Microglial Phagocytosis/Cell Health High-Content Assay
Emily R. Mason, Emily R. Mason, Disha M. Soni, Disha M. Soni, Shaoyou Chu, Shaoyou Chu
Abstract
We report a microglial phagocytosis/cell health high-content assay that has been used to test small molecule chemical probes and support our drug discovery projects targeting microglia for Alzheimer's disease therapy. The assay measures phagocytosis and cell health (cell count and nuclear intensity) simultaneously in 384-well plates processed with an automatic liquid handler. The mix-and-read live cell imaging assay is highly reproducible with capacity to meet drug discovery research needs. Assay procedures take 4 days including plating cells, treating cells, adding pHrodo-myelin/membrane debris to cells for phagocytosis, staining cell nuclei before performing high-content imaging, and analysis. Three selected parameters are measured from cells: 1) mean total fluorescence intensity per cell of pHrodo-myelin/membrane debris in phagocytosis vesicles to quantify phagocytosis; 2) cell counts per well (measuring compound effects on proliferation and cell death); and 3) average nuclear intensity (measuring compound induced apoptosis). The assay has been used on HMC3 cells (an immortalized human microglial cell line), BV2 cells (an immortalized mouse microglial cell line), and primary microglia isolated from mouse brains. Simultaneous measurements of phagocytosis and cell health allow for the distinction of compound effects on regulation of phagocytosis from cellular stress/toxicity related changes, a distinguishing feature of the assay. The combination of cell counts and nuclear intensity as indicators of cell health is also an effective way to measure cell stress and compound cytotoxicity, which may have broad applications as simultaneous profiling measurements for other phenotypic assays. © 2023 The Authors. Current Protocols published by Wiley Periodicals LLC.
This article was corrected on 06 April 2023. See the end of the full text for details.
Basic Protocol : Microglial phagocytosis/cell health high-content assay protocol
Support Protocol : Procedures to isolate myelin/membrane debris from mouse brain and label with pHrodo
INTRODUCTION
Microglia, the resident immune cells of the brain parenchyma, play critical functions to maintain homeostasis in the brain tissue environment, ensuring normal neuronal activities (Kettenmann et al., 2011; Nimmerjahn et al., 2005; Salter & Stevens, 2017; Wake et al., 2013). Cleaning debris of dead cells and extracellular abnormal protein aggregates through phagocytosis is the major homeostasis function of microglia (Edler et al., 2021; Ennerfelt et al., 2022; Franco-Bocanegra et al., 2021; Mundt et al., 2022; Nizami et al., 2019; Witting et al., 2000). Decline of phagocytosis activity has been observed in Alzheimer's disease (AD) brains (Lewcock et al., 2020; Mundt et al., 2022), especially in plaque associated microglia (Gyoneva et al., 2016; Plescher et al., 2018). Accumulation of Aβ plaques and Tau tangles in AD brains suggest that microglia lack the capacity to maintain homeostasis in diseased brains, and that this deficiency contributes to the neurodegenerative pathology leading to dementia. We hypothesize that targeting microglia with therapeutic small molecule agents to stimulate phagocytosis may be a potential therapeutic strategy for AD. Selected targets include main regulators of TREM2 signaling pathway, such as INPP5D and PLCG2, which have been identified as risk factors related to AD pathogenesis (Andreone et al., 2020; Ennerfelt et al., 2022; Lewcock et al., 2020; Nugent et al., 2020). Since TREM2 regulates microglial phagocytosis activities, quantitative measurement of phagocytosis can be a phenotypic assay to test cellular efficacy for drug discovery projects targeting the pathway. Safety of potential drug molecules is another important factor to be monitored during drug discovery studies. Learning from various reported assays measuring phagocytosis via cellular imaging (Andreone et al., 2020; Phanse et al., 2012; Vance et al., 2016; Witting et al., 2000; Yeo et al., 2013) or flow cytometry (Harvath & Terle, 1999; Meena et al., 2021; Phanse et al., 2012), we developed and established a cellular assay that can measure both the efficacy by quantifying microglial phagocytosis, and simultaneously measure cell health, while maintaining the capacity and reproducibility required for guiding the drug discovery process. This microglial phagocytosis/cell health high-content assay is presented here. The simultaneous measurements of phagocytosis and cell health enable the identification of regulation of microglial phagocytosis in the absence of cell stress/toxicity caused by compounds, a distinguishing feature of the assay. The combination of cell counts and nuclear intensity for cell health is also an effective way to measure cell stress and compound cytotoxicity, and this strategy of simultaneous profiling measurements may have broad applications for other phenotypic assays.
Basic Protocol: MICROGLIAL PHAGOCYTOSIS/CELL HEALTH HIGH CONTENT ASSAY PROTOCOL
The assay introduced here is a simplified cellular imaging assay without any fixation and washing steps, while producing multiparameter measurements with high capacity using a 384-well plate format. The assay procedures include plating cells on day 1, incubating cell plates overnight before treating cells on day 2, adding pHrodo-myelin/membrane debris to cell plates on day 3, and 20 hr later, staining cell nuclei with Hoechst-33342 for 30 min before performing high-content imaging on day 4.Three selected parameters are measured from cells: 1) mean total fluorescence intensity per cell of pHrodo-myelin/membrane debris in phagocytosis vesicles to quantify phagocytosis; 2) cell counts per well to quantify treatment effects on proliferation and cell death; and 3) average nuclear intensity to quantify treatment-induced nuclear changes such as in apoptosis. The assay has been used on HMC3 cells (an immortalized human microglial cell line), BV2 cells (an immortalized mouse microglial cell line), and primary microglia isolated from mouse brains. For initial assay attempts, we recommend using HMC3 cells available from the ATCC.
Materials
-
HMC3 microglial cell line (ATCC, cat. no. CRL-3304) (Janabi et al., 1995), or BV2 immortalized mouse microglial cell line (kindly gifted by Dr. Michelle Block, Indiana University School of Medicine) (Blasi et al., 1990; Taetzsch et al., 2015), or primary microglia isolated from C57BL/6J mouse brain (Saura et al., 2003)
-
Maintenance medium (see recipe)
-
Assay medium (see recipe)
-
TrypLE Express (ThermoFisher Scientific, cat. no. 12605-028)
-
Dulbecco's phosphate-buffered saline (DPBS, 1×) no calcium, no magnesium (ThermoFisher Scientific, cat. no. 14190-144)
-
0.4% trypan blue solution (Gibco, cat. no. 15250061)
-
Sterile water: filter water using MilliQ Millipak 0.22-µm filter (Millipore Sigma, cat. no. MPGP002A1), then autoclave
-
Serum-free medium: 500 ml DMEM, high glucose, GlutaMAX supplement (ThermoFisher Scientific, cat. no. 10566-016)
-
70% ethanol
-
Lipopolysaccharides (LPS) (Sigma-Aldrich cat. no. L2143)
-
Cytochalasin D (ENZO, cat. no. MBL-T109-0001)
-
Idelalisib (Medchemexpress, cat. no. HY-13026)
-
Saracatinib (Medchemexpress, cat. no. HY-10234)
-
DMSO, ≥99.9% (Sigma-Aldrich, cat. no. 276855-100 ml)
-
Hoechst 33342 (Invitrogen, cat. no. H3570)
-
pHrodo-myelin/membrane debris (1 mg/ml, 55 µl-aliquots) (see Support Protocol below for preparation)
-
CellXpert C170i cell culture incubator (Eppendorf, cat. no. 2231000868)
-
Biosafety cabinet: 1300 series class II, type A2 biological safety cabinet (ThermoFisher Scientific, cat. no. 1323TS, model 1371)
-
T-75 flasks (uncoated) (Fisher Scientific, cat. no. FB012937)
-
2-ml microcentrifuge tubes (Fisher Scientific, cat. no. 05-408-138)
-
10 ml serological pipettes (Fisher Scientific, cat. no. 13-678-11E)
-
Countess II automated cell counter (ThermoFisher Scientific, cat. no. AMQAX1000)
-
Countess cell counting chamber slides (ThermoFisher Scientific, cat. no. C10283)
-
50-ml conical tubes (Thermo Scientific, cat. no. 339652)
-
384-well clear-bottom cell culture plates (Corning, cat. no. 353962)
-
Thermo Scientific Multidrop 384 (cat. no. 5840150 or 5840157) set in the biosafety cabinet
-
96-well compound plates (Thermo Scientific, cat. no. 249946)
-
15-ml conical tubes (Thermo Scientific, cat. no. 339650)
-
OT-2 pipetting robot (Opentrons, SKU 999-00111, 999-00002, or 999-00002)
-
OT-2 accessories and tips: HEPA module (Opentrons, model GEN1), P300 GEN2 multichannel pipette (Opentrons), P20 GEN2 multichannel pipette (Opentrons), 15-ml reservoir (12-well, Opentrons, SKU 999-00076, or Nest, cat. no. 360112; or similar), 96 tips/box and refills (20 μl and 200 μl or 300 μl)
-
Ultrasonic bath (Fisher Scientific, ca. no. 15336130; model: Branson 1800), or similar
-
Sonicator (Fisher Scientific, model: FB50)
-
ArrayScan XTI high-content analysis (HCA) Reader (Thermo Scientific), and associated image analysis software (Thermo Scientific HCS Studio).
Day 1: Cell plating
1.Culture the cells in the T-75 flasks with 10 ml maintenance medium per flask, until they are 70%-90% confluent, changing the medium every 2-3 days depending on cell density.
2.Pre-warm the assay medium and TrypLE to 22°C-37°C.
3.In the biological safety cabinet, gently remove the medium from the cells in the T-75 flasks and gently rinse the cells twice with 5-10 ml DPBS per flask.
4.Immediately after removing the second DPBS rinse, coat the cells with 2 ml TrypLE per flask.
5.Replace the lids on the flasks and return the cells to the incubator (37°C, 5% CO2) for 5-10 min.
6.After the 5-10 min incubation period, add 4 ml assay medium to each flask and mix so that the fetal bovine serum (FBS) in the medium inactivates the TrypLE. Use the resuspension to rinse any remaining cells off the bottom surface of the flask, then stand the flask up on its end (opposite the cap) to pool the resuspension over a smaller surface area.
7.Mix the cells (gently and carefully; avoid making bubbles) ten times with a 10 ml serological pipette.
8.Remove 11 μl cell resuspension into a 2-ml microcentrifuge tube for counting.
9.Add 11 μl 0.4% trypan blue solution to the cell sample and gently mix with pipetting three times.
10.Transfer 10 μl dyed sample into each chamber on the cell counting slide, load the slide into the cell counter, and read the cell count and viability. Make sure the viability of the cells to be used for the assay is >90%.
11.In a 50-ml conical tube, prepare 35 ml cell solution for plating one 384-well plate (prepare 20 ml more for each additional cell plate if needed), at the concentration needed to achieve the desired number of cells per well. Plating volume for the 384-well plate is at 45 μl/well; HMC3 cells are prepared at 13,333 cells/ml to yield 600 cells/45 μl/well, and BV2 cells at 8,889 cells/ml to yield 400 cells/45 μl/well; and mouse primary microglia at 44,444 cells/ml to yield 2,000 cells/45 μl/well. Each 384-well plate can test 16 different treatments in duplicate in 10-point concentration serial dilutions. Plate the number of plates for an experiment accordingly.
12.Prepare the following in separate 50-ml conical tubes for washing tubes of the 384 Multidrop (or similar instrument can be used for plating cells into 384-well plates):
-
30 ml sterile water
-
25 ml serum-free medium
This is the volume needed for plating 2 cell lines; add 10 ml per additional cell line.
-
15-20 ml 70% ethanol
13.Prepare the Multidrop and set it to dispense 45 μl/well into all the wells of a 384-well plate.
14.Label an empty clear-bottomed 384-well plate and set it on the plate carrier of the Multidrop. Remove the lid and set it aside.
15.Run ∼10 ml of serum-free medium through the instrument into a waste container.
16.Draw up some air into the tubing, then gently mix the first 50-ml conical tube of cells for plating. Draw up the cell suspension into the instrument. Gently agitate the cell suspension constantly, until plating is completed.
17.Initiate the cell plating program to plate the cells.
18.If plating multiple plates of the same cell type, be sure to add more cell suspension to the 50-ml conical tube, then repeat the plating.
19.Check the plate visually for any obvious volume errors, which can be corrected manually.
20.When the plating step is completed, empty the tubes of residual cell suspension.
21.Prepare tubes for plating the next cell line by sequentially running 20 ml sterile water followed by 10 ml serum-free medium through the tubes, then hold the tubes up in the air and draw up some air.
22.Repeat steps 16-19 with the second cell line.
23.Check the plates under a microscope to ensure that the cells were plated evenly and with no visible contamination.
24.Clean tubes by sequentially by running 30 ml sterile water through the instrument followed by 10 ml 70% ethanol until all the ethanol has run through into the waste container.
25.Power off the instrument. Clean and store the instrument and accessories.
26.Incubate plated cells overnight (16-24 hr) at 37°C with 5% CO2 before treatment.
Day 2: Compound serial dilution and treating cells
The following procedures are for a single plate of each cell type, with duplicates of each compound dilution series within each plate.
Protocols for the OT-2 (or other similar liquid handling) robot (compound dilution protocol and compound distribution protocol) will need to be designed and tested before proceeding. Multichannel pipettes in the robot are oriented along the Y (e.g., plate column) axis using the protocols.
27.Use a 96-well compound plate for serial dilutions. Start by manually filling column 2 wells with 97 µl assay medium, then add 3 µl 20 mM compound in DMSO in each well to get 600 µM and 3% DMSO of each compound as 10×.
28.Make 3% DMSO in assay medium (30 µl DMSO and 970 µl medium/ml), prepare 6 ml for each compound plate, add the 3% DMSO-medium at 60 µl/well to wells in column 3-12.
29.Prepare a 1:3 dilution series by sequential transferring/mixing (three times) 30 μl from each well to the next well across the rows, from column 2 to column 11.Column 12 will remain without compound, as the vehicle control column. An example plate map of compound dilution is shown in Figure 1A.
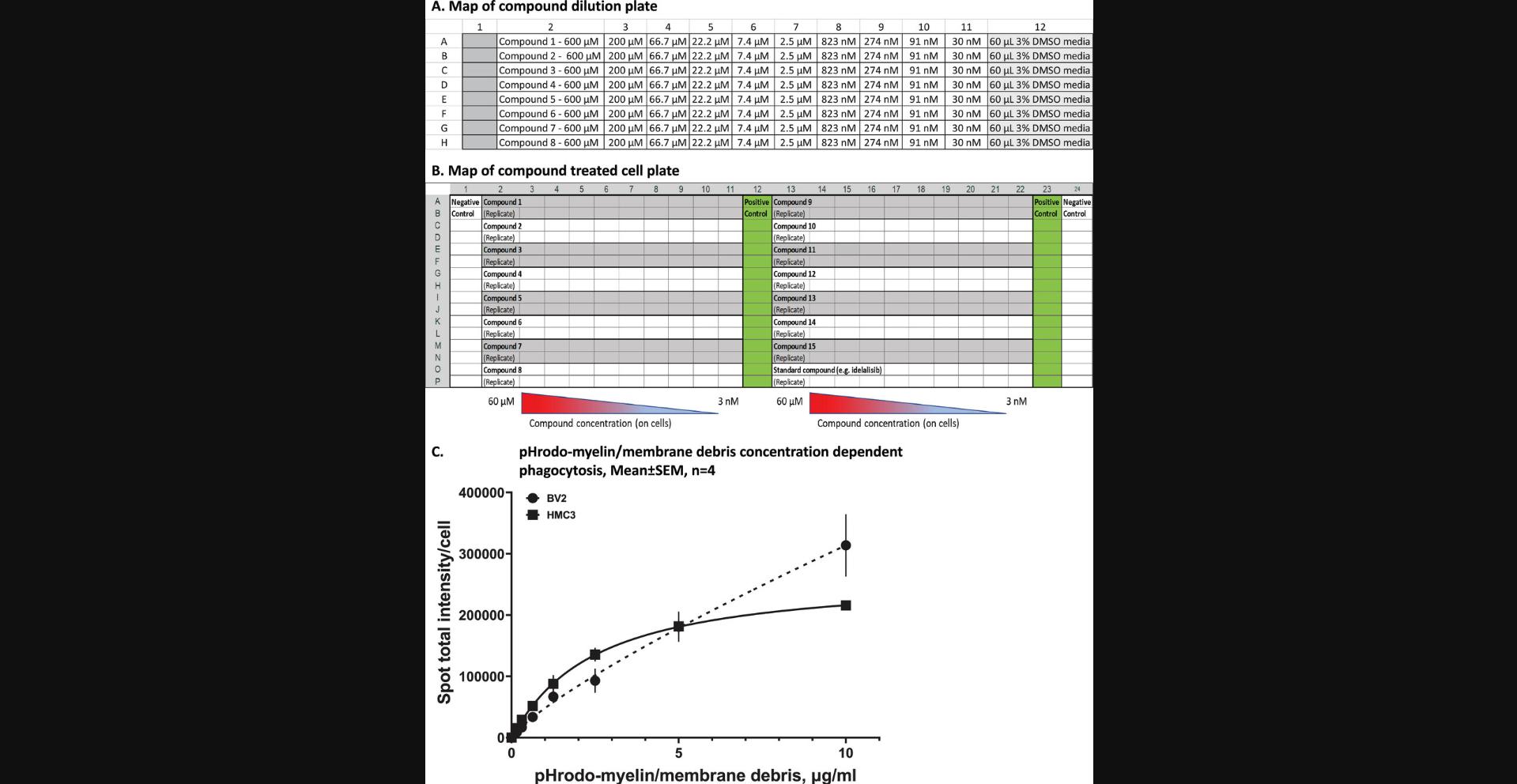
30.Transfer and position the compound plates, cell plates, and a box of 20 µl tips securely in the proper positions within the robot enclosure, according to the protocol setup on the robot app/software. Remove any lids or covers from these items.
31.Use the 8-channel pipette to pick up 20 µl control DMSO-medium from column 12, and transfer it to columns 1, 12, 23, and 24 in each cell plate, distributing 5 µl/well, twice per column since it is a 96-well to 384-well transfer. Change tips after this step.
32.Pick up 10 μl compounds from column 2 of the compound plate, transfer to column 2 of a 384-well cell plate through two duplicate actions (5 μl/well, 2 distributions/column); repeat these actions (pick up and transfer) through column 3-11 to treat the left half of the 384-well cell plate.
33.Repeat step 32 with a new set of tips and the second compound plate to treat the right half (column 13-22) of the 384-well cell plate.
34.Return cell plates to the incubator at 37°C with 5% CO2 for 24 hr before proceeding with the next step.
An example map of treated cell plate is shown in Figure 1B.
Day 3: pHrodo-myelin/membrane debris seeding
The pHrodo-myelin/cell debris seeding protocol uses the 20 μl 8-channel pipette to transfer 5 μl pHrodo-myelin/membrane debris from the compound plate to the corresponding wells in the cell plates so that final cell exposure to 5 μg/ml that was experimentally determined (Fig. 1C), changing tips after every transfer (from the compound plate to the cell plate) to avoid potential cross-contamination of compounds into the pHrodo-myelin/cell debris in the compound plate. The following protocol is for two cell plates; adjust volumes accordingly if using additional plates.
35.Pre-warm 5 ml cell culture assay medium to 37°C.
36.For each cell plate, thaw 2 aliquots of pHrodo-labeled myelin/cell debris (ea. 55 µl at 1 mg/ml) in the water bath at room temperature.
37.Vortex the aliquots briefly, then sonicate them in the sonicating water bath for 2 min, vortex the aliquots again, and transfer them into the biosafety cabinet.
38.In the biosafety cabinet, dilute each pHrodo-myelin/membrane debris aliquot with 1.1 ml assay medium (yielding 50 µg/ml pHrodo-myelin/membrane debris), pool the four aliquot dilutions in a 15-ml conical tube, and mix thoroughly.
39.Distribute 260 µl/well diluted pHrodo-myelin/membrane debris to wells in the first two columns (one column per cell plate) of a clean compound plate for seeding cells with the OT-2.
40.Use 20 μl tips to transfer 5 μl/well of the pHrodo-myelin/membrane debris from the compound plate to two 384-well cell plates; seed control columns 12 and 23 first, changing tips after every seeding to avoid cross contamination among wells when seeding wells in columns 2-11 and 13-22.Total seeded wells cover columns 2-23 of a 384-well cell plate; leave wells in columns 1 and 24 without seeding as negative controls.
41.Return cell plates to the incubator at 37°C with 5% CO2 for 20 hr before next step.
Day 4: Nuclear staining and imaging
The following protocol is for two cell plates; adjust volumes accordingly if using additional plates.
42.Perform nuclear staining as follows:
-
Pre-warm 22 ml of assay medium to room temperature.
-
Add 22 μl of 10 mg/ml Hoechst 33342 into 22 ml assay medium; mix well.
-
Use 200 μl tips to transfer 20 μl/well of the diluted Hoechst 33342 to 384-well cell plates, final cell exposure to 2.6 μg/ml Hoechst 33342; return cell plates into incubator for 30 min at 37°C with 5% CO2for incubation.
This step is performed with the OT-2 automatic pipetting robot but can be done with similar instruments.
-
Remove cell plates from the incubator for imaging.
43.Perform imaging as follows:
- Power on the ArrayScan XTI high-content imaging instrument, start Thermo Scientific HCS Studio Cell Analysis Software, choose the template of General Spot Measurement Tool. Set two channels, channel-1 for nuclear fluorescence (Hoechst 33342, ex. 386 nm, em. 440 nm) and channel-2 for phagocytosis vesicle fluorescence (pHrodo red, ex. 560 nm, em. >585 nm).
The plate imaging alignment may need to be optimized on the instrument at this time if not properly centered on wells.
-
Load the plate and optimize the imaging settings using the 10× objective, adjust appropriate imaging settings for each cell type to be imaged including automatic focusing on nucleus, find optimal exposure time for each channel, set the software to take a z-stack of 3 images with 6.7 μm apart to generate a projected image for analysis and taking 4 projected images/well.
-
Take example images from max and min wells and analyze these images through image analysis process: 1) segment cells on nucleus to identify individual cells; 2) expand identified edge of nucleus 30-50 μm to cover whole cell area; 3) identify phagocytosis vesicles in cell area for analysis.
-
Start the imaging, collecting four images per well. For analysis, collect cell counts per well, average nuclear intensity, and mean total phagocytosis spot intensity/cell, plus any other additional parameters of interest, for quantitation.
In the ArrayScan system, choose the template of “General Spot Measurement Tool” that allows to identify and analyze both the nuclei and the phagocytosed vesicles. A separate analysis protocol is needed for each cell type since different cell sizes and morphology need to be optimized for each specific cell type before starting the automated analysis. The imaging and analysis can be done with other high-content imaging systems using the similar analysis method.
Support Protocol: PROCEDURES TO ISOLATE MYELIN/MEMBRANE DEBRIS FROM MOUSE BRAIN AND LABEL WITH pHrodo
When developing the phagocytosis assay, we questioned what would be a proper ligand for measuring microglial phagocytosis. There are many options from which to choose, such as commercially available recombinant Aβ, Tau, microbeads, zymosan, and bacteria, or experimentally isolated dead neurons, synaptosomes or myelin from mouse brains. Since our project targets the TREM2 pathway, and since myelin/membrane debris has been shown to be a good ligand for TREM2 binding (Andreone et al., 2020; Ennerfelt et al., 2022; Nugent et al., 2020) and a natural material in the brain that is cleaned up by microglia in both homeostasis and neurodegenerative diseases, we decided to use myelin/membrane debris for the microglial phagocytosis/cell health assay. Isolation of crude myelin/membrane debris from 6-8 week old wild type C57BL/6J mouse brains was followed and modified on established literature protocols using sucrose gradients (0.32 M/0.85 M) and ultracentrifugation (Andreone et al., 2020; Erwig et al., 2019). The crude myelin/membrane debris was diluted to 1 mg/ml (protein equivalent) in DPBS, stored in a −80°C freezer, and subsequently labeled with pHrodo red dye.
Additional Materials (also see Basic Protocol)
-
6-8 week old C57BL/6J mice (B6; The Jackson Laboratory, 000664), 3 mice for each preparation
-
Anesthesia (see recipe)
-
Ultrapure distilled water (Invitrogen cat. no.10977-015); or autoclaved MilliQ water
-
Sucrose (Thermo Scientific, cat. no. A1558336) for preparing 0.3 M, 0.32 M and 0.85 M solutions in distilled water, 20 ml each, keep on ice.
-
Lysis buffer (see recipe)
-
Pierce Rapid Gold BCA Protein assay kit (Thermo Scientific, cat. no. A53226)
-
pHrodo red, 10 mM (see recipe)
-
NaHCO3, 1M (see recipe)
-
pH 4 buffer (Fisher Scientific, cat. no. Orinon 910104)
-
Glass petri dish (Fisher Scientific, cat. no. 08749B)
-
Razor blades
-
Glass dounce homogenizer (Fisher Scientific, cat. no. 06-435B)
-
5-ml ultracentrifuge tubes (SETON Scientific, cat. no. 5022) or similar 5-10 ml tubes for ultracentrifuge
-
Sterilized Pasteur pipette
-
Beckman Optima XPN-80 ultracentrifuge and SW 40 Ti rotor, 4°C (or similar)
-
Eppendorf centrifuge 5424R, 4°C
-
Microcentrifuge (BT labsystems, model. BT604)
-
Benchtop shaker (Fisher Scientific, cat. No. 88861045)
-
96-well clear-bottom cell culture plates (Thermo Scientific, cat. no. 165305)
1.Anesthetize 6-8 week old C57BL/6J mice (up to 3 mice for each prep) with anesthesia through intraperitoneal (ip) injection of 20 μl/gram body weight, then euthanize by cervical dislocation. (All experiments were approved by the Institutional Animal Care and Use Committee at Indiana University).
2.Remove brain tissues and place each brain in 1.2 ml cold lysis buffer in a glass petri dish on ice, cut into fine pieces with a razor blade and then dissociate via dounce homogenization (20× loose, then 20× tight).
3.Transfer the homogenate to 5-ml ultracentrifuge tubes with 1.2 ml/tube. Weigh loaded tubes to have balanced pairs for ultracentrifugation.
4.Add 1.2 ml of 0.32 M sucrose carefully underneath with a sterilized Pasteur pipette, and then add 1.2 ml of 0.85 M sucrose underneath both layers.
5.Precool the Beckman Optima XPN-80 ultracentrifuge to 4°C, load samples, and spin 30 min at 75,000 × g , 4°C (in an SW 40 Ti rotor, or similar) with low acceleration and deceleration.
6.Carefully remove myelin at the 0.32 M/0.85 M sucrose interface from centrifuge tubes and resuspended in 10 ml cold distilled water in a 15 ml tube, mix well.
7.Transfer the myelin/membrane debris to 2-ml microcentrifuge tubes with 1.2 ml/tube.
8.In a microcentrifuge (Eppendorf centrifuge 5424R), spin 10 min at 14,000 × g , 4°C, with maximum acceleration and deceleration; discard supernatant, resuspend pellets in 1.2 ml cold distilled water for each tube and vortex. Repeat three more times; after final centrifugation step, resuspend myelin/membrane debris with DPBS at 1 ml/tube.
9.Combine myelin from the 2-ml tubes into one 15-ml tube on ice for sonication at max amplitude for 15 s, sonicate three times.
10.Perform BCA assay to determine protein concentration, then dilute with DPBS to 1 mg/ml protein concentration, make 5 ml/tube aliquots (using 15-ml tubes) store in −20°C or −80°C freezer, or process for pHrodo labeling immediately through the following procedures.
11.Transfer 15 ml thawed 1 mg/ml myelin/membrane debris into a 50-ml conical tube.
12.Add 1.65 ml 1 M NaHCO3 to the 15 ml myelin/membrane debris. Mix by pipetting.
13.Add 150 μl 10 mM pHrodo red in DMSO to the myelin/membrane debris in the 50-ml conical tube. Mix by pipetting.
14.React for 45 min at room temperature: wrap the 50-ml tube with aluminum foil and set the tube in a rack on a shaker with gentle shaking.
15.Remove unbound dye as follows:
-
Transfer labeled myelin/membrane debris from the 50-ml tube to about 10-12 2-ml centrifuge tubes with 1.5 ml/vial (weigh tubes for balance before centrifuging).
-
Spin 10 min at 14,000 × g, 4°C, using the Eppendorf centrifuge 5424R.
-
Discard supernatant.
-
Resuspend labeled myelin/membrane debris in cold DPBS (keep on ice) by vortexing.
-
Repeat b-d three more times to remove unbound pHrodo red dye
16.Resuspend the labeled myelin/membrane debris in cold DPBS to total 15 ml (same as original volume of the unlabeled myelin, to keep the concentration at about 1 mg/ml); pool in a 50-ml conical tube on ice.
17.Sonicate the labeled myelin/membrane debris solution on ice (put the sonicator probe into the myelin solution in the 50-ml tube kept on ice in a beaker) at max strength for 15 s; perform this step three times.
18.Make aliquots of 55 μl/vial in 2 ml vials (two aliquots are required for each 384-well plate for one assay); store at −20°C (up to 3 months) or −80°C (up to 1 year) or use immediately. The final molar ratio of pHrodo red/myelin is at least 2 (counting myelin basal protein with MW 20 kD as the average MW of the sample).
19.Check the quality of the pHrodo-myelin/membrane debris prep with a fluorescent microscope as follows:
-
Prepare pHrodo-myelin/membrane debris at 5 μg/ml in DPBS (1:200 dilution of 1 mg/ml stock with DPBS).
-
Transfer 100 μl of the prep to a well of a 96-well clear-bottom cell culture plate.
-
Image with a fluorescent microscope using a 20× lens to get both transmitted light and fluorescent (ex. 560, em. 585 nm) images.
When in DPBS (about pH 7), the sample should show little or no fluorescence.
- Add 200 μl of a pH 4 buffer to the well and repeat step c.
The pHrodo-myelin/membrane debris should show strong red fluorescence in acid solution (around pH 4.2) (Fig.2Aand2B).
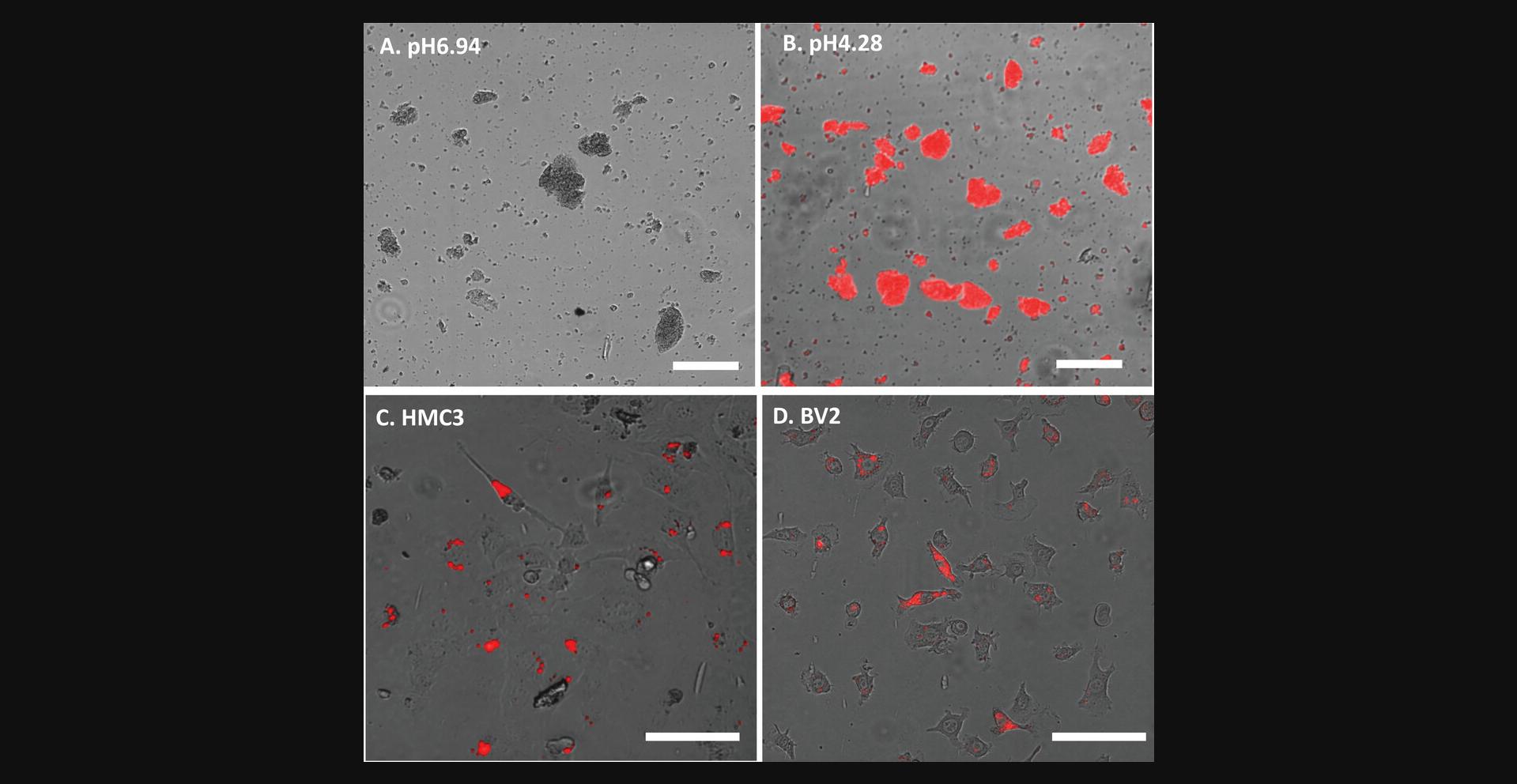
20.Check pHrodo-myelin/membrane debris signal in phagocytosis as follows:
-
Plate cells in a clear-bottomed microplate (1,000 cells/45 μl/well for a 384 well plate, or 5,000 cells/90 μl/well for a 96-well plate).
-
Incubate overnight in an incubator at 37°C with 5% CO2.
-
On day 2, prepare a 1:20 dilution (as 10× for seeding to cells) of the 1 mg/ml pHrodo-myelin/membrane debris (e.g., 5 μl mixed with 95 μl medium),
-
Add the diluted pHrodo-myelin/membrane debris into the cell plate (e.g., 5 μl/well for a 384-well plate with 45 μl cells/well plated, or 10 μl/well for a 96-well plate with 90 μl cells/well plated) so that cells are exposed to 5 μg/ml pHrodo-myelin/membrane debris.
-
Incubate at 37°C with 5% CO2for 4-5 hr.
-
Check phagocytosis with a fluorescent microscope as above in step 19 (Fig.2Cand2D).
Using 5 μg/ml pHrodo-myelin/membrane debris for the assay was determined with concentration dependent phagocytosis on both HMC3 and BV2 cells (Fig.1C).
REAGENTS AND SOLUTIONS
Anesthesia
- 1.25 g 2,2,2-tribromoethanol (ACROS organics cat. No. 421430500)
- 2.5 ml amylene hydrate (ACROS organics cat. no. 166620010)
- Dissolve by heating to 40°C with stirring
- Add distilled water to total volume of 100 ml
- Filter through 5-micron filter
- Aliquot and cover with foil
- Store at 4°C and use before the expiration date or when pH reaches >5.0
Assay medium
- 500 ml Dulbecco's modified eagle medium (DMEM), high glucose,
- GlutaMAX supplement, HEPES (ThermoFisher Scientific, cat. no. 10564-011)
- 50 ml Corning regular fetal bovine serum (Fisher Scientific, cat. no. MT35010CV)
- 5 ml penicillin/streptomycin (Sigma-Aldrich, cat. no. P4333, with 10,000 U penicillin and 10 mg streptomycin/ml)
- Filter through disposable PES filter units (Fisher Scientific, cat. no. FB12566504)
- Store up to 4 weeks at 4°C
Lysis buffer
- Prepare 10 ml:
- 10 mM HEPES
- 5 mM EDTA
- 0.3 M sucrose
- Supplement with protease inhibitor (Roche, 04-693-159-001) or similar
- Prepare fresh before use and store on ice
Maintenance medium
- 500 ml DMEM, high glucose, GlutaMAX supplement (ThermoFisher Scientific, cat. no. 10566-016)
- 50 ml Corning regular FBS (Fisher Scientific, cat. no. MT35010CV)
- 5 ml penicillin/streptomycin (Sigma-Aldrich, cat. no. P4333, with 10,000 units penicillin and 10 mg streptomycin/ml)
- Filter through disposable PES filter units (Fisher Scientific, cat. no. FB12566504)
- Store up to 4 weeks at 4°C
NaHCO3, 1 M
- 168 mg NaHCO3 (MW 84.01) (Fisher Scientific, cat. no. S233-500)
- 2 ml sterile MilliQ water
- Store at −20°C up to 6 months
pHrodo red, 10 mM
- 1 mg pHrodo red, warmed to room temperature and spun briefly with a microcentrifuge before opening (1 mg vial, Thermo Fisher, cat. No. P36600)
- 150 μl DMSO, ≥99.9% (Sigma-Aldrich, cat. no. 276855-100 ml)
- Vortex and mix with pipetting to ensure that the dye is well dissolved
- Prepare fresh immediately before using
COMMENTARY
Background Information
Microglial phagocytosis has been studied for its role in brain development (Cunningham et al., 2013; Matcovitch-Natan et al., 2016; Wake et al., 2013) and maintenance of homeostasis through surveillance and cleaning of cell debris, including dead cells and abnormal protein aggregates (Li & Barres, 2018; Mundt et al., 2022; Nimmerjahn et al., 2005; Salter & Stevens, 2017; Streit et al., 2004). Drug discovery targeting microglial phagocytosis function has been proposed as a therapeutic strategy for Alzheimer's disease and other neurodegenerative diseases (Nizami et al., 2019; Salter & Stevens, 2017). A reliable cellular assay capable of measuring drug-regulated phagocytosis activity while distinguishing that activity from phagocytosis modulation caused by cell stress is needed for drug discovery research targeting microglial phagocytosis. This need motivated us to develop and establish a high-content microglial phagocytosis/cell health assay to meet the challenge.
The primary advantage of the assay is the capability to measure phagocytosis and cell health simultaneously, allowing for the discernment of drug efficacy on phagocytosis (either stimulation or inhibition) in the absence of cell stress, since microglia under stress can show either higher or lower phagocytosis than that of resting microglia, as we have repeatedly observed. The multiplex feature of the assay has advantages over assays utilizing flow cytometry or imaging techniques to measure only phagocytosis alone. In addition, the assay with 384-well plates has the capacity required for screening or supporting molecule structure-activity-relationship studies for drug discovery projects. Another distinguishing feature of the assay is that it does not have washing steps and is a mix-and-read assay. Using pHrodo (an acid-activated fluorophore) -labeled myelin/membrane debris is the key to avoid any washing step for measuring cellular phagocytosis in the assay. Only live cells have bright phagocytosis vesicles with well-maintained inner acidic pH, and these are easily distinguished from extracellular pHrodo-myelin/membrane debris which shows negligible or low signal in a neutral pH environment. The washing-free protocol makes the cell count/well a reliable measurement for the evaluation of cell proliferation and viability under drug treatments (>24 hr), which combined with the measurement of nuclear intensity allowed estimation of treatment induced apoptosis. We think that combined measurements of cell count and nuclear intensity allow estimating overall status of cell population under experimental treatments, named them cell health measurements. One of the limitations of the 384-well plate assay is that it requires the use of an automatic pipetting instrument for liquid additions since it is difficult to keep uniformity of 384-well plates with manual operations. However, the assay can be performed with 96-well or other cell plates, through manual operations, to meet individual research needs. Another limitation is the necessity of an automatic high-content imaging system to handle the large numbers of images and analyses. The assay can be done manually as well if not used for drug discovery screening projects, by imaging with a common fluorescent microscope and performing image analysis with a proper software.
Critical Parameters
Cells
It is critical to check the cell viabilities when starting the assay. When plating cells, we check to ensure that viability is always >90%. Plating densities need to be experimentally determined and kept consistent to make the assay reproducible. The assay medium contains HEPES to maintain pH since during imaging the cell plates will be out of the incubator and exposed to room temperature and environmental air. We established this assay with microglial cell lines, HMC3 (immortalized human microglia) and BV2 (immortalized mouse microglia), and we have also used it with mouse primary microglia. The protocol can be used for other cells as well, such as macrophages and iPSC-derived microglia, to measure phagocytosis and cell heath simultaneously.
Ligands
The pHrodo-myelin/membrane debris was chosen as the ligand for our studies targeting TREM2 pathway because TREM2 has been shown to bind membrane lipids, especially the phosphatidylserine (PS) of dead cells. The protocol can be used to study the phagocytosis of other ligands such as synaptosomes, zymosan, bacteria, protein aggregates (Aβ, Tau), and microbeads, to suit individual research purposes. The seeding concentration and incubation time need to be experimentally established and kept consistent for reproducibility. Choosing 20-24 hr as the pHrodo-myelin incubation time was based on our observation that maximal phagocytosis took 4 hr and remained maximal for 24 hr, so that maximal phagocytosis can be measured. For experiments aiming to measure the initial phase of phagocytosis, the time course of phagocytosis needs to be experimentally determined.
Imaging
Collecting well-focused images is critical for quantitative high-content analysis since out-of-focus images always lead to inaccurate quantitative measurements. We set up automatic imaging using 3 focus planes separated by 6.7 μm along the Z-axis for every field, then save a single projected image formed from those 3 images for analysis. This is critical to ensure quality of the quantitative imaging analysis for subcellular measurements.
Troubleshooting
Troubleshooting steps are presented in Table 1.
Problem | Possible cause | Solution |
---|---|---|
Poor phagocytosis signal | Poor cell viability | Make sure cell viability >90%; cell plating density may be too high, check if medium turned yellow at the end, then adjust cell plating density accordingly |
Highly variable data |
1. Uneven cell plating, drug or pHrodo-myelin addition to wells; 2. Imaging was out of focus; 3. Max and min signals were not set properly |
1. Mix cell suspension well before plating; make sure the multidrop and automatic liquid handler is in normal condition; 2. Make sure the proper image projection of 3 focal planes is used; control wells should be uniform; 3. Check imaging setting and signal dynamic in range for covering max and min |
Data Analysis
All collected raw data are normalized to the untreated control with following formula: % of control = 100 × (X-Min)/(Max-Min). Factors in the formula are: X is raw data from a treated well, Max is an average measurement from pHrodo-myelin seeded but untreated wells, and Min is an average measurement from no pHrodo-myelin untreated wells. Normalized data are used for curve fitting that is done with the variable slope (four parameters) using the Prism software.
Understanding Results
The 384-well plate assay design is shown in Figure 3. Each plate contains two Min (negative) control columns (columns 1 & 24, without pHrodo-myelin seeding) and two Max (positive) (Fig. 3A) control columns (columns 12 & 23, with pHrodo-myelin) (Fig. 3B) so that these controls come from edge and middle wells, respectively. The rest of the wells are used for testing compounds in 10-point concentration response, in duplicates for each compound; a total of 16 compounds can be tested in one plate.
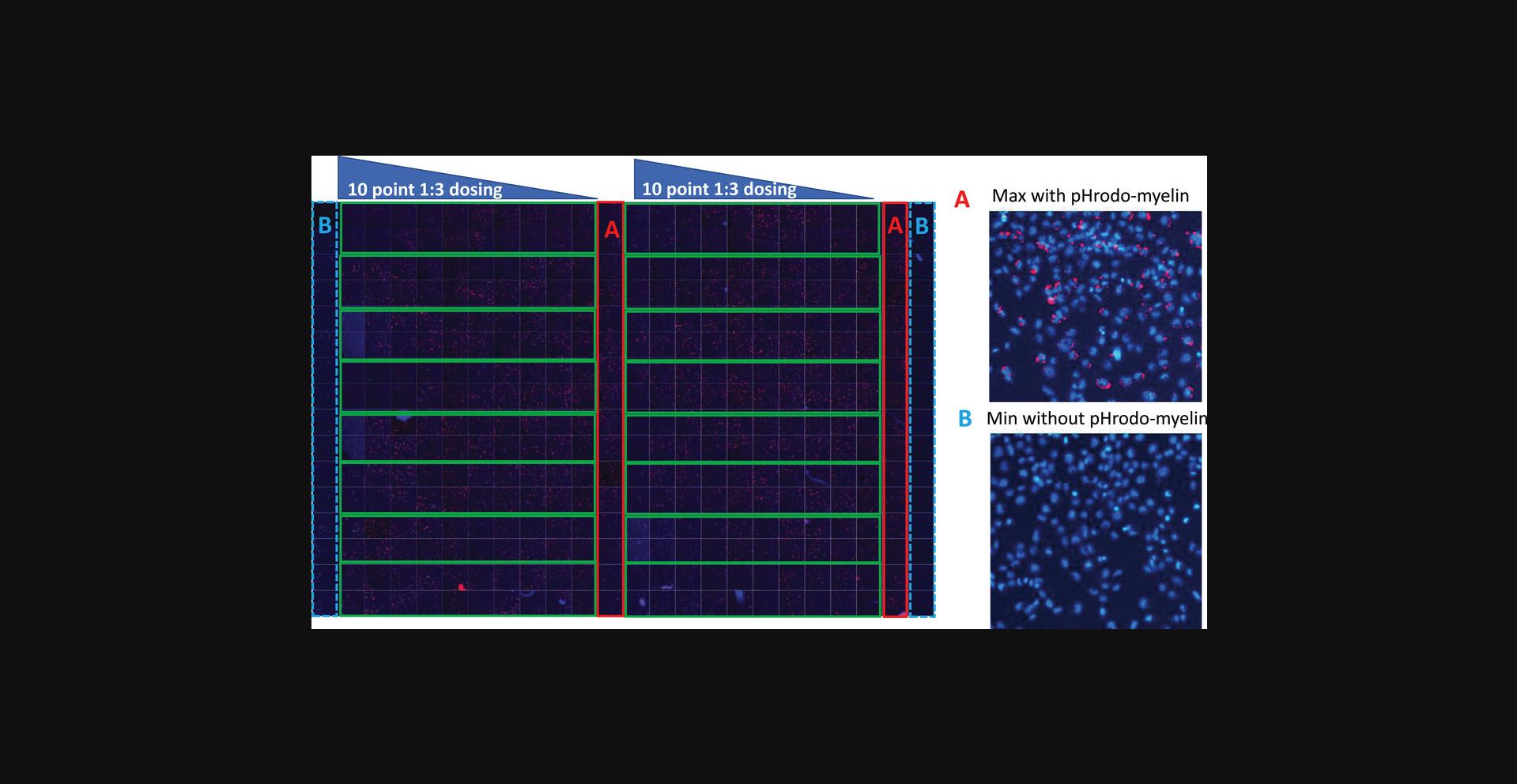
To ensure that the measurements reflect the steady state of compound treatment effects and cell health, the assay was set with a total compound treatment time of 48 hr, including pHrodo-myelin/membrane debris seeding performed 20-24 hr after compound addition. Figure 4 shows the image analysis process to identify individual cells and phagocytosis vesicles. Among many available quantitative measurements from the image analysis, we selected three parameters for the assay:
-
- The mean total intensity of phagocytosis vesicles/cell — to measure phagocytosis. There are multiple parameters generated from the image analysis, including phagocytosis vesicle area, number, and various fluorescence intensity measurements. Comparing these parameters, the total fluorescence intensity of vesicles/cell is the most sensitive one and was chosen for quantifying phagocytosis.
-
- The total cell counts — to measure overall cell viability, which is affected by proliferation and cell death. We chose to take 4 images/well (with the 10× objective lens) that cover about 50% of the total area of a well in a 384-well plate to represent cell count of the well.
-
- The average nuclear intensity — to measure drug-induced apoptosis, with high signal indicating nuclear condescension (a sign of early apoptosis) and low signal indicating DNA degradation (a sign of later apoptosis, when activated caspases digest DNA).
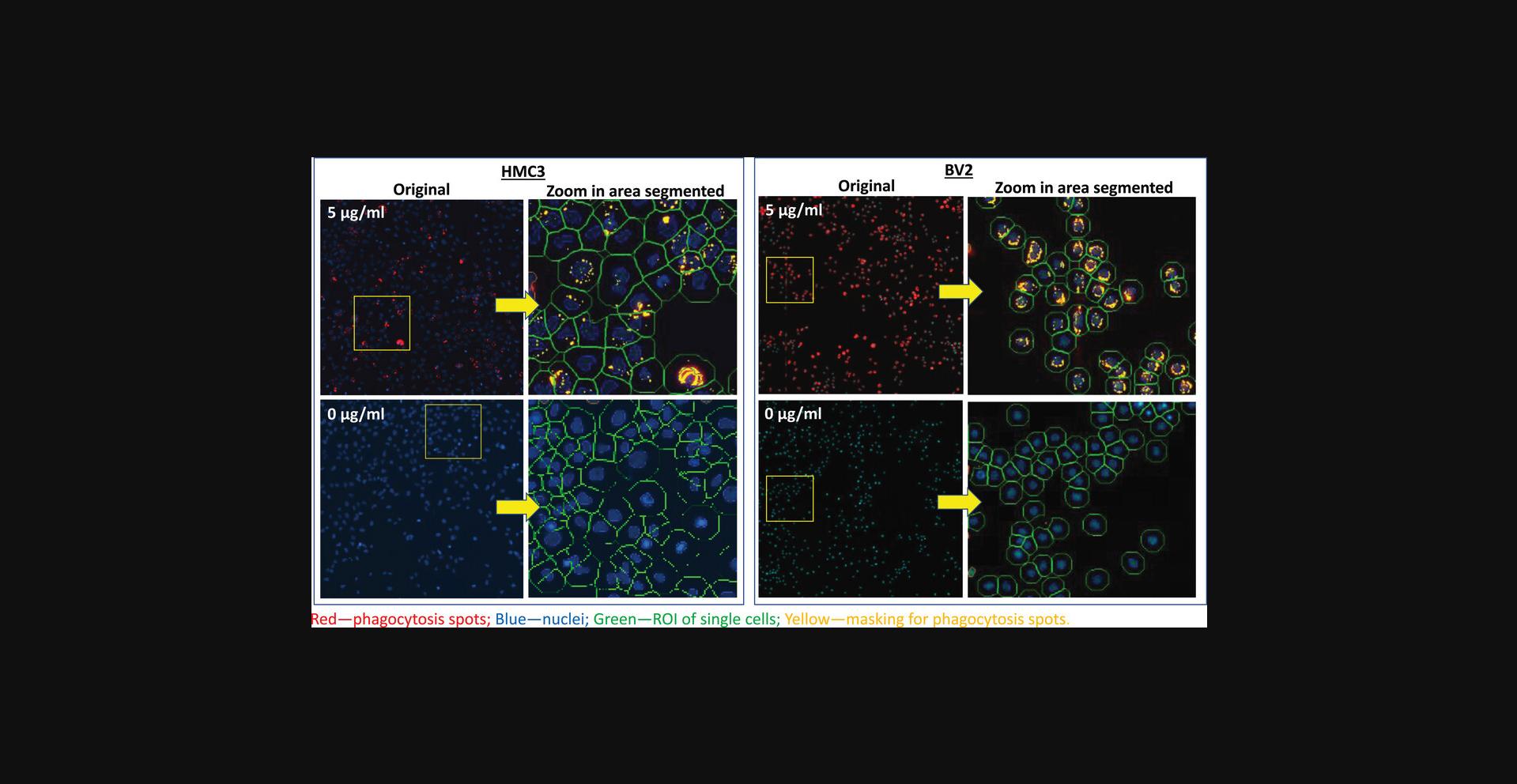
When evaluating the effects of a treatment, the combination of the three measurements allows for the determination of treatment effects on phagocytosis and of treatment caused cytotoxicity. We tested cytochalasin D in the assay, for its known effect of blocking actin filament formation (Casella et al., 1981), thus inhibiting phagocytosis and other actin-mediated cell activities (Meena et al., 2021; Melzer et al., 2019; Murai et al., 1993; Tilney & Portnoy, 1989; Vance et al., 2016). We observed that 24-hr cytochalasin D treatment (Fig. 5) inhibited HMC3 cell phagocytosis with IC50 = 138 nM, with lower potencies for its effects on cell count (IC50 = 367 nM) and nuclear intensity (EC50 = 488 nM). These results indicated that cytochalasin D inhibited phagocytosis in HMC3 cells, with cytotoxicity effects indicated by a cell number decrease and a concurrent nuclear intensity increase. It has been shown that cytochalasin D inhibits cell division at the end of the mitotic phase of the cell cycle and causes cells to round up (Margadant et al., 2013); which can explain our observation of decreased cell counts and increased nuclear intensity in HMC3 cells. When testing LPS on the HMC3 and BV2 microglia, results showed that HMC3 cells did not respond to LPS (Fig. 6A), while BV2 cells showed stimulation of phagocytosis parallel with decreased cell count, both in concentration-dependent responses, and no nuclear intensity changes up to 10 µg/ml LPS (Fig. 6B). The experiment was performed at least two times. These experimental results validated the assay performance. Observations that HMC3 cells failed to respond to the LPS suggested that HMC3 cells at basal status might lack Toll-like receptor 4 (TLR4) or related signaling molecules since LPS is known to bind to Toll-like receptor 4 (TLR4), thus stimulating immune cells. Different responses from different cell lines are not uncommon, and the mechanisms behind these differences remain to be studied.
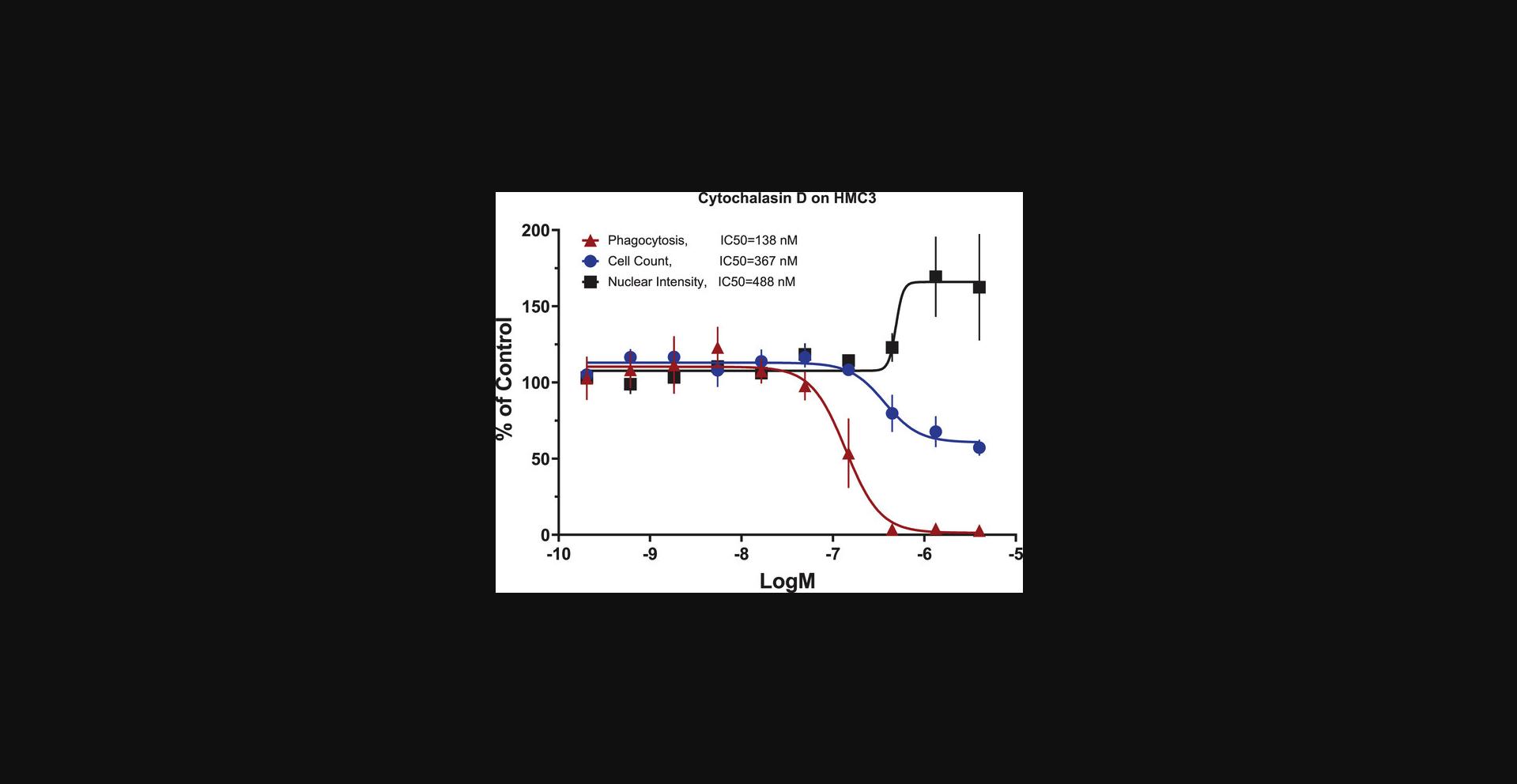
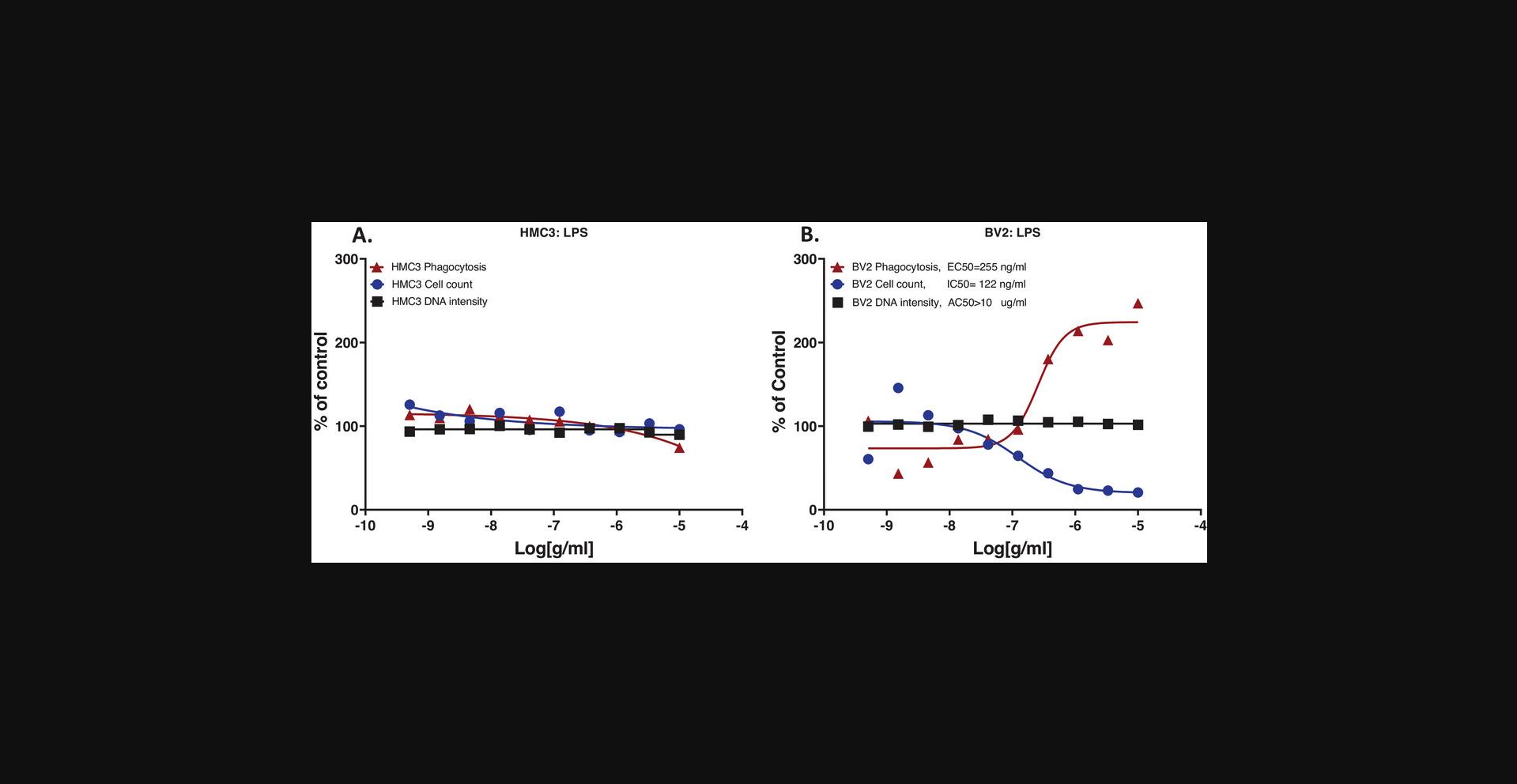
To explore cellular signaling regulation of phagocytosis with the assay, we chose a highly selective human PI3Kd inhibitor, idelalisib (Somoza et al., 2015), as a reference compound, based on knowledge that PI3K regulates cellular phagocytosis (Desale & Chinnathambi, 2021; Gillooly et al., 2001). Idelalisib (48-hr treatment) inhibited HMC3 cell phagocytosis in a concentration-dependent manner with an IC50 around 500 nM, with no significant cell count decrease or nuclear intensity changes (Fig. 7A). In comparison, idelalisib did not inhibit phagocytosis in BV2 cells, but increased phagocytosis in parallel with decreasing cell counts and increasing nuclear intensity, suggesting that the stimulation of phagocytosis was related to cell stress (Fig. 7B). These results were very reproducible, with similar results observed in more than 30 repeated assays, or a 100% success rate.
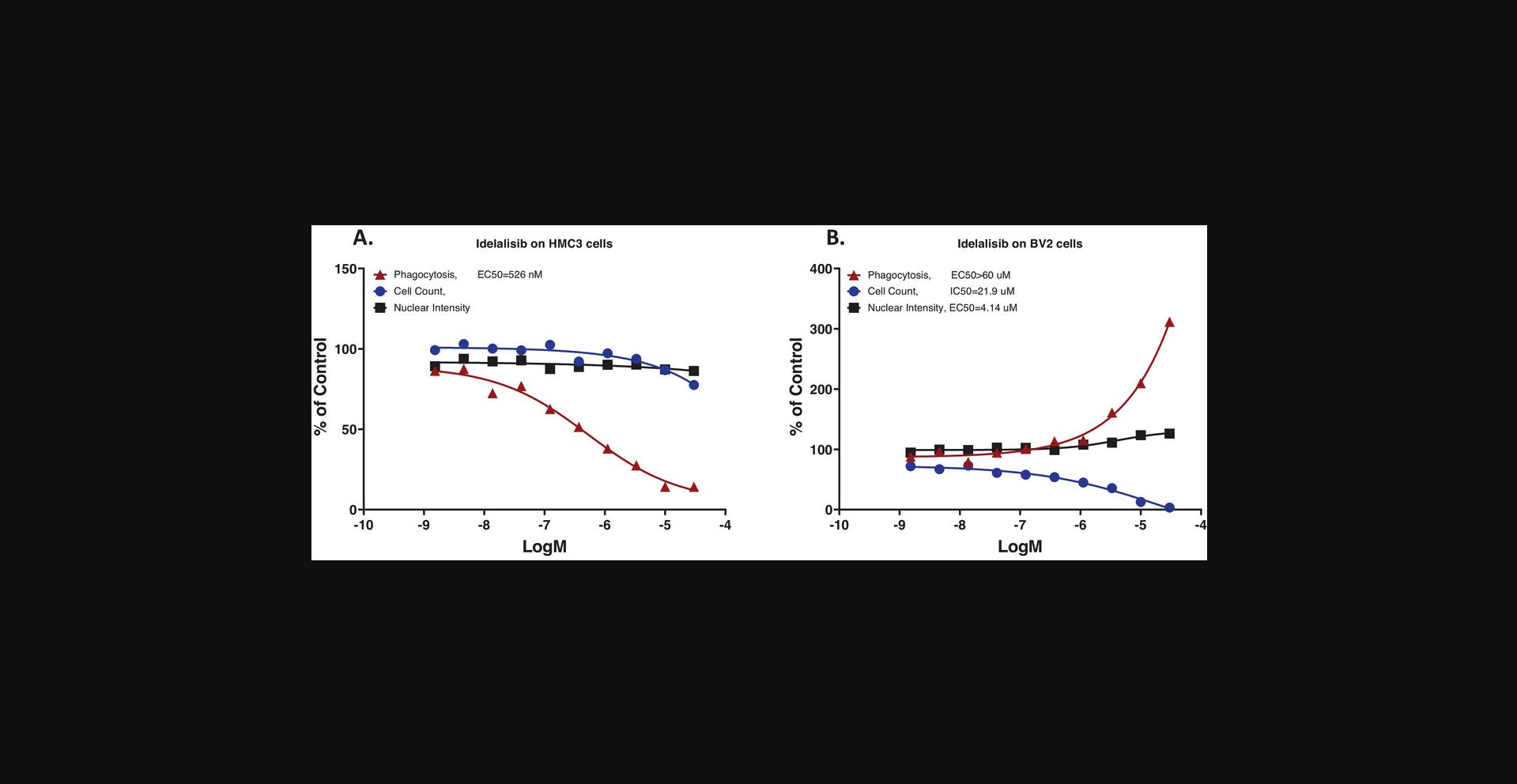
Saracatinib (AZD0530), an inhibitor of the Src/abl family of tyrosine-kinase including Fyn and Lyn, has been repurposed for potential AD therapy and has been in clinical trials (Nygaard, 2018; Nygaard et al., 2015; van Dyck et al., 2019) since some Src kinases have been found to be involved in AD pathogenesis (Dhawan & Combs, 2012). When testing saracatinib in our assay, we observed that it was highly active in stimulating HMC3 cell phagocytosis in the concentration range without cytotoxicity, EC50 <200nM (Fig. 8A and images). In comparison, BV2 cells responded to saracatinib differently, with no stimulated phagocytosis in the sub-μM concentration range, and when concentrations >1 μM showing cell count decreases and biphasic nuclear intensity changes (first increased then decreased), which indicate nuclear condensation followed by DNA degradation (Fig. 8B and images). Together, these results suggest that the phagocytosis increases under the saracatinib treatment as a stress reaction in BV2 cells. Similar results were observed in two repeat experiments. The mechanisms behind the different responses from the two microglial cell lines to saracatinib may be from possible differ in target structures in human versus mouse and remain to be studied. The observation that saracatinib significantly stimulates phagocytosis in HMC3 cells is interesting and may constitute an additional mechanism contributing to the therapeutic efficacy of the drug for AD.
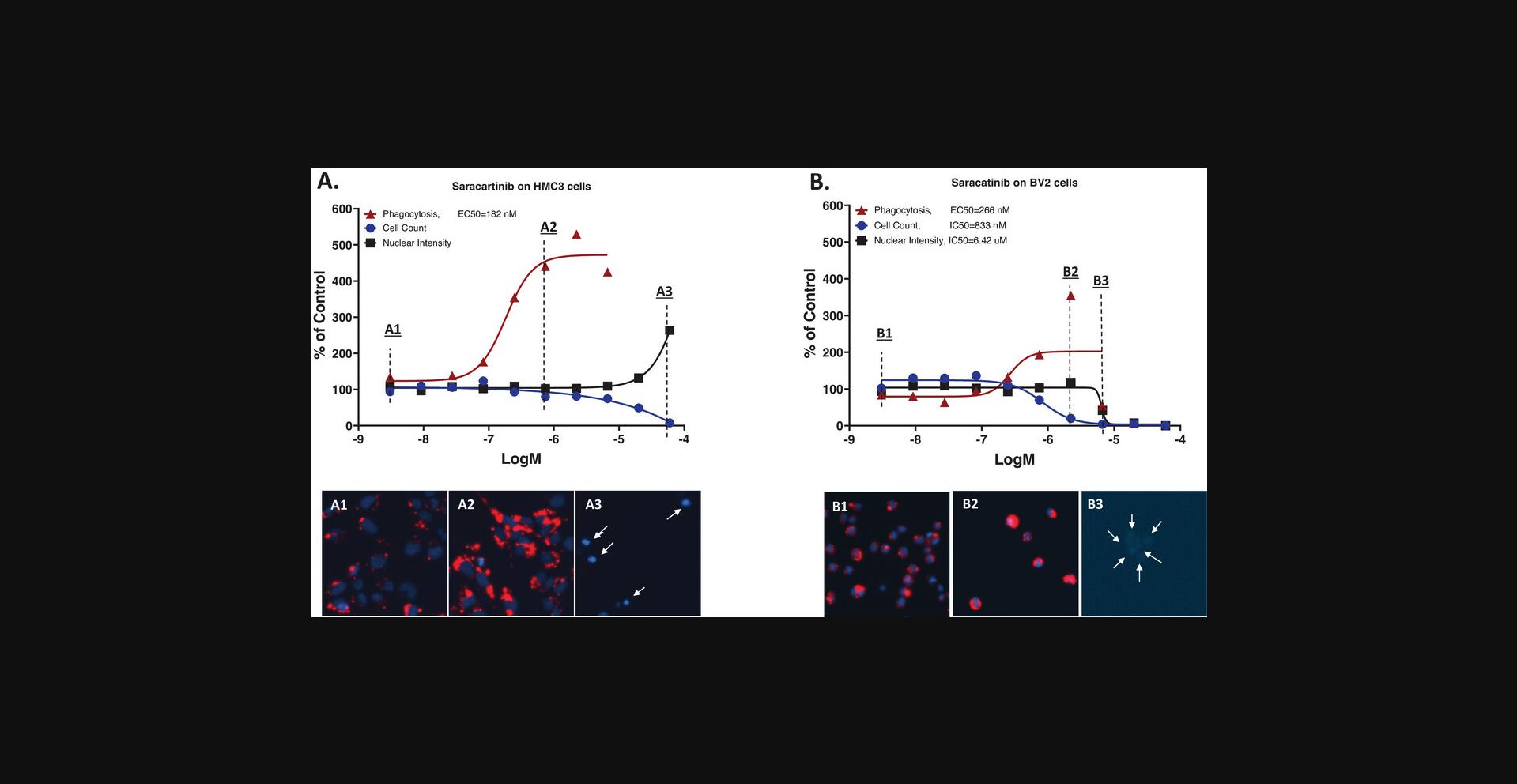
Based on the results of testing idelalisib (inhibition effect) and saracatinib (stimulation effect), we showed that microglial phagocytosis of pHrodo-myelin/membrane debris can be regulated by small molecule drugs; therefore, this assay can serve as a good in vitro model for drug discovery of small molecules targeting specific cellular signaling pathways with the aim to regulate the phagocytosis. We suggest that idelalisib and saracatinib can be used as references for the phagocytosis assay on microglia such as HMC3 cells. The assay was applied for the characterization of chemical probes targeting microglia, with results contributing to two papers (Potjewyd et al., 2022a, 2022b). Currently, the assay is being used in our AD drug discovery projects targeting the TREM2 pathway of microglia, specifically for the selection of INPP5D inhibitors and PLCG2 activators respectively. In conclusion, the microglial phagocytosis and cell health high-content assay provides a high-capacity tool measuring regulation of phagocytosis and cell health simultaneously, which can be helpful for drug discovery studies targeting the microglial phagocytosis.
Time Considerations
Time considerations for the protocols are presented in Table 2.
Step | Time |
---|---|
: Microglial phagocytosis/cell health high-content assay | |
Day 1 | |
Detach and count cells | 20 min |
Plate cells | 1 hr |
Incubation | overnight |
Day 2 | |
Compound plate preparation | 30 min |
Compound distribution to cells | 20 min |
Incubation | overnight |
Day 3 | |
Prep pHrodo-myelin/membrane debris | 20 min |
Add pHrodo-myelin/membrane debris to cells | 20 min |
Incubation | overnight |
Day 4 | |
Nuclear Staining | 45 min |
Imaging | 3 hr |
Data analysis | 3 hr |
: Procedures to isolate myelin/membrane debris from mouse brain and label with pHrodo | |
Prepare solutions | 30 min |
Collect mouse brain tissue | 30 min |
Homogenize brain and prep for ultracentrifugation | 20 min |
Ultracentrifugation | 45 min |
Collect myelin/membrane debris from centrifuge tubes | 10 min |
Wash collected myelin/membrane debris | 60 min |
Sonicate collected myelin/membrane debris | 5 min |
BCA protein assay | 60 min |
Thaw and solubilize sample and reagents | 30 min |
Add dye and NaHCO3 to myelin/membrane debris | 5 min |
Incubate in dark (on shaker) | 45 min |
Remove unbound dye | 60 min |
Resuspend and sonicate labeled myelin/membrane debris | 20 min |
Aliquot labeled myelin/membrane debris | 20 min |
Check prep quality in fluorescent microscope | 30 min |
Set cell culture for testing phagocytosis | overnight |
Check phagocytosis with cells | 5 hr |
Acknowledgments
The research is supported by a grant from the National Institute on Aging, National Institutes of Health, no. U54AG065181 (PIs: Dr. Alan Palkowitz and Dr. Bruce T. Lamb) to TREAD-AD IUSM/Purdue Alzheimer's Disease Drug Discovery Center. The authors want to acknowledge colleagues of the Center for their collaborations, encouragements and teamwork on our drug discovery studies. The authors thank Dr. Jingwei Meng of the Chemical Genomics Core Facility at the Indiana University School of Medicine for helping on applications of the ArrayScan XTI High Content imaging system.
Author Contributions
Shaoyou Chu : Conceptualization, assay development, experiment designs, analysis methods, protocol confirmation, data curation and interpretations, writing manuscript as well as review and editing. Emily Mason : assay development, experiment designs and implementation, collection, analysis, and interpretation of data; writing manuscript as well as review and editing. Disha Soni : assay development, experiment implementation, analysis methods, manuscript reviewing and editing.
Conflict of Interest
The authors declare no conflict of interest.
Open Research
Data Availability Statement
The data that support this study are available from the corresponding author upon reasonable request.
Literature Cited
- Andreone, B. J., Przybyla, L., Llapashtica, C., Rana, A., Davis, S. S., van Lengerich, B., Lin, K., Shi, J., Mei, Y., Astarita, G., Di Paolo, G., Sandmann, T., Monroe, K. M., & Lewcock, J. W. (2020). Alzheimer's-associated PLCgamma2 is a signaling node required for both TREM2 function and the inflammatory response in human microglia. Nature Neuroscience , 23(8), 927–938. https://doi.org/10.1038/s41593-020-0650-6
- Blasi, E., Barluzzi, R., Bocchini, V., Mazzolla, R., & Bistoni, F. (1990). Immortalization of murine microglial cells by a v-raf/v-myc carrying retrovirus. Journal of Neuroimmunology , 27(2-3), 229–237. https://doi.org/10.1016/0165-5728(90)90073-v
- Casella, J. F., Flanagan, M. D., & Lin, S. (1981). Cytochalasin D inhibits actin polymerization and induces depolymerization of actin filaments formed during platelet shape change. Nature , 293(5830), 302–305. https://doi.org/10.1038/293302a0
- Cunningham, C. L., Martinez-Cerdeno, V., & Noctor, S. C. (2013). Microglia regulate the number of neural precursor cells in the developing cerebral cortex. Journal of Neuroscience , 33(10), 4216–4233. https://doi.org/10.1523/Jneurosci.3441-12.2013
- Desale, S. E., & Chinnathambi, S. (2021). Phosphoinositides signaling modulates microglial actin remodeling and phagocytosis in Alzheimer's disease. Cell Communication and Signaling , 19(1), 28. https://doi.org/10.1186/s12964-021-00715-0
- Dhawan, G., & Combs, C. K. (2012). Inhibition of Src kinase activity attenuates amyloid associated microgliosis in a murine model of Alzheimer's disease. Journal of Neuroinflammation , 9, 117. https://doi.org/10.1186/1742-2094-9-117
- Edler, M. K., Mhatre-Winters, I., & Richardson, J. R. (2021). Microglia in aging and Alzheimer's disease: A comparative species review. Cells , 10(5), 1138. https://doi.org/10.3390/cells10051138
- Ennerfelt, H., Frost, E. L., Shapiro, D. A., Holliday, C., Zengeler, K. E., Voithofer, G., Bolte, A. C., Lammert, C. R., Kulas, J. A., Ulland, T. K., & Lukens, J. R. (2022). SYK coordinates neuroprotective microglial responses in neurodegenerative disease. Cell , 185(22), 4135–4152.e4122. https://doi.org/10.1016/j.cell.2022.09.030
- Erwig, M. S., Hesse, D., Jung, R. B., Uecker, M., Kusch, K., Tenzer, S., Jahn, O., & Werner, H. B. (2019). Myelin: Methods for purification and proteome analysis. Oligodendrocytes , 1936, 37–63. https://doi.org/10.1007/978-1-4939-9072-6_3
- Franco-Bocanegra, D. K., Gourari, Y., McAuley, C., Chatelet, D. S., Johnston, D. A., Nicoll, J. A. R., & Boche, D. (2021). Microglial morphology in Alzheimer's disease and after Abeta immunotherapy. Scientific Reports , 11(1), 15955. https://doi.org/10.1038/s41598-021-95535-0
- Gillooly, D. J., Simonsen, A., & Stenmark, H. (2001). Phosphoinositides and phagocytosis. Journal of Cell Biology , 155(1), 15–17. https://doi.org/10.1083/jcb.200109001
- Gyoneva, S., Swanger, S. A., Zhang, J., Weinshenker, D., & Traynelis, S. F. (2016). Altered motility of plaque-associated microglia in a model of Alzheimer's disease. Neuroscience , 330, 410–420. https://doi.org/10.1016/j.neuroscience.2016.05.061
- Harvath, L., & Terle, D. A. (1999). Assay for phagocytosis. Methods in Molecular Biology , 115, 281–290. https://doi.org/10.1385/1-59259-213-9:281
- Janabi, N., Peudenier, S., Heron, B., Ng, K. H., & Tardieu, M. (1995). Establishment of human microglial cell lines after transfection of primary cultures of embryonic microglial cells with the SV40 large T antigen. Neuroscience Letters , 195(2), 105–108. https://doi.org/10.1016/0304-3940(94)11792-h
- Kettenmann, H., Hanisch, U. K., Noda, M., & Verkhratsky, A. (2011). Physiology of microglia. Physiological Reviews , 91(2), 461–553. https://doi.org/10.1152/physrev.00011.2010
- Lewcock, J. W., Schlepckow, K., Di Paolo, G., Tahirovic, S., Monroe, K. M., & Haass, C. (2020). Emerging microglia biology defines novel therapeutic approaches for Alzheimer's disease. Neuron , 108(5), 801–821. https://doi.org/10.1016/j.neuron.2020.09.029
- Li, Q. Y., & Barres, B. A. (2018). Microglia and macrophages in brain homeostasis and disease. Nature Reviews Immunology , 18(4), 225–242. https://doi.org/10.1038/nri.2017.125
- Margadant, C., Cremers, L., Sonnenberg, A., & Boonstra, J. (2013). MAPK uncouples cell cycle progression from cell spreading and cytoskeletal organization in cycling cells. Cellular and Molecular Life Sciences , 70(2), 293–307. https://doi.org/10.1007/s00018-012-1130-2
- Matcovitch-Natan, O., Winter, D. R., Giladi, A., Vargas Aguilar, S., Spinrad, A., Sarrazin, S., Ben-Yehuda, H., David, E., Zelada González, F., Perrin, P., Keren-Shaul, H., Gury, M., Lara-Astaiso, D., Thaiss, C. A., Cohen, M., Bahar Halpern, K., Baruch, K., Deczkowska, A., Lorenzo-Vivas, E., … Amit, I. (2016). Microglia development follows a stepwise program to regulate brain homeostasis. Science , 353(6301), aad8670. https://doi.org/10.1126/science.aad8670
- Meena, J., Goswami, D. G., Anish, C., & Panda, A. K. (2021). Cellular uptake of polylactide particles induces size dependent cytoskeletal remodeling in antigen presenting cells. Biomaterials Science , 9(23), 7962–7976. https://doi.org/10.1039/d1bm01312b
- Melzer, C., von der Ohe, J., & Hass, R. (2019). Involvement of actin cytoskeletal components in breast cancer cell fusion with human mesenchymal stroma/stem-like cells. International Journal of Molecular Sciences , 20(4), 876. https://doi.org/10.3390/ijms20040876
- Mundt, S., Greter, M., & Becher, B. (2022). The CNS mononuclear phagocyte system in health and disease. Neuron , 110(21), 3497–3512. https://doi.org/10.1016/j.neuron.2022.10.005
- Murai, M., Seki, K., Sakurada, J., Usui, A., & Masuda, S. (1993). Effects of cytochalasins B and D on Staphylococcus aureus adherence to and ingestion by mouse renal cells from primary culture. Microbiology and Immunology , 37(1), 69–73. https://doi.org/10.1111/j.1348-0421.1993.tb03181.x
- Nimmerjahn, A., Kirchhoff, F., & Helmchen, F. (2005). Resting microglial cells are highly dynamic surveillants of brain parenchyma in vivo. Science , 308(5726), 1314–1318. https://doi.org/10.1126/science.1110647
- Nizami, S., Hall-Roberts, H., Warrier, S., Cowley, S. A., & Di Daniel, E. (2019). Microglial inflammation and phagocytosis in Alzheimer's disease: Potential therapeutic targets. British Journal of Pharmacology , 176(18), 3515–3532. https://doi.org/10.1111/bph.14618
- Nugent, A. A., Lin, K., Van Lengerich, B., Lianoglou, S., Przybyla, L., Davis, S. S., Llapashtica, C., Wang, J., Kim, D. J., Xia, D., Lucas, A., Baskaran, S., Haddick, P. C. G., Lenser, M., Earr, T. K., Shi, J., Dugas, J. C., Andreone, B. J., Logan, T., … Di Paolo, G. (2020). TREM2 regulates microglial cholesterol metabolism upon chronic phagocytic challenge. Neuron , 105(5), 837–854.e839. https://doi.org/10.1016/j.neuron.2019.12.007
- Nygaard, H. B. (2018). Targeting Fyn kinase in Alzheimer's disease. Biological Psychiatry , 83(4), 369–376. https://doi.org/10.1016/j.biopsych.2017.06.004
- Nygaard, H. B., Wagner, A. F., Bowen, G. S., Good, S. P., MacAvoy, M. G., Strittmatter, K. A., Kaufman, A. C., Rosenberg, B. J., Sekine-Konno, T., Varma, P., Chen, K., Koleske, A. J., Reiman, E. M., Strittmatter, S. M., & van Dyck, C. H. (2015). A phase Ib multiple ascending dose study of the safety, tolerability, and central nervous system availability of AZD0530 (saracatinib) in Alzheimer's disease. Alzheimer's Research & Therapy, 7(1), 35. https://doi.org/10.1186/s13195-015-0119-0
- Phanse, Y., Ramer-Tait, A. E., Friend, S. L., Carrillo-Conde, B., Lueth, P., Oster, C. J., Phillips, G. J., Narasimhan, B., Wannemuehler, M. J., & Bellaire, B. H. (2012). Analyzing cellular internalization of nanoparticles and bacteria by multi-spectral imaging flow cytometry. Journal of Visualized Experiments: JoVE , (64), e3884. https://doi.org/10.3791/3884
- Plescher, M., Seifert, G., Hansen, J. N., Bedner, P., Steinhauser, C., & Halle, A. (2018). Plaque-dependent morphological and electrophysiological heterogeneity of microglia in an Alzheimer's disease mouse model. Glia , 66(7), 1464–1480. https://doi.org/10.1002/glia.23318
- Potjewyd, F. M., Annor-Gyamfi, J. K., Aube, J., Chu, S., Conlon, I. L., Frankowski, K. J., Guduru, S. K. R., Hardy, B. P., Hopkins, M. D., Kinoshita, C., Kireev, D. B., Mason, E. R., Moerk, C. T., Nwogbo, F., Pearce, K. H. Jr., Richardson, T. I., Rogers, D. A., Soni, D. M., Stashko, M., … Axtman, A. D. (2022a). Use of AD Informer Set compounds to explore validity of novel targets in Alzheimer's disease pathology. Alzheimer's & Dementia, 8(1), e12253. https://doi.org/10.1002/trc2.12253
- Potjewyd, F. M., Annor-Gyamfi, J. K., Aube, J., Chu, S., Conlon, I. L., Frankowski, K. J., Guduru, S. K. R., Hardy, B. P., Hopkins, M. D., Kinoshita, C., Kireev, D. B., Mason, E. R., Moerk, C. T., Nwogbo, F., Pearce, K. H. Jr., Richardson, T. I., Rogers, D. A., Soni, D. M., Stashko, M., … Axtman, A. D. (2022b). AD Informer Set: Chemical tools to facilitate Alzheimer's disease drug discovery. Alzheimer's & Dementia, 8(1), e12246. https://doi.org/10.1002/trc2.12246
- Salter, M. W., & Stevens, B. (2017). Microglia emerge as central players in brain disease. Nature Medicine , 23(9), 1018–1027. https://doi.org/10.1038/nm.4397
- Saura, J., Tusell, J. M., & Serratosa, J. (2003). High-yield isolation of murine microglia by mild trypsinization. Glia , 44(3), 183–189. https://doi.org/10.1002/glia.10274
- Somoza, J. R., Koditek, D., Villaseñor, A. G., Novikov, N., Wong, M. H., Liclican, A., Xing, W., Lagpacan, L., Wang, R., Schultz, B. E., Papalia, G. A., Samuel, D., Lad, L., & Mcgrath, M. E. (2015). Structural, biochemical, and biophysical characterization of idelalisib binding to phosphoinositide 3-kinase delta. Journal of Biological Chemistry , 290(13), 8439–8446. https://doi.org/10.1074/jbc.M114.634683
- Streit, W. J., Mrak, R. E., & Griffin, W. S. (2004). Microglia and neuroinflammation: A pathological perspective. Journal of Neuroinflammation , 1(1), 14. https://doi.org/10.1186/1742-2094-1-14
- Taetzsch, T., Levesque, S., Mcgraw, C., Brookins, S., Luqa, R., Bonini, M G., Mason, R P., Oh, U., & Block, M. L. (2015). Redox regulation of NF-kappaB p50 and M1 polarization in microglia. Glia , 63(3), 423–440. https://doi.org/10.1002/glia.22762
- Tilney, L. G., & Portnoy, D. A. (1989). Actin filaments and the growth, movement, and spread of the intracellular bacterial parasite, Listeria monocytogenes. Journal of Cell Biology , 109(4 Pt 1), 1597–1608. https://doi.org/10.1083/jcb.109.4.1597
- Van Dyck, C. H., Nygaard, H. B., Chen, K., Donohue, M. C., Raman, R., Rissman, R. A., Brewer, J. B., Koeppe, R. A., Chow, T. W., Rafii, M. S., Gessert, D., Choi, J., Turner, R. S., Kaye, J. A., Gale, S. A., Reiman, E. M., Aisen, P. S., & Strittmatter, S. M. (2019). Effect of AZD0530 on cerebral metabolic decline in Alzheimer disease: A randomized clinical trial. JAMA Neurology , 76(10), 1219–1229. https://doi.org/10.1001/jamaneurol.2019.2050
- Vance, D. T., Dufresne, J., Florentinus-Mefailoski, A., Tucholska, M., Trimble, W., Grinstein, S., & Marshall, J. G. (2016). A phagocytosis assay for oxidized low-density lipoprotein versus immunoglobulin G-coated microbeads in human U937 macrophages. Analytical Biochemistry , 500, 24–34. https://doi.org/10.1016/j.ab.2016.01.007
- Wake, H., Moorhouse, A. J., Miyamoto, A., & Nabekura, J. (2013). Microglia: Actively surveying and shaping neuronal circuit structure and function. Trends in Neuroscience , 36(4), 209–217. https://doi.org/10.1016/j.tins.2012.11.007
- Witting, A., Muller, P., Herrmann, A., Kettenmann, H., & Nolte, C. (2000). Phagocytic clearance of apoptotic neurons by Microglia/Brain macrophages in vitro: Involvement of lectin-, integrin-, and phosphatidylserine-mediated recognition. Journal of Neurochemistry , 75(3), 1060–1070. https://doi.org/10.1046/j.1471-4159.2000.0751060.x
- Yeo, J. C., Wall, A. A., Stow, J. L., & Hamilton, N. A. (2013). High-throughput quantification of early stages of phagocytosis. Biotechniques , 55(3), 115–124. https://doi.org/10.2144/000114075
Corrections
In this publication, the following corrections have been made:
In the figure legend for the Fig. 6 (B), “EC50 = 256 nM” and “IC50 = 122 nM” have been replaced with the correct amounts.
The current version online now includes these corrections and may be considered the authoritative version of record.
Citing Literature
Number of times cited according to CrossRef: 2
- Ashley Wagner, Zhimin Yan, Marianna Kulka, A Human Microglial Cell Line Expresses γ-Aminobutyric Acid (GABA) Receptors and Responds to GABA and Muscimol by Increasing Production of IL-8, Neuroglia, 10.3390/neuroglia4030012, 4 , 3, (172-187), (2023).
- Cynthia D. Jesudason, Emily R. Mason, Shaoyou Chu, Adrian L. Oblak, June Javens‐Wolfe, Mustapha Moussaif, Greg Durst, Philip Hipskind, Daniel E. Beck, Jiajun Dong, Ovini Amarasinghe, Zhong‐Yin Zhang, Adam K. Hamdani, Kratika Singhal, Andrew D. Mesecar, Sarah Souza, Marlene Jacobson, Jerry Di Salvo, Disha M. Soni, Murugesh Kandasamy, Andrea R. Masters, Sara K Quinney, Suzanne Doolen, Hasi Huhe, Stacey J. Sukoff Rizzo, Bruce T. Lamb, Alan D. Palkowitz, Timothy I. Richardson, SHIP1 therapeutic target enablement: Identification and evaluation of inhibitors for the treatment of late‐onset Alzheimer's disease, Alzheimer's & Dementia: Translational Research & Clinical Interventions, 10.1002/trc2.12429, 9 , 4, (2023).