Scaled Isolation of Mesenchymal Stem/Stromal Cell-Derived Extracellular Vesicles
Verena Börger, Verena Börger, Simon Staubach, Simon Staubach, Robin Dittrich, Robin Dittrich, Oumaima Stambouli, Oumaima Stambouli, Bernd Giebel, Bernd Giebel
Abstract
Mesenchymal stem/stromal cells (MSCs) provide therapeutic effects in many diseases. Contrary to initial hypotheses, they act in a paracrine rather than a cellular manner. To this end, extracellular vesicles (EVs) have been found to mediate the therapeutic effects, even when harvested from MSC-conditioned cell culture supernatants. Lacking self-replicating activity and being so small that MSC-EV preparations can be sterilized by filtration, EVs provide several advantages as therapeutic agents over cellular therapeutics. At present, methods allowing EV preparation from larger volumes are scarce and regularly require special equipment. We have developed a polyethylene glycol−based precipitation protocol allowing extraction of EVs from several liters of conditioned medium. MSC-EVs prepared with this method have been successfully applied to a human graft-versus-host disease patient and to several animal models. Although the method comes with its own limitations, it is extremely helpful for the initial evaluation of EV-based therapeutic approaches. Here, we introduce the technique in detail and discuss all critical steps. © 2020 The Authors.
Basic Protocol 1 : Preparation of MSC-conditioned medium for scaled MSC-EV production
Basic Protocol 2 : PEG precipitation OF MSC-EV from MSC-conditioned medium
INTRODUCTION
At the turn of the millennium, mesenchymal stem/stromal cells (MSCs) were reported as multipotent cells (Pittenger et al., 1999). Considering them as an allogenic stem cell source for cell replacement strategies, several groups studied their interaction with immune cells. Quickly, it was demonstrated that MSCs were able to suppress pro-inflammatory immune responses (Bartholomew et al., 2002; Di Nicola et al., 2002). Due to their beneficial effects in various preclinical animal models, they were quickly translated into the clinic, either intended as cellular replacement or as immunomodulating cells. Up to now, MSCs have been applied in more than 1000 different clinical trials for the treatment of numerous different diseases (Heldring, Mager, Wood, Le Blanc, & Andaloussi, 2015). Despite positive effects in various settings, the MSCs were barely detected in affected tissues, resulting in the hypothesis that they mainly act via their secretome rather than in a direct cellular manner (Caplan & Correa, 2011; Caplan & Dennis, 2006). Indeed, it was quickly demonstrated using the examples of an acute kidney injury model and a myocardial infarction model that MSCs exert their therapeutic effects via small extracellular vesicles (EVs), such as exosomes and microvesicles, having diameters of up to 200 nm. Indeed, an array of different head-to-head studies confirmed that preparations that are highly enriched for such vesicles exert comparable therapeutic activities as their parental MSCs (Bruno et al., 2009; Doeppner et al., 2015; He et al., 2012).
Compared to cellular transplants, EV products provide some significant advantages. Due to their small sizes, MSC-EV preparations can be processed by filtration through filters with 0.22-µm pores, which is considered as sterilization. In contrast to cells, EVs are not self-replicating and thus lack any endogenous tumor-formation potential. Furthermore, their overall handling is much easier than that of cellular products. All of these features are essential requirements for an off-the-shelf product (Lener et al., 2015).
Although we are not aware of the exact mode of action, it appears MSC-EV preparations act multimodally. Among other activities, they can modulate pro-inflammatory into regulatory immune responses, presumably an essential requirement for regenerative processes (Börger et al., 2017; Giebel & Hermann, 2019).
A bottleneck in preparing MSC-EVs for clinical applications is the fact that liters of conditioned medium (CM) need to be prepared for the treatment of an individual patient. Classically, EVs have been prepared by differential centrifugation procedures, in which the initial volume is reduced during ultracentrifugation (see Current Protocols article: Thery, Amigorena, Raposo, & Clayton, 2006). Since even the largest rotors cannot process more than 400 ml in a single, typically 2-hr run, a challenge in the field is to find other, more effective methods of volume reduction. All available methods have their own limitations, such as purity, scalability, and time/cost factor (Watson et al., 2018). Currently, tangential flow filtration (TFF) is increasingly discussed as an effective method for volume reduction (Nordin, Bostancioglu, Corso, & El Andaloussi, 2019). However, this requires specific equipment, and optimization for clinical grade production is still ongoing. Here, we present a scalable, easy-to-handle and cost-effective procedure for the preparation of EVs from larger MSC-CM volumes, which could potentially be used for the clinical-grade production of MSC-EVs (Figs. 1 and 2).
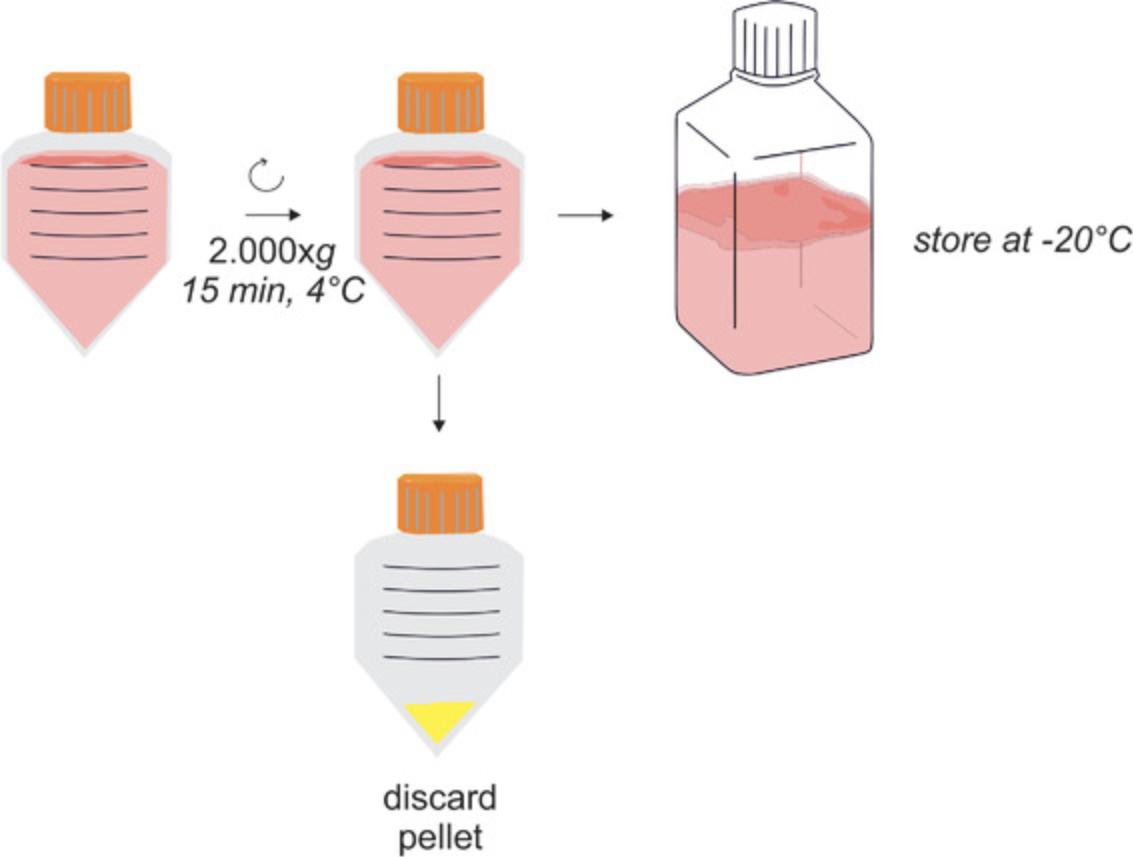
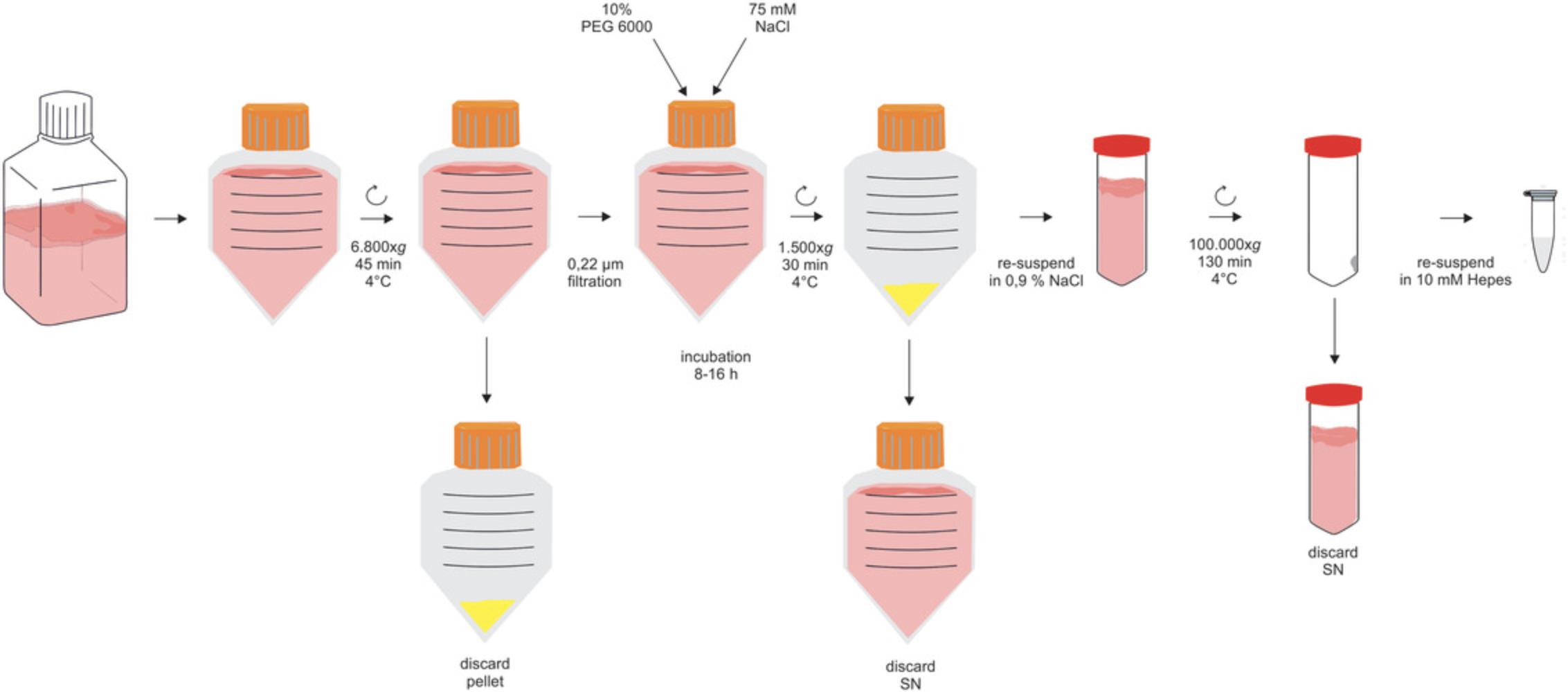
Facing the situation of a treatment-refractory graft-versus-host disease (GvHD) patient for whom no additional treatment options existed in 2011, we considered treating her with MSC-EVs and had to address the challenge of effectively extracting small EVs (<200 nm) from more than 4 L of MSC-CM. As EVs share many physical properties with viruses, especially lentiviruses (Nguyen, Booth, Gould, & Hildreth, 2003), which can effectively be prepared from larger supernatants by polyethylene glycol (PEG) precipitation, we established and optimized a PEG precipitation protocol for small EVs (Ludwig et al., 2018). Subsequently, the protocol was used to prepare MSC-EVs for the successful treatment of the GvHD patient (Kordelas et al., 2014). Notably, as demonstrated at the example of an ischemic stroke mouse model, PEG-prepared MSC-EVs showed comparable effects as their parental a MSCs (Doeppner et al., 2015).
PEG precipitation allows the scaled preparation of functional EVs
Briefly, after obtaining MSC-CM (Basic Protocol 1), the protocol (Basic Protocol 2) starts with a 2000 × g centrifugation step (Fig. 1). It is followed by a mid-speed centrifugation (depending on the maximum rotation speed of the rotor: 6800 to 10,000 × g) and a filtration step to successively remove contaminating cells, larger debris, and EVs that are larger than 200 nm (Fig. 2). Next, PEG precipitation occurs overnight, and the small EVs are pelleted at 1500 × g. To remove the PEG effectively, the precipitated EVs are washed with 0.9% NaCl and are pelleted again by ultracentrifugation. Thereafter, they are resuspended in the buffer of choice, e.g., HEPES or 0.9% NaCl, and stored −80°C until use (Fig. 2).
Basic Protocol 1: PREPARATION OF MSC-CONDITIONED MEDIUM FOR SCALED MSC-EV PRODUCTION
For scaled MSC-EV production, MSCs derived from bone marrow aspirates of healthy individuals are raised in 4-layered tissue culture stacks in human platelet lysate (hPL)−supplemented cultivation medium. During the expansion process, CM is harvested every 48 hr in a cumulative manner. Of note, to obtain optimal cell expansion required for optimal MSC-EV yield, we do not remove the EVs from the hPL-supplemented cultivation medium.
NOTE : All steps should be performed under sterile conditions.
Materials
-
Mesenchymal stem/stromal cells (MSCs; see appropriate articles in Current Protocols in Stem Cell Biology)
-
Cultivation medium (see recipe)
-
1× trypsin/EDTA solution (see recipe) or other suitable enzymatic detachment reagent
-
Phosphate-buffered saline (PBS; Gibco, cat. no. 70013-016)
-
0.4% trypan blue (Sigma, cat. no. T8154)
-
4-layered tissue culture stack (Polystyrene Cell Factory System; ThermoFisher Scientific, cat. no. 140360)
-
Microscope
-
500-ml centrifugation tubes (Corning, cat. no. 431123)
-
Neubauer chamber (hemocytometer)
-
Medium-speed centrifuge (e.g., Avanti J26XP with rotor JS-5.3, Beckman Coulter)
-
Additional reagents and equipment for cell culture, including counting viable cells by trypan blue exclusion (see Current Protocols article: Phelan & May, 2015)
Cultivation of MSCs for scaled CM collection
1.Seed MSCs at a density of around 800 to 1500 MSCs/cm2 in a 4-layered tissue culture stack containing 400 ml cultivation medium. Document the number of seeded cells.
2.Examine the confluence of the cells with a microscope daily.
3.Change medium when cells have reached ∼50% confluency.
4.Collect the CM every 48 hr from MSCs showing 50% to 90% confluency.
5.For passaging, detach MSCs with suitable enzymatic detachment reagents and harvest the cells. For example, use 150 ml of 1× trypsin/EDTA for 5 min, at 37°C. Stop reaction by adding an equal volume of fresh cultivation medium; transfer to a 500-ml centrifugation tube, and spin down for 5 min at 900 × g.
6.Resuspend pelleted cells in an appropriate volume of cultivation medium or PBS and determine the number of viable cells by trypan blue staining in a Neubauer chamber (hemocytometer; see Current Protocols article: Phelan & May, 2015).
7.Calculate the cell equivalents from which the CM was harvested:

): cell number during medium harvest at a time point

; n 0: number of originally seeded cells.
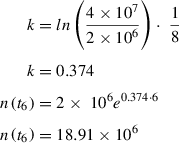
Preparation of MSC-CM
8.Transfer the collected MSC-CM to a new centrifuge tube.
9.Centrifuge the CM 15 min at 2000 × g , 4°C.
10.Transfer supernatant to sterile storage containers.
11.Store CM at −20°C until further processing.
Basic Protocol 2: PEG PRECIPITATION OF MSC-EV FROM MSC-CONDITIONED MEDIUM
The protein content of the CM is critical; thus, only serum- or hPL-supplemented media should be used. CM is harvested in in a cumulative manner, and can be pooled after thawing.
-
Conditioned medium (CM; Basic Protocol 1)
-
3.75 M NaCl (see recipe)
-
50% (w/v) PEG 6000 (see recipe)
-
0.9% sodium chloride (B. Braun, cat. no. 151072)
-
Medium or buffer of choice: e.g.,10 mM HEPES buffer (see recipe)
-
Medium-speed centrifuge (e.g., Avanti J26XP with rotor JS-5.3, Beckman Coulter)
-
Rapid flow filter system (e.g., Nalgene, cat. no. 595-4520)
-
500-ml centrifuge tubes (Corning, cat. no. 431123)
-
Polycarbonate tubes for ultracentrifugation (Beckman Coulter cat. no. 355622)
-
Ultracentrifuge (e.g., L7-65 with rotor Ti45, Beckman Coulter)
-
Low-retention tubes (Kisker, cat. no. G017)
1.Thaw CM at 4°C or at room temperature.
2.Transfer CM to centrifuge tubes and—depending on the rotor—centrifuge 45 min at a minimum of 6800 × g and maximum of 10,000 × g , 4°C.
3.Perform a bottle-top filtration of the CM using 0.22-µm filters.
4.Add PEG 6000 and NaCl to the filtered CM to a final concentration of 10% or 75 mM, respectively:
CM [ml] | 50% PEG 6000 [ml] | 3.75 M NaCl [ml] |
10 | 2.56 | 0.26 |
40 | 10.26 | 1.03 |
100 | 25.64 | 2.56 |
400 | 102.56 | 10.26 |
1000 | 256.41 | 25.64 |
2000 | 512.82 | 51.28 |
4000 | 1025.64 | 102.56 |
10,000 | 2564.10 | 256.41 |
5.Incubate the suspension for 8-16 hr at 4°C (overnight).
6.Mix the suspension well before transferring to centrifuge tubes.
7.Centrifuge 30 min at 1500 × g , 4°C.
8.Remove and discard the supernatant carefully using a pipette; keep the white pellet.
9.Resuspend the pellet in 10 ml 0.9% NaCl until the pellet is completely dispersed.
10.Transfer the suspension to ultracentrifuge tubes.
11.To transfer residual material, rinse the centrifuge tubes from the PEG precipitation with 25 ml of 0.9% NaCl.
12.Transfer each washing to the same ultracentrifuge tube as its original pellet.
13.Add 0.9% NaCl to the tubes to a final volume of 65 ml. Close the tubes with their lids.
14.Balance/tare tubes before loading tubes opposite to each other.
15.Ultracentrifuge 130 min at 100,000 × g , 4°C.
16.Take the tubes out of the rotor and place on ice.
17.Carefully remove the supernatant with a pipette, and discard it.
18.Resuspend the pellet in an appropriate volume of the medium or buffer of choice.
19.Add half of the calculated amount of buffer to the ultracentrifuge tubes, rinse the walls, and resuspend the pellet for a minimum of 4 min.
20.Add buffer to the final volume.
21.Store small aliquots of the EV preparation in appropriate containers at −80°C.
Volume supernatant [ml]: | 4300 | Cell count: | 6.45 × 108 |
---|---|---|---|
Complete medium (after 6800-10,000 × g centrifugation) | |||
Protein concentration [ng/µl]: From BCA |
5,224.69 |
Protein total [mg]: From BCA |
22,466 |
Particle concentration [per ml]: From NTA |
2.0 × 109 |
Particle total: From NTA |
8.6 × 1013 |
Particle size [nm]: From NTA_Average Size (×50 Value) |
100.3 | ||
EVs | |||
Resuspended in: |
⊠ 10 mM HEPES NaCl ☐ Other: __________ |
Volume [ml]: | 16.1 |
Protein concentration [ng/µl]: From BCA |
5518.45 |
Protein total [mg]: From BCA |
88.85 |
Particle concentration [pro ml]: From NTA | 2.5 × 1011 |
Particle total: From NTA |
4.03 × 1012 |
Particle size [nm]: From NTA_Average Size (×50 Value) |
116.3 | ||
Recovery [%]: (Particle total EV/particle total CM) |
4.69 | Particle/mg protein: | 4.5 × 1010 |
REAGENTS AND SOLUTIONS
Cultivation medium
- DMEM, low-glucose (Pan Biotech, cat. no. P04-01500)
- 10% human platelet lysate (hPL; in house production; available also from Macopharma and PL Bioscience)
- 100 U/ml, penicillin-streptomycin-glutamine (Life Technologies, cat. no. 10378016)
- 5 IU/ml heparin (Ratiopharm, cat. no. N68743.06)
- Store up to 4 weeks at 4°C
HEPES buffer, 10 mM
Add 1 ml of 1 M HEPES (Gibco, cat. no. 15630049) to 99 ml of 0.9% NaCl (B. Braun, cat. no. 151072). Sterilize by filtration using a 0.22-µm bottle-top filter. Store up to 6 months at 4°C.
NaCl, 3.75 M
Weigh 219 g sodium chloride (Sigma Aldrich, cat. no. 71376), transfer to a 1 L glass bottle, make up to 1000 ml with distillated water, and autoclave. Store up to 6 months at room temperature.
PEG 6000, 50% (w/v)
Weigh 250 g PEG 6000 (Sigma Aldrich, cat. no. 81260), transfer to a 1-L glass bottle, and make up to 500 ml with distilled water (50% w/v). Shake the bottle to mix the components, and use a magnetic stirrer until the PEG is completely dissolved, shaking from time to time. Autoclave, and store at room temperature for up to 6 months.
Trypsin/EDTA, 1×
- 50 ml 10× trypsin/EDTA (PAN Biotech, cat. no. P10-024100)
- 450 ml phosphate-buffered saline (PBS; Gibco, cat. no. 70013-016)
- Store up to 4 weeks at 4°C
COMMENTARY
Background Information
The method given here is applicable for the large-scale isolation of EVs from CM of various cell types. EVs harvested with this method from MSC-conditioned media have been successfully applied to various preclinical models (Doeppner et al., 2015; Drommelschmidt et al., 2017; Gussenhoven et al., 2019; Ophelders et al., 2016; Wang et al., 2020). EVs share several features with viruses, such as their size and a comparable molecular assembly. Since viruses can be concentrated by PEG precipitation (Kanarek & Tribe, 1967; Kohno et al., 2002; Vajda, 1978), we adopted and optimized protocols originally developed for viruses to be used with EVs (Ludwig et al., 2018). The principle of the PEG precipitation is based on replacement of water molecules that form a hydrate envelope around the EVs. Due to the hydrophobic effect, the EVs precipitate surrounded by PEG, leading to a massive volume reduction, mandatory for using subsequent ultracentrifugation-based methods. Our group established the method as a low-cost alternative to commercially available EV precipitation products.
Critical Parameters and Troubleshooting
Table 2 lists problems that may arise with the protocols in this article along with possible solutions.
Problem | Possible solution |
---|---|
Preparation of CM | |
Filtration of MSC-CM causes filter clogging | Always centrifuge the CM first and filter it in a second step; alternatively, use different material for the filter membrane |
PEG precipitation | |
PEG incompletely dissolved | Increase the time and speed of magnetic stirring and check after autoclaving the solution for residual solids |
Absence of white precipitate pellet after PEG precipitation and subsequent 1500 × g centrifugation step |
Check for correct volumes of added reagents Check on cultivation medium used; was serum or hPL added? Incubation time of PEG precipitation should be between 8-16 hr; modified incubation times may affect the recovery |
Pellet after 1500 × g cannot be resuspended | The precipitate from a maximum of 750 ml of CM should be applied to the 65 ml transferred to the ultracentrifuge tubes |
Ultracentrifugation | |
Precipitates become visible after filling ultracentrifuge tubes with samples of the resuspended PEG pellet |
Check the volume equivalents of CM which was pelleted, resuspended, and transferred to the ultracentrifuge tube; do not load more than 750 ml original CM equivalents per ultracentrifuge tube Invest more effort to disperse the pellet correctly |
Lack of visible EV pellet after 100,000 × g centrifugation | Check the seeded cell number; analyze obtained EVs with appropriate methods (like NTA or Western blot). If EVs are detectable, check the ultracentrifugation speed. It might be too low, or the run was interrupted. |
High protein concentration in obtained EV preparations | Removal of residual supernatant after the 1500 × g step will decrease the protein concentration; EVs may not have been carefully resuspended following PEG precipitation (proteins stick to EVs) |
For a scalable system with larger volumes (>10 L), the described method is limited. As an alternative method, TFF appears feasible. Depending on the system, TFF can be scaled to process hundreds of liters in relatively short time intervals. TFF devices from some companies are provided as automated, scalable systems, both as benchtop devices for research labs and as big machines for industry. First attempts to prepare EVs using TFF have already been published (Busatto et al., 2018; Heinemann et al., 2014). Notably, expensive hardware needs to be purchased. In contrast, for the PEG precipitation, only centrifuges are required, which should belong to each lab working with EVs.
Although the described method can be scaled for EV preparation, there are some bottlenecks to be discussed. The method needs to be considered an open system, including numerous handling steps that increase the risk of contamination. The method is only scalable to mid-range. The limiting factor is the centrifuge size. For example, only up to 5 L can be processed if only one ultracentrifuge run is going to be performed to remove residual PEG from the samples. In total, this is still 14-fold more than the amount that can be processed by conventional differential centrifugation protocols. For larger volumes, several runs need to be performed.
Time Consideration
The given method is applicable for large-scale isolation of CM from MSCs. With this approach, up to 10 L can be processed within 24 hr (including overnight incubation) on 2 subsequent days.
In detail, for the preparation of the medium in advance of the PEG precipitation, approximately 2 hr are needed, including the centrifugation and filtration of the CM. For the precipitation itself, we recommend overnight incubation. On the following day, another 5 hr need to be invested, including the 130 min for the ultracentrifugation run.
Acknowledgments
B.G. received funding from ERA-NET EuroTransBio, from the LeitmarktAgentur.NRW and European Union (European Regional Development Fund 2014–2020), and from the EU Commission for the Horizon 2020 project EVPRO [H2O20-NMBP-TR-IND-2018]. Open access funding enabled and organized by Projekt DEAL.
We thank Michel Bremer and Tobias Tertel for assistance in figure layout and Yanis Mouloud for proofreading.
Author Contributions
Verena Börger : Conceptualization; project administration; writing-original draft; writing-review & editing. Simon Staubach : Conceptualization; project administration; writing-review & editing. Robin Dittrich : Data curation; writing-review & editing. Oumaima Stambouli : Writing-review & editing. Bernd Giebel : Methodology; supervision; writing-review & editing.
Literature Cited
- Bartholomew, A., Sturgeon, C., Siatskas, M., Ferrer, K., McIntosh, K., Patil, S., … Hoffman, R. (2002). Mesenchymal stem cells suppress lymphocyte proliferation in vitro and prolong skin graft survival in vivo. Experimental Hematology , 30, 42–48. doi: 10.1016/S0301-472X(01)00769-X.
- Börger, V., Bremer, M., Ferrer-Tur, R., Gockeln, L., Stambouli, O., Becic, A., & Giebel, B. (2017). Mesenchymal stem/stromal cell-derived extracellular vesicles and their potential as novel immunomodulatory therapeutic agents. International Journal of Molecular Sciences , 18, 1450. doi: 10.3390/ijms18071450.
- Bruno, S., Grange, C., Deregibus, M. C., Calogero, R. A., Saviozzi, S., Collino, F., … Camussi, G. (2009). Mesenchymal stem cell-derived microvesicles protect against acute tubular injury. Journal of the American Society of Nephrology , 20, 1053–1067. doi: 10.1681/ASN.2008070798.
- Busatto, S., Vilanilam, G., Ticer, T., Lin, W. L., Dickson, D. W., Shapiro, S., … Wolfram, J. (2018). Tangential flow filtration for highly efficient concentration of extracellular vesicles from large volumes of fluid. Cells , 7, 273. doi: 10.3390/cells7120273.
- Caplan, A. I., & Correa, D. (2011). The MSC: An injury drugstore. Cell Stem Cell , 9, 11–15. doi: 10.1016/j.stem.2011.06.008.
- Caplan, A. I., & Dennis, J. E. (2006). Mesenchymal stem cells as trophic mediators. Journal of Cellular Biochemistry , 98, 1076–1084. doi: 10.1002/jcb.20886.
- Di Nicola, M., Carlo-Stella, C., Magni, M., Milanesi, M., Longoni, P. D., Matteucci, P., … Gianni, A. M. (2002). Human bone marrow stromal cells suppress T-lymphocyte proliferation induced by cellular or nonspecific mitogenic stimuli. Blood , 99, 3838–3843. doi: 10.1182/blood.V99.10.3838.
- Doeppner, T. R., Herz, J., Gorgens, A., Schlechter, J., Ludwig, A. K., Radtke, S., … Hermann, D. M. (2015). Extracellular vesicles improve post-stroke neuroregeneration and prevent postischemic immunosuppression. Stem Cells Translational Medicine , 4, 1131–1143. doi: 10.5966/sctm.2015-0078.
- Drommelschmidt, K., Serdar, M., Bendix, I., Herz, J., Bertling, F., Prager, S., … Felderhoff-Müser, U. (2017). Mesenchymal stem cell-derived extracellular vesicles ameliorate inflammation-induced preterm brain injury. Brain, Behavior, and Immunity , 60, 220–232. doi: 10.1016/j.bbi.2016.11.011.
- Giebel, B., & Hermann, D. M. (2019). Identification of the right cell sources for the production of therapeutically active extracellular vesicles in ischemic stroke. Annals of Translational Medicine , 7, 188. doi: 10.21037/atm.2019.03.49.
- Gussenhoven, R., Klein, L., Ophelders, D., Habets, D. H. J., Giebel, B., Kramer, B. W., … Wolfs, T. (2019). Annexin A1 as neuroprotective determinant for blood-brain barrier integrity in neonatal hypoxic-ischemic encephalopathy. Journal of Clinical Medicine , 8, 137. doi: 10.3390/jcm8020137.
- He, J., Wang, Y., Sun, S., Yu, M., Wang, C., Pei, X., … Zhao, W. (2012). Bone marrow stem cells−derived microvesicles protect against renal injury in the mouse remnant kidney model. Nephrology , 17, 493–500. doi: 10.1111/j.1440-1797.2012.01589.x.
- Heinemann, M. L., Ilmer, M., Silva, L. P., Hawke, D. H., Recio, A., Vorontsova, M. A., … Vykoukal, J. (2014). Benchtop isolation and characterization of functional exosomes by sequential filtration. Journal of Chromatography A , 1371, 125–135. doi: 10.1016/j.chroma.2014.10.026.
- Heldring, N., Mager, I., Wood, M. J., Le Blanc, K., & Andaloussi, S. E. (2015). Therapeutic potential of multipotent mesenchymal stromal cells and their extracellular vesicles. Human Gene Therapy , 26, 506–517. doi: 10.1089/hum.2015.072.
- Kanarek, A. D., & Tribe, G. W. (1967). Concentration of certain myxoviruses with polyethylene glycol. Nature , 214, 927–928. doi: 10.1038/214927a0.
- Kohno, T., Mohan, S., Goto, T., Morita, C., Nakano, T., Hong, W., … Sano, K. (2002). A new improved method for the concentration of HIV-1 infective particles. Journal of Virological Methods , 106, 167–173. doi: 10.1016/S0166-0934(02)00162-3.
- Kordelas, L., Rebmann, V., Ludwig, A. K., Radtke, S., Ruesing, J., Doeppner, T. R., … Giebel, B. (2014). MSC-derived exosomes: A novel tool to treat therapy-refractory graft-versus-host disease. Leukemia , 28, 970–973. doi: 10.1038/leu.2014.41.
- Lener, T., Gimona, M., Aigner, L., Borger, V., Buzas, E., Camussi, G., … Giebel, B. (2015). Applying extracellular vesicles based therapeutics in clinical trials—an ISEV position paper. Journal of Extracellular Vesicles , 4, 30087. doi: 10.3402/jev.v4.30087.
- Ludwig, A. K., De Miroschedji, K., Doeppner, T. R., Borger, V., Ruesing, J., Rebmann, V., … Giebel, B. (2018). Precipitation with polyethylene glycol followed by washing and pelleting by ultracentrifugation enriches extracellular vesicles from tissue culture supernatants in small and large scales. Journal of Extracellular Vesicles , 7, 1528109. doi: 10.1080/20013078.2018.1528109.
- Nguyen, D. G., Booth, A., Gould, S. J., & Hildreth, J. E. (2003). Evidence that HIV budding in primary macrophages occurs through the exosome release pathway. Journal of Biological Chemistry , 278, 52347–52354. doi: 10.1074/jbc.M309009200.
- Nordin, J. Z., Bostancioglu, R. B., Corso, G., & El Andaloussi, S. (2019). Tangential flow filtration with or without subsequent bind-elute size exclusion chromatography for purification of extracellular vesicles. Methods in Molecular Biology , 1953, 287–299. doi: 10.1007/978-1-4939-9145-7_18.
- Ophelders, D. R., Wolfs, T. G., Jellema, R. K., Zwanenburg, A., Andriessen, P., Delhaas, T., … Kramer, B. W. (2016). Mesenchymal stromal cell-derived extracellular vesicles protect the fetal brain after hypoxia-ischemia. Stem Cells Translational Medicine , 5, 754–763. doi: 10.5966/sctm.2015-0197.
- Pittenger, M. F., Mackay, A. M., Beck, S. C., Jaiswal, R. K., Douglas, R., Mosca, J. D., … Marshak, D. R. (1999). Multilineage potential of adult human mesenchymal stem cells. Science , 284, 143–147. doi: 10.1126/science.284.5411.143.
- Phelan, K. & May, K. M. (2015). Basic techniques in mammalian cell tissue culture. Current Protocols in Cell Biology , 66, 1.1.1–1.1.22. doi: 10.1002/0471143030.cb0101s66.
- Thery, C., Amigorena, S., Raposo, G., & Clayton, A. (2006). Isolation and characterization of exosomes from cell culture supernatants and biological fluids. Current Protocols in Cell Biology , 30, 3.22.1–3.22.29. doi: 10.1002/0471143030.cb0322s30.
- Vajda, B. P. (1978). Concentration and purification of viruses and bacteriophages with polyethylene glycol. Folia Microbiologica , 23, 88–96. doi: 10.1007/BF02876605.
- Wang, C., Börger, V., Sardari, M., Murke, F., Skuljec, J., Pul, R., … Hermann, D. M. (2020). Mesenchymal stromal cell-derived small extracellular vesicles induce ischemic neuroprotection by modulating leukocytes and specifically neutrophils. Stroke , 51(6), 1825–1834. doi: 10.1161/STROKEAHA.119.028012.
- Watson, D. C., Yung, B. C., Bergamaschi, C., Chowdhury, B., Bear, J., Stellas, D., … Pavlakis, G. N. (2018). Scalable, cGMP-compatible purification of extracellular vesicles carrying bioactive human heterodimeric IL-15/lactadherin complexes. Journal of Extracellular Vesicles , 7, 1442088. doi: 10.1080/20013078.2018.1442088.
Citing Literature
Number of times cited according to CrossRef: 34
- Xinya Zheng, Hongru Ai, Kewen Qian, Guangyao Li, Shuyi Zhang, Yitan Zou, Changhai Lei, Wenyan Fu, Shi Hu, Small extracellular vesicles purification and scale-up, Frontiers in Immunology, 10.3389/fimmu.2024.1344681, 15 , (2024).
- Timo Wadenpohl, Mikhail Shein, Julia Steinberg, Julian Bernhardt Lehmann, Anne Kathrin Schütz, Stephanie Jung, Economical large-scale purification of extracellular vesicles from urine, Separation and Purification Technology, 10.1016/j.seppur.2023.126155, 335 , (126155), (2024).
- Yanis Mouloud, Simon Staubach, Oumaima Stambouli, Shakiba Mokhtari, Tanja J Kutzner, Denise Zwanziger, Hatim Hemeda, Bernd Giebel, Calcium chloride declotted human platelet lysate promotes the expansion of mesenchymal stromal cells and allows manufacturing of immunomodulatory active extracellular vesicle products, Cytotherapy, 10.1016/j.jcyt.2024.04.069, 26 , 9, (988-998), (2024).
- Ricardo Malvicini, Giada De Lazzari, Anna Maria Tolomeo, Diego Santa-Cruz, Mujib Ullah, Carmine Cirillo, Paolo Grumati, Natalia Pacienza, Maurizio Muraca, Gustavo Yannarelli, Influence of the isolation method on characteristics and functional activity of mesenchymal stromal cell-derived extracellular vesicles, Cytotherapy, 10.1016/j.jcyt.2023.11.001, 26 , 2, (157-170), (2024).
- Sitong Zhang, Qiyue Wang, Daniel En Liang Tan, Vritika Sikka, Cheng Han Ng, Yan Xian, Dan Li, Mark Muthiah, Nicholas W. S. Chew, Gert Storm, Lingjun Tong, Jiong‐Wei Wang, Gut‐liver axis: Potential mechanisms of action of food‐derived extracellular vesicles, Journal of Extracellular Vesicles, 10.1002/jev2.12466, 13 , 6, (2024).
- Vivian V. T. Nguyen, Joshua A. Welsh, Tobias Tertel, Andre Choo, Simonides I. Wakker, Kyra A. Y. Defourny, Bernd Giebel, Pieter Vader, Jayanthi Padmanabhan, Sai Kiang Lim, Esther N. M. Nolte‐'t Hoen, Marianne C. Verhaar, R. Beklem Bostancioglu, Antje M. Zickler, Jia Mei Hong, Jennifer C. Jones, Samir EL Andaloussi, Bas W. M. Balkom, André Görgens, Inter‐laboratory multiplex bead‐based surface protein profiling of MSC‐derived EV preparations identifies MSC‐EV surface marker signatures, Journal of Extracellular Vesicles, 10.1002/jev2.12463, 13 , 6, (2024).
- Andreas Nicodemou, Soňa Bernátová, Michaela Čeháková, Ľuboš Danišovič, Emerging Roles of Mesenchymal Stem/Stromal-Cell-Derived Extracellular Vesicles in Cancer Therapy, Pharmaceutics, 10.3390/pharmaceutics15051453, 15 , 5, (1453), (2023).
- Manho Kim, Hyejun Jang, Wijin Kim, Doyeon Kim, Ju Hyun Park, Therapeutic Applications of Plant-Derived Extracellular Vesicles as Antioxidants for Oxidative Stress-Related Diseases, Antioxidants, 10.3390/antiox12061286, 12 , 6, (1286), (2023).
- Manho Kim, Hyejun Jang, Ju Hyun Park, Balloon Flower Root-Derived Extracellular Vesicles: In Vitro Assessment of Anti-Inflammatory, Proliferative, and Antioxidant Effects for Chronic Wound Healing, Antioxidants, 10.3390/antiox12061146, 12 , 6, (1146), (2023).
- Robert Ossendorff, Sibylle Grad, Tobias Tertel, Dieter C. Wirtz, Bernd Giebel, Verena Börger, Frank A. Schildberg, Immunomodulatory potential of mesenchymal stromal cell-derived extracellular vesicles in chondrocyte inflammation, Frontiers in Immunology, 10.3389/fimmu.2023.1198198, 14 , (2023).
- Tobias Tertel, Robin Dittrich, Pierre Arsène, Arne Jensen, Bernd Giebel, EV products obtained from iPSC-derived MSCs show batch-to-batch variations in their ability to modulate allogeneic immune responses in vitro, Frontiers in Cell and Developmental Biology, 10.3389/fcell.2023.1282860, 11 , (2023).
- Viktoria Jakl, Melanie Ehmele, Martina Winkelmann, Simon Ehrenberg, Tim Eiseler, Benedikt Friemert, Markus Thomas Rojewski, Hubert Schrezenmeier, A novel approach for large-scale manufacturing of small extracellular vesicles from bone marrow-derived mesenchymal stromal cells using a hollow fiber bioreactor, Frontiers in Bioengineering and Biotechnology, 10.3389/fbioe.2023.1107055, 11 , (2023).
- Nicole Labusek, Yanis Mouloud, Christian Köster, Eva Diesterbeck, Tobias Tertel, Constanze Wiek, Helmut Hanenberg, Peter A. Horn, Ursula Felderhoff-Müser, Ivo Bendix, Bernd Giebel, Josephine Herz, Extracellular vesicles from immortalized mesenchymal stromal cells protect against neonatal hypoxic-ischemic brain injury, Inflammation and Regeneration, 10.1186/s41232-023-00274-6, 43 , 1, (2023).
- Nicole Labusek, Parnian Ghari, Yanis Mouloud, Christian Köster, Eva Diesterbeck, Martin Hadamitzky, Ursula Felderhoff-Müser, Ivo Bendix, Bernd Giebel, Josephine Herz, Hypothermia combined with extracellular vesicles from clonally expanded immortalized mesenchymal stromal cells improves neurodevelopmental impairment in neonatal hypoxic-ischemic brain injury, Journal of Neuroinflammation, 10.1186/s12974-023-02961-0, 20 , 1, (2023).
- Soraya Williams, Maria Fernandez-Rhodes, Alice Law, Ben Peacock, Mark P. Lewis, Owen G. Davies, Comparison of extracellular vesicle isolation processes for therapeutic applications, Journal of Tissue Engineering, 10.1177/20417314231174609, 14 , (2023).
- Soraya Williams, Aveen R Jalal, Mark P Lewis, Owen G Davies, A survey to evaluate parameters governing the selection and application of extracellular vesicle isolation methods, Journal of Tissue Engineering, 10.1177/20417314231155114, 14 , (2023).
- Faezeh Shekari, Morteza Abyadeh, Anna Meyfour, Mehdi Mirzaei, Nitin Chitranshi, Vivek Gupta, Stuart L. Graham, Ghasem Hosseini Salekdeh, Extracellular vesicles as reconfigurable therapeutics for eye diseases: Promises and hurdles, Progress in Neurobiology, 10.1016/j.pneurobio.2023.102437, 225 , (102437), (2023).
- Michel Bremer, Fabiola Nardi Bauer, Tobias Tertel, Robin Dittrich, Peter A. Horn, Verena Börger, Bernd Giebel, Qualification of a multidonor mixed lymphocyte reaction assay for the functional characterization of immunomodulatory extracellular vesicles, Cytotherapy, 10.1016/j.jcyt.2023.03.009, 25 , 8, (847-857), (2023).
- Rabea J. Madel, Verena Börger, Robin Dittrich, Michel Bremer, Tobias Tertel, Nhi Ngo Thi Phuong, Hideo A. Baba, Lambros Kordelas, Simon Staubach, Frank Stein, Per Haberkant, Matthias Hackl, Regina Grillari, Johannes Grillari, Jan Buer, Peter A. Horn, Astrid M. Westendorf, Sven Brandau, Carsten J. Kirschning, Bernd Giebel, Independent human mesenchymal stromal cell–derived extracellular vesicle preparations differentially attenuate symptoms in an advanced murine graft-versus-host disease model, Cytotherapy, 10.1016/j.jcyt.2023.03.008, 25 , 8, (821-836), (2023).
- Fabiola Nardi Bauer, Tobias Tertel, Oumaima Stambouli, Chen Wang, Robin Dittrich, Simon Staubach, Verena Börger, Dirk M. Hermann, Sven Brandau, Bernd Giebel, CD73 activity of mesenchymal stromal cell-derived extracellular vesicle preparations is detergent-resistant and does not correlate with immunomodulatory capabilities, Cytotherapy, 10.1016/j.jcyt.2022.09.006, 25 , 2, (138-147), (2023).
- Manho Kim, Ju Hyun Park, Isolation of Aloe saponaria-Derived Extracellular Vesicles and Investigation of Their Potential for Chronic Wound Healing, Pharmaceutics, 10.3390/pharmaceutics14091905, 14 , 9, (1905), (2022).
- Maja Kosanović, Bojana Milutinovic, Sofija Glamočlija, Ingrid Mena Morlans, Alberto Ortiz, Milica Bozic, Extracellular Vesicles and Acute Kidney Injury: Potential Therapeutic Avenue for Renal Repair and Regeneration, International Journal of Molecular Sciences, 10.3390/ijms23073792, 23 , 7, (3792), (2022).
- Ciarra Almeria, Sebastian Kreß, Viktoria Weber, Dominik Egger, Cornelia Kasper, Heterogeneity of mesenchymal stem cell-derived extracellular vesicles is highly impacted by the tissue/cell source and culture conditions, Cell & Bioscience, 10.1186/s13578-022-00786-7, 12 , 1, (2022).
- Yolande F. M. Ramos, Tobias Tertel, Georgina Shaw, Simon Staubach, Rodrigo Coutinho de Almeida, Eka Suchiman, Thomas B. Kuipers, Hailiang Mei, Frank Barry, Mary Murphy, Bernd Giebel, Ingrid Meulenbelt, Characterizing the secretome of licensed hiPSC-derived MSCs, Stem Cell Research & Therapy, 10.1186/s13287-022-03117-2, 13 , 1, (2022).
- Duc M. Hoang, Phuong T. Pham, Trung Q. Bach, Anh T. L. Ngo, Quyen T. Nguyen, Trang T. K. Phan, Giang H. Nguyen, Phuong T. T. Le, Van T. Hoang, Nicholas R. Forsyth, Michael Heke, Liem Thanh Nguyen, Stem cell-based therapy for human diseases, Signal Transduction and Targeted Therapy, 10.1038/s41392-022-01134-4, 7 , 1, (2022).
- Tobias Tertel, Melanie Schoppet, Oumaima Stambouli, Ali Al-Jipouri, Patrick F. James, Bernd Giebel, Imaging flow cytometry challenges the usefulness of classically used extracellular vesicle labeling dyes and qualifies the novel dye Exoria for the labeling of mesenchymal stromal cell–extracellular vesicle preparations, Cytotherapy, 10.1016/j.jcyt.2022.02.003, 24 , 6, (619-628), (2022).
- Biji Mathew, Leianne A. Torres, Lorea Gamboa Acha, Sophie Tran, Alice Liu, Raj Patel, Mohansrinivas Chennakesavalu, Anagha Aneesh, Chun-Chieh Huang, Douglas L. Feinstein, Shafigh Mehraeen, Sriram Ravindran, Steven Roth, Uptake and Distribution of Administered Bone Marrow Mesenchymal Stem Cell Extracellular Vesicles in Retina, Cells, 10.3390/cells10040730, 10 , 4, (730), (2021).
- Lien Van Hoecke, Caroline Van Cauwenberghe, Verena Börger, Arnout Bruggeman, Jonas Castelein, Griet Van Imschoot, Elien Van Wonterghem, Robin Dittrich, Wouter Claeys, Junhua Xie, Bernd Giebel, Roosmarijn E. Vandenbroucke, Anti-Inflammatory Mesenchymal Stromal Cell-Derived Extracellular Vesicles Improve Pathology in Niemann–Pick Type C Disease, Biomedicines, 10.3390/biomedicines9121864, 9 , 12, (1864), (2021).
- Jinbing He, Shuai Ping, Fangyang Yu, Xi Yuan, Jiang Wang, Jun Qi, Mesenchymal Stem Cell-Derived Exosomes: Therapeutic Implications for Rotator Cuff Injury, Regenerative Medicine, 10.2217/rme-2020-0183, 16 , 8, (803-815), (2021).
- Yao Sun, Guoliang Liu, Kai Zhang, Qian Cao, Tongjun Liu, Jiannan Li, Mesenchymal stem cells-derived exosomes for drug delivery, Stem Cell Research & Therapy, 10.1186/s13287-021-02629-7, 12 , 1, (2021).
- Simon Staubach, Fabiola Nardi Bauer, Tobias Tertel, Verena Börger, Oumaima Stambouli, Denise Salzig, Bernd Giebel, Scaled preparation of extracellular vesicles from conditioned media, Advanced Drug Delivery Reviews, 10.1016/j.addr.2021.113940, 177 , (113940), (2021).
- Zi-Yuan Feng, Qing-Yi Zhang, Jie Tan, Hui-Qi Xie, Techniques for increasing the yield of stem cell-derived exosomes: what factors may be involved?, Science China Life Sciences, 10.1007/s11427-021-1997-2, 65 , 7, (1325-1341), (2021).
- Jonas Gregorius, Chen Wang, Oumaima Stambouli, Tanja Hussner, Yachao Qi, Tobias Tertel, Verena Börger, Ayan Mohamud Yusuf, Nina Hagemann, Dongpei Yin, Robin Dittrich, Yanis Mouloud, Fabian D. Mairinger, Fouzi El Magraoui, Aurel Popa-Wagner, Christoph Kleinschnitz, Thorsten R. Doeppner, Matthias Gunzer, Helmut E. Meyer, Bernd Giebel, Dirk M. Hermann, Small extracellular vesicles obtained from hypoxic mesenchymal stromal cells have unique characteristics that promote cerebral angiogenesis, brain remodeling and neurological recovery after focal cerebral ischemia in mice, Basic Research in Cardiology, 10.1007/s00395-021-00881-9, 116 , 1, (2021).
- Daniel Besser, Advancing Stem Cell Technologies and Applications: A Special Collection from the PluriCore Network in the German Stem Cell Network (GSCN), Current Protocols in Stem Cell Biology, 10.1002/cpsc.129, 55 , 1, (2020).