Update: Induction of Inflammatory Bowel Disease in Immunodeficient Mice by Injection of Naïve CD4+ T cells (T Cell Transfer Model of Colitis)
Claire F. Pearson, Claire F. Pearson, Kevin J. Maloy, Kevin J. Maloy
Abstract
The intestinal inflammation induced by injection of naïve CD4+ T cells into lymphocyte-deficient hosts (more commonly known as the T cell transfer model of colitis) shares many features of idiopathic inflammatory bowel disease (IBD) in humans, such as epithelial cell hyperplasia, crypt abscess formation, and dense lamina propria lymphocyte infiltration. As such, it provides a useful tool for studying mucosal immune regulation as it relates to the pathogenesis and treatment of IBD in humans. In the IBD model described here, colitis is induced in Rag (recombination-activating gene)-deficient mice by reconstitution of these mice with naïve CD4+CD45RBhi T cells through adoptive T cell transfer. Although different recipient hosts of cell transfer can be used, Rag-deficient mice are the best characterized and support studies that are both flexible and reproduceable. As described in the Basic Protocol, in most studies the transferred cells consist of naïve CD4+ T cells (CD45RBhi T cells) derived by fluorescence-activated cell sorting from total CD4+ T cells previously purified using immunomagnetic negative selection beads. In a Support Protocol, methods to characterize colonic disease progression are described, including the monitoring of weight loss and diarrhea and the histological assessment of colon pathology. © 2024 The Author(s). Current Protocols published by Wiley Periodicals LLC.
Basic Protocol : Induction of IBD in Rag-deficient mice by the transfer of naïve CD4+CD45RBhi T cells
Support Protocol : Monitoring development of colitis
INTRODUCTION
Historically, most animal studies of IBD utilized chemically induced or polymer/microbial-induced models of intestinal inflammation, which generally cause acute or subacute inflammatory responses, rather than the chronic inflammation that is characteristic of the human disease. The advent of mouse strains with deletion of specific genes led to the development of a raft of new mouse models of IBD. This initially included mice with targeted disruptions of the interleukin 10 gene (IL-10KO mice; Kuhn et al., 1993), the IL-2 gene (IL-2KO mice; Sadlack et al., 1993), the T cell receptor α chain gene (TCR-αKO mice; Mombaerts et al., 1993), and the signaling G protein subunit Gαi2 gene (Gαi2K0 mice; Rudolph et al., 1995); however, in later years many additional single-gene-deletion models of IBD were reported. The original version of the T cell transfer colitis model using lymphocyte-deficient SCID mice restored with CD4+CD45RBhi T cells is part of this group as well, because it depends on mice with a gene deletion (Morrissey et al., 1993; Powrie et al., 1993).These IBD models represented a significant step forward in gastrointestinal research as the intestinal lesions that develop in these models share many histological features present in human IBD, including chronic inflammation, epithelial cell hyperplasia, ulceration, and depletion of mucin-secreting goblet cells. In addition, these IBD models share the fact that in all cases colitis development occurs as a result of an altered immune system and thus establish that an intact immune system is necessary for prevention of intestinal inflammation (Powrie, 1995). The CD4+CD45RBhi T cell transfer model of IBD has several advantages over other models, as the incidence of disease is high (∼90%) and the kinetics and course of disease are highly reproducible (see Understanding Results). This is in contrast to the spontaneous models in various knockout mice, in which disease occurs with variable incidence and can take 3-4 months to develop. The CD4+CD45RBhi T cell transfer model can be used to study CD4+ T cell–mediated inflammatory responses and their regulation in vivo , as well as the effects of various therapeutic strategies on these processes.
This article describes the transfer of naïve CD4+ T cells into immunodeficient mice to initiate colitis, with or without regulatory T cells that ameliorate disease (Basic Protocol). A Support Protocol provides methods to characterize colonic disease progression, including the monitoring of weight loss and diarrhea and the histological assessment of colon pathology.
NOTE : All protocols involving animals must be reviewed and approved by the appropriate Animal Care and Use Committee and must follow regulations for the care and use of laboratory animals.
Basic Protocol: INDUCTION OF IBD IN RAG-DEFICIENT MICE BY THE TRANSFER OF NAÏVE CD4+CD45RBhi T CELLS
In this protocol, CD4+ T cell subsets are isolated from the spleen by a process of negative selection using magnetic beads followed by cell sorting. These consist of CD4+CD45RBhi T cells that are injected into immunodeficient hosts either alone (for induction of colitis) or in combination with CD4+CD25+CD45RBlo regulatory T (Treg) cells (given concomitantly for protection from colitis, or injected at a later time point for cure of colitis). Following this cell isolation and injection, the recipient mice are monitored for the development of intestinal disease. Transfer of CD4+CD45RBhi T cells from C57BL/6J mice to C57BL/6J Rag1 –/– mice is used here as an example, but the model has also been shown to work when transferring CD4+CD45RBhi T cells from different inbred strains into MHC-matched T cell–deficient recipients, including BALB/c into CB.17 SCID (Powrie et al., 1993), 129 SvEv into 129 SvEv Rag2 –/– (Powrie et al., 1996), and C57BL/6J mice into Tcrb –/– Tcrd –/– mice (Ostanin et al., 2006). This protocol is described using female donor and recipient mice, but male donors and recipients may also be used.
Materials
-
6- to 8-week-old female C57BL/6J Rag1–/– mice (recipient mice; C57BL/6J Rag2–/– mice may also be used)
-
6- to 12-week-old female C57BL/6J mice (donor mice)
-
PBS/BSA, with and without EDTA (see recipe)
-
0.025% (w/v) trypan blue
-
MojoSortTM Mouse CD4 T cell isolation kit (Biolegend) or EasySepTM Mouse CD4+ T cell isolation kit (StemCell Technologies)
-
Phycoerythrin-conjugated anti-CD25 antibody (CD25-PE; Biolegend or equivalent)
-
Peridinin-chlorophyll-protein-Cyanine 5.5-conjugated anti-CD4 antibody (CD4-PerCP Cy5.5, Biolegend or equivalent)
-
Allophycocyanin–conjugated anti-CD45RB antibody (CD45RB-APC; Biolegend or equivalent)
-
4,6-Diamidino-2-phenylindole (DAPI; ThermoFisher or equivalent)
-
Heat-inactivated fetal bovine serum (FBS)
-
70-mm nylon cell strainers (Fisher Scientific or equivalent)
-
1-ml disposable syringe
-
15- and 50-ml conical polypropylene centrifuge tubes
-
Magnet (StemCell Technologies EasySep magnet or Biolegend Mojo magnet or equivalent)
-
4- and 5-ml polystyrene tubes for cell sorting (Falcon or equivalent)
-
Fluorescence cell sorter (Becton Dickinson Aria III or equivalent)
-
Top-pan balance
-
Additional equipment for removal of lymphoid organs (Reeves & Reeves, 1992), preparation of single-cell suspensions (Kruisbeek, 2001), negative selection of CD4+ cells using magnetic beads (Horgan et al., 2009, immunofluorescence staining of single-cell suspensions (Holmes et al., 2001), trypan blue exclusion (Strober, 2015), management of immunocompromised animals (Donovan & Brown, 2007), cell sorting (Holmes et al., 2001; McKinnon, 2018), intraperitoneal injection (Donovan & Brown, 2006a), unique identification of mice (Donovan & Brown, 2006b), and CO2 euthanasia (Donovan & Brown, 2006c)
NOTE : All manipulations should be carried out at 4°C unless otherwise stated.
NOTE : Protocols involving animals must be reviewed and approved by the appropriate Institutional Animal Care and Use Committee. Institutional guidelines for the care and use of laboratory animals must be followed.
Prepare single-cell suspension
1.Extract 10 spleens from normal C57BL6/J mice and place them into 20 ml of sterile PBS/BSA.
2.Generate a single-cell suspension by forcing the spleens through a 70-μm nylon mesh or cell strainer using the plunger from a 1-ml disposable syringe. Wash the mesh with 10 ml PBS/BSA and add the wash to the single-cell suspension.
3.Pellet the cells by centrifugation for 5 min at 300 × g , 4°C, and resuspend cells in PBS/BSA/EDTA solution (1 ml per spleen).
Enrich for CD4+ cells using magnetic beads
4.Count cells using trypan blue uptake to exclude dead cells from the count.
5.Utilize a kit protocol to enrich the cells. Procedures described in steps 6-11 are derived from the MojoSort protocol and should be modified if other kits are used.
6.Pellet the cells as above and resuspend in PBS/BSA/EDTA at 1 × 108 cells/ml. Add 10 μl Biotin-Antibody cocktail per 100 μl of cells, mix well, and incubate at 4°C for 15 min.
7.Vortex Streptavidin Nanobeads and add 10 μl nanobeads for each 100 μl of cells; mix well and incubate the mixture at 4°C for 15 min.
8.If <2.5 ml total solution is obtained, increase the volume to 2.5 ml with PBS/BSA/EDTA; then pipet up and down to disrupt any aggregates.
9.Place the tube in the magnet for 5 min.
10.Pour out in one smooth motion and collect the liquid. Do not discard as this contains the CD4+ T cells.
11.Repeat steps 8-10 to increase the yield.
12.Pellet collected cells (5 min at 300 × g , 4°C) and resuspend in 5 ml PBS/BSA/EDTA.
13.Count viable cells again. Remove five aliquots of 5 × 105 cells into 4-ml polystyrene tubes as controls for cell sorting.
Label with fluorochrome-conjugated antibodies
14.Pellet remainder of cells (5 min at 300 × g , 4°C) and resuspend in a 5-ml polypropylene tube at 1-2 × 107 cells/ml in PBS/BSA/EDTA containing 15 μg/ml CD25-PE (1:100), 2 μg/ml CD4-PerCP-Cy5.5 (1:400), and 15 μg/ml CD45RB-APC (1:100). Incubate for 30 min at 4°C in the dark.
15.During incubation of samples, if using cells for single-color controls, pellet control aliquots of cells (step 13) at 300 × g , 4°C, for 5 min and resuspend in 100 μl PBS/BSA containing one of the following per tube:
- Tube a: nothing
- Tube b: 15 μg/ml CD45RB-FITC
- Tube c: 1 μg/ml CD25-PE
- Tube d: 1 μg/ml CD4-PerCP-Cy5.5
- Tube e: 1 μg/ml DAPI
16.Incubate cells with mAb at 4°C for 30 min in the dark and then wash twice with 3 ml PBS/BSA. Resuspend single-color control cell pellets in 300 μl PBS/BSA and stored at 4°C until analysis.
17.Pellet labeled cells (step 14) and wash twice with 10 ml PBS/BSA. Resuspend in PBS/BSA at 1 × 107/ml at store at 4°C until analysis.
Perform cell sorting
18.Set up a cell sorter (McKinnon, 2018) by running unstained cells (step 15, tube a).
19.Determine appropriate compensation (Holmes et al., 2001) for four-color analysis using FITC-, PE, and PerCP-Cy5.5-conjugated mAbs by analyzing cells stained with CD45RB-FITC alone (tube b), with CD25-PE alone (tube c), with CD4-PerCP-Cy5.5 alone (tube d), and with DAPI alone (tube e).
20.Analyze labeled cells (step 17) on the cell sorter and set appropriate gates (Holmes et al., 2001). Use DAPI to exclude nonviable cells, and use forward scatter height and width to exclude doublet cells.
21.Sort the cell suspension into DAPI– CD4+CD25loCD45RBhi and DAPI– CD4+CD25hiCD45RBlo T cell populations as shown in Figure 1.
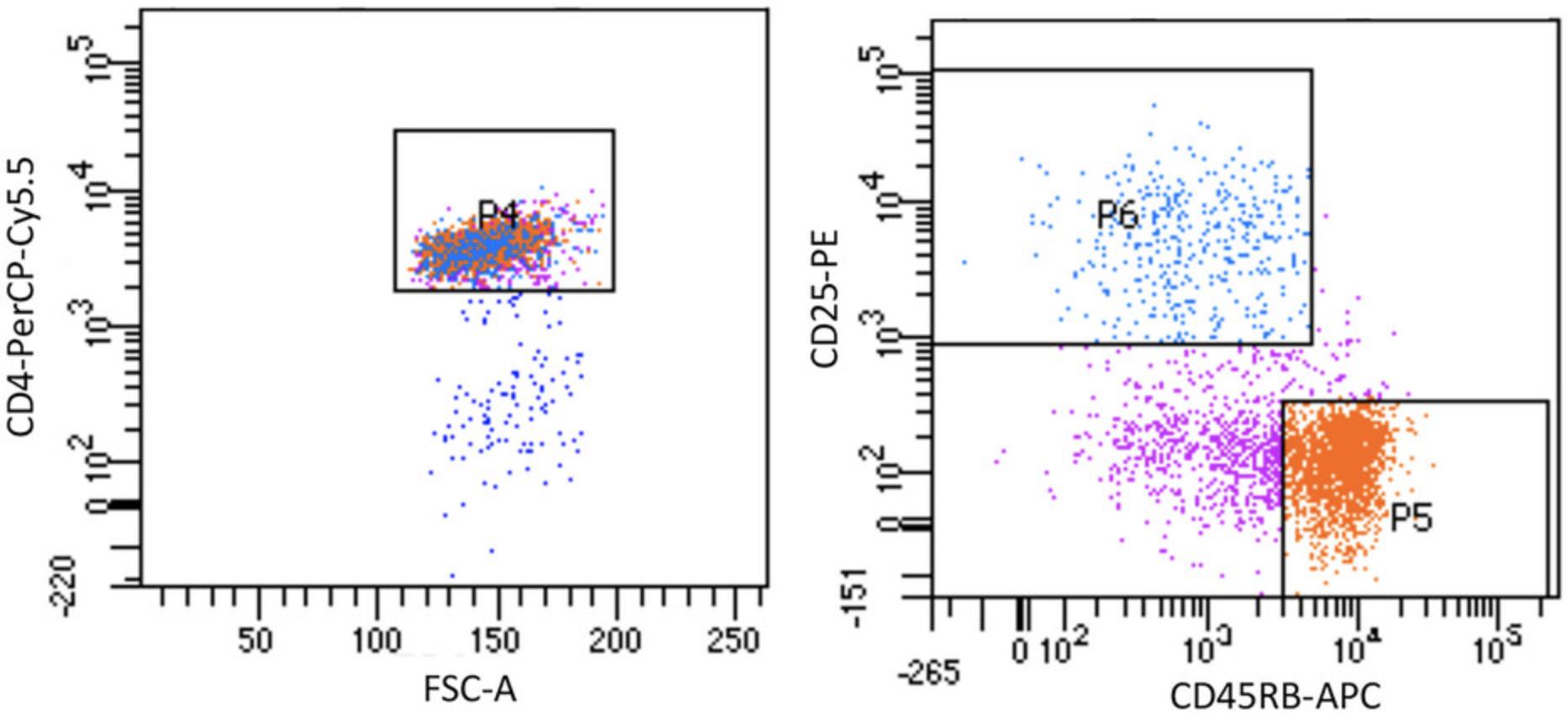
22.Pellet sorted cells (300 × g , 4°C, 5 min) in collection tubes, resuspend in 2 ml PBS/BSA/EDTA, and pool into a 50-ml centrifuge tube.
23.Count viable cells by trypan blue exclusion.
24.Remove 1 × 105 cells from each sorted population and re-analyze on the cell sorter to assess the purity of the sorted T cells.
Inject immunodeficient recipients
25.Pellet sorted cells from step 22 (300 × g , 4°C, 5 min). Resuspend the CD4+CD45RBhi (naïve) T cells at 4 × 106/ml in PBS, and resuspend the CD4+CD25hiCD45RBlo (regulatory) T cells (Tregs) at 2 × 106/ml.
26.To induce colitis in recipient mice, take 4 × 105 CD4+CD45RBhi T cells (equivalent to 100 μl of cell suspension) for each immunodeficient recipient to be restored with T cells and dilute the cell suspension with an equal volume of PBS; then inject the cells (now suspended in 200 μl volume) intraperitoneally (Donovan & Brown, 2006a) into female C57BL/6J Rag1 KO mice.
27.Mark mice for unique identification by hole punching the ears or other method of identification (Donovan & Brown, 2006b).
28.Weigh mice immediately following T cell transfers, and then at least weekly thereafter, using a top-pan balance.
Support Protocol: MONITORING DEVELOPMENT OF COLITIS
Development of colitis is accompanied by progressive weight loss and the onset and persistence of loose stools containing a high proportion of mucus. Weight loss can be variable depending on the microbiota and can be cage dependent. Recipient mice usually stop gaining weight in the first 2 weeks after T cell transfer and then lose weight over the next 2-6 weeks. At this time point, mice may undergo a rapid decline in health that requires close monitoring to allow cell harvest and other investigation prior to demise or recovery by injection of Tregs. The presence of colitis is identified by histological analysis of the colon. Characteristic lesions include a mononuclear cell infiltrate into the mucosa and muscularis, epithelial cell hyperplasia, ulceration, and depletion of mucin-secreting goblet cells.
Materials
-
Female C57BL/6J Rag1 –/– mice that have received adoptively transferred CD4+CD45RBhi T cells, weighed weekly (see Basic Protocol)
-
Phosphate-buffered saline (PBS), ice cold
-
10% (v/v) buffered formalin (Baxter)
-
Hematoxylin counterstain (Mayer's; Merck)
-
Dissecting instruments
-
19-G blunted-ended needle
-
Tissue-processing histology cassettes (Fisher Scientific)
-
Histology pencil (Fisher Scientific)
-
Additional reagents and equipment for CO2 euthanasia (Donovan & Brown, 2006c) and (optional) paraffin embedding, sectioning, and hematoxylin staining (Hofman & Taylor, 2013)
1.When T cell–transferred mice develop loose stools or lose 10%-15% of their initial body weight, euthanize in an atmosphere of 100% CO2 (Donovan & Brown, 2006c).
2.Open the abdomen, cut the colon below the cecum and just above the anus, and transfer the colon to ice-cold PBS. Using a 19-G blunt-ended needle, wash out the contents of the lumen with PBS.
3.Cut a small portion (∼1 cm) of each of the ascending (proximal), transverse (mid), and descending (distal) colon and place into a histology cassette. Assign each sample a code and label using a histology pencil. Fix in 10% buffered formalin for ≥24 hr.
4.Paraffin embed samples and cut two to three 5-μm transverse sections per sample. Mount on microscope slides and stain with hematoxylin counterstain.
5.Analyze stained sections by light microscopy to determine the extent of intestinal inflammation. Grade each pathological parameter semi-quantitatively on a scale from 0 (no change) to 3 (most severe) as described in Table 1, giving a total score for each section of 0-12.
Epithelium | Hyperplasia and/or | Goblet cell depletion |
0 | None | None |
1 | Mild (1.5× longer) | Mild (25%) |
2 | Moderate (2-3× longer) | Marked (25%-50%) |
3 | Severe (>3× longer) | Substantial (>50%) |
Inflammation in lamina propria | ||
0 | None—few leukocytes | |
1 | Mild—some increase in leukocytes at tips of crypts OR many lymphoid follicles | |
2 | Moderate—marked infiltrate (notable broadening of crypt) | |
3 | Severe—dense infiltrate throughout | |
Area affected (% of section) | ||
0 | None | |
1 | Up to 25% | |
2 | 25%-50% | |
3 | More than 50% | |
Markers of severe inflammation | ||
0 | None | |
1 | Submucosal inflammation OR few crypt abscesses (<5) | |
2 | Submucosal inflammation AND few crypt abscesses (<5) | |
2 | Many crypt abscesses (>5) OR extensive submucosal inflammation | |
3 | Many crypt abscesses (>5) AND extensive submucosal inflammation | |
3 | Ulceration OR extensive fibrosis |
REAGENTS AND SOLUTIONS
PBS/BSA(/EDTA)
Prepare a 1:10 dilution of 1% BSA stock solution (Sigma, Fraction V) in phosphate-buffered saline (PBS). For PBS/BSA/EDTA, add 10 mM EDTA. Sterilize by filtration with a 0.2-μm filter unit (Corning) and store at 4°C for up to 1 month.
COMMENTARY
Background Information
Transfer of naïve CD4+CD45RBhi T cells isolated from the spleen of normal mice to co-isogenic SCID or Rag–/– mice leads to the development of IBD in the recipients (Morrissey et al., 1993; Powrie et al., 1993). Intestinal lesions, which extend diffusely from the cecum to the rectum, are characterized by extensive leukocytic infiltrates (in some cases granulomatous in nature and composed primarily of macrophages and CD4+ T cells), epithelial cell hyperplasia with glandular elongation, ulceration, and loss of mucin-secreting goblet cells. In addition to intestinal inflammation, some mice also develop gastritis, hepatitis, myocarditis, and inflammation in the lung (Leach et al., 1996).
IBD induced in T-cell-deficient mice by transfer of CD45RBhiCD4+ T cells involves activation of Th1 cells, and disease is ameliorated by treatment with anti-IFN-γ mAb (Powrie et al., 1994). Subsequent studies demonstrated that CD45RBhiCD4+ T cell transfer IBD is dependent on the production of IL-23 and that this cytokine (in association with IL-12) acts on CD4+ T cells to promote pathogenic Th1 cell and Th17 cell responses in the gut (Ahern et al., 2010; Izcue et al., 2008; Kullberg et al., 2006). In addition, there is marked accumulation of activated monocytes and neutrophils in the inflamed colon, and dysregulated hematopoiesis contributes to pathogenesis (Griseri et al., 2012).
The contemporaneous discovery that IL23R gene polymorphisms were associated with increased risk of IBD in humans (Duerr et al., 2006) further validated the relevance of this preclinical mouse model for human IBD. Accordingly, recent clinical trials have shown that antibodies targeting IL-23 can reduce disease in many human IBD patients (McDonald et al., 2022).
Intestinal bacteria play a critical role in the pathogenesis of disease as colitis failed to develop in CD4+CD45RBhi T cell–restored immunodeficient mice raised under germ-free conditions (Aranda et al., 1997; Powrie & Leach, 1995). Evidence suggests that IBD develops as a result of an abnormal immune response to commensal bacteria (Maloy & Powrie, 2011), and several studies have highlighted the effect that particular bacteria can have on the disease process. For example, experimental infection with the gram-negative bacterium Helicobacter hepaticus leads to development of mild to moderate typhlocolitis in Rag-deficient mice, even in the absence of T cells (Maloy et al., 2003). However, severe disease, often including rectal prolapse, was observed in the presence of both CD4+CD45RBhi T cells and H. hepaticus infection, indicating that an abnormal immune response in the presence of a single enteric pathogen can lead to very severe immune pathology (Cahill et al., 1997; Maloy et al., 2003). A recent study using a similar naïve CD4+ T cell adoptive transfer colitis model provided compelling evidence that cognate recognition of microbiota-derived antigens drives the expansion of colitogenic CD4+ T cells in the intestine (Muschaweck et al., 2021). Using TCR sequencing, the authors observed the accumulation of a few dominant clonotypes in each Rag–/– recipient that were shared between Th1 and Th17 effector subsets. In addition, modulation of the microbiota using antibiotics correlated with changes in the colonic T cell repertoire, suggesting that recognition of specific microbiota components was responsible for expansion of dominant T cell clones (Muschaweck et al., 2021).
IBD induced by transfer of CD4+CD45RBhi T cells can be inhibited in a dose-dependent fashion by co-transfer of the reciprocal CD4+CD25hiCD45RBlo T cell population, showing that Treg cells contained within this population inhibit the development of pathogenic inflammatory responses in the intestine (Powrie et al., 1993). Transfer of the CD4+CD25hiCD45RBlo T cell population can also be used to “cure” established colitis in mice that were previously injected with CD4+CD45RBhi T cells (Mottet et al., 2003).
The immune-suppressive properties of CD4+CD25hiCD45RBlo Treg cells were shown to involve the production of transforming growth factor beta (TGF-β), which acts on donor T cells to suppress the accumulation of pathogenic CD4+ T effector cells in the gut (Fahlen et al., 2005). The CD4+CD25hiCD45RBlo Treg cells express the co-inhibitory receptor CTLA-4, which is also required for their suppression of colitis (Read et al., 2006). Additional factors that potentiate the suppressive function of intestinal CD4+CD25hiCD45RBlo Treg cells have been identified, including the alarmin IL-33 that is released by epithelial cells during tissue damage (Schiering et al., 2014).
Taken together, these studies highlight the utility of the CD4+CD45RBhi T cell transfer model of IBD for the identification of cellular, molecular, and environmental factors that contribute to the induction or suppression of chronic intestinal inflammation, which has opened new avenues for therapeutic intervention in human IBD.
Critical Parameters and Troubleshooting
Successful induction and/or regulation of intestinal disease in the cell transfer model described here is dependent on several factors. One factor of particular importance is the purity of the CD4+CD45RBhi T cells and CD4+CD25+CD45RBlo Treg cells being transferred, as contamination of the CD4+CD45RBhi T cell population with as few as 10%-15% CD4+CD25+CD45RBlo T cells can lead to inhibition of disease. For this reason, cell sorting is recommended for preparation of T cell subpopulations, using a program that utilizes both the CD45RB and CD25 cell-surface markers to allow mutual exclusion of cells with one or the other marker (Fig. 1).
Specific-pathogen-free (SPF) mice are also essential for the successful use of this model. This is evident from the fact that the presence of pathogens in either the donor or recipient mice can significantly alter the results obtained. If Rag-deficient mice rapidly develop wasting disease (i.e., within 2 weeks after CD4+CD45RBhi T cell transfer) or there is significant mortality in the experimental groups, suspect the presence of pathogens such as Pneumocystis carinii or mouse hepatitis virus. Often Rag-deficient mice can harbor pathogens without displaying clinical signs; however, transfer of donor T cells, particularly the CD4+CD45RBhi T subset, leads to activation of an immune response that induces immune pathology (Roths & Sidman, 1992). To minimize the chances of infection, the authors house their colonies in individually ventilated cages, which provide a source of high-efficiency particulate air (HEPA)-filtered air to individual cages.
As noted above, the presence of H. hepaticus in the recipient Rag-deficient mice can have a dramatic exacerbating effect on disease severity. Although H. hepaticus is not a bona fide pathogen of normal immune-competent mice, most animal facilities now include H. hepaticus screening as part of their regular monitoring. There are several related intestinal Helicobacter spp. that have been associated with intestinal inflammation in rodents, and it is therefore recommended that recipient Rag-deficient mice are screened for the presence of intestinal Helicobacter spp. (Feng et al., 2005).
Mouse age may also play a role in disease induction, and recipient mice older than 16 weeks at transfer are less susceptible to developing colitis. In addition, the of use mice younger than 6 weeks old at transfer is not recommended due to their smaller starting weight.
Protection from and cure of colitis in experiments may require some optimization of cell numbers. The number of CD4+CD25+CD45RBlo Treg cells required to block disease may alter depending on the local microenvironment and how quickly disease develops in normal circumstances. More Treg cells may be required in facilities where colitis occurs faster. It is important to keep cells on ice when not actually being sorted, especially when sorting a large number of Treg cells, as long delays before injection can reduce colitis induction efficacy.
Understanding Results
Rag–/– recipient mice stop gaining weight about 2 weeks after reconstitution with CD4+CD45RBhi T cells. By 3 weeks they start to lose weight and develop loose stools. Weight loss is progressive, and by 6-8 weeks mice in this group can have lost up to 15%-20% of their body weight, although it is possible for some mice to maintain weight but still develop disease. In contrast, Rag–/– mice restored with a mixture of CD4+CD45RBhi T cells and CD4+CD25+CD45RBlo Treg cells gain weight and thrive throughout the course of the experiment (Fig. 2). Upon necropsy, ∼80% of Rag–/– mice that received CD4+CD45RBhi T cells exhibit a thickened colon that is visible macroscopically. Microscopic analysis reveals inflammation and epithelial cell hyperplasia that affects the entire circumference of the bowel wall (Fig. 3) and is present diffusely from cecum to rectum. The majority of mice in this group develop colitis that is scored between 5 and 12 (Table 1). In contrast, colons from Rag–/– mice successfully restored with a mixture of CD4+CD45RBhi T cells and CD4+CD25+CD45RBlo Treg cells generally look indistinguishable from normal C57BL/6J mice, or show minimal signs of pathology (Fig. 3).
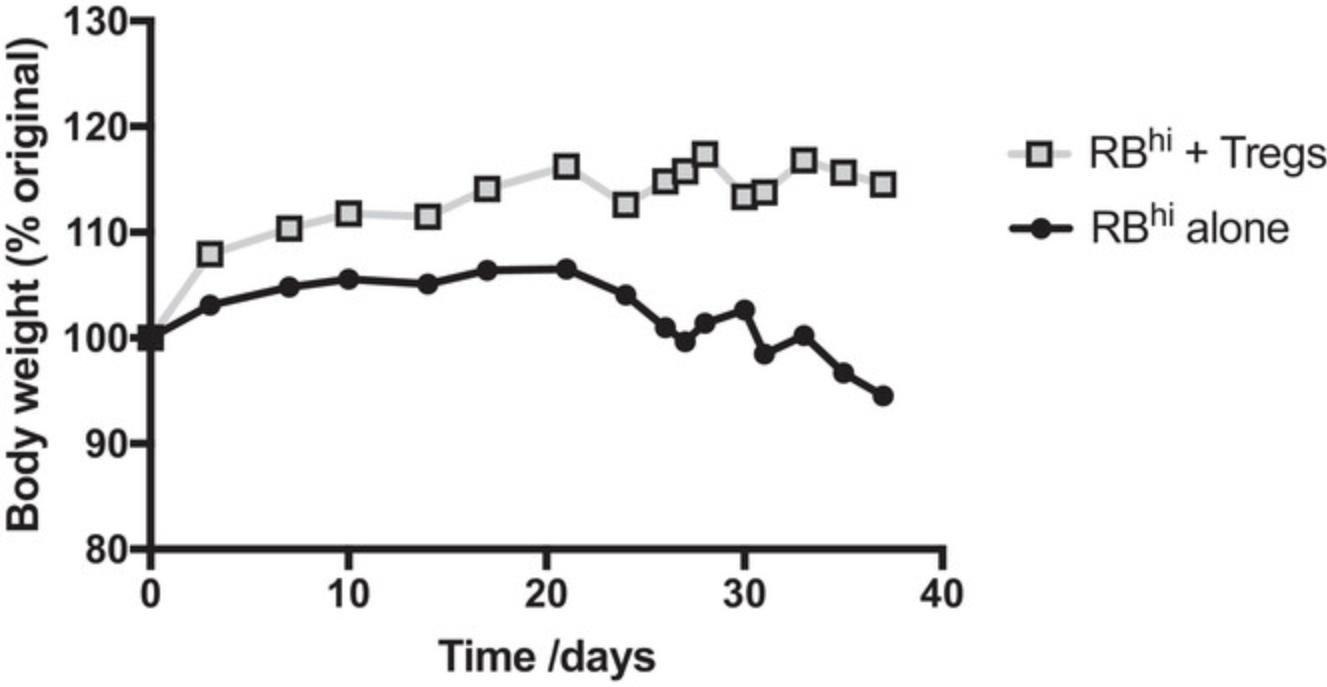
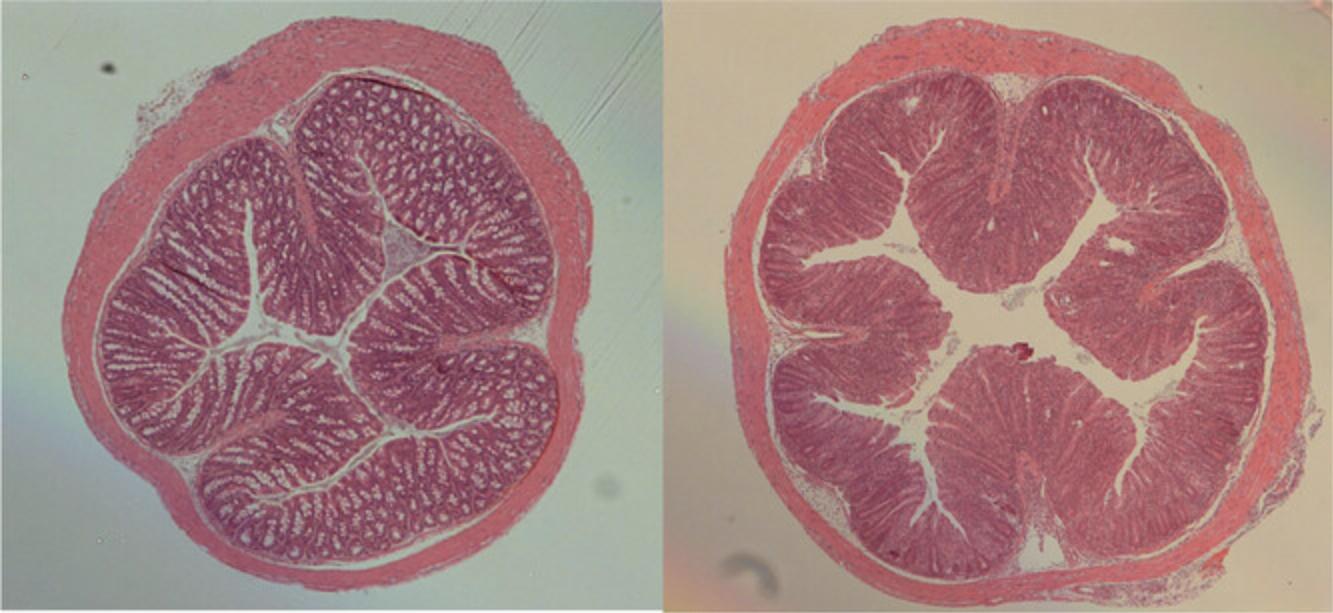
Time Considerations
The preparation of CD4+CD45RBhi T cells and CD4+CD25+CD45RBlo Treg cells typically takes a full day. It takes ∼2 hr to prepare an enriched CD4+ population from whole spleens. Staining the cells for cell sorting will take 1 hr. The time required to sort cells is dependent upon the sort rate and proportion of events falling in the required sort gates; however, as a rough guide, sorting 107 CD4+CD45RBhi T cells at 5000 events/s will take 2-3 hr. Sorted cells can be pooled as the sort progresses. Time should also be left for reanalysis (30 min) and injection of the sorted CD4+ subpopulations into recipients (1 hr).
Acknowledgments
KJM is supported by the Wellcome Trust (Investigator Award 102972/Z/13/Z) and by the MRC (Project Grant MR/X002004/1). CFP is supported by the Kennedy Trust (KENN202115) and by the Wellcome Trust (Investigator Award 212240/Z/18/Z awarded to Fiona Powrie). We would like to thank the original authors, Simon Read and Fiona Powrie, for their original article and the contributions of that article to this updated version.
Author Contributions
Claire F. Pearson: Writing—original draft; writing—review and editing. Kevin J. Maloy: Writing—original draft; writing—review and editing.
Conflict of Interest
The authors declare no conflicts of interest.
Open Research
Data Availability Statement
Data sharing not applicable—no new data generated.
Literature Cited
- Ahern, P. P., Schiering, C., Buonocore, S., McGeachy, M. J., Cua, D. J., Maloy, K. J., & Powrie, F. (2010). Interleukin-23 drives intestinal inflammation through direct activity on T cells. Immunity , 33(2), 279–288. https://doi.org/10.1016/j.immuni.2010.08.010
- Aranda, R., Sydora, B. C., McAllister, P. L., Binder, S. W., Yang, H. Y., Targan, S. R., & Kronenberg, M. (1997). Analysis of intestinal lymphocytes in mouse colitis mediated by transfer of CD4+, CD45RBhigh T cells to SCID recipients. Journal of Immunology , 158(7), 3464–3473. https://www.ncbi.nlm.nih.gov/pubmed/9120308
- Cahill, R. J., Foltz, C. J., Fox, J. G., Dangler, C. A., Powrie, F., & Schauer, D. B. (1997). Inflammatory bowel disease: An immunity-mediated condition triggered by bacterial infection with Helicobacter hepaticus. Infection and Immunity , 65(8), 3126–3131. https://doi.org/10.1128/iai.65.8.3126-3131.1997
- Donovan, J., & Brown, P. (2006a). Parenteral injections. Current Protocols in Immunology , 73, 1.6.1–1.6.10. https://doi.org/10.1002/0471142735.im0106s73
- Donovan, J., & Brown, P. (2006b). Animal identification. Current Protocols in Immunology , 73, 1.5.1–1.5.4. https://doi.org/10.1002/0471142735.im0105s73
- Donovan, J., & Brown, P. (2006c). Euthanasia. Current Protocols in Immunology , 73, 1.8.1–1.8.4. https://doi.org/10.1002/0471142735.im0108s73
- Donovan, J., & Brown, P. (2007). Managing immunocompromised animals. Current Protocols in Immunology , 77, 1.2.1–1.2.5. https://doi.org/10.1002/0471142735.im0102s77
- Duerr, R. H., Taylor, K. D., Brant, S. R., Rioux, J. D., Silverberg, M. S., Daly, M. J., Steinhart, A. H., Abraham, C., Regueiro, M., Griffiths, A., Dassopoulos, T., Bitton, A., Yang, H., Targan, S., Datta, L. W., Kistner, E. O., Schumm, L. P., Lee, A. T., Gregersen, P. K., … Cho, J. H. (2006). A genome-wide association study identifies IL23R as an inflammatory bowel disease gene. Science , 314(5804), 1461–1463. https://doi.org/10.1126/science.1135245
- Fahlen, L., Read, S., Gorelik, L., Hurst, S. D., Coffman, R. L., Flavell, R. A., & Powrie, F. (2005). T cells that cannot respond to TGF-beta escape control by CD4+CD25+ regulatory T cells. Journal of Experimental Medicine , 201(5), 737–746. https://doi.org/10.1084/jem.20040685
- Feng, S., Ku, K., Hodzic, E., Lorenzana, E., Freet, K., & Barthold, S. W. (2005). Differential detection of five mouse-infecting helicobacter species by multiplex PCR. Clinical and Diagnostic Laboratory Immunology , 12(4), 531–536. https://doi.org/10.1128/CDLI.12.4.531-536.2005
- Griseri, T., McKenzie, B. S., Schiering, C., & Powrie, F. (2012). Dysregulated hematopoietic stem and progenitor cell activity promotes interleukin-23-driven chronic intestinal inflammation. Immunity , 37(6), 1116–1129. https://doi.org/10.1016/j.immuni.2012.08.025
- Hofman, F. M., & Taylor, C. R. (2013). Immunohistochemistry. Current Protocols in Immunology , 103, 21.4.1–21.4.26. https://doi.org/10.1002/0471142735.im2104s103
- Holmes, K., Lantz, L. M., Fowlkes, B. J., Schmid, I., & Giorgi, J. V. (2001). Preparation of cells and reagents for flow cytometry. Current Protocols in Immunology , 44, 5.3.1–5.3.24. https://doi.org/10.1002/0471142735.im0503s44
- Horgan, K., Shaw, S., & Boirivant, M. (2009). Immunomagnetic purification of T cell subpopulations. Current Protocols in Immunology , 85, 7.4.1–7.4.9. https://doi.org/10.1002/0471142735.im0704s85
- Izcue, A., Hue, S., Buonocore, S., Arancibia-Carcamo, C. V., Ahern, P. P., Iwakura, Y., Maloy, K. J., & Powrie, F. (2008). Interleukin-23 restrains regulatory T cell activity to drive T cell-dependent colitis. Immunity , 28(4), 559–570. https://doi.org/10.1016/j.immuni.2008.02.019
- Kruisbeek, A. M. (2001). Isolation of mouse mononuclear cells. Current Protocols in Immunology , 39, 3.1.1–3.1.5. https://doi.org/10.1002/0471142735.im0301s39
- Kuhn, R., Lohler, J., Rennick, D., Rajewsky, K., & Muller, W. (1993). Interleukin-10-deficient mice develop chronic enterocolitis. Cell , 75(2), 263–274. https://doi.org/10.1016/0092-8674(93)80068-p
- Kullberg, M. C., Jankovic, D., Feng, C. G., Hue, S., Gorelick, P. L., McKenzie, B. S., Cua, D. J., Powrie, F., Cheever, A. W., Maloy, K. J., & Sher, A. (2006). IL-23 plays a key role in Helicobacter hepaticus-induced T cell-dependent colitis. Journal of Experimental Medicine , 203(11), 2485–2494. https://doi.org/10.1084/jem.20061082
- Leach, M. W., Bean, A. G., Mauze, S., Coffman, R. L., & Powrie, F. (1996). Inflammatory bowel disease in C.B-17 scid mice reconstituted with the CD45RBhigh subset of CD4+ T cells. American Journal of Pathology , 148(5), 1503–1515. https://www.ncbi.nlm.nih.gov/pubmed/8623920
- Maloy, K. J., & Powrie, F. (2011). Intestinal homeostasis and its breakdown in inflammatory bowel disease. Nature , 474(7351), 298–306. https://doi.org/10.1038/nature10208
- Maloy, K. J., Salaun, L., Cahill, R., Dougan, G., Saunders, N. J., & Powrie, F. (2003). CD4+CD25+ T(R) cells suppress innate immune pathology through cytokine-dependent mechanisms. Journal of Experimental Medicine , 197(1), 111–119. https://doi.org/10.1084/jem.20021345
- McDonald, B. D., Dyer, E. C., & Rubin, D. T. (2022). IL-23 monoclonal antibodies for IBD: So many, so different? Journal of Crohn's and Colitis , 16(Supplement_2), ii42–ii53. https://doi.org/10.1093/ecco-jcc/jjac038
- McKinnon, K. M. (2018). Flow cytometry: An overview. Current Protocols in Immunology , 120, 5.1.1–5.1.11. https://doi.org/10.1002/cpim.40
- Mombaerts, P., Mizoguchi, E., Grusby, M. J., Glimcher, L. H., Bhan, A. K., & Tonegawa, S. (1993). Spontaneous development of inflammatory bowel disease in T cell receptor mutant mice. Cell , 75(2), 274–282. https://doi.org/10.1016/0092-8674(93)80069-q
- Morrissey, P. J., Charrier, K., Braddy, S., Liggitt, D., & Watson, J. D. (1993). CD4+ T cells that express high levels of CD45RB induce wasting disease when transferred into congenic severe combined immunodeficient mice. Disease development is prevented by cotransfer of purified CD4+ T cells. Journal of Experimental Medicine , 178(1), 237–244. https://doi.org/10.1084/jem.178.1.237
- Mottet, C., Uhlig, H. H., & Powrie, F. (2003). Cutting edge: Cure of colitis by CD4+CD25+ regulatory T cells. Journal of Immunology , 170(8), 3939–3943. https://doi.org/10.4049/jimmunol.170.8.3939
- Muschaweck, M., Kopplin, L., Ticconi, F., Schippers, A., Iljazovic, A., Galvez, E. J. C., Abdallah, A. T., Wagner, N., Costa, I. G., Strowig, T., & Pabst, O. (2021). Cognate recognition of microbial antigens defines constricted CD4+ T cell receptor repertoires in the inflamed colon. Immunity , 54(11), 2565–2577.e2566. https://doi.org/10.1016/j.immuni.2021.08.014
- Ostanin, D. V., Pavlick, K. P., Bharwani, S., D'Souza, D., Furr, K. L., Brown, C. M., & Grisham, M. B. (2006). T cell-induced inflammation of the small and large intestine in immunodeficient mice. American journal of physiology Gastrointestinal and liver physiology , 290(1), G109–119. https://doi.org/10.1152/ajpgi.00214.2005
- Powrie, F. (1995). T cells in inflammatory bowel disease: Protective and pathogenic roles. Immunity , 3(2), 171–174. https://doi.org/10.1016/1074-7613(95)90086-1
- Powrie, F., Carlino, J., Leach, M. W., Mauze, S., & Coffman, R. L. (1996). A critical role for transforming growth factor-beta but not interleukin 4 in the suppression of T helper type 1-mediated colitis by CD45RB(low) CD4+ T cells. Journal of Experimental Medicine , 183(6), 2669–2674. https://doi.org/10.1084/jem.183.6.2669
- Powrie, F., & Leach, M. W. (1995). Genetic and spontaneous models of inflammatory bowel disease in rodents: Evidence for abnormalities in mucosal immune regulation. Therapeutic Immunology , 2(2), 115–123. https://www.ncbi.nlm.nih.gov/pubmed/8729882
- Powrie, F., Leach, M. W., Mauze, S., Caddle, L. B., & Coffman, R. L. (1993). Phenotypically distinct subsets of CD4+ T cells induce or protect from chronic intestinal inflammation in C. B-17 scid mice. International Immunology , 5(11), 1461–1471. https://doi.org/10.1093/intimm/5.11.1461
- Powrie, F., Leach, M. W., Mauze, S., Menon, S., Caddle, L. B., & Coffman, R. L. (1994). Inhibition of Th1 responses prevents inflammatory bowel disease in scid mice reconstituted with CD45RBhi CD4+ T cells. Immunity , 1(7), 553–562. https://doi.org/10.1016/1074-7613(94)90045-0
- Read, S., Greenwald, R., Izcue, A., Robinson, N., Mandelbrot, D., Francisco, L., Sharpe, A. H., & Powrie, F. (2006). Blockade of CTLA-4 on CD4+CD25+ regulatory T cells abrogates their function in vivo. Journal of Immunology , 177(7), 4376–4383. https://doi.org/10.4049/jimmunol.177.7.4376
- Reeves, J. P., & Reeves, P. A. (1992). Removal of lymphoid organs. Current Protocols in Immunology , 1, 1.9.1–1.9.3. https://doi.org/10.1002/0471142735.im0109s01
- Roths, J. B., & Sidman, C. L. (1992). Both immunity and hyperresponsiveness to Pneumocystis carinii result from transfer of CD4+ but not CD8+ T cells into severe combined immunodeficiency mice. Journal of Clinical Investigation , 90(2), 673–678. https://doi.org/10.1172/JCI115910
- Rudolph, U., Finegold, M. J., Rich, S. S., Harriman, G. R., Srinivasan, Y., Brabet, P., Bradley, A., & Birnbaumer, L. (1995). Gi2 alpha protein deficiency: A model of inflammatory bowel disease. Journal of Clinical Immunology , 15(6 Suppl), 101S–105S. https://doi.org/10.1007/BF01540899
- Sadlack, B., Merz, H., Schorle, H., Schimpl, A., Feller, A. C., & Horak, I. (1993). Ulcerative colitis-like disease in mice with a disrupted interleukin-2 gene. Cell , 75(2), 253–261. https://doi.org/10.1016/0092-8674(93)80067-o
- Schiering, C., Krausgruber, T., Chomka, A., Frohlich, A., Adelmann, K., Wohlfert, E. A., Pott, J., Griseri, T., Bollrath, J., Hegazy, A. N., Harrison, O. J., Owens, B. M. J., Lohning, M., Belkaid, Y., Fallon, P. G., & Powrie, F. (2014). The alarmin IL-33 promotes regulatory T-cell function in the intestine. Nature , 513(7519), 564–568. https://doi.org/10.1038/nature13577
- Strober, W. (2015). Trypan blue exclusion test of cell viability. Current Protocols in Immunology , 111, A3.B.1–A3.B.3. https://doi.org/10.1002/0471142735.ima03bs111