Generating Enteric Nervous System Progenitors from Human Pluripotent Stem Cells
Antigoni Gogolou, Antigoni Gogolou, Thomas J.R. Frith, Thomas J.R. Frith, Anestis Tsakiridis, Anestis Tsakiridis
Abstract
The intrinsic innervation of the gastrointestinal (GI) tract is comprised of enteric neurons and glia, which are buried within the wall of the bowel and organized into two concentric plexuses that run along the length of the gut forming the enteric nervous system (ENS). The ENS regulates vital GI functions including gut motility, blood flow, fluid secretion, and absorption and thus maintains gut homeostasis. During vertebrate development it originates predominantly from the vagal neural crest (NC), a multipotent cell population that emerges from the caudal hindbrain region, migrates to and within the gut to ultimately generate neurons and glia in response to gut-derived signals. Loss of GI innervation due to congenital or acquired defects in ENS development causes enteric neuropathies which lack curative treatment. Human pluripotent stem cells (hPSCs) offer a promising in vitro source of enteric neurons for modeling human ENS development and pathology and potential use in cell therapy applications. Here we describe in detail a differentiation strategy for the derivation of enteric neural progenitors and neurons from hPSCs through a vagal NC intermediate. Using a combination of instructive signals and retinoic acid in a dose/time dependent manner, vagal NC cells commit into the ENS lineage and develop into enteric neurons and glia upon culture in neurotrophic media. © 2021 The Authors. Current Protocols published by Wiley Periodicals LLC.
Basic Protocol 1 : Generation of vagal neural crest/early ENS progenitors from hPSCs
Basic Protocol 2 : Differentiation of hPSC-derived vagal NC/early ENS progenitors to enteric neurons and glia
INTRODUCTION
The enteric nervous system (ENS) is a complex and elegantly shaped network of neurons and supporting glial cells which provides the intrinsic gut innervation to control a plethora of bowel functions, such as intestinal motility, fluid secretion, movement across the epithelium, and vascular tone (Furness, 2012). The ENS is organized into ganglionated structures forming two major plexuses, the myenteric and submucosal plexus, located within the wall of gastrointestinal (GI) tract and extending longitudinally (Furness, 2006). During early embryonic development, the majority of enteric ganglia develops from the vagal neural crest (NC; Anderson, Stewart, & Young, 2006; Le Douarin & Teillet, 1973). Vagal NC is a transient multipotent cell population emerging from the dorsal tip of the neural tube at the level of somites 1 to 7 and is marked by the expression of HOX gene paralogous groups (PG) 3-5 (Burke, Nelson, Morgan, & Tabin, 1995; Chan et al., 2005; Fu, Lui, Sham, Cheung, & Tam, 2003; Kam & Lui, 2015). Apart from generating most of the ENS, vagal NC cells also contribute to cardiovascular system structures, lung, thymus, and pancreas (Hutchins et al., 2018; Le Douarin, Creuzet, Couly, & Dupin, 2004). Following delamination, vagal NC cells emigrate to the gut, a process partially orchestrated by somite-derived retinoic acid (RA; Niederreither et al., 2003; Simkin, Zhang, Rollo, & Newgreen, 2013; Uribe, Hong, & Bronner, 2018), and commit to a developmental program, which involves highly coordinated events such as progressive ENS progenitor specification and proliferation, rostro-caudal migration, and differentiation towards neurons and glia (reviewed in Kang, Fung, & Vanden Berghe, 2021; Lake & Heuckeroth, 2013). Dysregulation of these processes could result in absence or dysfunction of enteric ganglia along various lengths of the intestine, a common cause of enteric neuropathies such as Hirschsprung's disease (HSCR; Heanue & Pachnis, 2007; Obermayr, Hotta, Enomoto, & Young, 2013).
Seminal findings on the persistence of enteric neural progenitor cells in the fetal and post-natal gut offer an autologous cell therapy as a promising strategy to treat enteric neuropathies (Burns & Thapar, 2014). However, limiting factors such as the need for a sufficient pool of ENS progenitors that could be harvested have set a roadblock to this therapeutic approach. Human pluripotent stem cells (hPSCs) offer an alternative, accessible, and infinite source of such progenitor cells (reviewed in Stamp, 2017) and a number of hPSC differentiation strategies for the production of ENS progenitors and their derivatives have been reported over the past few years (Barber, Studer, & Fattahi, 2019; Fattahi et al., 2016; Lai et al., 2017; Lau et al., 2019; Li et al., 2018; Schlieve et al., 2017; Workman et al., 2017). We have recently described the generation of NC populations corresponding to various levels along the anteroposterior (A-P) axis from hPSCs, including vagal NC (Frith et al., 2018). Based on this, we developed a step-by-step differentiation strategy for the generation of ENS progenitors via an hPSC-derived vagal NC intermediate (Frith et al., 2020). Our approach relies on the induction of a HOX-negative NC population with a default cranial identity (Hackland et al., 2017), expressing SOX10, PAX3, PAX7 , which is subsequently steered toward a caudal hindbrain/cervical axial identity, reflected by the upregulation of HOX PG members (3-5), following exposure to RA (Frith et al., 2018; Frith et al., 2020). Moreover, we found that RA treatment drives the acquisition of an ENS progenitor identity marked by the induction of ASCL1, PHOX2B. These vagal NC/early ENS progenitors can colonize and differentiate efficiently within the ENS of adult mice following transplantation and thus serve as an attractive cell population for use in cell therapy approaches aiming to treat enteric neuropathies such as HSCR (Frith et al., 2020). Here we provide a detailed description of the protocol for their in vitro production from hPSCs as well as their subsequent differentiation into enteric neurons and glia (Fig. 1).
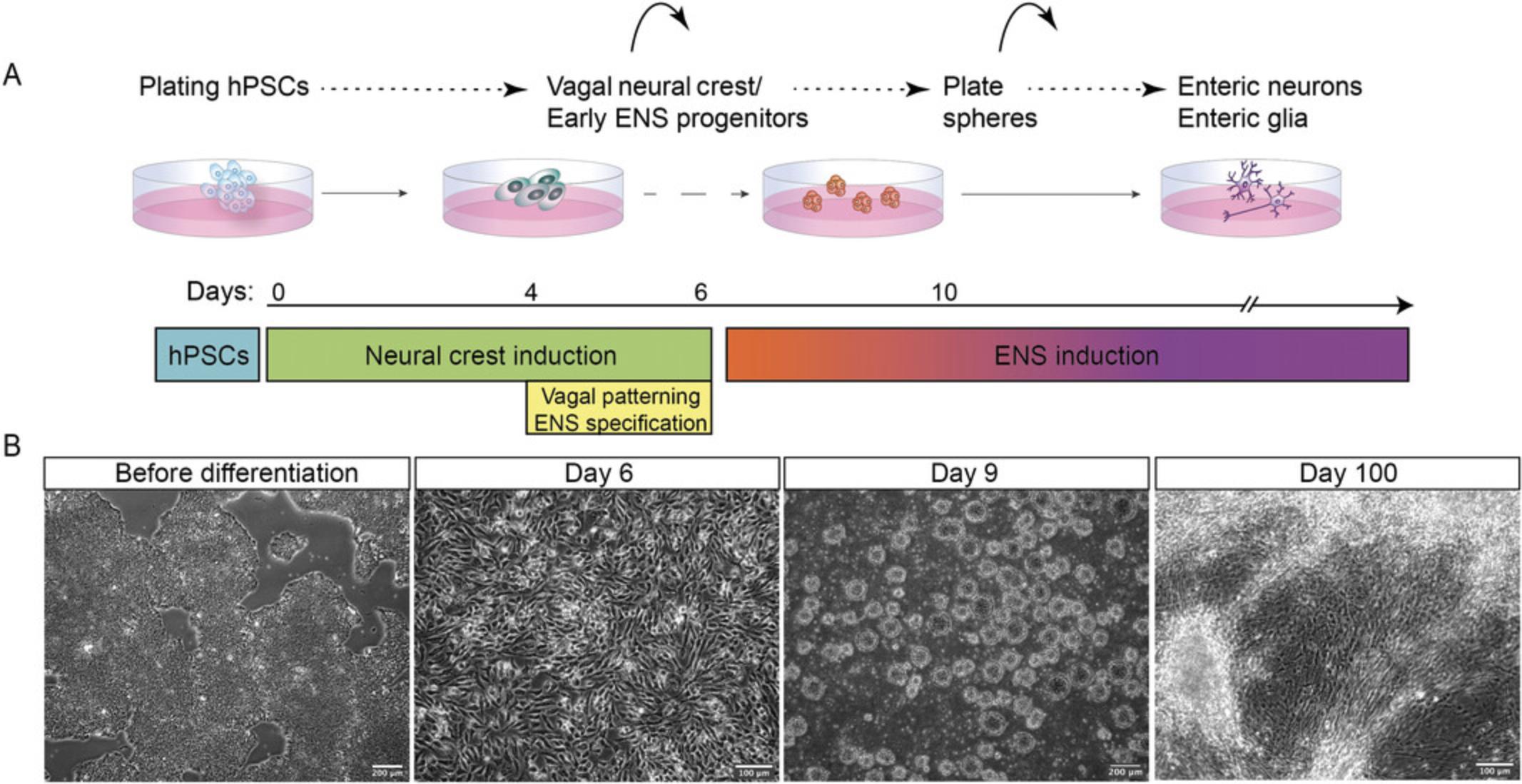
Basic Protocol 1: GENERATION OF VAGAL NEURAL CREST/EARLY ENS PROGENITORS FROM hPSCs
The differentiation protocol (Fig. 2A) begins with plating hPSCs into a culture regime that allows precise control of BMP signaling levels, achieved by simultaneous treatment with BMP4 recombinant protein along with a BMP type-I receptor inhibitor (DMH-1, an approach dubbed as Top-Down inhibition; Hackland et al., 2017), WNT signaling activation through GSK-3 inhibition mediated by the WNT agonist CHIR99021, and TFG-β pathway inhibition by employing the ALK receptor inhibitor SB431542.Following 4 days of treatment, the resulting population displays an anterior/cranial NC character reflected by the upregulation of SOX10, PAX3, PAX7 , p75, and the lack of HOX gene expression. Medium supplementation with RA during days 4 to 6 induces a vagal axial identity marked by the upregulation of the corresponding HOX genes (HOXB4, HOXB5), a step which coincides with the acquisition of an ENS precursor character as evidenced by ASCL1, PHOX2B expression.
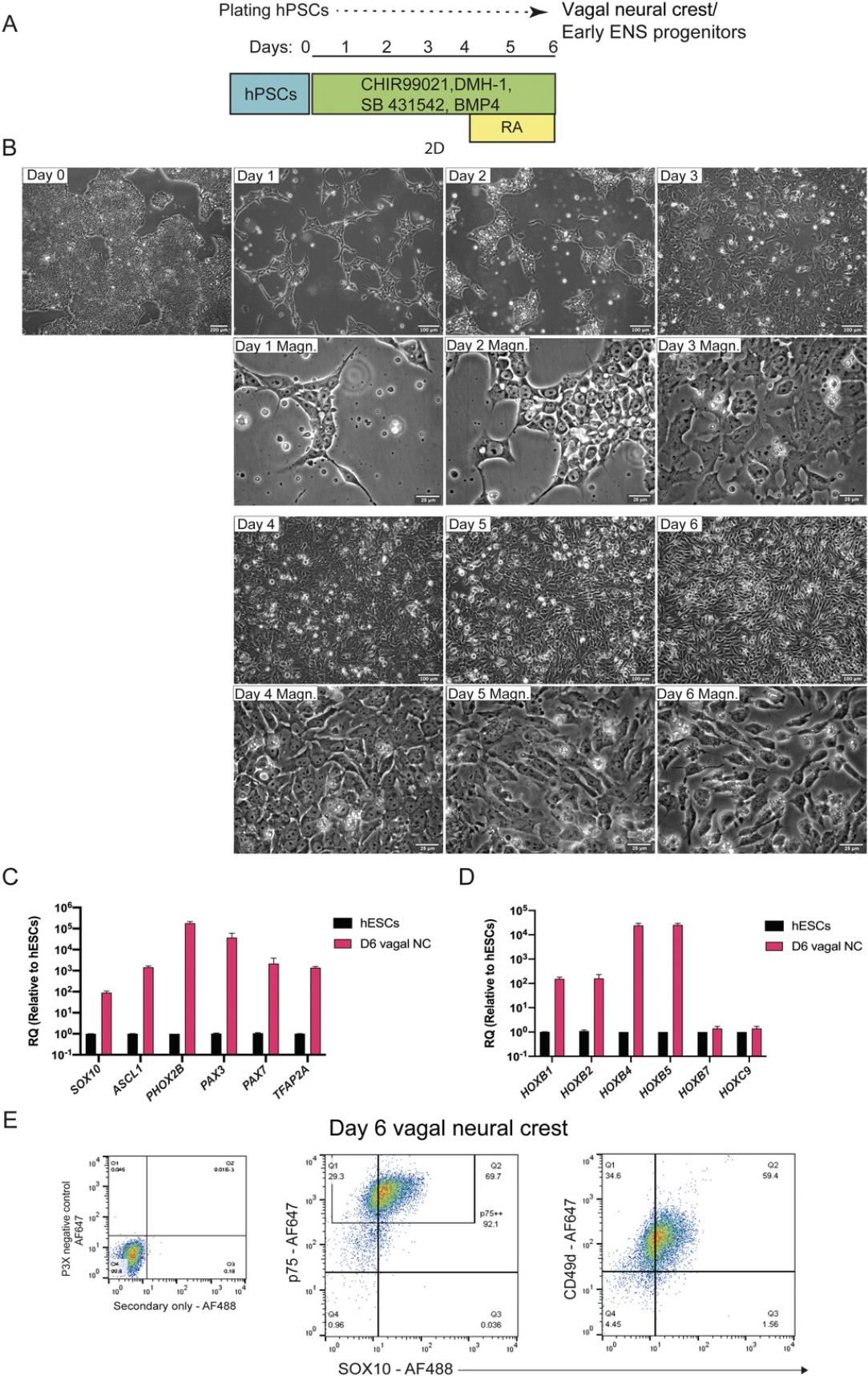
Materials
- Human pluripotent stem cells (hPSCs), e.g., cell lines used for this study: hESC line H9 (WA09), see Thomson et al., 1998 and H9SOX10:GFP, see Chambers et al., 2012
- Dulbecco's Modified Eagle's Medium/Nutrient Mixture F-12 Ham (DMEM/F12; Merck Life Science, cat. no. D6421; store at 4°C)
- mTeSR™1 (Stemcell Technologies, cat. no. 85850; prepare as per manufacturer's instructions)
- ReLeSR™ (Stemcell Technologies, cat. no. 05873; store at room temperature)
- Dulbecco's Phosphate Buffered Saline (Merck Life Science, cat. no. D8537; store at room temperature)
- N2 Supplement (Thermo Fisher Scientific, cat. no. 17502048; aliquot and store at −20°C; minimize freeze-thaw cycles)
- GlutaMAX™ Supplement (Thermo Fisher Scientific, cat. no. 35050038; store at 4°C)
- MEM Non-Essential Amino Acids Solution (Thermo Fisher Scientific, cat. no. 11140050; store at 4°C)
- CHIR99021 (Tocris, cat. no. 4423; aliquot and store at −20°C; minimize freeze-thaw cycles)
- SB 431542 (Tocris, cat. no. 1614; aliquot and store at −20°C; minimize freeze-thaw cycles)
- Human BMP4 Recombinant Protein (Thermo Fisher Scientific, cat. no. PHC9534; aliquot and store at −20°C; minimize freeze-thaw cycles)
- DMH-1 (Tocris, cat. no. 4126; aliquot and store at −20°C; minimize freeze-thaw cycles)
- Penicillin-Streptomycin (5,000 U/ml; Thermo Fisher Scientific, cat. no. 15070063; aliquot and store at −20°C as per manufacturer's instructions)
- Y-27632 2HCl (AdooQ Bioscience, cat. no. A11001; aliquot and store at −20°C; minimize freeze-thaw cycles)
- All-trans retinoic acid (RA; Merck Life Science, cat. no. R2625; stock solution of 10-2 M in DMSO: store at −80°C; prepare and store aliquots of 10-3 M in DMSO at −20°C in light protected vials; dilute retinoic acid with tissue culture medium right before use in subdued light)
- Geltrex™ LDEV-Free Reduced Growth Factor Basement Membrane Matrix (Thermo Fisher Scientific, cat. no. A1413202; defrost as per manufacturer's instructions; make stock aliquots and store at −20°C for up to 18 months from date of manufacture; store unused diluted Geltrex™ Matrix solution, 1% final concentration, at 4°C for up to 1 week)
- Accutase® solution (Merck Life Science, cat. no. A6964; prepare 25 ml aliquots and store at −20°C; once defrosted, store at 4°C)
- Neural crest basal medium (see recipe, Table 1)
- Neural crest induction medium (see recipe, Table 2)
Component | Stock concentration | Final concentration | Volume for 50 ml |
---|---|---|---|
DMEM/F12 | 1× | Not applicable | 48.5 ml |
N2 supplement | 100× | 1× | 0.5 ml |
MEM non-essential amino acids solution | 100× | 1× | 0.5 ml |
GlutaMAX supplement | 100× | 1× | 0.5 ml |
- a
Penicillin-streptomycin can be added in the basal medium (final concentration 1×) to prevent bacterial contamination. Neural crest basal medium can be prepared and stored at 4°C for 1 week.
Final volume | |||||
---|---|---|---|---|---|
Component | Stock concentration | Final concentration | 10 ml | 25 ml | 50 ml |
Neural crest basal | Not applicable | Not applicable | 10 ml | 25 ml | 50 ml |
CHIR99021 | 10 mM | 1 μΜ | 1 μl | 2.5 μl | 5 μl |
DMH-1 | 10 mM | 1 μΜ | 1 μl | 2.5 μl | 5 μl |
SB 431542 | 10 mM | 2 μΜ | 2 μl | 5 μl | 10 μl |
BMP4 | 50 μg/ml | 20 ng/ml | 4 μl | 10 μl | 20 μl |
All-trans retinoic acid (only add between Day 4-Day 6)b | 1 mM | 1 μΜ | 10 μl | 25 μl | 50 μl |
- a
We recommend that neural crest induction medium is made fresh on the day of feeding. At Day 0, when hPSCs are plated into neural crest induction conditions Y-27632 2HCl, a Rho-associated coil kinase (ROCK) inhibitor, must be added at a final concentration of 10 μM to help cell attachment and increase cell survival.
- b
All-trans retinoic acid is light sensitive, so work in the dark and keep medium out of direct light.
- Biosafety cabinet
- Incubator maintained at 37°C with 95% humidified atmosphere and 5% CO2 levels
- Centrifuge (Thermo Fisher Scientific Heraeus Megafuge 1.0)
- Inverted microscope (Olympus CKX41)
- Fluorescence Olympus inverted microscope 1X53
- InCell Analyser 2200 (GE Healthcare)
- Applied Biosystems QuantStudio™ 12K Flex thermocycler (Thermo Fisher Scientific)
- BD FACSJazz (BD Biosciences)
- Neubauer Improved Hemocytometer Counting Chamber (Hawksley)
- Single channel pipets and tips
- Pipet controller and serological pipets
- Cell culture plasticware and consumables: 15-, 50-ml conical tubes; multi-well plates and T12.5 flasks; syringes; syringe filters, 0.22 µm; filtration units; glass pipets
NOTE : Tissue culture operations are carried out using aseptic technique and sterile handling.
hPSC culture and maintenance
1.Culture hPSCs in mTeSR™1 on Geltrex-coated T12.5 flasks.
2.Passage hPSC cultures every 4-5 days until they reach 80%-85% confluency using ReLeSR™, as described in the protocol provided from Stemcell Technologies.
Prior to differentiation
3.Monitor cells through an inverted microscope. Grow cultures to ∼80%-85% confluency for an optimal NC induction (Fig. 2B).
4.Before starting the differentiation, coat plates with Geltrex:
-
Thaw a Geltrex aliquot on ice to prevent unwanted gelling when handling small volumes.
-
Dilute Geltrex 1:100 in pre-chilled DMEM/F12 (4°C), i.e., 200 μl Geltrex in 20 ml DMEM/F12.
-
Transfer sufficient Geltrex solution to each well of a multi-well plate, i.e., 100-200 μl Geltrex solution per cm2is enough to coat the growth surface.
-
Incubate Geltrex-coated plates at 37°C for a minimum of 1 hr. Alternatively, coated plates can be stored at 4°C for up to 2 weeks when sealed with Parafilm to prevent them from drying out.
5.Prepare neural crest basal medium (see Table 1).
6.Prepare neural crest induction medium by supplementing neural crest basal medium with small molecules and mix thoroughly (see Table 2).
Differentiation of hPSCs into vagal NC cells/early ENS progenitors (6 days in total)
7.Day 0: Plating hPSCs for neural crest differentiation:
-
Grow hPSCs until they reach ∼80%-85% confluency.
-
Aspirate mTeSR™1 medium from the flask and add 1× PBS (200 μl/cm2) to wash cell colonies. Remove PBS.
-
Add Accutase solution (100 μl per cm2) and incubate at 37°C for 5-7 min. Observe cells under the microscope to ensure cells have detached and a single-cell suspension is obtained.
-
Neutralize Accutase solution by adding pre-warmed DMEM/F12 medium and triturate cells using a serological pipet in order to dissociate them into a single-cell suspension.
-
Transfer cell suspension to a conical centrifuge tube.
Cells are lifted up in suspension. Do not aspirate Accutase solution as this will result in losing the cell population.
-
Agitate conical tube to ensure adequate mixing and transfer 10 μl cell suspension to a hemocytometer.
-
Count cells using the hemocytometer.
-
Meanwhile centrifuge cell suspension at 300 ×gfor 4 min at room temperature.
-
After centrifugation, aspirate supernatant carefully and dislodge cell pellet in neural crest induction medium supplemented with 10 μM Y-27632 2HCl (see Table2).
We recommend resuspending cells at a ratio of 1 million cells per ml.
- Plate cells at a density of 30,000 to 40,000 cells per cm2on a Geltrex pre-coated plate in neural crest induction medium supplemented with 10 μM Y-27632 2HCl.
That is, plate 30 μl of cell suspension (∼30,000 cells) per cm2.
For a well of a 6-well plate with a surface area of ∼10 cm2, transfer 300 μl of cell suspension.
Cell density is very critical for neural crest differentiation efficiency and it is cell line dependent. We highly recommend performing cell density titration for every cell line used to ensure optimal yield. Representative images of non-NC like cells compromising the protocol's efficiency due to inefficient seeding density are displayed in Figure3.
-
Following cell plating, top up each well with neural crest induction medium supplemented with 10 μM Y-27632 2HCl to obtain a final volume of 200 μl per cm2.
-
Place cells in a 37°C incubator and move plate back and forth, side to side to ensure cells are evenly distributed throughout the growth surface.
-
Incubate cells at 37°C in a humidified atmosphere of 5% CO2in air.
Do not disturb the cells until they have attached to the bottom of the plate.
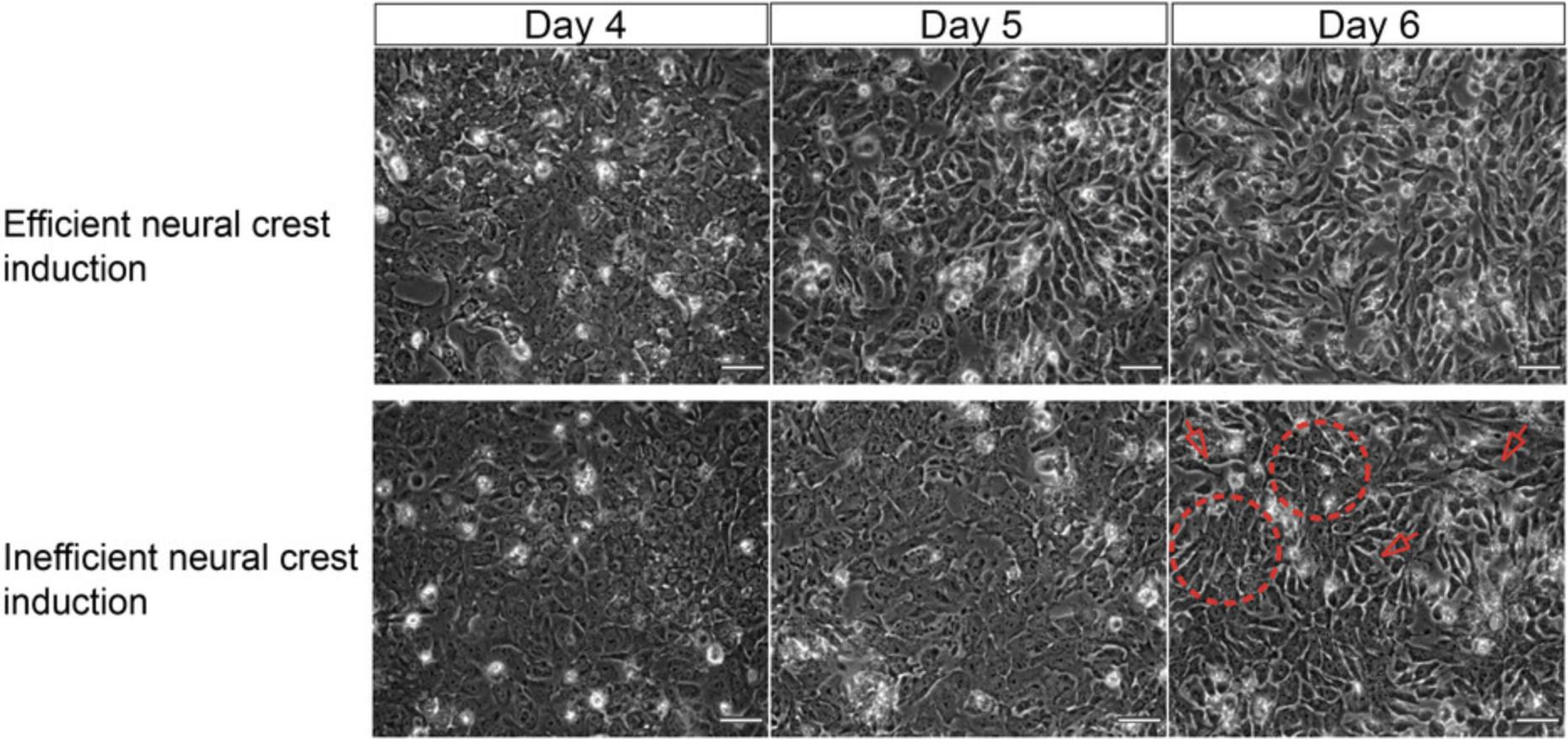
8.Day 2 (48 hr following plating):
- Feed cells by aspirating old medium and adding 200 μl freshly made neural crest induction medium without Y-27632 2HCl per cm2. Always add medium down the side wall of the well and not directly on top of the cell colonies.
By Day 2, cells form spider's-web like colonies and exhibit a spread out, spiky characteristic morphology due to treatment with Y-27632 2HCl (Fig.2B).
-
Incubate cells at 37°C for 48 hr.
9.Day 4 (vagal axial patterning and ENS specification):
-
Supplement neural crest induction medium with 1 μM all-trans retinoic acid.
-
Replace culture medium with freshly made and supplemented medium (200 μl/cm2).
Retinoic acid is extremely sensitive to light and high temperature; do not warm up medium with retinoic acid and keep plate out of light.
At Day 4, cells reach ∼95% of confluency (Fig.2B), an indicator of the success of the protocol. If cells are under confluent at this point, it is very likely that the differentiation will not work.
-
Incubate cells at 37°C for 24 hr.
10.Day 5:
- Refresh medium with 200 μl per cm2of freshly made neural crest induction medium supplemented with 1 μM all-trans retinoic acid.
We found that replacing medium at this stage to ensure sufficient levels of retinoic acid is important to maintain the expression of retinoic acid induced ENS progenitor genes ASCL1, PHOX2B.
Do not be alarmed by the increased cell death following treatment with retinoic acid; it is part of the process.
Cells begin to look darker and spiky after treatment with retinoic acid (Fig.2B).
-
Incubate cells at 37°C for 24 hr.
11.Day 6:
-
End of vagal neural crest differentiation: Cells cover 95%-100% of the growth surface forming a monolayer, appear dark and spiky (Fig.2B).
-
Cells are ready for replating in non-adherent conditions prior to the generation of enteric neurons and glia (see Basic Protocol2).
Optional: Assess vagal neural crest induction by flow cytometry, immunocytochemistry, or quantitative polymerase chain reaction (qPCR; for details about experimental techniques refer to Frith et al.,2018; Frith et al.,2020).
Understanding results
At the end of the differentiation, vagal NC cells/early ENS progenitors should form an almost confluent monolayer, exhibiting stellate-like morphology and appearing darker compared to hPSCs. The characteristic morphology of the cells as the NC protocol progresses is depicted in Figures 2B and 3.The efficiency of NC induction is indicated by the upregulation of NC-associated transcripts such as SOX10, PAX3, PAX7 , and TFAP2A as well as ENS progenitor markers (e.g., ASCL1, PHOX2B ; Fig. 2C and Table 3). The acquisition of a vagal axial identity can be verified by the marked increase in the expression HOX PG (3-5) transcripts (e.g., HOXB4, HOXB5 ; Fig. 2D). At this stage we also found that typically around 45%-65% of cells are positive for SOX10 protein following intracellular staining and analysis by flow cytometry and this SOX10+ population also co-expresses high levels of p75 (Hackland et al., 2017; Fig. 2E and Table 4) and the surface marker alpha-4 integrin (CD49D; Fattahi et al., 2016; Fig. 2E).
Gene | Forward | Reverse | Roche UPL probe |
---|---|---|---|
GAPDH | 5′-agccacatcgctcagacac-3′ | 5′-gcccaatacgaccaaatcc-3′ | # 60 |
SOX10 | 5′-ggctcccccatgtcagat-3′ | 5′-ctgtcttcggggtggttg-3′ | # 21 |
PAX3 | 5′-aggaggccgacttggaga-3′ | 5′-cttcatctgattggggtgct-3′ | # 13 |
PAX7 | 5′-gaaaacccaggcatgttcag-3′ | 5′-gcggctaatcgaactcactaa-3′ | # 66 |
ASCL1 | 5′-cgacttcaccaactggttctg-3′ | 5′-atgcaggttgtgcgatca-3′ | # 38 |
PHOX2B | 5′-ctaccccgacatctacactcg-3′ | 5′-ctcctgcttgcgaaacttg-3′ | # 17 |
TFAP2A | 5′-aacatgctcctggctacaaaa-3′ | 5′-aggggagatcggtcctga-3′ | # 62 |
HOXB1 | 5′-ccagctagggggcttgtc-3′ | 5′-atgctgcggaggatatgg-3′ | # 39 |
HOXB2 | 5′-aatccgccacgtctcctt-3′ | 5′-gctgcgtgttggtgtaagc-3′ | # 70 |
HOXB4 | 5′-ctggatgcgcaaagttcac-3′ | 5′-agcggttgtagtgaaattcctt-3′ | # 62 |
HOXB5 | 5′-aagcttcacatcagccatga-3′ | 5′-cggttgaagtggaactccttt-3′ | # 1 |
HOXB7 | 5′-ctacccctggatgcgaag-3′ | 5′-caggtagcgattgtagtgaaattct-3′ | # 1 |
HOXC9 | 5′-gcagcaagcacaaagagga-3′ | 5′-cgtctggtacttggtgtaggg-3′ | # 85 |
Antibody | Company | Catalog number | Species | Class |
---|---|---|---|---|
Anti-CD49d | BioLegend | 304302 | Mouse | Monoclonal |
Anti-SOX10 | Cell Signaling Technology | 89356 | Rabbit | Monoclonal |
Anti-p75 | Cell Signaling Technology | 8238 | Rabbit | Monoclonal |
- a
Working dilutions have to be optimized depending on antibody data using a series of dilutions in a titration experiment.
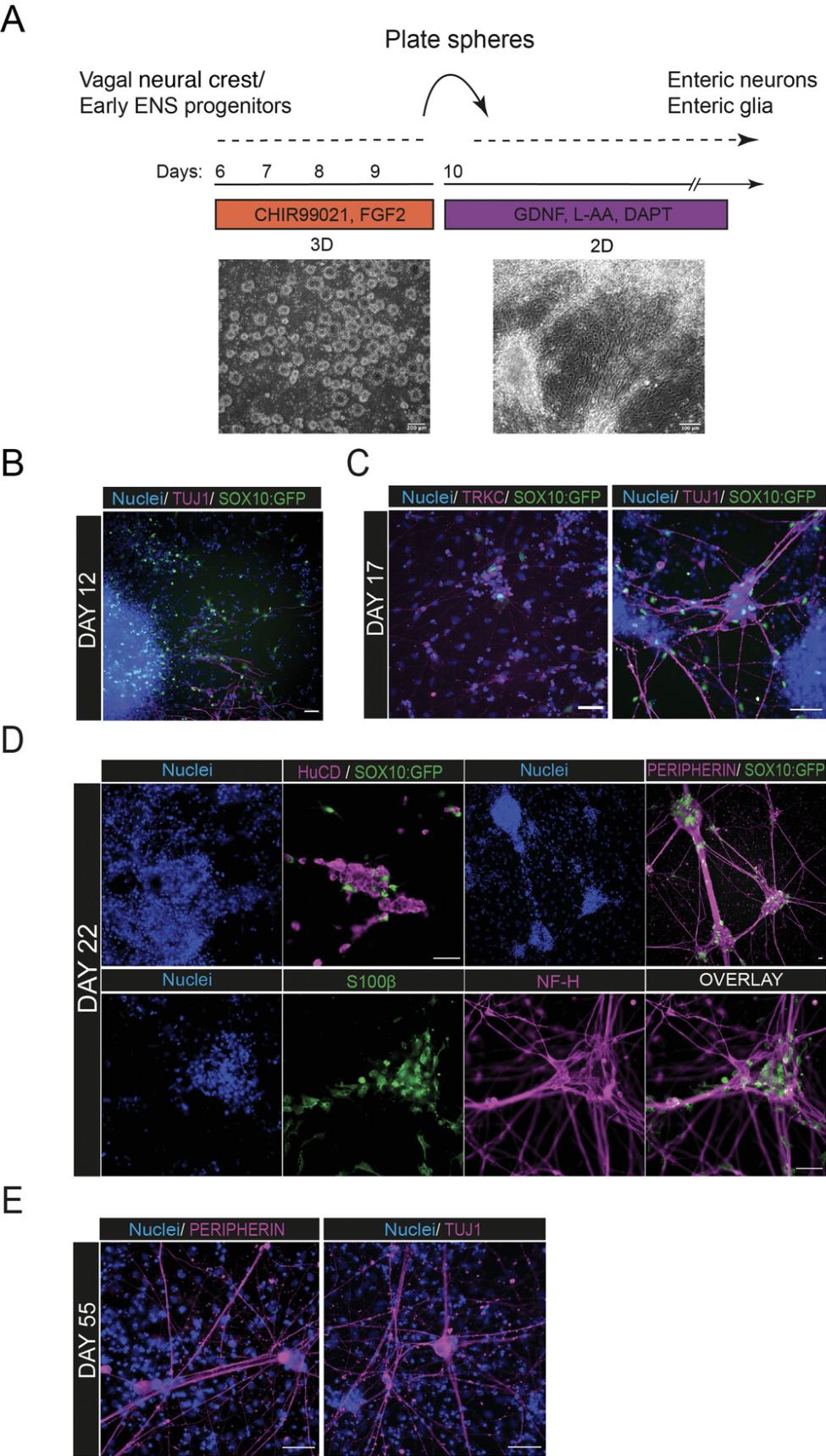
Basic Protocol 2: DIFFERENTIATION OF hPSC-DERIVED VAGAL NC/EARLY ENS PROGENITORS TO ENTERIC NEURONS AND GLIA
The protocol (Fig. 4A) continues with re-plating vagal NC cells generated from 2D monolayer differentiation into non-adherent conditions that promote formation of 3D cell sphere aggregates. These are then subsequently re-plated in adherent conditions and in the presence of neurotrophic medium to promote further differentiation toward ENS components. During that stage, SOX10+ progenitor cells migrate out of the re-plated spheres to give rise to enteric neurons and glia. The emergence of neurons, marked by downregulation of SOX10 and induction of neural markers, precedes glial occurrence, which is accompanied by sustained expression of SOX10 and upregulation of glial markers.
Materials
-
Vagal NC cells (from Basic Protocol 1)
-
Dulbecco's Modified Eagle's Medium/Nutrient Mixture F-12 Ham (Merck Life Science, cat. no. D6421; store at 4°C)
-
Neurobasal™ Medium (Thermo Fisher Scientific, cat. no. 21103049; store at 4°C)
-
Dulbecco's Phosphate Buffered Saline (Merck Life Science, cat. no. D8537; store at room temperature)
-
N2 Supplement (Thermo Fisher Scientific, cat. no. 17502048; aliquot and store at −20°C; minimize freeze-thaw cycles)
-
B27 Supplement (Thermo Fisher Scientific, cat. no. 17504001; aliquot and store at −20°C; minimize freeze-thaw cycles)
-
GlutaMAX™ Supplement (Thermo Fisher Scientific, cat. no. 35050038; store at 4°C)
-
MEM Non-Essential Amino Acids Solution (Thermo Fisher Scientific, cat. no. 11140050; store at 4°C)
-
CHIR99021 (Tocris, cat. no. 4423; aliquot and store at −20°C; minimize freeze-thaw cycles)
-
Recombinant Human FGF basic (146 aa) protein CF (R&D Systems, cat. no. 233-FB/CF; reconstitute powder following manufacturer's instructions, aliquot and freeze at −20°C; minimize freeze-thaw cycles)
-
5,000 U/ml Penicillin-Streptomycin (Thermo Fisher Scientific, cat. no. 15070063; aliquot and store at −20°C as per manufacturer's instructions
-
Y-27632 2HCl (AdooQ Bioscience, cat. no. A11001; aliquot and store at −20°C; minimize freeze-thaw cycles)
-
Accutase® solution (Merck Life Science, cat. no. A6964; make 25 ml aliquots and store at −20°C; once defrosted, store at 4°C)
-
Sphere culture basal medium (see recipe, Table 5)
-
Complete sphere culture medium (see recipe, Table 6)
-
BrainPhys™ Neuronal Medium (Stemcell Technologies, cat. no. 05790; store at 4°C)
-
Recombinant human glial cell-derived neurotrophic factor (GDNF; PeproTech, cat. no. 450-10; reconstitute powder as per manufacturer's instructions, aliquot, and store at −20°C; minimize freeze-thaw cycles)
-
(2 S)-N -[(3,5-Difluorophenyl)acetyl]-L-alanyl-2-phenyl]glycine 1,1-dimethylethyl ester (DAPT; γ-secretase inhibitor; Tocris, cat. no. 2634; aliquot and store at −20°C; minimize freeze-thaw cycles)
-
L-Ascorbic acid 2-phosphate sesquimagnesium salt (Santa Cruz Biotechnology, cat. no. sc-228390; reconstitute powder as per manufacturer's instructions, aliquot, and store at −20°C; minimize freeze-thaw cycles)
-
Vitronectin (VTN-N) Recombinant Human Protein, Truncated (Thermo Fisher Scientific, cat. no. A14700; prepare 100 μl aliquots and store at −80°C)
-
Geltrex™ LDEV-Free Reduced Growth Factor Basement Membrane Matrix (Thermo Fisher Scientific, cat. no. A1413202; defrost as per manufacturer's instructions; make stock aliquots and store at −20°C for up to 18 months from date of manufacture; store unused diluted GeltrexTM Matrix solution, 1% final concentration, at 4°C for up to 1 week)
-
Poly-L-ornithine solution (Merck Life Science, cat. no. P4957; store at 4°C; dilute 0.1mg/ml poly-L-ornithine stock solution with sterile cell culture grade water to yield 20 µg/ml for plastic and 50 µg/ml for glass surfaces)
-
Cell culture grade water
-
ENS basal medium (see recipe, Table 7)
-
ENS complete culture medium (see recipe, Table 8)
-
Corning™ Costar™ Ultra-Low Attachment 6-well Microplates 3471 (Thermo Fisher Scientific, cat. no. 10154431)
-
Biosafety cabinet
-
Incubator maintained at 37°C with 95% humidified atmosphere and 5% CO2 levels
-
Centrifuge (Thermo Fisher Scientific Heraeus Megafuge 1.0)
-
Inverted microscope (Olympus CKX41)
-
Fluorescence Olympus inverted microscope 1X53
-
InCell Analyser 2200 (GE Healthcare)
-
Single channel pipets and tips
-
Pipet controller and serological pipets
-
Cell culture plasticware and consumables: 15-, 50-ml conical tubes; multi-well plates; syringes; syringe filters, 0.22 µm; filtration units; glass pipets
Prior to sphere formation
1.Prepare sphere basal culture medium (see Table 5).
Component | Stock concentration | Final concentration | Volume for 50 ml |
---|---|---|---|
DMEM/F12 | 1× | 0.5× | 23.75 ml |
Neurobasal | 1× | 0.5× | 23.75 ml |
B27 supplement | 50× | 1× | 1 ml |
N2 supplement | 100× | 1× | 0.5 ml |
MEM non-essential amino acids solution | 100× | 1× | 0.5 ml |
GlutaMAX supplement | 100× | 1× | 0.5 ml |
- a
Penicillin-streptomycin can be added in the basal medium (final concentration 1×). Sphere basal medium can be prepared and stored at 4°C for 1 week.
Final volume | |||||
---|---|---|---|---|---|
Component | Stock concentration | Final concentration | 10 ml | 25 ml | 50 ml |
Sphere basal | Not applicable | Not applicable | 10 ml | 25 ml | 50 ml |
CHIR99021 | 10 mM | 3 μΜ | 3 μl | 7.5 μl | 15 μl |
Recombinant human FGF2 | 10 μg/ml | 10 ng/ml | 10 μl | 25 μl | 50 μl |
Y-27632 2HCl | 10 mM | 10 μΜ | 10 μl | 25 μl | 50 μl |
- a
We recommend using freshly made medium for sphere generation. Complete sphere culture medium is supplemented with Y-27632 2HCl, a Rho-associated coil kinase (ROCK) inhibitor which increases cell survival and improves formation of spheres (especially after breaking).
Component | Stock concentration | Final concentration | Volume for 50 ml |
---|---|---|---|
BrainPhys™ Neuronal Medium | 1× | Not applicable | 47.5 ml |
B27 supplement | 50× | 1× | 1 ml |
N2 supplement | 100× | 1× | 0.5 ml |
MEM non-essential amino acids solution | 100× | 1× | 0.5 ml |
GlutaMAX supplement | 100× | 1× | 0.5 ml |
- a
Penicillin-streptomycin can be added in the basal medium (final concentration 1×). ENS basal medium can be prepared and stored at 4°C for 1 week.
Final volume | |||||
---|---|---|---|---|---|
Component | Stock concentration | Final concentration | 10 ml | 25 ml | 50 ml |
ENS basal | Not applicable | Not applicable | 10 ml | 25 ml | 50 ml |
Recombinant human GDNF | 10 μg/ml | 10 ng/ml | 10 μl | 25 μl | 50 μl |
DAPT | 10 mM | 10 μΜ | 10 μl | 25 μl | 50 μl |
Ascorbic acid | 100 mM | 100 μΜ | 10 μl | 25 μl | 50 μl |
- a
Make ENS complete medium fresh before use. No ROCK inhibitor is added at the final plating.
2.Prepare complete sphere culture medium (see Table 6).
Sphere formation (3-4 days in total)
3.Day 6 (re-plating vagal neural crest into non-adherent conditions):
-
Aspirate medium from Day 6 vagal neural crest cells/early ENS progenitors carefully to avoid disturbing the monolayer on the bottom of the well.
-
Perform a quick wash with 1× PBS.
-
Lift cells into single-cell suspension by adding Accutase solution (100 μl per cm2) and incubate at 37°C for 5-7 min.
-
Neutralize Accutase solution with pre-warmed DMEM/F12 and using a serological pipet, triturate cell suspension a few times to ensure complete cell dissociation.
Do not aspirate Accutase solution at this step, as it contains cells that have been detached.
No need for counting cells; cells are plated down at a 1:1 ratio, e.g., the equivalent of a well of a 6-well plate is transferred into a well of an ultra-low attachment plate.
-
Transfer cell suspension into a conical tube.
-
Place conical tube in a centrifuge and spin at 300 ×gfor 4 min at room temperature.
-
Aspirate and discard supernatant very carefully without disturbing cell pellet.
-
Resuspend pellet in complete sphere culture medium (see Table6).
For example: When a full 6-well plate of vagal neural crest cells/early ENS progenitors is harvested for sphere formation, the resulting cell pellet is resuspended in 12 ml complete sphere medium and 2 ml of cell suspension is transferred per well for subsequent plating.
- Transfer cell suspension to each well of the 6-well ultra-low attachment plate.
That is, 2 ml of cell suspension transferred into each well of the non-adherent 6-well plate.
Be gentle while transferring cells to the plate; avoid scraping the bottom of the well with the pipet tip.
- Place plates into a plate holder and centrifuge at 100 ×gfor 2 min at room temperature.
This step collates single cells in the middle of the well to increase cell-cell contact and assist sphere formation.
Following centrifugation, cell suspension is visible at the center of the well.
- Incubate cells at 37°C for 24 hr.
Do not disturb plates for the first 24 hr.
4.Day 7 (24 hr after plating):
- Inspect cultures visually using an inverted microscope.
Floating cell aggregates appear in culture. They are quite fragile without well-defined spherical shape yet.
Do not be alarmed by the increased cell death or number of single cells floating; many of them will eventually form spheres in the next 24 hr.
-
Place plates in the incubator for another 24 hr.
5.Day 8 (48 hr after plating):
-
Spheres should be visible at this stage and have a better and/or round shape compared to Day 7 (Fig.4A).
-
Perform half medium change to supplement cells with fresh medium (see Table6).
1.Swirl plate to collect spheres from the circumference to the center of the well. 2.Hold plate at an angle towards you and let spheres settle to form sediment at the bottom corner of the well. 3.Remove and discard 1 ml medium very carefully by placing the tip of a P1000 pipet at an angle facing the side of each well.
Do not aspirate all medium, as this increases the risk of losing cells, too.
Do not use a vacuum aspirator to perform medium change at this point; the suction force is likely to cause sphere loss.
- c.Incubate plates at 37°C for 24 hr.
6.Day 9 and Day 10:
-
Spheres are re-plated into neurotrophic medium for further differentiation towards ENS derivatives.
-
If spheres have fused, forming large irregularly shaped aggregates, we prefer to break them up into smaller spheres and culture them for an additional day prior to culture in neurotrophic medium (see Troubleshooting, Table10).
Transfer spheres to a 50-ml conical tube and triturate sphere suspension two to three times by pipetting towards the side of the tube. We advise using a serological pipet with wide tip opening, i.e., 10-ml stripette which is less harsh for the spheres.
Gentle handling of spheres is highly recommended; do not break the spheres too much, as this will result in excessive cell death.
Problem | Possible cause | Solution |
---|---|---|
Poor cell plating |
Geltrex solution is overdiluted or has dried out or is not well dispersed across the well Cells are overgrown prior to NC induction |
Increase Geltrex concentration Ensure growth surface area is fully coated with Geltrex solution and it has not dried out Harvest cells from a less confluent flask at Day 0 |
Cells are not dispersed uniformly on the surface of the well following plating | Inadequate agitation to distribute cells | Tap plate gently in a “North, South, East, West” direction to disperse cells evenly across the surface of the well |
Poor NC induction as determined by p75/SOX10 or CD49d/SOX10 through flow cytometry analysis |
Cell cultures are too dense or not dense enough Bad quality small molecules/reagents Not precise levels of BMP signaling Poor quality hPSCs |
Determine optimal seeding density at Day 0 (beginning of the protocol) Prepare fresh NC medium and minimize freeze-thaw cycles of small molecules Titrate concentration of BMP4 and DMH-1 inhibitor for an optimal BMP signaling activity Assess quality of hPSCs |
Inadequate vagal axial patterning as determined by expression of posterior HOX genes |
Infrequent medium change with all-trans retinoic acid Low all-trans retinoic acid activity |
Replace all-trans retinoic acid and ensure it is stored appropriately |
Inadequate ENS specification on vagal NC determined by the mRNA levels of ASCL1, PHOX2B |
Infrequent medium change with all-trans retinoic acid Low all-trans retinoic acid activity |
Refresh medium at Day 5 supplemented with all-trans retinoic acid |
Free floating spheres form big “tube-like” structures | Increased cell numbers/well | Triturate cell aggregates using a 10-ml serological pipet by pipetting 2-3 times to break them up into small spheres |
No neurons observed |
Absence of small molecules or low activity of growth factors Poor induction of vagal NC/early ENS progenitors Spheres are broken up too much prior to sphere plating Not enough spheres/well |
Prepare fresh ENS medium and minimize freeze-thaw cycles of small molecules Monitor/assess differentiation efficiency of previous steps at Day 6 and Day 9/10 Determine optimal sphere resuspension volume prior to plating for enteric neuron differentiation; test different volumes/sphere densities for plating |
Prior to sphere plating
7.Prepare poly-L-ornithine/Geltrex coated plates:
-
Coat plastic growth surface with 15 μg/ml poly-L-ornithine solution prepared in cell culture grade water (50 μg/ml poly-L-ornithine solution is recommended for glass surfaces); 200 μl per cm2is enough to cover the growth area. Incubate plates for a minimum of 1 hr or overnight at 37°C.
-
Wash plates thoroughly twice using 1× PBS.
-
Aspirate 1× PBS and coat each well of the plate with diluted Geltrex solution (1:100) as previously shown (Basic Protocol1).
-
Let plates stand for 1 hr at 37°C.
8.Prepare ENS basal medium (see Table 7).
9.Prepare ENS complete medium (see Table 8).
Antibody | Company | Catalog number | Species | Class |
---|---|---|---|---|
Anti-beta III Tubulin (TuJ1) | Abcam | ab78078 | Mouse | Monoclonal |
Anti-TrkC | Cell Signaling Technology | 3376 | Rabbit | Monoclonal |
Anti-peripherin | MilliporeSigma | AB1530 | Rabbit | Polyclonal |
Anti-neurofilament heavy polypeptide (NF-H) | Abcam | ab8135 | Rabbit | Polyclonal |
Anti-S100 (β-subunit) | MilliporeSigma | S2532 | Mouse | Monoclonal |
Anti-HuC/HuD | Invitrogen | A-21271 | Mouse | Monoclonal |
- a
Working dilutions have to be optimized depending on antibody data using a series of dilutions in a titration experiment.
Plating spheres into ENS induction medium (12 days and beyond)
10.Day 9 and Day 10:
-
Swirl plates and group spheres together at the center of the well.
-
Collect spheres in a 50-ml conical tube using a 10-ml serological pipet.
Sphere collection requires gentle handling to avoid breaking up the spheres, resulting in single-cell suspension and concomitant reduced survival.
-
Let spheres settle to the bottom of the tube by gravity sedimentation for 5 min at room temperature before proceeding to step d.
-
When the supernatant is clear and no cell aggregates are observed within the solution, carefully remove and discard medium without disturbing sphere pellet.
Aspirating medium with a serological pipet or a P1000 pipet is highly recommended as the suction force of an aspiration pump will disturb the pellet and result in sphere loss.
- Resuspend sphere pellet using a 10-ml serological pipet in ENS complete culture medium (see Table8) and distribute sphere suspension to each well.
One well of spheres is resuspended in 3 ml of ENS complete culture medium and 1 ml of sphere suspension is transferred to a well of a 12-well plate for subsequent enteric neuron differentiation. For a 24-well plate, 0.5 ml of sphere suspension is transferred per well.
The differentiation is much more robust when the cells are plated at a very high density and deposited as whole spheres.
-
Move plates in a back-forth, right-left direction to distribute spheres evenly.
-
Incubate plates at 37°C for 48 hr.
Do not disturb plates for the first 24 hr.
After 24 hr, spheres have attached to the culture vessel and cells start migrating out of them.
11.Day 11 and Day 12 (48 hr after plating spheres) and for the rest of the differentiation:
-
Perform half medium change every other day by removing half of the old medium from each well and replacing it with freshly made ENS complete culture medium.
-
Incubate cells at 37°C in a humidified atmosphere of 5% CO2in air.
Increased cell death is expected during this stage of the differentiation. However, the wells should remain confluent to allow neural induction to occur otherwise the differentiation will fail.
Cells could be maintained in culture under ENS conditions for extended periods to allow further maturation. To date, cells have been successfully cultured in ENS medium up to day 100.
- After day 30-35, perform half medium change biweekly (every 3-4 days) and increase medium volume from 200 μl/cm2to 400 μl/cm2.
If cells start dethatching or axons seem to wobble with plate movement, supplement medium with vitronectin (1:100) once a week.
Understanding results
The neurons tend to form at regions of high density across the well, converge, and create big clumps of neuronal cell bodies that are interconnected with axonal processes that span the whole well (Fig. 4). Neurons first appear and migrate out of the spheres around day 12 of the protocol (2 days after culture in neurotrophic medium; Fig. 4B). Between day 17-22, neural fibers are visible and cells express pan-neuronal markers such as neurofilament heavy chain (NF-H), HuCD, TUJ1, PERIPHERIN, and TRKC (Fig. 4C and D), markers widely used in the literature for enteric neuron characterization (Table 9). Glial cells expressing S100β and SOX10 emerge around day 20-22 and grow in close vicinity to the neuronal cell bodies (Fig. 4D). At this stage, the cell cultures are comprised of a mixture of neural and glial cells that differentiate and mature further as the protocol progresses (Fig. 4E). For a more detailed expression analysis of the generated neurons, refer to the original study of Frith et al. (2020). We regularly observe emergence of contaminant cells that lack the characteristic neuronal morphology and are not immunoreactive to ENS lineage markers (ASCL1 , PHOX2B , SOX10). These do not appear to influence negatively the induction of neurons and/or glia.
REAGENTS AND SOLUTIONS
Complete sphere culture medium
- See Table 6.
Enteric nervous system (ENS) basal medium
- See Table 7.
Enteric nervous system (ENS) complete culture medium
- See Table 8.
Neural crest basal medium
- See Table 1.
Neural crest induction medium
- See Table 2.
Sphere culture basal medium
- See Table 5.
COMMENTARY
Background Information
The in vitro generation of enteric neural progenitors from hPSCs holds a great promise for the development of cell-therapy-based approaches and the study of the specification of ENS lineages and hence has attracted a considerable amount of research interest. To date, differentiation strategies have either implemented embryoid body formation and culture of hPSC in the presence of EGF, FGF signals (Li et al., 2018; Workman et al., 2017) or employed a monolayer differentiation approach that relies on transforming growth factor β (TGF-β) signaling suppression, bone morphogenetic protein (BMP) signaling regulation and WNT pathway stimulation to generate an NC-like population (Barber et al., 2019; Lau et al., 2019). Patterning of the in vitro derived NC to a vagal axial identity is routinely achieved by retinoic acid (RA) addition (Barber et al., 2019; Lau et al., 2019; Workman et al., 2017) while further commitment along the ENS trajectory has been mediated by co-culture with intestinal (Workman et al., 2017)/colonic organoids (Lau et al., 2019), gut tissue explants (Li et al., 2018), or further differentiation following culture in neurotrophic medium (Barber et al., 2019; Lau et al., 2019).
The protocol described here outlines an efficient method of directed differentiation that generates ENS progenitors from hPSCs via a vagal NC induction step following RA treatment. The derivation of NC relies on the combined WNT signaling stimulation and TFG-β pathway inhibition together with precise levels of BMP signaling. The latter is achieved by the introduction of saturating levels of exogenous BMP which is titrated by the simultaneous addition of a BMP receptor inhibitor (DMH-1) to counteract the effects of variable endogenous BMP production and the concomitant variations in NC induction (Hackland et al., 2017). The resulting population, which displays a cranial NC character (Frith et al., 2018), is then further differentiated toward a vagal NC/early ENS progenitor state following exposure to RA in a dose-dependent fashion (Frith et al., 2020). The obtained ENS precursor population is marked by the expression of ASCL1 , PHOX2B , SOX10 , p75, and CD49d. This approach is quicker than previously published protocols that yield equivalent ENS progenitors after 10 to 15 days of hPSC differentiation (Fattahi et al., 2016; Workman et al., 2017) and crucially the ENS progenitors can colonize the ENS of adult mice following transplantation (Frith et al., 2020). They can also be employed for the subsequent generation of electrophysiologically active enteric neurons in vitro without the requirement for an in vivo physical niche and instructive gut-derived signals.
Critical Parameters
Directed differentiation of hPSCs can be very challenging and often results in a great variability in the protocol's yield among independent replicates or no induction at all (Fig. 3). We found that an inefficient vagal NC differentiation is likely to reflect a poor quality hPSC starting population, a non-optimal seeding density, or sometimes could be due to inadequate medium change and the accompanied depletion of medium components essential for the progression of the differentiation. Thus, it is very important that attention should be paid to these critical parameters, as stated below, for a successful differentiation outcome.
Quality of hPSC cultures
Culture of hPSCs should be performed following good laboratory practice guidelines.
Cultures should be monitored for bacterial and fungal contamination and should be routinely examined for mycoplasma. To avoid contamination, antibiotics such as gentamycin or penicillin-streptomycin could be added in the culture medium. Cell cultures should be examined regularly for morphological changes that indicate unwanted differentiation. Spontaneously differentiated cells grown in hPSC cultures impair differentiation efficiency and therefore, their presence should be eliminated by manual scraping. Screening for markers such as NANOG, OCT4, SOX2 using qPCR or surface proteins SSEA4, SSEA3, TRA-1-60, through flow cytometry, or immunostaining is highly recommended to assess the presence of undifferentiated hPSCs (Thomson et al., 1998; Wright & Andrews, 2009). Regular karyotyping of hPSCs should also be employed to determine genetic aberrations that might occur during propagation of stem cell cultures (Baker et al., 2016). We routinely culture hPSCs on Geltrex or vitronectin coated flasks using mTeSR™1 or Essential 8 medium and use ReLeSR™ as passaging reagent. Alternative maintenance conditions have not been tested and may affect the differentiation efficiency of the current protocol. We found that passaging cells using EDTA- based dissociation solution yields lower NC efficiency.
Seeding density
Seeding density determines NC efficiency in a cell-line dependent manner. Therefore, it is highly recommended that plating densities for NC induction are optimized for each cell line/end user.
Medium change
Induction and maintained expression of ENS progenitor genes (ASCL1, PHOX2B) depends on retinoic acid addition in NC medium. We found that daily medium replacement with retinoic acid is required to retain high ASCL1, PHOX2B transcript levels.
Troubleshooting
See Table 10 for common problems encountered when performing these protocols and suggested solutions.
Time Considerations
Basic Protocol 1: 6 days.
Basic Protocol 2: 16 days or more. (To date, we have kept enteric neurons alive until day 100 but we have not extended culture past this time point).
Acknowledgments
This work is supported by the European Union Horizon 2020 Framework Programme ((H2020-EU.1.2.2; grant agreement ID 824070) and the Medical Research Council (MR/V002163/1).
Author Contributions
Antigoni Gogolou: Investigation, Methodology, Writing-original draft, Writing-review and editing, Thomas J.R. Frith: Conceptualization, Investigation, Methodology, Writing-review and editing, Anestis Tsakiridis: Conceptualization, Funding acquisition, Investigation, Supervision, Writing-review & editing.
Conflict of Interest
The authors declare no conflict of interest.
Open Research
Data Availability Statement
The data that support the findings of this study are available on request from the corresponding author. The data are not publicly available due to privacy or ethical restrictions.
Literature Cited
- Anderson, R. B., Stewart, A. L., & Young, H. M. (2006). Phenotypes of neural-crest-derived cells in vagal and sacral pathways. Cell and Tissue Research , 323(1), 11–25. doi: 10.1007/s00441-005-0047-6.
- Baker, D., Hirst, A. J., Gokhale, P. J., Juarez, M. A., Williams, S., Wheeler, M., … Barbaric, I. (2016). Detecting genetic mosaicism in cultures of human pluripotent stem cells. Stem Cell Reports , 7(5), 998–1012. doi: 10.1016/j.stemcr.2016.10.003.
- Barber, K., Studer, L., & Fattahi, F. (2019). Derivation of enteric neuron lineages from human pluripotent stem cells. Nature Protocols , 14(4), 1261–1279. doi: 10.1038/s41596-019-0141-y.
- Burke, A. C., Nelson, C. E., Morgan, B. A., & Tabin, C. (1995). Hox genes and the evolution of vertebrate axial morphology. Development , 121(2), 333–346. doi: 10.1242/dev.121.2.333.
- Burns, A. J., & Thapar, N. (2014). Neural stem cell therapies for enteric nervous system disorders. Nature Reviews Gastroenterology & Hepatology, 11(5), 317–328. doi: 10.1038/nrgastro.2013.226.
- Chambers, S. M., Qi, Y., Mica, Y., Lee, G., Zhang, X. J., Niu, L., … Studer, L. (2012). Combined small-molecule inhibition accelerates developmental timing and converts human pluripotent stem cells into nociceptors. Nature Biotechnology , 30(7), 715–720. doi: 10.1038/nbt.2249.
- Chan, K. K., Chen, Y. S., Yau, T. O., Fu, M., Lui, V. C., Tam, P. K., & Sham, M. H. (2005). Hoxb3 vagal neural crest-specific enhancer element for controlling enteric nervous system development. Developmental Dynamics , 233(2), 473–483. doi: 10.1002/dvdy.20347.
- Fattahi, F., Steinbeck, J. A., Kriks, S., Tchieu, J., Zimmer, B., Kishinevsky, S., … Studer, L. (2016). Deriving human ENS lineages for cell therapy and drug discovery in Hirschsprung disease. Nature , 531(7592), 105–109. doi: 10.1038/nature16951.
- Frith, T. J., Granata, I., Wind, M., Stout, E., Thompson, O., Neumann, K., … Tsakiridis, A. (2018). Human axial progenitors generate trunk neural crest cells in vitro. Elife , 7. doi: 10.7554/eLife.35786.
- Frith, T. J. R., Gogolou, A., Hackland, J. O. S., Hewitt, Z. A., Moore, H. D., Barbaric, I., … McCann, C. J. (2020). Retinoic acid accelerates the specification of enteric neural progenitors from in-vitro-derived neural crest. Stem Cell Reports , 15(3), 557–565. doi: 10.1016/j.stemcr.2020.07.024.
- Fu, M., Lui, V. C., Sham, M. H., Cheung, A. N., & Tam, P. K. (2003). HOXB5 expression is spatially and temporarily regulated in human embryonic gut during neural crest cell colonization and differentiation of enteric neuroblasts. Developmental Dynamics , 228(1), 1–10. doi: 10.1002/dvdy.10350.
- Furness, J. B. (2006). The enteric nervous system, Malden, Massachusetts: Blackwell Publishing.
- Furness, J. B. (2012). The enteric nervous system and neurogastroenterology. Nature Reviews Gastroenterology & Hepatology, 9(5), 286–294. doi: 10.1038/nrgastro.2012.32.
- Hackland, J. O. S., Frith, T. J. R., Thompson, O., Marin Navarro, A., Garcia-Castro, M. I., Unger, C., & Andrews, P. W. (2017). Top-down inhibition of BMP signaling enables robust induction of hPSCs into neural crest in fully defined, Xeno-free conditions. Stem Cell Reports , 9(4), 1043–1052. doi: 10.1016/j.stemcr.2017.08.008.
- Heanue, T. A., & Pachnis, V. (2007). Enteric nervous system development and Hirschsprung's disease: advances in genetic and stem cell studies. Nature Reviews Neuroscience , 8(6), 466–479. doi: 10.1038/nrn2137.
- Hutchins, E. J., Kunttas, E., Piacentino, M. L., Howard, A. G. A. t., Bronner, M. E., & Uribe, R. A. (2018). Migration and diversification of the vagal neural crest. Developmental Biology , 444(Suppl 1), S98–S109. doi: 10.1016/j.ydbio.2018.07.004.
- Kam, M. K., & Lui, V. C. (2015). Roles of Hoxb5 in the development of vagal and trunk neural crest cells. Development Growth and Differentiation , 57(2), 158–168. doi: 10.1111/dgd.12199.
- Kang, Y. N., Fung, C., & Vanden Berghe, P. (2021). Gut innervation and enteric nervous system development: a spatial, temporal and molecular tour de force. Development , 148(3), dev182543. doi: 10.1242/dev.182543.
- Lai, F. P., Lau, S. T., Wong, J. K., Gui, H., Wang, R. X., Zhou, T., … Ngan, E. S. (2017). Correction of Hirschsprung-associated mutations in human induced pluripotent stem cells via clustered regularly interspaced short palindromic repeats/Cas9, restores neural crest cell function. Gastroenterology , 153(1), 139–153.e8. doi: 10.1053/j.gastro.2017.03.014.
- Lake, J. I., & Heuckeroth, R. O. (2013). Enteric nervous system development: migration, differentiation, and disease. American Journal of Physiology. Gastrointestinal and Liver Physiology , 305(1), G1–24. doi: 10.1152/ajpgi.00452.2012.
- Lau, S. T., Li, Z., Pui-Ling Lai, F., Nga-Chu Lui, K., Li, P., Munera, J. O., … Ngan, E. S. (2019). Activation of hedgehog signaling promotes development of mouse and human enteric neural crest cells, based on single-cell transcriptome analyses. Gastroenterology , 157(6), 1556–1571.e5. doi: 10.1053/j.gastro.2019.08.019.
- Le Douarin, N. M., Creuzet, S., Couly, G., & Dupin, E. (2004). Neural crest cell plasticity and its limits. Development , 131(19), 4637–4650. doi: 10.1242/dev.01350.
- Le Douarin, N. M., & Teillet, M. A. (1973). The migration of neural crest cells to the wall of the digestive tract in avian embryo. Journal of Embryology and Experimental Morphology , 30(1), 31–48. doi: 10.1242/dev.30.1.31.
- Li, W., Huang, L., Zeng, J., Lin, W., Li, K., Sun, J., … Xiang, A. P. (2018). Characterization and transplantation of enteric neural crest cells from human induced pluripotent stem cells. Molecular Psychiatry , 23(3), 499–508. doi: 10.1038/mp.2016.191.
- Niederreither, K., Vermot, J., Le Roux, I., Schuhbaur, B., Chambon, P., & Dolle, P. (2003). The regional pattern of retinoic acid synthesis by RALDH2 is essential for the development of posterior pharyngeal arches and the enteric nervous system. Development , 130(11), 2525–2534. doi: 10.1242/dev.00463.
- Obermayr, F., Hotta, R., Enomoto, H., & Young, H. M. (2013). Development and developmental disorders of the enteric nervous system. Nature Reviews Gastroenterology & Hepatology, 10(1), 43–57. doi: 10.1038/nrgastro.2012.234.
- Schlieve, C. R., Fowler, K. L., Thornton, M., Huang, S., Hajjali, I., Hou, X., … Grikscheit, T. C. (2017). Neural crest cell implantation restores enteric nervous system function and alters the gastrointestinal transcriptome in human tissue-engineered small intestine. Stem Cell Reports , 9(3), 883–896. doi: 10.1016/j.stemcr.2017.07.017.
- Simkin, J. E., Zhang, D., Rollo, B. N., & Newgreen, D. F. (2013). Retinoic acid upregulates ret and induces chain migration and population expansion in vagal neural crest cells to colonise the embryonic gut. PLoS One , 8(5), e64077. doi: 10.1371/journal.pone.0064077.
- Stamp, L. A. (2017). Cell therapy for GI motility disorders: comparison of cell sources and proposed steps for treating Hirschsprung disease. American Journal of Physiology. Gastrointestinal and Liver Physiology , 312(4), G348–G354. doi: 10.1152/ajpgi.00018.2017.
- Thomson, J. A., Itskovitz-Eldor, J., Shapiro, S. S., Waknitz, M. A., Swiergiel, J. J., Marshall, V. S., & Jones, J. M. (1998). Embryonic stem cell lines derived from human blastocysts. Science , 282(5391), 1145–1147. doi: 10.1126/science.282.5391.1145.
- Uribe, R. A., Hong, S. S., & Bronner, M. E. (2018). Retinoic acid temporally orchestrates colonization of the gut by vagal neural crest cells. Developmental Biology , 433(1), 17–32. doi: 10.1016/j.ydbio.2017.10.021.
- Workman, M. J., Mahe, M. M., Trisno, S., Poling, H. M., Watson, C. L., Sundaram, N., … Wells, J. M. (2017). Engineered human pluripotent-stem-cell-derived intestinal tissues with a functional enteric nervous system. Nature Medicine , 23(1), 49–59. doi: 10.1038/nm.4233.
- Wright, A. J., & Andrews, P. W. (2009). Surface marker antigens in the characterization of human embryonic stem cells. Stem Cell Research , 3(1), 3–11. doi: 10.1016/j.scr.2009.04.001.
Citing Literature
Number of times cited according to CrossRef: 6
- Robert O. Heuckeroth, Hirschsprung Disease, Pediatric Neurogastroenterology, 10.1007/978-3-031-15229-0_26, (355-370), (2023).
- Jesse Gardner-Russell, Jakob Kuriakose, Marlene M. Hao, Lincon A. Stamp, Upper Gastrointestinal Motility, Disease and Potential of Stem Cell Therapy, The Enteric Nervous System II, 10.1007/978-3-031-05843-1_29, (319-328), (2023).
- Robert L. Drury, New and Emerging Technologies for Integrative Ambulatory Autonomic Assessment and Intervention as a Catalyst in the Synergy of Remote Geocoded Biosensing, Algorithmic Networked Cloud Computing, Deep Learning, and Regenerative/Biomic Medicine: Further Real, Autonomic Nervous System - Special Interest Topics, 10.5772/intechopen.104092, (2022).
- Regina Luttge, Nanofabricating neural networks: Strategies, advances, and challenges, Journal of Vacuum Science & Technology B, 10.1116/6.0001649, 40 , 2, (020801), (2022).
- Dosh Whye, Delaney Wood, Kristina H. Kim, Cidi Chen, Nina Makhortova, Mustafa Sahin, Elizabeth D. Buttermore, Dynamic 3D Combinatorial Generation of hPSC‐Derived Neuromesodermal Organoids With Diverse Regional and Cellular Identities, Current Protocols, 10.1002/cpz1.568, 2 , 10, (2022).
- Noémi Császár, Istvan Bokkon, Hypnotherapy and IBS: Implicit and Simple Stress Memory in ENS?, SSRN Electronic Journal, 10.2139/ssrn.4148409.