Mouse Model of Chronic Social Stress-Induced Excessive Pavlovian Aversion Learning-Memory
Hannes Sigrist, Hannes Sigrist, David E. Hogg, David E. Hogg, Alena Senn, Alena Senn, Christopher R. Pryce, Christopher R. Pryce
Abstract
Increased experience of aversive stimuli/events is a psychological-neurobiological state of major importance in psychiatry. It occurs commonly in generalized anxiety disorder, post-traumatic stress disorder, and major depression. A sustained period of exposure to threat (chronic stressor) is a common risk factor, and a major symptom is generalized excessive perception of, and reactivity to, aversive stimuli. In rodents, Pavlovian aversion learning and memory (PAL, PAM), quantified in terms of the conditioned defensive behavior freezing, is an extensively studied behavioral paradigm, and well understood in terms of underlying neural circuitry. In mice, chronic social stress (CSS) is a 15-day resident-intruder paradigm in which C57BL/6 adult males are exposed continuously and distally to dominant-aggressive CD-1 male mice (sustained threat) interspersed with a brief daily period of proximal attack (acute threat). To ensure that physical wounding is minimized, proximal attacks are limited to 30 to 60 s/day and lower incisor teeth of CD-1 mice are blunted. Control (comparison) mice are maintained in littermate pairs. The CSS and CD-1 mice are maintained in distal contact during subsequent behavioral testing. For PAL, CSS and control (CON) mice are placed in a conditioning chamber (context) and exposed to a tone [conditioned stimulus (CS)] and mild, brief foot shock [unconditioned stimulus (US)]. For PAM, mice are placed in the same context and presented with CS repetitions. The CSS mice acquire (learn) and express (memory) a higher level of freezing than CON mice, indicating that CSS leads to generalized hypersensitivity to aversion, i.e., chronic social aversion leads to increased aversion salience of foot shock. Distinctive features of the model include the following: high reproducibility; rare, mild wounding only; male specificity; absence of “susceptible” vs “resilient” subgroups; behavioral effects dependent on continued presence of CD-1 mice; and preclinical validation of novel compounds for normalizing aversion hypersensitivity with accurate feedforward prediction of efficacy in human patients. © 2024 The Authors. Current Protocols published by Wiley Periodicals LLC.
Basic Protocol 1 : Chronic social stress (CSS)
Basic Protocol 2 : Pavlovian aversion learning and memory (PALM)
INTRODUCTION
A major reason for the paucity of progress in discovering novel and more efficacious drugs for treating neuropsychiatric disorders has been the limited validity of animal models. With the conception of the research domain criteria (RDoC) framework has come new emphasis on the neurobehavioral study of specific psychological/psychopathological processes, in contrast to entire complex and heterogenous disorders (Cuthbert, 2020). This has provided impetus to translational research because there is realistic potential to develop and study animal models of these specific neurobehavioral processes and their disruption. One of the RDoC domains is negative valence systems, which are the neurobehavioral systems that underlie emotional and motivational responses to aversive stimuli/events, or threats, that are acute, potential, or sustained (https://www.nimh.nih.gov/research/research-funded-by-nimh/rdoc/about-rdoc).
Acute threat processing is one of the best understood neurobehavioral systems in both neuroscience and psychiatry (Berridge, 2019; LeDoux & Pine, 2016; Phelps & LeDoux, 2005; Ressler & Maren, 2019). This understanding, in rodents and humans, has been obtained using the paradigm Pavlovian aversion learning and memory (PALM), often referred to as “fear conditioning.” Of major importance to the neural circuitry underlying PALM are the amygdala and hippocampus, subcortical regions that are highly analogous in structure and function in rodents and primates. In rodents, the conditioned stimulus (CS) and unconditioned stimulus (US) typically comprise tone and electric foot shock, respectively (Johansen et al., 2011; Lüthi & Lüscher, 2014). The main unconditioned responses (URs) to the US are running, jumping, and retreating, and the main conditioned response (CR) to the CS is freezing. According to Pavlovian learning theory, the major determinant of the rate and asymptote of learning and memory is the emotional salience of the US; accordingly, across successive CS-US trials, the higher the foot shock amplitude, the faster the increase in and higher the maximum value of the percent time spent freezing to the tone (Rescorla, 1988; Rescorla & Wagner, 1972).
While the RDoC framework identifies acute, potential, and sustained threats as separate constructs, it is also the case that these negative valence systems are interdependent; that is, in psychiatric disorders, such as major depressive disorder (MDD) and generalized anxiety disorder (GAD), where responsiveness to acute or potential aversion is generally excessive, sustained threat by a specific aversive stimulus is a common etiological risk factor (Agid et al., 2000; Brown et al., 1995; Kendler & Gardner, 2010; Kendler et al., 1999, 2002; Kessler, 1997). Aversive psychosocial stimuli are of particularly high salience and psychosocial stressors are common and major risk factors (Keller et al., 2007). In rodents, and specifically males, territory-related social interactions are defined by differences in status, and aggression and escape/avoidance are common. In the laboratory, resident-intruder paradigms (Koolhaas et al., 2013; Pryce & Fuchs, 2017) have been developed in which this natural situation is utilized such that the home cage of the resident mouse is modified by a divider to allow introduction of a conspecific intruder and continuous distal sensory communication. In addition, for a short period per day there is proximate interaction and the resident attacks and establishes its dominance relative to the intruder. The subordinate status of the intruder combined with no possibility to escape/avoid the dominant mouse constitutes a sustained threat. A common disadvantage of such paradigms is that wounding is common, thereby substantially compromising the etiological validity of a manipulation that is intended to be a psychosocial stressor [e.g., Golden et al. (2011)].
We have established a refined resident-intruder paradigm in male mice, referred to as chronic social stress (CSS) (Azzinnari et al., 2014; Pryce & Fuchs, 2017). This 15-day paradigm minimizes wounding and the chronic social threat exposure results in a number of changes in the behavioral responses to emotionally salient stimuli of relevance to major symptoms in stress-related psychiatric disorders, both rewarding (Bergamini et al., 2016, 2018; Kukelova et al., 2018; Madur et al., 2023; Münster et al., 2022) and aversive (Adamcyzk et al., 2022; Azzinnari et al., 2014; Cathomas et al., 2019; Fuertig et al., 2016; Just et al., 2018). One of these aversion-directed responses is increased PALM, such that male mouse CSS-PALM constitutes a model of sustained psychosocial threat-induced generalized hyper-responsiveness to aversion. In this article we provide detailed step-by-step descriptions of CSS and PALM and of the respective materials required for each.
STRATEGIC PLANNING
Given that CSS and PALM are two protocols that together constitute a causal model, the temporal relationship between the two is of critical importance. Chronic social stress is a 15-day protocol and in our typical experimental design we conduct the Pavlovian aversion learning test two days after completion of CSS. In contrast, there is flexibility regarding when the subsequent Pavlovian aversion memory test is conducted, with the aversion memory-dependent increase in freezing behavior in CSS relative to control mice having been demonstrated up to 12 days post-learning. After completion of CSS, it is essential that each CSS mouse is maintained in the same divided cage as a CD-1 mouse, without physical attack, across the period of behavioral testing (Fig. 1).
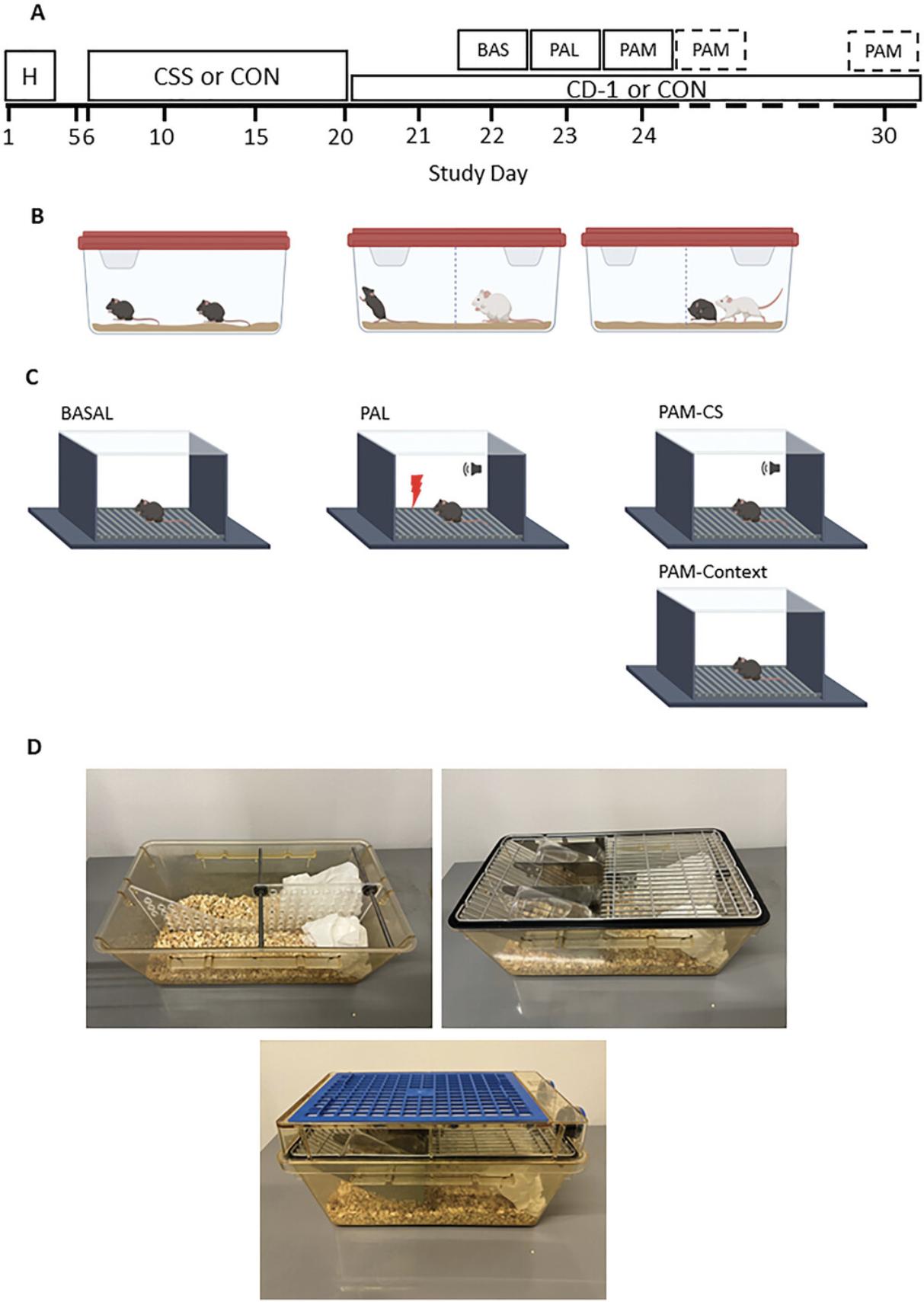
The following points are also important for strategic planning:
All in vivo procedures must be approved by the regional and national responsible authority and, if applicable, a local institutional committee. In the case of European countries, this will be a regional veterinary office that is applying the legislation according to national and/or European law. Submit a detailed description of the experiments planned with the CSS-PALM model and obtain legal approval that the planned experiments are ethical. The CSS-PALM model is typically conducted with C57BL/6J male mice that are bred in-house or obtained from a supplier (e.g., Janvier Labs: https://janvier-labs.com). It has also been conducted with transgenic mice to investigate gene × environment interaction effects [e.g., Cathomas et al. (2019)]. Wean mice at age 21 days into groups of 2 to 4 male littermates and cage all mice as pairs of littermates from age 6 weeks. House mice in transparent plastic cages measuring ∼33 cm × 21 cm × 14 cm (L × W × H), with a metal wire grid including a food hopper and space for a water bottle. Provide sawdust, paper tissue as nesting material, and a sleeping shelter. Cages are placed in a rack connected to an individually ventilated caging system with 40 to 50 air changes per hour. The aggressor mice (see Basic Protocol 1) can be kept in the same room as the C57BL/6J mice as long as an individually ventilated caging system is used. The housing room environment should be automated with a reversed light/dark (L:D) cycle with lights off at, e.g., 07:00 to 19:00, to eliminate the stress of disturbance during the inactive, light phase. Temperature needs to be in the range of 21.5° to 23.0°C and humidity of 40% to 60%. Change home cages each 7 to 10 days, to ensure a clean environment. Provide food and water ad libitum.
NOTE : All national and institutional ethical guidelines for the care and use of animals were followed. The experiments with the CSS-PALM model described were conducted in accordance with Swiss law and under the following animal experimentation licenses issued by the Veterinary Office of the Canton of Zurich: ZH170/2012, ZHZH149/2015, ZH155/2018 and ZH038/2022. All protocols involving animals must be reviewed and approved by the appropriate Animal Care and Use Committee and must follow regulations for the care and use of laboratory animals.
Basic Protocol 1: CHRONIC SOCIAL STRESS (CSS)
C57BL/6 male mice are chronically exposed to the distal stimuli, i.e., olfactory, auditory, visual, emitted by aggressive, ex-breeder CD-1 male mice, interspersed with daily brief proximal exposure to these same stimuli combined with physical attack and somatosensory pain. Together, this constitutes a chronic exposure to stimuli associated with uncontrollable and unpredictable aversion.
Materials
- C57BL/6J male mice
n = 24 in 12 littermate pairs, aged 10 to 12 weeks at experiment onset, bred in-house or obtained from a supplier (e.g., Janvier Labs). Each littermate pair is contributed by a different breeding pair.
- Swiss-Webster/Rj (CD-1) male mice
n = 20, aged 8 to 9 months at experiment onset, ex-breeder and separated from female directly before dispatch from supplier (e.g., Janvier Labs), and transported singly.
- Cages
Made from transparent polycarbonate measuring 33 cm × 21 cm × 14 cm, fitted with a metal wire grid comprising two lateral spaces for water bottles either side of a central food hopper, and with a flat top (Fig. 1D). These were obtained from Tecniplast, but the wire grids and tops are no longer available from this supplier.
- Bench, with sufficient room for placement of 3 cages and with a small adjustable lamp with white light to allow visual inspection of mice
- Precision weighing balance with dynamic function to allow for mouse movement (e.g., Mettler Toledo, dynamic balance range)
- Central longitudinal dividers
Made from transparent Plexiglas (5 mm) and cut to a form that fits into the cage such that there are no gaps at the front and back and top and bottom (Fig. 1D). In small holes at the front and back × top and bottom of the divider, metal rods are fixed securely, and are of a length that prevents the divider from tipping or being moved from side to side. The metal rods at the bottom of the divider are pressed down into the sawdust. The divider contains holes (Ø = 10 mm) at a horizontal and vertical distance of 15 mm (distance from hole-center to adjacent hole-center), to allow sensory contact between the mice while preventing physical contact.
- Experimental room, situated as close as possible to the housing room and equipped with infrared lighting
- Stopwatches
- Clip board with data sheet and pen
- Isoflurane anesthesia system with an induction chamber
- Rodent tooth cutting forceps
1.Order CD-1 mice from the supplier to arrive 1 week prior to the onset of the CSS protocol to allow acclimatization including adjustment to the reversed L:D cycle. Cage CD-1 mice singly and provide with wood stick for gnawing, paper tissue and sleeping shelter. Weigh and inspect all CD-1 mice to ensure that behavior and physical status are in order on 3 separate days in this week.
2.On day 1 of experiment (Fig. 1A), handle both BL/6J mice per littermate pair for 2 min each. Gently scoop up each mouse in turn into the cupped hands; briefly enclose the mouse in the hands if it is jumping back into the cage. Do not pick up the mouse using its tail. Weigh both mice. Repeat on days 2 and 3.After handling on day 2, conduct an ear punch of pinna, the right ear of one mouse and left ear of the other, for identification.
3.Using mean body weight per littermate pair, allocate mice to control (CON) and CSS groups by counterbalancing on body weight.
4.On the afternoon of day 5, place a divider into the home cages of the CD-1 mice. Place a CSS mouse on one side of the divider and the CD-1 mouse on the other. Replace the standard metal grid with the customized metal grid containing two water bottles and the central hopper filled with food. Replace the standard top with the flat top. Repeat with the remaining 11 mice allocated to CSS.
5.In the experimental room on the morning or afternoon of day 6:
-
Briefly handle, visually check, and weigh all CON mice.
-
Take the first CSS/CD-1 cage and remove the water bottles.
-
Place the first CSS mouse in the same compartment as the CD-1 mouse and close the cage.
-
Immediately start a stopwatch set to count down from 10 min.
-
Using another stopwatch set to count down from 1 min, count the time that the CD-1 mouse is attacking the CSS mouse, where attack includes the behaviors chase, box, and bite.
Often, one sequence of attack comprising these behaviors is followed by another such sequence, separated by an interval of 1 to 2 s. Therefore, at the end of each attack sequence, wait for 2 s before stopping the stopwatch and only stop it if another attack sequence has not been initiated within 2 s. Maintain a mental estimate of the cumulative duration of biting.
- Keep the two mice together in the same compartment for a maximum of 10 min, or a cumulative total of 60 s of attack, or an estimated cumulative total of 10 s of biting, whichever occurs first.
The cumulative total of attack time should be at least 30 s after 10 min. Typically, 60 s cumulative attack occurs within 5 to 6 min and during this time CSS mice are bitten for 3 s.
-
As soon as one of the criteria is reached, open the cage, and place the CD-1 mouse in the opposite compartment, so that the CSS mouse remains in the compartment where the attack occurred.
-
Weigh the CD-1 mouse.
-
Check the CSS mouse for wounds by gently stroking a clean white tissue along the back, flanks, and underside, and checking for blood spots; in the rare case of a blood spot, check for the site of the wound to ensure it is a surface wound and not a deep wound.
-
Return the mice to their compartments, the water bottles and lid, and return the cage to the rack.
-
Conduct the procedure with each of the other CSS/CD-1 pairs in turn.
With practice, it is possible to conduct the proximal attack sessions with two cages in parallel.
6.Directly after completing the protocol with all CSS mice on day 6:
-
Trim the lower incisor teeth of all CD-1 mice, to markedly reduce the incidence of wounding.
-
Place the mice singly in an anesthesia induction chamber with an inflow of 4% isoflurane at 800 to 1000 ml/min, for 30 to 40 s.
-
Remove the mouse and use a rodent tooth cutting forceps to remove 1.0 to 1.50 mm, including the sharp peaks of both lower incisors, thereby blunting them. Do not trim the upper incisors. The lower incisors regrow rapidly and continuously.
-
Check the teeth every 3rdday of the CSS procedure and repeat the trimming as necessary. On days of teeth trimming, cut 2 food pellets into smaller pieces and place on the floor of the compartment of the CD-1 mouse to facilitate feeding.
7.On the morning or afternoon of day 7:
-
Briefly handle, visually check, and then weigh all CON mice.
-
Remove the CSS mouse from the CSS/CD-1 cage in position 1 (#1) on the rack and place it in an empty “waiting cage” containing sawdust, tissue, water, and food.
-
Take cages #1, #2, and #3 to the experimental room (assuming that CSS will be conducted with the mice in cages #2 and #3 simultaneously).
-
Remove the water bottles.
-
With cage #2, remove, visually inspect, and weigh the CSS mouse.
-
Place this CSS mouse in the space for the water bottle on the side opposite to the CD-1 mouse in cage #1, and cover with the flat lid of the cage top to prevent escape.
-
Repeat for the CSS mouse in cage #3 and place in the water bottle space in cage #2.
-
Prepare the stopwatches and conduct the CSS protocol as described in step 5.
-
Repeat the procedure with all CSS/CD-1 cages: the final CSS mouse is in the waiting cage, and it is removed, visually inspected, and weighed, and placed in the CD-1 cage #12.
8.Repeat the procedures in step 7 on each of days 8 to 20, so that CSS mice undergo 15 proximal attacks and subsequent circadian (range 20 to 28 hr) distal periods, together with 12 different CD-1 mice. On days of CD-1 mouse teeth checking/trimming, reduce cumulative total of attack duration to 30 s maximum.
9.In the first 5 days of the CSS protocol (experimental days 6 to 10) it is possible that 2 to 3 of the 12 CSS mice will respond to attacks by CD-1 mice with retaliation or, in rare cases, will even initiate aggression. It is also possible that CD-1 mice will respond to this with submissive behavior. Such CD-1 mice are removed from the experiment and euthanized and replaced with a new CD-1 mouse. Also see Table 1.
Problem | Possible cause | Solution |
---|---|---|
CD-1 mouse submissive to BL/6 CSS mouse | Not sufficiently territorial | Replace CD-1 mouse |
CD-1 mouse does not attack for at least 30 s during 10 min | Not sufficiently aggressive | Replace CD-1 mouse |
CD-1 mouse causes surface wound to two BL/6 CSS mice on 2 consecutive days | Too aggressive | Replace CD-1 mouse |
CD-1 mouse that is usually aggressive is non-aggressive on day after teeth trimming | Teeth trimmed too low | Provide with food pieces on floor of cage for 2 days |
CD-1 mouse loses ≥10 % body weight on 2 consecutive days | Chronic stress and/or teeth trimmed to low | Remove from experiment and euthanize |
BL/6 CSS mouse receives surface wound | Insufficient active avoidance | Reduce daily maximum attack time from 60 s to 15-30 s until wound healed |
BL/6 CSS mouse receives 1 surface wound on 3 different days | Insufficient active avoidance | Remove BL/6 CSS mouse from experiment and euthanize |
10.From day 20 until the end of the experiment, maintain each CSS mouse with the same CD-1 mouse, separated by the divider and without any further proximal attacks.
11.As described above, it is essential to apply daily checks of C57BL/6J and CD-1 mice to ensure well-being, comprising weighing, checking for wounds, observing behavior for signs of pain or injury. The criteria that are applied to decide whether a mouse must be withdrawn from the experiment (humane end points) are the following:
-
C57BL/6J (CSS) mouse receives an open (deeper than skin only) bite wound on any day; receives 1 surface wound (skin only) on each of any 3 days; pain-related behavior of arched-back and poor gait/locomotion occurs on any day; body weight is <90% baseline (determined at handling on days 1 to 3) on any day; body weight is <95% baseline and coat is ungroomed on any 2 consecutive days. ∼1 per 100 CSS mice meets one of these termination criteria.
-
CD-1 mouse pain-related behavior of arched-back and poor gait/locomotion occurs on any day; stereotypy occurs on any day; body weight is <90% baseline on any 2 consecutive days. ∼3 per 100 CD-1 mice meets one of these termination criteria.
Basic Protocol 2: PAVLOVIAN AVERSION LEARNING AND MEMORY (PALM)
The CON and CSS mice undergo Pavlovian aversion learning (PAL) to test their acquisition of the predictive association between an initially neutral tone CS and context and an unconditioned aversive foot shock, measured as the percent time spent freezing during exposure to the CS and/or context. One or more days later, the mice undergo a Pavlovian aversion memory (PAM) test of their recall and expression of context aversion and CS-context aversion, again measured as percent time spent freezing. The CSS mice acquire and express higher CS-context aversion than do CON mice, indicating that chronic social stress leads to hyper-responsiveness to aversion in general.
Additional Materials (also see Basic Protocol 1)
-
70% ethanol, for cleaning and reducing mouse odors on floors and walls of conditioning arenas
-
Behavioral test system, for the automated conducting of PAL and PAM, including:
-
Attenuation chamber equipped with low-level white illumination (10 lux) and low-level white noise
-
Arena (context) with lid
-
Floor comprising stainless steel rods and that allows delivery of electrified shocks that serve as the unconditioned stimulus (US)
-
Loudspeaker for delivery of tone that serves as a conditioned stimulus (CS)
-
System for measurement of activity
This includes the unconditioned responses (UR) of running and jumping to the US; it also includes the absence of activity, primarily in the form of the conditioned response (CR) of freezing to the CS and/or context. The system should comprise an even number of conditioning units so that CON and CSS mice can be allocated to different units. For example, the Multi-Conditioning System (TSE Systems).
1.Conduct behavioral testing as close as possible to the housing room and under infrared lighting, as for the CSS protocol (Basic Protocol 1).
2.At experimental day 22, conduct a basal freezing test (BAS) with all CON and CSS C57BL/6J mice (Fig. 1A).
- Using a conditioning system equipped with four conditioning units, assign two units to CON mice and two to CSS mice.
This avoids potential effects of any stress-related odors from CSS mice impacting on the behavior of CON mice.
-
Transfer one CON cage and two CSS cages to the experimental room and weigh the mice.
-
Place all four mice singly in the arena (context) of the conditioning units.
-
Run a 15-min test in the absence of tone and foot shock to establish the basal % time spent freezing.
Activity and absence thereof can be detected using an infrared beam movement detection system or via video recording and subsequent image analysis.
-
At the end of the test, remove the mice and return them to their home cages and the housing room.
-
Clean the floors by removing fecal pellets and wiping with absorbent paper and then 70% ethanol; clean the arena walls with 70% ethanol.
Freezing is defined as episodes of at least 1500 ms (1000 ms can also be used) during which no activity is detected. Within each time period of interest (e.g., 15-min basal freezing test, 18 s CS, 120 s inter-trial interval in PAL test), the cumulative total time of freezing episodes is calculated and expressed as a percentage of the time period of interest.
3.Instead of a separate 15-min basal freezing test at day 22, a 6-min basal freezing test can be run directly before the PAL test on day 23.
4.At experimental day 23, conduct a PAL test with all CON and CSS C57BL/6J mice.
-
Run the mice in the same order as for the BAS test and in the same conditioning units.
-
Transfer one CON cage and two CSS cages to the experimental room and weigh the mice.
-
Place all four mice singly in the conditioning units.
-
If a BAS was not conducted on day 22, run a 6-min BAS test (see step 3).
-
Directly thereafter, run the PAL test: an interval of 120 s is followed by 6 CS-US trials of a novel auditory tone at 6.5 kHz and 85 dB (tone CS) presented via a loudspeaker for 20 s, with seconds 19 to 20 coincident with a 2 s foot shock (US) at 0.15 mA. The CS-US trials are separated by an inter-trial interval (ITI) of 120 s, and the test ends with a final interval of 120 s.
-
At the end of the test, remove the mice and return them to their home cages and the housing room.
-
Clean the floors by removing fecal pellets and wiping with absorbent paper and then 70% ethanol; clean the arena walls with 70% ethanol.
The % time spent freezing is measured during the 6 CS presentations, indicating acquisition of the association CS-and-context with US, and the 5 ITIs, indicating acquisition of the association of context with US.
5.At any day(s) between experimental days 24 and 30, conduct one or two PAM tests with all CON and CSS C57BL/6J mice.
-
Run the mice in the same order as for the PAL test and in the same conditioning units.
-
Transfer one CON cage and two CSS cages to the experimental room and weigh the mice.
-
Place all four mice singly in the conditioning units, using the same arenas as for the basal freezing test and the PAL test. One option is to first run a 10-min context memory test in the absence of CS and US; the % time spent freezing per min is measured, indicating recall and expression of context aversion memory specifically.
-
Directly thereafter, run a CS memory test comprising 10 trials of the tone CS for 30 s separated by ITIs of 90 s. Another option is to not run a context memory test: in this case, start the CS memory test with an initial interval of 120 s prior to the first tone CS trial.
The % time spent freezing is measured during the 10 CS, indicating recall and expression of CS-context aversion memory, and during the 9 ITIs, indicating recall and expression of context aversion memory. If a second day of PAM testing is conducted, run the CS memory test only.
6.Euthanize the C57BL/6J mice and CD-1 mice as soon as possible after the end of the experiment and at the latest within 3 days. If the brains of the CON and CSS C57BL/6J mice are to be investigated, conduct brain collection under anesthesia at the latest on the day following completion of PALM testing, and using a method compatible with the methods to be used for brain analysis. Euthanize CD-1 mice with carbon dioxide asphyxiation; after asphyxia check mice for absence of breathing movements and conduct bilateral pneumothorax to ensure euthanasia.
COMMENTARY
Critical Parameters
The purpose of the CSS-PALM procedure is to provide a model in which chronic exposure to psychosocial aversion leads to hyper-responsiveness to aversion in general, which is detected by measuring the conditioned response of freezing in response to Pavlovian CS and context associated with a mild foot shock US. This model can then be applied to investigate the brain-induced peripheral etiological processes and the brain-level pathophysiological processes that underlie psychosocial stress-induced generalized hyper-responsiveness to aversion. With respect to peripheral etiological processes, stress-responsive systems, such as the immune-inflammatory system and the hypothalamic-pituitary-adrenal (HPA) neuroendocrine system are considered major candidates (Azzinnari et al., 2014; Fuertig et al., 2016). Accordingly, it is essential that chronic social defeat/stress protocols minimize the bite wounding, which would otherwise itself activate these systems. Here, CSS represents a substantial advance over other protocols that do not involve the trimming of the lower incisor teeth of the aggressor mice or the timing of the cumulative duration of daily attack to ensure that this remains within a maximum of 1 min [e.g., Golden et al. (2011) and Wohleb et al. (2011)]. It is only by following the current protocol strictly that a chronic psychosocial stress procedure with etiological validity, i.e., that is not confounded by substantial wounding, can be achieved.
Three major characteristics of aversive stimuli that determine the extent to which they are stressors are their chronicity, uncontrollability, and unpredictability (Koolhaas et al., 2011; Pryce et al., 2011). Our CSS protocol has a duration of 15 days, 50% longer than the CSD protocol, which has a duration of 10 days (Golden et al., 2011) and substantially shorter than the protocol of chronic unpredictable mild stress (CUMS), which has a duration of 35 to 56 days (Willner, 2017). The uncontrollability of CSS is manifested in the combination of dominance and aggression of CD-1 mice during the daily proximal sessions; despite the subordinate behaviors of the CSS mice, including vocalization and upright stance in response to approach by the CD-1 mouse, and slow active approach toward the CD-1 mice, each of which should typically function to prevent agonistic encounters, the CD-1 mice continue to be aggressive and to attack. The unpredictability of CSS is manifested in the rotation of CSS mice across CD-1 mice and the differences in body size and behavior between the latter. In contrast to CSD (Golden et al., 2011), the CD-1 mice are not pre-selected for a short attack latency; therefore, there is unpredictability in the latency from the onset of the proximal session until the first attack. Furthermore, some CD-1 mice approach CSS mice slowly and inspect and even groom them interspersed with periods of attack, while others approach rapidly to attack and reach the 60 s maximum attack duration within 2 to 3 min. Also, the CSS mice differ in their behavior during the proximal sessions. One difference is that 10% to 25% of CSS mice display either spontaneous or retaliatory attacks of CD-1 mice during days 1 to 5 of the 15-day CSS protocol. Another difference is that ∼20% to 30% of CSS mice display slow approach toward followed by sitting directly in front of the CD-1 mouse; this is a form of submissive presentation and the mice that display this also actively avoid CD-1 mice when chased and emit submissive vocalization and upright stance when the CD-1 mice approach. It is likely that this submissive slow approach is what is mistakenly interpreted as “resilient” behavior in CSD mice in the social interaction test [e.g., Krishnan et al. (2007)].
Major features of the CSS-PALM model are its robustness and reproducibility. A sample size of 12 mice per CON and CSS groups is sufficient to detect the CSS effects on PAL and PAM. Furthermore, with this same sample size it is possible to detect the pharmacological reversal of the CSS effects on PAL and PAM. Importantly, the model is reproducible; this has been demonstrated by the large number of personnel within the laboratory who have conducted experiments with the model. While to our knowledge the CSS-PALM model has not yet been established in another laboratory, scientists have visited our laboratory to observe the CSS procedure and then successfully established a reproducible model of CSS-induced increase in aversion sensitivity using another behavioral test e.g., light-dark box (Michal Ślęzak, personal communication). At the same time, and related to this robustness and reproducibility, it is important to note that there are no subgroups of CSS-PALM mice that would be the equivalent of the so-called “susceptible” and “resilient” subgroups in the chronic social defeat-social interaction test (CSD-SI test) paradigm, where high and low passive avoidance of CD-1 mice is the measure used for subgrouping (Golden et al., 2011; Krishnan et al., 2007). As can be seen in Figure 2A, the inter-individual variability in freezing behavior is highly similar in CSS and CON mice. While it cannot be tested empirically, our interpretation is that mice that as CON mice acquire relatively low levels of freezing are those that acquire relatively low levels of freezing as CSS mice, and that mice that as CON mice acquire relatively high levels of freezing are those that acquire relatively high levels of freezing as CSS mice (Fig. 2B). That is, the C57BL/6J mice differ in their innate/trait propensities to acquire PALM, and CSS induces a state that is rather consistent across mice and additive to the innate propensity (Fig. 2C). Indeed, it has been demonstrated that the so-called “susceptible” and “resilient” states in the CSD-SI test paradigm are also based on corresponding pre-CSD innate propensities (Milic et al., 2021). Accordingly, comparing susceptible mice with all CON mice, rather than only with CON mice with a high innate passive avoidance, introduces a statistical bias that will exaggerate CSD effects. If subgroups were to be analyzed in the CSS-PALM model, it would need to be conducted as illustrated in Figure 2C.
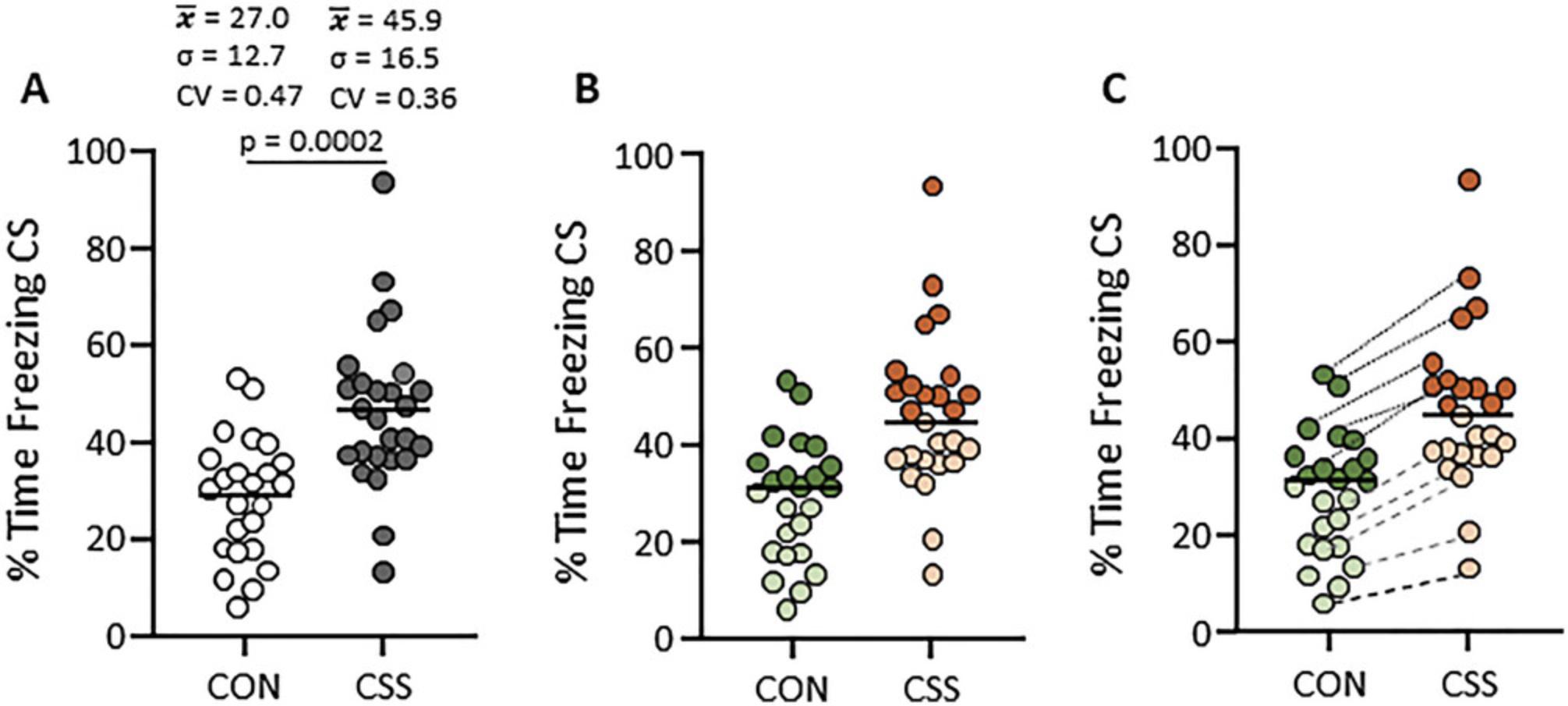
Therefore, the robustness of the CSS-PALM model is associated with not over-expecting what it can deliver with respect to subgroups relevant to human susceptibility and resilience based on multiple genetic, epigenetic, and environmental factors. Another area of animal model research that has been impeded by over expectation is the increasing insistence that the model is established in females and males, rather than one sex, i.e., either female or male, being sufficient. Regarding rodent resident-intruder or CSS/CSD protocols, these are male-specific because rodent females are not territorial. Some researchers have undertaken extreme endeavors to establish CSD protocols for female mice (e.g., Takahashi et al., 2017). Another approach, which we would encourage, is the following: if the male mouse protocol is applied to establish that a certain brain region, cell type or protein is important in regulating the behavioral readout, then the priority is to establish whether control female and male mice are similar or different with respect to that region, cell type or protein, rather than to produce a female version of the stress-behavior model. Below we present an example for this; the male mouse CSS-PALM model was used to provide preclinical validation for a novel mechanism of pharmacological action, and it accurately predicted efficacy in depressed patients, both men and women (see Understanding Results).
A final critical parameter of the model is that the excessive PALM in CSS mice is dependent on their being distally exposed to the CD-1 mice throughout the test period; post-CSS separation from the CD-1 mice and their cages results in CSS mice with PALM behavior resembling that of CON mice (see Understanding Results). Therefore, as well as the maintaining of CSS mice next to CD-1 mice being a major practical bridge linking the two stages of the model, the evidence that this is essential to the model provides critical insight into the etiopathophysiological processes via which CSS induces excessive PALM. It suggests strongly that the physical presence of the aversion-conditioned CD-1 mouse stimuli causes direct changes in the brain that underpin increased PALM, such that on removal of the aversive stimuli these brain-behavior effects can attenuate rapidly. A brain-periphery-brain causation would be expected to require longer to return to homeostasis/allostasis following separation from the CD-1 mice, such that the CSS effects on PALM would be expected to persist for longer. Therefore, while we have substantive evidence that, e.g., the immune-inflammatory and HPA systems are activated by psychosocial stress in CSS mice, it is much more likely that the CSS-induced effects on PALM are mediated by changes in brain processes directly. We hypothesize that these include CSS-induced increased glutamate and neuropeptide signaling in the neural circuitry underlying PALM, including the regions of amygdala and hippocampus, and the data presented in the Understanding Results section provide support for this.
Troubleshooting
See Table 1 for a list of potential problems, causes, and solutions for the CSS-PALM model.
Statistical Analysis
Freezing behavior is defined as episodes of at least 1.5 s (or 1 s can also be applied) during which the infrared beam system (or video record analysis) detects an absence of movement; the cumulative duration of these episodes is calculated and expressed as a percentage of the duration of the current trial or interval. These data are then analyzed in full-factorial statistical models using either GraphPad Prism or SPSS. Either analysis of variance (ANOVA) or linear mixed model can be applied. In ANOVA, there is a between-subjects factor of group (and of dose in the case of pharmacological experiments) and within-subjects (repeated measures) factor of either trial, inter-trial interval or interval, depending on test phase. In linear mixed model, there are fixed effects of group, dose, and depending on the experiment and test phase, either trial, inter-trial interval or interval, and a random effect of mouse subject. Post hoc testing of, e.g., significant within-subjects trial main effect or group × trial interaction effects, requires correction for multiple comparisons; usually Tukey's or Sidak's test is recommended.
Understanding Results
A representative example of the effect of the CSS protocol on freezing behavior in the PALM tests is given in Figure 3. In line with the mild intensity of the footshocks that constitute the Pavlovian US, CON mice acquire a low level of the conditioned response of freezing to the tone CS, ∼20% to 30% of the 18 s that the CS is presented at CS-US trials 5 to 6 (Fig. 3A). In CSS mice, the acquisition of freezing to the CS is increased to ∼40% to 50% of the CS time during trials 5 to 6. One possibility would be that CSS leads to hyperalgesia such that the physical aversion salience of the US is higher; that this is not the case is indicated by the absence of a CSS effect on distance moved during the 2 s footshock presentations (Fig. 3B). This indicates that the increase in salience is at the psycho-emotional level. At the PALM-context test on the following day, the % time spent freezing is relatively low in CON and CSS mice, but it is nonetheless significantly higher in the CSS mice (Fig. 3C). Directly afterwards, in the PALM-CS-context test when the CS is first presented, there is a marked increase in freezing by both CON and CSS mice to ∼40% and ∼60% time spent freezing, respectively, during the 30 s that the CS is presented at trials 1 to 2 (Fig. 3D). Both CON and CSS mice display a moderate and similar decrease in CS memory expression across the 10 CS trials.
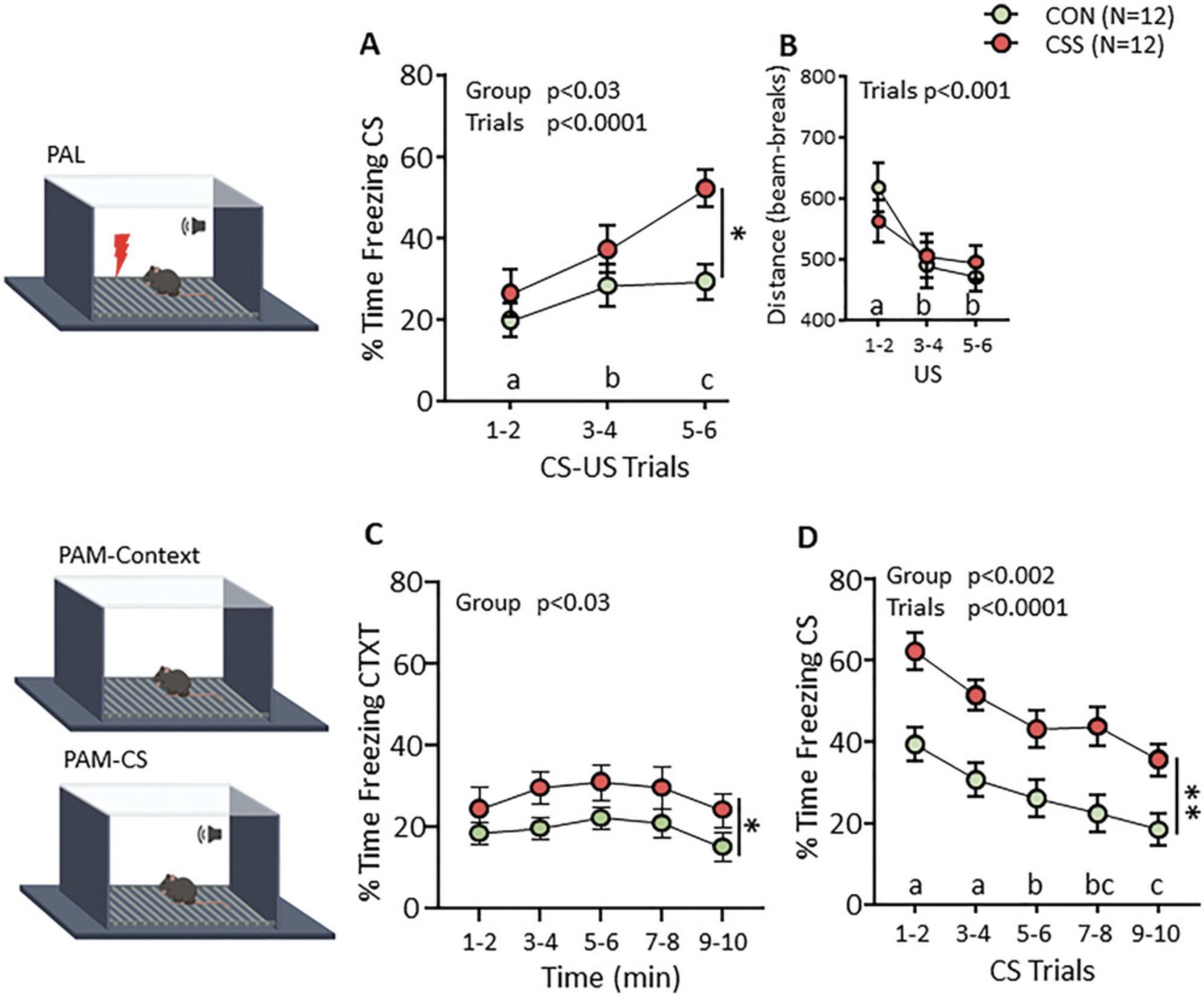
That it is essential to maintain CSS mice next to the CD-1 mice after the 15-day CSS protocol and during PALM testing is shown by the data in Figure 4. In addition to the usual CON and CSS groups, the experiment included a CSS group in which the C57BL/6J mice were separated from the CD-1 mice at day 15 of CSS (day 20 of the experiment) and then caged alone for the period of PALM testing (CSS-Alone group) (Fig. 4A). During the PAL test, the CSS-Alone mice acquired CS freezing at a similar level to CON mice and at a significantly attenuated level compared with CSS mice (Fig. 4B). Similarly in the PAM-CS-context test, while CSS mice displayed the expected increase in freezing compared with CON mice, this was not the case for CSS-Alone mice (Fig. 4C).
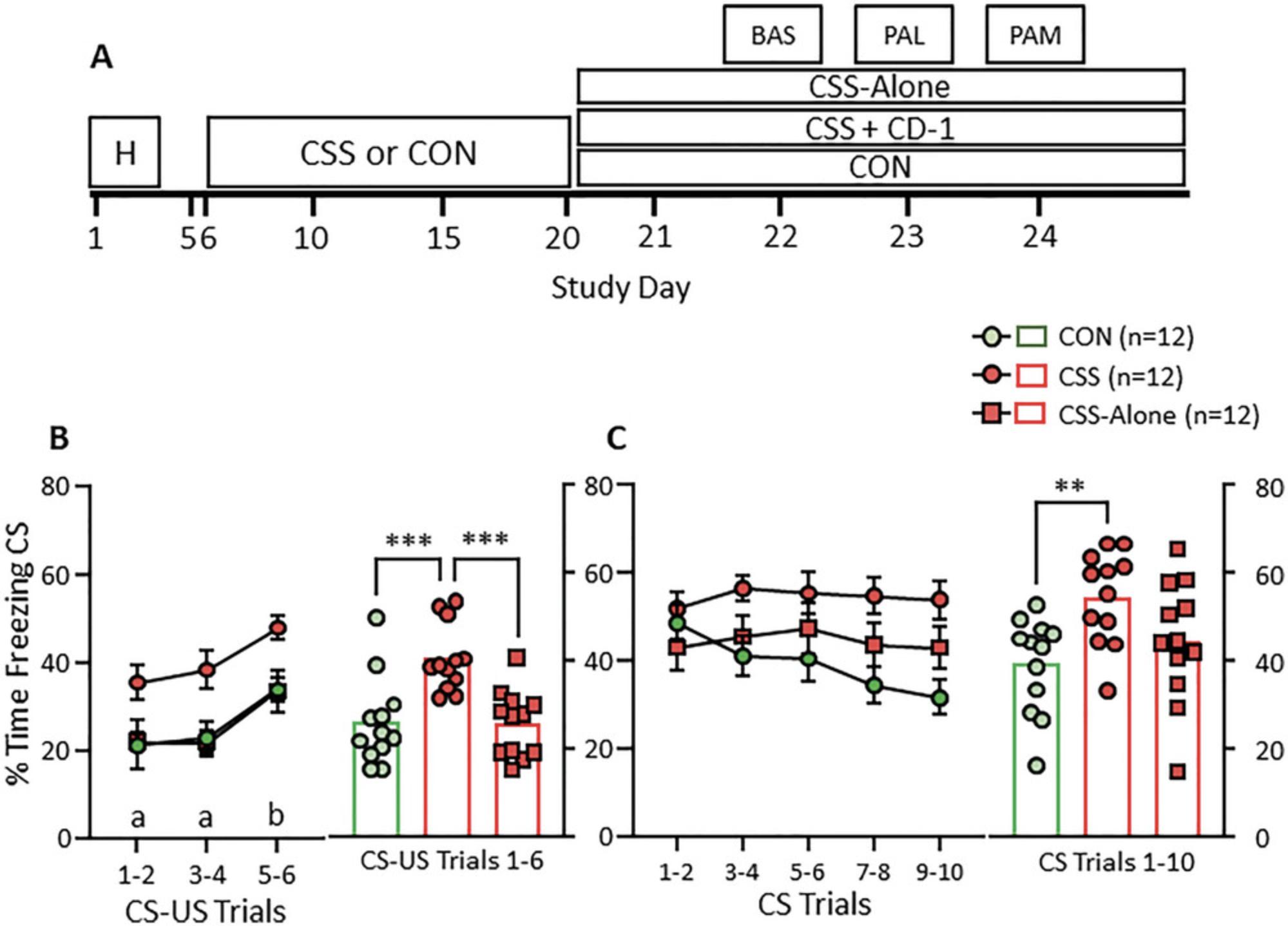
As indicated above, the CSS-PALM model can be applied to increase understanding of the neurobiological bases of chronic social stress-induced excessive aversion processing; specifically, to identify brain (sub)regions, cell types, and proteins that are altered in structure and/or function in CSS compared with CON mice. Directly related to this, the model can be applied to investigate the preclinical validity of novel neuropharmacological mechanisms of action. In these experiments, CON and CSS mice will receive vehicle only or the compound of interest at one or more doses; of major interest is whether the conditioned freezing behavior of CSS mice that received compound is reduced to a level lower than that in CSS mice that received vehicle and is similar to that in CON mice that received vehicle. One example of such a study was conducted with an inhibitor of the transient receptor potential canonical (TRPC) 4 and 5 channels. The TRPC channels are receptor-bound, calcium-permeable nonselective cation channels. Of the 7 mammalian TRPC channels, TRPC4 and TRPC5 are of most potential relevance to emotional disorders and their pharmacotherapy (Fowler et al., 2007; Klipec et al., 2016; Riccio et al., 2009, 2014). TRPC4 and 5 channel signaling occurs in response to activation of Gαq protein-coupled receptors (GPCRs), and the GαqPCRs with which TRPC4/5 channels co-localize, thereby enhancing neuronal excitability, include metabotropic glutamate receptor 1 (mGluR1) and cholecystokinin 2 receptor (CCK2) (Faber et al., 2006; Phelan et al., 2012; Riccio et al., 2009, 2014). Following the CSS/CON protocol, mice underwent the baseline freezing test on day 22, and then the PAL test on day 23; CSS mice acquired more CS freezing than did CON mice. Directly after the PAL and at 2 hr prior to the PAM test on day 24, a potent, small-molecule TRPC4/5 channel inhibitor (TRPC4/5-INH), or vehicle only (VEH), were administered; therefore, TRPC4/5-INH effects on post-conditioning consolidation and/or recall of CS-context memory could be studied (Fig. 5A and B). While there was no evidence for an effect on these processes in CON mice, the high CS-context freezing in the PAM test by CSS mice was normalized by TRPC4/5-INH (Fig. 5C) (Just et al., 2018). Based largely on this preclinical validation evidence, human studies have subsequently been conducted with a small-molecule TRPC4/5 channel inhibitor. In a single dose, randomized, placebo-controlled phase I BOLD-fMRI study with male and female major depressive disorder patients, TRPC4/5 channel inhibitor reduced the BOLD-signal response to emotional faces in amygdala and insula, and to a greater extent than was the case for either placebo or the antidepressant citalopram (Grimm et al., 2022). Therefore, the male mouse CSS-PALM model has accurately predicted that a novel mechanism of action will be efficacious in reducing aversion sensitivity in human subjects, women and men, with a current diagnosis of MDD.
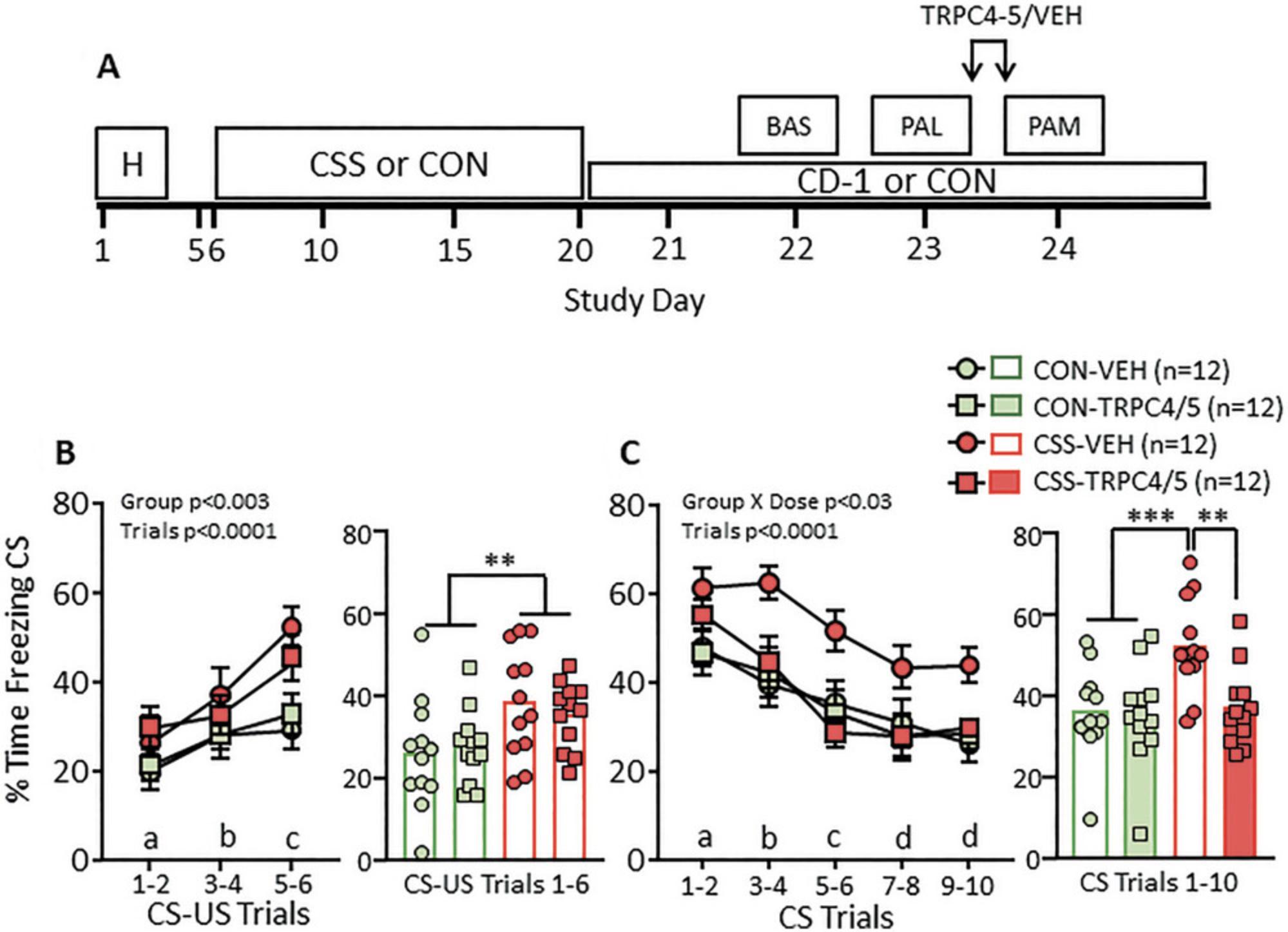
Time Considerations
As illustrated in Figure 1, the maximum duration of the experiment with 12 CON mice and 12 CSS mice is 30 days, with days 1 to 3 required for handling, days 6 to 20 for CSS and days 22 to 30 for behavioral testing. In the case of pharmacological experiments where multiples of 12 mice per group are required for each compound dose to be tested, it is recommended to divide the experiment into counterbalanced runs of 24 mice. For example, the experiment presented in Figure 5 was conducted over two runs, with each run comprising CON-VEH, n = 6; CON-TRPC4/5-INH, n = 6; CSS-VEH, n = 6; and CSS-TRPC4/5-INH, n = 6.
Acknowledgements
The CSS-PALM model development and application research was funded by the Swiss National Science Foundation (grants 31003A_130499, 31003A_141137, 31003A_160147, and 31003A_179381, to C.R.P.). The TRPC4/5 inhibitor project was conducted in collaboration with and funded by Boehringer-Ingelheim Pharma GmbH (to C.R.P.). We are extremely grateful to all of the following colleagues who contributed to the development of the chronic social stress protocol and/or conducted experiments with the CSS-PALM model: Jamie Albiez, Rene Amport, Sandra Auer, Damiano Azzinnari, Mischa Baer, Girogio Bergamini, Caroline Brook, Michaela Buerge, Flurin Cathomas, Redas Dulinskas, Alexandra Greter, Céline Hofer, Damaris Holder, Nagiua Haymour, Christian Ineichen, Diana Kukelova, Lorraine Madur, Sophie Meerwein, Jonas Mechtersheimer, Agata Panek, Giulia Poggi, Deniz Queisser, and Chenfeng Zhang.
Open access funding provided by Universitat Zurich.
Author Contributions
Hannes Sigrist : Conceptualization; data curation; investigation; methodology; writing original draft. Alena Senn : Investigation; methodology; writing original draft. David E. Hogg : Investigation; methodology; writing original draft. Christopher R. Pryce : Conceptualization; data curation; funding acquisition; methodology; project administration; supervision; writing original draft; writing review and editing.
Conflict of Interest
C.R.P. receives research funding from Boehringer Ingelheim Pharma GmbH. The other authors have no conflict of interest to declare.
Open Research
Data Availability Statement
Data are available on request from the authors.
Literature Cited
- Adamcyzk, I., Kukelova, D., Just, S., Giovannini, R., Sigrist, H., Amport, R., Cuomo-Haymour, N., Poggi, G., & Pryce, C. R. (2022). Somatostatin receptor 4 agonism normalizes stress-related excessive amygdala glutamate release and Pavlovian aversion learning and memory in rodents. Biological Psychiatry: Global Open Sience , 2, 470–479. https://doi.org/10.1016/j.bpsgos.2021.11.006
- Agid, O., Kohn, Y., & Lerer, B. (2000). Environmental stress and psychiatric illness. Biomedicine and Pharmacotherapy , 54, 135–141. https://doi.org/10.1016/S0753-3322(00)89046-0
- Azzinnari, D., Sigrist, H., Staehli, S., Palme, R., Hildebrandt, T., Leparc, G., Hengerer, B., Seifritz, E., & Pryce, C. R. (2014). Mouse social stress induces increased fear conditioning, helplessness and fatigue to physical challenge together with markers of altered immune and dopamine function. Neuropharmacology , 85, 328–341. https://doi.org/10.1016/j.neuropharm.2014.05.039
- Bergamini, G., Cathomas, F., Auer, S., Sigrist, H., Seifritz, E., Patterson, M., Gabriel, C., & Pryce, C. R. (2016). Mouse psychosocial stress reduces motivation and cognitive function in operant reward tests: A model for reward pathology with effects of agomelatine. European Neuropsychopharmacology , 26, 1448–1464. https://doi.org/10.1016/j.euroneuro.2016.06.009
- Bergamini, G., Mechtersheimer, J., Azzinnari, D., Sigrist, H., Buerge, M., Dallmann, R., Freije, R., Seifritz, E., Ferger, B., Suter, T., & Pryce, C. R. (2018). Chronic social stress induces peripheral and central immune activation, blunted mesolimbic dopamine function, and reduced reward-directed behaviour. Neurobiology of Stress , 8, 42–56. https://doi.org/10.1016/j.ynstr.2018.01.004
- Berridge, K. C. (2019). Affective valence in the brain: Modules or modes? Nature Reviews Neuroscience , 20(4), 225–234. https://doi.org/10.1038/s41583-019-0122-8
- Brown, G. W., Harris, T. O., & Hepworth, C. (1995). Loss, humiliation and entrapment among women developing depression: A patient and non-patient comparison. Psychological Medicine , 25, 7–21. https://doi.org/10.1017/S003329170002804X
- Cathomas, F., Azzinnari, D., Bergamini, G., Sigrist, H., Buerge, M., Hoop, V., Wicki, B., Goetze, L., Soares, S., Kukelova, D., Seifritz, E., Goebbels, S., Nave, K.-A., Ghandour, M. S., Seoighe, C., Hildebrandt, T., Leparc, G., Klein, H., Stupka, E., … Pryce, C. R. (2019). Oligodendrocyte gene expression is reduced by and influences effects of chronic social stress in mice. Genes, Brain and Behavior , 18, e12475. https://doi.org/10.1111/gbb.12475
- Cuthbert, B. N. (2020). The role of RDoC in future classification of mental disorders. Dialogues in Clinical Neuroscience , 22(1), 81–85. https://doi.org/10.31887/DCNS.2020.22.1/bcuthbert
- Faber, E. S., Sedlak, P., Vidovic, M., & Sah, P. (2006). Synaptic activation of transient receptor potential channels by metabotropic glutamate receptors in the lateral amygdala. Neuroscience , 137(3), 781–794. https://doi.org/10.1016/j.neuroscience.2005.09.027
- Fowler, M. A., Sidiropoulou, K., Ozkan, E. D., Phillips, C. W., & Cooper, D. C. (2007). Corticolimbic expression of TRPC4 and TRPC5 channels in the rodent brain. PLoS ONE , 2(6), e573. https://doi.org/10.1371/journal.pone.0000573
- Fuertig, R., Azzinnari, D., Bergamini, G., Cathomas, F., Sigrist, H., Seifritz, E., Vavassori, S., Luippold, A., Hengerer, B., Ceci, A., & Pryce, C. R. (2016). Mouse chronic social stress increases blood and brain kynurenine pathway activity and fear behaviour: Both effects are reversed by inhibition of indoleamine 2 3-dioxygenase. Brain, Behavior, and Immunity , 54, 59–72. https://doi.org/10.1016/j.bbi.2015.12.020
- Golden, S. A., Covington, H. E., Berton, O., & Russo, S. J. (2011). A standardized protocol for repeated social defeat stress in mice. Nature Protocols , 6, 1183–1191. https://doi.org/10.1038/nprot.2011.361
- Grimm, S., Keicher, C., Paret, C., Niedtfeld, I., Beckmann, C., Mennes, M., Just, S., Sharma, V., Fuertig, R., Herich, L., Mack, S., Thamer, C., Schultheis, C., Weigand, A., Schmahl, C., & Wunder, A. (2022). The effects of transient receptor potential cation channel inhibition by BI 1358894 on cortico-limbic brain reactivity to negative emotional stimuli in major depressive disorder. European Neuropsychopharmacology , 65, 44–51. https://doi.org/10.1016/j.euroneuro.2022.10.009
- Johansen, J. P., Cain, C. K., Ostroff, L. E., & LeDoux, J. E. (2011). Molecular mechanisms of fear learning and memory. Cell , 147, 509–524. https://doi.org/10.1016/j.cell.2011.10.009
- Just, S., Chenard, B. L., Ceci, A., Strassmaier, T., Chong, J. A., Blair, N. T., Gallaschun, R. J., Del Camino, D., Cantin, S., D'Amours, M., Eickmeier, C., Fanger, C. M., Hecker, C., Hessler, D. P., Hengerer, B., Kroker, K. S., Malekiani, S., Mihalek, R., McLaughlin, J., … Moran, M. M. (2018). Treatment with HC-070, a potent inhibitor of TRPC4 and TRPC5, leads to anxiolytic and antidepressant effects in mice. PLoS ONE , 13(1), e0191225. https://doi.org/10.1371/journal.pone.0191225
- Keller, M. C., Neale, M. C., & Kendler, K. S. (2007). Association of different adverse life events with distinct patterns of depressive symptoms. American Journal of Psychiatry , 164, 1521–1529. https://doi.org/10.1176/appi.ajp.2007.06091564
- Kendler, K. S., & Gardner, C. O. (2010). Dependent stressful life events and prior depressive episodes in the prediction of major depression. Archives of General Psychiatry , 67, 1120–1127. https://doi.org/10.1001/archgenpsychiatry.2010.136
- Kendler, K. S., Gardner, C. O., & Prescott, C. A. (2002). Toward a comprehensive development model for major depression in women. American Journal of Psychiatry , 159, 1133–1145. https://doi.org/10.1176/appi.ajp.159.7.1133
- Kendler, K. S., Karkowski, L. M., & Prescott, C. A. (1999). Causal relationship between stressful life events and the onset of major depression. American Journal of Psychiatry , 156(6), 837–841. https://doi.org/10.1176/ajp.156.6.837
- Kessler, R. C. (1997). The effects of stressful life events on depression. Annual Review of Psychology , 48, 191–214. https://doi.org/10.1146/annurev.psych.48.1.191
- Klipec, W. D., Burrow, K. R., O'Neill, C., Cao, J. L., Lawyer, C. R., Ostertag, E., Fowler, M., Bachtell, R. K., Illig, K. R., & Cooper, D. C. (2016). Loss of the trpc4 gene is associated with a reduction in cocaine self-administration and reduced spontaneous ventral tegmental area dopamine neuronal activity, without deficits in learning for natural rewards. Behavioural Brain Research , 306, 117–127. https://doi.org/10.1016/j.bbr.2016.03.027
- Koolhaas, J. M., Bartolomucci, A., Buwalda, B., de Boer, S. F., Flügge, G., Korte, S. M., Meerlo, P., Murison, R., Olivier, B., Palanza, P., Richter-Levin, G., Sgoifo, A., Steimer, T., Stiedl, O., van Dijk, G., Wöhr, M., & Fuchs, E. (2011). Stress revisited: A critical evaluation of the stress concept. Neuroscience and Biobehavioral Reviews , 35, 1291–1301. https://doi.org/10.1016/j.neubiorev.2011.02.003
- Koolhaas, J. M., Coppens, C. M., de Boer, S. F., Buwalda, B., Meerlo, P., & Timmermans, P. J. (2013). The resident-intruder paradigm: A standardized test for aggression, violence and social stress. Journal of visualized experiments: JoVE , (77), e4367. https://doi.org/10.3791/4367
- Krishnan, V., Han, M.-H., Graham, D. L., Berton, O., Renthal, W., Russo, S. J., LaPlant, Q., Graham, A., Lutter, M., Lagace, D. C., Ghose, S., Reister, R., Tannous, P., Green, T. A., Neve, R. L., Chakravarty, S., Kumar, A., Eisch, A. J., Self, D. W., … Nestler, E. J. (2007). Molecular adaptations underlying susceptibility and resistance to social defeat in brain reward regions. Cell , 131, 391–404. https://doi.org/10.1016/j.cell.2007.09.018
- Kukelova, D., Bergamini, G., Sigrist, H., Seifritz, E., Hengerer, B., & Pryce, C. R. (2018). Chronic social stress leads to reduced gustatory reward salience and effort valuation in mice. Frontiers in Behavioral Neuroscience , 12(134), 1–14. https://doi.org/10.3389/fnbeh.2018.00134
- LeDoux, J. E., & Pine, D. S. (2016). Using neuroscience to help understand fear and anxiety: A two-system framework. American Journal of Psychiatry , 173, 1083–1093. https://doi.org/10.1176/appi.ajp.2016.16030353
- Lüthi, A., & Lüscher, C. (2014). Pathological circuit function underlying addiction and anxiety disorders. Nature Neuroscience , 17, 1635–1643. https://doi.org/10.1038/nn.3849
- Madur, L., Ineichen, C., Bergamini, G., Greter, A., Poggi, G., Cuomo-Haymour, N., Sigrist, H., Sych, Y., Paterna, J. C., Bornemann, K. D., Viollet, C., Fernandez-Albert, F., Alanis-Lobato, G., Hengerer, B., & Pryce, C. R. (2023). Stress deficits in reward behaviour are associated with and replicated by dysregulated amygdala-nucleus accumbens pathway function in mice. Communications Biology , 6(1), 422. https://doi.org/10.1038/s42003-023-04811-4
- Milic, M., Schmitt, U., Lutz, B., & Müller, M. B. (2021). Individual baseline behavioral traits predict the resilience phenotype after chronic social defeat. Neurobiology of Stress , 14, 100290. https://doi.org/10.1016/j.ynstr.2020.100290
- Münster, A., Sommer, S., Kúkeľová, D., Sigrist, H., Koros, E., Deiana, S., Klinder, K., Baader-Pagler, T., Mayer-Wrangowski, S., Ferger, B., Bretschneider, T., Pryce, C. R., Hauber, W., & von Heimendahl, M. (2022). Effects of GPR139 agonism on effort expenditure for food reward in rodent models: Evidence for pro-motivational actions. Neuropharmacology , 213, 109078. https://doi.org/10.1016/j.neuropharm.2022.109078
- Phelan, K. D., Mock, M. M., Kretz, O., Shwe, U. T., Kozhemyakin, M., Greenfield, L. J., Dietrich, A., Birnbaumer, L., Freichel, M., Flockerzi, V., & Zheng, F. (2012). Heteromeric canonical transient receptor potential 1 and 4 channels play a critical role in epileptiform burst firing and seizure-induced neurodegeneration. Molecular Pharmacology , 81(3), 384–392. https://doi.org/10.1124/mol.111.075341
- Phelps, E. A., & LeDoux, J. E. (2005). Contributions of the amygdala to emotion processing: From animal models to human behavior. Neuron , 48, 175–187. https://doi.org/10.1016/j.neuron.2005.09.025
- Pryce, C. R., Azzinnari, D., Spinelli, S., Seifritz, E., Tegethoff, M., & Meinlschmidt, G. (2011). Helplessness: A systematic translational review of theory and evidence for its relevance to understanding and treating depression. Pharmacology & Therapeutics, 132, 242–267. https://doi.org/10.1016/j.pharmthera.2011.06.006
- Pryce, C. R., & Fuchs, E. (2017). Chronic psychosocial stressors in adulthood: Studies in mice, rats and tree shrews. Neurobiology of Stress , 6, 94–103. https://doi.org/10.1016/j.ynstr.2016.10.001
- Rescorla, R. A. (1988). Behavioral studies of Pavlovian conditioning. Annual Review of Neuroscience , 11, 329–352. https://doi.org/10.1146/annurev.ne.11.030188.001553
- Rescorla, R. A., & Wagner, A. R. (1972). A theory of Pavlovian conditioning: Variations in the effectiveness of reinforcement and nonreinforcement. In A. H. Black & W. F. Prokasy (Eds.), Classical conditioning II: Current research and theory (pp. 64–99). Appleton-Century-Crofts.
- Ressler, R. L., & Maren, S. (2019). Synaptic encoding of fear memories in the amygdala. Current Opinion in Neurobiology , 54, 54–59. https://doi.org/10.1016/j.conb.2018.08.012
- Riccio, A., Li, Y., Moon, J., Kim, K. S., Smith, K. S., Rudolph, U., Gapon, S., Yao, G. L., Tsvetkov, E., Rodig, S. J., Van't Veer, A., Meloni, E. G., Carlezon, W. A. Jr., Bolshakov, V. Y., & Clapham, D. E. (2009). Essential role for TRPC5 in amygdala function and fear-related behavior. Cell , 137(4), 761–772. https://doi.org/10.1016/j.cell.2009.03.039
- Riccio, A., Li, Y., Tsvetkov, E., Gapon, S., Yao, G. L., Smith, K. S., Engin, E., Rudolph, U., Bolshakov, V. Y., & Clapham, D. E. (2014). Decreased anxiety-like behavior and Gαq/11-dependent responses in the amygdala of mice lacking TRPC4 channels. Journal of Neuroscience , 34(10), 3653–3667. https://doi.org/10.1523/jneurosci.2274-13.2014
- Takahashi, A., Chung, J. R., Zhang, S., Zhang, H., Grossman, Y., Aleyasin, H., Flanigan, M. E., Pfau, M. L., Menard, C., Dumitriu, D., Hodes, G. E., McEwen, B. S., Nestler, E. J., Han, M. H., & Russo, S. J. (2017). Establishment of a repeated social defeat stress model in female mice. Scientific Reports , 7(1), 12838. https://doi.org/10.1038/s41598-017-12811-8
- Willner, P. (2017). The chronic mild stress (CMS) model of depression: History, evaluation and usage. Neurobiology of Stress , 6, 78–93. https://doi.org/10.1016/j.ynstr.2016.08.002
- Wohleb, E. S., Hanke, M. L., Corona, A. W., Powell, N. D., Stiner, L. M., Bailey, M. T., Nelson, R. J., Godbout, J. P., & Sheridan, J. F. (2011). B-adrenergic receptor antagonism prevents anxiety-like behavior and microglial reactivity induced by repeated social defeat. Journal of Neuroscience , 31, 6277–6288. https://doi.org/10.1523/JNEUROSCI.0450-11.2011
Citing Literature
Number of times cited according to CrossRef: 2
- Chenfeng Zhang, Redas Dulinskas, Christian Ineichen, Alexandra Greter, Hannes Sigrist, Yulong Li, Gregorio Alanis-Lobato, Bastian Hengerer, Christopher R. Pryce, Chronic stress deficits in reward behaviour co-occur with low nucleus accumbens dopamine activity during reward anticipation specifically, Communications Biology, 10.1038/s42003-024-06658-9, 7 , 1, (2024).
- Chenfeng Zhang, Diana Kúkeľová, Hannes Sigrist, Bastian Hengerer, Ramona F. Kratzer, Philipp Mracek, Azar Omrani, Moritz von Heimendahl, Christopher R. Pryce, Orphan receptor-GPR52 inverse agonist efficacy in ameliorating chronic stress-related deficits in reward motivation and phasic accumbal dopamine activity in mice, Translational Psychiatry, 10.1038/s41398-024-03081-w, 14 , 1, (2024).