Solid-Supported Membrane (SSM)-Based Electrophysiology Assays Using Surface Electrogenic Event Reader Technology (SURFE²R) in Early Drug Discovery
Antje Pommereau, Antje Pommereau, Thomas Licher, Thomas Licher, Felix Bärenz, Felix Bärenz
drug discovery
high-throughput screening
solid-supported membrane (SSM)-based electrophysiology
SURFE²R assay
Abstract
This article presents detailed descriptions of procedures and troubleshooting tips for solid-supported membrane (SSM)-based electrophysiology assays (SURFE²R) to measure electrogenic solute carrier transporter proteins (SLCs) and assess the effects of compounds that modulate their activity. SURFE²R allows the use of the standard 96-well format, making it an ideal platform for tertiary assays in a drug-discovery campaign. The assays are performed with cell-line-derived membrane fractions or proteoliposomes containing the transporter of interest. Three main protocols are described for the isolation of membrane fractions from cell culture and the generation of proteoliposomes containing the transporter of interest. Additionally, detailed protocols for SURFE²R single concentration and dose-response experiments are included to measure the potencies of test compounds in stimulating or inhibiting transporter function (EC50 or IC50 values, respectively) and kinetic functional assays to calculate apparent affinity (k M) and maximal velocity (V max) of substrate uptake. © 2023 Sanofi. Current Protocols published by Wiley Periodicals LLC.
This article was corrected on 23 March 2023. See the end of the full text for details.
PROTOCOL GROUP 1 : Sample preparation for SSM-based electrophysiology assays
Support Protocol 1 : Production of cell batches
Support Protocol 2 : Simple isolation of cell membranes
Alternate Protocol 1 : Isolation of cell membranes with sucrose gradient pre-purification
Support Protocol 3 : Production and isolation of liposomes
Support Protocol 4 : Preparation of sensor with isolated cell membranes
Alternate Protocol 2 : Preparation of sensor with isolated proteoliposomes
PROTOCOL GROUP 2 : Determination of assay parameters for SSM-based electrophysiology assay
Support Protocol 5 : Assay with stable buffer
Alternate Protocol 3 : Assay with ion gradient
Support Protocol 6 : Determination of membrane/liposome concentration
Support Protocol 7 : Determination of substrate dependency k M
PROTOCOL GROUP 3 : Determination of advanced assay parameters for SSM-based electrophysiology assays
Support Protocol 8 : Assessment of ion concentration dependency
Support Protocol 9 : Assessment of pH dependency
Support Protocol 10 : Assessment of DMSO dependency
Support Protocol 11 : Assessment of signal stability with multiple activations
PROTOCOL GROUP 4 : Compound testing through SSM-based electrophysiology assays using SURFE²R apparatus
Support Protocol 12 : Assessment of signal specificity of a published inhibitor or unknown compound(s)
Support Protocol 13 : Compound wash-out
Support Protocol 14 : Statistical analysis
INTRODUCTION
Cellular transporters are membrane-bound proteins that control the uptake or efflux of essential metabolites, compounds, and drugs such as carbohydrates, amino acids, nucleotides, and inorganic ions, into the cell or cellular organelles (International Transporter et al., 2010; Lin, Yee, Kim, & Giacomini, 2015; Pizzagalli et al., 2003). The transport mechanism by which the substrates are imported can be divided into passive and active transport processes (Lin et al., 2015). Passive transporters passage solutes across membranes down their electrochemical gradient, whereas active transporters create ion or solute gradients across membranes, utilizing energy-coupling mechanisms (Lin et al., 2015). Membrane-bound solute carrier transporter proteins (SLC transporters) are essential to cells, as they sustain cellular homeostasis by moving soluble molecules such as nutrients, drugs, and waste compounds across membranes (Casiraghi, Bensimon, & Superti-Furga, 2021; Hediger, Clemencon, Burrier, & Bruford, 2013; Hediger et al., 2004; International Transporter et al., 2010; Lin et al., 2015; Pizzagalli et al., 2003; Schlessinger, Yee, Sali, & Giacomini, 2013). More than 2000 of all human genes are transport related, and the SLC families represent a significant portion of these genes (Perland & Fredriksson, 2017): More than 300 different SLC human transporter genes exist and additional orphan SLC transporters are being identified and characterized constantly (Lin et al., 2015; Perland & Fredriksson, 2017; Schlessinger et al., 2013). Phylogenetic classification of SLC families has resulted in four major groups including 15 SLC families, which are clustered into four groups named α, β, δ, and γ (Perland & Fredriksson, 2017). SLC transporters are integral membrane proteins and contain hydrophobic transmembrane alpha helices connected to each other by hydrophilic intra- and extracellular loops. Some SLCs are only functional either as monomers or as obligate homo- or hetero-oligomers.
There remain few drugs targeting SLCs, as most SLCs are challenging drug targets due to a lack of appropriate tools, including specific assay technologies, appreciation of their redundant and integrated roles, and suitable chemical probes (Dvorak et al., 2021; International Transporter et al., 2010; Superti-Furga et al., 2020).The interest in SLC-oriented drug discovery is constantly increasing; however, one major challenge in this field is the sparse implementation of high-throughput screening (HTS) assays for drug-discovery campaigns (Fig. 1; Avram, Halip, Curpan, & Oprea, 2021; Dvorak et al., 2021; Garibsingh et al., 2018; Superti-Furga et al., 2020). This warrants the development of novel assays that circumvent the drawbacks commonly associated with label-based or cell-free screening assays (Dvorak et al., 2021; Superti-Furga et al., 2020). Ion transporter assay technologies that use labelled ligands and substrates suffer from limited sensitivity and robustness and high costs, but, on the other hand, they are ideal assay systems for HTS in the pharmaceutical industry (Fig. 1; Dunlop, Bowlby, Peri, Vasilyev, & Arias, 2008). Because of their simplicity, radioactive and fluorescence-based influx assays are still ideal primary assays (Fig. 2).
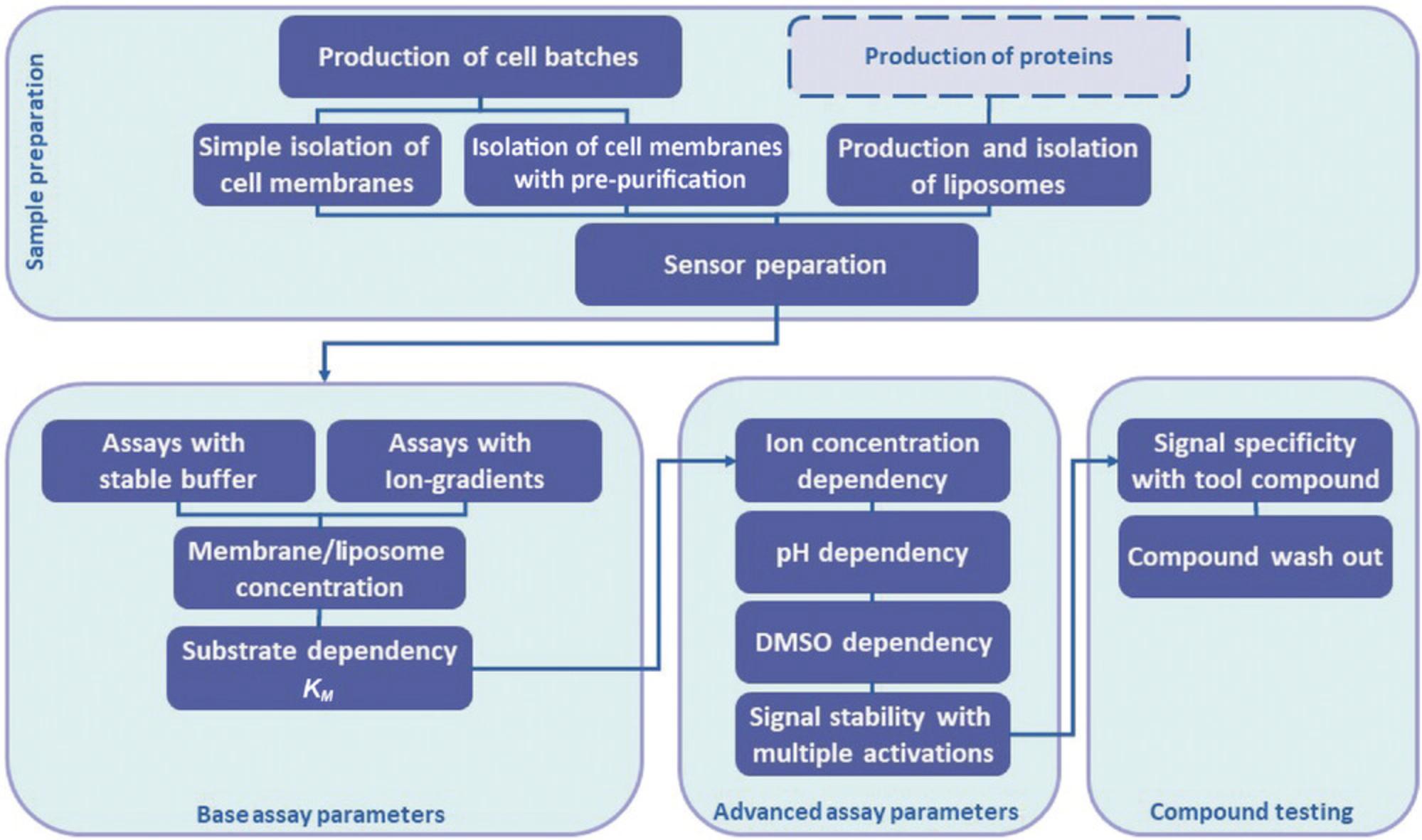
An ideal label-free and reliable method for the measurement of ion transporters is solid-supported membrane (SSM)-based electrophysiology, which was established in the late 1990s. SSM was especially developed for the measurement of transporters such as SLC membrane transport proteins, which are difficult to investigate using conventional electrophysiology (Bazzone & Barthmes, 2020; Bazzone, Barthmes, & Fendler, 2017; Bazzone et al., 2013; Dvorak et al., 2021; Nanion Technologies; Schulz, Garcia-Celma, & Fendler, 2008). The methodology differs from classical electrophysiology, such as patch-clamp methods, as no living cells are needed for analysis. SSM requires diverse native or artificial membrane vesicles such as reconstituted transporter protein in proteoliposomes or membrane preparations from organelles, cells, or tissue samples (Bazzone et al., 2013, 2017; Dvorak et al., 2021; Nanion Technologies, 2021; Schulz et al., 2008). SSM-based electrophysiology uses a membrane sample that is added to an SSM consisting of a lipid monolayer on top of a thiolated gold coated sensor chip, in advance of the experiment. This leads to the stable adsorption of the added membranes to the SSM and the formation of a capacitively coupled compound membrane (Bazzone & Barthmes, 2020; Bazzone et al., 2013, 2017; Dvorak et al., 2021; Nanion Technologies, 2021; Schulz et al., 2008). A substrate gradient established by fast solution exchange is the main driving force, and the transport of charged substrates or ions into the liposomes or vesicles generates a membrane potential (Bazzone & Barthmes, 2020; Bazzone et al., 2013, 2017; Dvorak et al., 2021; Nanion Technologies, 2021; Schulz et al., 2008). The potential can be detected via capacitive coupling between the membrane and the SSM on the gold layer of the sensor. As soon as the membrane potential equals the chemical driving force, the transport process comes to a halt and this is the reason why any current measured with SSM-based electrophysiology is transient, whereas the peak current amplitude reflects the transporter activity under steady-state conditions (Bazzone & Barthmes, 2020; Bazzone et al., 2013, 2017; Nanion Technologies, 2021). Transporters previously studied using SSM-based electrophysiology include symporters and exchangers and ATP-driven ion pumps. In addition, redox- and light-driven ion pumps, as well as receptors and ion channels, have been characterized.
The surface electrogenic event reader technology (SURFE²R; Nanion Technologies Munich) employs SSM-based electrophysiology and allows the measurement of up to 10 × 109 transporters at a time to yield the best signal-to-noise ratio (Bazzone & Barthmes, 2020; Dvorak et al., 2021; Nanion Technologies, 2021). Because SURFE²R allows the usage of the 96-well format, it is an ideal platform for secondary and tertiary assays in a drug-discovery campaign (Fig. 2; Dvorak et al., 2021; Gerbeth-Kreul et al., 2021).
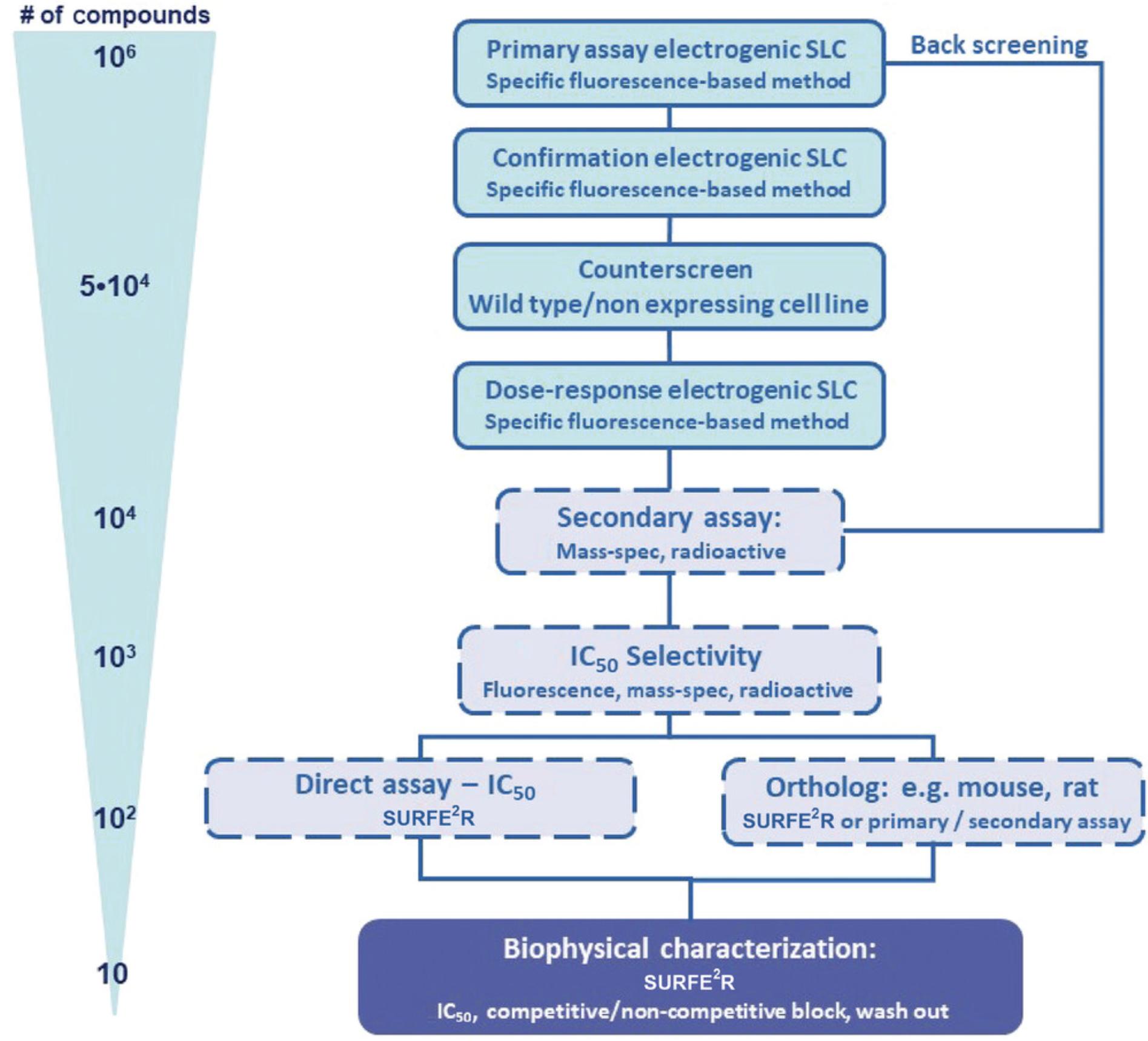
Described in this article are SURFE²R-based assay methods to pharmacologically characterize electrogenic SLCs in the context of drug discovery. Figure 1 gives an overview of the necessary steps during an assay development for the pharmacological characterization of an unknown solute carrier. The described methodology uses rather diverse native or artificial membrane vesicles such as reconstituted transporter protein in proteoliposomes or membrane preparations from organelles, cell, or tissue samples (Figs. 1 and 3). Therefore, the article contains protocols for the isolation of membrane fractions from cell culture and the generation of proteoliposomes containing the transporter of interest. Additionally, a detailed description of SURFE²R assays for single concentration, for dose response to measure potencies of test compounds for stimulation or inhibition of SLC transporter function (EC50 or IC50 values, respectively), as well as kinetic functional assays to calculate apparent affinity (k M) and maximal velocity (V max) of substrate uptake are presented (Fig. 1). Finally, an assay procedure for SURFE²R single concentration and dose-response assays to measure potencies of test compounds for stimulation or inhibition of transporter function (EC50 or IC50 values, respectively) is described (Fig. 1). Useful tips for troubleshooting experimental problems and optimization of critical factors that can affect the outcome of the results are provided. The final protocol deals with data handling and data evaluation.
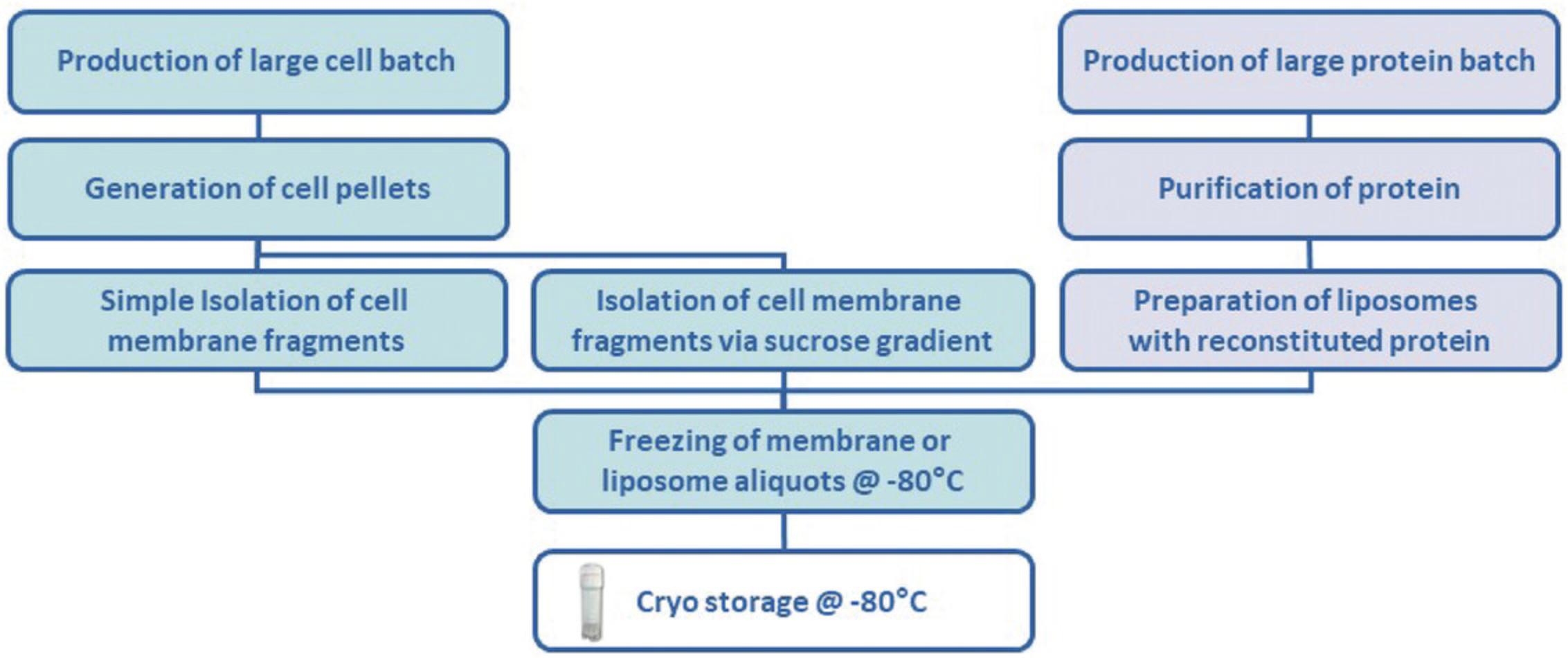
Protocol Group 1 comprises a set of sample preparation protocols including cell batch production, membrane isolation and proteoliposomes preparation (Figs. 1 and 3). Protocol Group 2 provides a set of protocols for setting up a SURFE²R drug-discovery assay for an uncharacterized electrogenic SLC (Figs. 1 and 4). Protocol Group 3 includes different protocols for setup using advanced assay parameters, and Protocol Group 4 provides protocols for testing compounds.
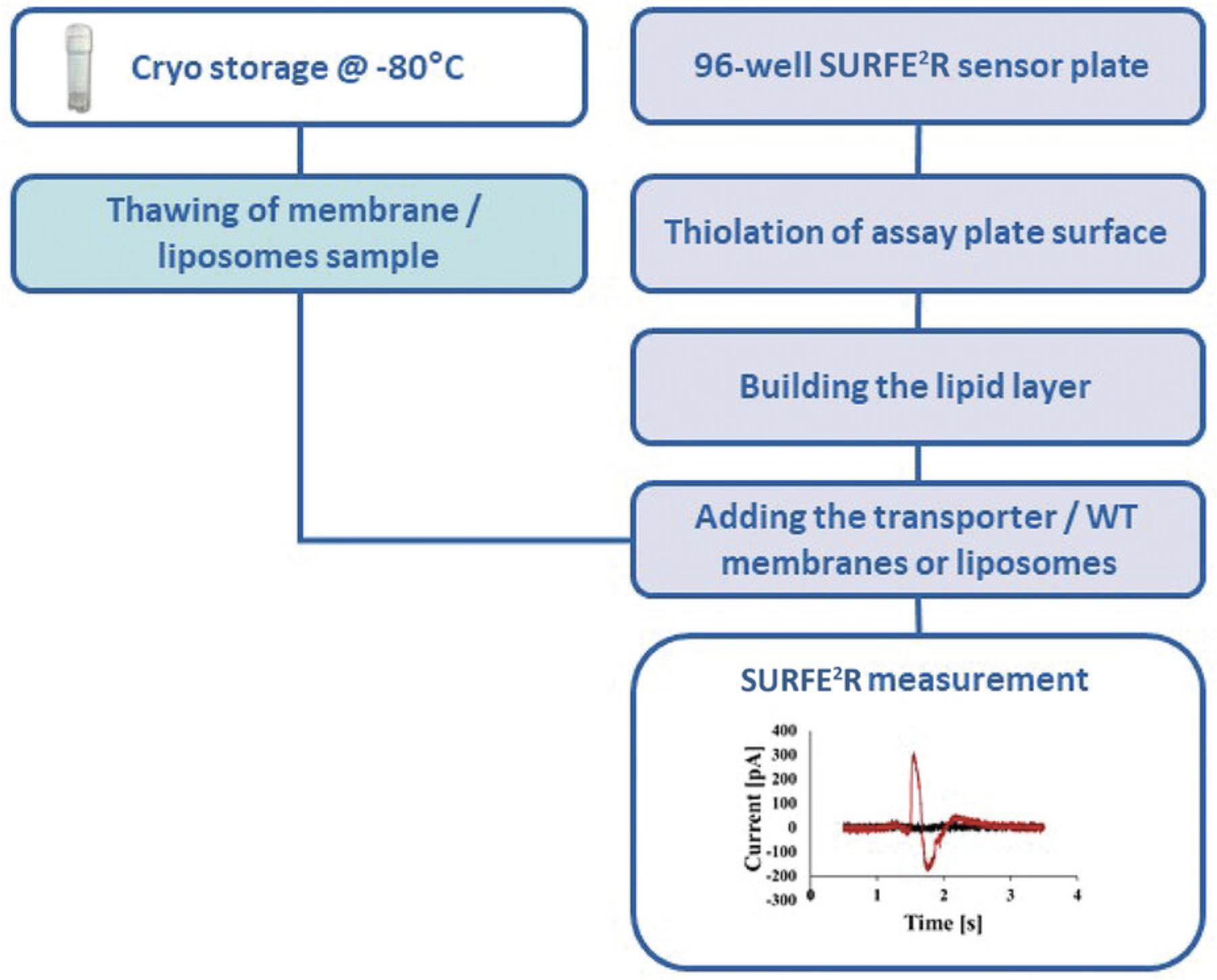
PROTOCOL GROUP 1: SAMPLE PREPARATION FOR SSM-BASED ELECTROPHYSIOLOGY ASSAYS
Protocol Group 1 includes Support Protocols 1-4 and Alternate Protocols 1 and 2, describing sample preparation methods.
Support Protocol 1: PRODUCTION OF CELL BATCHES
Recombinantly (over-) expressed SLC protein can generally be studied in bacteria and mammalian cell lines (Figs. 1 and 3). Support Protocol 1 describes the production of cell pellets from mammalian HEK293 (human embryonic kidney cells) and CHO (Chinese hamster ovary) cells overexpressing the desired SLC transporter. The SLC protein can be stably overexpressed in a mammalian cell line via a genomically stable TET-ON (tetracycline-controlled promoter) controlled cellular overexpression system or via transient plasmid-controlled overexpression.
Materials
-
Cell line (e.g., HEK293 human embryonic kidney cells or CHO Chinese hamster ovary cells) overexpressing the desired SLC transport, as frozen stock
-
Cell culture medium appropriate for cell line: either DMEM (high glucose, with GlutaMAX™ Supplement; Gibco cat. no. 61965026) or Ham's F-12 (with GlutaMAX™ Supplement; Gibco cat. no. 31765068), with 10% (v/v) fetal bovine serum (FCS; PAN cat. no. P30-3602) and prewarmed to 37°C
-
Dulbecco's phosphate-buffered saline, no calcium, no magnesium (DPBS; Gibco cat. no. 14190169)
-
Detachin cell detachment solution (Genlantis cat. no. T100100)
-
Greiner Bio-One™ Cellstar™ flask (cat. no. 660175)
-
Incubator: Heraeus BBD6220
-
Centrifuge: Hereaus Multifuge 3SR+ with Rotor XY with Sorvall rotor 75006445
Cell culture
1.Thaw the frozen cell stock and add 30 ml prewarmed cell culture medium to a Greiner flask. Allow cells to grow for 24 hr (longer if required) in an incubator at 37°C, 7.5% CO2, and 95% relative humidity until they reach 80%-90 % confluency.
2.Split the cells into two fresh new cell flasks (all solutions must be prewarmed to 37°C):
-
Remove medium.
-
Add 10 ml DPBS, swirl over the cell layer, and remove medium.
-
Add 3 ml Detachin, swirl over the cell layer, and place the cell tube in the incubator for 2 min.
-
Add 10 ml fresh cell culture medium.
-
Resuspend the cells in medium.
-
Transfer 6.5 ml of the cell suspension into each of two fresh Greiner flasks.
-
Add 23.5 ml medium to each flask obtain a final volume of 30 ml.
-
Place the flasks in the incubator for 24 hr.
Repeat this procedure until you have ∼100 Greiner flasks to harvest 25 × 108 to 35 × 108 cells.
Cell pellets
3.Detach the cells (from steps 1 and 2) and resuspend them in medium in a Greiner cell culture flask. All solutions must be prewarmed to 37°C.
-
Remove medium.
-
Add 10 ml DPBS, swing the flask back and forth once, and again remove the supernatant by suction.
-
Add 3 ml Detachin, swing the flask back and forth once, and place it in the incubator for 2 min.
-
Add 10 ml medium.
-
Resuspend the cells in the medium.
-
Pool the cell suspension in 50-ml tubes
-
Centrifuge 4 min at 400 ×g, room temperature.
-
Remove supernatant by suction.
-
Pool the pellets in 50-100 ml DPBS.
-
Divide the suspension into aliquots in 50-ml tubes.
-
Centrifuge for 15 min at 400 ×g, 4°C.
-
Discard the supernatant.
-
Freeze the pellets at −80°C.
The pellets are in the 50-ml tube in which the cells were pooled. The total amount of cells, in all 50-ml tubes, is between 12 × 108and 25 × 108.
Support Protocol 2: SIMPLE ISOLATION OF CELL MEMBRANES
Support Protocol 2 describes the simple isolation of crude cell membranes from HEK293 or CHO cell pellets (Support Protocol 1) using an ultracentrifugation protocol (Figs. 1 and 3). The generation of cell membrane fractions is based on ultracentrifugation of crude cell lysates. Depending on the required purity of the membrane fraction and the desired subcellular membrane fraction, a simple ultracentrifugation or a sucrose-gradient ultracentrifugation protocol can be used. Support Protocol 2 describes the simple ultracentrifugation procedure to generate a membrane fraction, but if the isolated fraction generates artefacts in the assay or the signal-to-noise ratio is insufficient, a sucrose-gradient-based membrane isolation procedure can help to generate a membrane fraction of higher purity, resulting in less background effects and higher signal amplitudes (Alternate Protocol 1).
NOTE : Use pre-cooled equipment and work on ice throughout the preparation.
Materials
-
Cell pellets from Support Protocol 1, step 3m
-
Tris buffer 1 (see recipe)
-
Tris cOmplete buffer (see recipe)
-
Homogenizer: Minilys Personal Homogenizer (Bertin Technologies)
-
Homogenizer tubes: 1.4-mm ceramic beads in 7-ml standard tubes (Bertin Technologies cat. no. P000940-LYSK0-A)
-
Centrifuge: Hereaus Multifuge 3SR+ with Rotor XY with Sorvall rotor 75006445
-
High-speed centrifuge: Kendro Sorvall Discovery 90SE with rotor SW-28
-
High-speed polycarbonate round-bottom centrifuge tubes (Nalgene cat. no. 3117-0380)
-
Spectrophotometer: Thermo NanoDrop ND-1000
1.Thaw the cell pellets (normally 12 × 108 to 25 × 108 cells) quickly in your hand.
2.Add 5 ml Tris buffer 1 and resuspend the cells.
3.Combine the cell suspension of the individual tubes and add ∼5.5 ml of the suspension to each homogenization tube.
4.Homogenize with the Minilys homogenizer for 5 sec (speed: step 2).
5.Pool the homogenized cells in 15-ml tubes.
6.Centrifuge 10 min at 300 × g , 4°C.
7.Remove the supernatant and transfer it to a high-speed centrifuge tube.
8.Discard the pellet.
9.Centrifuge the supernatants for 90 min at 48,000 × g , 4°C.
10.Discard the supernatant.
11.Add 10 ml Tris buffer 1 to the pellet.
12.Resuspend the pellets and homogenize again as in steps 3 and 4.
13.Pool the homogenized cells in a high-speed centrifuge tube.
14.Centrifuge again for 120 min at 48,000 × g , 4°C.
15.Discard supernatant.
16.Add 5 ml Tris cOmplete buffer to the pellet.
17.Resuspend the pellets and homogenize again.
18.Determinate the total protein concentration of the membrane suspension with NanoDrop spectrophotometer (based on the absorbance at 280 nm, A 280).
19.Freeze aliquots 20-60 µl in size at −80°C.
20.Store at −80°C.
Alternate Protocol 1: ISOLATION OF CELL MEMBRANES WITH SUCROSE GRADIENT PRE-PURIFICATION
Alternate Protocol 1 describes the isolation of membranes derived from HEK293 or CHO cells using a sucrose gradient ultracentrifugation procedure (Figs. 1 and 3). The sucrose gradient protocol for membrane isolation should be applied in the case of low assay signals or high background by using membranes prepared by a standard ultracentrifugation protocol. Membrane isolation based on sucrose gradient centrifugation generates membrane fractions of higher purity, significantly decreasing cross-contamination from other subcellular fractions.
Materials
-
Cell pellets from Support Protocol 1, step 3m
-
Homogenization buffer (see recipe)
-
Tris buffer 2 (see recipe)
-
Sucrose gradient buffers: 70%, 45%, 31%, and 9% (w/v; see recipe)
-
Storage buffer without additives (see recipe)
-
Storage buffer with glycerol and DTT (see recipe)
-
Homogenizer: Minilys Personal Homogenizer (Bertin Technologies)
-
Homogenizer Tube: 1.4-mm ceramic beads in 7-ml standard tubes (Bertin Technologies cat. no. P000940-LYSK0-A)
-
High-speed centrifuge: Kendro Sorvall Discovery 90SE with rotor SW-28
-
High-speed polycarbonate round-bottom centrifuge tubes (Nalgene cat. no. 3117-0380)
-
Spectrophotometer: Thermo NanoDrop ND-1000
Homogenization and lysis of frozen cell pellets
1.Starting with a 5-ml frozen cell pellet, resuspend the pellet with 10 ml homogenization buffer.
2.Combine the cell suspension and add ∼5.5 ml of the suspension to each homogenization tube.
3.Homogenize with a Minilys homogenizer (5 s, speed: step 2).
4.Pool the homogenized cells in a high-speed tube.
5.Centrifuge for 10 min at 6000 × g , 4°C.
6.Transfer supernatant into centrifuge tubes; discard the pellet.
7.Centrifuge the supernatant for 30 min at 100,000 × g , 4°C.
Sucrose gradient centrifugation
8.Discard the supernatant.
9.Resuspend the pellet in 1000 µl Tris buffer 2.
10.Transfer suspension in high-speed tubes (if you started with a 5-ml pellet, divide the suspension into two high-speed centrifuge tubes).
11.Measure the volume and add 70% sucrose to a final concentration of 51% sucrose.
12.Mix well, but avoid air bubbles.
13.Carefully add the sucrose solutions layer by layer, being careful not to mix the different layers:
-
8 ml of 45% sucrose;
-
8 ml of 31% sucrose;
-
5 ml of 9% sucrose.
14.Centrifuge 3 hr at 100,000 × g , 4°C.
15.Aspirate bands between the 9% and 31% sucrose layers and between the 31% and 45% sucrose layers with a Pasteur pipette, and transfer each band to a fresh centrifuge tube (if possible, without any sucrose solution).
16.Fill up to at least 3-5 times the volume with storage buffer (without additives), mix well, and centrifuge 30 min at 100,000 × g , 4°C.
17.Resuspend pellet carefully in 500-1000 µl storage buffer with glycerol and DTT.
18.Mix well, but avoid air bubbles.
19.If necessary, add more buffer until the suspension is viscous and homogeneous.
20.Determinate the total protein concentration of the membrane suspension by measuring the absorbance at 280 nm (A 280). Before freezing, the isolated membrane stock has to be diluted to a concentration of 5 mg/ml. The yield varies greatly from transporter to transporter. In most cases the overall amount is between 2.0 and 4.0 ml yield for the 31%/45% fraction and 0.1-0.2 ml yield for the 9%/31% fraction.
21.Freeze 20-50 µl aliquots at −80°C.
22.Store at −80°C.
Support Protocol 3: PRODUCTION AND ISOLATION OF LIPOSOMES
Proteoliposomes are artificial lipid membranes (liposomes with a reconstituted protein). Proteoliposomes have a major advantage as compared with natural membrane systems because they can be obtained with fewer components, thereby allowing a more direct measurement of the activity of the protein of interest with a lower background. On the other hand, they have the disadvantages of requiring methodological standardization for incorporation of each specific protein, as well as verification that the reconstitution procedure has yielded the correct orientation of the protein in the proteoliposomes. Support Protocol 3 describes the isolation of liposomes for SURFE²R assay (Figs. 1 and 3).
Materials
-
E. Coli POLAR Lipid (Avanti Lipids cat. no. 100600P)
-
Chloroform (Sigma cat. no. 372978)
-
Hydration buffer with DTT (see recipe)
-
Protein to be tested (we always use our own protein preparations, but this also could be commercially obtained)
-
Octyl β-D-glucopyranoside (n -octylglucoside; Sigma cat. no. O8001)
-
Bio-Beads SM-2 Adsorbent Media (Bio-Rad cat. no. 152-3920)
-
Reconstitution buffer with DTT (see recipe)
-
Vacuum concentrator: SpeedVac Thermo Fisher Scientific SPD121P
-
Shaker: IKA™ VXR Basic Vibrax™ Vortex Shaker Basic Vx2E
-
Extruder: LiposoFast Liposome Factory (Avestin; Sigma cat. no. Z373400)
-
400-nm and 100-nm membranes: LiposoFast Liposome Factory membrane (Sigma cat. nos. Z373435 and Z373419)
-
Centrifuge: Beckman TLX ultracentrifuge with rotor TLA-100.3
-
1.5-ml tubes for high-speed centrifugation with snap-on caps (Beckman cat. no. 357448)
Lipid film generation
1.Dissolve 10 mg E. Coli POLAR Lipid in 1 ml chloroform or 100 mg in 10 ml chloroform.
2.Transfer 1-ml aliquots into glass vials.
3.Evaporate the solvent in a SpeedVac.
4.Store the lipid film at −80°C up to 12 months.
Liposome constitution
5.Thaw 1 vial E. Coli POLAR Lipid film briefly in hand.
6.Add 1 ml hydration buffer with DTT to the lipid film (10 mg/ml lipid).
7.Shake for 90 min at 37°C.
8.Press the solution 15 times carefully through an extruder at room temperature using a 400-nm or 100-nm membrane.
9.Mix 750 µl lipid suspension (7.5 mg lipid) with 0.15 mg of the protein to be tested (protein-to-lipid ratio [PLR] 1:50).
10.Add 7.5 mg n -octylglucoside (1%) to the lipid/protein mix.
11.Add 225 mg Bio-Beads (30%).
12.Shake overnight at 4°C.
13.Centrifuge in high-speed tubes for 10 min at 17,000 × g , 4°C. Bio-Beads should deposit at the bottoms of the tubes.
14.Transfer the supernatant to another tube and add 225 mg fresh Bio-Beads.
15.Shake 60 min at 4°C.
16.Centrifuge again in high-speed tubes for 10 min at 17,000 × g , 4°C, to collect the Bio-Beads at the bottoms of the tubes.
17.Transfer the supernatant to a high-speed centrifuge tube and centrifuge 60 min at 130,000 × g , 4°C.
18.Discard the supernatant.
19.Resuspend the pellet in 1 ml of reconstitution buffer with DTT.
20.Transfer 50-μl aliquots of the liposome solution into separate microcentrifuge tubes.
21.Store the liposomes at −80°C.
Support Protocol 4: SENSOR PREPARATION WITH ISOLATED CELL MEMBRANES
Support Protocol 4 describes the sensor preparation for SURFE²R assay measurements (Fig. 4). At this step of the assay development, it's necessary to compare wild-type membrane fractions with a membrane fraction containing the overexpressed SLC of interest. The comparison provides information if the observed signals are SLC specific.
Materials
-
Thiol solution (see recipe)
-
Isopropanol (2-propanol; Fisher Scientific cat. no. P/7500/PC17)
-
Lipid solution (see recipe)
-
Assay buffer 1 or 2 (see recipes)Membranes of interest, depending on the transporter to be tested
-
Wild-type membranes, as control
-
Washer: Blue®Washer (BlueCatBio)
-
Centrifuge: Eppendorf 5810R with rotor A-4-81
-
Pipettor: Analytik Jena CyBio FeliX integrated in the SURFE²R 96SE
-
Ultrasonic processor: Dr. Hielscher UP50H
-
SURFE²R 96SE sensor plates (Nanion Technologies Munich cat. no. 182001)
NOTE : Use assay buffer 1 if you want to work with Support Protocol 5, or assay buffer 2 if you want to work with Alternate Protocol 3
Thiolation
1a. Fill the sensor wells with 50 µl thiol solution and incubate 2 hr at room temperature.
1b. Alternatively, fill the sensor wells with 100 µl thiol solution and incubate overnight at room temperature.
2.Remove the solution with BlueCatBio BlueWasher (program “gentle spin”).
3.Rinse the wells twice with 100 µl 2-propanol and remove the solution with BlueWasher.
4.Rinse the wells twice with 100 µl ddH2O and remove the solution with BlueWasher.
5.Leave the sensor resting for 10 min at room temperature to allow it to dry out completely.
Lipid layer
6.Apply 2 µl of the lipid solution to the surface of the thiolated sensor using the FeliX pipettor.
7.Immediately fill the sensor with 100 µl assay buffer using the FeliX pipettor.
8.The membrane now can be applied directly, or the lipid plate can be stored overnight at 4°C before proceeding.
Membranes
9.Use wild-type membranes and membranes with the transporter in the same concentrations.
10.Defrost the membrane aliquot in your hand quickly.
11.Dilute to the required concentration with assay buffer (normally start with a concentration of 1 mg/ml).
12.Sonicate 10 times using the ultrasonic processor at an amplitude of 20% and a 0.5-s cycle time ratio.
13.Add 5 µl of the membrane suspension to the sensor (the appropriate concentration will depend on assay conditions).
14.Centrifuge the sensor plate 30 min at 2000 × g , 7°C.
15.Perform Support Protocol 5 or Alternate Protocol 3 to measure the transport of the substrate.
Alternate Protocol 2: SENSOR PREPARATION WITH ISOLATED PROTEOLIPOSOMES
Alternate Protocol 2 describes the sensor preparation with isolated proteoliposomes for SURFE²R assay measurements (Fig. 4). At this step of the assay development, it's again necessary to compare empty liposomes with liposomes containing the purified SLC of interest. The comparison provides information if the observed signals are SLC specific.
Materials
-
1-Octadecanethiol (thiol; Sigma cat. no. O1858)
-
Lipid: 1,2-diphytanoyl-sn -glyero-3-phosphocholine (Avanti cat. no. 850356)
-
Isopropanol (2-propanol; Fisher Scientific cat. no. P/7500/PC17)
-
N -Decan (Sigma cat. no. D901)
-
Liposomes: depending on the protein to be tested
-
Liposomes: empty without protein (as control)
-
Thiol solution (see recipe)
-
Lipid solution (see recipe)
-
Assay buffer 1 or 2 (see recipes)
-
Washer: Blue®Washer (BlueCatBio)
-
Centrifuge: Eppendorf 5810R with rotor A-4-81
-
Pipettor: Analytik Jena CyBio FeliX integrated in the SURFE²R 96SE
-
Ultrasonic processor: Dr. Hielscher UP50H
-
SURFE²R 96SE sensor plates (Nanion Technologies Munich cat. no. 182001)
NOTE : Use assay buffer 1 if you want to work with Support Protocol 5, or assay buffer 2 if you want to work with Alternate Protocol 3
Thiolation
1a. Fill the sensor wells with 50 µl thiol solution and incubate 2 hr at room temperature.
1b. Alternatively, fill the sensor wells with 100 µl of the thiol solution and incubate overnight at room temperature.
2.Remove the solution with the BlueWasher (program “gentle spin”).
3.Rinse the wells two times with 100 µl 2-propanol and remove the solution with the BlueWasher.
4.Rinse the wells two times with 100 µl ddH2O and remove the solution with BlueWasher.
5.Leave the sensor resting for 10 min to dry out completely at room temperature.
Lipid layer
6.Apply 2 µl of the lipid solution to the surface of the thiolated sensor using the FeliX pipettor.
7.Immediately fill the sensor with 100 µl assay buffer using the FeliX pipettor.
8.The liposomes can now be applied directly, or the lipid plate can be stored overnight at 4°C before proceeding.
Liposomes
9.Use proteoliposomes with and without protein at the same concentration.
10.Defrost each liposome aliquot quickly in your hand.
11.Dilute to the required concentration with assay buffer (normally start with a concentration of 15 µg/ml).
12.Sonicate 10 times using the ultrasonic processor (amplitude of 20% and a 0.5-s cycle time ratio).
13.Add 5 µl of the liposome suspension to the sensor plate (concentration will depend on assay conditions).
14.Centrifuge the sensor plate 90 min at 2000 × g , 7°C.
15.Perform Support Protocol 5 or Alternate Protocol 3 to measure the transport of the substrate.
PROTOCOL GROUP 2: DETERMINATION OF ASSAY PARAMETERS FOR SSM-BASED ELECTROPHYSIOLOGY ASSAY
Protocol Group 2 includes Support Protocol 5-7 and Alternate Protocol 3, describing the measurement and determination of basic assay parameters.
Support Protocol 5: ASSAY WITH STABLE BUFFER
The assay development starts with a physiological buffer and uses the "single solution exchange" protocol of the SURFE²R apparatus (Nanion Technologies Munich; Figs. 1 and 4). The physiological buffer is used to attach the membrane or liposomes (non-activating buffer). For the first experiment, membranes are attached at a concentration of 5 µg/well and liposomes with a protein concentration of 100 ng/well, and in the following step a high concentration of substrate (0.5-1.0 mM in assay buffer = activation buffer) is used to test substrate transport. It is always important to additionally spot wild-type cell membrane or empty liposomes on the plate as a control and to test those under the same assay conditions. Some substrates or endogenous proteins generate artefacts on SURFE²R. If a control does not generate currents, you can be sure that the SLC currents are selective and specific due to the transport of the substrate. It is also essential to ensure that the assay buffer and activation buffer have the same composition, aside from the absence or presence of the substrate. Figure 5 provides schematic representation of a current trace recorded using a transporter of interest in the presence (red) or absence (black) of the corresponding substrate. The transporter is activated by a concentration jump of substrate that leads to an ion/substrate symport into the vesicles. Ion influx charges the vesicles; at the same time, the sensor also charges, and this charging current is measured and contains information about the kinetics of the transporter.
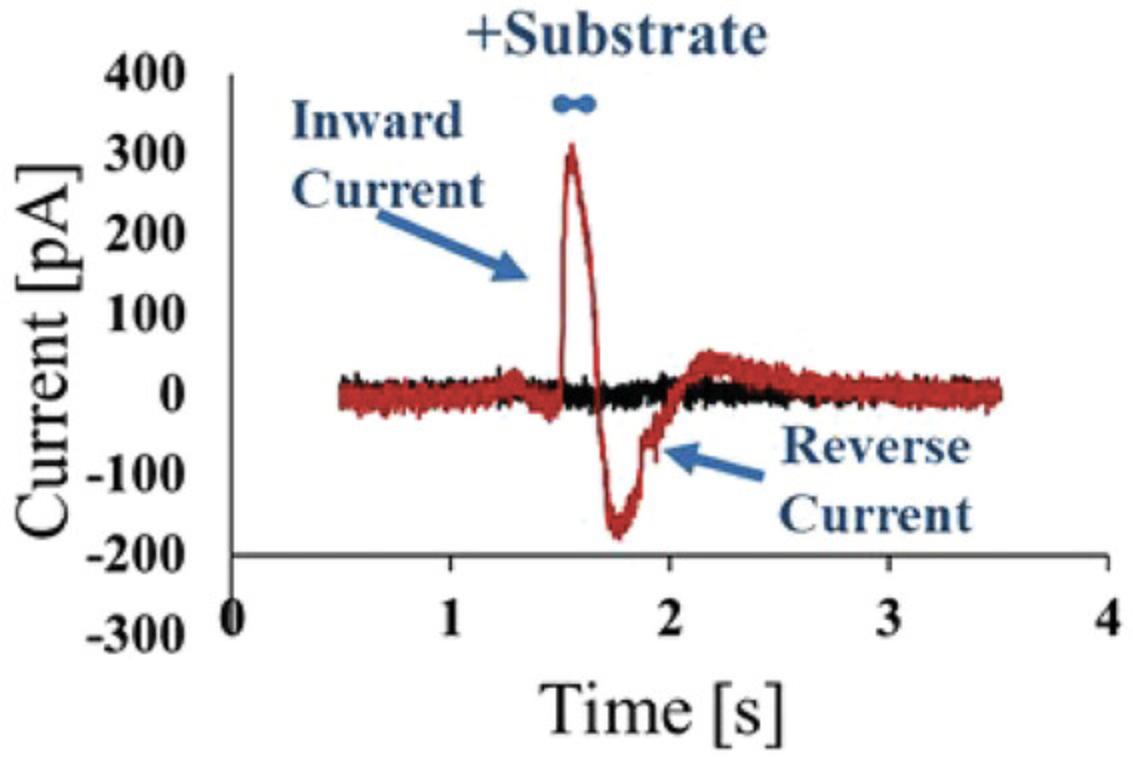
NOTE : The exact buffer composition must be adapted to the respective assay. Below, an assay for the transporter SLC6A19 is described as an example of the "Assay with stable buffer" protocol.
Materials
- Appropriate buffer and activation buffer depending on the cell line and assay
- Sensors to which thiol, lipid, and membranes (Support Protocol 4) or liposomes (Alternate Protocol 2) have already been attached on the gold surface
- Assay buffer 1 (see recipe)
- Activation buffer 1 (see recipe)
- SURFE²R 96SE apparatus (Nanion Technologies Munich)
1.Start with sensors to which thiol, lipid, and membranes or liposomes have already been attached on the gold surface (from Support Protocol 4 or Alternate Protocol 2).
2.For the first experimental measurements, perform 3-4 activations without substrate to prove baseline stability. The first activation without substrate is normally done to wash out the remaining storage buffer components. By the second activation without substrate, all residual attachment solutions will have been washed out, so from that point on the baseline will be mostly stable. When starting the assay development, add two more activations without substrate. In the following experiments, the number of activations without substrate is then adjusted to the necessary number.
3.Select the protocol "single solution exchange" on the 96SE, which comprises:
-
Activation with assay buffer (to rinse out the remaining solutions from the attachment);
-
Activation with assay buffer (as a control that no signal can be measured here);
-
Repetition of step b (to check that there really is no measurable signal);
-
Activation with activation buffer (measuring with substrate whether a signal is visible).
4.If a current is measurable and there is a difference from the wild-type cell or the empty liposomes, begin the next measurements for the determination of the membrane or liposome concentration.
Alternate Protocol 3: ASSAY WITH ION GRADIENT
For some assays, e.g., for the exchange of sodium and potassium, an ion gradient must be established. In these cases, the existing "double solution exchange" protocol from Nanion Technologies Munich is applied. The physiological buffer is used to attach the membrane or liposomes and later becomes the non-activating buffer. For the first experiment, membranes are spotted on the plate at a concentration of 5 µg/well and liposomes at a protein concentration of 100 ng/well. Afterwards, a high concentration of substrate (0.5-1.0 mM in assay buffer = activation buffer) is applied to test substrate transport. It is always important to spot wild-type cell membranes or empty liposomes on the plate as a control and to test the those under the same assay conditions. Some substrates generate artefacts on SURFE²R: If a control does not generate currents, you can be sure that the SLC currents are selective and specific due to the transport of the substrate. It is also essential to ensure that the assay buffer and activation buffer always have the same composition, aside from the presence or absence of the substrate.
NOTE : The exact buffer composition must be adapted to the respective assay. Below, an assay for the transporter SLC* is described as an example of the "double solution exchange without ion gradient" protocol.
Materials
-
Appropriate buffer and activation buffer for the cell line and assay
-
Assay buffer 2 (see recipe)
-
Control buffer (see recipe)
-
Activation buffer 2 (see recipe)
-
Sensors to which thiol, lipid, and membranes (Support Protocol 4) or liposomes (Alternate Protocol 2) have already been attached on the gold surface
-
SURFE²R 96SE apparatus (Nanion Technologies Munich)
1.Use sensors to which thiol, lipid, and membranes (Support Protocol 4) or liposomes (Alternate Protocol 2) have already been attached on the gold surface.
2.For the first experimental measurements, perform 3-4 activations without substrate need to prove baseline stability. The first activation without substrate is normally done to wash out the remaining storage buffer components. By the second activation without substrate, all residual attachment solutions have been washed out, so from that point on the baseline swill be mostly stable. When starting the assay development, add two more activations without substrate. In the following experiments, the number of activations without substrate is then adjusted to the necessary number
3.Select the protocol "double solution exchange" on the SURFE²R 96SE, which comprises:
-
Wash-out of the sensor with assay buffer (to rinse out the remaining solutions from the attachment);
-
Wash-out of the sensor with control buffer (to build the ion gradient);
-
Activation with control buffer (as a control that no signal can be measured here);
-
Washing of the sensor with assay buffer (to rinse out the control buffer);
-
Repetition of steps b and c (as a control that really no signal is measurable);
-
Wash-out of the sensor with assay buffer (to rinse out the control buffer);
-
Wash-out of the sensor with control buffer (to build the ion gradient);
-
Activation with activation buffer (measurement with substrate whether a signal is visible).
4.If a current is measurable and there is a difference from the wild-type cell or the empty liposomes, the next measurements for the determination of the membrane or liposome concentration can be initiated.
Support Protocol 6: DETERMINATION MEMBRANE OR LIPOSOME CONCENTRATION
After the establishment of stable and substrate-dependent signals, it is important to titrate the concentration of membranes or liposomes (Fig. 1). Either insufficient amounts or excessive amounts of membranes/liposomes can have negatively influence the signal quality. To achieve the best signal-to-noise ratio, it is advisable to perform a dilution series of the membranes/liposomes, which are attached to the plate and sequentially activated with high substrate concentration. The evaluation software (Data Control) supplied by Nanion Technologies Munich, the manufacturer of the manufacturer of the SURFE²R 96SE, can be used to determine the maximum peak or the integral of the signal. The data are plotted in a diagram, so that the optimum concentration can be determined (at the highest signal) and later experiments can then be carried out with this concentration.
Materials
-
Sensors to which thiol and lipid (Support Protocol 4) or liposomes (Alternate Protocol 2) have already been attached on the gold surface
-
Membrane, depending on the transporter to be tested
-
Proteoliposomes, depending on the transporter to be tested
-
Additional reagents and equipment for Support Protocol 4 or Alternate Protocol 2 and for Support Protocol 5 or Alternate Protocol 3
1.Use sensors to which thiol and lipid (Support Protocol 4) or liposomes (Alternate Protocol 2) have already been attached on the gold surface (from steps 1-8 of each protocol).
2.Attach membranes/liposomes as described in the Support Protocol 4/Alternate Protocol 2 (steps 9-15).
3.Use different membrane concentrations in a range from 0.1 to 2.5 mg/ml or liposome concentrations in a range from 3 to 30 µg/ml.
4.Perform step 3 of Support Protocol 5 or of Alternate Protocol 3.
5.Determine the area under the curve using the Data Control software.
6.Plot the individual values against each other.
Support Protocol 7: DETERMINATION OF SUBSTRATE DEPENDENCY kM
In transporter studies it is mandatory to determine enzyme/transporter kinetics. The Michaelis constant (k M) is defined as the substrate concentration at which the enzymatic reaction rate is half of its maximal value (V max). It indicates the affinity of an enzyme for a given substrate: The lower the k M, the higher the affinity of the enzyme for the substrate. Support Protocol 8 describes the determination of SLC transporter k M values using SURFE²R assay (Fig. 1).
There are two ways to determine the k M value, presented as Methods A and B below.
Materials
-
Sensors to which thiol and lipid (Support Protocol 4) or liposomes (Alternate Protocol 2) have already been attached on the gold surface
-
Membranes at a concentration depending on the result from Support Protocol 6
-
Proteoliposomes at a concentration depending on the result from Support Protocol 6
-
Additional reagents and equipment for Support Protocol 4 or Alternate Protocol 2 and for Support Protocol 5 or Alternate Protocol 3
Method A: kM determination in different wells
1a. Use sensors to which thiol and lipid (Support Protocol 4) or liposomes (Alternate Protocol 2) have already been attached on the gold surface (steps 1-8).
2a. Attach membranes/liposomes as described in the Support Protocol 4/Alternate Protocol 2 (steps 9-15).
3a. Use membrane concentration/liposome concentration determined in Support Protocol 6.
4a. Prepare 12 different concentrations of substrate in assay buffer (“single solution exchange”) or in control buffer (“double solution exchange”). By default, the maximum concentration is 10 mM, diluted 1:2 with the corresponding buffer to obtain a concentration of 5 mM. Thus, dilute the 5 mM substrate solution again 1:2 to obtain a final concentration of 2.5 mM. Repeat this dilution step 11 times to obtain a concentration series for the concentration range from 10 mM to 5 µM. At least 5 ml of each concentration must be prepared.
5a. Place the 12 different substrate solutions in a reservoir with 12 columns; this allows eight measurements for each substrate concentration.
6a. Perform step 3 of Support Protocol 5 or of Alternate Protocol 3
7a. Determine the area under the curve using the Data Control software.
8a. Average the area under the curve per concentration.
9a. Calculate the k M.
Method B: kM determination using cumulative concentrations per well
1b. Use sensors to which thiol and lipid (Support Protocol 4) or liposomes (Alternate Protocol 2) have already been attached on the gold surface (from steps 1-8 of each protocol).
2b. Attach membranes/liposomes as described in the Support Protocol 4/Alternate Protocol 2 (steps 9-15)
3b. Use membrane concentration/liposome concentration determined in Support Protocol 6.
4b. Via single solution exchange : Run the selected protocol "single solution exchange" on the 96SE, which comprises:
-
Washing of the sensor with assay buffer (to rinse out the remaining solutions from the attachment);
-
Activation with assay buffer (as a control that no signal can be measured here);
-
Repetition of step b (to check that there really is no measurable signal);
-
Activation of 8 or more sensors with activation buffer using the lowest substrate concentration;
-
Washing of the sensor with assay buffer (to rinse out the remaining activation buffer);
-
A pause included in the program on SURFE²R after washout, during which one fills the substrate template well with the next higher substrate concentration;
-
Repetition of steps d-f 11 more times, so that in the end all 12 concentrations have been tested in succession on one sensor.
The signals obtained from this are averaged for each concentration and the kMvalue is determined using the Michaelis-Menten constant.
4c. Via double solution exchange: Alternatively, run the selected protocol “double solution exchange” on the 96SE, which comprises:
-
Washing of the sensor with assay buffer (to rinse out the remaining solutions from the attachment);
-
Washing of the sensor with control buffer (to build the ion gradient);
-
Activation with control buffer (as a control that no signal can be measured here);
-
Washing of the sensor with assay buffer (to rinse out the control buffer);
-
Repetition of steps b and c (as a control that really no signal is measurable);
-
Washing of the sensor with assay buffer (to rinse out the control buffer);
-
Washing of the sensor with control buffer (to build the ion gradient);
-
Activation of 8 or more sensors with activation buffer using the lowest substrate concentration;
-
Washing of the sensor with assay buffer (to rinse out the remaining activation buffer);
-
A pause included in the program on SURFE²R after washout, during which one fills the substrate template well with the next higher substrate concentration
-
Repetition of steps of g-j 11 more times, so that in the end all 12 concentrations have been tested in succession on one sensor.
The signals obtained from this are averaged for each concentration and the kMvalue is determined using the Michaelis-Menten constant.
PROTOCOL GROUP 3: DETERMINATION OF ADVANCED ASSAY PARAMETERS FOR SSM-BASED ELECTROPHYSIOLOGY ASSAYS
Protocol Group 3 includes Support Protocols 8-11, describing the determination of advanced assay parameters.
Support Protocol 8: ASSESSMENT OF ION CONCENTRATION DEPENDENCY
Many SLC transporters are ion dependent, e.g., in regard to sodium for secondary transporters. If such a dependency exists, the cotransporter ion needs to be titrated to obtain the best signal-to-noise ratio (Fig. 1). It is essential to ensure that the total ion concentration in the assay buffer does not change. If, for example, the concentration of sodium chloride is titrated, the missing sodium chloride must be compensated for with choline chloride (example: 140 mM NaCl + 0 mM choline chloride; 80 mM NaCl + 40 mM choline chloride). Prepare for each of the different assay buffers the appropriate activation buffer with substrate.
Materials
-
Membranes at a concentration depending on the results of Support Protocol 6
-
Proteoliposomes at a concentration depending on the results of Support Protocol 6
-
Substrate at a concentration depending on the results of Support Protocol 7
-
Choline chloride buffer 1 or 2 (see recipes)
-
Sensors to which thiol and lipid (Support Protocol 4) or liposomes (Alternate Protocol 2) have already been attached on the gold surface
-
Additional reagents and equipment for Support Protocol 4 or Alternate Protocol 2 and for Support Protocol 5 or Alternate Protocol 3
1.Use sensors to which thiol and lipid (Support Protocol 4)/liposomes (Alternate Protocol 2) have already been attached on the gold surface (steps 1-8 of each protocol).
2.Attach membranes/liposomes as described in Support Protocol 4/Alternate Protocol 2 (steps 9-15).
3.Use membrane concentration/liposome concentration determined in Support Protocol 6.
Method A: single solution exchange without ion gradient
4a. Mix assay buffer 1 and choline chloride buffer 2 in different ratios to obtain 12 different buffers with different sodium/choline concentrations (e.g., 50% assay buffer 1 + 50% choline chloride buffer 1 = 70 mM sodium/70 choline). The range from 140 mM to 0 mM sodium chloride or choline chloride should be covered.
5a. Place the 12 different assay buffers in a reservoir with 12 columns; this allows eight measurements for each substrate concentration.
6a. Prepare the activation buffer with each of the 12 mixed assay buffers and place them in a 12-column reservoir too.
7a. Using the substrate concentration determined in Support Protocol 7, perform Support Protocol 5, step 3.
8a. Determine the area under the curve using the Data Control software.
9a. Plot the individual values against each other.
Method B: double solution exchange with ion gradient
4b. Mix control buffer and choline chloride buffer 2 in different ratios so that you get 12 different buffers with different sodium/choline concentrations (e.g., 50% assay buffer 1 + 50% choline chloride buffer 1 = 70 mM sodium/70 choline). The range from 140 mM to 0 mM sodium chloride or choline chloride should be covered.
5b. Place the 12 different control buffers in a reservoir with 12 columns; this allows eight measurements for each substrate concentration.
6b. Prepare the activation buffer with each of the 12 mixed control buffers and place them in a 12-column reservoir too.
7b. Using substrate concentration determined in Support Protocol 7, perform Alternate Protocol 3, step 3.
8b. Determine the area under the curve using Data Control.
9b. Plot the individual values against each other.
Support Protocol 9: ASSESSMENT OF pH DEPENDENCY
For SLC transporters that are pH dependent, the pH of the different buffers must be adjusted to different values and tested (range 5.5-8.0, Fig. 1). It is important that the assay buffer and the activation buffer, or the assay buffer, control buffer, and activation buffer, each have the same composition and pH with and without substrate. After the experiment has been carried out with the different pH values, the integral of the signals is plotted against the pH to determine the best signal.
Materials
-
Sensors to which thiol and lipid (Support Protocol 4)/liposomes (Alternate Protocol 2) have already been attached on the gold surface
-
Membranes at a concentration depending on the results of Support Protocol 6
-
Proteoliposomes at a concentration depending on the results of Support Protocol 6
-
Substrate at a concentration depending on the results of Support Protocol 7
-
Additional reagents and equipment for Support Protocol 4 or Alternate Protocol 2 (with buffer composition depending on the results of Support Protocol 8 if needed for an ion-dependent transporter) and for Support Protocol 5 or Alternate Protocol 3 (with buffer composition depending on the results of Support Protocol 8 if needed for an ion-dependent transporter)
1.Start with sensors to which thiol and lipid (Support Protocol 4) or liposomes (Alternate Protocol 2) have already been attached on the gold surface (from steps 1-8 of each protocol).
2.Attach membranes/liposomes as described in Support Protocol 4/Alternate Protocol 2 (steps 9-15).
3.Use membrane concentration/liposome concentration determined in Support Protocol 6.
4.Use the materials and buffers called for in Support Protocol 4 [5]/Alternate Protocol 2 [3] (use Support Protocol 8 for materials and buffers if needed for an ion-dependent transporter).
5.Prepare all buffers with different pH values: 5.5, 6.0, 6.5, 7.0, 7.5, and 8.0.
6.Apply the substrate concentration determined in Support Protocol 7.
7.Place the six different solutions with different pH in a reservoir with 6 columns; this allows 16 measurements for to be taken for each pH.
8.Perform step 3 of Support Protocol 5 or of Alternate Protocol 3
9.Determine the area under the curve using the Data Control software.
10.Average the area under the curve per pH.
11.Plot the individual pH values against AUC values.
Support Protocol 10: ASSESSMENT OF DMSO DEPENDENCY
Compounds applied to the transporter are usually dissolved in DMSO. The effect of different DMSO concentrations on the transport signal needs to be tested prior to the use of compounds (Fig. 1). For this purpose, DMSO is added to the buffers (0%-2%). After the measurement, the integral of the signal is plotted against the DMSO concentration, and the generated data indicate at what concentration DMSO interferes with the transporter signal. The concentration of DMSO with the minimal effect on the signal is selected for further experiments.
Materials
-
Sensors to which thiol and lipid (Support Protocol 4) or liposomes (Alternate Protocol 2) have already been attached on the gold surface
-
Membranes at a concentration depending on the result from Support Protocol 6
-
Proteoliposomes at a concentration depending on the result from Support Protocol 6
-
Substrate at a concentration depending on the result from Support Protocol 7
-
Dimethylsulfoxide (DMSO; Sigma cat. no. D2650)
-
Additional reagents and equipment for Support Protocol 4 or Alternate Protocol 2 (with buffer composition depending on the result from Support Protocol 8 if needed for an ion-dependent transporter, or Support Protocol 9 if needed for a pH-dependent transporter) and for Support Protocol 5 or Alternate Protocol 3 (with buffer composition depending on the result from Support Protocol 8 if needed for an ion-dependent transporter or Support Protocol 9 if needed for a pH-dependent transporter)
1.Use sensors to which thiol and lipid (Support Protocol 4) or liposomes (Alternate Protocol 2) have already been attached on the gold surface (steps 1-8 of each protocol).
2.Attach membranes/liposomes as described in Support Protocol 4/Alternate Protocol 2 (steps 9-15).
3.Set up the experiment as follows:
-
Use the membrane concentration/liposome concentration determined in Support Protocol6;
-
Use materials and buffers from Support Protocol4[5]/Alternate Protocol2[3] (using Support Protocol8for materials and buffers if needed for an ion-dependent transporter);
-
Use materials and buffers from Support Protocol4[5]/Alternate Protocol2[3] (using Support Protocol9for materials and buffers if needed for a pH-dependent transporter);
-
Add DMSO in different concentrations (0%-2%) to all buffers (e.g., 0.0, 0.1, 0.2, 0.4, 0.6, 0.8, 1.0, 1.2, 1.4, 1.6, 1.8, and 2.0%);
-
Use substrate concentration determined in Support Protocol7.
4.Place the 12 different solutions in a reservoir with 12 columns; this allows eight measurements to be taken for each DMSO concentration.
5.Run step 3 of Support Protocol 5 or of Alternate Protocol 3
6.Determine the area under the curve using the Data Control software.
7.Average the area under the curve per DMSO concentration.
8.Plot the observed values against each other.
Support Protocol 11: ASSESSMENT OF SIGNAL STABILITY WITH MULTIPLE ACTIVATIONS
During subsequent measurement of compounds, several activations are made per well during which the signal must be stable, as otherwise the compound effect cannot be discriminated from background (Figs. 1, 5, and 6). Therefore, it is necessary to check the stability of the signal. For this purpose, several activations are applied on each individual well and the reproducibility of the measured signal is tested. The deviation of the signals within a well should not be >8%. In general signals should be detectable and robust for >80% of the plate.

NOTE : It is imperative that the signal be stable over multiple activations. If this is not the case, measurement of inhibition of compounds is not possible.
Materials
-
Sensors to which thiol and lipid (Support Protocol 4) or liposomes (Alternate Protocol 2) have already been attached on the gold surface
-
Membranes at a concentration depending on the results of Support Protocol 6
-
Proteoliposomes at a concentration depending on the results of Support Protocol 6
-
Substrate at a concentration depending on the results of Support Protocol 7
-
Dimethylsulfoxide (DMSO; Sigma cat. no. D2650)
-
Additional reagents and equipment for Support Protocol 4 or Alternate Protocol 2 (with buffer composition depending on the results of Support Protocol 8 if needed for an ion-dependent transporter or Support Protocol 9 if needed for a pH-dependent transporter) and for Support Protocol 5 or Alternate Protocol 3 (with buffer composition depending on the results of Support Protocol 8 if needed for an ion-dependent transporter or Support Protocol 9 if needed for a pH-dependent transporter)
1.Take the previously prepared sensors to which thiol and lipid (Support Protocol 4) or liposomes (Alternate Protocol 2) have already been attached on the gold surface (from steps 1-8 of each protocol).
2.Attach membranes/liposomes according to Support Protocol 4/Alternate Protocol 2 (steps 9-15).
3.Apply the membrane or liposome concentration determined in Support Protocol 6.
4.Refer to materials and buffers from Support Protocol 4 [5]/Alternate Protocol 2 [3] (use Support Protocol 8 for materials and buffers if necessary for an ion-dependent transporter).
5.Take materials and buffers from Support Protocol 4 [5]/Alternate Protocol 2 [3] (use Support Protocol 9 for materials and buffers if necessary for a pH-dependent transporter).
6.Apply DMSO concentration determined in Support Protocol 10
7.Take the substrate concentration determined in Support Protocol 7.
8.Perform step 3 of Support Protocol 5 or of Alternate Protocol 3
9.Activate 6 times with activation buffer to check if the signal on each sensor is stable.
10.Determine the area under the curve using the Data Control software.
11.Compare activation (+ activation buffer) 3-5; the deviation between the individual measurements should be <8%.
PROTOCOL GROUP 4: COMPOUND TESTING THROUGH SSM-BASED ELECTROPHYSIOLOGY ASSAYS USING SURFE2R APPARATUS
Protocol Group 4 includes Support Protocols 12 and 13, describing the measurement of compound effects, and Support Protocol 14, describing the statistical analysis of data generated using these protocols.
Support Protocol 12: ASSESSMENT OF SIGNAL SPECIFICITY OF A PUBLISHED INHIBITOR OR UNKNOWN COMPOUND
Inhibitors of various transporters have already been published in literature (Gerbeth-Kreul et al., 2021). If this is the case for the transporter of interest, specific legacy inhibitors are available which can be tested at the end of the assay development to proof assay specificity (Figs. 1 and 7). If legacy compounds show reproducible activation or inhibition of the transport activity, depending on their published mode of action, proofs that the assay signals are reflecting a SLC specific transport of the substrate and no artifacts. To perform this proof-of-concept experiment with legacy compounds, proceed as described in Support Protocol 12 below.
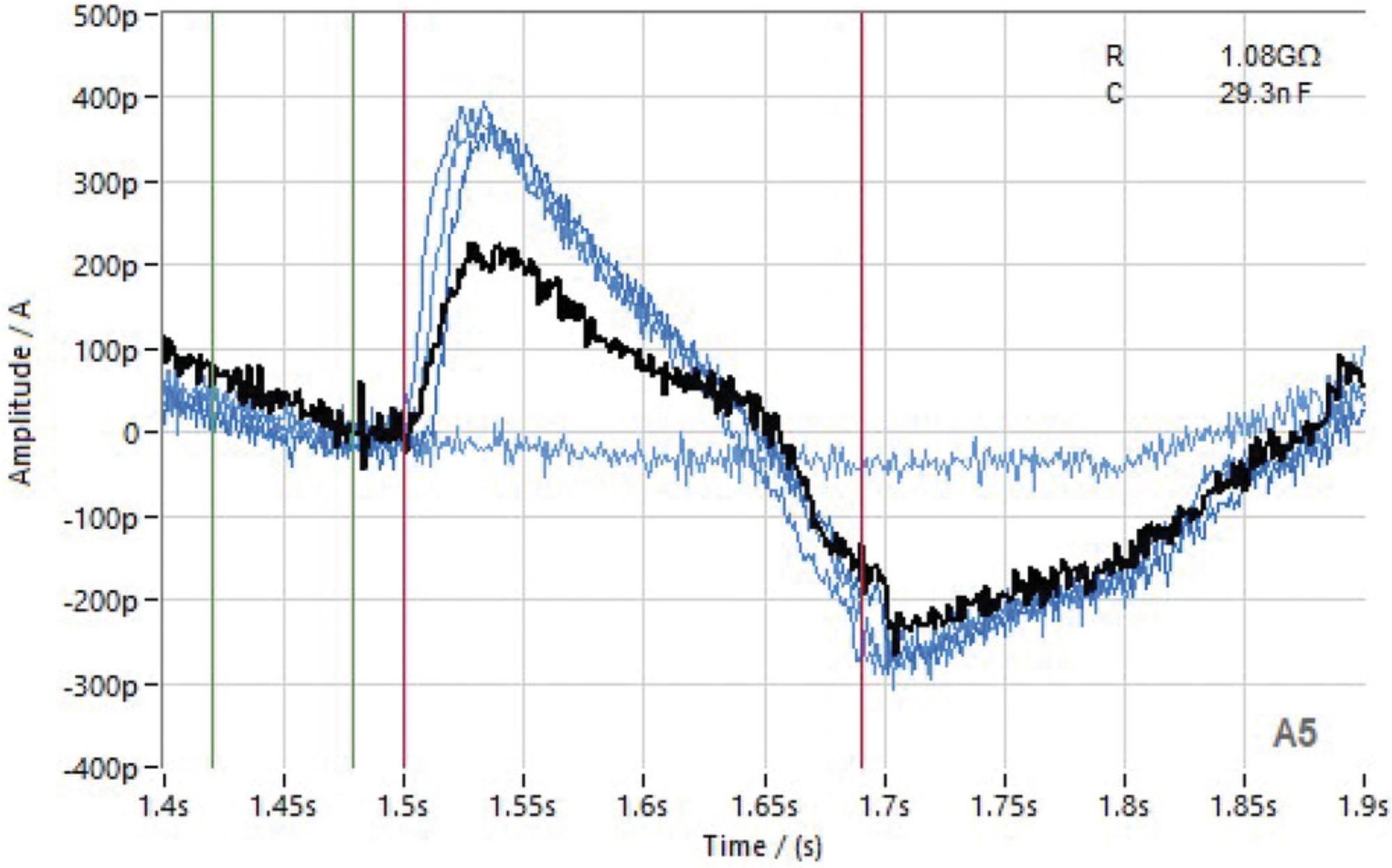
Materials
-
Sensors to which thiol and lipid (Support Protocol 4) or liposomes (Alternate Protocol 2) have already been attached on the gold surface
-
Membranes at a concentration depending on the result from Support Protocol 2
-
Proteoliposomes at a concentration depending on the result from Support Protocol 6
-
Substrate at a concentration depending on the result from Support Protocol 7
-
Dimethylsulfoxide (DMSO; Sigma cat. no. D2650)
-
Published inhibitor (standard) or unknown compound, at 10 mM in DMSO
-
96-well U-bottom plates (Greiner cat. no. 650201)
-
96-well Masterblock (Greiner cat. no. 780285)
-
Additional reagents and equipment for Support Protocol 4 or Alternate Protocol 2 (with buffer composition depending on the results of Support Protocol 8 if needed for an ion-dependent transporter or Support Protocol 9 if needed for a pH-dependent transporter) and for Support Protocol 5 or Alternate Protocol 3 (with buffer composition depending on the results of Support Protocol 8 if needed for an ion-dependent transporter or Support Protocol 9 if needed for a pH-dependent transporter)
1.Use sensors to which thiol and lipid (Support Protocol 4) or liposomes (Alternate Protocol 2) have already been attached on the gold surface (from steps 1-8 of each protocol).
2.Attach membranes/liposomes as described in Support Protocol 4/Alternate Protocol 2 (steps 9-15).
3.Set up the experiment as follows:
-
Use membrane concentration/liposome concentration determined in Support Protocol6;
-
Use materials and buffers from Support Protocol4[5]/Alternate Protocol2[3] (use Support Protocol8for materials and buffers if necessary for an ion-dependent transporter);
-
Use materials and buffers from Support Protocol4[5]/Alternate Protocol2[3] (use Support Protocol9for materials and buffers if necessary for a Ph-dependent transporter);
-
Use DMSO concentration determined in Support Protocol10;
-
Use substrate concentration determined in Support Protocol7.
Workflow for compound single concentration
4a. Prepare the compound plate according to the following workflow, using 96-well plates (single solution exchange).
-
Plate assignment:Column 1: No compound (high control), adjust DMSO concentrationColumn 2: For known compound, standard at IC50concentration (control); for unknown compound, no compound (high control), adjust DMSO concentrationColumns 3-12: 80 different compounds (concentration depending on assay: 10 or 30 µM)
-
Required compound plates:Plates 1 and 2: Compound in assay buffer determined during assay development (250 µl per well)Plate 3: Compound in activation buffer determined during assay development (250 µl per well)
4b. Double solution exchange : Prepare the compound plate according to the following workflow, using 96-well plates or 96-well Masterblock.
-
Plate assignment:Column 1: No compound (high control), adjust DMSO concentrationColumn 2: For known compound, standard at IC50concentration (control); for unknown compound, no compound (high control), adjust DMSO concentrationColumns 3-12: 80 different compounds (concentration depending on assay: 10 or 30 µM)
-
Required compound plates:Plate 1: Compound in assay buffer determined during assay development (700 µl per well, 96-well Masterblock)Plate 2: Compound in control buffer determined during assay development (250 µl per well, 96-well plate)Plate 3: Compound in activation buffer determined during assay development (100 µl per well, 96-well plate)
Workflow for compound IC50
4c. Single solution exchange : Prepare the compound plate according to the following workflow, using 96-well plates.
-
Plate assignment:Column 1: No compound (high control), adjust DMSO concentrationColumn 2: Standard in IC50concentration (control)Wells A3-A12: Compound 1 in 10 different dilutionsWells B3-B12: Compound 2 in 10 different dilutionsRepeat these steps in rows C-H with compounds 3-8
-
Required compound plates:Plates 1 and 2: Compound in assay buffer determined during assay development (250 µl per well)Plate 3: Compound in activation buffer determined during assay development (250 µl per well)
4d. Double solution exchange : Prepare the compound plate according to the following workflow, using 96-well plates or 96-well Masterblock (double solution exchange).
-
Plate assignment:Column 1: No compound (high control), adjust DMSO concentrationColumn 2: Standard in IC50concentration (control)Wells A3-A12: Compound 1 in 10 different dilutionsWells B3-B12: Compound 2 in 10 different dilutionsRepeat these steps in rows C-H with compounds 3-8
-
Required compound plates:Plate 1: Compound in assay buffer determined during assay development (700 µl per well, 96-well Masterblock)Plate 2: Compound in control buffer determined during assay development (250 µl per well, 96-well plate)Plate 3: Compound in activation buffer determined during assay development (100 µl per well, 96-well plate)
5.Activate according to the following major steps using the “single solution exchange” or “double solution exchange” protocol:
-
Activate with buffer but no substrate (to rinse; assay or control buffer);
-
Activate with buffer but no substrate (control; assay or control buffer);
-
Activate with buffer plus substrate (activation buffer);
-
Activate with buffer plus substrate (activation buffer);
-
Activate with buffer plus substrate (activation buffer);
-
Wash with assay buffer + compound or control buffer + compound;
-
Incubate with compound for 15-30 min (depending on the assay);
-
Activate with buffer (plus compound) plus substrate (activation buffer + compound).
6.Determine the area under the curve using the Data Control software.
7.Compare activations 3-5; the deviation between the individual measurements should be <8%.
8.Calculate the inhibition or the IC50 of the compounds.
Support Protocol 13: COMPOUND WASH-OUT
Support Protocol 13 describes the wash-out procedure after compound measurement (Figs. 1 and 6). First measurements are done in the absence of activation buffer with substrate to establish a baseline control using a sensor recovery time of 5 min (Fig. 6). The stability of activation is monitored by performing several activations with substrate with a sensor recovery time of 5 min (Fig. 6). Compounds are incubated for 30 min, and measurements are done in the presence of activation buffer containing the substrate. Followed by a compound wash-out procedure where several activations are done in the absence of compound.
Materials
-
Sensors to which thiol and lipid (Support Protocol 4) or liposomes (Alternate Protocol 2) have already been attached on the gold surface
-
Membranes at a concentration depending on the results of Support Protocol 6
-
Proteoliposomes at a concentration depending on the results of Support Protocol 6
-
Substrate at a concentration depending on the results of Support Protocol 7
-
Dimethylsulfoxide (DMSO; Sigma cat. no. D2650)
-
Published inhibitor (standard) or unknown compound, at 10 mM in DMSO
-
96-well U-bottom plates (Greiner cat. no. 650201)
-
96-well Masterblock (Greiner cat. no. 780285)
-
Additional reagents and equipment for Support Protocol 4 or Alternate Protocol 2 (with buffer composition depending on the results of Support Protocol 8, if necessary for an ion-dependent transporter, or Support Protocol 9, if necessary for a pH-dependent transporter) and for Support Protocol 5 or Alternate Protocol 3 (with buffer composition depending on the results of Support Protocol 8, if necessary for an ion-dependent transporter, or Support Protocol 9, if necessary for a pH-dependent transporter)
1.Use the sensors to which thiol and lipid (Support Protocol 4) or liposomes (Alternate Protocol 2) have already been attached on the gold surface (from steps 1-8 of each protocol).
2.Attach membranes/liposomes as described in Support Protocol 4/Alternate Protocol 2 (steps 9-15):
-
Use membrane concentration/liposome concentration determined in Support Protocol6;
-
Use materials and buffers from Support Protocol4[5]/Alternate Protocol2[3] (use Support Protocol8for materials and buffers if necessary for an ion-dependent transporter);
-
Use materials and buffers from Support Protocol4[5]/Alternate Protocol2[3] (use Support Protocol9for materials and buffers if necessary for a pH-dependent transporter);
-
Use DMSO concentration determined in Support Protocol10;
-
Use substrate concentration determined in Support Protocol7.
3.Measure compounds by performing Support Protocol 12.
4.Repeat step 6c of Support Protocol 12 to determine whether the compound can be washed out.
5.Repeat this step until the original signal is again achieved (provided that the compound is amenable to wash-out).
6.Determine the area under the curve using the Data Control software.
7.Compare these values with the original signals from step 3 of this protocol.
Support Protocol 14: STATISTICAL ANALYSIS
The SURFE²R (Nanion Technologies Munich) has a standard evaluation software called Data Control 96 that can calculate parameters like current maximum or area under the curve (AUC) of each individual recording in an automated way. For raw data analysis, the parameters “max” (maximum height of the signal/peak) and “AUC” (area of the signal/peak) are used.
At the beginning of an assay development and by measuring the different dependencies, only AUCs are determined. Later in assay development, i.e., when IC50 values of the standard inhibitors are determined, both parameters can be used for data quantification. AUC data provide usually more consistent and reliable results. Note that the Z ' factor is not calculated, because each well has its own controls and the deviation of 100% signals from well to well would have too large an influence.
The SURFE²R software, Data Control 96 (Nanion Technologies Munich), is able to calculate the AUC of signal minus the AUC baseline, so that this value can be used for later evaluation (AUC calculated = AUC signal – AUC baseline). For this purpose, the sections in which the signal or the baseline are to be expected must be defined. An advantage of the SURFE²Rs and of this procedure is that each well—i.e., each sensor—has its own low control and its own high control to which the compound measurements are referred.
Evaluation of which wells should be used for the calculation
IMPORTANT NOTE : Only if the signal deviation of activations 3-5 is <8% should a given well be used for the later calculation of an inhibition.
- 1.Average “AUC calculated” (activations 3-5 plus substrate):
- Average “AUC calculated” = (AUC calculated 3 + AUC calculated 4 + AUC calculated 5)/3
- 2.SD = σ = √(∑ x −¯ x)2 /n):
- SD “AUC calculated” (activations 3-5 plus substrate)
- SD “AUC calculated” = SD AUC 3-5
- 3.%CV “AUC calculated”:
- %CV “AUC calculated” = 100/average “AUC calculated” × STDEV “AUC calculated”
Evaluation of % inhibition
Each well is calculated individually. Only those wells for which the % CV of activations 3-5 = <8% are used.
- 1.100% AUC:
- 100% AUC = average “AUC calculated” (activations 3-5) – AUC activation 2 (without substrate)
- 2.AUC inhibition:
- AUC inhibition = AUC activation 6 (with inhibitor) – AUC activation 2 (without substrate)
- 3.% inhibition:
- % inhibition = 100 – (100/100% AUC × AUC inhibition).
REAGENTS AND SOLUTIONS
Activation buffer 1
Add the appropriate amount of L-leucine (Sigma cat. no. 76526; MW 131.17 g/mol) to assay buffer 1 (see recipe): e.g., 13.1 mg/100 ml if preparing buffer with 1 mM L-leucine. Prepare fresh.
Activation buffer 2
Add the appropriate amount of L-glutamic acid (Sigma cat. no. G5889; MW 169.11 g/mol) to control buffer (see recipe): e.g., 1 mM = 16.9 mg/10 ml if preparing buffer with 1 mM L-glutamic acid. Prepare fresh.
Assay buffer 1
- 140 mM (8.18 g/L) NaCl (Sigma cat. no. 71378; MW 58.44 g/mol)
- 30 mM (7.15 g/L) HEPES (Sigma cat. no. H3375; MW 238.30 g/mol)
- 4 mM (813 mg/L) magnesium chloride hexahydrate (MgCl2; Sigma cat. no. M2670; MW 203.30 g/mol)
- 0.5 mM (73.5 mg/L) calcium chloride dihydrate (CaCl2; Sigma 223506; MW147.02 g/mol)
- Adjust pH to 7.4 with N -methyl-D-glucamine (Sigma #M2004)
- Store up to 6 months at 4°C
Assay buffer 2
- 140 mM (10.56 g/L) potassium chloride (KCl; Sigma cat. no. P9333; MW 75.44 g/mol)
- 30 mM (7.15 g/L) HEPES (MW 238.30 g/mol)
- 2 mM (407 mg/L) MgCl2 (MW 203.30 g/mol)
- Adjust pH to 7.4 with potassium hydroxide solution (KOH; Sigma cat. no. 1.09107)
- Store up to 6 months at 4°C
Choline chloride buffer 1
- 140 mM (19.54 g/L) choline chloride (Sigma cat. no. C7527; MW 139.62 g/mol)
- 30 mM (7.15 g/L) HEPES (MW 238.30 g/mol)
- 4 mM (813 mg/L) MgCl2 (MW 203.30 g/mol)
- 0.5 mM (73.5 mg/L) CaCl2 (MW147.02 g/mol)
- Adjust pH to 7.4 with N -methyl-D-glucamine
- Store up to 6 months at 4°C
Choline chloride buffer 2
- 140 mM (19.54 g/L) choline chloride (MW 139.62 g/mol)
- 30 mM (7.15 g/L) HEPES (MW 238.30 g/mol)
- 2 mM (407 mg/L) MgCl2 (MW 203.30 g/mol)
- Adjust pH to 7.4 with sodium hydroxide (NaOH; Sigma cat. no. 1.09137)
- Store up to 6 months at 4°C
Control buffer
- 140 mM (8.18 g/L) NaCl (MW 58.44 g/mol)
- 30 mM (7.15 g/L) HEPES (MW 238.30 g/mol)
- 2 mM (407 mg/L) MgCl2 (MW 203.30 g/mol)
- Adjust pH to 7.4 with NaOH
- Store up to 6 months at 4°C
DTT stock solution, 100 mM
Dilute 154 mg DL-dithiothreitol (DTT; Sigma cat. no. D0632; MW 154.25 g/mol) in 10 ml ddH2O. Freeze aliquots from 50 µl for up to 12 months at −80°C.
Homogenization buffer
Dissolve 4.28 g sucrose (Sigma cat. no. S7903; MW 342.3 g/mol) in 50 ml Tris buffer 2 (see recipe) to give a 250 mM sucrose final concentration. Add 1 tablet cOmplete. Store at 4°C. Prepare fresh daily.
Hydration buffer without DTT
- 25 mM (2.98 g/500 ml) HEPES (MW 238.30 g/mol)
- 25 mM (1.51 g/500 ml) Tris(hydroxymethyl)aminomethane (Tris; Merck cat. no. 1.08387; MW 121.14 g/mol)
- 100 mM (3.73 g/500 ml) potassium chloride (KCl; Sigma cat. no. P9333; MW 74.55 g/mol)
- Adjust pH to 7.0 with hydrochloric acid (HCl; Merck cat. no. 1.09057)
- Store up to 6 months at 4°C
Hydration buffer with DTT
Add 200 µl DTT stock solution (see recipe) to 10 ml hydration buffer without DTT (see recipe) to give a 2 mM DTT final concentration. Prepare fresh.
Lipid solution, 10 mM
Dissolve 8.4 mg 1,2-diphytanoyl-sn-glyero-3-phosphocholine (Avanti cat. no. 850356) in 1 ml N-decan (Sigma cat. no. D901). Divide into aliquots in glass vials (500 µl/plate). Store up to 12 months at −80°C. Do not refreeze after thawing.
Reconstitution buffer without DTT
- 300 mM (11.19 g/500 ml) KCl (MW 74.55 g/mol)
- 75 mM () MOPS (Sigma cat. no. M1254; MW 209.26 g/mol; 7.85 g/500 ml)
- 5 mM (0.51 g/500 ml) MgCl2 (MW 203.3 g/mol)
- Adjust pH to 7.0 with KOH
- Store up to 6 months at 4°C
Reconstitution buffer with DTT
Add 200 µl DTT stock solution (see recipe) to 10 ml reconstitution buffer without DTT (see recipe) to give a 2 mM DTT final concentration. Prepare fresh daily.
Storage buffer (without additives)
- 140 mM (10.44 g/L) KCl (MW 74.55 g/mol)
- 2 mM (407 mg/L) MgCl2 (MW 203.30 g/mol)
- 5 mM (292 mg/L) NaCl (MW 58.44 g/mol)
- 30 mM (7.15 g/L) HEPES (MW 238.30 g/mol)
- Adjust pH to 7.5 with N -methyl-D-glucamine
- Store up to 6 months at 4°C
Storage buffer with glycerol and DTT
Dilute glycerol (Sigma cat. no. G5516) to 5% (w/v) in storage buffer without additives (see recipe) by dissolving 5 g glycerol in 100 ml storage buffer. Add 200 µl DTT stock solution (see recipe) to give a 0.2 mM DTT final concentration. Store at 4°C. Prepare fresh daily.
Sucrose gradient buffers
- 70% (w/v) : 70 g sucrose/100 ml in 10 mM Tris buffer 2 (see recipe)
- 45% (w/v) : 45 g sucrose/100 ml in 10 mM Tris buffer 2 (see recipe)
- 31% (w/v) : 31 g sucrose/100 ml in 10 mM Tris buffer 2 (see recipe)
- 9% (w/v) : 9 g sucrose/100 ml in 10 mM Tris buffer 2 (see recipe)
- Store up to 6 months at 4°C
Thiol solution, 0.5 mM
Dilute 71.6 mg 1-octadecanethiol (Sigma cat. no. O1858) in 500 ml isopropanol (2-propanol; Fisher Scientific cat. no. P/7500/PC17). Store up to 12 months at room temperature.
Tris buffer 1
- 10 mM (1.21 g/L) Tris(hydroxymethyl)aminomethane (Tris; Merck cat. no. 1.08387; MW 121.1 g/mol)
- 5 mM (1.86 g/L) EDTA (MW 372.2 g/mol)
- Adjust pH to 7.2 with HCl
- Store up to 6 months at 4°C.
Tris buffer 2
10 mM (1.21 g/L) Tris. Adjust pH to 7.5 with HCl. Store up to 6 months at 4°C
Tris cOmplete buffer
Dissolve 1 table cOmplete™ Protease Inhibitor Cocktail (Roche cat. no. 11697498001) in 50 ml Tris buffer 1 (see recipe). Store at 4°C. Prepare fresh daily.
COMMENTARY
Background Information
High-throughput screening (HTS) campaigns in the pharmaceutical industry aim to rapidly identify active compounds or biologics that modulate a particular biomolecular pathway and provide starting points for drug design. Reproducible, standardized, and robust assays, based on read-out systems that are compatible with automated screening platforms, are key assets for modern drug-discovery approaches. Automated robotic systems are normally used to rapidly test thousands to millions of compound samples for biological activity at the cellular or molecular level. As an pharmaceutical industry HTS typically aims to screen thousand or even more samples per day, relatively simple and automation-compatible assay designs, robotic-assisted sample handling, and automated data processing are essential. SLCs are still attractive targets to the pharmaceutical industry because of their relevance to a wide range of diverse indications and diseases.
Ion transporter assay technologies using labelled ligands and substrates or manual patch clamping suffer from limited sensitivity and robustness and high assay costs, but at the same time are ideal assay systems for HTS in pharmaceutical industry (Dunlop et al., 2008). Because of their simplicity, radioactive and florescence-based influx assays are frequently used in SLC high-throughput screening studies as entry assay (Fig. 2; Fardel, Le Vee, Jouan, Denizot, & Parmentier, 2015). Compounds from HTS with the desired target activity are further validated using so-called secondary and tertiary assays, which are designed to confirm primary hits through a series of biochemical or cellular assays detecting the functional response of the compounds, ensuring that the identified hits can be reliably translated into leads (Fig. 2). Secondary and tertiary assays normally use direct read-outs and are therefore label free, significantly reducing assay artefacts, compound interference, and false-negative or false-positive hits. Label-free assay technologies used in SLC drug-discovery campaigns include mass-spectrometry-based substrate import assays, high-throughput electrophysiology, and solid-supported membrane (SSM)-based electrophysiology, which can be used to measure the activity of electrogenic SLCs (Bazzone & Barthmes, 2020; Bazzone et al., 2013, 2017). Validated compounds with target selective activity are called lead compounds and are used as starting points for medicinal chemical optimization ( Figs. 1 and 2).
The surface electrogenic event reader technology (SURFE²R, Nanion Technologies Munich) employs SSM-based electrophysiology and allows up to 100 sequential measurements to be performed on the same sample. It makes it an ideal system to confirm and to counter-screen primary hits (Figs. 1 and 2) and allows the determination of parameters such as EC50 or IC50 values (Figs. 1 and 2; Dvorak et al., 2021; Gerbeth-Kreul et al., 2021; Nanion Technologies, 2021). Measurements can be done with purified membranes or vesicles from cells or with proteoliposomes, enabling label-free electrical measurements (Nanion Technologies, 2021). SURFE²R studies require only 0.1-1 µg protein per sensor, which suffices for up to 100 experiments (Nanion Technologies,, 2021). The technology allows real-time data acquisition with a high time resolution and a high signal amplification compared to conventional patch-clamp studies and additionally makes it possible to resolve fast binding kinetics and obtain EC50 or IC50 determinations with high throughput (Fig. 1; Gerbeth-Kreul et al., 2021; Nanion Technologies,, 2021). Additionally, it enables measurement of the transport rates of transporters with a low rate of transport because many transporters are spotted on the sensor, in stark contrast to patch clamp.
Critical Parameters and Troubleshooting
Support Protocol 1
Cell cultivation must be performed under sterile conditions to avoid contamination of the cells. In addition, it is important to ensure that the cell layer is only grown to a maximum confluence of 80%-90%, as otherwise the functionality of the cells cannot be guaranteed. The overexpression of the SLC transporter of interest should be controlled by western blot analysis of the total cell lysates and the corresponding membrane fraction, or alternatively by fluorescence microscopy if the overexpressed SLC contains a fluorescent protein tag or by immunofluorescence if SLC-transporter-specific antibodies are available.
Support Protocol 2
During membrane preparation, all steps should be performed refrigerated or on ice. Centrifuges must be precooled, buffers at 4°C must be used, and all supernatants transferred between the individual steps must be stored on ice. Thawing of cell pellets should be performed as quickly as possible.
Alternate Protocol 1
All steps must be done on ice, and centrifuges and buffers must be precooled and stored at 4°C. All supernatants obtained during the preparation must be stored on ice. Thawing of cell pellets should be done as quickly as possible. When setting up the sucrose gradient, handle the individual sucrose fractions very carefully. The different sucrose layers should not mix, as otherwise the purity of the fraction of interest decreases significantly. Aspirate the individual fractions very carefully with a Pasteur pipette.
NOTE : Test whether there is any positive influence on the stability of the membrane if the storage buffer is mixed with a low concentration of the ion to be transported.
Support Protocol 3
If your SLC of interest cannot be stably expressed in any expression system or, alternatively, does not integrate into the cell membrane after overexpression, reconstitution of recombinant SLC in proteoliposomes is a good alternative. Additionally, if your SSM-based measurements reveal high background or artefacts, using proteoliposomes containing the SLC of interest can significantly decrease currents related to artefacts.
Support Protocol 4
Thiolation
Make sure that the wells are dry and no residual isopropanol is left in the wells after rinsing. Avoid precipitation of thiol stock solution.
Membranes/liposomes
When adding the membrane or liposomes, make sure that the gold layer or the thiol layer is not touched with the pipette, as this can damage the thiol layer.
Support Protocol 5
It is essential to ensure that the ion concentration of the buffers is always identical. This means that in the "single solution exchange" protocol, a larger amount of assay buffer needs to be prepared, and the corresponding substrate is added to the assay buffer. This is important because small differences in osmolarity can influence the measurement. Some substrates change the pH of the buffer. As this can also have an impact on the assay signals or generate artifacts, it is necessary to confirm the pH of the activation buffer when developing new assays. If the difference in pH between the buffers with and without substrate is >0.1, the pH must be adjusted. It is imperative to treat a wild-type cell line identically to the transporter cell line because some substrates generate an artifact that occurs like a specific signal. If a significant signal difference between wild-type and transporter cell line can be observed, the signals are specific.
Alternate Protocol 3
It is essential to ensure that the ion concentration of the different buffers is identical. In the "double solution exchange" protocol, an ion gradient must be established by using three different buffers (assay buffer, control buffer, and activation buffer). The difference between the assay buffer and control buffer is, for example, that potassium is exchanged for sodium. The activation buffer consists of control buffer plus substrate. It is necessary that the ion concentrations of the activation and control buffer be identical, which can be tested by measuring osmolarity. Some substrates change the pH of the buffer. Because this can also have an influence on the signals or generate artifacts, it is essential to check the pH of the activation buffer when developing new assays. If the difference in pH between the buffers with and without substrate is >0.1, the pH must be adjusted or readjusted. It is imperative to treat a wild-type cell line identically to the transporter cell line because some substrates generate an artifact that looks like a signal. A specific signal can be identified only if a difference between wild-type and transporter cell line can be detected.
Support Protocol 12
It is imperative that the signal be stable over multiple activations. If this is not the case, measurement of inhibition of compounds is not possible.
Support Protocol 13
The DMSO concentration must be identical in each and every well tested.
Anticipated Results
The number of isolated membranes produced does not differ significantly between the cell types described (HEK293 or CHO in Support Protocol 2). Using the simple membrane isolation procedure usually produces 160-200 60-µl membrane aliquots at a concentration of 20 mg/ml, or 192-240 mg total protein. When applying the sucrose gradient ultracentrifugation protocol (Alternate Protocol 1) for membrane isolation, the authors obtain a much greater yield and more active membrane compared with the simple isolation protocol. The most active membrane fraction is usually present in the 31%/45% fraction. Fraction 9%/31% sucrose usually comprises 5-10 20-µl aliquots at a concentration of 5 mg/ml, or 0.5-1.0 mg protein. Fraction 31%/45% sucrose normally comprises 40-80 50-µl aliquots at a concentration of 5 mg/ml, or 10-20 mg protein. Proteoliposome isolations with the SLC of interest produce on average 19-20 50-µl aliquots (PLR 1:50) starting from 7.5 mg of lipid formulation and 150 µg of protein.
The SLC families that have been analyzed using SSM-based electrophysiology are SLC1, SLC6, SLC8, SLC15, and SLCO (Bazzone et al., 2017; Gerbeth-Kreul et al., 2021; Nanion Technologies, 2021). Because SSM-based electrophysiology has been used to assess the function of mitochondrial proteins, this technique may be applied to intracellular SLCs (Watzke, 2010). Interestingly, Barland & Perez (2021) developed an SSM-based electrophysiology method to select inhibitory and non-inhibitory nanobodies targeting an electrogenic secondary transporter and to calculate the nanobodies’ inhibitory constants. This technique may be especially useful for selecting inhibitory nanobodies targeting transporters for which labeled substrates are not available (Barland & Perez, 2021). Bazzone et al. (2022) reported an SSM-based electrophysiological approach to study sugar binding and Na+/glucose cotransport by SGLT1 in membrane vesicles. Rapid solution exchange enables the observation of substrate-induced electrogenic events such as conformational transitions, opening up novel perspectives for in-depth functional studies of SGLT1 and other transporters (Bazzone et al., 2022). Gerbeth-Kreul et al. (2021) evaluated the electrogenic amino acid transporter B0AT1 (SLC6A19). Electrophysiological characterization indicated that leucine-induced currents are B0AT1 dependent and can be inhibited using an established in-house tool compound (Gerbeth-Kreul et al., 2021). Active compounds from primary screens of large compound libraries were validated and false-positive hits were identified. These results clearly demonstrate the suitability of the SSM-based technique as a direct electrophysiological method for rapid and automated identification of small molecules that can inhibit B0AT1 activity (Gerbeth-Kreul et al., 2021).
Overall, SSM-based electrophysiology is an ideal methodology in cases where conventional electrophysiology cannot be applied and is also attractive for screening applications in drug discovery, especially given its robustness and its potential for automation.
Figure 7 illustrates the original current signals from SURFE2R (activations 2-6 in one well) using an SLC6 transporter as an example. Activation 2 was done without substrate, activations 3-5 with substrate, and activation 6 with substrate and inhibitor. The area integrals in the range between 1.5 and 1.69 s are determined using the Data Control software and can then be exported to an Excel file1 (Table 2). The range for determining the integral can differ from transporter to transporter because of the transport speed.
Protocol(s) | Problem | Possible cause | Solution |
---|---|---|---|
Support Protocol 1 | SLC not overexpressed—no signal in western blot or fluorescence microscopy | Overexpressed protein degraded; tagging of protein leads to protein degradation | Use alternative overexpression system with a gene promoter with milder overexpression, apply alternative protein tag, or switch tag from SLC N to C terminus; express desired SLC in other mammalian cells that reflect its in vivo expression pattern; check literature to see if coexpression of a SLC interaction partner is needed for stable expression |
Overexpressed SLC not localized at the membrane | Overexpression leads to cytoplasmic accumulation of unfolded SLC protein, protein tag | Switch N-terminal to C-terminal protein tag; use a gene promoter with milder overexpression | |
Cell contamination | Sterile conditions not maintained | work under sterile conditions and use antibiotics in medium | |
Cell layer >90% confluency | Cell seeding too high | Grow the cells to a maximum confluence of 80% | |
Support Protocol 2 | Low yield in isolating cell membranes from frozen cell pellet | Transfer of the supernatant (step 7) not carried out thoroughly; supernatant was not carefully removed (step 10 or 15) so that part of the pellet was sucked out | Transfer the complete supernatant, working carefully so that the pellet does not get lost |
Poor assay signal using cell membranes from frozen cell pellet | Cells were not cooled or only poorly cooled during processing | Always work on ice or with refrigerated equipment | |
Alternate Protocol 1 | Low yield using cell membranes from frozen cell pellets with sucrose gradient ultracentrifugation isolation procedure | Transfer of the supernatant from step 7 not carried out thoroughly | Carefully remove the complete supernatant under step 9 |
No visual band separation after step 15 using cell membranes from frozen cell pellets with sucrose gradient ultracentrifugation | Different sucrose solutions became mixed | The different sucrose solutions must be prepared and handled very carefully, with no shaking or mixing of the different solutions | |
Poor assay signal using cell membranes from frozen cell pellets with sucrose gradient ultracentrifugation | Cells were not cooled or only poorly cooled during processing | Always work on ice or with refrigerated equipment | |
Support Protocol 3 | The membrane in the extruder in step 8 is clogged liposomes | The lipid film did not dissolve well in the hydration buffer | Shake longer in step 7; if using 100-nm membrane, try processing the lipid first with 400-nm and then with 100-nm membrane |
Low yield in isolating liposomes | Transfer of the supernatant from step 14 or 17 not carried out thoroughly | Carefully transfer the complete supernatant without any loss of substantial amount | |
Parts of the pellet were sucked out during assay step 18 | Increase caution and do not suck out parts of the pellet | ||
Support Protocol 4 & Alternate Protocol 2 | Thiolation for sensor preparation: Well not dry after 10 min at room temperature | Too much ddH2O remains from rinsing the sensor | Let sensor stand for another 5-10 min at room temperature |
The membranes or liposomes did not attach properly during sensor preparation | When the lipid or membrane/liposome is attached, the thiol layer is damaged | Make sure not to touch the gold layer or the thiol layer with the pipette | |
Support Protocol 5/Alternate 3 | No signal during assay development | Transporter not overexpressed or membrane recovery failed; incorrect buffer constitution depending on transport mechanism (sodium, potassium, or pH dependency) | Re-transfect cells; produce new cell membrane; apply the double exchange protocol |
Poor signal during assay development | Transporter not sufficiently overexpressed or membrane recovery failed | Re-transfect cells; produce new cell membrane | |
Similar signal seen with the transporter and wild-type membranes | Problems with the substrate (artefacts); substrate may be transported nonspecifically by the wild-type membranes | Test alternative substrates | |
Support Protocol 13 | No inhibition with the published compound | No direct inhibitor | Change tool compound |
No inhibition with the published compound | Compound not properly dissolved due to poor solubility | Choose lower concentration of the compound |
Sweep results | Sweep 002 | Sweep 003 | Sweep 004 | Sweep 005 | Sweep 006 | |
---|---|---|---|---|---|---|
Parameter | AUC | AUC | AUC | AUC | AUC | |
Well | A01 | −5.17E-12 | 1.71E-11 | 1.60E-11 | 1.83E-11 | −1.05E-11 |
B01 | −2.47E-12 | 1.72E-11 | 1.53E-11 | 1.58E-11 | −1.16E-11 | |
C01 | −4.20E-12 | 1.83E-11 | 1.64E-11 | 1.83E-11 | −9.15E-12 | |
A05 | −5.02E-12 | 2.36E-11 | 2.22E-11 | 2.28E-11 | 1.36E-11 | |
B05 | −2.12E-12 | 1.46E-11 | 1.45E-11 | 1.74E-11 | 8.33E-12 | |
C05 | −3.88E-12 | 1.16E-11 | 1.09E-11 | 1.17E-11 | 3.14E-12 | |
F12 | −3.61E-12 | 2.36E-11 | 2.27E-11 | 2.48E-11 | 2.25E-11 | |
G12 | −2.42E-12 | 2.97E-11 | 2.93E-11 | 3.04E-11 | 2.72E-11 | |
H12 | −7.74E-12 | −8.06E-12 | −8.42E-12 | −7.55E-12 | −1.20E-11 |
Evaluation of which wells are used for the calculation
NOTE : Only if the signal deviation of the activations 3-5 is below 8% should the respective well be used for the later calculation of an inhibition.
Average “AUC calculated” (activations 3-5, with substrate) Average “AUC calculated” = (AUC calculated 3 + AUC calculated 4 + AUC calculated 5)/3
$$\begin{equation*} {\rm{SD\ }} = {\rm{\ \sigma \ }} = {\rm{\ }}\sqrt {\left( {\sum x - \bar{x}} \right)2/n)} \end{equation*}$$
SD “AUC calculated” (activations 3-5, with substrate) SD “AUC calculated” = SD AUC 3-5 %CV “AUC calculated” %CV “AUC calculated” = 100/average “AUC calculated” × STDEV “AUC calculated”
Example Well A5 :
- 1.Average = (2.36E-11 + 2.22E-11 + 2.28E-11)/3:
Average = 2.29E-11
- 2.SD = (2.36E-11 + 2.22E-11 + 2.28E-11)
SD = 6.04117E-13
- 3.%CV = 100/2.29E-11*6.04117E-13
%CV = 2.64
Evaluation of % inhibition
Each well is calculated individually. Only those wells for which the %CV of activations 3-5 = <8% are used.
100% AUC:
100% AUC = average “AUC calculated” (activation 3-5) – AUC activation 2 (without substrate)
AUC inhibition:
AUC inhibition = AUC activation 6 (with inhibitor) – AUC activation 2 (without substrate)
% inhibition:
% inhibition = 100 – (100/100% AUC × AUC inhibition)
Example Well A5
- 1.100% AUC
= Average (AUC activations 3-5) – (AUC activation 2) = Average (2.36E-11 + 2.22E-11 + 2.28E-11) – (-5.02E-12) 100% AUC = 2.79E-11
- 2.AUC inhibition
= (AUC activation 6) – (AUC activation 2) = (1.36E-11) – (-5.02E-12) AUC inhibition = 1.86E-11
- 3.% inhibition
= % inhibition = 100-(100/100% AUC × AUC inhibition) = % inhibition = 100-(100/2.79E-11 × 1.86E-11) % inhibition = 33.12
Time Considerations
Cultivating 5 × 108 to 10 × 108 cells for membrane isolation takes ∼8 days in total. The cultivation procedure requires that (1) the number of cells thawed be sufficient for a 175-cm2 flask to be semi-confluent the next day and (2) the cells double from day to day.
The complete membrane preparation procedure applying the simple isolation protocol takes ∼5 hr, including all centrifugation steps, and the gradient-centrifugation protocol ∼7 hr. The production of the proteoliposomes takes a complete working day. The practical work in the lab is ∼2 hr, plus 19-20 hr for centrifugation and shaking of the samples.
In general, the SURFE²R protocols at are very similar and differ only in the number of activations. This number determines the total duration of the actual program. An activation in the “single solution exchange” protocol takes ∼6 min, whereas an activation in the "double solution exchange" protocol takes ∼8 min. For measurements with inhibitors, the duration of the program is extended by the corresponding incubation time. The calculations always refer to the measurement of a complete plate with 96 sensors. The SURFE²R assay sensor preparation (Support Protocol 5) takes on average 30 min in total (for pipetting of the thiol solution, preparation of the membranes, etc.). The overall procedure, including incubation times and centrifugation, takes 5 hr in total, but a few steps can be done overnight. The time for attaching membranes or proteoliposomes are identical. The measurement of a published inhibitor or unknown compounds include eight compounds at 10 different concentrations (IC50) or 80 compounds at one concentration (single concentrations) per sensor plate. In contrast to the previous protocols, the compound measurement protocol is extended by the compound incubation time. Assuming an incubation time of 30 min, this protocol takes ∼66 min with "single solution exchange" and a total of six activations (two without substrate, three with substrate, and one with substrate and inhibitor). In the "double solution exchange" program, the SURFE²R requires ∼78 min. Additionally, the protocol requires additional 30 min for diluting and preparing the inhibitor.
Acknowledgments
We thank the numerous members of our laboratory who have worked with SSM-based electrophysiology within the last couple of years.
RESOLUTE has received funding from the Innovative Medicines Initiative 2 Joint Undertaking under grant agreement No 777372.This Joint Undertaking receives support from the European Union's Horizon 2020 research and innovation program and EFPIA. This article reflects only the authors' views and neither IMI nor the European Union and EFPIA are responsible for any use that may be made of the information contained therein.
Author Contributions
Antje Pommereau : Data curation, formal analysis, investigation, validation; Thomas Licher : Conceptualization, Supervision, writing—review and editing; Felix Bärenz : Conceptualization, project administration, supervision, writing—original draft, writing—review and editing.
Conflict of Interest
The authors declare no conflict of interest.
Open Research
Data Availability Statement
The data that support the findings of this study are available on request from the corresponding author. The data are not publicly available due to privacy or ethical restrictions.
Literature Cited
- Avram, S., Halip, L., Curpan, R., & Oprea, T. I. (2021). Novel drug targets in 2020. Nature Reviews Drug Discovery , 20(5), 333. doi: 10.1038/d41573-021-00057-z
- Barland, N., & Perez, C. (2021). Selection of transporter-targeted inhibitory nanobodies by solid-supported-membrane (SSM)-based electrophysiology. Journal of Visualized Experiments: JoVE , 2021, 171. doi: 10.3791/62578
- Bazzone, A., & Barthmes, M. (2020). Functional characterization of SLC transporters using solid supported membranes. Methods in Molecular Biology , 2168, 73–103. doi: 10.1007/978-1-0716-0724-4_4
- Bazzone, A., Barthmes, M., & Fendler, K. (2017). SSM-based electrophysiology for transporter research. Methods in Enzymology , 594, 31–83. doi: 10.1016/bs.mie.2017.05.008
- Bazzone, A., Costa, W. S., Braner, M., Calinescu, O., Hatahet, L., & Fendler, K. (2013). Introduction to solid supported membrane based electrophysiology. Journal of Visualized Experiments: JoVE , (75), e50230. doi: 10.3791/50230
- Bazzone, A., Korner, A., Meincke, M., Bhatt, M., Dondapati, S., Barthmes, M., … Fertig, N. (2022). SSM-based electrophysiology, a label-free real-time method reveals sugar binding & transport events in SGLT1. Biosensors & Bioelectronics, 197, 113763. doi: 10.1016/j.bios.2021.113763
- Casiraghi, A., Bensimon, A., & Superti-Furga, G. (2021). Recent developments in ligands and chemical probes targeting solute carrier transporters. Current Opinion in Chemical Biology , 62, 53–63. doi: 10.1016/j.cbpa.2021.01.012
- Dunlop, J., Bowlby, M., Peri, R., Vasilyev, D., & Arias, R. (2008). High-throughput electrophysiology: An emerging paradigm for ion-channel screening and physiology. Nature Reviews Drug Discovery , 7(4), 358–368. doi: 10.1038/nrd2552
- Dvorak, V., Wiedmer, T., Ingles-Prieto, A., Altermatt, P., Batoulis, H., Barenz, F., … Superti-Furga, G. (2021). An overview of cell-based assay platforms for the solute carrier family of transporters. Frontiers in Pharmacology , 12, 722889. doi: 10.3389/fphar.2021.722889
- Fardel, O., Le Vee, M., Jouan, E., Denizot, C., & Parmentier, Y. (2015). Nature and uses of fluorescent dyes for drug transporter studies. Expert Opinion on Drug Metabolism & Toxicology, 11(8), 1233–1251. doi: 10.1517/17425255.2015.1053462
- Garibsingh, R. A., Otte, N. J., Ndaru, E., Colas, C., Grewer, C., Holst, J., & Schlessinger, A. (2018). Homology modeling informs ligand discovery for the glutamine transporter ASCT2. Frontiers in Chemistry , 6, 279. doi: 10.3389/fchem.2018.00279
- Gerbeth-Kreul, C., Pommereau, A., Ruf, S., Kane, J. L. Jr., Kuntzweiler, T., Hessler, G., … Licher, T. (2021). A solid supported membrane-based technology for electrophysical screening of B(0)AT1-modulating compounds. SLAS Discovery , 26(6), 783–797. doi: 10.1177/24725552211011180
- Hediger, M. A., Clemencon, B., Burrier, R. E., & Bruford, E. A. (2013). The ABCs of membrane transporters in health and disease (SLC series): Introduction. Molecular Aspects of Medicine , 34(2-3), 95–107. doi: 10.1016/j.mam.2012.12.009
- Hediger, M. A., Romero, M. F., Peng, J. B., Rolfs, A., Takanaga, H., & Bruford, E. A. (2004). The ABCs of solute carriers: Physiological, pathological and therapeutic implications of human membrane transport proteins. Pflugers Archiv: European journal of physiology , 447(5), 465–468. doi: 10.1007/s00424-003-1192-y
- International Transporter, C., Giacomini, K. M., Huang, S. M., Tweedie, D. J., Benet, L. Z., Brouwer, K. L., … Zhang, L. (2010). Membrane transporters in drug development. Nature Reviews Drug Discovery , 9(3), 215–236. doi: 10.1038/nrd3028
- Lin, L., Yee, S. W., Kim, R. B., & Giacomini, K. M. (2015). SLC transporters as therapeutic targets: Emerging opportunities. Nature Reviews Drug Discovery , 14(8), 543–560. doi: 10.1038/nrd4626
- Nanion Technologies Munich. (2021). The solid supported membrane (SSM): “Measuring transporter currents” and “SURFE2R 96SE - high throughput transporter research”. Retrieved from https://www.nanion.de/en/products/surfe2r-96se.html
- Perland, E., & Fredriksson, R. (2017). Classification systems of secondary active transporters. Trends in Pharmacological Sciences , 38(3), 305–315. doi: 10.1016/j.tips.2016.11.008
- Pizzagalli, F., Varga, Z., Huber, R. D., Folkers, G., Meier, P. J., & St-Pierre, M. V. (2003). Identification of steroid sulfate transport processes in the human mammary gland. Journal of Clinical Endocrinology and Metabolism , 88(8), 3902–3912. doi: 10.1210/jc.2003-030174
- Schlessinger, A., Yee, S. W., Sali, A., & Giacomini, K. M. (2013). SLC classification: An update. Clinical Pharmacology & Therapeutics, 94(1), 19–23. doi: 10.1038/clpt.2013.73
- Schulz, P., Garcia-Celma, J. J., & Fendler, K. (2008). SSM-based electrophysiology. Methods (San Diego, Calif.) , 46(2), 97–103. doi: 10.1016/j.ymeth.2008.07.002
- Superti-Furga, G., Lackner, D., Wiedmer, T., Ingles-Prieto, A., Barbosa, B., Girardi, E., … Steppan, C. M. (2020). The RESOLUTE consortium: Unlocking SLC transporters for drug discovery. Nature Reviews Drug Discovery , 19(7), 429–430. doi: 10.1038/d41573-020-00056-6
- Watzke, N., Diekert, K., & Obrdlik, P. (2010). Electrophysiology of respiratory chain complexes and the ADP-ATP exchanger in native mitochondrial membranes. Biochemistry , 49(48), 10308–10318. doi: 10.1021/bi1011755
Corrections
In this publication, the following correction was made.
A second paragraph was added to the Acknowledgments section on p.37:
“RESOLUTE has received funding from the Innovative Medicines Initiative 2 Joint Undertaking under grant agreement No 777372. This Joint Undertaking receives support from the European Union's Horizon 2020 research and innovation program and EFPIA. This article reflects only the authors' views and neither IMI nor the European Union and EFPIA are responsible for any use that may be made of the information contained therein.”
The current version now includes this correction and may be considered the authoritative version of record.
Citing Literature
Number of times cited according to CrossRef: 2
- Daniela Digles, Alvaro Ingles-Prieto, Vojtech Dvorak, Tamara A. M. Mocking, Ulrich Goldmann, Andrea Garofoli, Evert J. Homan, Alberto Di Silvio, Lucia Azzollini, Francesca Sassone, Mario Fogazza, Felix Bärenz, Antje Pommereau, Yasmin Zuschlag, Jasper F. Ooms, Jeppe Tranberg-Jensen, Jesper S. Hansen, Josefina Stanka, Hubert J. Sijben, Helena Batoulis, Eckhard Bender, Riccardo Martini, Adriaan P. IJzerman, David B. Sauer, Laura H. Heitman, Vania Manolova, Juergen Reinhardt, Alexander Ehrmann, Philipp Leippe, Gerhard F. Ecker, Kilian V. M. Huber, Thomas Licher, Lia Scarabottolo, Tabea Wiedmer, Giulio Superti-Furga, Advancing drug discovery through assay development: a survey of tool compounds within the human solute carrier superfamily, Frontiers in Pharmacology, 10.3389/fphar.2024.1401599, 15 , (2024).
- Andre Bazzone, Maria Barthmes, Cecilia George, Nina Brinkwirth, Rocco Zerlotti, Valentin Prinz, Kim Cole, Søren Friis, Alexander Dickson, Simon Rice, Jongwon Lim, May Fern Toh, Milad Mohammadi, Davide Pau, David J. Stone, John J. Renger, Niels Fertig, A Comparative Study on the Lysosomal Cation Channel TMEM175 Using Automated Whole-Cell Patch-Clamp, Lysosomal Patch-Clamp, and Solid Supported Membrane-Based Electrophysiology: Functional Characterization and High-Throughput Screening Assay Development, International Journal of Molecular Sciences, 10.3390/ijms241612788, 24 , 16, (12788), (2023).