Do-it-Yourself Mock Community Standard for Multi-Step Assessment of Microbiome Protocols
Joanna Colovas, Joanna Colovas, Ari Fina Bintarti, Ari Fina Bintarti, Marco E. Mechan Llontop, Marco E. Mechan Llontop, Keara L. Grady, Keara L. Grady, Ashley Shade, Ashley Shade
Abstract
Microbiomes provide critical functions that support animals, plants, and ecosystems. High-throughput sequencing (HTS) has become an essential tool for the cultivation-independent study of microbiomes found in diverse environments, but requires effective and meaningful controls. One such critical control is a mock microbial community, which is used as a positive control for nucleic acid extraction, marker gene amplification, and sequencing. While mock community standards can be purchased, they can be costly and often include only medically relevant microbial strains that are not expected to be major players in non-human microbiomes. As an alternative, it is possible to design and construct a do-it-yourself (DIY) mock community, which can then be used as a positive control that is specifically customized to the protocol needs of a particular study system. In this article, we describe protocols to select appropriate microbial strains for the construction of a mock community. We first describe the steps to verify the identity of community members via Sanger sequencing. Then, we provide guidance on assembling and storing the DIY mock community as viable whole cells. This includes steps to create standard growth curves referenced to plate counts for each member, so that the community members can be quantified and later compared in terms of their “expected versus returned” relative contributions after sequencing. We also describe appropriate methods for the cryostorage of the fully assembled mock community as viable whole cells, so that they can be used as a unit in a microbiome analysis, from the lysis and nucleic acid extraction steps onwards. Finally, we provide an example of returned data and interpretation of DIY mock community sequences, discussing how to assess possible contamination and identify protocol biases for particular members. Overall, DIY mock communities serve to determine success and possible bias in a cultivation-independent microbiome analysis. © 2022 The Authors. Current Protocols published by Wiley Periodicals LLC.
Basic Protocol 1 : Strain identification and verification using Sanger sequencing
Basic Protocol 2 : Creation of glycerol stocks of each mock community strain for long-term cryostorage
Basic Protocol 3 : Assessment of strain freezer viability without cryoprotectant
Basic Protocol 4 : Creation of standard curve to determine CFU/ml of a liquid culture as a function of optical density
Basic Protocol 5 : Full mock community assembly using community concentration calculations and standard curves
INTRODUCTION
Microbiomes are currently at the forefront of scientific research, gaining much traction since the beginning of the human microbiome project in 2007, which sought to characterize the microbiome of key body sites relevant for human health. Now, interest has broadened to include studying the microbiomes from many environmental systems and non-human animals, including agriculturally relevant crops and critical engineered systems like wastewater treatment. Of the many approaches to study and characterize a microbiome, a relevant and powerful tool is the use of high-throughput sequencing (HTS). HTS can be used as a cultivation-independent method that can provide large amounts of taxonomic or functional information about the microbiome, based on returned DNA sequences. The sequence data provided from HTS can be used to characterize specific microbiomes, and has provided significant insights, but, as for all scientific protocols, several controls are required to ensure a high-quality and robust process. In HTS, controls are used to assess sequence read quality, to estimate microbiome coverage, to provide evidence that protocols worked as expected, and to identify biases and contamination.
In a typical HTS workflow to evaluate a microbial community, a sample is first collected from the study site of interest. Next, nucleic acid, typically DNA, is extracted from the whole sample, to produce a mixture of metagenomic nucleic acids from many microorganisms of the original sample. The quality and quantity of nucleic acid is then assessed using agarose gel electrophoresis or spectrophotometric or comparable methods (e.g., Qubit, Nanodrop, PicoGreen, BioAnalyzer). If the study includes marker gene sequencing, a PCR with the appropriate primers is performed, to ensure that amplification is possible from the metagenomic DNA. Finally, DNA is sequenced via HTS (e.g., using Illumina, Oxford Nanopore, or PacBio platforms) and analyzed appropriately for the chosen platform. Untargeted sequencing returns sequences from any genome location of the microbiome members, while targeted sequencing returns information only for a gene of interest (e.g., 16S rRNA gene or ITS gene as marker genes).
Key for microbiome HTS studies is the use of proper controls. One important positive control is the use of a mock community standard. This positive control is useful for assessing multiple parts of the laboratory protocols leading to and including the sequencing of DNA, including cell lysis and DNA extraction, and marker gene amplification using PCR (Eisenhofer et al., 2019; Nguyen, Smith, Peay, & Kennedy, 2015). A mock microbial community standard consists of either whole cells of the different microbial strains, or their extracted DNA, with the former used from the protocol's initial lysis step onward and the latter used from the amplification step onward. Here, we focus on the whole-cell standard that contains known proportions of cells from different taxa, because it can be processed alongside experimental samples through all laboratory protocols and then sequenced, to properly assess: (1) DNA and RNA extraction, including molecular weight and relative concentration; (2) marker gene amplification; (3) sequencing; (4) contamination; and (5) taxonomic bias in protocols based on mock community composition. For marker gene analyses, mock communities can also be used to determine error rates in sequencing (Knight, Vrbanac, & Taylor, 2018; Schloss et al., 2009) and to inform decisions for bioinformatically determining operational taxonomic units (OTUs). They can also be used as a “gold standard” to assess bioinformatic tools and outcomes based on known, simple communities (Bokulich et al., 2013; Karstens et al., 2019). The Mothur suite and SOP, first developed in 2009 by Schloss and colleagues, provides the theoretical basis for the development and usage of a custom mock community (see Internet Resources).
There are several mock community standards available for purchase for microbiome analyses, such as those from ZymoBIOMICS, the NITE Biological Resource Center (NBRC), the American Type Culture Collection (ATCC), and more (Tang, 2017). For-purchase mock community standards, however, may not be ideal for every study system, for various reasons. First, they may not necessarily have a taxonomic representation that is relevant to the environmental system of interest. This simply reflects the market in microbiome research: there is more research funding available for biomedical research, and so the biggest consumer base will be most interested in human microbiome strains assembled into mock communities (Highlander, 2014). This will be of limited relevance to those studying non-human systems. A positive sequencing control that better resembles the study system is necessary to more precisely evaluate the lysis and amplification of common microbial members found in the system. This allows for quantification of issues pertaining to nucleic acid extraction success (such as that from particular system members), and the assessment of marker gene amplification and sequencing success from particular system members, as well as the estimation of taxonomic bias in a sample. Second, these standards can be costly and, therefore, could limit experimental designs or force researchers to opt for suboptimal designs with reduced experimental controls when budgets are limited.
In this context, it could be useful to construct a do-it-yourself (DIY) mock community control that is not only better aligned to the taxa and needs of a particular experiment or environmental system, but is also more economical. The former consideration is especially important for environmental systems that contain difficult-to-lyse members that are expected to be present in high abundance, or for studies that are targeting a particular member that is cultivable but not available in off-the-shelf mock communities. Processing the mock community (cells) through DNA extraction, alongside experimental samples, through sequencing, will allow researchers to assess the effectiveness of lysis of the members of interest and, therefore, representation in the ultimate dataset via sequence analysis.
Here, we provide a generalized protocol for making a simple mock community (Figure 1). We provide guidance for choosing strains for a mock community standard made from whole cells, and what information must be known a priori about each included strain. After choosing strains and acquiring stocks, they must be verified, quantified using standard growth curves with plating, and then assembled together in defined quantities to produce an expected mock community composition. Then, these need to be cryopreserved, to maintain cell viability. These steps are necessary to make certain that all strains are correctly identified and properly stored, and can survive freezing during mock community assembly and usage. Freezer viability can also be assessed without cryoprotectant, which may interfere with nucleic acid extraction or other downstream molecular protocols. This protocol provides a resource to construct DIY mock community for use as a system-specific and low-cost positive control.
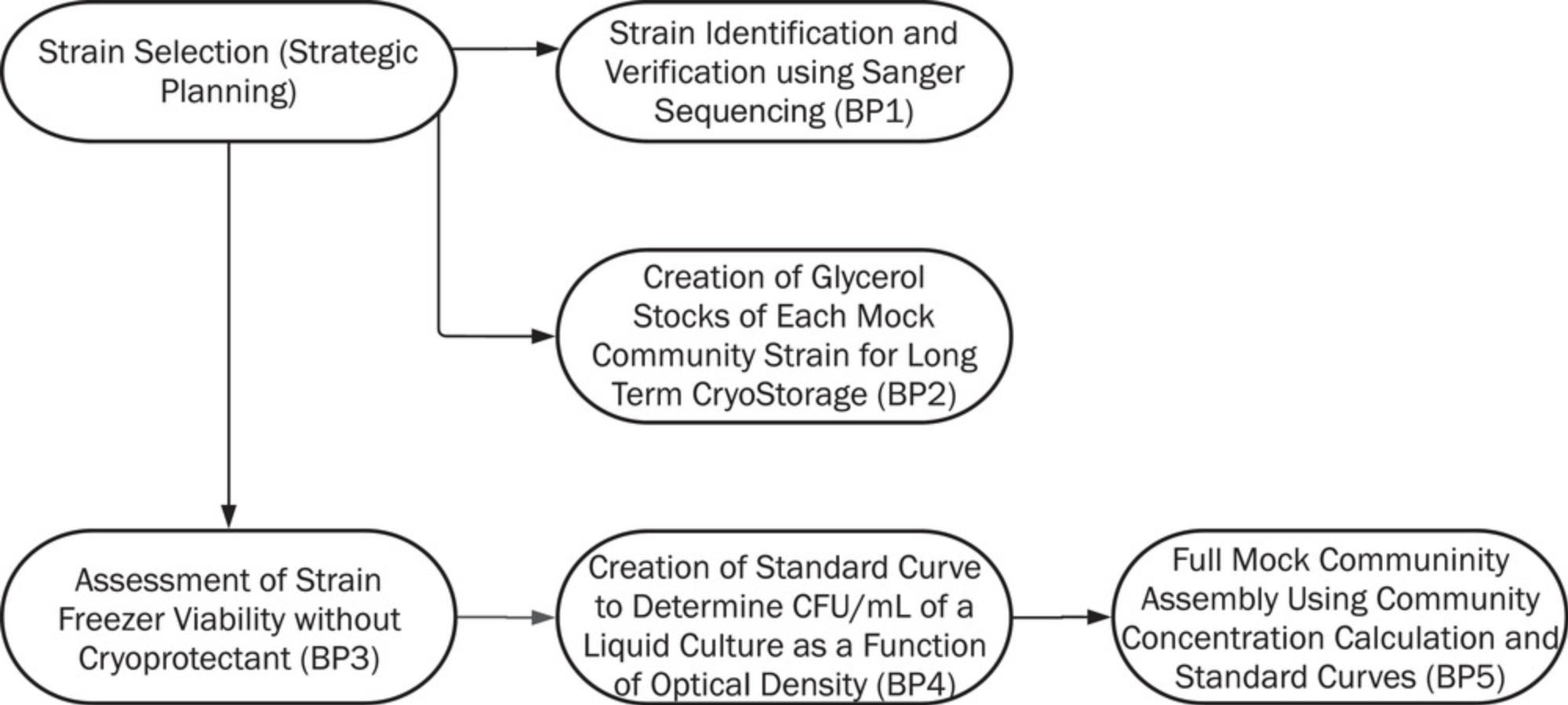
In Basic Protocol 1, we describe how each strain chosen for the mock community is verified using Sanger sequencing. This protocol allows users to confirm the identity of each member and obtain a reference sequence for a marker gene region such as 16S rRNA or ITS regions. In Basic Protocol 2, we provide steps to create glycerol stocks of each mock community member for long-term cryostorage at −80ºC. As we recommend that mock community aliquots be stored without a cryoprotectant for ease of use, Basic Protocol 3 assesses the viability of each strain after storage as pelleted cells at −80ºC. In Basic Protocol 4, users will create a standard growth curve for each strain to determine CFU/ml of liquid culture as a function of optical density. Finally, in Basic Protocol 5, the standard curves are applied to assemble the mock community with standardized input for each member. Figure 1 shows an overview and the connectivity of all the protocols included.
STRATEGIC PLANNING
While DIY mock community design, creation, and assembly present a significant up-front time investment, several of the protocols can be completed in parallel, for example, strain verification and standard curve creation. Additionally, Basic Protocols 1–4 only need to be completed once per strain, and can then be used to recreate or make a DIY mock community that includes the strain. Basic Protocol 5 can be repeated as needed to generate freezer stocks of mock community aliquots, and we recommend using a minimum of one mock community aliquot per set of nucleic acid extractions from the study system.
For several of the following protocols, prepared liquid cultures or DNA from each strain is used. For Basic Protocol 1, genomic DNA (gDNA) from each included strain is needed for Sanger sequencing. gDNA should be available for each strain in the mock community at a concentration of 12-20 ng/µl (50-200 ng total DNA needed). As each strain may require a different extraction method to obtain quality DNA with high molecular weight, this step is left to be determined by researchers based on their experience with nucleic acid extraction protocols for the same or related strains. Different strains used in the same mock community may require different genomic DNA extraction protocols (e.g., due to different lysis requirements), and it is not necessary to use the same extraction for all included strains. We recommend checking DNA quality and performing a gel extraction on the PCR product to achieve high-quality Sanger sequences. In Basic Protocols 2–5, liquid culture grown to stationary phase is needed in varying quantities. Check each protocol for specific amounts. During the preparation of liquid culture to stationary phase, begin each culture by inoculating a pure, single colony of each strain from solid agar medium into the required amount of liquid medium (see Troubleshooting for other alternatives). Then, shake and incubate overnight (12-24 hr) at an appropriate growth temperature, which may differ by strain. In Basic Protocols 2 and 3, approximately 5 ml of overnight liquid culture is necessary for each strain. Observe overnight liquid cultures for turbidity after incubation.
Selecting strains for the DIY mock community
All strains selected to be included in the DIY mock community must be cultivable in the laboratory. These strains can be acquired from biological strain collection supply companies, such as the American Type Culture Collection (ATCC) or the German Collection of Microorganisms and Cell Culture (DSMZ), or from well-curated, in-laboratory strain collections. When possible, strains should be available to other researchers. If possible, the mock community should include representative strains from the major expected lineages in the focal environment. If strains are not culturable, consider including close relatives. If representative strains are yet unknown for a new study system, it is worthwhile to create a diversified mock community that contains phylogenetically distributed lineages. Major considerations include: multi-domain diversity (bacteria, archaea, and eukaryotes including fungi or microeukaryotes; Bakker, 2018; Lindahl et al., 2013); representative diversity in cell wall/membrane structure among selected strains that reflect that of the study system; and inclusion of taxa of interest for the research question that are important to ensure representation. For example, for cell lysis considerations, phyllosphere bacteria often require augmented protocols to lyse UV- and desiccation-protected cells, and so a mock community relevant for the phyllosphere should include cells with such features among the selected strains. If desired, endospore formers or other differentiated structure-forming bacteria or fungi may be included to be further representative of microbes with difficult-to-lyse structures, if their inclusion is relevant to the driving research question.
We recommend that users aim to prepare a DIY mock community comprising 6-15 members. However, there are certainly examples of mock communities that contain fewer or more members—this is at the discretion of the researcher, and should be informed by expectations of the targeted microbiome. For many environments, it is not feasible to manually curate a mock community that has richness (number of unique species) equivalent to the expected richness in the focal study system, which, depending on the environment, can include dozens to tens of thousands of taxa. However, it may be possible to include all represented taxa from synthetic communities, or simple engineered systems that have dozens or fewer taxa. Generally, low richness of mock communities relative to that of highly diverse microbiomes is expected to result in more sequence variants per included strain, because of both errors that accumulate during PCR amplification and the low diversity of the available target sequences (e.g., making many copies of few targets can propagate errors; Schloss, Gevers, & Westcott, 2011.)
To summarize, selecting strains for the mock community requires knowledge of several aspects of each strain as well as of the study system of interest. Contemplate the diversity of bacterial, fungal, or other microbial lineages found in the study system, and determine if there are particular members that dominate the system or are otherwise important to the ecology of the community. Taxonomic breadth should include Gram-positive and Gram-negative species, as well as any known members of the study system with other types of cell walls, membranes, or structures that would cause difficulty in cell lysis, such as the presence of spore formation. Beyond lysis efficiency, consider the GC content of the DNA of each member of the mock community. This helps inform biases in DNA extraction efficiency and during downstream processes.
At a minimum, the 16S ribosomal RNA (rRNA) sequence of each bacterial stain; the ITS1 and ITS2 regions of each fungal strain; or other marker gene information should be known for each included strain a priori. This informs the resulting sequencing data in terms of sequencing success, contamination, and taxonomic bias in protocols, and can be obtained from databases such as UNITE and rrnDB (Nilsson et al., 2018; Stoddard, Smith, Hein, Roller, & Schmidt, 2015). The sequence of each copy of the marker gene per included strain should also be known a priori , if possible. Copy number of these marker genes as well as number of unique copies becomes important when first examining the amplification of sequences, and also when clustering sequence reads into taxonomic units. The number of unique copies of the marker gene for each strain and the percent sequence identity among them will inform the expected number of taxa after mock community sequencing. This is especially important when operational taxonomic units (OTUs) are based on 100% sequence identity (e.g., using the amplicon sequence variant (ASV), zOTU, or ESV definitions at 100%; Starke, Pylro, & Morais, 2020; Strube, 2021), as each unique copy of a marker gene will become a distinct taxonomic unit, including any propagated PCR errors. The gene copy numbers for many cultivable strains or close relatives are available in sequenced genomes in the National Center for Biotechnology Information (NCBI) database or, in the case of the 16S rRNA gene, in the rrnDB (https://rrndb.umms.med.umich.edu/; Stoddard et al., 2015). If the genomic information for a strain is not available a priori , high-quality draft whole-genome sequencing or marker gene amplification with Sanger sequencing to detect consistent mutations in the gene can inform as to gene copy numbers and variability among them. Optional but useful information is the amplification efficiency of the marker gene for each strain.
Medium considerations
As each mock community will have a different composition of members, researchers should use the literature and their own experience with the included strains to select appropriate growth media. Both solid and liquid media are required for different parts of the protocol. We list this below, for planning purposes.
Total Medium/Strain
- ∼38 solid agar plates
- ∼400 ml liquid medium
Basic Protocol 1: Strain Identification and Verification Using Sanger Sequencing
- 2 solid agar plates/strain (before protocol begins)
- 5 ml liquid medium/strain
Basic Protocol 2: Creation of Glycerol Stocks of Each Mock Community Strain for Long-Term Cryostorage
- 2 solid agar plates/strain (before protocol begins)
- 5 ml liquid medium/strain
Basic Protocol 3: Assessment of Strain Freezer Viability Without Cryoprotectant
- 2 solid agar plates/strain
- 5 ml liquid medium/strain
Basic Protocol 4: Creation of Standard Curve to Determine CFU/ml of a Liquid Culture as a Function of Optical Density
- 30 solid agar plates/strain
- 350 ml liquid medium/strain (may change depending on dilution volume)
Basic Protocol 5: Full Mock Community Assembly Using Community Concentration Calculations and Standard Curves
- 2 solid agar plates/strain (before protocol begins)
- 25 ml liquid medium/strain
Basic Protocol 1: STRAIN IDENTIFICATION AND VERIFICATION USING SANGER SEQUENCING
After choosing the desired cultivable bacterial and fungal strains for a DIY mock community (see Strategic Planning), it is important to verify the identity of each chosen strain once procured, as well as to have known and verified marker gene sequences of each strain if a targeted sequencing approach is used. This is what the user will do in Basic Protocol 1, via Sanger sequencing of gDNA previously extracted by users according to optimized protocols for their strains (Strategic Planning). DNA should be extracted from each culture and examined using agarose gel electrophoresis, to be sure that it is of sufficiently high quality and molecular weight for amplification and sequencing.
Here, PCR with a high-fidelity polymerase will be used to amplify the appropriate target gene from genomic DNA. The PCR products are then cleaned using agarose gel extraction and submitted for Sanger sequencing to a genomics facility. When reads are returned, the forward and reverse reads of the target gene are merged using a sequence analysis program to identify their overlapping regions and build a consensus sequence. Agreement in the overlapping regions of the forward and reverse read allows greater confidence in the consensus. Using the curated consensus sequence and the NCBI BLAST algorithm (Altschul, Gish, Miller, Myers, & Lipman, 1990), each strain is identified with respect to its nearest taxonomic relatives.
Materials
- Nuclease-free water (Fisher Scientific, cat. no. BP561-1)
- Phusion High-Fidelity DNA Polymerase (New England Biolabs, cat. no. M0530S) and 5× buffer
- Deoxynucleotide triphosphates (dNTPs; New England Biolabs, cat. no. N0447S)
- Dimethylsulfoxide (DMSO)
- PCR primers appropriate for each marker gene region, (IDT, 100 µM stock; 20 µM working primers; See Table 1 for examples)
- Template genomic DNA from each community member, extracted from pure culture: 12-20 ng/µl (50-200 ng total DNA; Strategic Planning)
- TBE buffer (see recipe)
- 1 Kb DNA ladder (New England Biolabs, cat. no. N3232S)
- Ethidium bromide (Sigma-Aldrich, cat. no. E1510) or other DNA visualization stain
- Monarch Gel Extraction Kit (New England Biolabs, cat. no. T1020S)
Primer | Sequence (5′-3′) | Reference |
---|---|---|
Bacterial universal 27F | AGA GTT TGA TCC TGG CTC AG | Lane (1991) |
Bacterial universal 1492R | GGT TAC CTT GTT ACG ACT T | |
Fungal ITS1-F | TCC GTA GGT GAA CCT GCG G | Kageyama, Ohyama, and Hyakumachi (1997) |
Fungal ITS4-R | TCC TCC GCT TAT TGA TAT GC |
-
Thermocycler
-
Labeled 1.5-ml microcentrifuge tubes
-
UV light source
-
UV protection
-
Razor blade
-
Balance
-
Vortex
-
Microcentrifuge
-
Dry block incubator or water bath for 1.5-ml microcentrifuge tubes
-
Facility for performing Sanger sequencing (also see Current Protocols article: Shendure et al., 2011)
-
Software for analyzing Sanger sequencing data
-
Additional reagents and equipment for the polymerase chain reaction (see Current Protocols article: Kramer & Coen, 2001), agarose gel electrophoresis (see Current Protocols article: Voytas, 2001), and Sanger sequencing (see Current Protocols article: Shendure et al., 2011)
Phusion PCR reaction
Details of PCR are provided in Kramer & Coen (2001).
1.For each DNA sample, set up a PCR reaction using the relevant primer pair for the sample (refer to Table 1 for recommended relevant primer pairs), as follows:
Reagent | Volume per reaction |
Nuclease-free water | 23.6 µl |
5× Phusion buffer | 8 µl |
10 µM dNTPs | 0.8 µl |
100% DMSO | 1.2 µl |
20 µM forward primer | 1.0 µl |
20 µM reverse primer | 1.0 µl |
50 units/µl Phusion DNA polymerase | 0.4 µl |
DNA template (12-60 ng/µl) |
4 µl |
Total volume per reaction | 40 µl |
2.Run the PCR with the following conditions, depending on the target gene:
16S full-length for bacteria (e.g., primers 27F and 1492R) | ITS full length for fungi (e.g., primers ITS1F and ITS4R) | |||
Step | Temperature | Time | Temperature | Time |
1. Initial denaturation | 9°C | 1 min | 9°C | 1 min |
2. Denaturation | 9°C | 10 s | 9°C | 10 s |
3. Annealing | 5°C | 20 s | 5°C | 20 s |
4. Extension | 7°C | 45 s | 7°C | 20 s |
5. Go to step 2 (×25 cycles) | - | - | - | - |
5. Final extension | 7°C | 5 min | 7°C | 5 min |
6. Hold | 4°C | ∞ | 4°C | ∞ |
Lid | 9°C | - | 9°C | - |
Running the sample on a gel and gel extraction using Monarch Gel Extraction Kit
All centrifugation steps should be completed at 16,000 × g and at room temperature.
3.Prepare a 1% (w/v) agarose gel using TBE buffer with DNA gel visualization stain (here, ethidium bromide) at a volume that matches the gel casting apparatus of choice, and let solidify with a sample comb.
4.Once the gel has solidified, remove sample combs and fill gel electrophoresis apparatus with TBE buffer.
5.Add a 1 Kb DNA ladder to one well of the agarose gel. Add all of the PCR product from each reaction to a different well of the agarose gel.
6.Run the gel with voltage and time parameters recommended by the manufacturer, and then visualize using ethidium bromide (Voytas, 2001) or other DNA visualization stain to ensure that DNA bands are the correct size.
7.While the gel is running, weigh and label 1.5-ml microcentrifuge tubes, using one per sample. Pre-weigh each tube.
8.After running the gel, and using a UV light box, excise each desired gel band using a razor blade and transfer to the appropriately labeled 1.5-ml microcentrifuge tube.
9.Weigh the gel slice inside the tube (subtracting previously recorded weight of tube alone) and add 4 volumes of Gel Dissolving Buffer (from the Monarch kit) to the gel slice.
10.Incubate the sample at between 37 and 55°C (typically 50ºC), vortexing periodically until the gel slice is completely dissolved (∼5-10 min).
11.Insert spin column into a collection tube (both from the Monarch kit) and load the sample onto the column. Spin for 1 min, then discard flowthrough.
12.Re-insert spin column into collection tube.
13.Add 200 µl of DNA Wash Buffer (from kit) and spin for 1 min.
14.Repeat step 11.
15.Transfer spin column to a clean 1.5-ml microcentrifuge tube.
16.Add at least 6 µl of DNA Elution Buffer to the center of the matrix.
17.Wait for 1 min, and then spin for 1 min to elute DNA.
Perform Sanger sequencing
18.Prepare DNA and submit for Sanger sequencing according to the sequencing center specifications.
19.Upon the return of Sanger sequencing data, find the consensus sequence of each read by combining the replicate reads in each direction, and then merging the forward and reverse reads using a tool such as the freely available FinchTV program (see Internet Resources).
20.Use a nucleotide sequence match (e.g., BLAST) in NCBI, the Ribosomal Database Project, SilVA, or other database to verify the identity of each strain based on the quality sequence returned (Quast et al., 2013; Stoddard et al., 2015).
Basic Protocol 2: CREATION OF GLYCEROL STOCKS OF EACH MOCK COMMUNITY STRAIN FOR LONG-TERM CRYOSTORAGE
Once the included strains for a mock community have been chosen and verified (Basic Protocol 1), an essential step is to create separate high-quality freezer stocks of each strain so that they can be re-grown and used to assemble fresh stocks of mock communities as needed. Freezer stocks contain glycerol and growth medium to preserve bacterial and fungal strains for long-term storage at −80ºC. In this protocol, users will take an aliquot of an overnight liquid culture of each strain and combine with glycerol (a cryoprotectant) for long-term freezer storage of multiple replicates of each mock community strain.
Materials
-
50% (v/v) glycerol (J.T. Baker, cat. no. 2136-1), sterile, autoclaved
-
Overnight liquid cultures of desired strains, ∼5 ml culture/strain
-
1.5-ml externally threaded cryovials (VWR, cat. no. 10018-734)
-
P1000 Micropipette and micropipette tips
-
−80ºC Freezer
-
Freezer boxes
1.Label each cryovial with the strain and date.
2.Using aseptic technique, transfer 750 µl of 50% (v/v) glycerol into each labeled cryovial.
3.Add 750 µl of an overnight strain culture into each labeled cryovial.
4.Repeat steps 1-3 at least five times for each strain to create multiple glycerol stocks of each strain.
5.Place glycerol stocks into a labeled freezer box, and place into a −80ºC freezer for long-term storage.
Basic Protocol 3: ASSESSMENT OF STRAIN FREEZER VIABILITY WITHOUT CRYOPROTECTANT
During storage of a fully assembled, whole-cell-based mock community, strains are stored as a combination of pelleted cells, including each community member at its desired concentration and without the addition of a cryoprotective agent. Cryoprotectants can interfere with downstream activities, and can be difficult to remove from stocks, so we elected to exclude a cryoprotectant from mock community stocks. Because of this exclusion, it is first necessary to ensure that the cells of each included strain are viable after freezing, to verify that the created mock community can be used as a valid lysis control for DNA extraction. Verification of viability after freezing is determined by pelleting each strain and plating on solid agar after subjecting it to a freeze-thaw cycle. If strains grow after freeze-thaw, at least some cells are viable after freezing in pelleted form.
In summary, as a positive extraction control, the mock community helps determine if all cells are lysed during DNA extraction to release their DNA. We recommend the use of viable strains in a mock community, as membrane integrity is compromised when microbial strains are not viable. If strains are not viable after freezer storage testing, additional cryoprotectant agents must be added for the mock community to be a valid positive control for reporting on the lysis efficiency of a DNA extraction.
Materials
-
Overnight liquid culture of desired strains, ∼5 ml culture/strain
-
Phosphate-buffered saline (PBS; see recipe), ∼1 ml /strain
-
Solid medium plates; two per strain
-
1.5-ml microcentrifuge tubes: one per strain
-
Centrifuge with microcentrifuge tube rotor
-
−80ºC Freezer
-
Vortex
-
Glass beads (or glass spreader)
-
Incubator(s)
-
Additional reagents and equipment for aseptic techniques in inoculating and transferring cultures (see Current Protocols article: Bykowski & Stevenson, 2020)
Freezing
1.Vortex the overnight culture of each strain.
2.Add ∼1 ml of each strain from its overnight culture to a separate, labeled 1.5-ml microcentrifuge tube.
3.Centrifuge 10 min at 5000 × g , room temperature, and discard the supernatant.
4.Resuspend with approximately 500 µl of sterile PBS.
5.Centrifuge 10 min at 5000 × g , room temperature, to wash the medium from the cells.
6.Discard the PBS supernatant and store pellets at −80ºC at least overnight.
Post-freezer viability
7.Thaw the frozen pellets from step 6.
8.Rehydrate each pellet with 500 µl of sterile PBS.
9.Vortex for 1 min, or until pellet has been disrupted.
10.Inoculate up to 50 µl of the mixture on two separate replicates of the corresponding agar plate for each strain.
11.Add glass beads and shake to evenly spread the liquid onto the agar plate.
12.Incubate for 24-48 hr at each strain's preferred growth temperature to ensure cells are viable.
Basic Protocol 4: CREATION OF STANDARD CURVE TO DETERMINE CFU/ml OF A LIQUID CULTURE AS A FUNCTION OF OPTICAL DENSITY
In each mock community aliquot, strains are normalized to a standard concentration, so that users can be aware of the exact input into the system or experimental techniques, and the expected output is then calculated. To do this, standard curves must be created to ensure that the concentration of each member is known and standardized in each community, so that the bias can be determined from the number of cells as input compared to the number of cell-equivalents observed as output from the marker gene analysis (after accounting for any multiple copies of the marker gene per strain). Because it is tedious to complete a dilution series and plate count each time for many different strains, we provide more efficient instructions to create standard curves for each member. These curves will be created by first completing serial two-fold dilutions of each strain and measuring the optical density at 600 nm (OD600). Then, a ten-fold dilution will be completed for each of the two-fold dilutions, and plated on solid agar. After overnight growth and incubation, colonies will be counted to determine the number of colony-forming units (CFUs) per ml of liquid culture for each stain. This is done in stationary phase, so that the chromosomes of each strain are not being duplicated. By using information on the copy number and unique copy number of a specific marker gene, it is possible to ultimately calculate the exact number of microbial cells per strain that have been lysed and that have released their DNA in the workflow. The standard curves are created by plotting the OD600 on the x axis and the colony-forming units (CFU)/ml on the y axis. A trendline and the associated equation should be added, and an r -squared value greater than 0.93 is sufficient to proceed. The linear equation should be recorded for future use when assembling new mock community aliquots. The CFU/ml may be averaged for replicates of the same dilution factor. After Basic Protocol 4, users will have standard curves that can be used for mock community assembly, described in Basic Protocol 5.
For the purposes of Basic Protocol 4, the overnight liquid culture will be referred to as the “1:1” dilution.
Materials
-
Liquid medium specific for each strain
-
1:1 tubes: overnight liquid cultures of desired strains (∼10 ml/strain)
-
Solid (agar) medium specific for each strain, ∼20-24 agar plates/strain (100 mm × 15 mm, VWR, cat. no. 25384-302)
-
10% (v/v) bleach
-
10-ml test tubes (Fisher Scientific, cat. no. 14-925E)
-
Vortex
-
Glass pipettes, 10 and 25 ml
-
P1000 micropipette and corresponding pipette pips
-
P200 micropipette and corresponding pipette tips
-
Spectrophotometer with 600-nm laser
-
Cuvettes for spectrophotometer (1.5 ml)
-
Glass beads (or glass spreader)
-
Parafilm M (VWR, cat. no. 52858-000)
-
Microsoft Excel or other graphing software
-
Additional reagents and equipment for aseptic techniques in inoculating and transferring cultures (see Current Protocols article: Bykowski & Stevenson, 2020)
1.Label 10-ml test tubes appropriately for the creation of the standard curve.
Dilution factor | 1:1 (original overnight culture) | 1:2 | 1:4 | 1:8 | 1:16 |
---|---|---|---|---|---|
1:1 1:101 |
1:2 1:101 |
1:4 1:101 |
1:8 1:101 |
1:16 1:101 |
|
1:1 1:102 |
1:2 1:102 |
1:4 1:102 |
1:8 1:102 |
1:16 1:102 |
|
1:1 1:103 |
1:2 1:103 |
1:4 1:103 |
1:8 1:103 |
1:16 1:103 |
|
1:1 1:104 |
1:2 1:104 |
1:4 1:104 |
1:8 1:104 |
1:16 1:104 |
|
1:1 1:105 |
1:2 1:105 |
1:4 1:105 |
1:8 1:105 |
1:16 1:105 |
|
1:1 1:106 |
1:2 1:106 |
1:4 1:106 |
1:8 1:106 |
1:16 1:106 |
- a
Each square represents one tube or well.
Serial two-fold dilution and OD600 measurement
See Bykowski and Stevenson (2020) for basic microbiological techniques including aseptic transfer.
2.Use aseptic technique to transfer the appropriate volume of medium from the 1:1 tube to the 1:2 one, for a 2-fold dilution. Vortex gently or pipette up and down to mix.
3.Use aseptic technique to transfer equal amounts of the 1:2 dilution and sterile liquid medium into the 1:4 dilution tube. Vortex or mix gently.
4.Use aseptic technique to transfer equal amounts of the 1:4 dilution and sterile liquid medium into the 1:8 dilution tube. Vortex or mix gently.
5.Use aseptic technique to transfer equal amounts of the 1:8 dilution and sterile liquid medium into the 1:16 dilution tube. Vortex or mix gently.
6.Use aseptic technique to pipette each dilution factor (1:1, 1:2, 1:4, 1:8, 1:16) into a cuvette, and a control of corresponding sterile liquid medium as a blank.
7.Insert each cuvette into a spectrophotometer to obtain and record optical density readings at a wavelength of 600 nm (OD600).
Serial ten-fold dilution and plating of each two-fold dilution
8.For each dilution factor (1:1, 1:2, 1:4, 1:8, 1:16), complete the following. Using aseptic technique, take 1 volume of culture from the dilution factor tube and add it to corresponding tube labeled 1:101, which should contain 9 units of sterile liquid medium. Vortex or mix gently.
9.Using aseptic technique and a pipette, take 1 volume of culture from each 1:101 tube and add it to a corresponding tube labeled 1:102, which should already contain 9 volumes of sterile liquid medium. Vortex or mix gently.
10.Using aseptic technique and a pipette, take 1 volume of culture from each 1:102 tube and add it to the corresponding tube labeled 1:103, which should already contain 9 volumes of sterile liquid medium. Vortex or mix gently.
11.Using aseptic technique and a pipette, take 1 volume of culture from each 1:103 tube and add it to the corresponding tube labeled 1:104, which should already contain 9 volumes of sterile liquid medium. Vortex or mix gently.
12.Using aseptic technique and a pipette, take 1 volume of culture from each 1:104 tube and add it to the corresponding tube labeled 1:105, which should already contain 9 volumes of sterile liquid medium. Vortex or mix gently.
13.Using aseptic technique and a pipette, take 1 volume of culture from each 1:105 tube and add it to the corresponding tube labeled 1:106, which should already contain 9 volumes of sterile liquid medium. Vortex or mix gently.
Approximate OD600 | Suggested plating |
---|---|
2.0 | 10-7, 10-6, 10-5 |
1.8 | 10-7, 10-6, 10-5 |
1.6 | 10-7, 10-6, 10-5 |
1.4 | 10-7, 10-6, 10-5 |
1.2 | 10-6, 10-5, 10-4 |
1.0 | 10-6, 10-5, 10-4 |
0.8 | 10-6, 10-5, 10-4 |
0.6 | 10-6, 10-5, 10-4 |
0.4 | 10-5, 10-4, 10-3 |
0.2 | 10-5, 10-4, 10-3 |
-
aIf cells on the plate are notably small or slow growing, consider plating one less dilution than noted here.
-
bFungi will likely not reach these concentrations and should be plated using one dilution factor less, as well as given extra incubation time.
-
cE.g., fungal OD600 = 1.4; suggested plating: 10-7, 10-6, 10-5, but for fungi 10-6, 10-5, 10-4.
Dilution | OD600 | CFU (via plate count) | Dilution factor | Plating volume (ml) | Final CFU/ml |
---|---|---|---|---|---|
1:1 | 1.936 | 89 | 10-6 | 0.05 | 1,780,000,000 |
1:2 | 1.338 | 30 | 10-6 | 0.05 | 1,200,000,000 |
1:4 | 0.773 | 105 | 10-5 | 0.05 | 8,48000,000 |
1:8 | 0.395 | 284 | 10-4 | 0.05 | 4,54,400,000 |
1:16 | 0.192 | 124 | 10-4 | 0.05 | 396,800,000 |
14.Label six agar growth plates—two for each dilution factor—with the date, the strain, the medium, and the dilution (see Table 3 for sample data and suggested plating based in the OD600 of the culture).
15.Using aseptic technique, take 50 µl from the desired test tube for each dilution factor and add it to the correctly labeled plate.
16.Repeat step 17 for each of the five remaining plates using the correct dilution tubes and plates, respectively.
17.Add 5-10 glass beads to each plate and shake slowly from side to side, rotating plates every few seconds to spread the liquid completely on each plate.
18.Remove glass beads and place them into a 10% (v/v) bleach solution.
19.Seal agar plates using Parafilm and incubate for ∼24 hr at ideal growth temperature for the strain.
20.Count and record the number of colony-forming units (CFU) on each plate after 24 hr.
Use the following formula to count the CFU per ml of culture for each counted plate:
- CFU/ml = CFU/volume of plated culture (ml) × dilution factor)
Example calculation: count of 282 CFUs on the 10-4 dilution factor plate. A total of 50 µl of culture were plated.
CFU/ml = (282)/(0.05 ml × 10-4) = 56,400,000 CFU/ml
Colony count can be used when the number of colonies per plate is between 30 and 300.
21.For each dilution factor, plot the optical density against the average CFU/ml using Microsoft Excel or other graphing software.
22.Add a linear trendline to the graph as well as the equation of the trendline. Make note of the equation of the trendline for use in Basic Protocol 5.
Basic Protocol 5: FULL MOCK COMMUNITY ASSEMBLY USING COMMUNITY CONCENTRATION CALCULATIONS AND STANDARD CURVES
Mock communities can be constructed and assembled in new batches as needed, and each batch should be clearly identified with the date created in case batch contamination is discovered later. Every time the mock community is constructed, the concentration of each member must be known. To build the mock community each time, use glycerol stocks of each strain from Basic Protocol 2.After strains have been freshly grown from their freezer stocks, they can be cultured using liquid medium overnight. The OD600 will be measured for each strain in liquid culture, and the number of cells in each liquid culture will be calculated using the linear trendline equations from Basic Protocol 4 for each member. This will allow for normalization of each member to a known and standardized concentration in each mock community aliquot. After each member has been normalized to a standard concentration, the growth medium will be washed from the cells of each strain, and then the individual microbial strains will be combined into the final aliquot. Please note that this generalized protocol creates ten mock communities at once. It is recommended to generate as many mock communities as required to complete sample processing of a focal study, and then to store them in a −80ºC freezer to ensure their ready availability. The assembly of many communities at once provides greater efficiency for construction and uniformity across sample processing batches within a study.
Materials
-
Freezer stocks of each member of the full community, created during Basic Protocol 2
-
Solid (agar) medium specific for each strain, ∼20-24 agar plates/strain (100 mm × 15 mm; VWR, cat. no. 25384-302), 2 plates/strain
-
Phosphate-buffered saline (PBS; see recipe), 40 ml PBS/strain/group of 10 communities
-
Shaking incubator with flask holder
-
50-ml Erlenmeyer flask, one per strain
-
50-ml conical tubes; (Corning cat. no. 430290)
-
Vortex
-
Centrifuge with 50-ml conical tube rotor
-
Glass pipettes, 10 and 25 ml
-
Motorized pipette controller (“pipette gun”)
-
P1000 micropipette and corresponding pipette tips
-
P200 micropipette and corresponding pipette tips
-
Cuvettes (1.5 ml), one per strain and one per medium type
-
Spectrophotometer
-
Additional reagents and equipment for aseptic techniques in inoculating and transferring cultures (see Current Protocols article: Bykowski & Stevenson, 2020)
Fresh growth of each community member from freezer stock
See Bykowski and Stevenson (2020) for basic microbiological techniques including aseptic transfer and streaking of plates.
1.Thaw one glycerol stock for each community member (from Basic Protocol 2).
2.Using aseptic technique, streak each member on corresponding solid agar plates for single colonies and incubate overnight appropriately.
Overnight growth and normalizing cell concentration
3.Prepare an overnight culture of each strain by taking a labeled 50-ml Erlenmeyer flask containing 25 ml of sterilized liquid medium and inoculating the medium using a sterilized loop (Bykowski & Stevenson, 2020) with a single bacterial or fungal colony from the solid agar.
4.Place the inoculated flask into a shaking incubator overnight with appropriate conditions for each strain.
5.Use aseptic technique and a spectrophotometer to obtain and record optical density readings at a wavelength of 600 nm (OD600).
6.Use the trendline equations from Basic Protocol 4 to evaluate each equation at the OD600 to find the number of cells in culture.
7.Using the following equation, calculate the necessary amount of each culture for standardization and final assembly of the mock community:
Necessary volume in ml = [(final solution volume in ml) × (final concentration in number of cells)]/(Overnight culture concentration in CFU/ml).
Strain | OD600 | Stock concentration (CFU/ml) | Final solution volume (ml) | Final concentration (CFU/ml) | Volume from culture (ml) |
---|---|---|---|---|---|
Example | 1.23 | 9,000,000 (use OD600 in the trendline equation) | 30 | 1.00E+08 | = (30 × 1.00E+08)/(9,000,000) |
B. subtilis | 1.02 | 470,000,000 | 30 | 1.00E+08 | 6.383 |
C. neoformans | 0.99 | 38,993,550 | 30 | 1.00E+07 | 7.694 |
E. coli | 1.66 | 3,260,000,000 | 30 | 1.00E+08 | 0.920 |
P. aeruginosa | 1.00 | 700,000,000 | 30 | 1.00E+08 | 4.286 |
R. tropici | 0.85 | 1,650,000,000 | 30 | 1.00E+08 | 1.818 |
S. aureus | 1.75 | 1,720,000,000 | 30 | 1.00E+08 | 1.744 |
S. cerevisiae | 1.25 | 30,500,000 | 30 | 1.00E+07 | 9.836 |
S. enterica | 1.40 | 4,000,000,000 | 30 | 1.00E+08 | 0.750 |
Streptomyces sp. | 0.87 | 1,694,620 | 30 | 1.00E+06 | 17.703 |
Cell washing for removal of growth medium
8.Using aseptic technique (Bykowski & Stevenson, 2020), aliquot the necessary amount of each culture into separate, labeled 50-ml conical tubes.
- Each culture should stay separate in its own conical tube.
9.Centrifuge each tube 10 min at 5000 × g and discard the growth medium supernatant.
10.Resuspend each culture with 5 ml of sterile PBS to rinse and remove any remaining growth medium.
11.Centrifuge 10 min at 5000 × g to wash the cells, and discard the supernatant PBS wash.
Full community assembly
12.Add the desired final volume of PBS solution to each 50-ml conical tube and vortex to resuspend and distribute cells.
13.Using a new set of labeled 50-ml conical tubes for each known community to be assembled, aliquot the same amount of each strain into each conical tube.
14.Centrifuge each assembled mock community 10 min at 5000 × g and discard the PBS supernatant, leaving ∼1 ml.
15.Vortex to resuspend the cells in the remaining 1 ml of supernatant and use a micropipette to transfer the sample from its 50-ml conical tube to a 1.5-ml microcentrifuge tube.
Preparation for long-term storage
16.Centrifuge the microcentrifuge tubes 15 min at 5000 × g.
17.Discard the PBS supernatant and store each mock community pellet at −80ºC until ready to use.
REAGENTS AND SOLUTIONS
Phosphate-buffered saline (PBS)
- Add the following to a flask:
- 800 ml distilled H2O
- 8 g NaCl
- 0.2 g KCl
- 1.44 g Na2HPO4
- 0.24 g KH2PO4
- Adjust pH to 7.4 with HCl
- Add distilled H2O to a final volume of 1 L
- Autoclave and store at room temperature for up to 2 years
TBE buffer, 5×
- Add the following to a flask:
- 800 ml distilled H2O
- 54.51 g Tris base (MW: 121.14 g/mol)
- 27.82 g boric acid (MW: 61.83 g/mol)
- 3.72 g UltraPure EDTA (MW: 372.24 g/mol)
- Adjust pH to 8.3 with HCl
- Add distilled H2O to a final volume of 1 L
- Autoclave and store at room temperature for up to 2 years
COMMENTARY
Background Information
Microbiome research seeks to characterize the specific bacterial, fungal, and viral communities present in an environment or host of scientific interest. Often, to characterize and fully understand the microbial community, high-throughput sequencing is used to generate large amounts of genomic sequence data, and can be used to provide taxonomic or functional insight. This culture-independent methodology requires appropriate positive and negative controls to gain greater insight on nucleic acid extraction, marker gene amplification, sequencing efforts, contamination, and taxonomic bias. We have provided methodology to create a custom mock community for use as a positive control during lysis, nucleic acid extraction, amplification, and sequencing, as well as for the interpretation of these results.
DIY community as a positive DNA extraction control
DNA extraction controls, both positive and negative, are important to determine if and to what degree there is contamination introduced in protocols or in a final experimental result. They can also inform on the quality of DNA sequencing efforts using error calculations of known strains against the resulting data. Negative controls monitor that no result is generated when there is no sample input, e.g., any results generated are the result of error or contamination introduced during experimental methodology. Negative controls also are used to determine any “noise” generated that can be systematically removed using bioinformatic decontamination tools (Davis, Proctor, Holmes, Relman, & Callahan, 2018). A positive control ensures that a laboratory protocol is providing the expected output, and can be used to inform on source of contamination, including plate-based contamination originating at a sequencing center. Although they have been employed since the early days of 454 pyrosequencing (Amend, Seifert, & Bruns, 2010; Caporaso et al., 2011), positive controls are sometimes overlooked or excluded, resulting in incomplete study designs. Positive controls are used to verify success of DNA extractions in studies that include samples of low microbial biomass, but can and should be widely employed to provide increased information about DNA extraction, amplification, and sequencing success (Karstens et al., 2019). Low-biomass samples may yield little to no microbial nucleic acids because of low DNA input resulting from constraints of the study system or sample type, or samples obtained may be of low quality. Mock community positive controls may be diluted to simulate the low biomass of the study system, which can help determine detection limits of experimental protocols or help identify success/failure in a protocol (Eisenhofer et al., 2019; Highlander, 2014; Knight et al., 2018; Salter et al., 2014). Without positive controls included alongside low-biomass extractions, it becomes impossible to determine if there was a protocol failure or true absence of extractable microbial biomass.
Though mock communities are less consistently used for studies of high-microbial-biomass samples, these samples can also benefit from their inclusion. For example, the sequencing from the positive controls can inform as to the step at which contamination occurred (DNA extraction, amplification, or library preparation, and sequencing). Indeed, if positive controls return unexpected sequences that are not from the input strains, or sequences from host organelles, these reads are contaminants. If both the positive and negative controls contain similar contaminants, contamination may be uniform across the sequenced samples from one run, and contaminants can be removed bioinformatically. If, with due diligence, the positive and negative controls show sequencing contamination that was not detected prior to library preparation and sequencing (e.g., at a sequencing facility), and their returned sequences show different patterns of contamination (e.g., positive and negative controls on the same plate have different contaminants), contamination is assumed to be non-uniform across samples, and this may require discarding all data from the same sequencing run and re-sequencing. If paired positive and negative controls from the same sample processing batch have contaminants, but those from other batches do not, then only the sequencing from contaminated batch(es) need be discarded.
After sequencing, the mock community data can be used to calculate the sequencing error rate by comparing the known input sequences to the output (Schloss et al., 2009). Known sequences from the mock community can be used to construct a local BLAST database of nucleotide sequences. A local BLAST database ensures that all true amplicon sequence variants (ASVs) are discovered and retained during sequence clustering into operational taxonomic units. This will help determine errors and approximate their occurrence(s), and can inform the quality control methods for the identification and determination of found taxa. This can also inform as to the variation in quality and clustering of taxa across mock communities from different processing batches but sequenced at the same time. Mock community data can also be uploaded and explored with the public resource “mockrobiota” to compare laboratory data with other mock community sequences. This is especially useful and relevant if multiple mock communities have been used, if the creator of a mock community makes the community available to others, or if the data is available in public repositories (Bokulich et al., 2016).
The creation of a customized mock community is useful for several reasons, including customization to study needs and practical cost savings. The DIY mock community informs robust outcomes along all stages, from microbiome sample processing to sequence analysis. Although there has been a repository for existing mock community data (Bokulich et al., 2016), this protocol adds value by providing a protocol resource to create a customized mock community for microbiome analysis.
Critical Parameters
There are several steps and stages of this protocol that are key to obtaining robust results. Beginning with strain selection, it is critical to have a priori knowledge for each included strain (e.g., cell lysis considerations, growth requirements, marker gene sequences, and copies), otherwise there may be gaps in the full realization of the DIY community as a positive control. Another critical point is the creation of standard curves for each strain, as described in Basic Protocol 4. If this information is inaccurate, there will not be standardized amounts of each strain in the fully assembled community and the expected input will not match the output, even if all other processes proceed perfectly. Additionally, the standardized amount of each strain in the mock community, per Basic Protocol 5, should be given special attention and consideration to best capture the needs of the system and represent that system with accuracy.
Troubleshooting
See Table 6 for common problems, their causes, and potential solutions.
Problem | Possible cause | Solution(s) |
---|---|---|
DNA extraction method yields low-quality DNA that may be fragmented or present in low concentration | DNA extraction kit is not sufficient to cause complete lysis of cells | Try DNA extractions using a different method, or adding mechanical lysis techniques such as bead beating |
PCR amplification yields non-specific bands or bands of unexpected length | Chimeras are created, or PCR primers matched to unforeseen DNA regions | Perform a gel extraction of only desired bands |
Sanger sequences are poor quality (less than 60% quality score) | Strains were not amenable to Sanger sequencing, high G/C content | Repeat Sanger sequencing protocols, ensuring high-quality DNA, and verify that appropriate buffers and methods are used based on the G/C content of their DNA |
Microbes have difficulty reaching stationary phase during growth | Not enough homogenization or aeration for aerobic cultures during shaking incubation |
|
Microbes do not grow well when transferred from solid agar medium to liquid culture | Too few starting cells | Instead of inoculating colonies directly into 25 ml of liquid medium, inoculate first into 5 ml of liquid medium and incubate overnight while shaking. The next day, dilute the culture 1:1000 into 25 ml of liquid medium and incubate overnight while shaking. |
Strains are not viable after freezing in glycerol | Not all strains are viable after freezer storage in glycerol |
|
Cells are not viable after freezing in pelleted form | Cells require a cryoprotectant |
|
Drastic differences in OD600 as compared to the number of colonies obtained by direct plate count methods | Incorrectly blanked spectrophotometer; aggregation of cells; OD600 is beyond detection limit of the spectrophotometer |
|
Standard curve does not have an r2 value of ≥ 0.93 | Incorrect dilutions were plated and grown; too much time has elapsed since plating and plate counting; contamination |
|
Strains do not stay pelleted in conical tubes | Angled centrifuge rotor, not enough time in centrifuge | Centrifuge for longer, use upright (bucket) centrifuge rotor |
Understanding Results
How do I use the mock community in a microbiome study?
Beyond the protocols included here, we provide guidance for using the mock community as a positive sequencing control. The Mothur suite and SOP, developed by Schloss and colleagues, provide the theoretical basis for the development and usage of a custom mock community. In the example data shown here, these positive controls were sequenced as part of a larger study at the Michigan State University Genomics Core Facility using the Illumina MiSeq platform V2 Standard flow cell in a 2 × 250-bp paired-end format for 16S rRNA gene amplicons. Raw sequencing data was processed with QIIME2 version 2021.8.0 (Bolyen et al., 2019) with the DADA2 denoising module from package q2-dada2 version 2021.8.0. We used the SILVA database version 138 (Quast et al., 2013) to assign taxonomy to the generated Amplicon Sequence Variants (ASVs, 100% sequence identity). Data visualization was performed in R version 4.1.3.
Figure 6A shows the expected relative abundance of each strain added in equal amounts of total cells into a DIY mock community. Figure 6B shows the relative abundance of the mock community members after the data were subsampled to 147,356 sequencing reads (to match the observational effort of the larger study, data not shown). The exact subsampling depth is not important unless the mock community was not comprehensively observed with the sequencing effort, which is unlikely given the small number of strains included and typical sequencing output from standard sequencing platforms (e.g., Illumina). Finally, Figure 6C shows the relative abundance of the mock community members after their total sequences per strain were corrected for their known copy number of the 16S rRNA gene (e.g., a strain with 5 marker gene copies and a sequencing return of 50 reads would be corrected to 10 to achieve a number comparable to a genome equivalent). Ideally, the corrected abundances should reflect the proportional expected input for each strain (e.g., Figure 6A), and discrepancies could be attributed to error in standardizing the input cells across strains when constructing the mock communities, or to methodological bias (e.g., lysis, primer bias, etc.). While the exact source of bias may not always be apparent, biases can be disclosed and discussed when interpreting results. In these example data, there may be a bias against Staphylococcus in the extraction or amplification, because its corrected proportion in Figure 6C is much lower than expected based on input in Figure 6A.
Finally, as previously mentioned, any taxa in the mock community that were not expected based on included strains can be examined as contaminants and checked against published information regarding the “kit-ome,” if a nucleic acid extraction kit was used (Salter et al., 2014). Systematic contaminants or “noise” sequences from taxa that are not expected to be present in the study samples and found in both the mock community(ies) and negative control(s) can be bioinformatically removed using software packages such as Decontam for R (see Internet Resources). These are deduced to have been introduced during library preparation and sequencing if there was no measurable DNA in the negative control and its “amplification check” PCR (performed with due diligence on the extracted DNA prior to library preparation) returned no detectable amplicons. Thus, with both the positive and negative controls, researchers can gain insight into the source and type of contamination and attempt to determine if the contamination is uniform in their samples and can be removed appropriately, or if contamination is non-uniform and samples may need to be re-sequenced.
Time Considerations
Basic Protocol 1
- Total time: 4 hr
- Hands-on time: 2 hr, 40 min
- Inactive time: 1 hr, 20 min
- Time to submit and receive Sanger results not included in estimate
Basic Protocol 2
- Total time: 24 hr, 40 min
- Hands-on time: 40 min
- Inactive time: 24 hr incubation (one overnight incubation)
Basic Protocol 3
- Total time: 25 hr, 40 min
- Hands-on time: 1 hr, 40 min
- Inactive time: 24 hr incubation (one overnight incubation)
Basic Protocol 4
- Total time: 52 hr
- Hands-on time: 3 hr
- Inactive time: 48 hr incubation (two overnight incubations)
Basic Protocol 5
- Total time: 26 hr, 20 min
- Hands-on time: 2 hr, 20 min
- Inactive time: 24 hr incubation (one overnight incubation)
Acknowledgments
This work was supported by the United States Department of Agriculture National Institute of Food and Agriculture (USDA NIFA) 2019-67019-29305. KLG and MEML acknowledge support from the Great Lakes Bioenergy Research Center, U.S. Department of Energy, Office of Science, Office of Biological and Environmental Research under award number DE-SC0018409. AS acknowledges support from the USDA NIFA and Michigan State University AgBioResearch
The authors would like to thank Dr. Patrick Schloss at the University of Michigan for the development of his widely used microbial sequence analysis pipeline and Mothur suite of tools.
Author Contributions
Joanna Colovas : methodology, visualization, writing original draft, writing review and editing; Ari Fina Bintarti : conceptualization, methodology, writing review and editing; Marco E. Mechan Llontop : data curation, formal analysis, visualization, writing review and editing; Keara Grady : methodology, writing review and editing; Ashley Shade : conceptualization, funding acquisition, methodology, project administration, writing review and editing.
Conflict of Interest
The authors declare no conflict of interests.
Open Research
Data Availability Statement
The data that support the protocol are openly available in repository Mock_Community_Standard_Colovas_2022 at https://github.com/ShadeLab/Mock_Community_Standard_Colovas_2022, and in the NCBI BioProject Mock community standard for multi-step assessment of microbiome protocols with BioProject ID PRJNA841769 at https://www.ncbi.nlm.nih.gov/bioproject/?term=PRJNA841769.
Literature Cited
- Altschul, S. F., Gish, W., Miller, W., Myers, E. W., & Lipman, D. J. (1990). Basic local alignment search tool. Journal of Molecular Biology , 215(3), 403–410. doi: 10.1016/s0022-2836(05)80360-2
- Amend, A. S., Seifert, K. A., & Bruns, T. D. (2010). Quantifying microbial communities with 454 pyrosequencing: Does read abundance count? Molecular Ecology , 19(24), 5555–5565. doi: 10.1111/j.1365-294/2010.04898.x
- Bakker, M. G. (2018). A fungal mock community control for amplicon sequencing experiments. Molecular Ecology Resources , 18(3), 541–556. doi: 10.1111/1755-0998.12760
- Bokulich, N. A., Subramanian, S., Faith, J. J., Gevers, D., Gordon, J. I., Knight, R., … Caporaso, J. G. (2013). Quality-filtering vastly improves diversity estimates from illumina amplicon sequencing. Nature Methods , 10(1), 57–59. doi: 10.1038/nmeth.2276
- Bokulich, N. A., Rideout, J. R., Mercurio, W. G., Shiffer, A., Wolfe, B., Maurice, C. F., … Caporaso, J. G. (2016). mockrobiota: A public resource for microbiome bioinformatics benchmarking. mSystems , 1(5), e00062–16. doi: 10.1128/mSystems.00062-16
- Bolyen, E., Rideout, J. R., Dillon, M. R., Bokulich, N. A., Abnet, C. C., Al-Ghalith, G. A., … Caporaso, J. G. (2019). Reproducible, interactive, scalable and extensible microbiome data science using QIIME 2. Nature Biotechnology , 37, 852–857. doi: 10.1038/s41587-019-0209-9
- Bykowski, T., & Stevenson, B. (2020). Aseptic technique. Current Protocols in Microbiology , 56, e98. doi: 10.1002/cpmc.98
- Caporaso, J. G., Lauber, C. L., Walters, W. A., Berg-Lyons, D., Lozupone, C. A., Turnbaugh, P. J., … Knight, R. (2011). Global patterns of 16S rrna diversity at a depth of millions of sequences per sample. Proceedings of the National Academy of Sciences , 108(Supplement_1), 4516–4522. doi: 10.1073/pnas.1000080107
- Davis, N. M., Proctor, D. M., Holmes, S. P., Relman, D. A., & Callahan, B. J. (2018). Simple statistical identification and removal of contaminant sequences in marker-gene and metagenomics data. Microbiome , 6, 226. doi: 10.1186/s40168-018-0605-2
- Eisenhofer, R., Minich, J. J., Marotz, C., Cooper, A., Knight, R., & Weyrich, L. S. (2019). Contamination in low microbial biomass microbiome studies: Issues and recommendations. Trends in Microbiology , 27(2), 105–117. doi: 10.1016/j.tim.2018.11.003
- Highlander, S. (2014). Mock community analysis. In K. Nelson (Ed.), Encyclopedia of metagenomics. New York: Springer. doi: 10.1007/978-1-4614-6418-1_54-1
- Karstens, L., Asquith, M., Davin, S., Fair, D., Gregory, W. T., Wolfe, A. J., … McWeeney, S. (2019). Controlling for contaminants in low-biomass 16S rRNA gene sequencing experiments. mSystems , 4, e00290–19. doi: 10.1128/mSystems.00290-19
- Kageyama, K., Ohyama, A., & Hyakumachi, M. (1997). Detection of pythium ultimum using polymerase chain reaction with species-specific primers. Plant Disease , 81(10), 1155–1160. doi: 10.1094/pdis.1997.81.10.1155
- Kearse, M., Moir, R., Wilson, A., Stones-Havas, S., Cheung, M., Sturrock, S., … Drummond, A. (2012). Geneious Basic: An integrated and extendable desktop software platform for the organization and analysis of sequence data, Bioinformatics , 28(12), 1647–1649. doi: 10.1093/bioinformatics/bts199
- Knight, R., Vrbanac, A., Taylor, B. C., Aksenov, A., Callewaert, C., Debelius, J., … Dorrestein, P. C. (2018). Best practices for analysing microbiomes. Nature Reviews Microbiology , 16, 410–422. doi: 10.1038/s41579-018-0029-9
- Kramer, M. F., & Coen, D. M. (2001). Enzymatic amplification of DNA by PCR: Standard procedures and optimization, Current Protocols in Molecular Biology , 56, 15.1.1–15.1.14. doi: 10.1002/0471142727.mb1501s56
- Lane, D. J. (1991). 16S/23S rRNA sequencing. Nucleic acid techniques in bacterial systematics (pp. 125–175). Chichester: John Wiley & Sons.
- Lindahl, B. D., Nilsson, R. H., Tedersoo, L., Abarenkov, K., Carlsen, T., Kjøller, R., … Kauserud, H. (2013). Fungal community analysis by high-throughput sequencing of amplified markers—a user's guide. New Phytologist , 199, 288–299. doi: 10.1111/nph.12243
- Nguyen, N. H., Smith, D., Peay, K., & Kennedy, P. (2015). Parsing ecological signal from noise in next generation amplicon sequencing. New Phytologist , 205(4), 1389–1393. doi: 10.1111/nph.12923
- Nilsson, R. H., Larsson, K. H., Taylor, A. F. S., Bengtsson-Palme, J., Jeppesen, T. S., Schigel, D., … Abarenkov, K. (2018). The UNITE database for molecular identification of fungi: Handling dark taxa and parallel taxonomic classifications. Nucleic Acids Research , 47(D1), D259–D264. doi: 10.1093/nar/gky1022
- Quast, C., Pruesse, E., Yilmaz, P., Gerken, J., Schweer, T., Yarza, P., … Glöckner, F. O. (2013). The SILVA ribosomal RNA gene database project: Improved data processing and web-based tools. Opens external link in new window. Nucleic Acids Research , 41(D1), D590–D596.
- Salter, S. J., Cox, M. J., Turek, E. M., Calus, S. T., Cookson, W. O., Moffatt, M. F., … Walker, A. W. (2014). Reagent and laboratory contamination can critically impact sequence-based microbiome analyses. BMC Biology , 12(1), 1–12.
- Schloss, P. D., Gevers, D., & Westcott, S. L. (2011). Reducing the effects of PCR amplification and sequencing artifacts on 16S rRNA-based studies. PLoS One , 6(12), e27310. doi: 10.1371/journal.pone.0027310
- Schloss, P. D., Westcott, S. L., Ryabin, T., Hall, J. R., Hartmann, M., Hollister, E. B., … Weber, C. F. (2009). Introducing Mothur: Open-source, platform-independent, community-supported software for describing and comparing microbial communities. Applied and Environmental Microbiology , 75(23), 7537–7541. doi: 10.1128/aem.01541-09
- Shendure, J. A., Porreca, G. J., Church, G. M., Gardner, A. E., Hendrickson, C. L., Kieleczawa, J., & Slatko, B. E. (2011). Overview of DNA sequencing strategies. Current Protocols in Molecular Biology , 96, 7.1.1–7.1.23. doi: 10.1002/0471142727.mb0701s96
- Starke, R., Pylro, V. S., & Morais, D. K. (2020). 16S rRNA gene copy number normalization does not provide more reliable conclusions in metataxonomic surveys. Microbial Ecology , 81, 535–539. doi: 10.1007/s00248-020-01586-7
- Stoddard, S. F., Smith, B. J., Hein, R., Roller, B. R. K., & Schmidt, T. M. (2015). rrn DB: Improved tools for interpreting rRNA gene abundance in bacteria and archaea and a new foundation for future development. Nucleic Acids Research , 43(Database issue), D593–598. doi: 10.1093/nar/gku1201
- Strube, M. L. (2021). RibDif: Can individual species be differentiated by 16S sequencing? Bioinformatics Advances , 1(1), vbab020. doi: 10.1093/bioadv/vbab020
- Tang, S. (2017). Microbiomics special edition. Guideline for Use of the ZymoBIOMICS® Microbial Community Standard. Irvine, CA: Zymo Research..
- Voytas, D. (2001). Agarose gel electrophoresis. Current Protocols in Molecular Biology , 51, 2.5A.1–2.5A.9. doi: 10.1002/0471142727.mb0205as51
Key References
- Schloss et al. (2009). See above.
The Mothur suite provides the theoretical basis for the development and usage of a custom mock community.
Internet Resources
Construction of a local BLAST database __
* <https://www.ncbi.nlm.nih.gov/books/NBK569841/>
Once the Sanger sequences have returned from a mock community, they can be assembled into a local BLAST database for quick and easy use for future data analysis of sequenced mock communities.
Decontam package for R from Bioconductor __
* <https://bioconductor.org/packages/release/bioc/html/decontam.html>
The Decontam package for R helps to identify contaminants in marker-gene and metagenomic sequencing data.
FinchTV download __
* <https://digitalworldbiology.com/FinchTV>
FinchTV is a free chromatogram viewer available freely online for use in analyzing the Sanger sequences generated in Basic Protocol 1.
Mothur MiSeq SOP calculation of 16S sequencing error rate __
* <https://mothur.org/wiki/miseq_sop/#assessing-error-rates>
The Mothur program is commonly used to clean, tidy, and analyze microbial sequence data. After a mock community has been sequenced alongside experimental samples, the resulting data can be used to calculate 16S/ITS sequencing error rates, and to determine what biases, if any, are present in the dataset.
Citing Literature
Number of times cited according to CrossRef: 6
- Samuel E. Barnett, Ashley Shade, Arrive and wait: Inactive bacterial taxa contribute to perceived soil microbiome resilience after a multidecadal press disturbance, Ecology Letters, 10.1111/ele.14393, 27 , 3, (2024).
- Sreejata Bandopadhyay, Xingxing Li, Alan W. Bowsher, Robert L. Last, Ashley Shade, Disentangling plant- and environment-mediated drivers of active rhizosphere bacterial community dynamics during short-term drought, Nature Communications, 10.1038/s41467-024-50463-1, 15 , 1, (2024).
- Andrew J. Hoisington, Christopher E. Stamper, Joseph C. Ellis, Christopher A. Lowry, Lisa A. Brenner, Quantifying variation across 16S rRNA gene sequencing runs in human microbiome studies, Applied Microbiology and Biotechnology, 10.1007/s00253-024-13198-z, 108 , 1, (2024).
- Giulia Zarantonello, Argelia Cuenca, Nanopore‐Enabled Microbiome Analysis: Investigating Environmental and Host‐Associated Samples in Rainbow Trout Aquaculture, Current Protocols, 10.1002/cpz1.1069, 4 , 6, (2024).
- Anna Wiesinger, Jasmin Wenderlein, Sebastian Ulrich, Stephanie Hiereth, Lidia Chitimia-Dobler, Reinhard K. Straubinger, Revealing the Tick Microbiome: Insights into Midgut and Salivary Gland Microbiota of Female Ixodes ricinus Ticks, International Journal of Molecular Sciences, 10.3390/ijms24021100, 24 , 2, (1100), (2023).
- Christopher J. Sweeney, Rishabh Kaushik, Melanie Bottoms, Considerations for the inclusion of metabarcoding data in the plant protection product risk assessment of the soil microbiome, Integrated Environmental Assessment and Management, 10.1002/ieam.4812, 20 , 2, (337-358), (2023).