Streptozotocin-Induced Diabetic Models in Mice and Rats
Brian L. Furman, Brian L. Furman
high-fat
hyperglycemia
insulin deficiency
insulin resistance
insulitis
mouse or rat
nicotinamide
streptozotocin
type 1 diabetes mellitus
type 2 diabetes mellitus
Abstract
Streptozotocin (STZ) is an antibiotic that causes pancreatic islet β-cell destruction and is widely used experimentally to produce a model of type 1 diabetes mellitus (T1DM). Detailed in this article are protocols for producing STZ-induced insulin deficiency and hyperglycemia in mice and rats. Also described are protocols for creating animal models for type 2 diabetes using STZ. These animals are employed for assessing the pathological consequences of diabetes and for screening potential therapies for the treatment of this condition. © 2021 The Authors.
INTRODUCTION
Streptozotocin (STZ) was initially isolated from Streptomyces achromogenes in 1960, with its diabetogenic properties not described until 1963 (Rakieten, Rakieten, & Nadkarni, 1963). This action was characterized by Junod, Lambert, Stauffacher, and Renold (1969) based on earlier work (Junod et al., 1967) showing that the diabetogenic effects are due to selective destruction of pancreatic islet β-cells. As a result of this action, the animals experience insulin deficiency, hyperglycemia, polydipsia, and polyuria, all of which are characteristic of human type 1 diabetes mellitus (T1DM; Kolb, 1987).
Several animal species, including the mouse, rat, and monkey, are sensitive to the pancreatic β-cell cytotoxic effects of STZ, with the rabbit being less so (Lazar, Golden, Furman, & Lieberman, 1968). Currently, STZ is most often used to induce diabetes in rats and mice.
Described in this article are two protocols used to produce STZ-induced diabetes in mice (Basic Protocol 1) and rats (Basic Protocol 2). Basic Protocol 1 employs multiple administrations of low-dose STZ to produce diabetic mice, and is increasingly used as an animal model for diabetes. Its growing popularity is due to the fact that the resultant pathology resembles human T1DM with chronic pancreatic islet inflammation, insulitis, and insulin deficiency; its lower cost compared to other animal models of T1DM, such as spontaneously diabetic BB (Biobreeding) rats (Lenzen, 2017) or NOD (Non-obese Diabetic) mice (Pearson, Wong, & Wen, 2016), is also an attraction. The procedure described in Basic Protocol 2 is used in rats to induce diabetes with STZ.
Although these models are valuable for evaluating treatments for T1DM, the majority of diabetic patients (∼90%) suffer from type 2 diabetes (T2D). The global prevalence of diabetes is predicted to be 9% by 2030 (Wou, Unwin, Huang, & Roglic, 2019), with a predicted world population of 8.5 billion, which would suggest around 690 million people with T2 diabetes. These people show an increased mortality compared with non-diabetic populations, for example, a 28% increase in mortality from coronary heart disease (de Souza et al., 2015). Two potentially useful STZ models of T2D are being developed, both of which are included in this article. The first (Basic Protocol 3) entails concurrent administration of nicotinamide to partially protect the β-cells against STZ (Masiello et al., 1998). This model is based on the work of Junod et al. (1969), who investigated the earlier finding of Schein, Cooney, and Vernon (1967) that nicotinamide protects against the diabetogenic effect of STZ. This compound combination produces a model of insulin-deficient, but not insulin-resistant, T2D. It is characterized by stable, moderate hyperglycemia associated with an approximately 60% loss of β-cell function (Ghasemi, Khalifi, & Jedi, 2014; Masiello et al., 1998). However, as most patients with T2D display insulin resistance in addition to impaired insulin secretion, another model (Basic Protocol 4) has been developed to more accurately mimic the human condition. In this case, the animals are exposed to a high-fat diet to produce insulin resistance, followed by administration of a moderate dose of STZ to reduce β-cell capacity (Reed et al., 2000). The result is hyperglycemia, associated with hyperinsulinemia and insulin resistance (Chao et al., 2018).
NOTE : All experimental protocols using live animals must first be reviewed and approved by an Institutional Animal Care and Use Committee (IACUC) and must comply with the guidelines as established by the IACUC regarding the care and use of laboratory animals in scientific experiments. They must also comply with governmental legislation, such as the UK Animals (Scientific Procedures) Act, 1986, as amended in 2012 (https://www.gov.uk/government/uploads/system/uploads/attachment_data/file/308593/ConsolidatedASPA1Jan2013.pdf). The care of diabetic animals must take into account the marked glycosuria, polyuria, and polydipsia characteristic of the disease, with due attention given to access to drinking water, as well as to cage hygiene and comfort of the animals.
CAUTION : STZ should be handled with care, as it is a know carcinogen.
Basic Protocol 1: INDUCTION OF TYPE 1 DIABETES MELLITUS IN MICE USING REPEATED LOW DOSES OF STREPTOZOTOCIN
Streptozotocin (STZ) is a highly selective pancreatic islet β-cell-cytotoxic agent that is often administered at a single high dose to cause, within 48 hr, complete β-cell necrosis and diabetes (Kolb, 1987; also see Alternate Protocol). However, after administering multiple, low doses of STZ to mice for 5 days, Like and Rossini (1976) noted a delayed onset of hyperglycemia which, for kinetic reasons, could not be due to a direct, rapid, toxic action of the drug. This multiple, low-dose STZ approach only partially damages pancreatic islets, triggering an inflammatory process that causes the further loss of β-cell activity that ultimately results in insulin deficiency and hyperglycemia. This response more closely resembles T1DM in pathogenesis and morphologic changes than the single, high-dose STZ protocol (Kolb, 1987; Kolb-Bachofen, Epstein, Kiesel, & Kolb, 1988; Like & Rossini, 1976; Weide & Lacy, 1991). The multiple, low-dose STZ approach is now widely used to produce an animal model of T1DM (Wu & Huan, 2007).
Using this protocol, diabetes is induced by the administration of multiple, low doses of STZ (40 mg/kg, intraperitoneally, i.p.) to mice on 5 consecutive days. The model is used for testing the effectiveness of potential antidiabetic agents. Screening assays can entail administration of the test agent prior to and/or following induction of diabetes, depending on study objectives.
Materials
-
C57BL/6 or CD-1 male mice: ∼ 25 g, 8 to 12 weeks old (Jackson Laboratory or Taconic); 12 to 20 animals per treatment group are recommended; Balb/cJ mice are resistant to the induction of diabetes using this treatment regimen (see Zunino, Simons, Sambrook, & Gething, 1994).
-
Standard rodent chow diet (Harlan)
-
50 mM sodium citrate buffer (enzyme-grade; Fisher), pH 4.5: prepared immediately before use
-
Streptozotocin (STZ; Sigma)
-
10% (w/v) sucrose (Sigma): prepared just before use
-
Test compound(s)
-
Rodent cages
-
Temperature-, humidity-, and light-controlled housing
-
1.5-ml microcentrifuge tubes
-
Aluminum foil
-
1-ml syringes
-
25-G needles
-
One Touch Basic blood glucose monitoring system (Lifescan)
-
Additional reagents and equipment for injection of mice (see Current Protocols article: Donovan & Brown, 2006a) and blood collection from mice (see Current Protocols article: Donovan & Brown, 2006b)
Prepare animals
1.At least 5 days prior to initiating the experiment, house two to five male mice per cage at 24°C ± 1°C and 55% ± 5% humidity, with a 12-hr light-dark cycle (light on at 8:00 and off at 20:00). Allow the mice free access to food and water.
2.Weigh all mice accurately to 1 g and randomly divide them into control and experimental groups.
3.On experimental day 1, 4 hr prior to STZ treatment, remove all food from cages for all groups. Provide water as normal.
Treat animals with STZ
4.Weigh 4 mg of STZ into a 1.5-ml microcentrifuge tube and cover the tubes with aluminum foil; use one tube for three mice. Prepare the citrate buffer.
5.Immediately prior to injection, dissolve the STZ in 50 mM sodium citrate buffer (pH 4.5) to a final concentration of 4 mg/ml.
6.Using 1-ml syringes and 25-G needles, inject the STZ solution i.p. (see Current Protocols article: Donovan & Brown, 2006a) at 40 mg/kg (1.0 ml/100 g) in the experimental group animals. Inject an equal volume of citrate buffer (pH 4.5) i.p. into the control group mice.
7.Return the mice to their home cages. Provide free access to normal food and 10% sucrose water.
8.Repeat steps 3 to 7 on days 2 to 5 (the next four consecutive days).
9.On experimental day 6, replace the 10% sucrose water with regular water.
10a. For studies involving early-stage T1DM : On experimental day 14 (9 days after the last STZ injection), fast all mice for 6 hr (e.g., from 7:00-13:00). Analyze blood glucose from a tail-vein blood sample (see Current Protocols article: Donovan & Brown, 2006b) using a One Touch Basic blood glucose monitoring system to ensure hyperglycemia in the STZ-treated subjects.
10b. For re-test of animals failing the first test for diabetes and for studies involving later-stage T1DM : On experimental day 28, fast all mice for 6 hr (e.g., from 7:00-13:00). Quantify blood glucose from a tail-vein blood sample (see Current Protocols article: Donovan & Brown, 2006b) using a One Touch Basic blood glucose monitoring system to confirm STZ injection-induced hyperglycemia.
11a. To examine a test agent for its ability to correct diabetes or affect hyperglycemia : Begin treatment with a test hypoglycemic agent once the diabetic state is established as defined in step 10. Include groups that receive appropriate vehicle injections as a control. Maintain the duration of treatment with test substance according to the experimental design.
11b. To study a chronic condition or diabetic complications : Repeat, at week 7, steps 3 to 8, omitting the 10% sucrose water, to maintain hyperglycemia in the STZ-treatment group (Kunjathoor, Wilson, & LeBoeuf, 1996).
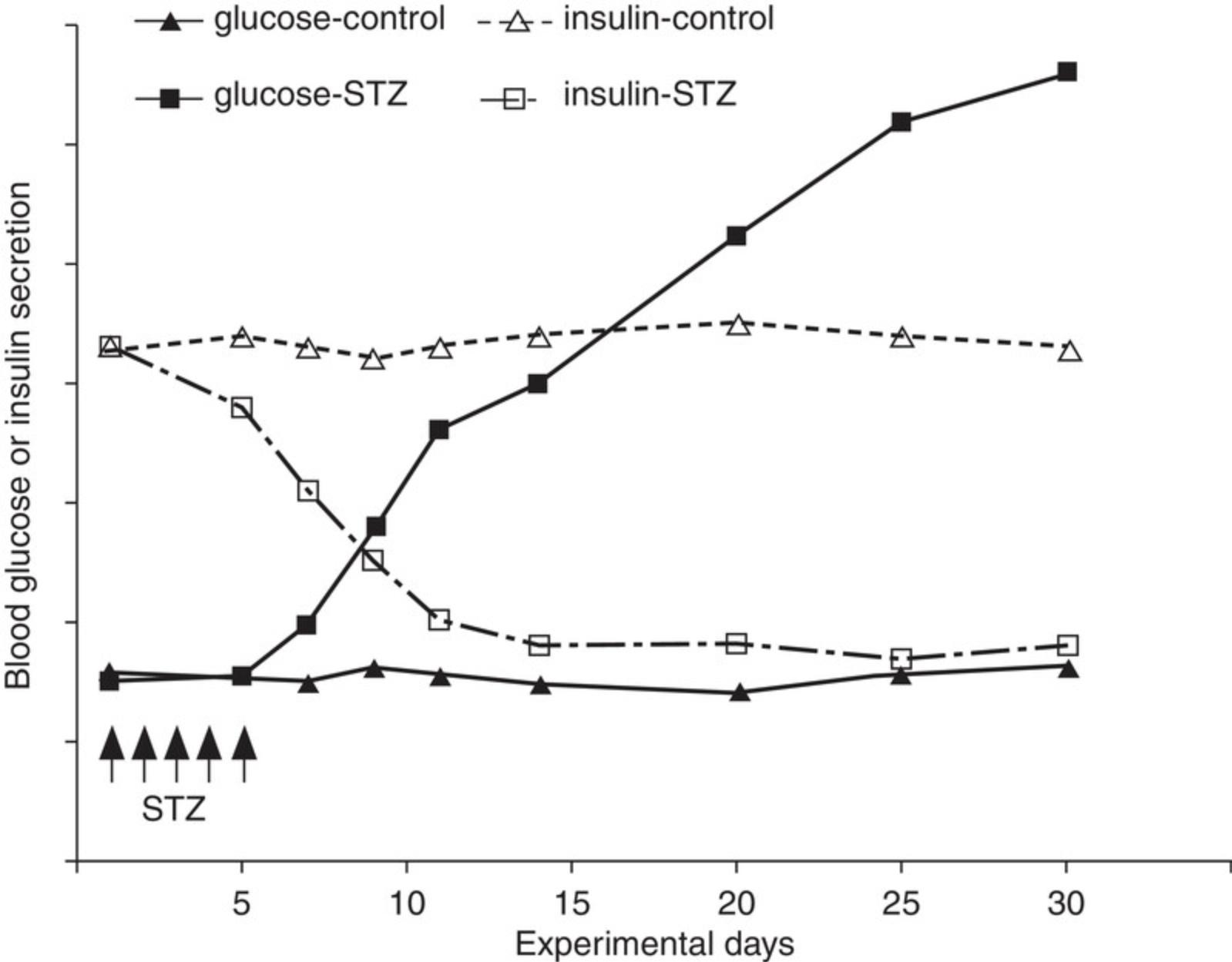
Alternate Protocol: INDUCTION OF TYPE 1 DIABETES MELLITUS IN MICE USING A SINGLE, HIGH DOSE OF STREPTOZOTOCIN
A single, high dose of STZ (200 mg/kg) is directly toxic to pancreatic β-cells, rapidly causing diabetes, with blood glucose concentrations of >500 mg/dl within 48 hr (Like & Rossini, 1976). Although multiple, low doses of STZ are associated with fewer toxic effects than a single, high dose of STZ, many investigators still prefer the single high-dose STZ approach for generating diabetic animals.
For materials, see Basic Protocol 1.
Prepare animals
1.At least 5 days prior to the initiating the experiment, house two to five male mice per cage at 24°C ± 1°C and 55% ± 5% humidity, with a 12-hr light-dark cycle (light on at 8:00 and off at 20:00) with free access to food and water.
2.Weigh all mice accurately to 1 g and randomly divide them into control and experimental groups.
3.On experimental day 1, 4 hr prior to STZ treatment, remove food from all animal cages. Provide water as normal.
Treat animals with STZ
4.Immediately prior to injection, dissolve the STZ in sodium citrate buffer (pH 4.5) to a final concentration of 20 mg/ml.
5.Inject STZ i.p. (see Current Protocols article: Donovan & Brown, 2006a) into the experimental animals at 200 mg/kg (1.0 ml/100 g). Inject an equal volume of citrate buffer (pH 4.5) i.p. into the control group mice.
6.Return the mice to their cages. Provide normal food and 10% sucrose water and closely monitor the mice every 2 hr for 12 hr for marked hypoactivity, unresponsiveness, or convulsions.
7.On experimental day 3, replace the 10% sucrose water with regular water.
8a. For studies involving early-stage T1DM : On experimental day 10, fast all mice for 6 hr (e.g., from 7 a.m. to 1 p.m.), then measure blood glucose via a tail-vein blood sample (see Current Protocols article: Donovan & Brown, 2006b) using a One Touch Basic blood glucose monitoring system to ensure hyperglycemia.
8b. For re-test of groups failing the first test for diabetes or for studies involving later-stage T1DM : On experimental day 21, fast all mice for 6 hr (e.g., from 7 a.m. to 1 p.m.). Test the blood glucose concentration in a tail-vein blood sample (see Current Protocols article: Donovan & Brown, 2006b) using a One Touch Basic blood glucose monitoring system to confirm that the STZ treatment induced hyperglycemia.
9a. To examine a test agent for its ability to correct diabetes or affect hyperglycemia : Once the diabetic state is confirmed as specified in step 8, treat the animals with a test anti-hyperglycemic agent. Include as controls animals that receive appropriate vehicle injections.
9b. To study a chronic condition or diabetic complications : Extend the protocol longer, depending on the experimental needs.
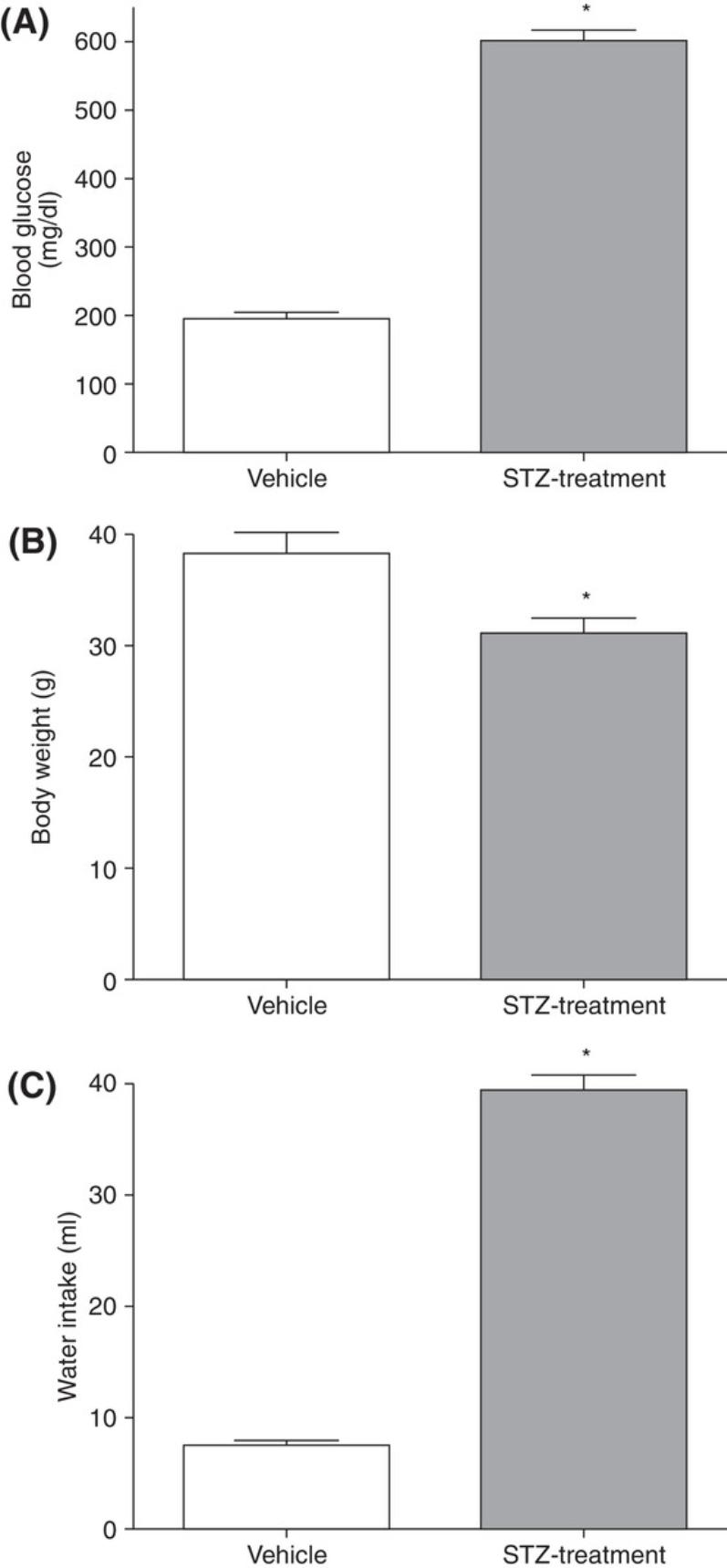
Basic Protocol 2: STREPTOZOTOCIN-INDUCED TYPE 1 DIABETES MELLITUS IN RATS
The rat is commonly used as an STZ-induced diabetic model. As in the mouse, the production of a diabetic state in rats is dependent on the dose of STZ (Arison, Ciaccio, Glitzer, Cassaro, & Pruss, 1967; Ganda, Rossini, & Like, 1976; Junod et al., 1969). The most frequently used procedure is to administer one dose of STZ (40 to 70 mg/kg) to rats aged 8 to 10 weeks (Brondum, Nilsson, & Aalkjaer, 2005). Many investigators use a single dose of approximately 65 mg/kg to establish diabetes using the procedure described in this protocol.
This protocol describes administration of a single dose of STZ (65 mg/kg, i.p.) to rats to generate a T1DM state. The diabetic rats can be used to study the pathogenesis of T1DM, as well as to evaluate antidiabetic agents (Bond, Failla, & Unger, 1983).
Materials
-
Sprague-Dawley or Wistar male rats: 150 to 200 g, 8 to 10 weeks old (Charles River Breeding Laboratories); 10 to 16 per treatment group recommended
-
Standard rodent chow diet (Harlan)
-
50 mM sodium citrate buffer (enzyme grade; Fisher), pH 4.5: prepared just before use
-
Streptozotocin (STZ; Sigma)
-
10% (w/v) sucrose (Sigma): prepared just before use
-
Test compound(s)
-
Rodent cages
-
Temperature-, humidity-, and light-controlled housing
-
1.5-ml microcentrifuge tubes
-
Aluminum foil
-
1-ml syringes
-
23-G needles
-
One Touch Basic blood glucose monitoring system (Lifescan)
-
Additional reagents and equipment for injection of rats (see Current Protocols article: Donovan & Brown, 2006a), blood collection from rats (see Current Protocols article: Donovan & Brown, 2006b), and anesthesia of rats (see Current Protocols article: Donovan & Brown, 1998)
Prepare animals
1.At least 5 days prior to the start of the experiment, house two to five male rats per cage at 24°C ± 1°C and 55% ± 5% humidity, with a 12-hr light-dark cycle (light on at 8:00 and light off at 20:00) Allow the rats free access to food and water.
2.Weigh all rats accurately to 1 g and randomly divide them into control and experimental groups.
3.On experimental day 1, fast all rats for 6 to 8 hr prior to STZ treatment. Provide water as normal.
Treat animals with STZ
4.Weigh 32.5 mg STZ into a 1.5-ml microcentrifuge tube and cover the tube with aluminum foil; use one tube for each rat. Prepare the citrate buffer.
5.Immediately prior to injection, dissolve STZ in 50 mM sodium citrate buffer (pH 4.5) to a final concentration of 32.5 mg/ml.
6.Using a 1-ml syringe and 23-G needle, inject the STZ solution i.p. (Donovan & Brown, 2006a) at 65 mg/kg (2.0 ml/kg) for the study group. Inject an equal volume of citrate buffer (pH 4.5) i.p. for the control group. Alternatively, using a 1-ml syringe and 25-G needle, inject the STZ solution i.v. at 65 mg/kg (2.0 ml/kg) for the study group. Inject an equal volume of citrate buffer (pH 4.5) i.v. for the control group.
7.Return the rats to their cages. Provide normal food and 10% sucrose water.
8.On experimental day 2, switch the 10% sucrose water to regular water.
9a. For studies involving early-stage T1DM : On experimental day 10, fast all rats for 6 to 8 hr (between 7 a.m. and 1 to 3 p.m.). Test the blood glucose concentration from a tail vein blood sample (see Current Protocols article: Donovan & Brown, 2006b) using a One Touch Basic blood glucose monitoring system to check hyperglycemia.
9b. For re-test of groups failing the first test for diabetes or for studies involving later-stage T1DM : On experimental day 21, fast all mice for 6 to 8 hr (e.g., from 7:00-13:00 or 15:00). Test the blood glucose concentrations from a tail vein blood sample (see Current Protocols article: Donovan & Brown, 2006b) using a One Touch Basic blood glucose monitoring system to confirm STZ injection-induced hyperglycemia.
10.If a test agent or compound is being assessed for its ability to correct hyperglycemia, extend the protocol longer, depending on the intent of the experiment. Treat groups of animals as described in steps 3 to 9 to establish a diabetic state, and then treat the animals with the potential restorative therapy. Include groups that receive appropriate vehicle injections as controls.
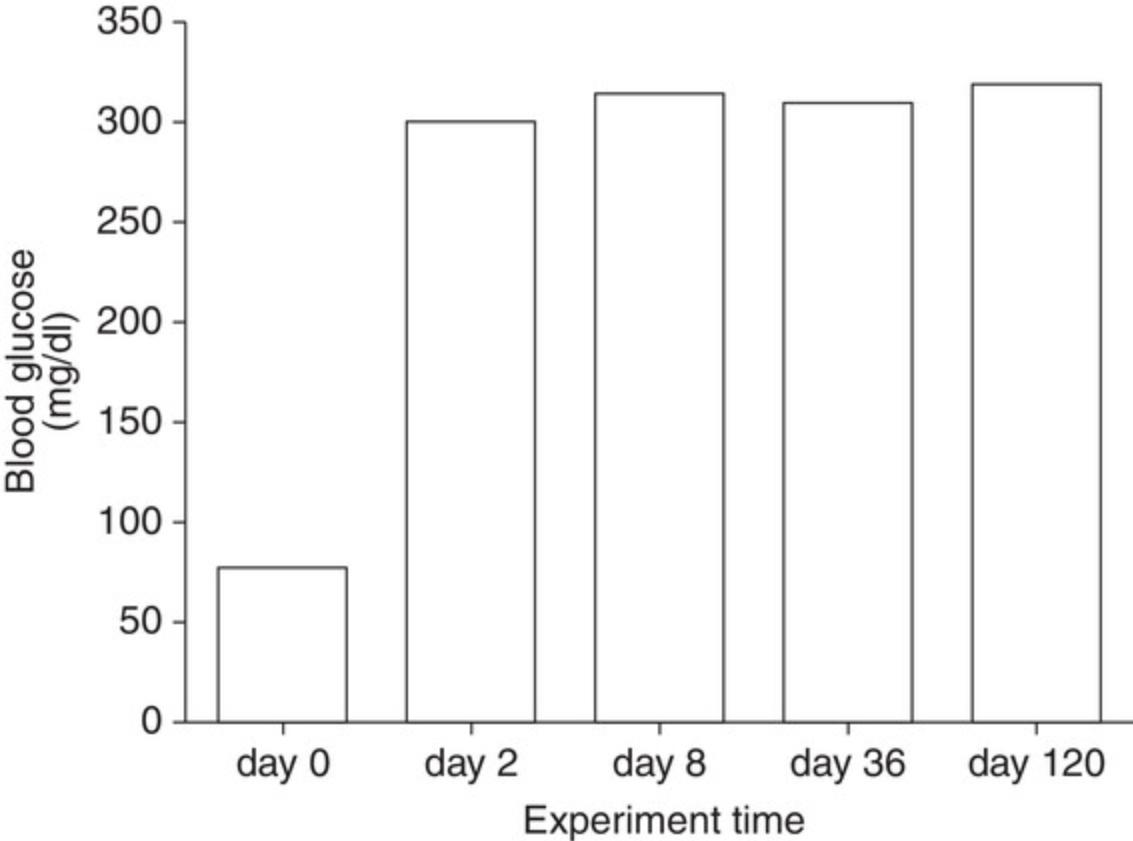
Basic Protocol 3: THE STREPTOZOTOCIN-NICOTINAMIDE RAT MODEL
This model uses concurrent administration of nicotinamide to afford partial protection of β-cells against STZ (Masiello et al., 1998). It is based on the work of Junod et al. (1969), who systematically investigated the early demonstration (Schein et al., 1967) that nicotinamide protected against the diabetogenic effect of STZ. This regimen produces a model of insulin-deficient, but not insulin-resistant, T2D, characterized by stable, moderate hyperglycemia, associated with 60% loss of β-cell function (Ghasemi et al., 2014; Masiello et al., 1998).
Materials
-
Sprague-Dawley or Wistar male rats: 150 to 200 g, 8 to 10 weeks old (Charles River Breeding Laboratories); 10 to 16 per treatment group, recommended
-
Standard rodent chow diet (Harlan)
-
Nicotinamide (Sigma)
-
0.9% (w/v) sodium chloride
-
50 mM sodium citrate buffer (enzyme-grade; Fisher), pH 4.5: prepared immediately before use
-
Streptozotocin (STZ; Sigma)
-
Test compound(s)
-
Rodent cages
-
Temperature-, humidity-, and light-controlled housing
-
1-ml syringes
-
23- and 25-G needles
-
One Touch Basic blood glucose monitoring system (Lifescan)
-
Additional reagents and equipment for injection of rats (Donovan & Brown, 2006a), blood collection from rats (Donovan & Brown, 2006b), and anesthesia of rats (Donovan & Brown, 1998)
Prepare animals
1.At least 5 days prior to the start of the experiment, house two to five male rats per cage at 24°C ± 1°C and 55% ± 5% humidity, with a 12-hr light-dark cycle (light on at 8:00 and off at 20:00). Allow the rats to have free access to food and water.
2.Weigh all rats accurately to 1 g, and randomly divide them into control and experimental groups.
3.On experimental day 1, fast all rats for 6 to 8 hr (from 7:00 to 13:00-15:00) prior to STZ treatment. Provide water as normal.
Induce diabetes with STZ and nicotinamide
4.Dissolve nicotinamide in 0.9% sodium chloride solution to a concentration of 230 mg/ml.
5.Weigh 32.5 mg STZ into a 1.5-ml microcentrifuge tube and cover the tube with aluminum foil; use one tube for each rat. Prepare the citrate buffer.
6.Using a 1-ml syringe and a 23-G needle, inject nicotinamide i.p. (see Current Protocols article: Donovan & Brown, 2006a) at a dose of 230 mg/kg (1.0 ml/kg).
7.Immediately prior to injection, dissolve STZ in 50 mM sodium citrate buffer, (pH 4.5 (see step 5), to a final concentration of 32.5 mg/ml.
8.Using a 1-ml syringe and 25-G needle, inject the STZ solution i.v. (Donovan & Brown, 2006a) at 65 mg/kg (2.0 ml/kg) for the experimental group. The control animals receive an i.v. injection of an equal volume of citrate buffer (pH 4.5) only.
9.Return the rats to their cages. Provide normal food and drinking water.
10.At around 8:00 a.m. on experimental day 10, test the blood glucose concentrations from a tail vein blood sample (Donovan & Brown, 2006b) using a One Touch Basic blood glucose monitoring system.
Treat animals with test agent(s)
11.If a test agent is being assessed for its ability to correct hyperglycemia, the diabetic animals can usually be maintained for several weeks. For testing of drug candidates, treat animals as described in steps 3 to 11 to establish the diabetic state and with the candidate drug, with vehicle injections in control subjects.
Basic Protocol 4: THE FAT-FED STREPTOZOTOCIN RAT MODEL
While the nicotinamide-STZ rat or mouse provides a model for insulin-deficient T2D, most patients with T2D display insulin resistance in addition to impaired insulin secretion. A model with these characteristics is created by administering moderate doses of STZ to animals rendered insulin resistant by prior consumption of a high-fat diet. This produces hyperglycemia, associated with hyperinsulinemia and insulin resistance (Reed et al., 2000).
Materials
-
Sprague-Dawley or Wistar male rats: 150 to 200 g, 8 to 10 weeks old (Charles River Breeding Laboratories); 10 to 16 per treatment group
-
A high-fat diet: 60% fat by caloric content (D12492 diet; Research Diets, http://www.researchdiets.com/opensource-diets/diet-induced-disease-models/obesity)
-
A low-fat diet from the same supplier for control animals (with the only difference between the diets being the % of the caloric intake provided by fat)
-
50 mM sodium citrate buffer (enzyme grade; Fisher), pH 4.5: prepared immediately before use
-
Streptozotocin (STZ; Sigma)
-
Test compound(s)
-
Rodent cages
-
Temperature-, humidity-, and light-controlled housing
-
1-ml syringes
-
23-G needles
-
One Touch Basic blood glucose monitoring system (Lifescan)
-
Additional reagents and equipment for injection of rats (see Current Protocols article: Donovan & Brown, 2006a) and blood collection from rats (see Current Protocols article: Donovan & Brown, 2006b)
Prepare animals
1.At least 5 days prior to initiating the experiment, house two to four male rats per cage at 24°C ± 1°C and 55% ± 5% humidity, with a 12-hr light-dark cycle (light on at 8:00 a.m. and off at 8:00 p.m.). Allow free access to food and water.
2.Weigh all rats accurately to 1 g and randomly divide them into control and experimental groups.
3.Place rats on the high-fat diet for 3 weeks.
4.On day 22, fast all rats for 6 to 8 hr (from 7:00 a.m. until 1:00-3:00 p.m.) prior to STZ treatment. Provide water as normal. Treat animals with STZ.
5.Weigh 40 mg STZ into a 1.5-ml microcentrifuge tube and cover the tube with aluminum foil. Prepare the citrate buffer.
6.Immediately prior to injection, dissolve STZ in the 50 mM sodium citrate buffer (pH 4.5) to a final concentration of 40 mg/ml.
7.Using a 1-ml syringe and 23-G needle, inject STZ i.p. (see Current Protocols article: Donovan & Brown, 2006a) into the experimental group at 40 mg/kg (1.0 ml/kg). Inject an equal volume of citrate buffer (pH 4.5) intraperitoneally into the control animals.
8.Return the rats to their cages. Provide the high-fat or control diet food as before, and normal drinking water.
9.At around 8:00 a.m., 10 days after STZ administration, measure the blood glucose concentrations in a tail-vein blood sample (Donovan & Brown, 2006b) using a One Touch Basic blood glucose monitoring system.
10.The animals can generally be maintained as hyperglycemic for several weeks for the testing of antihyperglycemic drug candidates. Treat groups of animals as described in steps 3 to 10 to establish the diabetic state, after which initiate the administration of test compounds to measure the hypoglycemic response in comparison to diabetic animals treated with vehicle only.
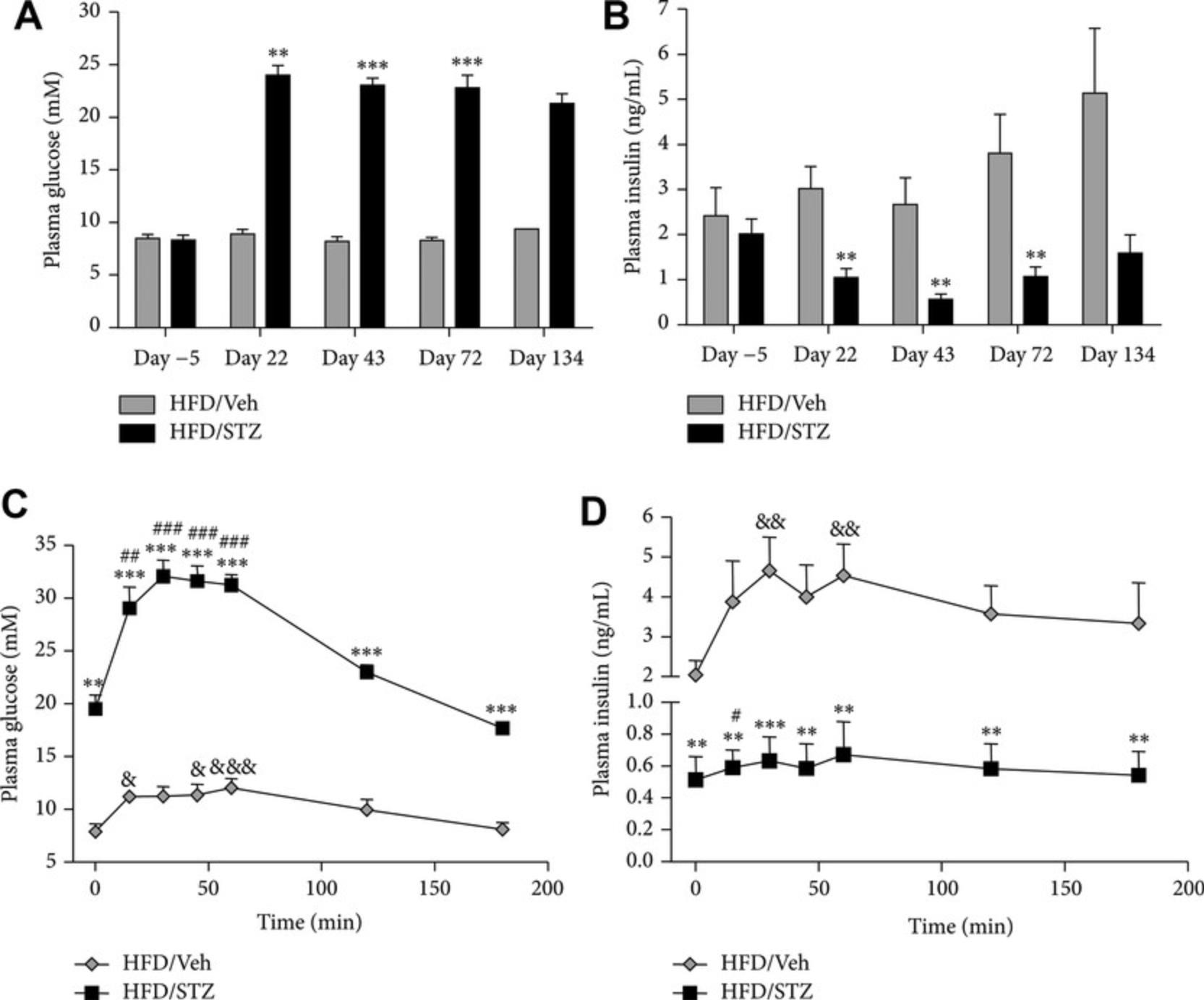
COMMENTARY
Background Information
Described in this article are methods for using STZ to selectively destroy pancreatic islet β-cells in mice and rats to generate animal models of T1DM and T2D. The T1DM animals can develop diabetic complications, e.g., diabetic neuropathy (Usuki et al., 2007), diabetic nephropathy (Breyer et al., 2005), and diabetic atherosclerosis (Wu & Huan, 2007). The models are used not only to study the pathological consequences of T1DM, but also to assess and evaluate experimental approaches for the treatment of this condition, in particular therapeutic approaches for reducing hyperglycemia. Rat and mouse models of diabetes have distinct advantages over other species, including the size of the animals, short induction period, ease of inducing the condition, and cost effectiveness (Wu & Huan, 2007).
Multiple, low-dose STZ-induced diabetic mouse models may more closely resemble human T1DM than models in which hyperglycemia is induced by a single large dose of the toxin, because of the association of hyperglycemia with lymphocytic infiltration of the pancreatic islets, marked β-cell apoptosis, insulitis, and insulin deficiency (Bonnevie-Nielsen, Steffes, & Lernmark, 1981; Kolb, 1987; Like & Rossini, 1976; Weide & Lacy, 1991). Moreover, as there is evidence for the contribution of autoimmunity in this model, it is much more suitable for studying the underlying pathogenesis of T1D than the high-dose STZ models where direct, toxin-induced necrosis of the β-cell is the predominant mode of cell death (Lin et al., 2010). However, because STZ may be toxic to organs and tissues other than the pancreatic islet β-cells, STZ models do not precisely mimic the human condition. For this reason, extrapolation of the findings directly to humans is not always possible. This is particularly true when using a single, high dose of STZ, which directly destroys β-cells rapidly and completely, therefore, lacking some features of T1DM, such as pancreatic insulitis (Kolb, 1987).
While T2D is the predominant form of this condition in humans, the challenges in developing an animal model for it are greater than for T1DM. Genetic models, especially the Zucker diabetic fatty rat and the db/db mouse, perhaps come closest to resembling the human disease. Nonetheless, their use is limited because they display some important differences from the human condition (Wang, Chandrasekera, & Pippin, 2014), and they are very expensive. Detailed in this article are two T2D models that are used most often. The nicotinamide STZ rat, a model for non-insulin-dependent, insulin-deficient T2D, is limited in not being insulin-resistant, a major feature of most human cases. The use of high-fat feeding to induce insulin resistance, followed by low-to-moderate doses of STZ to produce mild to moderate insulin deficiency, may currently be the most useful of the T2D models. The high-fat model is generally considered the best for characterizing many of the complications associated with human diabetes.
As none of these models precisely mimic human T1D or T2D, the choice of a model depends on the aim of the study. The models described in this article are useful for evaluating potential anti-diabetic agents, as well as for studying diabetes-induced long-term complications. Because of their limited construct validity (Furman, Candasamy, Bhattamisra, & Veettil, 2020), these models are of less use as tools for defining the etiology of the condition, although, in the context of T1D, the multiple low-dose STZ mouse model may be of some value in this regard.
Critical Parameters and Troubleshooting
General comments
While Basic Protocols 1 (along with its Alternate Protocol) and 2 are generally accepted as established procedures for studying T1DM, a consensus has yet to form on the best protocols for the STZ-nicotinamide and the STZ combined with a high-fat diet models for T2D. For the nicotinamide-STZ model, the main variables are the doses of nicotinamide (60 to 290 mg/kg, i.p.) and streptozotocin (45 to 65 mg/kg, i.p. or i.v.) employed, although there is general agreement on the time interval (15 min) between injection of the two agents (Ghasemi et al., 2014). In the case of the STZ/high-fat model, there is variability in the % fat in the diet (40% to 60%), the duration of the high-fat diet before STZ injection (2 to 12 weeks), the inclusion (or not) of sucrose, and the dose and route of administration of STZ (15, 35, or 50 mg/kg i.v., or 25 to 50 mg/kg, i.p.; Skovso, 2014). Indeed, in some cases, STZ was administered to neonates followed by the high-fat diet (e.g., Mancini, Ortiz, Croxatto, & Gallo, 2013). Yorek (2016) recommended 30 mg/kg STZ i.p. as the optimal dose in 12-week old Sprague-Dawley rats fed a high-fat diet for 8 weeks. A useful evaluation of the STZ combined with a high-fat diet model has been presented by Gheibi et al. (2017).
STZ stability
Streptozotocin should be stored at −20°C to avoid degradation. After weighing, the microcentrifuge tube containing the sample of STZ must be covered with aluminum foil to protect it from light. As STZ is unstable in solution, even at an acidic pH, it must not be mixed into citrate buffer until immediately prior to injection. The STZ solution should be prepared fresh and injected within 5 min of being dissolved because it decomposes in citrate buffer within 15 to 20 min. Although Ghasemi et al. (2010) suggest that STZ solutions may not be as unstable as previously believed, to reduce variability it is best to administer it within 5 min of its preparation.
Animal gender sensitivity to STZ
There is a strong influence of gender on the development of diabetes in laboratory animals. While females are resistant to the effects of low-dose STZ, this can be overcome by increasing the dose (Kolb, 1987). Because pancreatic islet β-cells of males are more prone than those of females to STZ-induced cytotoxicity, male animals are more popular for study (Kolb, 1987). The greater sensitivity of male mice to STZ across several strains was confirmed by Gurley et al. (2006). Although the precise reason for this gender difference remains undefined, estrogens are known to reduce the sensitivity of male rats to STZ-induced diabetes (Paik, Michelis, Kim, & Shin, 1982). Notably, however, the diabetes produced by a high dose of STZ (95 mg/kg) in rats protected by nicotinamide is more severe in female than in male rats (Vital, Larrieta, & Hiriart, 2006).
Animal strain sensitivity to STZ
Different strains of animals display different sensitivities to STZ. For mice, CD-1 and C57BL/6 are reliably sensitive to this toxin (Like & Rossini, 1976; Rossini, Appel, Williams, & Like, 1977), as are Sprague-Dawley and Wistar rats. DBA/2 is the most sensitive mouse strain, followed by C57BL6, according to Gurley et al. (2006). In contrast, Balb/cJ mice are resistant to the diabetes-inducing effects of multiple low doses of STZ (Zunino et al., 1994), as well as to single doses (Gurley et al., 2006). If blood glucose concentrations fail to rise above 150 mg/dl by weeks 3 or 4 after terminating STZ administration, it is possible that the animal strain being employed is insensitive to this toxin. Overall, it is recommended to use male mice of the most sensitive strain, rather than to increase the dose of STZ, which would carry the risk of non-specific toxicity (Gurley et al., 2006).
Fatal hypoglycemia after STZ injection
Some animals die quickly after STZ treatment due to massive islet β-cell necrosis and a sudden release of insulin that results in fatal hypoglycemia, usually within 48 hr of STZ injection. To prevent this, it is best to routinely provide animals with 10% sucrose water after STZ treatment (see http://www.AMDCC.org). If the number of animal deaths is >20% when using the single, high-dose STZ diabetic mouse protocol, treat the animals with 1 ml of 5% glucose solution i.p. 6 hr after STZ injection instead of providing 10% sucrose water (Huang & Wu, 2005). To avoid fatal hypoglycemia in the multiple, low-dose STZ-treated diabetic mice, provide 10% sucrose water for 6 days, beginning on experimental day 1. If mortality is high (>20%) in the single-dose, STZ-treated diabetic rats, provide 10% sucrose water for 2 days after the STZ injection. Generally, severe hypoglycemia is more likely to occur after high, single doses of STZ.
Fasting and nonfasting blood glucose concentrations
Because mice and rats are nocturnal feeders, an overnight fast before measuring blood glucose concentrations usually translates into a fast of ∼24 hr. This 24-hr fast can activate several physiologic responses that obscure the reliability of glucose readings. Because of this, fasting should be initiated on the morning of blood sampling. The National Institutes of Health (NIH) and the Animal Models of Diabetic Complications Consortium (AMDCC) have established a protocol of fasting mice from 7 a.m. to 1 p.m., with blood drawn at 1 p.m. (Breyer et al., 2005; http://www.AMDCC.org). Similarly, the accepted rat fasting time is 6 to 8 hr, between 7 a.m. and 1 to 3 p.m., after which the blood sample is taken for glucose analysis.
Blood glucose concentrations between fasting and nonfasting animals are quite different. The absolute concentrations of blood glucose in a fasting state are lower and less variable than in a nonfasting state. There is no standardized hyperglycemia concentration for mice or rats because different institutions and investigators use different, nonstandardized, fasting and nonfasting methods. However, there are three key points of general agreement: (1) use the same approach to test blood glucose concentrations for both control and STZ-treatment groups in the same experiment, e.g., either a non-fasting state or a fasting state for both control and STZ-treatment groups; (2) never use fasting and non-fasting states in the same experiment; and (3), hyperglycemia is defined as a blood glucose concentration in the STZ-treatment groups that is significantly higher than in the control groups.
Generally speaking, the blood glucose concentration in the STZ-treatment groups for non-fasting hyperglycemia should be >200 mg/dl (11.1 mmol/L), whereas for fasting diabetic animals the blood glucose should be >150 mg/dl (glucose of 18 mg/dl = 1 mM). The most important point is that there should be a statistically significant difference between the STZ-treatment and control groups. Usually, by 3 weeks after STZ injection, more than 50% of the animals develop severe hyperglycemia, with blood glucose concentrations attaining concentrations of 300 to 600 mg/dl (Deeds et al., 2011). If the study involves a chronic condition or diabetic complications (e.g., STZ-induced diabetic atherosclerosis) using multiple, low-dose STZ-induced diabetic mice, a second round of STZ injections is needed at week 7 to ensure maintenance of the diabetic state.
Understanding Results
For diabetic mice, typical time-dependent daily changes of glucose and insulin secretion are summarized in Figure 1. This figure illustrates glucose and insulin secretory responses to multiple, low doses of STZ in treated mice. Usually, the blood glucose concentrations in the STZ-injection groups are significantly higher than in the control groups on experimental day 10. By experimental weeks 3 to 4, blood glucose concentrations indicate severe hyperglycemia (300 to 600 mg/dl; 16.7 to 33.3 mmol/L) in ∼50% of STZ-treated animals. Insulin concentrations are low, although this parameter is not normally measured. Besides glucose and insulin concentration changes, mice also display, within 3 weeks of STZ treatment, typical T1DM features such as a loss of body weight and polydipsia (Flood, Mooradian, & Morley, 1990). Illustrated in Figure 2 are the typical features of mouse diabetes. In diabetic rats, hyperglycemia lasts for months (Arison et al., 1967). Shown in Figure 3 are the typical features of diabetes in rats.
The diabetes models described in this article can be used to assess diabetic mechanisms, screen compounds, or evaluate therapeutic options. For example, inosine, an immunomodulator/anti-inflammatory agent, significantly reduces blood glucose in the multiple low-dose STZ-induced diabetic mouse model (Fig 5; Mabley et al., 2003). In the single high-dose STZ-induced mouse model, syngeneic islet transplantation partially restores β-cell function and corrects hyperglycemia (Fig. 6; Yin et al., 2006). The STZ-induced diabetic rat model has also been used to evaluate antidiabetic drugs with, for example, valsartan (an angiotensin II receptor antagonist), displaying a dose-dependent anti-diabetic effect (Fig. 7; Chan et al., 2003). The high face validity of these models (Furman et al., 2020) accounts for their ability to predict effects of drug candidates working through well-known mechanisms; indeed, all known antidiabetic drugs are effective in these animal models. Their limited construct validity may, however, result in apparent preclinical effectiveness of agents that do not show clinical efficacy (Furman et al., 2020).
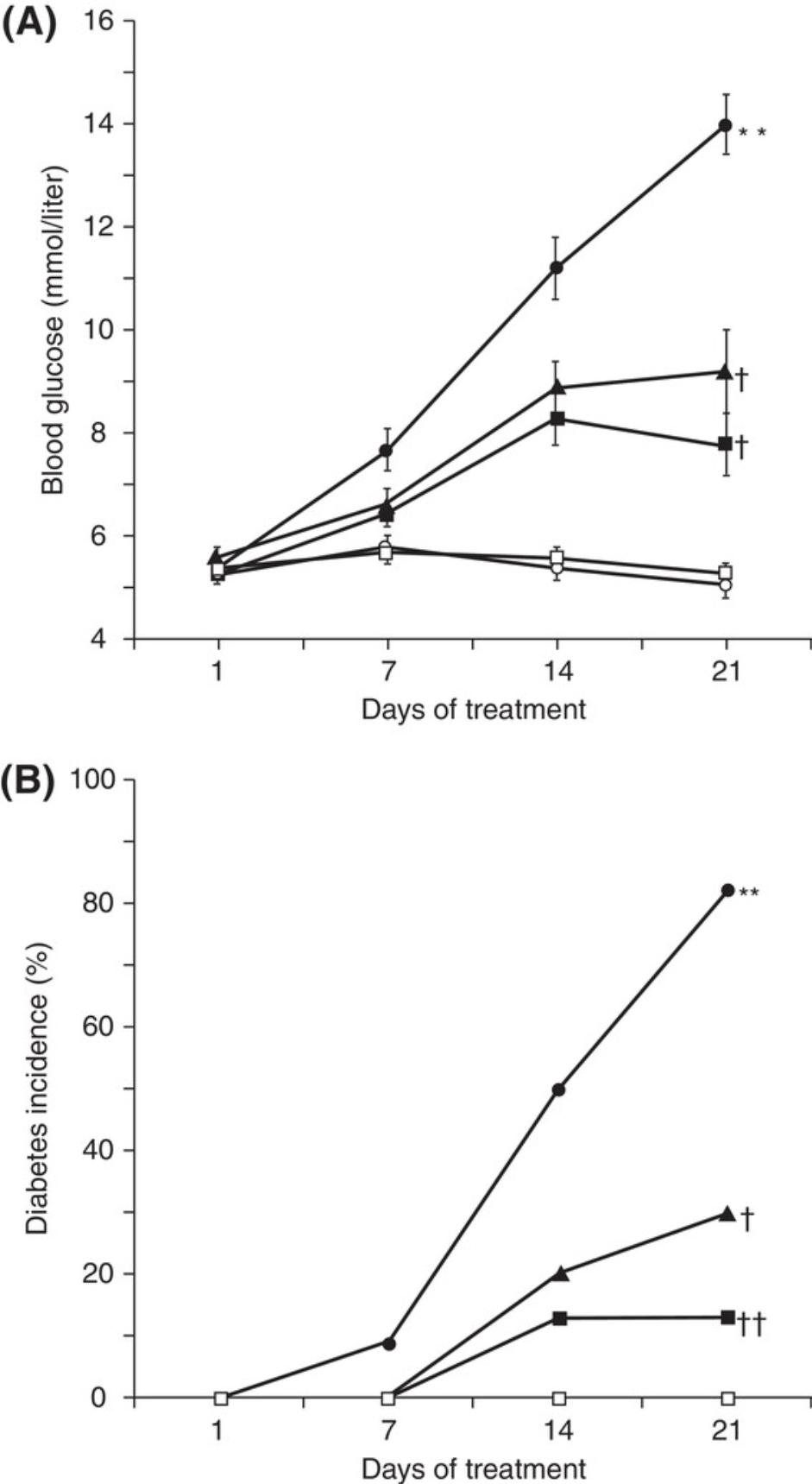
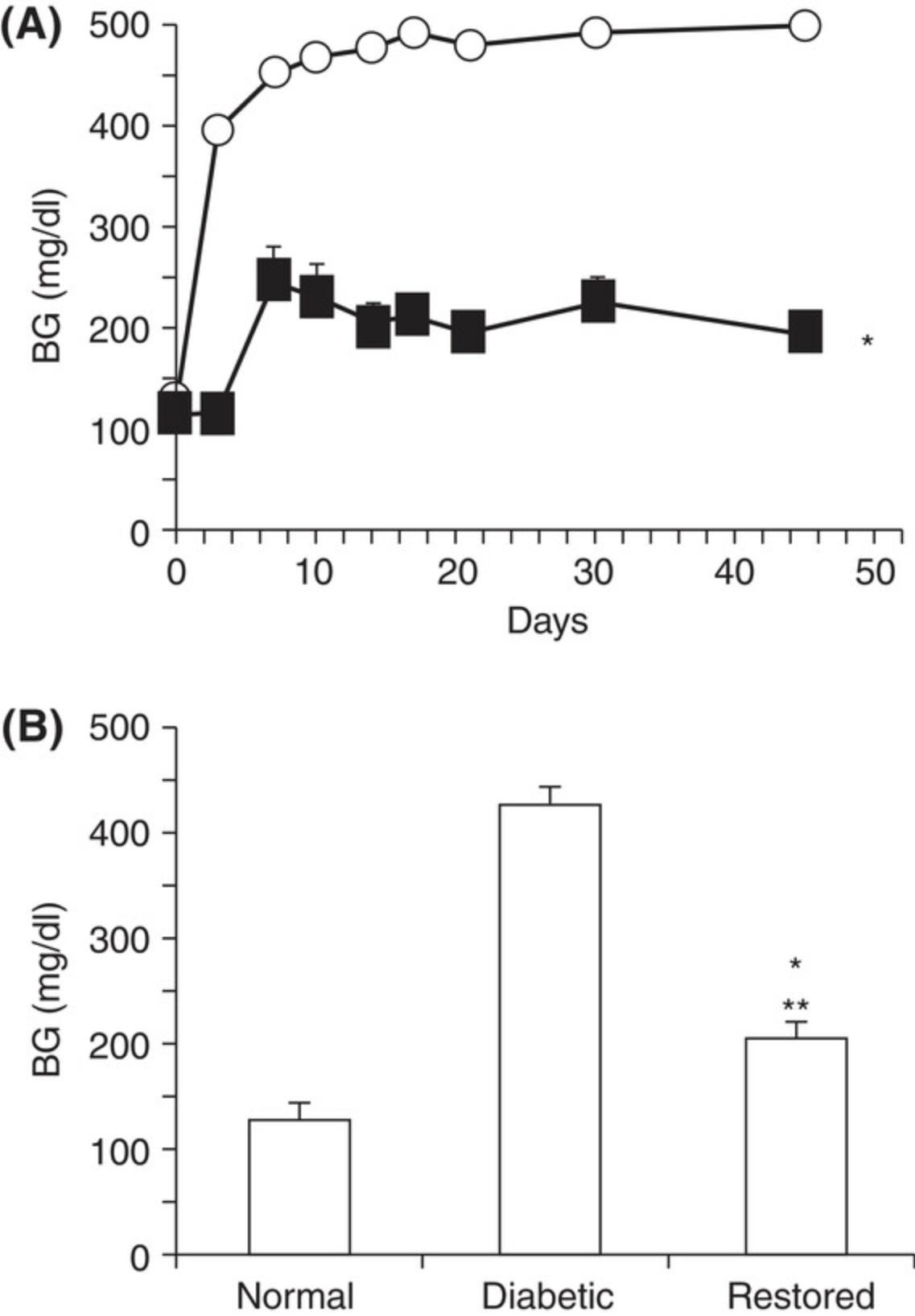
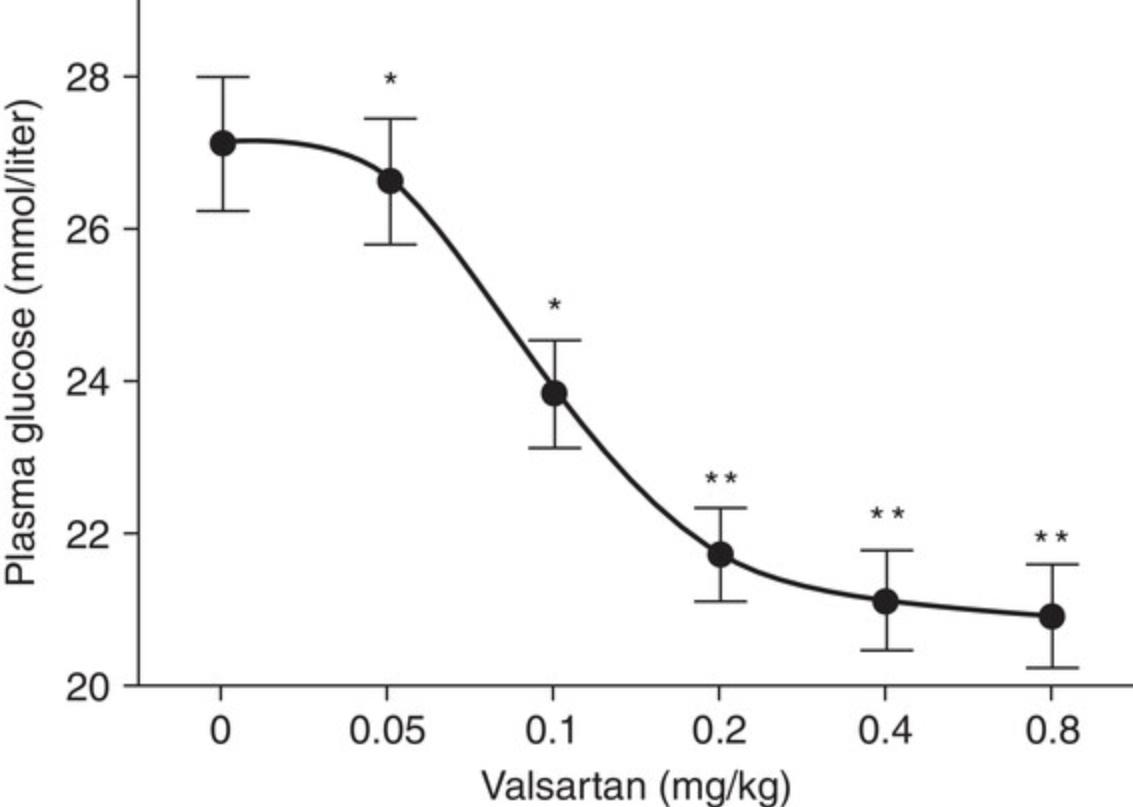
In studies on the high-fat STZ model (Fig. 4), it has been shown that both linagliptin (3 mg/kg daily) and metformin (200 mg/kg daily) reduce diabetes-induced changes in mechanical pain threshold (Byrne, Cheetham, Vickers, & Chapman, 2015). Rats rendered diabetic using the STZ protocol also develop nephropathy, making this a useful model for studying potential treatments for this condition (e.g., Luo et al., 2009). However, caution is required in applying these models of diabetic nephropathy because of the complication of STZ-induced acute tubular necrosis (Tay et al., 2005).
Time Considerations
Approximately 1 working day is needed to perform the STZ injections. On the first day of the experiment, mice must fast for 4 hr and rats for 6 to 8 hr before the STZ injection. The citrate buffer can be prepared during the fasting period. The STZ must be prepared immediately before injection to avoid decomposition. To prevent fatal hypoglycemia in single high-dose STZ-induced diabetic mice, treat the animals with 1 ml of 5% glucose i.p. at 6 hr after the STZ injection.
It takes several hours to test the blood glucose concentrations on the different post-STZ-injection days, depending on the protocol. The amount of time required over subsequent days or weeks depends on the aim of the study.
If the animals are to be used to study diabetic complications (e.g., STZ-induced diabetic atherosclerosis), the STZ treatment must be repeated at experimental week 7. This requires ∼1 working day. The animals can then be used for several more weeks or month, depending on the study design.
Acknowledgments
The author acknowledges the contributions of Kenneth K. Wu (Merck Research Laboratories) and Youming Huan (Mount Sinai School of Medicine) to a previous version of this article.
Conflict of Interest
The authors declare no conflict of interest.
Open Research
Data Availability Statement
Data sharing not applicable – no new data generated.
Literature Cited
- Arison, R. N., Ciaccio, E. I., Glitzer, M. S., Cassaro, J. A., & Pruss, M. P. (1967). Light and electron microscopy of lesions in rats rendered diabetic with streptozotocin. Diabetes , 16, 51–56. doi: 10.2337/diab.16.1.51.
- Bond, J. S., Failla, M. L., & Unger, D. F. (1983). Elevated manganese concentration and arginase activity in livers of streptozotocin-induced diabetic rats. Journal of Biological Chemistry , 258, 8004–8009. doi: 10.1016/S0021-9258(20)82019-5.
- Bonnevie-Nielsen, V., Steffes, M. W., & Lernmark, A. (1981). A major loss in islet mass and B-cell function precedes hyperglycemia in mice given multiple low doses of streptozotocin. Diabetes , 30, 424–429. doi: 10.2337/diab.30.5.424.
- Breyer, M. D., Bottinger, E., Brosius, F. C. 3rd, Coffman, T. M., Harris, R. C., Heilig, C. W., … AMDCC (2005). Mouse models of diabetic nephropathy. Journal of the American Society of Nephrology , 16, 27–45. doi: 10.1681/ASN.2004080648.
- Brondum, E., Nilsson, H., & Aalkjaer, C. (2005). Functional abnormalities in isolated arteries from Goto-Kakizaki and streptozotocin-treated diabetic rat models. Hormone and Metabolic Research , 37, 56–60. doi: 10.1055/s-2005-861370.
- Byrne, F. M., Cheetham, S., Vickers, S., & Chapman, V. (2015). Characterisation of pain responses in the high fat diet/streptozotocin model of diabetes and the analgesic effects of antidiabetic treatments. Journal of Diabetes Research , 2015, 752481. doi: 10.1155/2015/752481.
- Chan, P., Wong, K. L., Liu, I. M., Tzeng, T. F., Yang, T. L., & Cheng, J. T. (2003). Antihyperglycemic action of angiotensin II receptor antagonist, valsartan, in streptozotocin-induced diabetic rats. Journal of Hypertension , 21, 761–769. doi: 10.1097/00004872-200304000-00020.
- Chao, P. C., Li, Y., Chang, C. H., Shieh, J. P., Cheng, J. T., & Cheng, K. C. (2018). Investigation of insulin resistance in the popularly used four rat models of type-2 diabetes. Biomedicine & Pharmacotherapy, 101, 155–161
- Deeds, M. C., Anderson, J. M., Armstrong, A. S., Gastineau, D. A., Hiddinga, H. J., Jahangir, A., … Kudva, Y. C. (2011). Single dose streptozotocin induced diabetes: Considerations for study design in islet transplantation models. Laboratory Animals , 45, 131–140. doi: 10.1258/la.2010.010090.
- de Souza, R. J., Mente, A., Maroleanu, A., Cozma, A. I., Ha, V., Kishibe, T., … Anand, S. S. (2015). Intake of saturated and trans unsaturated fatty acids and risk of all cause mortality, cardiovascular disease, and type 2 diabetes: Systematic review and meta-analysis of observational studies. BMJ , 351, h3978.
- Donovan, J., & Brown, P. (1998). Anesthesia. Current Protocols in Immunology , 27, 1.4.1–1.4.5. doi: 10.1002/0471142735.im0104s27.
- Donovan, J., & Brown, P. (2006a). Parenteral injections. Current Protocols in Immunology , 73, 1.6.1–1.6.10. doi: 10.1002/0471142735.im0106s73.
- Donovan, J., & Brown, P. (2006b). Blood collection. Current Protocols in Immunology , 73, 1.7.1–1.7.9. doi: 10.1002/0471142735.im0107s73.
- Flood, J. F., Mooradian, A. D., & Morley, J. E. (1990). Characteristics of learning and memory in streptozotocin-induced diabetic mice. Diabetes , 39, 1391–1398. doi: 10.2337/diab.39.11.1391.
- Furman, B. L., Candasamy, M., Bhattamisra, S. K., & Veettil, S. K. (2020). Reduction of blood glucose by plant extracts and their use in the treatment of diabetes mellitus; discrepancies in effectiveness between animal and human studies. Journal of Ethnopharmacology , 247, 112264. doi: 10.1016/j.jep.2019.112264.
- Ganda, O. P., Rossini, A. A., & Like, A. A. (1976). Studies on streptozotocin diabetes. Diabetes , 25, 595–603. doi: 10.2337/diab.25.7.595.
- Ghasemi, M., Shafroodi, H., Gholipour, T., Nezami, B. G., Ebrahimi, F., & Dehpour, A. R. (2010). ATP-sensitive potassium channels contribute to the time-dependent alteration in pentylenetetrazole-induced seizure threshold in diabetic mice. Seizure: The Journal of the British Epilepsy Association , 19, 53–58. doi: 10.1016/j.seizure.2009.11.003.
- Ghasemi, A., Khalifi, S., & Jedi, S. S. (2014). Streptozotocin-nicotinamide-induced rat model of type 2 diabetes. Acta Physiologica Hungarica , 101, 408–420. doi: 10.1556/APhysiol.101.2014.4.2.
- Gheibi, S., Kashfi, K., & Ghasemi, A. (2017). A practical guide for induction of type-2 diabetes in rat: Incorporating a high-fat diet and streptozotocin. Biomedicine & Pharmacotherapy, 95, 605–613
- Gurley, S. B., Clare, S. E., Snow, K. P., Hu, A., Meyer, T. W., & Coffman, T. M. (2006). Impact of genetic background on nephropathy in diabetic mice. American Journal of Physiology. Renal Physiology , 290, F214–22 doi: 10.1152/ajprenal.00204.2005.
- Hartnell, J. M., Storrie, M. C., & Mooradian, A. D. (1990). Diabetes-related changes in chromatin structure of brain, liver, and intestinal epithelium. Diabetes , 39, 348–353. doi: 10.2337/diab.39.3.348.
- Huang, F., & Wu, W. (2005). Antidiabetic effect of a new peptide from Squalus mitsukurii liver (S-8300) in streptozocin-induced diabetic mice. Journal of Pharmacy and Pharmacology , 57, 1575–1580. doi: 10.1211/jpp.57.12.0007.
- Junod, A., Lambert, A. E., Stauffacher, W., & Renold, A. E. (1969). Diabetogenic action of streptozotocin: Relationship of dose to metabolic response. Journal of Clinical Investigation , 48, 2129–2139. doi: 10.1172/JCI106180.
- Junod, A., Lambert, A. E., Orci, L., Pictet, R., Gonet, A. E., & Renold, A. E. (1967). Studies of the diabetogenic action of streptozotocin. Proceedings of the Society for Experimental Biology and Medicine , 126, 201–205. doi: 10.3181/00379727-126-32401.
- Kolb, H. (1987). Mouse models of insulin dependent diabetes: Low-dose streptozocin-induced diabetes and nonobese diabetic (NOD) mice. Diabetes Metabolism Reviews , 3, 751–778. doi: 10.1002/dmr.5610030308.
- Kolb-Bachofen, V., Epstein, S., Kiesel, U., & Kolb, H. (1988). Low-dose streptozocin-induced diabetes in mice. Electron microscopy reveals single-cell insulitis before diabetes onset. Diabetes , 37, 21–27. doi: 10.2337/diab.37.1.21.
- Kunjathoor, V. V., Wilson, D. L., & LeBoeuf, R. C. (1996). Increased atherosclerosis in streptozotocin-induced diabetic mice. Journal of Clinical Investigation , 97, 1767–1773. doi: 10.1172/JCI118604.
- Lazar, M., Golden, P., Furman, M., & Lieberman, T. W. (1968). Resistance of the rabbit to streptozocin. Lancet , 26, 919. doi: 10.1016/S0140-6736(68)91094-5.
- Lenzen, S. (2017). Animal models of human type 1 diabetes for evaluating combination therapies and successful translation to the patient with type 1 diabetes. Diabetes Metabolism Research and Reviews , 33(7). doi: 10.1002/dmrr.2915.
- Like, A. A., & Rossini, A. A. (1976). Streptozotocin-induced pancreatic insulitis: New model of diabetes mellitus. Science , 193, 415–417. doi: 10.1126/science.180605.
- Lin, M., Yin, N., Murphy, B., Medof, M. E., Segerer, S., Heeger, P. S., & Schrüppel, B. (2010). Immune cell-derived c3 is required for autoimmune diabetes induced by multiple low doses of streptozotocin. Diabetes , 59, 2247–2252. doi: 10.2337/db10-0044.
- Luo, P., Zhou, Y., Chang, H. H., Zhang, J., Seki, T., Wang, C. Y., … Wang, M. H. (2009). Glomerular 20-HETE, EETs, and TGF-beta1 in diabetic nephropathy. American Journal of Physiology: Renal Physiology , 96, F556–F563. doi: 10.1152/ajprenal.90613.2008.
- Mabley, J. G., Rabinovitch, A., Suarez-Pinzon, W., Haskó, G., Pacher, P., Power, R., … Szabó, C. (2003). Inosine protects against the development of diabetes in multiple-low-dose streptozotocin and nonobese diabetic mouse models of type 1 diabetes. Molecular Medicine , 9, 96–104. doi: 10.2119/2003-00016.Mabley.
- Mancini, J. E., Ortiz, G., Croxatto, J. O., & Gallo, J. E. (2013). Retinal upregulation of inflammatory and proangiogenic markers in a model of neonatal diabetic rats fed on a high-fat-diet. BMC Ophthalmology , 13, 14. doi: 10.1186/1471-2415-13-14.
- Masiello, P. (2006). Animal models of type 2 diabetes with reduced pancreatic beta-cell mass. International Journal of Biochemistry & Cell Biology, 38, 873–893.
- Masiello, P., Broca, C., Gross, R., Roye, M., Manteghetti, M., Hillaire-Buys, D., … Ribes, G. (1998). Experimental NIDDM: Development of a new model in adult rats administered streptozotocin and nicotinamide. Diabetes , 47, 224–229. doi: 10.2337/diab.47.2.224.
- Paik, S. G., Michelis, M. A., Kim, Y. T., & Shin, S. (1982). Induction of insulin-dependent diabetes by streptozotocin. Inhibition by estrogens and potentiation by androgens. Diabetes , 31, 724–729. doi: 10.2337/diab.31.8.724.
- Pearson, J. A., Wong, F. S., & Wen, L. (2016). The importance of the Non Obese Diabetic (NOD) mouse model in autoimmune diabetes. Journal of Autoimmunity , 66, 76–88. doi: 10.1016/j.jaut.2015.08.019.
- Rakieten, N., Rakieten, M. L., & Nadkarni, M. V. (1963). Studies on the diabetogenic action of streptozotocin. Cancer Chemotherapy Reports Part 1 , 29, 91.
- Reed, M. J., Meszaros, K., Entes, L. J., Claypool, M. D., Pinkett, J. G., Gadbois, T. M., & Reaven, G. M. (2000). A new rat model of type 2 diabetes: The fat-fed, streptozotocin-treated rat. Metabolism , 49, 1390–1394. doi: 10.1053/meta.2000.17721.
- Rossini, A. A., Appel, M. C., Williams, R. M., & Like, A. A. (1977). Genetic influence of the streptozotocin-induced insulitis and hyperglycemia. Diabetes , 26, 916–920. doi: 10.2337/diab.26.10.916.
- Schein, P. S., Cooney, D. A., & Vernon, M. L. (1967). The use of nicotinamide to modify the toxicity of streptozotocin diabetes without loss of antitumor activity. Cancer Research , 27, 2324–2332.
- Skovso, S. (2014). Modeling type 2 diabetes in rats using high fat diet and streptozotocin. Journal of Diabetes Investigation , 5, 349–358. doi: 10.1111/jdi.12235.
- Tay, Y. C., Wang, Y., Kairaitis, L., Rangan, G. K., Zhang, C., & Harris, D. C. (2005). Can murine diabetic nephropathy be separated from superimposed acute renal failure? Kidney International , 68, 391–398. doi: 10.1111/j.1523-1755.2005.00405.x.
- Usuki, S., Ito, Y., Morikawa, K., Kise, M., Ariga, T., Rivner, M., & Yu, R. K. (2007). Effect of pregerminated brown rice intake on diabetic neuropathy in streptozotocin-induced diabetic rats. Nutrition and Metabolism , 4, 25. doi: 10.1186/1743-7075-4-25.
- Vital, P., Larrieta, E., & Hiriart, M. (2006). Sexual dimorphism in insulin sensitivity and susceptibility to develop diabetes in rats. Journal of Endocrinology , 190, 425–432. doi: 10.1677/joe.1.06596.
- Wang, B., Chandrasekera, P. C., & Pippin, J. J. (2014). Leptin- and leptin receptor-deficient rodent models: Relevance for human type 2 diabetes. Current Diabetes Reviews , 10, 131–145. doi: 10.2174/1573399810666140508121012.
- Weide, L. G., & Lacy, P. E. (1991). Low-dose streptozocin-induced autoimmune diabetes in islet transplantation model. Diabetes , 40, 1157–1162. doi: 10.2337/diab.40.9.1157.
- Wou, C., Unwin, N., Huang, Y., & Roglic, G. (2019). Implications of the growing burden of diabetes for premature cardiovascular disease mortality and the attainment of the Sustainable Development Goal target 3.4. Cardiovascular Diagnosis and Therapy , 9(2), 140–149 doi: 10.21037/cdt.2018.09.04.
- Wu, K. K., & Huan, Y. (2007). Diabetic atherosclerosis mouse models. Atherosclerosis , 191, 241–249. doi: 10.1016/j.atherosclerosis.2006.08.030.
- Yin, D., Tao, J., Lee, D. D., Shen, J., Hara, M., Lopez, J., … Chong, A. S. (2006). Recovery of islet beta-cell function in streptozotocin-induced diabetic mice: An indirect role for the spleen. Diabetes , 55, 3256–3263. doi: 10.2337/db05-1275.
- Yorek, M. A. (2016). Alternatives to the streptozotocin-diabetic rodent. International Review of Neurobiology , 127, 89–112 doi: 10.1016/bs.irn.2016.03.002.
- Zhang, M., Lv, X. Y., Li, J., Xu, Z.G., & Chen, L. (2008). The characterization of high-fat diet and multiple low-dose streptozotocin induced type 2 diabetes rat model. Experimental Diabetes Research , 2008, 704045. doi: 10.1155/2008/704045.
- Zunino, S. J., Simons, L. F., Sambrook, J. F., & Gething, M. J. (1994). Interleukin-1 promotes hyperglycemia and insulitis in mice normally resistant to streptozotocin-induced diabetes. American Journal of Pathology , 145, 661–670.
Internet Resources
Web site for Animal Models of Diabetic Complications Consortium (AMDCC), providing new animal models of diabetic complications, with the goal of identifying the most appropriate animal models to study the etiology, prevention, and treatment of diabetic complications.
Citing Literature
Number of times cited according to CrossRef: 129
- Naif AlSuhaymi, Therapeutic Effects of Nigella sativa Oil and Whole Seeds on STZ‐Induced Diabetic Rats: A Biochemical and Immunohistochemical Study, Oxidative Medicine and Cellular Longevity, 10.1155/2024/5594090, 2024 , 1, (2024).
- Kareem El‐Ghazawi, Ukpong B. Eyo, Shayn M. Peirce, Brain Microvascular Pericyte Pathology Linking Alzheimer's Disease to Diabetes, Microcirculation, 10.1111/micc.12877, 31 , 7, (2024).
- Yolander Valentine, Barbara S. Nikolajczyk, T cells in obesity‐associated inflammation: The devil is in the details, Immunological Reviews, 10.1111/imr.13354, 324 , 1, (25-41), (2024).
- Zijun Chen, Yuxi Wang, Guanhua Zhang, Jian Zheng, Lei Tian, Yingliang Song, Xiangdong Liu, Role of LRP5/6/GSK‐3β/β‐catenin in the differences in exenatide‐ and insulin‐promoted T2D osteogenesis and osteomodulation, British Journal of Pharmacology, 10.1111/bph.16421, 181 , 19, (3556-3575), (2024).
- Shaimaa M. Elnahas, Hend Abd El‐Halim Mansour, Mamdouh R. El‐Sawi, Amoura M. Abou‐El‐Naga, Therapeutic effect of Momordica charantia on cardiomyopathy in a diabetic maternal rat model, Journal of Experimental Zoology Part A: Ecological and Integrative Physiology, 10.1002/jez.2854, 341 , 9, (977-990), (2024).
- Zhanjuan Zhao, Jinwen Pang, Di Zhao, Ning Guo, Yiman Guo, Feiyan Kong, Huizhong Yang, Jianxi Zhao, Exploring the efficacy of photodynamic antimicrobial chemotherapy on diabetic foot ulcers in rats, Journal of Biophotonics, 10.1002/jbio.202300568, 17 , 7, (2024).
- Mohammad Yasin Zamanian, Hashem O. Alsaab, Maryam Golmohammadi, Alexey Yumashev, Abeer Mhussan Jabba, Mohammed Kadhem Abid, Abhishek Joshi, Ahmed Hussien Alawadi, Noor S. Jafer, Farzaneh Kianifar, Samuel Baker Obakiro, NF‐κB pathway as a molecular target for curcumin in diabetes mellitus treatment: Focusing on oxidative stress and inflammation, Cell Biochemistry and Function, 10.1002/cbf.4030, 42 , 4, (2024).
- Maha Abdelmonem, Shimaa O. Ali, Asmaa K. Al‐Mokaddem, Heba R. Ghaiad, Ameliorating diabetes‐induced testicular dysfunction by modulating PKC/Nrf2/Bcl‐2 signaling: Protective role of sulbutiamine, BioFactors, 10.1002/biof.2046, 50 , 4, (845-862), (2024).
- Wenyu Dai, Rui Shu, Fan Yang, Bin Li, Hannah M. Johnson, Sheng Yu, Hang Yang, Yau Kei Chan, Weizhong Yang, Ding Bai, Yi Deng, Engineered Bio‐Heterojunction Confers Extra‐ and Intracellular Bacterial Ferroptosis and Hunger‐Triggered Cell Protection for Diabetic Wound Repair, Advanced Materials, 10.1002/adma.202305277, 36 , 9, (2024).
- Li-Li Dai, Sung-Bo Cho, Hui-Fang Li, Li-Sha A, Xiao-Ping Ji, Sirigunqiqige Pan, Ming-Lan Bao, Laxinamujila Bai, Gen-Na Ba, Ming-Hai Fu, Lomatogonium rotatum extract alleviates diabetes mellitus induced by a high-fat, high-sugar diet and streptozotocin in rats , World Journal of Diabetes, 10.4239/wjd.v14.i6.846, 14 , 6, (846-861), (2023).
- Neng Fisheri Kurniati, Almira Fathadina, Combination of Empagliflozin and Liraglutide protects heart against isoproterenol-induced myocardial infarction in rats, Pharmacia, 10.3897/pharmacia.70.e96975, 70 , 1, (171-180), (2023).
- Abdollah Amini, Fahimeh Ghasemi Moravej, Atarodalsadat Mostafavinia, Hossein Ahmadi, Sufan Chien, Mohammad Bayat, Photobiomodulation Therapy Improves Inflammatory Responses by Modifying Stereological Parameters, microRNA-21 and FGF2 Expression, Journal of Lasers in Medical Sciences, 10.34172/jlms.2023.16, 14 , (e16), (2023).
- Maria Andonova, Petko Dzhelebov, Krastina Trifonova, Penka Yonkova, Nikola Kostadinov, Krasimira Nancheva, Veselin Ivanov, Krasimira Gospodinova, Nikola Nizamov, Ilia Tsachev, Chavdar Chernev, Metabolic Markers Associated with Progression of Type 2 Diabetes Induced by High-Fat Diet and Single Low Dose Streptozotocin in Rats, Veterinary Sciences, 10.3390/vetsci10070431, 10 , 7, (431), (2023).
- Najlaa Bassalat, Sleman Kadan, Sarit Melamed, Tamar Yaron, Zipora Tietel, Dina Karam, Asmaa Kmail, Mahmud Masalha, Hilal Zaid, In Vivo and In Vitro Antidiabetic Efficacy of Aqueous and Methanolic Extracts of Orthosiphon Stamineus Benth, Pharmaceutics, 10.3390/pharmaceutics15030945, 15 , 3, (945), (2023).
- Hala Zuhayri, Alice A. Samarinova, Alexey V. Borisov, David A. Lopez Guardado, Houssain Baalbaki, Natalya A. Krivova, Yury V. Kistenev, Quantitative Assessment of Low-Dose Photodynamic Therapy Effects on Diabetic Wound Healing Using Raman Spectroscopy, Pharmaceutics, 10.3390/pharmaceutics15020595, 15 , 2, (595), (2023).
- Anastasia Sklifasovskaya, Mikhail Blagonravov, Madina Azova, Vyacheslav Goryachev, Myocardial Glutathione Synthase and TRXIP Expression Are Significantly Elevated in Hypertension and Diabetes: Influence of Stress on Antioxidant Pathways, Pathophysiology, 10.3390/pathophysiology30020021, 30 , 2, (248-259), (2023).
- Dolores G. Aguila-Muñoz, Fabiola E. Jiménez-Montejo, Víctor E. López-López, Aarón Mendieta-Moctezuma, Jorge Rodríguez-Antolín, Jorge Cornejo-Garrido, María C. Cruz-López, Evaluation of α-Glucosidase Inhibition and Antihyperglycemic Activity of Extracts Obtained from Leaves and Flowers of Rumex crispus L., Molecules, 10.3390/molecules28155760, 28 , 15, (5760), (2023).
- Tri Widyawati, Rony Abdi Syahputra, Siti Syarifah, Imam Bagus Sumantri, Analysis of Antidiabetic Activity of Squalene via In Silico and In Vivo Assay, Molecules, 10.3390/molecules28093783, 28 , 9, (3783), (2023).
- I Made Wisnu Adhi Putra, Nanang Fakhrudin, Arief Nurrochmad, Subagus Wahyuono, A Review of Medicinal Plants with Renoprotective Activity in Diabetic Nephropathy Animal Models, Life, 10.3390/life13020560, 13 , 2, (560), (2023).
- Valery Golderman, Zehavit Goldberg, Shany Guly Gofrit, Amir Dori, Nicola Maggio, Joab Chapman, Ifat Sher, Ygal Rotenstreich, Efrat Shavit-Stein, PARIN5, a Novel Thrombin Receptor Antagonist Modulates a Streptozotocin Mice Model for Diabetic Encephalopathy, International Journal of Molecular Sciences, 10.3390/ijms24032021, 24 , 3, (2021), (2023).
- So-Young Chang, Jun Hee Lee, Se Cheol Oh, Min Young Lee, Nam Kyu Lim, Human Fibroblast Growth Factor-Treated Adipose-Derived Stem Cells Facilitate Wound Healing and Revascularization in Rats with Streptozotocin-Induced Diabetes Mellitus, Cells, 10.3390/cells12081146, 12 , 8, (1146), (2023).
- Jeremy Sprouse, Chethan Sampath, Pandu Gangula, 17β-Estradiol Suppresses Gastric Inflammatory and Apoptotic Stress Responses and Restores nNOS-Mediated Gastric Emptying in Streptozotocin (STZ)-Induced Diabetic Female Mice, Antioxidants, 10.3390/antiox12030758, 12 , 3, (758), (2023).
- Khalaf F. Alsharif, Asmaa A. Hamad, Mohamed A. Alblihd, Fatma Abo Zakaib Ali, Sherine Ahmed Mohammed, Abdulrahman Theyab, Osama M. Al-Amer, Malik Saad Almuqati, Abdulraheem Ali Almalki, Alaa Jameel A. Albarakati, Khalid J. Alzahrani, Ashraf Albrakati, Mohammad Hamed Albarakati, Doaa Abass, Maha S. Lokman, Ehab Kotb Elmahallawy, Melatonin downregulates the increased hepatic alpha-fetoprotein expression and restores pancreatic beta cells in a streptozotocin-induced diabetic rat model: a clinical, biochemical, immunohistochemical, and descriptive histopathological study, Frontiers in Veterinary Science, 10.3389/fvets.2023.1214533, 10 , (2023).
- Namphung Suemanotham, Sataporn Phochantachinda, Duangthip Chatchaisak, Walasinee Sakcamduang, Anchana Chansawhang, Pornsiri Pitchakarn, Boonrat Chantong, Antidiabetic effects of Andrographis paniculata supplementation on biochemical parameters, inflammatory responses, and oxidative stress in canine diabetes, Frontiers in Pharmacology, 10.3389/fphar.2023.1077228, 14 , (2023).
- Manda Wang, Gangqiang Wang, Xiaoli Pang, Jiacheng Ma, Jinghan Yuan, Yanrong Pan, Yu Fu, Ismail Laher, Shunchang Li, MOTS-c repairs myocardial damage by inhibiting the CCN1/ERK1/2/EGR1 pathway in diabetic rats, Frontiers in Nutrition, 10.3389/fnut.2022.1060684, 9 , (2023).
- Sofía Bordet, Juan Pablo Luaces, Maria Ines Herrera, Liliana Mirta Gonzalez, Tamara Kobiec, Santiago Perez-Lloret, Matilde Otero-Losada, Francisco Capani, Neuroprotection from protein misfolding in cerebral hypoperfusion concurrent with metabolic syndrome. A translational perspective, Frontiers in Neuroscience, 10.3389/fnins.2023.1215041, 17 , (2023).
- Alberto Mesa-Lombardo, Nuria García-Magro, Angel Nuñez, Yasmina B. Martin, Locus coeruleus inhibition of vibrissal responses in the trigeminal subnucleus caudalis are reduced in a diabetic mouse model, Frontiers in Cellular Neuroscience, 10.3389/fncel.2023.1208121, 17 , (2023).
- Jay V. Patankar, Marvin Bubeck, Miguel Gonzalez Acera, Christoph Becker, Breaking bad: necroptosis in the pathogenesis of gastrointestinal diseases, Frontiers in Immunology, 10.3389/fimmu.2023.1203903, 14 , (2023).
- Yeshiwas Guadie Zeleke, Seyfe Asrade Atnafie, Tezera Jemere Aragaw, Anti-Diabetic Activities of Hydro-Methanolic Crude Extract and Solvent Fractions of Heteromorpha arborescens (Apiaceae) Leaves in Mice, Journal of Experimental Pharmacology, 10.2147/JEP.S392742, Volume 15 , (107-121), (2023).
- Carlos Poblete Jara, Guilherme Nogueira, Joseane Morari, Thaís Paulino do Prado, Renan de Medeiros Bezerra, Lício A. Velloso, William Velander, Eliana Pereira de Araújo, An older diabetes-induced mice model for studying skin wound healing, PLOS ONE, 10.1371/journal.pone.0281373, 18 , 2, (e0281373), (2023).
- Emuesiri Goodies Moke, Eric Kelly Inanemo Omogbai, SammyDavies Ehiosu Osagie-Eweka, Adaeze Phina Uchendu, Odion Martha Obayuwana, Elizabeth Okoro-Akpandu, Benneth Ben-Azu, Antihypertensive and antihyperglycemic effects of combinations of losartan with metformin and/or glibenclamide in desoxycorticosterone acetate and streptozotocin-induced hypertensive diabetic rats, Laboratory Animal Research, 10.1186/s42826-023-00159-2, 39 , 1, (2023).
- Wakuma Wakene Jifar, Ahmed S. BaHammam, Yadeta Babu Bayane, Biruk Tafese Moges, Teshome Bekana, In Vivo Hypolipidemic, Hypoglycemic, Antihyperglycemic, and In Vitro Antioxidant Effects of Podocarpus gracilis Leaf Extract and Fractions in Diabetic Mice, Evidence-Based Complementary and Alternative Medicine, 10.1155/2023/9187837, 2023 , 1, (2023).
- Xiaoqian Zhang, Jing Fang, Ruipeng Ge, Jing Wu, Mengjuan Ye, Xiao Cai, Guanghui Deng, Mengzhen Ma, Jiahui Lv, Nianjun Yu, Liang Yao, Daiyin Peng, Regulation of Glucolipid Metabolism in Mice Using Dendrobium huoshanense Extract and PI3k/Akt Signaling Pathway Activation, Journal of Food Biochemistry, 10.1155/2023/8878821, 2023 , 1, (2023).
- Brandon M. Bauer, Supriyo Bhattacharya, Elizabeth Bloom-Saldana, Jose M. Irimia-Dominguez, Patrick T. Fueger, Dose-dependent progression of multiple low-dose streptozotocin-induced diabetes in mice, Physiological Genomics, 10.1152/physiolgenomics.00032.2023, 55 , 9, (381-391), (2023).
- Sahar Oudeh, Abbas Javahery Vayghan, Mahmood Ahmadi‐hamedani, Duration of the diabetic state altered platelet indices from baseline values in a streptozotocin‐induced rat model for type 1 diabetes mellitus, Veterinary Clinical Pathology, 10.1111/vcp.13229, 52 , 2, (236-242), (2023).
- Belinda L. Sun, Xiaoguang Sun, Carrie L. Kempf, Jin H. Song, Nancy G. Casanova, Sara M. Camp, Vivian Reyes Hernon, Michael Fallon, Christian Bime, Diego R. Martin, Cristina Travelli, Donna D. Zhang, Joe G. N. Garcia, Involvement of eNAMPT/TLR4 inflammatory signaling in progression of non‐alcoholic fatty liver disease, steatohepatitis, and fibrosis, The FASEB Journal, 10.1096/fj.202201972RR, 37 , 3, (2023).
- Swee Ching Tan, Ramkumar Rajendran, Subrat Kumar Bhattamisra, Purushotham Krishnappa, Fabian Davamani, Ebenezer Chitra, Stephen Ambu, Brian Furman, Mayuren Candasamy, Effect of madecassoside in reducing oxidative stress and blood glucose in streptozotocin–nicotinamide-induced diabetes in rats, Journal of Pharmacy and Pharmacology, 10.1093/jpp/rgad063, 75 , 8, (1034-1045), (2023).
- Madhuri Basak, Kiran Das, Tarun Mahata, Abhishek Singh Sengar, Sumit Kumar Verma, Sayan Biswas, Kakali Bhadra, Adele Stewart, Biswanath Maity, RGS7-ATF3-Tip60 Complex Promotes Hepatic Steatosis and Fibrosis by Directly Inducing TNFα, Antioxidants & Redox Signaling, 10.1089/ars.2021.0174, 38 , 1-3, (137-159), (2023).
- Chang Woo Chae, Jee Hyeon Yoon, Jae Ryong Lim, Ji Yong Park, Ji Hyeon Cho, Young Hyun Jung, Gee Euhn Choi, Hyun Jik Lee, Ho Jae Han, TRIM16-mediated lysophagy suppresses high-glucose-accumulated neuronal Aβ, Autophagy, 10.1080/15548627.2023.2229659, 19 , 10, (2752-2768), (2023).
- Pengmin Ji, Qifeng Shi, Yan Liu, Min Han, Yong Su, Ran Sun, Huimin Zhou, Weizu Li, Weiping Li, Ginsenoside Rg1 treatment alleviates renal fibrosis by inhibiting the NOX4–MAPK pathway in T2DM mice, Renal Failure, 10.1080/0886022X.2023.2197075, 45 , 1, (2023).
- Eric Priyo Prasetyo, Galih Sampoerno, Devi Eka Juniarti, Febriastuti Cahyani, Widya Saraswati, Mefina Kuntjoro, Evelyn Tjendronegoro, Effect of Lipopolysaccharide-Induced Apical Periodontitis in Diabetes Mellitus Rats on Periapical Inflammation, European Journal of Dentistry, 10.1055/s-0042-1758790, (2023).
- Jialin Cui, Enze Shi, Yingjie Wang, Tiantian Liu, Network pharmacology study of the mechanism underlying the therapeutic effect of Zhujing pill and its main component oleanolic acid against diabetic retinopathy, Bioscience Reports, 10.1042/BSR20220893, 43 , 1, (2023).
- Preetam Guha Ray, Debasis Maity, Jinbo Huang, Henryk Zulewski, Martin Fussenegger, A versatile bioelectronic interface programmed for hormone sensing, Nature Communications, 10.1038/s41467-023-39015-1, 14 , 1, (2023).
- Yudian Zhang, Zihua Li, Xinyi Liu, Xinyu Chen, Shujie Zhang, Yuemeng Chen, Jiangnan Chen, Jin Chen, Fuqing Wu, Guo-Qiang Chen, 3-Hydroxybutyrate ameliorates insulin resistance by inhibiting PPARγ Ser273 phosphorylation in type 2 diabetic mice, Signal Transduction and Targeted Therapy, 10.1038/s41392-023-01415-6, 8 , 1, (2023).
- Akurange Sujeevi Dammadinna Wickramasinghe, Anoja Priyadarshani Attanayake, Pabasara Kalansuriya, Herbal Extracts Encapsulated Nanoliposomes as Potential Glucose-lowering Agents: An in Vitro and in Vivo Approach Using Three Herbal Extracts, Journal of Pharmaceutical Sciences, 10.1016/j.xphs.2023.06.017, 112 , 9, (2538-2551), (2023).
- Afife Busra Ugur Kaplan, Meltem Cetin, Cemil Bayram, Serkan Yildirim, Ali Taghizadehghalehjoughi, Ahmet Hacimuftuoglu, In Vivo Evaluation of Nanoemulsion Formulations for Metformin and Repaglinide Alone and Combination, Journal of Pharmaceutical Sciences, 10.1016/j.xphs.2023.01.008, 112 , 5, (1411-1426), (2023).
- Dario Gerace, Quan Zhou, Jennifer Hyoje-Ryu Kenty, Adrian Veres, Elad Sintov, Xi Wang, Kyle R. Boulanger, Hongfei Li, Douglas A. Melton, Engineering human stem cell-derived islets to evade immune rejection and promote localized immune tolerance, Cell Reports Medicine, 10.1016/j.xcrm.2022.100879, 4 , 1, (100879), (2023).
- Somaia A G Eltobshy, Refka Messiha, Emile Metias, Mohamed Sarhan, Randa El-Gamal, Ahmed El-Shaieb, Mohammad Ghalwash, Effect of SGLT2 Inhibitor on Cardiomyopathy in a Rat Model of T2DM: Possible involvement of Cardiac Aquaporins, Tissue and Cell, 10.1016/j.tice.2023.102200, (102200), (2023).
- Nursarah Syamimi Anuar, Syahirah Ain Shafie, Muhammad Aiman Faris Maznan, Noor Syaffinaz Noor Mohamad Zin, Nur Ain Sabrina Azmi, Rohaizad Abdul Raoof, Diyas Myrzakozha, Nurdiana Samsulrizal, Lauric acid improves hormonal profiles, antioxidant properties, sperm quality and histomorphometric changes in testis and epididymis of streptozotocin-induced diabetic infertility rats, Toxicology and Applied Pharmacology, 10.1016/j.taap.2023.116558, 470 , (116558), (2023).
- Jozaa Z. AlTamimi, Nora A. AlFaris, Ghedeir M. Alshammari, Reham I. Alagal, Dalal H. Aljabryn, Mohammed Abdo Yahya, Esculeoside A alleviates reproductive toxicity in streptozotocin-diabetic rats’ s model by activating Nrf2 signaling, Saudi Journal of Biological Sciences, 10.1016/j.sjbs.2023.103780, 30 , 9, (103780), (2023).
- Judith Gruber, Ruth Hanssen, Mishal Qubad, Aicha Bouzouina, Vivi Schack, Hannah Sochor, Carmen Schiweck, Mareike Aichholzer, Silke Matura, David A. Slattery, Yurdaguel Zopf, Stephanie L. Borgland, Andreas Reif, Sharmili Edwin Thanarajah, Impact of insulin and insulin resistance on brain dopamine signalling and reward processing – An underexplored mechanism in the pathophysiology of depression?, Neuroscience & Biobehavioral Reviews, 10.1016/j.neubiorev.2023.105179, 149 , (105179), (2023).
- Emuesiri Goodies Moke, Eric K.I. Omogbai, Sammy D.E. Osagie-Eweka, Adaeze P. Uchendu, Adrian I. Omogbiya, Benneth Ben-Azu, Anthony T. Eduviere, Kesiena E. Edje, Emuesiri K. Umukoro, Kenneth K. Anachuna, Jerome N. Asiwe, Ejiroghene Ahante, Ighohwo J. Oghoghovwe, Co-administration of metformin and/or glibenclamide with losartan reverse N-nitro-l-arginine-methyl ester-streptozotocin-induced hypertensive diabetes and haemodynamic sequelae in rats, Microvascular Research, 10.1016/j.mvr.2023.104497, 147 , (104497), (2023).
- Tet Soon Wong, Fatahiya Mohamed Tap, Zanariah Hashim, Fadzilah Adibah Abdul Majid, Nor Hafizah Zakaria, Parsaoran Siahaan, Abeer Mogadem, Dual actions of gallic acid and andrographolide trigger AdipoR1 to stimulate insulin secretion in a streptozotocin-induced diabetes rat model, Journal of Traditional and Complementary Medicine, 10.1016/j.jtcme.2022.09.002, 13 , 1, (11-19), (2023).
- İstemihan Çelikbaş, Esra Mavi, Ceylan Hepokur, The evaluation of the effects of natural zeolite (Clinoptilolite) in diabetic rats on bone healing in dental extracting socket, Journal of Oral Biology and Craniofacial Research, 10.1016/j.jobcr.2022.10.007, 13 , 1, (36-40), (2023).
- Supannika Sorin, Yubin Zhou, Kanyarat Thithuan, Kullanat Khawkhiaw, Fuchun Zeng, Tummarat Ruangpratyakul, Surang Chomphoo, Wunchana Seubwai, Sopit Wongkham, Charupong Saengboonmee, High glucose enhances the aggressiveness of lung adenocarcinoma via activating epidermal growth factor receptor/signal transducer and activator of transcription 3 pathways, The Journal of Nutritional Biochemistry, 10.1016/j.jnutbio.2023.109399, 119 , (109399), (2023).
- Linhui Qin, Yingwu Mei, Chengcheng An, Rui Ning, Haifeng Zhang, Docosahexaenoic acid administration improves diabetes-induced cardiac fibrosis through enhancing fatty acid oxidation in cardiac fibroblast, The Journal of Nutritional Biochemistry, 10.1016/j.jnutbio.2022.109244, 113 , (109244), (2023).
- Daria A. Kotova, Aleksandra D. Ivanova, Matvei S. Pochechuev, Ilya V. Kelmanson, Yulia V. Khramova, Alisa Tiaglik, Mark A. Sudoplatov, Arina P. Trifonova, Anna Fedotova, Kseniia Morozova, Veronika A. Katrukha, Anastasia D. Sergeeva, Roman I. Raevskii, Mariia P. Pestriakova, Maxim A. Solotenkov, Evgeny A. Stepanov, Aleksandra S. Tsopina, Aleksandr A. Moshchenko, Milena Shestopalova, Anton Zalygin, Ilya V. Fedotov, Andrei B. Fedotov, Vladimir Oleinikov, Vsevolod V. Belousov, Alexey Semyanov, Nadezda Brazhe, Aleksei M. Zheltikov, Dmitry S. Bilan, Hyperglycemia exacerbates ischemic stroke not through increased generation of hydrogen peroxide, Free Radical Biology and Medicine, 10.1016/j.freeradbiomed.2023.08.004, 208 , (153-164), (2023).
- Wenhui Yan, Tingli Guo, Na Liu, Xin Cui, Xiaotong Wei, Yuzhuo Sun, Hao Hu, Lina Chen, Erythropoietin ameliorates cognitive deficits by improving hippocampal and synaptic damage in streptozotocin-induced diabetic mice, Cellular Signalling, 10.1016/j.cellsig.2023.110614, 106 , (110614), (2023).
- Sikha Thoduvayil, Jonathan S. Weerakkody, Ramalingam Venkat Kalyana Sundaram, Mackenzie Topper, Manindra Bera, Jeff Coleman, Xia Li, Malaiyalam Mariappan, Sathish Ramakrishnan, Rapid Quantification of First and Second Phase Insulin Secretion Dynamics using an In vitro Platform for Improving Insulin Therapy, Cell Calcium, 10.1016/j.ceca.2023.102766, 113 , (102766), (2023).
- Yuanlin Zhang, Ehsan Ranaei Pirmardan, Hua Jiang, Aliaa Barakat, Ali Hafezi-Moghadam, VEGFR-2 adhesive nanoprobes reveal early diabetic retinopathy in vivo, Biosensors and Bioelectronics, 10.1016/j.bios.2023.115476, 237 , (115476), (2023).
- Shivani Singla, Vinod Kumar, Gopabandhu Jena, 3-aminobenzamide protects against colitis associated diabetes mellitus in male BALB/c mice: Role of PARP-1, NLRP3, SIRT-1, AMPK, Biochimie, 10.1016/j.biochi.2023.03.009, 211 , (96-109), (2023).
- Johnathan T. Przybysz, Adrianne M. DiBrog, Katherine A. Kern, Ashmita Mukherjee, Jason E. Japa, Mariana H. Waite, Elizabeth G. Mietlicki-Baase, Macronutrient intake: Hormonal controls, pathological states, and methodological considerations, Appetite, 10.1016/j.appet.2022.106365, 180 , (106365), (2023).
- Ping Chen, Jun Li, Zhan Li, Duxia Yu, Ning Ma, Zian Xia, Xianglei Meng, Xingdang Liu, 18F-FP-CIT dopamine transporter PET findings in the striatum and retina of type 1 diabetic rats, Annals of Nuclear Medicine, 10.1007/s12149-022-01818-5, 37 , 4, (219-226), (2023).
- Nurliyana Ain Abdul Ghani, Nurul Alimah Abdul Nasir, Lidawani Lambuk, Muhammad Zulfiqah Sadikan, Renu Agarwal, Norlina Ramli, The effect of palm oil-derived tocotrienol-rich fraction in preserving normal retinal vascular diameter in streptozotocin-induced diabetic rats, Graefe's Archive for Clinical and Experimental Ophthalmology, 10.1007/s00417-022-05965-3, 261 , 6, (1587-1596), (2023).
- Aidan McGinnis, Michael Wang, Ru-Rong Ji, Animal Models of Pain and Anti-inflammatory Treatments, Neuroimmune Interactions in Pain, 10.1007/978-3-031-29231-6_3, (43-85), (2023).
- Lea Julie Dalco, Kunjan R. Dave, Diabetic Rodent Models for Chronic Stroke Studies, Neural Repair, 10.1007/978-1-0716-2926-0_30, (429-439), (2023).
- Hisashi Matsuda, Akifumi Nagatomo, Misaki Hatakeyama, Yoshiaki Manse, Masayuki Yoshikawa, Toshio Morikawa, Suppressive effects of Bombycis Feces (bombyx feces) and Bombyx Batryticatus (stiff silkworm) extracts on blood glucose level elevation in disaccharides‐loaded rats, Traditional & Kampo Medicine, 10.1002/tkm2.1368, 10 , 2, (132-141), (2023).
- Xiaoying Chen, Zhifei Zhang, Huiming Niu, Xinmiao Tian, Huibin Tian, Weiwei Yao, Huanshan He, Huaiping Shi, Cong Li, Jun Luo, Goat Milk Improves Glucose Metabolism in Type 2 Diabetic Mice and Protects Pancreatic β‐Cell Functions, Molecular Nutrition & Food ResearchMolecular Nutrition & Food ResearchMolecular Nutrition & Food Research, 10.1002/mnfr.202200842, 68 , 1, (2023).
- Qianyu Qu, Penggang He, Yuqi Zhang, Shujuan Yang, Peibin Zeng, The Intervention of Probiotics on Type 2 Diabetes Mellitus in Animal Models, Molecular Nutrition & Food ResearchMolecular Nutrition & Food ResearchMolecular Nutrition & Food Research, 10.1002/mnfr.202200815, 68 , 1, (2023).
- Heba A. Mubarak, Manal M. Kamal, Yossra Mahmoud, Fatma S. Abd‐Elsamea, Eman Abdelbary, Marwa G. Gamea, Reham I. El‐Mahdy, The ameliorating effects of mesenchymal stem cells compared to α‐tocopherol on apoptosis and autophagy in streptozotocin‐induced diabetic rats: Implication of PI3K/Akt signaling pathway and entero‐insular axis, Journal of Cellular Biochemistry, 10.1002/jcb.30482, 124 , 11, (1705-1719), (2023).
- Isabella Liba Pandolfo, Mirian Bonifacio, Izabelle Dias Benfato, Matheus Almeida Cruz, Márcia Regina Nagaoka, Carolina Prado de França Carvalho, Camila Aparecida Machado Oliveira, Ana Cláudia Muniz Renno, Photobiomodulation in diabetic rats: Effects on morphological, pancreatic parameters, and glucose homeostasis, Journal of Biophotonics, 10.1002/jbio.202300182, 16 , 11, (2023).
- Yangbo Lv, Shuiquan Lin, Mingsheng Liu, Lihui Wang, Xiaoyu Wang, Li Cui, Jianguang Xu, Impacts of pre‐existing diabetes mellitus on colorectal cancer in a mice model, Cancer Medicine, 10.1002/cam4.5868, 12 , 10, (11641-11650), (2023).
- Debasis Maity, Preetam Guha Ray, Peter Buchmann, Maysam Mansouri, Martin Fussenegger, Blood‐Glucose‐Powered Metabolic Fuel Cell for Self‐Sufficient Bioelectronics, Advanced Materials, 10.1002/adma.202300890, 35 , 21, (2023).
- Xiaoyu Han, Shuyu Chen, Zhengwei Cai, Ying Zhu, Weiwei Yi, Mengtong Guan, Bo Liao, Ying Zhang, Jieliang Shen, Wenguo Cui, Dingqun Bai, A Diagnostic and Therapeutic Hydrogel to Promote Vascularization via Blood Sugar Reduction for Wound Healing, Advanced Functional Materials, 10.1002/adfm.202213008, 33 , 14, (2023).
- Xue Yuan, Vedanshi Amin, Tianli Zhu, Mizuho Kittaka, Yasuyoshi Ueki, Teresita M. Bellido, Hakan Turkkahraman, Type 1 diabetes mellitus leads to gingivitis and an early compensatory increase in bone remodeling, Journal of Periodontology, 10.1002/JPER.22-0192, 94 , 2, (277-289), (2023).
- Harvey N Mayrovitz, Raneem Maqsood, Aneil S Tawakalzada, Do Magnetic Fields Have a Place in Treating Vascular Complications in Diabetes?, Cureus, 10.7759/cureus.24883, (2022).
- Mi-Hye Kwon, Min-Ji Choi, Fang-Yuan Liu, Fitri Rahma Fridayana, Lashkari Niloofar, Guo Nan Yin, Ji-Kan Ryu, Functional and Immunofluorescence Evaluations of Vascular and Neural Integrities in Urinary Bladder of Streptozotocin-Induced Diabetic Mice, International Neurourology Journal, 10.5213/inj.2244152.076, 26 , 3, (201-209), (2022).
- Eva Nurinda, Nurul Kusumawardani, Ari Susiana Wulandari , Annisa Fatmawati, E. Emelda, Husnatun Nisa, Nurjani A. Hasan, Wahyu Fajar Iriyanti, Mardiatun Rohmah, Puji Lestari, Veriani Aprilia, Pharmacological Study: Synergistic Antidiabetic Activity of Cinnamon Bark and Zingiber Extract in Streptozotocin-Induced Diabetic Rats, Open Access Macedonian Journal of Medical Sciences, 10.3889/oamjms.2022.9462, 10 , T8, (1-6), (2022).
- Valentina Boscaro, Matteo Rivoira, Barbara Sgorbini, Valentina Bordano, Francesca Dadone, Margherita Gallicchio, Aline Pons, Elisa Benetti, Arianna Carolina Rosa, Evidence-Based Anti-Diabetic Properties of Plant from the Occitan Valleys of the Piedmont Alps, Pharmaceutics, 10.3390/pharmaceutics14112371, 14 , 11, (2371), (2022).
- Abdullah A. Al-Ghanayem, Mohammed Sanad Alhussaini, Mohammed Asad, Babu Joseph, Moringa oleifera Leaf Extract Promotes Healing of Infected Wounds in Diabetic Rats: Evidence of Antimicrobial, Antioxidant and Proliferative Properties, Pharmaceuticals, 10.3390/ph15050528, 15 , 5, (528), (2022).
- Zhen Li, Shengguang Wang, Xinyu Wang, Peng Gao, Shiming Zhang, Yingning Mo, Dongsheng Zhao, Long Dai, Pharmacodynamic Interactions between Puerarin and Metformin in Type-2 Diabetic Rats, Molecules, 10.3390/molecules27217197, 27 , 21, (7197), (2022).
- Alexey Orlov, Savva Semenov, Gleb Rukhovich, Anastasia Sarycheva, Oxana Kovaleva, Alexander Semenov, Elena Ermakova, Ekaterina Gubareva, Anna E. Bugrova, Alexey Kononikhin, Elena I. Fedoros, Evgeny Nikolaev, Alexander Zherebker, Hepatoprotective Activity of Lignin-Derived Polyphenols Dereplicated Using High-Resolution Mass Spectrometry, In Vivo Experiments, and Deep Learning, International Journal of Molecular Sciences, 10.3390/ijms232416025, 23 , 24, (16025), (2022).
- Shuchan Sun, Awaguli Dawuti, Difei Gong, Ranran Wang, Tianyi Yuan, Shoubao Wang, Cheng Xing, Yang Lu, Guanhua Du, Lianghua Fang, Puerarin-V Improve Mitochondrial Respiration and Cardiac Function in a Rat Model of Diabetic Cardiomyopathy via Inhibiting Pyroptosis Pathway through P2X7 Receptors, International Journal of Molecular Sciences, 10.3390/ijms232113015, 23 , 21, (13015), (2022).
- Peir-Haur Hung, Yung-Chien Hsu, Tsung-Hsien Chen, Cheng Ho, Chun-Liang Lin, The Histone Demethylase Inhibitor GSK-J4 Is a Therapeutic Target for the Kidney Fibrosis of Diabetic Kidney Disease via DKK1 Modulation, International Journal of Molecular Sciences, 10.3390/ijms23169407, 23 , 16, (9407), (2022).
- Tyler N. Kambis, Hamid R. Shahshahan, Paras K. Mishra, Metabolites and Genes behind Cardiac Metabolic Remodeling in Mice with Type 1 Diabetes Mellitus, International Journal of Molecular Sciences, 10.3390/ijms23031392, 23 , 3, (1392), (2022).
- Bao Ting Zhu, Pathogenic Mechanism of Autoimmune Diabetes Mellitus in Humans: Potential Role of Streptozotocin-Induced Selective Autoimmunity against Human Islet β-Cells, Cells, 10.3390/cells11030492, 11 , 3, (492), (2022).
- Ioanna Prapa, Amalia E. Yanni, Anastasios Nikolaou, Nikolaos Kostomitsopoulos, Nick Kalogeropoulos, Eugenia Bezirtzoglou, Vaios T. Karathanos, Yiannis Kourkoutas, Dietary Pistachio (Pistacia vera L.) Beneficially Alters Fatty Acid Profiles in Streptozotocin-Induced Diabetic Rat, Applied Sciences, 10.3390/app12094606, 12 , 9, (4606), (2022).
- B. M. Williams, C. L. Cliff, K. Lee, P. E. Squires, C. E. Hills, The Role of the NLRP3 Inflammasome in Mediating Glomerular and Tubular Injury in Diabetic Nephropathy, Frontiers in Physiology, 10.3389/fphys.2022.907504, 13 , (2022).
- Yu-Zhong Chen, Jia Gu, Wei-Ting Chuang, Ya-Fang Du, Lin Zhang, Meng-Lan Lu, Jia-Ying Xu, Hao-Qiu Li, Yan Liu, Hao-Tian Feng, Yun-Hong Li, Li-Qiang Qin, Slowly Digestible Carbohydrate Diet Ameliorates Hyperglycemia and Hyperlipidemia in High-Fat Diet/Streptozocin-Induced Diabetic Mice, Frontiers in Nutrition, 10.3389/fnut.2022.854725, 9 , (2022).
- Jie Li, Zhen Wang, Xinyu Nan, Mingjie Yin, Hui Fang, Hotspots and frontier trends of diabetic associated cognitive decline research based on rat and mouse models from 2012 to 2021: A bibliometric study, Frontiers in Neurology, 10.3389/fneur.2022.1073224, 13 , (2022).
- Xiaoxia Guo, Chong Wang, Ranran Zhang, Xuliang Hao, Lei Lv, Yan Ni, Xiaohong Fan, Weiliang Zhang, Yunhong Jiao, Wei Song, Qi Dong, Yuqi Qi, Meiqing Song, Xuemei Qin, Scrophulariae Radix-Atractylodes sinensis pair and metformin inhibit inflammation by modulating gut microbiota of high-fat diet/streptozotocin-induced diabetes in rats, Frontiers in Microbiology, 10.3389/fmicb.2022.900021, 13 , (2022).
- Yinliang Chen, Jinbao Yang, Ying Wang, Weike Shen, Jinlin Liu, Meng Yuan, Xiaoyu Hao, Li Zhong, Rui Guo, Identification and Analysis of Hub Genes in Diabetic Cardiomyopathy: Potential Role of Cytochrome P450 1A1 in Mitochondrial Metabolism and STZ-Induced Myocardial Dysfunction, Frontiers in Cardiovascular Medicine, 10.3389/fcvm.2022.835244, 9 , (2022).
- Ryo Ichikawa, Sosuke Masuda, Junta Nakahara, Mio Kobayashi, Risako Yamashita, Suzuka Uomoto, Ohshima Kanami, Erika Hara, Yuko Ito, Makoto Shibutani, Toshinori Yoshida, Inhibition of autophagy with expression of NADPH oxidase subunit p22phox in preneoplastic lesions in a high-fat diet and streptozotocin-related hepatocarcinogenesis rat model, The Journal of Toxicological Sciences, 10.2131/jts.47.289, 47 , 7, (289-300), (2022).
- Ekowati Retnaningtyas, Budi Susatia, Siti Nur Arifah, Sri Rahayu Lestari, The improvement of insulin level after hydrogen-rich water therapy in streptozotocin-induced diabetic rats, Veterinary World, 10.14202/vetworld.2022.182-187, (182-187), (2022).
- Shatw Khalid Ali, Rojgar H. Ali, Effects of antidiabetic agents on Alzheimer’s disease biomarkers in experimentally induced hyperglycemic rat model by streptozocin, PLOS ONE, 10.1371/journal.pone.0271138, 17 , 7, (e0271138), (2022).
- Abdulaziz K. Al Mouslem, Antidiabetic and Hypolipidemic Potentials of Extract of Picris Babylonica in Streptozotocin-Induced Diabetic Model in Rats, Biomedical and Pharmacology Journal, 10.13005/bpj/2362, 15 , 1, (261-267), (2022).
- Andrew D Biancolin, Hyerin Jeong, Kimberly W Y Mak, Zixuan Yuan, Patricia L Brubaker, Disrupted and Elevated Circadian Secretion of Glucagon-Like Peptide-1 in a Murine Model of Type 2 Diabetes, Endocrinology, 10.1210/endocr/bqac118, 163 , 9, (2022).
- Wenjie Lu, Lingfei Yu, Lujun Wang, Songlin Liu, Manman Li, Zijun Wu, Shengqi Chen, Rongfeng Hu, Haiping Hao, Metformin Hydrochloride Mucosal Nanoparticles-Based Enteric Capsule for Prolonged Intestinal Residence Time, Improved Bioavailability, and Hypoglycemic Effect, AAPS PharmSciTech, 10.1208/s12249-022-02402-w, 24 , 1, (2022).
- Faheem Haider, Barkat Ali Khan, Muhammad Khalid Khan, Formulation and Evaluation of Topical Linezolid Nanoemulsion for Open Incision Wound in Diabetic Animal Model, AAPS PharmSciTech, 10.1208/s12249-022-02288-8, 23 , 5, (2022).
- Pingchuan Ma, Rufan Mo, Huabao Liao, Chengjie Qiu, Genhao Wu, Caixia Yang, Yunxiao Zhang, Yiran Zhao, Xue-Jun Song, Gut microbiota depletion by antibiotics ameliorates somatic neuropathic pain induced by nerve injury, chemotherapy, and diabetes in mice, Journal of Neuroinflammation, 10.1186/s12974-022-02523-w, 19 , 1, (2022).
- See more