Triplet-Primed PCR Assays for Accurate Screening of FMR1 CGG Repeat Expansion and Genotype Verification
Indhu-Shree Rajan-Babu, Indhu-Shree Rajan-Babu, Mulias Lian, Mulias Lian, Samuel S. Chong, Samuel S. Chong
capillary electrophoresis
FMR1
fragile X syndrome
melting curve analysis
screening
triplet-primed PCR
Abstract
Fragile X syndrome and other fragile X‒associated disorders are caused by the full-mutation (>200 copies) and premutation (55 to 200 copies) expansion, respectively, of the CGG short tandem repeat in the fragile X messenger ribonucleoprotein 1 (FMR1) gene. Clinical diagnostic laboratories use Southern blot analysis and polymerase chain reaction (PCR)-based tests to detect and/or size the FMR1 CGG repeats. The development of sensitive and high-throughput triplet-primed PCR (TP-PCR) assays has diminished the need to subject all samples to Southern blot analysis, which is both labor- and time-intensive. In this article, we describe two direct TP-PCR (dTP-PCR) assays for the detection of FMR1 CGG repeat expansions. We outline a protocol that is based on melting curve analysis of dTP-PCR amplicons for a rapid and cost-effective first-tier screening and identification of individuals with premutation and full-mutation expansions. We also describe a protocol that employs capillary electrophoresis to resolve the dTP-PCR amplicon fragments and to estimate the repeat sizes of normal (5 to 44 copies), intermediate (45 to 54 copies), and premutation alleles, as well as to detect full mutations and determine the structure of the FMR1 alleles. © 2022 The Authors. Current Protocols published by Wiley Periodicals LLC.
Basic Protocol 1 : Direct triplet-primed PCR master mix preparation and amplification of the FMR1 CGG repeat locus for melting curve analysis
Basic Protocol 2 : Melting curve analysis of direct triplet-primed PCR amplicons on the Rotor-Gene Q MD × 5plex high-resolution melt platform
Alternate Protocol : Melting curve analysis of direct triplet-primed PCR amplicons on the LightCycler 480 system
Basic Protocol 3 : Generation of direct triplet-primed PCR melting curve analysis profiles
Basic Protocol 4 : Direct triplet-primed PCR master mix preparation and amplification of the FMR1 CGG repeat locus for capillary electrophoresis
Basic Protocol 5 : Generation of control FMR1 plasmids for direct triplet-primed PCR melting curve analysis
Basic Protocol 6 : Sanger sequencing assay to verify FMR1 CGG repeat size and structure of plasmid DNA controls
INTRODUCTION
Fragile X syndrome (FXS; MIM no. 300624) is the most common single-gene cause of intellectual disability and autism spectrum disorder (Hagerman et al., 2017). In >99% of patients with FXS, the molecular cause is expansion of a CGG short tandem repeat sequence in the 5′-untranslated region of the fragile X messenger ribonucleoprotein 1 (FMR1 ; MIM no. 309550) gene (Hunter, Berry-Kravis, Hipp, & Todd, 1993; Verkerk et al., 1991). About 1:7000 males and 1:11,000 females harbor the FXS-causing full-mutation expansions with >200 CGG repeats (Hunter et al., 2014). In contrast, premutation alleles with 55 to 200 CGG repeats are more common in the general population and occur in 1:850 males and 1:300 females (Hunter et al., 2014). Carriers of premutation alleles may develop fragile X–associated tremor/ataxia syndrome (MIM no. 300623), fragile X–associated primary ovarian insufficiency (MIM no. 311360), or fragile X–associated neuropsychiatric disorder later in their life (Hunter et al., 1993). Moreover, premutation alleles, especially those in the upper end of the repeat size spectrum, tend to expand further during meiosis. In women with premutation with ≥90 CGG repeats, Nolin et al. reported an 80% to 100% risk of full-mutation transmission to their offspring (Nolin et al., 2003). In addition to the maternal CGG repeat size, the density of AGG interruptions within the premutation alleles can influence the full-mutation transmission risk (Nolin et al., 2013, 2019). AGG interruptions add stability to the FMR1 alleles by inhibiting the formation of secondary structures around CGG repeats that induce strand slippage and repeat expansion (Latham, Coppinger, Hadd, & Nolin, 2014). In general, the expanded FMR1 alleles lack or have fewer AGG interruptions compared with normal alleles with 5 to 44 CGG repeats that are more stable. Intermediate alleles with 45 to 54 CGG repeats exhibit some degree of instability and may expand into a premutation or full mutation in the subsequent generations when the alleles are large and uninterrupted (Nolin et al., 2013). Therefore, precise CGG repeat size determination or FMR1 genotyping and AGG interruption mapping are critical in the molecular diagnostic evaluation of FXS.
Clinical diagnostic laboratories use Southern blot and polymerase chain reaction (PCR)-based tests for FXS diagnosis (Spector et al., 2021). Triplet-primed (TP)-PCR-based FMR1 tests (Chen et al., 2010, 2011; Hantash et al., 2010; Rajan-Babu, Law, Yoon, Lee, & Chong, 2015; Rajan-Babu, Teo, et al., 2015) are more sensitive and have a significantly shorter turnaround time and higher throughput compared with Southern blot analysis. Increased reliance on PCR-based assays has reduced the requirement to perform Southern blot analysis on all cases (Spector et al., 2021) and has enabled more rapid processing of large volumes of samples (Bailey et al., 2017; Lee et al., 2020; Tassone et al., 2012). In contrast to conventional PCR that may fail to amplify larger expansions, TP-PCRs will detect all FMR1 full mutations, irrespective of the number of repeats they contain. This is possible because TP-PCRs use a three-primer system (Rajan-Babu & Chong, 2019), which includes a fluorophore-labeled, locus-specific primer that hybridizes to the unique sequence either 5′ or 3′ of the CGG repeats; a TP primer with CGG repeats at its 3′ end to enable random annealing to the FMR1 CGG repeat sequence; and a tail primer that is complementary to the 5′ overhanging sequence of the TP primer to extend and amplify the TP primer products. Owing to this design, in samples with expansion, TP-PCR will produce highly heterogeneous amplicon fragments that exhibit a characteristic “stutter” pattern upon electrophoresis. Full-mutation cases will have TP-PCR amplicon peaks that cross the 200-repeat pathogenicity threshold for FXS. Coupling TP-PCRs with capillary electrophoresis (CE) allows reliable FMR1 full-mutation detection and characterization of AGG interruption patterns, in addition to the accurate sizing of alleles in the normal to premutation size ranges and resolving zygosity in females (Chen et al., 2010; Rajan-Babu, Law, et al., 2015; Rajan-Babu, Teo, et al., 2015).
FXS molecular testing of newborns and women of reproductive age can enable identification of individuals with FMR1 expansions who are likely to benefit from diagnosis and post-test genetic counseling (Hunter et al., 1993). The reported average age of diagnosis of FXS is ∼35 to 37 months in boys and 41.6 months in girls (Bailey, Raspa, Bishop, & Holiday, 2009). This delay in FXS diagnosis impedes the implementation of treatments and interventions that are most effective when started within the first year (Okoniewski et al., 2019) and also increases the risk of families who are unaware of the genetic cause in their first child getting pregnant with their second child (Bailey et al., 2009). One of the factors that have hampered large-scale screening efforts targeted toward the identification of individuals with FMR1 expansions is the lack of a cost-effective, sensitive, and scalable FXS genetic test (Okoniewski et al., 2019; Riley & Wheeler, 2017). To address the need for a rapid first-tier FXS screening test, we developed a direct TP-PCR and melting curve analysis (dTP-PCR MCA) assay (Rajan-Babu, Law, et al., 2015), which is cost-effective (US$3.20 per sample), scalable (∼1500 samples in 24 hr), and 100% sensitive in detecting FMR1 expansions and their low-level mosaicisms (down to 1% full mutation in the presence of 99% normal allele). The dTP-PCR MCA assay uses internal size controls (i.e., one or two samples with a known CGG repeat size) to establish the thresholds to demarcate the profiles of expanded and nonexpanded samples and does not differentiate premutation and full-mutation samples. To further precisely genotype the FMR1 alleles and to characterize the AGG interruptions in samples that are positive for an expansion in the dTP-PCR MCA screening, we developed a complementary CE-based method, the dTP-PCR CE (Rajan-Babu, Law, et al., 2015).
In this article, we present protocols to implement the screening (dTP-PCR MCA) and genotyping (dTP-PCR CE) methods. We also describe a protocol to isolate, purify, and clone FMR1 fragments with desired CGG repeat size to create custom plasmid DNAs for use as internal controls in the dTP-PCR MCA assay. First, we provide details on preparation of the dTP-PCR master mix and amplification of the FMR1 CGG repeat locus for MCA (Basic Protocol 1). Second, we describe the MCA program setup for dTP-PCR amplicons on the Rotor-Gene Q (RGQ) MD × 5plex High-Resolution Melt (HRM) Platform (Basic Protocol 2) or LightCycler 480 System (Alternate Protocol). Third, we provide details on the generation and analysis of dTP-PCR MCA profiles (Basic Protocol 3). Fourth, we explain preparation of the dTP-PCR master mix and amplification of the FMR1 CGG repeat locus for CE (Basic Protocol 4). Lastly, we outline the protocol for amplification, isolation, purification, and cloning of the FMR1 amplicon fragments (Basic Protocol 5) and a Sanger sequencing protocol to verify CGG repeat size and structure of the cloned FMR1 plasmid controls (Basic Protocol 6).
Basic Protocol 1: DIRECT TRIPLET-PRIMED PCR MASTER MIX PREPARATION AND AMPLIFICATION OF THE FMR1 CGG REPEAT LOCUS FOR MELTING CURVE ANALYSIS
This protocol outlines preparation of the master mix for dTP-PCR amplification of the FMR1 CGG repeat locus for MCA of the generated amplicons.
Materials
-
70% ethanol
-
DNA AWAY surface decontaminant (e.g., Thermo Scientific, cat. no. 7010)
-
100 ng/µl test genomic DNA sample
-
100 ng/µl control genomic DNA (e.g., Coriell Institute, sample ID NA20232)
-
100 ng/µl control genomic DNA (e.g., Coriell Institute, sample ID NA20230)
-
FMR1 -specific reverse primer, f : 5′-AGCCCCGCACTTCCACCACCAGCTCCTCCA-3′ (Fu et al., 1991)
-
Tail primer: 5′-TGCTCTGGACCCTGAAGTGTGCCGTTGATA-3′
-
TP primer: 5′-TGCTCTGGACCCTGAAGTGTGCCGTTGATA[CGG]5-3′
-
HotStarTaq DNA polymerase (e.g., Qiagen, cat. no. 203205) containing:
- Q-solution
- HotStarTaq PCR buffer
- HotStarTaq PCR polymerase
-
Water, HPLC grade (e.g., Sigma-Aldrich, cat. no. 270733)
-
dNTPs, PCR grade (e.g., Roche Applied Science, cat. no. 11969064001)
-
10,000× SYBR Green I Nucleic Acid Gel Stain in DMSO (e.g., Invitrogen, cat. no. S7563)
-
10× Tris-EDTA (TE) buffer, pH 8.0, ultrapure (e.g., 1st BASE, cat. no. BUF-3020-10)
-
Minicentrifuge (e.g., micro ONE Mini Personal Centrifuge; TOMY, model no. Capsulefuge PMC-880)
-
1.5-ml nonstick, RNase-free microcentrifuge tubes (e.g., Applied Biosystems, cat. no. AM12450)
-
0.2-ml MicroAmp reaction tube with cap (e.g., Applied Biosystems, cat. no. N8010540) or PCR tubes
-
Vortex mixer (e.g., Genie 2; Scientific Industries, model no. G-560E)
-
Thermal cycler (e.g., GeneAmp PCR System 9700; Applied Biosystems, cat. no. 4339386)
Master mix preparation
1.Using appropriate personal protective equipment, decontaminate benchtop thoroughly with 70% ethanol and then with DNA AWAY.
2.Thaw reagents and test and control genomic DNA samples on ice.
3.Flick and then spin down reagent, primer, and genomic DNA in the minicentrifuge before setting up the PCR.
4.Prepare dTP-PCR master mix on ice by carefully dispensing the following reagents into a clean 1.5-ml microcentrifuge tube in the given order:
- 7.50 µl of 5× Q-solution
- 1.50 µl of 10× HotStarTaq PCR buffer
- 1.21 µl HPLC-grade water
- 1.00 µl HotStarTaq DNA polymerase
- 0.90 µl of 10 µM FMR1 -specific reverse primer, f
- 0.36 µl of 25 µM tail primer
- 0.36 µl of 25 nM TP primer
- 0.26 µl of 50 mM dGTP
- 0.26 µl of 50 mM dCTP
- 0.26 µl of 10 mM dATP
- 0.26 µl of 10 mM dTTP
- 0.15 µl of 10× SYBR Green I Nucleic Acid Gel Stain.
Use aerosol-barrier pipette tips to minimize contamination.
Ensure the reagents, DNA samples, and master mix are placed on ice throughout the assay setup to prevent reagent degradation and suboptimal results.
Note that SYBR Green is light sensitive. Wrap the SYBR Green and master mix tubes with aluminum foil to avoid direct light exposure.
The reagent volumes listed are for a single reaction. To calculate the total volume required for the assay, simply multiply the volumes provided with the total number of reactions.
We recommend that internal CGG repeat size controls (e.g., NA20232, 46 repeats; NA20230, 54 repeats) are included in each MCA setup to differentiate the melt peaks of samples with FMR1 premutation and full-mutation expansions from those of the normal or nonexpanded samples. In addition, a negative or nontemplate PCR control is essential in each run to ensure the absence of amplicon or DNA contaminants in the reagents used.
To generate plasmid DNA controls for MCA, follow Basic Protocol 5 to amplify, isolate, purify, and clone fragments of desired FMR1 CGG repeat counts.
5.Mix master mix by pipetting up and down.
6.Spin down master mix tube in the minicentrifuge.
7.Label 0.2-ml MicroAmp reaction tubes or PCR tubes, and place on ice.
8.Aliquot 14 µl master mix into each labeled PCR tube.
9.Add 1 µl of 100 ng/μl test genomic DNA and control genomic DNA into the appropriate PCR tubes. To the nontemplate PCR control tube, add 1 µl HPLC-grade water.
10.Vortex or flick PCR tubes briefly, and then spin down in the minicentrifuge.
dTP-PCR amplification
11.Set the following program on the thermal cycler, and then transfer PCR tubes into the instrument and start the amplification:
1 cycle | 15 min | 95°C | (initial denaturation) |
40 cycles | 45 s | 99°C | (denaturation) |
45 s | 55°C | (annealing) | |
8 min | 70°C | (extension) | |
1 cycle | 10 min | 72°C | (final extension) |
Hold | indefinitely | 4°C. |
12.Remove PCR tubes once the thermal cycler enters the hold step.
13.Briefly spin tubes in a minicentrifuge to ensure the amplicons are collected down and are ready to be transferred for MCA.
Basic Protocol 2: MELTING CURVE ANALYSIS OF DIRECT TRIPLET-PRIMED PCR AMPLICONS ON THE ROTOR-GENE Q MD × 5plex HIGH-RESOLUTION MELT PLATFORM
This protocol outlines the program for MCA on the RGQ MD × 5plex HRM Platform (Rajan-Babu et al., 2016).
Materials
- Amplicons of interest (see Basic Protocol 1)
- 0.1-ml strip tubes and caps (e.g., Qiagen, cat. no. 981103)
- Minicentrifuge (e.g., mySPIN 6 Mini Centrifuge; Thermo Scientific, cat. no. 75004061)
- RGQ MD × 5plex HRM Platform (e.g., Qiagen, cat. no. 9002370)
1.Transfer amplicons into 0.1-ml strip tubes.
2.Apply caps and spin strip tubes down in the minicentrifuge.
3.Transfer strip tubes into the RGQ MD × 5plex Platform.
4.Program the HRM module as follows and initiate analysis:
1 cycle | 1 min | 95°C | (denaturation) |
Ramp | 0.50°C | 65°C to 95°C | (temperature ramp; include 5-s hold at each step). |
Alternate Protocol: MELTING CURVE ANALYSIS OF DIRECT TRIPLET-PRIMED PCR AMPLICONS ON THE LightCycler 480 SYSTEM
This protocol outlines the program for MCA on the LightCycler 480 System (Rajan-Babu, Law, et al., 2015).
Materials
- Amplicons of interest (see Basic Protocol 1)
- 96- or 384-well LightCycler 480 Multiwell Plate, white (e.g., Roche Applied Science, cat. nos. 04729692001 and 04729749001, respectively)
- Microcentrifuge with plate adaptor (e.g., Eppendorf, model no. 5810)
- LightCycler 480 System (e.g., Roche Applied Science)
1.Transfer amplicons into the LightCycler 480 Multiwell Plate and seal plate.
2.Spin down plate in a microcentrifuge with plate adaptor 1 min at 100 × g , room temperature.
3.Transfer plate to the LightCycler 480 System.
4.Set up the MCA program as follows and initiate analysis:
1 cycle | 1 min | 95°C | (denaturation) |
1 cycle | 1 min | 60°C | (temperature hold-step) |
Ramp | 0.01°C/s | 60°C to 95°C | (temperature ramp). |
Basic Protocol 3: GENERATION OF DIRECT TRIPLET-PRIMED PCR MELTING CURVE ANALYSIS PROFILES
This protocol outlines the steps in plotting the melt peaks of the test and control DNA samples.
Materials
- MCA data from RGQ HRM or LightCycler 480 run exported in text (.txt) format (see Basic Protocol 2 or Alternate Protocol)
- Microsoft Excel
1.Open exported MCA data file in Microsoft Excel.
2.Select one of the temperature columns and the –d F /d T values of the samples and controls of interest, and choose “X Y (Scatter)” from the Charts option under the Insert tab. Then select “Scatter with Smooth Lines” to create the melt peak plots.
3.Format minimum and maximum bounds of the x - and y -axis, as required.
4.Observe melt peak profiles to identify samples with an expanded allele that display a higher melt peak temperature (Tm) relative to the internal repeat size controls used.
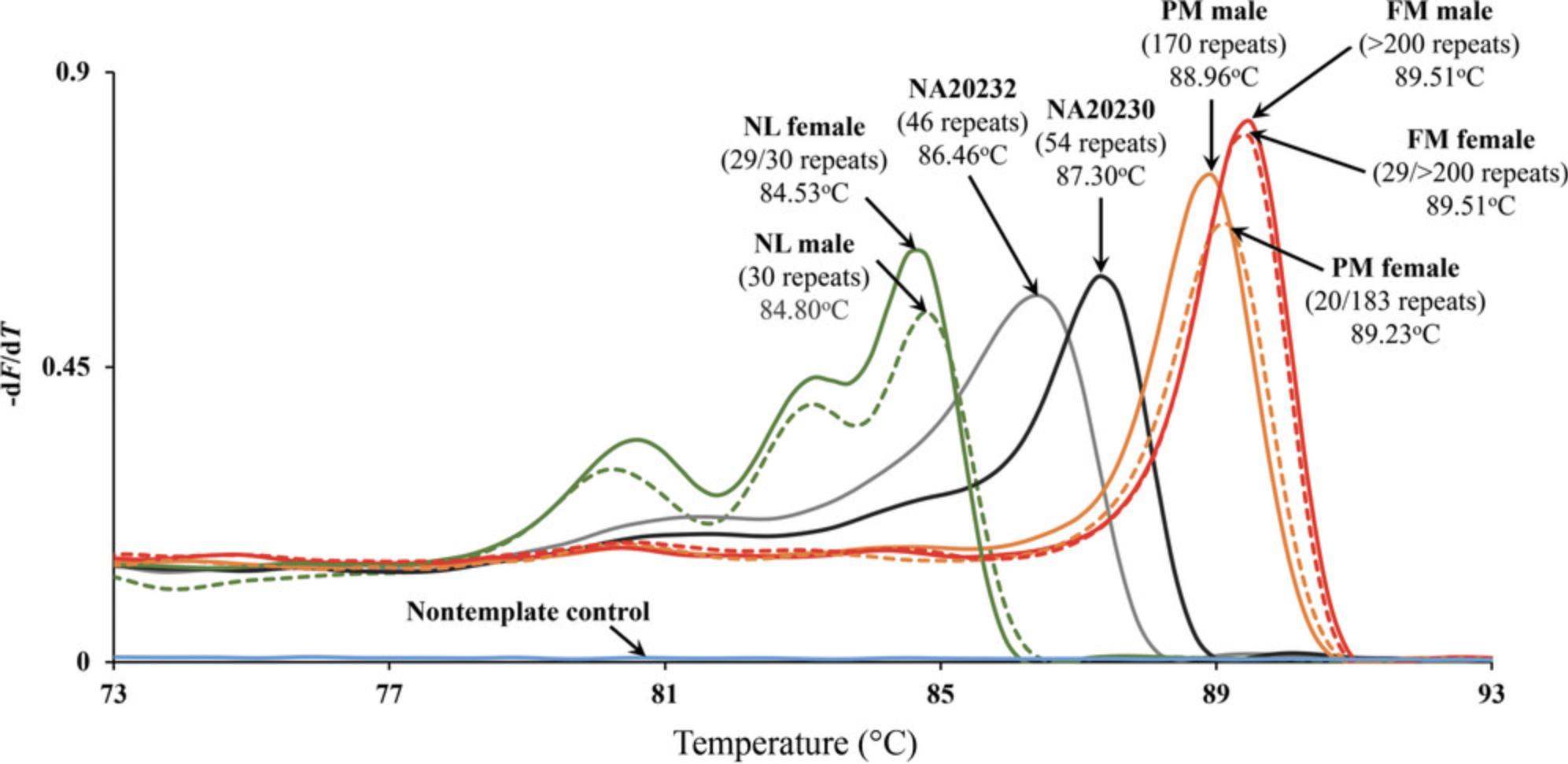
5.Mark samples with potential expansion for repeat size verification with dTP-PCR CE (see Basic Protocol 4).
Basic Protocol 4: DIRECT TRIPLET-PRIMED PCR MASTER MIX PREPARATION AND AMPLIFICATION OF THE FMR1 CGG REPEAT LOCUS FOR CAPILLARY ELECTROPHORESIS
FMR1 genotypes (normal, intermediate, premutation, and full mutation) of the samples and the repeat structure (i.e., presence and distribution of AGG interruptions) can be verified using dTP-PCR CE. This protocol outlines the master mix preparation and dTP-PCR amplification for CE and FMR1 genotype analysis of the generated amplicons.
Materials
-
HotStarTaq DNA polymerase (e.g., Qiagen, cat. no. 203205) containing:
- Q-solution
- HotStarTaq PCR buffer
- HotStarTaq PCR polymerase
-
Taq extender PCR additive (e.g., Agilent Technologies, cat. no. 600148)
-
Water, HPLC grade (e.g., Sigma-Aldrich, cat. no. 270733)
-
Fam -labeled FMR1 -specific reverse primer, f : 5′-AGCCCCGCACTTCCACCACCAGCTCCTCCA-3′ (Fu et al., 1991)
-
Tail primer: 5′-TGCTCTGGACCCTGAAGTGTGCCGTTGATA-3′
-
TP primer: 5′-TGCTCTGGACCCTGAAGTGTGCCGTTGATA[CGG]5-3′
-
dNTPs, PCR grade (e.g., Roche Applied Science, cat. no. 11969064001)
-
100 ng/µl genomic DNA sample
-
100 ng/µl control genomic DNA (e.g., Coriell Institute)
-
10× TE buffer, pH 8.0, ultrapure (e.g., 1st BASE, cat. no. BUF-3020-10)
-
Hi-Di formamide (e.g., Applied Biosystems, cat. no. 4311320)
-
MapMarker X-rhodamine-labeled DNA fragments, 50 to 1000 bp (e.g., BioVentures, cat. no. D1-MM1000)
-
POP-7 polymer
-
1.5-ml nonstick, RNase-free microcentrifuge tubes (e.g., Applied Biosystems, cat. no. AM12450)
-
Minicentrifuge (e.g., micro ONE Mini Personal Centrifuge; TOMY, model no. Capsulefuge PMC-880)
-
0.2-ml MicroAmp reaction tube with cap (e.g., Applied Biosystems, cat. no. N8010540) or PCR tubes
-
Vortex mixer (e.g., Genie 2; Scientific Industries, model no. G-560E)
-
Thermal cycler (e.g., GeneAmp PCR System 9700 Fast; Applied Biosystems, cat. no. 4339386)
-
96-well MicroAmp Optical reaction plate with barcode (e.g., Applied Biosystems, cat. no. 4306737)
-
96-well plate septa (e.g., Applied Biosystems, cat. no. 4315933)
-
Microcentrifuge with plate adaptor (e.g., Eppendorf, model no. 5810)
-
Genetic analyzer (e.g., Applied Biosystems)
-
GeneMapper or Peak Scanner software (e.g., Applied Biosystems)
Master mix preparation
1.Follow Basic Protocol 1 steps 1, 2, and 3.
2.Prepare dTP-PCR master mix on ice by carefully dispensing the following reagents into a clean 1.5-ml microcentrifuge tube in the given order:
- 7.50 µl of 5× Q-solution
- 1.50 µl of 10× HotStarTaq PCR buffer
- 1.00 µl HotStarTaq DNA polymerase
- 1.00 µl Taq extender additive
- 0.90 µl HPLC-grade water
- 0.36 µl of 25 µM Fam -labeled FMR1 -specific reverse primer, f
- 0.36 µl of 25 µM tail primer
- 0.36 µl of 25 nM TP primer
- 0.26 µl of 50 mM dGTP
- 0.26 µl of 50 mM dCTP
- 0.26 µl of 10 mM dATP
- 0.26 µl of 10 mM dTTP.
Use aerosol-barrier pipette tips to minimize contamination.
The reagent volumes listed are for a single reaction. To calculate the total volume required for the assay, simply multiply the volumes provided with the total number of reactions.
Include at least one positive control (e.g., sample with a known FMR1 expansion) and a negative or nontemplate PCR control in each dTP-PCR CE assay to ensure reliable amplification and repeat sizing and absence of amplicon or DNA contaminants, respectively.
Ensure the reagents, DNA samples, and master mix are placed on ice throughout the assay setup to prevent reagent degradation and suboptimal results.
3.Mix master mix by pipetting up and down.
4.Spin down master mix in the minicentrifuge.
5.Label 0.2-ml MicroAmp reaction tubes or PCR tubes, and place on ice.
6.Aliquot 14 µl master mix into each labeled PCR tube.
7.Add 1 µl of 100 ng/μl genomic DNA and control genomic DNA into the appropriate PCR tubes. To the nontemplate PCR control tube, add 1 µl HPLC-grade water.
8.Vortex or flick PCR tubes briefly, and then spin down in the minicentrifuge.
dTP-PCR amplification
9.Set the following program on the thermal cycler, and then transfer PCR tubes into the instrument and start the amplification:
1 cycle | 15 min | 95°C | (initial denaturation) |
40 cycles | 45 s | 99°C | (denaturation) |
45 s | 55°C | (annealing) | |
8 min with 15-s autoextension | 70°C | (extension) | |
1 cycle | 10 min | 72°C | (final extension) |
Hold | indefinitely | 4°C. |
10.Remove PCR tubes once the thermal cycler enters the hold step.
11.Briefly spin tubes in a minicentrifuge to ensure the amplicons are collected down and ready for CE.
Genotype analysis
12.Aliquot 4 µl of each dTP-PCR amplicon into individual wells in a barcoded 96-well MicroAmp Optical reaction plate.
13.Add 9 µl Hi-Di formamide and 0.5 µl MapMarker 1000 to the amplicons.
14.Seal plate with clean rubber septa.
15.Spin down plate in a microcentrifuge with plate adaptor 1 min at 100 × g , room temperature.
16.Denature amplicon/Hi-Di mix at 95°C for 5 min.
17.Place reaction plate on ice.
18.Set up genetic analyzer, and ensure instrument has sufficient polymer.
19.Replenish buffer and water reservoirs of the instrument, if necessary, as per the manufacturer's instructions.
20.Ensure injection time and voltage in the genetic analyzer's run module are set to 18 s and 1.2 kV, respectively, and run time and voltage to 50 min and 15 kV, respectively. Leave the rest of the parameters at default values.
21.Once the run is completed, analyze data using GeneMapper or Peak Scanner software following the software manual guidelines.
22.Scan electropherograms to assess the FMR1 genotypes, repeat counts, and number and placement of any AGG interruptions.
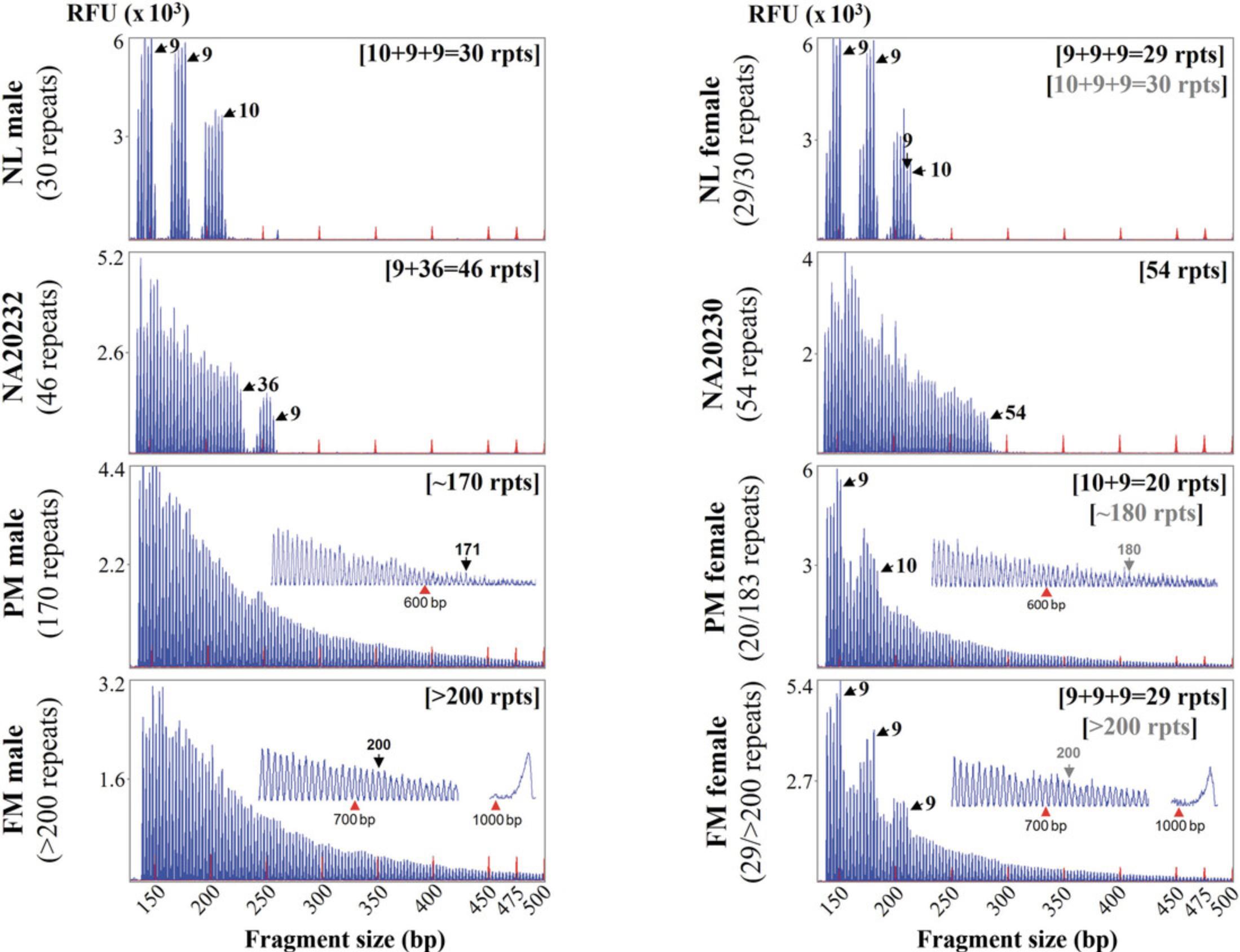
Basic Protocol 5: GENERATION OF CONTROL FMR1 PLASMIDS FOR DIRECT TRIPLET-PRIMED PCR MELTING CURVE ANALYSIS
This protocol outlines the steps to generate plasmids with FMR1 CGG repeat length of choice for use as internal size controls in dTP-PCR MCA. Briefly, the steps outlined include the flanking PCR protocol to amplify across the FMR1 CGG repeat sequence and describe isolation, concentration, purification, and cloning of the amplicon fragments.
Materials
-
HotStarTaq DNA polymerase (e.g., Qiagen, cat. no. 203205) containing:
- Q-solution
- HotStarTaq PCR buffer
- HotStarTaq PCR polymerase
-
Water, HPLC grade (e.g., Sigma-Aldrich, cat. no. 270733)
-
FMR1 -specific forward primer, c : 5′-GCTCAGCTCCGTTTCGGTTTCACTTCCGGT-3′ (Fu et al., 1991)
-
FMR1 -specific reverse primer, f3 : 5′-GCACTTCCACCACCAGCTCCTCCATCTTCT-3′
-
dNTPs, PCR grade (e.g., Roche Applied Science, cat. no. 11969064001)
-
100 ng/µl genomic DNA sample
-
70% and 100% ethanol (e.g., Fisher Chemical, cat. no. E/0650DF/17)
-
3 M sodium acetate (e.g., Sigma-Aldrich, cat. no. S2404)
-
Glycogen, molecular biology grade (e.g., Thermo Scientific, cat. no. R0561)
-
10× TE buffer, pH 8.0, ultrapure (e.g., 1st BASE, cat. no. BUF-3020-10)
-
pCRII-TOPO TA Cloning Kit, dual promoter, without competent cells (e.g., Invitrogen, cat. no. 450640)
-
Maximum efficiency DH5α competent cells (e.g., Invitrogen, cat. no. 18258012)
-
S.O.C. medium (e.g., Invitrogen, cat. no. 15544034)
-
Luria-Bertani (LB) agar plates containing 100 μg/ml ampicillin (e.g., Sigma-Aldrich, cat. no. L5667)
-
LB broth (e.g., Invitrogen, cat. no. 10855001) containing 100 μg/ml ampicillin (e.g., Sigma-Aldrich, cat. no. A5354)
-
Glycerol (e.g., Sigma-Aldrich, cat. no. G5516)
-
Plasma midi prep kit (e.g., Qiagen Plasmid Mini or Axyprep Plasmid MiniPrep Kit)
-
Minicentrifuge (e.g., micro ONE Mini Personal Centrifuge; TOMY, model no. Capsulefuge PMC-880)
-
0.2-ml MicroAmp reaction tube with cap (e.g., Applied Biosystems, cat. no. N8010540) or PCR tubes
-
Vortex mixer (e.g., Genie 2; Scientific Industries, model no. G-560E)
-
Thermal cycler (e.g., GeneAmp PCR System 9700 Fast; Applied Biosystems, cat. no. 4339386)
-
1.5-ml nonstick, RNase-free microcentrifuge tubes (e.g., Applied Biosystems, cat. no. AM12450)
-
Microcentrifuge (e.g., Eppendorf, model no. 5810)
-
Microvolume spectrophotometer (e.g., NanoDrop 1000; Thermo Scientific, model no. ND-1000)
-
42°C water bath (e.g., Memmert, model no. MEM-EW-WB14L4)
-
Laboratory shaker (e.g., New Brunswick Scientific, model no. Innova 4330)
-
Cell spreader, sterile (e.g., VWR International, cat. no. 60828-680)
-
37°C incubator
-
15-ml conical centrifuge tubes (e.g., Fisher Scientific, cat. no. 14-959-53A)
Master mix preparation
1.Follow Basic Protocol 1 steps 1, 2, and 3.
2.Prepare flanking PCR master mix on ice by carefully dispensing the following reagents into a clean 1.5-ml microcentrifuge tube in the given order:
- 25.00 µl of 5× Q-solution
- 12.00 µl HPLC-grade water
- 5.00 µl of 10× HotStarTaq PCR buffer
- 2.50 µl of 10 µM FMR1 -specific forward primer, c
- 2.50 µl of 10 µM FMR1 -specific reverse primer, f3
- 1.00 µl of 25 mM dNTPs
- 1.00 µl HotStarTaq DNA polymerase.
Ensure the reagents, genomic DNA, and master mix are placed on ice throughout the assay setup to prevent reagent degradation and suboptimal results.
The reagent volumes listed are for a single reaction. For each sample, set up five to six reactions.
3.Mix master mix by pipetting up and down.
4.Spin down master mix in the minicentrifuge.
5.Label 0.2-ml MicroAmp reaction tubes or PCR tubes, and place on ice.
6.Aliquot 49 µl master mix into each labeled PCR tube.
7.Add 1 µl of 100 ng/μl genomic DNA into each aliquoted master mix. To the nontemplate PCR control tube, add 1 µl HPLC-grade water.
8.Vortex or flick PCR tubes briefly, and then spin down in the minicentrifuge.
Flanking PCR amplification
9.Set the following program on the thermal cycler, and then transfer PCR tubes into the instrument and start the amplification:
1 cycle | 15 min | 95°C | (initial denaturation) |
40 cycles | 45 s | 98°C | (denaturation) |
45 s | 55°C | (annealing) | |
8 min with 15-s autoextension | 70°C | (extension) | |
1 cycle | 10 min | 72°C | (final extension) |
Hold | indefinitely | 4°C. |
10.Remove PCR tubes once the thermal cycler enters the hold step, and spin down briefly.
11.Pool PCR products of each sample (∼300 µl) into a clean 1.5-ml microcentrifuge tube.
12.Prechill 70% and 100% ethanol at –20°C, and set the microcentrifuge or refrigerated microcentrifuge temperature to 4°C.
Purification and concentration of PCR products
13.To each pooled PCR product, add 1/10 volume of 3 M sodium acetate (e.g., 1/10 × 290 = 29 µl), 3 volumes of prechilled 100% ethanol (3 × 290 = 870 µl), and 1 µl glycogen.
14.Mix contents by gently inverting the tubes.
15.Incubate tubes at –20°C for 20 to 30 min.
16.Centrifuge tubes 20 min at 16,060 × g , 4°C.
17.Pipette supernatant out carefully without disturbing or dislodging the pellet.
18.Add 500 µl prechilled 70% ethanol to the tubes, and centrifuge samples 10 to 15 min at 16,060 × g , 4°C.
19.Pipette supernatant out carefully and discard.
20.Spin down tubes briefly in the minicentrifuge, and aspirate and discard any residual liquid.
21.Leave tubes open and air-dry until no ethanol remains.
22.Once the pellet is dry, add 50 µl HPLC-grade water or 1× TE buffer.
23.Flick tubes to dissolve the pellet and spin down.
24.Quantify concentrated PCR products using a microvolume spectrophotometer.
25.Store concentrated PCR products at –20°C until further use.
Bacterial transformation
26.Prepare ligation reaction by adding 1 µl salt solution and 1 µl pCRII-TOPO TA vector to 4 µl of each concentrated PCR product (from step 24).
27.Add ligation reaction to the thawed DH5α competent cells (50 µl per tube).
28.Gently tap tubes to mix.
29.Incubate cells on ice for 30 min.
30.Immerse two-thirds of the tubes into the 42°C water bath, and heat shock cells for 20 s.
31.Place tubes on ice for 2 min.
32.Add 0.95 ml room temperature S.O.C. medium to each tube.
33.Shake tubes at 225 rpm for 1 hr at 37°C.
34.Centrifuge tubes 5 min at 16,060 × g , room temperature.
35.Remove 800 µl supernatant and discard.
36.Resuspend pellet in remaining supernatant.
Bacterial culture
37.Using a sterile cell spreader, spread cells from each tube on individual, labeled LB agar plates containing 100 μg/ml ampicillin.
38.Incubate plates overnight at 37°C.
39.Randomly pick 10 to 20 colonies from each plate using clean pipette tips.
40.Suspend each colony in an individual PCR tube containing 10 µl of 1× TE buffer, and mark colony numbers on the PCR tubes and LB plates.
41.Set up dTP-PCR CE assay (as outlined in Basic Protocol 4) with 1 µl picked colony from each PCR tube.
42.Examine electropherograms to identify colonies that have the inserts with expected FMR1 CGG repeat size and structure.
43.Create a starter culture by inoculating the remaining 9 µl colonies of interest into individual 15-ml tubes with 5 ml LB broth containing 100 μg/ml ampicillin.
44.Shake tubes at 250 rpm overnight at 37°C.
45.Expand culture by performing an overnight incubation at 37°C with 250 rpm in 50 to 100 ml LB broth containing 100 μg/ml ampicillin.
46.Prepare glycerol stocks for future use by adding 400 µl culture to 400 µl glycerol and pipetting up and down gently to mix. Store glycerol stocks at –80°C.
Isolation and quantification of plasmid DNAs
47.Use the Qiagen Plasmid Mini or Axyprep Plasmid MiniPrep Kit to isolate plasmid DNA samples as per the manufacturer's instructions.
48.Quantify extracted plasmid DNAs using a microvolume spectrophotometer, and store samples at –20°C.
49.Use 1 pg extracted plasmid DNA as the template for dTP-PCR MCA (see Basic Protocol 1) or dTP-PCR CE (see Basic Protocol 4) assays.
Basic Protocol 6: SANGER SEQUENCING ASSAY TO VERIFY FMR1 CGG REPEAT SIZE AND STRUCTURE OF PLASMID DNA CONTROLS
This protocol outlines the Sanger sequencing assay for verification of the FMR1 CGG repeat genotypes of the cloned and extracted plasmid DNAs.
Materials
-
Q-solution, from HotStarTaq DNA polymerase kit (e.g., Qiagen, cat. no. 203207)
-
2.5× BigDye Terminator v3.1 Cycle Sequencing Kit (e.g., Applied Biosystems, cat. no. 4337455)
-
Water, HPLC grade (e.g., Sigma-Aldrich, cat. no. 270733)
-
FMR1 -specific forward primer, c : 5′-GCTCAGCTCCGTTTCGGTTTCACTTCCGGT-3′
-
100 ng/µl plasmid DNA template (see Basic Protocol 5)
-
70% and 100% ethanol (e.g., Fisher Chemical, cat. no. E/0650DF/17)
-
3 M sodium acetate, pH 5.2 (e.g., Thermo Scientific, cat. no. R1181)
-
Hi-Di formamide (e.g., Applied Biosystems, cat. no. 4311320)
-
POP-7 polymer
-
1.5-ml nonstick, RNase-free microcentrifuge tubes (e.g., Applied Biosystems, cat. no. AM12450)
-
0.2-ml MicroAmp reaction tube with cap (e.g., Applied Biosystems, cat. no. N8010540) or PCR tubes
-
Thermal cycler (e.g., GeneAmp PCR System 9700 Fast; Applied Biosystems, cat. no. 4339386)
-
Vortex mixer (e.g., Genie 2; Scientific Industries, model no. G-560E)
-
Microcentrifuge with plate adaptor (e.g., Heraeus, model no. 75005521)
-
96-well MicroAmp Optical reaction plate with barcode (e.g., Applied Biosystems, cat. no. 4306737)
-
96-well plate septa (e.g., Applied Biosystems, cat. no. 4315933)
-
Genetic analyzer (e.g., Applied Biosystems)
-
GeneMapper (e.g., Applied Biosystems) or Chromas (e.g., Technelysium Pty) software
Cycle sequencing reaction
1.Follow Basic Protocol 1 steps 1, 2 , and 3.
2.Prepare cycle sequencing reaction master mix on ice by dispensing the following reagents into a clean 1.5-ml microcentrifuge tube in the given order:
- 9.20 µl of 5× Q-solution
- 8 µl of 2.5× BigDye Terminator Mix v3.1
- 0.48 µl HPLC-grade water
- 0.32 µl of 10 µM FMR1 -specific forward primer, c.
The reagent volumes listed are for a single reaction. To calculate the volume required for the assay, simply multiply the volumes provided with the total number of reactions.
3.Dispense 18 µl cycle sequencing reaction master mix into each labeled PCR tube.
4.Add 2 µl of 100 ng/μl plasmid DNA template to the appropriate PCR tubes.
5.Perform cycle sequencing reaction on a thermal cycler under the following condition:
1 cycle | 1 min | 96°C | (initial denaturation) |
25 cycles | 10 s | 98°C | (denaturation) |
5 s | 55°C | (annealing) | |
4 min | 60°C | (extension) | |
Hold | indefinitely | 4°C. |
6.Once the cycle sequencing reaction is completed, store products at 4°C.
Precipitation of cycle sequencing products
7.Prepare a precipitation mix by dispensing the following reagents into a clean 1.5-ml microcentrifuge tube:
- 62.5 µl of 100% ethanol
- 14.5 µl HPLC-grade water
- 3 µl of 3 M sodium acetate.
The reagent volumes listed are for a single reaction. To calculate the volume required for the assay, simply multiply the volumes provided with the total number of reactions.
8.To clean, labeled 1.5-ml microcentrifuge tubes, add 80 µl precipitation mix, and transfer cycle sequencing products into the corresponding tubes.
9.Briefly vortex or flick tubes, and then spin down in the minicentrifuge.
10.Leave tubes at room temperature away from light for 15 min.
11.Centrifuge tubes 20 min at 16,060 × g , 4°C.
12.Aspirate and discard supernatant.
13.Add 250 µl of 70% ethanol into each tube.
14.Centrifuge tubes 5 min at 16,060 × g , 4°C.
15.Aspirate and discard supernatant.
16.Repeat steps 14, 15, and 16 once more.
17.Air-dry tubes.
18.Add 9 µl Hi-Di formamide to each tube.
19.Vortex tubes to dislodge and suspend pellets.
Sanger sequencing
20.Load products into a clean 96-well MicroAmp Optical reaction plate.
21.Seal plate with a clean rubber septa and spin down.
22.Denature plate at 95°C for 5 min.
23.Place reaction plate on ice, and set up genetic analyzer, ensuring the instrument has sufficient polymer.
24.Replenish buffer and water reservoirs of the instrument, if necessary, as per the manufacturer's instructions.
25.Initiate the CE run.
26.Once the run is completed, visualize and analyze chromatograms using GeneMapper or Chromas software.
![Details are in the caption following the image FMR1 plasmid chromatograms with normal and premutation alleles. (A) Plasmid with a (CGG)<sub>10</sub>AGG(CGG)<sub>32</sub> repeat structure generated from the intermediate allele (45 repeats [rpts]; (CGG)<sub>10</sub>AGG(CGG)<sub>34</sub>) of the Coriell sample NA20235. (B) Plasmid with a (CGG)<sub>9</sub>AGG(CGG)<sub>9</sub>AGG(CGG)<sub>36</sub> repeat structure generated from the premutation allele (56 rpts; (CGG)<sub>9</sub>AGG(CGG)<sub>9</sub>AGG(CGG)<sub>36</sub>) of the Coriell sample CD00014. (C) Plasmid with a (CGG)<sub>9</sub>AGG(CGG)<sub>18</sub>AGG(CGG)<sub>18</sub>AGG(CGG)<sub>10</sub> repeat structure generated from the premutation allele (58 rpts; (CGG)<sub>9</sub>AGG(CGG)<sub>18</sub>AGG(CGG)<sub>18</sub>AGG(CGG)<sub>10</sub>) of an in-house sample.](https://static.yanyin.tech/literature_test/cpz1427-fig-0003-m.jpg)
COMMENTARY
Background Information
The TP-PCR method was originally developed to detect (CAG)n expansions that cause myotonic dystrophy type 1 (MIM no. 160900; Warner et al., 1996) and has been optimized for the FMR1 locus. The dTP-PCR protocols described in this article signify a dual approach that includes an MCA-based rapid, scalable, and cost-effective first-tier screening test to detect samples with expanded alleles and a CE-based FMR1 genotyping assay that can be implemented on the screen-positive samples for confirmatory repeat size and AGG interruption structure evaluation. The dTP-PCR MCA assay will identify both premutation and full-mutation individuals, whereas the CE assay can be used to determine the precise FMR1 genotypes and to characterize the number and placement of AGG interruptions at the 3′ ends of the expanded and nonexpanded alleles. The MCA protocol is highly sensitive in detecting low-level mosaicisms of full-mutation expansions (Rajan-Babu, Law, et al., 2015) and has been shown to yield reliable results on DNA inputs ranging from as little as 1 ng to 1 µg and DNA extracted from a wide variety of sources including cell lines, peripheral blood, buccal swabs, saliva, and dried blood spots (Rajan-Babu et al., 2016). These methods are ideal for large-scale studies such as screening newborns, women of reproductive age, and at-risk populations (e.g., individuals with intellectual disability and autism).
Critical Parameters
It is critical to adhere to the reagent concentrations and volumes listed for the dTP-PCR MCA and CE assays, as minute changes in the volumes and concentrations of the reagents can affect the results. Irregular or uneven electropherogram peaks could result from altered reagent volumes and concentrations, and so it is important to use well-calibrated pipettes and avoid pipetting errors. Repeated freezing and thawing of the reagents can yield peaks with reduced relative fluorescence units or –d F /d T. Repeat the experiments with freshly prepared reagents if reagent degradation is suspected. The recommended genomic DNA concentration for the dTP-PCR MCA assays is 100 ng, but lower (1 ng) or higher (1 µg) concentrations can be used to successfully detect FMR1 expansions. However, reducing the DNA concentration can produce weaker fluorescence signal intensities. For the dTP-PCR CE assay, we do not recommend using <100 ng of genomic DNA per sample, as this may affect accurate sizing of large premutation and reliable detection of full-mutation expansions. It is also imperative to use DNA that is free of impurities, as residual DNA precipitation reagents (such as sodium acetate and glycogen) and other contaminants produce suboptimal results. For MCA, block-based real-time PCR systems can have edge effects that could have an impact on the Tms recorded. The systems with centrifugal rotary design offer greater temperature uniformity and help minimize variations arising from the edge effects of block-based systems. For each MCA run, use at least one internal size control, such as a large normal sample with 44 CGG repeats to generate the temperature threshold and identify all samples with >44 CGG repeats or a large intermediate sample with 54 CCG repeats to generate the temperature threshold and identify all samples with >54 CGG repeats.
Troubleshooting
One of the potential outcomes of the dTP-PCR protocols is false positives. Even the presence of minute amounts of amplicon contaminants from expanded samples can result in false-positive MCA outcomes owing to the assay's high sensitivity. If the nontemplate PCR controls display unusual peaks with very low fluorescence signal and a return to baseline that exceeds the threshold temperatures of the internal size controls, repeat the assay with freshly prepared reagents and cleaned pipettes.
If the DNA samples used have contaminants, the CE amplicon peaks may appear unusual and make it harder to discern the AGG interruption patterns, especially in female samples. If impurities are suspected in the DNA samples, purify the samples following the standard phenol-chloroform protocol and repeat the assay.
The TP primer is 1000× diluted compared with the FMR1 locus-specific and tail primers. Using this recommended concentration ratio is essential to ensure detection and sizing of large FMR1 expansions. If steeper drops in the fluorescence intensities of the dTP-PCR CE amplicon peaks are observed, redilute the TP primer and repeat the assay. For MCA, degradation of the SYBR Green I Nucleic Acid Gel Stain over time can result in melt peaks with a low –d F /d T. Redilute SYBR Green I and repeat the assay if the SYBR Green I working stock solution is more than 1 or 2 weeks old. Always include a positive control in each dTP-PCR MCA and CE protocol. This will help verify whether the assay is yielding expected results and point out errors or mistakes in the assay setup.
Understanding Results
Basic Protocols 1, 2, and 3 for dTP-PCR amplification, MCA, and generation of melt peak profiles will produce results as in the example data presented in Figure 1. The melt peak profiles of eight samples with distinct FMR1 genotypes (normal male, 30 repeats; premutation male, 118 repeats; full-mutation male, >200 repeats; normal female, 29/30 repeats; premutation female, 20/183 repeats; full-mutation female, 29/>200 repeats), two intermediate-sized internal repeat size controls (NA20232, 46 repeats; NA20230, 54 repeats) from the Coriell Institute (https://www.coriell.org/), and a nontemplate PCR control are displayed. The internal repeat size controls will provide the temperature thresholds to help demarcate expanded and nonexpanded samples. Essentially, samples that have a Tm exceeding that of NA20230 are considered to have a premutation or full-mutation expansion. As shown in Figure 1, premutation and full-mutation males and females displayed right-shifted melt peaks with Tms higher than that of NA20230, whereas the normal nonexpanded samples had a lower Tm relative to that of NA20232. It is important to note that AGG interruptions present within the CGG repeat sequence can influence the Tm. For instance, NA20230 has 54 uninterrupted CGG repeats, which may display a higher Tm compared with premutation samples that have 55 or 56 interrupted CGG repeats. If the goal of the screening effort is to characterize samples with intermediate alleles in addition to those with premutation and full-mutation alleles, we recommend verifying the repeat sizes of samples whose Tm falls between the Tms of NA20232 and NA20230, as well those with Tms that exceed NA20230 using the dTP-PCR CE genotyping assay (see Basic Protocol 4). The dTP-PCR MCA protocol does not differentiate between premutation and full-mutation expansions, and so a second-tier sizing assay such as the dTP-PCR CE is crucial to tell them apart.
The dTP-PCR CE (see Basic Protocol 4) of normal, premutation, and full-mutation samples and intermediate controls subjected to dTP-PCR MCA are presented in Figure 2. As shown, the expanded and nonexpanded samples generate distinct TP-PCR electropherograms. Contrasting flanking PCR assays that amplify across CGG repeats and produce amplicon fragments that are proportional to the number of distinctly sized FMR1 alleles in the samples, the dTP-PCR CE assay with a TP primer that has a 3′ end composed of (CGG)5 will anneal to every stretch of five uninterrupted CGG repeats and generate a highly heterogeneous mixture of amplicon fragments that differ from each other by a CGG repeat. The first peak originates from the five CGG repeats at the 3′ end of the FMR1 alleles, and so it is counted to have five CGG repeats, with the successive peaks counted serially. When the FMR1 CGG repeats are interrupted, the TP primer will fail to anneal across the stretch of five repeats that has the interruption, and as a result the electropherograms of male samples with a single interrupted FMR1 allele will have no-amplicon five-peak gaps interspersing the amplicon peaks. The number of five-peak gaps will correspond with the number of AGG interruptions. In heterozygous female samples with a normal and expanded allele, the overlapping amplicon peaks from the interrupted normal and 3′ uninterrupted expanded alleles can be visualized, with the spikes in amplicon peaks representing the AGG interruption pattern of the normal allele. In Figure 2, the normal samples have one or two FMR1 alleles with 29 or 30 CGG repeats and two AGG interruptions after every 9 or 10 CGG repeats. The control samples have an intermediate allele each, with a single AGG interruption detected in NA20232 (CGG9AGGCGG36) and none in NA20230. The premutation and full-mutation samples have no AGGs at the 3′ end of the expanded FMR1 alleles, and the amplicon peaks can be seen extending beyond the 55- and 200-repeat thresholds, respectively. For full-mutation samples, in addition to the TP-PCR amplicon stutters of >200 repeats, a full-length peak around 1050 bp can also be observed.
Time Considerations
The time required to complete the dTP-PCR and MCA protocol is ∼10 hr and to complete the dTP-PCR and CE protocol is ∼16 hr. The flanking PCR takes 16 hr; agarose gel electrophoresis, ∼30 to 40 min; amplicon concentration and purification, ∼1.4 hr; cloning and generation of plasmids with FMR1 CGG repeat inserts, ∼72 hr; plasmid DNA extraction, ∼1.3 hr; and Sanger sequencing analysis, ∼3 hr.
Acknowledgments
This study was supported by National University Health System (NUHS) grant NUHSRO/2015/056/STB/BBP to SSC. ISRB was a recipient of a research scholarship from the National University of Singapore.
Author Contributions
Indhu-Shree Rajan-Babu : development and assay validation, experimentation, data analysis, writing—original draft; Mulias Lian : writing—reviewing and editing; Samuel S. Chong : development and assay validation, funding acquisition, writing—reviewing and editing.
Conflict of Interest
The authors declare no conflict of interest.
Open Research
Data Availability Statement
The data, tools, and material (or their source) that support the protocol are available from the corresponding author upon reasonable request.
Literature Cited
- Bailey, D. B., Berry-Kravis, E., Gane, L. W., Guarda, S., Hagerman, R., Powell, C. M., … Wheeler, A. (2017). Fragile X newborn screening: Lessons learned from a multisite screening study. Pediatrics , 139, S216–S225. doi: 10.1542/peds.2016-1159H.
- Bailey, D. B., Raspa, M., Bishop, E., & Holiday, D. (2009). No change in the age of diagnosis for fragile X syndrome: Findings from a national parent survey. Pediatrics , 124, 527–533. doi: 10.1542/peds.2008-2992.
- Chen, L., Hadd, A., Sah, S., Filipovic-Sadic, S., Krosting, J., Sekinger, E., … Latham, G. J. (2010). An information-rich CGG repeat primed PCR that detects the full range of fragile X expanded alleles and minimizes the need for Southern blot analysis. The Journal of Molecular Diagnostics , 12, 589–600. doi: 10.2353/jmoldx.2010.090227.
- Chen, L., Hadd, A., Sah, S., Houghton, J. F., Filipovic-Sadic, S., Zhang, W., … Latham, G. J. (2011). High-resolution methylation polymerase chain reaction for fragile X analysis: Evidence for novel FMR1 methylation patterns undetected in Southern blot analyses. Genetics in Medicine , 13, 528–538. doi: 10.1097/GIM.0b013e31820a780f.
- Fu, Y. H., Kuhl, D. P., Pizzuti, A., Pieretti, M., Sutcliffe, J. S., Richards, S., … Warren, S. T. (1991). Variation of the CGG repeat at the fragile X site results in genetic instability: Resolution of the Sherman paradox. Cell , 67, 1047–1058. doi: 10.1016/0092-8674(91)90283-5.
- Hagerman, R. J., Berry-Kravis, E., Hazlett, H. C., Bailey, D. B., Moine, H., Kooy, R. F., … Hagerman, P. J. (2017). Fragile X syndrome. Nature Reviews. Disease Primers , 3, 17065. doi: 10.1038/nrdp.2017.65.
- Hantash, F. M., Goos, D. G., Tsao, D., Quan, F., Buller-Burckle, A., Peng, M., … Strom, C. M. (2010). Qualitative assessment of FMR1 (CGG)n triplet repeat status in normal, intermediate, premutation, full mutation, and mosaic carriers in both sexes: Implications for fragile X syndrome carrier and newborn screening. Genetics in Medicine , 12, 162–173. doi: 10.1097/GIM.0b013e3181d0d40e.
- Hunter, J. E., Berry-Kravis, E., Hipp, H., & Todd, P. K. (1993). FMR1 disorders. In M. P. Adam, H. H. Ardinger, R. A. Pagon, S. E. Wallace, L. J. Bean, G. Mirzaa, & A. Amemiya (Eds.), GeneReviews. Seattle: University of Washington. Retrieved from http://www.ncbi.nlm.nih.gov/books/NBK1384/.
- Hunter, J., Rivero-Arias, O., Angelov, A., Kim, E., Fotheringham, I., & Leal, J. (2014). Epidemiology of fragile X syndrome: A systematic review and meta-analysis. American Journal of Medical Genetics. Part A , 164A, 1648–1658. doi: 10.1002/ajmg.a.36511.
- Latham, G. J., Coppinger, J., Hadd, A. G., & Nolin, S. L. (2014). The role of AGG interruptions in fragile X repeat expansions: A twenty-year perspective. Frontiers in Genetics , 5, 244. doi: 10.3389/fgene.2014.00244.
- Lee, S., Taylor, J. L., Redmond, C., Hadd, A. G., Kemppainen, J. A., Haynes, B. C., … Latham, G. J. (2020). Validation of fragile X screening in the newborn population using a fit-for-purpose FMR1 PCR assay system. The Journal of Molecular Diagnostics , 22, 346–354. doi: 10.1016/j.jmoldx.2019.11.002.
- Nolin, S. L., Brown, W. T., Glicksman, A., Houck, G. E., Gargano, A. D., Sullivan, A., … Sherman, S. L. (2003). Expansion of the fragile X CGG repeat in females with premutation or intermediate alleles. American Journal of Human Genetics , 72, 454–464. doi: 10.1086/367713.
- Nolin, S. L., Glicksman, A., Tortora, N., Allen, E., Macpherson, J., Mila, M., … Hadd, A. G. (2019). Expansions and contractions of the FMR1 CGG repeat in 5,508 transmissions of normal, intermediate, and premutation alleles. American Journal of Medical Genetics. Part A , 179, 1148–1156. doi: 10.1002/ajmg.a.61165.
- Nolin, S. L., Sah, S., Glicksman, A., Sherman, S. L., Allen, E., Berry-Kravis, E., … Hadd, A. G. (2013). Fragile X AGG analysis provides new risk predictions for 45–69 repeat alleles. American Journal of Medical Genetics. Part A , 161A, 771–778. doi: 10.1002/ajmg.a.35833.
- Okoniewski, K. C., Wheeler, A. C., Lee, S., Boyea, B., Raspa, M., Taylor, J. L., & Bailey, D. B. (2019). Early identification of fragile X syndrome through expanded newborn screening. Brain Sciences , 9, 4. doi: 10.3390/brainsci9010004.
- Rajan-Babu, I. S., Law, H. Y., Yoon, C. S., Lee, C. G., & Chong, S. S. (2015). Simplified strategy for rapid first-line screening of fragile X syndrome: Closed-tube triplet-primed PCR and amplicon melt peak analysis. Expert Reviews in Molecular Medicine , 17, e7. doi: 10.1017/erm.2015.5.
- Rajan-Babu, I. S., Lian, M., Tran, A. H., Dang, T. T., Le, H. T., Thanh, M. N., … Chong, S. S. (2016). Defining the performance parameters of a rapid screening tool for FMR1 CGG-repeat expansions based on direct triplet-primed PCR and melt curve analysis. The Journal of Molecular Diagnostics , 18, 719–730. doi: 10.1016/j.jmoldx.2016.05.002.
- Rajan-Babu, I. S., Teo, C. R., Lian, M., Lee, C. G., Law, H. Y., & Chong, S. S. (2015). Single-tube methylation-specific duplex-PCR assay for rapid and accurate diagnosis of fragile X mental retardation 1-related disorders. Expert Review of Molecular Diagnostics , 15, 431–441. doi: 10.1586/14737159.2015.1001749.
- Rajan-Babu, I.-S., & Chong, S. S. (2019). Triplet-repeat primed PCR and capillary electrophoresis for characterizing the fragile X mental retardation 1 CGG repeat hyperexpansions. Methods in Molecular Biology , 1972, 199–210. doi: 10.1007/978-1-4939-9213-3_14.
- Riley, C., & Wheeler, A. (2017). Assessing the fragile X syndrome newborn screening landscape. Pediatrics , 139, S207–S215. doi: 10.1542/peds.2016-1159G.
- Spector, E., Behlmann, A., Kronquist, K., Rose, N. C., Lyon, E., & Reddi, H. V. (2021). Laboratory testing for fragile X, 2021 revision: A technical standard of the American College of Medical Genetics and Genomics (ACMG). Genetics in Medicine , 23, 799–812. doi: 10.1038/s41436-021-01115-y.
- Tassone, F., Iong, K. P., Tong, T.-H., Lo, J., Gane, L. W., Berry-Kravis, E., … Hagerman, R. J. (2012). FMR1 CGG allele size and prevalence ascertained through newborn screening in the United States. Genome Medicine , 4, 100. doi: 10.1186/gm401.
- Verkerk, A. J., Pieretti, M., Sutcliffe, J. S., Fu, Y. H., Kuhl, D. P., Pizzuti, A., … Zhang, F. P. (1991). Identification of a gene (FMR-1) containing a CGG repeat coincident with a breakpoint cluster region exhibiting length variation in fragile X syndrome. Cell , 65, 905–914. doi: 10.1016/0092-8674(91)90397-h.
- Warner, J. P., Barron, L. H., Goudie, D., Kelly, K., Dow, D., Fitzpatrick, D. R., & Brock, D. J. (1996). A general method for the detection of large CAG repeat expansions by fluorescent PCR. Journal of Medical Genetics , 33, 1022–1026. doi: 10.1136/jmg.33.12.1022.