An Efficient Agrobacterium-Mediated Transformation Protocol for Hexaploid and Tetraploid Wheat
Mark A. Smedley, Mark A. Smedley, Sadiye Hayta, Sadiye Hayta, Martha Clarke, Martha Clarke, Wendy A. Harwood, Wendy A. Harwood, Macarena Forner, Macarena Forner
Abstract
Wheat, though a key crop plant with considerable influence on world food security, has nonetheless trailed behind other major cereals in the advancement of gene transformation technology for its improvement. New breeding technologies such as genome editing allow precise DNA manipulation, but their potential is limited by low regeneration efficiencies in tissue culture and the lack of transformable genotypes. We developed, in the hexaploid spring wheat cultivar “Fielder,” a robust, reproducible Agrobacterium tumefaciens –mediated transformation system with transformation efficiencies of up to 33%. The system requires immature embryos as starting material and includes a centrifugation pretreatment before the inoculation with Agrobacterium. This high-throughput, highly efficient, and repeatable transformation system has been used effectively to introduce genes of interest for overexpression, RNA interference, and CRISPR-Cas-based genome editing. With slight modifications reported here, the standard protocol can be applied to the hexaploid wheat “Cadenza” and the tetraploid durum wheat “Kronos” with efficiencies of up to 4% and 10%, respectively. The system has also been employed to assess the developmental gene fusion GRF -GIF with outstanding results. In our hands, this technology combined with our transformation system improved transformation efficiency to 77.5% in Fielder. This combination should help alleviate the genotype dependence of wheat transformation, allowing new genome-editing tools to be used directly in more elite wheat varieties. © 2021 The Authors.
This article was corrected on 20 July 2022. See the end of the full text for details.
Basic Protocol 1 : Growing of donor plants
Basic Protocol 2 : Transformation of Agrobacterium with vector by electroporation
Basic Protocol 3 : Starting material collection, sterilization, and embryo inoculation
Basic Protocol 4 : Selection, regeneration, rooting, and acclimatization of transformants
INTRODUCTION
Unrivalled in its geographic range of cultivation, wheat accounts for ∼20% of the calorific value and ∼25% of the daily protein intake of the world's population (Shewry, 2009). One of the three major cereal crops, after maize (Zea mays) and rice (Oryza sativa ; Shewry, 2009), wheat has more influence on global food security than any other crop (Wingen et al., 2017). Notwithstanding wheat's importance, however, it has until recently trailed behind the other major cereals in regard to the development of genomic tools and resources for its improvement (Adamski et al., 2020; Borrill, Harrington, & Uauy, 2019). Despite recent advances in wheat genomic resources, it remains one of the most challenging major cereals to genetically transform (Hayta et al., 2019). Transgenic approaches are required for gene functional studies, crop improvement, and the delivery of components for new breeding technologies such as genome editing (Borrill et al., 2019).
Having developed a highly reproducible Agrobacterium -mediated transformation system for the hexaploid spring wheat cultivar Fielder, we successfully applied this protocol to the hexaploid Cadenza and the tetraploid durum wheat Kronos with slight modifications. With transformation efficiencies of up to 33% in Fielder, 10% in Kronos, and 4% in Cadenza, this protocol is robust and efficient.
The detailed protocol described here uses immature embryos as the starting material. These are pretreated by centrifugation before being inoculated with Agrobacterium strain AGL1 (Lazo, Stein, & Ludwig, 1991) containing a binary vector that confers hygromycin resistance in planta. The protocol has been used successfully for gene overexpression studies, gene complementation (Connorton et al., 2017), RNA interference (RNAi), and genome editing based on clustered regularly interspaced short palindromic repeats (CRISPR) and CRISPR-associated protein (Cas) in wheat (Rey et al., 2018; Smedley et al., 2021). To date, our protocol has been used in our lab to introduce more than 150 constructs into wheat. The protocol has been transferred to other laboratories worldwide that are now transforming wheat routinely.
A recent and monumental development for wheat transformation by the Dubcovsky laboratory at the University of California, Davis, is a protein fusion consisting of two developmental regulator genes overexpressed on the T-DNA (Debernardi et al., 2020). The wheat transcription factor GROWTH-REGULATING FACTOR 4 (GRF4) and its cofactor GRF-INTERACTING FACTOR 1 (GIF1), when expressed as a protein fusion, substantially improve the regeneration of plant cells growing in vitro. When included and tested with our wheat transformation protocol, this fusion increased transformation efficiency to 77.5% in Fielder (Debernardi et al., 2020). It is envisaged that these developmental regulators will alleviate some of the cultivar dependence of wheat transformation and, in so doing, enable the transformation of more elite wheat cultivars.
This step-by-step guide of our wheat transformation protocol is supplemented with an online video (https://www.youtube.com/watch?v=PTYoTdeS_Wo).
STRATEGIC PLANNING
Collection of immature embryos that are healthy and at the correct developmental stage is a key factor for wheat transformation. The conditions of the controlled environment growth rooms should be adjusted to grow plant material vigorously so that the immature embryos reach the early milk stage, GS73 (Zadoks, Chang, & Konzak, 1974), ∼14 days post anthesis. In addition, particular care needs to be taken to reduce the risk of spreading pathogens. The parent plants should not be sprayed with insecticides or fungicides at any stage of growth, and therefore high levels of plant hygiene must be maintained. As the plant material develops to the right developmental stage over 12-14 weeks, the seeds from the variety to be transformed should be sown every week in small batches. This will ensure a continuous supply of immature embryos at the right developmental stage for transformation.
Basic Protocol 1: GROWING OF DONOR WHEAT PLANTS
This basic protocol describes growing of donor plants used to produce immature embryos used in the transformation system.
NOTE : Sequentially sow small batches of wheat seeds at weekly intervals to ensure a continued supply of immature embryos. Donor plants are grown at 20 ± 1°C day and 15 ± 1°C night temperatures, 70% humidity, with light levels of 600 μmol m−2 s−1 provided by fluorescent tubes and tungsten lighting in controlled environment growth rooms.
Materials
-
John Innes cereal mix (see recipe)
-
Wheat seeds
-
Controlled environment growth room
-
24CT seed tray inserts with 5-cm-square cells
-
Seed tray
-
Small dibber (or pencil)
-
Medium dibber (optional)
-
13-cm-diameter pots
-
Watering can fitted with a fine rose
1.Place a 24CT insert inside a seed tray and fill with John Innes cereal mix.
2.Using a small dibber (or pencil) make a hole ∼1.5-2 cm deep in the center of the cereal mix within each module
3.Place a single wheat seed in each hole and cover with a little more cereal mix (if needed). Tap the tray to level the cereal mix.
4.Using a watering can with a fine rose fitted, gently water the seeds.
5.Place the tray in a controlled environment room set to the conditions listed above (note). Plants should be monitored daily. The compost should be kept moist but not waterlogged.
6.Filling 13-cm-diameter pots with John Innes cereal mix.
7.Make a small hole in the center of the compost using a medium-sized dibber or your finger. The hole should be about the size of the 5-cm modules in which your wheat seedlings germinated.
8.Water the seedlings that are about to be transplanted. Loosen the seedling roots from the module's walls by gently squeezing and release the seedling.
9.Keep the root ball intact as much as possible by supporting the seedling from underneath. Gently plant the seedling into the hole in the fresh compost. Firm the compost around the roots gently.
10.Water using a watering can with a fine rose fitted to it.
11.Grow the plants in a controlled environmental room under the conditions listed above until they produce donor embryos (see Basic Protocol 3 and Time Considerations). Continue to monitor plants daily. The compost should be kept moist but do not overwater.
Basic Protocol 2: TRANSFORMATION OF AGROBACTERIUM WITH VECTOR BY ELECTROPORATION
We recommend using a reporter-gene construct in your initial experiments for optimization of the protocol to your local conditions. The pGoldenGreenGate (pGGG) we describe here contains the hygromycin resistance gene (hpt) and Cat1 intron under the control of a rice Actin1 promoter, and the β-glucuronidase gene (GUS) with two introns (GUS 2Int) under the control of the rice ubiquitin promoter (Addgene cat. no. 165418), along with the helper plasmid pAL155 containing an additional VirG gene (Fig. 1). This combination gave the highest transformation efficiency within our system (Hayta et al., 2019).
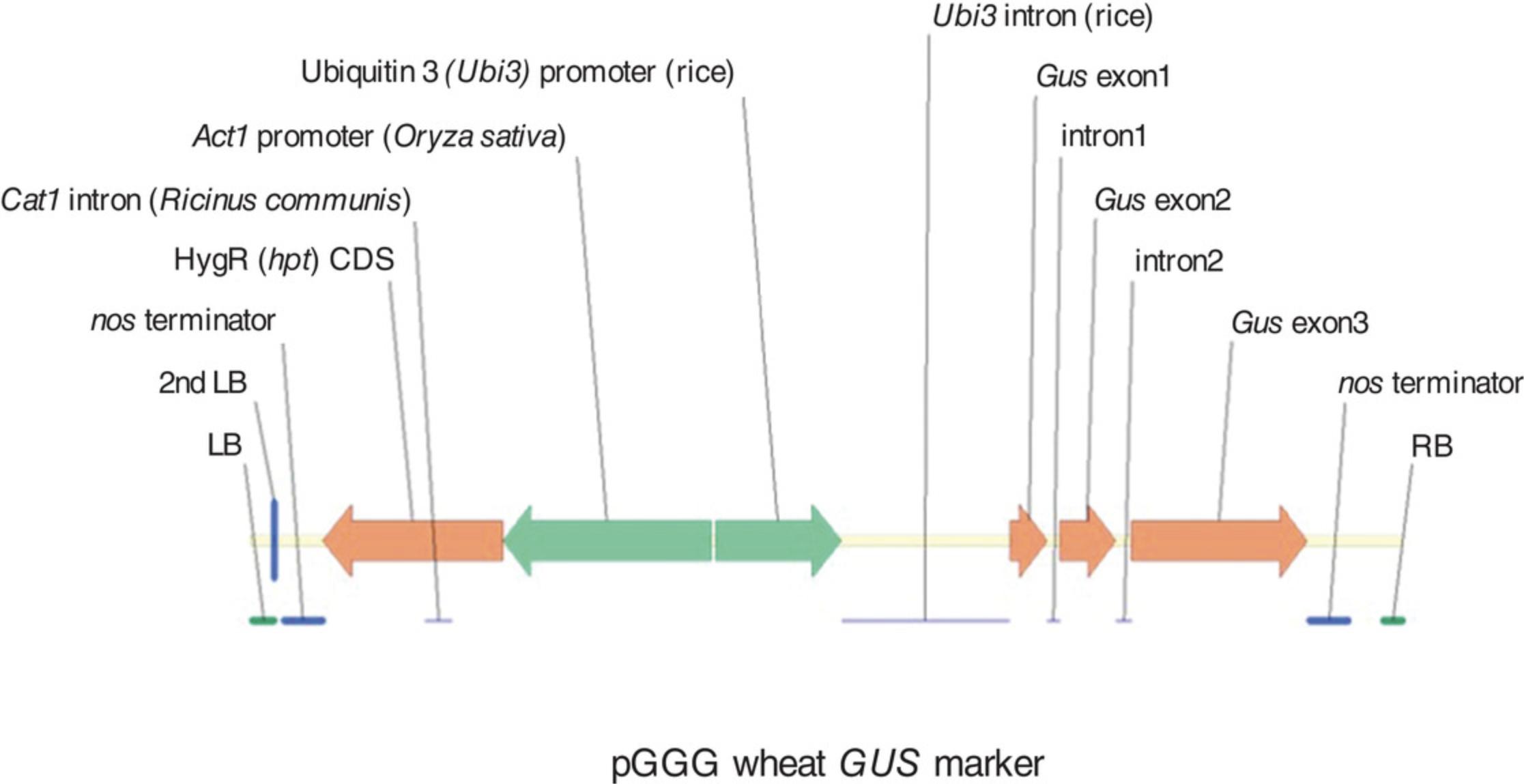
NOTE: The following steps should be carried out in a laminar flow cabinet under aseptic conditions. Ensure cells, cuvette, and DNA are chilled on ice.
Materials
-
Electrocompetent cells of hypervirulent Agrobacterium tumefaciens strain AGL1
-
Binary vector DNA to be used
-
Helper plasmid DNA, if using
-
LB medium, solid and liquid (Bertani, 1951)
-
Antibiotics to select for the Agrobacterium strain and binary vector used (e.g., rifampicin or kanamycin; see step 7)
-
MG/L medium, liquid
-
Wheat inoculation medium (WIM), liquid
-
100 mM acetosyringone (AS; see recipe)
-
Electroporation cuvette with 2-mm separation (Sarstedt Limited 67.742), prechilled on ice
-
Bio-Rad GenePulser or similar electroporation device
-
15-ml Falcon tubes (Life Sciences 430790), sterile
-
Parafilm strips (Parafilm®-PM-992)
-
Electric shaker
-
90-mm single-vent petri plates (Thermo Scientific 101R20)
-
50-ml Falcon tube (Starlab E1450-0800)
-
Aluminum foil
Transformation of Agrobacterium with vector
1.Thaw 50 µl electrocompetent cells of hypervirulent Agrobacterium tumefaciens strain AGL1 on ice. This should take ∼5 min.
2.Add 1 µl (∼100 ng) binary vector DNA (and the same amount of helper plasmid, if using) to the competent cells, gently mix by flicking, and place back on ice.
3.Transfer the cells and DNA mixture to a prechilled cuvette on ice. Gently tap to ensure the contents are at the bottom of the cuvette. Maintain on ice.
4.Electroporate using a Bio-Rad GenePulser, with the settings 2.50 kV, 25 μFD, 400 Ω, and time constant between 8.0 and 9.5 ms.
5.Immediately add 100 µl liquid LB medium to the cuvette to allow cells to recover. Transfer the cells to 500 µl LB medium in a sterile Falcon tube. Wrap the lid of the Falcon tube with Parafilm to secure.
6.Incubate the cells at room temperature, lay the Falcon tube horizontally on a shaker, and gently shake at 100 rpm for 4-6 hr.
7.Spread 20 and 40 µl of the electroporation culture, respectively, onto two plates solid LB medium supplemented with appropriate antibiotics.
8.Incubate the plate upturned at 28°C for ∼48 hr.
Preparation of Agrobacterium for transformation
9.From the inoculated plate, select single colonies of Agrobacterium AGL1, containing the desired vector, and inoculate 10 ml liquid LB medium containing the appropriate antibiotics. Incubate at 28°C in a rotary shaker, shaken at 200 rpm for ∼65 hr.
10.Prepare Agrobacterium standard inoculums for transformation as previously described by Tingay et al. (1997). Mix equal quantities of the Agrobacterium culture and 30% sterile glycerol. Make 400-µl aliquots in 0.5-ml microcentrifuge and freeze at −80°C.
11.The day before wheat transformation, use a single 400-µl standard Agrobacterium inoculum to inoculate 10 ml of MG/L (Garfinkel & Nester, 1980) liquid medium without antibiotics and incubate at 28°C in a rotary shaker, shaken at 200 rpm overnight (∼16 hr).
12.On the day of transformation, pellet the bacteria by centrifugation in a 50-ml Falcon tube at 3100 rpm for 10 min at 24°C. Discard the supernatant, and resuspend the cells gently in 10 ml wheat inoculation medium (WIM) to an optical density of 0.5 OD (600 nm). Then, add 100 mM acetosyringone (AS) to 100 µM final concentration.
13.Incubate the culture for 4-6 hr at room temperature with gentle agitation (80 rpm) in the dark (wrap Falcon tube with aluminum foil) before proceeding to Basic Protocol 3.
Basic Protocol 3: STARTING MATERIAL COLLECTION, STERILIZATION, AND EMBRYO INOCULATION
This protocol describes the collection of starting material at the correct stage, their sterilization, and then the inoculation of immature embryos.
Materials
-
Donor wheat plants with spikes (Basic Protocol 1)
-
70% (v/v) ethanol
-
Distilled water, sterile
-
10% (v/v) sodium hypochlorite (Fluka 71696)
-
Wheat inoculation medium (WIM; see recipe)
-
Silwet L-77
-
Wheat co-cultivation medium plates (see recipe)
-
150-ml Sterilin jar
-
Fine-point forceps
-
1.7-ml microcentrifuge tubes
-
90-mm-diameter single-vent petri plates (Thermo Scientific No 101R20)
-
50-mm-diameter petri plate, sterile
-
Scissors
-
Micropore tape
Collection and sterilization of immature seeds
1.Collect the wheat spikes ∼14 days post anthesis, when the immature embryos are 1-1.5 mm in diameter and at the early milk stage GS73 (Fig. 2A).
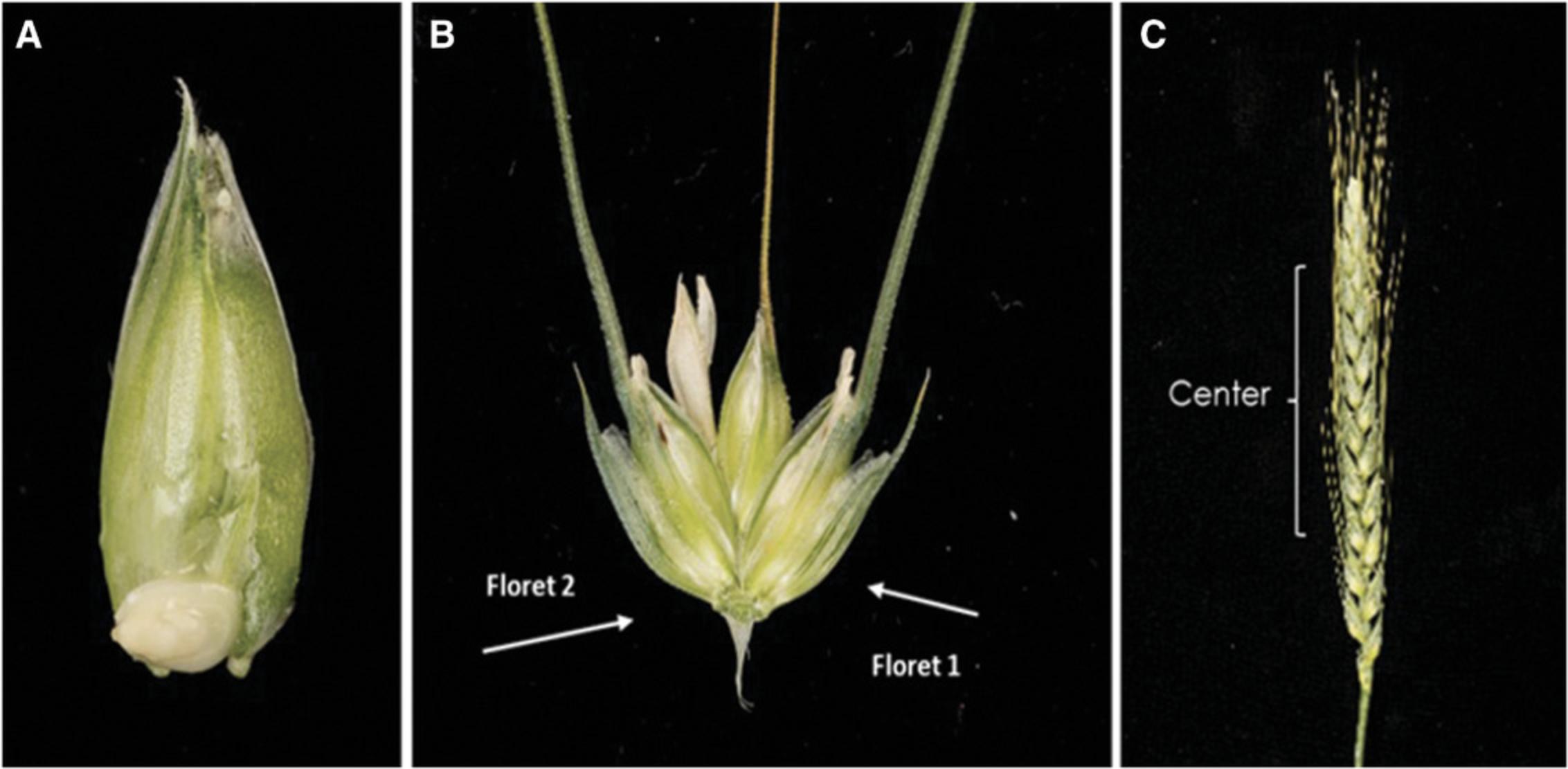
2.Use kernels from florets 1 and 2 (Fig. 2B) on central spikelet (Fig. 2C) for transformation. Cut off the awns from the ears ∼3-5 mm from the grain.
3.Separate the immature grains from the ear and place in a 150-ml Sterilin jar.
4.Sterilize the grains under aseptic conditions (e.g., laminar flow cabinet) using 70% (v/v) ethanol for 1 min. Rinse once with sterile distilled water and then place in 10 ml 10% (v/v) sodium hypochlorite and let stand 7 min. Then wash the grains three times with sterile distilled water.
Isolation of immature embryos, inoculation with Agrobacterium, and co-cultivation
5.Isolate the embryos (Fig. 3A) from the immature grains using fine forceps.
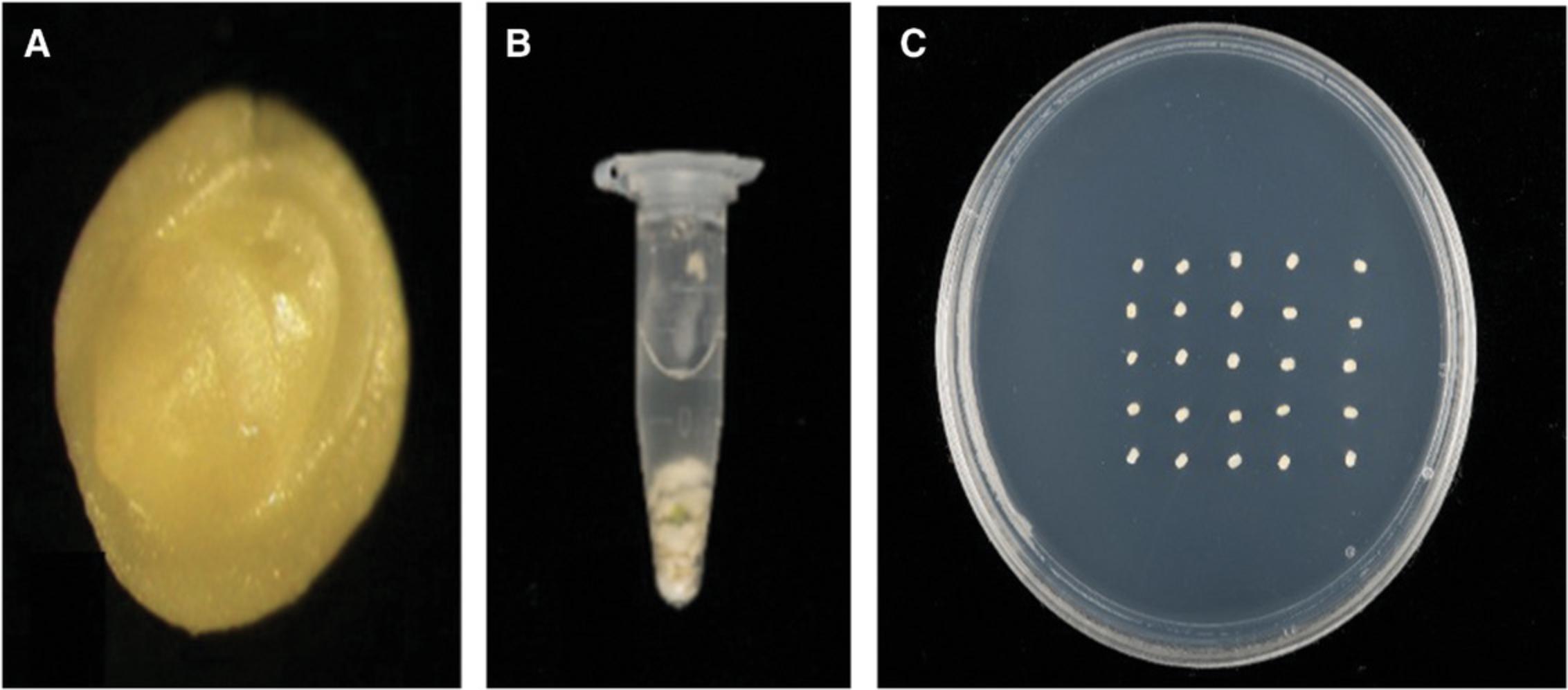
6.Transfer embryos to 1.7-ml microcentrifuge tubes containing 1 ml WIM with 0.05% Silwet L-77, placing ∼100 embryos in each tube (Fig. 3B).
7.After isolating all the embryos, remove the WIM and add fresh WIM to the microcentrifuge tube(s). Centrifuge the isolated embryos 10 min at 14,000 rpm, 4°C (Ishida, Tsunashima, Hiei, & Komari, 2015).
8.Remove WIM with a pipet, add 1 ml Agrobacterium solution, and invert the tubes repeatedly for 30 s. Incubate at room temperature for at least 20 min.
9.After the incubation period, pour the Agrobacterium suspension with the embryos into an empty sterile 50-mm-diameter petri plate and then remove the suspension with a pipet.
10.Transfer 25 embryos, scutellum side up, to a fresh plate(s) of wheat co-cultivation medium in 90-mm-diameter single-vent petri plates.
11.Seal the petri plates with Micropore tape and incubated at 24 ± 1°C in the dark for 3 days of co-cultivation (Fig. 3C).
Basic Protocol 4: SELECTION, REGENERATION, ROOTING, AND ACCLIMATIZATION OF TRANSFORMANTS
This protocol describes the resting period immediately after transformation, the selection process, plantlet regeneration and rooting, and finally acclimation from in vitro culture to soil. The resting period is a 5-day period following co-cultivation in which the Agrobacterium is removed by using timentin; however, the plant material is not subjected to selection pressure. This resting period allows the material to recover from the Agrobacterium infection and initiates callus induction. The selection process that follows puts the calli produced from the immature embryos under selection pressure using the antibiotic hygromycin. Cells that contain the T-DNA and are therefore resistant to hygromycin can proliferate on medium containing hygromycin. The selection pressure continues through the remaining in vitro steps; however, it is these first two phases that are referred to as Selection 1 and 2.During these two selection stages it is important that subcultures are done at the scheduled time, as delay may result in the growth of latent Agrobacterium , causing overgrowth and browning of callus, and/or the reduced stringency of selection may result in the growth of “escapes”, i.e., non-transformed plants.
Materials
-
Inoculated wheat embryos (Basic Protocol 3)
-
Wheat callus induction medium (WCI; see recipe), solid and liquid
-
Wheat regeneration medium (WRM; see recipe), solid
-
Extract-N-Amp™ Plant Tissue PCR Kits (Sigma XNAP-1KT)
-
REDExtract-N-Amp PCR Reaction Mix (Sigma XNAS)
-
Primer pair HygF 5′-AGGCTCTCGATGAGCTGATGCTTT-3′ and HygR 5′-AGCTGCATCATCGAAATTGCCGTC-3′
-
GUS solution (see recipe)
-
90-mm-diameter petri plate (Thermo Scientific No 101R20)
-
Deep petri dishes (tissue culture dish, 90 mm diameter × 20 mm, Falcon 353003)
-
De Wit culture tubes (Duchefa W 1607)
-
De Wit tray (Duchefa T1608)
-
Clear plastic propagator lid
-
Micropore tape
-
Additional reagents and equipment for agarose gel electrophoresis (Voytas, 2000)
Resting period, callus induction, and selection of transformed material
1.After 3 days of co-cultivation, excise the embryogenic axes from the embryos using forceps (Fig. 4A).
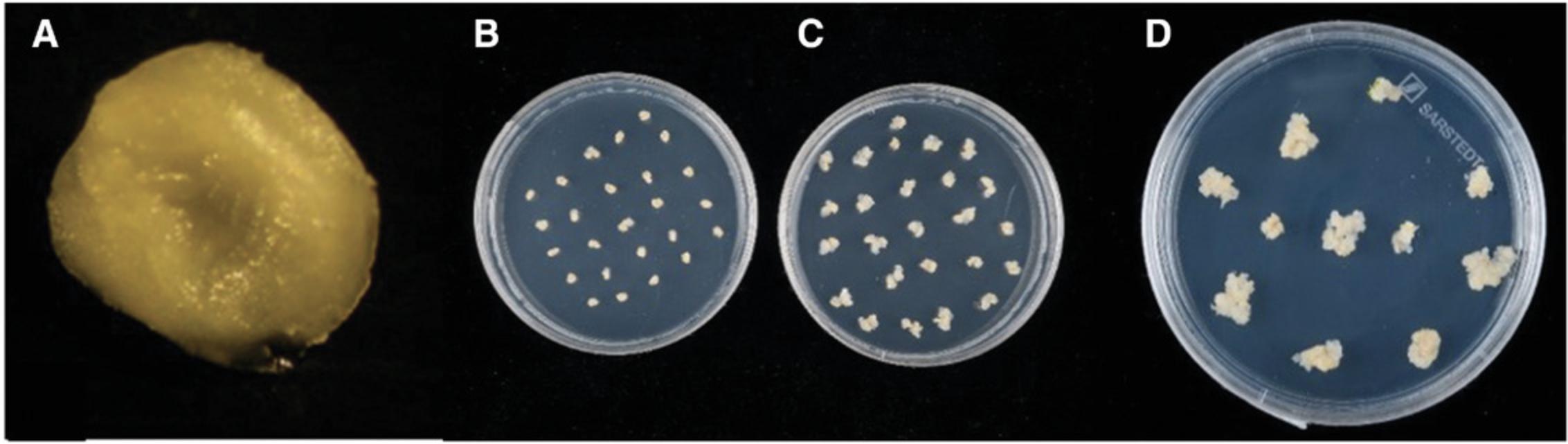
2.Transfer the embryos to fresh wheat callus induction medium plates (WCI) and incubate at 24 ± 1°C in the dark for 5 days.
3.Selection 1 : After the 5-day resting period, transfer the embryos, scutellum side up, to fresh WCI plates as described above with the addition of 15 mg/liter hygromycin (10 mg/liter for Cadenza; Fig. 4B), and incubate at 24 ± 1°C in the dark for 2 weeks (Fig. 4C).
4.Selection 2 : After 2 weeks, split the calli at the into clumps of ∼4 mm2. Callus pieces derived from a single embryo should be labelled to keep track of their origin. Transfer the calli to fresh selection plates (WCI plates) as above, but with 30 mg/liter hygromycin (15 mg/liter for Cadenza), reducing the number of calli per plate by approximately half. Incubate at 24 ± 1°C in the dark for 2 weeks (Fig. 4D).
5.After 2 weeks, transfer the calli to a lit culture room under fluorescent lights (100 μmol/m2/s) at 24 ± 1°C with a 16-hr photoperiod and covered with a single layer of paper towel for a further week.
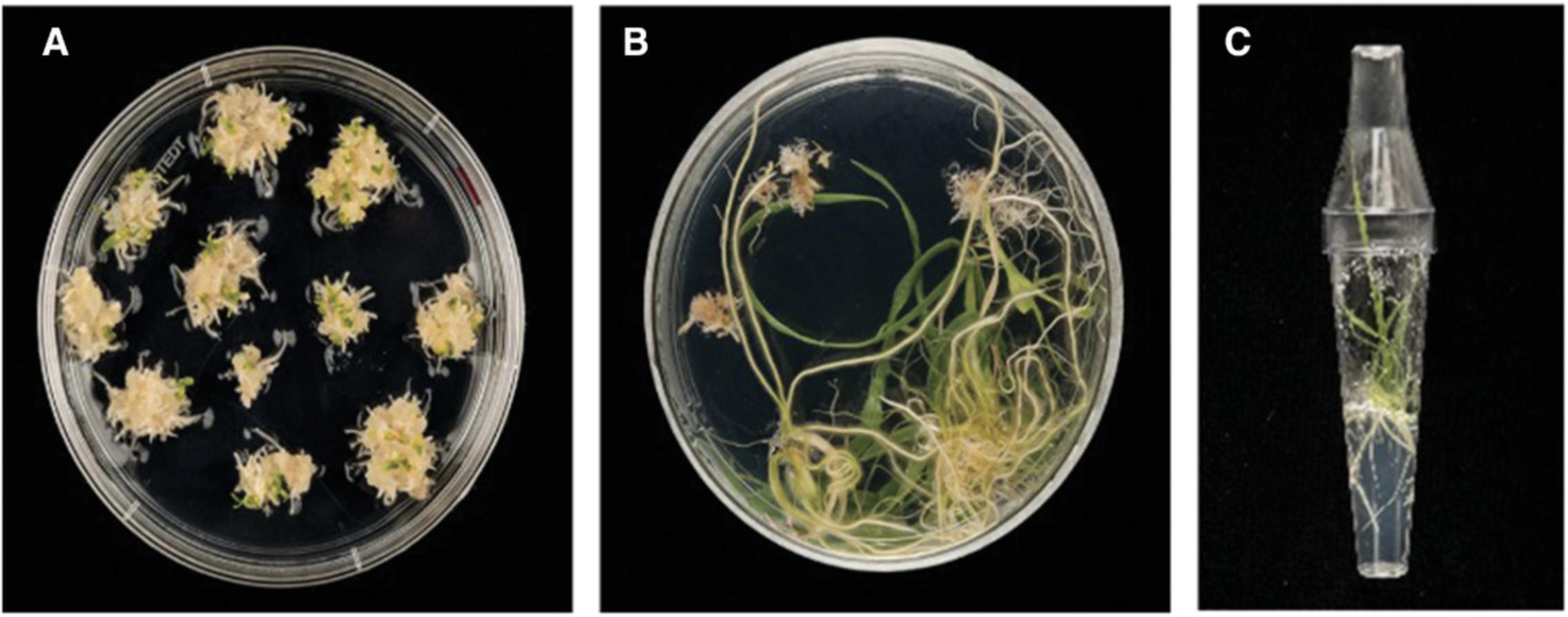
Regeneration of transgenic plants
6.After the 3 weeks on the selection 2 plates, transfer the calli a final time to wheat regeneration medium (WRM) in deep petri dishes (90 mm diameter × 20 mm). Remove the tissue paper covering the plates. Culture calli under fluorescent lights (100 μmol/m2/s) at 24 ± 1°C with a 16-hr photoperiod.
7.Transfer regenerated shoots that are 1-2 cm in length with visible roots (Fig. 5B) to De Wit culture tubes containing 8 ml WCI without growth regulators and supplemented with 15 mg/liter hygromycin.
Acclimatization
8.After ∼10 days, gently remove regenerated plantlets with strong root systems from the tubes using long forceps. Wash the roots with cool running water to remove any remaining tissue culture medium.
9.Plant the plants in cereal mix in 24CT trays and cover with a clear plastic propagator lid. The plants should remain covered with the propagator lid for ∼1 week to maintain high humidity around them while they become established in soil.
10.Once the plants are well established in the soil, leaf samples can be collected for further analysis to confirm the presence of the introduced genes.
Screening
Successful transformation should be confirmed by PCR amplification of the hygromycin resistance gene and/or copy number analysis performed by quantitative PCR.
DNA extraction
11.Harvest 0.5- to 0.7-cm leaf samples into PCR tubes, and extract DNA with Extract-N-Amp™ Plant Tissue PCR Kits following the manufacturer's instructions.
HygR (hpt) polymerase chain reaction (PCR)
12.Amplify the 335-bp amplicon of the hygromycin resistance gene (hpt) by PCR using the primer pair HygF 5′-AGGCTCTCGATGAGCTGATGCTTT-3′, HygR 5′-AGCTGCATCATCGAAATTGCCGTC-3′, along with REDExtract-N-Amp PCR Reaction Mix (cat. no. XNAS), in a 20-µl volume per reaction. Each reaction should comprise 10 µl 2× PCR Reaction Mix (REDExtract-N-Amp), 4 µl DNA extract (∼50 ng DNA), 1 µl (10 mM) of each primer (Hyg F and Hyg R), and 4 µl sterile laboratory-grade water, in 20 µl total volume.
13.Perform PCR in a thermal cycler under the following conditions:
Initial step: | 3 min | 95°C | |
34 cycles: | 30 s | 95°C | (denaturation) |
30 s | 58°C | (annealing) | |
1 min | 72°C | (extension) | |
Final extension: | 7 min | 72°C | |
Final hold: | - | 10°C. |
14.Resolve the PCR products by gel electrophoresis on a 1% agarose gel containing 0.1 μg/ml ethidium bromide.
GUS histochemical assay
NOTE : We suggest carrying out initial experiments with a control vector expressing a reporter gene such as GUS to assess and optimize the system for your local conditions. Using a reporter construct will allow you to follow the transformation process through the different stages.
15.Immerse the plant material in GUS assay substrate at 37°C under dark conditions overnight (∼ 16 hr).
16.Remove the GUS solution, and for green plant material, immerse in 70% ethanol to remove chlorophyll. Leave all green samples in 70% ethanol to remove chlorophyll and other plant pigments prior to visualization and photography.
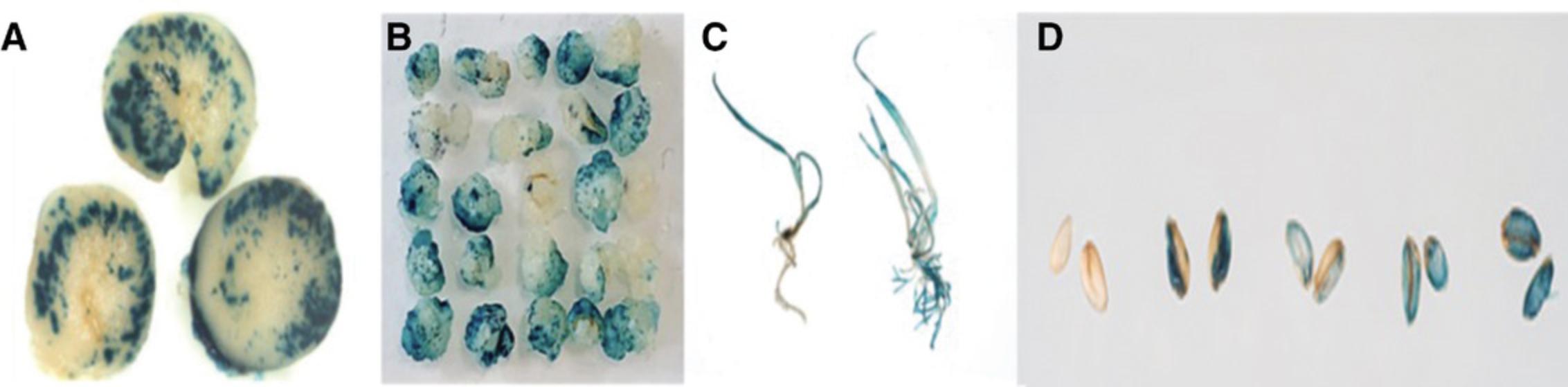
REAGENTS AND SOLUTIONS
NOTE: Use ultrapure water in all recipes unless otherwise noted.
John Innes cereal mix
- 40% (v/v) medium-grade peat
- 40% (v/v) sterilized soil
- 20% (v/v) horticultural grit
- 1.3 kg/m3 PG Mix 14-16-18 + Te Base Fertilizer
- 1 kg/m3 Osmocote Mini 16-8-11 2 mg + Te 0.02% B
- Wetting agent (e.g., 500 g/m3 H2Gro®, ICL Specialty Fertilizers, Ipswich, UK)
- 3 kg/m3 Maglime
- 300 g/m³ Exemptor
Bacterial culture media
GUS solution
- 1 mmol/liter 5-bromo-4-chloro-3-indolyl glucuronide (X-gluc)
- 100 mmol/liter sodium phosphate
- 10 mmol/liter Na2EDTA
- 0.1% Triton X-100
- pH 7
LB medium
- 5 g/liter yeast extract (Duchefa Y1333)
- 10 g/liter NaCl
- 10 g/liter tryptone
- 1 g/liter Bactoagar (Difco)
MG/L, pH 7
- 5.0 g/liter tryptone
- 2.5 g/liter yeast
- 100 mg/liter NaCl
- 5 g/liter mannitol
- 1 g/liter glutamic acid
- 250 mg/liter KH2PO4
- 100 mg/liter MgSO4
- 1 μg/liter biotin
Medium additive stocks
Acetosyringone (AS), 100 mM
Dissolve 490 mg AS (Sigma D134406-1G) in 25 ml dimethyl sulfoxide (DMSO). Divide into 1-ml aliquots and store at −20°C.
AgNO3, 5 mM
Dissolve 42.5 mg AgNO3 (Sigma S7276) in a total volume of 50 ml water. Filter sterilize, divide into 1-ml aliquots, and store at −20°C.
CuSO4, 5 mM
Dissolve 125 mg of CuSO4·5H2O (Sigma C3036) in a total volume of 100 ml water. Filter sterilize, divide into 1-ml aliquots, and store at −20°C.
2,4-Dichlorophenoxyacetic acid (2,4-D)
Dissolve 125 mg 2,4-D (Melford D0911) in 50 ml 100% ethanol. Does not require filter sterilization.
Hygromycin, 50 mg/L
Purchase as 1 g hygromycin in 20-ml volume, sterile (Sigma 10843555001). Prepare 1-ml aliquots in microcentrifuge tubes without filter sterilization.
Picloram, 2.2 mg/ml
Dissolve 110 mg picloram (Melford P0914) in a few drops of 1 N NaOH and make up the volume to 50 ml with water.
Timentin, 160 mg/L
Add 12.5 ml distilled water to the bottle of timentin (2 g; Melford T0190.0002). Prepare 1-ml aliquots in microcentrifuge tubes without filter sterilization.
Vitamin stock for callus induction (WCI) medium, 100×
- 100 mg/liter thiamine HCl
- 35 g/liter myoinositol
- 69 g/liter proline
- Filter sterilize and store at 4°C.
Zeatin
Dissolve 25 mg zeatin (Melford Z0917) in a few drops of 1 N NaOH and make up the volume to 50 ml with water.
Plant culture media
Make up all media and stocks using water from an ultra-pure water. All media should be adjusted to pH 5.8 and all reagents should be added slowly under sterile conditions in a laminar flow hood.
Prepare all medium components for solid media except the gelling agent as double concentrates and filter sterilize. Make up the gelling agent separately as a double concentrate (in H2O) and autoclave.
After autoclaving, maintain the 2× gelling agents at 60°C and warm the filter-sterilized 2× medium components to 60°C prior to mixing the two together and pouring the plates. Add the phytohormones and antibiotics as filter-sterilized stocks just before pouring.
All media in petri plates and tubes should be stored at 10°C. Use of media >2 weeks old is not recommended.
Wheat inoculation medium (WIM)
- 0.44 g/liter Murashige and Skoog (MS) medium (Duchefa M0222)
- 10 g/liter glucose (Sigma G70Z1)
- 0.5 g/liter 2-(N -morpholino)ethanesulfonic acid (MES; Sigma M8250)
Wheat callus induction (WCI)
- 4.3 g/liter MS medium (Duchefa M0221)
- 30 g/liter maltose
- 1.0 g/liter casein hydrolysate
- 10 ml 100× vitamin stock (see recipe)
- 2 mg/liter picloram (Sigma P5575)
- 0.5 mg/liter 2,4-D (see recipe)
- 1.25 mg/liter CuSO4·5H2O (see recipe)
- 160 mg/liter timentin
- 5 g/liter agarose (Sigma A6013)
Wheat co-cultivation medium
Prepare WIM supplemented with 100 μM AS (see recipe), 5 µM AgNO3 (see recipe), 1.25 mg/liter CuSO4·5H2O (see recipe), and 8 g/liter agarose (Sigma A6013).
Wheat regeneration medium (WRM)-Fielder
- 4.3 g/liter MS medium (Duchefa M0221)
- 30 g/liter maltose
- 1.0 g/liter casein hydrolysate
- 10 ml vitamin stock (see recipe)
- 1.0 mg/liter zeatin (see recipe)
- 1.25 mg/liter CuSO4·5H2O (see recipe)
- 3.5 g/liter phytagel (Sigma P8169)
- 160 mg/liter timentin (see recipe)
- 20 mg/liter hygromycin (see recipe)
WRM2-Kronos
- 4.3 g/liter MS medium (Duchefa M0221)
- 30 g/liter maltose
- 1.0 g/liter casein hydrolysate
- 10 ml vitamin stock (see recipe)
- 2.5 mg/liter zeatin (see recipe)
- 1.25 mg/liter CuSO4·5H2O (see recipe)
- 5 g/liter agarose (Sigma A6013)
- 160 mg/liter timentin (see recipe)
- 20 mg/liter hygromycin (see recipe)
WRM3-Cadenza
- 4.3 g/liter MS (Duchefa M0221)
- 30 g/liter maltose
- 1.0 g/liter casein hydrolysate
- 10 ml of vitamin stock (see recipe)
- 1.0 mg/liter zeatin (see recipe)
- 1.25 mg/liter CuSO4·5H2O (see recipe)
- 5 g/liter agarose (Sigma A6013)
- 160 mg/liter timentin (see recipe)
- 15 mg/liter hygromycin (see recipe)
COMMENTARY
The first Agrobacterium -mediated transformation of wheat was reported in 1997, just after those of the other major cereals rice and maize (Cheng et al., 1997). Despite wheat's universal importance, transformation efficiencies remained ∼5% for many years. Efficient patented transformation systems were developed by Japan Tobacco Inc. (www.jti.co.jp; Ishida et al., 2015). However, expensive specialist training and/or specialist vectors are required to successfully reproduce this method. This restricts wheat transformation to those universities, institutes, and companies that are able to afford these licensed technologies (Borrill et al., 2019). The protocol reported here is based on the freely available open-access wheat transformation method reported in Hayta et al. (2019). Wheat transformation is genotype dependent, and only a few varieties can currently be transformed. The original Hayta et al. protocol was developed for the wheat cultivar Fielder, which remains the wheat variety most efficiently transformable using this methodology. However, we also report slight modifications to the protocol that enable the transformation of the hexaploid wheat Cadenza and the tetraploid wheat Kronos. New developments in genome-editing technologies provide great opportunities for the manipulation of genes within wheat, but these new technologies are dependent on transformation technology for their delivery (Adamski et al., 2020; Debernardi et al., 2020).
Here, we report our robust, reproducible, and transferrable wheat transformation system that is widely available to the research community. The protocol is supplemented with an online video (see Internet Resources).
Critical Parameters and Troubleshooting
The growth of parent material can dramatically affect transformation efficiency. In our experience, one feature essential for success is the availability of good-quality, heathy parent material grown under controlled environmental conditions. High levels of plant hygiene need to be adhered to, as parent plants should not have pesticides or fungicides applied. The collection of healthy immature embryos at the correct stage is important for good transformation efficiency.
In experiments, vector type and selection cassette affect transformation efficiency; vector backbones have an effect on transformation efficiency and copy number. We recommend use of the pGoldenGreenGate (pGGG) vector with the Os Actin promoter::hpt ::nos terminator for selection and the inclusion of an additional VirG gene on the helper plasmid pAL155 for higher transformation efficiency (Hayta et al., 2019).
We use one plant from each embryo to calculate transformation efficiency. In gene functional studies introducing overexpression vectors or for gene complementation, it is important to have plants from independent transformation events. For genome-editing studies in wheat using CRISPR-Cas technology, we recommend taking all the plants that regenerate within culture, as editing could occur at any time, resulting in many independent editing events from an initial explant.
Time Considerations
Growing parent plants for donor material takes an average of 8-10 weeks from sowing to the collection of immature embryos. The transformation process for isolation and inoculation of embryos to the regeneration of plantlets in vitro that are ready for transfer to soil takes ∼11-12 weeks. Transferred plants then take a further 12-14 weeks to mature to seed harvest.
Acknowledgments
We acknowledge and thank Ruby O'Grady from the communications team and Phil Robinson from the photography team at the John Innes Centre for the transformation video and photos used and referenced in this chapter; Mark Youles of TSL SynBio for supplying Golden Gate components; and JIC Horticultural Services. We acknowledge funding support from the Biotechnology and Biological Sciences Research Council (BBSRC) Institute Strategic Programme GEN (BB/P013511/1) to the John Innes Centre. All or part of some figures here were reproduced from Plant Methods (Hayta et al., 2019; https://doi.org/10.1186/s13007-019-0503-z). Copyright permission has been granted to reproduce complete figures or portions of figures as applicable.
Author Contributions
Sadiye Hayta : Investigation; Project administration; Supervision; writing-original draft. Mark A. Smedley : Investigation; Methodology; Supervision; writing-review & editing. Martha Clarke : Methodology. Macarena Forner : Methodology. Wendy A. Harwood : Project administration; Resources; writing-review & editing.
Conflict of Interest
The authors declare no conflict of interest.
Open Research
Data Availability Statement
Data sharing not applicable–no new data generated.
Literature Cited
- Adamski, N. M., Borrill, P., Brinton, J., Harrington, S. A., Marchal, C., Bentley, A. R., … Uauy, C. (2020). A roadmap for gene functional characterisation in crops with large genomes: Lessons from polyploid wheat. eLife , 9, e55646. doi: 10.7554/eLife.55646.
- Bertani, G. (1951). Studies on lysogenesis. I. The mode of phage liberation by lysogenic Escherichia coli. Journal of Bacteriology , 62(3), 293–300. doi: 10.1128/jb.62.3.293-300.1951.
- Borrill, P., Harrington, S. A., & Uauy, C. (2019). Applying the latest advances in genomics and phenomics for trait discovery in polyploid wheat. The Plant Journal , 97(1), 56–72. doi: 10.1111/tpj.14150.
- Cheng, M., Fry, J. E., Pang, S., Zhou, H., Hironaka, C. M., Duncan, D. R., … Wan, Y. (1997). Genetic transformation of wheat mediated by Agrobacterium tumefaciens. Plant Physiology , 115(3), 971–980. doi: 10.1104/pp.115.3.971.
- Connorton, J. M., Jones, E. R., Rodríguez-Ramiro, I., Fairweather-Tait, S., Uauy, C., & Balk, J. (2017). Wheat vacuolar iron transporter TaVIT2 transports Fe and Mn and is effective for biofortification. Plant Physiology , 174(4), 2434. doi: 10.1104/pp.17.00672.
- Debernardi, J. M., Tricoli, D. M., Ercoli, M. F., Hayta, S., Ronald, P., Palatnik, J. F., & Dubcovsky, J. (2020). A GRF–GIF chimeric protein improves the regeneration efficiency of transgenic plants. Nature Biotechnology , 38, 1274–1279. doi: 10.1038/s41587-020-0703-0.
- Garfinkel, D. J., & Nester, E. W. (1980). Agrobacterium tumefaciens mutants affected in crown gall tumorigenesis and octopine catabolism. Journal of Bacteriology , 144(2), 732–743. doi: 10.1128/JB.144.2.732-743.1980.
- Hayta, S., Smedley, M. A., Demir, S. U., Blundell, R., Hinchliffe, A., Atkinson, N., & Harwood, W. A. (2019). An efficient and reproducible Agrobacterium -mediated transformation method for hexaploid wheat (Triticum aestivum L.). Plant Methods , 15(1), 121. doi: 10.1186/s13007-019-0503-z.
- Hellens, R. P., Edwards, E. A., Leyland, N. R., Bean, S., & Mullineaux, P. M. (2000). pGreen: A versatile and flexible binary Ti vector for Agrobacterium-mediated plant transformation. Plant Molecular Biology , 42(6), 819–832. doi: 10.1023/a:1006496308160.
- Ishida, Y., Tsunashima, M., Hiei, Y., & Komari, T. (2015). Wheat (Triticum aestivum L.) transformation using immature embryos. Methods in Molecular Biology , 1223, 189–198. doi: 10.1007/978-1-4939-1695-5_15.
- Lazo, G. R., Stein, P. A., & Ludwig, R. A. (1991). A DNA transformation-competent Arabidopsis genomic library in Agrobacterium. Bio/Technology , 9(10), 963–967. doi: 10.1038/nbt1091-963.
- Rey, M.-D., Martín, A. C., Smedley, M., Hayta, S., Harwood, W., Shaw, P., & Moore, G. (2018). Magnesium increases homoeologous crossover frequency during meiosis in ZIP4 (Ph1 Gene) mutant wheat-wild relative hybrids. Frontiers in Plant Science , 9, 509–509. doi: 10.3389/fpls.2018.00509.
- Shewry, P. R. (2009). Wheat. Journal of Experimental Botany , 60(6), 1537–1553. doi: 10.1093/jxb/erp058.
- Smedley, M. A., & Harwood, W. A. (2015). GatewayR-compatible plant transformation vectors. Methods in Molecular Biology , 1223, 3–16. doi: 10.1007/978-1-4939-1695-5_1.
- Smedley, M. A., Hayta, S., Clark, M., & Harwood, W. A. (2021). CRISPR-Cas9 based genome editing in wheat. Current Protocols , 1, e65.
- Tingay, S., McElroy, D., Kalla, R., Fieg, S., Wang, M., Thornton, S., & Brettell, R. (1997). Agrobacterium tumefaciens -mediated barley transformation. The Plant Journal , 11(6), 1369–1376. doi: 10.1046/j.1365-313X.1997.11061369.x.
- Voytas, D. (2000). Agarose gel electrophoresis. Current Protocols in Molecular Biology , 51, 2.5A.1–2.5A.9. doi: 10.1002/0471142727.mb0205as51.
- Wingen, L. U., West, C., Leverington-Waite, M., Collier, S., Orford, S., Goram, R., … Griffiths, S. (2017). Wheat landrace genome diversity. Genetics , 205(4), 1657–1676. doi: 10.1534/genetics.116.194688.
- Zadoks, J. C., Chang, T. T., & Konzak, C. F. (1974). A decimal code for the growth stages of cereals. Weed Research , 14(6), 415–421. doi: 10.1111/j.1365-3180.1974.tb01084.x.
Key Reference
- Hayta, S., Smedley, M. A., Demir, S. U. et al. (2019). An efficient and reproducible Agrobacterium -mediated transformation method for hexaploid wheat (Triticum aestivum L.). Plant Methods , 15, 121. doi: 10.1186/s13007-019-0503-z.
Internet Resources
Online video of the procedure described in this article.
Corrections
In this publication, author's conflict of interest and data availability statement have been added.
The current version online now includes this information and may be considered the authoritative version of record.
Citing Literature
Number of times cited according to CrossRef: 32
- Muhammad Jawad Akbar Awan, Imran Amin, Awais Rasheed, Nasir A. Saeed, Shahid Mansoor, Knockout mutation in TaD27 enhances number of productive tillers in hexaploid wheat, Frontiers in Genome Editing, 10.3389/fgeed.2024.1455761, 6 , (2024).
- Tom Lawrenson, Martha Clarke, Rachel Kirby, Macarena Forner, Burkhard Steuernagel, James K. M. Brown, Wendy Harwood, An optimised CRISPR Cas9 and Cas12a mutagenesis toolkit for Barley and Wheat, Plant Methods, 10.1186/s13007-024-01234-y, 20 , 1, (2024).
- Francisco M. Ayala, Itzell Eurídice Hernández-Sánchez, Monika Chodasiewicz, Brande B.H. Wulff, Radim Svačina, Engineering a One Health Super Wheat, Annual Review of Phytopathology, 10.1146/annurev-phyto-121423-042128, (2024).
- Md Reyazul Islam, Marina Youngblood, Hye-In Kim, Ivonne González-Gamboa, Andrea Gabriela Monroy-Borrego, Adam A. Caparco, Gregory V. Lowry, Nicole F. Steinmetz, Juan Pablo Giraldo, DNA Delivery by Virus-Like Nanocarriers in Plant Cells, Nano Letters, 10.1021/acs.nanolett.3c04735, 24 , 26, (7833-7842), (2024).
- Adam Gauley, Marianna Pasquariello, Guilherme V. Yoshikawa, Abdul Kader Alabdullah, Sadiye Hayta, Mark A. Smedley, Laura E. Dixon, Scott A. Boden, Photoperiod-1 regulates the wheat inflorescence transcriptome to influence spikelet architecture and flowering time, Current Biology, 10.1016/j.cub.2024.04.029, 34 , 11, (2330-2343.e4), (2024).
- Weiwei Mao, Xiaobo Wang, Yongming Chen, Yongfa Wang, Liang Ma, Xiaoming Xie, Xiaojia Wu, Jin Xu, Yuqi Zhang, Yue Zhao, Xuejun Tian, Weilong Guo, Zhaorong Hu, Mingming Xin, Yingyin Yao, Zhongfu Ni, Qixin Sun, Huiru Peng, Map-based cloning and characterization reveal that an R2R3 MYB gene confers red glume in wheat, The Crop Journal, 10.1016/j.cj.2024.03.002, (2024).
- Muhammet Çağrı Oğuz, Ezgi Oğuz, Mustafa Güler, Seed priming with essential oils for sustainable wheat agriculture in semi-arid region, PeerJ, 10.7717/peerj.15126, 11 , (e15126), (2023).
- Diana Lucia Zuluaga, Emanuela Blanco, Giacomo Mangini, Gabriella Sonnante, Pasquale Luca Curci, A Survey of the Transcriptomic Resources in Durum Wheat: Stress Responses, Data Integration and Exploitation, Plants, 10.3390/plants12061267, 12 , 6, (1267), (2023).
- Zelalem Eshetu Bekalu, Michael Panting, Inger Bæksted Holme, Henrik Brinch-Pedersen, Opportunities and Challenges of In Vitro Tissue Culture Systems in the Era of Crop Genome Editing, International Journal of Molecular Sciences, 10.3390/ijms241511920, 24 , 15, (11920), (2023).
- Forrest Wold-McGimsey, Caitlynd Krosch, Rocío Alarcón-Reverte, Karl Ravet, Andrew Katz, John Stromberger, Richard Esten Mason, Stephen Pearce, Multi-target genome editing reduces polyphenol oxidase activity in wheat (Triticum aestivum L.) grains, Frontiers in Plant Science, 10.3389/fpls.2023.1247680, 14 , (2023).
- Tracie N. Draeger, María-Dolores Rey, Sadiye Hayta, Mark Smedley, Azahara C. Martin, Graham Moore, DMC1 stabilizes crossovers at high and low temperatures during wheat meiosis, Frontiers in Plant Science, 10.3389/fpls.2023.1208285, 14 , (2023).
- Tracie N. Draeger, María-Dolores Rey, Sadiye Hayta, Mark Smedley, Abdul Kader Alabdullah, Graham Moore, Azahara C. Martín, ZIP4 is required for normal progression of synapsis and for over 95% of crossovers in wheat meiosis, Frontiers in Plant Science, 10.3389/fpls.2023.1189998, 14 , (2023).
- Louie Cris Lopos, Natalia V. Bykova, Janeen Robinson, Susan Brown, Kerry Ward, Andriy Bilichak, Diversity of transgene integration and gene-editing events in wheat (Triticum aestivum L.) transgenic plants generated using Agrobacterium-mediated transformation, Frontiers in Genome Editing, 10.3389/fgeed.2023.1265103, 5 , (2023).
- Лариса Броннікова, Дослідження характеру акумуляції розподілу вільного проліну в органах рослин за умов норми та стресу, Notes in Current Biology, 10.29038/NCBio.23.1-1, 1 (5), (3-8), (2023).
- Yan Yan, Yue‐Ting Guo, Chao‐Yan Chang, Xiao‐Ming Li, Mei‐Qi Zhang, Ci‐Hang Ding, Dangqun Cui, Congwei Sun, Yan Ren, Meng‐Lu Wang, Chaojie Xie, Zhongfu Ni, Qixin Sun, Feng Chen, Jin‐Ying Gou (缑金营), HSP90.2 modulates 2Q2‐mediated wheat resistance against powdery mildew, Plant, Cell & EnvironmentPlant, Cell & EnvironmentPlant, Cell & Environment, 10.1111/pce.14579, 46 , 6, (1935-1945), (2023).
- Lara Esch, Qi Yang Ngai, J. Elaine Barclay, Rose McNelly, Sadiye Hayta, Mark A. Smedley, Alison M. Smith, David Seung, Increasing amyloplast size in wheat endosperm through mutation of PARC6 affects starch granule morphology, New Phytologist, 10.1111/nph.19118, 240 , 1, (224-241), (2023).
- Xiaona Liang, Xiaomin Bie, Yuliang Qiu, Ke Wang, Zujun Yang, Yuqi Jia, Zhiying Xu, Mei Yu, Lipu Du, Zhishan Lin, Xingguo Ye, Development of powdery mildew resistant derivatives of wheat variety Fielder for use in genetic transformation, The Crop Journal, 10.1016/j.cj.2022.06.012, 11 , 2, (573-583), (2023).
- Shahnam Azizi-Dargahlou, Mahin pouresmaeil, Agrobacterium tumefaciens-Mediated Plant Transformation: A Review, Molecular Biotechnology, 10.1007/s12033-023-00788-x, 66 , 7, (1563-1580), (2023).
- Esma Yigider, Mahmut Sinan Taspinar, Guleray Agar, Advances in bread wheat production through CRISPR/Cas9 technology: a comprehensive review of quality and other aspects, Planta, 10.1007/s00425-023-04199-9, 258 , 3, (2023).
- Bal Krishna Joshi, Hari Kumar Shrestha, Dipendra Kumar Ayer, Plant Breeding Strategies and Methods for Food Security: Review on the Technology, Emerging Solutions in Sustainable Food and Nutrition Security, 10.1007/978-3-031-40908-0_9, (213-254), (2023).
- Eun Ji Park, Jae-Ryeong Sim, Yu-Jeong Yang, Saet Buyl Lee, Beom-Gi Kim, Sewon Kim, Jong-Yeol Lee, Agrobacterium -Mediated Transformation of Wheat , Korean Journal of Breeding Science, 10.9787/KJBS.2022.54.4.358, 54 , 4, (358-368), (2022).
- Manar S. Ibrahim, Saifeldeen M. Ibrahim, Identifying biotic stress-associated molecular markers in wheat using differential gene expression and machine learning techniques, Highlights in BioScience, 10.36462/H.BioSci.202203, (2022).
- Edina Türkösi, László Ivanizs, András Farkas, Eszter Gaál, Klaudia Kruppa, Péter Kovács, Éva Szakács, Kitti Szőke-Pázsi, Mahmoud Said, Petr Cápal, Simon Griffiths, Jaroslav Doležel, István Molnár, Transfer of the ph1b Deletion Chromosome 5B From Chinese Spring Wheat Into a Winter Wheat Line and Induction of Chromosome Rearrangements in Wheat-Aegilops biuncialis Hybrids, Frontiers in Plant Science, 10.3389/fpls.2022.875676, 13 , (2022).
- Yun-Yan Ling, Zhi-Lin Ling, Rui-Lin Zhao, Construction of a heat-resistant strain of Lentinus edodes by fungal Hsp20 protein overexpression and genetic transformation, Frontiers in Microbiology, 10.3389/fmicb.2022.1009885, 13 , (2022).
- T. I. K. Munaweera, N. U. Jayawardana, Rathiverni Rajaratnam, Nipunika Dissanayake, Modern plant biotechnology as a strategy in addressing climate change and attaining food security, Agriculture & Food Security, 10.1186/s40066-022-00369-2, 11 , 1, (2022).
- B. R. Kuluev, E. V. Mikhailova, A. R. Kuluev, A. A. Galimova, E. A. Zaikina, E. K. Khlestkina, Genome Editing in Species of the Tribe Triticeae with the CRISPR/Cas System, Molecular Biology, 10.1134/S0026893322060127, 56 , 6, (885-901), (2022).
- Jilin Chen, Shaoya Li, Yubing He, Jingying Li, Lanqin Xia, An update on precision genome editing by homology-directed repair in plants, Plant Physiology, 10.1093/plphys/kiac037, 188 , 4, (1780-1794), (2022).
- Waqar Afzal Malik, Imran Mahmood, Abdul Razzaq, Maria Afzal, Ghulam Abbas Shah, Asif Iqbal, Muhammad Zain, Allah Ditta, Saeed Ahmed Asad, Ishfaq Ahmad, Naimatullah Mangi, Wuwei Ye, Exploring potential of copper and silver nano particles to establish efficient callogenesis and regeneration system for wheat ( Triticum aestivum L.) , GM Crops & Food, 10.1080/21645698.2021.1917975, 12 , 1, (564-585), (2021).
- Azahara C. Martín, Abdul Kader Alabdullah, Graham Moore, A separation-of-function ZIP4 wheat mutant allows crossover between related chromosomes and is meiotically stable, Scientific Reports, 10.1038/s41598-021-01379-z, 11 , 1, (2021).
- Shaoya Li, Chen Zhang, Jingying Li, Lei Yan, Ning Wang, Lanqin Xia, Present and future prospects for wheat improvement through genome editing and advanced technologies, Plant Communications, 10.1016/j.xplc.2021.100211, 2 , 4, (100211), (2021).
- Jing Liu, Zhaoyan Chen, Zhihui Wang, Zhaoheng Zhang, Xiaoming Xie, Zihao Wang, Lingling Chai, Long Song, Xuejiao Cheng, Man Feng, Xiaobo Wang, Yanhong Liu, Zhaorong Hu, Jiewen Xing, Zhenqi Su, Huiru Peng, Mingming Xin, Yingyin Yao, Weilong Guo, Qixin Sun, Jie Liu, Zhongfu Ni, Ectopic expression of VRT-A2 underlies the origin of Triticum polonicum and Triticum petropavlovskyi with long outer glumes and grains, Molecular Plant, 10.1016/j.molp.2021.05.021, 14 , 9, (1472-1488), (2021).
- Mark A. Smedley, Sadiye Hayta, Martha Clarke, Wendy A. Harwood, CRISPR‐Cas9 Based Genome Editing in Wheat, Current Protocols, 10.1002/cpz1.65, 1 , 3, (2021).