Stage-Specific Generation of Human Pluripotent Stem Cell Derived Lung Models to Measure CFTR Function
Shuk Yee Ngan, Shuk Yee Ngan, Henry T. Quach, Henry T. Quach, Onofrio Laselva, Onofrio Laselva, Elena N. Huang, Elena N. Huang, Maria Mangos, Maria Mangos, Sunny Xia, Sunny Xia, Christine E. Bear, Christine E. Bear, Amy P. Wong, Amy P. Wong
Abstract
Human embryonic stem cells (ES) and induced pluripotent stem cells (iPSC) are powerful tools that have the potential to generate in vitro human lung epithelial cells. However, challenges in efficiency and reproducibility remain in utilizing the cells for therapy discovery platforms. Here, we optimize our previously published protocols to efficiently generate three developmental stages of the lung model (fetal lung epithelial progenitors, fLEP; immature airway epithelial spheroid, AES; air-liquid interface culture, ALI), and demonstrate its potential for cystic fibrosis (CF) drug discovery platforms. The stepwise approach directs differentiation from hPSC to definitive endoderm, anterior ventral foregut endoderm, and fetal lung progenitor cells. The article also describes the generation of immature airway epithelial spheroids in Matrigel with epithelial cells sorted by a magnetic-activated cell sorting system, and the generation of adult-like airway epithelia through air-liquid interface conditions. We demonstrate that this optimized procedure generates remarkably higher cystic fibrosis transmembrane conductance regulator (CFTR) expression and function than our previous method, and thus is uniquely suitable for CF research applications. © 2022 The Authors. Current Protocols published by Wiley Periodicals LLC.
Basic Protocol 1 : hESC/hiPSC differentiation to fetal lung progenitors
Basic Protocol 2 : Formation of airway epithelial spheroids
Alternate Protocol 1 : Cryopreservation of airway epithelial spheroids
Basic Protocol 3 : Differentiation and maturation in air-liquid interface culture
Alternate Protocol 2 : Differentiation and maturation of epithelial progenitors from airway epithelial spheroids in ALI culture
INTRODUCTION
Human pluripotent stem cells (hPSCs) have revolutionized the generation of organ-specific cells for drug discovery and disease modeling (Aurora & Spence, 2016; Kolios & Moodley, 2013). Pluripotent stem cells (PSC, including embryonic and induced) are defined by their functional ability to self-renew indefinitely and their capacity to differentiate into any cell type in the embryo proper (Takahashi et al., 2007; Thomson et al., 1998). Cellular differentiation is the process by which stem cells acquire more specialized phenotype and function such that with each successive stage of lineage differentiation, the cells lose their capacity to become other cell types. Protocols to generate robust lung epithelial cell types from human PSC have now been established by our lab and others (H. J. Chen et al., 2019; Dye et al., 2015; Hawkins et al., 2021; Magro-Lopez, Palmer, Manso, Liste, & Zambrano, 2018; McCauley et al., 2017; Miller et al., 2018; Rodrigues Toste de Carvalho et al., 2021; Wong et al., 2012, 2015). Generation of patient-specific cells offers an incredible opportunity to model patient-specific differences and responses.
Many of the directed differentiation protocols to generate human lung epithelial cells are based on a few founding protocols and generally involve stage-specific signaling of cells modeling developmental trajectories (Y.-W. Chen et al., 2017; Hawkins et al., 2021; S. X. L. Huang et al., 2014; Jacob et al., 2017; McCauley et al., 2017; Wong et al., 2012). Each stage of differentiation relies on efficient generation of the cells from definitive endoderm (D'Amour et al., 2005; Wong et al., 2015), to anterior ventral foregut endoderm (Green et al., 2011), to primitive fetal lung cells expressing NKX2-1 (S. X. L. Huang et al., 2014; McCauley et al., 2017), to multipotent lung progenitors (Hawkins et al., 2021), and finally to maturation in air liquid interface (ALI) (Hawkins et al., 2021; McCauley et al., 2017; Wong et al., 2015). As such, hPSCs are a great resource of cells to model developmental (Hurley et al., 2020; McCauley et al., 2017; Miller et al., 2020), genetic (Y.-W. Chen et al., 2017; Wong et al., 2012, 2015), and acquired human lung diseases (Huang et al., 2020; Porotto et al., 2019), and have potential uses in airway regeneration (Shafa et al., 2018; Shojaie et al., 2015). A caveat of current developments in using hPSC-derived cells for therapy discovery is the inability to generate reproducible cells for testing. This is true for cystic fibrosis (CF), an autosomal recessive genetic disease induced by mutations on the cystic fibrosis transmembrane conductance regulator (CFTR) gene that encodes CFTR protein. Therefore, a differentiation protocol optimized for high CFTR expression and models that can measure CFTR protein function is ideal. Here we show a unique use of human PSC-derived stage-specific lung epithelial cells in in vitro testing of cystic fibrosis transmembrane conductance regulator (CFTR) function as a means for rapid testing of potential modulators and facilitating therapeutic discovery. Generating predictive cell-based models is important for advancement in precision medicine.
This article describes a detailed approach to generating 2D and 3D lung epithelial models for studying CFTR transport activity. Specifically, we focus on derivation of renewable fetal lung epithelial progenitors (fLEP), immature airway epithelial spheroids (AES), and mature airway epithelial cells in ALI. Incorporating some recent improvements in lung differentiation protocols, our new protocol generates epithelial cells with greater expression of CFTR and higher CFTR-specific function compared to our previous method (Wong et al., 2015).
Our new differentiation protocol enables testing of new CFTR modulators in various lung models generated from hPSC, especially in fetal lung cells that express higher abundance of CFTR for rapid high-throughput testing (Jiang et al., 2021). This is important, as reproducibility and efficiency of generating proper cells coupled with cost-efficiency of cell production without starting from iPSC every time will enable development of rapid screens for testing new and emerging compounds for CF therapy.
This article describes a detailed approach to efficient generation of anterior ventral foregut and fetal lung cells, isolation of fetal epithelial cells, generation of 3D AES, differentiation of 3D AES, and maturation in ALI conditions. It also describes the use of the different lung cells in CFTR functional analysis and the potential use of these cells in CF iPSC therapy testing. Line-to-line variability in terms of efficiency of generating fLEP is expected; however, with the sorting strategy and renewability of these cells, use of the fLEP cells should reduce batch-to-batch variability. Specific optimization of the protocol for each individual PSC line is highly recommended, as well as use of a gold-standard hPSC line in parallel to benchmark technical differences.
Basic Protocol 1: hESC/hiPSC DIFFERENTIATION TO FETAL LUNG PROGENITORS
The following protocol is designed for generating fetal lung progenitors from hESC/iPSC lines. The protocol is optimized from our previous protocol (Wong et al., 2015; Fig. 1). hPSC are induced into definitive endoderm using the STEMDiff definitive endoderm (DE) kit (following manufacturer's protocol exactly). This is followed by the anterior ventral foregut endoderm (AVFE) stage, which expresses NKX2-1 indicating lung lineages, then fetal lung progenitors (fLEP), which express high CFTR levels.
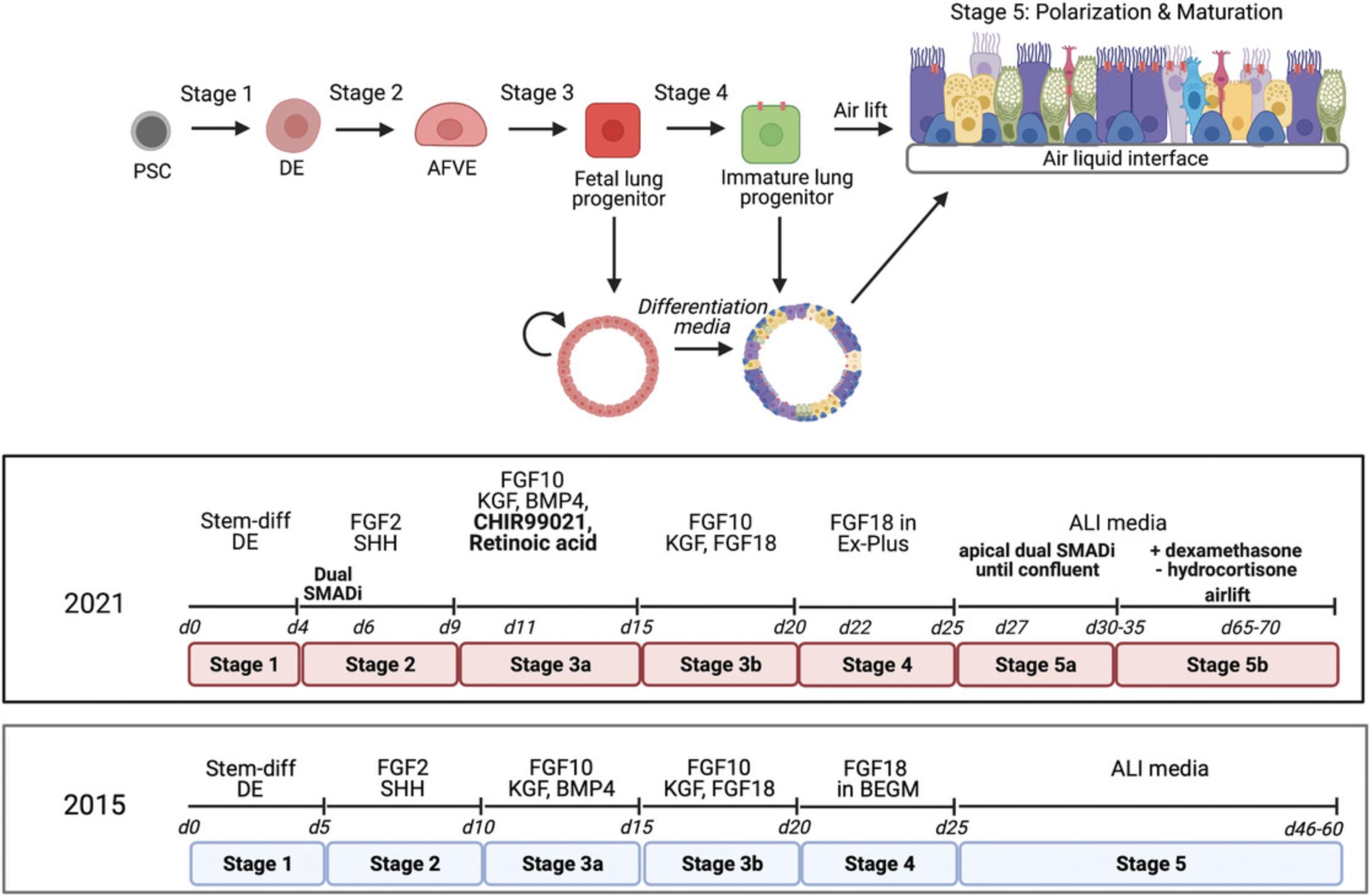
Materials
- hES-certified Matrigel™ (Corning; cat. no. 354277)
- Human embryonic stem cells/induced pluripotent stem cells (e.g., CA1/ BU3-NKX2-1GFP/P63tdTomato)
- ReLeSR™ (STEMCELL Technologies; cat. no. 05872)
- mTeSR™1 medium (STEMCELL Technologies; cat. no. 858500)
- STEMdiff™ Definitive Endoderm Kit (STEMCELL Technologies; cat. no. 05110)
- DPBS, 1×, without calcium and magnesium (Corning; cat. no. 21031CV)
- Anterior ventral foregut endoderm (AVFE) medium (see recipe)
- Dorsomorphin (STEMCELL Technologies; cat. no. 72102)
- SB431542 (STEMCELL Technologies; cat. no. 72234)
- ROCK inhibitor (Y-27632; STEMCELL Technologies; cat. no. 72304)
- Fetal lung epithelial progenitor (fLEP) medium S3A (see recipe)
- Fetal lung epithelial progenitor (fLEP) medium S3B (see recipe)
- Primers for checking gene expression level in culture (see Table 1)
- Antibodies for validating cell identity (see Table 2)
- Sterile 6-well plate (Sarstedt; cat. no. 83.3920)
Gene | Forward (5′→3′) | Reverse (5′→3′) |
---|---|---|
ASCL1 | GGGCTCTTACGACCCGCTCA | AGGTTGTGCGATCACCCTGCTT |
CCSP (SCGB1A1) | TTCAGCGTGTCATCGAAACCCC | ACAGTGAGCTTTGGGCTATTTTT |
CFTR | CTATGACCCGGATAACAAGGAGG | CAAAAATGGCTGGGTGTAGGA |
FOXA2 | GGGAGCGGTGAAGATGGA | TCATGTTGCTCACGGAGGAGTA |
FOXG1 | CTCCGTCAACCTGCTCGCGG | CTGGCGCTCATGGACGTGCT |
FOXI1 | CGGGCAAAGGGAATTACTGG | AGGCTCCATCCAAGATGTCC |
FOXJ1 | GAGCGGCGCTTTCAAGAAG | GGCCTCGGTATTCA CCGTC |
FOXP2 | AATCTGCGACAGAGACAATAAGC | TCCACTTGTTTGCTGCTGTAAA |
GAPDH | ACAACTTTGGTATCGTGGAAGG | GCCATCACGCCACAGTTTC |
KRT5 | GGAGTTGGACCAGTCAACATC | TGGAGTAGTAGCTTCCACTGC |
KRT8 | GGTGGACCCCAACATCCA | GCTGCAGGAGGCTCCACTT |
KRT13 | AGGACGCCAAGATGATTGGTT | GTGGTAACAGAGGTGCTACGG |
KRT18 | CAGAGATCGAGGCTCTCAAGGA | GTCAACCCAGAGCTGGCAAT |
MUC5ac | CCATTGCTATTATGCCCTGTGT | TGGTGGACGGACAGTCACT |
SCGB3A2 | CGGAATTCCCCAGATAACTGTCA | ACATCTAGACACCAAGTGTGATAGC |
SFPTC | CACCTGAAACGCCTTCTTATCG | TGGCTCATGTGGAGACCCAT |
SOX2 | TACAGCATGTCCTACTCGCAG | GAGGAAGAGGTAACCACAGGG |
SOX9 | GAGGAAGTCGGTGAAGAACG | CCAACATCGAGACCTTCGAT |
TFF1 | CCCCGTGAAAGACAGAATTGT | GGTGTCGTCGAAACAGCAG |
Antibodies | Isotype | Host | Company | Cat. no. or Clone ID | Dilution | Assay |
---|---|---|---|---|---|---|
Primary antibodies | ||||||
Acetylated α-tubulin | IgG | Mouse | Sigma Aldrich | T7451 | 1:200 | IF |
β-tubulin | IgG | Rabbit | Abcam | Ab15568 | 1:200 | IF |
Calnexin | IgG | Rabbit | Sigma Aldrich | C4731 | 1:2500 | WB |
CFTR | IgG2b | Mouse | courtesy of J.R. Riordan | 596 | 1:1000 | WB |
CFTR | IgG1 | Mouse | Millipore | 05-581 | 1:500 | IF |
CGRP | IgG | Mouse | Sigma | C9487 | 1:200 | IF |
ΔN P63 | IgG | Rabbit | BioLegend | 619002 | 1:200 | IF/FC |
E-cadherin | IgG | Rat | BioLegend | 147302 | 1:500 | IF |
FOXA2 | IgG | Goat | Santa Cruz | sc-6554 | 1:200 | IF |
FOXI1 | IgG | Goat | Sigma | SAB2501502 | 1:200 | IF |
FOXJ1 | IgG | Mouse | Invitrogen | 149965-82 | 1:200 | IF/FC |
KRT5 | IgG | Rabbit | Abcam | Ab53121 | 1:200 | IF |
KRT14 | IgG3 | Mouse | Abcam | ab7800 | 1:200 | IF |
KRT8/18 | IgG | Rabbit | Abcam | ab53280 | 1:200 | IF |
MUC5ac | IgG | Mouse | Thermo Scientific | MA5-12178 | 1:200 | IF |
pan-cytokeratin | IgG1 | Rabbit | Abcam | ab234297 | 1:500 | IF |
PDX1 | IgG | Mouse | Santa Cruz | sc-390792 | 1:500 | FC |
SCGB3A2 | IgG | Goat | Santa Cruz | sc-48320 | 1:200 | IF |
SOX2 | IgG | Goat | R&D | AF2018 | 1:200 | IF/FC |
SOX9 | IgG2a | Mouse | Abcam | ab79976 | 1:200 | IF |
TTF1 | IgG | Rabbit | Abcam | ab76013 | 1:100 | IF/FC |
Alexa Fluor 647 Phalloidin | Invitrogen | A22287 | 1:500 | IF | ||
ZO-1 | IgG1k | Mouse | Invitrogen | 33-9100 | 1:200 | IF |
Secondary | ||||||
Anti-rabbit IgG (H+L) Alexa Fluor 488 | IgG | Donkey | Invitrogen | A11008 | 1:500 | IF |
Anti-mouse IgG (H+L) Alexa Fluor 546 | IgG | Donkey | Invitrogen | A10036 | 1:500 | IF |
Anti-goat IgG (H+L) Alexa Fluor 568 | IgG | Donkey | Invitrogen | A11057 | 1:500 | IF |
Anti-mouse IgG (H+L) Alexa Fluor 647 | IgG | Donkey | Invitrogen | A31571 | 1:500 | IF |
Anti-mouse IgG (H+L)-HRP conjugate | IgG | Goat | BIO-RAD | 170-6516 | 1:700 | WB |
Anti-rabbit IgG (H+L)-peroxidase | IgG | Goat | Jackson ImmunoResearch | 111-035-003 | 1:5000 |
WB |
1.Prepare hES-certified Matrigel-coated 6-well plate as per manufacturer's protocol.
2.Dissociate hPSC at ∼80% confluency using ReLeSR at 37°C for 2 min.
3.Seed the cells at 1:3 dilution ratio on the Matrigel-coated plate with mTeSR1 medium.
4.Day 0 : When confluency reaches 80%, change medium to STEMdiff endoderm basal medium supplemented with CJ and MR from the STEMdiff kit according to the manufacturer's instructions.
5.Day 1-3 : Change medium with STEMdiff endoderm basal medium supplemented with CJ daily.
6.Day 4 : Remove old medium and wash with DPBS (without Ca or Mg).
7.Add AVFE medium supplemented with 2 µM dorsomorphin and 10 µM SB431542.
8.Day 6 : For ALI culture on transwell, continue differentiation according to Basic Protocol 3.For AES formation, perform medium change with AVFE medium without dorsomorphin and SB431542 every 2 days until day 10.
9.Day 10 : Perform medium change with fLEP S3A medium supplemented with 10 µM Y-27632.
10.Day 12-16 : Perform medium change with fLEP S3A medium without Y-27632 every 2 days.
11.Day 16 : Perform medium change with fLEP S3B medium supplemented with 10 µM Y-27632.
12.Day 18-21 : Perform medium change with fLEP S3B medium without Y-27632 every 2 days.
Basic Protocol 2: FORMATION OF AIRWAY EPITHELIAL SPHEROIDS
The protocol below describes airway epithelial spheroid (AES) generation from iPSC-derived fetal lung epithelial progenitors (fLEPs). A column-based magnetic-activated cell sorting (MACS) strategy is used to enrich CD326+ fetal lung epithelial cells (fLEC) and improve CFTR-expressing AES formation.
Materials
-
hPSC-derived fetal lung epithelial progenitors (fLEPs; see Basic Protocol 1)
-
TrypLE (Gibco; cat. no. 25950CQC)
-
Sorting buffer (see recipe)
-
Trypan blue solution 0.4% (Gibco; cat. no. 15250061)
-
Anti-CD326 microbeads (Human) (Miltenyi; cat. no. 130-061-101)
-
Growth factor–reduced (GFR) Matrigel™ (Corning; cat. no. 354230)
-
Airway epithelial spheroid (AES) expansion medium (see recipe)
-
Dispase (1 U/ml; STEMCELL Technologies; cat. no. 07923)
-
DMEM/F12 (Wisent; cat. no. 319-085-CL)
-
0.25% trypsin-EDTA (see Current Protocols article: Phelan & May, 2015)
-
Airway epithelial spheroid (AES) differentiation medium (see recipe)
-
Primers for checking gene expression level in culture (See Table 1)
-
Antibodies for validating cell identity (See Table 2)
-
Centrifuge
-
0.4-µm cell strainer (Falcon; cat. no. 352340)
-
Hemocytometer
-
MS Columns (Miltenyi; cat. no. 130-042-201)
-
MiniMACS™ Separator (Miltenyi; cat. no. 130-042-102)
-
MACS® MultiStand (Miltenyi; cat. no. 130-042-303)
-
24-well culture plates
-
12-well transwell plate (Costar; cat. no. C3460)
-
Permeable support for 12-well plate (Falcon; cat. No. 353180)
-
Additional reagents and equipment for general cell culture techniques including determining viable cells by trypan blue exclusion and trypsinization of cells (see Current Protocols article: Phelan & May, 2015)
1.Day 21 : Remove medium from the fetal lung epithelial progenitors (fLEPs).
2.Treat fLEPs with 1 ml of TrypLE per well for 5 min at 37°C.
3.Collect the cells in sorting buffer, and centrifuge 3 min at 300 × g , room temperature. Carefully aspirate the supernatant without disturbing the cell pellet.
4.Strain the cells using a 0.4-µm cell strainer to obtain a single-cell suspension.
5.Calculate total viable cell number using trypan blue and a hemocytometer (Phelan & May, 2015).
6.Centrifuge the fLEP cells 3 min at 300 × g , room temperature. Carefully aspirate the supernatant without disturbing the cell pellet, and resuspend the fLEP cells in CD326 microbeads (Human) as per manufacturer's protocol.
7.After 30 min of incubation on ice, add 10 ml of sorting buffer.
8.Centrifuge 10 min at 300 × g , room temperature, to remove residual antibodies. Carefully aspirate the supernatant without disturbing the cell pellet.
9.Sort for CD326+ cells using column-based MACS sorting system as per manufacturer's protocol.
10.Resuspend CD326+ cells, which are now called fetal lung epithelial cells (fLEC), in growth factor–reduced (GFR) Matrigel in a 50 µl volume at 200-400 cells per µl.
11.Seed 50 µl of GFR-Matrigel containing the cells at one drop per well on a 24-well plate (or multiple droplets per well on a 6-well plate).
12.Reverse plate and incubate at 37°C for 15 min.
13.Add warm AES expansion medium (1 ml per well on 24-well plate and 2 ml/well on 6-well plate).
14.Change the medium every other day.
15.For AES differentiation, release Stage 3 AES by removing old medium and then dissolving GFR-Matrigel with dispase (500 µl/well of a 24 well plate or 2 ml/well of a 6 well plate) at 37°C for 1 hr.
16.Collect AES in 10 ml of DMEM/F12 medium per 1 ml of dispase.
17.Centrifuge 3 min at 300 × g , room temperature. Carefully aspirate the supernatant without disturbing the cell pellet.
18.Repeat steps 16-17.
19.Dissociate AES using 1 ml of 0.25% trypsin-EDTA (Phelan & May, 2015) at 37°C for 15 min.
20.Neutralize trypsin with 1 ml of sorting buffer.
21.Strain cells with 0.4-µm strainers to improve single-cell dissociation.
22.Centrifuge 3 min at 300 × g , room temperature. Carefully aspirate the supernatant without disturbing the cell pellet.
23.Re-plate single-cell suspension at 100 cells/µl in 200 µl of a 1:1 mixture of GFR-Matrigel and AES differentiation medium to the apical membrane of a 12-well transwell plate.
24.Allow GFR-Matrigel mix with cells to solidify at 37°C for 1 hr.
25.Add 1.5 ml of AES differentiation medium to the basolateral side.
26.Perform medium change every 2 days until AES form.
Alternate Protocol 1: CRYOPRESERVATION OF AIRWAY EPITHELIAL SPHEROIDS
This alternate protocol provides instructions on how to cryopreserve and recover airway epithelial spheroids (AES) from Basic Protocol 2.
Additional Materials (also see Basic Protocols 1 and 2)
-
mFreSR™ (STEMCELL Technologies; cat. no. 05855)
-
Liquid nitrogen
-
Sterile cryovials (Thermo Fisher; cat. no. 5011-0012)
-
Mr. Frosty™ slow-cooling cryopreservation unit
-
Liquid nitrogen tank
1.Follow steps 15-18 of Basic Protocol 2 to release AES from GFR-Matrigel.
2.Resuspend 3-5 domes of AES into 0.5 ml of mFreSR™.
3.Transfer to cryovials.
4.Store in −80°C freezer with a slow-cooling system unit (e.g., Mr. Frosty™).
5.Transfer to a liquid nitrogen tank for long-term storage.
6.Thaw frozen vials in a 37°C water bath.
7.Resuspend cell/mFreSR™ mixture in 5 ml of DMEM/F12.
8.Centrifuge 5 min at 300 × g, room temperature. Carefully aspirate the supernatant without disturbing the cell pellet.
9.Resuspend in 100 µl of GFR-Matrigel, and seed two domes (see Basic Protocol 2).
10.Invert plate and incubate at 37°C for 15 min.
11.Add 1.5 ml of warm AES expansion medium supplemented with 10 µM Y-27632.
Basic Protocol 3: DIFFERENTIATION AND MATURATION IN AIR-LIQUID INTERFACE CULTURE
In this protocol, we will be generating well-differentiated airway epithelial cells under air-liquid interface (ALI) conditions by culturing AVFE (Basic Protocol 1, day 6) onto collagen-coated transwells. Our ALI protocol entails a two-step method that first allows expansion of the cells seeded onto the transwell membrane to form a monolayer of cells, and directed differentiation and polarization towards generating mature airway epithelial tissue thereafter. Further differentiation of the cells in ALI, as we previously described (Wong et al., 2012, 2015), induces maturation and polarization of the epithelium such that by the end of 5 weeks in ALI, a pseudostratified epithelium is formed, reminiscent of the large airways. This has led to an improved level of CFTR protein and measurable function (up to 40% function above baseline).
Materials
-
Collagen-IV from human placenta (Sigma Aldrich; cat. no. C7521)
-
DMEM/F12 (Wisent; cat. no. 319-085-CL)
-
AVFE (Basic Protocol 1, step 8)
-
DPBS, 1×, without calcium and magnesium (Corning; cat. no. 21031CV)
-
TrypLE (Gibco; cat. no. 25950CQC)
-
Anterior ventral foregut endoderm (AVFE) medium (see recipe)
-
ROCK inhibitor (Y-27632; STEMCELL Technologies; cat. no. 72304)
-
Fetal lung epithelial progenitor (fLEP) medium S3A (see recipe)
-
Fetal lung epithelial progenitor (fLEP) medium S3B (see recipe)
-
PneumaCult Ex Plus (STEMCELL Technologies; cat. no. 05040)
-
Recombinant FGF18 (Sigma-Aldrich; cat. no. F7301)
-
Air-liquid interface (S5A) medium (see recipe)
-
Air-liquid interface (S5B) medium (see recipe)
-
Primers for checking gene expression level in culture (See Table 1)
-
Antibodies for validating cell identity (See Table 2)
-
Biosafety cabinet
-
12-well transwell plates (Costar; cat. no. C3460) or Permeable support for 12-well plate (Falcon; cat. no. 353180)
-
Incubator (37°C, 5% CO2)
1.Day 5 : Add 150-200 µl of collagen-IV (60 µg/ml) onto the apical side of each transwell evenly. Dry in biosafety cabinet overnight (18 hr minimum).
2.Day 6 : When the transwells are completely dry, wash the transwells with DMEM/F-12 three times. Dry in biosafety cabinet for 1 hr.
3.Remove medium from anterior ventral foregut endoderm (AVFE) cells and wash with DPBS.
4.Treat cells with 1 ml of TrypLE for 5 min at 37°C.
5.Collect the cells in AVFE medium and centrifuge 5 min at 300 × g , room temperature. Carefully aspirate the supernatant without disturbing the cell pellet.
6.Resuspend the cells in AVFE medium with 10 µM Y-27632 at 2000 cells/µl.
7.Gently seed 250 µl of the cell suspension onto the apical side of each transwell evenly, and transfer seeded cells into the incubator.
8.Day 7 : Remove old medium and add 500 µl of fresh AVFE medium on the apical side. Change AVFE medium every other day until day 10.
9.Day 10 : Perform medium change with fLEP S3A medium supplemented with 10 µM Y-27632 on the apical side.
10.Day 12-16 : Perform medium change with fLEP S3A medium every 2 days on the apical side.
11.Day 16 : Perform medium change with fLEP S3B medium supplemented with 10 µM Y-27632 on the apical side.
12.Day 18-21 : Perform medium change with fLEP S3B medium every 2 days on the apical side.
13.Day 21 : Perform medium change with PneumaCult-Ex Plus medium supplemented with 10 µM Y-27632 and 10 ng/ml FGF18 on the apical side.
14.Day 23-26 : Perform medium change with PneumaCult-Ex Plus medium supplemented with 10 ng/ml of FGF18 every 2 days on the apical side.
15.Day 26 : Perform medium change with S5A medium supplemented with 10 µM Y-27632 on the apical side.
16.Day 28+ : Perform medium change with S5A every 2 days on the apical side until confluent.
17.Once confluent, remove medium from apical side and add 1.5 ml per transwell of S5B medium on the basolateral side.
18.Perform medium change with 1.5 ml per transwell of S5B medium two times per week on the basolateral side for at least 5 weeks.
Alternate Protocol 2: DIFFERENTIATION AND MATURATION OF EPITHELIAL PROGENITORS FROM AIRWAY EPITHELIAL SPHEROIDS IN ALI CULTURE
Our well-differentiated airway epithelial cells under ALI culture conditions can also be generated from CD326+ airway epithelial spheroids (AES). Similar to Basic Protocol 3, this involves dissociating AES and seeding them onto collagen-coated transwells.
Additional Materials (also see Basic Protocol 3)
- Dispase (1 U/ml; STEMCELL Technologies; cat. no. 07923)
- 0.25% trypsin-EDTA (see Current Protocols article: Phelan & May, 2015)
- Sorting buffer (see recipe)
- 0.4-µm cell strainer (Falcon; cat. no. 352340)
1.Add 150-200 µL of collagen-IV (60 µg/ml) onto the apical side of each transwell evenly. Dry in biosafety cabinet overnight (18 hr minimum).
2.When the transwells are completely dry, wash the transwells with DMEM/F-12 three times. Dry in biosafety cabinet for 1 hr.
3.Gently resuspend GFR-Matrigel-embedded AES using 1 U/ml dispase (500 µl/well of a 24-well plate or 2 ml/well of a 6-well plate) and incubate for 30 min at 37 °C.
4.Collect the AES in 10 ml of DMEM/F-12 per 1 ml of dispase and centrifuge 5 min at 300 × g, room temperature. Carefully aspirate the supernatant without disturbing the cell pellet.
5.Add 1 ml of 0.25% trypsin-EDTA to the pelleted AES and incubate for 5 min at 37°C.
6.Collect the cells in 1 ml of sorting buffer and strain cell suspension using 0.4-µm strainer.
7.Centrifuge 5 min at 300 × g, room temperature. Carefully aspirate the supernatant without disturbing the cell pellet.
8.Resuspend the cells in S5A medium with 10 µM Y-27632 at 2000 cells/µl.
9.Gently seed 250 µl of the cell suspension onto the apical side of each transwell evenly.
10.After 24 hr, remove old medium and add fresh S5A medium on the apical side. Change medium every other day for a total of 5 days.
11.When confluent, remove medium from apical chamber and add 1.5 ml per transwell of S5B medium supplemented with 10 µM Y-27632 on the basolateral side.
12.Medium change with 1.5 ml per transwell of S5B medium two times per week on the basolateral side for at least 5 weeks.
REAGENTS AND SOLUTIONS
Air-liquid interface (S5A) medium
- PneumaCult-ALI medium with supplements (no hydrocortisone added) (STEMCELL Technologies; cat. no. 05001)
- 50 nM dexamethasone (Sigma-Aldrich; cat. no. D4902)
- 10 µM SB431542 (STEMCELL Technologies; cat. no. 72234)
- 2 µM dorsomorphin (STEMCELL Technologies; cat. no. 72102)
- Store up to 2 weeks at 4°C
Air-liquid interface (S5B) medium
- PneumaCult-ALI medium with supplements (no hydrocortisone added) (STEMCELL Technologies; cat. no. 05001)
- 50 nM dexamethasone (Sigma-Aldrich; cat. no. D4902)
- Store up to 2 weeks at 4°C
Airway epithelial spheroid (AES) differentiation medium
- 500 ml Pneumacult-ALI Complete Medium (STEMCELL Technologies; cat. no. 05001; as per manufacturer's protocol) supplemented with:
- 10 µM SB431542 (STEMCELL Technologies; cat. no. 72234)
- 3 µM CHIR99021 (STEMCELL Technologies; cat. no. 72054)
- 10 µM Y-27632 (STEMCELL Technologies; cat. no. 72304)
- 10 µM DAPT (Abcam; cat. no. ab120633)
- 10 ng/ml recombinant FGF10 (Peprotech; cat. no.100-26)
- 10 ng/ml recombinant FGF7 (KGF, Peprotech; cat. no.100-19)
- 10 ng/ml recombinant FGF18 (Sigma-Aldrich; cat. no. F7301)
- 1 µM forskolin (STEMCELL Technologies; cat. no. 100-0249)
- 1 µM all-trans retinoic acid (Millipore Sigma-Aldrich; cat. no. R2625)
- 2 µM IBMX (Millipore Sigma-Aldrich; cat. no. I5879)
- Store up to 2 weeks at 4°C away from light
Airway epithelial spheroid (AES) expansion medium
- PneumaCult-Ex Plus (STEMCELL Technologies; cat. no. 05040)
- 1 µM A83-01 (STEMCELL Technologies; cat. no. 72022)
- 1 µM DMH1 (STEMCELL Technologies; cat. no. 73632)
- Store up to 1 month at 4°C
Anterior ventral foregut endoderm (AVFE) medium
- 450 mL KnockOut DMEM (Gibco; cat. no.10829-018)
- 10% KnockOut serum replacement (KOSR; Gibco; cat. no. 10828-028)
- 1% penicillin/streptomycin (Gibco; cat. no. 15-140-122)
- 2 mM Glutamax (Gibco; cat. no. 35050-061)
- 0.15 mM 1-mono-thioglycerol (MTG; Sigma-Aldrich; cat. no. M6145)
- 1 mM non-essential amino acid (Gibco; cat. no. 11140-050)
- 500 ng/ml recombinant fibroblast growth factor 2 (Peprotech; cat. no.100-18B)
- 50 ng/ml recombinant sonic hedgehog (Peprotech; cat. no. 100-45)
- Store up to 2 months at 4°C
Collagen IV solution
Dissolve collagen IV powder in 0.02 N glacial acetic acid at 600 µg/ml. Filter the solution prior to freezing. Small aliquots can be stored at −20°C for 6 months.
Complete serum-free differentiation basal medium (cSFDM)
- 75% IMDM (Gibco; cat. no. 12440053)
- 25% Ham's F12 (Gibco; cat. no. 11765062)
- 1% B-27 supplement (Invitrogen; cat. no. 17504-44)
- 0.5% N-2 supplement (Invitrogen; cat. no. 17502-048)
- 0.05% BSA (Invitrogen; cat. no. 15260-037)
- 2 mM Glutamax (Gibco; cat. no. 35050-061)
- 50 µg/ml ascorbic acid (Sigma-Aldrich; cat. no. A4544)
- 0.15 mM 1-mono-thioglycerol (MTG; Sigma-Aldrich; cat. no. M6145)
- 1% penicillin/streptomycin (Gibco; cat. no. 15-140-122)
- Store up to 1 month at 4°C
Fetal lung epithelial progenitor (fLEP) medium S3A
- cSFDM (see recipe) supplemented with:
- 10 ng/ml recombinant FGF7 (KGF, Peprotech; cat. no.100-19)
- 50 ng/ml recombinant FGF10 (Peprotech; cat. no.100-26)
- 10 ng/ml recombinant bone morphometric protein-4 (BMP4; R&D Systems; cat. no.314-BP)
- 3 µM CHIR99021 (STEMCELL Technologies; cat. no.72054)
- 100 nM all-trans retinoic acid (Sigma-Aldrich; cat. no. R2625)
- Store up to 2 weeks at 4°C away from light
Fetal lung epithelial progenitor (fLEP) medium S3B
- cSFDM (see recipe) supplemented with:
- 50 ng/ml recombinant FGF7 (KGF, Peprotech; cat. no.100-19)
- 50 ng/ml recombinant FGF10 (Peprotech; cat. no.100-26)
- 10 ng/ml recombinant FGF18 (Sigma-Aldrich; cat. no. F7301)
- Store up to 2 weeks at 4°C
Sorting buffer
- Dulbecco's phosphate-buffered saline (DPBS; without magnesium and calcium; Corning; cat. no. 21031CV)
- 2% fetal bovine serum (Wisent Bioproducts; cat. no. 080150)
- Store up to 3 months at 4°C
COMMENTARY
Background Information
Previously, we developed a protocol to generate CFTR-functional mature air-liquid interface (ALI) cultures (Wong et al., 2015). Here, we present an improved hPSC directed differentiation protocol capable of generating airway epithelial spheroids (AES) and a mature ALI with increased CFTR function more efficiently than before. In comparison to our previous protocols, each stage of differentiation is optimized to improve lung lineages and CFTR function. At the fetal lung epithelial progenitor (fLEP) stage, the NKX2-1 reporter line (Hawkins et al., 2021; Jacob et al., 2019; McCauley et al., 2017) and surface markers (CPM and CD47; Gotoh et al., 2014; Hawkins et al., 2017) are used to enrich or isolate NKX2-1+ primordial lung progenitors. While our protocol does not rely on FACS sorting of fluorescent reporters or specific cell surface molecules to enrich for NKX2-1+ cells, we preferentially sort for pan-epithelial cell surface antigen EpCAM (or CD326) by magnetic activated cell sorting (MACS) to establish fetal lung epithelial cells (fLEC). Our reason for this is that it remains unknown whether NKX2-1 is the only marker of lung progenitors or whether NKX2-1 is required to specify lung. In Nkx2.1 null mice, the lung anlage forms; however, lung development arrests at the pseudoglandular stage (E12) (Minoo, Su, Drum, Bringas, & Kimura, 1999), which would suggest the lung bud can develop in the absence of NKX2-1 , and more importantly, NKX2-1 is not required to specify lung but required for lung epithelial development.
To generate proximal lineages, low Wnt treatment on sorted NKX2-1+ cells is able to generate proximal-airway organoids (McCauley et al., 2017). Recently, Hawkins et al. (2021) developed the protocol to generate proximal lineage ALI culture through basal cell–enriched organoids. While dual SMAD inhibition treatment enriches for a basal cell population in our differentiation, our basal cell–enriched organoids do not contain all the cell types seen in the proximal airway. However, defined AES differentiation medium supplemented with growth factors differentiates the basal cells and generates 3D epithelial spheroids containing basal, ciliated, club, and goblet cells. Replating the undifferentiated basal cell organoids onto transwells for ALI culture, as we previously described (Wong et al., 2012, 2015), induces maturation and polarization of the epithelium such that by the end of 5 weeks in ALI, a pseudostratified epithelium is formed containing many of the proximal airway epithelial cells. Our new ALI culture showed an improved level of CFTR protein and measurable function (up to 40% function above baseline) compared to our old protocol.
Critical Parameters
- (i) Start with at least 80% confluency for differentiation into DE : One aspect that significantly impacts the robustness of the differentiation (Basic Protocol 1) is the starting hPSC confluency. Prior to DE induction, we have found that 80% confluency yields high DE efficiency. Co-expression of C-X-C chemokine receptor type 4 (CXCR4) and CD117 can be used to evaluate DE differentiation efficiency (Wong et al., 2015) When under-confluent (<80%), differentiation efficiency is significantly reduced and, depending on the cell line, can affect downstream yield of fetal lung epithelial progenitors.
- (ii) Validate efficient AVFE generation from DE : One major factor affecting the robustness of lung differentiation is lineage contamination. High levels of non-lung lineages at the AVFE stage can affect downstream differentiation efficiency. qRT-PCR or flow cytometry can be used to evaluate yield of non-lung lineage markers, such as pancreatic (PDX1) and intestine (CDX2). If high levels of non-lung lineage markers are observed, we recommend that users titrate the concentration of growth factors for the cell line due to line-to-line variation. High cell confluency affects AVFE differentiation efficiency due to the high consumption of growth factors in the medium. We recommend medium change daily instead of every 2 days; alternatively, replating AVFE cells can improve AVFE differentiation efficiency and expand the number of AVFE cells for downstream differentiation.
- (iii) Collagen coating : A sensitive aspect of Basic Protocol 3 is the preparation of collagen-coated transwells. One common oversight is partially covering the membrane and subsequently preventing proper coating and collagen polymerization from occurring. It is therefore important to check each transwell for full collagen coverage. It is also crucial to allow sufficient time for the collagen-IV to air dry onto the transwells. While 18 hr has been sufficient for our purposes, it is important for the user to optimize the amount of time needed to fully dry the membrane and allow for proper collagen polymerization. Lastly, when preparing these transwells, first-time users will discover that the acidity of collagen-IV impacts the effectiveness of subsequent cell seeding. Therefore, multiple washes with DMEM/F12 prior to seeding are important to remove residual collagen solution and reduce its acidity. Phenol red in the DMEM/F12 should be used as an indicator for acidity, and users should wash the membranes until there is an absence of DMEM/F12 color change.
- (iv) Single cell dissociation for seeding : To ensure good cell seeding efficiency onto collagen-coated transwells (Basic Protocol 3), it is crucial to handle cells with care during single cell dissociation. Users should pay close attention to the timing when conducting TrypLE dissociation and add sufficient medium for proper inactivation. Users may supplement media with FBS. Once dissociated, cells should be handled on ice when possible to improve viability.
- (v) Cell seeding into transwell : During optimization of our previous protocol, we observed that replating cells onto collagen-IV-coated plates and transwells has the most success with regard to adherence and efficiency on day 6 of the differentiation protocol, but this was largely user dependent. Shear forces from manual cell dissociation to re-plate the cells can affect cell viability. The ROCK inhibitor Y-27632 is added to improve viability. Due to line-to-line variability in proliferation rate, continuous cell growth may cause over-confluency in later stages of differentiation, hence causing the cell layer to peel off. To resolve this problem, users may need to reduce seeding density for some of the cell lines and optimize the appropriate cell seeding density for each line.
- (vi) Generating hanging-drop Matrigel dome : The hanging-drop Matrigel dome technique is required for Basic Protocol 2 to generate AES. This process is time-sensitive due to the sensitivity of the dissociated fLEC and Matrigel's proclivity for solidification. Therefore, prior to executing this method, users are recommended to prepare the dissociated cells, pre-counted and ready to be embedded, on ice, with sufficient Matrigel thawed on ice. When plating, it is important to only use the first stop of the pipettor to minimize air bubble generation within the domes. Lastly, the technique that presents the most difficulty even with experienced users is inverting the plate. Ideally, the plate should be inverted quickly without interfering with the dome's formation. While not ideal, it is possible for users to allow the Matrigel to solidify marginally and invert the plate afterwards, for a higher success rate. It is also important to keep in mind that Matrigel is not defined and can present lot-to-lot variation. Therefore, users should be mindful that the incubation times for effective Matrigel solidification are guidelines. Prior to adding medium, users should first verify whether the Matrigel dome is fully solid and add incubation time for Matrigel solidification when needed.
- (vii) Careful removal of mucus to allow consistent air exposure in ALI culture : Air exposure is crucial for the polarization of differentiation during the ALI stage. To improve ALI differentiation efficiency and consistency, it is important to ensure that cells in the apical chamber are not covered by medium or mucus. We recommended removing the liquid/mucus gently with a micropipettor to avoid damage during suctioning. Keep in mind that accumulated mucus can impair epithelial integrity and present the risk of accidental stripping of the epithelium from the transwells when removing the mucus.
Troubleshooting
Tables 3-5 list common problems that may arise with Basic Protocols 1, 2, and 3, respectively, along with their probable causes and solutions.
Problem | Possible cause | Solution |
---|---|---|
Cell death after 1 day in definitive endoderm medium | hPSCs not confluent enough prior to DE differentiation | Ensure hPSC confluency >80% |
Low yield of NKX2-1-expressing cell | Inefficient DE differentiation | Perform quality check on DE based on CXCR4+/CD117+ immunostaining. If DE efficiency is low, optimize hPSC confluency and quality for differentiation. |
Problem | Possible cause | Solution |
---|---|---|
Low yield of CD326+ cells (fLEP) after MACS | CD326+ cells were not tagged properly | Ensure single cell suspension prior to tagging with beads by straining cell suspension |
Differentiation efficiency (DE) may be too low | Improve differentiation efficiency by following troubleshooting for Basic Protocol 1 (see Table 3) | |
Matrigel does not form dome structure when plating on TC plates | TC plate coating may not support dome formation | Try different brands of plates (e.g., Sarstedt adhering plate) |
Matrigel is too cold when plating | Let Matrigel sit at room temperature for 30 s before plating on a tissue culture plate. Pre-warm the tissue culture plate in incubator at 37°C for 5 min. | |
Matrigel is diluted by cell suspension | Increase cell density before resuspending in Matrigel | |
Matrigel domes collapsed next day after seeding | Medium is not warm enough | Ensure the medium is lukewarm before adding to the well |
Matrigel has not fully solidified before medium is added | Increase incubation time for the Matrigel to solidify |
Problem | Possible cause | Solution |
---|---|---|
Cells unable to attach to transwells | Residual collagen on transwell | Wash with DMEM/F12 prior to seeding |
Cell death at Stage 4 | PneumaCult-Ex Plus is too old | Use fresh PneumaCult-Ex Plus supplemented with FGF18 for Stage 4 cells. Avoid freeze-thawing PneumaCult-Ex Plus. |
Cell layer start peeling at later stage of ALI culturing | Over-confluent cell layer detached from transwell | Decrease cell seeding density on day 6 |
Suction on apical chamber is too rough | Use a P200 pipette instead of regular suctioning to remove liquid on the apical chamber | |
Medium was added directly onto the cells and disrupted cell layer | Add medium on the wall of the chamber and not directly on the cells |
Understanding Results
This procedure includes directed differentiation towards mature proximal airway epithelia (Fig. 1). Using a commercially defined kit to generate highly efficient DE (>95%), cells are pushed towards AVFE differentiation using sonic hedgehog and fibroblast growth factor 2 (Wong et al., 2012, 2015), with a brief dual SMAD inhibition [transforming growth factor beta (TGFβ) and BMP inhibition] period (2 days) (Huang et al., 2014) in medium containing supplements favoring AVFE differentiation. At the end of this stage, >90% of the cells are SOX2+ with no detectable midgut pancreatic and duodenal homeobox-1 (PDX1+) and a low level of hindgut caudal type homeobox-2 (CDX2+) cells (4.2%) (Fig. 2A). Gene expression analysis of foregut endoderm shows marked up-regulation of the foregut markers forkhead box G1 (FOXG1) and SRY-box 2 (SOX2), and modest elevation in the earliest lung marker NKX2-1 , in comparison to McCauley et al. (2017), Miller et al. (2018), and Wong et al. (2015). Compared to fetal control RNA (whole fetal lung from gestational week 18 tissue), airway epithelial–specific lineage markers were not highly expressed by bulk gene expression analysis (using quantitative real-time reverse transcription polymerase chain reaction (qRT-PCR) (Fig. 2B).
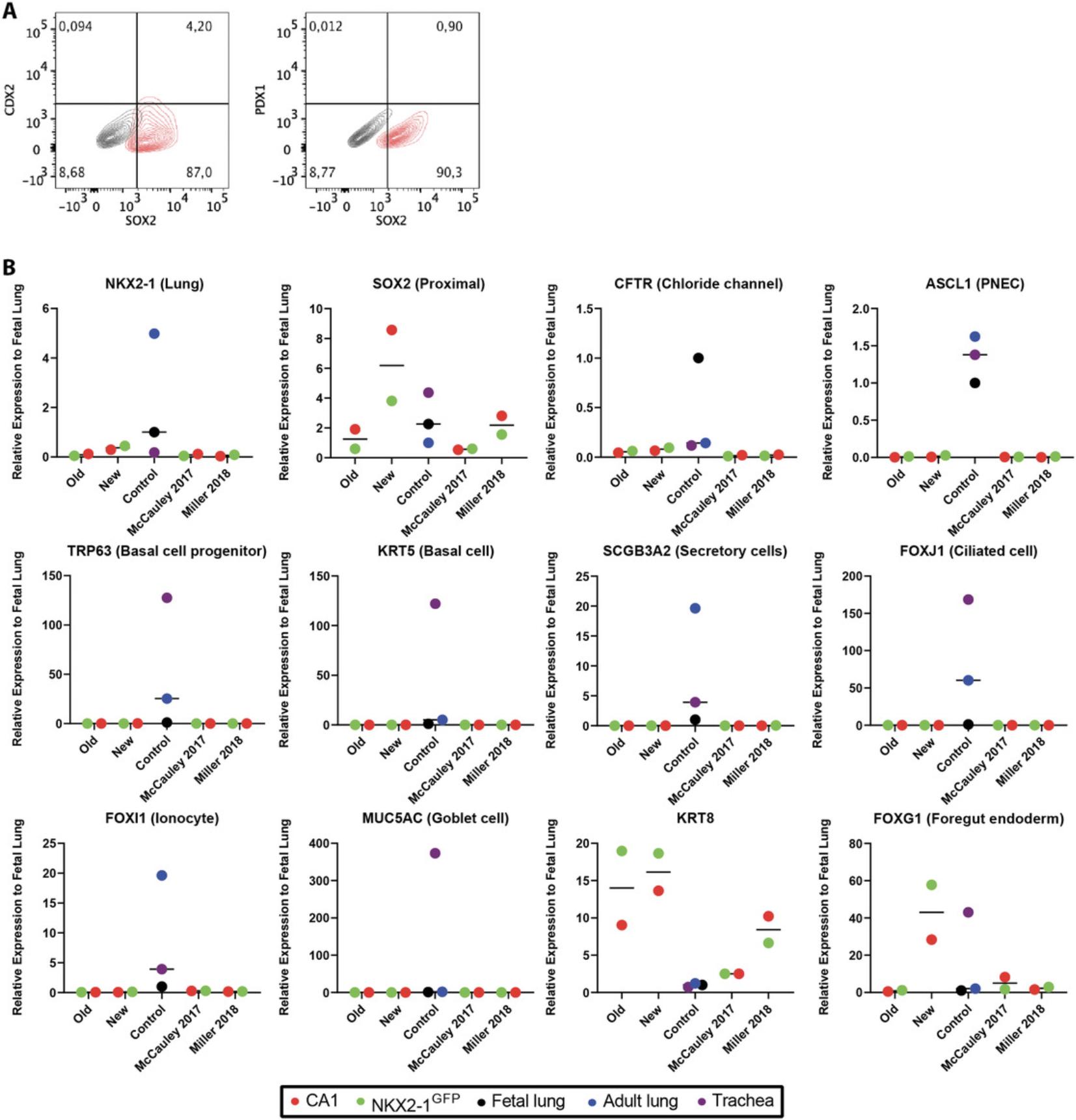
Next, fLEP from Stage 3 of the differentiation were generated by adding Wnt pathway activator CHIR99021 and retinoic acid, both of which influence lung specification (Nikolić et al., 2017), to media supplemented with fibroblast growth factors (FGFs) and bone morphogenetic protein 4 (BMP4), favoring conditions that drive fetal lung growth (Wong et al., 2012). Immunofluorescence staining of fLEP cultures for multipotent progenitor cell markers (SOX2, SOX9) and lung marker NKX2-1 (or thyroid transcriptional factor 1, TTF1, the protein herein) showed TTF1+ cells expressing both SOX2+ and SOX9+, and TTF1+SOX2+ and TTF1+SOX9+ double-positive cells were found using both hPSC lines, suggesting emergence of a heterogeneous lung progenitor population in the fLEP cultures (Fig. 3A). At this stage, we compared our new and old protocols with McCauley et al. (2017) and Miller et al. (2018) using gene expression analysis by qRT-PCR of the cell cultures (Fig. 3B). We show abundant levels of CFTR expression with highest expression observed in our new differentiation protocol. The high expression level of CFTR is reminiscent of first-trimester fetal lung tissue where CFTR has previously been found to be abundantly expressed in the developing lung epithelium. An increase in gene expression associated with basal (transformation related protein 63, TRP63 , or TP63 ; and cytokeratin-5, KRT5) and ciliated (forkhead box J1, FOXJ1) cells was also observed. Using flow cytometric analysis, an increase in TTF1+ and TTF1+SOX2+, TTF1+SOX9+ cells was only seen in the NKX2-1-EGFP cell line with our new protocol compared to our old (Fig. 4A, B). However, an increase in P63+ cells was seen in both cell lines in the new protocol, with our protocol yielding the highest percentage of P63+ cells (60%) compared to others. Finally, using our previously established modified apical chloride conductance (ACC) assay measuring membrane potential changes through changes in fluorescence intensity (fluorescence imaging plate reader, FLiPR; Jiang et al., 2021), we found that CFTR activity was enhanced by about 30% in our new protocol compared to our old protocol (Fig. 5A,B) upon forskolin stimulation. Specificity of CFTR function was confirmed using CFTR inhibitor-172 treatment. Both hPSC lines demonstrated enhanced CFTR function, averaging around 32% of forskolin-induced CFTR function in our new protocol compared to our old (∼10% function; Fig. 5C). Higher levels of the mature CFTR protein Band C (representing a fully glycosylated functional form of the protein expressed on the plasma membrane, ∼170 kDa) was observed in our new differentiation protocol compared to our previous protocol (Fig. 5D), providing a powerful source of cells for rapid high-throughput functional screens of novel CFTR modulators.
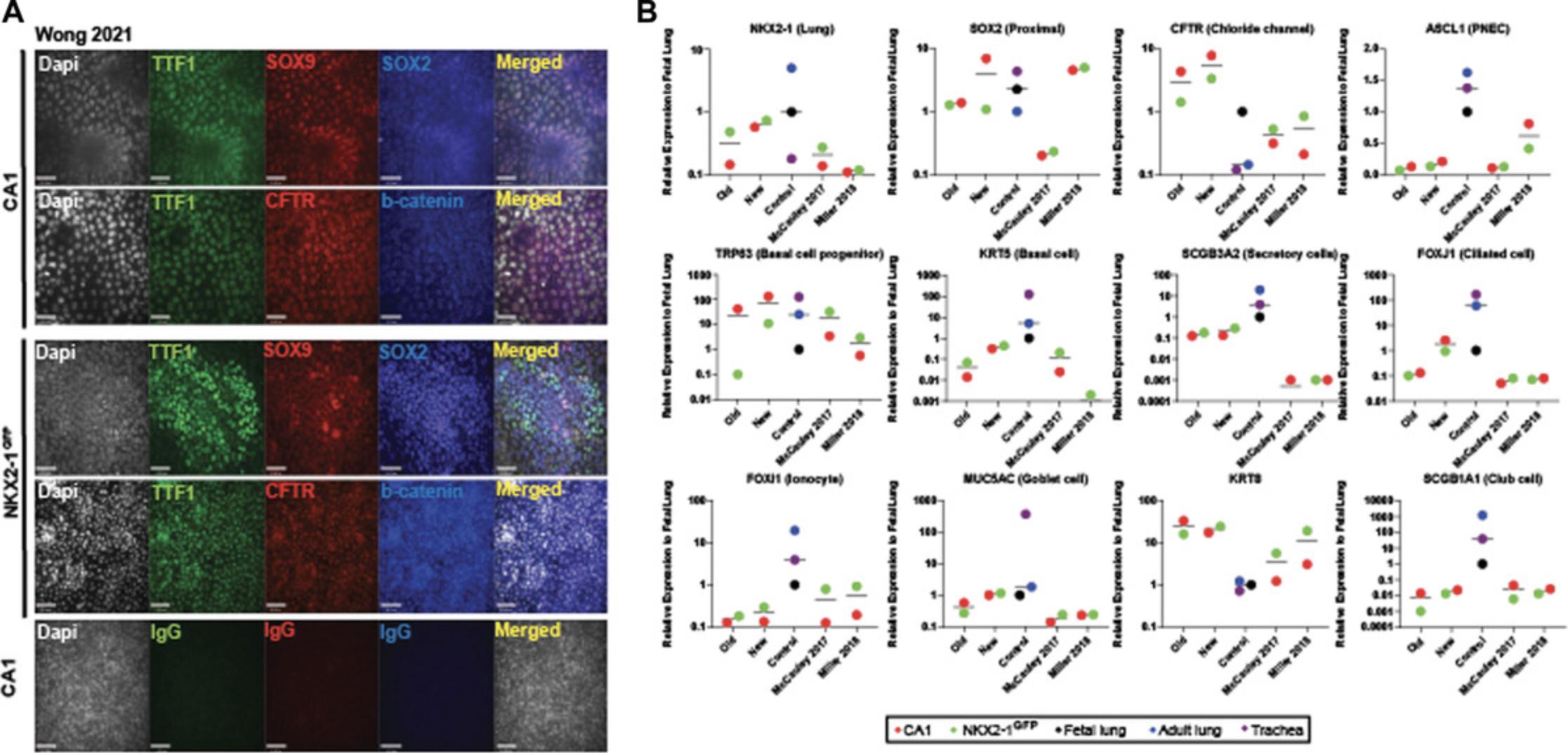
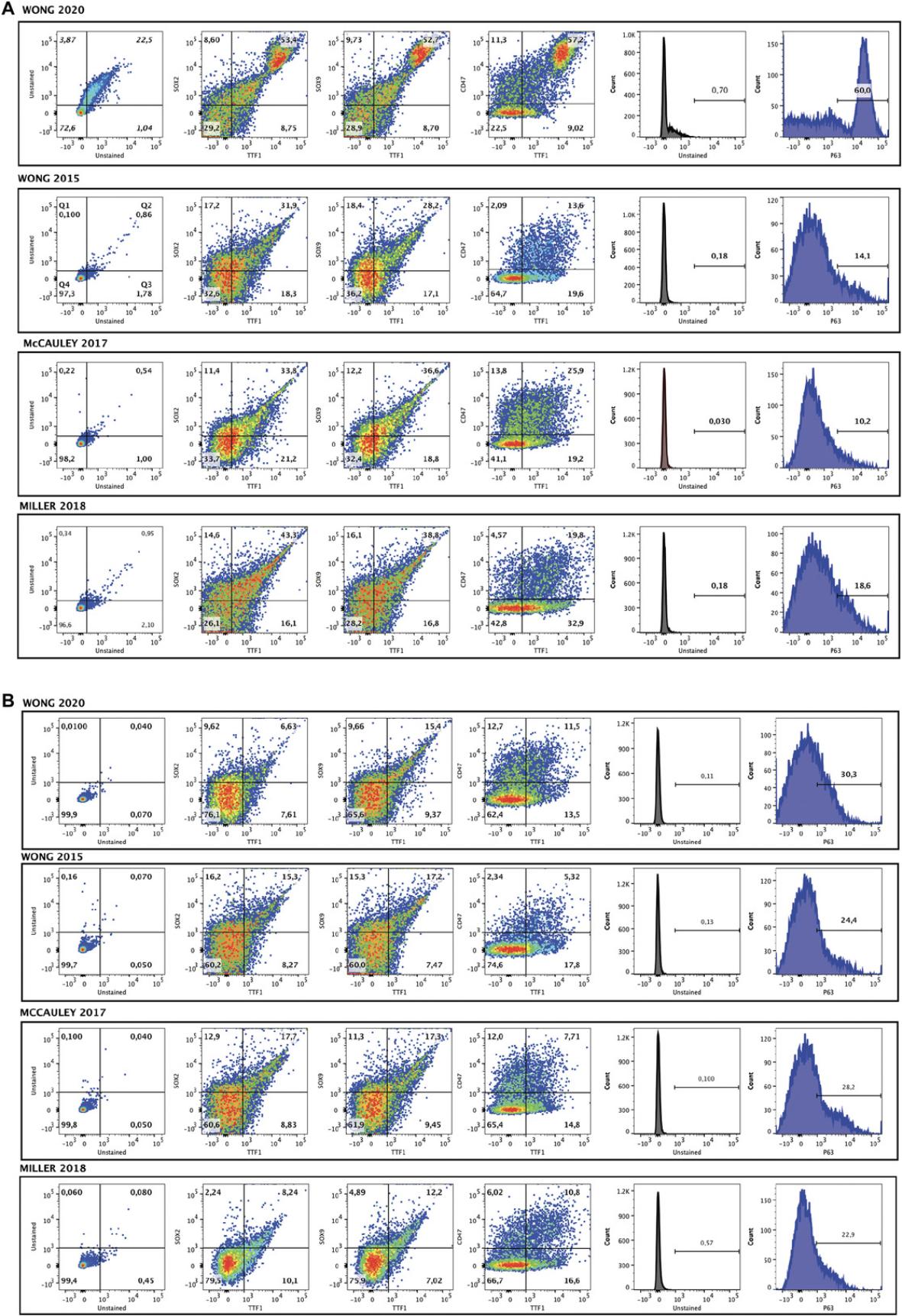
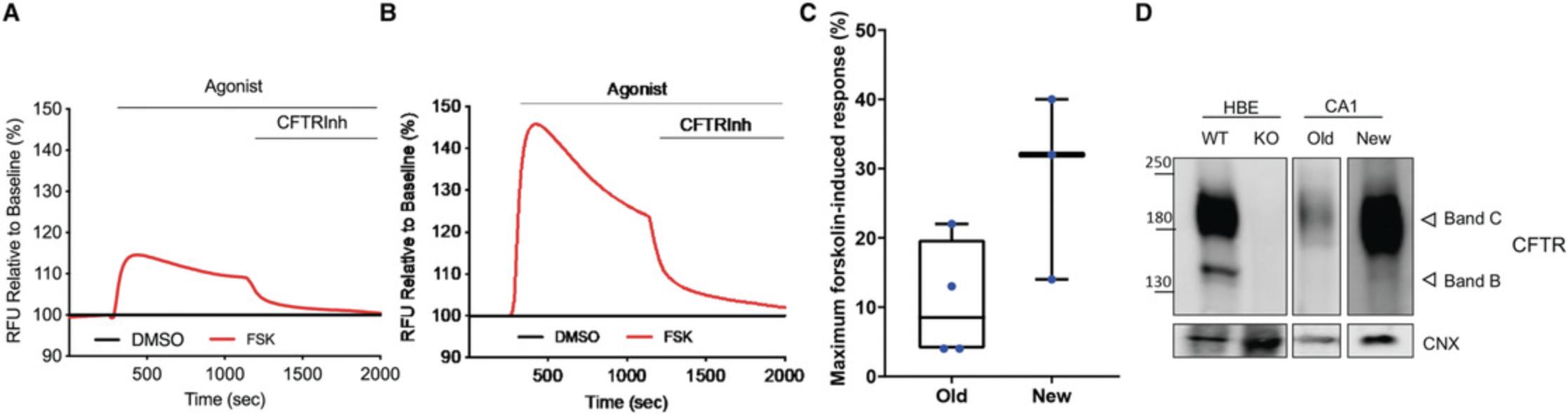
AES were generated from hPSC-derived CD326+ fLEC isolated by column-based magnetic-activated cell sorting (Fig. 6A). Within 4 days, AES emerged. These spheroids were capable of propagation in expansion medium containing dual SMAD inhibitors for >4 passages (up to 10-15 passages; data not shown) with decreasing expansion potential in late passages (Fig. 6B,C). We tested this AES generation potential in three independent hPSC lines and found varying efficiencies of AES generation from CD326+ single cells (Fig. 6D). The dual SMAD inhibition, A83-01 and DMH1, suppresses basal cell spontaneous differentiation to secretory and ciliary cells, and maintains basal stem cell proliferation (Hawkins et al., 2021; Mou et al., 2016). Renewable AES are composed of mostly ΔNP63+ basal stem cells (>90%), confirmed using a double fluorescence reporter hPSC line BU3-NKX2-1GFP/P63tdTomato (NGPT) (Hawkins et al., 2021), where tdTomato fluorescence was observed making up the majority of the cells in the spheroids (Fig. 6E,F).
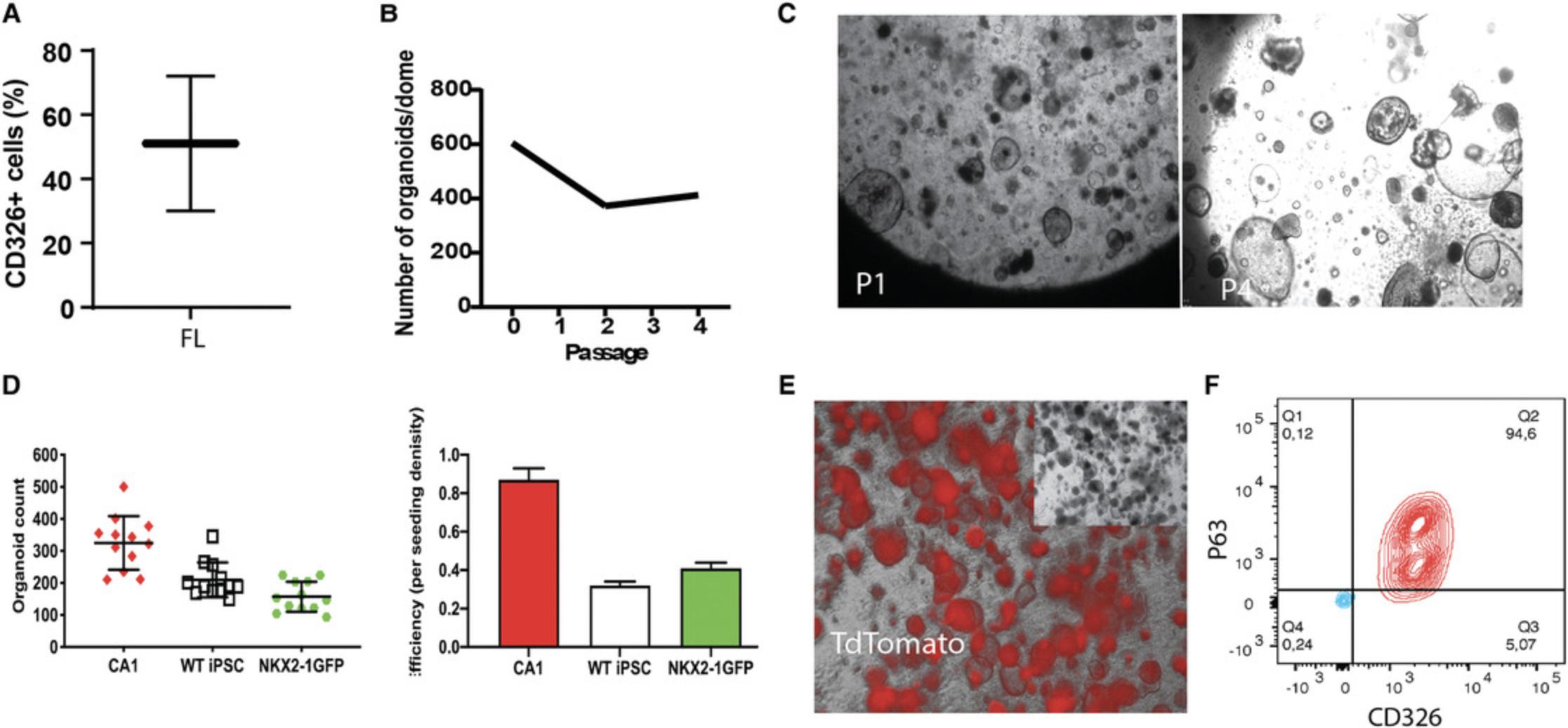
To determine the multi-epithelial differentiation potential of the basal stem cells in the AES, the spheroids were cultured in differentiation medium for up to 14 days. AES shares morphological similarities to spheroids derived from primary bronchial epithelial cells (Fig. 7A). The differentiated spheroids expressed ΔNP63 and KRT14, acetylated α-tubulin, cytokeratin-8/18 (KRT8/18), secretoglobin 1A1 (SCGB1A1), and mucin 5ac (MUC5AC) (Fig. 7B). qPCR analysis reveals up-regulation of several genes associated with specific epithelial lineages found in the airways; basal cell marker KRT5 , ciliated cell marker FOXJ1 , luminal epithelial cell marker KRT8 , and secretory cell marker MUC16 were found in differentiated AES in comparison to AES in expansion medium (Fig. 7C). A near two-fold up-regulation of CFTR is also observed (Fig. 7C) and can be found on the apical membrane (lumen side) of the spheroid along tight junction protein ZO-1 (Fig. 7B). The lung (TTF1+) epithelial (E-cadherin+, panCK+) spheroids express proximal marker SOX2, suggesting that these are AES and do not contain cells of the distal respiratory phenotype. To assess CFTR protein expression, we assayed for the presence of Band C (∼170 kDa) protein by gel electrophoresis, indicative of a mature, apically localized protein capable of function. Only spheroids derived from CD326+ cells express mature CFTR (Fig. 7D).
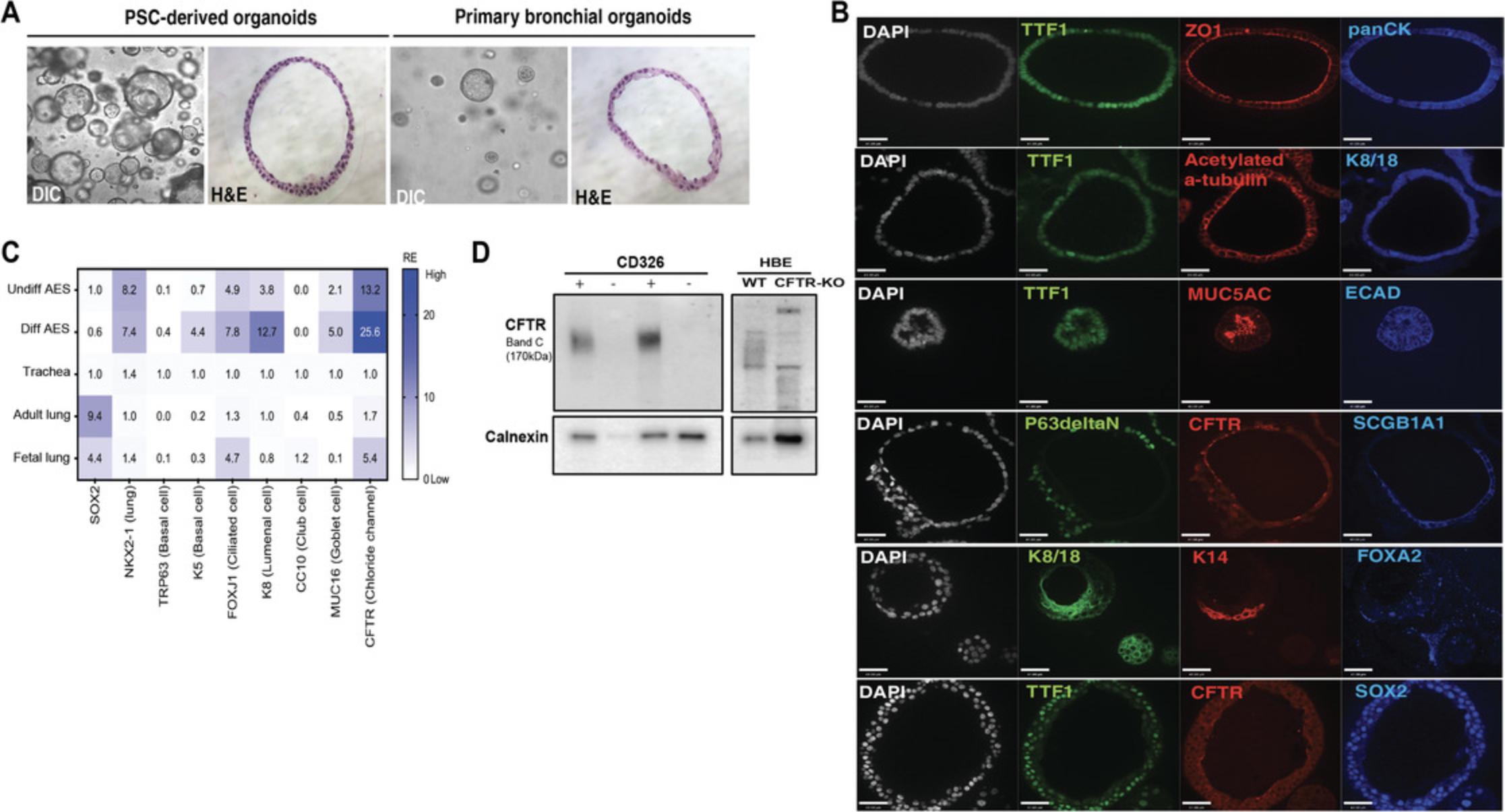
Next, we generate functional mature airway epithelial tissue by culturing the cells on collagen-coated transwells, as previously described (Wong et al., 2015), to generate ALI cultures. During this period, the epithelial cells undergo additional differentiation, maturation, and polarization to form a pseudostratified epithelium reminiscent of the mature airways. Our modified ALI protocol entails a two-step method that first allows expansion of the cells seeded onto the transwell membrane to form a monolayer of cells prior to differentiation and polarization. Characterization of the genes expressed in the cell population in the ALI cultures show an average up-regulation of CFTR expression in the new protocol by >500-fold and >30-fold compared to the old and McCauley et al. (2017) protocols respectively, while many of the other epithelial cell lineage markers remained relatively consistent (Fig. 8). Importantly, there were differences in expression levels for many of the genes between the two hPSC lines tested. Immunostaining of the ALI cultures in our new protocol shows that TTF1 expression is restricted to some cell types, and the majority co-expressed with SOX2 indicate proximal airway phenotype (Fig. 9A, A′) similar to adult lung tissue (Fig. 9A′′). A pseudostratified airway epithelium composed of basolateral-localized basal cells (ΔNP63+) and luminal secretory (SCGB3A2+) and ciliated (acetylated α-tubulin+) cells is extended to the luminal/apical side (Fig. 9B), similar to adult airway tissue (Fig. 9B′). Non-immune-reactive immunoglobulins (IgGs) were used as controls for nonspecific binding (Fig. 9C). Bulk gene expression analysis showed that, using the new protocol, both hPSC lines are capable of generating many of the mature airway epithelial cell types observed in airways (Fig. 8). Protein analysis of CFTR shows high levels of Band C CFTR protein species (Fig. 9D). Importantly, a nearly 3-fold higher CFTR function was detected in the new ALI cultures compared to our previous protocol (Fig. 9E,F).
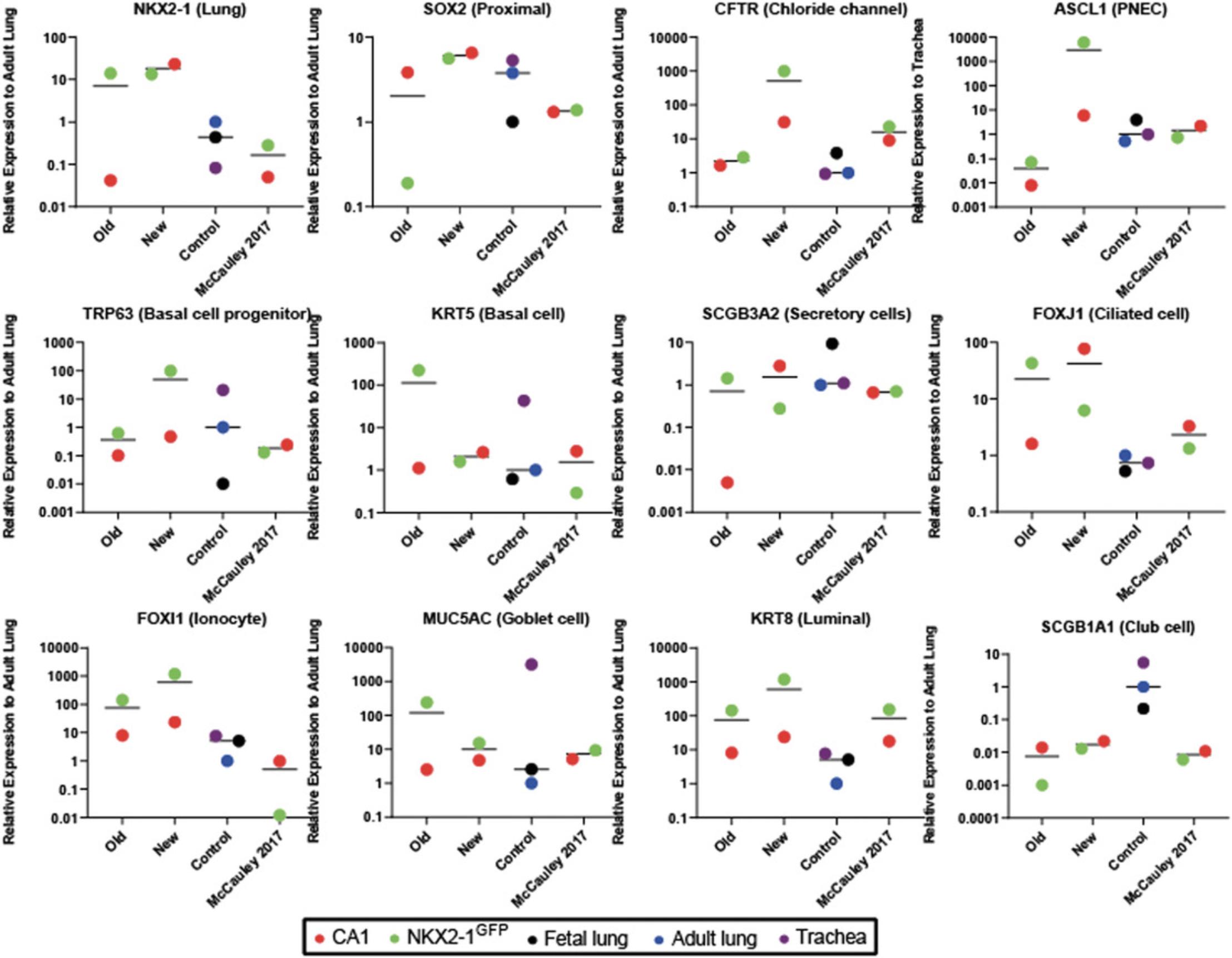
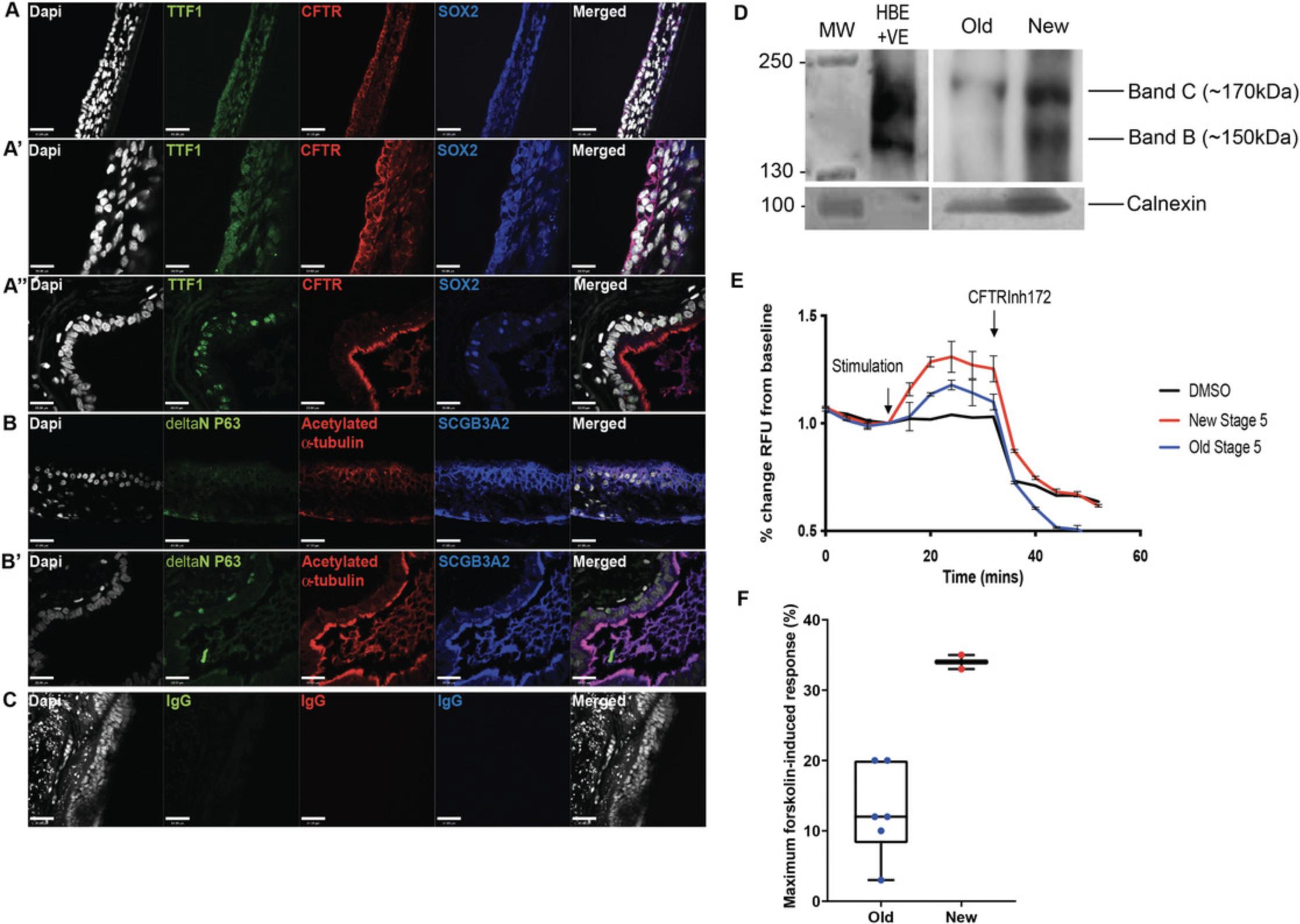
Time Considerations
Differentiation of mature airway cells from human pluripotent stem cell (hPSC) culture (Fig. 1) using this protocol requires more than 2 months to complete. However, this timeline does not include hPSC maintenance and expansion. Nonetheless, with the generation of renewable fetal organoids in Stage 3, one does not need to re-initiate hPSC differentiation from the start each time lung cells are needed.
We show the generation of three stage-specific lung epithelial models derived from hPSC. On day 21, the fLEP cells express cell markers associated with lung epithelial progenitors and high CFTR expression. The fLEP can be used to study signaling pathways regulating cell lineage development, and for CFTR-modulator screens as recently shown (Jiang et al., 2021).
The undifferentiated AES model after MACS can be serially passaged and cryopreserved without losing cell phenotype. This is significant, as it minimizes the cell differentiation time for downstream analysis. Under appropriate differentiation conditions, AES generates mature airway cell types with cellular organization similar to the native airways.
The ALI culture model represents an adult-like phenotype in vitro. The hPSC-derived ALI culture contains mature pulmonary cell types and forms pseudostratified epithelia. This model has been used for disease modeling such as cystic fibrosis (Wong et al., 2012, 2015), and represents a good model to study post-natal airway.
The three unique models (fLEP, AES and ALI culture) offer a powerful repertoire of lung models for investigators to use to study human lung development, disease, and therapies.
Acknowledgments
The authors would like to thank Dr. D. Kotton and Dr. F. Hawkins (Boston University) for providing the human iPSC reporter lines, and Dr. Nagy (Lunenfeld Tanenbaum Research Institute, Toronto) for the human ESC CA1 line. Monoclonal CFTR antibodies #596 were courtesy of J.R. Riordan (University of North Carolina, Chapel Hill, North Carolina, USA). We would also like to thank Dr. J. Dierolf for generating the BU3-NKX2-1GFP/P63tdTomato airway epithelial spheroids and corresponding representative fluorescent image. Small-molecule CFTR inhibitor-172 was obtained from CFFT. This work was supported by the CF Canada SickKids Program in Individual Therapy (CFIT) (in part) by a SickKids CFIT Microsynergy grant (awarded to A.P.W in 2017) and Vertex Pharmaceutical CF Research Innovation Award (awarded to A.P.W in 2017), the Genome Canada and the Ontario Genomics Institute (OGI-148), the SickKids Foundation & CIHR-IHDCYH grant (NI20-1070), and the Stem Cell Network Early Career Investigator—Innovation Award (FY21/ECI23 Wong).
Author Contributions
Shuk Yee Ngan : data curation, formal analysis, validation, visualization, writing original draft; Henry T. Quach : visualization, writing original draft, writing review and editing; Onofrio Laselva : data curation, formal analysis, writing review and editing; Elena N. Huang : data curation, writing review and editing; Maria Mangos : data curation; Sunny Xia : data curation, formal analysis; Christine E. Bear : resources, supervision; Amy P. Wong : conceptualization, data curation, formal analysis, funding acquisition, investigation, methodology, project administration, supervision, validation, visualization, writing original draft, writing review and editing.
Conflict of Interest
The authors declare no conflict of interest.
Open Research
Data Availability Statement
The data that support the findings of this study are available from the corresponding author upon reasonable request.
Supporting Information
Filename | Description |
---|---|
cpz1341-sup-0001-TableS1-S2.docx19.5 KB | Supporting Information |
Please note: The publisher is not responsible for the content or functionality of any supporting information supplied by the authors. Any queries (other than missing content) should be directed to the corresponding author for the article.
Literature Cited
- Aurora, M., & Spence, J. R. (2016). hPSC-derived lung and intestinal organoids as models of human fetal tissue. Developmental Biology , 420(2), 230–238. doi: 10.1016/j.ydbio.2016.06.006.
- Chen, H. J., Poran, A., Unni, A. M., Huang, S. X., Elemento, O., Snoeck, H.-W., & Varmus, H. (2019). Generation of pulmonary neuroendocrine cells and SCLC-like tumors from human embryonic stem cells. Journal of Experimental Medicine , 216(3), 674–687. doi: 10.1084/jem.20181155.
- Chen, Y.-W., Huang, S. X., de Carvalho, A. L. R. T., Ho, S.-H., Islam, M. N., Volpi, S., … Snoeck, H.-W. (2017). A three-dimensional model of human lung development and disease from pluripotent stem cells. Nature Cell Biology , 19(5), 542–549. doi: 10.1038/ncb3510.
- D'Amour, K. A., Agulnick, A. D., Eliazer, S., Kelly, O. G., Kroon, E., & Baetge, E. E. (2005). Efficient differentiation of human embryonic stem cells to definitive endoderm. Nature Biotechnology , 23(12), 1534–1541. doi: 10.1038/nbt1163.
- Dye, B. R., Hill, D. R., Ferguson, M. A., Tsai, Y.-H., Nagy, M. S., Dyal, R., … Spence, J. R. (2015). In vitro generation of human pluripotent stem cell derived lung organoids. ELife , 4, e05098. doi: 10.7554/eLife.05098.
- Gotoh, S., Ito, I., Nagasaki, T., Yamamoto, Y., Konishi, S., Korogi, Y., … Mishima, M. (2014). Generation of alveolar epithelial spheroids via isolated progenitor cells from human pluripotent stem cells. Stem Cell Reports , 3(3), 394–403. doi: 10.1016/j.stemcr.2014.07.005.
- Green, M. D., Chen, A., Nostro, M.-C., d'Souza, S. L., Schaniel, C., Lemischka, I. R., … Snoeck, H.-W. (2011). Generation of anterior foregut endoderm from human embryonic and induced pluripotent stem cells. Nature Biotechnology , 29(3), 267–272. doi: 10.1038/nbt.1788.
- Hawkins, F. J., Suzuki, S., Beermann, M. L., Barillà, C., Wang, R., Villacorta-Martin, C., … Kotton, D. N. (2021). Derivation of airway basal stem cells from human pluripotent stem cells. Cell Stem Cell , 28(1), 79–95.e8. doi: 10.1016/j.stem.2020.09.017.
- Hawkins, F., Kramer, P., Jacob, A., Driver, I., Thomas, D. C., McCauley, K. B., … Kotton, D. N. (2017). Prospective isolation of NKX2-1–expressing human lung progenitors derived from pluripotent stem cells. Journal of Clinical Investigation , 127(6), 2277–2294. doi: 10.1172/JCI89950.
- Huang, J., Hume, A. J., Abo, K. M., Werder, R. B., Villacorta-Martin, C., Alysandratos, K.-D., … Kotton, D. N. (2020). SARS-CoV-2 Infection of pluripotent stem cell-derived human lung alveolar type 2 cells elicits a rapid epithelial-intrinsic inflammatory response. Cell Stem Cell , 27(6), 962–973.e7. doi: 10.1016/j.stem.2020.09.013.
- Huang, S. X. L., Islam, M. N., O'Neill, J., Hu, Z., Yang, Y.-G., Chen, Y.-W., … Snoeck, H.-W. (2014). Efficient generation of lung and airway epithelial cells from human pluripotent stem cells. Nature Biotechnology , 32(1), 84–91. doi: 10.1038/nbt.2754.
- Hurley, K., Ding, J., Villacorta-Martin, C., Herriges, M. J., Jacob, A., Vedaie, M., … Kotton, D. N. (2020). Reconstructed single-cell fate trajectories define lineage plasticity windows during differentiation of human PSC-derived distal lung progenitors. Cell Stem Cell , 26(4), 593–608.e8. doi: 10.1016/j.stem.2019.12.009.
- Jacob, A., Morley, M., Hawkins, F., McCauley, K. B., Jean, J. C., Heins, H., … Kotton, D. N. (2017). Differentiation of human pluripotent stem cells into functional lung alveolar epithelial cells. Cell Stem Cell , 21(4), 472–488.e10. doi: 10.1016/j.stem.2017.08.014.
- Jacob, A., Vedaie, M., Roberts, D. A., Thomas, D. C., Villacorta-Martin, C., Alysandratos, K.-D., … Kotton, D. N. (2019). Derivation of self-renewing lung alveolar epithelial type II cells from human pluripotent stem cells. Nature Protocols , 14(12), 3303–3332. doi: 10.1038/s41596-019-0220-0.
- Jiang, J. X., Wellhauser, L., Laselva, O., Utkina, I., Bozoky, Z., Gunawardena, T., … Bear, C. E. (2021). A new platform for high-throughput therapy testing on iPSC-derived lung progenitor cells from cystic fibrosis patients. Stem Cell Reports , 16(11), 2825-2837. doi: 10.1016/j.stemcr.2021.09.020.
- Kolios, G., & Moodley, Y. (2013). Introduction to stem cells and regenerative medicine. Respiration , 85(1), 3–10. doi: 10.1159/000345615.
- Magro-Lopez, E., Palmer, C., Manso, J., Liste, I., & Zambrano, A. (2018). Effects of lung and airway epithelial maturation cocktail on the structure of lung bud organoids. Stem Cell Research & Therapy, 9(1), 186. doi: 10.1186/s13287-018-0943-9.
- McCauley, K. B., Hawkins, F., Serra, M., Thomas, D. C., Jacob, A., & Kotton, D. N. (2017). Efficient derivation of functional human airway epithelium from pluripotent stem cells via temporal regulation of Wnt signaling. Cell Stem Cell , 20(6), 844–857.e6. doi: 10.1016/j.stem.2017.03.001.
- Miller, A. J., Hill, D. R., Nagy, M. S., Aoki, Y., Dye, B. R., Chin, A. M., … Spence, J. R. (2018). In vitro induction and in vivo engraftment of lung bud tip progenitor cells derived from human pluripotent stem cells. Stem Cell Reports , 10(1), 101–119. doi: 10.1016/j.stemcr.2017.11.012.
- Miller, A. J., Yu, Q., Czerwinski, M., Tsai, Y.-H., Conway, R. F., Wu, A., … Spence, J. R. (2020). In vitro and in vivo development of the human airway at single-cell resolution. Developmental Cell , 54(6), 818. doi: 10.1016/j.devcel.2020.09.012.
- Minoo, P., Su, G., Drum, H., Bringas, P., & Kimura, S. (1999). Defects in tracheoesophageal and lung morphogenesis inNkx2.1(−/−) mouse embryos. Developmental Biology , 209(1), 60–71. doi: 10.1006/dbio.1999.9234.
- Mou, H., Vinarsky, V., Tata, P. R., Brazauskas, K., Choi, S. H., Crooke, A. K., … Rajagopal, J. (2016). Dual SMAD signaling inhibition enables long-term expansion of diverse epithelial basal cells. Cell Stem Cell , 19(2), 217–231. doi: 10.1016/j.stem.2016.05.012.
- Nikolić, M. Z., Caritg, O., Jeng, Q., Johnson, J.-A., Sun, D., Howell, K. J., … Rawlins, E. L. (2017). Human embryonic lung epithelial tips are multipotent progenitors that can be expanded in vitro as long-term self-renewing organoids. ELife , 6, e26575. doi: 10.7554/eLife.26575.
- Phelan, K., & May, K.M. (2015). Basic techniques in mammalian cell tissue culture. Current Protocols in Cell Biology , 66, 1.1.1–1.1.22.doi: 10.1002/0471143030.cb0101s66.
- Porotto, M., Ferren, M., Chen, Y-W., Siu, Y., Makhsous, N., Rima, B., … Moscona, A. (2019). Authentic modeling of human respiratory virus infection in human pluripotent stem cell-derived lung organoids. mBio , 10(3), e00723-19. doi: 10.1128/mbio.00723-19.
- Rodrigues Toste de Carvalho, A. L., Liu, H.-Y., Chen, Y.-W., Porotto, M., Moscona, A., & Snoeck, H.-W. (2021). The in vitro multilineage differentiation and maturation of lung and airway cells from human pluripotent stem cell–derived lung progenitors in 3D. Nature Protocols , 16(4), 1802–1829. doi: 10.1038/s41596-020-00476-z.
- Shafa, M., Ionescu, L. I., Vadivel, A., Collins, J. J. P., Xu, L., Zhong, S., … Thébaud, B. (2018). Human induced pluripotent stem cell–derived lung progenitor and alveolar epithelial cells attenuate hyperoxia-induced lung injury. Cytotherapy , 20(1), 108–125. doi: 10.1016/j.jcyt.2017.09.003.
- Shojaie, S., Ermini, L., Ackerley, C., Wang, J., Chin, S., Yeganeh, B., … Post, M. (2015). Acellular lung scaffolds direct differentiation of endoderm to functional airway epithelial cells: Requirement of matrix-bound HS proteoglycans. Stem Cell Reports , 4(3), 419–430. doi: 10.1016/j.stemcr.2015.01.004.
- Takahashi, K., Tanabe, K., Ohnuki, M., Narita, M., Ichisaka, T., Tomoda, K., & Yamanaka, S. (2007). Induction of pluripotent stem cells from adult human fibroblasts by defined factors. Cell , 131(5), 861–872. doi: 10.1016/j.cell.2007.11.019.
- Thomson, J. A., Itskovitz-Eldor, J., Shapiro, S. S., Waknitz, M. A., Swiergiel, J. J., Marshall, V. S., & Jones, J. M. (1998). Embryonic stem cell lines derived from human blastocysts. Science , 282(5391), 1145–1147. doi: 10.1126/science.282.5391.1145.
- Wong, A. P., Bear, C. E., Chin, S., Pasceri, P., Thompson, T. O., Huan, L.-J., … Rossant, J. (2012). Directed differentiation of human pluripotent stem cells into mature airway epithelia expressing functional CFTR protein. Nature Biotechnology , 30(9), 876–882. doi: 10.1038/nbt.2328.
- Wong, A. P., Chin, S., Xia, S., Garner, J., Bear, C. E., & Rossant, J. (2015). Efficient generation of functional CFTR-expressing airway epithelial cells from human pluripotent stem cells. Nature Protocols , 10(3), 363–381. doi: 10.1038/nprot.2015.021.
Citing Literature
Number of times cited according to CrossRef: 2
- Jeremy D. Newton, Yuetong Song, Siwan Park, Kayshani R. Kanagarajah, Amy P. Wong, Edmond W. K. Young, Tunable In Situ Synthesis of Ultrathin Extracellular Matrix‐Derived Membranes in Organ‐on‐a‐Chip Devices, Advanced Healthcare Materials, 10.1002/adhm.202401158, 13 , 20, (2024).
- Iris A. L. Silva, Onofrio Laselva, Miquéias Lopes-Pacheco, Advances in Preclinical In Vitro Models for the Translation of Precision Medicine for Cystic Fibrosis, Journal of Personalized Medicine, 10.3390/jpm12081321, 12 , 8, (1321), (2022).