4 and 5) takes 15 min.
Preparing the inoculum for the bioreactor and inoculating the vessel (Basic Protocol 3, Alternate Protocols 1 through 3) takes ∼1 hr depending on reducing time if using an anaerobic chamber. The vessel is also grown in batch for 24 to 72 hr prior to turning on the medium flow rate and proceeding with the experiment.
If inoculating with fecal samples, the vessels should be run for at least 2 weeks but with an aim for at least 28 days to reach a community equilibrium. For more simple synthetic bacterial communities (up to 50 strains), 1 week is usually sufficient.
Sampling the bioreactor (Basic Protocol 4) takes ∼10 min per vessel. Harvesting the contents of the vessel at the conclusion of the experiment (Basic Protocol 5) takes ∼45 min.
Time taken to sterilize and clean the sampling needles (Support Protocol 6) is dependent on the number of needles being cleaned but generally takes ∼1 min per needle, not including the autoclave time.
Cleaning the vessel, effluent bottle, and support bottles (Basic Protocol 6 and Support Protocol 7) will take ∼3 hr in total including autoclave time.
Acknowledgments
The authors acknowledge funding support for the development of this model and the associated article through grants to EAV from the Canadian Institutes of Health Research, Natural Sciences and Engineering Research Council, the Canada Research Chairs program, and the Canada Foundation for Innovation.
Author Contributions
Connor M. Gianetto-Hill : Methodology, visualization, writing original draft; Sarah J. Vancuren : Methodology, visualization, writing original draft; Brendan Daisley : Methodology, visualization, writing review and editing; Simone Renwick : Methodology, writing review and editing; Jacob Wilde : Methodology, writing review and editing; Kathleen Schroeter : Investigation, validation, writing review and editing; Michelle C. Daigneault : Methodology, validation, writing review and editing; Emma Allen-Vercoe : Conceptualization, funding acquisition, methodology, supervision, writing review and editing.
Conflict of Interest
We describe herein the use of Infors HT bioreactors, although none of the authors are affiliated with Infors HT, and Infors has not been involved in the preparation of this article in any way. Author EAV has used bioreactor vessels in the described manner to drive research that led to the development of a spin-off company, NuBiyota LLC, by which KS and MD are or were employed. EAV is the co-founder and Chief Scientific Officer (CSO) of NuBiyota, however NuBiyota has had no influence over the contents of this paper, and was not involved in providing funding support.
Open Research
Data Availability Statement
Data sharing not applicable to this article as no datasets were generated or analyzed during the current study.
Supporting Information
Filename | Description |
---|---|
cpz1737-sup-0001-TableS1.docx40.5 KB | Supplemental Figure S1: Images of the pump head with and without tubing and after placement on the drive shaft of the control unit. (A) Front of empty pump head. (B) Back of empty pump head. (C) Front of pump head with pump tubing. (D) Back of pump head with pump tubing. (E) Pump head on drive shaft with pump tubing. (F) Pump head on drive shaft without pump tubing. |
cpz1737-sup-0002-FigureS1.png570.9 KB | Supplemental Table S1: Comparison of growth medium compositions for bioreactor experiments supporting human gut microbiota, as published in the scientific literature. Units in g/L. |
Please note: The publisher is not responsible for the content or functionality of any supporting information supplied by the authors. Any queries (other than missing content) should be directed to the corresponding author for the article.
Literature Cited
- Allison, C., McFarlan, C., & MacFarlane, G. T. (1989). Studies on mixed populations of human intestinal bacteria grown in single-stage and multistage continuous culture systems. Applied and Environmental Microbiology , 55(3), 672–678. https://doi.org/10.1128/aem.55.3.672-678.1989
- Attai, H., Wilde, J., Liu, R., Chopyk, J., Garcia, A. G., Allen-Vercoe, E., & Pride, D. (2022). Bacteriophage-mediated perturbation of defined bacterial communities in an in vitro model of the human gut. Microbiology Spectrum , 10(3), e0113522. https://doi.org/10.1128/spectrum.01135-22
- Bayer, G., Ganobis, C. M., Allen-Vercoe, E., & Philpott, D. J. (2021). Defined gut microbial communities: Promising tools to understand and combat disease. Microbes and Infection , 23(6-7), 104816. https://doi.org/10.1016/j.micinf.2021.104816
- Cremer, J., Arnoldini, M., & Hwa, T. (2017). Effect of water flow and chemical environment on microbiota growth and composition in the human colon. Proceedings of the National Academy of Sciences of the United States of America , 114(25), 6438–6443. https://doi.org/10.1073/pnas.1619598114
- Edwards, C. A., Duerden, B. I., & Read, N. W. (1985). Metabolism of mixed human colonic bacteria in a continuous culture mimicking the human cecal contents. Gastroenterology , 88(6), 1903–1909. https://doi.org/10.1016/0016-5085(85)90017-4
- Engevik, M. A., Danhof, H. A., Auchtung, J., Endres, B. T., Ruan, W., Basseres, E., Engevik, A. C., Wu, Q., Nicholson, M., Luna, R. A., Garey, K. W., Crawford, S. E., Estes, M. K., Lux, R., Yacyshyn, M. B., Yacyshyn, B., Savidge, T., Britton, R. A., & Versalovic, J. (2021). Fusobacteriumnucleatum adheres to clostridioides difficile via the RadD adhesin to enhance biofilm formation in intestinal mucus. Gastroenterology , 160(4), 1301–1314.e1308. https://doi.org/10.1053/j.gastro.2020.11.034
- Ganobis, C. M. (2022). Characterizing the mouse gut microbiome and improving mouse gut-derived microbial communities for mouse model studies. (Identifier: https://hdl.handle.net/10214/27392) [Doctoral dissertation, University of Guelph]. The Atrium, University of Guelph.
- Ganobis, C. M., Al-Abdul-Wahid, M. S., Renwick, S., Yen, S., Carriero, C., Aucoin, M. G., & Allen-Vercoe, E. (2020). 1D (1) H NMR as a tool for fecal metabolomics. Current Protocols in Chemical Biology , 12(3), e83. https://doi.org/10.1002/cpch.83
- Human Microbiome Project Consortium. (2012). Structure, function and diversity of the healthy human microbiome. Nature , 486, 207–214. https://doi.org/10.1038/nature11234
- Kim, H. J., Huh, D., Hamilton, G., & Ingber, D. E. (2012). Human gut-on-a-chip inhabited by microbial flora that experiences intestinal peristalsis-like motions and flow. Lab on A Chip , 12(12), 2165–2174. https://doi.org/10.1039/c2lc40074j
- Macfarlane, G. T., Macfarlane, S., & Gibson, G. R. (1998). Validation of a three-stage compound continuous culture system for investigating the effect of retention time on the ecology and metabolism of bacteria in the human colon. Microbial Ecology , 35(2), 180–187. https://doi.org/10.1007/s002489900072
- Marteau, P., Pochart, P., Dore, J., Bera-Maillet, C., Bernalier, A., & Corthier, G. (2001). Comparative study of bacterial groups within the human cecal and fecal microbiota. Applied and Environmental Microbiology , 67(10), 4939–4942. https://doi.org/10.1128/AEM.67.10.4939-4942.2001
- Martinez-Guryn, K., Leone, V., & Chang, E. B. (2019). Regional diversity of the gastrointestinal microbiome. Cell Host & Microbe, 26(3), 314–324. https://doi.org/10.1016/j.chom.2019.08.011
- McDonald, J. A., Fuentes, S., Schroeter, K., Heikamp-deJong, I., Khursigara, C. M., de Vos, W. M., & Allen-Vercoe, E. (2015). Simulating distal gut mucosal and luminal communities using packed-column biofilm reactors and an in vitro chemostat model. Journal of Microbiological Methods , 108, 36–44. https://doi.org/10.1016/j.mimet.2014.11.007
- McDonald, J. A., Schroeter, K., Fuentes, S., Heikamp-Dejong, I., Khursigara, C. M., de Vos, W. M., & Allen-Vercoe, E. (2013). Evaluation of microbial community reproducibility, stability and composition in a human distal gut chemostat model. Journal of Microbiological Methods , 95(2), 167–174. https://doi.org/10.1016/j.mimet.2013.08.008
- Miller, T. L., & Wolin, M. J. (1981). Fermentation by the human large intestine microbial community in an in vitro semicontinuous culture system. Applied and Environmental Microbiology , 42(3), 400–407. https://doi.org/10.1128/aem.42.3.400-407.1981
- Minekus, M., Smeets-Peeters, M., Bernalier, A., Marol-Bonnin, S., Havenaar, R., Marteau, P., Alric, M., Fonty, G., & Huis in't Veld, J. H. (1999). A computer-controlled system to simulate conditions of the large intestine with peristaltic mixing, water absorption and absorption of fermentation products. Applied Microbiology and Biotechnology , 53(1), 108–114. https://doi.org/10.1007/s002530051622
- Molly, K., Vande Woestyne, M., & Verstraete, W. (1993). Development of a 5-step multi-chamber reactor as a simulation of the human intestinal microbial ecosystem. Applied Microbiology and Biotechnology , 39(2), 254–258. https://doi.org/10.1007/BF00228615
- Oliphant, K., Parreira, V. R., Cochrane, K., & Allen-Vercoe, E. (2019). Drivers of human gut microbial community assembly: Coadaptation, determinism and stochasticity. The ISME Journal , 13(12), 3080–3092. https://doi.org/10.1038/s41396-019-0498-5
- Renwick, S., Ganobis, C. M., Elder, R. A., Gianetto-Hill, C., Higgins, G., Robinson, A. V., Vancuren, S. J., Wilde, J., & Allen-Vercoe, E. (2021). Culturing human gut microbiomes in the laboratory. Annual Review of Microbiology , 75, 49–69. https://doi.org/10.1146/annurev-micro-031021-084116
- Scheppach, W. (1994). Effects of short chain fatty acids on gut morphology and function. Gut , 35(1 Suppl), S35–38. https://doi.org/10.1136/gut.35.1_suppl.s35
- Ulluwishewa, D., Anderson, R. C., Young, W., McNabb, W. C., van Baarlen, P., Moughan, P. J., Wells, J. M., & Roy, N. C. (2015). Live Faecalibacterium prausnitzii in an apical anaerobic model of the intestinal epithelial barrier. Cellular Microbiology , 17(2), 226–240. https://doi.org/10.1111/cmi.12360
- Van den Abbeele, P., Roos, S., Eeckhaut, V., MacKenzie, D. A., Derde, M., Verstraete, W., Marzorati, M., Possemiers, S., Vanhoecke, B., Van Immerseel, F., & Van de Wiele, T. (2012). Incorporating a mucosal environment in a dynamic gut model results in a more representative colonization by lactobacilli. Microbial Biotechnology , 5(1), 106–115. https://doi.org/10.1111/j.1751-7915.2011.00308.x
- Vandeputte, D., Kathagen, G., D'Hoe, K., Vieira-Silva, S., Valles-Colomer, M., Sabino, J., Wang, J., Tito, R. Y., De Commer, L., Darzi, Y., Vermeire, S., Falony, G., & Raes, J. (2017). Quantitative microbiome profiling links gut community variation to microbial load. Nature , 551(7681), 507–511. https://doi.org/10.1038/nature24460
- Vijay, A., & Valdes, A. M. (2022). Role of the gut microbiome in chronic diseases: A narrative review. European Journal of Clinical Nutrition , 76(4), 489–501. https://doi.org/10.1038/s41430-021-00991-6
- Yen, S., McDonald, J. A., Schroeter, K., Oliphant, K., Sokolenko, S., Blondeel, E. J., Allen-Vercoe, E., & Aucoin, M. G. (2015). Metabolomic analysis of human fecal microbiota: A comparison of feces-derived communities and defined mixed communities. Journal of Proteome Research , 14(3), 1472–1482. https://doi.org/10.1021/pr5011247