Agrobacterium-mediated Genetic Transformation of Cassava
Rosana Segatto, Rosana Segatto, Tira Jones, Tira Jones, Danielle Stretch, Danielle Stretch, Claire Albin, Claire Albin, Raj Deepika Chauhan, Raj Deepika Chauhan, Nigel J. Taylor, Nigel J. Taylor
Abstract
The storage root crop cassava (Manihot esculenta Crantz) is predicted to remain central to future food and economic security for smallholder farming households and agricultural output in the tropics. Genetic improvement of cassava is required to meet changing farmer and consumer needs, evolving pests and diseases, and challenges presented by climate change. Transgenic and genome editing technologies offer significant potential for introducing desired traits into farmer-preferred varieties and breeding lines, and for studying the biology of this under-investigated crop species. A bottleneck in implementing genetic modification in this species has been access to robust methods for transformation of cassava cultivars and landraces. In this article, we provide a detailed protocol for Agrobacterium -mediated transformation of cassava and regeneration of genetically modified plants. Basic Protocol 1 describes how to establish and micropropagate in vitro cassava plantlets, and Alternate Protocol 1 details how to establish in vitro cultures from field or greenhouse cuttings. Basic Protocol 2 describes all steps necessary for genetic transformation in the model variety 60444, and Alternate Protocol 2 provides details for modifying this method for use with other cultivars. Finally, Basic Protocol 3 describes how to establish plants produced via Basic Protocol 2 and Alternate Protocol 2 in soil in a greenhouse. These methods have proven applications across more than a dozen genotypes and are capable of producing transgenic and gene-edited plants for experimental purposes, for testing under greenhouse and field conditions, and for development of plants suitable for subsequent regulatory approval and product deployment. © 2022 The Authors. Current Protocols published by Wiley Periodicals LLC.
Basic Protocol 1 : Establishment and propagation of in vitro cassava plantlets
Alternate Protocol 1 : Establishment of in vitro plants from field or greenhouse plants
Basic Protocol 2 : Genetic transformation of cassava variety 60444
Alternate Protocol 2 : Genetic transformation of additional cultivars
Basic Protocol 3 : Establishment and growth of plants in the greenhouse
INTRODUCTION
The starchy root crop cassava (Manihot esculenta Crantz) is a major staple for hundreds of millions of people across the world's tropical regions, where it is surpassed only by rice and maize in its importance as a source of dietary carbohydrates. Traditionally grown by smallholder farmers for on-farm consumption and sale in local markets, it is also becoming an important industrial crop, cultivated for its high-quality starch and as a source of biofuel, alcohol, and bioplastics (Wagaba et al., 2020). Cassava has been recognized as drought-tolerant and is therefore predicted to become increasingly important for future food, and economic security, and agricultural output in the tropics (Pushpalatha & Gangadharan, 2020).
Genetic improvement of cassava is required to meet changing farmer and consumer needs, evolving pests and diseases, and challenges presented by climate change, population growth, and urbanization. Conventional breeding in cassava is complicated by its high level of heterozygosity, inbreeding depression, and asynchronous flowering (Liu, Zheng, Ma, Gadidasu, & Zhang, 2011). Transgenic and genome-editing technologies offer important potential for introducing desired traits into farmer-preferred varieties and breeding lines and for studying the biology of this under-investigated crop species. Recovery of transgenic cassava plants was first reported in 1996 (Li, Sautter, Potrykus, & Puonti-Kaerlas, 1996; Schöpke et al., 1996). Multiple publications have subsequently described development and application of transgenic systems to produce plants genetically modified for disease resistance (Gomez et al., 2018; Wagaba et al., 2017), modified starch (Bull et al., 2018), nutritional enhancement (Narayanan et al., 2019), and reduced cyanogenesis (Gomez et al., 2021), among other traits.
The most frequently used protocol for genetic transformation of cassava relies on generation of embryogenic callus as target tissue for integration of transgenes and gene-editing tools via Agrobacterium tumefaciens. Important progress has been made to increase efficiency and expand capacity into cassava genotypes of importance in Africa, Asia, and the Americas (Chauhan, Beyene, & Taylor, 2018; Chavarriaga-Aguirre et al., 2016; Narayanan et al., 2021; Taylor et al., 2012; Utsumi et al., 2022). Nevertheless, production of transgenic cassava remains challenging for many researchers, with the capacity for reliable regeneration of modified plants restricted to only a few laboratories around the world.
We describe here highly detailed methodologies to enable the production of transgenic cassava plants across a range of genotypes. The protocols presented provide details for all stages needed to initiate and maintain cassava plants in vitro , produce totipotent target tissues, perform Agrobacterium -mediated transformation, regenerate robust transgenic plants, and establish them in the greenhouse. Specifically, Basic Protocol 1 describes how to maintain micropropagated plantlets in vitro , while Alternate Protocol 1 details how to establish in vitro cultures from cuttings obtained from field- or greenhouse-grown plants. Basic Protocol 2 describes the steps necessary for genetic transformation in the model variety 60444, while Alternate Protocol 2 provides details for modifying this method for other farmer-preferred cultivars. Finally, Basic Protocol 3 describes how to establish regenerated plants in soil in the greenhouse.
STRATEGIC PLANNING
The protocols described below assume that the researcher has access to basic plant tissue culture equipment and reagents. These should be obtained before commencing work to produce genetically modified cassava plants. Equipment includes laminar flow hoods in which plant materials can be handled axenically, autoclaves for sterilization of plant media, a temperature-controlled growth room with lighting to maintain plant cultures, and an environmentally controlled greenhouse if establishment of whole plants in soil is desired.
To initiate transformation, the user will need viable cultures of A. tumefaciens LBA4404 or a similar strain harboring a plasmid with genes of interest and preferably a visual marker. We recommend the use of green fluorescent protein (GFP) to track transformation success and progress, but alternatives are available and function well (for details, see Background Information).
Only high-quality chemicals from reputable suppliers should be used in order to reliably replicate these protocols. For planning purposes, lists of media with their components and specific uses are provided in Tables 1 and 2 for cassava cultivar 60444 and other cultivars, respectively. Instructions for preparing media are provided in Reagents and Solutions.
Medium | Purpose | Compositiona | Culture duration (days) | Scale |
---|---|---|---|---|
MS2 | Micropropagation | MS basal salts and vitamins | 42-56 | 6-8 plants per plate, 12-20 plates |
OES induction medium | Induction of OES from leaf explants | MS2, 50 μM picloram, 2 μM CuSO4 | 28 | 10 explants per plate, 10- 20 plates |
FEC induction medium | FEC induction and proliferation (3 cycles) | GD2, 50 μM picloram | 21-28 per cycle | 7-10 colonies per plate, 10- 20 plates |
Liquid inoculation medium | Agrobacterium inoculation of FEC | GD2, 200 μM acetosyringone | 30 min | 3-7 samples per construct |
Co-culture medium | FEC co-culture with Agrobacterium | GD2, 50 μM picloram, 200 μM acetosyringone | 2 | 3-7 samples per construct |
Resting medium | Resting phase | GD2, 50 μM picloram, 150 mg/L carbenicillin | 8 | 3-7 samples per construct |
Callus selection medium | Selection of transgenic callus | GD2, 50 μM picloram, 75 mg/L carbenicillin, 25 μM paromomycin | 21 | 4 plates per sample |
Stage 1 regeneration medium | Somatic embryo regeneration from FEC | MS2, 5 μM NAA, 75 mg/L carbenicillin, 45 μM paromomycin | 18-21 | 10 colonies per plate |
Stage 2 regeneration medium | Somatic embryo maturation | MS2, 0.5 μM NAA, 45 μM paromomycin | 18-21 | 4-6 embryos per plate |
Stage 3 regeneration medium (optional)b | Somatic embryo maturation | MS2, 0.05 μM NAA, 45 μM paromomycin | 18-21 | 4-6 embryos per plate |
Germination medium | Germination of somatic embryos | MS2, 2 μM BAP | 18-21 | 4-6 embryos per plate |
MS2 | Plantlet establishment/ rooting | MS basal salts and vitamins | 14-30 | 6-8 plants per plate |
- aAll contain 20 g/L sucrose and 8 g/L Noble agar.
- bSee Basic Protocol 2, step 58.
Medium | Purpose | Compositiona | Culture duration (days) | Scale |
---|---|---|---|---|
MS2 | Micropropagation | MS basal salts and vitamins | 42-56 | 6-8 plants per plate, 12-20 plates |
OES induction medium | Induction of OES from leaf explants | MS2, 50 μM picloram, 2 μM CuSO4 | 28 | 10 explants per plate, 10-20 plates |
FEC induction post-mesh medium | FEC induction | GD2, 50 μM picloram, 250 μM tyrosine | 3-5 | 7-10 colonies per plate, 10-20 plates |
FEC proliferation medium | FEC proliferation (3 cycles) | GD2, 50 μM picloram, 250 μM tyrosine, 50 mg/L moxalactam | 21-28 per cycle | 7-10 colonies per plate, 10- 20 plates |
Liquid inoculation medium | Agrobacterium inoculation of FEC | GD2, 200 μM acetosyringone | 45 min | 3-7 samples per gene construct |
Co-culture medium | FEC co-culture with Agrobacterium | GD2, 50 μM picloram, 200 μM acetosyringone | 4 | 3-7 samples per gene construct |
Resting medium | Resting phase | GD2, 50 μM picloram, 125 mg/L cefotaxime | 10 | 3-7 samples per gene construct |
Callus selection medium | Selection of transgenic callus | GD2, 50 μM picloram, 125 mg/L cefotaxime, 27.5 μM paromomycin | 21 | 4 plates per sample |
Stage 1 regeneration medium | Somatic embryo regeneration from FEC | MS2, 5 μM NAA, 30 mg/L timentin, 45 μM paromomycin | 18-21 | 10 colonies per plate |
Stage 2 regeneration medium | Somatic embryo maturation | MS2, 0.5 μM NAA, 45 μM paromomycin | 18-21 | 4-6 embryos per plate |
Stage 3 regeneration medium (optional) | Somatic embryo maturation | MS2, 0.05 μM NAA, 45 μM paromomycin | 18-21 | 4-6 embryos per plate |
Germination medium | Germination of somatic embryos | MS2, 2 μM BAP | 18-21 | 4-6 embryos per plate |
MS2 | Plantlet establishment/ rooting | MS basal salts and vitamins | 14-30 | 6-8 plants per plate |
- aAll contain 20 g/L sucrose and 8 g/L Noble agar.
If cultures of cassava plants established in vitro are already available, follow Basic Protocol 1 for micropropagation and multiplication, then proceed to Basic Protocol 2 for production of transgenic or gene-edited plants. If cassava plants need to be brought into culture from the greenhouse or field, start with Alternate Protocol 1, then multiply and maintain the in vitro plantlets prior to commencing Basic Protocol 2 or Alternate Protocol 2. Depending on the research goals, the recovered plants can be sent to the greenhouse for evaluation following details provided in Basic Protocol 3. If the researcher is inexperienced in cassava transformation, we recommend the use of the cassava cultivar 60444, as it offers ease of target tissue production, an amenable response to Agrobacterium transgene integration, and high regeneration efficiency.
NOTE : Before beginning work, regulatory approval from the relevant authorities must be in place to handle and produce transgenic bacteria and plants.
Basic Protocol 1: ESTABLISHMENT AND PROPAGATION OF IN VITRO CASSAVA PLANTLETS
Here we describe the steps required to establish in vitro cultures and how to micropropagate and multiply cassava plants. These mother plants generate the leaf explant material used to initiate the embryogenic target tissues for subsequent transgene integration. We provide directions to handle cassava plant material as in vitro plantlets obtained from germplasm collections such as those held by the International Center for Tropical Agriculture (CIAT) and the International Institute of Tropical Agriculture (IITA). Cassava genotypes obtained from germplasm collections are most often transported in glass or plastic test tubes. The steps below describe first how to establish mother plants from such cultures and then how to propagate them.
While growth and multiplication rates vary, this protocol has proven successful for micropropagation of many cassava varieties. Details are provided for establishing and growing mother plants in Petri dishes. Glass jars can also be used, but Petri dishes are preferred because they can be stacked three or four high, allowing large numbers of mother plants to be maintained in a relatively small space within growth chambers. Use of 100 × 25–mm Petri dishes is recommended for the MS2 agar plates to provide plants with maximum headspace for growth, although 100 × 15–mm sterile dishes also work well.
To provide sufficient numbers of explants for production of target tissues and recovery of transgenic plants, 20 Petri dishes carrying 7-8 mother plants each (140-160 in vitro plantlets) should be established. The established plantlets should be subcultured every 6-8 weeks to ensure continued healthy growth and production of shoot materials in optimal condition for induction of embryogenic tissues.
Materials
- In vitro cassava cultures (e.g., from CIAT)
- MS2 agar plates (see recipe)
- Laminar flow hood
- Sterile fine pointed forceps
- Sterile 100 × 15–mm Petri dishes (VWR, 25384-342)
- Miltex carbon steel no. 10 surgical blade (prod. no. 4-110)
- Parafilm or Saran Wrap cut into 5-cm wide strips
- 28°C growth chamber
Establish mother plants from in vitro plantlets
1.Under a laminar flow hood and using sterilized forceps, carefully extract a plantlet from a test tube or other container and place on a sterile Petri dish lid.
2.Using sterilized forceps and scalpel blade, trim away and discard old leaves. Cut the plantlet transversely to generate 2-3 nodes per stem segment.
3.Transfer stem segments to MS2 agar plates, submerging 0.5 cm of the stem into the medium, and establishing 6-8 shoot explants per plate (20 plates per cultivar are ideal).
4.Seal and label plates appropriately and place in a 28°C growth chamber under bright light (90 µMol m−2 s−1) with a 14-16 hr photoperiod.
Micropropagate mother plants
5.Open Petri dishes containing mother plants in the laminar flow hood.
6.Using sterile forceps and a scalpel blade, excise apical stem segments 2-3 cm in length. Trim away lower leaves and petioles from the stem and discard, leaving the apical meristem and the first mature leaf at the top of the micro cutting.
7.Place the cutting vertically into a new MS2 agar plate, submerging 0.5 cm of the stem into the medium, and placing 6-8 micro cuttings evenly spaced in each dish.
8.Seal and label plates appropriately and culture as above.
Alternate Protocol 1: ESTABLISHMENT OF IN VITRO PLANTS FROM FIELD OR GREENHOUSE PLANTS
This protocol describes how to initiate sterile cassava in vitro if the researcher does not have access to previously established cultures of a desired genotype. Specifically, it provides a guide for surface sterilization and establishment of in vitro plantlets from greenhouse- or field-grown cassava plants. Only disease-free plants should be used to initiate cultures of a given variety. If stem cuttings are collected from the field, it is recommended that diagnostics be performed before proceeding in order to detect the presence of diseases such as cassava mosaic disease (CMD), cassava brown streak disease (CBSD), cassava bacterial blight (CBB), and cassava frogskin disease, as appropriate for the geographies where collection took place.
Materials
- Field- or greenhouse-grown cassava plants
- 15% (v/v) bleach (sodium hypochlorite, 8.25%) in sterile diH2O or reverse-osmosis (RO) water
- Tween 20
- Sterile RO water
- MS2 Gelzan plates (see recipe)
- MS2 agar plates (see recipe)
- Straight single-edge razor blade
- 250- or 500-ml Erlenmeyer flasks
- Aluminum foil
- Rotary shaker (optional)
- Laminar flow hood
- 100 × 15–mm sterile Petri dishes (VWR, 25384-342)
- Waste bucket for bleach rinses
- Fine pointed forceps
- Miltex carbon steel no. 10 surgical blade (prod. no. 4-110)
- Parafilm or Saran Wrap cut into 5-cm-wide strips
- 28°C growth chamber
NOTE : As in Basic Protocol 1, the use of 100 × 25–mm Petri dishes is recommended for MS2 Gelzan and MS2 agar plates to allow unrestricted growth of new shoots and mother plants.
Cut and surface sterilize stem nodes
1.Cut upper, green-colored stem material from field- or greenhouse-grown cassava plants.
2.Using a straight single-edge razor blade, cut off and discard all leaves and petioles to within 0.5 cm of the axillary buds.
3.Cut individual nodes from the stem, leaving ∼1.0 cm of stem above and below the axillary bud.
4.Place a maximum of 25 nodes in a 500-ml flask (15 nodes for a 250-ml flask; Fig. 1A) and cover with aluminum foil.
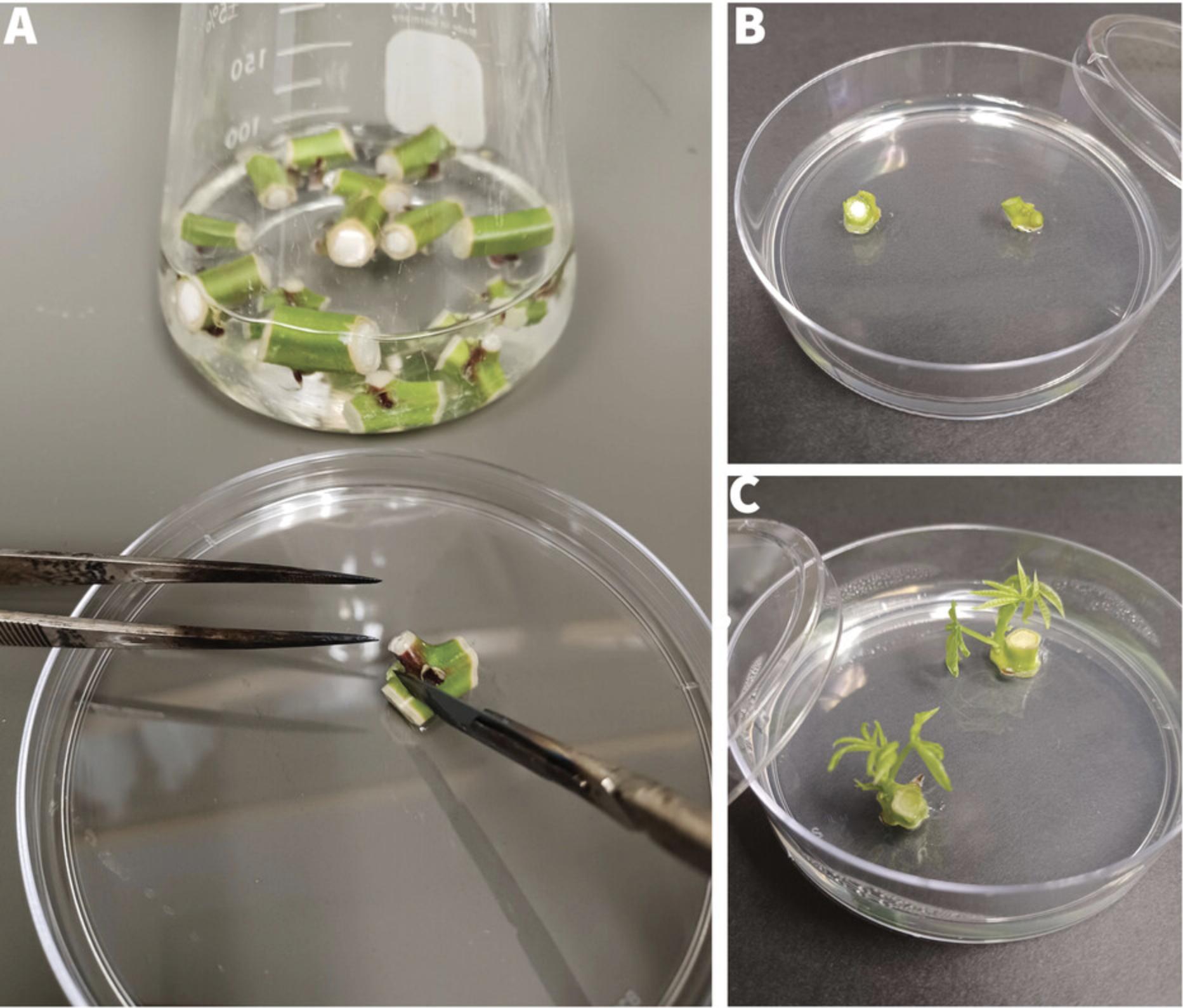
5.Pour 100 ml of 15% bleach into each flask containing cut nodal segments and add two drops of Tween 20. Cover the tops tightly with foil and swirl.
6.Shake for 30 min at 150 rpm on a rotary shaker at room temperature, or swirl flasks by hand in a vigorous manner every 2 min for 30-40 min.
7.Transfer flasks to a laminar flow hood. Remove foil and invert a Petri dish on the top of the flask. Tilt the flask over a large beaker to let the bleach solution pour out, using the Petri dish to retain nodes within the flask.
8.To stop the sterilization process, add 150 ml sterile water to each flask, swirl, and drain the liquid into a waste bucket.
9.Rinse at least five more times with fresh sterile water until nodes are thoroughly rinsed and a bleach smell is no longer present.
10.Drain liquid and transfer nodes to new sterile Petri dish lids (one per flask).
Grow shoots and establish mother plants
11.Under the laminar flow hood, use sterile forceps and scalpel blade to trim bleached tissues away from each nodal segment to expose green healthy tissue above and below the node, taking care not to damage the axillary bud (Fig. 1A).
12.Transfer trimmed nodes to an MS2 Gelzan plate, placing 2-3 nodes per dish, with the axillary buds facing up (Fig. 1B). Seal with Parafilm or Saran Wrap, label appropriately, and place in a 28°C growth chamber under bright light (90 µMol m−2 s−1) with a light duration of 14-16 hr.
13.Observe plates every two days and allow shoots to grow to 2-3 cm in length. Discard if bacterial or fungal growth is observed.
14.Use sterile forceps and scalpel blade to cut new shoot growth away from the original nodes. Insert new stems 0.5 cm deep into MS2 agar plates, placing 6-8 micro cuttings per dish.
15.Culture new micro cuttings to generate roots and establish mother plants.
Basic Protocol 2: GENETIC TRANSFORMATION OF CASSAVA VARIETY 60444
Here we describe steps required to produce transgenic plants in cassava variety 60444, a variety with high transformation efficiency. The process starts with production of organized embryogenic structures (OES) induced from immature leaf explants from in vitro plantlets produced in Basic Protocol 1.To begin production of target tissues, approximately 20 plates of 6- to 8-week-old mother plants should be available. After OES formation, friable embryogenic callus (FEC) tissues are produced in three cycles of 21 days. FEC tissues are amenable to transformation by Agrobacterium and subsequent selection and regeneration of somatic embryos. Finally, the latter are germinated to produce plantlets. The total time required 3-3.5 months from the time of inoculation with Agrobacterium to obtain transgenic plantlets.
Details are provided for use of A. tumefaciens LBA4404 carrying pCAMBIA2300 - based gene constructs. pCAMBIA2300 has an npt I gene for bacterial selection and an npt II gene for plant selection. Table 1 lists the appropriate media for each step of the procedure, along with media composition, culture duration, and scale.
Materials
- Twenty Petri dishes of micropropagated 60444 cassava plantlets (see Basic Protocol 1)
- OES induction plates (see recipe)
- FEC induction plates (see recipe)
- Glycerol stock of A. tumefaciens strain LBA4404
- LB plates (see recipe) with antibiotics for Agrobacterium selection (e.g., 30 mg/L rifampicin, 30 mg/L streptomycin, and 50 mg/L kanamycin)
- LB liquid medium (see recipe) with the same antibiotics
- YM liquid medium (see recipe) with the same antibiotics
- Liquid inoculation medium (see recipe)
- Co-culture plates (see recipe)
- GD2 liquid medium (see recipe)
- 250 mg/ml carbenicillin (see recipe)
- Resting plates (see recipe) with carbenicillin
- Callus selection plates (see recipe) with carbenicillin
- Stage 1 regeneration plates (see recipe) with carbenicillin
- Stage 2 regeneration plates (see recipe)
- Stage 3 regeneration plates (see recipe)
- Germination plates (see recipe)
- MS2 agar plates (see recipe)
- 100 × 15–mm sterile Petri dishes (VWR, 25384-342)
- Fine point forceps
- Miltex carbon steel no. 10 surgical blade (prod. no. 4-110)
- Laminar flow hood
- Dissecting microscope
- Sterile hypodermic needles (BD Precision Glide, 23-G × 1 IM TW, 0.6 × 25 mm)
- 60-ml sterile syringes
- Sterile stainless steel mesh (Timesetl, 1-mm2 pore size) cut into 36-cm2 pieces
- Sterile stainless steel spatula
- Parafilm or Saran Wrap cut into 5-cm-wide strips
- 28°C growth chamber
- Culture tubes (VWR, 60818-667)
- 28°C shaking incubator
- 250-ml Erlenmeyer flasks
- Inoculating loops
- Spectrophotometer
- 15- and 50-ml conical polystyrene tubes
- Sterile 12-well plates (Corning Costar, 3513)
- Sterile 100-µm nylon mesh (Sefar, 03-110/47) cut into 25-cm2 squares
- 10-ml pipettes, regular and wide bore (Corning Costar, 4492)
- Sterile 85-mm Whatman filter paper (1001-085, grade 1)
Induce OES
1.Select Petri dishes containing mother plants at 6-8 weeks since previous subculture onto MS2 agar plates.
2.Open one Petri dish and use fine forceps and a scalpel blade to excise the topmost (apical) 1-2 cm portions from 3-5 individual shoots (Fig. 2A). Transfer to a sterile Petri dish lid or bottom.
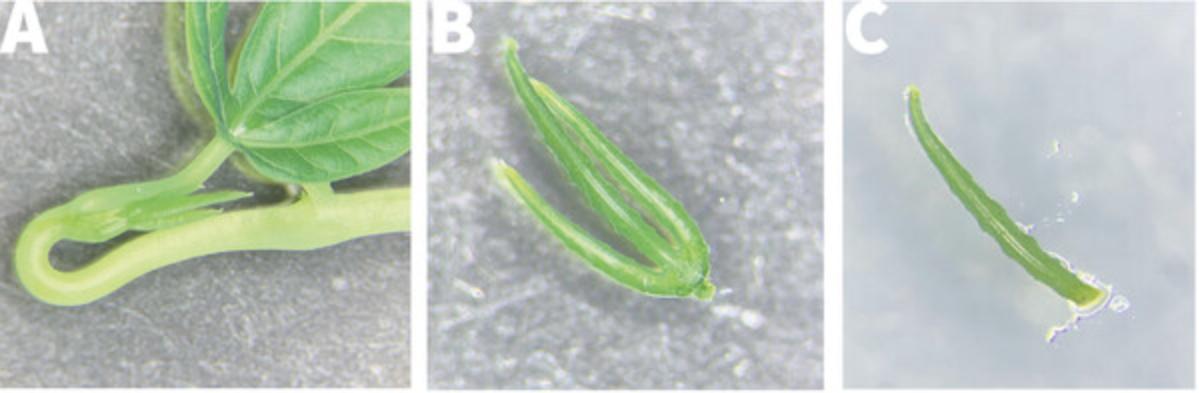
3.In a laminar flow hood under a dissecting microscope, select cuttings in which the youngest leaves are immature, unopened, and 2-5 mm in length.
4.Using fine forceps to hold one cutting, use a 23-G hypodermic needle on a syringe to make incisions at the petiole/lamina junction to separate the three lobes of the leaf from the petiole (Fig. 2B) and from each other (Fig. 2C).
5.Transfer 10-12 leaf lobe explants to an OES induction plate and orient to ensure that the midrib (abaxial side) is downwards and in contact with the medium (Fig. 2C).
6.Repeat steps 2-5 to obtain 100-200 leaf lobe explants in total.
7.Seal plates, label appropriately, and place in a 28°C growth chamber under low light (20 µMol m−2 s−1) for 28 days.
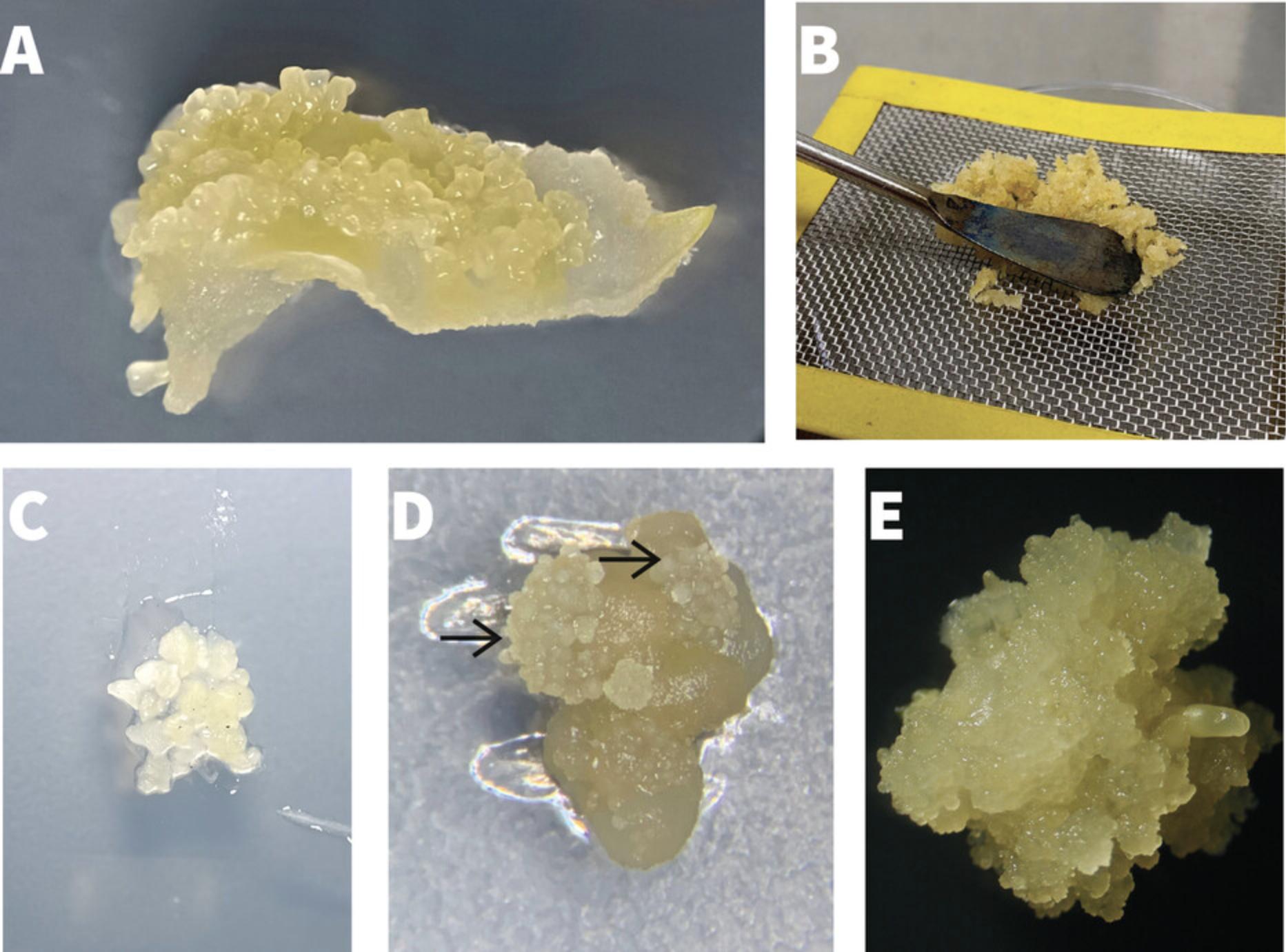
Induce and proliferate FEC
8.Open dishes and use fine forceps to transfer 2-3 explants to a sterile Petri dish lid.
9.Working under a dissecting microscope in a laminar flow hood, use a no. 10 scalpel blade and fine point forceps to excise the OES and separate them from the surrounding non-embryogenic, wet, mushy callus. Cut away all adhering callus tissue and place excised OES fragments onto an FEC induction plate. Cover with a lid to prevent desiccation.
10.Transfer all collected OES fragments to a sterile metal mesh (1-mm pore) placed on top of an empty sterile Petri dish (Fig. 3B).
11.Using a sterile stainless steel spatula, force the OES through the mesh, collecting the fragments below. Use a sterile needle to push adhering tissues through the mesh and add to tissue in the Petri dish.
12.Using sterile forceps, gently mix the meshed OES to homogenize the tissue mass.
13.Using fine forceps, transfer meshed OES to a new FEC induction plate and arrange fragments to form colonies of 10-15 fragments each (Fig. 3C). Establish 6-7 such colonies per FEC induction plate.
14.Seal plates with Parafilm or Saran Wrap, label appropriately, and place in a 28°C growth chamber under low light (20 µMol m−2 s−1) for 28 days (1st cycle).
15.Use fine forceps to select FEC tissues (Fig. 3D) from the colonies and transfer to fresh FEC induction plates. Do not subculture non-embryogenic tissues. Place groups of FEC tissues in a monolayer to generate colonies ∼0.5 cm in diameter, with 7-10 such colonies per dish, and incubate at 28°C in low light (20 µMol m−2 s−1) for 21 days (2nd cycle).
16.Use fine forceps to select FEC tissues from the colonies and transfer to fresh FEC induction plates to generate homogenous FEC tissues (Fig. 3E). Place FEC tissues to generate colonies ∼0.5 cm in diameter, with 7-10 colonies per dish, and incubate at 28°C in low light (20 µMol m-2 s-1) for 21 days (3rd cycle).
Prepare Agrobacterium suspension
17.Streak an Agrobacterium glycerol stock on an LB plate containing the appropriate antibiotics. Incubate inverted for 48 hr at 28°C.
18.Take a single Agrobacterium colony and inoculate a culture tube containing 2 ml LB liquid medium with the same antibiotics. Incubate on a shaking incubator at 250 rpm and 28°C for 8-16 hr.
19.Remove tube from shaker and allow any solids to settle. Take 0.5 ml and inoculate a 250-ml flask containing 20 ml YM liquid medium with the same antibiotics. Incubate overnight at 28°C with shaking at 250 rpm.
20.Determine the optical density (OD600) using a spectrophotometer.
21.Once an OD600 of 0.5 has been reached, transfer bacterial suspension to a sterile 50-ml tube and centrifuge 5 min at 8000 × g. Pour off supernatant and resuspend bacteria in 20 ml liquid inoculation medium. Repeat centrifugation and discard supernatant.
22.Resuspend bacterial pellet in liquid inoculation medium at an OD600 of 0.5.Calculate the resuspension volume of medium (V R) using the formula:
- V R = (V S × ODM)/ODT
where V S is the starting volume (in ml) and ODM and ODT are the measured and target OD600 values. For example:
- V R = (20 ml × 0.82)/0.5 = 32.8 ml
Inoculate FEC with Agrobacterium
23.Remove 10-20 plates of FEC cultures from the growth chamber at the end of the 3rd cycle (Fig. 4A).
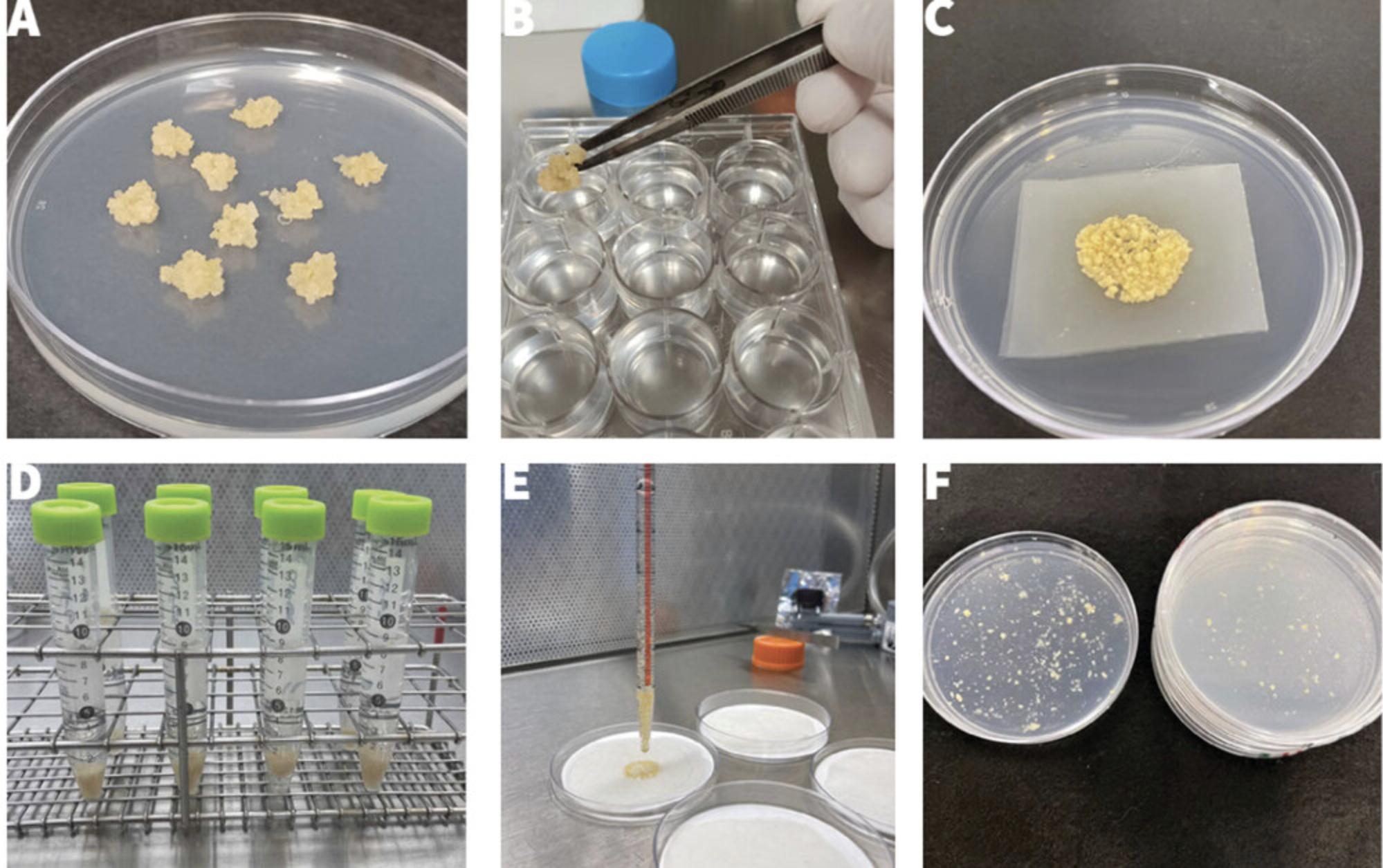
24.Using fine forceps and working under the dissection microscope, select and transfer good-quality FEC tissues to a fresh FEC induction plate.
25.Use forceps to gently mix the FEC and homogenize the tissue mass.
26.Vortex the prepared Agrobacterium suspension for 10 s and dispense 2 ml to each well of a sterile 12-well plate.
27.Using sterile forceps, transfer samples of homogenized FEC to each well (Fig. 4B) until the bottom is covered (one sample is ∼0.5-0.7 cm3). Stir with a pipette tip.
28.Place the lid on the plate and leave in the laminar flow hood for 30 min. Swirl gently by hand 2-3 times during this period to facilitate mixing.
Co-culture FEC and Agrobacterium
29.Place a piece of sterile nylon mesh on an empty sterile Petri dish lid.
30.Use a 10-ml wide-bore pipette to extract the FEC/Agrobacterium suspension from one well.
31.Touch the tip of the pipette to the mesh to prime it (so that moisture passes through the mesh), then transfer the tissue suspension onto the mesh.
32.Use sterile forceps to gently spread the sample to make a monolayer on the mesh.
33.Place the mesh with inoculated FEC on a co-culture plate (Fig. 4C) and label the plate accordingly.
34.Repeat steps 29-33 for each well individually.
35.Seal plates with Parafilm or Saran Wrap and incubate for 2 days at 22°C under bright light (90 µMol m−2 s−1).
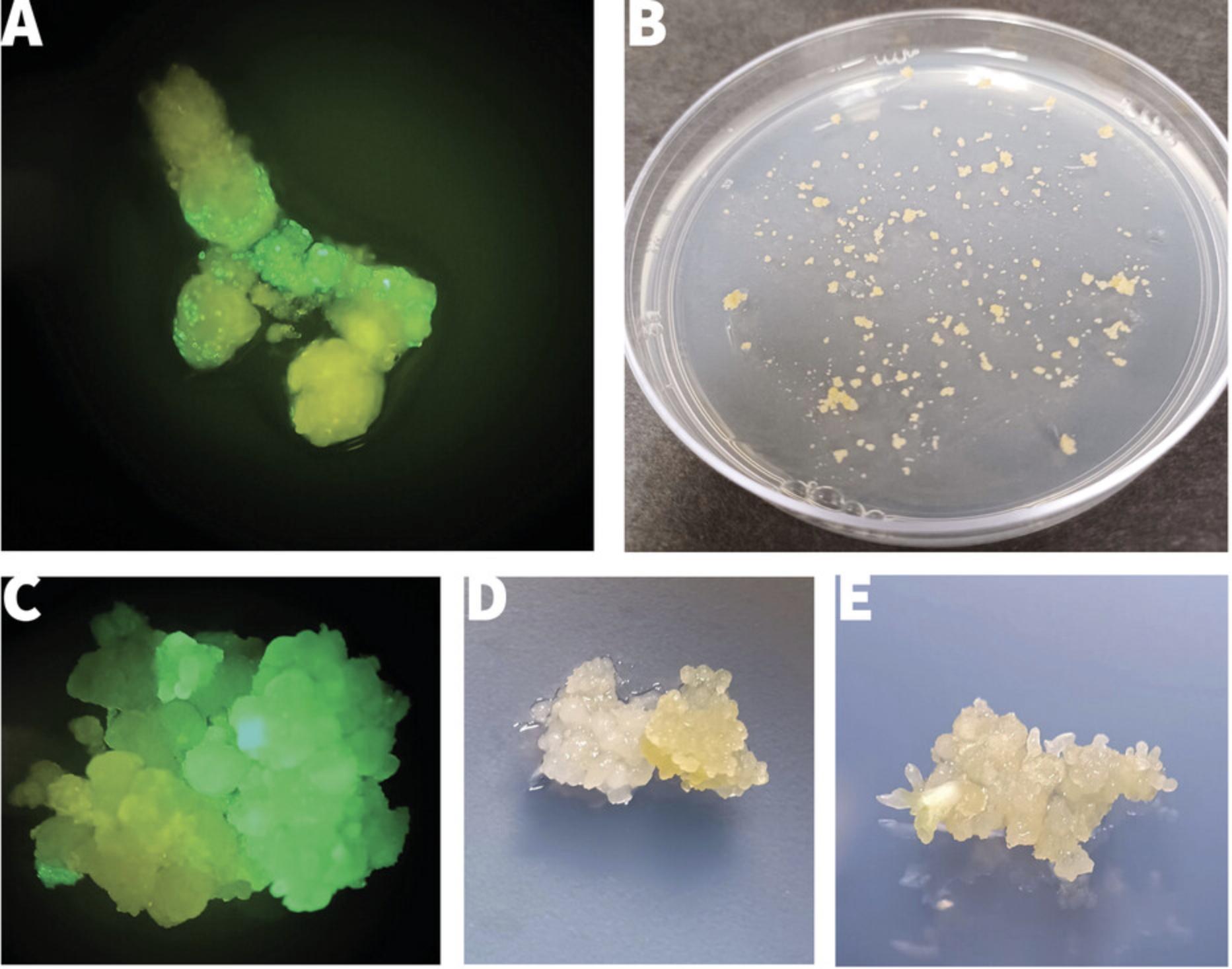
Wash FEC and culture on resting medium
36.Place one sterile 15-ml plastic tube per FEC sample/plate in a test tube rack and label accordingly.
37.Prepare a washing solution by adding 150 µl of 250 mg/ml carbenicillin to 250 ml GD2 liquid medium (final 150 mg/L carbenicillin). Pipette 8 ml to each 15-ml tube.
38.Using sterile forceps, transfer FEC from each plate/mesh to its corresponding tube and vortex 30 s to mix and disaggregate the FEC.
39.Allow tissues to settle for 10 min, then remove liquid using a sterile 10-ml pipette.
40.Add 8 ml fresh GD2 liquid medium containing 150 mg/L carbenicillin to each tube, vortex again, and allow to settle for 10 min (Fig. 4D).
41.Record the volume of FEC in cubic centimeters that has settled to the bottom of the tube.
42.Place a sheet of sterile filter paper on an empty sterile Petri dish and then place a piece of sterile 100-μm nylon mesh on top of the filter paper.
43.Use a 10-ml wide-bore pipette to extract 5 ml of medium from the first tube and discard, then transfer the remaining medium with FEC onto the nylon mesh, allowing the liquid medium to be absorbed into the filter paper (Fig. 4E).
44.Use the pipette or sterile fine forceps to spread the FEC in a monolayer on the mesh.
45.Transfer mesh with FEC to a fresh resting plate (Fig. 4F).
46.Repeat steps 42-45 for all samples.
47.Seal and label the plates appropriately and culture for 8 days at 28°C under low light (20 µMol m−2 s−1).
Recover transgenic tissues on callus selection medium
48.Transfer FEC from the mesh/plates to separate 15-ml tubes containing 8 ml GD2 liquid medium containing 150 mg/L carbenicillin as above (see steps 36-38).
49.Place four pieces of sterile nylon mesh on four separate sterile Petri dish lids. Use a 10-ml wide-bore pipette to extract the FEC from one tube and disperse equal portions across the four meshes.
50.Transfer FEC to callus selection plates by inverting the mesh onto the medium. Using sterile forceps, gently press on the mesh to transfer all of the FEC (Fig. 4C).
51.Repeat for each sample.
52.Seal plates, label appropriately, and culture for 21 days at 28°C under low light (20 µMol m−2 s−1).
Regenerate somatic embryos on stage 1 regeneration plates
53.Under a dissecting microscope, identify healthy growing colonies of FEC.
54.Using sterile fine forceps, transfer about 10 healthy colonies to a stage 1 regeneration plate. Spread colonies evenly onto the medium to form distinct groups.
55.Seal plates and place in the 28°C growth chamber in stacks of not more than three plates. Culture for 18-21 days under bright light (90 µMol m−2 s−1).
Mature somatic embryos on stage 2 regeneration plates
56.Use sterile forceps and a dissection microscope to select and transfer healthy, growing, and developmentally advanced colonies showing the presence of torpedo to early cotyledon-stage embryos (Fig. 5E) to stage 2 regeneration plates, establishing a maximum of 4-6 colonies per plate.
57.Seal plates and culture for another 18-21 days following step 55.
Germinate somatic embryos on germination plates
58.Using a dissection microscope, look for somatic embryos that have developed distinct green cotyledons (Fig. 6A-6C).
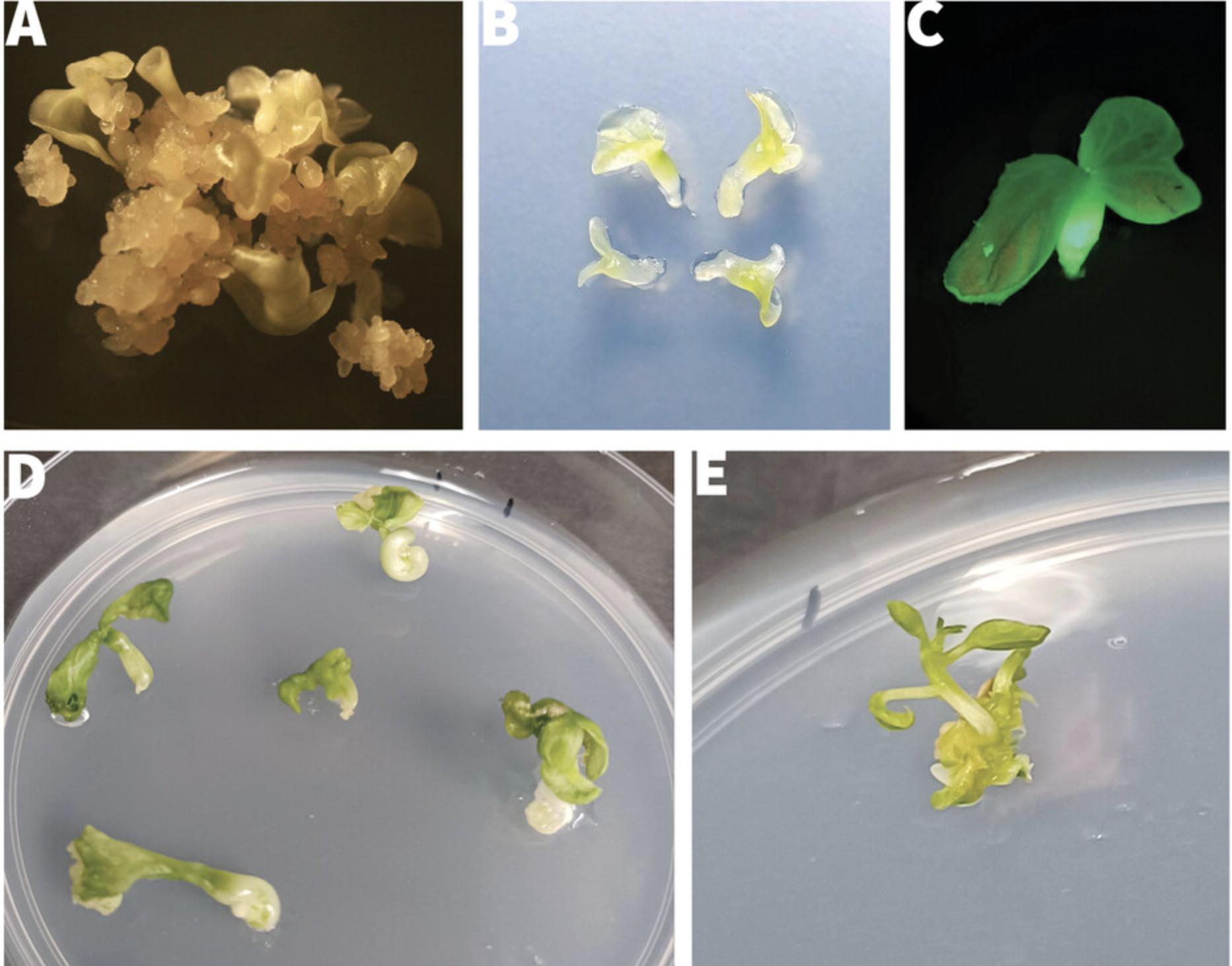
59.Using fine forceps, pick individual cotyledon-stage embryos and trim away any adhering callus using a scalpel blade.
60.Transfer individual embryos to germination plates and gently press into the medium until the underside of the cotyledons is in contact with the medium. Do not submerge the embryo.
61.Place a maximum of 6 embryos from a given callus colony on a dish (Fig. 6D) and label with the line number.
62.Seal plates and culture 18-21 days as in step 55 for embryos to mature and germinate (Fig. 6D).
Establishing regenerated plants on rooting medium
63.Check for shoot production under a dissecting microscope.
64.Using sterile forceps and a scalpel blade, cut the stem of germinated plantlets just above the cotyledons (Fig. 6E). Transfer shoots onto MS2 agar plates, inserting stem ∼0.5 cm into the medium to initiate rooting and plant establishment.
65.Allow plantlets to develop strong shoots and roots and then multiply by micropropagation as required (see Basic Protocol 1).
Alternate Protocol 2: GENETIC TRANSFORMATION OF ADDITIONAL CULTIVARS
Although Basic Protocol 2 is a robust method for production of plants from cassava variety 60444, it must be adapted to regenerate transgenic plants of other genotypes. Changes include the use of alternative media for production of FEC target tissues (e.g., inclusion of tyrosine to encourage FEC production), adjusted Agrobacterium ODs and co-culture, and use of different antibiotics due to the toxicity of carbenicillin to cultivars other than 60444.Table 2 lists the appropriate media for each step of the procedure, along with media composition, culture duration, and scale. The adjusted protocols have proved effective for recovery of transgenic plants in NASE 13, NASE 14, TME 204, TME 419, TME 3, TME 7, TME 14, TMS 01/0040, TMS 01/1206, TMS 91/02324, TMS 92/0326, and TMS 98/0505, plus additional East African landraces.
Additional Materials (also see Basic Protocol 2)
- FEC induction post-mesh plates (see recipe)
- FEC proliferation plates (see recipe)
- 100 mg/ml cefotaxime (see recipe)
- Resting plates (see recipe) with cefotaxime
- Callus selection plates (see recipe) with cefotaxime
- Stage 1 regeneration plates (see recipe) with timentin
Prepare FEC for transformation
1.Induce OES and prepare a meshed and homogenized OES sample as described (see Basic Protocol 2, steps 1-12).
2.Using fine forceps, transfer meshed OES to an FEC induction post-mesh plate and arrange to form colonies of 10-15 fragments each. Establish 6-7 such colonies per plate.
3.Seal plates, label appropriately, and place in a 28°C chamber in the dark for 5 days.
4.Using fine forceps, split each colony into two and subculture onto FEC proliferation plates, placing 6-7 colonies per plate. Seal plates and incubate for 28 days at 28°C in the dark (1st cycle).
5.Use fine forceps to select FEC tissues from the colonies and transfer to fresh FEC proliferation plates. Do not subculture non-embryogenic tissues. Place FEC tissues to generate colonies ∼0.5 cm in diameter, with 6-7 such colonies per plate, and incubate at 28°C in the dark for 21 days (2nd cycle).
6.Use fine forceps to select FEC tissues from the colonies and transfer to fresh FEC proliferation plates. Place FEC tissues to generate colonies ∼0.5 cm in diameter, with 6-7 such colonies per dish, and incubate in the dark for 18-22 days (3rd cycle).
Perform transformation
7.Prepare Agrobacterium strain LBA4404 as described (see Basic Protocol 2, steps 17-22).
8.Dilute Agrobacterium suspension by a factor of 10 with GD2 + 200 µM acetosyringone medium to reach an OD600 of 0.05.
9.Inoculate and co-culture FEC with diluted Agrobacterium as described (see Basic Protocol 2, steps 23-35), but extend the inoculation period to 45 min and the co-culture period to 4 days.
Establish regenerated plants from transformed FEC
10.Wash FEC and culture on resting medium as described (see Basic Protocol 2, steps 36-47) with the following modifications:
- a.
Replace carbenicillin in washing solution (steps 37 and 40) and resting plates (step 45) with 125 mg/L cefotaxime (final concentration).
Inclusion of 50 mg/L moxalactam in the resting medium has been found to be highly effective for enhancing transformation efficiency in some cultivars. It is not routinely added but is worth testing.
- b.In step 47, increase the culture time from 8 days to 10 days.
11.Recover transgenic tissues on callus selection medium as described (see Basic Protocol 2, steps 48-52) with the following modifications:
- a.In step 48, replace carbenicillin in the liquid medium with 125 mg/L cefotaxime (final).
- b.
In step 50, replace carbenicillin in callus selection plates with 125 mg/L cefotaxime and 27.5 µM paromomycin (final).
Sensitivity to paromomycin differs between cultivars. A lower concentration (25 µM) may be desirable at this stage for some cultivars (e.g., NASE 13) and should be tested empirically. Concentrations below 25 µM are not recommended due to the large proportion of non-transgenic escape plants that will be recovered.
12.Regenerate and germinate somatic embryos as described (see Basic Protocol 2, steps 53-62), but replace carbenicillin in stage 1 regeneration plates with 30 mg/L timentin (final).
13.Establish regenerated plants on rooting as described (see Basic Protocol 2, steps 63-65).
Basic Protocol 3: ESTABLISHMENT AND GROWTH OF PLANTS IN THE GREENHOUSE
Once transgenic plants have been obtained (Basic Protocol 2 or Alternate Protocol 2), there is often a need to establish them in soil for subsequent studies. Plants are micropropagated onto MS2 Gelzan medium and cultured for 3-4 weeks. It has been found to be highly beneficial to propagate plants in Gelzan prior to soil transfer. The use of 2.2 g/L Gelzan provides sufficient support for the plants but is soft enough for easy removal with minimal damage to the roots. Plants are then potted in soil and maintained at high humidity. This protocol describes the steps necessary for high efficiency transfer of plants from in vitro conditions to soil for growth in a greenhouse or growth chamber.
Materials
- In vitro cassava plantlets (see Basic Protocol 2 or Alternate Protocol 2)
- MS2 Gelzan plates (see recipe)
- Berger BM7 35% Bark HP (Hummert International, 10121500)
- Gnatrol (Hummert International, 01-2035)
- NPK 15-5-15 fertilizer (Hummert International, 07-5902)
- MOST micronutrients (mix of soluble traces, Hummert International, 07-5990)
- Sprint 330 chelated iron (Hummert International, 07-1511)
- NPK 10-30-20 fertilizer (Hummert International, 07-593300)
- NPK 15-16-17 fertilizer (optional ; Hummert International, 07-592500)
- 28°C greenhouse
- 7.6-cm plastic pots (Hummert International, 11631100)
- 27.8 × 54.5 × 6.2–cm tray with holes (1020 flat, Hummert International, 11-3000)
- 27.8 × 54.5 × 6.2–cm tray without holes (NH flat, Hummert International, 11-3050)
- 8-L watering can
- Low plastic dome (Hummert International, 11-3360)
- Tall plastic dome (Humi-Dome, Hummert International, 14-3852-2)
- Additional reagents and equipment for micropropagating in vitro plantlets (see Basic Protocol 1)
Establish plants in MS2 Gelzan
1.For each line to be propagated, micropropagate two ∼3-cm-tall plants (see Basic Protocol 1), trimming older leaves, into one MS2 Gelzan plate, spacing them evenly apart.
2.Label plates, seal, and place in a 28°C growth chamber under bright light (90 µMol m−2 s−1) for 4 weeks, stacking no more than two plates high.
Establish plants in soil
3.Transfer plates to the potting area.
4.Set up and label one 7.6-cm plastic pot for each plantlet to be potted. Keep pots for different lines separate to avoid mislabeling.
5.Fill pots half full and at a slant with Berger BM7 35%, making sure that the soil is loose and has no large clumps. Place pots in a tray with holes.
6.Add the following to an 8-L watering can and mix to dissolve completely:
- 7.6 L hand-warm tap water
- 24 g Gnatrol
- 12.5 g 15-5-15 fertilizer
- 2.1 g micronutrients
- 4.2 g Sprint 330
Gnatrol is recommended to control insects such as fungus gnats that are present in many greenhouses. An 8-L watering provides sufficient mixture for three trays containing 21 pots each. Scale appropriately.
7.Pour mixture into a tray without holes until the liquid is just over the bottom of the pots and let soil become moist for ∼20 min.
8.Remove lids from plates containing micropropagated plants and fill plates with warm water (33°-35°C).
9.Gently remove plants from plates by loosening the gel. Break up gel in spots free of roots first. Swirl plates to see if plants are loose and thus ready to be transferred. Use fingers to hold the root ball, taking care not to squeeze or damage the stem.
10.Lay each plant gently on the soil within a pot so that the apical meristem and 2-3 leaves are above the surface of the soil. Fill pot gently with Berger BM7 35%.
11.Water plants using a gentle stream from a hose. Place a low dome over pots to prevent wilting. Let tray sit in the Gnatrol mixture for 30 min until soil is saturated.
12.Remove the tray and drain away all water and Gnatrol mixture.
13.Replace low dome with a tall dome and place trays containing plants on a bench to provide 100% humidity. Allow plants to grow 7 days with at least 12 hr light, day temperature 26°C, and night temperature 25°C.
14.Remove trays containing plants from mist bench, puncture the tall dome, and place on the open greenhouse bench. Grow for 5-10 days with 12 hr light, day temperature 27°-32°C, night temperature 21°-26°C, relative humidity 60%-70%.
15.Remove dome gradually once leaves are fully expanded and plants look hardy. Arrange pots in the greenhouse with 10-15 cm spacing to allow vigorous growth.
16.Once established, water plants with micronutrients 28 days after transfer to soil following manufacturer instructions.
17.Apply 10-30-20 fertilizer (following manufacturer instructions) to hardened plants on the open bench as liquid feed each time a plant is watered until the plant has been in soil for 4-5 weeks. Then reduce fertilizer application to three times per week, watering on other occasions with regular water.
18.After 5 weeks, if production of storage roots is required, switch fertilizer to 15-16-17 fertilizer and apply three times per week (Taylor et al., 2012).
REAGENTS AND SOLUTIONS
For a direct comparison of media composition and uses, see Tables 1 and 2.
Growth Media for Agrobacterium
LB medium
For liquid medium : Place a 2-L graduated cylinder containing ∼1.5 L diH2O on a stir plate and begin stirring at ∼200-250 rpm. Slowly add 40 g LB powder and stir until all powder is dissolved (∼10 min). Bring volume to 2 L with diH2O. Set up six 500-ml orange-capped stock bottles with autoclave tape on the caps. Aliquot 340 ml medium to each bottle. Ensure that caps are threaded onto bottles but are still loose, then autoclave on liquid setting for 20 min. After cooling, add appropriate antibiotics and carefully tighten caps. Store up to 3 months at 4°C.
For agar plates : Add 4.5 g microbiology-grade agar to each bottle before autoclaving. After cooling, add appropriate antibiotics, carefully tighten caps, and gently swirl bottles. Dispense 30 ml per 100 × 15–mm plate. Store up to 3 months at 4°C.
YM liquid medium
- 600 ml Milli-Q water
- 0.4 g yeast extract
- 10.0 g mannitol
- 0.2 g magnesium sulfate (MgSO4·7H2O)
- 0.5 g dipotassium phosphate (K2HPO4)
- 0.1 g NaCl
Combine reagents in the order listed and stir until dissolved. Bring to 1 L with Milli-Q water and adjust pH to 7.1. Dispense into 250-ml bottles and autoclave on liquid setting for 20 min. Store up to 3 months at room temperature.
Gresshoff and Doy (GD) and Derivative Media
The following are based on GD basal medium (Gresshoff & Doy, 1974). GD2 medium containing 50 μM picloram is commonly referred to as GD2 50P.
GD2 medium and agar plates
- 600 ml Milli-Q water
- 40 ml 25× GD macronutrients (see recipe)
- 5 ml 200× Fe-EDTA (see recipe)
- 1 ml 1000× GD micronutrients (see recipe)
- 1 ml 1000× GD vitamins (see recipe)
- 20 g sucrose (Fisher Chemical, S5-12 kg)
For liquid medium : Combine reagents in the order listed and stir vigorously until dissolved. Bring to 1 L with Milli-Q water and adjust pH to 6.12 with 1 M NaOH. Dispense into 250-ml autoclave bottles and autoclave. Store up to 3 months at room temperature.
For agar plates : Place 8 g Noble agar (Difco, 214230) in an autoclave bottle. Add 1 L prepared liquid medium, swirl, and autoclave. Cool to 44°C in a water bath, then dispense into 100 × 15–mm Petri dishes at 30 ml per dish. Store up to 1 month at room temperature or 4°C for medium containing antibiotics.
Callus selection plates (GD2 50P + antibiotics)
- GD2 agar plates (see recipe)
- For cultivar 60444, add after autoclaving and cooling:
- 5 ml/L picloram stock (see recipe)
- 300 μl/L carbenicillin stock (see recipe; final 75 mg/L)
- 250 μl/L paromomycin stock (see recipe; final 25 μM)
- For other cultivars :
- Replace carbenicillin with 1.25 ml/L cefotaxime stock (see recipe; final 125 mg/L)
- Increase paromomycin to 275 μl/L (final 27.5 μM)
Co-culture plates (GD2 50P + 200 μM acetosyringone)
- GD2 agar plates (see recipe)
- After autoclaving and cooling, add:
- 5 ml/L picloram stock (see recipe)
- 2 ml/L acetosyringone stock (see recipe)
FEC induction plates (GD2 50P)
- GD2 agar plates (see recipe)
- After autoclaving and cooling, add 5 ml/L picloram (see recipe)
FEC induction post-mesh plates (GD2 50P + 250 μM tyrosine)
- GD2 agar plates (see recipe)
- After autoclaving and cooling, add:
- 5 ml/L picloram stock (see recipe)
- 25 ml/L l-tyrosine stock (see recipe)
FEC proliferation plates (GD2 50P + 250 μM tyrosine + 50 mg/L moxalactam)
- GD2 agar plates (see recipe)
- After autoclaving and cooling, add:
- 5 ml/L picloram stock (see recipe)
- 25 ml/L l-tyrosine stock (see recipe)
- 1 ml/L moxalactam stock (see recipe)
Liquid inoculation medium (GD2 + 200 μM acetosyringone)
- GD2 liquid medium (see recipe)
- After autoclaving, add 2 ml/L acetosyringone (see recipe)
Resting plates (GD2 50P + antibiotics)
- GD2 agar plates (see recipe)
- For cultivar 60444, add after autoclaving and cooling:
- 5 ml/L picloram stock (see recipe)
- 600 μl/L carbenicillin stock (see recipe; final 150 mg/L)
- For other cultivars, replace carbenicillin with :
- 1.25 ml/L cefotaxime stock (see recipe; final 125 mg/L)
Murashige and Skoog (MS) and Derivative Media
The following are based on MS basal medium (Murashige & Skoog, 1962). MS2 medium containing 50 μM picloram is commonly referred to as MS2 50P.
MS2 agar plates
- 600 ml Milli-Q water
- 4.31 g MS basal salts (Sigma, M5524)
- 1 ml 1000× MS vitamins (see recipe)
- 20 g sucrose (Fisher Chemical, S5-12kg)
Combine reagents and stir vigorously until dissolved. Bring to 1 L with diH2O and adjust pH to 6.15 with 1 M NaOH. Place 8 g Noble agar (Difco, 214230) in an autoclave bottle. Add prepared liquid medium, swirl, and autoclave. Cool to 44°C in a water bath, then dispense into 100 × 25–mm Petri dishes (VWR, 89107-632) at 40 ml per dish. Store up to 1 month at room temperature or 4°C for medium containing antibiotics.
Germination plates (MS2 + 2 μM BAP)
- MS2 agar plates (see recipe)
- Before autoclaving add 2 ml/L BAP stock (see recipe)
MS2 Gelzan plates
- MS2 agar plates (see recipe) with 2.2 g/L Gelzan (Sigma, G1910) in place of agar
OES induction plates (MS2 50P + 2 μM CuSO4)
- MS2 agar plates (see recipe)
- Before autoclaving, add 2 ml/L CuSO4 stock (see recipe)
- After autoclaving and cooling, add 5 ml/L picloram stock (see recipe)
- Dispense into 100 × 15–mm Petri dishes at 30 ml per dish
Stage 1 regeneration plates (MS2 + 5 μM NAA + antibiotics)
- MS2 agar plates (see recipe)
- Before autoclaving, add 5 ml/L NAA stock (see recipe)
- For cultivar 60444, add after autoclaving and cooling:
- 450 μl/L paromomycin stock (see recipe; final 45 μM)
- 300 μl/L carbenicillin stock (see recipe; final 75 mg/L)
- For other cultivars, replace carbenicillin with :
- 150 μl/L timentin stock (see recipe; final 30 mg/L)
Stage 2 regeneration plates (MS2 + 0.5 μM NAA + 45 μM paromomycin)
- MS2 agar plates
- Before autoclaving, add 0.5 ml/L NAA stock
- After autoclaving and cooling, add 450 μl/L paromomycin stock
Stage 3 regeneration plates (MS2 + 0.05 μM NAA + 45 μM paromomycin)
- MS2 agar plates
- Before autoclaving, add 0.05 ml/L NAA stock
- After autoclaving and cooling, add 450 μl/L paromomycin stock
Stock Solutions
Acetosyringone, 100 mM
- Place 196.2 mg acetosyringone (3′,5′-dimethoxy-4′-hydroxyacetophenone; Sigma, D134406) in a 15-ml conical tube. Add 5 ml of 100% ethanol to dissolve. Bring volume to 10 ml with Milli-Q water. Filter sterilize through a 0.22-µm syringe filter. Store up to 7 days at −20°C.
- A new acetosyringone stock should be prepared for each transformation experiment.
6-Benzylaminopurine (BAP), 1 mM
- Dissolve 11.26 mg BAP powder (Sigma, B3408) in a 50-ml tube by adding 1-2 ml of 1 M NaOH dropwise. Vortex to dissolve. Bring to 50 ml with Milli-Q water. Store up to 6 months at 4°C in a 50-ml conical tube.
Carbenicillin, 250 mg/ml
- Dissolve 12.5 g carbenicillin (Phytotech, C346) in 35 ml Milli-Q water in a 50-ml conical tube. Vortex to dissolve. Bring to 50 ml with Milli-Q water. Filter sterilize using a 0.22-µm syringe filter and aliquot into sterile 2-ml microcentrifuge tubes. Store up to 1 year at −20°C.
Cefotaxime, 100 mg/ml
- Dissolve 4.0 g cefotaxime powder (Phytotech, C381) in 30 ml of Milli-Q water. Vortex to dissolve. Bring to 40 ml with Milli-Q water. Filter sterilize using a 0.22-µm syringe filter and aliquot into sterile 2-ml microcentrifuge tubes. Store up to 1 year at −20°C.
Copper sulfate, 1 mM
- Weigh out 12.49 mg CuSO4·5H2O (Sigma, C3036) on a 5-figure analytical balance. Dissolve in a clear 50-ml conical tube by adding 10 ml Milli-Q H2O. Swirl or vortex to dissolve. Bring to 50 ml with Milli-Q water in a graduated cylinder. Store up to 6 months at 4°C in a clear 50-ml conical tube.
Fe-EDTA, 200×
- Add 3.725 g Na2EDTA 2H2O (Sigma, E5134) to a beaker containing 300 ml Milli-Q water and mix vigorously until dissolved. Slowly add 2.76 g iron sulfate 7H2O and continue to mix vigorously until dissolved. Bring to 500 ml. Wrap with aluminum foil and store up to 6 months at 4°C.
- Fe-EDTA may precipitate after storage. If this occurs, remove foil and microwave 5-6 min or until all the precipitate dissolves. This is only necessary once after the solution has been prepared.
GD macronutrients, 25×
- 25.0 g ammonium nitrate (Sigma, A3795)
- 1.625 g potassium chloride (Sigma, P9333)
- 8.675 g calcium nitrate 4H2O (Sigma, C2786)
- 0.4275 g magnesium sulphate (anhydrous) (Sigma, M2643)
- 25.0 g potassium nitrate (Sigma, P8291)
- 7.5 g potassium phosphate monobasic (Sigma, P5655)
Add reagents in the order listed to a beaker containing 600 ml Milli-Q water. Mix vigorously until dissolved. Bring volume to 1 L with Milli-Q water. Store up to 6 months at 4°C.
GD micronutrients, 1000×
- 1000 mg manganese sulphate H2O (Sigma, M7899)
- 25 mg cobalt (II) chloride 6H2O (Sigma, C2911)
- 25 mg copper (II) sulfate 5H2O (Sigma, C3036)
- 300 mg boric acid (Sigma, B6768)
- 25 mg molybdic acid (sodium salt) 2H2O (Sigma, M1651)
- 800 mg potassium iodide (Sigma, P8166)
- 300 mg zinc sulphate 7H2O (Sigma, Z1001)
Add reagents in the order listed to a beaker containing 400 ml Milli-Q water. Mix vigorously until dissolved. Bring to 1 L with Milli-Q water. Store in 50-ml conical tubes up to 6 months at 4°C.
GD vitamins, 1000×
- 0.1 g d-biotin (Sigma, B4639)
- 0.2 g glycine (free base, Sigma, G7403)
- 5 g myo-inositol (Sigma, I7508)
- 0.05 g nicotinic acid (free acid, Sigma, N4126)
- 0.05 g pyridoxine HCl (Sigma, P6280)
- 0.5 g thiamine HCl (Sigma, T1270)
Add reagents in the order listed to a beaker containing 400 ml Milli-Q water. Mix vigorously until dissolved. Bring to 500 ml with Milli-Q water. Filter sterilize using a 0.22-µm filter. Store in 50-ml conical tubes up to 6 months at 4°C.
Moxalactam, 50 mg/ml
- Dissolve 2.0 g moxalactam (Sigma, M8158) in 30 ml Milli-Q water. Vortex to dissolve. Bring to 40 ml with Milli-Q water. Filter sterilize using a 0.22-µm syringe filter and aliquot into sterile 2-ml microcentrifuge tubes. Store up to 1 year at −20°C.
MS vitamins, 1000×
- Dissolve MS vitamin powder (Sigma, M7150-100 ml) in 95 Milli-Q water in a beaker. Bring to 100 ml to a 1000× stock. Filter sterilize using a 0.22-µm filter. Store in 50-ml sterile conical tubes for up to 6 months at 4°C.
1-Naphthaleneacetic acid (NAA), 1 mM
- Dissolve 46.5 mg NAA powder (Sigma, N0640) in a 50-ml conical tube using 3 ml of 1 M NaOH. Vortex to dissolve. Bring to 250 ml with Milli-Q water in a graduated cylinder. Store up to 6 months at 4°C in a 250-ml Pyrex bottle.
Paromomycin, 100 mM
- Find the batch number on the paromomycin bottle (Sigma, P8692) and locate the Certificate of Analysis on the online Sigma product page. Find the purity of the batch by checking the µg paromomycin/mg anhydrous basis value. This should be greater than or equal to 675 (corresponding to 67.5% paromomycin). Calculate the amount needed for a 100 mM stock of 100% paromomycin and add this amount into a 50-ml conical tube. Bring to final volume with Milli-Q water and vortex. Filter sterilize through a 0.22-µm syringe filter and aliquot into sterile 2-ml microcentrifuge tubes. Store up to 1 year at −20°C.
Picloram, 10 mM
- Dissolve 1.208 g picloram (Sigma, P5575) in a 50-ml conical tube using 3-5 ml of 1 M NaOH. If necessary, place tube in a 50°C water bath to dissolve. Bring to 50 ml Milli-Q water and vortex to mix. Transfer to a 500-ml cylinder and bring to 500 ml with Milli-Q water. Adjust pH to 5.8 by adding 1 M HCl dropwise. Filter sterilize though a 0.22-µm Stericup filter. Store up to 6 months at 4°C.
Timentin, 200 mg/ml
- Dissolve 5.0 g timentin powder (Phytotech, T869) in 20 ml Milli-Q water and vortex to dissolve. Bring to 25 ml with Milli-Q water. Filter sterilize through a 0.22-µm syringe filter. Aliquot into sterile 2-ml microcentrifuge tubes and store up to 1 year at −20°C.
Tyrosine, 10 mM
- Dissolve 2.18 g l-tyrosine HCl (Sigma, T2006) in a 50-ml conical tube by adding 2-3 ml of 5 M NaOH. Transfer to a graduated cylinder and add Milli-Q water to 990 ml (pH will be 11-12). Add concentrated HCl dropwise to adjust pH to 5.8, switching to 1 M HCl as you approach the desired pH (precipitation will occur). Heat using a heated stir plate or microwave until completely dissolved. Allow solution to cool to 45°-50°C. Filter sterilize using a 0.22-µm Stericup 2. Store up to 6 months at 4°C.
COMMENTARY
Background Information
The genetic transformation systems described here are based on the production of totipotent embryogenic tissues that are amenable to T-DNA delivery by Agrobacterium. Selection of transgenic embryogenic callus is followed by regeneration of mature somatic embryos and their germination to produce genetically modified plantlets. Production of totipotent tissues commences by inducing somatic embryos from immature leaf explants cultured on MS-based medium supplemented with high levels of a potent auxin. In our hands, picloram is the most effective auxin for production of OES, but 2,4-dichlorophenoxyacetic acid (2,4-D) can be employed if the former is not available (Taylor et al., 1996). Totipotent FEC, used as the target tissue for transgene integration, is generated by subculture of the OES onto GD-based medium supplemented with picloram. Switching from MS-based to GD-based medium is critical for successful production of FEC, which is subsequently selected and proliferated to generate homogenous FEC target tissues for transformation with Agrobacterium. Transition back to MS-based medium with reduced auxin stimulates reorganization of the tissues to produce torpedo and cotyledon-stage embryos, which are then matured and germinated to recover plants.
The methods and technology described have proven to be robust for small- and large-scale recovery of transgenic and gene-edited cassava plants. The systems have been critical components of research programs in multiple laboratories investigating disease resistance (Beyene, Chauhan, et al., 2017; Gomez et al., 2018; Ogwok et al., 2012), nutritional enhancement (Beyene, Solomon, et al., 2017; Ihemere, Arias-Garzon, Lawrence, & Sayre, 2006; Narayanan, Beyene, Chauhan, Grusak, & Taylor, 2020), starch modification (Bull et al., 2018), post-harvest deterioration (Salcedo & Siritunga, 2011), herbicide tolerance (Hummel et al., 2018), and fundamental questions central to the biology of this under-investigated crop species. In some cases, these traits have been stacked to enhance potential value to farmers and consumers (Narayanan et al., 2021). The methods described have proven suitable for large-scale plant production within product development programs (up to 500-1000 independent transgenic events per year). Performance of regenerated plants has been demonstrated in the field (Narayanan et al., 2020; Ogwok et al., 2012; Wagaba et al., 2017) and meets the quality required for regulatory approval (Wagaba et al., 2020). Transgenic cassava plants produced following Alternate Protocol 1 have been integrated into breeding programs in East Africa, proving the potential utility of these systems to impact cassava yields for farmers. Recently, the methods described have been successfully adapted for genome editing (Gomez et al., 2018; Odipio et al., 2017), being sufficiently powerful to support homologous recombination and allele replacement (Hummel et al., 2018).
These protocols were originally developed to produce transgenic plants in the West African cassava variety 60444 (Schöpke et al., 1996; Taylor et al., 1996). When applied as described in Basic Protocol 1, the method is powerful, with a trained laboratory technician able to produce more than 1000 independent transgenic events per year. Twenty to thirty independent events can be recovered per cm3 SCV of starting material in a period of 4 months, from Agrobacterium transformation to plant recovery. Generation of transgenic plants in 60444 is especially effective because production of embryogenic target tissues and plant regeneration occur at high efficiencies, and because it is very amenable to Agrobacterium co-culture and T-DNA transfer (Bull et al., 2009; Taylor et al., 2012). Although no longer cultivated by farmers, 60444 remains a useful model cultivar for laboratory and greenhouse studies of transgene expression, cassava biology, and gene editing (Odipio et al., 2017; Taylor et al., 2012).
Cassava is a highly heterozygous species, with significant genetic variation between varieties. Many hundreds of cassava cultivars and landraces are cultivated across the tropics and have been selected to meet differing farmer, consumer, and processor needs. Expanding capacity for genetic transformation into a range of cassava varieties with relevance to cultivation in Africa, Asia, and Central/South America initially proved to be challenging. Over the last 10 years, significant success has been achieved in broadening the number of cassava cultivars that can be genetically transformed by the techniques described here. Alternate Protocol 1 and Table 2 provide adaptations to the culture media and transformation systems that are critical to this effort. The rationale behind these are detailed below.
Use of tyrosine for FEC induction
Supplementation of GD2 50P-based media with tyrosine is effective for encouraging production of FEC from fragmented OES. The amount of tyrosine required varies with the cultivar and should be assessed empirically when a new cultivar is brought into culture. For example, TME 7 responds well to 500 µM tyrosine, whereas TME 419 and NASE 13 require no more than 250 µM tyrosine to stimulate FEC production (Chauhan et al., 2015). Tyrosine is detrimental for induction of FEC in cv. 60444 and should not be used.
Moxalactam enhances transformation efficiency
Addition of moxalactam is highly effective for enhancing the quality of FEC produced and efficiency of T-DNA delivery when included in the later cycles of FEC production (cycles 2 and 3 on GD2 50P-based media), prior to inoculation with Agrobacterium. This response varies across cultivars. No effect is seen in 60444, but a 50-100× increase in transformation efficiency can be achieved in TME 204, TME 419, and other cultivars when this antibiotic is included in the GD2 50P FEC induction medium before and after transformation with Agrobacterium (Chauhan et al., 2015).
Reduction of Agrobacterium OD
In most plant transformation systems, Agrobacterium inoculations occur at bacterial densities of 0.3-0.7 OD600. However, significant reduction in the OD600 was found to be beneficial for genetic transformation of cassava FEC. While OD600 0.5 is effective in 60444, a reduction to OD600 0.05 or even 0.01 is dramatically superior in other cultivars, especially when combined with a 4-day co-culture period (Chauhan et al., 2015).
Use of alternative antibiotics to control Agrobacterium
A critical discovery was that carbenicillin is toxic to most cassava cultivars (but not 60444) and prevents growth and recovery of transgenic callus after Agrobacterium co-culture. Use of cefotaxime in place of carbenicillin overcomes this problem and actively encourages proliferation of FEC tissues (Chauhan et al., 2015). Timentin is favored for use in stage 1 regeneration medium, where the goal is to suppress FEC production and stimulate regeneration of somatic embryos (Chauhan et al., 2015).
Co-culture in the light
While co-culture of FEC with Agrobacterium under dark conditions results in T-DNA transfer, especially in 60444, performing this step under light conditions has proven to enhance genetic transformation.
Elimination of antibiotics in later regeneration stages
Washing FEC tissues after co-culture as described is effective for eliminating Agrobacterium regrowth. This allows antibiotics to be omitted after stage 1 regeneration medium (embryo induction). We have confirmed by PCR that Agrobacterium is not detected in or associated with regenerated plantlets when the procedures described here are followed.
The paromomycin selection agent should not be included in the germination medium. It is not required and its presence suppresses shoot germination from mature cotyledon-stage somatic embryos.
Metatopolin as an alternative cytokinin for stimulating shoot regeneration
We have seen positive results with the use of 2 µM metatopolin for embryo germination in place of BAP. Shoot regeneration from mature cotyledon-stage embryos can be accomplished by culture on MS2 + 2 µM metatopolin + Gelzan just as effectively as on MS2 + 2 µM BAP + agar (Chauhan & Taylor, 2018).
Benefits of methods presented
The genetic transformation methods detailed here bring multiple benefits. Understanding them provides researchers with important opportunities to adapt the systems for their specific needs and laboratory capacities.
Suitability for use with a range of chemical and visual selectable markers. This wide adaptability is important for enabling recovery of transgenic plants (see further discussion below).
Compatibility with different Agrobacterium strains. While LBA4404 is the most effective strain in our hands, success has also been achieved with EHA105, GV3101, and AGL1. AGL1 is employed in our laboratory in cases where rearrangement of gene constructs takes place in LBA4404.
Production of nonchimeric regenerates. Plants regenerated via Agrobacterium transformation of FEC are reliably nonchimeric in nature. This contrasts with other cassava regeneration systems, in which transgenic plants are recovered from transformation of OES tissues or matured somatic embryo–derived cotyledons (Jørgensen et al., 2005, Li et al., 1996). In our hands, the latter systems produce a high frequency of chimeric plants, which complicates the production and analysis of quality events.
Adaptability to other gene transfer technologies. FEC tissues produced following the methods described here are adaptable for use with direct gene transfer technologies such as microparticle bombardment (Chellappan et al., 2004) and as a source of totipotent protoplasts for transgene integration and genome editing (Sofiari, Raemakers, Bergervoet, Jacobsen, & Visser, 1998).
Selectable and visual marker systems
An important benefit of the tissue culture and transformation system described here is its adaptability for use with different visual and selectable marker systems. The ability to employ alternative selectable markers provides flexibility to meet the preferences of different laboratories and allows retransformation to stack transgenes within the same cassava plant (Okwuonu, Achi, Egesi, & Taylor, 2015)
Paromomycin. The protocols detailed here are based on use of npt II as the selectable marker gene and utilize paromomycin at 25 or 27.5 μM as the selection agent in the GD2 50P–based callus selection medium. This is increased to 45 μM in the regeneration media. The presence of paromomycin causes non-transgenic tissues to become bleached and die, making visual selection for putative transgenic tissues straightforward. The use of paromomycin as described will result in recovery of less than 1%-2% of non-transgenic escape lines. Paromomycin should not be included in the germination medium, as it suppresses germination of somatic embryos to produce plants. Our standard plasmids use the 35S promoter to drive expression of npt II. The Nos promoter is also effective for driving expression of npt II and is preferred in some cases due to the tendency of the 35S promoter to act in trans on other promoters within the T-DNA (Mette, van der Winden, Matzke, & Matzke, 1999).
Hygromycin. The culture systems described here have been successfully adapted to use hygromycin (Bull et al., 2009). If employing the hpt selectable marker gene, paromomycin can be replaced with hygromycin at 20 mg/L in the callus selection medium and 40 mg/L in the regeneration media (Okwuonu et al., 2015).
Glyphosate. Cassava transformation systems have also been adapted to use the herbicide glyphosate as a selection agent. Expression of mutated versions of the EPSPS gene have been used for transgenic and allelic replacement technologies (Hummel et al., 2018). Use of glyphosate is ineffective in the callus selection medium, but its inclusion at 2.5 mM in the regeneration media strongly suppresses development of green cotyledon stage embryos in non-transgenic tissues to facilitate recovery of genetically modified and edited events.
GFP and DsRed for system development, quality control, and tracking transformation efficiency. The tissue systems detailed in these protocols are highly suited for use with non-lethal, visual marker genes such as GFP and DsRed. Both can be used to track transformation efficiency from the single-cell stages immediately after co-culture to whole plantlet regeneration. GFP is especially effective due to the non-photosynthetic nature of the embryogenic tissues and is included as an internal control in all experiments performed in our laboratory. Examining tissues using a UV dissecting microscope provides accurate assessment for success of each transformation experiment and its progress into stages of early cell division, establishment of FEC colonies, and regeneration of somatic embryos. Use of GFP or DsRed is also recommended when adapting the system for use with a new cultivar, attempting to develop enhanced processes, or establishing cassava transformation in a new laboratory. The ability to rapidly determine and understand transgene expression, and to do so in a non-lethal manner, is very effective for training researchers in the methods and for learning where success and bottlenecks are occurring at each stage of the transformation process. When performed under a fluorescence microscope in a laminar flow hood, visual identification, manual selection, and subculture of GFP- or DsRed-expressing callus lines and somatic embryos to the next culture stage is also an effective process for recovery of transgenic tissues and plants. This can be beneficial as it negates the need for use of a selectable marker transgene such as npt II and hpt and inclusion of chemical selection agents in the media.
Critical Parameters
Multiple aspects of the protocols have been found critical to success. These have been discovered over almost 20 years of experience with these systems and are discussed below.
General
Work under a dissecting microscope. Perform tissue manipulations under a good-quality dissecting microscope at all steps except whole plant establishment and micropropagation. In our laboratory, we use the Olympus SZ51. Working under the microscope is essential for accuracy of explant preparation, OES isolation, FEC identification, selection and subculture, isolation and subculture of healthy callus on antibiotic selection medium, identifying maturing somatic embryos, and handling cotyledon-stage embryos. Constant observations under the microscope are also central for learning to recognize the tissues being produced and gaining the knowledge required to optimize the culture systems for quality results.
Establish a tissue culture and transformation pipeline. The protocols detailed here can be used as one-off processes to produce a small number (5-20) of independent transgenic or gene-edited plants. It is recommended, however, to establish a pipeline that routinely produces FEC target tissues so that they are available as needed for use in transformation and gene-editing experiments. When FEC tissues are generated in this manner, only 3-5 months are required from the time gene constructs are available to recovery of modified plants of a given cassava variety. Establishing a pipeline requires micropropagation of in vitro mother plants every 6-8 weeks so that they remain in optimal condition. Leaf explants should be established on OES induction medium every alternate week and tissues moved through the GD2 50P–based media to ensure that FEC tissues are generated and available for transformation experiments on a continuous basis to support research programs.
Media
Use only high-quality agar. Cassava is susceptible to the impurities found in lower-grade agar products. Whole plants and embryogenic cultures will fail to develop, grow slowly, and/or be of poor quality if grown on low-grade, impure agar. Use only the highest quality agar, such as Noble agar (Difco, 214230). If high-grade agar cannot be obtained, Gelzan can be used in its place and is effective especially for whole plant culture at the beginning and end stages of the protocols.
Make basal media from stocks in-house. It is recommended not to rely on commercially sourced basal media unless they have been tested and proven to be effective. It is preferable to make basal media from scratch using the individual chemical components to ensure quality and consistency. This is especially the case for Gresshoff and Doy basal media. It is recommended to not use GD basal salts medium sourced from Duchefa Biocheme, as we and several collaborating laboratories have found it to be ineffective for production of FEC from OES tissues.
Use deep Petri dishes to encourage somatic embryo development. Culture in deep Petri dishes (100 × 25 mm) encourages regeneration and maturation of somatic embryos. Use of deep dishes at stage 1 and 2 regeneration plates and germination plates is intentional, as we have found that the increased headspace significantly encourages somatic embryo development and shoot regeneration.
Use fresh media. We recommend keeping media for a maximum of 4 weeks. OES induction medium is optimal for up to 2 weeks. All media containing antibiotics should be kept refrigerated and warmed on the bench to room temperature before use.
Plant materials
Ensure high quality of mother plants. Mother plants for production of leaf explants must be kept in good condition by repeated subculture on fresh MS2 medium every 2-3 months. Propagate only strong, healthy plants. If a mother plant appears weak or slow-growing, do not micropropagate it to establish the next cycle of mother plants and do not use it as a source of leaf explants to initiate OES. In this manner, weak, slow-growing plants are eliminated in favor of strong, vigorously growing plants that provide the highest quality leaf explants.
Orient leaf explants correctly on the medium. Leaf lobe explants must be oriented so that the abaxial surface and midrib are in contact with the medium. Take care to orient each explant individually to ensure proper contact with the OES induction medium. Do not submerge explants into the medium. Failure to follow this process will result in little or no OES production.
Transfer only OES tissue onto FEC induction medium. Trim away and discard all non-embryogenic tissues from the OES before meshing and subculturing onto GD2 50P–based medium. It is important to take extra care and to perform this step under a dissecting microscope to be able to visualize the tissues accurately. Subculture of non-embryogenic tissues significantly reduces or negates FEC production.
Select FEC carefully and subculture to generate homogenous tissues lines. Use a dissecting microscope to visualize embryogenic tissues. Use fine forceps to select FEC and subculture to the next cycle of FEC induction. This ensures elimination of non-embryogenic tissues and production of the high-quality FEC tissues required for successful transformation.
Culture FEC for a maximum of three cycles on GD-based medium. It is highly recommended to not exceed three culture cycles of FEC on GD2 50P–based medium before transformation with Agrobacterium. Exceeding three cycles suppresses the regeneration potential of the embryogenic tissues and increases the frequency of offtype plants regenerated (Taylor et al., 2012).
Evenly spread tissues on callus selection medium. Ensure that FEC tissues are spread evenly on the callus selection medium and avoid clumping to ensure even exposure to the selection agent. This also allows transgenic events to be isolated early in the process to maximize the number of independent transgenic plant lines recovered in each experiment. If FEC tissues become clumped together when transferred onto the callus selection medium, use fine forceps to manually move FEC units apart and place arrange them on the same selection plate.
Do not overload plates with tissues. Statements for placing a specified number of callus tissues and somatic embryos per plate at each stage are intentional. Overloading the plates with tissues inhibits development, especially maturation of somatic embryos on stage 1 and regeneration media and shoot regeneration on germination medium. Exceeding the stated amounts will delay or prevent somatic embryo recovery and plant regeneration.
Subculture green cotyledon-stage embryos to germination medium. Allow somatic embryos to develop to the green cotyledon stage before subculture onto germination medium. Only distinct green embryos should be subcultured, as earlier stage embryos will not mature into the large green cotyledon-stage embryos capable of germinating to produce plantlets.
Trim mature embryos to encourage shoot regeneration. In some cases, mature cotyledon-stage embryos do not germinate on MS2 2BAP medium. Instead, large green cotyledon tissues develop. If this occurs, remove embryos and place on a sterile Petri dish lid. Use forceps and a scalpel blade to trim away excess foliose tissues to within 3-4 mm of the hypocotyl and apical region. Place trimmed embryos onto fresh germination medium and return to the culture chamber. Observe weekly for shoot regeneration.
Troubleshooting
See Table 3 for a list of problems encountered with the protocols along with their causes and solutions.
Problem | Possible cause | Solution |
---|---|---|
In vitro plant establishment and micropropagation | ||
Contamination of nodal explants during culture on MS2 medium | Woody or semi-woody nodal sections used | Use only new growth with fresh green stem |
Too many nodes in bleach solution | Use a maximum of 12-15 green nodes per 250-ml flask or 25 per 500-ml flask | |
Bleach stock solution too old | Use newly opened commercial bleach; ensure commercial bleach is at least 8% sodium hypochlorite | |
Insufficient surfactant | Ensure that two drops of Tween-20 were added | |
Insufficient agitation | Agitate at 150 rpm or by hand every 5 min | |
Endophytic contamination | Eliminate endophytic contamination by propagating shoot tips from newly sprouted material and subculturing to new MS2 plate; flame sterilize instruments after handling each shoot, dipping in ethanol (bead sterilizers have not been effective in killing some endophytes) | |
Shoots fail to grow from nodal explants | Excessive bleach used or extended sterilization period | Do not add >15% (v/v) bleach; do not exceed recommended 30-40 min sterilization period |
Insufficient material retained above and below axillary bud | Leave at least 0.75-1.0 cm above and below node before sterilization to allow bleaching of tissues from the cut surface without bleaching and damaging of axillary bud | |
Poor-quality stake material | Select only healthy new growth from sprouted stake cuttings | |
Poor health and growth of micropropagated mother plants | Poor quality gelling agent | Use highest-quality agar (Noble agar) or Gelzan |
Incorrect media composition | Ensure all media components were not expired and were added correctly | |
Poor quality starting material | Use healthy starting material no more than 8-10 weeks old | |
Production of embryogenic target tissues | ||
No or very little OES production from leaf explants | Mother plants in poor condition | Use mother plants no more than 6-8 weeks from previous subculture |
Leaf explants not oriented on medium correctly | Ensure abaxial side of explant and midrib are in contact with medium, but do not submerge into the medium | |
Medium too old or made incorrectly | Use freshly made induction medium or medium no older than 2 weeks; extend OES induction period from 4 to 5 weeks, if required (do not extend to point where distinct cotyledon-stage embryos start to form) | |
No or very little FEC produced from OES | Insufficient wounding of OES | Force OES tissues through 1-mm2 mesh to ensure wounding |
OES too mature | Use developmentally young OES (before it reaches late torpedo embryo stage) | |
FEC is slow growing and/or unhealthy brown color | Media made incorrectly | Ensure all media components were not expired and were added correctly |
Poorly sealed Petri dishes | Make sure Petri dishes are well sealed | |
FEC left too long on GD2 50P induction medium | Subculture FEC to fresh medium every 21-28 days | |
Too much tyrosine in GD2 50P induction medium | Reduce tyrosine in GD2 50P FEC induction medium | |
FEC cultures not homogenous by end of 3rd cycle, but a mix of tissue types | Did not select pure FEC when subculturing tissues | Select only distinct, pure FEC when subculturing, leaving hard brown callus and mushy, non-embryogenic callus tissues behind |
Insufficient number of plates of FEC produced for transformation experiments | Too few leaf explants established | Establish sufficient leaf explants (e.g., for 60444, set up 200 leaf explants) |
Not enough FEC subcultured | Select pure FEC when subculturing | |
Agrobacterium culture and FEC inoculation and co-culture | ||
No or minimal growth of mini-culture | Excess or incorrect antibiotic | Check for correct antibiotic type(s) and concentrations |
Insufficient time at 28°C | Prolong mini-culture time for up to 24 hr | |
Detrimental gene construct | Assess gene construct to determine if leaky expression may impact bacterial vitality and growth | |
No or minimal growth of overnight culture | Excess or incorrect antibiotics | Check for correct antibiotic type(s) and concentration |
Insufficient inoculum | Inoculate multiple flasks per gene construct with different inoculum amounts | |
Insufficient time at 28°C | Prolong culture time; check culture status every additional 2 hr | |
Detrimental gene construct | Assess gene construct to determine if leaky expression may impact bacterial vitality and growth | |
Recovery of transgenic tissues, regeneration of somatic embryos and plants | ||
No GFP expression at end of co-culture period | Agrobacterium delivery of T-DNA failed | Follow all proper Agrobacterium handling procedures and growth periods |
Poor-quality FEC tissue | Use FEC at 3rd cycle, 18-22 days since previous subculture | |
Tissue/genotype recalcitrance to Agrobacterium infection | Adjust and test effects of co-culture time (2-4 days); perform co-culture in dark versus light conditions; test alternative Agrobacterium strains | |
All FEC tissue dies/does not grow on callus selection medium | Incorrect paromomycin concentration in selection medium | Check paromomycin concentration |
Incorrect medium composition | Ensure all media components were not expired and were added correctly | |
No transgenic tissue present | Repeat transformation with fresh FEC target tissues | |
All FEC tissue grows on callus selection medium | Insufficient selection pressure | Check paromomycin concentration; prepare fresh antibiotic stock solutions and fresh selection medium |
All callus lines die on stage 1 regeneration medium with 45 μM paromomycin | Excessive paromomycin | Check paromomycin concentration |
Incorrect medium composition | Check medium composition | |
Cotyledon-stage embryos do not develop from healthy FEC callus on stage 1 and 2 regeneration medium | Insufficient culture time in reduced auxin conditions | Subculture tissues to stage 3 regeneration medium (MS2 + 0.05 μM NAA + 45 μM paromomycin) |
Cotyledon-stage embryos do not germinate on MS2 + 2 μM BAP medium | Poor-quality embryos selected for transfer to germination medium | Select best-quality embryos for subculture. Embryos should have two distinct cotyledons and a hypocotyl with visible immature apical meristem. Avoid trumpet-shaped embryos or those with no cotyledon development. |
Excessive number of cotyledons on a plate | Place no more than 6 embryos per plate | |
Some cultivars can be problematic at this stage, possibly because large foliose cotyledons inhibit shoot formation | Remove somatic embryos from germination medium and trim away excess cotyledon tissues within 2 mm of apical region; subculture cleaned embryos onto fresh germination medium | |
BAP maybe insufficient for germination | If embryos refuse to germinate, trim away excess cotyledon tissues and subculture onto MS2 + 2 μM metatopolin + Gelzan | |
Regenerated plants are not transgenic | Insufficient selection pressure at callus selection and stage 1-2 regeneration stages | Check paromomycin concentration; use freshly made medium |
Do not exceed recommend tissue amounts per plate at these stages | ||
Regenerated plants are offtype | Excess time in culture at FEC stages | Cycle FEC tissues for the appropriate times; do not exceed recommended number of FEC cycles (3 maximum) or time per cycle |
Transgenes impact phenotype | Research potential developmental impact of transgene(s) and modify expression cassetes as required | |
Transfer and establishment of in vitro plants in soil | ||
Failure of plantlets to establish in soil, plants die in 3-4 days; low-frequency plant establishment | Poor-quality or too-young material transferred to soil from tissue culture stage | Use plantlets 4-5 cm in height with well-developed root system |
Root or stem damage from improper handling of plants during transfer | Handle young plants gently, taking care not to damage the stem; use MS2 Gelzan medium for micropropagation to minimize root damage; place no more than 3 plants per plate of MS2 Gelzan medium | |
Insufficient humidity for plant establishment | Make sure pots are thoroughly watered; maintain humidity close to 100% | |
Plants die after 4-10 days in soil | Fungal contamination causes damping off | Use good-quality sterile potting soil; water with fungicide (e.g., Mancozeb) |
Fungus gnats or similar insect pests | Water with Bt-based insecticidal solution (e.g., Gnatrol) | |
Insufficient humidity for plant establishment | Maintain humidity close to 100% | |
Plants die on open bench 10-28 days after transfer | Plastic domes removed too early | Follow recommended hardening period |
Over- or underwatering | Do not allow soil to dry out; do not allow plants to sit in water | |
Plants grow slowly or develop poorly | Insufficient fertilizer | Water with micronutrients (e.g., MOST) 28 days after transfer |
Plants do not produce storage roots | Insufficient fertilizer; excessive nitrogen in fertilizer | Use a low-nitrogen fertilizer regime for storage root production (e.g., NPK 10-30-20) |
Pot too big | Use 7-9 cm pots; if the pot is too large, plants will produce shoot growth over storage root development |
Understanding Results
Using a visual marker to assess transformation efficiency
It is important to track the success of the transformation system within each experiment. This allows results to be understood, adjustments to be made, and experiments to be repeated, if required. The use of GFP or a similar non-lethal, visual marker is a powerful tool for understanding the genetic transformation system and assessing progress and efficiency of transgenic tissue and plant production. The non-photosynthetic nature of the FEC tissues facilitates easy visualization of fluorescent markers with minimal background interference, allowing visual tracking through all steps in the methodologies described in Basic Protocol 2 and Alternate Protocol 2. Transformation efficiencies can be determined at each stage of the process from co-culture with Agrobacterium to full plant recovery. Data can be collected as the number of GFP-expressing callus units and embryos per starting unit (cm3) of FEC sample (Chauhan et al., 2015). This provides valuable information regarding success and failures within the transformation system, allowing them to be identified and addressed in a timely manner. GFP is used as an internal control in all experiments performed in our laboratory, allowing efficiency of the transformation process to be tracked across experiments, cultivars, and gene constructs. In our hands, the use of an ER-targeted GFP expression cassette driven by the constitutive 35S promoter allows optimal visualization of the transformation process from the single-cell to whole-plant stages (Taylor et al., 2012). Expected results when using GFP are shown in Table 4 and described below.
Culture stage | Expected result | Notes |
---|---|---|
End of co-culture | 100s of single GFP-expressing cells per sample (Fig. 5A) | Large numbers of single GFP-expressing cells indicate successful T-DNA transfer |
End of resting stage | Dozens of 2- to 8-cell-stage GFP-expressing cell units per sample | A large reduction in single-cell expression is expected. Cell division should be visible by this time, indicating healthy tissues and successful transformation. |
End of callus selection stage | Growing GFP-expressing FEC colonies (Fig. 5C,D) | Rapid cell division indicates healthy tissues and resistance to antibiotic selection pressure. |
End of stage 1 regeneration | Growing GFP-expressing FEC colonies with development of torpedo to early cotyledon-stage embryos (Fig. 5E) | Presence of some dead, white colonies is expected and indicates efficacy of the elevated antibiotic selection pressure at this stage. |
End of stage 2 regeneration | GFP-expressing colonies consisting of torpedo and cotyledon-stage embryos (Fig. 6A-C) | Colonies displaying active growth and somatic embryo regeneration at this stage are considered transgenic and tracked onwards with a unique line number. If cotyledon-stage embryos have not developed, subculture to stage 3 regeneration medium (0.05 μM NAA). |
Shoot regeneration stage | Development of somatic embryos with large green cotyledons, followed by production and growth of shoots (Fig. 6D,E) |
Calculating efficiency of genetic transformation
Efficiency of transformation for a given gene construct/cultivar/Agrobacterium interaction within and across experiments can be calculated and expressed at each stage of the method. At the end of the co-culture and resting stages, a subjective 0-5 scoring is most easily used because counting many hundreds of GFP-expressing single cells for multiple transformed FEC samples is time-consuming and inaccurate. A visual assessment from 0 for no expression to 5 for extremely high numbers (e.g., 1000s) of expressing cells per sample is routinely used in our lab (Chauhan et al., 2015; Fig. 5). A score of 0 at the end of the resting stage indicates that the transformation failed. In this case, it is recommended to stop the experiment for all gene constructs and repeat the transformation process as soon as possible. For possible reasons that FEC transformations can fail, see Table 3.
By the end of the callus selection stage, the number of putatively transgenic callus lines recovered per unit of starting material can be accurately assessed. The amount of starting material per sample can be calculated by determining the settled cell volume (SCV in cm3) of FEC that was inoculated. SCV is determined by allowing the FEC tissues in a sample to settle to the bottom of a graduated tube (see Basic Protocol 2). This is best performed after co-culture in order not to complicate the inoculation stage, when multiple samples are handled at the same time. Once the SCV is known, the number of callus lines produced per starting sample can be determined, followed by the number of independent transgenic plants per SCV of starting material. In 60444 and TME 204, this is reliably as high as 20-30 plants/cm3 SVC (Chauhan et al., 2015; Taylor et al., 2012).
In some laboratories, the use of GFP or alternative fluorescent markers may not be possible. In this case, an alternative visual marker gene such as uid A (GUS) can be used to assess and track efficacy of transformation from co-culture through plant recovery. However, the tissues being assessed will be lost due to the lethal nature of the transgene visualization process. Alternatively, the system can be tracked by counting the number of healthy yellow callus lines recovered at the end of the callus selection stage and similarly through the putative transgenic tissues recovered at the end of stages 1 and 2 regeneration, and the number of independent lines that produce plants by germination on germination medium.
Time Considerations
For a detailed description and timing of each cultivation step in the processes used to recover transgenic cassava plants, refer to Tables 1 and 2. Time from co-culture of FEC tissues with Agrobacterium is 3-3.5 months for 60444 and ∼1 month longer for other varieties. As cassava is highly heterozygous and vegetatively propagated, the T0 generation is most often the product desired and upon which subsequent experimental procedures will be assessed. Recovery of transgenic plants within this timeframe therefore places cassava favorably compared to other crop species.
To prevent extended recovery time of transgenic cassava plants, it is highly recommended to establish a pipeline for production of FEC target tissues and to have them available for transformation every 2-3 weeks. In this manner extended times are not required between transformation and gene-editing experiments waiting for the next batch of FEC tissues to become available.
Acknowledgments
The methods and systems described here were developed with support from the United States Agency for International Development (USAID) from the American People, Bayer Crop Science, and the Bill & Melinda Gates Foundation. The authors acknowledge contributions from Ted Moll, Brent Trauterman, and Marina Kalyaeva.
Author Contributions
Rosana Segatto : Method development, writing (original draft and editing), photography; Tira Jones : Method development, photography; Danielle Stretch : Method development, photography; Claire Albin : Method development; Raj Deepika Chauhan : Method development; Nigel Taylor : Method development, writing (original draft and editing).
Conflict of Interest Statement
The authors declare that they have no conflict of interest.
Open Research
Data Availability Statement
Data sharing not applicable to this article as no datasets were generated or analyzed during the current study.
Literature Cited
- Beyene, G., Chauhan, R. D., Ilyas, M., Wagaba, H., Fauquet, C. M., Miano, D., … Taylor, N. J. (2017). A virus-derived stacked RNAi construct confers robust resistance to cassava brown streak disease. Frontiers in Plant Science , 7, 2052. doi: 10.3389/fpls.2016.02052
- Beyene, G., Solomon, F. R., Chauhan, R. D., Gaitán-Solis, E., Narayanan, N., Gehan, J., … Cahoon, E. B. (2017). Provitamin A biofortification of cassava enhances shelf life but reduces dry matter content of storage roots due to altered carbon partitioning into starch. Plant Biotechnology Journal , 16(6), 1186–1200. doi: 10.1111/pbi.12862
- Bull, S. E., Owiti, J. A., Niklaus, M., Beeching, J. R., Gruissem, W., & Vanderschuren, H. (2009). Agrobacterium -mediated transformation of friable embryogenic calli and regeneration of transgenic cassava. Nature Protocols , 4(12), 1845–1854. doi: 10.1038/nprot.2009.208
- Bull, S. E., Seung, D., Chanez, C., Mehta, D., Kuon, J. E., Truernit, E., … Vanderschuren, H. (2018). Accelerated ex situ breeding of GBSS- and PTST1-edited cassava for modified starch. Science Advances , 4(9), eaat6086. doi: 10.1126/SCIADV.AAT6086/SUPPL_FILE/AAT6086_SM.PDF
- Chauhan, R. D., Beyene, G., Kalyaeva, M., Fauquet, C. M., & Taylor, N. (2015). Improvements in Agrobacterium -mediated transformation of cassava (Manihot esculenta Crantz) for large-scale production of transgenic plants. Plant Cell Tissue and Organ Culture , 121(3), 591–603. doi: 10.1007/s11240-015-0729-z
- Chauhan, R. D., Beyene, G., & Taylor, N. J. (2018). Multiple morphogenic culture systems cause loss of resistance to cassava mosaic disease. BMC Plant Biology , 18(1), 132. doi: 10.1186/s12870-018-1354-x
- Chauhan, R. D., & Taylor, N. J. (2018). Meta-topolin stimulates de novo shoot organogenesis and plant regeneration in cassava. Plant Cell, Tissue and Organ Culture , 132(1), 219–224. doi: 10.1007/S11240-017-1315-3/FIGURES/3
- Chavarriaga-Aguirre, P., Brand, A., Medina, A., Prías, M., Escobar, R., Martinez, J., … Tohme, J. (2016). The potential of using biotechnology to improve cassava: A review. In Vitro Cellular and Developmental Biology - Plant , 52(5), 461–478. doi: 10.1007/s11627-016-9776-3
- Chellappan, P., Masona, M. V., Vanitharani, R., Taylor, N. J., & Fauquet, C. M. (2004). Broad spectrum resistance to ssDNA viruses associated with transgene-induced gene silencing in cassava. Plant Molecular Biology 2004 56:4 , 56(4), 601–611. doi: 10.1007/S11103-004-0147-9
- Gomez, M. A., Berkoff, K. C., Gill, B. K., Iavarone, A. T., Lieberman, S. E., Ma, J. M., … Danforth, D., (2021). CRISPR-Cas9-mediated knockout of CYP79D1 and CYP79D2 in cassava attenuates toxic cyanogen production. BioRxiv , 2021.10.08.462827. doi: 10.1101/2021.10.08.462827
- Gomez, M. A., Lin, Z. D., Moll, T., Chauhan, R. D., Hayden, L., Renninger, K., … Bart, R. S. (2018). Simultaneous CRISPR/Cas9-mediated editing of cassava eif4e isoforms ncbp-1 and ncbp-2 reduces cassava brown streak disease symptom severity and incidence. Plant Biotechnology Journal , 17(2), 421–434. doi: 10.1111/pbi.12987
- Gresshoff, P. M., & Doy, C. H. (1974). Derivation of a haploid cell line from Vitis vinifera and the importance of the stage of meiotic development of anthers for haploid culture of this and other genera. Zeitschrift Für Pflanzenphysiologie , 73(2), 132–141. doi: 10.1016/s0044-328x(74)80084-x
- Hummel, A. W., Chauhan, R. D., Cermak, T., Mutka, A. M., Vijayaraghavan, A., Boyher, A., … Taylor, N. J. (2018). Allele exchange at the EPSPS locus confers glyphosate tolerance in cassava. Plant Biotechnology Journal , 16(7), 1275–1282. doi: 10.1111/pbi.12868
- Ihemere, U., Arias-Garzon, D., Lawrence, S., & Sayre, R. (2006). Genetic modification of cassava for enhanced starch production. Plant Biotechnology Journal , 4(4), 453–465. doi: 10.1111/j.1467-7652.2006.00195.x
- Jørgensen, K., Bak, S., Busk, P. K., Sørensen, C., Olsen, C. E., Puonti-Kaerlas, J., & Møller, B. L. (2005). Cassava plants with a depleted cyanogenic glucoside content in leaves and tubers. Distribution of cyanogenic glucosides, their site of synthesis and transport, and blockage of the biosynthesis by RNA interference technology. Plant Physiology , 139(1), 363–374. doi: 10.1104/pp.105.065904
- Li, H.-Q., Sautter, C., Potrykus, I., & Puonti-Kaerlas, J. (1996). Genetic transformation of cassava (Manihot esculenta Crantz). Nature Biotechnology , 14(6), 736–740. doi: 10.1038/nbt0696-736
- Liu, J., Zheng, Q., Ma, Q., Gadidasu, K. K., & Zhang, P. (2011). Cassava genetic transformation and its application in breeding. Journal of Integrative Plant Biology , 53(7), 552–569. doi: 10.1111/j.1744-7909.2011.01048.x
- Mette, M. F., van der Winden, J., Matzke, M. A., & Matzke, A. J. (1999). Production of aberrant promoter transcripts contributes to methylation and silencing of unlinked homologous promoters in trans. EMBO Journal , 18(1), 241–248. doi: 10.1093/emboj/18.1.241
- Murashige, T., & Skoog, F. (1962). A revised medium for rapid growth and bio assays with tobacco tissue cultures. Physiologia Plantarum , 15(3), 473–497. doi: 10.1111/j.1399-3054.1962.tb08052.x
- Narayanan, N., Beyene, G., Chauhan, R. D., Gaitán-Solís, E., Gehan, J., Butts, P., … Taylor, N. J. (2019). Biofortification of field-grown cassava by engineering expression of an iron transporter and ferritin. Nature Biotechnology , 37(2), 144–151. doi: 10.1038/s41587-018-0002-1
- Narayanan, N., Beyene, G., Chauhan, R. D., Grusak, M. A., & Taylor, N. J. (2021). Stacking disease resistance and mineral biofortification in cassava varieties to enhance yields and consumer health. Plant Biotechnology Journal , 19(4), 844–854. doi: 10.1111/pbi.13511
- Odipio, J., Alicai, T., Ingelbrecht, I., Nusinow, D. A., Bart, R., & Taylor, N. J. (2017). Efficient CRISPR/cas9 genome editing of phytoene desaturase in cassava. Frontiers in Plant Science , 8, 1780. doi: 10.3389/fpls.2017.01780
- Ogwok, E., Odipio, J., Halsey, M., Gaitán-Solís, E., Bua, A., Taylor, N. J., … Alicai, T. (2012). Transgenic RNA interference (RNAi)-derived field resistance to cassava brown streak disease. Molecular Plant Pathology , 13(9), 1019–1031. doi: 10.1111/j.1364-3703.2012.00812.x
- Okwuonu, I. C., Achi, O. K., Egesi, C. N., & Taylor, N. J. (2015). Evaluation of red fluorescent protein (DsRed) as alternative visual marker of genetic transformation in cassava (Manihot esculenta Crantz). In Vitro Cellular & Developmental Biology - Plant, 51(5), 571–579. doi: 10.1007/s11627-015-9718-5
- Pushpalatha, R., & Gangadharan, B. (2020). Is cassava (Manihot esculenta Crantz) a climate “smart” crop? A review in the context of bridging future food demand gap. Tropical Plant Biol , 13, 201–211. doi: 10.1007/s12042-020-09255-2
- Salcedo, A., & Siritunga, D. (2011). Insights into the physiological, biochemical and molecular basis of postharvest deterioration in cassava (Manihot esculenta) roots. Journal of Experimental Agriculture International , 1(4), 414–431. doi: 10.9734/AJEA/2011/784
- Schöpke, C., Taylor, N., Cárcamo, R., Konan, N. K., Marmey, P., Henshaw, G. G., … Fauquet, C. (1996). Regeneration of transgenic cassava plants (Manihot esculenta Crantz) from microbombarded embryogenic suspension cultures. Nature Biotechnology , 14(6), 731–735. doi: 10.1038/nbt0696-731
- Sofiari, E., Raemakers, C. J. J. M., Bergervoet, J. E. M., Jacobsen, E., & Visser, R. G. F. (1998). Plant regeneration from protoplasts isolated from friable embryogenic callus of cassava. Plant Cell Reports , 18(1), 159–165. doi: 10.1007/S002990050550
- Taylor, N. J., Edwards, M., Kiernan, R. J., Davey, C. D. M., Blakesley, D., & Henshaw, G. G. (1996). Development of friable embryogenic callus and embryogenic suspension culture systems in cassava (Manihot esculenta Crantz). Nature Biotechnology , 14(6), 726–730. doi: 10.1038/nbt0696-726
- Taylor, N., Gaitán-Solís, E., Moll, T., Trauterman, B., Jones, T., Pranjal, A., … Fauquet, C. M. (2012). A high-throughput platform for the production and analysis of transgenic cassava (Manihot esculenta) plants. Tropical Plant Biology , 5(1), 127–139. doi: 10.1007/s12042-012-9099-4
- Utsumi, Y., Utsumi, C., Tanaka, M., Okamoto, Y., Takahashi, S., Huong, T. T., … Seki, M. (2022). Agrobacterium -mediated cassava transformation for the Asian elite variety KU50. Plant Molecular Biology , 109(3), 271–282. doi: 10.1007/S11103-021-01212-1/TABLES/3
- Wagaba, H., Beyene, G., Aleu, J., Odipio, J., Okao-Okuja, G., Chauhan, R. D., … Alicai, T. (2017). Field level RNAi-mediated resistance to cassava brown streak disease across multiple cropping cycles and diverse East African agro-ecological locations. Frontiers in Plant Science , 7, 2060. doi: 10.3389/FPLS.2016.02060/BIBTEX
- Wagaba, H., Kuria, P., Wangari, P., Aleu, J., Obiero, H., Beyene, G., … MacKenzie, D. J. (2020). Comparative compositional analysis of cassava brown streak disease resistant 4046 cassava and its non-transgenic parental cultivar. GM Crops & Food, 12(1), 158–169. doi: 10.1080/21645698.2020.1836924