Optimized Workflow for Proteomics and Phosphoproteomics With Limited Tissue Samples
Yan Wang, Yan Wang, Minghan Hu, Minghan Hu
Abstract
Proteomics and phosphoproteomics play crucial roles in elucidating the dynamics of post-transcriptional processes. While experimental methods and workflows have been established in this field, a persistent challenge arises when dealing with small samples containing a limited amount of protein. This limitation can significantly impact the recovery of peptides and phosphopeptides. In response to this challenge, we have developed a comprehensive experimental workflow tailored specifically for small-scale samples, with a special emphasis on neuronal tissues like the trigeminal ganglion. Our proposed workflow consists of seven steps aimed at optimizing the preparation of limited tissue samples for both proteomic and phosphoproteomic analyses. One noteworthy innovation in our approach involves the utilization of a dual enrichment strategy for phosphopeptides. Initially, we employ Fe-NTA Magnetic beads, renowned for their specificity and effectiveness in capturing phosphopeptides. Subsequently, we complement this approach with the TiO2-based method, which offers a broader spectrum of phosphopeptide recovery. This innovative workflow not only overcomes the challenges posed by limited sample sizes but also establishes a new benchmark for precision and efficiency in proteomic investigations. Published 2024. This article is a U.S. Government work and is in the public domain in the USA. Current Protocols published by Wiley Periodicals LLC.
Basic Protocol 1 : Protein extraction and digestion
Basic Protocol 2 : TMT labeling and peptide cleanup
Basic Protocol 3 : IMAC Fe-NTA magnetic beads phosphopeptide enrichment
Basic Protocol 4 : TiO2 enrichment
Basic Protocol 5 : Fe-NTA phosphopeptide Enrichment
Basic Protocol 6 : High pH peptide fractionation
Basic protocol 7 : LC-MS/MS analysis and database search
INTRODUCTION
The study of the proteome involves a comprehensive assessment of protein function and structure to gain a nuanced understanding of protein expression in specific tissues or cells (Breitkopf & Asara, 2012, Al-Amrani et al., 2021). Phosphorylation of proteins, a critical mechanism regulating cell signaling processes, such as gene expression and membrane transport, plays a pivotal role in both physiological and pathological events. However, the detection and identification of phosphorylated peptides on a proteome-wide scale present significant challenges due to the low abundance of phosphorylated proteins and potential losses during sample preparation (Dunn et al., 2010).
The trigeminal ganglion, a critical component of primary sensory ganglia, is responsible for transmitting pain and itch sensations. Its intricate structure comprises trigeminal ganglion cell bodies surrounded by a single layer of satellite glial cells, while Schwann cells wrap both distal and central afferent neuronal processes. The ganglion also accommodates fibroblasts, collagen fibers, small blood vessels, and various immune cells (Messlinger & Russo, 2019). Additionally, each mouse trigeminal ganglion is ∼0.1 g in size. As a result, studying the proteome and phosphoproteome of tiny neuronal tissues, such as the trigeminal ganglion, presents challenges due to the limitations imposed by tissue size and cell type composition.
To address these challenges, our customized workflow employs a high concentration of 5% SDS as a lysis buffer for protein extraction from the trigeminal ganglion. Additionally, a three-step process incorporating Fe-NTA magnetic beads enrichment and TiO2 enrichment, is implemented to enhance the yield of phosphopeptides. This protocol provides a comprehensive workflow outline facilitating high-quality phosphoproteomic analysis with limited neuronal tissue samples. Detailed procedures cover sample lysis, protein digestion, clearance, enrichment, and liquid chromatography-tandem mass spectrometry (LC-MS/MS) analysis.
NOTE : All protocols involving animals must be reviewed and approved by the appropriate Animal Care and Use Committee and must follow regulations for the care and use of laboratory animals.
Basic Protocol 1: PROTEIN EXTRACTION AND DIGESTION
Protein extraction is a pivotal initial step in proteomics, particularly when dealing with limited tissue samples or total protein. Achieving efficient protein extraction from neuronal tissues, such as the trigeminal ganglion, requires careful selection of the lysis buffer to optimize results. This part of the protocol focuses on the use of the 5% SDS lysis buffer for conducting protein extraction (Hu, Doyle et al., 2022, Wang, Veth et al., 2023).
Protein digestion is a critical tool for identifying, characterizing, and measuring proteins in proteomic research (Switzar, Giera et al., 2013). Predominantly, proteomics experiments rely on digestion of the protein into peptides prior to mass spectrometric (MS) analysis. Basic Protocol 1 describes the use of dithiothreitol (DTT) and subsequent iodoacetamide (IAA) for the reduction and alkylation of proteins, respectively, which leads to their denaturation. This refined protocol has been adapted from the original S-trap digestion method.
Materials
-
Mice
-
5% SDS lysis buffer (Fisher Scientific, cat. no. 54600046)
-
Pierce BCA protein assay kits (Thermo Fisher Scientific, cat. no. 23225)
-
S-TrapTM micro columns (≤ 100 µg) (Protifi, cat. no. C02-MICRO-10)
-
Trimethylammonium bicarbonate (TEAB) 50mM (see Reagents and Solutions)
-
0.5M Dithiothreitol (DTT) (see Reagents and Solutions)
-
0.55M Iodoacetamide (IAA) (see Reagents and Solutions)
-
Trypsin Gold, Mass Spectrometry Grade (Promega, cat. no. V5280)
-
12% Phosphoric acid solution (see Reagents and Solutions)
-
0.2% Formic acid in water (see Reagents and Solutions)
-
Dry ice bath or liquid nitrogen
-
−80°C freezer
-
KIMBLE KONTES DUALL tissue grinder, 1 ml (DWK life sciences, cat. no. 885460-0020)
-
Ice
-
1.5-ml protein LoBind tubes (Eppendorf, cat. no. 022431081)
-
Boiling water bath
-
Centrifuge (Eppendorf, cat. no. 5425 R) or equivalent refrigerated centrifuge
-
Incubator (Benchmark Scientific, cat. no. RS7166) or equivalent equipment
-
SpeedVac (CentriVap-84 Cold Trap, LabConco, cat. no. 7460020
-
Refrigerated CentriVap Benchtop Vacuum Concentrator with glass lid, LabConco, cat. no. 7310021) or equivalent equipment
-
Vortex Machine (Scientific Industries, inc., cat. no. SKU:SI-0236)
1.Dissect trigeminal ganglion tissue from mice and promptly freeze it using a dry ice bath (Katzenell, Cabrera et al., 2017). Store the tissue in −80°C freezer.
2.Pre-cool a grinder and pestle on ice.
3.Take the tissue out from the freezer. Transfer the tissue into a 1-ml homogenizer. Add 100 µl of 5% SDS lysis buffer and thoroughly homogenize the sample at room temperature.
4.Transfer the homogenized tissue and buffer into a 1.5-ml microcentrifuge tube with low protein binding properties, then boil the mixture for 2 min.
5.Centrifuge samples 10 min at 14,000 × g , room temperature, and collect the supernatants. Keep the samples.
6.Determine the protein concentration using a BCA protein assay kit. After testing the concentration, retain the sample for digestion as outlined in Basic Protocol 2.
7.Take 100 µg protein aliquot from the total protein extract supernatant from Basic Protocol 1 for each sample.
8.Add DTT to a final concentration of 2 mM. Incubate at 56°C for 30 min.
9.Add iodoacetamide (IAA) to a final concentration of 5 mM and incubate at room temperature for 45 min. Keep the reaction in the dark.
10.Add 12% aqueous phosphoric acid to the sample at a 1:10 (v/v) ratio, achieving a final concentration of 1.2% phosphoric acid. After adding, vortex the sample for thorough mixing.
11.Add 165 µl binding/wash buffer to every 27.5 µl acidified protein solution. Mix well by vortexing.
12.Transfer to the top of an S-Trap column; if total volume is >200 µl at this point, repeat this step and step 7 with ∼200 µl each time.
13.Centrifuge the micro column 1 to 2 min at 4000 × g , 4°C, until all buffer has passed through the S-Trap column.
14.Wash captured protein by adding 150 µl binding/wash buffer and repeating centrifugation three times.
15.Move S-Trap micro column to a clean 2-ml sample tube for protease digestion.
16.Add 20 µl digestion buffer (50 mM TEAB) containing protease at 1:10 to 1:25 (w/w) (compare with current sample volume) to the top of the micro column.
17.Cap the S-Trap column loosely to limit evaporation loss.
18.Incubate for 1 hr at 47°C for trypsin.
19.Elute peptides with 40 µl of 50 mM TEAB, and then centrifuge 1 min at 4000 × g , 4°C.
20.Add 40 µl of 0.2% aqueous formic acid to the column and then centrifuge 1 min at 4000 × g , 4°C.
21.Add 40 µl of 50% acetonitrile containing 0.2% formic acid and then centrifuge 1 min at 4000 × g , 4°C.
22.Pool eluted peptides and dry down in the SpeedVac. Eluted peptides are then resuspended for TMT labeling (Basic Protocol 3).
Basic Protocol 2: TMT LABELING AND PEPTIDE CLEANUP
After protein digestion, when dealing with multiple samples, tandem mass tags (TMT) can be employed for labeling. TMT reagents utilize the principle of isotopes. Different isotopic labels are conjugated to specific amino acid residues in peptides, allowing for the labeling of peptides from different sources. The TMT labeling reagents enable simultaneous identification and quantification of proteins in diverse samples using tandem mass spectrometry. The protocol is adapted from TMTpro label reagent set user guide.
Peptide cleanup is essential for the removal of secondary metabolite contamination produced during sample preparation (Waas, Pereckas et al., 2019). These compounds can negatively impact the efficiency of subsequent phosphopeptide enrichment processes. To ensure an optimal protein yield and to prepare high-quality protein samples for mass spectrometric analysis, Basic Protocol 2 second section outlines the procedure for peptide clean-up using the Thermo Fisher Scientific prep kit.
Materials
-
TMTpro label reagent set (Thermo Fisher, cat. no. A52045)
-
50 mM TEAB (see recipe)
-
5% hydroxylamine (see recipe)
-
20% formic acid solution (see recipe)
-
EasyPep™ Mini MS Sample Prep Kit (Thermo Fisher Scientific, cat. no. A4006)
-
Vortex
-
pH paper
-
SpeedVac (CentriVap-84 Cold Trap, LabConco, cat. no. 7460020; Refrigerated CentriVap Benchtop Vacuum Concentrator with glass lid, LabConco, cat. no. 7310021) or equivalent equipment
1.Just before using the kit, let the TMTpro label reagents reach room temperature inside the foil pouch.
2.Add 100 µl of 50 mM TEAB to resuspend peptides for each sample.
3.Add 40 µl TMT reagent, which is dissolved in 100% acetonitrile, to each buffered peptide sample. Allow this mixture to incubate at room temperature for 30 to 60 min.
4.To stop and acidify the labeling reaction, add 50 µl each of 5% hydroxylamine and 20% formic acid solution to each labeling reaction.
5.Take 1 µl drop from each tube to verify pH < 4 using pH paper.
6.After labeling, combine the samples into one tube and then proceed with the cleanup procedure.
Peptide Cleanup
7.Take off the white cap located at the bottom of the peptide cleanup column. Gently loosen the green top cap and put it into a 2-ml microcentrifuge tube.
8.Centrifuge the column 2 min at 3000 × g , 4°C, to remove any remaining liquid from the column, and then dispose of the liquid that flows through.
9.Transfer the protein digest sample, which has a total volume of ∼300 µl, into the dry peptide cleanup column.
10.Centrifuge the column 2 min at 1500 × g , 4°C, and dispose the flowthrough.
11.Add 300 µl wash solution A to the column.
12.Centrifuge once more 2 min at 1500 × g , 4°C, and discard the flowthrough.
13.Add 300 µl wash solution B to the column.
14.Centrifuge 2 min at 1500 × g , 4°C, and discard the flowthrough.
15.Repeat steps 7 and 8 one more time.
16.Transfer the peptide cleanup column to a fresh 2-ml microcentrifuge tube.
17.Add 300 µl elution solution to the column.
18.Centrifuge 2 min at 1500 × g , 4°C, to collect the purified peptide sample.
19.Use a vacuum centrifuge to dry the peptide sample. After drying, take 10% of the protein sample for fractionation (Basic Protocol 8) and analysis of background proteome. The remaining sample is used to enrich phosphopeptides using Fe-NTA magnetic beads.
Basic Protocol 3: IMAC Fe-NTA MAGNETIC BEADS PHOSPHOPEPTIDE ENRICHMENT
Mass spectrometry is pivotal for identifying protein phosphorylation sites and quantifying changes in phosphorylation levels. Nonetheless, analyzing protein phosphorylation via MS presents challenges, such as low stoichiometry, high hydrophilicity, suboptimal ionization, and incomplete fragmentation of phosphopeptides. Due to the comparatively low occurrence of phosphorylation modifications in complex protein mixtures, it becomes essential to enrich phosphopeptides to enhance the effectiveness of MS analysis.
PTMScan phospho-enrichment IMAC Fe-NTA magnetic beads utilize immobilized metal affinity chromatography to capture phosphorylated peptides. The negatively charged phosphate groups bind to the positively charged metal ions on the beads. Combined with liquid chromatography-tandem mass spectrometry (LC-MS/MS), this method facilitates the isolation, identification, and quantification of numerous phosphorylated cellular peptides. It achieves a high level of specificity and sensitivity, offering a comprehensive snapshot of phosphorylation in cellular and tissue samples (Liu et al., 2022). Basic Protocol 5 describes the detailed steps for using magnetic beads to enrich phosphopeptides.
Materials
-
Loading buffer (see recipe)
-
PTMScan phospho-enrichment IMAC Fe-NTA magnetic beads (Cell Signaling Technology, cat. no. 20432)
-
Binging/wash buffer (see recipe)
-
Elution buffer (see recipe)
-
ACN, LC-MS grade (Thermo Fisher, cat. no. 51101)
-
20% trifluoroacetic acid (TFA) (see recipe)
-
Ammonium hydroxide 28% (Sigma, cat. no. 338818)
-
Vortex
-
Centrifuge (Eppendorf, cat. no. 5425 R) or equivalent refrigerated centrifuge
-
Rotator (Barnstead/Thermolyne Labquake shaker, model 400110, cat. no. 26806) or equivalent instrument
-
20-µl pipette tip
-
1.5-ml protein LoBind tubes (Eppendorf, cat. no. 022431081)
-
Magnetic stand (Cell Signaling Technology, cat. no. 7017)
-
Parafilm
-
SpeedVac (see Basic Protocol 2 for details)
1.Add 0.95 ml loading buffer, containing 0.1% TFA and 85% ACN, to the digested peptide solution. Ensure thorough mixing by vortexing.
2.Centrifuge 5 min at 10,000 × g , 4°C, in a microcentrifuge to clear solution.
3.Rotating the PTMScan IMAC Fe-NTA magnetic beads end-over-end on a rotator for 10 min to resuspend the beads.
4.Cut off 2 mm from the tip of a 20-µl pipette tip using a razor blade or scissors. Use this tip to take 20 µl bead slurry and transfer to a 1.5-ml microcentrifuge tube.
5.Add 1 ml binding/wash buffer to wash the IMAC magnetic beads. Allow the beads to settle on a magnetic stand. Carefully remove the wash solution, taking care not to dislodge any beads. Wash 3 times.
6.Transfer the cleared peptide solution into the microcentrifuge tube containing IMAC beads.
7.Tighten the cap on the tube. Seal the top of the microcentrifuge tube with Parafilm to prevent any potential leakage. Incubate on the rotator 30 min at room temperature.
8.After incubation, allow the magnetic beads to settle on the magnetic stand, then discard the supernatant carefully; do not remove any beads. The depleted peptide solution may be frozen at −80°C and saved for later SMOAC enrichment (labeled W1).
9.Add 1 ml binding/wash buffer to wash beads, gently rotate the tube until the beads are completely resuspended.
10.Allow the beads to settle on the magnetic stand, then transfer the supernatant into a separate tube, labeled W2.
11.Wash two more times, transferring each subsequent supernatant into distinct tubes labeled W3 and W4, respectively.
12.Add 50 µl elution buffer, containing 50% ACN and 2.5% ammonia, to elute the phosphopeptides from the beads. Tap the bottom of the tube to resuspend the beads and then allow the beads to settle.
13.Rinse a fresh microcentrifuge tube with 0.5-ml ACN to remove any potential contaminants, vortex it, and discard the rinse.
14.Transfer the supernatant containing the eluted phosphopeptides to the rinsed tube.
15.Add 40 µl of 20% TFA to acidify the eluate.
16.Repeat the elution step (steps 12 to 14) once more and combine the resulting eluates.
17.Dry the eluted phosphopeptides and the combined washed supernatant (W1 to W4) in a SpeedVac at 25°C.
18.Freeze the eluted phosphopeptides at −80°C. The W1 to W4 tubes will then be used for Basic Protocol 6.
Basic Protocol 4: TiO2 ENRICHMENT
The Thermo Fisher High-Select TiO2 phosphopeptide enrichment kit offers an effective method for isolating phosphorylated peptides from complex protein digests and fractions, facilitating their analysis through mass spectrometry. TiO2 exhibits a strong affinity for phosphopeptides, as well as other acidic peptides, and is water insoluble. Phosphopeptides can be effectively captured by TiO2 beads in an acidic loading buffer and subsequently released using an alkaline elution buffer. This carefully optimized procedure, along with the specific buffers, enhances phosphopeptide yield, enabling direct MS analysis without requiring extra graphite or C18 purification steps. This method is adapted from the Thermo Fisher High-Select SMOAC protocol.
Materials
-
High-Select TiO2 phosphopeptide enrichment kit (Thermo Fisher, cat. no. A32993)
-
H2O, LC-MS grade (Thermo Fisher, cat. no. 51140)
-
0.1% formic acid, LC-MS grade (Thermo Fisher, cat. no. 85170)
-
2-ml protein LoBind tubes (Eppendorf, cat. no. 022431102)
-
Centrifuge (Eppendorf, cat. no. 5425 R) or equivalent refrigerated centrifuge
-
Vortex
-
Kimwipes
-
SpeedVac (see Basic Protocol 2 for details)
1.Remove all solutions from fridge to equilibrate the solutions to room temperature.
2.To prepare the column, place a centrifuge column adaptor in a 2-ml protein LoBind microcentrifuge tube and insert a TiO2 spin tip into the adaptor.
3.Add 20 µl wash buffer provided in the kit and centrifuge 2 min at 3000 × g , 4°C.
4.Add 20 µl binding/equilibration buffer and centrifuge 2 min at 3000 × g , 4°C.
5.Discard the flowthrough. Keep the microcentrifuge tube for step 10.
6.Transfer the prepared TiO2 spin tip and adaptor into a new 2-ml microcentrifuge tube.
7.Add 150 µl binding/equilibration buffer to completely suspend lyophilized peptide (W1 to W4).
8.Add 150 µl suspended peptide sample to the spin tip. Centrifuge 5 min at 1000 × g , 4°C.
9.Reapply sample in the microcentrifuge tube to the spin tip. Centrifuge 5 min at 1000 × g , 4°C.
10.Transfer the TiO2 spin tip and adaptor into the collection tube prepared from step 5.
11.Add 20 µl binding/equilibration buffer to wash column. Centrifuge 2 min at 3000 × g , 4°C.
12.Add 20 µl wash buffer to wash the column. Centrifuge 2 min at 3000 × g , 4°C. Save the wash fraction 1.
13.Transfer the TiO2 spin tip and adaptor into a fresh 2-ml microcentrifuge tube.
14.Repeat steps 11 and 12.
15.Add 20 µl LC-MS grade water. Centrifuge 2 min at 3000 × g , 4°C. Save the wash fraction 2.
16.Eliminate surplus liquid by gently blotting the bottom of the spin tip using a clean paper towel, such as a Kimwipe.
17.Place the spin tip and adaptor in a new 2-ml microcentrifuge tube.
18.Add 50 µl phosphopeptide elution buffer provided in the kit. Centrifuge 5 min at 1000 × g , 4°C.
19.Repeat step 18 once.
20.Promptly dry the eluate in a SpeedVac concentrator to eliminate phosphopeptide elution buffer.
21.Suspend the eluate with 50 µl of 0.1% formic acid for direct MS analysis.
22.Combine TiO2-FT from step 9, with the saved wash fraction 1 and 2 from step 12 and 15.
23.Dry the combined sample by using the SpeedVac. The dried sample can be stored at −80°C until the next procedure.
Basic Protocol 5: Fe-NTA PHOSPHOPEPTIDE ENRICHMENT
The Thermo Fisher High-Select Fe-NTA phosphopeptide enrichment kit offers a swift and effective means of isolating phosphorylated peptides, with a specificity >90%. The streamlined process allows for the enrichment of phosphopeptides from protein digests or peptide fractions, priming them for MS analysis. Each spin column, provided within the kit, is equipped with a specialized resin designed for phosphopeptide capture, boasting exceptional binding and recovery capabilities, accommodating up to 150 µg of phosphopeptides per column. This method is derived from the High-Select Fe-NTA phosphopeptide enrichment SMOAC protocol.
Materials
-
High-Select Fe-NTA phosphopeptide enrichment kit (Thermo Fisher, cat. no. A32992)
-
H2O, LC-MS grade (Thermo Fisher, cat. no. 51140)
-
0.1% formic acid, LC-MS grade (Thermo Fisher, cat. no. 85170)
-
2-ml protein LoBind tubes (Eppendorf, cat. no. 022431102)
-
Centrifuge (Eppendorf, cat. no. 5425 R) or equivalent refrigerated centrifuge
-
Vortex
-
SpeedVac (see Basic Protocol 2 for details)
1.Begin by equilibrating column, remove the spin column bottom closure, and loosen the screw cap.
2.Put column in a 2-ml microcentrifuge collection tube. Centrifuge 30 s at 1000 × g , 4°C, to eliminate storage buffer.
3.Take off the screw cap and keep it aside for use in later phosphopeptide binding step 8.
4.Add 200 µl binding/wash buffer. Centrifuge 30 s at 1000 × g , 4°C, and discard the flowthrough.
5.Repeat step 4 one more time.
6.Use a white Luer plug provided in the kit to cap the bottom of the column. Transfer the column with the plug into the empty microcentrifuge tube.
7.Add 200 µl binding/wash buffer. Completely suspend lyophilized peptide sample. Use vortex mixer with tube stand if necessary.
8.Add 200 µl peptide solution to the prepared spin column and secure the screw cap.
9.Mix the resin with the sample by gently tapping the bottom plug while holding the screw cap for 10 s, ensuring the resin becomes fully suspended.
10.Incubate for 30 min at room temperature.
11.Gently remove both the bottom plug and top screw cap. Do not compress the bottom plug, which could cause liquid to flow back into the column.
12.Put the column into the microcentrifuge tube. Centrifuge 30 s at 1000 × g , 4°C. Discard the flowthrough.
13.Add 200 µl binding/wash buffer provided in the kit to the column to wash column. Centrifuge 30 s at 1000 × g , 4°C.
14.Repeat wash step 13 two more times for a total of 3 washes. Discard the flowthrough.
15.Add 200 µl LC-MS grade water for an additional wash. Centrifuge 30 s at 1000 × g , 4°C. Discard the flowthrough.
16.To elute column, first place column in a new microcentrifuge tube. Add 100 µl elution buffer provided in the kit to the column. Centrifuge 30 s at 1000 × g , 4°C. Keep the flowthrough. Repeat this step once.
17.Immediately dry the collected eluate using a SpeedVac vacuum concentrator to eliminate the elution buffer.
18.Add 70 µl of 0.1% formic acid to suspend the dried eluate and then direct LC-MS analysis.
Basic Protocol 6: HIGH pH PEPTIDE FRACTIONATION
The Thermo Fisher Pierce high pH reversed-phase peptide fractionation kit is specifically designed to improve protein identification in complex samples via liquid chromatography-mass spectrometry (LC-MS) analysis, utilizing a high-pH reversed-phase chromatography approach. This method efficiently separates peptides based on their hydrophobic properties, offering a strong alternative to low-pH reversed-phase LC-MS gradients and demonstrating its distinct advantage over strong cation exchange (SCX) fractionation by eliminating the need for an additional desalting process before LC-MS analysis. The kit's protocol is a refined version of the original method used in the Pierce kigh pH reversed-phase peptide fractionation kit.
Materials
-
Pierce high pH reversed-phase peptide fractionation kit (Thermo Fisher, cat. no. 84868)
-
ACN, LC-MS grade (Thermo Fisher, cat. no. 51101)
-
0.1% TFA (see recipe)
-
H2O, LC-MS grade (Thermo Fisher, cat. no. 51140)
-
2-ml protein LoBind tubes (Eppendorf cat. no. 022431102) or Pierce low protein binding microcentrifuge tubes, 2-ml (Thermo Fisher, cat. no. 88379 or 88380)
-
Microcentrifuge with adjustable rotor speed up to 7000 × g
-
SpeedVac (see Basic Protocol 2 for details)
1.Take off and discard the protective white tip from the bottom of the column supplied in the peptide fractionation kit. Then, place the column into a 2-ml tube. Centrifuge 2 min at 5000 × g , 4°C. Discard the flowthrough.
2.Take off the top screw cap and add 300 µl ACN into the column. Secure the cap, place the spin column back into a 2-ml microcentrifuge tube and centrifuge 2 min at 5000 × g , 4°C. Discard flowthrough.
3.Repeat wash step 2 one more time.
4.Add 0.1% TFA to the spin column to wash. Centrifuge 2 min at 5000 × g , 4°C. Discard flowthrough. The column is now ready for use.
5.Prepare elution solutions as outlined in Table 1.
Fraction no. | Acetonitrile (%) | Acetonitrile (µl) | 0.1% Triethylamine (µl) |
---|---|---|---|
Wash | 5.0 | 50 | 950 |
1 | 10.0 | 100 | 900 |
2 | 12.5 | 125 | 875 |
3 | 15.0 | 150 | 850 |
4 | 17.5 | 175 | 825 |
5 | 20.0 | 200 | 800 |
6 | 22.5 | 225 | 775 |
7 | 25.0 | 250 | 750 |
8 | 30 | 300 | 700 |
9 | 37.5 | 375 | 625 |
10 | 50 | 500 | 500 |
6.Dissolve the peptide sample, ranging from 10 to 100 µg, in 300 µl of 0.1% TFA.
7.Transfer the spin column to a fresh 2-ml tube. Add 300 µl sample solution onto the column, reapply the cap on top. Centrifuge 1 min at 3000 × g , 4°C. Keep eluate flowthrough fraction as “flowthrough.”
8.Place the column into a new 2-ml tube. Add 300 µl of water onto the column. Centrifuge 1 min at 3000 × g , 4°C. Retain eluate as “wash” fraction.
9.Place the column into a new 2-ml tube. Add 300 µl elution solution (5% ACN, 0.1% TEA). Centrifuge 2 min at 3000 × g , 4°C, to collect the fraction.
10.Repeat step 9 for each elution step, using new 2-ml tubes and the respective elution solutions detailed in Table 1 for each fraction.
11.Use a SpeedVac or similar vacuum concentrator to evaporate all the liquid from each sample tube until dry.
Basic Protocol 7: LC-MS/MS ANALYSIS AND DATABASE SEARCH
In a typical LC-MS/MS analysis, peptides are separated based on hydrophobicity before entering mass spectrometry. The mass spectrometer operates in a “data dependent” mode where eluted peptides are isolated and fragmented inside the mass spectrometer. Accurate mass of the intact peptide and its fragments are recorded in the mass spec raw data. The raw data are then processed by a search engine to find the best match between each mass spectrum with a peptide from the designated protein database. After applying a false discovery rate (FDR) control, peptides with high confidence are inferred to proteins. A parsimony rule is applied so that the smallest list of proteins is reported to represent all peptides identified. Peptide quantification is based on abundance of TMT reporter ions.
Materials
-
Solvent A (see recipe)
-
Solvent B (see recipe)
-
Trap column, 75-μm × 2-cm Acclaim PepMap 100 trap column
-
Nanoscale HPLC system (Dionex Ultimate3000nano RSLC) with autosampler
-
Capillary analytical column, 75-μm × 250-mm Acclaim PepMap 100 column (3 μm, 100 Å)
-
High resolution mass spectrometer (Orbitrap Fusion Lumos from Thermo)
-
Data processing software (Proteome Discoverer 2.5)
1.Resuspend dry samples in an appropriate volume of solvent A before LC-MS analysis.
2.Load 1 μg tryptic digest onto a trap column and desalt it using an HPLC system at a flow rate of 4 μl/min for 5 min for each analysis.
3.Elute peptides onto a 75 µm × 250 mm Acclaim PepMap 100 column (3 µm, 100 Å).
4.Perform chromatographic separation using a two-part solvent system, with solvent A being 0.1% formic acid and solvent B combining 0.1% formic acid with 80% acetonitrile, at a flow rate of 300 nl/min.
5.Implement a gradient from 1% to 42% solvent B over 150 min.
6.Follow this by washing the column with 80% solvent B for 5 min and re-equilibrating it at 1% solvent B for 10 min before introducing the next sample.
7.Detect precursor masses in the Orbitrap with a resolution of 120,000 (m/z 200).
8.Detect higher energy collisional dissociation (HCD) fragment masses in the Orbitrap at a resolution of 50,000 (m/z 200), employing data-dependent MS/MS with a cycle time of 2 s and a dynamic exclusion period of 20 s.
9.The proteomics and phosphoproteomics data are subsequently analyzed using Proteome Discoverer (V2.5) against human protein database (Uniprot) with the following search parameters:
- a. Full trypsin cleavage with maximum 1 missed cleavage.
- b. Precursor tolerance 10 ppm.
- c. Fragment tolerance 0.02 Da.
- d. Static modification: carbamidomethylation of cysteine, TMTpro labeling of lysine and peptide N-terminus.
- e. Dynamic modification: oxidation of methionine, phosphorylation of serine, threonine, and tyrosine, and protein N-terminal acetylation.
10.A representative example of the results, like those depicted in Figure 1D, can be visualized as a volcano plot.
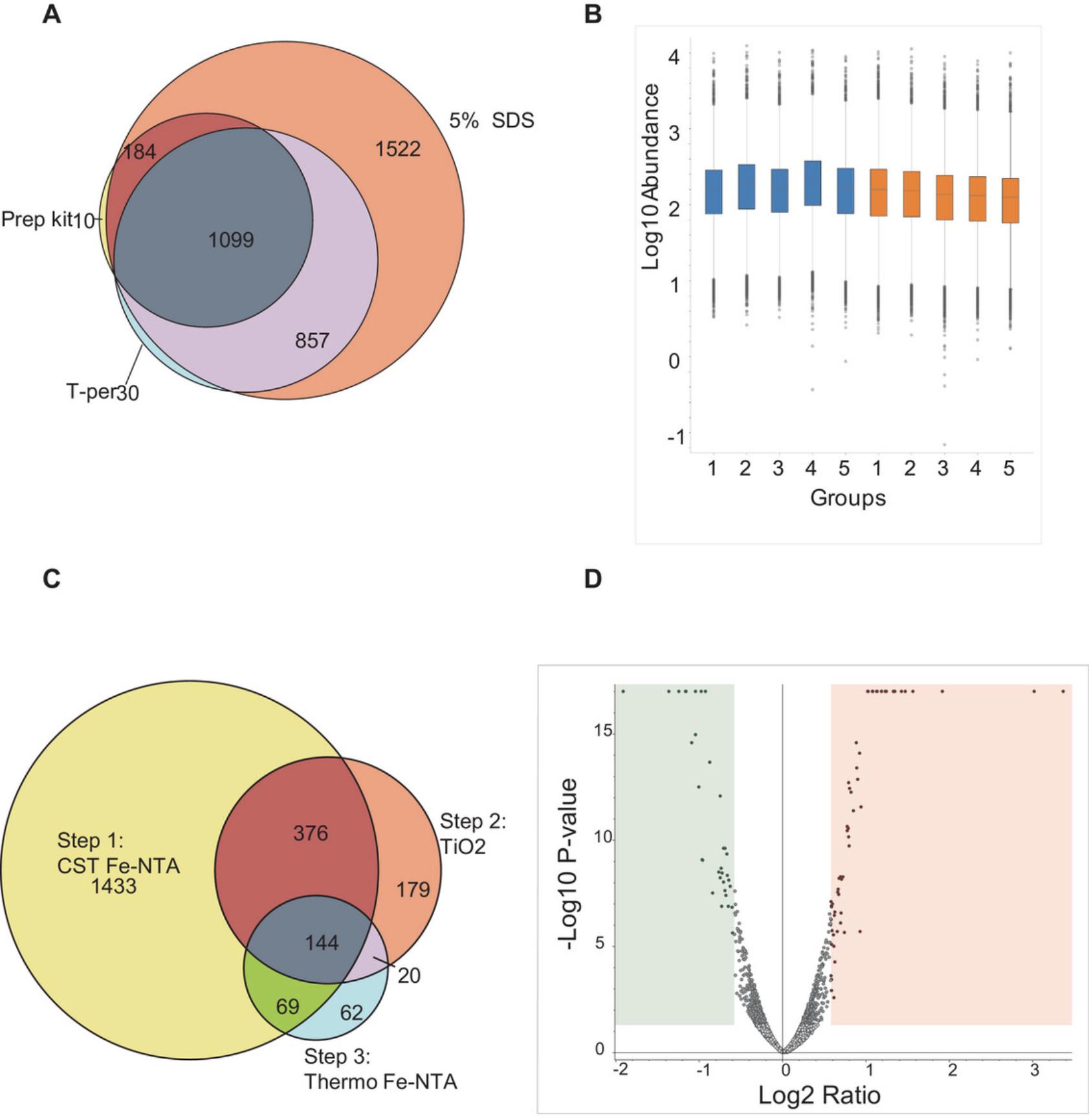
REAGENTS AND SOLUTIONS
Binding/wash buffer
- For 50 ml:
- 40 ml ACN, LC-MS grade (80% final concentration) (Thermo Fisher, cat. no. 51101)
- 9.75 ml H2O, LC-MS grade (Thermo Fisher, cat. no. 51140)
- 250 µl of 20% TFA (0.1% final concentration) (see recipe)
- Store up to 3 months at 4°C
DTT, 0.5 M
- 7.2 mg dithiothreitol (DTT) (Millipore Sigma, cat. no. D0632)
- 100 µl of 50 mM TEAB (see recipe)
- Prepare fresh the day of use
Elution buffer
- For 5 ml:
- 0.45 ml of 28% ammonia (2.5% final concentration) (Sigma, cat. no. 338818)
- 2.05 ml H2O, LC-MS grade (Thermo Fisher, cat. no. 51140)
- 2.5 ml ACN, LC-MS grade (50% final concentration) (Thermo Fisher, cat. no. 51101)
- Store up to 1 week at 4°C
Formic acid, 0.2%
- For 1 ml:
- 2 µl formic acid, LC-MS grade (Thermo Fisher, cat. no. 85178)
- 998 µl H2O, LC-MS grade (Thermo Fisher, cat. no. 51140)
- Store up to 1 week at 4°C
Formic acid, 20%
- For 1 ml:
- 200 µl formic acid, LC-MS grade (Thermo Fisher, cat. no. 85178)
- 800 µl H2O, LC-MS grade (Thermo Fisher, cat. no. 51140)
- Store up to 1 month at 4°C
Hydroxylamine, 5%
- 50 µl hydroxylamine (Thermo Fisher, cat. no. 90115)
- 450 µl of 100 mM TEAB (see recipe)
- Prepare fresh the day of use
IAA, 0.55 M
- 100 µl 50 mM TEAB (see recipe)
- 10.2 µg iodoacetamide (IAA) (Thermo Fisher, cat. no. A39271)
- Prepare fresh the day of use
Loading buffer
- For 5 ml:
- 4.25 ml Pierce ACN, LC-MS grade (85% final concentration) (Thermo Fisher, cat. no. 51101)
- 0.725 ml H2O, LC-MS grade (Thermo Fisher, cat. no. 51140)
- 25 µl of 20% TFA (0.1% final concentration) (see recipe)
- Store up to 2 weeks at 4°C
Phosphoric acid solution, 12%
- For 10 ml:
- 1.4 ml of 85% phosphoric acid (Sigma Aldrich, cat. no. 345245)
- 8.6 ml H2O, LC-MS grade (Thermo Fisher, cat. no. 51140)
- Store closed tightly as a corrosive hazardous material
- Do not store in metal containers
Solvent A
- 0.1% (v/v) formic acid, LC-MS grade (Thermo Fisher, cat. no. 85178)
- H2O, LC-MS grade (Thermo Fisher, cat. no. 51140)
- Store up to 2 weeks at room temperature
Solvent B
- 0.1% (v/v) formic acid, LC-MS grade (Thermo Fisher, cat. no. 85178)
- 80% (v/v) ACN, LC-MS grade (Thermo Fisher, cat. no. 51101)
- H2O, LC-MS grade (Thermo Fisher, cat. no. 51140)
- Store up to 2 weeks at room temperature
TEAB, 100 mM
- 500 µl of 1 M triethyl ammonium bicarbonate (TEAB) (Thermo Fisher, cat. no. 90114)
- 4.5 ml H2O, LC-MS grade (Thermo Fisher, cat. no. 51140)
- Store up to 1 month at 4°C
TEAB, 50 mM
- 1 ml of 100 mM TEAB (see recipe)
- 1 ml µl H2O, LC-MS grade (Thermo Fisher, cat. no. 51140)
- Store up to 1 month at 4°C
TFA, 0.1%
- For 10 ml:
- 10 µl Pierce trifluoroacetic acid (TFA), sequencing grade (Thermo Fisher, cat. no. 28904)
- 10 ml H2O, LC-MS grade (Thermo Fisher, cat. no. 51140)
- Store up to 2 weeks at 4°C
TFA, 20%
- 10 ml Pierce TFA, sequencing grade (Thermo Fisher, cat. no. 28904)
- 40 ml H2O, LC-MS grade (Thermo Fisher, cat. no. 51140)
- Store up to 1 month at room temperature
COMMENTARY
In a global phosphoproteomic analysis, the use of a large number of cells, tissues, or other biological material is recommended due to the inherently low levels of phosphorylated proteins in most biological systems and the potential loss occurring across multiple steps of sample preparation. Limited sample size poses a challenge in achieving optimal protein and peptide recoveries. To address this, we present a detailed method for enriching phosphoproteins, allowing for the use of limited sample size tissues while maintaining high-quality phosphoproteomic data. This method is suitable for small tissue samples while ensuring high-quality phosphoproteomic data. Our approach involves a 3-step sequential enrichment process: initially using Fe-NTA magnetic beads from Cell Signaling Technology, followed by TiO2 enrichment, and concluding with secondary Fe-NTA phosphopeptide enrichment.
Critical Parameters
Lysis buffer
In the lysis stage, selecting the appropriate lysis buffer is critical. This buffer aids in breaking down cells or tissues for protein extraction. Both the type and volume of the buffer might require adjustments based on the initial sample and the target protein concentration. Factors to consider include the cell or tissue type and size, along with the need for effective protein extraction. In our study, we evaluated three different lysis buffers for trigeminal ganglion tissue: T-per (Thermo Fisher Scientific, cat. no. 78510) with protease/phosphatase inhibitors, EasyPep™ Mini MS Sample Prep Kit lysis buffer (Thermo Fisher Scientific, cat. no. A40006), and a 5% SDS solution. Following the application of these lysis buffers, we proceeded with the Basic Protocol 1 for protein digestion and then assessed the protein yield. Our findings indicate that in the case of neuronal tissue, a 5% SDS lysis buffer is most effective. Further details on these findings will be provided in the Understanding Results section.
Protein concentration test
Determining protein concentration is a critical step in preparing samples for various downstream applications. Although this protocol does not delve deeply into the specific method used for determining protein concentration, it acknowledges the variability in available methods. Researchers often use techniques such as the BCA assay to quantify protein content.
Protein cleanup
Protein cleanup is crucial for removing contaminants and substances that might interfere with subsequent analyses. The timing of this cleanup, either before or after TMT labeling, is flexible. However, performing cleanup after TMT labeling can improve efficiency by allowing the combination of labeled samples into a single tube. This step streamlines the process, simplifying downstream processing and analysis for a more efficient workflow.
Phosphopeptide enrichment
When considering the sequence of phosphopeptide enrichment methods, it is advantageous to employ the CST Fe-NTA magnetic Beads method before utilizing the TiO2 and SMOAC methods. This is primarily because the final step in the SMOAC method involves repeating the Fe-NTA enrichment, that when combined with the initial Fe-NTA step, yields a more abundant result. This observation is corroborated by the Venn diagram presented in Figure 1C, which demonstrates that each enrichment step captures distinct phosphopeptides. See Understanding Results for a more comprehensive discussion of these findings.
Troubleshooting
Please see Table 2 a troubleshooting guide for proteomic and phosphoproteomics analysis for limited tissue.
Problem | Possible cause | Solution |
---|---|---|
Low protein yield | Insufficient lysis of the tissue | To enhance tissue lysis, consider increasing the volume of the lysis buffer or incorporating additional mechanical disruption methods; these steps can help prevent the formation of an insufficiently lysed tissue pellet after centrifugation |
Reduced effectiveness in tryptic digestion of proteins | The concentration of protease might be insufficient; additionally, the presence of bubbles on top of the trap could hinder the exposure of the sample to the digestive protease | Adjust the enzyme-to-protein ratio or extend the digestion time, such as by allowing for an overnight digest in an incubator at 37°C; to ensure there are no bubbles, conduct a visual inspection; if bubbles are present, gently flick the container until they rise to the surface |
Poor peptide recovery | The column dried out during digestion due to being uncapped | Rehydrate the protein-trapping matrix to solubilize the peptides; add digestion buffer without the enzyme and allow it to sit for 30 min, followed by centrifugation; subsequently, repeat the washing and elution steps |
Low binding efficiency of Fe-NTA magnetic beads | Wrong elution buffer used | Double check all buffer layouts; continue with the process, as the subsequent TiO2 step may still capture phosphopeptides |
Spin tip clogged | Particles present in the sample caused by the incomplete dissolution of the protein digest sample in binding/equilibration buffer | To ensure complete dissolution of the digested peptide sample, use a vortex mixer or centrifuge the sample prior to applying it to the spin tip |
Understanding Results
This protocol outlines a workflow for preparing limited protein tissue samples, applicable to other neuronal tissues. We conducted tests with various lysis buffers, as shown in Figure 1A and observed that different lysis buffers yielded varying protein quantities. When we employed T-per and added proteases, it resulted in a total recovery of 1995 proteins. By using a miniprep kit from Thermo Fisher, we successfully identified 1283 proteins. The utilization of a 5% SDS lysis buffer allowed us to identify a total of 3662 proteins. The Venn diagram illustrates the overlap and unique proteins identified with different lysis buffers. Consequently, for neuronal tissues, such as trigeminal ganglion or dorsal root ganglion, we recommend using a 5% SDS lysis buffer.
Following protein digestion and cleanup, TMT labeling is executed to consolidate the protein samples for subsequent workflow steps. This process is vital for ensuring efficient downstream analysis. Post-labeling, we routinely assess the effectiveness of TMT labeling. Typically, we analyze 1 μg of the labeled sample to confirm that each sample has been sufficiently and uniformly labeled, as illustrated in Figure 1B. Ensuring even labeling of samples is crucial, as it significantly impacts the accuracy and reliability of subsequent experimental stages, including quantitative analysis and the detection of subtle changes in protein expression across different samples.
In our protocol, we outline a three-step enrichment process aimed at improving phosphopeptide identification. We successfully identified a total of 4454 phosphopeptides. Figure 1C details the number of phosphopeptides identified via each enrichment step. The initial Fe-NTA method led to the identification of 1433 phosphopeptides, followed by the TiO2 step, which uncovered an additional 199 phosphopeptides. The third step, employing Fe-NTA, revealed 62 more phosphopeptides. There is a final fractionation step of the combined sample from the above three-step enrichments, resulting in a total of 4454 phosphopeptides. Each method uniquely captures different proteins, thereby enhancing the overall yield in phosphoproteomics. Furthermore, although not illustrated in the figure, we identified a total of 2923 phosphoproteins, corresponding to 13,460 peptide groups.
The volcano plot in Figure 1D displays the global phosphoproteome data from trigeminal ganglion. It plots log2-fold changes on the x-axis against p -values on the y-axis. Using high-sensitivity LC-MS/MS for proteomics and phosphoproteomics, we identified peptides and phosphopeptides with differential expression in the trigeminal ganglia. A peptide is deemed differentially expressed if it exhibits a fold change >1.5 and a p -value <.05. The plot, generated by Proteome Discoverer 2.5, shows the overall peptide profile, highlighting peptides with significant increases or decreases. In the treated group, analysis revealed 61 upregulated and 33 downregulated peptides within the total proteome dataset.
Time Considerations
Basic Protocol 1 takes ∼1 to 2 hr, including the BCA assay process. Basic Protocol 2 requires ∼4 to 5 hr, which includes the drying process. Drying times may vary depending on the equipment used. Basic Protocols 3 and 4 together take ∼1 to 2 hr. Basic Protocol 5 lasts 5 to 6 hr and incorporates the drying process. Basic Protocol 6 is completed in ∼2 to 3 hr, including the drying process. Basic Protocol 7 takes ∼4 to 5 hr including drying process. Basic Protocol 8 takes ∼6 hr.
Acknowledgments
This research was supported by the Intramural Research Program of the NIH, NIDCR (ZIA DE000664, ZIA DE000719). The NIDCR Mass Spectrometry Facility is supported by the Division of Intramural Research, NIDCR/NIH (ZIA DE000751).
Author Contributions
Minghan Hu : Conceptualization; investigation; methodology; resources; writing original draft; writing review and editing. Yan Wang : Methodology; resources; software; visualization; writing review and editing.
Conflict of Interest
The authors declare no conflict of interest.
Open Research
Data Availability Statement
Data sharing is not applicable to this article as no new data were created or analyzed in this study.
Literature Cited
- Al-Amrani, S., Al-Jabri, Z., Al-Zaabi, A., Alshekaili, J., & Al-Khabori, M. (2021). Proteomics: Concepts and applications in human medicine. World Journal of Biological Chemistry , 12(5), 57–69. https://doi.org/10.4331/wjbc.v12.i5.57
- Breitkopf, S. B., & Asara, J. M. (2012). Determining in vivo phosphorylation sites using mass spectrometry. Current Protocols in Molecular Biology , 18, Unit18 19 11–27.
- Dunn, J. D., Reid, G. E., & Bruening, M. L. (2010). Techniques for phosphopeptide enrichment prior to analysis by mass spectrometry. Mass Spectrometry Reviews , 29(1), 29–54. https://doi.org/10.1002/mas.20219
- Hu, M., Doyle, A. D., Yamada, K. M., & Kulkarni, A. B. (2022). Visualization of trigeminal ganglion sensory neuronal signaling regulated by Cdk5. Cell reports , 38(10), 110458.
- Katzenell, S., Cabrera, J. R., North, B. J., & Leib, D. A. (2017). Isolation, purification, and culture of primary murine sensory neurons. Methods in Molecular Biology , 1656, 229–251.
- Liu, X., Rossio, V., Thakurta, S. G., Flora, A., Foster, L., Bomgarden, R. D., Gygi, S. P., & Paulo, J. A. (2022). Fe(3+)-NTA magnetic beads as an alternative to spin column-based phosphopeptide enrichment. Journal of Proteomics , 260, 104561. https://doi.org/10.1016/j.jprot.2022.104561
- Messlinger, K., & Russo, A. F. (2019). Current understanding of trigeminal ganglion structure and function in headache. Cephalalgia , 39(13), 1661–1674. https://doi.org/10.1177/0333102418786261
- Switzar, L., Giera, M., & Niessen, W. M. (2013). Protein digestion: An overview of the available techniques and recent developments. Journal of Proteome Research , 12(3), 1067–1077. https://doi.org/10.1021/pr301201x
- Waas, M., Pereckas, M., Jones Lipinski, R. A., Ashwood, C., & Gundry, R. L. (2019). SP2: Rapid and automatable contaminant removal from peptide samples for proteomic analyses. Journal of Proteome Research , 18(4), 1644–1656. https://doi.org/10.1021/acs.jproteome.8b00916
- Wang, F., Veth, T., Kuipers, M., Altelaar, M., & Stecker, K. E. (2023). Optimized suspension trapping method for phosphoproteomics sample preparation. Analytical Chemistry , 95(25), 9471–9479.