Plant Sample Collection and Shipment for Multi-omic Analyses and Phytosanitary Evaluation
Saleh Alseekh, Saleh Alseekh, Alisdair R. Fernie, Alisdair R. Fernie, Esra Karakas, Esra Karakas, Paola Ferrante, Paola Ferrante, Roland Schafleitner, Roland Schafleitner, Giovanni Giuliano, Giovanni Giuliano
Abstract
Plant sample preparation for analyses is a fundamental step in high-throughput omics strategies. Especially for plant metabolomics, quenching of hydrolytic enzymes able to affect metabolite concentrations is crucial for the accuracy of results. Given that DNA is usually less labile than metabolites, most sampling and shipment procedures able to preserve the metabolome are also suitable for preventing the degradation of plant DNA or of DNA of pathogens in the plant tissue. In this article, we describe all the steps of sample collection, shipment (including the phytosanitary issues of moving plant samples), and processing for combined genomics and metabolomics from a single sample, as well as the protocols used in our laboratories for downstream approaches for crop plants, allowing collection of multi-omic datasets in large experimental setups. The protocols have been adjusted to apply to both freeze-dried and fresh-frozen material to allow the processing of crop plant samples that will require long-distance transport. © 2023 The Authors. Current Protocols published by Wiley Periodicals LLC.
Basic Protocol 1 : Preparation of freeze-dried leaf disks for multiplexed PCR or DArT-Seq genotyping
Basic Protocol 2 : Medium-throughput preparation of pathogen-free nucleic acids for most genotyping-resequencing applications or pathogen detection
Alternate Protocol : Low-throughput extraction of high-quality DNA for resequencing using commercial kits
Support Protocol : DNA quality control
Basic Protocol 3 : Preparation of freeze-dried plant material for metabolomics
Basic Protocol 4 : Preparation of fresh-frozen plant material for metabolomics
Basic Protocol 5 : Preparation and shipment of metabolite extracts for metabolomic analyses
Basic Protocol 6 : Sample shipping and long-term storage
INTRODUCTION
In plant breeding, hundreds to tens of thousands of tissue samples are generated and analyzed by omics technologies (Holtorf et al., 2002; Schauer & Fernie, 2006; Sumner et al., 2003). The combination of genomic, phenomic/metabolomic, and disease resistance datasets collected from large diversity panels or segregating populations in multi-location trials provides unprecedented power for the identification of novel genomic regions/quantitative trait loci (QTLs)/candidate genes controlling important agronomic, disease resistance, or metabolic traits (Cao et al., 2022; Demirjian et al., 2023; Fang & Luo, 2019; Korte & Farlow, 2013; McLeod et al., 2023; Sulli et al., 2021).
Both plant pathogens and plant metabolites display remarkable diversity, with tens of thousands of pathogenic bacteria, fungi, and viruses and over one million distinct chemical structures known (Wurtzel & Kutchan, 2016).
Often, plant samples must be shipped to specialized laboratories that are well equipped for conducting genomic and metabolomic analyses, which may require cross-country shipment of plant samples. Countries have different regulations for shipping plant products such as plant samples. In general, for the import of an unprocessed plant product, a phytosanitary certificate issued by the National Plant Protection Organization of the exporting country is required. Grinding, freezing, and drying are generally not considered as processing, and samples treated with agents able to inactivate pathogen(s) (e.g., heat, detergents, or chaotropic agents) are generally not considered as a plant product and generally do not require a phytosanitary certificate. However, samples for measuring labile metabolites or proteins, where pathogen-inactivating treatments cannot be applied, may require a phytosanitary certificate for import to the destination country. In the European Union, laboratories can request exemptions from the requirement for phytosanitary certificates for performing analyses from the national authorities, facilitating shipment of plant samples to such laboratories.
Plant DNA barcoding is currently a widely used tool for rapid and accurate identification of plant species. Proper classification of plant material in a genebank is an indispensable activity because the worldwide distribution of misclassified material could have very detrimental effects on research programs. DNA barcoding relies on the presence of sequence variation in the genome (barcode) flanked by conserved sequences based on which primers are designed (Fig. 1). In contrast to the animal kingdom, in which mitochondrial cytochrome c oxidase 1 (CO1) was identified as a universal barcode (Hebert et al., 2003), in plants, it was not possible to identify loci that work across all species due to inadequate variation in the target loci and/or low PCR efficiency due to sequence variation in the primer-binding region (Chase & Fay, 2009; Chen et al., 2010). Furthermore, in the plant kingdom, deletion of genes is an important factor that hampers the use of unique universal barcodes: for example, algae do not have the matK locus containing a plastidial gene encoding an organelle maturase able to splice group II introns that is frequently used for barcoding in vascular plants because it is one of the most variable coding genes (Hilu et al., 2003). In the last few years, a different approach has been followed, consisting of selecting specific barcodes for each plant group that are able to discriminate across species in a given group (Barchi et al., 2019; Peyraud et al., 2017).
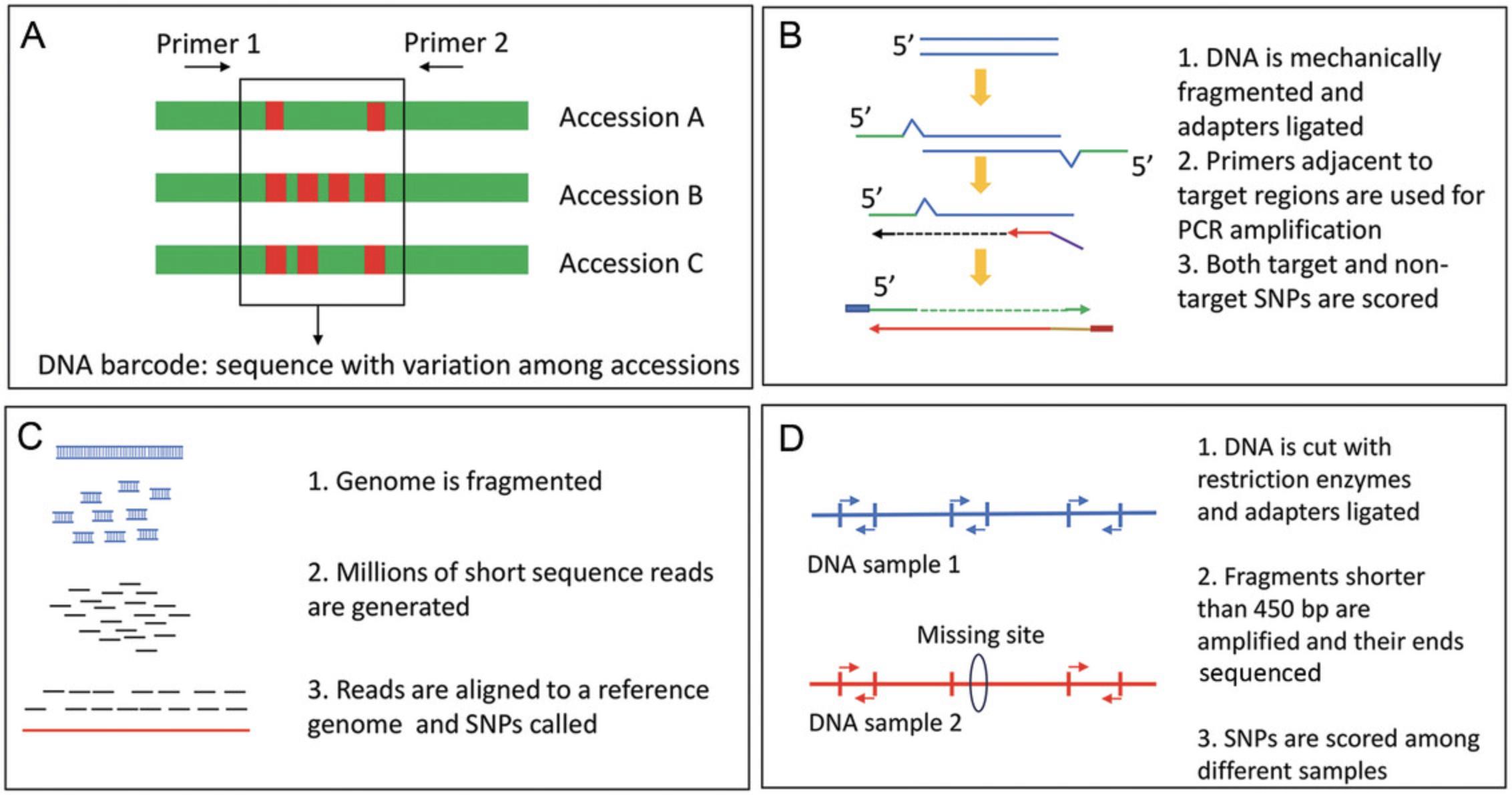
Metabolomics is an approach of study that involves the comprehensive analysis of small molecules, known as metabolites, present in biological samples. It provides insights into the metabolic processes occurring in an organism or system (Fiehn, 2002). Metabolomics can help in identifying and quantifying essential vitamins (e.g., vitamin C, B vitamins) and antioxidants (e.g., flavonoids, polyphenols), which play crucial roles in the protection of cells from oxidative damage and are associated with various health benefits (Delfin et al., 2019). There are also anti-nutritional/toxic substances other than health-promoting compounds. Consuming materials containing high levels of anti-nutritional or toxic substances can lead to food poisoning or adverse health effects. Crop wild relatives (CWRs) and wild food plants (WFPs) are the plant species closely related to cultivated crops that serve as a genetic resource for improving crop resilience, yield, and nutritional content (Bohra et al., 2022). In some cases, CWRs and WFPs can lead to food poisoning (Smith et al., 2008). Metabolomics data assess the potential risks associated with varieties or species and can guide decisions on at which certain level or under what conditions these can be consumed.
For metabolomics approaches, sample preparation, acquisition of data using analytical chemical methods, and data mining have immense importance. Variability among samples can emerge from various origins, encompassing both inherent biological diversity and factors associated with sample collection and storage (Gibon & Rolin, 2012). Sample preparation is a critical step for the isolated compounds and the accuracy of results.
In this article, we describe approaches and protocols for the collection and shipment of samples for genomic and metabolomic analyses for genome-wise association study (GWAS)/QTL analyses on large collections (Figs. 1 and 2), as well as a protocol for complying with phytosanitary regulations for shipping plant samples. Basic Protocols 1 and 2 allow the application of genomic approaches, including both non-targeted [whole-genome resequencing, genotyping by sequencing, Diversity Arrays Technology sequencing (DArT-Seq)] and targeted [multiplexed PCR, single primer enrichment technology (SPET)] ones (Table 1). In addition to that, the Alternate and Support Protocols focus on the extraction of high-quality DNA and DNA quality control (QC). Furthermore, Basic Protocols 3 and 4 describe the preparation of freeze-dried and fresh-frozen plant material for metabolomics approaches, and Basic Protocol 5 describes the metabolomics extraction analysis, including extract shipment. Sample shipment and long-term storage, including obtaining phytosanitary certificates, are described in the Basic Protocol 6.
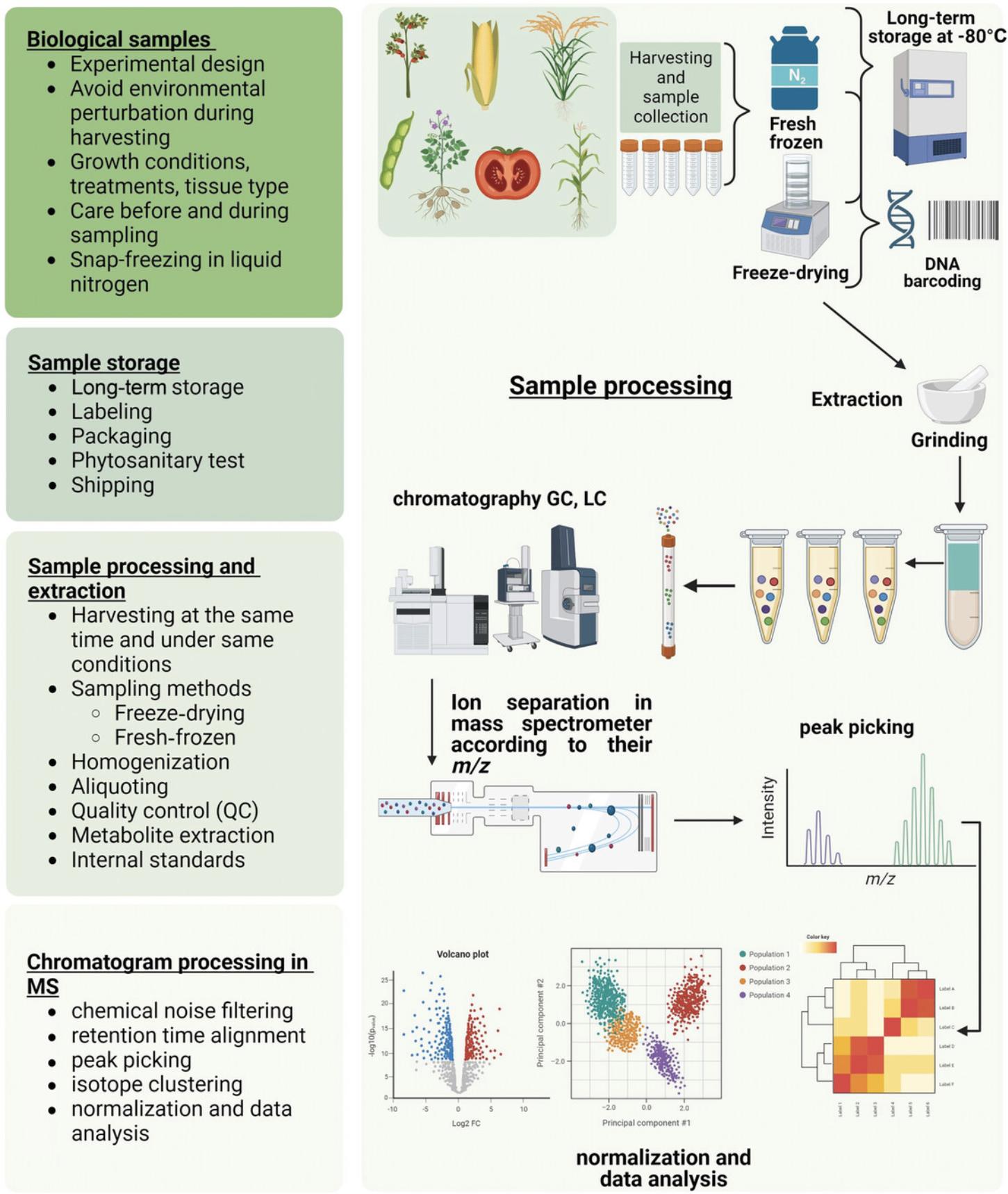
Approach | No. of high-quality markers | Cost/sample | Comments | Reference |
---|---|---|---|---|
Multiplexed PCR and resequencing | 10-103 | 5-15 € | Targeted method; requires a priori genome knowledge; robust to low DNA quantity/ quality | (Onda et al., 2018) |
DArT-Seq | 103-104 | 20-40 € | Untargeted method; robust to low DNA quantity/quality | (Wenzl et al., 2004) |
Genotyping by sequencing | 103-104 | 20-40 € | Untargeted method; requires good-quality DNA; high % of missing data | (Elshire et al., 2011) |
SPET | 104-105 | 20-50 € | Targeted method; requires a priori genome knowledge; requires good-quality DNA | (Barchi et al., 2019) |
Genome resequencing | 106-107 | 50-300 € | Untargeted method; requires good-quality DNA; sequencing depth can be decreased to 1×, in combination with imputation methods | (Kumar et al., 2021) |
SAMPLING FOR GENOMIC ANALYSES
The protocols below are adapted for freeze-dried leaf disk samples for DArT-Seq genotyping and multiplexed PCR analysis. No particular cautions are required for DNA preparation for genomics analyses. Young, healthy leaves are usually the preferred materials for DNA preparation, and no particular pooling scheme is required. Usually, freeze-drying the tissue before homogenization and extraction results in higher-molecular-weight DNA with a size up to 40 kb, with fragments of 20 to 25 kb predominating. The protocols below describe sample preparation for multiplex PCR or DArT-Seq genotyping (Basic Protocol 1), preparation of pathogen-free nucleic acids for most genotyping-resequencing applications or pathogen detection (Basic Protocol 2), and extraction of high-quality DNA for resequencing or specific genotyping applications using commercial kits (Alternate Protocol).
Basic Protocol 1: PREPARATION OF FREEZE-DRIED LEAF DISKS FOR MULTIPLEXED PCR OR DArT-Seq GENOTYPING
Leaf disks are collected in screw-cap microcentrifuge tubes, freeze-dried, and shipped to a centralized laboratory/service provider for DNA extraction/genotyping. The freeze-drying (lyophilization) method offers a robust and stable format that also facilitates easier shipment and storage. Lyophilization is used to remove moisture from various types of samples while preserving their structure (Maisl et al., 2023).
Materials
-
Young leaves from plant of interest
-
Liquid nitrogen
-
2-ml screw-cap polypropylene microcentrifuge tubes, with O-ring and frosted writing surface (e.g., Brand BR780758)
-
Parafilm
-
Refrigerated microcentrifuge, 4°C
-
Breathe-Easier Sealing Film (e.g., VWR, 10141-844)
-
Tube rack, 4°C
-
Freeze-dryer
1.Label 2-ml screw-cap polypropylene microcentrifuge tube on the frosted surface.
2.Collect 1 to 2 leaf disks per plant from young leaves of plants of interest by punching the leaves directly on the plant between the tube rim and a clean Parafilm surface held with the other hand. Twist the tube to make a clean cut. Avoid veins. Keep on ice until step 3.
3.Centrifuge briefly in a refrigerated microcentrifuge to move the disks to the tube bottom.
4.Freeze the tubes containing the leaf disks in liquid nitrogen and store at −80°C until freeze-drying is performed.
5.For freeze-drying, open the tube and cover the tube opening with a piece of Breathe-Easier Sealing Film.
6.Place the sample tube in a cold tube rack quickly to avoid defrosting and freeze-dry overnight in a freeze-dryer.
7.Remove the sealing film and cap tube tightly.
8.Store at room temperature.
Basic Protocol 2: MEDIUM-THROUGHPUT PREPARATION OF PATHOGEN-FREE NUCLEIC ACIDS FOR MOST GENOTYPING-RESEQUENCING APPLICATIONS OR PATHOGEN DETECTION
This protocol is for laboratories on a limited budget that do not wish to use commercial kits (Alternate Protocol) or automated DNA extractors. Nucleic acids are extracted in a 96-well format using a phenol-chloroform-sodium dodecyl sulfate (SDS) method, precipitated with isopropanol, and shipped as a pellet. In our hands, the nucleic acids can be used for most genotyping applications and for detection of pathogen DNA/RNA using quantitative or end-point PCR.
Materials
-
Extraction buffer (see recipe)
-
Phenol-chloroform (e.g., Thermo Fisher, 17909)
-
Isopropanol
-
80% (v/v) ethanol
-
TE buffer (10 mM Tris /0.1 mM EDTA, pH 8.0) with freshly added 100 ng/µl RNase A (DNase-free, e.g., Thermo Fisher, EN0531)
-
Nuclease-free water (DEPC-treated or, e.g., Thermo Fisher, AM9935)
-
5-mm hole puncher
-
Collection microtubes racked 10 × 96 (Qiagen, cat. no. 19560)
-
Microsoft Excel
-
Gauze
-
Rubber band
-
Tungsten carbide beads, 3 mm (e.g., Qiagen, 69997)
-
Collection microtube caps 120 × 8 (Qiagen, cat. no. 19566)
-
Mixer Mill homogenizer (Retsch, model no. MM 400)
-
8-channel micropipets, variable volume (100 to 500 µl and 10 to 50 µl)
-
Refrigerated high-RCF (4000 to 5000 × g) microplate centrifuge
-
96-well deepwell plates, with polypropylene sealing mats (e.g., Thermo Fisher, 260252)
-
Benchtop plate mixer
-
Bubble wrap
-
Transparent packing tape
-
Polypropylene PCR plates, with plate seals
-
37°C or 60°C heating block
-
Additional reagents and equipment for collecting plant tissue disks (see Basic Protocol 1, step 2)
Preparation of 192 samples
1.Using a 5-mm hole puncher, collect two young leaf disks or disks of cotyledon tissue (see Basic Protocol 1, step 2) per tube in collection microtubes racked 10 × 96.Enter the sample names in a Microsoft Excel file for documentation.
2.Mark two sets of collection microtubes racked 10 × 96 as 1 and 2 and pay attention to the orientation in order not to confuse the samples.
3.Cover all the collection microtubes with gauze and a rubber band and freeze-dry leaf material.
4.Add 1 tungsten carbide bead to each collection microtube and cover the tubes with collection microtube caps 120 × 8.
5.Disrupt the leaf tissue with a Mixer Mill homogenizer for 1 min at 25 Hz.
6.Using an 8-channel micropipet, add 400 µl extraction buffer and 100 µl phenol-chloroform to each well. Seal the microtubes with collection microtube caps.
7.Homogenize in homogenizer for 2 min at 25 Hz.
8.Centrifuge 10 min at maximum speed, 4°C, in a refrigerated high-RCF microplate centrifuge.
9.While centrifuging, prepare two clean 96-well deepwell plates on ice, numbered 1 and 2, with 250 µl isopropanol per well and two sealing mats, numbered 1 and 2 and with a mark at position A1.
10.Without disturbing the interface, collect 300 µl from each microtube using the 8-channel micropipet and transfer it to the isopropanol-filled plates, paying attention to the plate orientation.
11.Seal plates with a mat and mix with a benchtop plate mixer for 30 s. Leave on ice for 20 min.
12.Centrifuge 20 min at maximum speed, 4°C.
13.Discard supernatant, blot dry on a clean paper towel, and place upright on the bench.
14.Using the 8-channel micropipet, add 200 µl of 80% ethanol to each well.
15.Paying attention not to invert the mats and their orientation, seal the plates and mix with a benchtop plate mixer for 30 s.
16.Centrifuge 10 min at maximum speed, 4°C.
17.Discard the supernatant carefully using the 8-channel micropipet.
18.Air-dry the plate for 10 min at room temperature to allow evaporation of residual ethanol.
19.Paying attention not to invert the mats and their orientation, seal the plates again. Wrap in bubble wrap and seal with transparent packaging tape to avoid plate breakage during transport.
20.Ship at room temperature.
Upon receipt of the samples
21.Centrifuge 2 min at maximum speed to collect nucleic acid pellet at the bottom of the plate.
22a. For DNA genotyping/resequencing or pathogen DNA detection : Add 50 µl TE buffer with freshly added 100 ng/µl RNase A to each well. Pipet up and down 5 times. Transfer samples to a polypropylene PCR plate. Incubate 30 min at 37°C in a heating block. Seal and store at −20°C.
22b. For RNA virus detection : Add 50 µl nuclease-free water to each well. Pipet up and down 5 times. Transfer to a polypropylene PCR plate. Incubate for 10 min at 60°C in a heating block. Seal and store at −20°C.
Alternate Protocol: LOW-THROUGHPUT EXTRACTION OF HIGH-QUALITY DNA FOR RESEQUENCING USING COMMERCIAL KITS
To maintain the high quality standards required for sequencing, it is recommended to use commercial kits to purify DNA from plant tissues. Below, the Qiagen DNeasy Plant Mini Kit and one for the LGC Sbeadex Maxi Plant Kit are mentioned as examples, but there are several different kits on the market that can be used for obtaining high-quality DNA. Some kits, like the LGC Sbeadex Maxi Plant Kit, are easily adaptable to high-throughput automation. Freeze-drying is required to obtain high-molecular-weight DNA; otherwise, fresh leaf material can be used.
Materials
-
Liquid nitrogen
-
Qiagen DNeasy Plant Mini Kit (Qiagen, 69104) or LGC Sbeadex Maxi Plant Kit (LGC, 41602)
-
Analytical balance
-
2-ml safe-lock microcentrifuge tubes (e.g., Eppendorf, 0030120094)
-
Tungsten carbide beads, 3 mm (e.g., Qiagen, 69997)
-
Mixer Mill homogenizer (Retsch, model no. MM 400) with pre-cooled or non-pre-cooled adapter set
-
Bag with silica gel
-
Additional reagents and equipment for harvesting (see Basic Protocol 1, step 2) and freeze-drying (see Basic Protocol 1, steps 4 to 6) leaf material
DNA extraction from fresh leaf material
1a. Harvest leaf material (see Basic Protocol 1, step 2) and place a sample (≤100 mg of young leaf for Qiagen DNeasy Plant Mini Kit or 40 to 120 mg for LGC Sbeadex Maxi Plant Kit depending on the plant species) in a 2-ml safe-lock microcentrifuge tube together with 1 tungsten carbide bead. Freeze the tube in liquid nitrogen.
2a. To disrupt tissue, put the tube in a pre-cooled adapter set in a Mixer Mill homogenizer and grind the samples for 1 min at 25 Hz.
3a. Refreeze the tubes in liquid nitrogen and repeat step 2a, reversing the position of the tubes within the adapter set.
4a. After the sample is ground to a fine powder, carefully follow the protocol recommended by the manufacturer for the Qiagen DNeasy Plant Mini Kit or LGC Sbeadex Maxi Plant Kit.
5a. If the leaf samples are not processed immediately, store at −80°C.
DNA extraction from freeze-dried material
1b. Harvest leaf material (see Basic Protocol 1, step 2) and place a sample in a 2-ml safe-lock microcentrifuge tube. Freeze-dry it following the instructions in Basic Protocol 1, steps 4 to 6, and then weigh ≤20 mg (Qiagen DNeasy Plant Mini Kit) or 10 to 30 mg (LGC Sbeadex Maxi Plant Kit ) freeze-dried tissue into a 2-ml safe-lock microcentrifuge tube.
2b. Add 1 tungsten carbide bead to the tube, put the tube in a non-pre-cooled adapter set in a Mixer Mill homogenizer, and grind the sample for 1 min at 25 Hz.
3b. Repeat step 2b, reversing the position of the tubes within the adapter set.
4b. After the sample is ground, carefully follow the protocol recommended by the manufacturer (for the Qiagen DNeasy Plant Mini Kit or LGC Sbeadex Maxi Plant Kit).
5b. If the leaf samples are not processed immediately, store at room temperature in a bag with silica gel.
Support Protocol: DNA QUALITY CONTROL
Before shipment to a DNA genotyping/resequencing facility, it may be necessary to perform QC. Usually, DNA is shipped in dry ice, or if EDTA is present (0.1 mM EDTA), it can be shipped using ice pads if the transit time is fast (24 to 48 hr).
For genomic analyses, DNA isolation is a crucial step because low-quality DNA may impair the analyses (Table 1). Therefore, QC of DNA must be performed before the analyses, depending on the number of samples. A total of 3 to 4 QC samples can be added to each batch, which contains around 60 samples. To perform QC, usually 6 µl is required for each test (2 µl for agarose gel electrophoresis, 2 µl for spectrophotometer readings, and 2 µl for fluorimetric determination of DNA concentration). QC usually consists of the following steps:
- 1)Agarose (0.8% w/v) gel electrophoresis to check DNA integrity and RNA contamination: Use a high-molecular-weight marker like phage lambda DNA not digested and/or digested with HindIII to evaluate the size of extracted DNA. Moreover, loading different amounts of the reference marker (e.g., 25, 50, and 100 ng total), the fluorescence can be used to have a rough estimation of the DNA concentration of the unknown samples.
- 2)Determination of 260/230 and 260/280 ratios by a spectrophotometer: This is only for samples isolated with a commercial kit like the Qiagen DNeasy Plant Mini Kit or LGC Sbeadex Maxi Plant Kit (see Alternate Protocol) that completely eliminates oligoribonucleotides remaining after RNase digestion. Optimal 260/280 and 260/230 ratios are 1.7 to 2.0 and 2.0 to 2.2, respectively.
- 3)Fluorimetric determination of DNA concentration based on a DNA intercalating agent: Picogreen (Thermo Fisher, P7589), a Qubit dsDNA High Sensitivity Assay Kit (Thermo Fisher Scientific, Q33230), or similar can be used. Depending on the specific protocol/kit used and on the species, the DNA concentration can range from 10 to 20 ng/µl up to 200 ng/µl. It is possible to increase the DNA concentration by reducing the elution volume.
SAMPLING FOR METABOLOMICS ANALYSES
For most metabolomics experiments, plant materials need to grow under field or greenhouse conditions, which may cause large variations in transcriptomic and metabolomic datasets. Evaluation of large sample sets becomes relatively simpler with the use of variables under strictly controlled conditions (Alseekh et al., 2018). Harvesting several plant materials of each variety may provide stronger information about the degree of similarity between collected samples (Markert, 1995). Therefore, in large experiments, it is important to detect the number of lines and associated biological replicates. Plant sampling has great importance in metabolomics experiments. Special care is necessary to avoid rapid metabolic changes, which may then affect the results considerably during harvesting and storage (Gibon & Rolin, 2012).
Plant metabolomics experiments are generally performed at the organ level (e.g., developing fruit, whole leaf, root) for most of the crop species, such as maize, tomato, and rice, and to reduce the variation within the genotype, it is recommended to have pooled samples per replicate. The harvesting time needs to be taken into account because the levels of plant metabolites vary throughout the day (Queiroz, 1974).
Once the material is harvested for metabolomics, it should be frozen immediately to prevent any enzymatic reactions (Basic Protocol 4). The time and method of sampling depend on the plant's life cycle, which then influences the analysis. Different methods are applied while harvesting different plant tissues; for instance, when a plant leaf is harvested, it should immediately be frozen to prevent any enzymatic reactions. However, fruit samples can be transported to the laboratory inside collection bags and can be frozen after obtaining the specific part according to the desired method to be performed.
Sampling can be performed as freeze-dried (lyophilized) or fresh-frozen (liquid nitrogen) samples based on the intended analysis (Basic Protocols 3 and 4, respectively). Placing the samples in a liquid nitrogen tank to freeze them can be a simple and rapid way to prepare them for metabolomics analyses. As an important factor, storage conditions have to be controlled for the stability of the samples. Usually, the sample storage temperature is −80°C for transcriptomics and metabolomics analysis to avoid sample thawing.
As plant samples potentially can be contaminated with pathogens, a phytosanitary certificate is generally required for cross-country shipment of untreated plant samples (see Basic Protocol 6, “Phytosanitary Certificate and Import Permit”).
Basic Protocol 3: PREPARATION OF FREEZE-DRIED PLANT MATERIAL FOR METABOLOMICS
Drying is required for some specific analyses, especially for metabolomics, because water provides the medium for enzyme-mediated reactions that may cause metabolite decomposition (Kim & Verpoorte, 2010). Different methods are available for drying samples, such as ambient air-drying, oven-drying, freeze-drying, and trap-drying (Harbourne et al., 2009). Among these methods, the most common and suitable method to dry plant material is freeze-drying.
During the process of freeze-drying, the material is first frozen and then exposed to low pressure and subjected to a vacuum to remove the frozen water through sublimation, which reduces oxidative denaturation. Before complete drying, samples are kept at low temperatures so that enzyme activity is inhibited and thus enzyme-catalyzed degradation is reduced.
Materials
-
Liquid nitrogen
-
Dry ice (optional)
-
Storage tubes or bags
-
Permanent marker
-
Dewar
-
Freeze-dryer with vacuum pump (e.g., Christ, model no. Alpha 2-4)
-
Mixer Mill homogenizer (Retsch, model no. MM 400)
-
Tungsten carbide beads, 3 mm (e.g., Qiagen, 69997)
-
Vacuum bags
-
Additional reagents and equipment for harvesting plant tissue (see Basic Protocol 1, step 2)
1.Label as many storage tubes or bags as samples with a permanent marker before harvesting to avoid sample mixture. Then, harvest appropriate plant tissue (e.g., source leaves and sink leaves, stems, and/or roots; see Basic Protocol 1, step 2) according to the experimental aim.
2.Immediately freeze tissue in liquid nitrogen in a dewar and store it at −80°C until further processing.
3.Turn on and set up a clean and properly working freeze-dryer with a vacuum pump according to the manufacturer's instructions.
4.Place the sample on dry ice or in liquid nitrogen until ready to place in the freeze-dryer. Remove the lid of the sample and quickly place it into the freeze-dryer.
5.Follow the manufacturer's instructions to ensure the freezing temperature is reached.
6.Once the desired level of dryness is achieved, gradually increase the temperature inside the freeze-dryer to atmospheric pressure.
7.Grind the sample using a Mixer Mill homogenizer and tungsten carbide beads until a fine-powdered form is obtained. Keep samples at a low temperature with liquid nitrogen or dry ice during the grinding process.
8.After lyophilization, keep samples in vacuum-sealed bags for long-term storage.
Basic Protocol 4: PREPARATION OF FRESH-FROZEN PLANT MATERIAL FOR METABOLOMICS
In this protocol, samples are frozen in liquid nitrogen or directly transferred to an ultra-low-temperature freezer as soon as possible after collection. Fresh-frozen samples can be stored for several years, as they are kept stable at low temperatures.
Materials
-
Liquid nitrogen
-
Dry ice (optional)
-
2-ml tubes
-
Mixer Mill homogenizer (Retsch, model no. MM 400)
-
Analytical balance
-
Additional reagents and equipment for harvesting plant tissue (see Basic Protocol 1, step 2)
1.Harvest plant material from plants (see Basic Protocol 1, step 2), place it inside a 2-ml tube, and freeze it in liquid nitrogen.
2.Use a Mixer Mill homogenizer to grind plant samples that are frozen in liquid nitrogen, grinding to a fine powder for 2 min at 25 Hz. Then, place the samples either on dry ice or in liquid nitrogen until the next step to avoid sample melting.
3.Label transferring 2-ml tubes before cooling down in liquid nitrogen.
4.Aliquot (50 mg) frozen ground material into each labeled transferring 2-ml tube.
5.For long-term storage, store material at −80°C.
Basic Protocol 5: PREPARATION AND SHIPMENT OF METABOLITE EXTRACTS FOR METABOLOMIC ANALYSES
The protocol below is adapted for shipment to countries with strict phytosanitary regulations (see Basic Protocol 6), in which freeze-drying or pulverizing of biological materials is considered insufficient for the inactivation of possible pathogens. The introduction of a chaotropic agent like chloroform during the extraction ensures such inactivation. The protocol is adapted for the analysis of both semi-polar and non-polar metabolites, but, because it involves a drying step, not for volatile ones.
Semi-polar and non-polar internal standards are added to correct for sample loss during the procedure. It is suggested to use QC samples for the large number of samples, which then can help to make sure that samples are treated in the same way in the case of using batches. Samples must be shipped in dry ice, stored at −80°C upon arrival, and analyzed as soon as possible due to the intrinsic instability of extracts. See Basic Protocol 6 for further shipping information.
Materials
-
Freeze-dried plant tissue (see Basic Protocol 3)
-
Methanol (LC-MS grade) spiked with fixed concentration of freshly prepared internal semi-polar standard (e.g., 3 ng/ml formononetin)
-
Chloroform (LC-MS grade) spiked with fixed concentration of freshly prepared internal non-polar standard (e.g., 50 µg/ml DL-alpha-tocopherol acetate).
-
Tris-NaCl buffer (1 M NaCl and 50 mM Tris-HCl, pH 7.5)
-
100% methanol (LC-MS grade)
-
100% chloroform (LC-MS grade)
-
Dry ice
-
1.5-ml (conical) and 2-ml (round-bottom) safe-lock microcentrifuge tubes (e.g., Eppendorf, 0030120094 and 003012008620)
-
Analytical balance
-
Tungsten carbide beads, 3 mm (e.g., Qiagen, 69997)
-
Rotary shaker for microcentrifuge tubes
-
Vortex
-
Refrigerated microcentrifuge, 4°C
-
500-µl Hamilton syringe
-
Rotary evaporator for microcentrifuge tubes (e.g., Thermo Fisher SpeedVac or Eppendorf Concentrator Plus)
1.Prepare and label as many 2-ml (round-bottom) safe-lock microcentrifuge tubes as samples.
2.Working under dim light, weigh 5 to 25 mg freeze-dried plant tissue in each tube (quantity depends on the tissue and analytical method used). Place tubes on ice.
3.Add a clean tungsten carbide bead to each tube.
4.Add 200 µl methanol spiked with a fixed concentration of freshly prepared internal semi-polar standard (e.g., 3 ng/ml formononetin).
5.Shake for 10 min in rotary shaker for microcentrifuge tubes.
6.Add 400 µl chloroform spiked with a fixed concentration of the freshly prepared internal non-polar standard (e.g., 50 µg/ml DL-alpha-tocopherol acetate).
7.Shake for 10 min in the rotary shaker.
8.Add 200 µl Tris-NaCl buffer.
9.Vortex for 10 s.
10.Centrifuge the samples for 10 min at maximum speed at 4°C in a refrigerated microcentrifuge.
11.Collect 300 µl of upper phase (semi-polar metabolites) with a 500-µl Hamilton syringe in a 1.5-ml (conical) safe-lock microcentrifuge tube without disturbing the interface. Wash the syringe with 100% methanol between samples.
12.Collect 300 µl of lower phase (non-polar metabolites) with the 500-µl Hamilton syringe in a 1.5-ml (conical) safe-lock microcentrifuge tube without disturbing the interface. Wash the syringe with 100% chloroform between samples.
13.Dry completely in the dark in a rotary evaporator for microcentrifuge tubes. Dry chloroform extracts first (they dry almost immediately) and then dry the semi-polar extracts.
14.As soon as pellets are dry, cap and store at −80°C in the dark.
Basic Protocol 6: SAMPLE SHIPPING AND LONG-TERM STORAGE
Sample Shipping
1.Phytosanitary certificate and import permit
Countries have different regulations, with some plant products regulated and some not. In general, for the import of unprocessed plant products, a phytosanitary certificate issued by the National Plant Protection Organization of the exporting country is required. Grinding, freezing, and drying are not considered as processing. Certain plants and plant products or plants originating from certain countries or regions may be completely banned from import. The following process can be used as guidance in obtaining a phytosanitary certificate and import permit, if needed:
-
- Check whether the movement of plant samples is legally possible and whether a phytosanitary certificate or other documents are required.
National contact points can inform whether a phytosanitary certificate is required for importing plant samples: https://www.ippc.int/en/countries/all/contactpoints/. The legal environments for plant imports across countries can be found here: https://www.ippc.int/en/countries/all/legislation/.
For the EU, regulation (EU) 2016/2031 of the European Parliament and the Council regulates the import of plant material from third countries into the EU (https://eur-lex.europa.eu/legal-content/EN/TXT/HTML/?uri=CELEX:32019R2072#d1e32-216-1), and Article 13 of this regulation lists plants, unprocessed plant products, and other objects for which a plant passport is required for their movement within the EU territory.
Professional operators can request exemptions from the national authorities from import bans or the requirement for phytosanitary certificates for official testing, scientific or educational purposes, trials, varietal selections, or breeding following the Commission Delegated Regulation (EU) 2019/829 that supplements Regulation (EU) 2016/2031.If granted, a letter of authority will be issued that must accompany shipments in lieu of a phytosanitary certificate.
In the USA, import of plant samples is regulated by Plant Protection and Quarantine (PPQ) under the authority of the Plant Protection Act (https://www.aphis.usda.gov/aphis/ourfocus/planthealth). A Controlled Import Permit (CIP) is required to import plant samples that are not intended for plating and are imported solely for destructive analysis. A containment facility may be required for importation of the regulated material. The facility will be evaluated before the permit is issued. Information and forms to apply for a CIP to import plant samples for destructive analysis can be found here: https://www.aphis.usda.gov/aphis/ourfocus/planthealth/import-information/permits/plants-and-plant-products-permits/prohibited/cip. The application is filed online and the CIP to import restricted or not authorized plant material is issued electronically via the ePermit system.
In Canada, movement of plants and plant parts is governed by Plant Protection Regulations (https://laws-lois.justice.gc.ca/eng/regulations/SOR-95-212/FullText.html). Samples for scientific research can be imported after requesting an import permit from the Canadian Food Inspection Agency (CFIA, https://inspection.canada.ca/importing-food-plants-or-animals/plant-and-plant-product-imports/eng/1299168480001/1299168593866), and the permit must be obtained before the shipment arrives in Canada.
-
- Check whether an additional declaration on phytosanitary certificates is required.
For the EU, Annex VII of Commission Implementing Regulation (EU) 2019/2072 lists plants, plant products, and other objects originating from third countries, and the corresponding special requirements for their introduction into the EU territory is set out. For export to third countries, the Plant Health Authority in the importing country informs about additional declarations.
-
- Obtain a phytosanitary certificate for the cross-border movement of plant products.
The sender of the material contacts the Plant Health Authority in the exporting country and requests a phytosanitary certificate. Some countries can issue electronic phytosanitary certificates using the electronic certification tool TRACES NT (https://webgate.ec.europa.eu/tracesnt/login).
-
- Make sure that a phytosanitary certificate or a Letter of Authority [please refer to (1)] is accompanying the consignment.
2.Biosecurity
- Check the plant health status and plant samples for any signs of pests or diseases before packaging. 2.Check and comply with international regulations for shipping plant materials because some countries have strict rules to prevent the spread of pests and diseases. Pest risk usually increases with plant age and size, so larger plants have more potential for exposure to the diversity of pests (Eschen et al., 2017).
3.Labeling
- Properly label samples and carefully print the address of the sender and recipient along with the contact information on the shipping box.
- Include all the special handling instructions, tracking number, plant species, and quantity of samples and indicate if the phytosanitary certificate is included.
4.Packaging
- Select appropriate containers, choosing containers that are suitable for the type of samples being shipped, such as vials, tubes, bags, or boxes.
- Pack fresh-frozen or fresh-frozen powdered samples on dry ice. 3.Use bubble wrap when sending samples with dry ice, which can prevent shifting of the samples and minimize the risk of sample loss during transit when the ice dissipates. Insulation materials such as Styrofoam can be used for temperature-sensitive plant materials to provide thermal protection. 4.Indicate the sample shipping list on the box. 5.Place lyophilized plant samples in vacuum-sealed bags so that samples do not moisturize and can be stored longer. 6.Ensure that samples are packed tightly to minimize movement and potential breakage/opening.
- Include silica gel packets inside the shipping container in order to minimize the potential damage caused by humidity.
- Laboratories might have specific packing requirements for shipping. For instance, fresh leaf samples should be double-bagged, and for lyophilized samples, static electricity may cause dried tissue samples to move unexpectedly and cling to the surface (Shillito & Shan, 2022). Therefore, it is suggested to consult with the laboratory if static electricity is an issue and if they have a recommended way to remedy it.
5.Documentation
- Include a detailed packaging list with a description of each sample.
- Clarify the quantity of the samples and provide all the permits and customs forms.
6.Shipping method
- Choose a suitable shipping method that aligns with the urgency of the shipment and any special requirements.
- Keep a record of the tracking number provided by the shipping service to monitor the progress of the shipment.
- Track the status of the shipment online and address any issues promptly.
- Inform the recipient about the tracking number and the expected delivery date of shipment.
Long-Term Storage
It has been shown that different ways and durations of storage can influence the stability of plant materials (Houba et al., 1995). Different enzymes have shown distinct activities after keeping samples at −20°C or −80°C (Hartmann & Asch, 2019). For a long safe storage period, we suggest keeping fresh-frozen samples at −80°C for several months or years. Freeze-dried samples must be kept in vacuum-sealed bags, and for short-term storage, silica gel packets can be placed inside the sample boxes to minimize humidity. It is suggested to perform quality checking during storage or after shipment. Performing physicochemical or biochemical analyses at different time points during sample storage can be helpful while carrying out the quality check (Fish & Davis, 2003; Phillips et al., 2005).
REAGENTS AND SOLUTIONS
Extraction buffer
100 mM Tris·HCl, pH 8.0 (from 0.5 M stock, stored at 4°C) 200 mM NaCl (from 5 M stock, stored at room temperature) 20 mM EDTA, pH 8.0 (from 0.5 M stock, stored at 4°C) 0.5% (w/v) SDS (from 5% stock, stored at room temperature) 0.1% (v/v) 2-mercaptoethanol (stock stored at 4°C) Prepare fresh immediately before use from stock solutions and keep at room temperature
COMMENTARY
Background Information
Metabolomics has been practiced for over 20 years as a companion to other omics technologies such as transcriptomics, genomics, and proteomics (Oliver et al., 1998). Mass spectrometry is a well-established interface with both liquid chromatography (LC-MS) and gas chromatography (GC-MS). The approach also provides important clues about analyte structure from fragmentation patterns as well as the retention time obtained from the chromatographic dimension.
Freeze-drying is a standard method for post-harvest treatment of samples because it reduces their weight and volume and makes them easier to handle, store, transport, weigh, homogenize, and extract, with minimal degradation of the analytes. We have routinely observed a more efficient homogenization/extraction of metabolites from freeze-dried than from frozen plant tissues, as well as a higher molecular weight of the extracted DNA. Because freeze-drying occurs at low temperatures, it prevents the denaturation of heat-sensitive compounds, including enzymes, vitamins, and phytochemicals. Moreover, it effectively eliminates water from plant samples, thereby inhibiting the growth of microorganisms like bacteria and fungi. On the other hand, fresh-frozen plant samples retain the biological activity of plant tissues and components, including enzymes, cellular structures, and metabolites, which is why these samples have been frequently used for enzyme activity assays and metabolic profiling studies. Although fresh-frozen plant sampling has numerous advantages, it also requires access to proper freezing equipment and storage facilities to maintain consistently low temperatures. Additionally, freezing may not be suitable for all types of plant materials; therefore, researchers should choose the sampling method that best suits their specific research plant materials.
Critical Parameters
Experimental design is the first important step when performing any kind of process. For complex experiments, it is necessary to observe the effect of size and estimate the number of replicates because these parameters can significantly change the statistical results of the intended analysis (Trutschel et al., 2015). In plant metabolomics, several factors can contribute to variability across samples, so an appropriate experimental design should be considered to minimize any potential sources of undesired variation. In addition to careful experimental design, fresh-frozen plant materials must be aliquoted quickly, using pre-cooled tubes and spatulas to avoid sample thawing, as well as subsequently stored deep-frozen at a constant −80°C until the first application starts. For detailed metabolic analysis, various plant tissues can be used at a desired developmental stage or time point.
For large sample sets, we recommend using QC samples that are distributed among other samples to correct for batch-to-batch variation. It is highly recommended to split the experiment into several batches in the interest of the intended analysis. QC samples can be pooled from the samples as a reference material, and they should be processed as the samples are.
When transportation or storage of extracts is part of the process, enhancing the chemical stability of compounds can be achieved by first drying the extracts and then storing them at low temperatures (−20°C or −80°C). For prolonged storage, extracts have been successfully stored at −80°C for up to 3 months; investigations into even longer storage times are still pending.
Time Considerations
The time required varies according to the protocol used and the number of samples and resources available in the laboratory.
The time for harvesting and preparation of samples in Basic Protocols 1 and 3 can vary greatly according to the number of samples to be collected in the experiment. The time needed for freeze-drying also depends on the samples.
For DNA extraction using Basic Protocol 2, 192 samples (in two 96-well plates) or 48 samples (in individual Eppendorf tubes) can be processed in 3 to 4 hr.
For DNA extraction using commercial kits (Alternate Protocol), the time needed depends on the number of processed samples and whether automation is used or not. For samples processed manually, usually, 2 to 3 hr is required to extract DNA from 24 samples.
The harvest time and experimental design constraints have been mentioned in the Critical Parameters section. In Basic Protocol 4, around 60 samples can be ground and prepared for the metabolomics extraction in a day. Additionally, in Basic Protocol 5, 60 samples can easily be extracted per day, and drying can be performed overnight. We recommend using electronic pipets if available.
Acknowledgments
We thank Olivia Demurtas (ENEA) for advice on Basic Protocol 5. This work was supported by the European Commission Horizon EU programme, project PRO-GRACE (contract no. 101094738). The World Vegetable Center contribution was funded by National Science and Technology Council, Republic of China, contract no. 2023W02061, and by long-term strategic donors from the UK government, the United States Agency for International Development (USAID), the Australian Centre for International Agricultural Research (ACIAR), Germany, Thailand, Philippines, Korea, and Japan.
Open access funding enabled and organized by Projekt DEAL.
Author Contributions
Esra Karakas : Conceptualization; methodology; writing—original draft; writing—review and editing. Paola Ferrante : Methodology; visualization; writing—review and editing. Roland Schafleitner : Methodology; visualization; writing—review and editing. Giovanni Giuliano : Methodology; visualization; writing—review and editing. Alisdair R. Fernie : Conceptualization; methodology; writing—original draft; writing—review and editing. Saleh Alseekh : Conceptualization; methodology; writing—original draft; writing—review and editing.
Conflict of Interest
The authors declare no conflicts of interest.
Open Research
Data Availability Statement
The data are openly available in a public repository that issues datasets with DOIs.
Literature Cited
- Alseekh, S., Wu, S., Brotman, Y., & Fernie, A. R. (2018). Guidelines for sample normalization to minimize batch variation for large-scale metabolic profiling of plant natural genetic variance. In C. António (Ed.), Plant metabolomics (pp. 33–46). https://doi.org/10.1007/978-1-4939-7819-9_3
- Barchi, L., Acquadro, A., Alonso, D., Aprea, G., Bassolino, L., Demurtas, O., Ferrante, P., Gramazio, P., Mini, P., & Portis, E. (2019). Single primer enrichment technology (SPET) for high-throughput genotyping in tomato and eggplant germplasm. Frontiers in Plant Science , 10, 1005. https://doi.org/10.3389/fpls.2019.01005
- Bohra, A., Kilian, B., Sivasankar, S., Caccamo, M., Mba, C., McCouch, S. R., & Varshney, R. K. (2022). Reap the crop wild relatives for breeding future crops. Trends in Biotechnology , 40, 412–431. https://doi.org/10.1016/j.tibtech.2021.08.009
- Cao, Y., Zhang, K., Yu, H., Chen, S., Xu, D., Zhao, H., Zhang, Z., Yang, Y., Gu, X., & Liu, X. (2022). Pepper variome reveals the history and key loci associated with fruit domestication and diversification. Molecular Plant , 15, 1744–1758. https://doi.org/10.1016/j.molp.2022.09.021
- Chase, M. W., & Fay, M. F. (2009). Barcoding of plants and fungi. Science , 325, 682–683. https://doi.org/10.1126/science.1176906
- Chen, S., Yao, H., Han, J., Liu, C., Song, J., Shi, L., Zhu, Y., Ma, X., Gao, T., & Pang, X. (2010). Validation of the ITS2 region as a novel DNA barcode for identifying medicinal plant species. PLOS ONE , 5, e8613. https://doi.org/10.1371/journal.pone.0008613
- Delfin, J. C., Watanabe, M., & Tohge, T. (2019). Understanding the function and regulation of plant secondary metabolism through metabolomics approaches. Theoretical and Experimental Plant Physiology , 31, 127–138. https://doi.org/10.1007/s40626-018-0126-1
- Demirjian, C., Vailleau, F., Berthomé, R., & Roux, F. (2023). Genome-wide association studies in plant pathosystems: Success or failure? Trends in Plant Science , 28(4), 471–485. https://doi.org/10.1016/j.tplants.2022.11.006
- Elshire, R. J., Glaubitz, J. C., Sun, Q., Poland, J. A., Kawamoto, K., Buckler, E. S., & Mitchell, S. E. (2011). A robust, simple genotyping-by-sequencing (GBS) approach for high diversity species. PLOS ONE , 6, e19379. https://doi.org/10.1371/journal.pone.0019379
- Eschen, R., Douma, J. C., Grégoire, J.-C., Mayer, F., Rigaux, L., & Potting, R. P. J. (2017). A risk categorisation and analysis of the geographic and temporal dynamics of the European import of plants for planting. Biological Invasions , 19, 3243–3257. https://doi.org/10.1007/s10530-017-1465-6
- Fang, C., & Luo, J. (2019). Metabolic GWAS-based dissection of genetic bases underlying the diversity of plant metabolism. The Plant Journal , 97, 91–100. https://doi.org/10.1111/tpj.14097
- Fiehn, O. (2002). Metabolomics–the link between genotypes and phenotypes. Plant Molecular Biology , 48, 155–171. https://doi.org/10.1023/A:1013713905833
- Fish, W. W., & Davis, A. R. (2003). The effects of frozen storage conditions on lycopene stability in watermelon tissue. Journal of Agricultural and Food Chemistry , 51, 3582–3585. https://doi.org/10.1021/jf030022f
- Gibon, Y., & Rolin, D. (2012). Aspects of experimental design for plant metabolomics experiments and guidelines for growth of plant material. In N. W. Hardy & R. D. Hall (Eds.), Plant metabolomics (pp. 13–30). https://doi.org/10.1007/978-1-61779-594-7_2
- Harbourne, N., Marete, E., Jacquier, J. C., & O'Riordan, D. (2009). Effect of drying methods on the phenolic constituents of meadowsweet (Filipendula ulmaria) and willow (Salix alba). LWT-Food Science and Technology , 42, 1468–1473. https://doi.org/10.1016/j.lwt.2009.05.005
- Hartmann, J., & Asch, F. (2019). Extraction, storage duration, and storage temperature affect the activity of ascorbate peroxidase, glutathione reductase, and superoxide dismutase in Rice tissue. Biology , 8, 70. https://doi.org/10.3390/biology8040070
- Hebert, P. D. N., Ratnasingham, S., & De Waard, J. R. (2003). Barcoding animal life: Cytochrome c oxidase subunit 1 divergences among closely related species. Proceedings of the Royal Society of London. Series B: Biological Sciences , 270, S96–S99. https://doi.org/10.1098/rsbl.2003.0025
- Hilu, K. W., Borsch, T., Müller, K., Soltis, D. E., Soltis, P. S., Savolainen, V., Chase, M. W., Powell, M. P., Alice, L. A., Evans, R., Sauquet, H., Neinhuis, C., Slotta, T. A., Rohwer, J. G., Campbell, C. S., & Chatrou, L. W. (2003). Angiosperm phylogeny based on< 011>matK sequence information. American Journal of Botany , 90(12), 1758–1776.
- Holtorf, H., Guitton, M. C., & Reski, R. (2002) Plant functional genomics. Naturwissenschaften , 89, 235–249. https://doi.org/10.1007/s00114-002-0321-3
- Houba, V. J. G., Novozamsky, I., & Van der Lee, J. J. (1995). Influence of storage of plant samples on their chemical composition. Science of the Total Environment , 176, 73–79. https://doi.org/10.1016/0048-9697(95)04831-6
- Kim, H. K., & Verpoorte, R. (2010). Sample preparation for plant metabolomics. Phytochemical Analysis: An International Journal of Plant Chemical and Biochemical Techniques , 21, 4–13. https://doi.org/10.1002/pca.1188
- Korte, A., & Farlow, A. (2013). The advantages and limitations of trait analysis with GWAS: A review. Plant Methods , 9, 1–9. https://doi.org/10.1186/1746-4811-9-29
- Kumar, P., Choudhary, M., Jat, B. S., Kumar, B., Singh, V., Kumar, V., Singla, D., & Rakshit, S. (2021). Skim sequencing: An advanced NGS technology for crop improvement. Journal of Genetics , 100, 1–10. https://doi.org/10.1007/s12041-021-01285-3
- Maisl, C., Doppler, M., Seidl, B., Bueschl, C., & Schuhmacher, R. (2023). Untargeted plant metabolomics: Evaluation of lyophilization as a sample preparation technique. Metabolites , 13, 686. https://doi.org/10.3390/metabo13060686
- Markert, B. (1995). Sample preparation (cleaning, drying, homogenization) for trace element analysis in plant matrices. Science of the Total Environment , 176, 45–61. https://doi.org/10.1016/0048-9697(95)04829-4
- McLeod, L., Barchi, L., Tumino, G., Tripodi, P., Salinier, J., Gros, C., Boyaci, H. F., Ozalp, R., Borovsky, Y., & Schafleitner, R. (2023). Multi-environment association study highlights candidate genes for robust agronomic quantitative trait loci in a novel worldwide Capsicum core collection. The Plant Journal , 116(5), 1508–1528. https://doi.org/10.1111/tpj.16425
- Oliver, S. G., Winson, M. K., Kell, D. B., & Baganz, F. (1998). Systematic functional analysis of the yeast genome. Trends in Biotechnology , 16, 373–378. https://doi.org/10.1016/S0167-7799(98)01214-1
- Onda, Y., Takahagi, K., Shimizu, M., Inoue, K., & Mochida, K. (2018). Multiplex PCR targeted amplicon sequencing (MTA-Seq): Simple, flexible, and versatile SNP genotyping by highly multiplexed PCR amplicon sequencing. Frontiers in Plant Science , 9, 201. https://doi.org/10.3389/fpls.2018.00201
- Peyraud, R., Dubiella, U., Barbacci, A., Genin, S., Raffaele, S., & Roby, D. (2017). Advances on plant–pathogen interactions from molecular toward systems biology perspectives. The Plant Journal , 90, 720–737. https://doi.org/10.1111/tpj.13429
- Phillips, K. M., Wunderlich, K. M., Holden, J. M., Exler, J., Gebhardt, S. E., Haytowitz, D. B., Beecher, G. R., & Doherty, R. F. (2005). Stability of 5-methyltetrahydrofolate in frozen fresh fruits and vegetables. Food Chemistry , 92, 587–595. https://doi.org/10.1016/j.foodchem.2004.08.007
- Queiroz, O. (1974). Circadian rhythms and metabolic patterns. Annual Review of Plant Physiology , 25, 115–134. https://doi.org/10.1146/annurev.pp.25.060174.000555
- Schauer, N., & Fernie, A. R. (2006). Plant metabolomics: Towards biological function and mechanism. Trends in Plant Science , 11, 508–516. https://doi.org/10.1016/j.tplants.2006.08.007
- Shillito, R., & Shan, G. (2022). Application of sampling and detection methods in agricultural plant biotechnology. Elsevier.
- Smith, S. W., Giesbrecht, E., Thompson, M., Nelson, L. S., & Hoffman, R. S. (2008). Solanaceous steroidal glycoalkaloids and poisoning by Solanum torvum, the normally edible susumber berry. Toxicon , 52, 667–676. https://doi.org/10.1016/j.toxicon.2008.07.016
- Sulli, M., Barchi, L., Toppino, L., Diretto, G., Sala, T., Lanteri, S., Rotino, G. L., & Giuliano, G. (2021). An eggplant recombinant inbred population allows the discovery of metabolic QTLs controlling fruit nutritional quality. Frontiers in Plant Science , 12, 638195. https://doi.org/10.3389/fpls.2021.638195
- Sumner, L. W., Mendes, P., & Dixon, R. A. (2003). Plant metabolomics: Large-scale phytochemistry in the functional genomics era. Phytochemistry , 62, 817–836. https://doi.org/10.1016/S0031-9422(02)00708-2
- Trutschel, D., Schmidt, S., Grosse, I., & Neumann, S. (2015). Experiment design beyond gut feeling: Statistical tests and power to detect differential metabolites in mass spectrometry data. Metabolomics , 11, 851–860. https://doi.org/10.1007/s11306-014-0742-y
- Wenzl, P., Carling, J., Kudrna, D., Jaccoud, D., Huttner, E., Kleinhofs, A., & Kilian, A. (2004). Diversity Arrays Technology (DArT) for whole-genome profiling of barley. Proceedings of the National Academy of Sciences , 101, 9915–9920. https://doi.org/10.1073/pnas.0401076101
- Wolkers, W. F., & Oldenhof, H. (2015). Cryopreservation and freeze-drying protocols. Springer.
- Wurtzel, E. T., & Kutchan, T. M. (2016). Plant metabolism, the diverse chemistry set of the future. Science , 353, 1232–1236. https://doi.org/10.1126/science.aad2062