Establishment of a Four-Cell In Vitro Blood-Brain Barrier Model With Human Primary Brain Cells
Johid R. Malik, Johid R. Malik, Ukamaka O. Modebelu, Ukamaka O. Modebelu, Courtney V. Fletcher, Courtney V. Fletcher, Anthony T. Podany, Anthony T. Podany, Kimberly K. Scarsi, Kimberly K. Scarsi, Siddappa N. Byrareddy, Siddappa N. Byrareddy, Robbyn K. Anand, Robbyn K. Anand, Shilpa Buch, Shilpa Buch, Susmia Sil, Susmia Sil, Jennifer Le, Jennifer Le, John S. Bradley, Ashley N. Brown, Debapriya Sutar, Sean N. Avedissian
Abstract
The blood-brain barrier (BBB) constitutes a crucial protective anatomical layer with a microenvironment that tightly controls material transit. Constructing an in vitro BBB model to replicate in vivo features requires the sequential layering of constituent cell types. Maintaining heightened integrity in the observed tight junctions during both the establishment and post-experiment phases is crucial to the success of these models. We have developed an in vitro BBB model that replicates the cellular composition and spatial orientation of in vivo BBB observed in humans. The experiment includes comprehensive procedures and steps aimed at enhancing the integration of the four-cell model. Departing from conventional in vitro BBB models, our methodology eliminates the necessity for pre-coated plates to facilitate cell adhesion, thereby improving cell visualization throughout the procedure. An in-house coating strategy and a simple yet effective approach significantly reduce costs and provides superior imaging of cells and corresponding tight junction protein expression. Also, our BBB model includes all four primary cell types that are structural parts of the human BBB. With its innovative and user-friendly features, our in-house optimized in vitro four-cell-based BBB model showcases novel methodology and provides a promising experimental platform for drug screening processes. © 2024 The Authors. Current Protocols published by Wiley Periodicals LLC.
Basic Protocol 1 : Coating and culture system
Basic Protocol 2 : Cell seeding and Transwell insert handling
Basic Protocol 3 : Assessment of model functionality
INTRODUCTION
The blood-brain barrier (BBB) is a structurally dynamic microenvironment that protects the central nervous system (CNS) by acting as an entry and exit checkpoint for molecules to and from CNS (Cecchelli et al., 2007). Its major cellular components are brain microvascular endothelial cells (BMECs), astrocytes, pericytes, and neuronal projections, which play crucial roles in paracellular exchange, cytoplasmic intake, and exocytosis of essential molecules (Liu et al., 2012). In an optimal scenario aiming to mimic the in vivo BBB, it is important that an in vitro BBB model include all four major cell types. These protocols introduce a novel in vitro BBB model that employs the four primary human brain cells and enables more comprehensive image analysis compared to previous work (Appelt-Menzel et al., 2017; Bagchi et al., 2019; Wang et al., 2015). In vitro humanized BBB models are essential for understanding drug transport across the human BBB (https://www.nal.usda.gov/animal-health-and-welfare/animal-use-alternatives), and the Transwell in vitro BBB models mimicking physiological barriers offer a method for screening potential drug candidates for CNS penetration (Ghaffarian & Muro, 2013; Santaguida et al., 2006).
Immortalized BMECs have been used to explore the complexity and functionality of the human BBB (Sano et al., 2010; Weksler et al., 2005). Although BMEC monolayer BBB models have been successfully established on Transwell membranes, understanding of complexity, inter-cellular communication, and response mechanisms was lacking in these models (Banks et al., 2018). Further, using immortalized cells results in poor BBB properties in regard to intervascular networks and expression of tight junction proteins and causes loss of crucial in vivo BBB permeability functions (Rist et al., 1997; Watanabe et al., 2013). Though the human induced pluripotent stem cell (hiPSC)-based BBB models provide a consistent source of cells with better reproducibility and better barrier formation, their technical complexity and higher cost makes these undesirable for regular drug-screening processes or other, similar work in a relatively smaller lab setup (Lippmann et al. 2012). Recently, physiologically humanized in vitro BBB models have been developed that, again, are expensive and require dedicated space for different steps of BBB development. A microfluidics-based BBB model provides a three-dimensional (3D) network and can also be designed to incorporate recirculation, but the fabrication of the microfluidic device itself requires substantial investment and a dedicated clean room if 3D printers are to be utilized to develop the fluidic chips. Furthermore, this model lacks a means to measure the crucial parameter of resistance between tissue layers, or trans-endothelial electrical resistance (TEER, discussed in the next paragraph; Hajal et al., 2022). More details on the recent development and comparative study of various in vitro models have been compiled by Shah and Dong (Shah & Dong, 2022). Given the technical complexities, costs, and space requirements, the static model is a preferred experimental setup for drug screening and toxicological studies, and more recently researchers have employed four cell types in constructing these in vitro BBB models (Bagchi et al., 2019), improving on earlier methodologies (Allen & Bayraktutan, 2009; Hind et al., 2015; Stone et al., 2019). However, the manufacturer's discontinuation of the specific precoated plates used by previous researchers (Stone et al., 2019) requires modification of available resources and standardization of protocols.
Our enhanced methodology addresses these challenges and mitigates dependence on specific aspects of the BBB model (Stone et al., 2019). We determined that polyester membranes with a 3-µm pore size are superior to polycarbonate for visual confirmation of cell adherence and growth under a microscope (Stone et al., 2019). Shifting from translucent polycarbonate to polyester Transwell plates enhanced visualization and allowed improved confirmation of cell adherence. The initial TEER value is assessed using an EVOM2 (epithelial volt-ohm) meter, which is specifically designed to measure electrical resistance in tissue culture, as detailed on the manufacturer's website (https://www.wpiinc.com/company/our-history/). This instrument accurately measures the electrical resistance across the layers of cells. In addition to ensuring superior cell visibility and enhanced imaging on the polyester Transwell membrane, our protocols also yield higher and more stable TEER in the BBB model compared to other earlier models. The optimal coating combination of collagen, followed by fibronectin and poly-L-lysine, identified for use with polycarbonate membrane has been successfully carried forward with polyester membrane (Paradis et al., 2016). The study also demonstrated the expression of specific surface proteins using protein-specific antibodies (Paradis et al., 2016). The basolateral astrocyte-pericyte co-culture and apical human brain endothelial cells (hBMECs) are identified with astrocyte-specific anti-S100B antibody (red), and the co-cultured pericyte along with hBMECs in the contact culture is recognized by anti-CD146 (green) antibody. In the absence of serum, claudin 5 detection on astrocytes and pericytes is poor, as documented, but the presence of zinc increases claudin 5 expressions. No visible difference is noted for zona occludens-1 (ZO-1), and similar fluorescence visibilities for claudin 5 are detected in hBMECs regardless of the presence of zinc. Inclusion of zinc also induces elevated claudin 5 expression not only in our BBB model but also in neuronal cells. The functionality of the BBB model is assessed based on the penetration of dolutegravir (an antiretroviral) through the intact BBB.
Here, we detail the pertinent materials and stepwise basic protocols for our four-cell-based BBB model, including (1) coating strategies for cells and culturing system (Basic Protocol 1); (2) cell seeding, Transwell membrane, and four-cell integration (Basic Protocol 2); and (3) assessment of the functionality of the four-cell model by measuring TEER and fluorescent intensity (Basic Protocol 3). In addition, we analyze the penetration of dolutegravir (DTG) as a proof of concept to demonstrate the functionality of our BBB model. The timing and steps for the entire experiment, from astrocyte seeding till termination of BBB setup, are outlined in Figure 1.
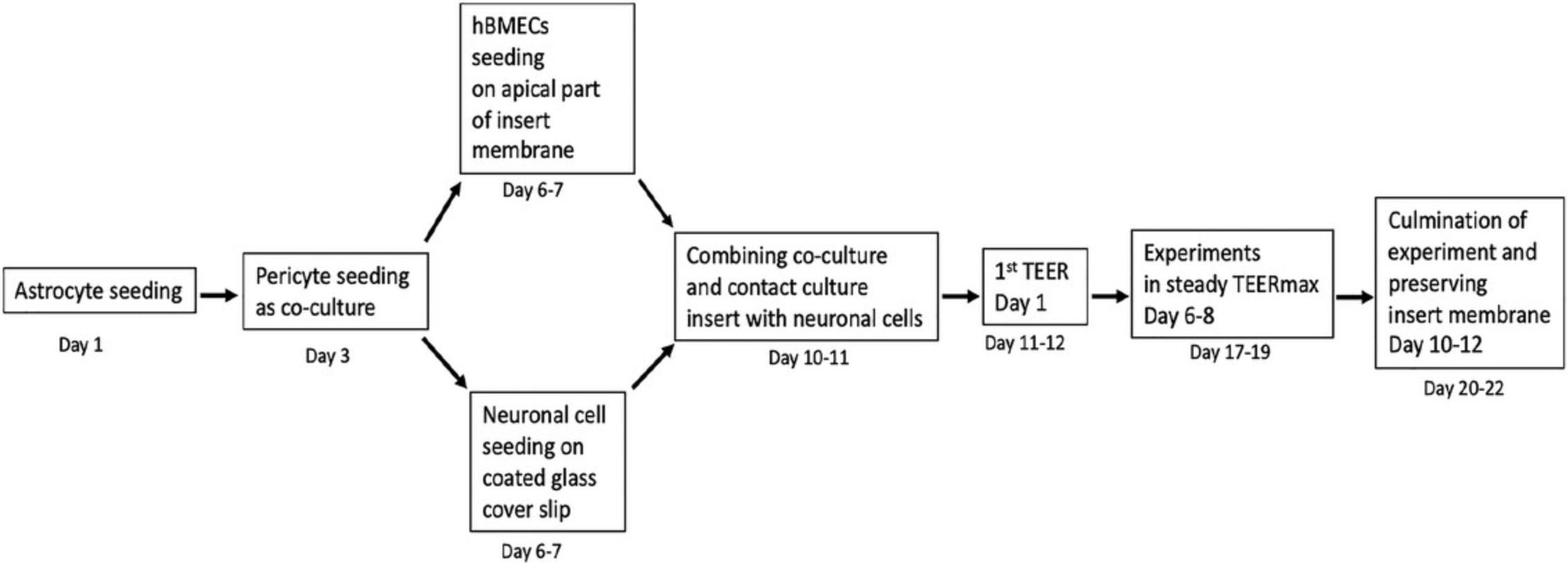
NOTE : Always turn your UV light and cell culture cabinet 30 min before the start of the experiment to help prevent and kill microbes/contaminants. Ensure that an extra set of coated flasks and Transwell plates with inserts is ready and available throughout the experiment. Exercise extreme caution with the cell medium (through appropriate labeling) to avoid mixing up the cell types.
NOTE : Thawing cells for subculture is a critical step, and cells should not be left longer than 2 min in a 37°C water bath. Do not centrifuge thawed cells; rather, immediately add them into the medium to dilute the DMSO in the freezing mix (10:1 medium:DMSO). Maintain a single-cell suspension by gentle pipetting, which enhances the chances of achieving a monolayer in the cell culture flask/Transwell membrane. Use personal protective equipment (lab coat, hand gloves, 70% ethanol for sterilizing items, eye protection) at all times. This will decrease the risk of contamination. After every experiment, clean the cell culture cabinet with 70% ethanol and turn on the UV lamp for 15 min; then turn off the cabinet after the designed time has elapsed. Because the BBB model will develop in Transwell plates, which do not represent a completely closed environment, extra care should be taken with the culture incubator and the handling of the plates. All media used for all types of cells in culture flasks and Transwell plates are the corresponding complete media (CM; see recipe).
Basic Protocol 1: COATING AND CULTURE SYSTEM
Given that human primary cells are adherent cells, they require a surface for optimal growth. However, specific cell types require specific treatment for attachment to any surface. The coating with the various proteins and reagents mentioned above acts as an extracellular matrix for enhanced adherence and better growth. For cell culture, only fibronectin is required; however, this is insufficient by itself for growing cells on Transwell membranes. Here, collagen I provides the base, fibronectin provides additional matrix strength, and poly-L-lysine facilitates quick initial attachment.
NOTE : The steps below apply to astrocytes, pericytes, and hBMECs. The experiments are conducted within a biosafety cabinet, maintaining aseptic conditions, particularly due to the susceptibility of primary cells to potential contaminates. For astrocyte, pericyte, and hBMEC culture initiation, all three cell types are seeded into the flask on the same day; however, if needed, pericytes and hBMECs can later be split and brought back to 90% confluency to match the timeline for seeding on already astrocyte-seeded Transwell membrane. The neurons are used directly from the frozen vial for seeding on a glass coverslip in a separate 12-well plate.
Materials
-
Dulbecco's phosphate-buffered saline (DPBS; Gibco, cat. no. 14190144)
-
Bovine plasma fibronectin (cat. no. 8248, ScienCell Research Laboratory [SCRL])
-
Bovine collagen I working solution (see recipe)
-
Poly-L-lysine (Sigma, cat. no. P4707-50ML)
-
Sterile water
-
FBS (cat. no. 0010, SCRL)
-
Penicillin/streptomycin solution (P/S; cat. no. 0503, SCRL)
-
Appropriate cells, media, and supplements:
- Primary human brain astrocytes (SCRL, cat. no. 1800) and complete medium (CM; see recipe) prepared with Astrocyte Medium (AM; SCRL, cat. no. 1801) and Astrocyte Growth Supplement (AGS; SCRL, cat. no. 1852)
- Primary human brain pericytes (SCRL, cat. no. 1200) and CM (see recipe) prepared with Pericyte Medium (PM; SCRL, cat. no. 1201) and Pericyte Growth Supplement (PGS; SCRL, cat. no. 1252)
- Human brain microvascular endothelial cells (hBMECs; SCRL, cat. no. 1000) and CM (see recipe) prepared with Endothelial Cell Medium (ECM; SCRL, cat. no. 1001) and Endothelial Growth Supplement (ECGS; SCRL, cat. no. 1052)
- Human neurons (SCRL, cat. no. 1520) and CM (see recipe) prepared with Neuronal Medium (SCRL, cat. no. 1521) and Neuronal Growth Supplement (SCRL, cat. no. 1562)
-
70% (v/v) ethanol
-
Biosafety cabinet
-
150-cm2 culture flasks (TPP cat. no. 90076)
-
Refrigerator and freezer
-
12-well Transwell plate with inserts (Corning cat. no. 3462)
-
CO2 cell culture incubator, 37°C
-
50-ml tubes
-
10 ml sero-pipet
-
Pipets
-
200- and 500-ml glass beakers
-
EVOS Floid light microscope (Life Sciences)
-
37°C water bath
-
Centrifuge for 50-ml and smaller sample volumes
Cell culture initiation
Flask coating
1.Prepare in advance for all potential experimental procedures. Coat a 150-cm2 flask with 10 ml of fibronectin in DPBS and incubate overnight at 37°C (add the required volume of fibronectin in DPBS to make it 3 µg/ml and mix it by gently pipeting)
2.The following day, remove the solution from the flask and rinse with 10 ml DPBS. Store the flask at 4°C.
Transwell insert coating
3.Coat Transwell inserts by adding 1 ml bovine collagen I working solution to each well of a 12-well Transwell plate with inserts. Incubate plate overnight at 4°C to facilitate even coating of the surfaces.
4.Next day, remove the solution by aspirating it with a pipet and then wash the insert well plate once with DPBS.
5.Perform the second coating step by adding 1 ml of 3 µg/ml of fibronectin in DPBS to each plate well and incubating overnight at 37°C.
6.Next day, aspirate the solution from the insert wells and wash the plate once with DPBS before storing it at 4°C for future use.
Cell culture procedures
7.On the day of the experiment, remove the coated flask (from step 2) from the 4°C refrigerator and place it in the biosafety cabinet.
8.Prewarm all the medium components (FBS, PS, and the four types of media and supplements) in a 37°C water bath.
9.Prepare the required volume of complete medium (CM; see recipe) appropriate for the cells to be cultured.
10.Dispense 20 ml CM into the precoated 150-cm2 flask and place it in the 37°C CO2 incubator to prewarm for ∼10-15 min.
11.Meanwhile, retrieve the frozen cell vials, promptly thaw them in a 37°C water bath, and disinfect the outsides of the vials with 70% ethanol before transferring them into the biosafety cabinet. Introduce the cells from the vial into the flask containing prewarmed medium.
12.Transfer the flask with the cells into the 37°C CO2 incubator until they reach 90% confluency. Change the medium every 48 hr using the appropriate CM for each cell type to ensure optimal cell growth.
Basic Protocol 2: CELL SEEDING AND TRANSWELL INSERT HANDLING
The Transwell inserts with delicate polyester membranes support the optimal growth of any cells. The development of the BBB model starts with seeding different types of cells on either side of the Transwell insert membrane.
NOTE : When inverting the a plate on the plate lid in the biosafety cabinet, avoid touching with hands directly; instead, use forceps, and take special care not to touch near the Transwell membrane. The volume of AM-containing astrocytes is standardized to ensure optimal contact of the cell medium drop sitting on the Transwell membrane, with the bottom of the well covering it for proper surface tension. This ensures complete covering of the entire membrane surface with cell medium for even distribution of the cells across the membrane.
Materials
-
Cultures of astrocytes, pericytes, hBMECs, and neurons (Basic Protocol 1)
-
Astrocyte, pericyte, hBMEC, and neuronal complete media and supplements (Basic Protocol 1)
-
Dulbecco's phosphate-buffered saline (DPBS; Gibco, cat. no. 14190144)
-
0.25% trypsin/EDTA (cat. no. CC-5012, Lonza)
-
Trypan blue (ThermoFisher scientific, cat. no. T10282)
-
Fibronectin- and collagen-coated Transwell plates (Basic Protocol 1)
-
Biosafety cabinet
-
37°C water bath
-
10-ml sero-pipet
-
CO2 cell culture incubator, 37°C
-
EVOS Floid light microscope, Life Science.
-
Cell counting chamber slides (Invitrogen, cat. no. C10228)
-
Cell counter (Countess, Invitrogen cat. no. C10281)
-
200- and 500-ml glass beakers
-
50- and 1-ml centrifuge tubes
-
Centrifuge for 50-ml and smaller sample volumes
-
Forceps
-
16- to 18-mm-diameter, 0.15-mm-thick round glass coverslip
Astrocyte cell collection and seeding on Transwell inserts
Astrocytes are the first type of cells to be seeded on the basolateral part of the Transwell insert membrane. Carry out all steps inside the biosafety cabinet.
1.When the astrocytes reach 90% confluency, transfer astrocyte-containing flask to the biosafety cabinet.
2.Decant the medium from the flask, wash the flask once with 10 ml DPBS, add another 10 ml DPBS, and incubate the flask in the culture incubator for 10-15 min to ensure easy detachment of cells.
3.Remove trypsin/EDTA from −20°C storage and prewarm it to 37°C.
4.After 10-15 min, remove the flask of cells from the incubator, pipet out the DPBS, and add 5 ml warmed trypsin/EDTA (150-cm2 flask).
5.Return the flask to the 37°C CO2 incubator and perform visual checks every 30 sec for 2 min under a microscope to observe cell detachment. Continue observing the flask until all cells appear to have detached from the bottom of the flask.
6.When all cells appear to be floating, immediately dilute trypsin/EDTA in the flask by adding 45 ml DPBS.
7.Transfer the DPBS cell mixture from the flask to a 50-ml tube using a 10-ml pipet. Centrifuge for 5-10 min at 200 RCF, room temperature.
8.Discard the supernatant and disperse the pellet in 10 ml astrocyte CM.
9.Mix a 10-µl well-dispersed sample of cells with 10 µl trypan blue dye in a 1-ml centrifuge tube. Determine the cell count using a cell counter.
10.Remove the collagen- and fibronectin-coated insert plate from the 4°C refrigerator and give it a final coating by placing it on top of a 50-µl drop of 0.01% poly-L-lysine and then allowing it to sit for 5 min at room temperature. Remove the excess poly-L-lysine from the insert and allow it to air dry.
11.Flip the coated and air-dried Transwell inserts to an inverted position on the plate lid so that the bottom of the Transwell membrane faces upwards (Fig. 2).
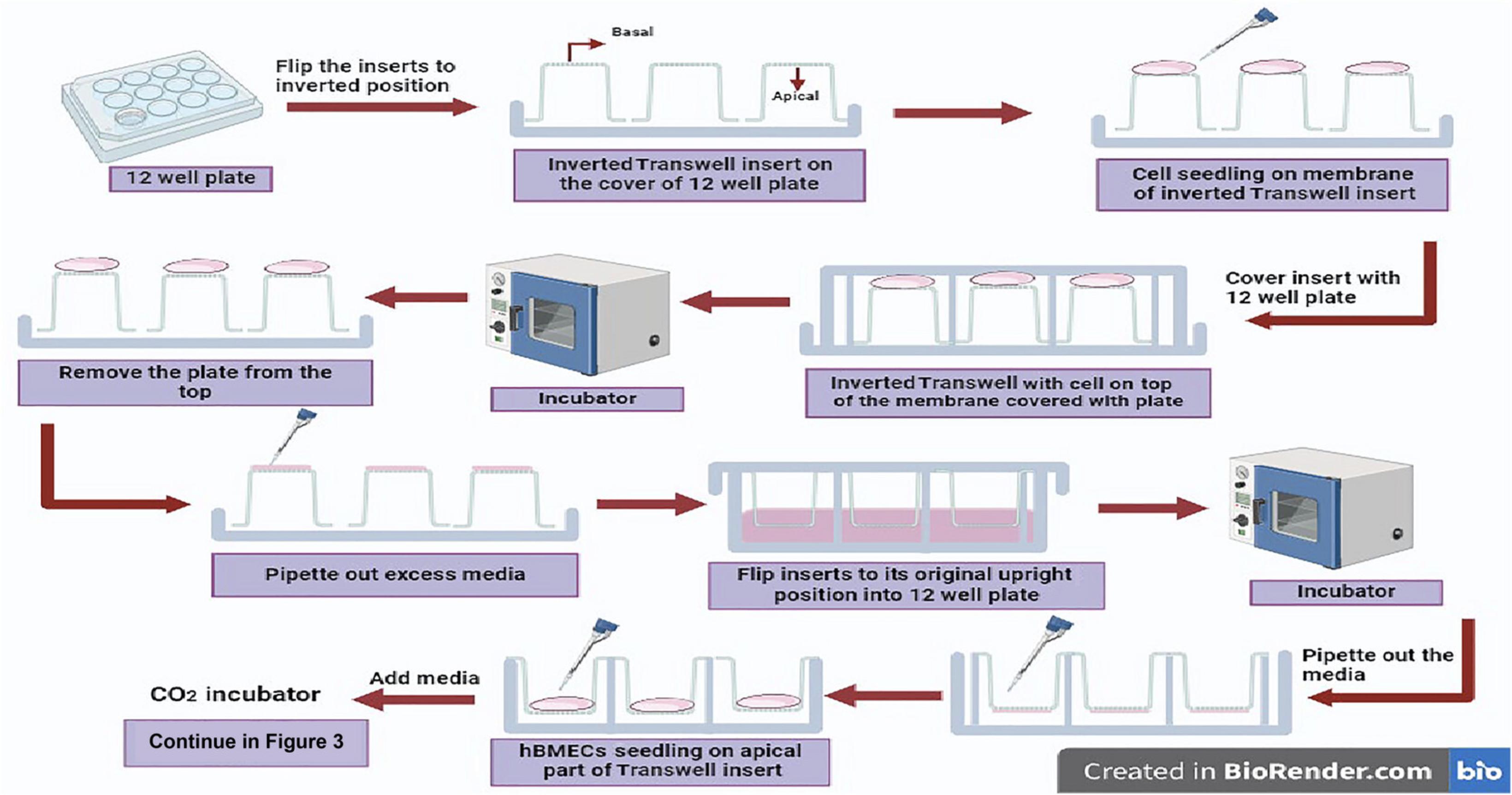
12.Prepare astrocyte cell suspension with cell concentration of 4 × 106 cells/ml in astrocyte CM (Carefully add 150 µl of the cell suspension on the basolateral side of the inverted Transwell insert membranes placed on the plate lid (Fig. 2).
13.Immediately place the plate back onto the lid containing the inverted Transwell inserts with seeded cells (Fig. 2), and incubate the plate in that inverted position in a 37°C CO2 incubator for 3 hr. Next, aspirate the excess medium, return each Transwell insert to its original position, and add 1.5 ml of fresh astrocyte CM to each well, covering the apical compartment. Incubate for 48 hr to allow astrocytes to grow (Fig. 2).
Pericyte cell seeding on insert membrane
14.Perform pericyte seeding by repeating steps 1-11, except starting with pericytes and using pericyte CM in step 8.
15.Prepare pericyte cell suspension with a cell concentration of 1.2 × 105/150 µl in pericyte CM (maintaining a 1:5 ratio of astrocytes to pericytes). Carefully add 150 µl of the cell suspension on the basolateral side of the inverted Transwell membrane, on top of the astrocytes, and incubate the plate in a 37°C CO2 incubator for 3 hr.
16.Aspirate any excess medium and return the Transwell inserts to their normal position. Add 1.5 ml of a 1:1 mixture of astrocyte CM/pericyte CM to each Transwell well and continue incubating for an additional 5 days, changing medium every 48 hr.
SeedihBMEC seeding on insert membrane
17.Perform hBMEC seeding by repeating steps 1-11 starting with hBMECs, except using endothelial CM in step 8.
18.To prevent the cells growing on the Transwell membrane from drying out, in advance, prepare hBMEC cell suspension with a cell concentration of 7.51 × 104/200 µl in endothelial CM. Carefully add 200 µl of the cell suspension on the apical side of each Transwell insert and incubate the plate in a 37°C CO2 incubator for 6 hr.
19.After 7 days from the initial seeding of astrocytes and subsequent seeding of pericytes on the same Transwell membrane, aspirate medium from the apical and basal regions of the Transwell inserts with hBMCs. Meanwhile, aspirate the apical layer medium from the plate containing astrocytes and pericytes, leaving the basal medium.
20.Seed the hBMECs from step 18 (7.51 × 104/200 µl hBMECs in endothelial CM) onto the apical side of the Transwell inserts containing astrocytes and pericytes in the basolateral part (Fig. 2).
21.Incubate the hBMEC-seeded Transwell plates for 6 h. Add 400 µl endothelial CM to the apical region of the Transwell membrane and 0.7 mL of 1:1 complete AM:PM in the basal region, continue incubating the plate (see the time schematic in Fig. 1), changing the medium every 48 hr.
Seeding of neurons on a glass coverslip
Neurons are the fourth type of cells in this in vitro BBB model. Because neurons do not divide after maturation, they should not be cultured; instead, the neuron cell vial is used directly and should contain the required number of neuron cells initially.
22.Working in parallel, on same the day as hBMEC seeding (day 7 after the initial seeding with astrocytes), coat 16- to 18-mm-diameter, 0.15-mm-thick round glass coverslips with poly-L-lysine by dipping the required number of coverslips in a 50-ml tube containing poly-L-lysine for 5 min at room temperature and then air drying them. After coating the glass coverslip for 5 min, keep the individual pieces of glass coverslip on a paper towel inside the biosafety cabinet to allow them to air dry. Once dry, place each coverslip at the bottom of a well in a separate sterile 12-well plate (without inserts). Next, add 200 µl of 2.5 × 104 human neurons in neuronal CM onto each coated coverslip (Fig. 3A).
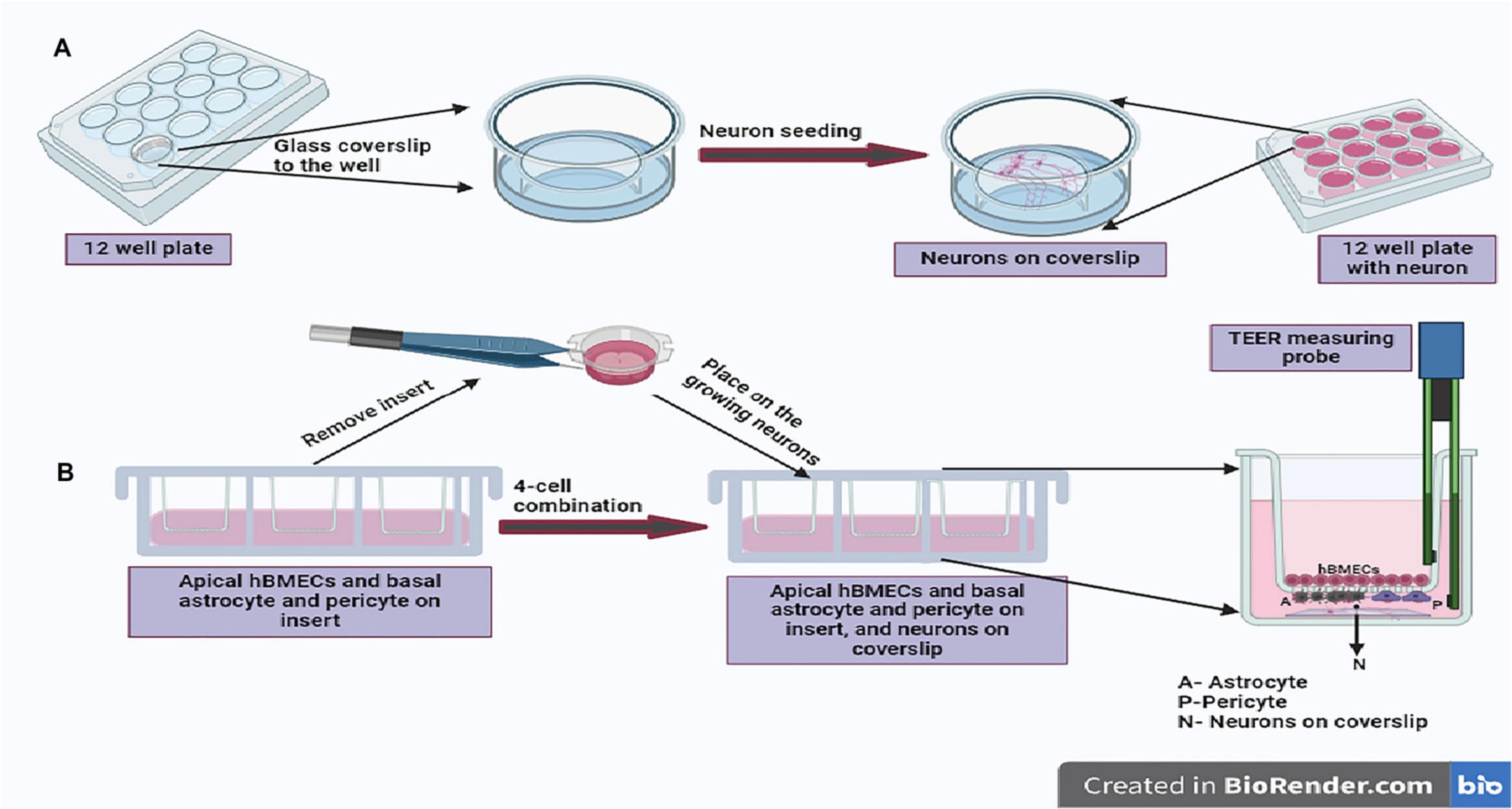
23.Incubate the neurons for 2 hr in a 37°C CO2 incubator and then add another 500-800 µl neuronal CM. Continue incubation, changing the medium every 48 hr.
Integration of all four cell types for an in vitro BBB model (Fig. 3B)
Astrocytes, pericytes, and hBMECs are grown together on one Transwell insert, while neurons are seeded in separate plates on glass coverslips; thus, to prepare an in vitro BBB reflecting the in vivo composition, the insert with the cells must be combined with the growing neurons. This is usually done on the 10th to 11th day after the commencement of the experiment (Fig. 1).
24.Remove the plate with the growing co-culture comprising astrocytes and pericytes in the basolateral part and hBMECs in the apical part of the insert membrane (step 21) from the incubator. Remove each Transwell insert, remove any excess medium from the insert by aspiration, and place the insert meticulously into a well of the 12-well plate containing neurons on the coverslip at the well bottom, being careful to orient it with hBMECs above and astrocytes/pericytes below and not to disturb the neurons at the bottom of the well growing on glass coverslips (step 23).
25.Replenish the apical side of the Transwell insert with 0.5 ml fresh endothelial CM, and fill the basolateral side of the Transwell with 1 ml of a mixture of astrocyte, pericyte, and neuronal CM in a 1:1:2 ratio.
Basic Protocol 3: ASSESSMENT OF MODEL FUNCTIONALITY
Any in vitro BBB model should not only have the cell composition of human BBB but also have similar functional properties. Though in vivo TEER values are high, a moderate TEER is expected in the in vitro model. Also, the permeability across the barrier should be tested to validate the integrity of the BBB model. Following are the methods and related steps that we applied in our BBB model.
Materials
-
70% (v/v) ethanol
-
Endothelial CM (see recipe)
-
Transwell inserts bearing BBB models (Basic Protocol 2)
-
Paraformaldehyde (Thermo Scientific, cat. no. J19943-K2)
-
Dulbecco's phosphate-buffered saline (DPBS; Gibco, cat. no. 14190144)
-
Goat serum (Abcam, cat. no. ab7481)
-
Tween-20 (Sigma, cat. no. P2287-100ML)
-
Bovine serum albumin (BSA)
-
Wash buffer (see recipe)
-
Appropriately conjugated (labeled) antibodies for claudin 5 (Invitrogen, cat. no. 362588) and ZO-1 (Invitrogen, cat. no. MA3-39100 A647)
-
Appropriately conjugated (labeled) antibodies for cell markers: anti-S1OOβ for astrocytes (Abcam, cat. no. ab196175), anti-CD146 (Abcam, cat. no. ab196448 for pericytes and hBMECs), and anti-NeuN antibody for neuron (Abcam, ab190565)
-
DAPI-(4′,6-diamidino-2-phenylindole) mounting dye (ProLong Diamond Antifade Mounting medium with DAPI, Invitrogen cat. no. P3692)
-
Dolutegravir (DTG; Adooq Bioscience)
-
DTG solution without DTG (see recipe)
-
EVOM2 for TEER measurement (World Precision Instruments [WPI])
-
Scalpel
-
Scissors
-
Beakers
-
Glass slides
-
Glass coverslips
-
Forceps
-
Measuring cylinders
-
Magnetic stirrer
-
Magnetic beads
-
Vortex
-
Confocal microscope (Zeiss LSM800 microscope)
-
Fiji ImageJ software
-
Liquid chromatography-tandem mass spectrometer (LC/MS/MS)
Trans-epithelial electrical resistance (TEER) measurements
The simpler and relevant indicator of barrier integrity can simply be assessed by measuring the TEER value of the developed BBB model. Though this is not an accurate parameter for measuring permeability across the barrier, a higher TEER value is achieved only when there is strong barrier formation. Extra care should be taken in performing this protocol to ensure accurate results.
1.Carefully wash the resistance measuring probe in 70% (v/v) ethanol, air dry, and then equilibrate in endothelial CM for 15 min inside the biosafety cabinet.
2.To achieve a stable TEER value, 10-15 min before initiation of TEER measurement, remove the plates with inserts from the CO2 incubator and place inside the biosafety cabinet.
3.After this equilibration is complete, gently position the probe into the Transwell insert well, with the shorter arm just above the hBMECs (apical) layer and the longer arm just above the neurons on the coverslip at the bottom of the well (Fig. 3B).
4.Monitor and note down the TEER values daily until the completion of the experiment.
Processing of the Transwell membrane
After completion of the experiment, the Transwell membrane can be used for various cell detection methods. For any such desired analysis, the cells on the membrane must be fixed to keep the cellular entities intact.
5.As the experiment concludes, wash the insert membrane with cells twice by dipping the insert into a beaker containing PBS. Then, the cells can be fixed by adding 0.5 ml of 4% paraformaldehyde to the membranes containing the cells for 10 min at room temperature.
6.Subsequently, wash the fixed membrane with DPBS, as mentioned in step 5 for washing, and delicately section the membrane using a fresh scalpel into four equal pieces for further staining. Allow the pieces to air dry and then place each on a glass slide, working carefully to avoid creating air bubbles between the membrane and the slide.
Staining cells on the membranes for imaging
The cells on the membrane can easily be stained with a desired antibody for analysis under a confocal microscope.
7.In a fresh 12-well plate, incubate a portion of the membrane containing cells on both sides in a blocking buffer containing 5% goat serum, 0.1% Triton X-100, and 1% BSA for 2 hr. Subsequently, wash the membrane sample twice in the well by adding and then removing 0.5 ml wash buffer (DPBS with 0.1% Tween-20).
8.Apply the conjugated antibodies to claudin 5 at a 1:200 dilution and antibodies to ZO-1 at a 1:100 dilution in wash buffer to the samples and incubate overnight at 4°C.
9.Using another piece of the same insert membrane, perform the same procedure (steps 7 and 8) for cell identification, but using conjugated cell marker antibodies: anti-S100β for astrocytes, anti-CD146 for pericytes and hBMECs, and anti-NeuN for neurons.
10.The following day, decant the primary antibody solution and wash the samples twice with 0.5 ml of wash buffer. After the washes, again fix the samples as mentioned in step 5 but this time in dark. This step fixes the antibodies bound to the samples’ already-fixed cellular proteins.
11.After fixation, wash the samples with PBS, air dry, and place them on a glass coverslip that has already a drop of DAPI added.
12.Add another drop of DAPI on top of the piece of membrane.
13.Finally, place another glass coverslip cautiously onto the DAPI-treated sample membrane with cells.
14.Use an LSM800 microscope (Zeiss LSM800) or another fluorescence microscope to image the expression of tight junction proteins (claudin 5 and Zo-1) and cellular marker proteins (S100β, CD146, and NeuN).
Measurement of fluorescence intensity
15.Assess the fluorescence pixel intensity of the confocal images to analyze tight junction proteins by importing the images into Fiji ImageJ software for image analysis (Schindelin et al., 2012).
16.In the import window, select the cells to be analyzed and to be compared by using the selection tool.
17.Select the visually brightest cell in each sample and determine its average intensity by aggregating the cell area. The software provides the mean intensity for the entire selected area.
18.Copy the intensity number or export the data from the opened window menu in an Excel sheet. The data export can be performed by right-clicking on the picture or from the drop-down menu.
19.The data can then be used to generate bar graphs or line graphs.
Analysis of drug penetration for functional proof of BBB model
Dolutegravir (DTG) is an integrase-strand transfer inhibitor widely employed in antiretroviral therapy (ART) for the treatment of human immunodeficiency virus (HIV) infection. DTG penetration or any known penetration/permeability assay should be employed as proof of concept to demonstrate the functionality of our BBB model. The drug analysis experiment is carried forward from step 4 of Basic Protocol 3, before ending the BBB experiment.
20.Prepare the designated medium (endothelial CM) containing 4000 ng/ml DTG by adding 4 µl of DTG solution to the endothelial CM.
21.Add 0.5 ml of drug-containing medium to the apical side only of the Transwell membrane. Incubate for 48 hrs at 37°C in a CO2 incubator.
22.After the completion of the incubation period, collect medium from the apical and basal compartments of the four-cell model.
23.Determine the concentration of DTG in the collected medium using a validated LC-MS/MS methodology (Dyavar et al., 2019; Fletcher et al., 2014; Podany et al., 2014)
REAGENTS AND SOLUTIONS
Bovine collagen I working solution
Working inside a biosafety cabinet, in a 50-ml tube, mix 99% acetic acid (density 1.048 g/cm3; cat. no. A113-50, Fisher Chemicals) into sterile water to make a 0.1 N acetic acid solution. Add 10 mg/ml bovine collagen I (Gibco, cat. no. A10644-01) to the tube to a final concentration of 3 µg/ml collagen I. Always prepare fresh.
Complete media (CM)
- Combine and mix in a 50-ml tube :
- 500 µl of appropriate 100× growth supplement (AG, PGS, EGS, or NGS, as applicable)
- 500 µl of 100× Pen/Strep
- 2.5 ml fetal bovine serum (FBS)
- Medium (AM, PM, ECM, or NM, as applicable) to 50 ml total
- Store up to 2 weeks at 4°C
Four appropriate types of CM need to be prepared, suitable for astrocytes, pericytes, hBMECs, and neurons; see Materials list of Basic Protocol 1 for details of the growth supplements and media needed.
Final composition: 2.5% (v/v) FBS, 1× growth supplement, and 1× Pen/Strep.
Dolutegravir (DTG) solution
- To prepare vehicle, combine and mix in a 50-ml tube :
- 4 ml dimethyl sulfoxide (DMSO; Sigma-Aldrich)
- 12.5 ml polyethene glycol 400 (PEG 400; Applichem)
- 7.5 ml propylene glycol (PG; Fisher Scientific)
- 5 ml of 98% (v/v) ethanol (Sigma-Aldrich)
- 3.5 ml kolliphor (Cremophor EL poloxamer 188, Sigma-Aldrich)
- 17.5 ml DPBS
- Store up to 6 months at −20°C
To prepare DTG solution (just before use), weigh out 4 mg DTG, add it to 4 ml of the vehicle solution, and vortex.
Wash buffer (1 L)
Add 1 ml Tween-20 to 1 L of 1× DPBS and mix well on a magnetic stirrer before use. Always prepare fresh.
COMMENTARY
Background Information
It is essential to understand the structural and functional aspects of the BBB to achieve successful CNS drug delivery. There has been considerable debate regarding the fact that relying on the rodent BBB models does not provide critical answers about the human BBB as there are significant differences between species related to transporter expression, the complexity of tight junctions, and drug receptors. Obtaining an in vitro humanized BBB model is a crucial experiment and methodology to understand drug transport across the BBB.
Because of the BBB, most CNS-targeted drugs have suboptimal concentrations in brain tissues, and thus CNS pathogenesis is challenging to treat. Though animal models can be used for neuronal studies, fundamental species differences must be taken into consideration. Thus, a more species-specific model for entry of different entities into the brain, is desired. The properties of the BBB vary between species, in part due to the distinct properties of the BBB-associated cells. Moreover, within any given individual, there are substantial differences between BBB-associated brain endothelial cells and capillary endothelial cells. The BBB endothelial cells exhibit extensive fenestration and enhanced intercellular barrier junctions showing pinocytotic function. When resources are limiting factors, a monolayer of brain endothelial cells can also be used as the most simplified BBB representative for studying the penetration of drugs/molecules. Pericytes are one of the most important cells for vascular development. However, in the brain, pericytes can communicate directly with microglial cells. Thus, any alteration in the properties of pericytes will not only cause a compromised BBB but also affect the brain's relay system. Astrocytes are unique to the brain and are an integral structural unit of the BBB, wherein astrocyte projections directly interact with the underlying neuron cells and preceding endothelial cells. Brain injury and a damaged BBB lead to the release of S100β protein, a cellular marker of astrocytes. As a part of BBB, these cells specify the unique features of the BBB to enable ionic homeostasis, optimal nutrition maintenance, and the filtration of harmful entities destined for the CNS. Despite providing an impeccable barrier, BBB regulates a restricted passage with passive diffusion of essential water-soluble nutrients across the BBB and the transporters on brain cells, helping provide other specific nutrients for brain tissues. Though very much necessary, the protective and less-than-semi-permeable nature of the BBB also hinders the effectiveness of several drugs targeted for CNS. For example, in brain cancer, based on the type of tumor, there is variability in the degree of BBB integrity around the tumor region, also known as the blood-tumor barrier (BTB). Thus, comprehensive study of the BBB is a prerequisite to identify BBB-penetrable drugs for maximum control of brain tumors. Although a leaky BBB is undesirable, to achieve the optimal concentration of a drug for a better treatment of neuronal pathogenesis, the integrity of the BBB can be modulated in a controlled way. The heterogeneous alteration in the BBB in brain tumors affects the permeability, bioavailability, and chemotherapeutic response of potential therapeutic chemo agents.
The method presented here is also cost-effective for screening a large number of drug candidates. Since the initial incorporation of endothelial cells onto a transparent collagen filter, Transwell technology has advanced significantly to make it more suitable for BBB studies. It is necessary to utilize primary human cells because nonhuman primary cells are not representative of their human counterpart and exhibit substantial differences in the properties of tight junction proteins. To completely recapitulate the BBB microenvironment with the associated paracellular stimuli, in vivo constituent cells of BBB should be used as primary cells in an in vitro model employing a Transwell membrane system for contact-based co-culture including neurons in a non-contact position (at the bottom of the well on the coverslip). Despite drawbacks related to its nature as a static model, the Transwell-insert-based human BBB model remains the most affordable and straightforward way to replicate conditions representing the BBB.
The in vitro BBB model described here can be used to investigate the penetration of molecules and explore the regulatory mechanisms and functional aspects of its constituent cells. This model is also suitable to study the regulation of transporter protein expression on individual cell types. Different efflux transporters, including ATP-binding cassette (ABC) proteins P-glycoprotein (Pgp), and breast cancer resistance protein (BCRP), are expressed by the component cells of the BBB. Pharmacologically, these efflux transporters play a significant role in regulating the concentration of molecules in the brain. Various studies, including those involving transporter knock-out animal models, have demonstrated the effects of these transporters on drug concentrations in the CNS. Reports indicate that several small-molecular drugs act as substrates for these transporter proteins and thus are barricaded by the BBB, reducing drug efficacy. As a means to evaluate the efficacy of any xenobiotic for brain pathogenesis, this model provides a simple, straightforward strategy for drug penetration analysis in which information can be gathered in a single experiment. Even if such an experiment is performed in triplicates, four drugs/molecules can be assessed for penetration across the BBB in a single plate. After the experiment, the collected sample size of 0.5 ml is also desirable, as it can be used in multiple downstream analytical assays. One can use mass spectrometry to quantify the level of drug passed through BBB, or the whole BBB plate can be subjected to live imaging if the drug/chemical agent is tagged with fluorescent dye. Such live imaging can reveal the real-time penetration of drugs across the BBB. At the same time, any alteration in BBB integrity can be monitored by analyzing the intactness of the tight junction proteins.
Critical Parameters
Using this protocol, we successfully developed an in vitro BBB model. As expected, working on the protocol revealed several steps that are critical for its success.
Intermittent visualization under a microscope is desired to confirm proper cell growth on the insert membrane; however, it is very difficult to observe the cells growing on the Transwell insert membrane. Some insert membranes are opaque, some are translucent, and some are relatively transparent. We tested polycarbonate- and polyester-based inserts and found that cell visibility under a microscope was better for cells growing on a polyester membrane (Fig. 4).
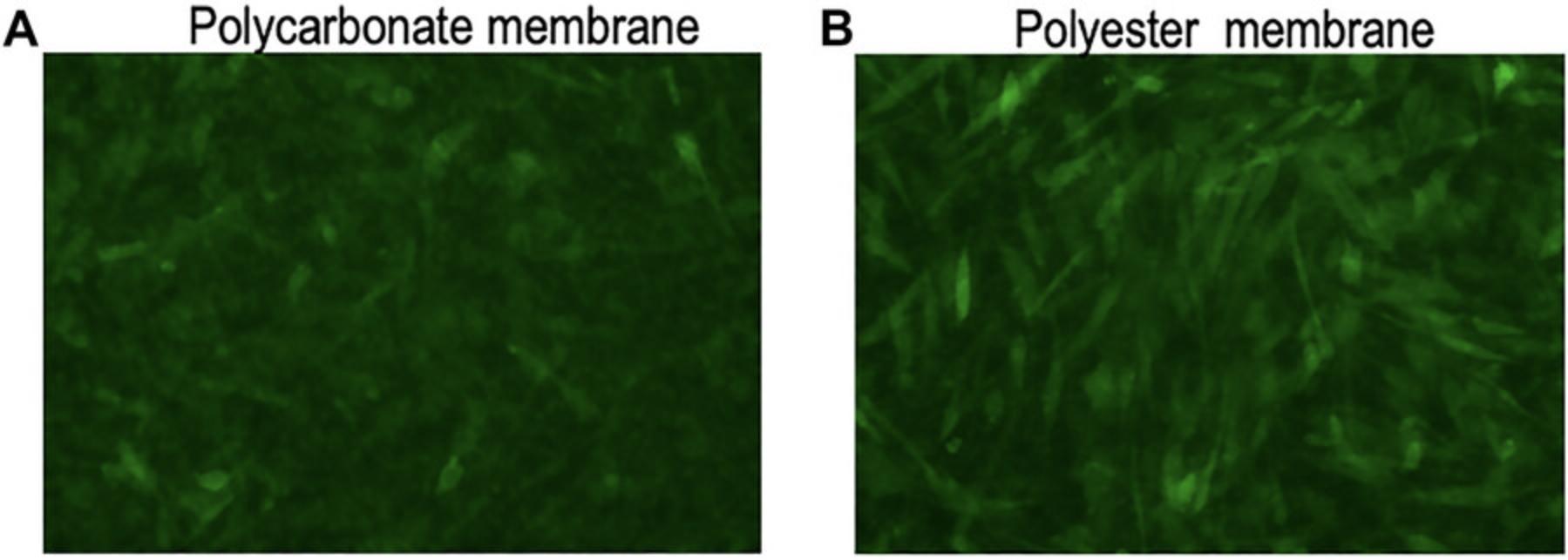
A critical feature of primary brain cells is their adherent nature. Thus, the optimal attachment of the cells to the flask or insert membrane highly depends on the coating strategy for the surfaces. Although fibronectin alone is sufficient for proper attachment and growth of cells in a culture flask, a better matrix is required for cells to grow on insert membranes. Because the developing BBB plates are not air-tight, handling and cell growth in the plates must be completely aseptic to avoid contamination. Though complete drying of the insert membrane is desired for astrocyte seeding, complete drying of the insert membrane before pericyte seeding can harm the membrane-attached astrocytes. Removing all the excess astrocyte medium from the insert membrane is sufficient for pericyte seeding. Neurons do not grow in suboptimal serum concentrations (<5%), and accordingly, care must be taken to keep the astrocyte/pericyte/neuron media ratio at 1:1:2 when combining all the cells together. After all cells are combined during BBB model development, minimal handling is desirable because this can be expected to allow uninterrupted cell communications and cell contact with each other. During TEER measurement, extra care is needed to avoid touching the apical hBMECs and basal neuron cells. Too much growth of cells on the membrane is not desirable as it can lead to overlapping cells and non-monolayer growth. This particularly affects the post-experiment processing and imaging of the insert membrane.
Troubleshooting
Important problems and related solutions are summarized in the table (Table 1) and also noted in the relevant sections.
Problem | Possible cause | Solution |
---|---|---|
Cells not attaching to the flask/insert membrane | Improper coating or degradation of coating reagents | Store the coating reagents at supplier-specified temperatures, and do not use old coated flask/inserts (≥2 weeks old). |
Non-uniform monolayer of cells | Cells not thoroughly dispersed | Use gentle pipetting to ensure a single-cell dispersion. Observe 10 µl of the cell suspension on a glass slide under a microscope to assess the dispersion; pipet more if the desired dispersion was not achieved. |
Loss of membrane integrity | Direct contact of the insert membrane with the pipette tip | While holding the insert with forceps, gently aspirate the excess medium from on top of the glass lining using a pipet. |
Cells at either the center or the periphery of the insert membrane | More or less than the optimal volume of cell medium was applied when seeding on top of membrane | Make sure that the optimal volume of cell media is applied during the cell seeding procedure. The drop of cell medium on the inverted insert membrane touches the bottom well, thus causing even spreading across the insert membrane. |
Inconsistency of TEER value | Higher temperature of the media and cells causes fluctuation in TEER values | The plate with the inserts should be placed in the biosafety cabinet for 10-15 min before TEER reading. |
The apical and basolateral compartment show the same amounts of permeability testing agents, which are known to be less permeable across the BBB | Overflowing or mixing of apical medium with basolateral medium while shifting from biosafety cabinet to CO2 incubator or vice versa | Use only 500 µl of medium in the apical region and 1 ml in the basal part of the insert when ready for the permeability-based assays. |
Understanding the Results
BBB model validation by TEER measurement
TEER value indicates the integrity of BBB model. We optimized several combinations, including plate type, coating reagents, cell types, and cell numbers, to provide a better and more stable TEER value. Based on our results (Figs. 5 and 6), we recommend using polyester Transwell inserts coated with collagen I, fibronectin, and poly-L-lysine (as described above) and all four different cell types, namely astrocytes, pericytes, endothelial cells, and neuron cells. This combination exhibited the best stable TEER value of ∼150 ohm/cm2.
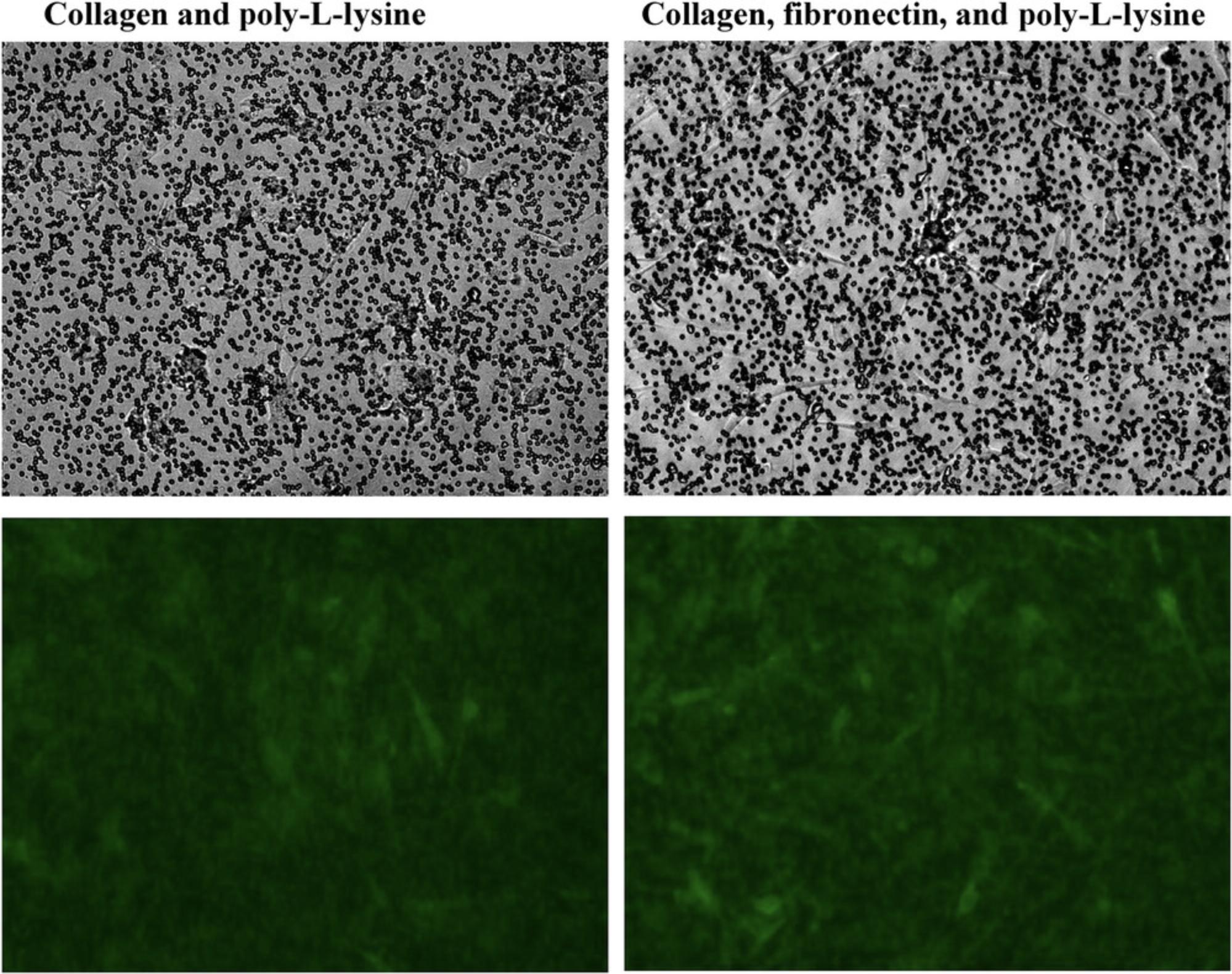
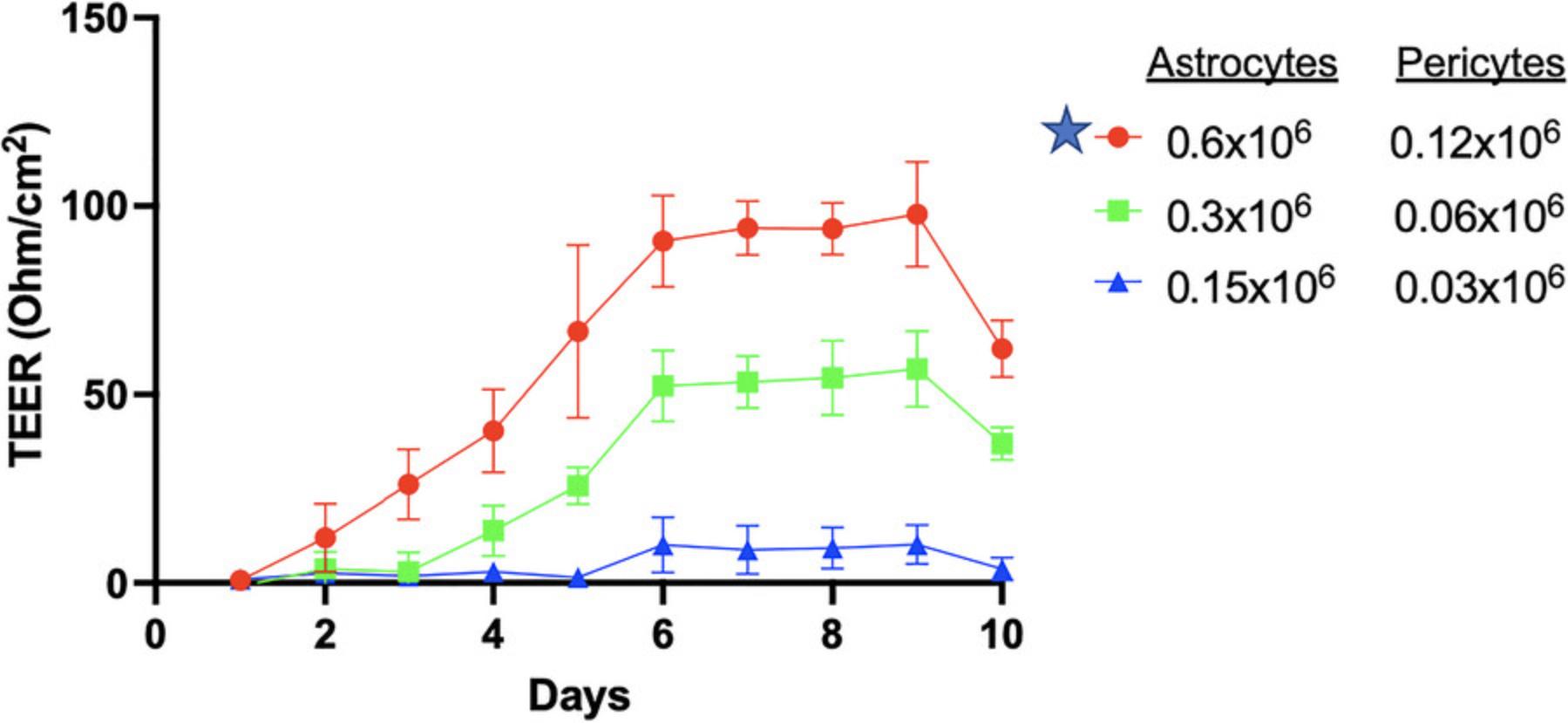
Including zinc in the media further improved the TEER value (Fig. 7). The zinc supplementation, added as ZnSO4, leads to a higher TEER value because of its effect on enhanced barrier protein expression. As depicted in Figure 7, zinc moderately increased the TEER to a maximum of 230 ohm/cm2 (day 10) compared to that seen for untreated controls (155 ohm/cm2) and serum-deprived conditions (≤80 ohm/cm2). Combining neurons with hBMECs, astrocytes, and pericytes significantly enhanced TEER, demonstrating the higher integrity of the four-cell versus the three cell BBB model (Fig. 7).
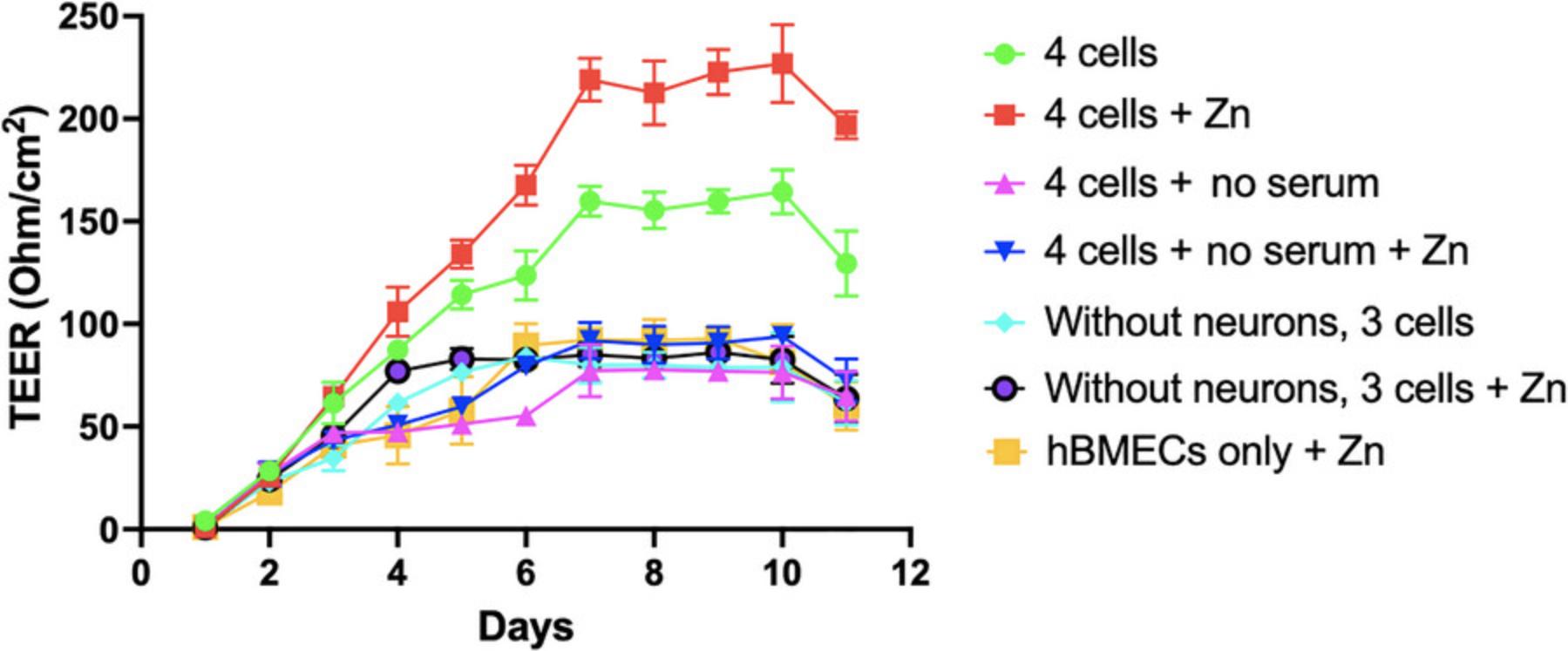
BBB validation by fluorescence microscopy
A functional and well-established BBB model should exhibit high expression of tight junction proteins claudin 5 and ZO-1. The presence of claudin 5 and ZO-1 on co-cultured astrocytes and pericytes at the basolateral portion of the polyester Transwell membrane can be assessed through immunostaining and laser-scanning microscopy (Fig. 8A). In our experiments, astrocytes, pericyte co-culture (Fig. 8A), and hBMECs (Fig. 8C) all exhibited basal-level expression of claudin 5 and ZO-1 tight junctions. However, addition of zinc to the medium for both astrocytes:pericyte co-culture (Fig. 8B) and hBMECs (Fig. 8C) resulted in increased expression of claudin 5 and ZO-1. Furthermore, there was a limited expression of barrier proteins on the three cell BBBs, with astrocytes, pericytes and hBMECs showing diminished presentation of claudin 5 (Fig. 9A and C) compared to the that in four-cell model depicted in (Fig. 8). Notably, introduction of zinc did not improve claudin 5 expression in the absence of neurons (Fig. 9B and D).
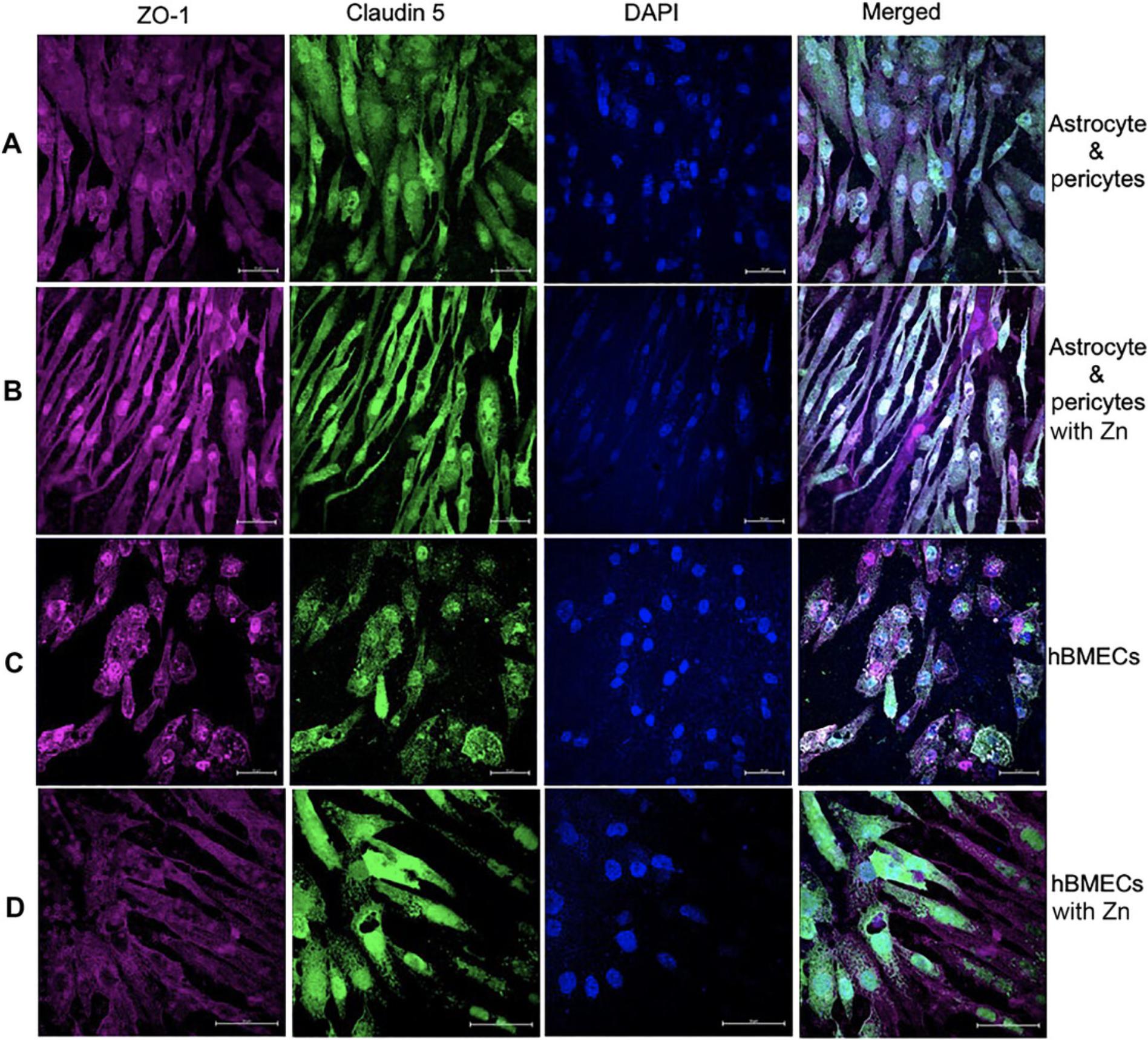
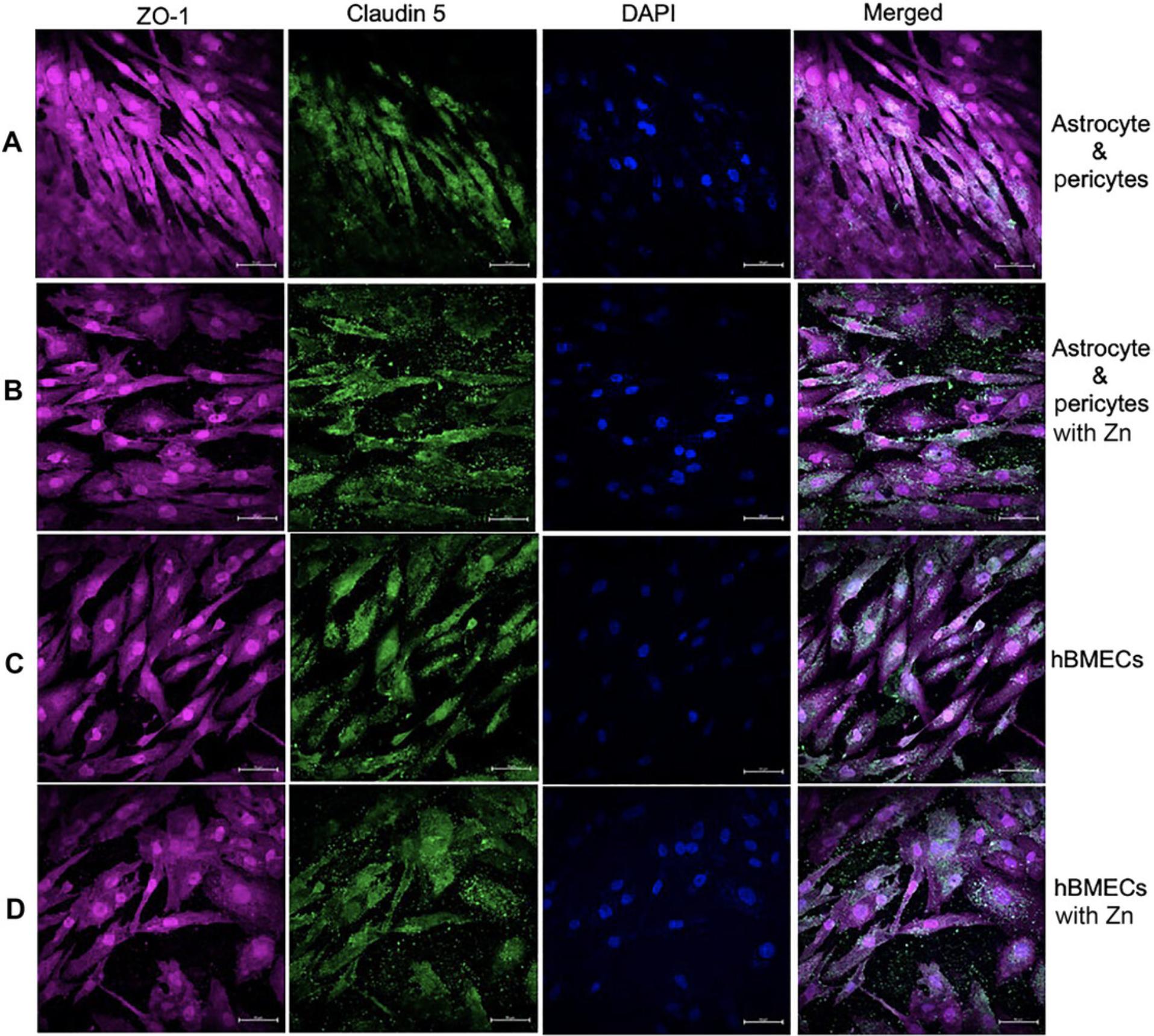
Anti-ZO-1 and anti-claudin 5 antibodies are used to assess the expression of these proteins on the hBMECs monolayer on the Transwell membrane, revealing that hBMECs generate tight junction proteins, but their expression is suboptimal when probed for ZO-1 and claudin 5 tight junction protein (Fig. 10A). Similarly, neuronal cells can be processed for detection of tight junction protein expression and other protein as illustrated in Figure 10B.
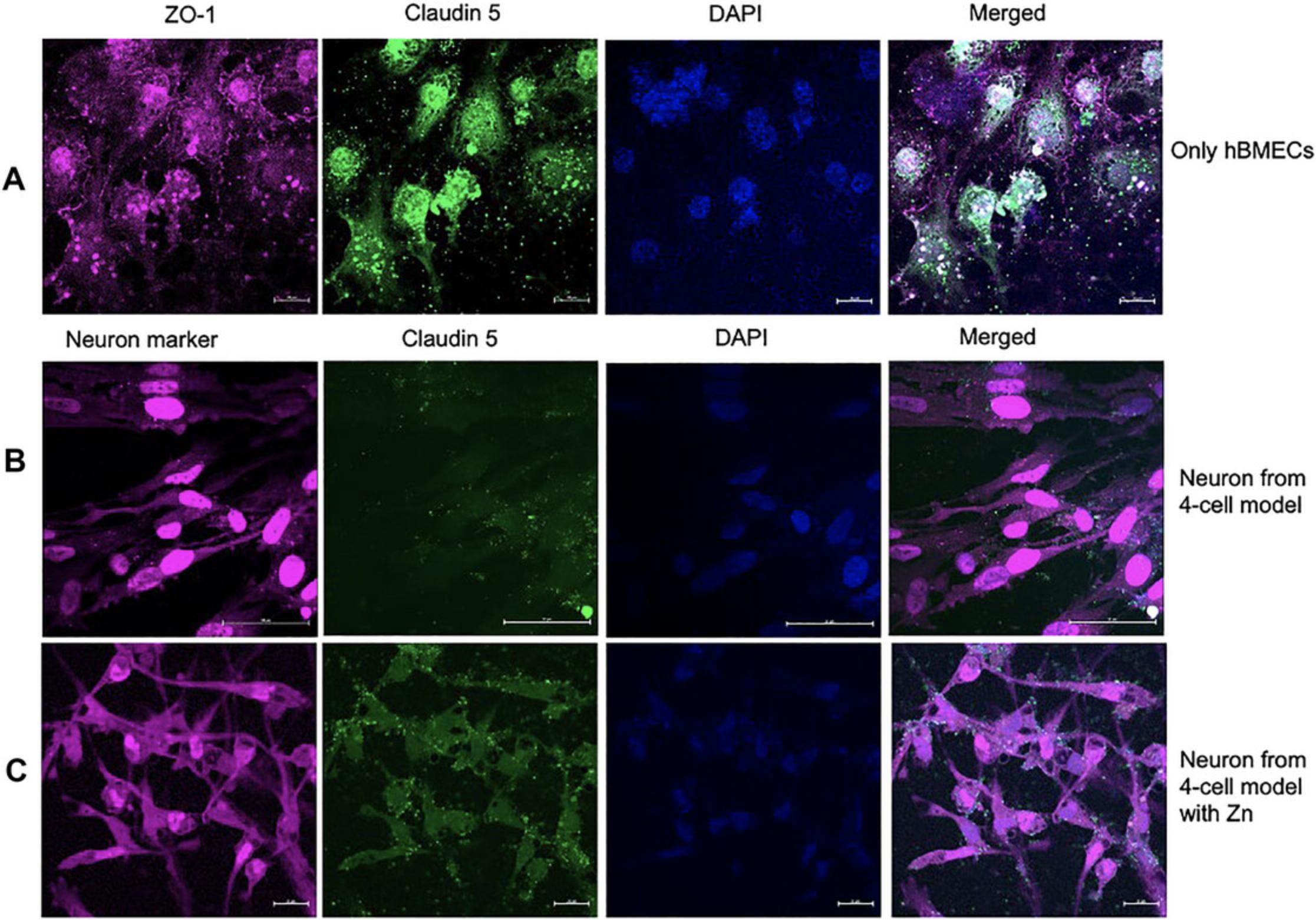
Cellular markers indicative of astrocytes (S100B), pericytes, and hBMECs (CD146) expressed on the cell surface should be validated using specific antibodies to ensure cell compositions. We detected astrocytes expressing S100B using an Alexa-647-conjugated antibody (red; Fig. 11A). Pericytes are seen in green due to staining using an Alexa-488-conjugated anti-CD146 antibody. Cellular integrity for the BBB model can be confirmed by probing for CD146 expression on hBMECs on the opposite side of the same membrane (Fig. 11B) using Alexa-488-conjugated anti-CD146 antibody.
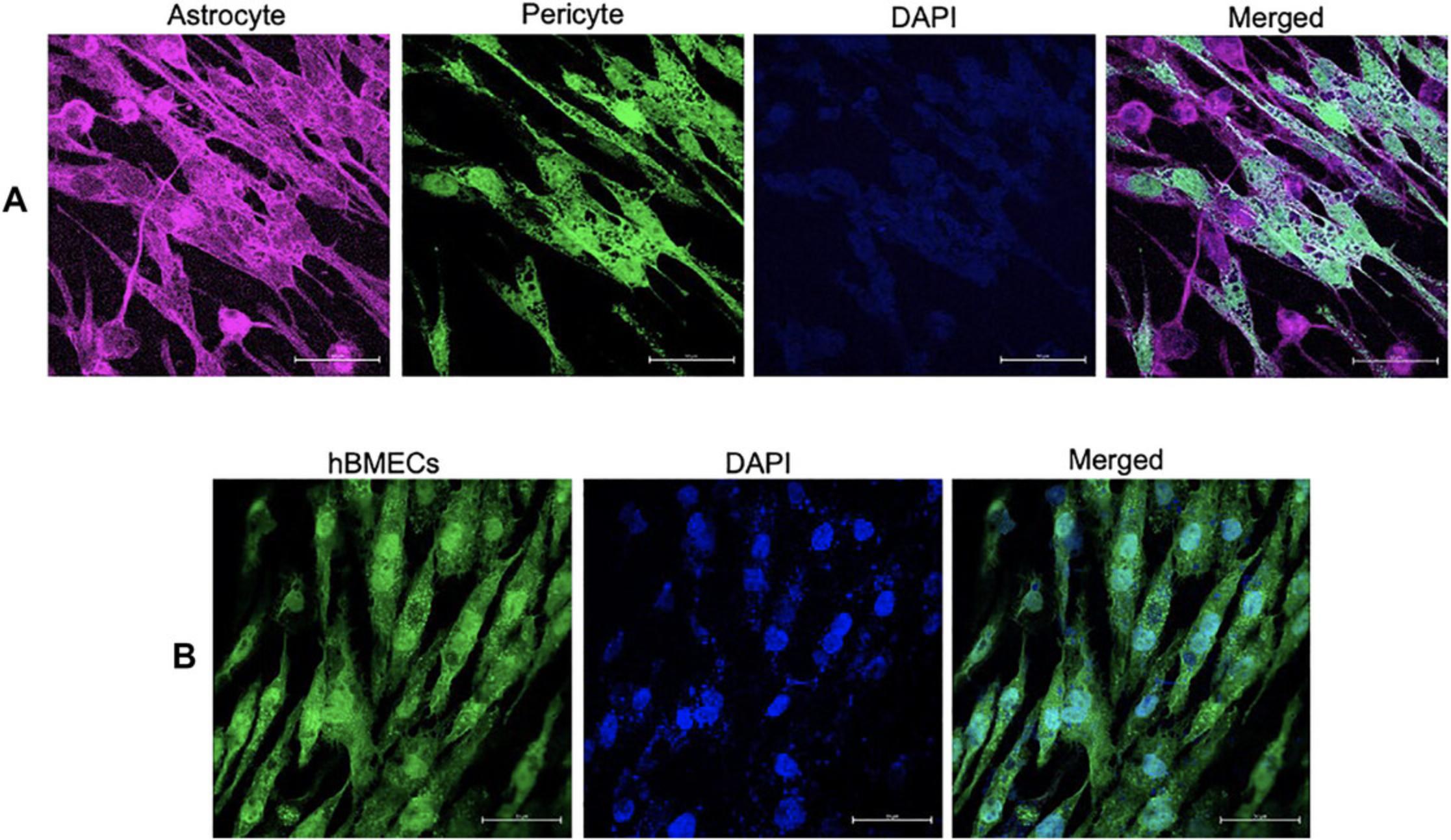
BBB tight junction protein analysis based on fluorescence intensity
A visible and distinct difference is expected between samples for protein detection. However, fluorescence intensity analysis provides a better understanding and quantification of the data. It is desirable to perform fluorescence intensity analysis of the claudin 5 protein or any other tight junction proteins in each represented cell within various combinations of the BBB representation. As depicted in Figure 12A, in the presence of zinc in the media, there was a 33% increase in claudin 5 intensity in astrocytes:pericytes and a 43% increase in hBMECs. Similarly, the analysis pointed to a more than 50% increase in claudin 5 intensity in astrocytes:pericytes from the four-cell model in the absence of serum. No significant differences in intensity were observed in other BBB representations. Neuronal cells from the four-cell model exhibited an intensity of 10, but the inclusion of zinc in the media elevated the intensity to 19.
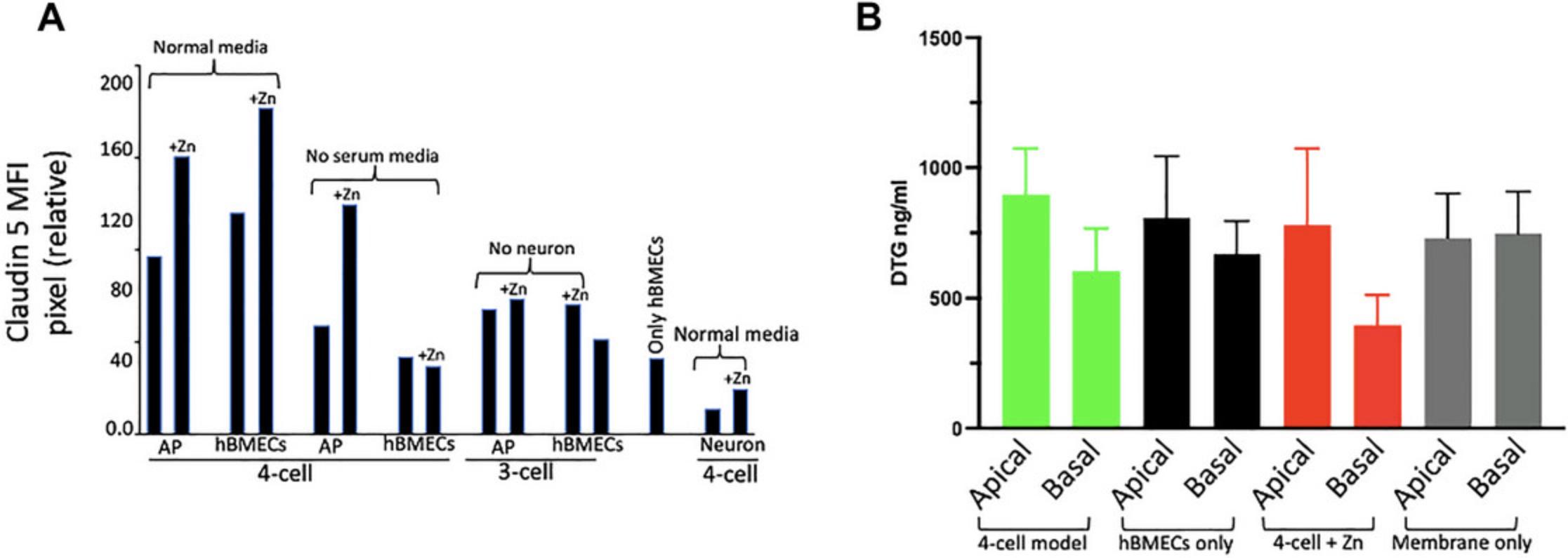
Assessment of functionality testing: Penetration of DTG
The functionality of the established BBB model can be assessed by examining the penetration of any known xenobiotic in comparison to what is already known or seen clinically. DTG, a drug used in treating HIV, shows low penetration into the CSF (<1%) (Gelé et al., 2019; Letendre et al., 2014). In our BBB model, we observed approximately 33% variance in DTG distribution across the Transwell membrane of the four-cell model (Fig. 12B). Additionally, we observed a roughly 17% reduction in drug penetration when the Transwell membrane featured only an hBMEC monolayer, without astrocyte, pericytes, and neurons. The data also lends support to the notion that the addition of zinc to the four-cell model enhances tight junction formation, as evidenced by a nearly 48% decrease in DTG penetration through the barrier (comparing apical and basal drug concentration).
Time Considerations
The timing should be carefully planned beforehand because once the experiment commences, with the seeding of astrocytes on the insert membrane, there are no options to stop or pause the experiment. A total of 20-21 days is required to develop the BBB model, and an additional 2-3 days are needed to complete the BBB-based experiments (i.e., drug administration, etc.). After the experiment, after being fixed with paraformaldehyde, insert membranes from the BBB model can be stored at 40°C for up to 2 months. Stepwise timing for the complete in vitro model are outlined in Figure 1.
Acknowledgments
We acknowledge support from the following grants from the U.S. National Institutes of Health: K23 MH125734 (to S.N.A.) and 1U01 DA058527 (to C.V.F. and S.N.A.). The content is solely the responsibility of the authors and does not necessarily represent the official views of the National Institutes of Health.
Author Contributions
Johid R. Malik : Formal analysis; investigation; methodology; validation; writing—original draft; writing—review and editing. Ukamaka O. Modebelu : Data curation; formal analysis; investigation; methodology; validation; writing—original draft; writing—review and editing. Courtney V. Fletcher : Investigation; methodology; project administration; supervision; writing—review and editing. Anthony T. Podany : Methodology; supervision; writing—review and editing. Kimberly K. Scarsi : Investigation; methodology; visualization; writing—review and editing. Siddappa N. Byrareddy : Investigation; methodology; visualization; writing—review and editing. Robbyn K Anand : Methodology; validation; writing—review and editing. Shilpa Buch : Investigation; methodology; writing—review and editing. Susmia Sil : Conceptualization; visualization; writing—review and editing. Jennifer Le : Writing—review and editing. John Bradley : Writing—review and editing. Ashley N. Brown : Methodology; writing—review and editing. Debapriya Sutar : Formal analysis; investigation; methodology; writing—review and editing. Sean N. Avedissian : funding acquisition; project administration; supervision; writing—review and editing.
Conflict of Interest
Authors have no conflicts to declare.
Open Research
Data Availability Statement
For this article, data sharing is not applicable because no data were generated for this protocol study. Instead, we have included the published data after obtaining the required permission from the publishing house.
Literature Cited
- Allen, C. L., & Bayraktutan, U. (2009). Antioxidants attenuate hyperglycaemia-mediated brain endothelial cell dysfunction and blood-brain barrier hyperpermeability. Diabetes, Obesity & Metabolism, 11(5), 480–490. https://doi.org/10.1111/j.1463-1326.2008.00987.x
- Appelt-Menzel, A., Cubukova, A., Günther, K., Edenhofer, F., Piontek, J., Krause, G., Stüber, T., Walles, H., Neuhaus, W., & Metzger, M. (2017). Establishment of a human blood-brain barrier co-culture model mimicking the neurovascular unit using induced pluri- and multipotent stem cells. Stem Cell Reports , 8(4), 894–906. https://doi.org/10.1016/j.stemcr.2017.02.021
- Bagchi, S., Chhibber, T., Lahooti, B., Verma, A., Borse, V., & Jayant, R. D. (2019). In-vitro blood-brain barrier models for drug screening and permeation studies: An overview. Drug Design, Development and Therapy , 13, 3591–3605. https://doi.org/10.2147/DDDT.S218708
- Banks, W. A., Kovac, A., & Morofuji, Y. (2018). Neurovascular unit crosstalk: Pericytes and astrocytes modify cytokine secretion patterns of brain endothelial cells. Journal of Cerebral Blood Flow and Metabolism , 38(6), 1104–1118. https://doi.org/10.1177/0271678X17740793
- Cecchelli, R., Berezowski, V., Lundquist, S., Culot, M., Renftel, M., Dehouck, M. P., & Fenart, L. (2007). Modelling of the blood-brain barrier in drug discovery and development. Nat Rev Drug Discovery , 6(8), 650–661. https://doi.org/10.1038/nrd2368
- Dyavar, S. R., Gautam, N., Podany, A. T., Winchester, L. C., Weinhold, J. A., Mykris, T. M., Campbell, K. M., Alnouti, Y., & Fletcher, C. V. (2019). Assessing the lymphoid tissue bioavailability of antiretrovirals in human primary lymphoid endothelial cells and in mice. Journal of Antimicrobial Chemotherapy , 74(10), 2974–2978. https://doi.org/10.1093/jac/dkz273
- Fletcher, C. V., Staskus, K., Wietgrefe, S. W., Rothenberger, M., Reilly, C., Chipman, J. G., Beilman, G. J., Khoruts, A., Thorkelson, A., Schmidt, T. E., Anderson, J., Perkey, K., Stevenson, M., Perelson, A. S., Douek, D. C., Haase, A. T., & Schacker, T. W. (2014). Persistent HIV-1 replication is associated with lower antiretroviral drug concentrations in lymphatic tissues. Proceedings of the National Academy of Sciences of the United States of America , 111(6), 2307–2312. https://doi.org/10.1073/pnas.1318249111
- Gelé, T., Furlan, V., Taburet, A. M., Pallier, C., Becker, P. H., Goujard, C., Gasnault, J., Barrail-Tran, A., & Chéret, A. (2019). Dolutegravir cerebrospinal fluid diffusion in HIV-1-infected patients with central nervous system impairment. Open Forum Infectious Diseases , 6(6), ofz174. https://doi.org/10.1093/ofid/ofz174
- Ghaffarian, R., & Muro, S. (2013). Models and methods to evaluate transport of drug delivery systems across cellular barriers. Journal of Visualized Experiments: JoVE , (80), e50638. https://doi.org/10.3791/50638
- Hajal, C., Offeddu, G. S., Shin, Y., Zhang, S., Morozova, O., Hickman, D., Knutson, C. G., & Kamm, R. D. (2022). Engineered human blood-brain barrier microfluidic model for vascular permeability analyses. Nature Protocols , 17(1), 95–128. https://doi.org/10.1038/s41596-021-00635-w
- Hind, W. H., Tufarelli, C., Neophytou, M., Anderson, S. I., England, T. J., & O'Sullivan, S. E. (2015). Endocannabinoids modulate human blood-brain barrier permeability in vitro. British Journal of Pharmacology , 172(12), 3015–3027. https://doi.org/10.1111/bph.13106
- Letendre, S. L., Mills, A. M., Tashima, K. T., Thomas, D. A., Min, S. S., Chen, S., Song, I. H., Piscitelli, S. C., & extended ING116070 study team. (2014). ING116070: A study of the pharmacokinetics and antiviral activity of dolutegravir in cerebrospinal fluid in HIV-1-infected, antiretroviral therapy-naive subjects. Clinical Infectious Diseases , 59(7), 1032–1037. https://doi.org/10.1093/cid/ciu477
- Lippmann, E. S., Azarin, S. M., Kay, J. E., Nessler, R. A., Wilson, H. K., Al-Ahmad, A., Palecek, S. P., & Shusta, E. V. (2012). Derivation of blood-brain barrier endothelial cells from human pluripotent stem cells. Nature Biotechnology , 30(8), 783–791. https://doi.org/10.1038/nbt.2247
- Liu, W. Y., Wang, Z. B., Zhang, L. C., Wei, X., & Li, L. (2012). Tight junction in blood-brain barrier: An overview of structure, regulation, and regulator substances. CNS Neuroscience & Therapeutics, 18(8), 609–615. https://doi.org/10.1111/j.1755-5949.2012.00340.x
- Paradis, A., Leblanc, D., & Dumais, N. (2016). Optimization of an in vitro human blood-brain barrier model: Application to blood monocyte transmigration assays. MethodsX , 3, 25–34. https://doi.org/10.1016/j.mex.2015.11.009
- Podany, A. T., Winchester, L. C., Robbins, B. L., & Fletcher, C. V. (2014). Quantification of cell-associated atazanavir, darunavir, lopinavir, ritonavir, and efavirenz concentrations in human mononuclear cell extracts. Antimicrobial Agents and Chemotherapy , 58(5), 2866–2870. https://doi.org/10.1128/AAC.02551-13
- Rist, R. J., Romero, I. A., Chan, M. W., Couraud, P. O., Roux, F., & Abbott, N. J. (1997). F-actin cytoskeleton and sucrose permeability of immortalised rat brain microvascular endothelial cell monolayers: Effects of cyclic AMP and astrocytic factors. Brain Research , 768(1-2), 10–18. https://doi.org/10.1016/s0006-8993(97)00586-6
- Sano, Y., Shimizu, F., Abe, M., Maeda, T., Kashiwamura, Y., Ohtsuki, S., Terasaki, T., Obinata, M., Kajiwara, K., Fujii, M., Suzuki, M., & Kanda, T. (2010). Establishment of a new conditionally immortalized human brain microvascular endothelial cell line retaining an in vivo blood-brain barrier function. Journal of Cellular Physiology , 225(2), 519–528. https://doi.org/10.1002/jcp.22232
- Santaguida, S., Janigro, D., Hossain, M., Oby, E., Rapp, E., & Cucullo, L. (2006). Side by side comparison between dynamic versus static models of blood-brain barrier in vitro: A permeability study. Brain Research , 1109(1), 1–13. https://doi.org/10.1016/j.brainres.2006.06.027
- Schindelin, J., Arganda-Carreras, I., Frise, E., Kaynig, V., Longair, M., Pietzsch, T., Preibisch, S., Rueden, C., Saalfeld, S., Schmid, B., Tinevez, J. Y., White, D. J., Hartenstein, V., Eliceiri, K., Tomancak, P., & Cardona, A. (2012). Fiji: An open-source platform for biological-image analysis. Nature Methods , 9(7), 676–682. https://doi.org/10.1038/nmeth.2019
- Shah, B., & Dong, X. (2022). Current status of in vitro models of the blood-brain barrier. Current Drug Delivery , 19(10), 1034–1046. https://doi.org/10.2174/1567201819666220303102614
- Stone, N. L., England, T. J., & O'Sullivan, S. E. (2019). A novel transwell blood brain barrier model using primary human cells. Frontiers in Cellular Neuroscience , 13, 230. https://doi.org/10.3389/fncel.2019.00230
- Animal Welfare Information Center. Animal Use Alternatives. National Agricultural Library, U.S. Department of Agriculture. https://www.nal.usda.gov/animal-health-and-welfare/animal-use-alternatives
- Wang, Y., Wang, N., Cai, B., Wang, G. Y., Li, J., & Piao, X. X. (2015). In vitro model of the blood-brain barrier established by co-culture of primary cerebral microvascular endothelial and astrocyte cells. Neural Regeneration Research , 10(12), 2011–2017. https://doi.org/10.4103/1673-5374.172320
- Watanabe, T., Dohgu, S., Takata, F., Nishioku, T., Nakashima, A., Futagami, K., Yamauchi, A., & Kataoka, Y. (2013). Paracellular barrier and tight junction protein expression in the immortalized brain endothelial cell lines bEND.3, bEND.5 and mouse brain endothelial cell 4. Biological & Pharmaceutical Bulletin, 36(3), 492–495. https://doi.org/10.1248/bpb.b12-00915
- Weksler, B. B., Subileau, E. A., Perrière, N., Charneau, P., Holloway, K., Leveque, M., Tricoire-Leignel, H., Nicotra, A., Bourdoulous, S., Turowski, P., Male, D. K., Roux, F., Greenwood, J., Romero, I. A., & Couraud, P. O. (2005). Blood-brain barrier-specific properties of a human adult brain endothelial cell line. FASEB Journal , 19(13), 1872–1874. https://doi.org/10.1096/fj.04-3458fje