Intelligent Characterization of Lentil Genetic Resources: Evolutionary History, Genetic Diversity of Germplasm, and the Need for Well-Represented Collections
Elena Bitocchi, Elena Bitocchi, Roberto Papa, Roberto Papa, Tania Gioia, Tania Gioia, Azalea Guerra-García, Azalea Guerra-García, Eric von Wettberg, Eric von Wettberg, Giuseppina Logozzo, Giuseppina Logozzo, Kirstin E Bett, Kirstin E Bett
Abstract
The genetic and phenotypic characterization of crops allows us to elucidate their evolutionary and domestication history, the genetic basis of important traits, and the use of variation present in landraces and wild relatives to enhance resilience. In this context, we aim to provide an overview of the main genetic resources developed for lentil and their main outcomes, and to suggest protocols for continued work on this important crop. Lens culinaris is the third-most-important cool-season grain and its use is increasing as a quick-cooking, nutritious, plant-based source of protein. L. culinaris was domesticated in the Fertile Crescent, and six additional wild taxa (L. orientalis , L. tomentosus, L. odemensis, L. lamottei , L. ervoides , and L. nigricans) are recognized. Numerous genetic diversity studies have shown that wild relatives present high levels of genetic variation and provide a reservoir of alleles that can be used for breeding programs. Furthermore, the integration of genetics/genomics and breeding techniques has resulted in identification of quantitative trait loci and genes related to attributes of interest. Genetic maps, massive genotyping, marker-assisted selection, and genomic selection are some of the genetic resources generated and applied in lentil. In addition, despite its size (∼4 Gbp) and complexity, the L. culinaris genome has been assembled, allowing a deeper understanding of its architecture. Still, major knowledge gaps exist in lentil, and a deeper understanding and characterization of germplasm resources, including wild relatives, is critical to lentil breeding and improvement. © 2021 The Authors. Current Protocols published by Wiley Periodicals LLC.
Basic Protocol 1 : Recording of lentil seed descriptors
Basic Protocol 2 : Lentil seed imaging
Basic Protocol 3 : Lentil seed increase
Basic Protocol 4 : Recording of primary lentil seed INCREASE descriptors
INTRODUCTION
Origin, Domestication, and Diffusion Out of the Center of Origin
Lentil (Lens culinaris Medik.) is an annual, herbaceous, self-pollinated grain legume crop that is increasingly used as a source of dietary protein, particularly for people with plant-based diets. Lentil is the third-most-important cool-season grain legume after chickpea and pea (FAOSTAT, http://www.fao.org/faostat/en/#data/QC). In 2019, lentil production was 5.9 M tonnes from 4.9 Mha across more than 50 countries, with Canada as the largest producer (2.2 M tonnes) followed by India (1.2 M tonnes) (FAOSTAT, http://www.fao.org/faostat/en/#data/QC). Like other legume crops, lentil establishes symbiotic relationships with nitrogen-fixing bacteria, reducing the use of fertilizers and enhancing the productivity of non-legume rotational crops. Therefore, lentils and other legume crops are an important component of farming systems, providing environmental and ecological benefits to the whole system, and are relevant for climate change mitigation (Marques, Kur, Bueno, & Wettberg, 2020; Young, Mudge, & Ellis, 2003).
The genus Lens Miller (2n = 2× = 14) is a member of the Fabaceae family and its taxonomy has gone through several modifications. Although five species were initially proposed, seven taxa can alternatively be recognized: L. culinaris (the domesticated lentil), L. orientalis , L. tomentosus , L. lamottei , L. odemensis , L. ervoides , and L. nigricans (Fig. 1A; Dissanayake, Braich, Cogan, Smith, & Kaur, 2020; Ogutcen, Ramsay, von Wettberg, & Bett, 2018; Wong et al., 2015). Despite taxonomic reorganizations, studies generally agree that L. orientalis is the most closely related wild progenitor of L. culinaris and that L. nigricans is the most distant relative (Fig. 1A; Cubero, 1981; Dissanayake et al., 2020; Ferguson, Maxted, Van Slageren, & Robertson, 2000; Ogutcen et al., 2018; Wong et al., 2015).
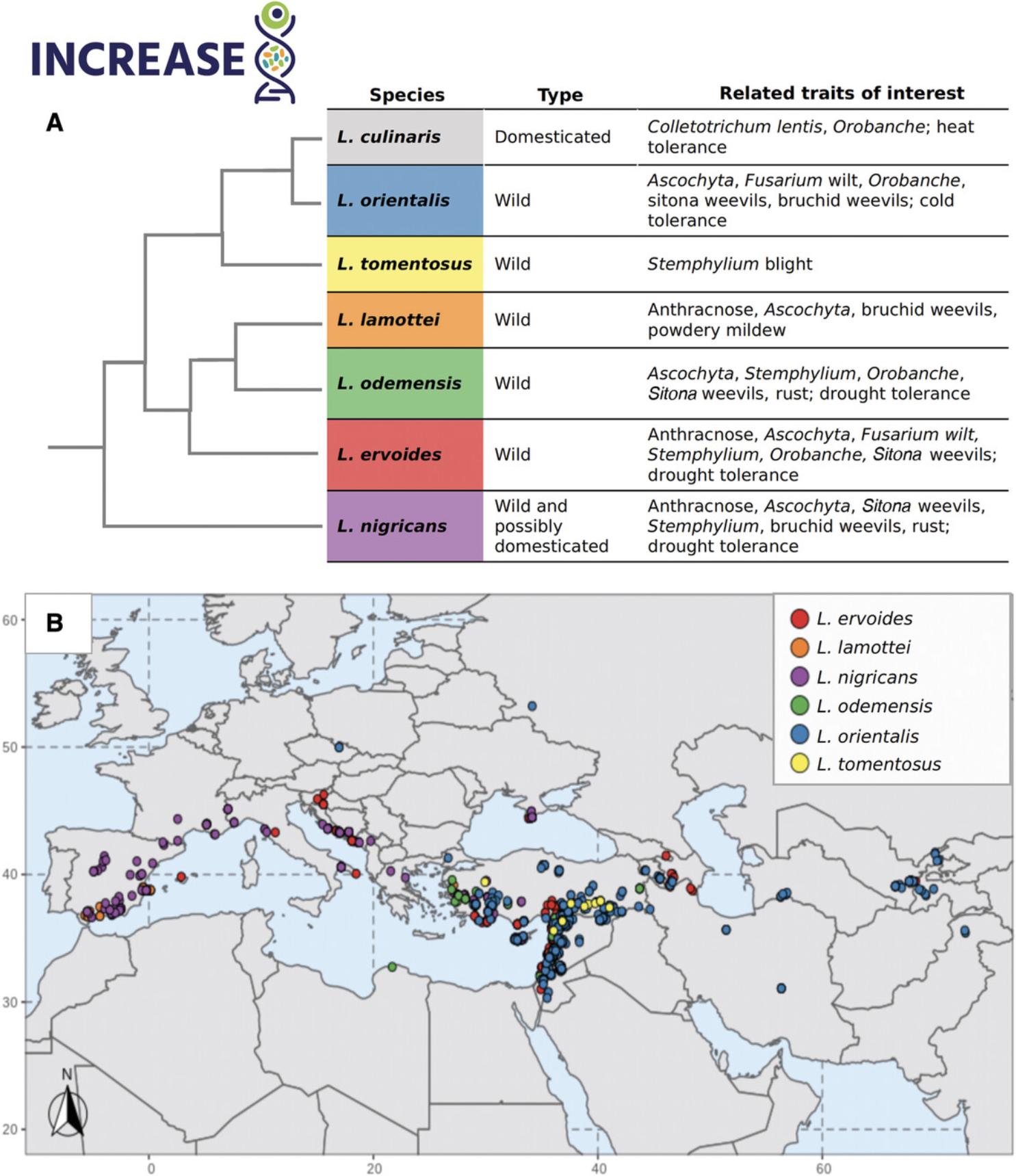
Wild lentil taxa are widely distributed in the Mediterranean region, with overlapping distributions in the Aegean area and southwestern Turkey (Fig. 1B; Ferguson, Roberston, et al. 1998). Most wild lentil species are found in Turkey, but their populations and their potential as a source of variation for cultivated lentil are threatened due to habitat loss (Singh et al., 2018).
There are different crossability levels within Lens species, and the fertility of hybrids depends on the compatibility of chromosomal arrangements of the wild parent. L. culinaris crosses easily with its closest relative L. orientalis , whereas successful crossing with L. odemensis depends on the parents involved (Singh et al., 2013). Successful crosses between L. culinaris and L. ervoides have been achieved using embryo rescue or by applying postfertilization growth hormones and embryo/ovule rescue techniques (Abbo & Ladizinsky, 1991; Ahmad, Fautrier, McNeil, Burritt, & Hill, 1995; Fiala, Tullu, Banniza, Séguin-Swartz, & Vandenberg, 2009; Fratini & Ruiz, 2006; Ladizinsky, Cohen, & Muehlbauer, 1985; Vail, Strelioff, Tullu, & Vandenberg, 2012). The exception is L. nigricans , for which no successful hybrids have been reported (Abbo & Ladizinsky, 1991; Ahmad et al., 1995; Ladizinsky, 1993).
Lentil appears early in archaeological records in the Fertile Crescent, suggesting it was among the first cultivated crops (Fuller, 2007; Ladizinsky, 1993; Ladizinsky, Braun, & Muehlbauer, 1983; Sonnante, Hammer, & Pignone, 2009). Genetic evidence also indicates southern Turkey as the area of origin of cultivated lentil (Alo, Furman, Akhunov, Dvorak, & Gepts, 2011) around 7000 B.C. From there it moved outwards in all directions during the Neolithic period (Cubero, 1984; Sonnante, Montemurro, et al., 2009). In archaeological remains from the Neolithic to the Bronze age, lentil seeds are small and nearly impossible to distinguish from wild lentils (Fuller, 2007). Ancient use of lentils mixed with barley and historical accounts of lentil cultivation suggest that lentil cultivation by the Bronze age had spread widely from the Fertile Crescent and was a key component of ancient crop rotations with cereals.
Early studies in the first half of the 20th century proposed that lentil was divided into two major types during the spread phase: microsperma (characterized by strongly pigmented flowers with almost spherical seeds, red and yellow cotyledons, seed diameter of 2-6 mm, distributed in South Asia) and macrosperma (lighter pigmented flowers with flattened seeds, yellow cotyledons, seed diameter of 6-9 mm, distributed in western Asia, Europe, and more recently North and South America) (Barulina, 1930; Cubero, 1984). However, Ferguson, Ford-Lloyd, et al. (1998) have reported that microsperma and macrosperma are not strictly associated with geography.
There has been debate about a second potential domestication event in the genus Lens , with some populations of L. nigricans occurring in disturbed human-associated sites in southern Europe. Ladizinsky and colleagues have noted that L. nigricans occurs in two distinct habitats: (1) rocky undisturbed fields with annual short-statured vegetation and (2) old vineyards and olive orchards. In the second habitat, seeds tend to be larger and have some cytological distinctions, such as reciprocal translocations and chromosomal rearrangements (Ladizinsky et al., 1983). Despite these intriguing observations, these populations have not been shown to lack seed dormancy or have indehiscent pods, two of the canonical traits expected of domesticated legumes (e.g., Hammer, 1984).
Genetic diversity of the genus Lens has been studied using different molecular marker types (see Supporting Information, Table S1). Most of these studies, however, were conducted on a relatively small scale and focused mostly on cultivated lentil. One of the first large-scale genetic studies in cultivated and wild lentils was performed by Alo et al. (2011), and it supported the hypothesis of southern Turkey as the center of origin for cultivated lentil and presented evidence that domestication led to a loss of ∼40% of the genetic diversity. Khazaei et al. (2016), examining accessions from 54 countries, identified three major cultivated lentil groups that reflected the world's agro-ecological zones: South Asia (sub-tropical savannah), Mediterranean, and northern temperate. Furthermore, Pavan et al. (2019) detected a correlation between genetic clustering, geography, and phenotypic traits (seed size and early flowering) in Mediterranean germplasm. Results of Khazaei et al. (2016) and Pavan et al. (2019) suggest that both natural and artificial selection have clearly shaped lentil diversity.
More recently, assessment of genetic diversity among 467 wild and cultivated lentil accessions using 420,000 SNPs from gene transcripts confirmed the phylogenetic relationships of Lens species, but unlike previous studies found only a weak correlation between geographical origin and genetic relationships (Dissanayake et al., 2020).
Worldwide Germplasm Collections
At the beginning of the last century, agronomists and geneticists recognized the need and importance of preserving and characterizing the genetic diversity of cultivated plants. The Russian scientist N. I. Vavilov, who led a worldwide collection of crops in the Soviet Union (Janick, 2015), included lentil in his collection. His second wife, E. I. Barulina, was the leading Soviet expert on lentils (Barulina, 1930; Vishnyakova, 2006). Since Vavilov, numerous germplasm banks have been created around the world, but wild accessions and landraces have tended to be abandoned without concern for their genetic value (Coyne et al., 2020).
During the domestication process and subsequent spreading and breeding, genetic bottlenecks occur, leading to a loss of genetic diversity. Crop wild relatives present wider genetic variation since they have longer evolutionary histories across diverse environments (Maxted et al., 2015; Meyer & Purugganan, 2013; Piperno, 2017; Ripple, Wolf, Newsome, Barnard, & Moomaw, 2019). Wild accessions and landraces are a source of diversity, and using a diverse gene pool for lentil breeding is important to reduce genetic erosion and enhance the resilience of future varieties. For example, several studies have reported traits of interest related to biotic and abiotic stress responses in wild lentil species (Fig. 1A; Supporting Information, Table S2; Coyne et al., 2020).
The world's largest lentil collection is in the ICARDA genebank, which conserves 14,597 Lens accessions, including 2580 breeding lines, 11,405 landraces, and 612 wild accessions (www.genebanks.org/genebanks/icarda; retrieved July 29, 2020). Coyne et al. (2020) noted that there are major germplasm gaps at the species and genotype levels, and a continuum is required to fill these gaps in the wild gene pool from unrepresented areas of diversity. Some other larger lentil germplasm collections are held by the European Cooperative Programme for Plant Genetic Resources (4598 acc., www.ecpgr.cgiar.org), the National Bureau of Plant Genetic Resources, India (7712 acc., www.nbpgr.ernet.in), the Australian Grains Genebank (5254 acc., https://grdc.com.au), the USDA Agricultural Research Service, USA (3187 acc., www.ars.usda.gov), the Vavilov Institute, Russia (2556 acc., www.vir.nw.ru), and Plant Gene Resources of Canada (1150 acc., https://pgrc.agr.cg.ca).
Around 76% of ICARDA lentil accessions have been characterized for morphological and phenological attributes (www.genesys-pgr.org; Kumar, Rajendran, Kumar, Hamwieh, & Baum, 2016; Kumari et al., 2018; Sharma et al., 2018; Sita et al., 2017). Under the Canadian-led project AGILE, a lentil diversity panel (LDP) consisting of 324 accessions was phenotyped for phenological attributes in nine different locations around the world for two seasons (Wright et al., 2020). This set has also been genotyped using an exome capture array (Ogutcen et al., 2018), and seeds from 321 lines have been deposited in the ICARDA genebank. This well-characterized set, along with the mini-core and FIGS sets from ICARDA and the INCREASE collections described below, will be useful for further studies into trait diversity.
Despite the presence of yield traits and resistance to biotic and abiotic stresses in wild lentil germplasm (Fig. 1A; Supporting Information, Table S2), only 4% of ICARDA accessions correspond to wild relatives (www.genesys-pgr.org). There is still a need for a deeper understanding and characterization of the genetic and phenotypic diversity in wild lentils in order to be able to use that variation (Singh et al., 2018). To understand crop diversification and adaptation, studies should be conducted in the geographic centers of origin, where a greater amount of diversity is found (Chen, Shapiro, Benrey, & Cibrián-Jaramillo, 2017).
Genomics of Genetic Resources (Whole Genome, Genome Scan, Genomic Diversity)
Massive sequencing technologies have led to the routine use of thousands or even millions of molecular markers. These tools can be used by breeders to study the genetic composition of crop varieties, the evolutionary relationships between cultivars and wild relatives, and the basis for modeling complex genotype-phenotype relationships (Cobb, DeClerck, Greenberg, Clark, & McCouch, 2013; Varshney, Terauchi, & McCouch, 2014). New genetic resources have been generated and accumulated in the last few years.
Genetic Maps, Quantitative Trait Loci, and Association Mapping
The availability of DNA-based molecular markers has facilitated the generation of genetic maps, which can be used to locate markers associated with target genes. The construction of the first linkage maps for lentil started using isozymes and morphological traits (Havey & Muehlbauer, 1989; Tahir & Muehlbauer, 1994; Vaillancourt & Slinkard, 1993; Zamir & Ladizinsky, 1984), followed by PCR-based markers (Eujayl, Baum, Powell, Erskine, & Pehu, 1998; Gupta, Verma, et al., 2012; Hamwieh et al., 2005; Phan et al., 2007; Rubeena, Ford, & Taylor, 2003; Saha, Sarker, Chen, Vandemark, & Muehlbauer, 2010b; Tullu, Tar'an, Warkentin, & Vandenberg, 2008; Verma et al., 2015), which have been replaced by SNPs (Ates et al., 2018; Fedoruk, Vandenberg, & Bett, 2013; Gujaria-Verma et al., 2014; Polanco et al., 2019). Interspecific mapping populations using wild and cultivated parents (L. culinaris × L. orientalis, L. culinaris × L. ervoides , and L. culinaris × L. odemensis) have also been used to generate genetic maps (Durán, Fratini, García, & de la Vega, 2004; Eujayl et al., 1998; Gujaria-Verma et al., 2014; Gupta, Verma, et al., 2012; Polanco et al., 2019).
Several genes and quantitative trait loci (QTLs) related to complex attributes have been mapped, such as resistance to Fusarium wilt (Eujayl et al., 1998; Hamwieh et al., 2005), anthracnose (Tullu, Buchwaldt, Warkentin, Taran, & Vandenberg, 2003), and Ascochyta blight (Chowdhury, Andrahennadi, Slinkard, & Vandenberg, 2001; Ford, Pang, & Taylor, 1999; Rodda et al., 2017; Sudheesh, Rodda, et al., 2016; Taylor, Ades, & Ford, 2006); height of first ramification, flowering time, plant height, pod dehiscence, and seed diameter (Durán et al., 2004); flowering time and seed size and shape (Fedoruk et al., 2013); winter survival and tolerance to winter injury (Kahraman et al., 2004); and others traits (Ates et al., 2016, 2018; Gupta, Taylor, et al., 2012; Kaur et al., 2014; Saha, Sarker, Chen, Vandemark, & Muehlbauer, 2010a,b; Tar'an et al., 2003).
More recently, marker-trait association or association mapping (AM) studies for quantitative traits have been used increasingly to study complex genetic attributes, although few AM studies have been reported in lentil. Khazaei et al. (2017) were the first to apply this approach using accessions with a wide range of variation for zinc and iron concentration in seeds, finding two SNPs linked to both mineral concentrations. SNPs associated with seed diameter, plumpness, and thickness (Khazaei, Fedoruk, Caron, Vandenberg, & Bett, 2018), and genes associated with Aphanomyces root rot resistance (Ma et al., 2020) have also been identified using AM. To identify candidate genes associated with days to flower, Neupane (2019) evaluated 324 lentil accessions in multiple locations around the world and found four QTLs associated with this key phenological trait.
Genomics-assisted Breeding
There are two main types of genomic-assisted breeding: marker-assisted selection (MAS) and genomic selection (GS). MAS uses molecular markers that map close to or within specific genes or QTLs associated with target traits to select individuals that carry favorable alleles (Mackay, Stone, & Ayroles, 2009; Zhu, Gore, Buckler, & Yu, 2008). Despite the number of QTL studies published to date, very few markers have made their way into lentil breeding programs. The SNP responsible for the zero-tannin seed coat phenotype (tan) has been converted to a KASP assay for use in breeding zero-tannin lentils (Mirali et al., 2016). In addition, KASP assays have been used routinely to screen for imidazolinone tolerance in Canadian and Australian breeding programs. Other markers that have been applied in Australia include those for boron tolerance and Ascochyta blight resistance (A. Shunmugam, pers. comm.). GS integrates marker data from a training population with phenotypic and pedigree data to predict how well a plant will perform as a parent for crossing (Varshney et al., 2014). GS has recently been applied to lentil, evaluating a diversity panel and two recombinant inbred line (RIL) populations for five phenology traits (Haile et al., 2020). The results of this study are the first empirical evidence of GS in lentil and suggest that high accuracy can be obtained within populations and across environments, but not across populations.
Genome Sequencing
Despite advances in high-throughput sequencing and bioinformatics tools, there are still challenges to sequencing Lens spp. because of their large and complex genomes. Lentil has an ∼4-Gbp genome (Arumuganathan & Earle, 1991), which is eight times that of Medicago truncatula , seven times that of common bean (Phaseolus vulgaris), and almost four times that of soybean (Glycine max). An initial draft assembly of the L. culinaris genome was developed using short-read technology (Ramsay et al., 2016; https://knowpulse.usask.ca/genome-assembly/Lc1.2). The genome of a single plant derived from the cultivar CDC Redberry (Vandenberg et al., 2006) was sequenced using different technologies (paired-end, mate-pair, and a few long-read libraries) over a wide range of sizes. Due to the limitations of short reads, it was only possible to assemble 2.7 Gbp using this approach; however, most of the genes appeared to be in the assembly. With the advent of long-read sequencing technology, coupled with Hi-C proximity by ligation mapping, a new version of the CDC Redberry assembly was developed that covers 3.8 Gbp of an estimated 3.92-Gbp genome (Ramsay et al., 2019a; https://knowpulse.usask.ca/genome-assembly/Lcu.2RBY). A complete long-read assembly of the Australian cultivar PBA Blitz is currently in the final stages of completion (S. Kaur, pers. comm.). Having these assemblies will allow us to start looking at structural variation, something that is difficult with simple genetic maps, and compare across legumes, reducing the effort to identify genetic control of traits of common interest.
Understanding the genomes of the other Lens species is important to help guide breeding efforts to introgress valuable traits while minimizing linkage drag following interspecific crossing. The Bett group in Canada and the Kaur group in Australia have sequenced at least one representative of each wild species using long-read technology. A complete assembly of the smaller L. ervoides genome (estimated 3.4-Gbp) based on accession IG 72815 is currently available (Ramsay et al., 2019b; https://knowpulse.usask.ca/genome-assembly/Ler.1DRT). Full genome assemblies are revealing differences in genome size as well as large-scale structural rearrangements among different species. For instance, L. ervoides is smaller due to fewer repeats, not a change in gene content (L. Ramsay, pers. comm.).
Reduced-representation Libraries
For large and complex genomes such as lentil, methods that generate reduced-representation libraries (RRLs) can be used to diminish the cost of sequencing. This approach includes restriction-associated DNA sequencing, e.g., genotyping by sequencing (GBS) and RAD-seq (Elshire et al., 2011). Based on more than 5000 SNPs discovered through GBS, Wong et al. (2015) showed genetic relationships among lentil species. Pavan et al. (2019) found that genetic clusters of Mediterranean lentils are related to geographic patterns and suggested post-domestication spreading routes. More recently, GBS was used to conduct an AM analysis to identify genes related to Aphanomyces root rot resistance in lentil (Ma et al., 2020). Although GBS is a cost-effective alternative for studies of neutral genetic variation, its extensive implementation in lentil is limited because it does not provide sufficient marker density and depth to confidently identify variants under selection in such large and complex genomes.
A different reduced-representation option is exome capture, which targets protein-coding regions of a genome (Hodges et al., 2007). Exome capture arrays allow targeting of a relatively wide range of closely related species, recovering orthologous loci, and focuses on regions that probably have been under selection, facilitating the identification of variants for marker-assisted selection in crops (Bragg, Potter, Bi, & Moritz, 2016).
Of the ∼4-Gbp lentil genome, only 130 Mbp have been identified as putative genic sequences (Ogutcen et al., 2018). This led to the development of an 85-Mbp exome capture array with more than 6.6 million variants, providing a significant amount of genomic data that can be used in different downstream analyses and marker-assisted breeding programs (Ogutcen et al., 2018). Furthermore, evidence of gene duplications across the lentil genome was documented (Ogutcen et al., 2018).
The transcriptome provides information about gene expression and regulation at a specific moment. One of the first lentil transcriptomes was developed by Kaur et al. (2011) using RNA isolated from various tissues and stages, which led to the identification of more than 25,000 unigenes. Sharpe et al. (2013) identified a large collection of SNPs and constructed the first comprehensive genetic map of cultivated lentil based on 3′-cDNA transcript profiling from eight cultivars and two wild accessions. Similar approaches to discovering polymorphisms, performing gene predictions, and constructing genetic maps have continued to be applied using transcriptomic approaches (Dissanayake et al., 2020; Sudheesh, Verma, et al., 2016; Temel, Deniz, Akkale, Kahri̇man, & Tanyolaç, 2015). Moreover, transcriptome analyses have been conducted to characterize differential expression and identify candidate genes associated with traits of interest, including seedling drought stress (Singh et al., 2017), heat response (Singh et al., 2019), Ascochyta blight resistance (Sari et al., 2018), and Stemphylium blight resistance (Cao, Li, Kapoor, & Banniza, 2019).
Pulse Genetic Resources on the Web
In order to maximize the usefulness of genetic data for breeding purposes, it should be curated and connected to phenotypic information. Several legume-focused databases have been developed, including the Legume Information System (LIS; Dash et al., 2016; https://legumeinfo.org), Pulse Crop Database (formerly Cool Season Food Legume Database; https://www.pulsedb.org/), and KnowPulse (https://knowpulse.usask.ca). Each of these resources has a specific focus and set of tools that are invaluable to their crop-specific and comparative communities. Efforts are underway to facilitate communication among these databases so that a researcher could query more than one database at once (L. Sanderson, pers. comm.).
INCREASE Intelligent Collections
Exploitation of genetic resources in crop breeding is limited in comparison to availability of materials, and the potential impact of their use is far from optimal (i.e., lack of comprehensive information regarding passport data and descriptors useful for users, accession heterogeneity, unharmonized data).
Collections are assembled and maintained on an accession basis, where each accession usually comprises a mixture of genotypes that represent a population. For the use of genetic resources, the value of an accession is limited, as information collected at the phenotype level is necessarily obtained from a mixture of genotypes, which greatly limits its value for breeding and research. Thus, a coordinated, interdisciplinary, and multisectorial effort is needed to exploit recent scientific and technological ground-breaking advances, ranging from genomics to information technology (IT) and artificial intelligence (AI), to develop an innovative platform for conservation and sustainable use of genetic resources. INCREASE—Intelligent Collections of Food Legumes Genetic Resources for European Agrofood Systems is a project recently funded through the European Union's Horizon 2020 research and innovation program (https://www.pulsesincrease.eu/) that aims to facilitate efficient and effective conservation and use of plant genetic resources in food and agriculture in order to promote agrobiodiversity and improve sustainable use. INCREASE will enhance the genotypic and phenotypic characterization of genetic resources of four important food legumes: lentil, common bean, chickpea, and lupin (Cortinovis et al., 2021; Kroc et al., 2021). This will be achieved by the INCREASE Intelligent Collection approach, which aims to provide tiers of characterization to increasingly large pools of germplasm. In detail, the INCREASE approach involves development of a set of nested-core collections of genetically purified accessions based on single homozygous genotypes, purified by one or two cycles of single seed descent (SSD) from a large sample of representative accessions from genebanks, including wild species, landraces, and cultivars, and from dynamic conservation on farms. A Reference-CORE (R-CORE) of ∼3000 lentil accessions will be genotyped using a custom-designed repeat depletion assay similar to the one described by Ichida and Abe (2019). A subset of R-CORE will constitute a Training-CORE (T-CORE) based on ∼450 accessions with comprehensive passport and georeferenced information. These will be used for phenotypic characterization (including transcriptomics and metabolomics) and genomic characterization using the repeat depletion assay. Moreover, a specific study will be conducted on lentil for resistance gene clusters (nucleotide-binding leucine-rich repeats or NLRs) using resistance-gene enrichment by applying long-read sequencing (Jupe et al., 2013). This will allow the lentil pan-NLRome to be characterized. A Hyper-CORE (H-CORE) of ∼50 genotypes sampled on an evolutionary transect will be used for deep genomic and phenotypic characterization to explore the phenotypic space (i.e., reaction norm) and identify key traits of interest for future phenotyping. The latest and most-advanced sequencing technologies will be applied to subsamples of H-CORE genotypes to obtain new de novo platinum genomes for lentil (i.e., a contiguous, haplotype-resolved representation of the entire genome). These Intelligent Collections will be shared as a tool among genebanks and will coexist with whole collections available in different genebanks to improve overall management of genetic diversity.
Protocols for Development of INCREASE Intelligent Collections
In order to associate phenotypes with reliable genotypic information, accessions that will become part of the INCREASE Intelligent Collection will be purified using an SSD approach for several cycles and will require the maintenance of thousands of lines. Here, we describe effective protocols and a list of general best practices and considerations for researchers when developing SSD collections of lentils for the purpose of promoting conservation of genetic resources and use in pre-breeding programs. The protocols can be applied to all lentil genotypes from wild lentil species to domesticated lentil lines.
The first step in developing an INCREASE Intelligent Collection is to collect seeds of heterogeneous accessions coming from ex situ or in situ conservation. At the beginning of each seed increase cycle and upon arrival of the seed, before using the seeds for any experiment, Basic Protocol 1 (Recording of Lentil Seed Descriptors) and Basic Protocol 2 (Lentil Seed Imaging) should be used, and seeds traits should be recorded for each accession. Then, one seed from each accession is grown in controlled conditions following Basic Protocol 3 (Lentil Seed Increase). During the first and the subsequent cycles of SSD development, all individual plants coming from each accession should be phenotyped using Basic Protocol 4 (Recording of Primary Seed Lentil INCREASE Descriptors). At the end of the first cycle, seeds harvested from each plant are phenotyped using Basic Protocols 1 and 2, and a single seed from the single plant is used for another cycle of selfing using Basic Protocol 3. During the selfing cycle, tissues are harvested to conduct standard and innovative genomics and molecular phenotyping (e.g., transcriptomics, metabolomics) that will be associated with each pure line developed (Fig. 2).
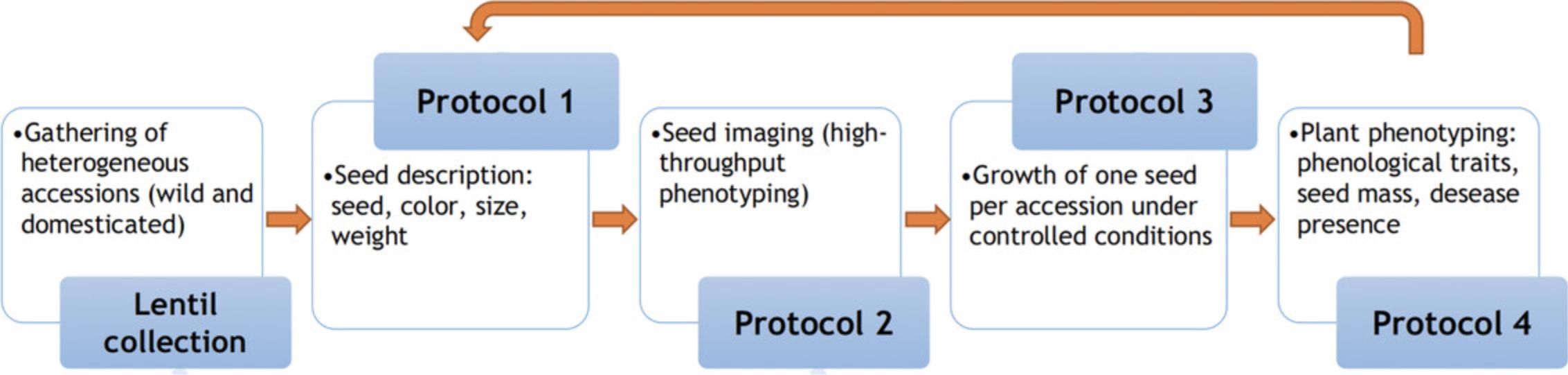
The four different phenotyping protocols reported in this article were developed starting from the International Plant Genetic Resources Institute (IPGRI) descriptors for lentil (IBPGR, 1985) and are based on the International Union for the Protection of New Varieties of Plants (UPOV) Guidelines for the Conduct of Tests for Distinctness, Uniformity and Stability for lentil (UPOV, 2015) with modifications for purposes related to SSD development activities.
Basic Protocol 1: RECORDING OF LENTIL SEED DESCRIPTORS
This protocol is used to characterize genetic resources of the INCREASE project during SSD and subsequent seed increase cycles under controlled conditions. It can also be adopted by genebanks and research institutions to characterize seeds. The protocol will be used at the beginning of each primary and/or secondary seed increase cycle, and as soon as possible upon arrival of the seed, before using seeds for any experiment. The protocol includes a list of descriptors to be scored on lentil seeds.
Materials
- Lentil seeds
- Ruler
- Analytical balance
- Template (spreadsheets) for data collection
1.Record the following traits to be categorized based on the UPOV guidelines for lentil (UPOV, 2015):
- Seed: shape in longitudinal section
- Seed: pattern of secondary color
- Seed: cotyledon color (see Fig. 3 for reference)
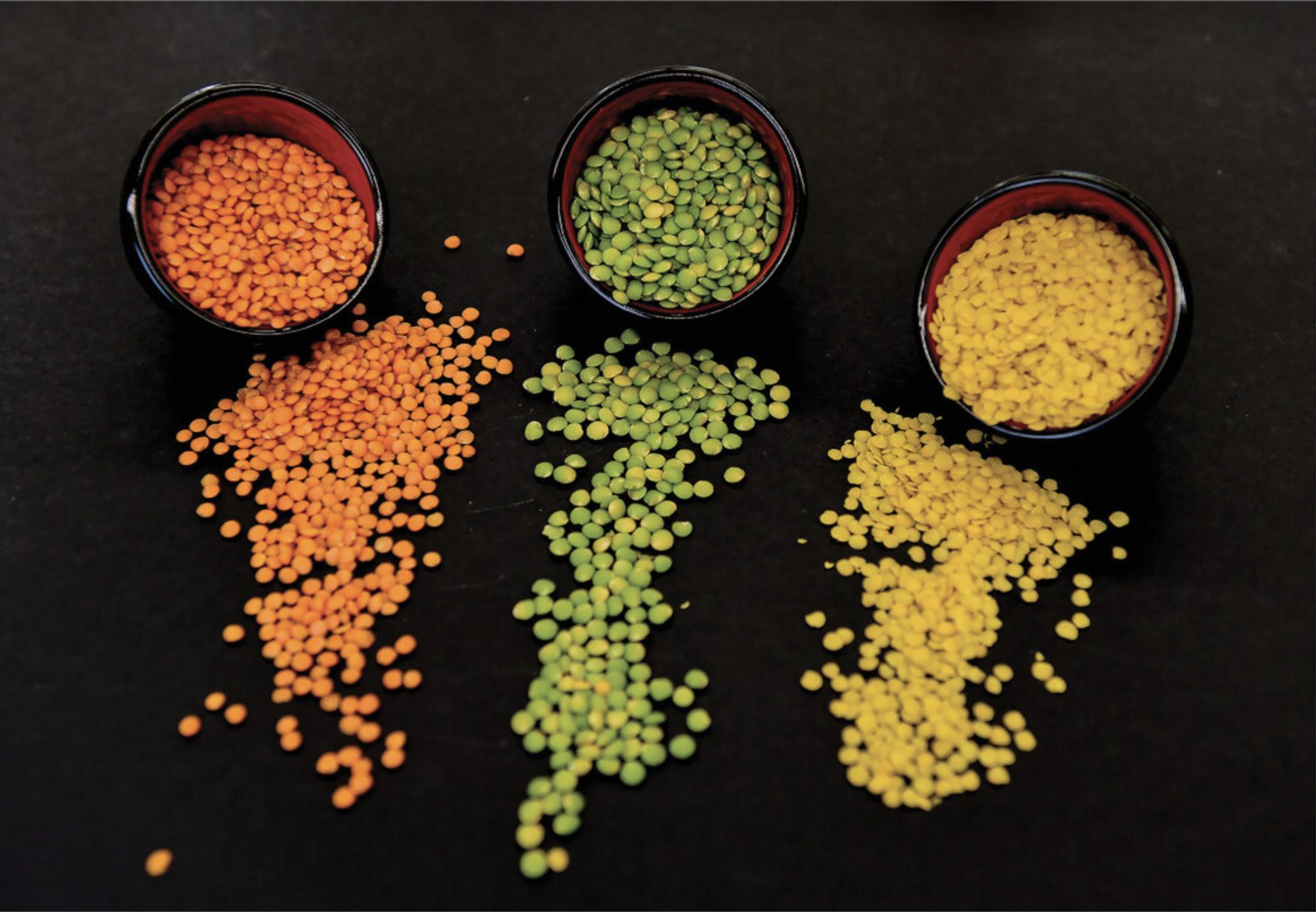
2.Record and measure the following traits:
- Seed: main color (primary color) based on visual observation and evaluation, and using the following categories (Vandenberg & Slinkard, 1990; Fig. 4): brown, tan, grey, green, black, clear (zero tannin), or other (describe).
- Seed: width (mm) based on measurement of ventral dimension, average of 5 seeds
- Seed: weight (g) measured on at least two samples of 100 intact seeds
If this number is not possible, seed weight should be measured on three samples of ten seeds. Immature, broken, or infected seeds should be excluded.
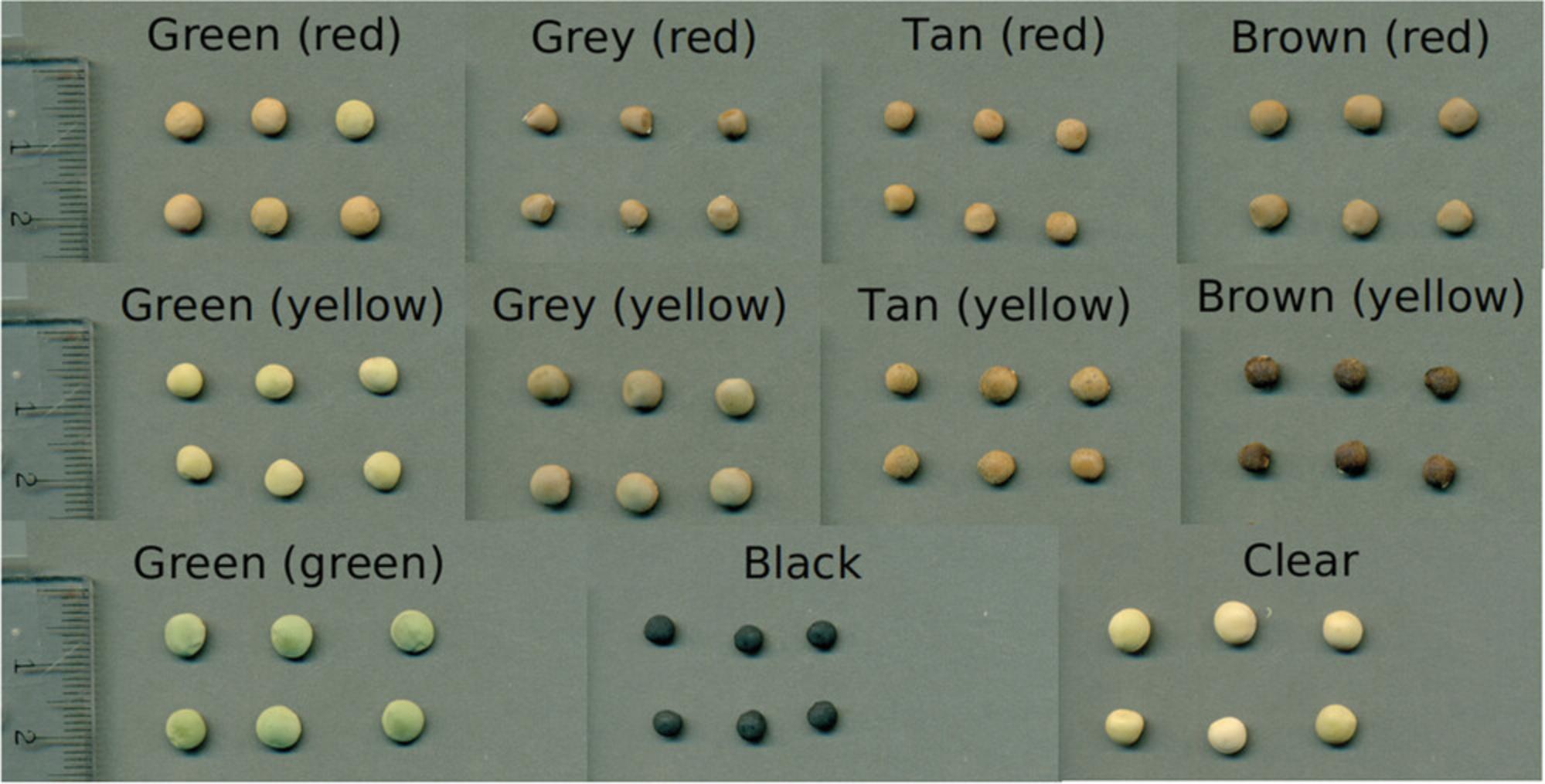
Basic Protocol 2: LENTIL SEED IMAGING
This protocol describes the steps used to acquire image-based data from lentil seeds with the aim of providing a visual quality control of the propagation result and reference images for seed shape, color, and size. A comprehensive paper on digitization of collections based on Nelson, Paul, Riccardi, & Mast (2012) is given in de la Hidalga, van Walsun, Rosin, Sun, & Wijers (2019). Accordingly, the following requirements have to be fulfilled as the best practice standard.
Two imaging protocols are used for phenotyping lentil seed. One will be implemented for all four crops included in the INCREASE project (lentil, common bean, lupin, and chickpea) and is fully described in a common bean review included in this series of articles (Cortinovis et al., 2021).
The second protocol is for high-throughput seed phenotyping and was developed and tested in lentil at the University of Saskatchewan (Halcro et al., 2020). The imaging system captures top- and side-view images of single seeds as they pass underneath integrated RGB cameras. The images are processed and analyzed using the open-source, batch-based software phenoSEED (Halcro et al., 2020), which returns data for seed size, shape, and color. The incorporation of three-dimensional shape analysis provides information about plumpness and symmetry.
The use of any imaging system for phenotyping eliminates potential operator biases and increases the rate of data acquisition. Operators of BELT were able to achieve 50 batches of seeds per hour, each batch containing around 200 seeds. That represents a significant improvement compared to manual dimensional measurement, which can be slow and imprecise (Halcro et al., 2020).
Materials
- Lentil seeds
- BELT imaging instrument (Halcro et al., 2020)
- Seed imaging station
- Python script phenoSEED (Halcro et al., 2020)
- Computer with at least 2.2 GHz CPU and 64 GB RAM
- Server for permanent storage of image files
1.If the seed envelope has a barcode, allow the machine to scan it by placing the empty envelope under the collection funnel.
2.Place lentil seeds in the feeder of the imaging system.
3.If the barcode was scanned properly, start processing the seeds by clicking the touchscreen. If the barcode was not scanned properly or if there is no barcode, manually enter the accession information.
4.Allow time for acquisition of images of individual seeds inside the imaging dome.
5.Following image acquisition, images are stored locally and named automatically according to sample ID (from barcode or information entered in step 3). Images are also displayed automatically, so the operator can quickly check them.
6.Run the Python script phenoSEED to extract information about seed size, shape, color, pattern type, and intensity.
Basic Protocol 3: LENTIL SEED INCREASE
This protocol describes the steps undertaken to plant and grow diverse lentil lines under controlled conditions.
Materials
-
Lentil seeds
-
Soilless, peat-based potting mix
-
Water
-
Fertilizer solutions (20-20-20 and 15-30-15)
-
2.7-L pots
-
Barcode identification labels (or similar)
-
Irrigation picks
-
Stakes for plant support
-
Mesh bags
-
Greenhouse with drip irrigation system
1.Fill 2.7-L pots with a soilless, peat-based potting mix that has been watered well.
2.Label each pot with identifying information.
3.Sow 2-3 seeds per pot.
4.Insert one irrigation pick into each pot.
5.Set growing conditions for a 17-hr daylength with supplemental lighting as required.
6.Set temperature at 22°C day/18°C night.
7.Thin to 1 plant per pot at the seedling stage.
8.Water with 20-20-20 and 15-30-15 fertilizer solutions for vegetative and reproductive growth stages, respectively. Apply first fertilizer ∼10-15 days after planting at a concentration of 1 g/L and volume of 500 ml per 2.7-L pot. Gradually increase the volume as the plants grow.
9.During the vegetative growth stage, insert a stake in each pot and attach to the plant with a twist/bubble tie or place a small cage in the pot to contain the plants (Fig. 5). Contain wild lentil plants in a mesh bag to catch the seeds before the pods shatter.
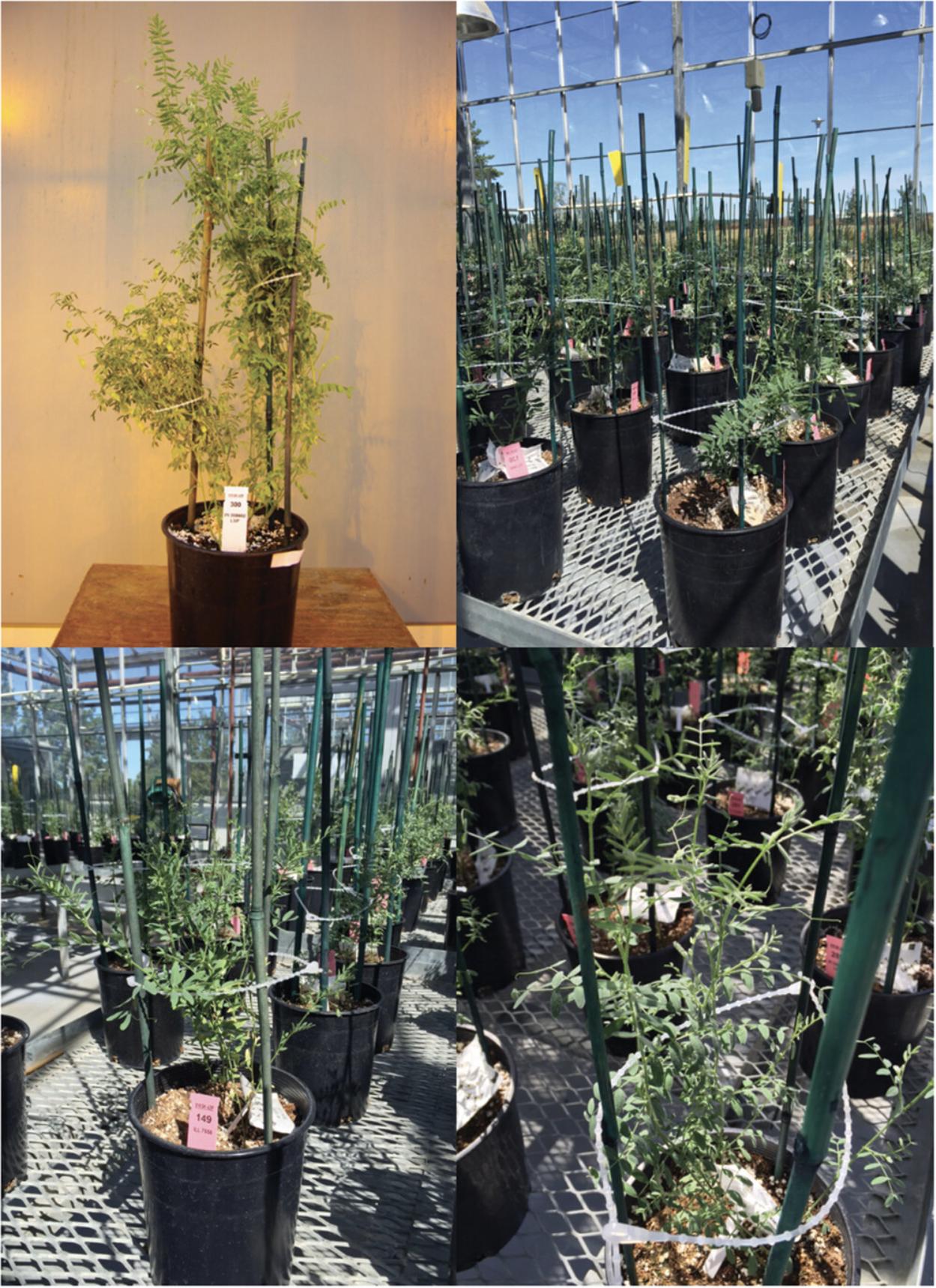
Basic Protocol 4: RECORDING OF PRIMARY LENTIL SEED INCREASE DESCRIPTORS
This protocol describes how to characterize lentil genetic resources of the INCREASE project during seed increase cycles under controlled conditions. It includes a list of phenotyping descriptors to be scored during the growth stages on each lentil genotype of the INCREASE Intelligent Collections (R-CORE, T-CORE, and H-CORE). The descriptors have been developed starting from IPGRI lentil descriptors (IBPGR, 1985) and based on the UPOV guidelines (UPOV, 2015), and have been modified specifically for purposes related to these SSD development activities. Before using the protocol, the following information is collected for each experimental site: data collector name; location name of experimental trial; latitude and longitude of experimental trial; altitude of experimental trial; insect-free conditions used (greenhouse/tunnel/growth chamber); and sowing date.
Materials
- Lentil plants
- Meter stick
- Analytical balance
- Template (spreadsheets) for data collection
Phenotype plants for mandatory traits (priority 1)
1.Record the date the seeds were sown.
2.Record the number of days after planting when 50% of seeds have emerged (days to emergence).
3.Record the number of days after planting when the first leaves begin to have an elongated tendril.
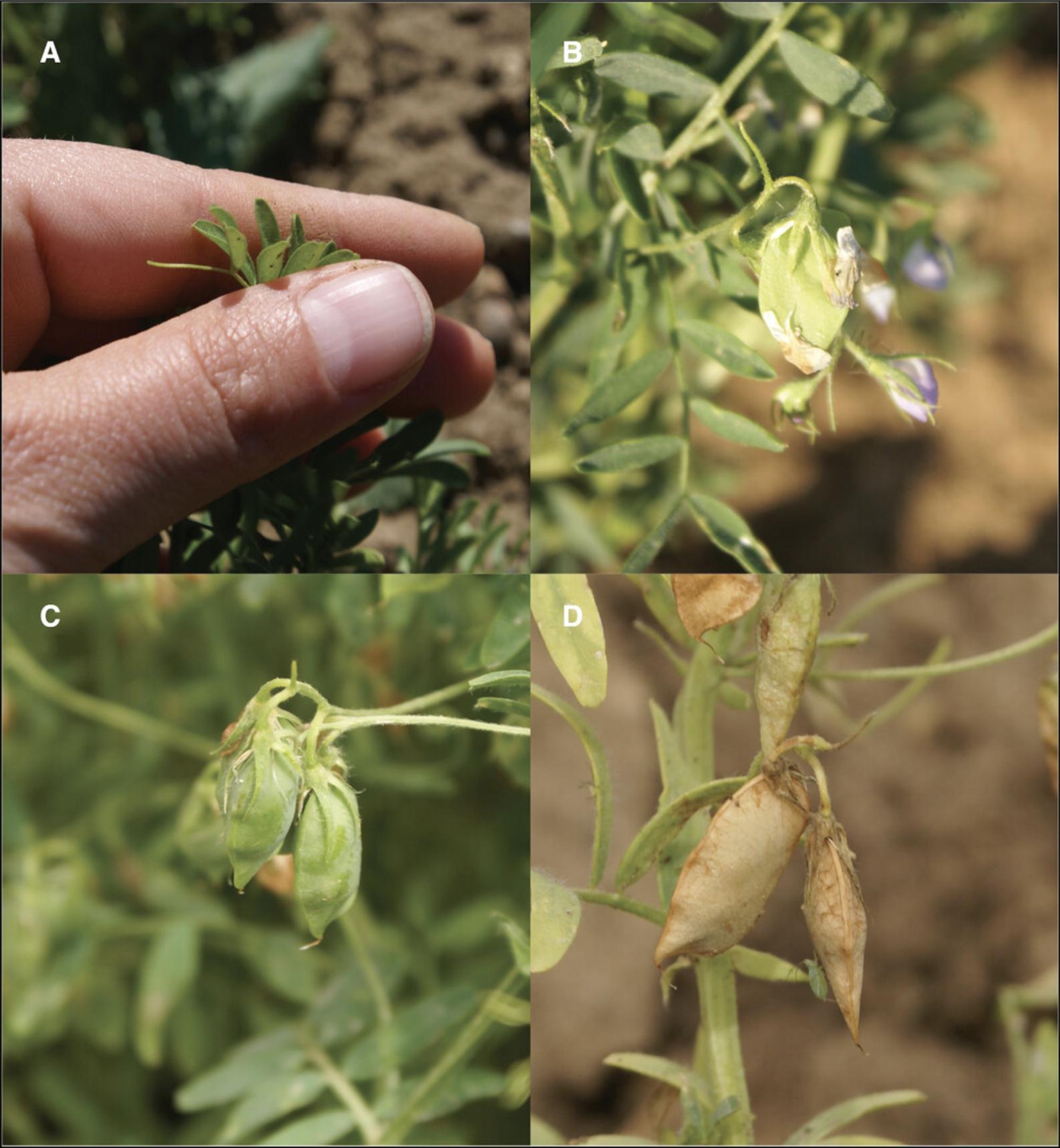
4.Record the number of days after planting when plants have at least one open flower (flower or standard petal is visible) at any node (R1).
5.Record the intensity of ramification when plants have at least one open flower according to categories of 1 = weak, 2 = medium, 3 = strong.
6.Record the presence/absence of anthocyanin pigmentation.
7.Before maturity, record leaf pubescence according to the categories 0 = absent, 1 = slight, 2 = dense.
8.Before maturity, record leaf color according to the categories 1 = light, 2 = medium, 3 = dark.
9.Record leaf shape for the first leaflet at the second flowering node according to the categories 1 = elliptical, 2 = obovate, 3 = rectangular.
10.Record leaf size for the first leaflet at the second flowering node according to the categories 1 = small, 2 = medium, 3 = large.
11.Record total number of flowers per node on the first floral level at the time of flowering.
12.Record flower size according to the categories 1 = small, 2 = medium, 3 = large.
13.Record color of standard in freshly opened flowers according to the categories 1 = white, 2 = pink, 3 = blue, 4 = other (specify).
14.Record the presence/absence of violet stripes of standard.
15.Record the number of days after planting when plants have pods that are visible without having to remove flower petals (R3, Fig. 6B) on nodes 10-13 of the basal primary branch.
16.Record the number of days after planting when plants have seeds in any single pod on nodes 10-13 of the basal primary branch and seeds that have swollen to their maximum size (physiological maturity) and fill more than half of the pod area (R5, Fig. 6C).
17.Record the number of days after planting when half of the plant's pods are mature (dry pods ready to be harvested; Fig. 6D).
18.Record the presence/absence of pod pigmentation.
19.Record pod color before dry harvest maturity (when the pod is not completely dry) according to the categories 1 = light green, 2 = medium green, 3 = dark green.
20.Record the distance (cm) from the soil to the end of the longest stem using a meter stick. Record values when half of the pods are mature (R7).
21.Record plant habit according to the categories 1 = upright, 2 = semi-upright, 3 = horizontal.
22.Record the distance (cm) from the soil to the bottom of the lowest pod using a meter stick. Record values when half of the pods are mature (R7).
23.Record the number of days after planting when 90% of pods are golden-brown (R8).
24.Record pod dehiscence one week after maturity according to the categories 0 = none, 1 = low, 2 = medium, 3 = high.
25.Record the number of days from planting to harvest.
26.Record pod shedding, after or during harvesting one week post-maturity, according to the categories 0 = none, 1 = low, 2 = medium, 3 = high.
27.Record pod length (average of three pods in cm) at dry harvest maturity with a meter stick. Make observations using pods that are completely dry but unopened.
28.Record pod width (average of three pods in cm) from suture to suture with a meter stick. Make observations using pods that are completely dry but unopened.
29.Record total mass (g) of all seeds harvested from each plant.
30.Record the total number of seeds harvested from each plant.
31.Record the mass (g) of 100 representative seeds.
32.Record the presence/absence of disease using the categories 0 = no disease present, 1 = disease present, 2 = unsure. If able, describe or make notes in a “Disease-specific Comments” column, including but not limited to the presence of many or specific diseases. Specific mention should be made of the following:
- Insects:
- aphid, weevil (Sitona spp.; Bruchus spp., …), pod borer (Etiella zinckenella Treit), other
- Fungi:
- rust (Uromyces fabae), blight (Ascochyta spp.), vascular wilt (Fusarium oxysporum), downy mildew (Peronospera lentis Gaum.), other
- Bacteria:
- virus parasitic weed (Orobanche spp.)
33.Record the presence of any stress using the categories 0 = no disease present, 1 = disease present, 2 = unsure. Make notes on specific stresses in a “Stress-specific Comments” column. Specific mention should be made of the following stresses:
- Winter kill: Proportion of plants emerged prior to winter that survive through winter
- Low temperature: Damage caused to aerial plant parts not associated with winter kill
- High temperature
- Drought
- High soil moisture
- Salinity
Phenotype plants for optional traits (priority 2)
34.Record the number of nodes on the primary stem when the first flower opens.
35.Record the total number of pods for each plant.
36.Record the mass (g) of dry, above-ground plant material from each plant excluding the seed.
COMMENTARY
Conclusion
This article presents an overview of the current genetic and genomic resources available for cultivated lentil and its wild relatives. Until recently, lentil was considered an orphan crop with respect to genetic resources, but this is changing and new data and tools have been generated. This includes genotypes of hundreds of accessions from around the world using several sequencing approaches; genetic maps; QTLs and genes associated with traits of interest; and most recently genome assemblies. As a result of the genetic and phenotypic characterization of Lens accessions preserved in genebanks, it has been possible to elucidate the domestication history and subsequent diversification of Lens , the structure of its populations and diversity levels, the genetic architecture of some important traits, and the implementation of marker-assisted and genomic selection.
Improving adaptation of cultivars is an ongoing process that frequently involves the introduction of alleles from close wild species and landraces. Despite the identification of loci associated with traits of interest in landraces and wild Lens , there is still great potential in terms of genetic variation that can be exploited to maintain and expand yield gains and to face agricultural challenges such as climate change and food security. Also, elucidating the adaptation of Lens taxa to different environments is needed to assist breeders in increasing diversity through introduction of germplasm from diverse regions. In this context, large-scale projects such as INCREASE (https://www.pulsesincrease.eu/) and EVOLVES (https://knowpulse.usask.ca/study/2691111) seek to improve the sustainable use of genetic resources by developing efficient and effective conservation tools to promote agrobiodiversity and its implementation.
Acknowledgments
We are grateful for financial support from the Western Grains Research Foundation, Saskatchewan Pulse Growers, the University of Saskatchewan, Genome Canada, and the European Union's Horizon 2020 research and innovation program project INCREASE—Intelligent Collections of Food Legumes Genetic Resources for European Agrofood Systems (grant agreement no. 862862). A.G.-G. is supported through the Genome Canada–funded project Enhancing the Value of Lentil Variation for Ecosystem Survival (EVOLVES) awarded to K.E.B. and managed by Genome Prairie. E.V.W is supported in part by the USDA Hatch program through the Vermont State Agricultural Experimental Station.
Author Contributions
Azalea Guerra-García : Writing-original draft, Tania Gioia : Writing-original draft, Eric von Wettberg : Writing-original draft, Writing-review & editing, Giuseppina Logozzo : Writing-review & editing, Roberto Papa : Funding acquisition, Writing-review & editing, Elena Bitocchi : Project administration, Kirstin E Bett : Funding acquisition, Writing-original draft, Writing-review & editing.
Conflict of Interest
The authors declare no conflict of interest.
Open Research
Data Availability Statement
Data sharing not applicable—no new data generated.
Supporting Information
Filename | Description |
---|---|
cpz1134-sup-0001-tableS1-S2.docx24.2 KB | Supplementary Information |
Please note: The publisher is not responsible for the content or functionality of any supporting information supplied by the authors. Any queries (other than missing content) should be directed to the corresponding author for the article.
Literature Cited
- Abbo, S., & Ladizinsky, G. (1991). Anatomical aspects of hybrid embryo abortion in the genus Lens L. Botanical Gazette , 152(3), 316–320. doi: 10.1086/337895.
- Abbo, S., & Ladizinsky, G. (1994). Genetical aspects of hybrid embryo abortion in the genus Lens L. Heredity , 72(2), 193–200. doi: 10.1038/hdy.1994.26.
- Ahmad, M., Fautrier, A. G., McNeil, D. L., Burritt, D. J., & Hill, G. D. (1995). Attempts to overcome postfertilization barrier in interspecific crosses of the genus Lens. Plant Breeding , 114(6), 558–560. doi: 10.1111/j.1439-0523.1995.tb00859.x.
- Alo, F., Furman, B. J., Akhunov, E., Dvorak, J., & Gepts, P. (2011). Leveraging genomic resources of model species for the assessment of diversity and phylogeny in wild and domesticated lentil. Journal of Heredity , 102(3), 315–329. doi: 10.1093/jhered/esr015.
- Arumuganathan, K., & Earle, E. D. (1991). Nuclear DNA content of some important plant species. Plant Molecular Biology Reporter , 9, 208–218. doi: 10.1007/BF02672069.
- Ates, D., Aldemir, S., Yagmur, B., Kahraman, A., Ozkan, H., Vandenberg, A., & Tanyolac, M. B. (2018). QTL mapping of genome regions controlling manganese uptake in lentil seed. G3 , 8(5), 1409–1416. doi: 10.1534/g3.118.200259.
- Ates, D., Sever, T., Aldemir, S., Yagmur, B., Temel, H. Y., Kaya, H. B., … Tanyolac, B. (2016). Identification QTLs controlling genes for Se uptake in lentil seeds. PLoS One , 11(3), e0149210. doi: 10.1371/journal.pone.0149210.
- Barulina, E. I. (1930). Lentils of the U.S.S.R. and of other countries: A botanic-agronomical monograph. Bulletin of Applied Botany, of Genetics and Plant-breeding , Institute of Plant Industry Lenin Academy of Agricultural Sciences in the U.S.S.R.
- Bayaa, B., Erskine, W., & Hamdi, A. (1994). Response of wild lentil to Ascochyta fabae f.sp. lentis from Syria. Genetic Resources and Crop Evolution , 41(2), 61–65. doi: 10.1007/bf00053049.
- Bayaa, B., Erskine, W., & Hamdi, A. (1995). Evaluation of a wild lentil collection for resistance to vascular wilt. Genetic Resources and Crop Evolution , 42(3), 231–235. doi: 10.1007/bf02431257.
- Bhadauria, V., Ramsay, L., Bett, K. E., & Banniza, S. (2017). QTL mapping reveals genetic determinants of fungal disease resistance in the wild lentil species Lens ervoides. Scientific Reports , 7(1), 3231. doi: 10.1038/s41598-017-03463-9.
- Bragg, J. G., Potter, S., Bi, K., & Moritz, C. (2016). Exon capture phylogenomics: Efficacy across scales of divergence. Molecular Ecology Resources , 16(5), 1059–1068. doi: 10.1111/1755-0998.12449.
- Buchwaldt, L., Anderson, K. L., Morrall, R. A. A., Gossen, B. D., & Bernier, C. C. (2004). Identification of lentil germ plasm resistant to Colletotrichum truncatum and characterization of two pathogen races. Phytopathology , 94(3), 236–243. doi: 10.1094/phyto.2004.94.3.236.
- Cao, Z., Li, L., Kapoor, K., & Banniza, S. (2019). Using a transcriptome sequencing approach to explore candidate resistance genes against stemphylium blight in the wild lentil species Lens ervoides. BMC Plant Biology , 19(1), 399. doi: 10.1186/s12870-019-2013-6.
- Chen, Y. H., Shapiro, L. R., Benrey, B., & Cibrián-Jaramillo, A. (2017). Back to the origin: In situ studies are needed to understand selection during crop diversification. Frontiers in Ecology and Evolution , 5, 125. doi: 10.3389/fevo.2017.00125.
- Chowdhury, M. A., Andrahennadi, C. P., Slinkard, A. E., & Vandenberg, A. (2001). RAPD and SCAR markers for resistance to acochyta blight in lentil. Euphytica , 118(3), 331–337. doi: 10.1023/A:1017581817201.
- Cobb, J. N., DeClerck, G., Greenberg, A., Clark, R., & McCouch, S. (2013). Next-generation phenotyping: Requirements and strategies for enhancing our understanding of genotype–phenotype relationships and its relevance to crop improvement. Theoretical and Applied Genetics , 126(4), 867–887. doi: 10.1007/s00122-013-2066-0.
- Cortinovis, G., Oppermann, M., Neumann, K., Graner, A., Gioia, T., Marsella, M., … Bitocchi, E. (2021) (in press). Common bean INCREASE Intelligent Collections: Towards the development, maintenance, and standardized phenotypic characterization of single seed descent genetic resources. Current Protocols , e133. doi: 10.1002/cpz1.133.
- Coyne, C. J., Kumar, S., von Wettberg, E. J. B., Marques, E., Berger, J. D., Redden, R. J., … Smýkal, P. (2020). Potential and limits of exploitation of crop wild relatives for pea, lentil, and chickpea improvement. Legume Science , 2(2), e36. doi: 10.1002/leg3.36.
- Cubero, J. I. (1981). Origin, taxonomy, and domestication. In C. Webb & G. Hawtin (Eds.), Lentils (pp. 15–38). Slough, U.K.: Commonwealth Agricultural Bureau.
- Cubero, J. I. (1984). Taxonomy, distribution and evolution of the lentil and its wild relatives. In J. R. Witcombe & W. Erskine (Eds.), Genetic resources and their exploitation—Chickpeas, faba beans and lentils (pp. 187–203). Dordrecht, The Netherlands: Springer.
- Dadu, R. H. R., Ford, R., Sambasivam, P., & Gupta, D. (2017). A novel Lens orientalis resistance source to the recently evolved highly aggressive Australian Ascochyta lentis isolates. Frontiers in Plant Science , 8, 1038. doi: 10.3389/fpls.2017.01038.
- Dash, S., Campbell, J. D., Cannon, E. K. S., Cleary, A. M., Huang, W., Kalberer, S. R., … Cannon, S. B. (2016). Legume information system (LegumeInfo.org): A key component of a set of federated data resources for the legume family. Nucleic Acids Research , 44(D1), D1181–D1188. doi: 10.1093/nar/gkv1159.
- de la Hidalga, N. A., van Walsun, M., Rosin, P., Sun, X., & Wijers, A. (2019). Quality management methodologies for digitisation operations. Zenodo. doi: 10.5281/zenodo.3469521.
- Dissanayake, R., Braich, S., Cogan, N. O. I., Smith, K., & Kaur, S. (2020). Characterization of genetic and allelic diversity amongst cultivated and wild lentil accessions for germplasm enhancement. Frontiers in Genetics , 11, 546. doi: 10.3389/fgene.2020.00546.
- Durán, Y., Fratini, R., García, P., & de la Vega, M. P. (2004). An intersubspecific genetic map of Lens. Theoretical and Applied Genetics , 108(7), 1265–1273. doi: 10.1007/s00122-003-1542-3.
- El-Bouhssini, M., Sarker, A., Erskine, W., & Joubi, A. (2008). First sources of resistance to Sitona weevil (Sitona crinitus Herbst) in wild Lens species. Genetic Resources and Crop Evolution , 55(1), 1–4. doi: 10.1007/s10722-007-9297-3.
- Elshire, R. J., Glaubitz, J. C., Sun, Q., Poland, J. A., Kawamoto, K., Buckler, E. S., & Mitchell, S. E. (2011). A robust, simple genotyping-by-sequencing (GBS) approach for high diversity species. PLoS One , 6(5), e19379. doi: 10.1371/journal.pone.0019379.
- Erskine, W., Muehlbauer, F. J., & Short, R. W. (1990). Stages of development in lentil. Experimental Agriculture , 26(3), 297–302. doi: 10.1017/s0014479700018457.
- Eujayl, I., Baum, M., Powell, W., Erskine, W., & Pehu, E. (1998). A genetic linkage map of lentil (Lens sp.) based on RAPD and AFLP markers using recombinant inbred lines. Theoretical and Applied Genetics , 97(1-2), 83–89. doi: 10.1007/s001220050869.
- Fedoruk, M. J., Vandenberg, A., & Bett, K. E. (2013). Quantitative trait loci analysis of seed quality characteristics in lentil using single nucleotide polymorphism markers. Plant Genome , 6(3). doi: 10.3835/plantgenome2013.05.0012.
- Ferguson, M. E., Ford-Lloyd, B. V., Robertson, L. D., Maxted, N., & Newbury, H. J. (1998). Mapping the geographical distribution of genetic variation in the genus Lens for the enhanced conservation of plant genetic diversity. Molecular Ecology , 7(12), 1743–1755. doi: 10.1046/j.1365-294x.1998.00513.x.
- Ferguson, M. E., Maxted, N., Van Slageren, M., & Robertson, L. D. (2000). A re-assessment of the taxonomy of Lens Mill. (Leguminosae, Papilionoideae, Vicieae). Botanical Journal of the Linnean Society , 133(1), 41–59. doi: 10.1111/j.1095-8339.2000.tb01536.x.
- Ferguson, M. E., Robertson, L. D., Ford-Lloyd, B. V., John Newbury, H., & Maxted, N. (1998). Contrasting genetic variation amongst lentil landraces from different geographical origins. Euphytica , 102(2), 265–273. doi: 10.1023/A:1018331432580.
- Fernández-Aparicio, M., Sillero, J. C., Pérez-de-Luque, A., & Rubiales, D. (2008). Identification of sources of resistance to crenate broomrape (Orobanche crenata) in Spanish lentil (Lens culinaris) germplasm. Weed Research , 48(1), 85–94. doi: 10.1111/j.1365-3180.2008.00604.x
- Fernández-Aparicio, M., Sillero, J. C., & Rubiales, D. (2009). Resistance to broomrape in wild lentils (Lens spp.). Plant Breeding , 128(3), 266–270. doi: 10.1111/j.1439-0523.2008.01559.x.
- Fiala, J. V., Tullu, A., Banniza, S., Séguin-Swartz, G., & Vandenberg, A. (2009). Interspecies transfer of resistance to anthracnose in lentil (Lens culinaris Medic.). Crop Science , 49(3), 825–830. doi: 10.2135/cropsci2008.05.0260.
- Ford, R., Pang, E. C. K., & Taylor, P. W. J. (1999). Genetics of resistance to ascochyta blight (Ascochyta lentis) of lentil and the identification of closely linked RAPD markers. Theoretical and Applied Genetics , 98(1), 93–98. doi: 10.1007/s001220051044.
- Fratini, R., & Ruiz, M. L. (2006). Interspecific hybridization in the genus Lens applying in vitro embryo rescue. Euphytica , 150(1-2), 271–280. doi: 10.1007/s10681-006-9118-3.
- Fuller, D. Q. (2007). Contrasting patterns in crop domestication and domestication rates: Recent archaeobotanical insights from the old world. Annals of Botany , 100(5), 903–924. doi: 10.1093/aob/mcm048.
- Gorim, L. Y., & Vandenberg, A. (2017). Evaluation of wild lentil species as genetic resources to improve drought tolerance in cultivated lentil. Frontiers in Plant Science , 8, 1129. doi: 10.3389/fpls.2017.01129.
- Gujaria-Verma, N., Vail, S. L., Carrasquilla-Garcia, N., Penmetsa, R. V., Cook, D. R., Farmer, A. D., … Bett, K. E. (2014). Genetic mapping of legume orthologs reveals high conservation of synteny between lentil species and the sequenced genomes of Medicago and chickpea. Frontiers in Plant Science , 5, 676. doi: 10.3389/fpls.2014.00676.
- Gupta, D., & Sharma, S. K. (2006). Evaluation of wild Lens taxa for agro-morphological traits, fungal diseases and moisture stress in North Western Indian hills. Genetic Resources and Crop Evolution , 53(6), 1233–1241. doi: 10.1007/s10722-005-2932-y.
- Gupta, D., Taylor, P. W. J., Inder, P., Phan, H. T. T., Ellwood, S. R., Mathur, P. N., … Ford, R. (2012). Integration of EST-SSR markers of Medicago truncatula into intraspecific linkage map of lentil and identification of QTL conferring resistance to ascochyta blight at seedling and pod stages. Molecular Breeding , 30(1), 429–439. doi: 10.1007/s11032-011-9634-2.
- Gupta, M., Verma, B., Kumar, N., Chahota, R. K., Rathour, R., Sharma, S. K., … Sharma, T. R. (2012). Construction of intersubspecific molecular genetic map of lentil based on ISSR, RAPD and SSR markers. Journal of Genetics , 91(3), 279–287. doi: 10.1007/s12041-012-0180-4.
- Haile, T. A., Heidecker, T., Wright, D., Neupane, S., Ramsay, L., Vandenberg, A., & Bett, K. E. (2020). Genomic selection for lentil breeding: Empirical evidence. Plant Genome , 13(1), 1. doi: 10.1002/tpg2.20002.
- Halcro, K., McNabb, K., Lockinger, A., Socquet-Juglard, D., Bett, K. E., & Noble, S. D. (2020). The BELT and phenoSEED platforms: Shape and colour phenotyping of seed samples. Plant Methods , 16, 49. doi: 10.1186/s13007-020-00591-8.
- Hamdi, A., Küsmenoĝlu, I., & Erskine, W. (1996). Sources of winter hardiness in wild lentil. Genetic Resources and Crop Evolution , 43(1), 63–67. doi: 10.1007/bf00126942.
- Hammer, K. (1984). The domestication syndrome. Kulturpflanzen , 32(1), 11–34. doi: 10.1007/bf02098682.
- Hamwieh, A., Udupa, S. M., Choumane, W., Sarker, A., Dreyer, F., Jung, C., & Baum, M. (2005). A genetic linkage map of Lens sp. based on microsatellite and AFLP markers and the localization of fusarium vascular wilt resistance. Theoretical and Applied Genetics , 110(4), 669–677. doi: 10.1007/s00122-004-1892-5.
- Havey, M. J., & Muehlbauer, F. J. (1989). Linkages between restriction fragment length, isozyme, and morphological markers in lentil. Theoretical and Applied Genetics , 77(3), 395–401. doi: 10.1007/bf00305835.
- Hodges, E., Xuan, Z., Balija, V., Kramer, M., Molla, M. N., Smith, S. W., … McCombie, W. R. (2007). Genome-wide in situ exon capture for selective resequencing. Nature Genetics , 39(12), 1522–1527. doi: 10.1038/ng.2007.42.
- Hoque, M. E., & Hasan, M. M. (2012). Molecular diversity analysis of lentil (Lens culinaris Medik.) through RAPD markers. Plant Tissue Culture & Biotechnology, 22(1), 51–58. doi: 10.3329/ptcb.v22i1.11260.
- Ichida, H., & Abe, T. (2019). An improved and robust method to efficiently deplete repetitive elements from complex plant genomes. Plant Science , 280, 455–460. doi: 10.1016/j.plantsci.2018.10.021.
- International Board for Plant Genetic Resources (IBPGR). (1985). Lentil descriptors. Rome: IBPGR.
- International Union for the Protection of New Varieties of Plants (UPOV). (2015). Lentil: Guidelines for the conduct of tests for distinctness, uniformity and stability. Retrieved from https://static.yanyin.tech/literature/current_protocol/10.1002/cpz1.134/attachments/tg210.pdf.
- Janick, J. (2015). Nikolai Ivanovich Vavilov: Plant geographer, geneticist, martyr of science. HortScience , 50(6), 772–776. doi: 10.21273/hortsci.50.6.772.
- Jupe, F., Witek, K., Verweij, W., Śliwka, J., Pritchard, L., Etherington, G. J., … Jones, D. G. (2013). Resistance gene enrichment sequencing (RenSeq) enables re-annotation of the NB-LRR gene family from sequenced plant genomes and rapid mapping of resistance loci in segregating populations. Plant Journal , 76, 530–544. doi: 10.1111/tpj.12307.
- Kahraman, A., Kusmenoglu, I., Aydin, N., Aydogan, A., Erskine, W., & Muehlbauer, F. J. (2004). QTL mapping of winter hardiness genes in lentil. Crop Science , 44(1), 13. doi: 10.2135/cropsci2004.0013.
- Kaur, S., Cogan, N. O. I., Pembleton, L. W., Shinozuka, M., Savin, K. W., Materne, M., & Forster, J. W. (2011). Transcriptome sequencing of lentil based on second-generation technology permits large-scale unigene assembly and SSR marker discovery. BMC Genomics , 12, 265. doi: 10.1186/1471-2164-12-265.
- Kaur, S., Cogan, N. O. I., Stephens, A., Noy, D., Butsch, M., Forster, J. W., & Materne, M. (2014). EST-SNP discovery and dense genetic mapping in lentil (Lens culinaris Medik.) enable candidate gene selection for boron tolerance. Theoretical and Applied Genetics , 127(3), 703–713. doi: 10.1007/s00122-013-2252-0.
- Khazaei, H., Caron, C. T., Fedoruk, M., Diapari, M., Vandenberg, A., Coyne, C. J., … Bett, K. E. (2016). Genetic diversity of cultivated lentil (Lens culinaris Medik.) and its relation to the world's agro-ecological zones. Frontiers in Plant Science , 7, 1093. doi: 10.3389/fpls.2016.01093.
- Khazaei, H., Fedoruk, M., Caron, C. T., Vandenberg, A., & Bett, K. E. (2018). Single nucleotide polymorphism markers associated with seed quality characteristics of cultivated lentil. Plant Genome , 11(1), 170051. doi: 10.3835/plantgenome2017.06.0051.
- Khazaei, H., Podder, R., Caron, C. T., Kundu, S. S., Diapari, M., Vandenberg, A., & Bett, K. E. (2017). Marker-trait association analysis of iron and zinc concentration in lentil (Lens culinaris Medik.) seeds. Plant Genome , 10(2). doi: 10.3835/plantgenome2017.02.0007.
- Kroc, M., Tomazewska, M., Czepiel, K., Bitocchi, E., Oppermann, M., Newmann, K., … Susek, K. (2021) (in press). Lupin INCREASE Intelligent Collections: Characterization and development of single seed descent genetic resources. Current Opinion in Plant Biology.
- Kumar, J., Thavarajah, D., Kumar, S., Sarker, A., & Singh, N. P. (2018). Analysis of genetic variability and genotype × environment interactions for iron and zinc content among diverse genotypes of lentil. Journal of Food Science and Technology , 55(9), 3592–3605. doi: 10.1007/s13197-018-3285-9.
- Kumar, S., Rajendran, K., Kumar, J., Hamwieh, A., & Baum, M. (2016). Current knowledge in lentil genomics and its application for crop improvement. In S. Kumar (Ed.), Crop breeding: Bioinformatics and preparing for climate change (pp. 309–327). Apple Academic Press. doi: 10.1201/9781315365084-11.
- Kumari, M., Mittal, R. K., Chahota, R. K., Thakur, K., Lata, S., & Gupta, D. (2018). Assessing genetic potential of elite interspecific and intraspecific advanced lentil lines for agronomic traits and their reaction to rust (Uromyces viciae-fabae). Crop and Pasture Science , 69(10), 999. doi: 10.1071/cp17145.
- Ladizinsky, G. (1993). Wild lentils. Critical Reviews in Plant Sciences , 12(3), 169. doi: 10.1080/713608047.
- Ladizinsky, G., Braun, D., & Muehlbauer, F. J. (1983). Evidence for domestication of Lens nigricans (M. Bieb.) Godron in S Europe. Botanical Journal of the Linnean Society , 87(2), 169–176. doi: 10.1111/j.1095-8339.1983.tb00988.x.
- Ladizinsky, G., Cohen, D., & Muehlbauer, F. J. (1985). Hybridization in the genus Lens by means of embryo culture. Theoretical and Applied Genetics , 70(1), 97–101. doi: 10.1007/bf00264489.
- Laserna-Ruiz, I., De-Los-Mozos-Pascual, M., Santana-Méridas, O., Sánchez-Vioque, R., & Rodríguez-Conde, M. F. (2012). Screening and selection of lentil (Lens Miller) germplasm resistant to seed bruchids (Bruchus spp.). Euphytica , 188(2), 153–162. doi: 10.1007/s10681-012-0752-7.
- Liu, J., & Jin, L. I. U. (2009). Analysis of genetic diversity population structure in lentil (Lens culinaris Medik.) germplasm by SSR markers. Acta Agronomica Sinica , 34(11), 1901–1909. doi: 10.3724/sp.j.1006.2008.01901.
- Ma, Y., Marzougui, A., Coyne, C. J., Sankaran, S., Main, D., Porter, L. D., … McGee, R. J. (2020). Dissecting the genetic architecture of Aphanomyces root rot resistance in lentil by QTL mapping and genome-wide association study. International Journal of Molecular Sciences , 21(6), 2129. doi: 10.3390/ijms21062129.
- Mackay, T. F. C., Stone, E. A., & Ayroles, J. F. (2009). The genetics of quantitative traits: Challenges and prospects. Nature Reviews Genetics , 10(8), 565–577. doi: 10.1038/nrg2612.
- Marques, E., Kur, A., Bueno, E., & Wettberg, E. (2020). Defining and improving the rotational and intercropping value of a crop using a plant-soil feedbacks approach. Crop Science , 60, 2195–2203. doi: 10.1002/csc2.20200.
- Maxted, N., Avagyan, A., Frese, L., Iriondo, J., Kell, S., Brehm, J. M., … Dulloo, E. (2015). Conservation planning for crop wild relative diversity. In R. Redden, S. S. Yadav, N. Maxted, M. E. Dulloo, L. Guarino, & P. Smith (Eds.), Crop wild relatives and climate change (pp. 88–107). Hoboken, NJ: Wiley-Blackwell. doi: 10.1002/9781118854396.ch6.
- Meyer, R. S., & Purugganan, M. D. (2013). Evolution of crop species: Genetics of domestication and diversification. Nature Reviews Genetics , 14(12), 840–852. doi: 10.1038/nrg3605.
- Mirali, M., Purves, R. W., Stonehouse, R., Song, R., Bett, K., & Vandenberg, A. (2016). Genetics and biochemistry of zero-tannin lentils. PLoS One , 11(10), e0164624. doi: 10.1371/journal.pone.0164624.
- Mohammadi, N., Puralibaba, H., Mohammadi Goltapeh, E., Babaie Ahari, A., & Pakdaman Sardrood, B. (2012). Advanced lentil lines screened for resistance to Fusarium oxysporum f. sp. lentis under greenhouse and field conditions. Phytoparasitica , 40(1), 69–76. doi: 10.1007/s12600-011-0201-5.
- Nelson, G., Paul, D., Riccardi, G., & Mast, A. (2012). Five task clusters that enable efficient and effective digitization of biological collections. ZooKeys , 209, 19.45. doi: 10.3897/zookeys.209.3135.
- Neupane, S. (2019). Flowering time response of diverse lentil (Lens culinaris Medik.) germplasm grown in multiple environments. M.S. thesis. University of Saskatchewan, Saskatoon, Canada.
- Ogutcen, E., Ramsay, L., von Wettberg, E. B., & Bett, K. E. (2018). Capturing variation in Lens (Fabaceae): Development and utility of an exome capture array for lentil. Applications in Plant Sciences , 6(7), e01165. doi: 10.1002/aps3.1165.
- Pavan, S., Bardaro, N., Fanelli, V., Marcotrigiano, A. R., Mangini, G., Taranto, F., … Ricciardi, L. (2019). Genotyping by sequencing of cultivated lentil (Lens culinaris Medik.) highlights population structure in the Mediterranean gene pool associated with geographic patterns and phenotypic variables. Frontiers in Genetics , 10, 872. doi: 10.3389/fgene.2019.00872.
- Phan, H. T. T., Ellwood, S. R., Hane, J. K., Ford, R., Materne, M., & Oliver, R. P. (2007). Extensive macrosynteny between Medicago truncatula and Lens culinaris ssp. culinaris. Theoretical and Applied Genetics , 114(3), 549–558. doi: 10.1007/s00122-006-0455-3.
- Piperno, D. R. (2017). Assessing elements of an extended evolutionary synthesis for plant domestication and agricultural origin research. Proceedings of the National Academy of Sciences of the United States of America , 114(25), 6429–6437. doi: 10.1073/pnas.1703658114.
- Podder, R., Banniza, S., & Vandenberg, A. (2013). Screening of wild and cultivated lentil germplasm for resistance to stemphylium blight. Plant Genetic Resources , 11(1), 26–35. doi: 10.1017/s1479262112000329.
- Polanco, C., de Miera, L. E. S., González, A. I., García, P., Fratini, R., Vaquero, F., … de la Vega, M. P. (2019). Construction of a high-density interspecific (Lens culinaris × L. odemensis) genetic map based on functional markers for mapping morphological and agronomical traits, and QTLs affecting resistance to Ascochyta in lentil. PLoS One , 14(3), e0214409. doi: 10.1371/journal.pone.0214409.
- Ramsay, L., Chan, C., Sharpe, A. G., Cook, D. R., Penmetsa, R. V., Chang, P., … Bett, K. E. (2016). Lens culinaris CDC Redberry Genome Assembly v1.2. Retrieved from https://knowpulse.usask.ca/genome-assembly/Lc1.2.
- Ramsay, L., Koh, C., Konkin, D., Cook, D., Penmetsa, V., Dongying, G., … Bett, K. E. (2019a). Lens culinaris CDC Redberry Genome Assembly v2.0. Retrieved from https://knowpulse.usask.ca/genome-assembly/Lcu.2RBY.
- Ramsay, L., Koh, K., Konkin, D., Stonehouse, R., Banniza, S. & Bett, K. E. (2019b). Lens ervoides IG 72815 Genome Assembly 1.0. Retrieved from https://knowpulse.usask.ca/genome-assembly/Ler.1DRT.
- Ripple, W. J., Wolf, C., Newsome, T. M., Barnard, P., & Moomaw, W. R. (2019). World scientists’ warning of a climate emergency. BioScience , 70, 8–12. doi: 10.1093/biosci/biz088.
- Rodda, M. S., Davidson, J., Javid, M., Sudheesh, S., Blake, S., Forster, J. W., & Kaur, S. (2017). Molecular breeding for ascochyta blight resistance in lentil: Current progress and future directions. Frontiers in Plant Science , 8, 1136. doi: 10.3389/fpls.2017.01136.
- Rubeena, R., Ford, R., & Taylor, P. W. J. (2003). Construction of an intraspecific linkage map of lentil (Lens culinaris ssp. culinaris). Theoretical and Applied Genetics , 107(5), 910–916. doi: 10.1007/s00122-003-1326-9.
- Saha, G. C., Sarker, A., Chen, W., Vandemark, G. J., & Muehlbauer, F. J. (2010a). Identification of markers associated with genes for rust resistance in Lens culinaris Medik. Euphytica , 175(2), 261–265. doi: 10.1007/s10681-010-0187-y.
- Saha, G. C., Sarker, A., Chen, W., Vandemark, G. J., & Muehlbauer, F. J. (2010b). Inheritance and linkage map positions of genes conferring resistance to stemphylium blight in lentil. Crop Science , 50(5), 1831–1839. doi: 10.2135/cropsci2009.12.0709.
- Sari, E., Bhadauria, V., Ramsay, L., Hossein Borhan, M., Lichtenzveig, J., Bett, K. E., … Banniza, S. (2018). Defense responses of lentil (Lens culinaris) genotypes carrying non-allelic ascochyta blight resistance genes to Ascochyta lentis infection. PLoS One , 13(9), e0204124. doi: 10.1371/journal.pone.0204124.
- Seyedimoradi, H., & Talebi, R. (2014). Detecting DNA polymorphism and genetic diversity in Lentil (Lens culinaris Medik.) germplasm: Comparison of ISSR and DAMD marker. Physiology and Molecular Biology of Plants , 20(4), 495–500. doi: 10.1007/s12298-014-0253-3.
- Sharma, S. R., Singh, S., Aggarwal, N., Kaur, J., Gill, R. K., Kushwah, A., … Kumar, S. (2018). Genetic variation for tolerance to post-emergence herbicide, imazethapyr in lentil (Lens culinaris Medik.). Archives of Agronomy and Soil Science , 64(13), 1818–1830. doi: 10.1080/03650340.2018.1463519.
- Sharpe, A. G., Ramsay, L., Sanderson, L.-A., Fedoruk, M. J., Clarke, W. E., Li, R., … Bett, K. E. (2013). Ancient orphan crop joins modern era: Gene-based SNP discovery and mapping in lentil. BMC Genomics , 14(1), 1–13. doi: 10.1186/1471-2164-14-192.
- Singh, D., Singh, C. K., Taunk, J., Jadon, V., Pal, M., & Gaikwad, K. (2019). Genome-wide transcriptome analysis reveals vital role of heat responsive genes in regulatory mechanisms of lentil (Lens culinaris Medikus). Scientific Reports , 9(1), 1–19. doi: 10.1038/s41598-019-49496-0.
- Singh, D., Singh, C. K., Taunk, J., Tomar, R. S. S., Chaturvedi, A. K., Gaikwad, K., & Pal, M. (2017). Transcriptome analysis of lentil (Lens culinaris Medikus) in response to seedling drought stress. BMC Genomics , 18(1), 206. doi: 10.1186/s12864-017-3596-7.
- Singh, M., Bisht, I. S., Dutta, M., Kumar, K., Kumar, S., & Bansal, K. C. (2014). Genetic studies on morpho-phenological traits in lentil (Lens culinaris Medikus) wide crosses. Journal of Genetics , 93(2), 561–566. doi: 10.1007/s12041-014-0409-5.
- Singh, M., Bisht, I. S., Kumar, S., Dutta, M., Bansal, K. C., Karale, M., … Datta, S. K. (2014). Global wild annual Lens collection: A potential resource for lentil genetic base broadening and yield enhancement. PLoS One , 9(9), e107781. doi: 10.1371/journal.pone.0107781.
- Singh, M., Rana, M. K., Kumar, K., Bisht, I. S., Dutta, M., Gautam, N. K., … Bansal, K. C. (2013). Broadening the genetic base of lentil cultivars through inter-sub-specific and interspecific crosses of Lens taxa. Plant Breeding , 132(6), 667–675. doi: 10.1111/pbr.12089.
- Singh, M., Sharma, S. K., Singh, B., Malhotra, N., Chandora, R., Sarker, A., … Gupta, D. (2018). Widening the genetic base of cultivated gene pool following introgression from wild Lens taxa. Plant Breeding , 137(4), 470–485. doi: 10.1111/pbr.12615.
- Sita, K., Sehgal, A., Kumar, J., Kumar, S., Singh, S., Siddique, K. H. M., & Nayyar, H. (2017). Identification of high-temperature tolerant lentil (Lens culinaris Medik.) genotypes through leaf and pollen traits. Frontiers in Plant Science , 8, 744. doi: 10.3389/fpls.2017.00744.
- Sonnante, G., Hammer, K., & Pignone, D. (2009). From the cradle of agriculture a handful of lentils: History of domestication. Rendiconti Lincei. Scienze Fisiche E Naturali , 20(1), 21–37. doi: 10.1007/s12210-009-0002-7.
- Sonnante, G., Montemurro, C., Morgese, A., Sabetta, W., Blanco, A., & Pasqualone, A. (2009). DNA microsatellite region for a reliable quantification of soft wheat adulteration in durum wheat-based foodstuffs by real-time PCR. Journal of Agricultural and Food Chemistry , 57(21), 10199–10204. doi: 10.1021/jf902624z.
- Sudheesh, S., Rodda, M. S., Davidson, J., Javid, M., Stephens, A., Slater, A. T., … Kaur, S. (2016). SNP-based linkage mapping for validation of QTLs for resistance to ascochyta blight in lentil. Frontiers in Plant Science , 7, 1604. doi: 10.3389/fpls.2016.01604.
- Sudheesh, S., Verma, P., Forster, J. W., Cogan, N. O. I., & Kaur, S. (2016). Generation and characterisation of a reference transcriptome for lentil (Lens culinaris Medik.). International Journal of Molecular Sciences , 17(11), 1887. doi: 10.3390/ijms17111887.
- Sultana, T., & Ghafoor, A. (2008). Genetic diversity in ex-situ conserved lens culinaris for botanical descriptors, biochemical and molecular markers and identification of landraces from indigenous genetic resources of Pakistan. Journal of Integrative Plant Biology , 50(4), 484–490. doi: 10.1111/j.1744-7909.2007.00632.x.
- Tahir, M., & Muehlbauer, F. J. (1994). Gene mapping in lentil with recombinant inbred lines. Journal of Heredity , 85(4), 306–310. doi: 10.1093/oxfordjournals.jhered.a111464.
- Tar'an, B., Buchwaldt, L., Tullu, A., Banniza, S., Warkentin, T. D., & Vandenberg, A. (2003). Using molecular markers to pyramid genes for resistance to ascochyta blight and anthracnose in lentil (Lens culinaris Medik). Euphytica , 134(2), 223–230. doi: 10.1023/b:eUph.0000003913.39616.fd.
- Taylor, P. W. J., Ades, P. K., & Ford, R. (2006). QTL mapping of resistance in lentil (Lens culinaris ssp. culinaris) to ascochyta blight (Ascochyta lentis). Plant Breeding , 125(5), 506–512. doi: 10.1111/j.1439-0523.2006.01259.x.
- Temel, H. Y., Deniz, G. Ö. L., Akkale, H. B. K., Kahri̇man, A., & Tanyolaç, M. B. (2015). Single nucleotide polymorphism discovery through Illumina-based transcriptome sequencing and mapping in lentil. Turkish Journal of Agriculture and Forestry , 39, 470–488. doi: 10.3906/tar-1409-70.
- Tullu, A., Banniza, S., Tar'an, B., Warkentin, T., & Vandenberg, A. (2010). Sources of resistance to ascochyta blight in wild species of lentil (Lens culinaris Medik.). Genetic Resources and Crop Evolution , 57, 1053–1063. doi: 10.1077/s10722-010-9547-7.
- Tullu, A., Buchwaldt, L., Lulsdorf, M., Banniza, S., Barlow, B., Slinkard, A. E., … Vandenberg, A. (2006). Sources of resistance to anthracnose (Colletotrichum truncatum) in wild Lens species. Genetic Resources and Crop Evolution , 53, 111–119. doi: 10.1007/s10722-004-1586-5.
- Tullu, A., Buchwaldt, L., Warkentin, T., Taran, B., & Vandenberg, A. (2003). Genetics of resistance to anthracnose and identification of AFLP and RAPD markers linked to the resistance gene in PI 320937 germplasm of lentil (Lens culinaris Medikus). Theoretical and Applied Genetics , 106(3), 428–434. doi: 10.1007/s00122-002-1042-x.
- Tullu, A., Tar'an, B., Breitkreutz, C., Banniza, S., Warkentin, T. D., Vandenberg, A., & Buckwaldt, L., (2006). A quantitative-trait locus for resistance to ascochyta blight [Ascochyta lentis] maps close to a gene for resistance to anthracose [Colletotrichum truncatum] in lentil. Canadian Journal of Plant Pathology , 28, 588–595. doi: 10.1080/07060660609507337.
- Tullu, A., Tar'an, B., Warkentin, T., & Vandenberg, A. (2008). Construction of an intraspecific linkage map and QTL analysis for earliness and plant height in lentil. Crop Science , 48(6), 2254–2264. doi: 10.2135/cropsci2007.11.0628.
- Vail, S., Strelioff, J. V., Tullu, A., & Vandenberg, A. (2012). Field evaluation of resistance to Colletotrichum truncatum in Lens culinaris , Lens ervoides , and Lens ervoides × Lens culinaris derivatives. Field Crops Research , 126, 145–151. doi: 10.1016/j.fcr.2011.10.002.
- Vail, S., & Vandenberg, A. (2011). Genetic control of interspecific-derived and juvenile resistance in lentil to Colletotrichum truncatum. Crop Science , 51(4), 1481–1490. doi: 10.2135/cropsci2010.07.0436.
- Vaillancourt, R. E., & Slinkard, A. E. (1993). Linkage of morphological and isozyme loci in lentil, Lens culinaris L. Canadian Journal of Plant Science , 73(4), 917–926. doi: 10.4141/cjps93-122.
- Vandenberg, A., Banniza, S., Warkentin, T. D., Ife, S., Barlow, B., McHale, S., … Dueck, S. (2006). CDC redberry lentil. Canadian Journal of Plant Science , 86(2), 497–498. doi: 10.4141/p05-071.
- Vandenberg, A., & Slinkard, A. E. (1990). Genetics of seed coat color and pattern in lentil. Journal of Heredity , 81(6), 484–488. doi: 10.1093/oxfordjournals.jhered.a111030.
- Varshney, R. K., Terauchi, R., & McCouch, S. R. (2014). Harvesting the promising fruits of genomics: Applying genome sequencing technologies to crop breeding. PLoS Biology , 12(6), e1001883. doi: 10.1371/journal.pbio.1001883.
- Verma, P., Goyal, R., Chahota, R. K., Sharma, T. R., Abdin, M. Z., & Bhatia, S. (2015). Construction of a genetic linkage map and identification of QTLs for seed weight and seed size traits in lentil (Lens culinaris Medik.). PLoS ONE , 10(10), e0139666. doi: 10.1371/journal.pone.0139666.
- Vishnyakova, M. A. (2006). Elena Ivanovna Barulina—Ucenica, soratnica, zena Nikolaja Ivanovica Vavilova. Sel'skochozjajstvennaja biologija , 5, 108–123.
- Wong, M. M. L., Gujaria-Verma, N., Ramsay, L., Yuan, H. Y., Caron, C., Diapari, M., … Bett, K. E. (2015). Classification and characterization of species within the genus lens using genotyping-by-sequencing (GBS). PLoS One , 10(3), e0122025. doi: 10.1371/journal.pone.0122025.
- Wright, D. M., Neupane, S., Heidecker, T., Haile, T. A., Chan, C., Coyne, C. J., … Bett, K. E. (2020). Understanding photothermal interactions will help expand production range and increase genetic diversity of lentil (Lens culinaris Medik.). Plants, People, Planet , 3(2), 171–181. doi: 10.1002/ppp3.10158.
- Ye, G., McNeil, D. L., & Hill, G. D. (2000). Two major genes confer ascochyta blight resistance in Lens orientalis. New Zealand Plant Protection , 53, 109–113. doi: 10.30843/nzpp.2000.53.3622.
- Young, N. D., Mudge, J., & Ellis, T. H. N. (2003). Legume genomes: More than peas in a pod. Current Opinion in Plant Biology , 6(2), 199–204. doi: 10.1016/s1369-5266(03)00006-2.
- Zamir, D., & Ladizinsky, G. (1984). Genetics of allozyme variants and linkage groups in lentil. Euphytica , 33(2), 329–336. doi: 10.1007/bf00021129.
- Zhu, C., Gore, M., Buckler, E. S., & Yu, J. (2008). Status and prospects of association mapping in plants. Plant Genome , 1(1), 5–20. doi: 10.3835/plantgenome2008.02.0089.
Citing Literature
Number of times cited according to CrossRef: 10
- Vijay Rani Rajpal, Apekshita Singh, Renu Kathpalia, Rakesh Kr. Thakur, Mohd. Kamran Khan, Anamika Pandey, Mehmet Hamurcu, Soom Nath Raina, The Prospects of gene introgression from crop wild relatives into cultivated lentil for climate change mitigation, Frontiers in Plant Science, 10.3389/fpls.2023.1127239, 14 , (2023).
- Anirban Roy, Parmeshwar K. Sahu, Camellia Das, Somnath Bhattacharyya, Aamir Raina, Suvendu Mondal, Conventional and new-breeding technologies for improving disease resistance in lentil (Lens culinaris Medik), Frontiers in Plant Science, 10.3389/fpls.2022.1001682, 13 , (2023).
- Mustafa Bulut, Regina Wendenburg, Elena Bitocchi, Elisa Bellucci, Magdalena Kroc, Tania Gioia, Karolina Susek, Roberto Papa, Alisdair R. Fernie, Saleh Alseekh, A comprehensive metabolomics and lipidomics atlas for the legumes common bean, chickpea, lentil and lupin, The Plant Journal, 10.1111/tpj.16329, 116 , 4, (1152-1171), (2023).
- Francesca Brescia, Fabiano Sillo, Elisabetta Franchi, Ilaria Pietrini, Vincenzo Montesano, Giovanni Marino, Matthew Haworth, Elisa Zampieri, Danilo Fusini, Martino Schillaci, Roberto Papa, Chiara Santamarina, Federico Vita, Walter Chitarra, Luca Nerva, Giannantonio Petruzzelli, Carmelo Mennone, Mauro Centritto, Raffaella Balestrini, The ‘microbiome counterattack’: Insights on the soil and root‐associated microbiome in diverse chickpea and lentil genotypes after an erratic rainfall event, Environmental Microbiology Reports, 10.1111/1758-2229.13167, 15 , 6, (459-483), (2023).
- Jitendra Kumar, Tadesse S. Gela, Debjyoti S. Gupta, Anup Chandra, Hamid Khazaei, Recent Advances in Lentil Genetics, Genomics, and Molecular Breeding, Lentils, 10.1002/9781119866923.ch2, (25-43), (2023).
- Kuldeep Tripathi, Jyoti Kumari, Padmavati G. Gore, Dwijesh C. Mishra, Amit Kumar Singh, Gyan P. Mishra, C. Gayacharan, H. K. Dikshit, Neeta Singh, D. P. Semwal, Reena Mehra, Rakesh Bhardwaj, Ruchi Bansal, J. C. Rana, Ashok Kumar, Veena Gupta, Kuldeep Singh, Ashutosh Sarker, Agro-Morphological Characterization of Lentil Germplasm of Indian National Genebank and Development of a Core Set for Efficient Utilization in Lentil Improvement Programs, Frontiers in Plant Science, 10.3389/fpls.2021.751429, 12 , (2022).
- Azalea Guerra‐Garcia, Teketel Haile, Ezgi Ogutcen, Kirstin E. Bett, Eric J. Wettberg, An evolutionary look into the history of lentil reveals unexpected diversity, Evolutionary Applications, 10.1111/eva.13467, 15 , 8, (1313-1325), (2022).
- Lorenzo Rocchetti, Tania Gioia, Giuseppina Logozzo, Creola Brezeanu, Luis Guasch Pereira, Lucía De Rosa, Stefania Marzario, Alice Pieri, Alisdair R. Fernie, Saleh Alseekh, Karolina Susek, Douglas R. Cook, Rajeev K. Varshney, Shiv Kumar Agrawal, Aladdin Hamwieh, Elena Bitocchi, Roberto Papa, Towards the Development, Maintenance and Standardized Phenotypic Characterization of Single‐Seed‐Descent Genetic Resources for Chickpea, Current Protocols, 10.1002/cpz1.371, 2 , 2, (2022).
- Diego Rubiales, Paolo Annicchiarico, Maria Carlota Vaz Patto, Bernadette Julier, Legume Breeding for the Agroecological Transition of Global Agri-Food Systems: A European Perspective, Frontiers in Plant Science, 10.3389/fpls.2021.782574, 12 , (2021).
- Elisa Bellucci, Orlando Mario Aguilar, Saleh Alseekh, Kirstin Bett, Creola Brezeanu, Douglas Cook, Lucía De la Rosa, Massimo Delledonne, Denise F. Dostatny, Juan J. Ferreira, Valérie Geffroy, Sofia Ghitarrini, Magdalena Kroc, Shiv Kumar Agrawal, Giuseppina Logozzo, Mario Marino, Tristan Mary‐Huard, Phil McClean, Vladimir Meglič, Tamara Messer, Frédéric Muel, Laura Nanni, Kerstin Neumann, Filippo Servalli, Silvia Străjeru, Rajeev K. Varshney, Marta W. Vasconcelos, Massimo Zaccardelli, Aleksei Zavarzin, Elena Bitocchi, Emanuele Frontoni, Alisdair R. Fernie, Tania Gioia, Andreas Graner, Luis Guasch, Lena Prochnow, Markus Oppermann, Karolina Susek, Maud Tenaillon, Roberto Papa, The INCREASE project: Intelligent Collections of food‐legume genetic resources for European agrofood systems, The Plant Journal, 10.1111/tpj.15472, 108 , 3, (646-660), (2021).