Measurement of Trogocytosis: Quantitative Analyses Validated with Rigorous Controls
Ronald P. Taylor, Ronald P. Taylor, Margaret A. Lindorfer, Margaret A. Lindorfer
Abstract
Trogocytosis is a process in which receptors on acceptor cells remove and internalize cognate ligands from donor cells. Trogocytosis has a profound and negative impact on mAb-based cancer immunotherapy, as seen in the treatment of chronic lymphocytic leukemia (CLL) with CD20 mAbs, such as rituximab (RTX) and ofatumumab (OFA). Our clinical observations of RTX/OFA-mediated loss of the CD20 target from circulating CLL cells have been replicated in our in vitro studies. Here we describe flow cytometry and fluorescence microscopy experiments, which demonstrate that acceptor cells, such as monocytes/macrophages that express FcγR, remove and internalize both antigen and donor cell-bound cognate IgG mAbs for several different mAb-donor cell pairs. Fluorescent mAbs and portions of the plasma cell membrane are transferred from donor cells to acceptor cells, which include the THP-1 monocytic cell line as well as freshly isolated monocytes. We describe rigorous controls to validate the reactions and eliminate dissociation or internalization as alternative mechanisms. Trogocytosis is likely to contribute to neutropenia, thrombocytopenia, and liver damage associated with use of antibody-drug conjugates. The methods we have described should allow for examination of strategies focused on blocking trogocytosis and its adverse effects. © 2023 The Authors. Current Protocols published by Wiley Periodicals LLC.
Basic Protocol 1 : Trogocytosis of mAb-opsonized donor cells mediated by adherent THP-1 cells
Alternate Protocol : Application of fluorescence microscopy to examine THP-1 cell-mediated trogocytosis
Support Protocol 1 : Alexa labeling of mAbs and determination of F/P ratios
Support Protocol 2 : Standard washing procedure
Support Protocol 3 : Labeling and opsonization of cells
Basic Protocol 2 : Trogocytosis mediated by human monocytes as acceptor cells
Support Protocol 4 : Isolation of human monocytes
Basic Protocol 3 : Trogocytosis mediated by THP-1 cells in solution
Support Protocol 5 : Retinoic acid treatment of THP-1 cells
Support Protocol 6 : Culturing of SCC-25, BT-474, MOLT-4 and THP-1 cell lines
INTRODUCTION
mAb-Mediated Loss of CD20 on B cells Requires an Explanation
We have reported that prolonged intravenous infusion of the usual high doses of CD20 mAbs rituximab (RTX) or ofatumumab (OFA) (600 to 2000 mg over ∼8 hr), used in targeting circulating malignant B cells in the treatment of chronic lymphocytic leukemia (CLL), leads to pronounced loss of CD20 from circulating B cells in the later phase of the infusion (Beurskens et al., 2012; Kennedy et al., 2004). Initially these IgG mAb-targeted CLL B cells were cleared from the circulation and presumably phagocytosed by fixed tissue macrophages in the liver (Kupffer cells) (Atkinson & Frank, 1974; Atkinson et al., 1973; Schreiber & Frank, 1972a, 1972b). However, ∼1 hour after the start of this RTX/OFA infusion, a different pattern became evident: due to re-equilibration of more CLL B cells into the circulation from other compartments including the spleen and lymphatics, and likely saturation of Kupffer cells, the concentration of circulating CLL B cells increased from the previous RTX/OFA-induced nadir, even though additional CD20 mAb was still being infused into the bloodstream. These B cells became covalently tagged with C3 activation fragments, indicating that RTX /OFA must have bound to CD20 proteins initially present on the re-equilibrated cells, thus forming immune complexes that activated complement. However, the levels of CD20 on these isolated cells were soon substantially reduced, typically down to ∼15% or less of pre-RTX/OFA infusion levels (Beurskens et al., 2012; Kennedy et al., 2004). In the absence of CD20 mAb therapy, CD20 levels on re-equilibrated circulating B cells from the lymphatics and spleen are comparable or greater than CD20 levels of circulating cells (Pavlasova et al., 2016). The pattern of recrudescence of “low CD20” B cells covalently tagged with C3 activation fragments has now been replicated without exception in studies of more than 100 CLL patients who received either RTX or OFA therapy at four different institutions: the University of Virginia School of Medicine, the NIH, the Mayo Clinic, and the University of Rochester School of Medicine (Beurskens et al., 2012; Kennedy et al., 2004; Wallace et al., 2023; Zent et al., 2014, 2016).
Based on these clinical observations, we designed an in vitro model to investigate the mechanism by which substantial amounts of CD20 could have been removed (i.e., trogocytosed) from these re-equilibrated cells in the presence of excess circulating amounts of RTX/OFA (>30 μg/ml) (Beum et al., 2006). The in vitro experiments we conducted were based on incubating Alexa Fluor 488 (Al488)-labeled RTX/OFA-opsonized B cells with acceptor cells such as THP-1 monocytes. We demonstrated that Fcγ receptor-expressing acceptor cells are capable of rapidly (10 to 45 min) removing both cell-bound Al488-labeled CD20 mAb as well as CD20 from opsonized B cells in a concerted reaction in which portions of the plasma membrane of the donor B cell are also transferred to the acceptor cell. These observations, applied to the special case of cell-bound IgG immune complexes interacting with Fcγ receptor-expressing cells, recapitulate the original definition of trogocytosis (transfer of plasma membrane-associated ligands from donor cells to cognate receptors on acceptor cells) for this special case (Joly & Hudrisier, 2003). Other antigen substrates (on donor cells) that we have demonstrated to be successfully targeted for trogocytosis with specific mAbs include CD5 (MOLT-4 T cells), Her2/Neu (BT-474 breast cancer cells), and the epidermal growth factor receptor (SSC-25 squamous carcinoma cells) (Beum et al., 2008). We have found that additional donor cells are also susceptible to trogocytosis of CD20 include several B cell lines (Raji, Daudi, Z138 cells), as well as B cells isolated from normal individuals and patients with CLL. Acceptor cells (all of which express Fcγ receptors) include human monocytes, macrophages, neutrophils, and NK cells (Beum et al., 2006; Beum, Lindorfer, & Taylor, 2008; Beum et al., 2008; Beum et al., 2011; Taylor & Lindorfer, 2015). Here, we describe in detail, the general methodology we have used to examine trogocytosis mediated by THP-1 cells and monocytes.
The measurement of the transfer of fluorescent or radioactive probes from one cell to another is straightforward and its demonstration provides powerful first order evidence for trogocytosis. However, it is also necessary to include critical control experiments to clearly demonstrate and validate the reaction. These experiments include careful interrogation of unambiguously identified donor and acceptor cells. We will describe experiments that demonstrate trogocytosis based on flow cytometry or fluorescence microscopy. These experiments include trogocytosis mediated by adherent THP-1 cells as well as by THP-1 cells and monocytes in solution. Several Al488 mAb-donor cell pairs are examined. The general methods we describe allow for measurement of the transfer of the Al488 mAbs along with portions of the donor cell plasma membrane from the donor to acceptor cells. We also follow trogocytosis by making use of a secondary developing agent, Al488 mAb HB43, specific for the Fc region of human IgG. Use of this reagent reinforces the lessons learned with the Al488 mAbs because it tracks removal of unlabeled mAbs from the donor cells.
NOTE : Appropriate informed consent is necessary for obtaining and use of human study material.
Basic Protocol 1: TROGOCYTOSIS OF mAb-OPSONIZED DONOR CELLS MEDIATED BY ADHERENT THP-1 CELLS
The experimental paradigm (Fig. 1) that we have employed for quantitative measurement of trogocytosis involves a 15 to 60 min incubation at 37°C of Al488- (or Al647-) mAb-opsonized donor cells with acceptor cells such as adherent THP-1 monocytic cells (see Support Protocol 1). All samples are run in duplicate or triplicate and usually three or more controls are included to validate the experimental trogocytosis condition. Opsonized donor cells are added to empty wells for a 45 min incubation at 37°C to determine if the cell-bound opsonizing mAb simply dissociates from (or is internalized by) the donor cells (Control 1). Opsonized donor cells (first held on ice for 45 min) are added to either empty wells (Control 2, no trogocytosis), or to wells with adherent THP-1 cells (Control 3, zero-time), followed by immediate quenching with cold BSA-PBS to test for the effect of simple processing of the samples (centrifugation and wash steps). In previous experiments we reported that samples that were simply held on ice and never exposed to THP-1 cells had identical signals to the zero-time samples. In this article we only report the zero-time controls. Finally, sample 4 is the experimental trogocytosis protocol in which opsonized donor cells are reacted with adherent acceptor THP-1 cells for 15 to 60 min at 37°C and are then quenched by addition of cold BSA-PBS. After quenching, the donor cells are transferred from the adhered THP-1 cells to a FACS tube by gentle pipetting, washed with BSA-PBS (see Support Protocol 2**),** and then the (putatively) trogocytosed donor cells, along with the control samples, are analyzed by flow cytometry.
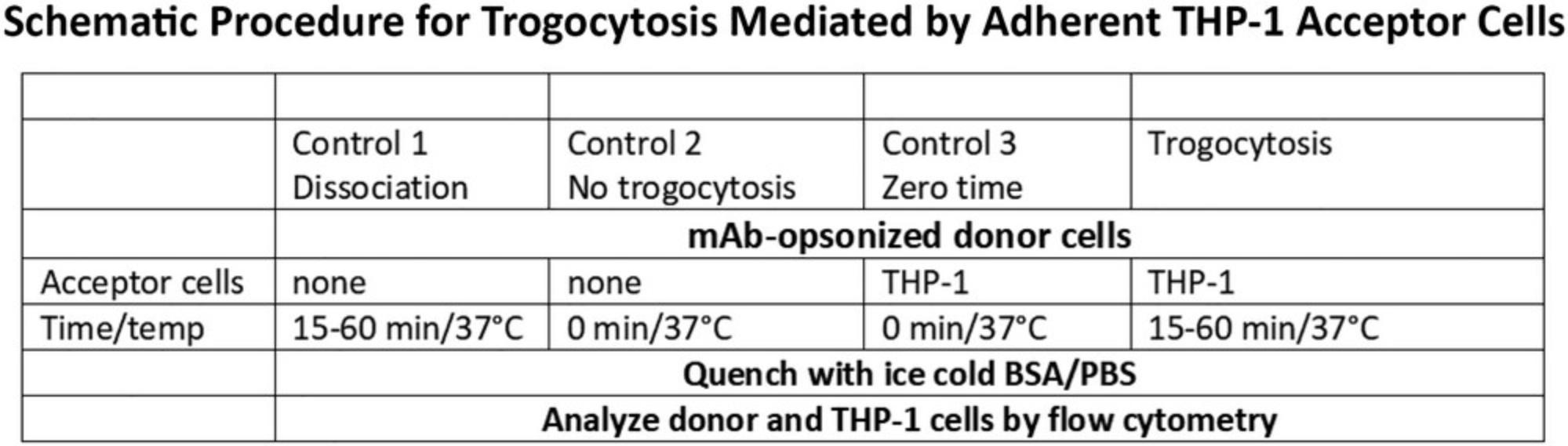
The flow cytometry analyses include several of the following determinations: The trogocytosed donor cells are analyzed for the level of (residual) bound Al488 mAb (FL1). Alternatively, the trogocytosed donor cells are re-opsonized with Al488 mAb, washed, and then analyzed by flow cytometry. This procedure, combined with the other measurements, tests for simple dissociation of cell-bound Al488 mAb and allows for determination of the total amount of target antigen available on the trogocytosed donor cells after the trogocytosis reaction. As is illustrated below, we find that the % trogocytosis, which reflects loss of the Al488 mAb from donor cells, is in very good agreement with the % trogocytosis determined based on the % available antigen after re-opsonization. In some experiments the donor cells are also stained with the membrane dye PKH 26, immediately before or after opsonization is equally effective (see Support Protocol 3). After the trogocytosis reaction the acceptor cells are examined for uptake of PKH26 (FL2 signal), which reflects transfer of plasma membrane fragments from the donor cells that occurred in concert with removal of the opsonizing mAb. These experiments make use of either 2 or 3 different colors in the flow cytometry analyses (FL1, FL2, FL4) and in all cases compensation controls with cells stained with a single dye are first examined. The level of PKH26 dye remaining on the donor cells is considerably greater than the amount of PKH26 taken up by the acceptor cells, and this allows for additional and reliable analyses of the donor and acceptor cells. As is illustrated in selected experiments below, trogocytosis always includes transfer of membrane fragments from the donor cell to the acceptor cell.
The acceptor cells, which in the illustrated examples are either THP-1 cells or freshly isolated human monocytes, are examined by flow cytometry. In reactions in which the THP-1 cells are adhered to wells, after the trogocytosed donor cells are gently removed from the wells followed by a wash, the adherent THP-1 cells are then removed from the wells by vigorous pipetting.
We will describe additional controls in several experiments that are designed to enhance the validity of the measurements. For example, we have determined that the processes we are studying are mediated by Fcγ receptors on acceptor cells (Beum et al., 2006), and we find (see below) that pre-incubation of acceptor cells with human IgG (to block Fc receptors) also substantially blocks trogocytosis. We have also demonstrated trogocytosis based on probing donor cells with Alexa-labeled mAb HB43, which binds to the Fc region of chimeric/humanized mAbs (see Basic Protocol 3). Use of mAb HB43 allows for evaluation of unlabeled cell-bound mAb [e.g., cetuximab (CET), trastuzumab (TRA)]. As already noted, it is certainly possible that the Al488 mAb simply dissociates from the opsonized donor cell, and then the released mAb may be taken up by Fcγ receptors on the acceptor cell. To test for this possibility, we incubate the opsonized donor cells in buffer alone for 45 min at 37°C (Control 1). The supernatant, possibly containing released opsonizing mAb, is then added to unreacted but adherent THP-1 cells to determine if they take up any putatively released Al488 mAb.
Materials
-
Cells
- THP-1 acceptor cells (ATCC, cat. no. TIB202)
- MOLT-4 donor cells (T cell antigen CD5; ATCC, cat. no. CRL-1582)
- BT-474 donor cells (Her/Neu+; ATCC, cat. no. HTB-20)
- SCC-25 donor cells (epidermal growth factor positive; ATCC, cat. no. CRL-1628)
- _All cells were grown in culture following ATCC guidelines, with special handling as noted in the cell culture section below (_Support Protocol Support Protocol 6 ).
-
Complete RPMI 1640 medium (see recipe)
-
Phorbol 12-myristate 13-acetate (PMA) (Sigma, cat. no. P8139)
-
mAbs
- Purified human IgG (Lampire Biological Laboratories, cat. no. 7403704)
- Cocktail, consisting of 120 μl biotinylated mAb to CD11b (0.2 mg/ml; BD Pharmingen, cat. no. 555387), 120 μl biotinylated mAb to CD14 (0.2 mg/ml; Caltag, cat. no. MHCD1415-4), and 160 μl BSA-PBS (see recipe).
- Rituximab (RTX), a chimeric (mouse/human) mAb, specific for CD20 (UVA Hospital Pharmacy)
- Trastuzumab (TRA), a humanized mAb specific for Her2/Neu on breast cancer cells (UVA Hospital Pharmacy)
- Cetuximab (CET) a chimeric (mouse/human) mAb specific for the human epidermal growth factor (UVA Hospital Pharmacy)
- mAb T101, a mouse mAb specific for CD5 expressed on T cells (a gift from Dr. J. Carrasquillo, NIH)
- HB43, a mouse mAb specific for the Fc region of human IgG (ATCC, cat. no. 1410 KG7)
- mAb HB43 binds to the Fc region of both TRA and CET but does not bind to mouse mAb T101.
-
BSA-PBS (see recipe)
-
Phosphate-buffered saline (PBS) (Current Protocols, 2006)
-
Alexa 647 (Al647) streptavidin (Molecular Probes, cat. no. S32357)
-
Ultra Rainbow Quantitative Particle Kit (Spherotech, cat. no. RCP-30-20A)
-
Hemocytometer (e.g., Weler Scientific 304811)
-
24-well plates (e.g., Corning Costar 3524)
-
Standard cell culture hood, 5% CO2 and 37°C for all cell culture and trogocytosis experiments
-
Simple light microscope for cell counting and inspection of adherent cells
-
Refrigerated centrifuge, 4°C (Eppendorf 5810R), equipped with a swinging bucket rotor (A-4-81)
-
Ice
-
FACS tubes, polystyrene, round bottom centrifuge tubes compatible with flow cytometer sample port (e.g., Thermo Fisher, cat. no. 341661)
-
FACSCalibur dual wavelength flow cytometer (Becton-Dickinson)
1.Count THP-1 cells using a hemocytometer. Suspend THP-1 cells at 1 × 106 cells/ml in complete RPMI 1640 medium supplemented with 1 μM PMA. Transfer 1 ml aliquots to the wells of a 24-well culture plate, cover and culture for 2 to 3 days at 37°C in 5% CO2 in a tissue culture incubator.
2.Inspect the wells under 10× magnification to verify that cells have adhered. Remove the medium by aspiration and incubate the adherent THP-1 cells for 1 h at 37°C with either 100 μl complete RPMI 1640 medium containing 2 mg/ml purified human IgG or complete RPMI 1640 medium alone (control).
3.Add 1-10 × 105 PKH26-labeled Al488 T101-opsonized donor cells or PKH26-labeled donor cells (not opsonized with Al488 T101) in 400 μl complete RPMI 1640 medium to the THP-1 cells into both the positive control wells and the IgG-containing wells (see Figure 1 and Support Protocol 3).
4.Centrifuge the plate 1 min at 50 × g , 4°C, to pellet the donor cells. Cover the plate and incubate for 45 min at 37°C, 5% CO2.
5.Add 2 ml ice-cold BSA-PBS to each well to quench the reaction samples. In additional controls, add PKH26-labeled Al488 T101-opsonized donor cells (held on ice for 45 min) to wells containing THP-1 cells, followed by immediate quenching with 2 ml cold BSA-PBS. This is the zero-time control.
6.Transfer all quenched samples to FACS tubes, wash the samples twice (see Support Protocol 2) and reconstitute in 250 μl PBS after the last wash.
7.Examine the PKH26-labeled donor cells by flow cytometry using an FL2 gate that excludes the weakly-positive PKH26 signal on the THP-1 cells. Count 8000 events.
8.Isolate the THP-1 cells after the trogocytosis reaction as follows:
-
Wash the wells previously used for the trogocytosis reaction 3 times with BSA-PBS.
-
Add 1 ml BSA-PBS and pipet vigorously 10 times to release the THP-1 cells.
-
Transfer the released THP-1 cells to FACS tubes.
-
Wash once (see Support Protocol2).
-
Reconstitute to 250 μl in PBS containing 2 mg/ml mouse IgG to reduce non-specific binding of probes.
9.Probe the released THP-1 cells as follows:
-
Incubate the THP-1 cells with 10 μl of a cocktail containing 0.06 μg/ul each of biotinylated antibodies to CD11b and to CD14.
-
Incubate these samples for 30 min on ice, and then wash twice (see Support Protocol2).
-
Resuspend the cells to 100 μl in BSA-PBS and add 10 μl of 0.1 mg/ml Al647 streptavidin.
-
After a 30 min incubation on ice, wash the samples twice in BSA-PBS and resuspend to 250 μl in PBS.
-
Analyze by flow cytometry based on FL1/FL2/FL4 (collect 12,000 events).
Compared to the zero-time samples, the released THP-1 cells (FL4 positive) should manifest increases in the Al488 and PKH26 (FL1 and FL2) signals, due to trogocytosis. However, these signals should remain at baseline for the THP-1 cells incubated with un-opsonized donors or cells subject to dissociation. Finally, the FL1 and FL2 signals for THP-1 cells blocked with human IgG should be close to baseline as well.
10.Test for dissociation of the Al488 mAb from the donor cells as follows:
-
Incubate the PKH26-labeled Al488 T101-opsonized donor cells in empty wells for 45 min at 37°C.
-
Centrifuge the plate 1 min at 50 ×g, 4°C.
-
Transfer the supernatant to THP-1 cells in a separate well followed by another incubation of 45 min at 37°C.
-
Release the THP-1 cells from the plate by vigorous pipetting and analyze them by flow cytometry to determine if they take up any Al488 or PKH26 that could have been released into the supernatant from the PKH26-labeled Al488 T101-opsonized donor cells.
Based on numerous calibration studies, we expect that there will be little, if any increase in theAl488 and PKH26 background signals (compared to background) for the released THP-1 cells.
MESF calculations for flow cytometry experiments
In order to obtain a rigorous quantitative measure of trogocytosis, mean fluorescence intensities, recorded as channel numbers, are converted to molecules of equivalent soluble fluorophore (MESF units) based on using a graduated series of fluorescent beads, with defined MESF values (Spherotech). The channel number of each standard bead is determined by flow cytometry under the exact instrument settings for each experiment. The slope (m) and intercept (b) of a plot of the log defined MESF vs experimental channel number are calculated using the equation: log MESF (y) = mx + b, where x = channel number. In the example in Figure 2 the derived values are m = 0.00405 and b = 2.865.Based on these values, it is then straight-forward to convert any given channel number into an MESF value, based on calculating anti-logs. For instance, in this example, a channel number of 600 would convert to an MESF of 197,701.
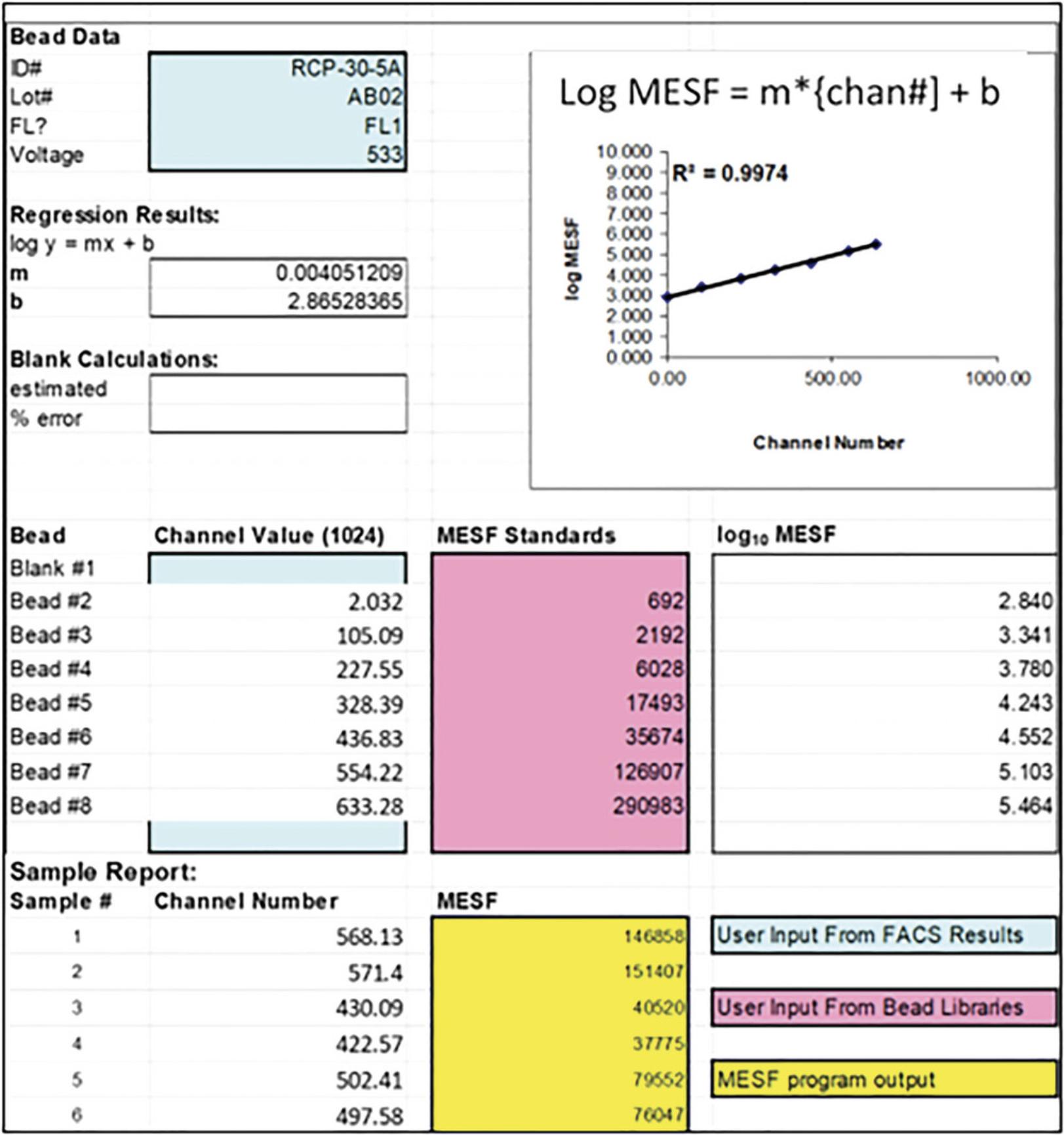
Figure 3 shows flow cytometry analyses of trogocytosis of donor PKH26-labeled MOLT 4 cells opsonized with Al488 T101 and then reacted with adherent acceptor THP-1 cells at donor to acceptor ratios of 1:10 (Fig. 3A) or 1:1 (Fig. 3B). The light scattering gating scheme identifies the MOLT 4 cells. Cells were reacted for 45 min at 37°C with THP-1 cells alone (trogocytosis, pink) or with THP-1 cells first blocked with 2 mg/ml human IgG (blocked, green). Alternatively, the donor cells were first incubated in medium alone for 45 min at 37°C and then combined with THP-1 cells and immediately quenched with cold BSA-PBS (blue, zero-time). The gating scheme was set to eliminate any cells that were weakly positive for PKH26, thus only showing the donor MOLT 4 cells. Figure 3A shows MESF values for the respective 3 samples reacted at donor:acceptor ratios of 1:10 were 29,000; 54,000; and 59,000. The % trogocytosis, based on comparing the trogocytosis sample with the zero-time sample is therefore ∼50%: 100 × [(59,000–29,000)/59,000)] %, and it is evident that human IgG substantially blocked trogocytosis. In Figure 3B, a similar but separate experiment with a donor:acceptor ratio of 1:1, the MESF values for the three samples were 37,000 (pink, trogocytosis); 49,000 (green, IgG block); and 54,000 (blue, zero-time), corresponding to ∼30% trogocytosis.
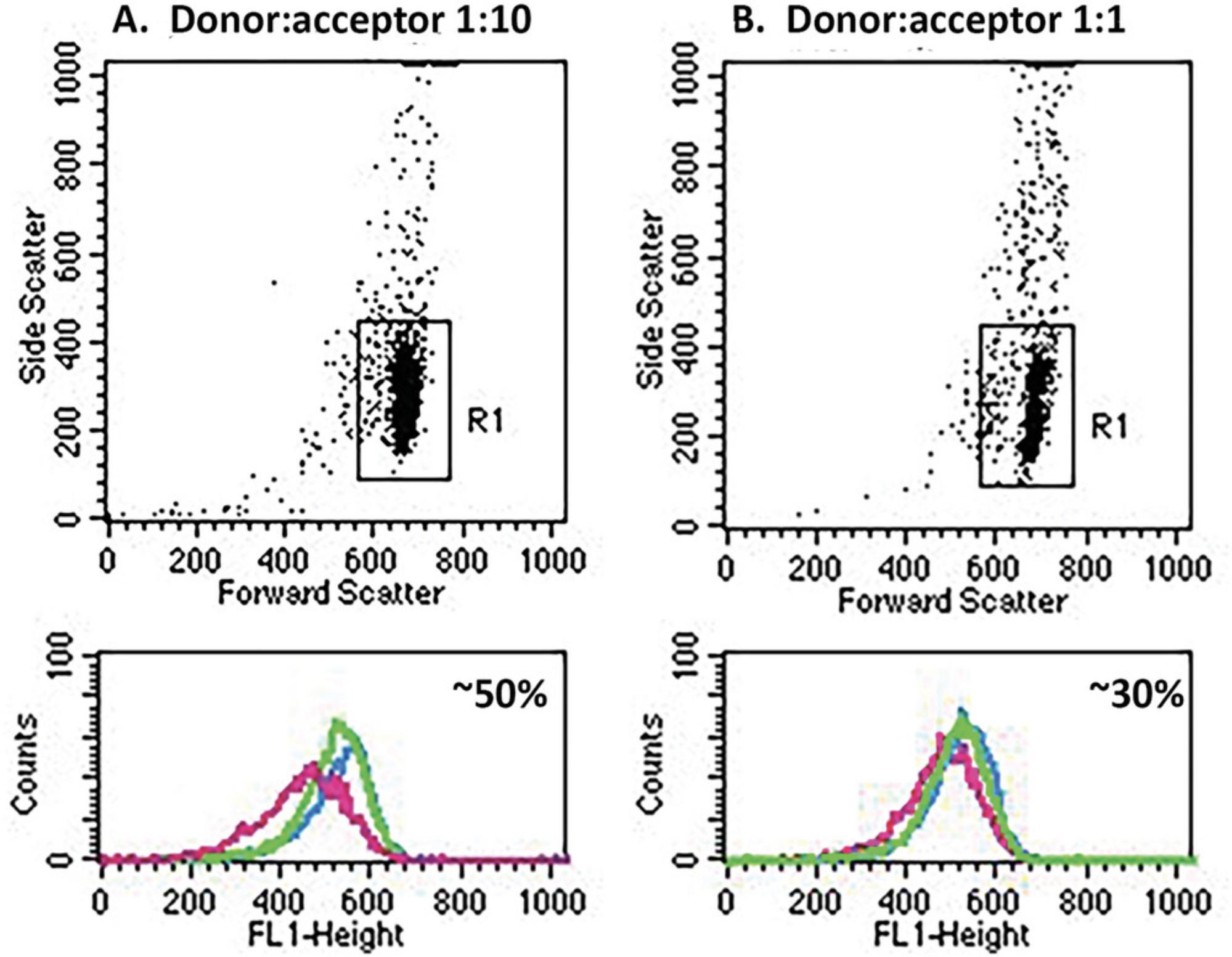
Figure 4 shows the flow cytometry analyses of the uptake of both Al488 T101 (FL1) and PKH26 (FL2) by THP-1 cells for the experiment illustrated in Figure 3B. The gating scheme was set to include cells weakly positive for PKH26 (Fig. 4A). Dot plots illustrate the uptake of Al488 (top plot) and PKH26 (bottom plot) for FL4 positive THP-1 cells identified by probing with biotinylated CD11b/CD14 and secondarily developed with Al647 streptavidin. The quadrants were defined by analysis of naïve THP-1 cells (not shown). Fig. 4B, trogocytosis; Fig. 4C, zero-time; Fig. 4D, blocked with human IgG; Fig. 4E, dissociation (see Step 10); Fig. 4F, THP-1 cells incubated with un-opsonized PKH26-labeled MOLT-4 cells. The results demonstrate that approximately 26% and 43% of the THP-1 cells acquire Al488 and PKH26, respectively (Fig. 4B). Uptake is substantially blocked by pre-incubation of the THP-1 cells with human IgG (Fig. 4D). It should be noted that in the zero-time sample (Fig. 4C), very few donor cells are demonstrable, because they were only added after the 45 min incubation and then immediately quenched and processed. In the dissociation control (Fig. 4E), the donor cells were incubated for 45 min at 37°C in the absence of THP-1 cells. The cells were pelleted, and the supernatant was transferred to THP-1 cells which were incubated for 45 min at 37°C and then washed and analyzed.
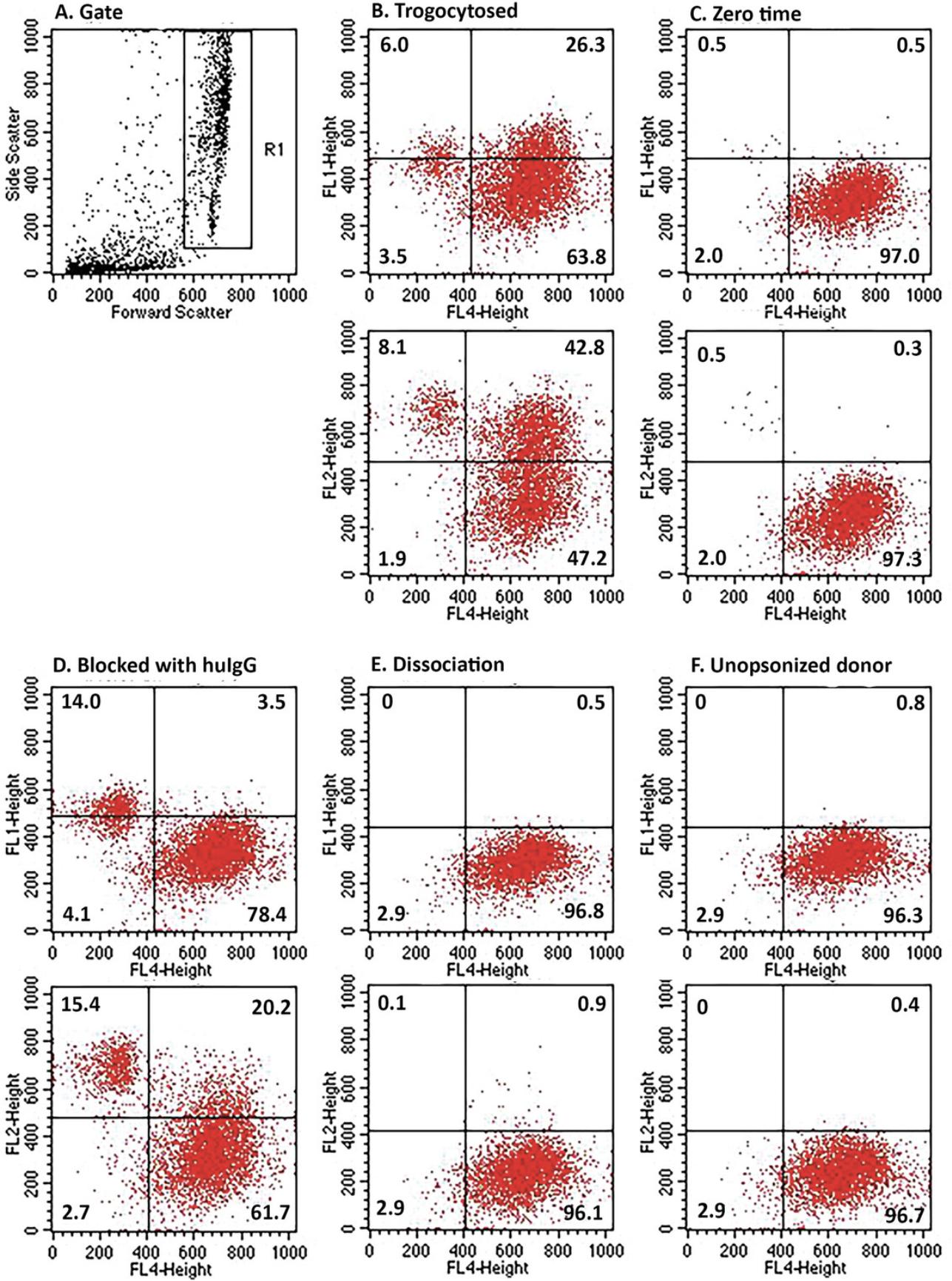
Alternate Protocol: APPLICATION OF FLUORESCENCE MICROSCOPY TO EXAMINE THP-1 CELL-MEDIATED TROGOCYTOSIS
This procedure provides an alternative and quite visual determination of trogocytosis. Indeed, in this case, “seeing is believing.”
Additional Materials (also see Basic Protocol 1)
- Microscope slides (e.g., Fisherbrand, cat. no. 12550423)
- Coverslips (e.g., Fisher, Eisco S24461)
- Fingernail polish
- Fluorescent microscope equipped with a digital camera (BX40 Olympus with a Magnafire digital camera) and filters suitable for FITC and Texas Red
1.Repeat steps 1 to 9 of Basic Protocol 1 with the following modification. In step 3 use Al488 mAb-opsonized donor cells that are not dyed with PKH26. Use pairs of mAb-opsonized cells, e.g., Al488 T101-opsonized MOLT-4 cells; Al488 CET-opsonized BT-474 cells; or Al488 TRA-opsonized SCC-25 cells (see Support Protocol 3).
2.Concentrate aliquots of the isolated THP-1 cells by centrifugation for 1 min at 800 × g , 4°C, followed by reconstitution in a small volume of PBS to ∼1 × 107 cells/ml.
3.Apply 10 μl of the concentrated cell suspension to a microscope slide, cover with a cover slip, and seal with fingernail polish.
4.Examine in a BX40 Olympus fluorescent microscope (100×) with oil, under either white light or based on the FITC or Texas Red filters.
Figure 5 shows the results of trogocytosis experiments in which the THP-1 cells were isolated and examined by fluorescence microscopy after the trogocytosis reaction (45 min, 37°C). This experiment is similar to the experiment described in Figure 4, except the donor cells were not labeled with PKH26. In this case they were reacted with one of the three respective Al488 cognate mAbs. After the trogocytosis reaction the THP-1 cells were identified by probing with biotinylated mAbs specific for CD11b and CD14 (see Basic Protocol 1, step 9) followed by Al647 streptavidin. It is clear that the red acceptor monocytes have taken up the green Al488 mAbs in all three cases.
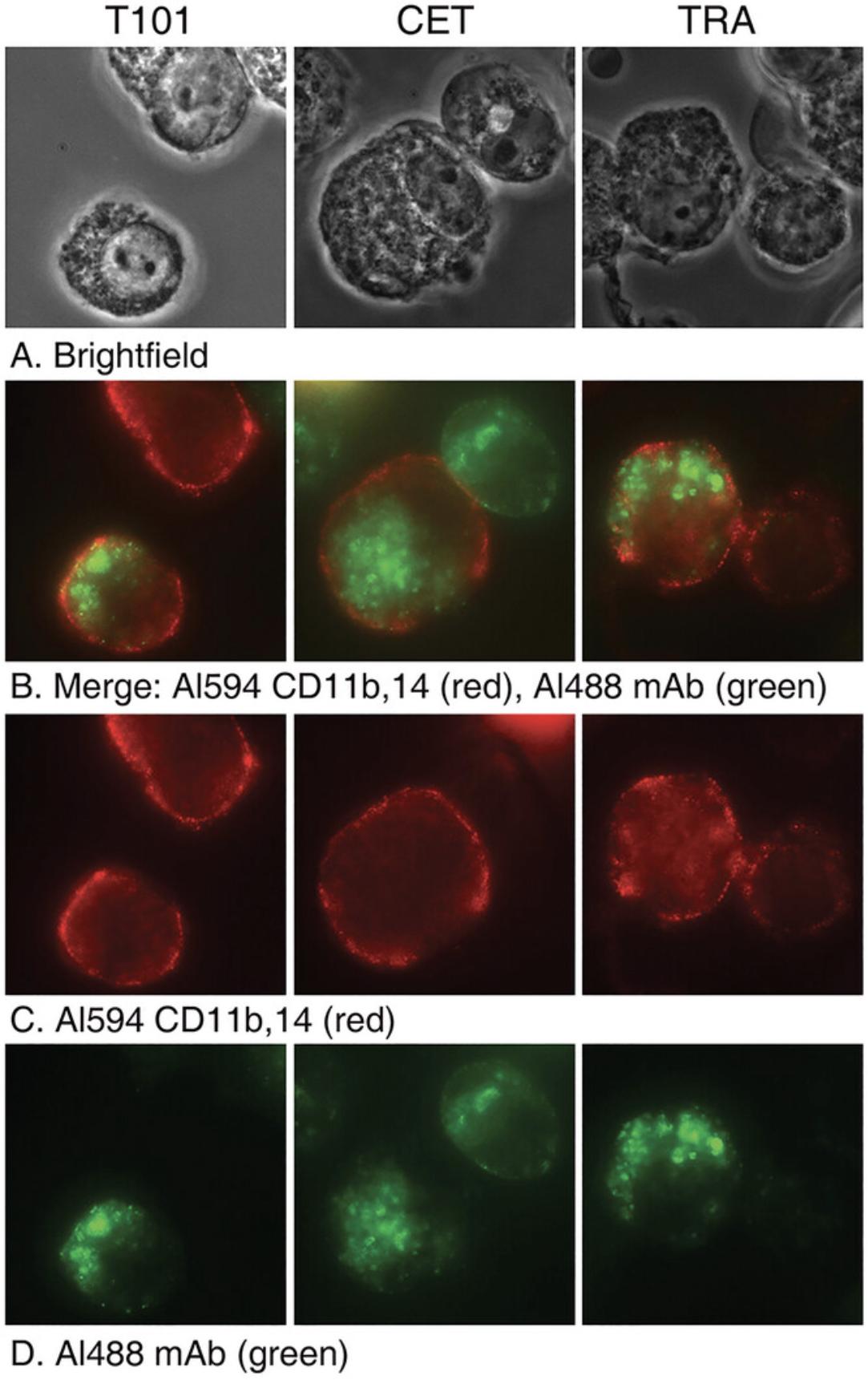
Support Protocol 1: ALEXA LABELING OF mAbs AND DETERMINATION OF F/P RATIOS
Almost all of our trogocytosis experiments require use of well-defined fluorescent mAbs. Here we describe our general labeling procedure.
Materials
-
Borate saline (BS) (see recipe)
-
Alexa 488 NHS (ThermoFisher, cat. no. A20000)
-
1 M bicarbonate buffer (see recipe)
-
Dimethylsulfoxide (DMSO), reagent grade
-
1 M Tris, pH 8 (Current Protocols, 2006)
-
Dialysis membranes, Spectra Por (Cole Parmer, cat. no. 131201)
-
Spectrophotometer
-
pH strip
-
Gel filtration columns (Bio-Rad, cat. no. PDP10)
1.Dialyze mAbs against BS to remove any competing primary amines.
2.Determine antibody concentration using a spectrophotometer by measuring the absorbance at 280 nm (A280). The A280 divided by 1.5 gives the mAb concentration in mg/ml.
3.Allow Alexa NHS vial to come to room temperature before opening the vial.
4.Raise pH of the mAb solution by adding a 10% volume of 1 M bicarbonate buffer.
5.Use pH strip to make sure the pH is >8.
6.Immediately before use, dissolve 1 mg Alexa 488 NHS in 100 µl DMSO.
7.Add 10 µl Alexa dye/mg mAb.
8.Mix gently by inversion. Do not vortex.
9.React, protected from light, 1 hr at room temperature.
10.Add 50 µl 1 M Tris, pH 8 to stop the reaction.
11.Remove unreacted dye from Alexa-mAb by gel filtration on BioRad columns equilibrated with BS buffer. The protein-dye conjugate exits in the void volume.
12.Measure the absorbance at 280 nm and 495 nm of the isolated Al488 mAbs.
13.Calculate mg/ml mAb concentration and F/P ratio according to manufacturer's directions as follows:
where 203,000 is the molar extinction coefficient (ε) in cm–1 M–1 of a typical IgG at 280 nm which is suitable for IgA, IgD, and IgE as well. The value CF280 is a correction factor for the fluorophore's contribution to the absorbance at 280 nm. For Alexa 488 the CF280 is 0.11.
14.
where εdye (in cm–1M–1) is the molar extinction coefficient of the specific dye. Use 71,000 as the molar extinction coefficient for Al488 at 495 nm. A similar calculation is used for Al647 mAbs, except Al647 has a different extinction coefficient and correction factor. See the ThermoFisher web site for a table of extinction coefficients and correction factors for Document Connect (thermofisher.com).
Support Protocol 2: STANDARD WASHING PROCEDURE
All of our procedures require multiple wash steps to insure the reliability of the measurements and the general wash procedure is now presented.
Additional Materials (also see Basic Protocol 1)
- 15-ml conical tubes (optional)
- Vortex
1.Dilute cells with ∼20 volumes of BSA-PBS.
2.Vortex.
3.Pellet by centrifuging 2 to 5 min at 800 × g , 4°C. Use either FACS tubes or 15-ml conical tubes, depending upon the volume of cells to be processed.
Support Protocol 3: LABELING AND OPSONIZATION OF CELLS
It is important to establish uniformity in fluorescent labeling of cells and mAbs. The procedures are presented here.
Additional Materials (also see Basic Protocol 1)
- Cognate donor cells, MOLT-4, BT-474, SCC-25 (see Basic Protocol 1)
- Alexa-labeled mAb (see Support Protocol 1)
- Acceptor cells, e.g., THP-1 (see Basic Protocol 1), human monocytes (see Support Protocol 4)
- PKH26 (Sigma, cat. no. PKH26GL)
- Diluent C (Sigma, cat. no. PKH26GL)
- FBS (ThermoFisher, cat. no. A4766801)
Opsonization of donor cells
1.Disperse donor cells in complete RPMI 1640 medium to 1-10 × 106 cells/ml and incubate 10 to 20 min at 37°C with specific Al488-labeled or Al647-labeled mAbs (3 to 10 μg/ml final).
2.Wash once with BSA-PBS.
3.Reconstitute in complete RPMI 1640 medium.
PKH26 labeling of cells
4.Follow the manufacturer's procedures with slight modifications.
5.Gently resuspend pelleted cells at 4 × 107 cells/ml in diluent C (Sigma) by carefully pipetting.
6.Immediately add PKH26 (2 μl per 500 μl cells) diluted in an equal volume of diluent C.
7.Mix gently by inversion. Do not vortex.
8.Incubate for 45 s at room temperature, then stop reaction by addition of equal volume of FBS.
9.Let stand 1 min.
10.Pellet cells by centrifuging 5 min at 200 × g , room temperature.
11.Wash 3 times in BSA-PBS, spinning 5 min at 200 × g , room temperature.
12.Resuspend in complete RPMI 1640 medium at desired density.
Basic Protocol 2: TROGOCYTOSIS MEDIATED BY HUMAN MONOCYTES AS ACCEPTOR CELLS
In this protocol monocytes are isolated from fresh blood and their ability to mediate trogocytosis of PKH26-labeled Al488 T101-opsonized MOLT4 cells is examined. Trogocytosis is conducted in solution phase; the donor and acceptor cells are mixed, briefly centrifuged, and then incubated for 60 min at 37°C. Controls that were used previously are included, and in addition, putatively trogocytosed cells (manifesting loss of opsonizing Al488 mAb) are re-opsonized to provide a direct and independent measure of the loss of the target antigen from the donor cells.
Additional Materials (also see Basic Protocol 1 and Support Protocols 2 to 4)
- mouse IgG (Lampire Biological Laboratories, cat. no. 7404304)
1.Label the donor cells with PKH26 followed by opsonization with the respective cognate Al488 mAbs (see Support Protocol 3).
2.Wash the cells 3 times in BSA-PBS, and reconstitute them in complete RPMI 1640 medium to a final concentration of 1 × 106 cells/ml. A total of at least 1 × 106 donor cells (in 1 ml) (see step 4) are needed for each experiment.
3.Incubate freshly isolated human monocytes in complete RPMI 1640 medium with or without blockade with 2 mg/ml human IgG for 1 hr at 37°C, at a cell concentration of 5 × 106 cells/ml.
4.Prepare the following incubation mixtures in FACS tubes, all in duplicate.
100 µl PKH26-labeled mAb-opsonized donor cells | A | 100 μl naïve monocytes |
B | 100 μl monocytes treated with human IgG | |
C | 100 μl medium | |
D | 100 μl medium |
In A to D (final volume of 200 μl) there are 1 × 105 donor cells. In A and B, there are 5 × 105 monocytes. Briefly centrifuge the samples 10 to 15 s at 300 × g , room temperature, to bring the donor and acceptor cells close together.
5.Perform the following:
-
Incubate samples for 60 min at 37°C in 5% CO2.
-
Vortex the tubes and place them on ice.
-
Add 100 μl of naïve monocytes (5 × 105cells) to sample C (zero-time), and immediately quench all samples with 4 ml of ice cold BSA-PBS.
-
Pellet and wash the samples once.
-
Resuspend the cells in 300 μl PBS and split each sample into two equal aliquots.
-
Hold on ice.
6.Examine the donor cells in one aliquot by flow cytometry. Collect 7000 events per sample.
7.Pellet the second aliquot and resuspend cells in 100 μl of BSA-PBS containing 2 mg/ml mouse IgG (added to prevent non-specific binding of probes).
8.Probe samples A, B, and C with the biotinylated CD11b/CD14 cocktail followed by Al647 streptavidin to identify monocytes as in Basic Protocol 1, step 9.This will allow for identification of monocytes based on the FL4 signal. Sample D is not probed as it has no monocytes. To test for the total amount of antigen remaining on the donor cells, also add 10 μl of 0.1 mg/ml of the opsonizing Al488 mAb to Samples A to D and incubate for 15 min, room temperature.
9.Wash the samples once and reconstitute to 250 μl with PBS and examine by flow cytometry.
Figure 6 shows that trogocytosis mediates loss of Al488 mAb T101 signal from opsonized MOLT4 cells incubated with freshly isolated human monocytes in solution for 1 hr, 37°C. FL2-positive donor cells were analyzed for FL1 signal. In addition to the trogocytosis sample (pink, 24,000 MESF), the controls include zero-time (blue, 64,000 MESF); IgG block (green, 64,000 MESF) and simple dissociation (yellow, 65,000 MESF). The yellow and blue histograms coincide with the green histogram. Based on these values, the % trogocytosis was ∼63%. In additional controls, the cells were re-opsonized with Al488 T101 to allow for measurement of total available antigen accessible. This second opsonization had only marginal effects on the Al488 signals associated with the cells (results not shown). The results for the trogocytosed sample before (pink, 24,000 MESF) and after re-opsonization (29,000 MESF) are quite similar. Moreover, the values for the zero-time samples before (64,000) and after re-opsonization (61,000) are quite similar as well.
Figure 7 shows analyses of the uptake by monocytes (identified as FL4-positive) of Al488 mAb T101 and PKH26 for the experiment illustrated in Figure 6.Under conditions that allowed for trogocytosis (Fig. 7B), approximately 37% and 33% of the monocytes took up Al488 (top plot) and PKH26 (bottom plot), respectively. However, when the reaction was blocked with human IgG (Fig. 7C), the % uptake was reduced to 1% and 3%. respectively. There is little, if any, uptake of either Al488 or PKH26 for the zero-time sample (Fig. 7D).
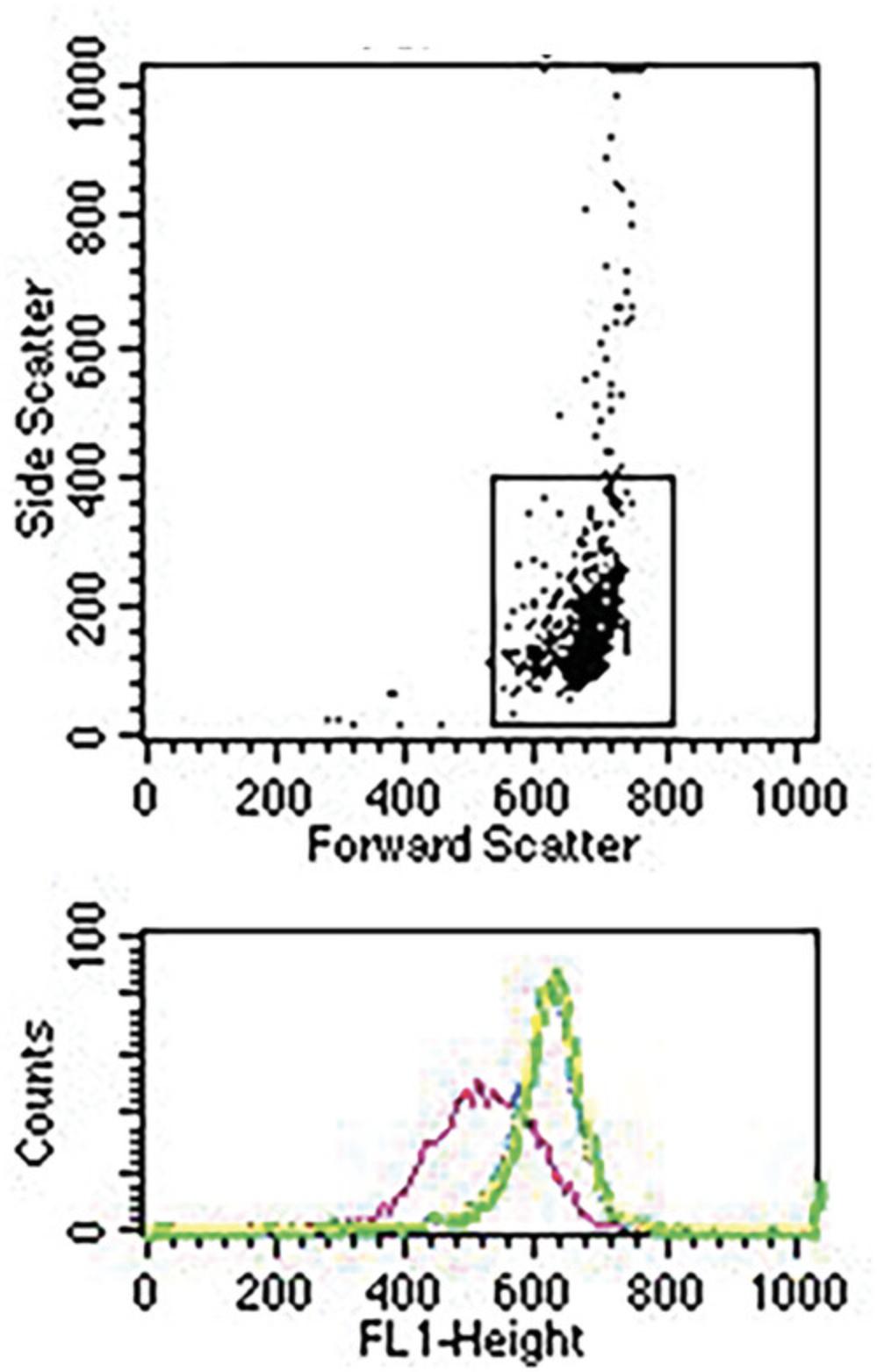
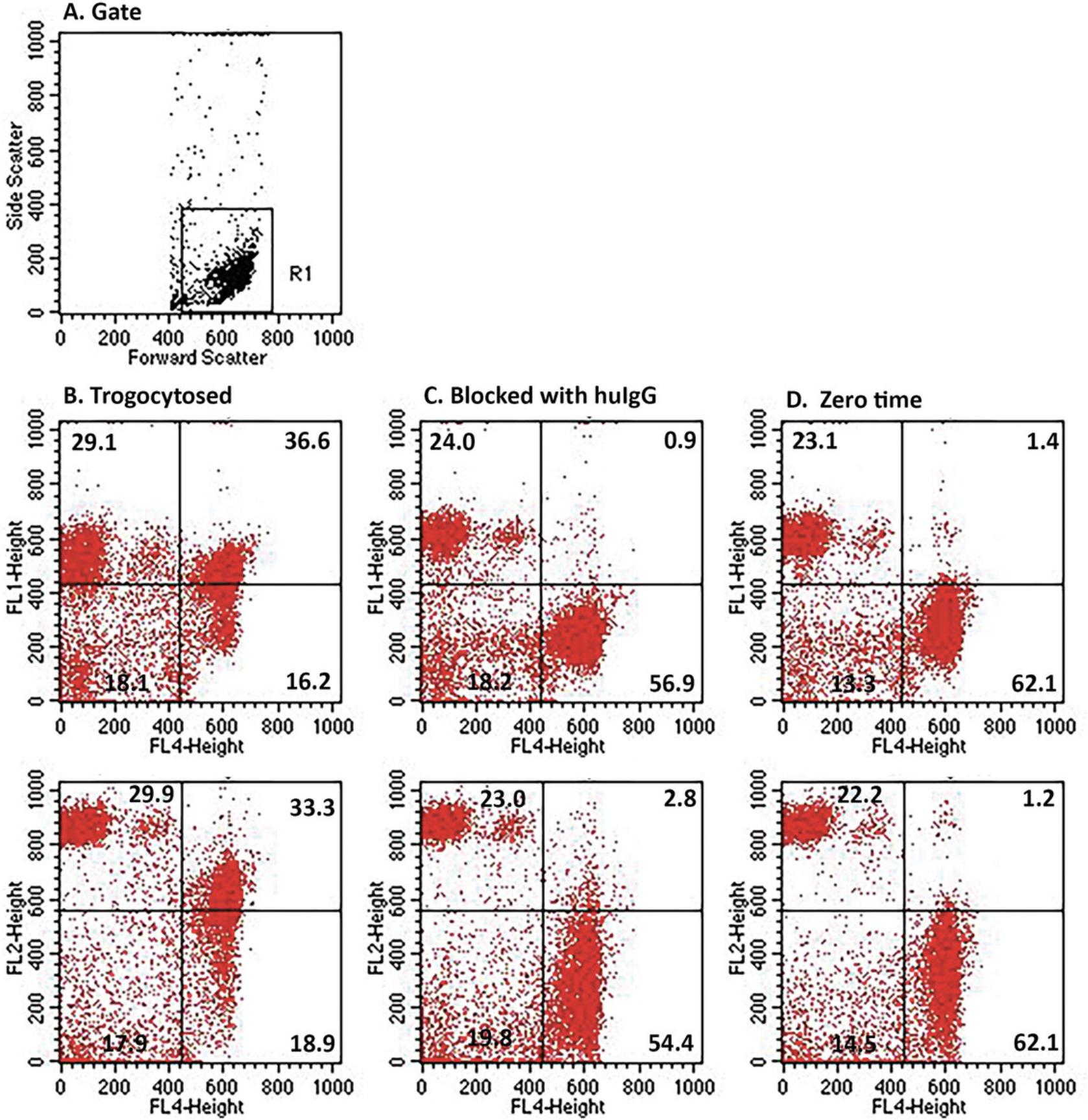
Support Protocol 4: ISOLATION OF HUMAN MONOCYTES
Demonstration of an immunologic phenomenon such as trogocytosis with a cell line should always, if possible, be recapitulated with primary cells. In this case we show that freshly isolated human monocytes are also proficient at mediating trogocytosis.
Additional Materials (also see Basic Protocol 1)
-
Ficoll Paque Plus (Millipore Sigma, cat. no. GE 17-1440-02)
-
Heparinzed green top tubes (Becton Dickinson, cat. no. 368037)
-
50-ml centrifuge tubes (Fisher, cat. no. 06-443-21)
-
Cell scraper (Fisher, cat. no. 08-100-241)
-
70-ml T75 flask (Corning Falcon, cat. no. 353022)
1.Collect 180 ml of whole blood in heparinized (green top) tubes.
2.Mix with an equal volume of PBS and carefully layer multiple 35 ml aliquots of diluted whole blood on 11 ml Ficoll Paque Plus in 50-ml centrifuge tubes.
3.Centrifuge 30 min at 400 × g , no brake, room temperature.
4.Transfer the white PBMC layer to 50-ml tubes, dilute with complete RPMI 1640 medium and perform 2 washes, centrifuging 2 min at 1600 × g , room temperature.
5.Reconstitute pooled cells to 30 ml in complete RPMI 1640 medium and isolate monocytes based on the Current Protocols in Immunology method (Fuss et al., 2009) as follows:
-
Transfer 30 ml of the PBMC suspension to a T75 flask and incubate 1.5 hr at 37°C in 5% CO2.
-
Carefully remove the non-adherent cells by aspiration followed by three gentle washes of the adherent cells with 10 ml each of complete RPMI 1640 medium.
-
Add 10 ml complete RPMI 1640 medium to the flask and use a cell scraper to remove the adhered monocytes.
-
Repeat this step 3 times and consolidate all the washes.
-
Centrifuge 2 min at 1200 ×g, 4°C.
-
Resuspend the pelleted cells in 2 ml complete RPMI 1640 medium.
Basic Protocol 3: TROGOCYTOSIS MEDIATED BY THP-1 CELLS IN SOLUTION
Although it is technically easier to study trogocytosis with adhered acceptor cells, it is important to demonstrate that this is a general phenomenon, which also can occur if cells are in solution, as illustrated in this experiment.
Additional Materials (also see Basic Protocol 1)
- Retinoic acid (see recipe)
- Al488 HB43 (see Support Protocol 1)
- TO-PRO-3 iodide (ThermoFisher, cat. no. T3065)
- 70% ethanol
NOTE : Perform all reactions in FACS tubes.
1.Prepare 1 ml of THP-1 cells (3 × 107 cells/ml in complete RPMI 1640 medium) previously treated for three days with 1 μM retinoic acid (see Support Protocol 5).
2.Label donor cells (e.g., BT-474, SCC-25) with PKH26 (see Support Protocol 3).
3.Opsonize the PKH26-labeled donor cells with cognate unlabeled mAb by combining 400 μl donor cells (2.4 × 106 cells) and 25 μl of 0.1 mg/ml unlabeled cetuximab. Incubate for 15 min at 37°C.
4.Wash the opsonized samples 3 times with BSA-PBS and reconstitute in complete RPMI 1640 medium to starting volume.
5.Initiate trogocytosis by combining 100 μl of opsonized samples with either 100 μl of retinoic acid-treated THP-1 cells or with 100 μl complete RPMI 1640 medium for 60 min at 37°C.
6.After the incubation, add 100 μl of retinoic acid-treated THP-1 cells to the medium-only samples from step 5 and then immediately quench all samples with 4 ml of cold BSA-PBS and pellet all the samples in the standard wash.
7.Reconstitute all samples to 500 μl with PBS. Combine 100 μl of each duplicate sample with 10 μl of 0.1 mg/ml Al488 HB43, specific for human IgG Fc, and incubate for 15 min at room temperature.
8.Meanwhile, add 20 μl of a 1:500 dilution of TO-PRO-3 in PBS to duplicate 100 μl sample aliquots. Incubate at least 5 min at room temperature.
9.Wash all samples twice with PBS, aspirate to leave 250 μl, and then analyze by flow cytometry for FL1/FL2/FL4 (10,000 events per sample) where the level of binding of Al488 HB43 (FL1) reflects how much opsonizing mAb is cell-bound, and/or is lost due to trogocytosis, and the TO-PRO-3 reports on cell viability.
10.Prepare positive controls for killing of cells. React separate 100 μl aliquots of untreated donor cells with 500 μl of 70% ethanol for 15 s. Wash all samples twice with PBS and resuspend to 100 μl with PBS. Probe with 20 μl a 1:500 dilution of TO-PRO-3 and analyze cells for killing, as described above.
Figure 8 shows the results of trogocytosis experiments in which unlabeled CET was used to opsonize cognate SCC-25 donor cells, which were then reacted with retinoic acid-activated THP-1 cells in solution. Analysis of trogocytosis is based on development with Al488 mAb HB43, specific for IgG Fc, which detects CET bound to cells. Based on the MESF values of the reacted (pink) versus the zero-time controls (green) shown in the FL1 histogram, the % trogocytosis was ∼65%. Based on the FL4 analyses of uptake of TO-PRO-3 it is evident that the cells were not killed during the procedures.
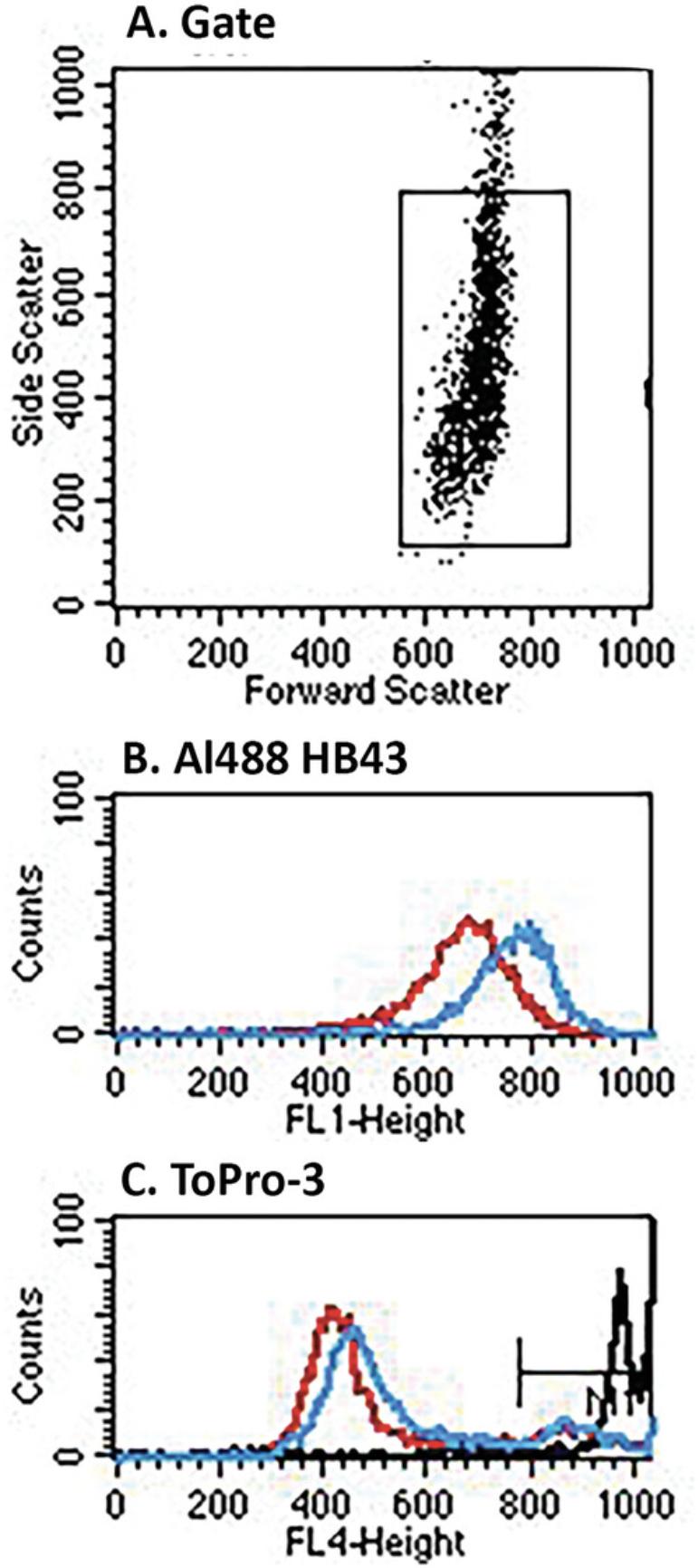
Support Protocol 5: RETINOIC ACID TREATMENT OF THP-1 CELLS
After retinoic acid treatment, THP-1 cells are not adherent, but they are able to promote trogocytosis in solution.
Materials
- See Basic Protocols 1 and 3
1.Culture 50 ml of THP-1 cells to ∼106 cell/ml.
2.Centrifuge the 50-ml culture 10 min at 200 × g , room temperature.
3.Resuspend pelleted cells in 50 ml fresh complete RPMI 1640 medium.
4.Add 50 μl retinoic acid stock solution (1 mM in absolute ethanol) to the resuspended cells. Mix by shaking. Discard the retinoic acid aliquot.
5.Use cells 3 to 5 days later.
Support Protocol 6: CULTURING OF SCC-25, BT-474, MOLT-4 AND THP-1 CELL LINES
All the donor cells and the acceptor THP-1 cells require special and variable culture conditions, as presented below.
Additional Materials (also see Basic Protocol 1)
- Trypsin-EDTA (Fisher Scientific, cat. no. 15400054)
- DMEM/12 medium (see recipe)
- Mercaptoethanol
Cells and specific culture conditions
SCC-25 cells
Grown in DMEM/F12 medium. These cells express high levels of EGFR and are a good model cell line for studies involving erbitux. They grow very rapidly as an attached monolayer. They should be split 1:40 once every week.
BT-474 cells
Grown in complete RPMI 1640 medium. These cells express high levels of Her-2/Neu and are a good model cell line for studies involving herceptin. They grow slowly (∼1/10 as fast as SCC-25) as an attached monolayer. They should be split 1:10 once every two weeks.
SCC-25 and BT-474 cells
Both SSC-25 cells and BT-474 cells are grown as monolayers in T75 flasks and are released as follows: the medium is withdrawn; and the adherent cells are treated with 5 ml trypsin-EDTA for 15 min followed by addition of 10 ml complete RPMI 1640 medium; the released cells are washed and reconstituted in complete RPMI 1640 medium.
MOLT-4 cells
Grown in complete RPMI 1640 medium. MOLT-4 cells grow rapidly in suspension and will attach to plastic to some degree. They should be split at least 1:20 once a week. The cells express CD5 and are useful for studies involving the anti-CD5 mAb T101.
THP-1 cells
Grown in complete RPMI 1640 medium supplemented with 50 μM mercaptoethanol. THP-1 cells are routinely cultured in suspension. This is a monocytic cell line that is differentiated to a macrophage state by retinoic acid or PMA, and under these conditions they are particularly useful for trogocytosis experiments. They grow quickly and should be split 1:10 once a week. THP-1 cells become adherent after 2 to 4 days in culture with 1 μM PMA. Alternatively, THP-1 cells are held in suspension for 2 to 4 days after combination with 1 to 2 μM retinoic acid.
REAGENTS AND SOLUTIONS
Use deionized water to prepare all reagents.
Bicarbonate buffer, 1 M
Dissolve 8.4 g NaHCO3 (Sigma, cat. no. S5761) in 100 ml dH2O. Adjust pH to 9 using NaOH or HCl. Store up to 2 months at 4°C.
Borate saline (BS)
- 0.15 M NaCl (Current Protocols, 2006)
- 0.03 M sodium borate (Sigma-Aldrich, cat. no. SX0355)
- Adjust pH to 7.8 using NaOH or HCl
- Store up to 6 months at 4°C.
BSA-PBS, 10 mg/ml
Dissolve 10 mg/ml bovine serum albumin (BSA) in PBS, pH 7.4 (Current Protocols, 2006). Store up to 2 weeks at 4°C.
Complete RPMI 1640 medium
- RPMI 1640 medium (ATCC, cat. no. 30-2001)
- 10% FBS (ThermoFisher)
- 1% penicillin/streptomycin solution (ThermoFisher) (final concentrations: 100 U/ml penicillin, 100 μg/ml streptomycin)
- Store up to 2 months at 4°C
RPMI 1640 medium from ATCC cat. no. 30-2001 contains the following components: 10 mM HEPES, 1 mM sodium pyruvate, 2 mM l-glutamine, 4.5 g/L glucose, 1.5 g/L sodium bicarbonate.
DMEM/F12 medium
- DMEM/F12 medium (ThermoFisher, cat. no. 11320033)
- 10% FBS (ThermoFisher, cat. no. 4766801)
- 1% penicillin/streptomycin solution (ThermoFisher, cat. no. 15140122) (final concentrations: 100 U/ml penicillin, 100 μg/ml streptomycin)
- 20 mM HEPES (ThermoFisher, cat. no. J63578.AK)
- 500 μg/ml hydrocortisone (ThermoFisher, cat. no. A16292.06)
- Store up to 2 months at 4°C
Phorbol 12-myristate 13-acetate (PMA)
Dissolve 1 mg PMA (Sigma, cat. no. P8139) in 1 ml DMSO (160 μM final concentration). Store single-use aliquots up to 2 years at –70°C. To use, reconstitute THP-1 cells at 1 × 106 cells/ml and add 100 μl/16 ml of cells (final concentration 1 μM).
Retinoic acid, 1 mM
Dissolve 50 mg retinoic acid (Sigma, cat. no. R2625) in 165 ml absolute ethanol. Store in single-use aliquots up to 2 years at –70°C.
COMMENTARY
Background Information
The immune complex clearance reaction
In the period between 1985 and 2007 our laboratory investigated transfer reactions involving a variety of complement-opsonized IgG-containing radio- or fluorescent-labeled immune-complexed substrates bound to: 1) primate erythrocytes via complement receptor 1 (CR1) (Cosio et al., 1987; Craig et al., 2000; Craig et al., 2002; Edberg et al., 1992; Edberg et al., 1987; Edberg et al., 1989; Gyimesi et al., 2004; Kimberly et al., 1989; Kuhn et al., 1998; Nardin et al., 1999; Taylor, Ferguson, et al., 1997; Taylor, Martin, et al., 1997; Taylor et al., 1992; Taylor, Sutherland, et al., 1997); 2) murine B cells or human cell lines via complement receptor 2 (CR2) (Lindorfer et al., 2003; Whipple et al., 2007; Whipple et al., 2004); and 3) platelets in rabbits and mice via the immune adherence complement receptor (Edberg et al., 1989; Taylor et al., 1985, 1986). Immune complexes were transferred to acceptor cells which included monocytes, macrophages and follicular dendritic cells and mouse and human macrophage-like cell lines. Not only were the immune-complexed substrates transferred to the acceptor cells, but almost all the CR1, CR2 and the platelet immune adherence receptors were removed from the respective donor cells. In all cases we found that transfer required recognition of the Fc regions of the immune complexes by Fcγ receptors on the acceptor cells.
We were able to document these reactions in vitro as well as in rabbit and mouse models and in non-human primate models in which mAbs specific for CR1 were used as surrogates for C3b, and the immunological significance of these processes have proven to be considerable. In agreement with the initial reports of Nelson and later Hebert and colleagues (Cornacoff et al., 1983; Hebert & Cosio, 1987; Nelson, 1953, 1955), we found that erythrocyte CR1 can serve as an anchor site to promote the safe and effective binding and subsequent clearance from the circulation of complement-opsonized immune complexes, both soluble and particulate (e.g., bacteria and viruses with bound IgG). The erythrocytes are not cleared and remain in the circulation, but as noted above, during this processing CR1 is removed from the erythrocytes, thereby providing a vital clue for our later mechanistic investigations. We also made use of a mAb specific for CR2, which analogously serves as a surrogate for its ligand C3d, to attach antigens to B cells in bispecific constructs (Whipple et al., 2007). We found that transfer of these prototype immune complexes from B cell CR2 to follicular dendritic cells (FDC) provoked an immune response to the transferred substrate antigens. In this case the transfer reaction was accompanied by substantial loss of CR2 from the B cells. This immunization protocol recapitulates Fearon's use of C3d opsonization to enhance immunity to selected antigens (Dempsey et al., 1996; Fearon & Carroll, 2000).
The detailed mechanisms for these transfer reactions were not altogether clear; our studies suggested that one or more proteases might be involved, but definitive proof for this was never established. It was only after we carefully examined and thoroughly characterized the processing of immune complexes composed of IgG anti-CD20 mAbs RTX or OFA, bound to CD20-positive B cells, that we were able to establish and define a general pattern for all of these reactions: antigens on target cells that are opsonized with IgG are rapidly and efficiently removed from the donor cell by Fcγ-expressing acceptor cells in a process previously defined as trogocytosis by Joly and Hudrisier (2003). Most generally, trogocytosis is a process in which receptors on acceptor cells, specific for cognate plasma-membrane expressed ligands on donor cells, promote formation of immunological synapses with the donor cells which include local merging of the respective plasma membranes of the cells. Then, the cognate ligands, as well as closely associated portions of the plasma membrane of the donor cells, are pinched off and transferred to and internalized by the acceptor cells. Application of this definition to the present case is as follows: immune complexes consisting of multimers of IgG antibodies bound to target antigens on the donor cell are taken up after chelation by Fc receptors on the acceptor cell, and the antigen on the donor cell, still bound to IgG, is transferred to the acceptor cell, along with small portions of the associated plasma membrane of the donor cell (Lindorfer & Taylor, 2022; Taylor & Lindorfer, 2015).
In 1985 Ross and colleagues first reported that CR1 on erythrocytes of patients with systemic lupus erythematosus is reduced considerably compared to its levels in normal individuals (Ross et al., 1985). This phenomenon can clearly be attributed to trogocytosis in which C3b-opsonized IgG containing complexes (most likely antibody/dsDNA immune complexes) are removed from the erythrocytes (along with CR1) and are taken up by Fcγ receptor expressing acceptor cells. It is also noteworthy that in lupus mouse models, the immune adherence receptor on mouse platelets is also reduced (Alexander et al., 2007; Miller et al., 1975), and this observation recapitulates analogous findings for CR1 loss from erythrocytes in SLE.
The methods to measure trogocytosis that are documented in this contribution should provide a template for analyses of other immunological systems that are suspected to be characterized by trogocytosis. For example, there is now substantial evidence that the CD38 mAb daratumumab, used in the treatment of multiple myeloma, mediates trogocytosis of both targeted plasma cells and other CD38 expressing cells (Krejcik et al., 2017). We suggest that as other immunotherapeutic mAbs are developed, these patterns will be repeated. Another particularly relevant observation that is most likely related to trogocytosis concerns the severe side effects associated with the use of antibody-drug conjugates (ADC) in cancer immunotherapy. Several lines of evidence strongly suggest that these agents can induce severe liver toxicity due to uptake of the toxic conjugates by liver sinusoidal endothelial cells that express FcγRIIB (Lindorfer & Taylor, 2022).
Based on these considerations, an important question for research on trogocytosis should focus on developing specific strategies to block this reaction when it negatively impacts on immunotherapies, which we have described for CD20 mAb-directed therapies. In addition to low dose, high frequency RTX/OFA treatments (Wallace et al., 2023; Williams et al., 2006; Zent et al., 2014; Zent et al., 2016), other approaches, including specific blocking of Fcγ receptors on acceptor cells, especially FcγRIIb appear to be particularly promising. We hope that the experimental protocols that we have described herein will be of use in future investigations in this area.
Critical Parameters and Troubleshooting
See Table 1 for a list of common problems, possible causes, and solutions.
Problem | Possible cause | Solution |
---|---|---|
Weak Alexa signal on donor cells | Sub-par labeling of mAb or inadequate opsonization | Re-label mAb or repeat opsonization step |
Very modest trogocytosis; little loss of opsonization mAb from donor cells | Not enough acceptor cells | Increase acceptor/donor cell ratio |
Poor differentiation between PKH26-labeled donor cells and unlabeled acceptor cells | FL2 voltage incorrect | Check voltages and gating scheme with single cell controls (e.g., unlabeled donor cells, PKH26-labeled donor cells, acceptor cells) |
Cell debris in PKH-26 labeled cells | Vortexing or excessive pipetting in labeling step | Cells are very fragile when suspended in Diluent C; resuspend by very gentle pipetting; do not vortex |
FL2 signal of PKH26-labeled cells is too intense | PKH26 input is too high, labeling time too long, and/or failure to stop labeling by addition of FBS | Reduce PKH26 input; stop labeling by addition of FBS |
Understanding Results
The experiments illustrated in Figures 3 and 4 clearly demonstrate that the acceptor cells can remove both the Al488 mAbs as well as PKH26 from the donor cells in a brief period (45 min). The controls reveal that simple dissociation of the mAbs from the donor cells followed by uptake by the acceptor cells does not occur to any measurable extent. The cells must be in contact. It is important to provide an alternative measure of trogocytosis, and this is illustrated in Figure 5, in which substantial amounts of Al488 mAbs are easily demonstrable on the acceptor T101 cells after incubating them with three different types of donor cells opsonized with their cognate mAbs. This experiment also additionally and independently illustrates the general nature of trogocytosis.
The experiments illustrated in Figures 6 and 7 demonstrate that human monocytes are fully capable of promoting trogocytosis of cells opsonized with mAb/donor cell pairs. The gating schemes clearly allow for isolation and examination of both the donor cells and acceptor monocytes. Although the total MESF signal on cells subjected to re-opsonization tends to be modestly greater than after a single opsonization, we do find that the apparent degree of trogocytosis is quite similar for the initial determination and after re-opsonization, thus again validating the trogocytosis paradigm. We also found that under conditions that precluded or should have blocked trogocytosis (zero-time or pre-incubation of acceptor monocytes with human IgG), uptake of the Al488 mAbs or PKH26 by the THP-1 cells was considerably less than under the condition that allowed for trogocytosis.
Time Considerations
Basic Protocol 1 can be completed in 1 day. Basic Protocol 2, that makes use of monocytes as acceptor cells, can be completed in one long day, 9 to 10 hr. Basic Protocol 3 can be completed in 1 day. Alternate Protocol can be completed in 1 day. Support Protocol 1 can be completed in 3 hr. Support Protocol 2 can be completed in 4 hr. Support Protocol 3: opsonization of cells can be completed in 1 hr; PKH26 labeling of cells can be completed in 2 hr. Support Protocol 4 requires 5 hr. Support Protocol 5 is conducted over 3 to 5 days.
Acknowledgments
This review received no external funding. We thank Dr. Paul Beum for his outstanding experimental work.
Author Contributions
Ronald Taylor : Conceptualization; data curation; funding acquisition; resources; supervision; writing—original draft; writing—review and editing. Margaret Lindorfer : Conceptualization; data curation; writing—original draft; writing—review and editing.
Conflict of Interest
The authors declare no conflict of interest.
Open Research
Data Availability Statement
The data, tools, and material (or their source) that support the protocol are available from the corresponding author upon reasonable request.
Literature Cited
- Alexander, J. J., Wang, Y., Chang, A., Jacob, A., Minto, A. W. M., Karmegam, M., Haas, M., & Quigg, R. J. (2007). Mouse podocyte complement factor H: The functional analog to human complement receptor 1. Journal of the American Academy of Nephrology , 18(4), 1157–1166. https://doi.org/10.1681/ASN.2006101125
- Atkinson, J. P., & Frank, M. M. (1974). Studies on the in vivo effects of antibody. Interaction of IgM antibody and complement in the immune clearance and destruction of erythrocytes in man. Journal of Clinical Investigation , 54, 339–348. https://doi.org/10.1172/JCI107769
- Atkinson, J. P., Schreiber, A. D., & Frank, M. M. (1973). Effects of corticosteroids and splenectomy on the immune clearance and destruction of erythrocytes. Journal of Clinical Investigation , 52(6), 1509–1517. https://doi.org/10.1172/JCI107325
- Beum, P. V., Kennedy, A. D., Williams, M. E., Lindorfer, M. A., & Taylor, R. P. (2006). The shaving reaction: Rituximab/CD20 complexes are removed from mantle cell lymphoma and chronic lymphocytic leukemia cells by THP-1 monocytes. Journal of Immunology , 176(4), 2600–2609. https://doi.org/10.4049/jimmunol.176.4.2600
- Beum, P. V., Lindorfer, M. A., & Taylor, R. P. (2008). Within peripheral blood mononuclear cells, antibody-dependent cellular cytotoxicity of rituximab-opsonized Daudi cells is promoted by NK cells and inhibited by monocytes due to shaving. Journal of Immunology , 181(4), 2916–2924. https://doi.org/10.4049/jimmunol.181.4.2916
- Beum, P. V., Mack, D. A., Pawluczkowycz, A. W., Lindorfer, M. A., & Taylor, R. P. (2008). Binding of rituximab, trastuzumab, cetuximab, or mAb T101 to cancer cells promotes trogocytosis mediated by THP-1 cells and monocytes. Journal of Immunology , 181(12), 8120–8132. https://doi.org/10.4049/jimmunol.181.11.8120
- Beum, P. V., Peek, E. M., Lindorfer, M. A., Beurskens, F. J., Engelberts, P. J., Parren, W. H. I., van de Winkel, J. G. J., & Taylor, R. P. (2011). Loss of CD20 and bound CD20 antibody from opsonized B cells occurs more rapidly because of trogocytosis mediated by Fc receptor-expressing effector cells than direct internalization by the B cells. Journal of Immunology , 187(6), 3438–3447. https://doi.org/10.4049/jimmunol.1101189
- Beurskens, F. J., Lindorfer, M. A., Farooqui, M., Beum, P. V., Engelberts, P., Mackus, W. J., Parren, P. W. H. I., Wiestner, A., & Taylor, R. P. (2012). Exhaustion of cytotoxic effector systems may limit monoclonal antibody-based immunotherapy in cancer patients. Journal of Immunology , 188(7), 3532–3541. https://doi.org/10.4049/jimmunol.11036938845
- Cornacoff, J. B., Hebert, L. A., Smead, W. L., van Aman, M. E., Birmingham, D. J., & Waxman, F. J. (1983). Primate erythrocyte-immune complex-clearing mechanism. Journal of Clinical Investigation , 71, 236–247. https://doi.org/10.1172/JCI110764
- Cosio, F. G., Hebert, L. A., Birmingham, D. J., Dorval, B. L., Bakaletz, A. P., Kujala, G. A., Edberg, J. C., & Taylor, R. P. (1987). Clearance of human antibody/DNA immune complexes and free DNA from the circulation of the nonhuman primate. Clinical Immunology and Immunopathology , 42(1), 1–9. https://doi.org/10.1016/0090-1229(87)90167-X
- Craig, M. L., Bankovich, A. J., McElhenney, J., & Taylor, R. P. (2000). Clearance of anti-double-stranded DNA antibodies: The natural immune complex clearance mechanism. Arthritis and Rheumatism , 43, 2265–2275. https://doi.org/10.1002/1529-0131(200010)43:10%3c2265::AID-ANR14%3e3.0.CO;2-J
- Craig, M. L., Bankovich, A. J., & Taylor, R. P. (2002). Visualization of the transfer reaction: Tracking immune complexes from erythrocyte complement receptor 1 to macrophages. Clinical Immunology , 105, 36–47. https://doi.org/10.1006/clim.2002.5266
- Current Protocols. (2006). Commonly Used Reagents. Current Protocols in Microbiology , 00, A.2A.1–A.2A.15. https://doi.org/10.1002/9780471729259.mca02as00
- Dempsey, P. W., Allison, M. E., Akkaraju, S., Goodnow, C. C., & Fearon, D. T. (1996). C3d of complement as a molecular adjuvant: Bridging innate and acquired immunity. Science , 271, 348–350. https://doi.org/10.1126/science.271.5247.348
- Edberg, J. C., Kimberly, R. P., & Taylor, R. P. (1992). Functional characterization of non-human primate erythrocyte immune adherence receptors: Implications for the uptake of immune complexes by the cells of the mononuclear phagocytic system. European Journal of Immunology , 22(6), 1333–1339. https://doi.org/10.1002/eji.1830220602
- Edberg, J. C., Kujala, G. A., & Taylor, R. P. (1987). Clearance kinetics and immunochemistry in rabbits of soluble antibody/DNA immune complexes. Effects of antibody class and DNA conformation. Journal of Immunology , 139, 180–187. https://doi.org/10.4049/jimmunol.139.1.180
- Edberg, J. C., Tosic, L., & Taylor, R. P. (1989). Immune adherence and the processing of soluble complement-fixing antibody/DNA immune complexes in mice. Clinical Immunology and Immunopathology , 51, 118–132. https://doi.org/10.1016/0090-1229(89)90212-2
- Fearon, D. T., & Carroll, M. C. (2000). Regulation of B lymphocyte responses to foreign and self-antigens by the CD19/CD21 complex. Annual Review of Immunology , 18, 393–422. https://doi.org/10.1146/annurev.immunol.18.1.393
- Fuss, I. J., Kanof, M. E., Smith, P. S., & Zola, H. (2009). Isolation of whole mononuclear cells from peripheral blood and cord blood. Current Protocols in Immunology , 85, 7.1.7–7.1.8. https://doi.org/10.1002/0471142735.im701s858892
- Gyimesi, E., Bankovich, A. J., Schuman, T. A., Goldberg, J. B., Lindorfer, M. A., & Taylor, R. P. (2004). Staphylococcus aureus bound to complement receptor 1 on human erythrocytes by bispecific monoclonal antibodies is phagocytosed by acceptor macrophages. Immunology Letters , 95(2), 185–192. https://doi.org/10.1016/j.imlet.2004.07.007
- Hebert, L. A., & Cosio, F. G. (1987). The erythrocyte-immune complex-glomerulonephritis connection in man. Kidney International , 31, 877–885. https://doi.org/10.1038/ki.1987.81
- Joly, E., & Hudrisier, D. (2003). What is trogocytosis and what is its purpose? Nature Immunology , 4(9), 815. https://doi.org/10.1038/ni0903-815
- Kennedy, A. D., Beum, P. V., Solga, M. D., DiLillo, D. J., Lindorfer, M. A., Hess, C. E., Densmore, J. J., Williams, M. E., & Taylor, R. P. (2004). Rituximab infusion promotes rapid complement depletion and acute CD20 loss in chronic lymphocytic leukemia. Journal of Immunology , 172(5), 3280–3288. https://doi.org/10.4049/jimmunol.172.5.3280
- Kimberly, R. P., Edberg, J. C., Merriam, L. T., Clarkson, S. B., Unkeless, J. C., & Taylor, R. P. (1989). In vivo handling of soluble complement fixing Ab/dsDNA immune complexes in chimpanzees. Journal of Clinical Investigation , 84(3), 962–970. https://doi.org/10.1172/JCI114259
- Krejcik, J., Frerichs, K. A., Nijhof, I. S., van Kessel, B., van Velzen, J. F., Bloem, A. C., Broekmans, M. E. C., Zweegman, S., van Meerloo, J., Musters, R. J. P., Poddighe, P. J., Groen, R. W. J., Chiu, C., Plesner, T., Lokhorst, H. M., Sasser, A. K., Mutis, T., & van de Donk, N. W. C. J. (2017). Monocytes and granulocytes reduce CD38 expression levels on myeloma cells in patients treated with daratumumab. Clinical Cancer Research , 23(24), 7498–7511. https://doi.org/10.1158/1078-0432.CCR-17-20273583
- Kuhn, S. E., Nardin, A., Klebba, P. E., & Taylor, R. P. (1998). Escherichia coli bound to the primate erythrocyte complement receptor via bispecific monoclonal antibodies are transferred to and phagocytosed by human monocytes in an in vitro model. Journal of Immunology , 160(10), 5088–5097. https://doi.org/10.4049/jimmunol.160.10.5088
- Lindorfer, M. A., Jinivizian, H. B., Foley, P. L., Kennedy, A. D., Solga, M. D., & Taylor, R. P. (2003). B cell complement receptor 2 transfer reaction. Journal of Immunology , 170(7), 3671–3678. https://doi.org/10.4049/jimmunol.170.7.3671
- Lindorfer, M. A., & Taylor, R. P. (2022). FcγR-mediated trogocytosis 2.0: Revisiting history gives rise to a unifying hypothesis. Antibodies , 11(3). https://doi.org/10.3390/antib110310458474
- Miller, G. W., Steinberg, A. D., Green, I., & Nussenzweig, V. (1975). Complement-dependent alterations in the handling of immune complexes by NZB/W mice. Journal of Immunology , 114(4), 1166–1170. https://doi.org/10.4049/jimmunol.114.4.1166
- Nardin, A., Schlimgen, R., Holers, V. M., & Taylor, R. P. (1999). A prototype pathogen bound ex vivo to human erythrocyte complement receptor 1 via bispecific monoclonal antibody complexes is cleared to the liver in a mouse model. European Journal of Immunology , 29(5), 1581–1586. https://doi.org/10.1002/(SICI)1521-4141(199905)29:05%3c1581::AID-IMMU1581%3e3.0.CO;2-V
- Nelson, R. A. (1953). The immune-adherence phenomenon. An immunologically specific reaction between microorganisms and erythrocytes leading to enhanced phagocytosis. Science , 118(3077), 733–737. https://doi.org/10.1126/science.118.3077.733
- Nelson, R. A. (1955). The immune-adherence phenomenon. A hypothetical role of erythrocytes in defense against bacteria and viruses. Proceedings of the Royal Society of Medicine , 49, 55–58. https://doi.org/10.1177/003591575604900122
- Pavlasova, G., Borsky, M., Seda, V., Cerna, K., Osickova, J., Doubek, M., Mayer, J., Calogero, R., Trbusek, M., Pospisilova, S., Davids, M. S., Kipps, T. J., Brown, J. R., & Mraz, M. (2016). Ibrutinib inhibits CD20 upregulation on CLL B cells mediated by the CXCR4/SDF-1 axis. Blood , 128, 1609–1613. https://doi.org/10.1182/blood-2016-04-7095198891
- Ross, G. D., Yount, W. J., Walport, M. J., Winfield, J. B., Parker, C. J., Fuller, C. R., Taylor, R. P., Myones, B. L., & Lachmann, P. J. (1985). Disease-associated loss of erythrocyte complement receptors (CR1, C3b receptors) in patients with systemic lupus erythematosus and other diseases involving autoantibodies and/or complement activation. Journal of Immunology , 135(3), 2005–2014. https://doi.org/10.4049/jimmunol.135.3.2005
- Schreiber, A. D., & Frank, M. M. (1972a). Role of antibody and complement in the immune clearance and destruction of erythrocytes. II. Molecular nature of IgG and IgM complement-fixing sites and effects of their interaction with serum. Journal of Clinical Investigation , 51, 583–589.5920. https://doi.org/10.1172/JCI106847
- Schreiber, A. D., & Frank, M. M. (1972b). Role of antibody and complement in the immune clearance and destruction of erythrocytes: I. In vivo effects of IgG and IgM complement fixing sites. Journal of Clinical Investigation , 51(3), 575–582.5919. https://doi.org/10.1172/JCI106846
- Taylor, R. P., Ferguson, P. J., Martin, E. N., Cooke, J., Greene, K. L., Grinspun, K., Guttman, M., & Kuhn, S. (1997). Immune complexes bound to the primate erythrocyte complement receptor (CR1) via anti-CR1 mAbs are cleared simultaneously with loss of CR1 in a concerted reaction in a rhesus monkey model. Clinical Immunology and Immunopathology , 82(1), 49–59.6589. https://doi.org/10.1006/clin.1996.4286
- Taylor, R. P., Kujala, G., Edberg, J. C., Foreman, P., Davis, J. S., & Wright, E. (1986). The kinetics of clearance of human antibody/dsDNA immune complexes from rabbit circulation. Effects of dsDNA size, rheumatoid factor, and reduction and alkylation of the antibodies. Journal of Immunology , 136(10), 3785–3792.6580. https://doi.org/10.4049/jimmunol.136.10.3785
- Taylor, R. P., Kujala, G., Wilson, K., Wright, E., & Harbin, A. (1985). In vivo and in vitro studies of the binding of antibody/dsDNA immune complexes to rabbit and guinea pig platelets. Journal of Immunology , 134(4), 2550–2558.6578. https://doi.org/10.4049/jimmunol.134.4.2550
- Taylor, R. P., & Lindorfer, M. A. (2015). FcγR-mediated trogocytosis impacts mAb-based therapies: Historical precedence and recent developments. Blood , 125(5), 762–766.6610. https://doi.org/10.1182/blood-2014-10-569244
- Taylor, R. P., Martin, E. N., Reinagel, M. L., Nardin, A., Craig, M., Choice, Q., Schlimgen, R., Greenbaum, S., Incardona, N. L., & Ochs, H. D. (1997). Bispecific monoclonal antibody complexes facilitate erythrocyte binding and liver clearance of a prototype particulate pathogen in a monkey model. Journal of Immunology , 159, 4035–4044.6588. https://doi.org/10.4049/jimmunol.159.8.4035
- Taylor, R. P., Reist, C. J., Sutherland, W. M., Otto, A., Labuguen, R. H., & Wright, E. L. (1992). In vivo binding and clearance of circulating antigen by bispecific heteropolymer-mediated binding to primate erythrocyte complement receptor. Journal of Immunology , 148(8), 2462–2468.8888. https://doi.org/10.4049/jimmunol.148.8.2462
- Taylor, R. P., Sutherland, W. M., Martin, E. N., Ferguson, P. J., Reinagel, M. L., Gilbert, E., Lopez, K., Incardona, N. L., & Ochs, H. D. (1997). Bispecific monoclonal antibody complexes bound to primate erythrocyte CR1 facilitate virus clearance in a monkey model. Journal of Immunology , 158(2), 842–850.6590. https://doi.org/10.4049/jimmunol.158.2.842
- Wallace, D. S., Zent, C. S., Baran, A. M., Reagan, P. M., Casulo, C., Rice, G., Friedberg, J. W., & Barr, P. M. (2023). Acalabrutinib and high-frequency low-dose subcutaneous rituximab for initial therapy of chronic lymphocytic leukemia. Blood Advances , 7(11), 2496–2503. https://doi.org/10.1182/bloodadvances.20220093828893
- Whipple, E. C., Ditto, A. H., Shanahan, R. S., Gatesman, J. J., Little, S. F., Taylor, R. P., & Lindorfer, M. A. (2007). Low doses of antigen coupled to anti-CR2 mAbs induce rapid and enduring IgG immune responses in mice and in cynomolgus monkeys. Molecular Immunology , 44(4), 377–388.8855. https://doi.org/10.1016/j.molimm.2006.02.032
- Whipple, E. C., Shanahan, R. S., Ditto, A. H., Taylor, R. P., & Lindorfer, M. A. (2004). Analyses of the in vivo trafficking of stoichiometric doses of an anti-complement receptor 1/2 monoclonal antibody infused intravenously in mice. Journal of Immunology , 173(4), 2297–2306. https://doi.org/10.4049/jimmunol.173.4.22978696
- Williams, M. E., Densmore, J. J., Pawluczkowycz, A. W., Beum, P. V., Kennedy, A. D., Lindorfer, M. A., Hamil, S. H., Eggleton, J. C., & Taylor, R. P. (2006). Thrice-weekly low-dose rituximab decreases CD20 loss via shaving and promotes enhanced targeting in chronic lymphocytic leukemia. Journal of Immunology , 177(10), 7435–7443.8856. https://doi.org/10.4049/jimmunol.177.10.7435
- Zent, C. S., Taylor, R. P., Lindorfer, M. A., Beum, P. V., LaPlant, B., Wu, W., Call, T. G., Bowen, D. A., Conte, M. J., Frederick, L. A., Link, B. K., Blackwell, S. E., Veeramani, S., Baig, N. A., Viswanatha, D. S., Weiner, G. J., & Witzig, T. E. (2014). Chemoimmunotherapy for relapsed/refractory and progressive 17p13 deleted chronic lymphocytic leukemia (CLL) combining pentostatin, alemtuzumab, and low dose rituximab is effective and tolerable and limits loss of CD20 expression by circulating CLL cells. American Journal of Hematology , 89(7), 757–765. https://doi.org/10.1002/ajh.237377527
- Zent, C. S., Wang, X. V., Ketterling, R. P., Hanson, C. A., Libby, E. N., Barrientos, J. C., Call, T. G., Chang, J. E., Liu, J. J., Calvo, A. R., Lazarus, H. M., Rowe, J. M., Luger, S. M., Litzow, M. R., & Tallman, M. S. (2016). A Phase II randomized trial comparing standard and low dose rituximab combined with alemtuzumab as initial treatment of progressive chronic lymphocytic leukemia in older patients: A trial of the ECOG-ACRIN Cancer Research Group (E1908). American Journal of Hematology , 91, 308–312. https://doi.org/10.1002/ajh.242657528