Cell Banking of hiPSCs: A Practical Guide to Cryopreservation and Quality Control in Basic Research
Thomas Eschenhagen, Aya Shibamiya, Aya Shibamiya, Elisabeth Schulze, Elisabeth Schulze, Dana Krauß, Dana Krauß, Christa Augustin, Christa Augustin, Marina Reinsch, Marina Reinsch, Mirja Loreen Schulze, Mirja Loreen Schulze, Simone Steuck, Simone Steuck, Giulia Mearini, Giulia Mearini, Ingra Mannhardt, Ingra Mannhardt, Thomas Schulze, Thomas Schulze, Birgit Klampe, Birgit Klampe, Tessa Werner, Umber Saleem, Anika Knaust, Sandra D. Laufer, Christiane Neuber, Marta Lemme, Charlotta Sophie Behrens, Malte Loos, Florian Weinberger, Sigrid Fuchs, Arne Hansen, Bärbel Maria Ulmer
Abstract
The reproducibility of stem cell research relies on the constant availability of quality-controlled cells. As the quality of human induced pluripotent stem cells (hiPSCs) can deteriorate in the course of a few passages, cell banking is key to achieve consistent results and low batch-to-batch variation. Here, we provide a cost-efficient route to generate master and working cell banks for basic research projects. In addition, we describe minimal protocols for quality assurance including tests for sterility, viability, pluripotency, and genetic integrity. © 2020 The Authors.
Basic Protocol 1 : Expansion of hiPSCs
Basic Protocol 2 : Cell banking of hiPSCs
Support Protocol 1 : Pluripotency assessment by flow cytometry
Support Protocol 2 : Thawing control: Viability and sterility
Support Protocol 3 : Potency, viral clearance, and pluripotency: Spontaneous differentiation and qRT-PCR
Support Protocol 4 : Identity: Short tandem repeat analysis
INTRODUCTION
Human induced pluripotent stem cells (hiPSCs) are widely utilized from basic research to clinical applications. Many laboratories create patient-specific or genetically modified hiPSC lines, and need to implement a strategy to cryopreserve not only large quantities of hiPSCs but also high-quality hiPSCs (Stacey, 2012; Stacey, Crook, Hei, & Ludwig, 2013). The basis for this is usually the creation of master and working cell banks (MCBs and WCBs). Basically, a MCB consists of multiple cryopreserved vials of one batch of hiPSCs; some of the vials are used for quality control and others to create WCBs. One WCB cryovial can be thawed per experiment and used without the need for long expansion phases, which are prone to cause genetic aberrations and contamination. This assures a reproducible, validated hiPSC quality that reduces batch-to-batch variability of hiPSC-derived cell types and assays (Volpato et al., 2020). Cell banking with MCBs and WCBs is a central part of quality control during the production of hiPSC-based medical products or hiPSC lines targeted for clinical application (Andrews et al., 2015; Sullivan et al., 2018). Directives and regulations for this are in place (Baghbaderani et al., 2015; Rao et al., 2018; Shafa et al., 2020; see also Internet Resources for EU regulations and directives for Good Laboratory Practice [GLP], Good Cell Culture Practice [GCCP], and Good Manufacturing Practice [GMP]). In addition, consortia such as the International Stem Cell Initiative and Stem Cell COREdinates define best practices and work on a consensus for hiPSC MCB testing, especially for clinical-grade hiPSCs (Andrews et al., 2015; Sullivan et al., 2018). Registries and hiPSC banks such as hPSCreg and EBiSC set gold standards for research-grade hiPSC lines (also see Internet Resources and Key References).
However, these activities are in stark contrast to the common standard of hiPSC quality testing in basic research, where the degree of quality assessment varies widely, with many publications not mentioning any quality control. Thus, a strategy for cell banking and minimal quality control is also necessary to improve reproducibility in the field of basic research.
This protocol is designed to serve as a practical guide to cryopreservation and quality control for basic research. Among other things, it should enable basic scientist to establish a cost-efficient, labor-saving workflow for quality-controlled, reproducible hiPSC-based experiments by detailing a selection of common, well-known assays. The protocol was used to produce MCBs in the course of the IndivuHeart project (ClinicalTrials.gov Identifier: NCT02417311), which attempted to compare hiPSC-derived cardiomyocytes in engineered heart tissues from 60 probands. The protocol details expansion starting from young hiPSC clones between passage numbers (p) 5 and 10 (Basic Protocol 1) and subsequent cell banking of MCBs and WCBs (Basic Protocol 2). Quality controls are described in Support Protocols 1-4.
NOTE : Prior approval from the local Institution Safety Board and Ethical Committee is required for research using hiPSCs. All work described in this protocol was reviewed and approved by the Ethical Committee of the University Medical Center Hamburg-Eppendorf (Az. 532/116/9.7.1991 and PV4798/21.10.2014), and all patients gave written informed consent.
NOTE : All procedures are to be performed using sterile materials in a Class II biological hazard flow hood or a laminar-flow hood, and proper aseptic technique should be used accordingly.
STRATEGIC PLANNING
This protocol was developed based on hiPSC lines derived from human dermal fibroblasts reprogrammed with CytotuneTM Sendai virus according to the manufacturer's instructions. We recommend freezing backup cryovials of fibroblasts and transduced fibroblasts, as well as backup vials of 6-10 clones per proband around passage (p) 5-10 (Fig. 1). Here we start with these uncharacterized p5-p10 hiPSC clones and end with the freezing of MCBs and WCBs around p18-p25 or p23-p30 (+ p5), respectively (Fig. 1). Quality controls are detailed in Support Protocols 1-4. The protocol can be adapted to any other reprogramming method, for example episomal transduction of reprogramming factors, by changing quality controls such as transgene clearance controls accordingly. Also, other primary cell types, such as lymphocytes or urinary cells, and other culture methods can be utilized in a similar workflow.
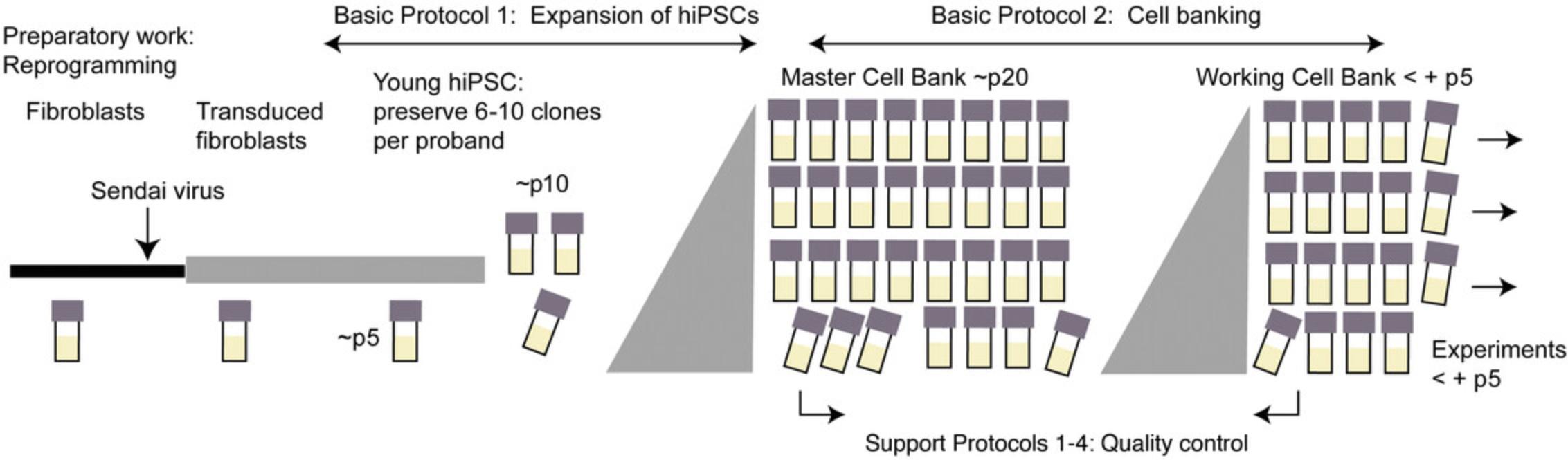
Basic Protocol 1: EXPANSION OF hiPSC
This protocol describes the thawing and expansion of one cryovial of an uncharacterized hiPSC clone in the range of p5-p10 to derive hiPSCs for an MCB. For details of general hiPSC culture, consult Frank, Zhang, Schöler, & Greber (2012).
Materials
-
Six-well plates or T75 flasks, coated with Geltrex (see recipe)
-
FTDA medium with and without Y-27632 (see recipe)
-
Washing medium (see recipe)
-
Reprogrammed, uncharacterized hiPSC clones, p5-p10
-
Y-27632 (rho kinase inhibitor) solution (see recipe)
-
Dulbecco's Phosphate-Buffered Saline (PBS; e.g., ThermoFisher 14190)
-
Accutase solution with Y-27632 (see recipe)
-
Trypan blue dye, optional
-
Water bath, 37°C
-
Incubator with CO2 and N2 gas supply with an O2 control system set to 37°C, 90% relative humidity (rH), 5% CO2, 5% O2 (hypoxic condition)
-
Warming cabinet or other dry warming device, 37°C
-
Freezers, −80°C and −150°C (or liquid nitrogen)
-
Dry ice
-
Serological plastic pipets (e.g., Sarstedt 86.1685.001, 86.1254.001, and 86.1253.001)
-
50- and 15-ml conical centrifugation tubes (e.g., Falcon®)
-
Centrifuge
-
Nunclon® Delta Surface six-well plates and T75 flasks (ThermoFisher)
-
Cell counter (e.g., CASY cell counter) or Neubauer chamber
Thawing of hiPSCs
1.Prewarm water bath and incubator (with Geltrex-coated plate inside). Prewarm washing medium and FTDA (both with Y-27632) in the warming cabinet.
2.Pick up frozen hiPSC cryovials stored in liquid nitrogen tank or −150°C freezer and transfer to the bench on dry ice. Thaw cryovial in water bath at 37°C for 2-3 min without shaking.
3.Draw up 5 ml washing medium in a pipet. Place the tip of the pipet into the cryovial, and gradually draw up the cell suspension into the washing medium inside the pipet. Slowly let cells and washing medium run down at the inner side of a 50-ml conical centrifugation tube. Centrifuge for 2 min at 200 × g , room temperature.
4.Aspirate supernatant, loosen cell pellet by tapping bottom of tube with finger, and resuspend carefully in FTDA medium with Y-27632.Aspirate Geltrex solution from the coated plates and plate cells.
5.Place the cells in an incubator under hypoxic condition at 37°C, 90% rH, 5% CO2 and 5% O2.
Feeding of hiPSCs
6.Monitor hiPSCs daily under the microscope.
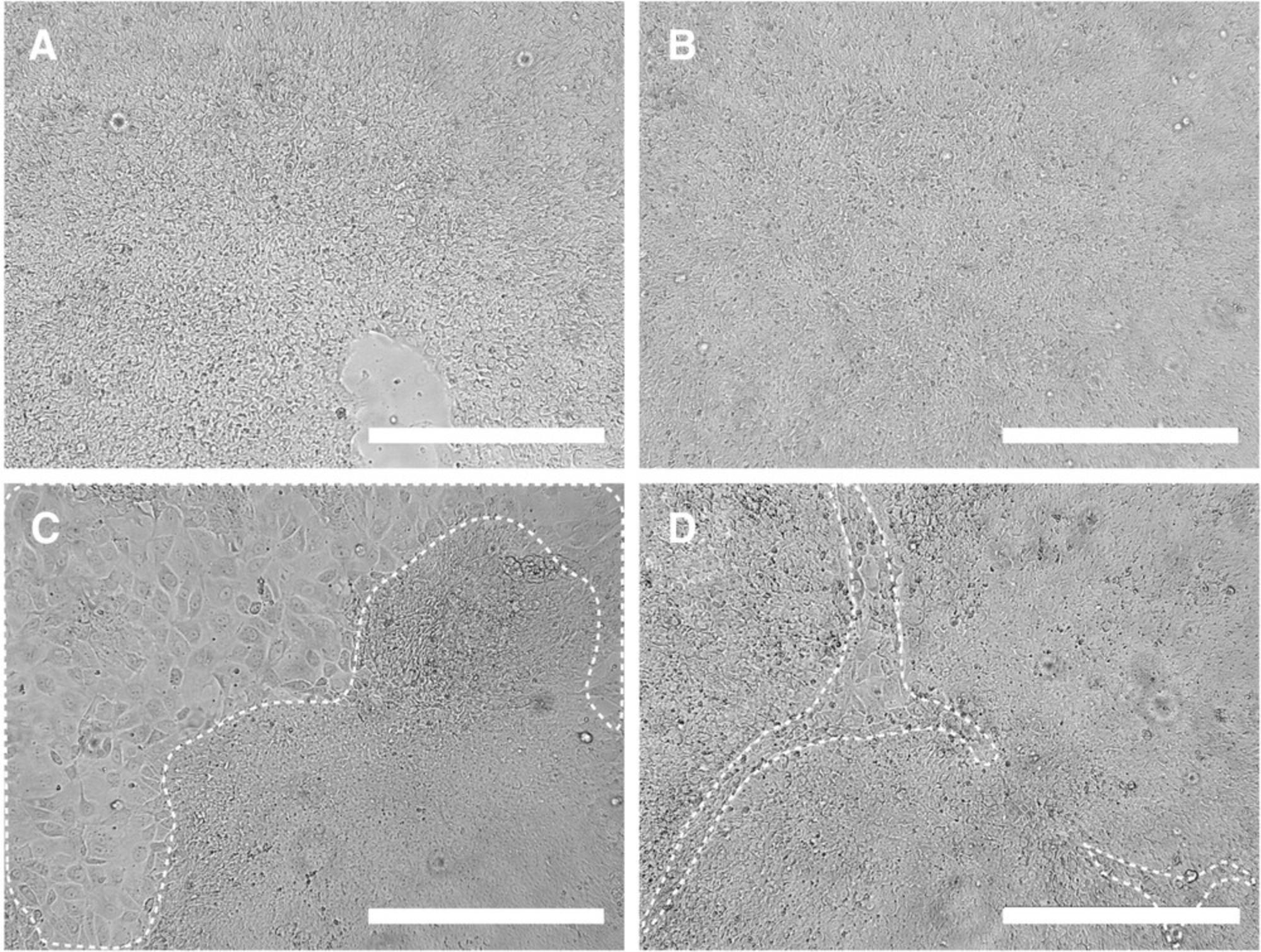
7.Prepare aliquots of FTDA medium without Y-27632, and prewarm to 37°C.
8.Aspirate most of the medium with one pipet for each cell line. Add 2-4 ml FTDA dropwise to each six-well plate.
9.Return the cells into the incubator.
Passaging
10.Prewarm Geltrex-coated plate, PBS, Accutase with Y-27632, and FTDA with Y-27632 to 37°C.
11.Aspirate medium, and wash with 1 ml PBS per 9.6 cm² (that is, per six-well plate).
12.Detach cells with 1 ml Accutase with Y-27632 per 9.6 cm² for 5-10 min in the incubator.
13.Wash off cells in 2 ml washing medium per 9.6 cm² and transfer to a 50-ml conical centrifugation tube.
14.Centrifuge 2 min at 200 × g , room temperature. Aspirate supernatant, loosen cells by tapping bottom of conical centrifugation tube with finger, and resuspend carefully in 500 µl to 1 ml of FTDA with Y-27632.
15.Count cells with CASY or manually with Neubauer chamber with Trypan Blue dye diluted 1:1 with cell suspension.
16.Dilute cell suspension with FTDA with Y-27632. Aspirate Geltrex solution from the coated plates, and plate cells at a seeding density of 4.5-7 × 104 cells per cm² surface.
17.Incubate at 37°C, 90% rH, 5% CO2 and 5% O2, and document data.
18.Around p12-p15, scale up culture from six-well plates to T75 flasks.
Basic Protocol 2: CELL BANKING OF hiPSCs
This section describes the freezing of a master cell bank (MCB) or working cell bank (WCB).
Materials
-
Two to four T75 flasks of confluent (90%-100%) hiPSCs at p18-p25 (Basic Protocol 1)
-
Fetal bovine serum, advanced (FBS; Capricorn Scientific GmbH FBS-11A)
-
Accutase solution with Y-27632 (see recipe)
-
Washing medium (see recipe)
-
Freezing medium (see recipe)
-
Pasteur pipets, sterile, autoclaved
-
Incubator with CO2 and N2 gas supply with an O2 control system set to 37°C, 90% relative humidity (rH), 5% CO2, 5% O2 (hypoxic condition)
-
50-ml conical centrifugation tube
-
Cryovials
-
Cryogenic storage labels or ethanol-resistant markers
-
Freezing container with isopropanol or freezing machine
-
Freezer, −80°C and −150°C (or liquid nitrogen tank)
Cryopreservation of MCB
Volumes listed below are suitable for one T75 flask. For six-well plates, adjust according to growth surface. Two T75 will usually give rise to 25-30 cryovials containing 1 million hiPSCs each.
1.Image cell morphology just before harvesting for MCB, and document.
2.Aspirate medium using a sterile, autoclaved Pasteur pipet for each cell line. Wash with 10 ml PBS per T75 flask.
3.Add 7 ml of Accutase with Y-27632 and incubate 7-10 min in incubator.
4.Resuspend cells in 10 ml washing medium. Centrifuge cells in 50-ml conical centrifugation tube for 3 min at 200 × g , room temperature.
5.Aspirate supernatant, loosen the pellet by tapping bottom of conical centrifugation tube, and carefully resuspend in 2-3 ml FBS.
6.Count cells in CASY or manually with Neubauer chamber.
7.Calculate the amount of freezing medium required to give a final concentration of 1 million cells per 1 ml of freezing medium.
8.Prepare fresh freezing medium (10% DMSO/90% FBS) in an extra tube in advance.
9.Add freezing medium to the cells. Invert and pipet very gentle, and aliquot immediately into labeled cryovials.
10.Transfer MCB vials in the freezing container to a −80°C freezer and let it sit overnight. The next day, transfer to −150°C or liquid nitrogen.
11.Document all the data in a file, and prepare detailed information in the storage list.
Cryopreservation of WCB
12.Thaw 1 vial of the MCB, expand further for 3-5 passages, and cryopreserve as WCB by repeating steps 1-11 and quality controls as specified in Figure 3 (see Support Protocols 1-4) .
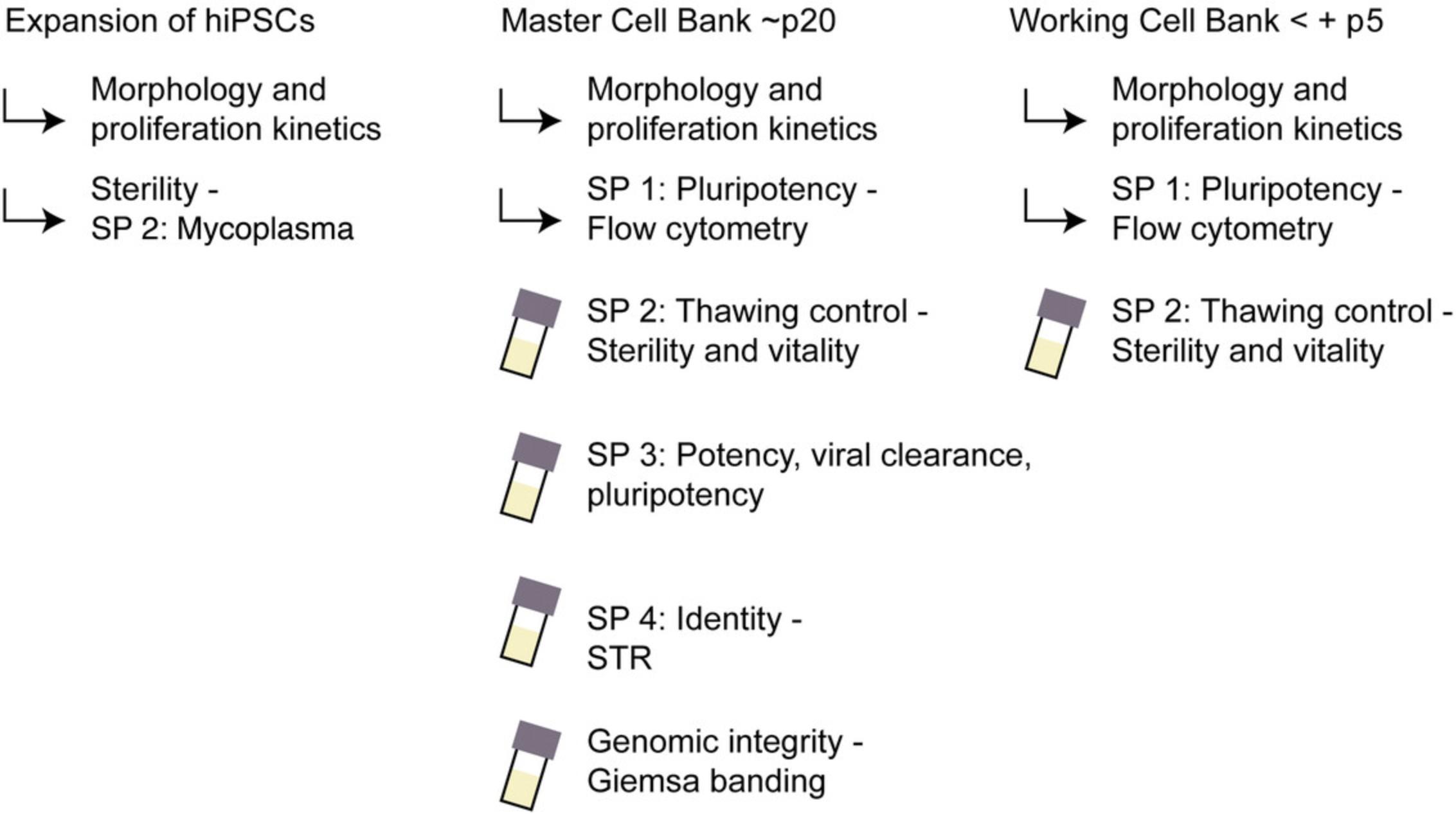
Support Protocol 1: ASSESSMENT OF STEM CELL MARKERS BY FLOW CYTOMETRY
Quantitative live stain of surface markers of stem cells and self-renewal can be done by flow cytometry. For representative results, either sample fresh hiPSCs during cell harvesting for MCB or harvest at least two passages after thawing (see Anticipated Results).
Materials
-
hiPSCs (Basic Protocol 1 or 2)
-
FACS buffer (see recipe)
-
FITC Mouse Anti-human TRA-1-60 (Becton Dickinson cat. no. 560876)
-
FITC Mouse IgM, Isotype Control (Becton Dickinson cat. no. 553474)
-
PE Anti-SSEA3 (Becton Dickinson cat. no. 560879)
-
PE Rat IgM, Isotype Control (Becton Dickinson cat. no. 553943)
-
Flow cytometry tubes compatible with flow cytometer
-
Flow cytometer and software: e.g., Canto II Flow Cytometer and Becton Dickinson FACSDiva Software 6.0
Live-cell staining for SSEA3 and TRA-1-60
1.Resuspend 1 million hiPSCs in 1 ml FACS buffer and incubate 15 min on ice.
2.Split the cell sample into two flow cytometry tubes.
3.Centrifuge 3 min at 200 × g , 4°C, and discard supernatant.
4.Add either PE anti-SSEA3 or FITC mouse anti-human TRA-1-60 antibody, diluted in 100 µl FACS buffer, or the respective isotype control antibodies.
5.Vortex at low speed to resuspend hiPSCs. Incubate at least 30 min on ice or at 4°C.
6.Add 2 ml FACS buffer, centrifuge 5 min at 200 × g , 4°C, and discard supernatant.
7.Repeat step 6 twice with 2 ml PBS.
8.Resuspend in final PBS volume that is adequate for FACS analysis (e.g., 250 µl).
9.Analyze samples by flow cytometry.
Support Protocol 2: THAWING CONTROL—VIABILITY AND STERILITY
Controls the viability, enables hiPSC recovery, and tests for bacterial and fungal contamination (Fig. 4). We recommend thawing two vials to check the reliability of the freezing process (Hunt, 2019).
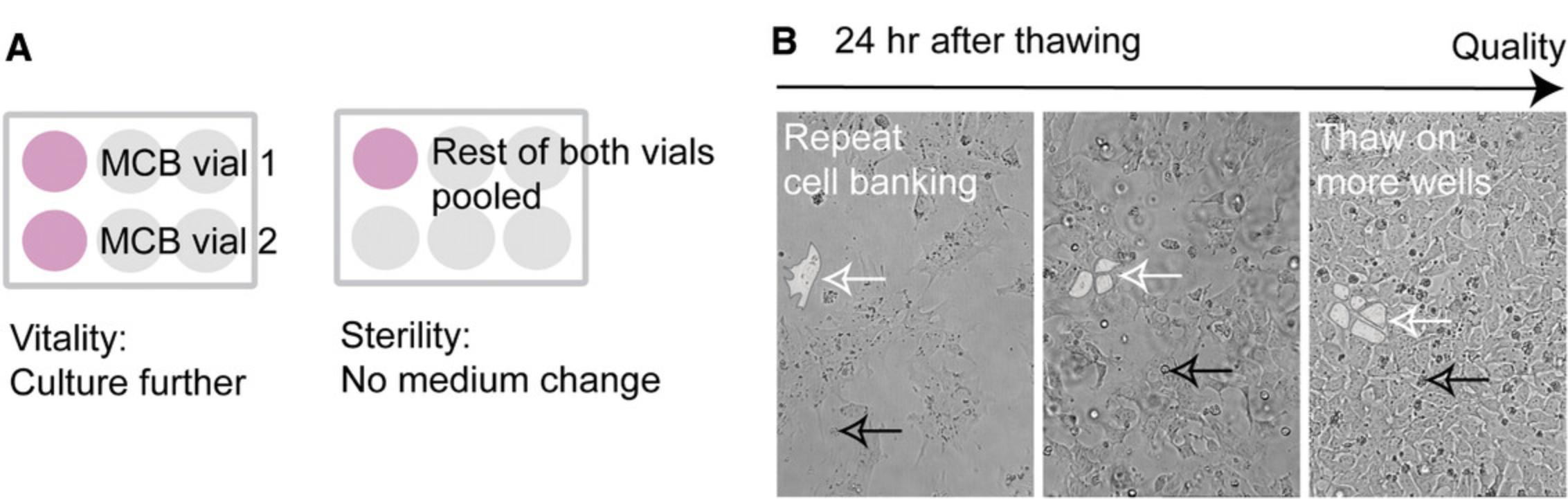
Materials
-
Two MCB cryovials (see Basic Protocol 2, step 10)
-
Six-well plates, coated with Geltrex (see recipe)
-
FTDA with Y-27632 (see recipe)
-
Microscope with camera for documentation
-
Material for mycoplasma PCR (see Table 1 and Breckwoldt et al., 2017)
Gene or other sequence | Function | Forward primer sequence (5′-3′) | Reverse primer sequence (5′-3′) |
---|---|---|---|
GUSB | Housekeeping gene | AAACGATTGCAGGGTTTCAC | CTCTCGTCGGTGACTGTTCA |
Sendai virus | Reprogramming vector | GGA TCA CTA GGT GAT ATC GAG C | ACC AGA CAA GAG TTT AAG AGA TAT GTA TC |
Mycoplasma | Bacterial contamination | TGC ACC ATC TGT CAC TCT GTT AAC CTC | ACT CCT ACG GGA GGC AGC AGT A |
NANOG | Pluripotency endogenous | GATTTGTGGGCCTGAAGAAA | AAGTGGGTTGTTTGCCTTTG |
SOX2 | Pluripotency endogenous | AGTCTCCAAGCGACGAAAAA | TTTCACGTTTGCAACTGTCC |
BRACHYURY (TBXT) | Mesoderm | TGCTTCCCTGAGACCCAGTT | GATCACTTCTTTCCTTTGCATCAAG |
TNNT2 | Mesoderm | TTTGGTTTGGACTCCTCCAT | CTGGAGAGAGGACGAAGACG |
SOX17 | Endoderm | CGCACGGAATTTGAACAGTA | GGATCAGGGACCTGTCACAC |
AFP | Endoderm | AGAACCTGTCACAAGCTGTG | GACAGCAAGCTGAGGATGTC |
FOXA2 | Endoderm | GAGCGGTGAAGATGGAAGG | TGTACGTGTTCATGCCGTT |
TUBB3 | Ectoderm | GGCCAAGGGTCACTACACG | GCAGTCGCAGTTTTCACACTC |
PAX6 | Ectoderm | TGGGCAGGTATTACGAGACTG | ACTCCCGCTTATACTGGGCTA |
SOX1 | Ectoderm | ACCAGGCCATGGATGAA | CTTAATTGCTGGGGAATTGG |
NCAM1 | Ectoderm | ATGGAAACTCTATTAAAGTGAACCTG | TAGACCTCATACTCAGCATTCCAGT |
Thaw cells
1.Prepare two separate Geltrex-coated six-well plates: a sterility control plate with one six-well per clone and a viability control plate with two six-well plates per clone.
2.Thaw two MCB cryovials separately as described in Basic Protocol 1, steps 1-5, resuspending cells in 2.3 ml each of FTDA with Y-27632 (Fig. 4A).
3.On the viability control plate, plate into each well 2 ml from each MCB cryovial.
4.On the sterility control plate, pool the remaining 300 µl from both vials and add 1 ml FTDA with Y-27632.
Viability control
5.Document recovery by photographing the cell density of the viability control plate.
6.Feed hiPSCs daily, and consider subjecting them to further analysis (Support Protocols 3 and 4.
Control for bacterial and fungal contamination including mycoplasma
7.Check regularly for bacterial contamination for 10 days, without changing the medium, by monitoring medium color and potential bacterial motion under the microscope at high magnification, as well as the growth speed and morphology of hiPSCs.
8.Optional: Send supernatant for sterility check.
9.After 72 hr, sample medium supernatant for detection of mycoplasma by PCR as described by Breckwoldt et al. (2017) or using an alternative commercially available mycoplasma detection test.
Support Protocol 3: POTENCY, VIRAL CLEARANCE, AND PLURIPOTENCY—SPONTANEOUS DIFFERENTIATION AND qRT-PCR
The protocol combines simple embryoid body (EB) formation with collagenase- (El-Mounayri et al., 2013) and FBS-based spontaneous differentiation medium (Fig. 5). The potency of hiPSCs in regard to differentiation into the three germ layers is examined by qRT-PCR (see Table 1; Bertero et al., 2016; Breckwoldt et al., 2017; Schaaf et al., 2014). The cDNA is also used to check for viral clearance. In addition, qRT-PCR with endogenous pluripotency factors can be used to better assess markers of stem cell status and self-renewal.
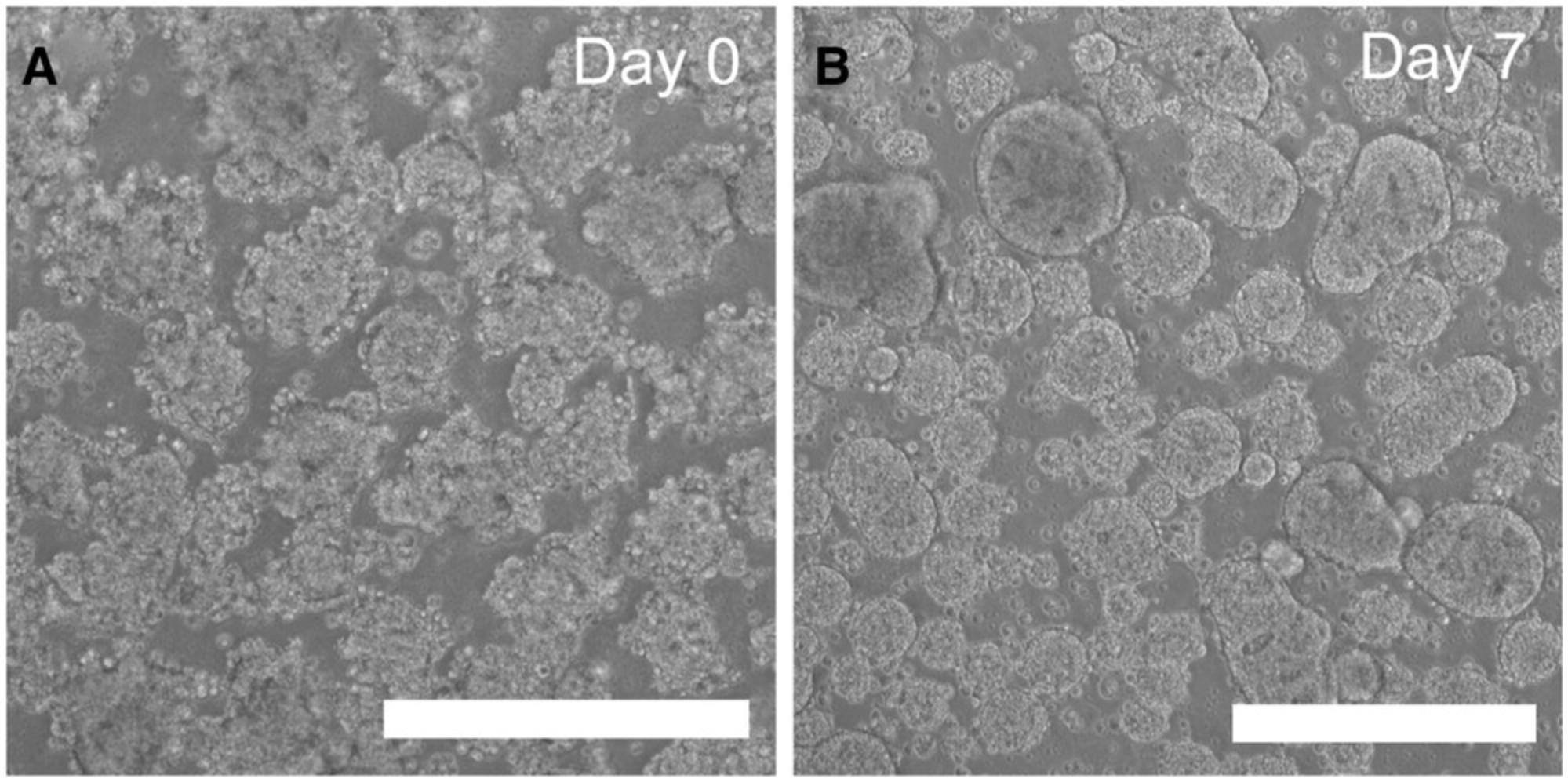
Materials
-
Twice-passaged, almost confluent (90%-100%) hiPSCs thawed from MCB (Basic Protocol 2, step 10)
-
Collagenase II solution (see recipe)
-
EB washing medium (see recipe)
-
Pluronic®-127-coated T25 suspension culture flask or two wells of a six-well plate (see recipe)
-
EB differentiation medium (see recipe)
-
RNA isolation kit (e.g., RNeasy Plus Mini kit, Qiagen 74134)
-
cDNA reverse transcription kit (e.g., High Capacity cDNA RT Kit, Applied Biosystems)
-
Specific primers (see Table 1)
-
qPCR mix with carboxyrhodamine (ROX; e.g., Eva Green qPCR Mix, Solis BioDyne)
-
50-ml conical centrifugation tubes
-
Liquid nitrogen
-
Nanodrop TM ND-1000 spectrophotometer (ThermoFisher Scientific)
-
qPCR machine (e.g., ABI PRISM 7900HT Sequence Detection System, Applied Biosystems)
EB-based spontaneous differentiation
1.Culture hiPSCs to 90%-100% confluency.
2.Aspirate medium, and incubate in 1 ml collagenase solution for 45 min to 2 hr until cell layer lifts off in large junks from the surface.
3.Transfer the cell suspension into a 50-ml conical centrifugation tube.
4.Rinse the wells with 1 ml EB washing medium and add to conical centrifugation tube.
5.Let cell clumps sediment and remove supernatant (first wash step).
6.Add 4 ml EB washing medium and centrifuge 3 min at 200 × g , room temperature.
7.Remove supernatant, and resolve the pellet by tapping the conical centrifugation with a finger. Add 4 ml differentiation medium.
8.Transfer 3 ml of cell suspension to a T25 flask previously coated with Pluronic® F127 and washed.
9.Transfer the remaining cell suspension into a 1.5-ml tube as the day 0 sample. Remove supernatant after a short centrifugation, snap-freeze cell pellet in liquid nitrogen, and store at −80°C.
10.Incubate T25 flask at 37°C, 90% rH, 5% CO2 under normoxic conditions. Cell aggregation will give rise to embryoid bodies overnight (Fig. 5B).
11.Change medium with EB differentiation medium every 2-3 days, for 7 days.
12.On day 7, take sample of EBs and transfer to a conical centrifugation tube. Let this sediment, and remove supernatant so that only about 1 ml of medium with EBs is left. Transfer to a labeled 1.5-ml tube. Centrifuge 1 min at 300 × g , room temperature. Remove supernatant, snap freeze cell pellet in liquid nitrogen, and store at −80°C.
RT-qPCR for germ layer markers, viral clearance, and stem cell markers
13.Isolate RNA and transcribe into cDNA.
14.Run RT-qPCR with primers for markers of each germ layer, for endogenous pluripotency genes (as listed in Table 1), and for reprogramming virus primers according to manufacturer's instructions.
15.Normalize expression to household gene and evaluate expression of differentiated cells as fold of hiPSCs (day 0).
Support Protocol 4: IDENTITY—SHORT TANDEM REPEAT ANALYSIS
For the identity test (Fig. 6), compare the short tandem repeat (STR) profile of the proband sample (e.g., genomic DNA isolated from buffy coats of blood or buccal swab, or preserved primary cells) to that of the MCB hiPSCs. The discrimination capacity of these combined STR-loci is high enough to differentiate all human beings except for identical twins.
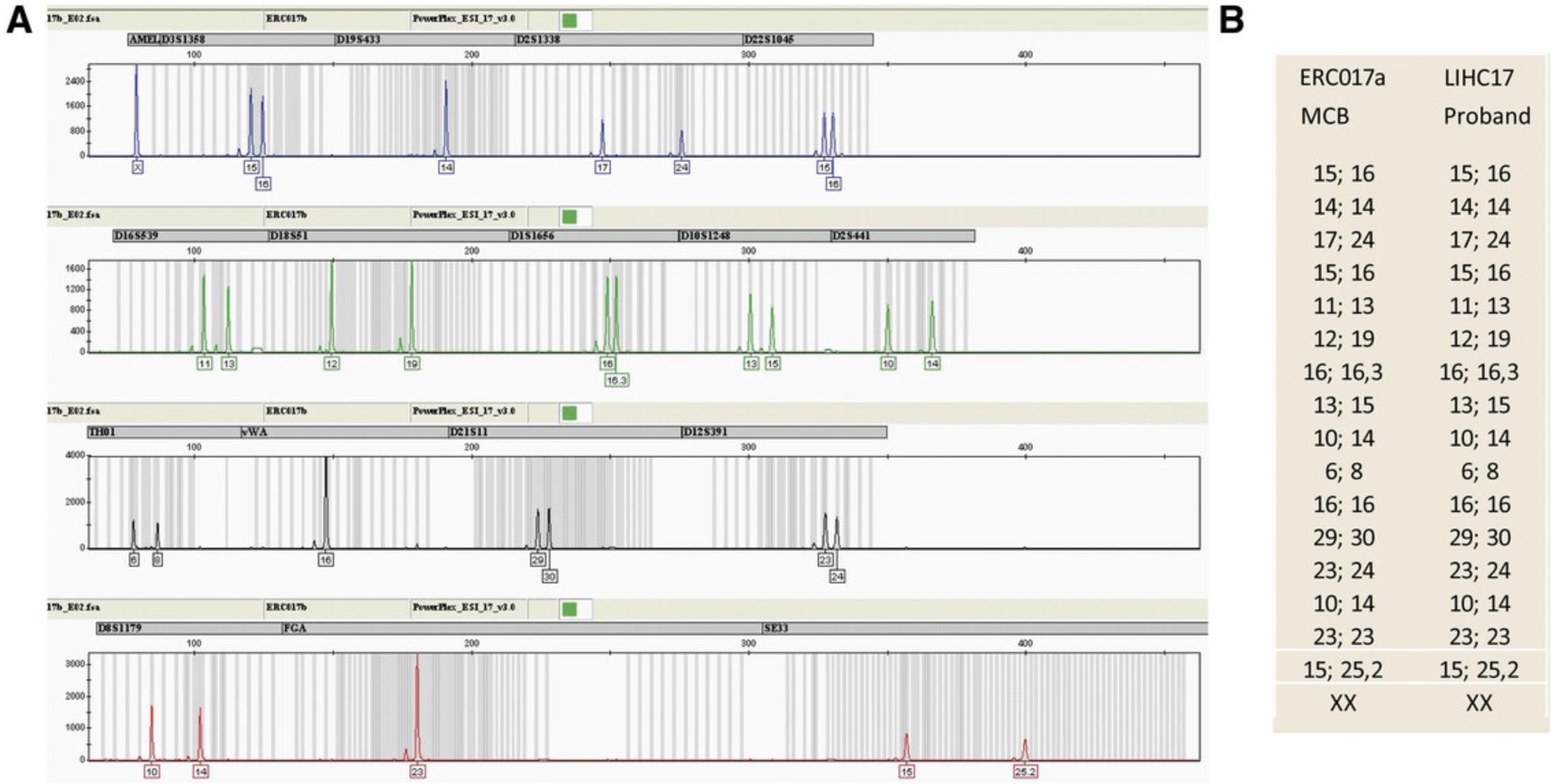
Materials
-
Proband sample (e.g., buccal swab)
-
MCB cryovial (Basic Protocol 2, step 10)
-
Chelex 100® resin (Bio-Rad 143-2832)
-
PowerQuant™ System (Promega PQ 5008)
-
PowerPlex ESI17 Fast™ PCR-amplification multiplex kit (Promega DC 1720) or comparable kit from another company (e.g., ThermoFisher, Qiagen, or Promega)
-
MicroAmp™ Optical Adhesive Film (Applied Biosystems 4311971)
-
HiDi Formamide (Applied Biosystems 4311320)
-
LiChrosolv water for chromatography (Merck 1.15333)
-
Forensic swabs (Sarstedt 80.634)
-
Heater, 56°C
-
96-well PCR plate (e.g., 96 PCR Plate half skirt, Sarstedt 72.1979.202)
-
ABI 7500 Realtime PCR System
-
ABI 3130 Genetic Analyser
-
Genemapper ID 3.2 (software for the identification of the alleles)
Sample preparation
1.Thaw 1 vial of MCB as described in Basic Protocol 1.
2.Centrifuge 3 min at 200 × g , room temperature, aspirate medium, and take sample with swab from cell pellet at the bottom of Falcon tube.
DNA extraction: Chelex method
Chelex®100 is an ion-exchange resin that inactivates DNA destructing nucleases by removing magnesium, exposure to 100°C disrupts the cell membranes, proteins and denatures the DNA yielding single stranded DNA (Butler, 2010; Walsh, Metzger, & Higuchi, 1991).
3.Prepare a 5% suspension of Chelex®100 resin in distilled water.
4.Transfer each swap (one with proband sample, one with MCB sample) into a 1.5-ml reaction tube.
5.Add 500 µl of Chelex®100 suspension to the swab in the tube and shake several times to mix the suspension.
6.Incubate 30 min at 56°C.
7.Remove the tubes from the heater and increase the temperature to 100°C.
8.Incubate the tubes 6 min at 100°C.
9.Vortex the tubes vigorously and centrifuge 1 min at 200 × g , room temperature.
10.DNA in the supernatant is now ready for PCR.
Optional: DNA quantification
The most sensitive and specific way to quantify human DNA is real-time quantitative PCR (qPCR). Here, we use the PowerQuant™ System (Promega) and the ABI 7500 Real Time PCR System (ThermoFisher). The PowerQuant™ System simultaneously quantifies the amount of total, of male, and of degraded gDNA. DNA quantification is performed according to the manufacturer´s protocol.
11.Prepare a serial dilution of the PowerQuant™ Male gDNA Standard (provided with PowerQuant kit).
12.Prepare a reaction mix of PowerQuant™ 2× Master Mix, PowerQuant™ 20× Primer Mix, and water for the number of reactions planned plus 10%. The reaction volume is 20 µl.
13.Add 18 µl of the reaction mix to the reaction wells of a 96-well PCR plate.
14.Add 2 µl of the gDNA standards the controls and the unknown DNA samples to the appropriate wells; seal the plate with MicroAmp Optical Adhesive Film. Centrifuge the plate briefly.
15.Start the thermal cycling run according to the manufacturer's instructions.
16.The analysis of the raw data is performed with the HID Real Time PCR software; the data are then imported into the PowerQuant Analysis Tool and stored there.
PCR amplification of STR loci with PowerPlex ESI17 Fast™ multiplex kit (Promega)
The Multiplex Kit PowerPlex ESI 17 fast contains the 16 so-called ESS (European Standard Set of STRs) systems plus amelogenin for sex determination.
- 17.Prepare a reaction mix:
- 2 µl 5× master mix including Taq polymerase
- 1 µl 10× primer mix
- 0.5 ng template DNA
- Water (amplification grade) as needed to 10 µl.
The optimal amount of template DNA for generating well-balanced STR-profiles is 0.5 ng of total template DNA.
17.Run the PCR in the thermocycler for 29 cycles according to the manufacturer´s protocol
18.Store amplified samples at 4°C until ready to perform capillary electrophoresis
Capillary electrophoresis of the PCR products on the ABI 3130 Genetic Analyzer
Five different fluorescent dyes are attached to the primers and to the size standard. The different fragment lengths of the STR alleles and the use of different fluorescent dyes allows simultaneous detection of all loci and alleles in a single run. The capillaries are 36 cm in length and are refilled with a special polymer for the separation of the alleles after each run.
19.Dilute each amplified sample by adding 30 µl of LiChrosolv® water.
20.Prepare a formamide size-standard mix (1000 µl HIDI formamide + 30 µl size standard)
21.Add 13 µl of this mixture to the number of wells of a 96-well plate that are needed for the amplified samples and allelic ladder. Add 2 µl of each PCR product and 2 µl of the allelic ladder, respectively.
22.Incubate the plate 3 min at 94°C, and then cool for 3 min in a freezer at −18°C.
23.Run the plate in the ABI 3130 Genetic Analyzer according to the manufacturer's protocols.
24.Analyze the raw data with Genemapper ID 3.2 software.
REAGENTS AND SOLUTIONS
NOTE : All media are prepared under sterile conditions and, if not provided sterile by the supplier, vacuum filtered with a 0.2-µm-pore-size filtration set (TPP, 99500).
Accutase containing Y-27632
Add 10 μM Y-27632 solution (see recipe below) to freshly thawed Accutase (Sigma-Aldrich, A6964) by diluting stock solution 1:1000. Store up to 2 weeks at 4°C.
Basic fibroblast growth factor (bFGF) solution
Dissolve bFGF (PeproTech, 100-18B) to a stock concentration of 100 μg/ml in PBS (Gibco, 10010-049) containing 0.1% (w/v) bovine serum albumin (BSA). Working concentration in FTDA is 30 ng/ml. Store aliquots for up to 6 months at −80°C, or thawed aliquots up to 1 week at 4°C. Always add fresh to medium.
Collagenase type 2 solution
Dissolve collagenase type 2 (Worthington LS004176) in DMEM (ThermoFisher 41965-039) to a concentration of 200 U/ml. Incubate at room temperature for 1 hr, divide into aliquots, and store up to 1 year at −20°C. Thaw aliquots at 4°C as needed, and add 6 µl/ml DNase solution fresh before use.
DNase solution
Dissolve 100 mg DNase I, type V (from bovine spleen, Sigma-Aldrich D8764), in 50 ml PBS, and divide into 2-ml aliquots. Store the aliquots up to 1 year at −20°C.
EB differentiation medium
- 1× L-glutamine (ThermoFisher 25030081)
- 1 mM HEPES solution (see recipe)
- 20% fetal bovine serum advanced (FBS, Capricorn Scientific GmbH, FBS-11A)
- 1% non-essential amino acid (NEAA; ThermoFisher 11140-050)
- 0.1% 1-thioglycerol (Sigma-Aldrich M6145)
- IMDM medium (ThermoFisher 12440-053)
- Prepare medium fresh.
EB washing medium
RPMI containing 6 µl/ml DNase II solution and 0.5% (v/v) human serum albumin. Add DNase I fresh to the medium before use.
FACS buffer for blocking and staining (live-cell staining)
PBS supplemented with 5% fetal bovine serum advanced (FBS, Capricorn Scientific GmbH, FBS-11A). Store up to 4 weeks at 4°C.
Freezing medium
Fetal bovine serum advanced (FBS, Capricorn Scientific GmbH, FBS-11A) with 10% DMSO (Sigma-Aldrich, D8418) and 10 μM Y-27632 (1:100 stock solution). Aliquot FBS and thaw fresh for each cryobanking process. DMSO releases heat during mixing with FBS. Alternatively, use Gamma Irradiated FBS (FBS, Capricorn Scientific GmbH, FBS-GI-12A) to avoid contamination with bovine viruses, as FBS cannot be fully sterilized. In addition, use of DMSO-free medium should be considered (Awan et al., 2020).
FTDA containing Y-27632
Supplement FTDA medium with bFGF solution (see recipe), and add Y-27632 solution (see recipe) in 1:1000 dilution to a final concentration of 10 μM.
Recipe
FTDA medium (Frank et al., 2012) FTDA medium (Frank et al., 2012)
DMEM/F12 (ThermoFisher 21331-020) containing: | |
L-Glutamine (ThermoFisher 25030081) | 2 mM |
Transferrin-selenium solution (see recipe; 1:10,000) | 5 mg/L (transferrin)/5 µg/L (Se) |
Human Serum Albumin (Biological Industries 05-720-1B) | 0.10% (v/v) |
Lipid mix (Sigma-Aldrich L5146) | 1× |
Insulin (Sigma-Aldrich I9278) | 5 mg/L |
Dorsomorphin (Tocris 3093) | 50 nM |
Activin A (R&D Systems 314-BP) | 2.5 ng/ml |
TGFβ1 (PeproTech 100-21C) | 0.5 ng/ml |
bFGF solution (see recipe; add fresh) | 30 ng/ml |
Supplement medium with bFGF immediately before use. FTDA without bFGF can be stored up to 2 weeks in the dark at 4°C. We do not recommend using antibiotics in hiPSC culture.
Geltrex solution and coating
Thaw Geltrex (LDEV-Free Reduced Growth Factor Basement Membrane Matrix, ThermoFisher A14132) on ice and dilute 1:100 (v/v) in cold (4°C) DMEM/F12 (ThermoFisher, 21331-020). Use this to coat six-well plate (ThermoFisher, 140675) or T75 flasks (ThermoFisher, 156472) according to manufacturers' instructions (more details is provided by the European Bank for Induced Pluripotent Stem Cells (EBiSC; see Internet Resources). Coated plates or flasks wrapped with Parafilm can be kept up to 2 week at 4°C. Warm before use and aspirate the supernatant.
HEPES, pH 7.4
Dissolve HEPES (Roth, 9105.4) in PBS to a concentration of 1 M. Adjust the pH to 7.4 with potassium hydroxide. Store up to 1 year at 4°C.
Pluronic® F-127 coating for low-attachment culture surface
Add 1 ml of 1% Pluronic® solution (see recipe) per well of a six-well plate or 2.5 ml per T25 flask at least 1 day before use, and incubate in humidified 37°C incubator for up to 2 weeks. Wash twice with PBS before use.
Pluronic® F-127 solution
Dissolve 1% Pluronic® F-127 (Sigma-Aldrich P2443) in 500 ml PBS (ThermoFisher 14190-094, [w/v]; 5 g). Store up to 1 year at 4°C.
Sodium selenite
Dissolve 382 μM sodium selenite (Sigma-Aldrich S5261) in PBS (e.g., 33 mg in 500 ml PBS). Store at 4°C for up to 1 year.
Transferrin–selenium solution
Dissolve 100 mg transferrin (Sigma-Aldrich T8158) in 2 ml sodium selenite (see recipe) and prepare aliquots of 55 μl. Store them up to 1 year at −80°C, and thaw fresh for preparation of FTDA.
Washing medium (for thawing and passaging)
DMEM/F12 (ThermoFisher 21331-020) containing 10 μM Y-27632.
Y-27632 solution
Dissolve the rho kinase inhibitor Y-27632 (Biorbyt orb60104) in injection-grade water to a stock concentration of 10 mM. Store aliquots up to 1 year at −20°C, and after thawing, up to 1 week at 4°C. Working concentration is 10 μM.
COMMENTARY
Background Information
The techniques described in this protocol aim to (i) fulfill regulatory, ethical, and safety requirements for basic research; (ii) meet common quality criteria for the publication or registration of lines in registries such as hPSCreg.eu, which combine release and informational criteria that characterize the cell lines; and (iii) distinguish hiPSCs of superior versus mediocre quality for successful hiPSC-based experiments (predictive criteria).
The main safety and regulatory aspects are as follows. (i) For basic research, donor consent and viral contamination. Points to consider for donor consent, data protection, and derivation of primary cells have been discussed previously (Lomax, Hull, Lowenthal, Rao, & Isasi, 2013; Morrison et al., 2017; Orzechowski, Schochow, Kühl, & Steger, 2020). Before reprogramming, the proband or the primary cells should be tested for HIV and hepatitis (be negative on viral screening for HIV1, HIV2, HBV, and HCV by qPCR; Andrews et al., 2015). After reprogramming, MCBs free of viral reprogramming vectors (i.e., showing vector clearance in Support Protocol 3) can be considered BSL-1 instead of BSL-2. (ii) Criteria for the registration of cell lines used for basic research purposes include proof of markers of stem cell status and proliferation, differentiation potency, genetic fidelity, and identity (see the Internet Resources and Key References for more detail). (iii) Which criteria are meaningful and assure successful hiPSC-based experiments strongly depends on the type of experiments being conducted in a given laboratory. However, in general, although there are incompletely pluripotent hiPSC lines with intrinsically mediocre quality, it is also possible for hiPSC lines of good quality to lose their differentiation potency during culture. Thus, we recommend evaluating certain parameters (vitality, morphology, signs of contaminations, proliferation, and maybe expression of stem cell markers; Table 2, Fig. 3) frequently during expansion for MCB, for each WCB and for critical experiments.
Attribute | Criteria | Analytical method | Alternatives |
---|---|---|---|
Proliferation | Short doubling time, <1 day | Counting cells during seeding and harvest (Basic Protocol 1) | Proliferation markers |
Morphology | Cobblestone-like, small stem cells, no differentiating cells | Microscopic control (Support Protocol 2) | Automated high-content screening |
Viability | >80% viable hiPSCs during expansion, >90% for freezing | CASY (Basic Protocol 1) | Trypan Blue staining, viability dye (e.g., eBioscience™ Fixable Viability Dye eFluor™ 450 65-0863-14) |
Aseptic culture: mycoplasma | Mycoplasma free | PCR on supernatant of confluent or 72-hr cultured hiPSCs (Support Protocol 2; Breckwoldt et al., 2017) | Broth-agar microbiological culture method, ELISA, DNA stain test |
Aseptic culture: bacteria | Contamination free | Microscopic control (Support Protocol 2) | Sterility test by membrane filtration or direct inoculation |
The assays described are commonly used standard assays that enable a cost- and labor-saving workflow to achieve minimal criteria (Table 3, Fig. 3); however, there are plenty of alternatives (Tables 2 and 3). We strongly encourage changing the quality tests depending on the requirements of the specific projects.
Attribute | Criteria | Analytical method(s) | Alternatives |
---|---|---|---|
Pluripotency | High expression of stem cell markers | Flow cytometry (Support Protocol 1) qRT-PCR (Support Protocol 3) | Pluritest, Scorecard, immunofluorescence, Taqman |
Potency | Differentiation into all three germ layers | EB-based spontaneous differentiation and qRT PCR (Support Protocol 3) | Teratoma assay, directed differentiation |
Genomic integrity | Normal karyotype | Metaphase cytogenetics, Giemsa banding (Breckwoldt et al., 2017) | FISH, array-CGH, sequencing, spectral karyotyping (SKY) NanoString |
Identity | Genomic DNA of hiPSCs at MCB level identical to sample derived directly from donor | STR analysis (Support Protocol 4) | |
Viral clearance | No detection of virus genome | Perform RT-PCR according to manufacturer's instructions (Support Protocol 4) | Depending on reprogramming method |
To assess differentiation potential, we describe EB-based spontaneous differentiation. However, results from EBiSC indicate that hiPSCs that lack spontaneous differentiation still showed differentiation potential in all three germ layers (O'Shea, Steeg, Chapman, Mackintosh, & Stacey, 2020). Directed differentiation, on the contrary, might test very specific pathways, and the optimal assay to use is still under discussion (The International Stem Cell Initiative, 2018). For a predictive assay, most probably directed differentiation into the cell type of interest is the most meaningful readout (Liu et al., 2019).
Human iPSC lines have repeatedly been shown to be prone to accumulate genetic aberrations (Henry, Hawkins, Boyle, & Bridger, 2019; Steinemann, Göhring, & Schlegelberger, 2013; Weissbein, Benvenisty, & Ben-David, 2014), occurring during reprogramming as well as during expansion of hiPSC clones. Moreover, hiPSC have high clonality, and subclones can overgrow the culture in the course of a few passages (Brenière-Letuffe et al., 2018). We assessed karyotype by G-banding; however, genetic aberrations can be analyzed by many other methods. Examples are qPCR to detect common karyotypic abnormalities or mosaicism (Assou et al., 2020), fluorescence in situ hybridization (FISH) or spectral karyotyping (SKY; Moralli et al., 2011), and sequencing approaches (Henry et al., 2019; Kyriakides, Halliwell, & Andrews, 2018).
Global confirmation of marker of stem cell status and self-renewal with methods like Pluritest or Scorecard is clearly more informative than the use of single markers (Fergus, Quintanilla, & Lakshmipathy, 2015; Müller, 2012), but is considerably more expensive.
Cell line identity is an often-neglected part of quality control in hiPSC basic research, although data shows that between 10% and 20% of hiPSC lines are misidentified (De Sousa et al., 2017; O'Shea et al., 2020; Yaffe, Noggle, & Solomon, 2016). Here, we describe STR profiling, which is the standard for authentication of human samples (Barallon et al., 2010).
From basic to translational research: requirements for preclinical or clinical-grade hiPSC banking
The proposed workflow specifies minimal scientific and technical elements of hiPSC quality control to enable reproducibility for basic research, which might be sufficient for preclinical proof-of-concept testing. However, it would clearly fall short of the regulatory requirements needed to generate data to be submitted for regulatory approval or clinical use (see Internet Resources and Key References, below). From a technical and scientific view, the applied assays for quality control of hiPSCs for both clinical and research work are comparable. However, for clinical applications, the scope changes from more informative assays about cell line characteristics to areas including traceability, risk assessment, adventitious agent testing, critical quality attributes, product characterization, and process standardization (Creasey, Stacey, Bharti, Sato, & Lubiniecki, 2019, Crook, Hei, & Stacey, 2010; O'Shea et al., 2020; Stacey, 2009; Stacey et al., 2019). For all reagents and materials that come in contact with hiPSCs, risk-assessment procedures need to be implemented, and processes and equipment must be qualified (e.g., DQ/OQ/IQ/PQ to apply for a GMP product manufacturing license; Rehakova, Souralova, & Koutna, 2020; Shafa et al., 2020; see Internet Resources). Qualification of raw materials and bioanalytics are very different, ranging from the selection of irradiated FBS to the gas quality for incubators. The occurrence of karyotypic and other genetic abnormalities in clinical-use hiPSCs affects genetic safety, as it might result in higher teratogenicity (Merkle et al., 2017; Rebuzzini, Zuccotti, Alberto, & Garagna, 2016; Stacey et al., 2019), and there is no clear consensus regarding methods for tumorigenicity assessment (Abbot et al., 2018; Andrews et al., 2015; Liu et al., 2019; Sato et al., 2019; Stacey et al., 2013). The use of the rho kinase inhibitor described here might increase the malignant potential by inducing subchromosomal abnormalities (Bai et al., 2015).
One other important aspect is documentation: Naming hiPSCs according to the standard nomenclature specified by (Kurtz et al., 2018) and recording the most important hiPSC parameters (see Basic Protocol 1) is recommended for any hiPSCs research project. However, for any more formal quality-management systems, e.g., for Good Laboratory Practice (GLP) or the more specific Good Cell Culture Practice (GCCP), accurate and extensive records are needed (Coecke et al., 2005).
Critical Parameters
The success of the cell-banking approach depends on the quality of the preserved stem cells. Most time-consuming are the quality checks at the MCB level (Table 3); thus, it is critical to preselect hiPSC lines based on the parameters specified in Table 2. We recommend freezing only fast-growing hiPSCs, as an accepted indicator for pluripotency (Kuo et al., 2020), and only cells with excellent morphology. Stringently discard lines that show evidence of spontaneous differentiation during expansion for MCB (Fig. 2). In addition, the passage number for the MCB should be kept as low as possible: On the one hand, genetic aberrations accumulate over culture time, whereas on the other hand a stable reprogramming state and full clearance of reprogramming vectors has to be ensured. Hence, passage number depends on both the reprogramming and culture methods. As the cells are passaged with high confluency two to three times per week in this protocol, cryobanking at around p20 corresponds to a comparably low number of population doublings. We found that lower passage numbers were inferior in regard to pluripotency and (cardiac) differentiation potency and included a higher frequency of clones that retained Sendai virus (data not shown).
Troubleshooting
Table 4 lists problems that may arise with these procedures, as well as their possible causes and solutions.
Problem(s) | Possible cause(s) | Solution(s) |
---|---|---|
Cells are not evenly distributed in culture well or flask | Poor distribution of cells during plating | Move six-well plate or flask back and forth from side to side three times to generate a wave. Check under the microscope after 30 min incubation whether cells are dispersed optimally across the well or flask surface. |
Cells detach during feeding | Suboptimal coating Harsh feeding Contamination | Control Geltrex coating. Feed dropwise. Early sign of contamination. |
Cell layer is less confluent than expected after 3-4 days of culture | Low seeding density due to miscounting Slow cells growth | Pool wells of the same cell line, e.g., two into one. Slow cell proliferation can be a sign of technical problems, loss of pluripotency, or contamination. For MCB production, only exponentially growing cell cultures should be used. |
Medium is turbid, yellow, or pink Bacterial movement or brown molecular motion is observed | Warning signs that might indicate bacterial contamination | Eliminate infected cultures and sterilize all equipment and consumables. Quarantine all the cultures running in parallel. |
Spontaneous differentiation occurs during expansion, or no recovery of bad morphology occurs | Poor quality of the original cell clone Technical problem(s) during stem cell culture | Do not proceed to MCB—use other clones. If not available, consider iPSC pool purification e.g., using magnetic-activated cell sorting (MACS) of TRA-1-60 or SSEA4. |
No EB formation occurs | hiPSCs too confluent before starting collagenase treatment | Start at 90% confluency (see Figures 2A and 4B). |
MCB vials have thawed | Blackout or freezer broken | Distribute MCB vials to several locations as mirror backups, ideally in another building or institution. |
PCR results during STR analysis are unclear | Amount of template DNA not optimal | DNA quantification is optional, but strongly recommended, as the subsequent PCR of STR markers will give much better results. |
Understanding Results
Only a fraction of MCB meets all quality criteria
Stringent quality controls result in many hiPSC lines that need to be discarded. We produced MCBs that met all quality criteria for 42 probands with the workflow described in this protocol. In this process, we discarded 67 clones during the expansion phase. Most of the discarded clones (65%) showed morphological signs of differentiation, and the rest slow growth, technical problems, or bacterial contamination. The first MCB passed all quality controls in only one-quarter of probands. Two MCBs had to be prepared for half of the probands and 3-5 MCBs for another quarter. The causes were mostly chromosomal aberrations (50%) or lack of Sendai virus clearance (25% of discarded clones, Fig. 7). We thus recommend thawing 3-6 young hiPSC clones per proband in parallel to account for the selection process.
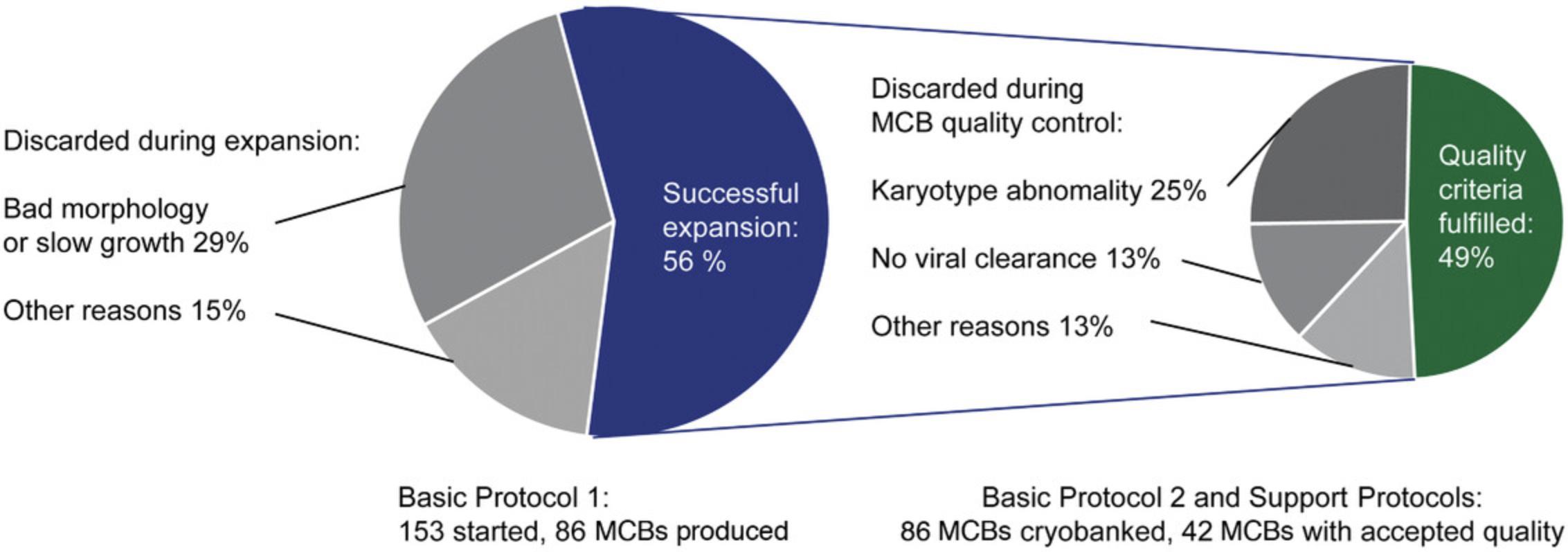
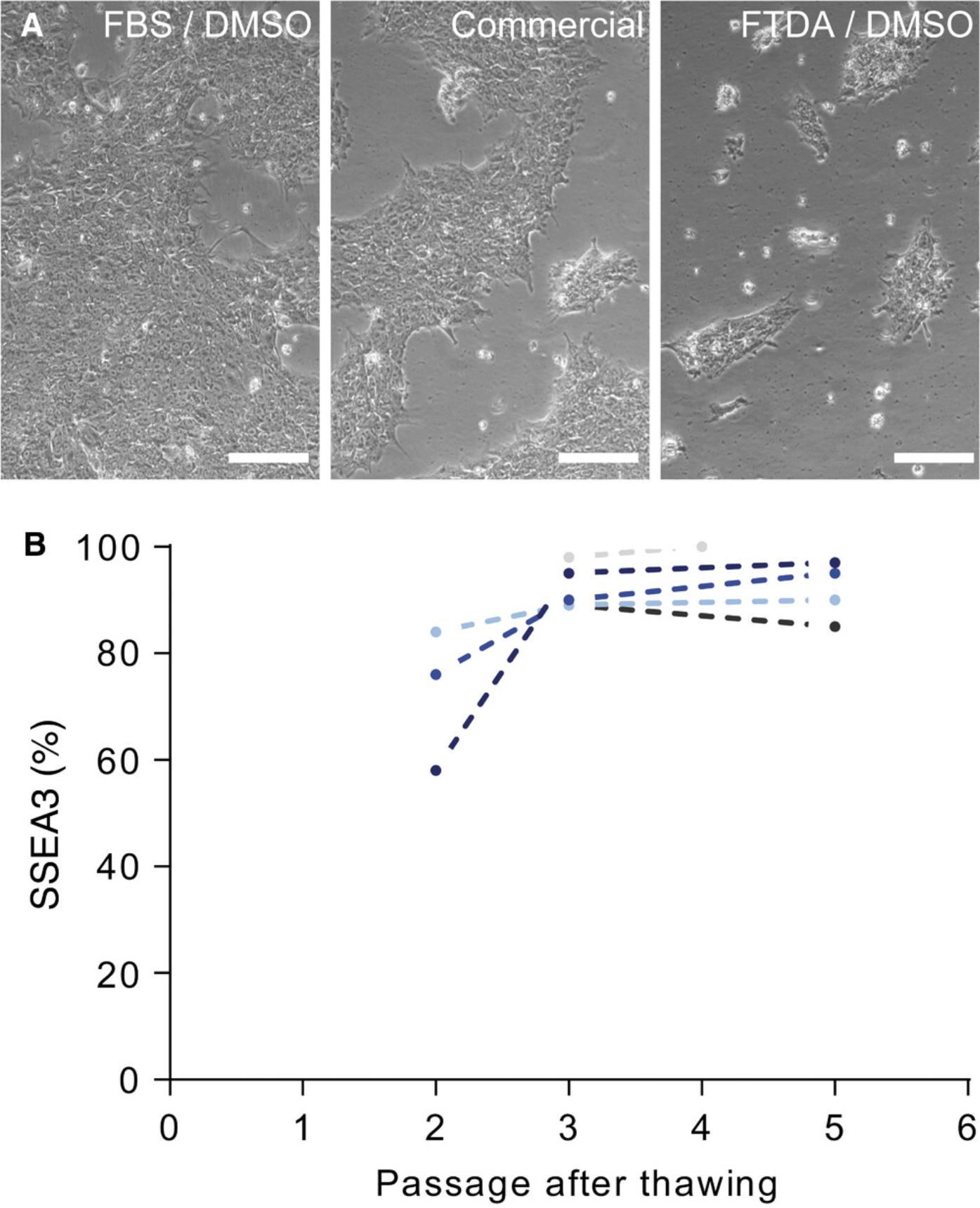
Recovery of thawed hiPSCs in 2-3 passages
Cell banking relies on the fast recovery of cells after thawing. We chose 90% FBS/10% DMSO as cryopreservation medium; however, there are a variety of xeno-free commercial freezing media available. In our hands, however, FBS-based medium was superior to commercial freezing medium (as determined by batch and time matched freezing and thawing, Fig. 7A). With Basic Protocol 2 described here, hiPSCs regularly regain pluripotency and vitality within 2-3 passages after thawing (Fig. 7B), allowing experiments (e.g., directed differentiation) to be started after three passages.
Time Considerations
For an efficient workflow, parallel cell culture of six to ten hiPSC lines, typically three to six clones from two probands, is recommended. From thawing of young clones at p10 to MCB at p20, about 5 weeks with daily hands-on work need to be scheduled. In-house quality control (Support Protocols 1-4) will take about 4-6 additional weeks.
Acknowledgments
We thank June Uebeler for expert technical help with mycoplasma detection. The FACS analysis was done with support from the HEXT FACS Core unit of the UKE. Jennifer Kaiser helped with karyotype analysis. We thank Prof. Kaomei Guan for sharing the protocol for spontaneous differentiation medium and expert advice for hiPSC characterization. Dr. Boris Greber and Dr. Nicole Dubois generously provided protocols for hiPSC culture and EB formation as well as hands-on training.
This study was supported by the European Research Council (ERC-AG IndivuHeart), the Deutsche Forschungsgemeinschaft (DFG Es 88/12-1, DFG HA 3423/5-1), and the German Centre for Cardiovascular Research (DZHK). Open access funding enabled and organized by Projekt DEAL.
Author Contributions
Aya Shibamiya : Conceptualization; data curation; formal analysis; investigation; methodology; project administration; validation; visualization; writing-original draft; writing-review & editing. Elisabeth Schulze : Investigation; methodology; project administration; writing-review & editing. Dana Krauß : Investigation; methodology. Christa Augustin : Conceptualization; data curation; investigation; methodology; project administration; supervision; visualization; writing-original draft; writing-review & editing. Marina Reinsch : Investigation; methodology; validation. Mirja Loreen Schulze : Investigation; methodology; visualization. Simone Steuck : Investigation; methodology. Giulia Mearini : Investigation. Ingra Mannhardt : Investigation; methodology. Thomas Schulze : Investigation. Birgit Klampe : Investigation. Tessa Werner : Investigation. Umber Saleem : Investigation. Anika Knaust : Investigation. Sandra D. Laufer : Investigation. Christiane Neuber : Investigation. Marta Lemme : Investigation. Charlotta Sophie Behrens : Investigation. Malte Loos : Investigation. Florian Weinberger : Project administration; supervision; writing-review & editing. Sigrid Fuchs : Investigation; methodology; visualization. Thomas Eschenhagen : Conceptualization; funding acquisition; project administration; resources; supervision; writing-review & editing. Arne Hansen : Project administration; resources; supervision; writing-review & editing. Bärbel Maria Ulmer : Conceptualization; data curation; formal analysis; funding acquisition; investigation; methodology; project administration; supervision; validation; visualization; writing-original draft; writing-review & editing.
Literature Cited
- Abbot, S., Agbanyo, F., Ahlfors, J. E., Baghbaderani, B. A., Bartido, S., Bharti, K., … Zoon, K. (2018). Report of the international conference on manufacturing and testing of pluripotent stem cells. Biologicals , 56, 67–83. doi: 10.1016/j.biologicals.2018.08.004.
- Andrews, P. W., Baker, D., Benvinisty, N., Miranda, B., Bruce, K., Brüstle, O., … Zhou, Q. (2015). Points to consider in the development of seed stocks of pluripotent stem cells for clinical applications: International Stem Cell Banking Initiative (ISCBI). Regenerative Medicine , 10(2 Suppl), 1–44. doi: 10.2217/rme.14.93.
- Andrews, P. W., Ben-David, U., Benvenisty, N., Coffey, P., Eggan, K., Knowles, B. B., … Stacey, G. N. (2017). Assessing the safety of human pluripotent stem cells and their derivatives for clinical applications. Stem Cell Reports , 9(1), 1–4. doi: 10.1016/j.stemcr.2017.05.029.
- Assou, S., Girault, N., Plinet, M., Bouckenheimer, J., Sansac, C., Combe, M., … De Vos, J. (2020). Recurrent genetic abnormalities in human pluripotent stem cells: Definition and routine detection in culture supernatant by targeted droplet digital PCR. Stem Cell Reports , 14(1), 1–8. doi: 10.1016/j.stemcr.2019.12.004.
- Awan, M., Buriak, I., Fleck, R., Fuller, B., Goltsev, A., Kerby, J., … Stacey, G. N. (2020). Dimethyl sulfoxide: A central player since the dawn of cryobiology, is efficacy balanced by toxicity? Regenerative Medicine , 15(3), 1463–1491. doi: 10.2217/rme-2019-0145.
- Baghbaderani, B. A., Tian, X., Neo, B. H., Burkall, A., Dimezzo, T., Sierra, G., … Rao, M. S. (2015). CGMP-manufactured human induced pluripotent stem cells are available for pre-clinical and clinical applications. Stem Cell Reports , 5(4), 647–659. doi: 10.1016/j.stemcr.2015.08.015.
- Bai, Q., Ramirez, J. M., Becker, F., Pantesco, V., Lavabre-Bertrand, T., Hovatta, O., … De Vos, J. (2015). Temporal analysis of genome alterations induced by single-cell passaging in human embryonic stem cells. Stem Cells and Development , 24(5), 653–662. doi: 10.1089/scd.2014.0292.
- Barallon, R., Bauer, S. R., Butler, J., Capes-Davis, A., Dirks, W. G., Elmore, E., … Kerrigan, L. (2010). Recommendation of short tandem repeat profiling for authenticating human cell lines, stem cells, and tissues. In Vitro Cellular and Developmental Biology - Animal , 46(9), 727–732. doi: 10.1007/s11626-010-9333-z.
- Bertero, A., Pawlowski, M., Ortmann, D., Snijders, K., Yiangou, L., De Brito, M. C., … Vallier, L. (2016). Optimized inducible shRNA and CRISPR/Cas9 platforms for in vitro studies of human development using hPSCs. Development (Cambridge) , 143(23), 4405–4418. doi: 10.1242/dev.138081.
- Breckwoldt, K., Letuffe-Brenière, D., Mannhardt, I., Schulze, T., Ulmer, B., Werner, T., … Hansen, A. (2017). Differentiation of cardiomyocytes and generation of human engineered heart tissue. Nature Protocols , 12(6), 1177–1197. doi: 10.1038/nprot.2017.033.
- Brenière-Letuffe, D., Domke-Shibamiya, A., Hansen, A., Eschenhagen, T., Fehse, B., Riecken, K., & Stenzig, J. (2018). Clonal dynamics studied in cultured induced pluripotent stem cells reveal major growth imbalances within a few weeks. Stem Cell Research & Therapy, 9(1), 165. doi: 10.1186/s13287-018-0893-2.
- Butler, J. M. (2010). Fundamentals of forensic DNA typing. San Francisco: Academic Press. doi: 10.1016/C2009-0-01945-X.
- Coecke, S., Balls, M., Bowe, G., Davis, J., Gstraunthaler, G., Hartung, T., … Stokes, W. (2005). Guidance on good cell culture practice: A Report of the Second ECVAM Task Force on good cell culture practice. ATLA Alternatives to Laboratory Animals , 33(3), 261–287. doi: 10.1177/026119290503300313.
- Creasey, A. A., Stacey, G., Bharti, K., Sato, Y., & Lubiniecki, A. (2019). A strategic road map to filing a Biologics License Application for a pluripotent stem cell derived therapeutic product. Biologicals , 59, 68–71. doi: 10.1016/j.biologicals.2019.03.007.
- Crook, J. M., Hei, D., & Stacey, G. (2010). The international stem cell banking initiative (ISCBI): Raising standards to bank on. In Vitro Cellular and Developmental Biology - Animal , 46(3–4), 169–172. doi: 10.1007/s11626-010-9301-7.
- De Sousa, P. A., Steeg, R., Wachter, E., Bruce, K., King, J., Hoeve, M., … Allsopp, T. E. (2017). Rapid establishment of the European Bank for induced Pluripotent Stem Cells (EBiSC) - the Hot Start experience. Stem Cell Research , 20, 105–114. doi: 10.1016/j.scr.2017.03.002.
- El-Mounayri, O., Mihic, A., Shikatani, E. A., Gagliardi, M., Steinbach, S. K., Dubois, N., … Husain, M. (2013). Serum-free differentiation of functional human coronary-like vascular smooth muscle cells from embryonic stem cells. Cardiovascular Research , 98(1), 125–135. doi: 10.1093/cvr/cvs357.
- Fergus, J., Quintanilla, R., & Lakshmipathy, U. (2015). Characterizing pluripotent stem cells using the TaqMan® hPSC scorecardTM panel. Methods in Molecular Biology , 1307, 25–37. doi: 10.1007/7651_2014_109.
- Fleming, D. O., & Hunt, D. L. (2006). Biological safety: Principles and practices ( 4th ed.). Washington, DC: ASM Press.
- Frank, S., Zhang, M., Schöler, H. R., & Greber, B. (2012). Small molecule-assisted, line-independent maintenance of human pluripotent stem cells in defined conditions. PLoS ONE , 7(7), e41958. doi: 10.1371/journal.pone.0041958.
- Henry, M. P., Hawkins, J. R., Boyle, J., & Bridger, J. M. (2019). The genomic health of human pluripotent stem cells: Genomic instability and the consequences on nuclear organization. Frontiers in Genetics , 10(JAN), 623. doi: 10.3389/fgene.2018.00623.
- Hunt, C. J. (2019). Technical considerations in the freezing, low-temperature storage and thawing of stem cells for cellular therapies. Transfusion Medicine and Hemotherapy , 46, 134–149. doi: 10.1159/000497289.
- Kim, J. H., Alderton, A., Crook, J. M., Benvenisty, N., Brandsten, C., Firpo, M., … Stacey, G. N. (2019). A report from a workshop of the international stem cell banking initiative, held in collaboration of global alliance for iPSC therapies and the harvard stem cell institute, Boston, 2017. Stem Cells , 37(9), 1130–1135. doi: 10.1002/stem.3003.
- Kuo, H. H., Gao, X., DeKeyser, J. M., Fetterman, K. A., Pinheiro, E. A., Weddle, C. J., … Burridge, P. W. (2020). Negligible-cost and weekend-free chemically defined human iPSC culture. Stem Cell Reports , 14(2), 256–270. doi: 10.1016/j.stemcr.2019.12.007.
- Kurtz, A., Seltmann, S., Bairoch, A., Bittner, M. S., Bruce, K., Capes-Davis, A., … Xu, R. H. (2018). A standard nomenclature for referencing and authentication of pluripotent stem cells. Stem Cell Reports , 10(1), 1–6. doi: 10.1016/j.stemcr.2017.12.002.
- Kyriakides, O., Halliwell, J. A., & Andrews, P. W. (2018). Acquired genetic and epigenetic variation in human pluripotent stem cells. Advances in Biochemical Engineering/Biotechnology , 163, 187–206. doi: 10.1007/10_2017_22.
- Liu, L. P., & Zheng, Y. W. (2019). Predicting differentiation potential of human pluripotent stem cells: Possibilities and challenges. World Journal of Stem Cells , 11, 375–382. doi: 10.4252/wjsc.v11.i7.375.
- Lomax, G. P., Hull, S. C., Lowenthal, J., Rao, M., & Isasi, R. (2013). The DISCUSS project: Induced pluripotent stem cell lines from previously collected research biospecimens and informed consent: Points to consider. Stem Cells Translational Medicine , 2(10), 727–730. doi: 10.5966/sctm.2013-0099.
- Merkle, F. T., Ghosh, S., Kamitaki, N., Mitchell, J., Avior, Y., Mello, C., … Eggan, K. (2017). Human pluripotent stem cells recurrently acquire and expand dominant negative P53 mutations. Nature , 545(7653), 229–233. doi: 10.1038/nature22312.
- Moralli, D., Yusuf, M., Mandegar, M. A., Khoja, S., Monaco, Z. L., & Volpi, E. V. (2011). An improved technique for chromosomal analysis of human ES and iPS cells. Stem Cell Reviews and Reports , 7(2), 471–477. doi: 10.1007/s12015-010-9224-4.
- Morrison, M., Bell, J., George, C., Harmon, S., Munsie, M., & Kaye, J. (2017). The European General Data Protection Regulation: Challenges and considerations for iPSC researchers and biobanks. Regenerative Medicine , 12(6), 693–703. doi: 10.2217/rme-2017-0068.
- Müller, F.-J. (2012). Assessment of human pluripotent stem cells with PluriTest. In StemBook (Vol. 10, Issues 2008–2012). Cambridge (MA): Harvard Stem Cell Institute. doi: 10.3824/stembook.1.84.1.
- Nikfarjam, L., & Farzaneh, P. (2012). Prevention and detection of mycoplasma contamination in cell culture. Cell Journal , 13, 203–212. doi: 10.22074/cellj.2012.1190.
- O'Shea, O., Steeg, R., Chapman, C., Mackintosh, P., & Stacey, G. N. (2020). Development and implementation of large-scale quality control for the European bank for induced Pluripotent Stem Cells. Stem Cell Research , 45, 101773. doi: 10.1016/j.scr.2020.101773.
- Orzechowski, M., Schochow, M., Kühl, M., & Steger, F. (2020). Donor information in research and drug evaluation with induced pluripotent stem cells (iPSCs). Stem Cell Research and Therapy , 11(1), 126. doi: 10.1186/s13287-020-01644-4.
- Rao, M. S., Pei, Y., Garcia, T. Y., Chew, S., Kasai, T., Hisai, T., … Zeng, X. (2018). Illustrating the potency of current Good Manufacturing Practice–compliant induced pluripotent stem cell lines as a source of multiple cell lineages using standardized protocols. Cytotherapy , 20(6), 861–872. doi: 10.1016/j.jcyt.2018.03.037.
- Rebuzzini, P., Zuccotti, M., Alberto, C., & Garagna, S. (2016). Achilles’ heel of pluripotent stem cells : Genetic, genomic and epigenetic variations during prolonged culture. Cellular and Molecular Life Sciences , 73(13), 2453–2466. doi: 10.1007/s00018-016-2171-8.
- Rehakova, D., Souralova, T., & Koutna, I. (2020). Clinical-grade human pluripotent stem cells for cell therapy: Characterization strategy. International Journal of Molecular Sciences , 21, 2435. doi: 10.3390/ijms21072435.
- Sato, Y., Bando, H., Di Piazza, M., Gowing, G., Herberts, C., Jackman, S., … van der Laan, J. W. (2019). Tumorigenicity assessment of cell therapy products: The need for global consensus and points to consider. Cytotherapy , 21, 1095–1111. doi: 10.1016/j.jcyt.2019.10.001.
- Schaaf, S., Eder, A., Vollert, I., Stöhr, A., Hansen, A., & Eschenhagen, T. (2014). Generation of strip-format fibrin-based engineered heart tissue (EHT). Methods in Molecular Biology , 1181, 121–129. doi: 10.1007/978-1-4939-1047-2_11.
- Seltmann, S., Lekschas, F., Müller, R., Stachelscheid, H., Bittner, M. S., Zhang, W., … Kurtz, A. (2016). hPSCreg-the human pluripotent stem cell registry. Nucleic Acids Research , 44(D1), D757–D763. doi: 10.1093/nar/gkv963.
- Shafa, M., Walsh, T., Panchalingam, K. M., Richardson, T., Menendez, L., Tian, X., … Baghbaderani, B. A. (2020). Long-term stability and differentiation potential of cryopreserved cGMP-compliant human induced pluripotent stem cells. International Journal of Molecular Sciences , 21(1), 108. doi: 10.3390/ijms21010108.
- Stacey, G. N. (2009). Consensus guidance for banking and supply of human embryonic stem cell lines for research purposes: The international stem cell banking initiative. Stem Cell Reviews and Reports , 5(4), 301–314. doi: 10.1007/s12015-009-9085-x.
- Stacey, G. N. (2012). Banking stem cells for research and clinical applications. Progress in Brain Research , 200, 41–58. doi: 10.1016/B978-0-444-59575-1.00003-X.
- Stacey, G. N., Andrews, P. W., Barbaric, I., Boiers, C., Chandra, A., Cossu, G., … Wagey, R. (2019). Stem cell culture conditions and stability: A joint workshop of the PluriMes Consortium and Pluripotent Stem Cell Platform. Regenerative Medicine , 14(3), 243–255. doi: 10.2217/rme-2019-0001.
- Stacey, G. N., Crook, J. M., Hei, D., & Ludwig, T. (2013). Banking human induced pluripotent stem cells: Lessons learned from embryonic stem cells? In Cell Stem Cell , 13, 385–388. doi: 10.1016/j.stem.2013.09.007.
- Steinemann, D., Göhring, G., & Schlegelberger, B. (2013). Genetic instability of modified stem cells—a first step towards malignant transformation? American Journal of Stem Cells , 2, 39–51.
- Sullivan, S., Stacey, G. N., Akazawa, C., Aoyama, N., Baptista, R., Bedford, P., … Song, J. (2018). Quality control guidelines for clinical-grade human induced pluripotent stem cell lines. Regenerative Medicine , 13(7), 859–866. doi: 10.2217/rme-2018-0095.
- The International Stem Cell Initiative (2018). Assessment of established techniques to determine developmental and malignant potential of human pluripotent stem cells. Nature Communications , 9, 1925. doi: 10.1038/s41467-018-04011-3.
- Volpato, V., & Webber, C. (2020). Addressing variability in iPSC-derived models of human disease: Guidelines to promote reproducibility. DMM Disease Models and Mechanisms , 13(1), dmm042317. doi: 10.1242/dmm.042317.
- Walsh, P. S., Metzger, D. A., & Higuchi, R. (1991). Chelex® 100 as a medium for simple extraction of DNA for PCR-based typing from forensic material. BioTechniques , 10(4), 506–513. doi: 10.2144/000114018.
- Weissbein, U., Benvenisty, N., & Ben-David, U. (2014). Quality control: Genome maintenance in pluripotent stem cells. The Journal of Cell Biology , 204(2), 153–163. doi: 10.1083/jcb.201310135.
- World Health Organization. Recommendations for the evaluation of animal cell cultures as substrates for the manufacture of biological medicinal products and for the characterization of cell banks. Proposed replacement of TRS 878, Annex 1, (2010). Retrieved from https://www.who.int/biologicals/Cell_Substrates_clean_version_18_April.pdf.
- Yaffe, M. P., Noggle, S. A., & Solomon, S. L. (2016). Raising the standards of stem cell line quality. Nature Cell Biology , 18, 236–237. doi: 10.1038/ncb3313.
Key References
- Breckwoldt et al. (2017). See above.
Description of cardiac differentiation and generation of engineered heart tissues containing some quality controls mentioned in this protocol.
- Frank et al. (2012). See above.
Description of FTDA-based cell culture FTDA medium for robust long-term maintenance of diverse hPSC lines.
- Andrews et al. (2015). See above.
Description of the requirements for early seed stocks of stem cell lines intended for clinical applications according to the International Stem Cell Banking Initiative (ISCBI); consensus on MCB testing is provided in the supplement.
- Sullivan et al. (2018). See above.
Quality control guidelines for clinical-grade hiPSCs.
- Coecke et al. (2005). See above.
Guidance on Good Cell Culture Practice.
- Kurtz et al. (2018). See above.
Standard nomenclature guideline for hiPSC lines.
- O'Shea et al. (2020). See above.
Recent report of lessons learned from the European Bank for Induced Pluripotent Stem Cells.
- Stacey (2009). See above.
Consensus guidance for cell banking based on knowledge of embryonic stem cell.
- Stacey et al. (2013). See above.
Review of lessons learned from embryonic stem cells.
Internet Resources
European registries and cell banks __
* [hPSCreg.eu](hPSCreg.eu)
Registry and certification of pluripotent stem cell lines (Seltmann et al., 2016). Collaboration with many partners worldwide allows a broad overview about accessible hiPSC lines.
* <https://ebisc.org/>
European Bank for Induced Pluripotent Stem Cells, a not-for-profit iPSC bank. Source for protocols, expert support, QC testing, and hiPSC lines (De Sousa et al., 2017; O'Shea et al., 2020).
International and European societies and consortia __
* <https://www.iscbi.org>
International Stem Cell Banking Initiative providing cell banking knowledge and consensus guidance on embryonic and also hiPSC banking (Crook et al., 2010; Stacey, 2009). Regular meeting reports provide helpful updates: (e.g.: Andrews et al., 2017; Kim et al., 2019)
* <https://coredinates.org/>
Stem Cell COREdinates is a group of core facilities working with hiPSCs sharing their expertise.
* <https://isscr.org>
International Society for Stem Cell Research, released helpful guidelines in 2016.
* <https://gscn.org>
German society for stem cell research.
International and European regulations __
* <https://www.iccbba.org/home>
Provides information on ISBT 128, the standard regarding terminology, identification, coding, and labeling of medical products of human origin, mandatory for cell lines intended for clinical use.
* <https://www.iso.org/>
International Standards: Includes Organization ISO9001:2000, a general quality-management standard for provision of services and products; ISO17025, for laboratory testing and monitoring including the use of cell lines for "batch release" testing of medical products; ISO13485, for diagnostic testing procedures including the use of cells or cell-derived reference materials; and ISO34 Guide for the preparation of reference materials (Stacey, 2009).
* <https://www.who.int/>
The "Recommendations for the evaluation of animal cell cultures as substrates for the manufacture of biological medicinal products and for the characterization of cell banks proposed replacement of TRS 878, Annex 1, 2010", providing recommendations for the characterization of cell banks.
* <https://eur-lex.europa.eu/>
Commission Directive 2006/86/EC implementing Directive 2004/23/EC of the European Parliament and of the Council: Requirements for the coding, processing, preservation, storage, and distribution of human tissues and cells; Commission Directive 2006/17/EC implementing Directive 2004/23/EC: requirements for the donation, procurement, and testing of human tissues and cells.
Citing Literature
Number of times cited according to CrossRef: 10
- Yalin Yildirim, Louisa Degener, Lukas Reuter, Johannes Petersen, Lilian Gabel, Annika Sommer, Christiane Pahrmann, Hermann Reichenspurner, Simon Pecha, Evaluation of cell survival in different 3D‐printed geometric shapes of human iPSC‐derived engineered heart tissue, Artificial Organs, 10.1111/aor.14833, 48 , 11, (1251-1263), (2024).
- Maksymilian Prondzynski, Paul Berkson, Michael A. Trembley, Yashasvi Tharani, Kevin Shani, Raul H. Bortolin, Mason E. Sweat, Joshua Mayourian, Dogacan Yucel, Albert M. Cordoves, Beatrice Gabbin, Cuilan Hou, Nnaemeka J. Anyanwu, Farina Nawar, Justin Cotton, Joseph Milosh, David Walker, Yan Zhang, Fujian Lu, Xujie Liu, Kevin Kit Parker, Vassilios J. Bezzerides, William T. Pu, Efficient and reproducible generation of human iPSC-derived cardiomyocytes and cardiac organoids in stirred suspension systems, Nature Communications, 10.1038/s41467-024-50224-0, 15 , 1, (2024).
- Ina Meiser, Monica Alstrup, Elham Khalesi, Bianca Stephan, Anna M. Speicher, Julia Majer, Chee Keong Kwok, Julia C. Neubauer, Mattias Hansson, Heiko Zimmermann, Application-Oriented Bulk Cryopreservation of Human iPSCs in Cryo Bags Followed by Direct Inoculation in Scalable Suspension Bioreactors for Expansion and Neural Differentiation, Cells, 10.3390/cells12141914, 12 , 14, (1914), (2023).
- Malte Loos, Birgit Klampe, Thomas Schulze, Xiaoke Yin, Konstantinos Theofilatos, Bärbel Maria Ulmer, Carl Schulz, Charlotta S. Behrens, Tessa Diana van Bergen, Eleonora Adami, Henrike Maatz, Michaela Schweizer, Susanne Brodesser, Boris V. Skryabin, Timofey S. Rozhdestvensky, Sara Bodbin, Konstantina Stathopoulou, Torsten Christ, Chris Denning, Norbert Hübner, Manuel Mayr, Friederike Cuello, Thomas Eschenhagen, Arne Hansen, Human model of primary carnitine deficiency cardiomyopathy reveals ferroptosis as a novel mechanism, Stem Cell Reports, 10.1016/j.stemcr.2023.09.002, 18 , 11, (2123-2137), (2023).
- Antonia T. L. Zech, Maksymilian Prondzynski, Sonia R. Singh, Niels Pietsch, Ellen Orthey, Erda Alizoti, Josefine Busch, Alexandra Madsen, Charlotta S. Behrens, Moritz Meyer-Jens, Giulia Mearini, Marc D. Lemoine, Elisabeth Krämer, Diogo Mosqueira, Sanamjeet Virdi, Daniela Indenbirken, Maren Depke, Manuela Gesell Salazar, Uwe Völker, Ingke Braren, William T. Pu, Thomas Eschenhagen, Elke Hammer, Saskia Schlossarek, Lucie Carrier, ACTN2 Mutant Causes Proteopathy in Human iPSC-Derived Cardiomyocytes, Cells, 10.3390/cells11172745, 11 , 17, (2745), (2022).
- Cristine Marie Yde Ohki, Rhiannon V. McNeill, Matthias Nieberler, Franziska Radtke, Sarah Kittel-Schneider, Edna Grünblatt, Promising Developments in the Use of Induced Pluripotent Stem Cells in Research of ADHD, 10.1007/7854_2022_346, (2022).
- Elise Aasebø, Annette K. Brenner, Maria Hernandez-Valladares, Even Birkeland, Håkon Reikvam, Frode Selheim, Frode S. Berven, Øystein Bruserud, Proteomic Characterization of Spontaneous Stress-Induced In Vitro Apoptosis of Human Acute Myeloid Leukemia Cells; Focus on Patient Heterogeneity and Endoplasmic Reticulum Stress, Hemato, 10.3390/hemato2030039, 2 , 3, (607-627), (2021).
- Xuelin He, Li Liu, Baode Chen, Chao Wu, Using Cell Type–Specific Genes to Identify Cell-Type Transitions Between Different in vitro Culture Conditions, Frontiers in Cell and Developmental Biology, 10.3389/fcell.2021.644261, 9 , (2021).
- Nele Warnecke, Bärbel M. Ulmer, Sandra D. Laufer, Aya Shibamiya, Elisabeth Krämer, Christiane Neuber, Sophia Hanke, Charlotta Behrens, Malte Loos, Julia Münch, Jirko Kühnisch, Sabine Klaassen, Thomas Eschenhagen, Monica Patten-Hamel, Lucie Carrier, Giulia Mearini, Generation of bi-allelic MYBPC3 truncating mutant and isogenic control from an iPSC line of a patient with hypertrophic cardiomyopathy, Stem Cell Research, 10.1016/j.scr.2021.102489, 55 , (102489), (2021).
- Daniel Besser, Advancing Stem Cell Technologies and Applications: A Special Collection from the PluriCore Network in the German Stem Cell Network (GSCN), Current Protocols in Stem Cell Biology, 10.1002/cpsc.129, 55 , 1, (2020).