Experimental Models of Alcohol Use Disorder and Their Application for Pathophysiological Investigations
Valerie Vierkant, Valerie Vierkant, Xueyi Xie, Xueyi Xie, Xuehua Wang, Xuehua Wang, Jun Wang, Jun Wang
Abstract
Alcohol use disorder (AUD) is a complex disorder characterized by compulsive alcohol use and a lack of control over alcohol intake. Several experimental methods using mouse models have been developed to improve research regarding this disorder. Mouse behavioral paradigms are advantageous in inducing alcohol dependence and evaluating alcohol intake, circumventing ethical issues, and increasing experimental control over human-based experiments. These behavioral methods typically fall under one of two categories: forced exposure and voluntary consumption. This paper highlights two common paradigms used to study AUD in rodent models: one forced exposure method (use of a vapor inhalation system for alcohol exposure) and one voluntary consumption method (the two-bottle choice procedure). The effectiveness and experimental validity of these behavioral paradigms for pathophysiological investigations of AUD and how they can be combined are also discussed, along with their individual strengths and weaknesses. © 2023 The Authors. Current Protocols published by Wiley Periodicals LLC.
Basic Protocol 1 : Vapor inhalation for exposure to alcohol
Basic Protocol 2 : Intermittent access two-bottle choice procedure (acquisition)
Basic Protocol 3 : Intermittent access two-bottle choice procedure (measurement)
Alternate Protocol : Sucrose fading to encourage voluntary alcohol consumption
INTRODUCTION
Alcohol use disorder (AUD), characterized by compulsive alcohol use and a lack of control over alcohol intake (Carvalho et al., 2019), is prevalent in the United States. In 2020, around 10% of the population aged 12 and older met the criteria for a diagnosis of AUD, which is associated with approximately 385 deaths every day (National Center for Drug Abuse Statistics, 2023). This condition causes many adverse acute and chronic health issues, such as depression, generalized anxiety disorder, increased risk of hypertension, and brain damage (Cargiulo, 2007). These significant and varied impacts of AUD show that it is a complex disorder requiring further study.
The use of laboratory animal models permits investigations of alcohol addiction without the ethical issues associated with studying the effects of alcohol on humans while still allowing the researcher to assess how alcohol affects complex behaviors (Tabakoff, 2000). In addition, using animal models permits precise experimental control over influences such as environmental and genetic factors (Nieto et al., 2021). The most commonly used animal models in behavioral paradigms for studying AUD are rodent models such as rats and mice (Tabakoff, 2000).
Despite the numerous benefits of working with animal models to study AUD, some complications arise from this approach. Most rodent models, including one of the most commonly used mouse models in AUD research (C57BL/6J), limit their intake of alcohol under normal circumstances and will rarely reach levels of intoxication on their own (Holleran & Winder, 2017; Nieto et al., 2021). This weakness has led to the development of multiple forced intake methods to enhance alcohol administration, such as the alcohol liquid diet, alcohol injection, or exposure to vaporized alcohol. Repeated use of these methods permits the induction of alcohol dependence and drives future voluntary alcohol consumption. However, these models can suffer from low external validity and do not translate into motivated drinking behavior (Holleran & Winder, 2017; Hwa et al., 2011). Other methods that permit the animal to voluntarily consume alcohol, such as the two-bottle choice and operant self-administration procedures, have aimed to increase this area of external validity. These procedures are thought to follow the progression of alcohol dependence followed by humans more closely, and they offer more insight into the behavioral aspects of alcohol drinking (Hwa et al., 2011; Vendruscolo & Roberts, 2014).
This article describes comprehensive methodologies for two common behavioral paradigms used to study alcohol dependence and how they can be applied for pathophysiological investigation. These methods include the intermittent access (IA) two-bottle choice procedure and using a vapor inhalation system for alcohol exposure. The vapor inhalation system is useful for pathophysiological investigations of AUD as it permits the researcher to control the dose, duration, and pattern of alcohol exposure, and it can drive increases in voluntary alcohol-drinking behavior (Gilpin et al., 2008). The IA two-bottle choice paradigm is beneficial because the animal models voluntarily consume alcohol, leading to levels of voluntary alcohol consumption that mimic behaviors similar to binge drinking (a human behavior that is a key risk factor for developing AUD) (Crowley et al., 2019; Hwa et al., 2011). Although both mice and rats can be used for these paradigms, the protocols described in this paper will focus on mice.
NOTE : The Institutional Animal Care & Use Committee (IACUC) for Texas A&M University reviewed and approved all experiments described in this protocol and the overall article. All protocols using live animals must first be reviewed and approved by an IACUC and must follow officially approved procedures for the care and use of laboratory animals.
STRATEGIC PLANNING
Training animals for alcohol consumption or exposure is a complex and time-consuming endeavor requiring repeated exposure. Due to this, researchers should consider the time required to train the animals when designing experimental plans. To fully comprehend the implications of training time, consult the Time Considerations, which provide more detail on this topic. By taking the time to ensure sufficient alcohol consumption/exposure, researchers can ensure the accuracy and reliability of their experimental results.
Basic Protocol 1: VAPOR INHALATION FOR EXPOSURE TO ALCOHOL
Basic Protocol 1 is a widely used method in rodent models to induce alcohol dependence via exposure to alcohol vapor. The procedure involves intermittently exposing rodent models to vaporized alcohol over an extended period, typically from several days to several weeks, to induce alcohol dependence. This protocol is based on the principle that continuous exposure to alcohol vapor produces physiological and behavioral changes similar to those observed in humans with AUD. This protocol requires careful monitoring of animal health and behavior to ensure the reproducibility and accuracy of experimental results.
Materials
-
Mice, 2-3 months old, male/female (e.g., C57BL/6), group-housed
-
200-proof ethanol (Koptex, UN1170, Ethyl Alcohol, 3, PGII)
-
Tap water
-
Vapor inhalation system (Any commercialized vapor system will work. Our system was adapted from Morton et al., 2014)
-
Access to air and vacuum systems
-
Rubber tubing (3/8 inch TYGON tubing)
-
2000-ml glass beaker (Pyrex, no. 5340, filter flask)
-
Standard drinking bottle for water (Tecniplast, animal water bottles, 600 ml)
-
Rat and mouse cubes (Specialty Feeds, SF00-100)
-
Animal cage with wire lid (Tecniplast ULTEM Animal Cages, 2000P001)
1.Construct/assemble the vapor inhalation system if necessary.
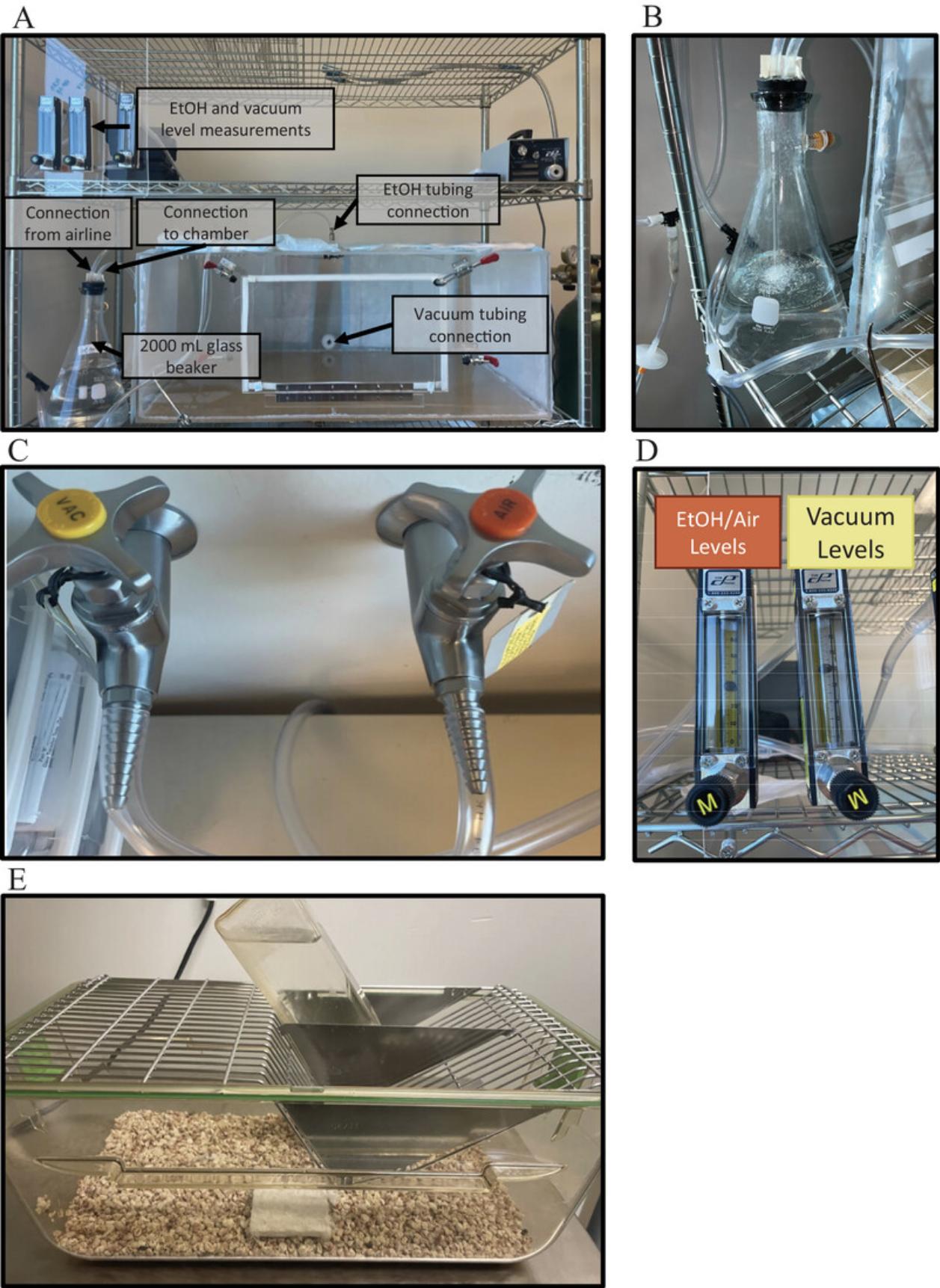
2.Fill the 2000 ml glass beaker with 200-proof ethanol.
3.Connect rubber tubing from the air line to the glass beaker.
4.Connect rubber tubing from the glass beaker to the vapor inhalation system.
5.Connect rubber tubing from the vacuum line to the vapor inhalation system.
6.Place the wire cover over the mouse cage(s).
7.Fill the standard drinking bottle with water.
8.Place the bottle in the cage so the animal can access it.
9.Place rat and mouse cubes on top of the wire cage so the animal can access them.
10.Place the cage(s) in the vapor inhalation system.
11.Turn on the vacuum system.
12.Turn on the air system.
13.Leave mice in the vapor inhalation system for the desired time.
14.Turn off the air and then the vacuum after the allotted time has passed.
15.Remove mice from the vapor inhalation system and return to the home cage.
16.Repeat protocol as often as experimental needs dictate.
Basic Protocol 2: INTERMITTENT ACCESS TWO-BOTTLE CHOICE PROCEDURE-ACQUISITION
Basic Protocols 2 and 3 are commonly used in AUD research to drive high levels of voluntary alcohol consumption or to determine animals’ preference between an alcohol solution versus water. This protocol involves offering rodent models access to both the alcohol solution and water and subsequently measuring the difference in consumption between these two liquids. Animals can be group- or single-housed, although this protocol will focus on group-housed animals. Two primary protocols are employed for group-housed animals: the acquisition phase (Basic Protocol 2) and the measurement phase (Basic Protocol 3). Throughout the procedure, it is essential to adhere to appropriate animal handling and care guidelines to ensure the well-being of the animals. Additionally, precise record-keeping and environmental control are necessary to ensure the reproducibility and accuracy of experimental results.
Materials
-
Mice, 2-3 months old, male/female (e.g., C57BL/6), group-housed
-
200-proof ethanol (Koptex, UN1170, Ethyl Alcohol, 3, PGII)
-
Tap water
-
Mouse home cage (Tecniplast, GM500 for mice, green line)
-
60-ml clear graduated bottles (Qorpak, clear graduated medium round bottles, GLC-01472)
-
Rubber rodent water bottle nozzle (Alternative Design, Neoprene Stopper, and Straight Open Sipper Tube)
-
Standard drinking bottle for water (Tecniplast, animal water bottles, 600 ml)
1.Select the schedule of access to alcohol.
2.Fill a 60-ml bottle with 20% alcohol solution and a standard drinking bottle with water for each cage.
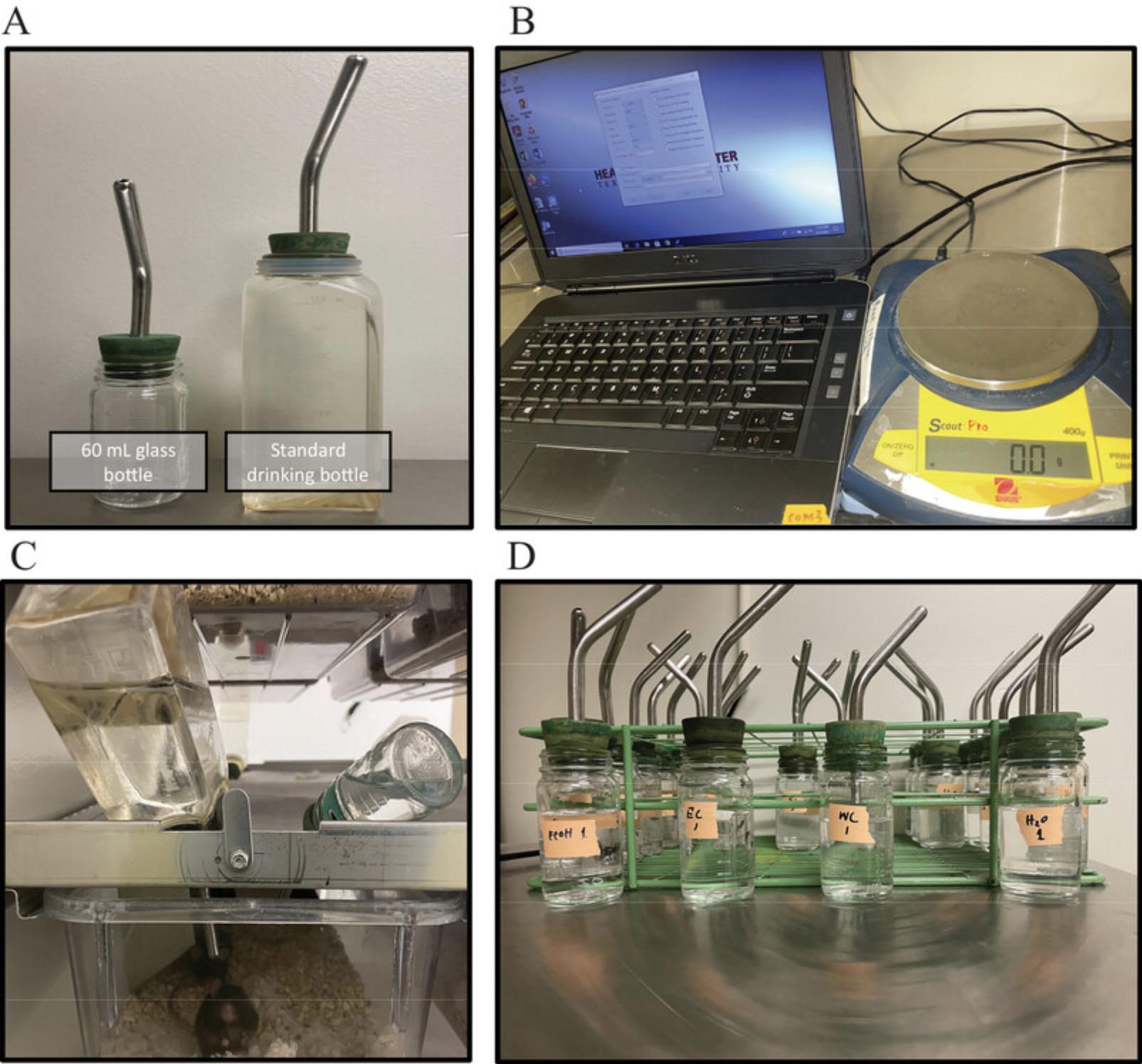
3.Attach rubber water bottle nozzles to each bottle.
4.Place a 60-ml bottle with alcohol and the standard drinking bottle for water into the home cage on days when the mice are scheduled to have access to alcohol.
5.After 24 hr, remove the 60-ml bottle from each home cage.
6.Repeat as necessary for the desired number of sessions.
7.Alternate placement (left or right) of each solution between sessions.
Basic Protocol 3: INTERMITTENT ACCESS TWO-BOTTLE CHOICE PROCEDURE MEASUREMENT
Basic Protocol 3 outlines the measurement phase for the two-bottle choice procedure. During this phase, rodent models are removed from group housing and individually subjected to the two-bottle choice procedure. The mice are given access to both the alcohol solution and water for 24 hr, with their alcohol consumption measured at the end of the 24-hr period. We recommend repeating this procedure at least twice, with an interval of around 24 hr between trials to ensure accuracy and reproducibility. This allows for the recording of the amount of alcohol consumed by individual animals. As discussed in Basic Protocol 2, it is essential to adhere to appropriate animal handling and care guidelines and maintain precise record-keeping and environmental control to ensure the well-being of the animals and the reproducibility and accuracy of experimental results.
Materials
-
Mice used in Basic Protocol 2
-
200-proof ethanol (Koptex, UN1170, Ethyl Alcohol, 3, PGII)
-
Tap water
-
Additional cages (enough for every mouse to be individually housed; Tecniplast, GM500 for mice, green line)
-
60-ml clear graduated bottles (Qorpak, clear graduated medium round bottles, GLC-01472)
-
Rubber rodent water bottle nozzle (i.e., Alternative Design, Neoprene Stopper, and Straight Open Sipper Tube)
-
Digital scale (Scout Pro)
1.Weigh the mice and ensure that the data is recorded.
2.Label each mouse to ensure data can be attributed to individual mice in future sessions.
3.Move mice into individual cages so that they are housed individually rather than group housed.
4.Fill a 60-ml bottle with 20% alcohol and a 60-ml bottle with tap water for each cage.
5.Attach a rubber nozzle to each bottle.
6.Label each alcohol and water bottle according to the cage/animal to which they are assigned.
7.Fill three extra 60-ml bottles with 20% alcohol and three extra 60-ml bottles with water and attach the rubber nozzles.
8.Weigh all bottles to assess starting levels of solution and ensure that the data is recorded.
9.Place alcohol and water bottles in each cage.
10.Place the surplus filled bottles in an empty cage.
11.Weigh all the bottles after 24 hr to assess how much of each solution has been consumed and ensure the data is recorded.
12.Return mice to their home cages.
13.Repeat the procedure at least once more after 24 to 48 hr of alcohol withdrawal.
Alternate Protocol 1: SUCROSE FADING TO ENCOURAGE VOLUNTARY ALCOHOL CONSUMPTION
Alternate Protocol 1 describes the use of sucrose fading within the two-bottle choice procedure. Sucrose fading is used whenever mice refuse to drink alcohol. This method involves initially using a high concentration of sucrose solution to stimulate drinking and gradually reducing the sucrose concentration over time until only alcohol is present. This process is used within the acquisition phase of the two-bottle choice procedure. However, even though consumption is not usually measured during the acquisition phase, measuring it during sucrose fading is useful to determine when the animals have reached higher consumption levels for each new solution. It is important to note that the concentrations of the solutions below are examples, and different concentrations can be used as needed so long as the sucrose is gradually faded out. In addition, if a slower sucrose fading protocol is necessary, then the concentration of alcohol in the solution can initially be reduced and then slowly increased before fading out the sucrose.
Additional Materials (to Basic Protocol 2)
- Purified sucrose crystals (Thermo Fisher Scientific, code: S25590B; Fig. 3B)
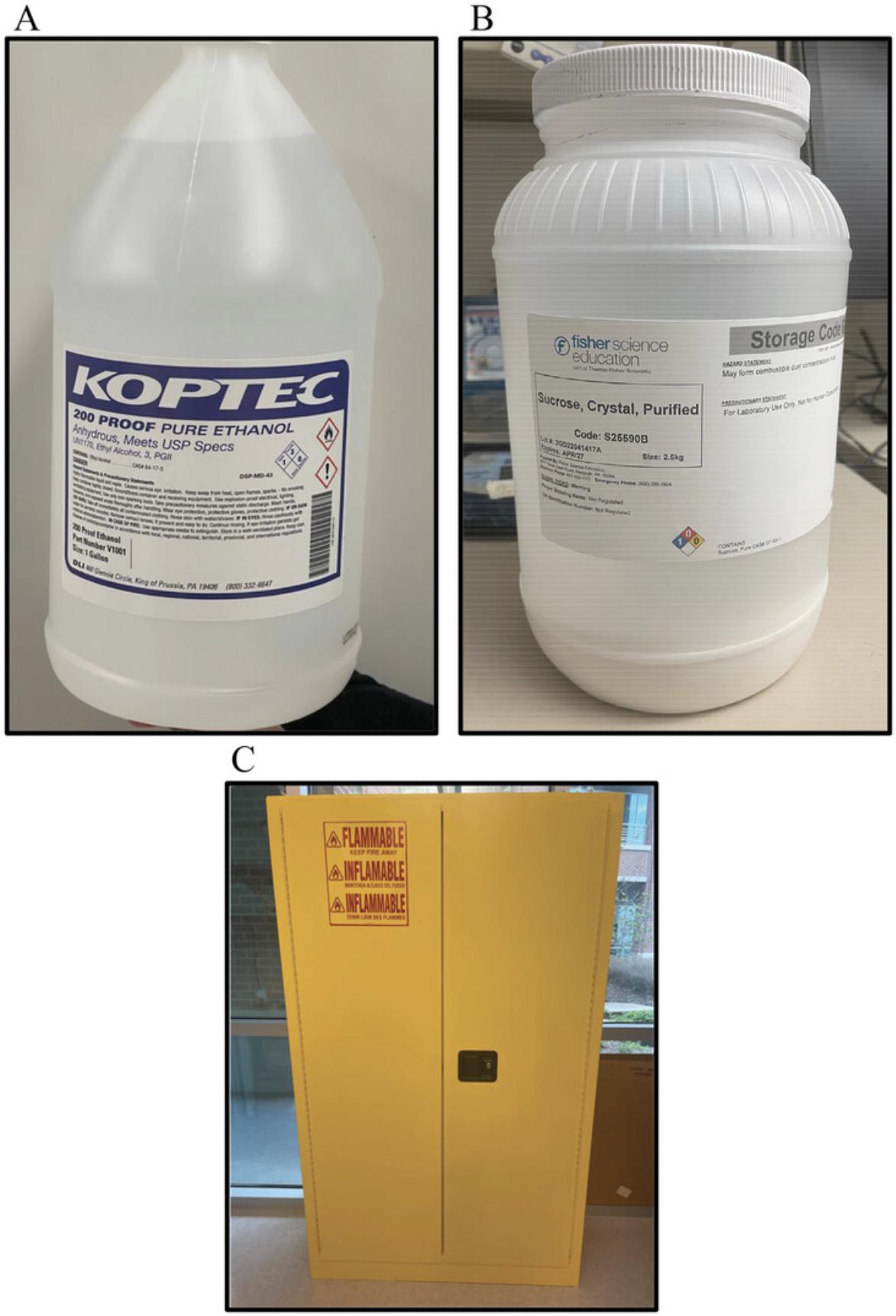
1.Select the schedule of access to alcohol.
2.Prepare 20% alcohol/20% sucrose, 20% alcohol/17% sucrose, 20% alcohol/15% sucrose, 20% alcohol/10% sucrose, 20% alcohol/5% sucrose, and 20% alcohol (0% sucrose).
3.Fill a 60-ml bottle with 20% alcohol/20% sucrose and ensure that the standard drinking bottle for water is filled.
4.Attach rubber nozzle to each bottle.
5.Measure the bottle with the alcohol solution to assess starting levels of solution and ensure that the data is recorded.
6.Place both bottles in the home cage on days when the mice are scheduled to have access to alcohol.
7.Weigh both bottles to assess how much of each solution was consumed by each cage after scheduled access to alcohol has ended and ensure that the data is recorded.
8.Repeat this procedure for the next two sessions with the same solution.
9.Alternate placement (left or right) of each solution between sessions.
10.Switch the alcohol solution to 20% alcohol/17% sucrose after completing the three sessions with 20% alcohol/20% sucrose.
11.Repeat the procedure with 20% alcohol/17% sucrose for three sessions.
12.Switch the alcohol solution to 20% alcohol/15% sucrose after completing the three sessions with 20% alcohol/17% sucrose.
13.Repeat the procedure with 20% alcohol/15% sucrose for three sessions.
14.Switch the alcohol solution to 20% alcohol/10% sucrose after completing the three sessions with 20% alcohol/15% sucrose.
15.Repeat the procedure with 20% alcohol/10% sucrose for three sessions.
16.Switch the alcohol solution to 20% alcohol/5% sucrose after completing the three sessions with 20% alcohol/10% sucrose.
17.Repeat the procedure with 20% alcohol/5% sucrose for three sessions.
18.Switch the alcohol solution to 20% alcohol (with 0% sucrose) after completing the three sessions with 20% alcohol/5% sucrose.
19.Repeat the procedure with 20% alcohol for the remainder of the duration of the two-bottle choice procedure.
REAGENTS AND SOLUTIONS
Alcohol, 20% (v/v)
- 200 ml of 200-proof pure ethanol
- 800 ml tap water
- Mix thoroughly
- Store at room temperature for up to 1 week
Alcohol/Sucrose, 20% (w/v)
- 200 ml of 200-proof pure ethanol
- 800 ml tap water
- 200 g sucrose
- Mix thoroughly
- Store at room temperature for up to 1 week
Alcohol/Sucrose, 20%/17% (w/v)
- 200 ml 200-proof pure ethanol
- 800 ml tap water
- 170 g sucrose
- Mix thoroughly
- Store at room temperature for up to 1 week
Alcohol/Sucrose, 20%/15% (w/v)
- 200 ml of 200 proof pure ethanol
- 800 ml tap water
- 150 g sucrose
- Mix thoroughly
- Store at room temperature for up to 1 week
Alcohol/Sucrose, 20%/10% (w/v)
- 200 ml of 200-proof pure ethanol
- 800 ml tap water
- 100 g sucrose
- Mix thoroughly
- Store at room temperature for up to 1 week
Alcohol/Sucrose, 20%/5% (w/v)
- 200 ml of 200-proof pure ethanol
- 800 ml tap water
- 50 g sucrose
- Mix thoroughly
- Store at room temperature for up to 1 week
Alcohol is classified as a Class IB flammable liquid that should be handled and stored with care. Store 200-proof ethanol (Fig. 3A) in an approved storage cabinet (Fig. 3C). There is no expiration date for 200-proof ethanol.
COMMENTARY
Background Information
Animal models have been employed to study AUD for decades. Several methods have been developed, and most behavioral paradigms fall under one of two categories: forced exposure (i.e., alcohol vapor inhalation) or voluntary consumption (i.e., the two-bottle choice procedure) (Crowley et al., 2019).
Alcohol vapor inhalation is one of the most prevalent forced exposure methods and is a relatively simple method for inducing physical alcohol dependence and driving future alcohol consumption behaviors. This makes it an excellent method to study withdrawal-related behaviors and physiological changes induced by alcohol exposure. The experimenter can control the doses of alcohol the animals receive, reducing the variability associated with consumption by individual animals. However, since forced exposure methods such as the vapor inhalation system do not require voluntary behavior to form alcohol dependency, it has some drawbacks for psychiatric investigations of AUD. The IA two-bottle choice paradigm offers advantages, as it permits a model wherein the animal voluntarily consumes alcohol. Thus, the IA two-bottle choice paradigm may offer more insight into the behavioral aspects of alcohol drinking and may be more beneficial for researchers examining the neuropsychiatric aspects of AUD. However, this benefit comes with the risk of the animals not choosing to drink or developing alcohol dependence.
Due to the drawbacks of the two-bottle choice procedure, it is common to combine alcohol vapor inhalation with a paradigm such as two-bottle choice that permits self-administration. Using the vapor inhalation system prior to using self-administration paradigms may drive higher amounts of alcohol-taking and -seeking behaviors. Figure 4 shows the increase in two-bottle choice response following exposure to alcohol vapor.
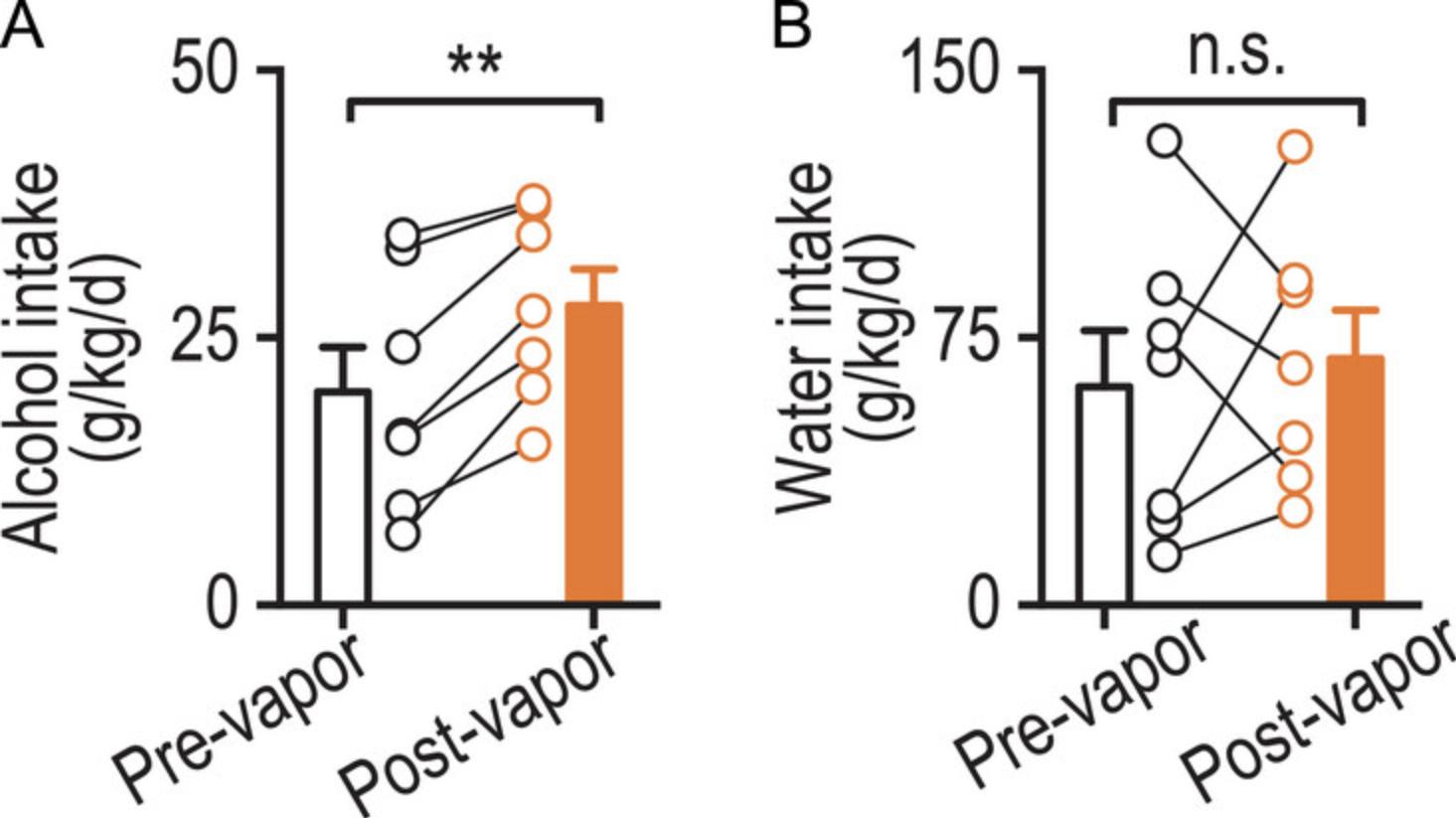
In addition to a vapor inhalation system, sucrose fading, where sucrose is initially added to the alcohol solution and then is slowly reduced over time, is used to help animals with a low alcohol preference respond to and consume alcohol (Tolliver et al., 1988). This method can be used in the two-bottle choice procedure or other voluntary consumption behavioral paradigms such as operant self-administration (see Fig. 5).
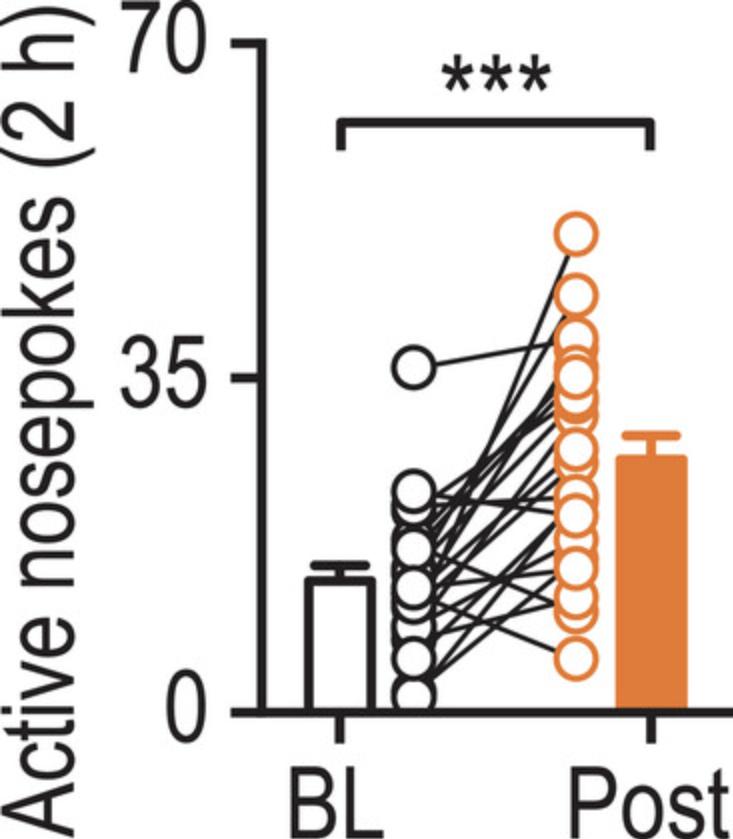
As mentioned, both mice and rats can be used within behavioral paradigms to research AUD. These protocols are delivered in very similar manners. However, a few important factors must be considered when deciding between mice or rats. A primary reason for the preferential use of mice for AUD research is that they have historically been superior genetic models, so more recombinant mouse models are readily available. However, it is important to note that rats are quickly becoming better genetic models due to improvements in genome editing technologies (Ellenbroek & Youn, 2016). At an anatomical level, several differences between rats and mice are relevant for studies into the pathophysiology of AUD. The serotonin receptor 5-HT6 is more highly expressed in rats than in mice, especially within the dorsal and ventral striatum (Ellenbroek & Youn, 2016). These receptors have been implicated in regulating addictive behaviors; for instance, a 5-HT6 receptor antagonist reduced cue-induced reinstatement of alcohol seeking (de Bruin et al., 2013). In the context of ethanol pharmacokinetics in mice versus rats, it has been shown that mice metabolize ethanol at a rate of 550 mg/kg/hr, whereas rats metabolize it at 300 mg/kg/hr. Mice have also been shown to develop sharper blood ethanol concentrations, meaning that they reached a peak blood ethanol concentration more quickly than rats but then rapidly declined from the peak concentration. On the other hand, rats have a more gradual decline from the peak blood ethanol concentration (Livy et al., 2003).
Mice and rats exhibit different alcohol intake and preference behaviors. Mice demonstrate a clear drop in baseline alcohol intake after extended voluntary home cage drinking, whereas rats seem to maintain relatively stable baseline alcohol consumption levels. Additionally, following abstinence from alcohol, rats exhibit increased post-abstinence drinking compared to mice. This relapse-like drinking is also more stable in rats, in which this relapse-like behavior remains consistent, whereas this behavior disappears in mice after around the 8th deprivation phase (Vengeliene et al., 2014). Mice and rats have also demonstrated different behavioral responses in paradigms looking at the motivational properties of alcohol; in the conditioned place preference task (a paradigm that examines the reinforcing properties of alcohol), it is difficult to demonstrate an alcohol place preference in rats, whereas mice have repeatedly been found to demonstrate a place preference. Additionally, in this behavioral paradigm, rats are more likely to show an aversion to alcohol (Tabakoff, 2000). Overall, the anatomical and behavioral differences between rats and mice should be considered when selecting which animal model to employ in pathophysiological investigations of AUD.
In conclusion, animal models have been critical in advancing research on AUD. Forced exposure methods have been used to induce physical dependence and withdrawal-related behaviors, while voluntary consumption methods offer insight into the behavioral aspects of alcohol drinking. By using these methods and techniques, researchers can improve the accuracy and reliability of their experimental results in AUD research.
Critical Parameters
To ensure the ethical conduct of animal research, it is crucial to follow all applicable rules and regulations before commencing any study that involves animal models. This involves obtaining approval from the Institutional Animal Care and Use Committee (IACUC) after reviewing and verifying all protocols. Additionally, all relevant local, institutional, and governmental regulations regarding animal welfare should be strictly adhered to during the study.
Effective training of animal models requires appropriate animal care prior to, during, and after the training process. Typically, rodent models are maintained on a reverse light cycle (where lights are off during the day and on at night) to allow their active phase to occur during the standard workday (Crowley et al., 2019).
The experimenter should also consider whether to house the animals singly or in groups, especially in the context of the two-bottle choice procedure. Social enrichment has been shown to reduce alcohol preference, so single-housed animals may drink more than group-housed animals (Holgate et al., 2017). Animals should be housed according to experimental needs. For the purpose of these protocols, however, it is assumed that the mice are group housed.
Finally, it is important to consider the sex of the animal models. In the two-bottle choice procedure, females drink more than males and display greater anxiety-related behaviors (Li et al., 2019). Thus, sex considerations should be considered when analyzing the behavioral data and interpreting results.
Troubleshooting
A troubleshooting guide detailing the most common problems, possible causes, and solutions for each protocol is provided in Table 1.
Use of a Vapor Inhalation System for Exposure to EtOH | ||
---|---|---|
Problem | Possible cause | Solution |
After turning on the air line, alcohol is not diffusing into the vapor inhalation system | Bad tubing connection | Ensure that the plastic tubing is securely attached to the air line and that the other end is placed in the alcohol |
Animal suffers health issues from vapor inhalation | Concentration of alcohol is too high | Lower the levels of alcohol being pumped into the vapor inhalation system |
Animal fails to establish dependency on alcohol | Concentration of alcohol is too low | Slowly increase the levels of alcohol being pumped into the vapor inhalation system until desired results are seen |
Two-Bottle Choice Procedure | ||
Problem | Possible cause | Solution |
Animal will not consume alcohol | Dislike of the taste of alcohol | Sucrose fading. See Alternate Protocol 1 for details |
Alcohol consumption gradually decreases | Intermittent access is not permitted | Ensure intermittent access. The time between consecutive drinking sessions should be around 24 hr |
Excess leakage from bottles | Insecure/loose seal | Tighten the lid; if that fails, replace the bottle with a new one; if in the measurement phase, make sure to exclude the bottle that leaked from the final data analysis |
Following data analysis, animal has negative levels of consumption for water or alcohol | Animal drank less than the average leakage of water or alcohol | Exclude data from analysis |
Sucrose Fading | ||
Problem | Possible cause | Solution |
Animal does not increase consumption levels following the sucrose fading procedure | Sucrose was faded out of the solution too quickly | Ensure that the animal undergoes a minimum of three sessions with each new concentration of sucrose. Maintain the level of sucrose until the response rate increases |
Statistical Analysis
A good method for statistical analysis of behavioral data is using Excel formulas to document changes in drinking behavior. Additionally, statistical analysis software such as OriginLab/OriginPro or Prism can be used for more complex analyses and producing figures and charts for data visualization.
The most common analysis method for the two-bottle choice procedure is measuring the amount of liquid the animals consumed throughout each session. Using data analysis programs or by hand, calculate the change in levels of alcohol solution (and water () by subtracting the weight of the bottle at 24 hr (B24) from the weight of the bottle at 0 hr (B0). Do this for each animal and each bottle of alcohol solution (Be) and water (Bw). Also calculate the change in the alcohol leak control () and water leak control ( bottles.
After calculating and for each animal, calculate the actual consumption of alcohol (Econ) and water (Wcon) given bottle leakage. To do this, take the average value of both and ( and , respectively). Subtract and from each bottle's and , respectively.
These equations yield and values for each animal tested during the two-bottle choice procedure. For further analysis, it is important to factor in the weight of the animals (acquired in step 1 of Basic Protocol 3) by dividing the and values for each animal by the animal's body weight (BW) in kilograms (kg). This gives the animal's alcohol and water consumption by g/kg/d. The EtOH consumption value must be multiplied by 0.2 because the solution is 20% EtOH. The final data can be used in different statistical analyses for further study depending on the nature of each unique study, such as with a paired t-test.
When applying this data for scientific investigations, it is important to account for baseline variance. One can calculate a percent variance in baseline and set a threshold for the maximum variance allowed. For example, the threshold can be set at 30% (as was done for Fig. 4 in the Understanding Results section). If data from a mouse exceeds this threshold (i.e., 45%), it should be excluded from further analysis. If two days of baseline are used, this percent variance in the baseline for alcohol (BVe) can be calculated by subtracting the first day's Econg/kg/d (Econg/kg/d1) from the second day's Econg/kg/d (Econg/kg/d2), dividing this value by Econg/kg/d1, and then multiplying the resulting value by 100. The same process can be followed for Wcong/kg/d values to calculate the percent variance in baseline for water (BVw). For further detail regarding the use and importance of accounting for the percent variance in baseline, refer to Figure 4.
Table 2 shows raw data obtained from the application of the two-bottle choice protocol before and after exposure to the alcohol vapor inhalation system (see Fig. 4 for a more detailed explanation of the data). Table 3 shows the Econg/kg/d and Wcong/kg/d obtained from these values. The data in Table 3 was developed by following the steps for the statistical analysis described above. Averaged body weights from each home cage were used for the statistical analysis. For alcohol and water bottles 1 through 4, the average weight was 0.03105 kg; for bottles 5 through 8, the average weight was 0.02295 kg; and for bottle 9, the average weight was 0.024 kg. It is important to note that this method should only be employed if all the mice in a cage have similar body weights (this can only occur if all mice are housed with only mice of the same sex and of similar ages). In Table 2, "On" refers to the bottle weight (in grams) when it was placed in the cage and “Off” refers to the weight when it was removed (B0 and B24, respectively). Sessions 1-2 occurred prior to the vapor chamber exposure and sessions 3-5 occurred after. In Table 3, “–” represents data excluded from analysis due to negative consumption levels after factoring in the leak controls or measurement errors such as the bottle leaking excessively.
Alcohol | Session 1 | Session 2 | Session 3 | Session 4 | Session 5 | |||||
---|---|---|---|---|---|---|---|---|---|---|
Bottle # | On | Off | On | Off | On | Off | On | Off | On | Off |
1 | 175.2 | 170.8 | 167.0 | 163.2 | 158.5 | 153.6 | 171.8 | 166.4 | 166.2 | 160.6 |
2 | 168.0 | 163.7 | 159.7 | 155.9 | 150.4 | 145.4 | 170.1 | 165.6 | 165.5 | 161.0 |
3 | 171.7 | 164.9 | 159.5 | 155.9 | 152.1 | 147.8 | 179.8 | 177.4 | 177.3 | 174.5 |
4 | 159.9 | 156.4 | 168.8 | 166.2 | 163.0 | 159.4 | 173.0 | 169.7 | 169.6 | 166.4 |
5 | 160.5 | 154.3 | 147.6 | 142.9 | 157.6 | 151.5 | 175.1 | 170.1 | 169.8 | 164.8 |
6 | 158.0 | 155.0 | 152.5 | 150.7 | 147.0 | 142.9 | 162.9 | 160.0 | 159.8 | 156.7 |
7 | 167.3 | 162.4 | 174.4 | 170.5 | 162.7 | 156.8 | 180.7 | 175.9 | 175.8 | 171.5 |
8 | 173.0 | 167.3 | 162.1 | 156.6 | 151.4 | 145.5 | 166.3 | 161.2 | 161.0 | 155.9 |
9 | 173.6 | 167.9 | 163.0 | 157.1 | 151.9 | 146.3 | 174.8 | 169.9 | 169.7 | 165.1 |
Water | Session 1 | Session 2 | Session 3 | Session 4 | Session 5 | |||||
Bottle # | On | Off | On | Off | On | Off | On | Off | On | Off |
1 | 177.6 | 121.5 | 173.6 | 172.0 | 169.2 | 165.6 | 165.6 | 164.3 | 164.3 | 162.6 |
2 | 176.1 | 173.9 | 170.8 | 169.3 | 167.0 | 165.1 | 165.1 | 164.2 | 164.2 | 162.3 |
3 | 176.9 | 176.1 | 176.2 | 174.3 | 171.4 | 168.5 | 168.5 | 166.4 | 166.4 | 163.7 |
4 | 169.7 | 166.7 | 164.6 | 160.5 | 155.7 | 149.4 | 162.2 | 166.9 | 166.8 | 163.5 |
5 | 174.4 | 170.4 | 167.2 | 164.9 | 163.4 | 162.2 | 162.2 | 160.7 | 160.6 | 158.6 |
6 | 176.2 | 172.8 | 169.3 | 165.8 | 163.8 | 161.0 | 161.0 | 159.1 | 159.1 | 157.0 |
7 | 174.7 | 172.0 | 169.0 | 162.9 | 159.9 | 157.0 | 157.0 | 155.5 | 155.4 | 151.5 |
8 | 179.7 | 177.1 | 173.6 | 172.9 | 169.6 | 166.3 | 166.1 | 163.8 | 163.5 | 160.6 |
9 | 175.0 | 172.1 | 168.8 | 166.3 | 164.0 | 162.4 | 162.4 | 161.0 | 161.0 | 158.2 |
Alcohol Control |
Session 1 | Session 2 | Session 3 | Session 4 | Session 5 | |||||
Bottle # | On | Off | On | Off | On | Off | On | Off | On | Off |
1 | 170.0 | 167.8 | 165.9 | 164.5 | 162.3 | 161.0 | 160.9 | 160.1 | 160.0 | 159.1 |
2 | 169.2 | 167.8 | 166.0 | 165.2 | 163.5 | 162.4 | 162.2 | 161.3 | 161.1 | 160.1 |
3 | 164.6 | 161.7 | 159.9 | 158.8 | 157.1 | 156.0 | 155.9 | 154.9 | 154.8 | 153.6 |
Water Control |
Session 1 | Session 2 | Session 3 | Session 4 | Session 5 | |||||
Bottle # | On | Off | On | Off | On | Off | On | Off | On | Off |
1 | 169.2 | 168.2 | 162.1 | 161.5 | 160.2 | 159.4 | 159.4 | 158.6 | 168.7 | 157.1 |
2 | 181.1 | 180.0 | 178.9 | 178.7 | 178.3 | 177.7 | 177.4 | 176.9 | 176.6 | 176.0 |
3 | 181.4 | 177.6 | 174.3 | 172.5 | 170.6 | 169.5 | 169.3 | 168.8 | 168.7 | 167.7 |
Alcohol | Econg/kg/d | ||||
---|---|---|---|---|---|
Bottle # | Session 1 | Session 2 | Session 3 | Session 4 | Session 5 |
1 | 14.4 | 17.4 | 24.0 | 29.0 | 29.4 |
2 | 13.7 | 17.4 | 24.7 | 23.2 | 22.3 |
3 | 29.8 | 16.1 | 20.2 | 9.7 | 11.4 |
4 | 8.6 | 9.7 | 15.7 | 15.5 | 14.0 |
5 | 35.1 | 31.4 | 43.0 | 35.7 | 34.6 |
6 | 7.3 | 6.1 | 25.6 | 17.4 | 18.0 |
7 | 23.8 | 24.4 | 41.2 | 34.0 | 28.5 |
8 | 30.8 | 38.3 | 41.2 | 36.6 | 35.4 |
9 | 29.4 | 40.0 | 36.9 | 33.3 | 29.7 |
Water | Wcong/kg/d | ||||
Bottle # | Session 1 | Session 2 | Session 3 | Session 4 | Session 5 |
1 | – | 23.6 | 89.1 | 22.5 | 29.0 |
2 | 7.5 | 20.4 | 34.4 | 9.7 | 35.4 |
3 | – | 33.3 | 66.6 | 48.3 | 61.2 |
4 | 33.3 | 104.1 | 176.1 | – | 80.5 |
5 | 88.6 | 62.5 | 16.0 | 39.2 | 52.3 |
6 | 62.5 | 114.7 | 85.7 | 56.6 | 56.6 |
7 | 32.0 | 228.0 | 90.1 | 39.2 | 135.1 |
8 | 27.6 | – | 107.5 | 74.1 | 91.5 |
9 | 38.9 | 68.1 | 31.9 | 33.3 | 83.3 |
Understanding Results
To demonstrate the application of the alcohol vapor inhalation system and two-bottle choice procedure, we conducted an experiment that integrated both methods. Wild-type mice were trained in the acquisition phase of the two-bottle choice procedure for 8 weeks, after which we utilized the two-bottle choice measurement protocol to establish baseline data for alcohol and water consumption. We then exposed the same mice to alcohol vapor inhalation for three 14-hr sessions followed by the two-bottle choice measurement protocol again to assess changes in drinking behavior. To determine the significance of these changes, we conducted a paired t -test analysis on both alcohol and water consumption (obtained using the steps presented in Statistical Analysis). Two mice (individuals 3 and 9, see Table 2) exceeded the percent variance in baseline we permitted (30%; see Statistical Analysis). Following the removal of these mice, there was a statistically significant increase in alcohol consumption post-exposure to vapor inhalation (p = 0.001) (Fig. 4A) but no significant increase in water consumption (Fig. 4B). This data represents the general behavior and patterns of consumption that should be obtained from the animals if following the protocols described within this paper. If the animals do not increase their voluntary consumption following vapor inhalation system exposure, it may be necessary to follow the methods discussed in the troubleshooting section of this paper or to expose the animals to more sessions in the vapor inhalation system.
Several studies have used the two-bottle choice procedure and the vapor inhalation system for pathophysiological investigations into AUD. Table 4 summarizes relevant studies that have utilized these behavioral paradigms in their investigations. Readers can reference these studies to gain a broader understanding of how these protocols can be applied.
Paper | Behavioral paradigm | Primary findings |
---|---|---|
(Ma et al., 2022) | Two-bottle choice (2BC) | 2BC drinking increases glutamatergic transmission from the thalamus to cholinergic interneurons in the dorsomedial striatum. Additionally, 2BC decreased the duration of pulse response in CINs. |
(Cheng et al., 2021) | 2BC | 2BC facilitates operant training. |
(Lu et al., 2019) | 2BC | 2BC causes a long-lasting potentiation of glutamatergic D2→D1 transmission. |
(Cheng et al., 2018) | 2BC | 2BC induces functional and structural plasticity in dopamine d1 receptor-expressing neurons of the dorsomedial striatum. |
(Renteria et al., 2020) | Vapor inhalation system (vapor) | Induction of alcohol dependence through the use of chronic intermittent exposure to alcohol with a vapor inhalation system biased the use of habitual decision-making processes controlling alcohol-seeking behaviors. |
(de Guglielmo et al., 2019) | Vapor | Chronic intermittent alcohol vapor was used to show that neurons in the central nucleus of the amygdala that are activated during alcohol withdrawal contain corticotropin-releasing factor neurons that play a role in the escalation of alcohol drinking and somatic signs of withdrawal. |
(Healey et al., 2020) | Vapor | Acute exposure to alcohol vapor was used to show that acyl-ghrelin (a gut-brain signaling hormone) is elevated in male rats following exposure to alcohol in early adolescence. |
(Finegersh & Homanics, 2014) | Vapor and 2BC | Chronic intermittent alcohol vapor was used to show that paternal alcohol exposure can cause epigenetic modifications within male offspring. The 2BC procedure (albeit with continuous access rather than intermittent access) was used to show that male offspring with paternal alcohol exposure had deceased alcohol preference. |
In addition to the two-bottle choice and vapor inhalation system protocols, we demonstrated the use of sucrose fading in operant self-administration (OSA). Sucrose fading can be used for two-bottle choice procedures and other voluntary consumption procedures such as OSA. We first trained wild-type mice to nose poke for a 20% alcohol, 10% sucrose solution (a common concentration of solution used within OSA) to establish a baseline prior to introducing sucrose. We then implemented an intensive sucrose fading protocol, starting with a solution containing 0% alcohol and 20% sucrose, and gradually increased the alcohol concentration to 20%. We then decreased the sucrose concentration to 10%. After the sucrose fading procedure, there was a significant increase in response to the initial alcohol solution (20% alcohol, 10% sucrose) (Fig. 5). These results support the efficacy of sucrose fading in increasing alcohol consumption in mice and represents the changing patterns of consumption that should be obtained if using sucrose fading to increase animal consumption in voluntary paradigms. If animals do not increase their consumption from baseline, refer to the troubleshooting section.
Time Considerations
Training for both forced and voluntary alcohol consumption is generally a lengthy process requiring multiple exposures. The length of time in an alcohol vapor inhalation system and for the two-bottle choice procedure can vary depending on experimental needs. Typically, however, both procedures require a minimum of days but can continue for up to several weeks. If the alcohol vapor inhalation system is being used to drive future voluntary alcohol drinking in voluntary consumption paradigms, three 14-hr sessions is typical. The acquisition phase of the two-bottle choice procedure can take around eight weeks, whereas the measurement phase may only take 2 sessions/4 days. Adding sucrose fading for the two-bottle choice procedure can vastly increase the length of time necessary to obtain the desired results.
The actual active time for the researcher spent on conducting the alcohol vapor inhalation system protocol is between 5 to 20 min, depending on how much set up is necessary. For the two-bottle choice procedure, active time for the researcher is between 10 to 20 min during the acquisition phase but may take closer to 20 to 30 min during the measurement phase.
Acknowledgments
This research was supported by NIAAA R01AA021505 (JW), R01AA027768 (JW), U01AA025932 (JW), and by an X-grant from the Presidential Excellence Fund at Texas A&M University (JW). The authors declare no competing financial interests.
Author Contributions
Valerie Vierkant : Conceptualization, data curation, formal analysis, investigation, methodology, validation, original draft writing; Xueyi Xie : Conceptualization, data curation, formal analysis, investigation, methodology, validation, visualization, draft review and editing; Xuehua Wang : Resources**; Jun Wang** : Conceptualization, funding acquisition, project administration, resources, supervision, validation, draft review and editing.
Conflict of Interest
The authors declare no conflict of interest.
Open Research
Data Availability Statement
The data that support the findings of this study are available from the corresponding author upon reasonable request.
Literature Cited
- Cargiulo, T. (2007). Understanding the health impact of alcohol dependence. American Journal of Health-System Pharmacy: AJHP: Official Journal of the American Society of Health-System Pharmacists , 64(5 Suppl 3), S5–11. https://doi.org/10.2146/ajhp060647
- Carvalho, A. F., Heilig, M., Perez, A., Probst, C., & Rehm, J. (2019). Alcohol use disorders. The Lancet , 394(10200), 781–792. https://doi.org/10.1016/S0140-6736(19)31775-1
- Cheng, Y., Wang, X., Wei, X., Xie, X., Melo, S., Miranda, R. C., & Wang, J. (2018). Prenatal exposure to alcohol induces functional and structural plasticity in dopamine D1 receptor-expressing neurons of the dorsomedial striatum. Alcohol: Clinical and Experimental Research , 42(8), 1493–1502. https://doi.org/10.1111/acer.13806
- Cheng, Y., Xie, X., Lu, J., Gangal, H., Wang, W., Melo, S., Wang, X., Jerger, J., Woodson, K., Garr, E., Huang, Y., Janak, P., & Wang, J. (2021). Optogenetic induction of orbitostriatal long-term potentiation in the dorsomedial striatum elicits a persistent reduction of alcohol-seeking behavior in rats. Neuropharmacology , 191, 108560. https://doi.org/10.1016/j.neuropharm.2021.108560
- Crowley, N. A., Dao, N. C., Magee, S. N., Bourcier, A. J., & Lowery-Gionta, E. G. (2019). Animal models of alcohol use disorder and the brain: From casual drinking to dependence. Translational Issues in Psychological Science , 5(3), 222–242. https://doi.org/10.1037/tps0000198
- de Bruin, N. M. W. J., McCreary, A. C., van Loevezijn, A., de Vries, T. J., Venhorst, J., van Drimmelen, M., & Kruse, C. G. (2013). A novel highly selective 5-HT6 receptor antagonist attenuates ethanol and nicotine seeking but does not affect inhibitory response control in Wistar rats. Behavioural Brain Research , 236, 157–165. https://doi.org/10.1016/j.bbr.2012.08.048
- de Guglielmo, G., Kallupi, M., Pomrenze, M. B., Crawford, E., Simpson, S., Schweitzer, P., Koob, G. F., Messing, R. O., & George, O. (2019). Inactivation of a CRF-dependent amygdalofugal pathway reverses addiction-like behaviors in alcohol-dependent rats. Nature Communications , 10(1), 1238. https://doi.org/10.1038/s41467-019-09183-0
- Ellenbroek, B., & Youn, J. (2016). Rodent models in neuroscience research: Is it a rat race? Disease Models & Mechanisms, 9(10), 1079–1087. https://doi.org/10.1242/dmm.026120
- Finegersh, A., & Homanics, G. E. (2014). Paternal alcohol exposure reduces alcohol drinking and increases behavioral sensitivity to alcohol selectively in male offspring. PLoS ONE , 9(6), e99078. https://doi.org/10.1371/journal.pone.0099078
- Gilpin, N. W., Richardson, H. N., Cole, M., & Koob, G. F. (2008). Vapor inhalation of alcohol in rats. Current Protocols in Neuroscience , 44(1), Unit 9.29. https://doi.org/10.1002/0471142301.ns0929s44
- Healey, K. L., Landin, J. D., Dubester, K., Kibble, S., Marquardt, K., Brutman, J. N., Davis, J. F., Swartzwelder, H. S., & Chandler, L. J. (2020). Effects of ethanol on plasma ghrelin levels in the rat during early and late adolescence. Alcohol , 85, 111–118. https://doi.org/10.1016/j.alcohol.2019.12.006
- Holgate, J. Y., Garcia, H., Chatterjee, S., & Bartlett, S. E. (2017). Social and environmental enrichment has different effects on ethanol and sucrose consumption in mice. Brain and Behavior , 7(8), e00767. https://doi.org/10.1002/brb3.767
- Holleran, K. M., & Winder, D. G. (2017). Preclinical voluntary drinking models for alcohol abstinence-induced affective disturbances in mice: Negative affect in alcohol abstinence. Genes, Brain and Behavior , 16(1), 8–14. https://doi.org/10.1111/gbb.12338
- Hwa, L. S., Chu, A., Levinson, S. A., Kayyali, T. M., DeBold, J. F., & Miczek, K. A. (2011). Persistent escalation of alcohol drinking in C57BL/6J mice with intermittent access to 20% ethanol. Alcohol: Clinical and Experimental Research , 35(11), 1938–1947. https://doi.org/10.1111/j.1530-0277.2011.01545.x
- Li, J., Chen, P., Han, X., Zuo, W., Mei, Q., Bian, E. Y., Umeugo, J., & Ye, J. (2019). Differences between male and female rats in alcohol drinking, negative affects and neuronal activity after acute and prolonged abstinence. Int J Physiol Pathophysiol Pharmacol , 11(4), 163–176.
- Livy, D. J., Parnell, S. E., & West, J. R. (2003). Blood ethanol concentration profiles: A comparison between rats and mice. Alcohol , 29(3), 165–171. https://doi.org/10.1016/S0741-8329(03)00025-9
- Lu, J., Cheng, Y., Wang, X., Woodson, K., Kemper, C., Disney, E., & Wang, J. (2019). Alcohol intake enhances glutamatergic transmission from D2 receptor-expressing afferents onto D1 receptor-expressing medium spiny neurons in the dorsomedial striatum. Neuropsychopharmacology , 44(6), 1123–1131. https://doi.org/10.1038/s41386-019-0332-9
- Ma, T., Huang, Z., Xie, X., Cheng, Y., Zhuang, X., Childs, M. J., Gangal, H., Wang, X., Smith, L. N., Smith, R. J., Zhou, Y., & Wang, J. (2022). Chronic alcohol drinking persistently suppresses thalamostriatal excitation of cholinergic neurons to impair cognitive flexibility. Journal of Clinical Investigation , 132(4), e154969. https://doi.org/10.1172/JCI154969
- Morton, R. A., Diaz, M. R., Topper, L. A., & Valenzuela, C. F. (2014). Construction of vapor chambers used to expose mice to alcohol during the equivalent of all three trimesters of human development. Journal of Visualized Experiments , 89, 51839. https://doi.org/10.3791/51839
- National Center for & Drug Abuse Statistics. (2023). Alcohol Abuse Statistics [2023]: National + State Data. NCDAS. https://drugabusestatistics.org/alcohol-abuse-statistics/
- Nieto, S. J., Grodin, E. N., Aguirre, C. G., Izquierdo, A., & Ray, L. A. (2021). Translational opportunities in animal and human models to study alcohol use disorder. Translational Psychiatry , 11(1), 496. https://doi.org/10.1038/s41398-021-01615-0
- Renteria, R., Cazares, C., & Gremel, C. M. (2020). Habitual ethanol seeking and licking microstructure of enhanced ethanol self-administration in ethanol-dependent mice. Alcoholism: Clinical and Experimental Research , 44(4), 880–891. https://doi.org/10.1111/acer.14302
- Tabakoff, B. (2000). Animal models in alcohol research. Alcohol Research and Health , 24(2), 77–84.
- Tolliver, G. A., Sadeghi, K. G., & Samson, H. H. (1988). Ethanol preference following the sucrose-fading initiation procedure. Alcohol , 5(1), 9–13. https://doi.org/10.1016/0741-8329(88)90036-5
- Vendruscolo, L. F., & Roberts, A. J. (2014). Operant alcohol self-administration in dependent rats: Focus on the vapor model. Alcohol , 48(3), 277–286. https://doi.org/10.1016/j.alcohol.2013.08.006
- Vengeliene, V., Bilbao, A., & Spanagel, R. (2014). The alcohol deprivation effect model for studying relapse behavior: A comparison between rats and mice. Alcohol , 48(3), 313–320. https://doi.org/10.1016/j.alcohol.2014.03.002
Citing Literature
Number of times cited according to CrossRef: 1
- Valerie Vierkant, Xueyi Xie, Zhenbo Huang, Lian He, Eric Bancroft, Xuehua Wang, Tran Nguyen, Rahul Srinivasan, Yubin Zhou, Jun Wang, Optogenetic inhibition of light‐captured alcohol‐taking striatal engrams facilitates extinction and suppresses reinstatement, Alcohol, Clinical and Experimental Research, 10.1111/acer.15412, 48 , 9, (1728-1739), (2024).