Purification of Mouse Embryonic Fibroblasts (MEFs)
Rita Ferreira, Rita Ferreira, Nadine Hein, Nadine Hein
Abstract
Mouse embryonic fibroblasts (MEFs) are primary fibroblasts purified from mouse embryos at a defined time post-fertilization. MEFs have versatile applications, including use as feeder cell layers or sources of untransformed primary cells for a variety of biological assays. MEFs are most commonly isolated between embryonic day (E)12.5 and E13.5 but can be isolated from embryos as early as E8.5 and as late as E15.5. The individual embryos are harvested by carefully removing uterine tissue, yolk sac, and placenta. The embryos are euthanized, and non-mesenchymal tissues, such as the fetal liver and heart, are removed before tissue homogenization. The remaining fetal tissue is homogenized by mechanical mincing using a sterile blade, followed by enzymatic digestion and resuspension. During tissue dissociation, the duration of trypsin-EDTA/DNase digestion and enzyme concentration are critical parameters to produce high-quality MEFs with the highest rates of cell viability and proliferation potential. MEFs can be cryopreserved at passage (P) 0 if >80% confluent, passaged for further expansion before freezing down, or directly utilized for downstream applications, i.e., preparation as feeder cell layers. Primary MEFs possess a limited proliferation capacity of ∼20 cell divisions, beyond which the percentage of senescent cells rapidly increases; thus, cultures should only be expanded/passaged to a maximum of P5. Critical for cell viability during cryopreservation and thawing of MEFs is the slow decrease in temperature when freezing, the rapid increase when thawing, the use of a cryoprotective agent, and an optimal cell density. While it is critical to generate high-quality MEFs to standardize and optimize preparation procedures and utilize fresh reagents, some variability in proliferation capacity and cell viability between MEF preparations remains. Thus, MEF preparation, culture, and cryopreservation procedures are continuously being optimized. © 2023 The Authors. Current Protocols published by Wiley Periodicals LLC.
Basic Protocol : Purification, passaging, and expansion of MEFs
Supporting Protocol : Cryopreservation and thawing of MEFs
INTRODUCTION
Mouse embryonic fibroblasts (MEFs) are embryo-derived primary fibroblasts with limited proliferation potential (Todaro & Green, 1963). MEFs have versatile applications. Most commonly, MEFs are prepared as feeder cell layers to maintain embryonic stem cells (ESCs) or induced pluripotent stem cells (iPSCs) in an undifferentiated state in prolonged culture (Evans & Kaufman, 1981; Jozefczuk et al., 2012). To be used as feeder cells, MEFs are expanded and mitotically inactivated by either chemical treatment with, i.e., Mitomycin C or γ-irradiation (Conner, 2001; Martin & Evans, 1975). Mitotic inactivated MEFs can then be seeded to form a confluent layer of cells (feeder layer) before seeding ESCs or iPSCs. The feeder layer provides a niche that inhibits cell differentiation. Inactivated MEFs remain metabolically active and support ESCs and iPSCs through the release of growth factors and extracellular matrix components, expression of adhesion molecules, and the support of cell-cell interaction (Llames et al., 2015; Saitoh et al., 2012). One critical MEF-secreted factor that maintains ESCs in their undifferentiated state is leukemia inhibitory factor (LIF) (Smith et al., 1988; Williams et al., 1988). Beyond feeder cells, MEFs derived from transgenic mice are used as in vitro cell systems to functionally test and analyze the impact of genetic alteration (Hannan et al., 2022). Primary non-transformed MEFs are a popular cell system to characterize “normal” cellular activities and signaling in response to various external stimuli or to investigate cellular processes such as mesenchymal differentiation in a non-cancerous background (Dastagir et al., 2014). Due to their limited expansion capacity, MEFs are best cryopreserved at a low passage number until required. The purification procedure and intrinsic batch-to-batch variation impact MEF quality. To minimize these effects and ensure high cell viability and expansion potential, procedures must be standardized, and only fresh reagents should be used. The following protocol describes the purification of MEFs at E13.5, passaging and expansion (Basic Protocol), cryopreservation and thawing (Supporting Protocol).
NOTE : Access to a laminar flow hood or equivalent, a tissue culture incubator, inverted microscope, bench-top centrifuge, −80°C freezer, and ultralow storage is essential for the following protocol.
NOTE : All steps after uterine horn dissection and reagent preparation must be performed under aseptic conditions.
STRATEGIC PLANNING
Depending on the expected litter size and mating success, a sufficient number of female mice must be set up for timed mating. If pregnancy rates are low, other steps outlined in the Troubleshooting section can be introduced to increase mating success (Byers et al., 2012) (https://www.jax.org/news-and-insights/jax-blog/2014/september/six-steps-for-setting-up-timed-pregnant-mice).
NOTE : All animal protocols must be reviewed and approved by the appropriate Animal Care and Use Committee and regulations followed for the care and use of laboratory animals.
Basic Protocol: PURIFICATION, PASSAGING, AND EXPANSION OF MEFs
This protocol describes the generation of MEFs from mouse embryos at E13.5.Mouse embryos are dissected from the pregnant female by removing the uterine horns under non-sterile conditions. The uterine horns are transferred to a laminar flow hood for further preparation under aseptic conditions. First, the uterine horns are rinsed twice in sterile DPBS-/- with antibiotic-antimycotic, followed by removing individual embryos from the uterine tissue. The yolk sac and placenta are then removed, and the exposed embryos are rinsed in sterile DPBS-/- with antibiotic-antimycotic. Before tissue dissociation, the embryo is decapitated, and fetal organs are dissected. The embryonic tissue is finely minced in trypsin-EDTA with DNAse, resuspended multiple times, and digested for 15 min at 37°C. Digestion is stopped by adding MEF harvest medium. If large tissue aggregates remain, the tissue suspension can be passed through a cell strainer or directly transferred to a 15-ml Falcon tube, spun, and the supernatant aspirated. The tissue pellet is resuspended in MEF harvest medium and transferred to a T25 flask. The following day, the medium is refreshed to remove non-attached cells, tissue aggregates, and debris. The T25 flask should be between 70% to 90% confluent.
NOTE : As mentioned above, all animal work must comply with national and institutional requirements for the use of animals in research.
NOTE : Pregnant female mice are humanely euthanized by cervical dislocation. This procedure is performed according to approved standard operating procedures. The uterine horns are immediately placed on ice until the embryos have been decapitated according to the approved institutional protocols.
NOTE : To minimize the risk of contamination, the female mouse is extensively sprayed with ethanol, and tissue harvest and dissociation are performed under aseptic conditions.
NOTE : MEFs should be cultured in complete MEF medium without antibiotic-antimycotic after the first passage.
NOTE : If MEFs are kept in long-term culture, i.e., for immortalization, testing the culture for mycoplasma is recommended.
Materials
-
Mice (female and male, 8-10 weeks of age)
-
MEF harvest medium (see recipe)
-
Dulbecco's Modified Eagle Medium (DMEM), high glucose GibcoTM (ThermoFisher Scientific, cat. no. 11965092)
-
Fetal bovine serum (FBS; Sigma-Aldrich, cat. no. F9423)
-
GlutaMAXTM (ThermoFisher Scientific, cat. no. 350500610)
-
MEM non-essential amino acids (NEAA) GibcoTM (ThermoFisher Scientific, cat. no. 11140050)
-
Antibiotic-antimycotic (ThermoFisher Scientific, cat. no. 15240096)
-
Dulbecco's phosphate-buffered saline, no calcium, no magnesium (DPBS-/-) GibcoTM (ThermoFisher Scientific, cat. no. 14190250)
-
DPBS-/- 2% FBS (see recipe)
-
DPBS-/- antibiotic/antimycotic (see recipe)
-
70% (v/v) Ethanol (EtOH) (Labchem, 5 L, cat. no. AJA726-5LPL)
-
E13.5 mouse embryos
-
0.25% trypsin-EDTA (ThermoFisher Scientific, cat. no. 25200056)
-
Deoxyribonuclease I (DNase I) (Sigma Aldrich, cat. no. D5025)
-
MEF culture medium (see Reagents and Solutions section for the recipe)
-
Laminar flow hood (Safemate 1.2, Vision ABC, cat. no. LDE0820)
-
Water bath (Stuart SWB series, cat. no. SWB15D)
-
10-cm tissue culture plate (TPP, cat. no. 93100)
-
10-ml sterile serological pipette (Corning™, Costar Stripette, cat. no. CORN4488)
-
5-ml sterile serological pipette (Corning™, Costar Stripette, cat. no. CORN4487)
-
12-well tissue culture plate (Nunclon™, cat. no. 150628)
-
50-ml Falcon tube (Corning™, cat. no. 352070)
-
Paper, Bench Kleenex with Poly 91 mm × 415 mm (RSBS, cat. no. 917203)
-
Dissection scissors (Able Scientific, Onyx, cat. no. AS09340-10)
-
Large dissection forceps (Able Scientific, Onyx, cat. no. 11106-13)
-
Fine pointed dissection forceps (ProSci Tech, Style 5, cat. no. T65-SS)
-
Blades (Personna, 0.23 mm, cat. no. 61-0045)
-
1-ml filter pipette tips (Neptune Scientific, cat. no. BT1250)
-
6-cm tissue culture plate (TPP, cat. no. 93060)
-
Tissue culture incubator (Binder, C 150, cat. no. 9040 – 0062)
-
Cell strainer PP/Nylon, sterile, 100 μm (Westlab cat. no. 664-791)
-
15-ml Falcon tube (Corning™, cat. no. 352097)
-
Benchtop centrifuge (Beckman Coulter, Allegra® X-12R, cat. no. 392304)
-
Sterile aspiration pipette (Corning™, Costar®, cat. no. 9186)
-
Vacuum trap (Clements, 2 L collection Jar complete, cat. no. SUC 80312)
-
T25 flask (Nunc™, cat. no. 156367)
-
T75 flask (Nunc™, EasYFlask™, cat. no. 156499)
-
Inverted microscope (Nikon, Eclipse TS100, cat. no. KCC-REM-NCY-TS100LED)
-
300-μl filter pipette tips (Neptune Scientific, cat. no. BT300)
-
Beckman Coulter Counter cups (Beckman Coulter, 25 ml, cat. no. A35471)
-
Beckman Coulter counter (Beckman Coulter, Z2, cat. no. 6605700)
1.Set up timed matings to obtain embryos for harvest at E13.5.
2.Prepare MEF harvest medium (see recipe) in the laminar flow hood and prewarm in a 37°C water bath. Prepare DPBS-/- 2% FBS and DPBS-/- antibiotic/antimycotic (see recipes) in the laminar flow hood and place on ice.
3.Add 8 ml sterile DPBS-/- 2% FBS to two 10-cm tissue culture plates using a 10-ml sterile serological pipette and place on ice.
4.Prepare 3 (or more) additional 10-cm tissue culture plates by adding 2 ml sterile DPBS-/- 2% FBS using a 5-ml sterile serological pipette.
5.Add 2 ml/well sterile DPBS-/- 2% FBS to two 12-well tissue culture plates and place on ice.
6.Add 1 ml/well sterile DPBS-/- 2% FBS to a 12-well tissue culture plate and place on ice.
7.Prepare two 50-ml Falcon tubes by adding 40 ml DPBS-/- 2% FBS (see recipe) per tube. These will be used to immerse the uterine horns in succession.
8.Euthanize the pregnant female by cervical dislocation.
9.Place the female mouse supine on a paper bench barrier and spray generously with 70% EtOH before opening the abdominal cavity using dissection scissors (Fig. 1).
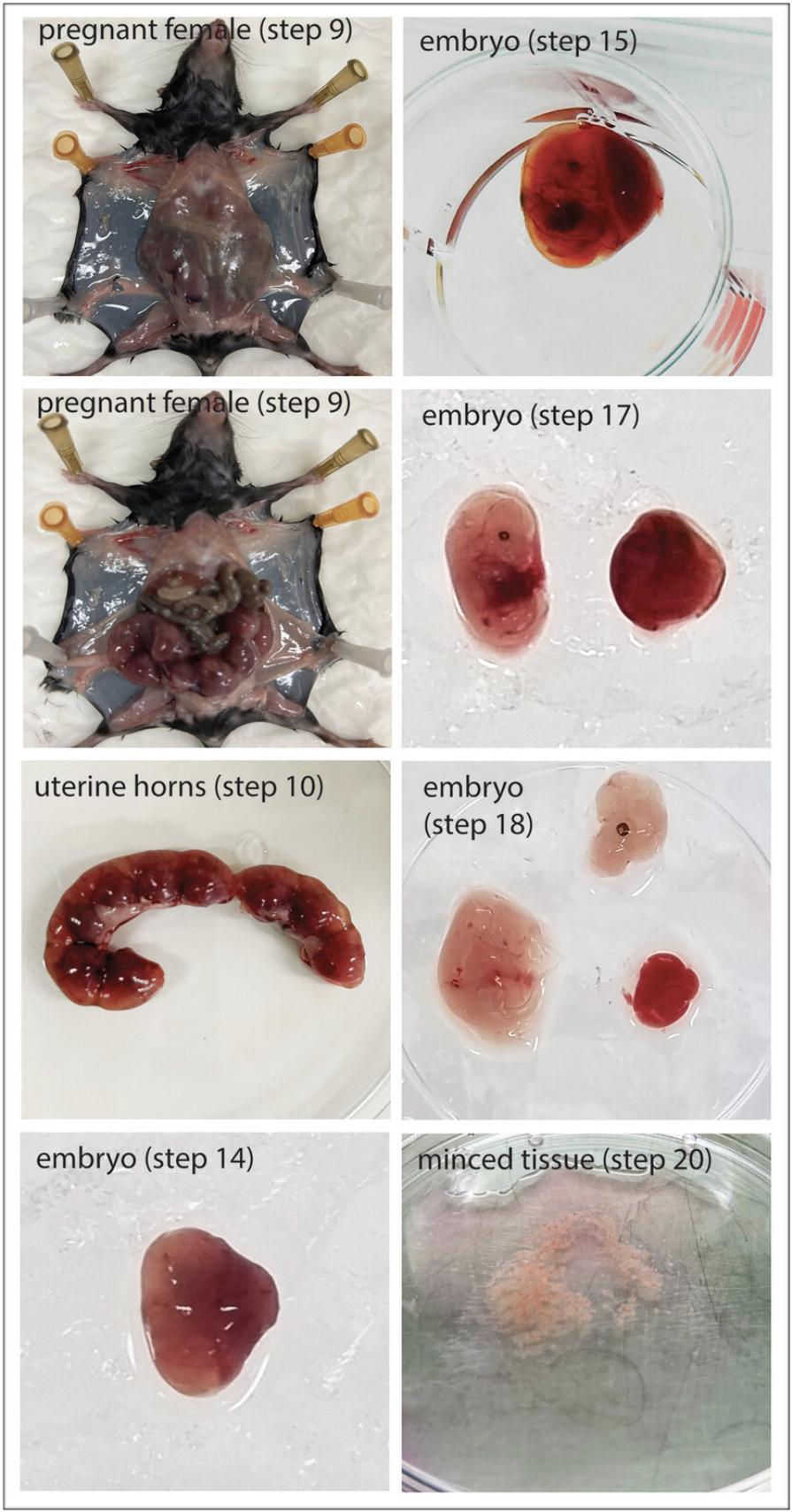
10.Use large dissection forceps to lift the uterus, remove membranes and fat tissue, and cut the cervix using dissection scissors to isolate the uterine horns. Place the uterine horns in a 10-cm tissue culture plate containing sterile DPBS-/- 2% FBS (Fig. 1).
11.Transfer the plate with uterine horns to the laminar flow hood to proceed aseptically.
12.Rinse the uterine horns twice by submerging them in two 50-ml Falcon tubes containing 40 ml sterile DPBS-/- antibiotic/antimycotic in succession.
13.Place uterine horns in a fresh 10-cm tissue culture plate containing 4 ml sterile DPBS-/- 2% FBS on ice.
14.Separate individual embryos by cutting the uterine wall between implantation sites using sterile dissection scissors. (Fig. 1).
15.Remove uterine tissue, rinse in 2 ml sterile DPBS-/- 2% FBS in a 12-well tissue culture plate, and place individual embryos into a new well of a 12-well tissue culture plate containing 1 ml sterile DPBS-/- 2% FBS, place on ice until all embryos are isolated (Fig. 1).
16.Place embryo in a 10-cm tissue culture plate with sterile DPBS-/- 2% FBS on ice.
17.Remove the yolk sac and placenta using two sterile fine-point dissection forceps (Fig. 1).
18.Decapitate embryo and remove the fetal liver and heart with two fine-point dissection forceps and rinse the embryo in 2 ml sterile DPBS-/- 2% FBS in a 12-well tissue culture plate (Fig. 1).
19.Transfer the embryo to a fresh 10-cm tissue culture plate containing 2 ml 0.25% trypsin-EDTA with DNase I (final concentration: 15 kU/ml) at room temperature (RT).
20.Mince embryo tissue finely with a sterile blade at RT until the tissue suspension becomes smooth and gloopy in consistency (Fig. 1).
21.Tilt plate and resuspend the tissue suspension with a 1-ml filter pipette tip.
22.Place a support (i.e., a 5- or 10-ml sterile serological pipette) under the plate to keep it at an angle and place in an incubator.
23.Incubate the tissue suspension at 37°C in a humidified 5% CO2 atmosphere for 15 min.
24.Add 4 ml warm MEF harvest medium to inactivate the 0.25% trypsin-EDTA with DNase I.
25.Pipette the tissue suspension up and down with a 5-ml sterile serological pipette until it is homogenous and transfer to a 15-ml Falcon tube. Keep at RT until all embryos are processed.
26.Spin the tissue suspension at 500 × g and RT for 5 min using a benchtop centrifuge to pellet the cells.
27.Carefully aspirate the supernatant using a sterile aspiration pipette and vacuum trap, or use a 10-ml sterile serological pipette if a vacuum trap is unavailable.
28.Resuspend the cell pellet in 5 ml prewarmed MEF harvest medium and transfer to a T25 flask.
29.Incubate for approximately 24 hr at 37°C, 5% CO2 under humidified conditions.
30.Check cell confluency the following day using an inverted microscope. If cell confluency has reached 80% to 90%, passage MEFs into a T75 flask for expansion or process for cryopreservation (see Supporting Protocol) (Fig. 2A).
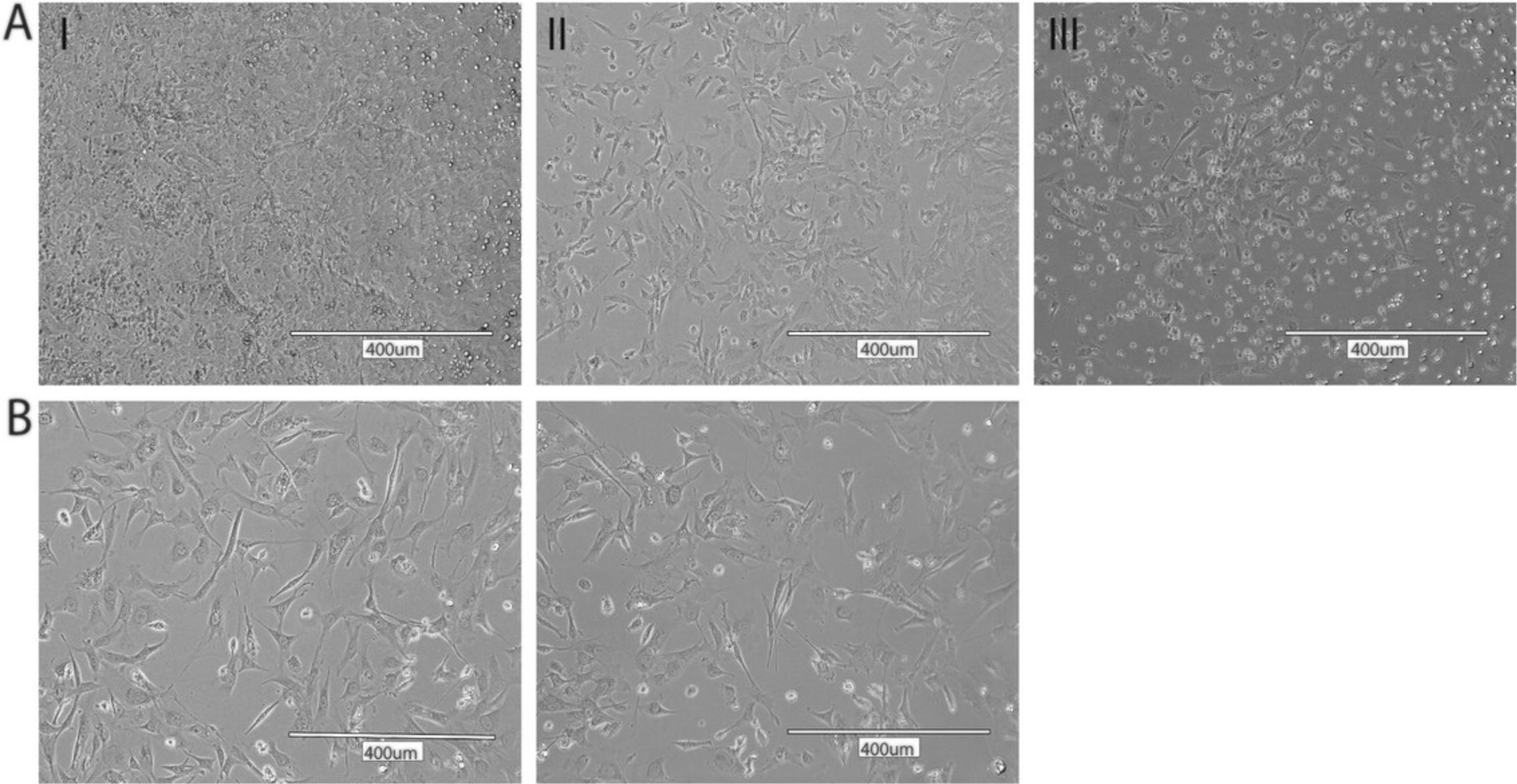
Passaging and expansion of MEFs
31.Prewarm MEF culture medium and DPBS-/- in a 37°C water bath and keep 0.25% trypsin-EDTA at RT.
32.Aspirate medium with a sterile aspiration pipette using a vacuum trap or a 10-ml sterile serological pipette if a vacuum trap is unavailable.
33.Wash cell monolayer once with sterile prewarmed DPBS-/-.
34.Aspirate DPBS-/- with a sterile aspiration pipette using a vacuum trap.
35.Add 0.25% trypsin-EDTA to the monolayer to detach adhered MEFs.
36.Incubate the flask at 37°C in a humidified 5% CO2 tissue culture incubator for around 5 min or until cells have detached.
37.Inactivate the 0.25% trypsin-EDTA digest by adding MEF culture medium and resuspending using a 5- or 10-ml sterile serological pipette.
38.Transfer an aliquot of the cell suspension to a Beckman Coulter Counter cup using a 300-μl filter pipette tip and perform a cell count using a Beckman Coulter Counter (according to manufacturer instructions: Beckman Coulter Z series User Manual 9914591-DA), an equivalent cell counter, or count manually using a hematocytometer (according to manufacturer instructions).
39.Transfer cells within the optimal MEF density range of ∼2- to 4 × 104 cells/cm2 to a fresh T75 flask and add MEF culture medium to a volume of 20 ml.
40.Incubate the T75 flask at 37°C in a humidified 5% CO2 atmosphere in a tissue culture incubator.
41.Check cell confluency daily using an inverted microscope. Freeze (see Support Protocol 1) or passage the cells when they reach 80%-90% confluency.
Support Protocol: CRYOPRESERVATION AND THAWING OF MEFs
MEFs can be cryopreserved at P0 if >80% confluent or after expansion at P1-5.The cells can be frozen naïve or, if isolated for use as feeder cells, after mitotic inactivation (Conner, 2001). When freezing mammalian cells, it is important to use a cryoprotective agent that prevents damage to the cell membrane by blocking ice crystal formation. Dimethyl Sulfoxide (DMSO) is most routinely used in mammalian tissue culture. Another aspect important for cryopreservation is the controlled gradual decrease in temperature at approximately −1°C per minute down to −80°C. Optimal cell recovery post cryopreservation requires rapid thawing of the cryovial and optimal cell density. To maintain high-quality cryopreserved MEFs, cryovials should be transferred to liquid nitrogen or specialized ultralow freezers (−150°C) for long-term storage.
Materials
- Mouse embryonic fibroblasts (MEFs)
- 70% (v/v) EtOH (Labchem, 5 L, cat. no. AJA726-5LPL)
- MEF culture medium (see recipe)
- Dulbecco's Modified Eagle Medium (DMEM), high glucose GibcoTM (ThermoFisher Scientific, cat. no. 11965092)
- Fetal bovine serum (FBS; Sigma-Aldrich, cat. no. F9423)
- GlutaMAXTM (ThermoFisher Scientific, cat. no. 350500610)
- MEM non-essential amino acids (NEAA) GibcoTM (ThermoFisher Scientific, cat. no. 11140050)
- MEF freeze-down medium (see recipe)
- DMSO (Sigma, cat. no. D8418)
- Dulbecco's phosphate-buffered saline, no calcium, no magnesium (DPBS-/-) GibcoTM (ThermoFisher Scientific, cat. no. 14190250)
- 0.25% trypsin-EDTA (ThermoFisher Scientific, cat. no. 25200056)
- T25 flask (Nunc™, cat. no. 156367)
- T75 flask (Nunc™, EasYFlask™, cat. no. 156499)
- Tissue culture incubator (Binder, C 150, cat. no. 9040 – 0062)
- Laminar flow hood (Safemate 1.2, Vision ABC, cat. no. LDE0820)
- Sterile aspiration pipette (Corning™, Costar®, cat. no. 9186)
- Vacuum trap (Clements, 2 L collection Jar complete, cat. no. SUC 80312)
- 10-ml sterile serological pipette (Corning™, Costar Stripette, cat. no. CORN4488)
- 5-ml sterile serological pipette (Corning™, Costar Stripette, cat. no. CORN4487)
- Inverted microscope (Nikon, Eclipse TS100, cat. no. KCC-REM-NCY-TS100LED)
- 15-ml Falcon tube (Corning™, cat. no. 352097)
- 300-μl filter pipette tips (Neptune Scientific, cat. no. BT300)
- Beckman Coulter Counter cups (Beckman Coulter, 25 ml, cat. no. A35471)
- Beckman Coulter Counter (Beckman Coulter, Z2, cat. no. 6605700)
- Benchtop centrifuge (Beckman Coulter, Allegra® X-12R, cat. no. 392304)
- 1-ml cryovials (Corning™, 1.2 ml, cat. no. 430487)
- 1-ml filter pipette tips (Neptune Scientific, cat. no. BT1250)
- CoolCell® LX Alcohol-Free Cryopreservation Container, ATCC, cat. no. ACS-6000)
- −80°C freezer (Haier, Salvum Ultimate, cat. no. DW-86L829BP)
- Water bath (Stuart, SWB Series, cat. no. SWB15D)
1.Expand MEFs in MEF culture medium in a T25 or T75 flask at 37°C, 5% CO2 and humified conditions in a tissue culture incubator until ∼80% confluent.
2.Prepare sterile MEF freeze-down medium under aseptic conditions in the laminar flow hood.
3.Aspirate medium with a sterile aspiration pipette and vacuum trap.
4.Wash cell monolayer once with sterile DPBS-/- using a 10-ml sterile serological pipette.
5.Aspirate DPBS-/- using a sterile aspiration pipette and vacuum trap.
6.Add prewarmed 0.25% trypsin-EDTA to the tissue culture flask to detach the cells using a 5-ml sterile serological pipette.
7.Incubate the flask at 37°C, 5% CO2 under humidified conditions in a tissue culture incubator for around 5 min or until cells have detached.
8.Add MEF culture medium to the flask to inactivate the 0.25% trypsin-EDTA digest and resuspend using a 5- or 10-ml sterile serological pipette to generate a homogeneous single-cell suspension.
9.Transfer the cell suspension to a 15-ml Falcon tube.
10.Transfer an aliquot of the cell suspension to a Beckman Coulter Counter cup using a 300-μl filter pipette tip and perform a cell count using a Beckman Coulter Counter (according to manufacturer instructions: Beckman Coulter Z series User Manual 9914591-DA), an equivalent cell counter, or count manually using a hematocytometer (according to manufacturer instructions).
11.Spin the cell suspension for 5 min at 500 × g and RT using a benchtop centrifuge.
12.Carefully aspirate the supernatant without disturbing the cell pellet using a sterile aspiration pipette and vacuum trap.
13.Resuspend the pellet thoroughly to dissociate any cell aggregates in the appropriate volume of freeze-down medium to freeze 2–5 × 106 cells/ml per cryovial.
14.Transfer 1 ml cell suspension in MEF freeze-down medium into each labeled cryovial using a 1-ml filter pipette tip.
15.Place the cryovials in a CoolCell® Cryopreservation container to allow a gradual temperature decrease at an optimal rate of −1°C per minute to −80°C.
16.Move the CoolCell® Cryopreservation container to a −80°C freezer for overnight storage.
17.Take cryovials from the CoolCell® Cryopreservation container and transfer cryopreserved MEFs to liquid nitrogen storage or an ultralow −150°C freezer for long-term storage.
Thawing of MEFs
18.Prepare and prewarm MEF culture medium in a 37°C water bath.
19.Add 9 ml prewarmed MEF culture medium to a 15-ml Falcon tube using a 10-ml sterile serological pipette.
20.Remove a MEF cryovial from liquid nitrogen or freezer and immediately place it in a 37°C water bath until defrosted.
21.Spray the MEF cryovial extensively with 70% EtOH to minimize the risk of contamination before opening in the laminar flow hood.
22.Transfer the thawed cell suspension dropwise into a 15-ml Falcon tube containing 9 ml prewarmed MEF culture medium using a 1-ml filter pipette tip.
23.Spin the cell suspension for 5 min at 500 × g and RT using a benchtop centrifuge to remove the DMSO from the MEF suspension.
24.Carefully aspirate the supernatant without disturbing the cell pellet using a sterile aspiration pipette and vacuum trap.
25.Resuspend the cell pellet carefully in 5 ml prewarmed MEF culture medium and transfer to a tissue culture flask using a sterile 5-ml serological pipette. If MEFs are cultured in a T75 flask, add 15 ml MEF culture medium to the flask using a 10-ml sterile serological pipette.
26.Incubate the tissue culture flask at 37°C, 5% CO2, under humidified conditions in a tissue culture incubator.
27.Check viability, morphology, and percent confluency of MEFs the following day using the inverted microscope (Fig. 2B).
REAGENTS AND SOLUTIONS
Prepare all recipes using aseptic technique.
DPBS-/-, 1× antibiotic-antimycotic (v/v): 495 ml DPBS-/-, 5 ml 100× antibiotic-antimycotic
DPBS-/-, 2% FBS, antibiotic-antimycotic: 485 ml DPBS-/-, 10 ml FBS, 5 ml 100× antibiotic-antimycotic
DPBS-/-, 2% FBS (v/v): 490 ml DPBS-/-, 10 ml FBS
MEF culture medium (DMEM, 10% FBS, NEAA, Glutamax): 440 ml DMEM high glucose, 50 ml fetal bovine serum, 5 ml 100× NEAA, 5 ml 100× Glutamax
MEF freeze-down medium (DMEM, 20% FBS, 10% DMSO): 50 ml DMEM high glucose, 10 ml FBS, 5 ml DMSO
MEF harvest medium (DMEM, 10% FBS, antibiotic-antimycotic, NEAA, 2 mM Glutamax): 445 ml DMEM high glucose, 50 ml fetal bovine serum, 5 ml 100× antibiotic-antimycotic, 5 ml 100× NEAA, 5 ml 100× Glutamax
COMMENTARY
Background Information
MEFs are a heterogenous fibroblast population of mesenchymal origin. Todaro and Green first studied and described mouse embryonic cell culture and growth characteristics in 1963. They culture-adapted mouse-derived embryo cells isolated from E15-17 to in vitro growth conditions through continuous passaging and generated the widely used NIH/3t3 fibroblast cell line, which has unlimited proliferative potential (Marigliani et al., 2019; Todaro & Green, 1963). The basic principle of isolating MEFs from embryos at a defined time after mating and tissue dissociation by mincing and enzymatic digestion has remained similar to the original procedure (Michalska, 2007; Qiu et al., 2016; Xu, 2005).
Over time, however, specific steps have been modified, such as the duration of tissue digestion, digestion with or without agitation, and the addition of DNase to the 0.25% trypsin-EDTA for genomic DNA degradation. The duration of tissue digestion in trypsin-EDTA varies from overnight at 4°C to 10-30 min at 37°C with or without agitation to achieve the best possible tissue homogenization without reducing cell viability and proliferation capacity. For the same reason, DNase I digestion of gDNA further improves tissue dissociation after mincing and facilitates pipetting of the tissue suspension. The time of harvest usually varies between E11.5 and E15.5, while E13.5-15.5 is considered optimal for MEF isolation. Though not essential, some protocols utilize gelatin-coated flasks to facilitate cell attachment of freshly isolated MEFs (Jozefczuk et al., 2012; Michalska, 2007; Qiu et al., 2016; Xu, 2005).
Primary MEFs are used for various applications. In the early 1980s, the first mouse ESC cultures were isolated and propagated on feeder cells, although Evans and Kaufman did not utilize primary MEFs (Evans & Kaufman, 1981). Mitotically inactivated MEFs are now one of the most commonly used feeder cell layers to maintain the undifferentiated growth of ESC and iPSC cultures. As MEF preparations can differ in quality and require frequent renewal due to their finite expansion potential, some laboratories use Sandos inbred mice (SIM) 6-thioguanine-resistant, ouabain-resistant (STO) cell lines generated from SIM (Ware & Axelrad, 1972) or switched to feeder-free culture systems. While still controversial in ESC cultures, Saitoh et al. showed that MEFs are superior to permanent STO-feeders when first establishing iPSCs (Saitoh et al., 2015). However, undifferentiated mESCs can also be maintained on other embryonic fibroblast-derived cell lines, including C3H 10T1/2 (Rathjen et al., 1990).
Generally speaking, MEFs are easy to obtain and culture. A major advantage of primary MEFs is their non-transformed state characterized by the absence of oncogenic features; thus, they are a popular cell model for studying “normal” biochemical processes and cellular signaling. MEFs can be a suitable model system for investigating mesoderm-type cell differentiation (Dastagir et al., 2014; Saeed et al., 2012). Furthermore, MEFs are an excellent in vitro model to study gene function and the functional consequences of genetic alteration. For example, MEFs are used to validate the loss or mutation of a specific gene and its effect on pathway activation and the epigenetic landscape (Hannan et al., 2022; Moss et al., 2019). Therefore, MEFs can be isolated from genetically modified mouse strains or genetically altered in vitro as a more simplistic means to analyze gene function. Recently, MEFs have been used as a model system to assess developmental toxicity (Hansen & Piorczynski, 2019).
A major disadvantage of MEFs is their limited proliferative capacity of approximately 20 cell divisions ex vivo (Karatza & Shall, 1984), equivalent to five passages. Thus, MEF stocks require frequent renewal, which is time-consuming. The non-physiological oxygen level is an environmental condition limiting the replicative life span of MEFs ex vivo (Parrinello et al., 2003).
Despite their limited proliferation potential, MEFs are easily immortalized through continuous passaging or genetic modifications that generate MEF-derived cell lines with infinite proliferative capacity. Immortalization through serial passage most likely occurs due to environmentally induced changes that cause a growth advantage and prevent apoptotic cell death and senescence.
Another disadvantage is considerable batch-to-batch variation, which leads to differences in cell viability and proliferative potential. These variations can be reduced through high technical skill and optimized protocols but have not been entirely resolved.
Critical Parameters
We recommend accounting for variability in mating success (Table 1), as not all matings produce pregnancies. Mating success can be increased by housing stud mice individually 1-2 weeks before mating and by selecting females that are in the proestrus or oestrus state (Byers et al., 2012) (https://www.jax.org/news-and-insights/jax-blog/2014/september/six-steps-for-setting-up-timed-pregnant-mice). Timed matings are routinely set up as 1 male with 2 females (8-10 weeks of age) overnight. The following morning, the female mice are examined for the presence of a vaginal plug.
Problem | Possible cause | Solution |
---|---|---|
Low mating success |
Female mouse is not in the proestrus-oestrus stage Oestrous cycles are not synchronized Low sperm count, low sperm viability |
Steps to improve mating success: see critical parameters |
Low/variable cell number per embryo |
Insufficient tissue homogenization Inefficient tissue digestion Intrinsic variability |
Extend time spent mincing tissue Increase the number of times the tissue is resuspended Use fresh 0.25% trypsin-EDTA |
Low cell viability after isolation or passaging | 0.25% trypsin-EDTA DNase digestion too long |
Do not exceed recommended digestion time or enzyme concentration. Do not passage MEFs below the recommended density per cm2 |
Culture Contamination |
Contamination is introduced during dissection of the uterine horns under non-sterile conditions, i.e., carryover of mouse hair Poor aseptic technique |
Improve aseptic techniques, spray mouse multiple times with 70% EtOH to account for evaporation, increase number of washes in sterile DPBS-/-, change dissection instruments after each step |
Low cell viability after thawing |
Cells were not exponentially proliferating at the time of cryopreservation Cell number per cryovial too low or high |
Ensure optimal rate of −1°C per min cooling by using a specific cryopreservation container Freeze cells at defined density |
The mouse strain utilized to generate MEFs depends on the downstream application. Certain mouse strains have smaller litter sizes that need to be accounted for—the number of female mice set up for timed mating may need to be increased. The difference in litter sizes may be due to wild-type inbred strain-specific differences. For example, C57BL/6 litter sizes range from 5 to 8 pups, while FVB/NJ mice have litter sizes of 8 to 10 pups, and differences can be the phenotypic consequence of gene ablation or modification (Ferreira et al., 2007). Thus, researchers must consider the average litter size when setting up the required number of timed matings. When using genetically modified mouse strains, it is important to check for increased mortality of embryos on specific days during embryonic development. Whenever possible, the embryonic day of MEF isolation should be before any phenotypic changes are observable to avoid isolating MEFs that fail to proliferate and/or survive in culture.
The size and state of the embryo will determine the proliferative capacity and overall quality of the derived MEFs. For example, suppose MEFs are not used for phenotypic characterization of specific genotypes but to generate feeder cells. In that case, we do not recommend generating MEFs from embryos that appear markedly smaller (∼25% smaller than embryos from the same female) or morphologically abnormal. In principle, MEFs can be isolated from any genetically modified mouse strain; however, if the genetic alteration is embryonic lethal before harvest (E13.5, for example), MEFs can be purified from embryos as early as E8.5.
Cell viability, cell number, and proliferative capacity are impacted by the efficiency and duration of the enzymatic tissue digestion (Table 1); thus, it is inadvisable to exceed the recommended digestion time or trypsin-EDTA concentration, and it is critical to only use tissue culture-grade reagents before their expiration date. High cell confluency (>70%) and low level of debris 24 hr post-harvest is indicative of a high-quality preparation. Ex vivo MEFs are cultured under oxidative conditions since physiological oxygen levels are around 3%, and MEFs grown in culture are exposed to 21% oxygen, which facilitates/accelerates replicative senescence (Parrinello et al., 2003).
Contamination of MEF cultures most likely occurs at the time of harvest or due to poor aseptic technique (Table 1). To minimize the risk of contamination during isolation, spray the euthanized female mouse extensively with 70% EtOH before dissecting the uterine horns. Once isolated, transfer the uterine horns in a 10-cm tissue culture plate to a laminar flow hood, rinse twice in sterile DPBS-/-, and perform all subsequent steps under aseptic conditions. To avoid contamination of media, reagents, and other consumables used for MEF harvest and culture, prepare all materials under strict aseptic conditions and purchase reagents, media, and single-use consumables from known suppliers. All reusable dissection equipment must be sterilized/autoclaved.
MEFs should only be frozen in the exponential proliferation phase at 80% to 90% confluence, and the number of cells per cryovial should not be less than 1 × 106 cells/vial nor exceed 5 × 106 cells/vial (Table 1). To ensure optimal cell recovery after thawing, remove DMSO and transfer to an appropriately sized tissue culture flask depending on the number of cells in the cryovial. Low cell numbers can affect cell recovery as MEFs require cell–cell interactions for optimal growth. During cryopreservation, a gradual decrease in temperature is essential to prevent cell damage, and rapid thawing minimizes the cytotoxic effects of the cryoprotectant.
Troubleshooting
Understanding Results
MEF preparations from wild-type embryos are expected to reach 80% to 90% confluence in a T25 flask within 1-2 days after purification. Depending on the genetic alteration, MEFs isolated from genetically modified animals may exhibit different proliferation rates. Many dead cells and debris are expected within the first 24 hr post-preparation and should decrease significantly after the first medium exchange. The average doubling time of P1-P4 wild-type MEFs can vary between 28 hr and 48 hr and decreases with further passages due to the limited proliferation potential. Between passages 0 and 4/5, MEF morphology remains mesenchymal fibroblastic, while MEFs passaged beyond P5/6 display an increased percentage of senescent cells with an enlarged, flat, and frequently multinucleated morphology (Chen et al., 2020; Dirac & Bernards, 2003; Manning & Kumar, 2010). Cell viability of high-quality cryopreserved MEFs after thawing is expected to be around 80%.
Time Considerations
The time required to purify MEFs includes the timed mating, which ranges from E12.5 to 15.5. At the time of harvest, the time required to isolate MEFs depends on the number of pregnant females and embryos per female. The preparation of reagents (FBS and other media supplements should be defrosted overnight at 4°C) and set-up of the harvest area and laminar flow hood takes around 30 min. Euthanasia and dissection of the uterine horns take around 5 min per mouse. Embryo isolation, tissue removal, mincing, and tissue digestion require approximately 30 min per embryo. These steps can be staggered so that the next embryo can be processed during tissue digestion for the previous embryo. Centrifugation, aspiration of the supernatant, resuspension of the tissue pellet, and transfer to a pre-labeled T25 flask will take another 10-20 min, depending on the number of samples. Overall, 2-2.5 hr should be allowed for the MEF purification from one pregnant female with a litter size of 8-9 embryos.
Passaging, including washing, trypsinization, resuspension, cell counting, and transfer to new flasks, is around 15-20 min per flask, prewarming of tissue culture reagents excluded.
Cryopreservation takes around 30 min per flask, including washing, trypsinization, resuspension, cell counting, centrifugation, resuspending in freeze-down medium, and transfer to prelabeled cryovials.
Thawing takes around 15-20 min.
Acknowledgments
This work and salaries were funded by the National Health and Medical Research Council of Australia Ideas Grants (#38658).
Open access publishing facilitated by Australian National University, as part of the Wiley - Australian National University agreement via the Council of Australian University Librarians.
Author Contributions
Rita Ferreira : Writing – review and editing. Nadine Hein : Conceptualization; methodology; visualization; writing – original draft.
Conflict of Interest
The authors have no conflict of interest.
Open Research
Data Availability Statement
Data sharing is not applicable – no new data were generated.
Literature Cited
- Byers, S. L., Wiles, M. V., Dunn, S. L., & Taft, R. A. (2012). Mouse estrous cycle identification tool and images. PLoS ONE , 7(4), e35538. https://doi.org/10.1371/journal.pone.0035538
- Chen, W., Wang, X., Wei, G., Huang, Y., Shi, Y., Li, D., Qiu, S., Zhou, B., Cao, J., Chen, M., Qin, P., Jin, W., & Ni, T. (2020). Single-cell transcriptome analysis reveals six subpopulations reflecting distinct cellular fates in senescent mouse embryonic fibroblasts. Frontiers in Genetics , 11, 867. https://doi.org/10.3389/fgene.2020.00867
- Conner, D. A. (2001). Mouse embryo fibroblast (MEF) feeder cell preparation. Current Protocols in Molecular Biology , 51, 23.2.1–23.2.7. https://doi.org/10.1002/0471142727.mb2302s51
- Dastagir, K., Reimers, K., Lazaridis, A., Jahn, S., Maurer, V., Strauss, S., Dastagir, N., Radtke, C., Kampmann, A., Bucan, V., & Vogt, P. M. (2014). Murine embryonic fibroblast cell lines differentiate into three mesenchymal lineages to different extents: New models to investigate differentiation processes. Cell Reprogramming , 16(4), 241–252. https://doi.org/10.1089/cell.2014.0005
- Dirac, A. M., & Bernards, R. (2003). Reversal of senescence in mouse fibroblasts through lentiviral suppression of p53. Journal of Biological Chemistry , 278(14), 11731–11734. https://doi.org/10.1074/jbc.C300023200
- Evans, M. J., & Kaufman, M. H. (1981). Establishment in culture of pluripotential cells from mouse embryos. Nature , 292(5819), 154–156. https://doi.org/10.1038/292154a0
- Ferreira, R., Wai, A., Shimizu, R., Gillemans, N., Rottier, R., von Lindern, M., Ohneda, K., Grosveld, F., Yamamoto, M., & Philipsen, S. (2007). Dynamic regulation of Gata factor levels is more important than their identity. Blood , 109(12), 5481–5490. https://doi.org/10.1182/blood-2006-11-060491
- Graham, M. D. (2022). The coulter principle: A history. Cytometry Part A: The Journal of the International Society for Analytical Cytology , 101(1), 8–11. https://doi.org/10.1002/cyto.a.24505
- Hannan, K. M., Soo, P., Wong, M. S., Lee, J. K., Hein, N., Poh, P., Wysoke, K. D., Williams, T. D., Montellese, C., Smith, L. K., Al-Obaidi, S. J., Nunez-Villacis, L., Pavy, M., He, J. S., Parsons, K. M., Loring, K. E., Morrison, T., Diesch, J., Burgio, G., … George, A. J. (2022). Nuclear stabilization of p53 requires a functional nucleolar surveillance pathway. Cell Reports , 41(5), 111571. https://doi.org/10.1016/j.celrep.2022.111571
- Hansen, J. M., & Piorczynski, T. B. (2019). Use of primary mouse embryonic fibroblasts in developmental toxicity assessments. Methods in Molecular Biology , 1965, 7–17. https://doi.org/10.1007/978-1-4939-9182-2_2
- Jozefczuk, J., Drews, K., & Adjaye, J. (2012). Preparation of mouse embryonic fibroblast cells suitable for culturing human embryonic and induced pluripotent stem cells. Journal of Visualized Experiments , 64, 3854. https://doi.org/10.3791/3854
- Karatza, C., & Shall, S. (1984). The reproductive potential of normal mouse embryo fibroblasts during culture in vitro. Journal of Cell Science , 66, 401–409. https://doi.org/10.1242/jcs.66.1.401
- Llames, S., Garcia-Perez, E., Meana, A., Larcher, F., & del Rio, M. (2015). Feeder layer cell actions and applications. Tissue Engineering Part B: Reviews , 21(4), 345–353. https://doi.org/10.1089/ten.TEB.2014.0547
- Manning, J. A., & Kumar, S. (2010). A potential role for NEDD1 and the centrosome in senescence of mouse embryonic fibroblasts. Cell Death & Disease, 1(4), e35. https://doi.org/10.1038/cddis.2010.12
- Marigliani, B., Balottin, L. B. L., & Augusto, E. F. P. (2019). Adaptation of mammalian cells to chemically defined media. Current Protocols in Toxicology , 82(1), e88. https://doi.org/10.1002/cptx.88
- Martin, G. R., & Evans, M. J. (1975). Differentiation of clonal lines of teratocarcinoma cells: Formation of embryoid bodies in vitro. PNAS , 72(4), 1441–1445. https://doi.org/10.1073/pnas.72.4.1441
- Michalska, A. E. (2007). Isolation and propagation of mouse embryonic fibroblasts and preparation of mouse embryonic feeder layer cells. Current Protocols in Stem Cell Biology , 3, 1C.3.1–1C.3.17. https://doi.org/10.1002/9780470151808.sc01c03s3
- Moss, T., Mars, J. C., Tremblay, M. G., & Sabourin-Felix, M. (2019). The chromatin landscape of the ribosomal RNA genes in mouse and human. Chromosome Research , 27(1–2), 31–40. https://doi.org/10.1007/s10577-018-09603-9
- Parrinello, S., Samper, E., Krtolica, A., Goldstein, J., Melov, S., & Campisi, J. (2003). Oxygen sensitivity severely limits the replicative lifespan of murine fibroblasts. Nature Cell Biology , 5(8), 741–747. https://doi.org/10.1038/ncb1024
- Qiu, L. Q., Lai, W. S., Stumpo, D. J., & Blackshear, P. J. (2016). Mouse embryonic fibroblast cell culture and stimulation. Bio-Protocol , 6(13). e1859. https://doi.org/10.21769/BioProtoc.1859
- Rathjen, P. D., Toth, S., Willis, A., Heath, J. K., & Smith, A. G. (1990). Differentiation inhibiting activity is produced in matrix-associated and diffusible forms that are generated by alternate promoter usage. Cell , 62(6), 1105–1114. https://doi.org/10.1016/0092-8674(90)90387-t
- Saeed, H., Taipaleenmaki, H., Aldahmash, A. M., Abdallah, B. M., & Kassem, M. (2012). Mouse embryonic fibroblasts (MEF) exhibit a similar but not identical phenotype to bone marrow stromal stem cells (BMSC). Stem Cell Reviews and Reports , 8(2), 318–328. https://doi.org/10.1007/s12015-011-9315-x
- Saitoh, I., Inada, E., Iwase, Y., Noguchi, H., Murakami, T., Soda, M., Kubota, N., Hasegawa, H., Akasaka, E., Matsumoto, Y., Oka, K., Yamasaki, Y., Hayasaki, H., & Sato, M. (2015). Choice of feeders is important when first establishing iPSCs derived from primarily cultured human deciduous tooth dental pulp cells. Cell Medicine , 8(1–2), 9–23. https://doi.org/10.3727/215517915X689038
- Saitoh, I., Sato, M., Iwase, Y., Inada, E., Nomura, T., Akasaka, E., Yamasaki, Y., & Noguchi, H. (2012). Generation of mouse STO feeder cell lines that confer resistance to several types of selective drugs. Cell Medicine , 3(1–3), 97–102. https://doi.org/10.3727/215517912X639414
- Smith, A. G., Heath, J. K., Donaldson, D. D., Wong, G. G., Moreau, J., Stahl, M., & Rogers, D. (1988). Inhibition of pluripotential embryonic stem cell differentiation by purified polypeptides. Nature , 336(6200), 688–690. https://doi.org/10.1038/336688a0
- Strober, W. (2015). Trypan blue exclusion test of cell viability. Current Protocols in Immunology , 111, A3.B.1–A3.B.3. https://doi.org/10.1002/0471142735.ima03bs111
- Todaro, G. J., & Green, H. (1963). Quantitative studies of the growth of mouse embryo cells in culture and their development into established lines. Journal of Cell Biology , 17(2), 299–313. https://doi.org/10.1083/jcb.17.2.299
- Ware, L. M., & Axelrad, A. A. (1972). Inherited resistance to N- and B-tropic murine leukemia viruses in vitro: Evidence that congenic mouse strains SIM and SIM.R differ at the Fv-1 locus. Virology , 50(2), 339–348. https://doi.org/10.1016/0042-6822(72)90385-6
- Williams, R. L., Hilton, D. J., Pease, S., Willson, T. A., Stewart, C. L., Gearing, D. P., Wagner, E. F., Metcalf, D., Nicola, N. A., & Gough, N. M. (1988). Myeloid leukaemia inhibitory factor maintains the developmental potential of embryonic stem cells. Nature , 336(6200), 684–687. https://doi.org/10.1038/336684a0
- Xu, J. (2005). Preparation, culture, and immortalization of mouse embryonic fibroblasts. Current Protocols in Molecular Biology , 70, 28.1.1–28.1.8. https://doi.org/10.1002/0471142727.mb2801s70
Internet Resources
Citing Literature
Number of times cited according to CrossRef: 1
- Meirong Wu, Xiaojing Zhang, Yanjuan Tu, Wenzhao Cheng, Yiming Zeng, Culture and expansion of murine proximal airway basal stem cells, Stem Cell Research & Therapy, 10.1186/s13287-024-03642-2, 15 , 1, (2024).