Multiplex Quantification of Plasma Biomarkers for Patients with Gaucher Disease Type 1
Iskren Menkovic, Iskren Menkovic, Michel Boutin, Michel Boutin, Pamela Lavoie, Pamela Lavoie, Christiane Auray-Blais, Christiane Auray-Blais
biomarker
Gaucher disease
Lyso-Gb1
Lyso-Gb1 analogs
mass spectrometry
N-palmitoyl-O-phosphocholineserine
sphingosylphosphorylcholine
Abstract
Gaucher disease (GD) is a lysosomal storage disorder caused by a deficiency of the enzyme beta-glucocerebrosidase. This leads to the accumulation of glycolipids in macrophages and ultimately results in tissue damage. Recent metabolomic studies highlighted several potential biomarkers in plasma specimens. In hopes of better understanding the distribution, importance, and clinical significance of these potential markers, a UPLC-MS/MS method was developed and validated to quantify lyso-Gb1 and six related analogs (with the following modifications on the sphingosine moiety: -C2H4 (-28 Da), -C2H4+O (-12 Da), -H2 (-2 Da), -H2+O (+14 Da), +O (+16 Da), and +H2O (+18 Da)), sphingosylphosphorylcholine, and N- palmitoyl-O-phosphocholineserine in plasma specimens of treated and untreated patients. This 12-min UPLC-MS/MS method involves a purification step via solid-phase extraction followed by evaporation under nitrogen flow and resuspension in an organic mix compatible with HILIC chromatography. This method is currently used for research purposes and might be used for monitoring, prognostics, and follow-up. © 2023 The Authors. Current Protocols published by Wiley Periodicals LLC.
INTRODUCTION
Gaucher disease (GD) is a lysosomal storage disorder caused by biallelic pathogenic variants in the GBA1 gene (Özdemir, 2022). This results in enzymatic deficiencies of beta-glucocerebrosidase (GCase, EC 3.2.1.45), which is involved in the degradation pathway of glucosylceramide and leads to the accumulation of several glycolipids within the lysosomes of tissues including the spleen, liver, bone marrow, and occasionally the lungs, ultimately resulting in multisystemic complications (Grabowski, Antommaria, Kolodny, and Mistry, 2021).
GD is characterized by marked phenotypic and genotypic variability (D'Amore et al., 2021; Grabowski et al., 2021; Özdemir, 2022). Over 300 different mutations have been described for the GBA gene (D'Amore et al., 2021) and result in phenotypes ranging from asymptomatic to in-utero death (Arévalo et al., 2022; Weinreb et al., 2021. Although GD phenotypes span a wide continuum, three types can be distinguished (Schiffmann et al., 2020; Silva García et al., 2021). Type 1 GD (OMIM 230800) accounts for approximately 90% to 95% of all GD cases, with an estimated disease prevalence of 1 in 52,000 individuals in the general population (Biegstraaten et al., 2018; Chis, Chis, and Dumitrascu, 2021; Giraldo, 2021). This number can, in certain communities, be significantly increased. Indeed, GD affects approximately 1 newborn out of 800 in Ashkenazi Jewish communities (Mistry et al., 2020; Stirnemann et al., 2017). Type 1 GD is known for being a more attenuated form of the disease (Mistry et al., 2020). Typically, neurological involvement is not observed in GD type 1, although some have described attenuated neurological features. Other clinical manifestations may include hepatosplenomegaly, cytopenia, and bone or pulmonary involvement (Essabar et al., 2015; Miyamoto et al., 2021). GD type 3 (OMIM 231000), known as the chronic neuronopathic form of the disease, generally involves some degree of neurological impairment in addition to the somatic symptoms potentially observed in GD type 1 (Donald et al., 2020). Finally, GD type 2 (OMIM 230900) is the most severe form of the disease. Neurological involvement may appear in the first months of life and lead to death within the first years of life (Gary, Ryan, Steward, and Sidransky, 2018; Marshall et al., 2016; Sam, Ryan, Daykin, and Sidransky, 2021).
Enzyme replacement therapy (ERT) and substrate reduction therapy are available (Gary et al., 2018; Marshall et al., 2016). Although some challenges remain, such as the inability of ERT to cross the blood-brain barrier, studies have demonstrated the clinical efficacy of these treatments (Andrade-Campos et al., 2017). Moreover, experts have argued about the potential benefits of early treatment initiation (Andrade-Campos et al., 2017; Charrow, 2015; Mistry et al., 2009). It is believed that starting treatment early would prevent the accumulation of cytotoxic metabolites that lead to permanent and irreversible tissue damage (Charrow, 2015; Mistry et al., 2009). To achieve this, sensitive molecular and biochemical tests are required. Several biomarkers are used in clinical labs: chitotriosidase, angiotensin-converting enzyme (ACE), tartrate-resistant acid phosphatase (TRAP5b), and C-C motif ligand 18 (CCL18) can be quantified in blood specimens for routine follow-up (Giraldo, López de Frutos, and Cebolla, 2018; Stirnemann et al., 2017). Unfortunately, these markers are not specific to GD and are not well adapted for diagnostic purposes (Giraldo et al., 2018; Stirnemann et al., 2017). In recent years, the analysis of lyso-Gb1 emerged as a gold standard for monitoring disease progression (Dinur et al., 2021; Revel-Vilk, Fuller, and Zimran, 2020). Studies revealed that lyso-Gb1 is a highly sensitive biomarker (Dinur et al., 2021; Revel-Vilk et al., 2020) and that lyso-Gb1 plasma levels correlate with disease severity (Revel-Vilk et al., 2020). Although lyso-Gb1 represents a significant upgrade from previous markers, it is possible, considering the major phenotypic variability associated with the disease, that other markers in patient plasma may correlate with specific clinical manifestations. To investigate this hypothesis, our research group used a metabolomic approach to identify metabolites potentially associated with the condition in untreated patient plasma samples (Menkovic et al., 2020). This specific metabolomic study using ultra-performance liquid chromatography time-of-flight mass spectrometry highlighted several potential biomarkers, namely lyso-Gb1 and four related analogs (with the following modifications on the sphingosine moiety: -C2H4 (-28 Da), -H2 (-2 Da), -H2+O (+14 Da), and +H2O (+18 Da)), sphingosylphosphorylcholine (SPC), and N- palmitoyl-O-phosphocholineserine (NPOPCS) (Menkovic et al., 2020). After structural identification of these metabolites, we developed and validated a quantification method for these compounds and two other lyso-Gb1 analogs (see Background Information) using ultra-performance liquid chromatography/tandem mass spectrometry (UPLC-MS/MS) to investigate marker distributions and their clinical significance (Menkovic et al., 2022a). The validation step revealed that the method had high sensitivity with low limits of quantification and detection as well as high levels of accuracy and precision (Menkovic et al., 2022a). Herein, we provide an in depth protocol for the method developed to study the distribution of metabolites identified in our metabolomic study.
Basic Protocol:
Materials
-
Surrogate matrix (see recipe)
-
H3PO4 (2% v/v in water) (see recipe)
-
Calibration curve/QC working solutions (see recipe)
-
Internal standard working solution (see recipe)
-
Methanol:Dimethyl sulfoxide (DMSO) 95:5 v/v solution (see recipe)
-
Formic acid 2% v/v solution in water (see recipe)
-
Formic acid 0.2% v/v in methanol (see recipe)
-
Ammonium hydroxide 2% v/v in methanol solution (see recipe)
-
Nitrogen evaporator (homemade)
-
Mobile phase A solution (see recipe)
-
Mobile phase B solution (see recipe)
-
Sodium citrate (light blue) blood collection tubes
-
Centrifuge (Beckman GPR Centrifuge Model 7C008)
-
1.5-ml polypropylene tubes (Sarstedt, cat. no. 72.690.300)
-
13 × 100–mm disposable culture tubes (Fisher Scientific, cat. no. 14-961-27)
-
16 × 150–mm disposable culture tubes (Fisher Scientific, cat. no. 14-961-31)
-
2-ml vials (Waters Corp. Milford, MA, USA, cat. no 186000307C)
-
Oasis MCX cartridges, 60 μm, 30 mg, LP (Waters, cat. no. 186000782)
-
Inserts, 250-μl, 6 × 29 mm (Chromatographic Specialities, cat. no. C221020M)
-
Preslit polytetrafluoroethylene (PTFE) silicone septum (Chromatographic Specialities, cat. no.C779200XBBM)
-
Halo HILIC 2.7 μm 4.6 × 150 mm (Advanced Materials Technology, cat. no. 92814701)
-
Xevo TQ-S mass spectrometer coupled with an Acquity I-Class UPLC system (Waters)
Plasma collection
1.Collect blood samples in sodium citrate vacutainer blood collection tubes (light blue).
2.Centrifuge at room temperature for 10 min at 1000 × g to extract plasma from the whole blood samples.
3.Transfer the plasma specimens into polypropylene tubes.
4.Keep at −20°C until shipping and analysis.
Experimental Protocol
5.Thaw the following solutions:
- - Quality control plasma specimens (healthy control samples)
- - Calibration curve/QC working solutions
- - Plasma samples to analyze
- - Internal standard working solution
6.Before use, vortex each solution for 10 s, followed by 1 min of sonication.
7.Repeat 3 times.
Sample Preparation
QC samples and calibration curve points
8.In fourteen 1.5-ml Eppendorf polypropylene tubes, add 200 μl of the surrogate matrix.
9.Add 500 μl H3PO4 (2% v/v in water).
10.Add 20 μl calibration curve or QC working solution.
11.Add 500 μl internal standard working solution.
12.Vortex 10 s.
Patients and Controls
13.In a 1.5-ml Eppendorf polypropylene tube, add 200 μl of plasma.
14.Add 500 μl H3PO4 (2% v/v in water).
15.Add 20 μl methanol:dimethyl sulfoxide (DMSO, 95:5 v/v).
16.Add 500 μl internal standard working solution.
17.Vortex 10 s.
Plasma specimen SPE extraction
18.Label one 13 × 100–mm disposable culture tube, one 16 × 150–mm disposable culture tube, and one 2-ml borosilicate vial for each sample ID.
19.Deposit an Oasis MCX cartridge into each 16 × 150–mm disposable culture tube.
20.Cartridge conditioning 1: Add 1200 μl methanol to the cartridge using an automatic pipette and let it pass through via gravity.
21.Cartridge conditioning 2: Add 1200 μl H3PO4 (2% v/v in water) to the cartridge using an automatic pipette and let it pass through via gravity.
22.Transfer previously prepared samples onto their assigned conditioned Oasis MCX cartridge using an automatic pipette and let it pass through the cartridge via gravity.
23.Wash step 1: Add 1200 μl formic acid (2% v/v in water) using a repeater pipette and let it pass through the cartridge via gravity.
24.Wash step 2: Add 1200 μl formic acid (0.2% v/v in methanol) using a repeater pipette and let it pass through the cartridge via gravity.
25.Transfer Oasis MCX cartridges to the assigned 13 × 100–mm disposable culture tubes.
26.Using a repeater pipette, elute samples with 1200 μl ammonium hydroxide (2% v/v in methanol) and let it pass through the cartridge via gravity.
27.Evaporate to dryness under a nitrogen flow at room temperature.
28.Resuspend with 100 μl mobile phase A with a pipette.
29.Vortex each tube for 15 s.
30.Sonicate for 30 s.
31.Transfer suspension to a 250-μl glass insert deposited in the assigned 2-ml glass vial with a pipette, then cap the vials.
32.Centrifuge the vials for 20 s at room temperature and 1300 × g.
33.Prepare the UPLC-MS/MS for sample analysis.
System preparation
34.Install the Halo HILIC chromatographic column on the UPLC system.
35.Install the following solutions on the UPLC system:
- - Mobile phase A
- - Mobile phase B
- - Weak needle wash
- - Strong wash
36.Set up the chromatographic separation parameters on the UPLC system, as shown in Table 1.
Parameter | Description |
---|---|
Autosampler Temperature | 10°C |
Column Temperature | 60°C |
Weak Wash Solvent | ACN |
Strong Wash Solvent | ACN |
Injection Mode | Partial Loop Injection |
Injection Volume | 10 μl |
Mobile Phase A | 94.5:2.5:2.5:0.5 ACN: MeOH:H2O:FA + 5 mM Amm. Form. |
Mobile Phase B | 10:89.5:0.5 ACN:H2O:FA + 5 mM Amm. Form. |
Flow rate | 0.85 ml/min |
Gradient (% Mobile Phase B) | 0.0-1.0 min: 15% |
1.0-6.0 min: 15% | |
6.0-7.0 min: 15%-60% (Linear Gradient) | |
7.0-10.0 min: 60% | |
10.0-12.0 min: 15% |
37.Set up the tandem mass spectrometry method parameters on the tandem mass spectrometry system, as shown in Table 2.
Mass Spectrometry Parameters | ||||
Capillary voltage | 3.2 kV | |||
Desolvation temperature | 550°C | |||
Desolvation gas flow | 750 L/hr | |||
Cone gas flow | 150 L/hr | |||
Source temperature | 150°C | |||
Analytes | ||||
Compound | Transitions (m/z) | Cone voltage (V) | Collision energy (V) | Dwell time (s) |
Lyso-Gb1 -28 Da | 434.31 > 254.25 | 38 | 18 | 0.028 |
Lyso-Gb1 -12 Da | 450.31 > 252.23 | 38 | 18 | 0.028 |
Lyso-Gb1 -2 Da | 460.32 > 280.26 | 38 | 18 | 0.028 |
Lyso-Gb1 | 462.34 > 282.29 | 38 | 18 | 0.028 |
Lyso-Gb1-(13C6) | 468.32 > 282.28 | 38 | 18 | 0.028 |
Lyso-Gb1 +14 Da | 476.36 > 296.29 | 38 | 18 | 0.028 |
Lyso-Gb1 +16 Da | 478.34 > 280.31 | 38 | 18 | 0.028 |
Lyso-Gb1 +18 Da | 480.36 > 318.31 | 38 | 18 | 0.028 |
SPC | 465.35 > 184.05 | 18 | 21 | 0.028 |
SPC-(D9) | 474.40 > 193.13 | 18 | 21 | 0.028 |
NPOPCS | 509.34 > 104.11 | 18 | 21 | 0.028 |
38.Set up the multiple reaction monitoring transitions in positive electrospray ionization mode, as shown in Table 2.
39.Inject 10 µl of the samples in the prepared UPLC-MS/MS system.
Data processing
40.Integrate the chromatographic peaks for each analyte and internal standard using TargetLynx software (Waters).
41.Calculate the ratio of the area of each analyte to the area of its corresponding internal standard. Lyso-Gb1-(13C6) is used as the internal standard for lyso-Gb1 and its analogs, while SPC-(D9) is used as the internal standard for SPC and NPOPCS. A chromatogram is shown in Figure 1.
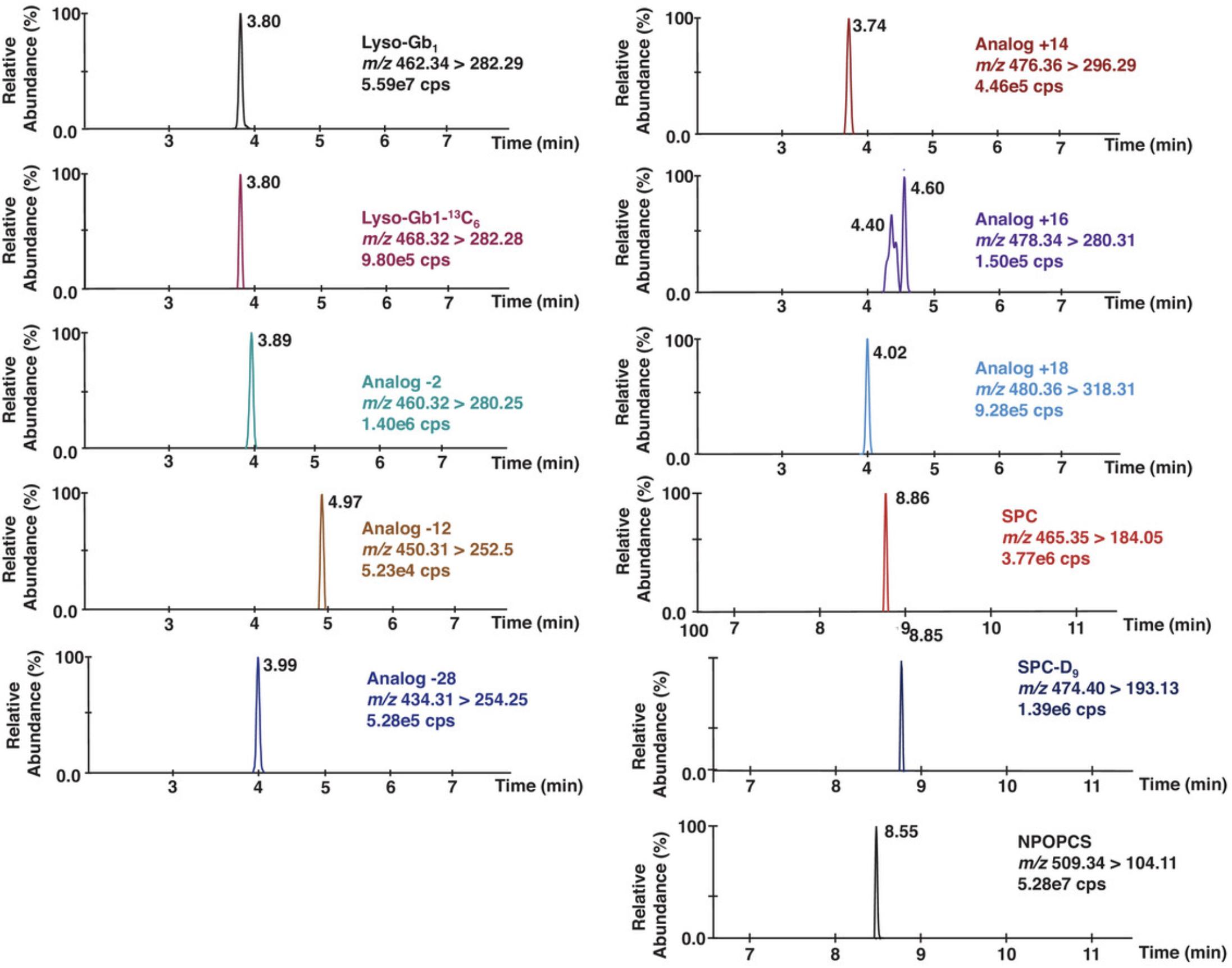
42.Calculate the analyte concentrations using the calibration curves (area ratios obtained in the calibrators plotted against the nominal concentration). A lyso-Gb1 calibration curve is used to quantify lyso-Gb1 and its analogs, while an SPC calibration curve is used to quantify SPC and NPOPCS.
43.For both lyso-Gb1 and SPC calibration curves, ensure that a minimum of 8 standards out of 10 have an accuracy (%bias) within ±15%, except for P1 (limit of quantitation), which should have an accuracy (%bias) within ±20%.
44.Ensure that the calibration curves have a coefficient of determination (r2) >0.995.
REAGENTS AND SOLUTIONS
Use Optima LC/MS grade water (Fisher, cat. no. W6-4) for all solutions unless otherwise indicated.
Calibration curve and quality control working solutions
Using Table 3 below, add specific volumes of each solution in a 4-ml amber glass vial with PTFE black phenolic cap to obtain the calibration curve and QC working solutions. All preparations should be made fresh, at room temperature, and used the same day.
Lyso-Gb1 working solutions | SPC working solutions | Lyso-Gb1 | SPC | |||||||
---|---|---|---|---|---|---|---|---|---|---|
Calibration Point | 200 nM (μl) | 4000 nM (μl) | 20,000 nM (μl) | 200 nM (μl) | 20,000 nM (μl) | DMSO (μl) | MeOH (μl) | Total Vol. (μl) | Conc. (nM) | Conc. (nM) |
P0 | - | - | - | - | - | 50 | 950 | 1000 | 0 | 0 |
P1 | 5 | - | - | 10 | - | 49 | 936 | 1000 | 1 | 2 |
P2 | 50 | - | - | 100 | - | 40 | 810 | 1000 | 10 | 20 |
P3 | 250 | - | - | 500 | - | 0 | 250 | 1000 | 50 | 100 |
P4 | - | 50 | - | - | 20 | 48 | 882 | 1000 | 200 | 400 |
P5 | - | 200 | - | - | 50 | 45 | 705 | 1000 | 800 | 1000 |
P6 | - | 600 | - | - | 80 | 42 | 278 | 1000 | 2400 | 1600 |
P7 | - | - | 200 | - | 125 | 38 | 637 | 1000 | 4000 | 2500 |
P8 | - | - | 300 | - | 160 | 34 | 506 | 1000 | 6000 | 3200 |
P9 | - | - | 400 | - | 320 | 18 | 262 | 1000 | 8000 | 6400 |
P10a | - | - | 500 | - | 600 | 50 | 950a | 1000 | 10,000 | 12,000 |
LQC | 150 | - | - | 300 | - | 20 | 530 | 1000 | 30 | 60 |
MQC | - | 625 | - | - | 130 | 37 | 208 | 1000 | 2500 | 2600 |
HQC | - | - | 250 | - | 375 | 12 | 363 | 1000 | 5000 | 7500 |
- a
Add 500 μl of lyso-Gb1 20,000 nM working solution and 600 μl of 20,000 nM working solution. Evaporate to dryness under a nitrogen stream and resuspend in 1 ml of a 95:5 MeOH:DMSO solution. Vortex for 10 s and sonicate for 30 s.
Formic acid, 2% v/v in water
-
1.In a 500-ml clear glass bottle, add 490 ml Optima LC/MS-grade water.
-
2.Add 10 ml formic acid (FA; >99%; Acros Organics, cat. no. EW-88147-15).
-
Keep at room temperature for up to 6 months.
Formic acid, 0.2% v/v in methanol
-
1.In a 250-ml clear glass bottle, add 100 ml Optima LC/MS-grade methanol and 99.6 ml Optima LC/MS-grade water.
-
2.Add 0.4 ml formic acid (FA; >99%; Acros Organics, cat. no. EW-88147-15).
-
Keep at room temperature for up to 6 months.
H3PO4 2% v/v solution in water
-
1.In a 500-ml clear glass bottle, add 336 ml Optima LC/MS-grade water (Fisher Scientific, cat. no. W6-4).
-
2.Add 8 ml ACS-certified O-phosphoric acid (H3PO4; 85%; Fisher Scientific, cat. no. A242-500).
-
Keep at room temperature for up to 18 months.
Internal standard working solutions (SPC-(D9): 10.6 nM and lyso-Gb1-(13C6): 10.7 nM)
-
1.In a 250-ml amber glass bottle, mix 40 μl SPC-(D9) stock solution (528 μM solution) with 40 μl lyso-Gb1-(13C6) internal standard (535 μM solution).
-
2.Complete volume with Optima LC/MS-grade methanol to 200 ml.
-
3.Proceed with a 1:10 dilution to obtain the internal standard working solution (20 ml internal standard solution added to 180 ml Optima LC/MS-grade methanol).
-
Keep at 4°C for up to 6 months.
Lyso-Gb1 stock solution (0.2 mM)
-
1.In a 20-ml clear glass vial with polytetrafluoroethylene (PTFE) black phenolic cap, weigh 0.923 mg glucosylsphingosine (lyso-Gb1; Matreya, cat. no. 1306) and dissolve in 10 ml Optima LC/MS-grade methanol.
-
2.Sonicate for 30 s and verify that dissolution is complete.
-
Keep at −20°C until use. Stable while frozen. Once thawed, use immediately and do not refreeze.
Lyso-Gb1-(13C6) internal standard stock solution (535 μM)
-
1.Dissolve 1 mg of 13C6-glucosylsphingosine (lyso-Gb1-(13C6); Matreya, cat. no. 2209) in its original container with small amounts of Optima LC/MS-grade methanol and transfer to a 4-ml amber glass vial with PTFE black phenolic cap (Duran Wheaton Kimble, cat. no W224682).
-
2.Bring volume to 4000 μl with Optima LC/MS-grade methanol.
-
3.Sonicate for 30 s, vortex for 10 s, and verify that dissolution is complete.
-
Keep frozen at −20 or −80°C until use. Stable while frozen. Once thawed, use immediately and do not refreeze.
Lyso-Gb1 working solution 1 (20,000 nM)
- In a 20-ml clear vial with PTFE black phenolic cap, dilute 1 ml lyso-Gb1 stock solution (0.2 mM) with 9 ml Optima LC/MS-grade methanol.
- Keep frozen at −20°C until use. Stable while frozen. Once thawed, use immediately and do not refreeze.
Lyso-Gb1 working solution 2 (4000 nM)
- In a 20-ml clear vial with PTFE black phenolic cap, dilute 2 ml lyso-Gb1 working solution 1 (20,000 nM) with 8 ml Optima LC/MS-grade methanol.
- Keep at −20°C until use. Stable while frozen. Once thawed, use immediately and do not refreeze.
Lyso-Gb1 working solution 3 (200 nM)
- In a 20-ml clear vial with PTFE black phenolic cap, dilute 0.1 ml lyso-Gb1 working solution 1 (20,000 nM) with 9.9 ml Optima LC/MS-grade methanol.
- Keep frozen at −20°C until use. Stable while frozen. Once thawed, use immediately and do not refreeze.
Methanol:Dimethyl sulfoxide (DMSO) 95:5 v/v solution
-
1.In a 20-ml clear glass vial with PTFE black phenolic cap (Duran Wheaton Kimble, cat. no W224589), add 19 ml Optima LC/MS grade methanol (Fisher Scientific, cat. no. A456-4).
-
2.Add 1 ml dimethyl sulfoxide (DMSO; >99%; Sigma Aldrich, cat. no. M81802).
-
Keep at 4°C for up to 1 week.
NH4OH 2% v/v in methanol
-
1.In a 250-ml clear glass bottle, add 56 ml Optima LC/MS grade methanol.
-
2.Add 4 ml ACS-grade ammonium hydroxide (NH4OH; 28%-30%; Fisher Scientific, cat. no. AC423300250).
-
Prepare fresh on the day of extraction.
SPC stock solution (0.2 mM)
-
1.In a 20-ml clear vial with PTFE black phenolic cap, weigh 0.929 mg sphingosylphosphorylcholine (SPC; Matreya, cat. no. 1318) standard and dissolve in 10 ml of 90:10 MeOH:DMSO.
-
2.Sonicate for 30 s and vortex for 10 s and verify that dissolution is complete.
-
Keep frozen at −20°C until use. Stable while frozen. Once thawed, use immediately and do not refreeze.
SPC working solution 1 (20,000 nM)
-
1.In a 20-ml clear vial with PTFE black phenolic cap, dilute 1 ml SPC stock solution (0.2 mM) with 9 ml of 90:10 MeOH:DMSO.
-
2.Vortex (10 s) and sonicate (30 s) the SPC stock solution before use.
-
Keep frozen at −20°C until use. Stable while frozen. Once thawed, use immediately and do not refreeze.
SPC working solution 2 (200 nM)
-
1.In a 20-ml clear vial with PTFE Black Phenolic Cap, dilute 0.1 ml SPC working solution 1 (20,000 nM) in 9.9 ml of 90:10 MeOH:DMSO.
-
2.It is extremely important to vortex (10 s) and sonicate (30 s) the SPC working solution before use.
-
Keep frozen at −20°C until use. Stable while frozen. Once thawed, use immediately and do not refreeze.
SPC-(D9) internal standard stock solution (528 μM)
-
1.Dissolve 1 mg deuterated sphingosylphosphorylcholine (SPC-(D9); Avanti Polar Lipids Alabaster, cat. no. 860685) in its original container with small amounts of Optima LC/MS-grade methanol and transfer to a 4-ml amber glass vial with PTFE black phenolic cap.
-
2.Add Optima LC/MS methanol to 4000 μl.
-
3.Vortex for 10 s and sonicate for 30 s.
-
4.Verify that dissolution is complete.
-
Keep frozen at −80°C until use. Stable while frozen. Once thawed, use immediately and do not refreeze.
Strong Wash–ACN 100%
- In a 1000-ml clear glass bottle, add 1000 ml OmniSolv LC-MS-grade acetonitrile (ACN; EMD Chemicals, cat. no. AX0156-1).
- Store at room temperature in a well-ventilated place for up to 2 months.
Surrogate matrix
- Dissolve 75 mg fatty-acid-free bovine serum albumin (BSA; ≥98%; Sigma Aldrich, cat. no. A7030) in 10 ml PBS (1×, pH 7.4) (Wisent Bioproduct, cat. no. 311-010-CL).
- Keep at 4°C for up to 2 weeks.
Weak Needle Wash–ACN 100%
- In a 1000-ml clear glass bottle, add 1000 ml OmniSolv LC/MS-grade acetonitrile (ACN; EMD Chemicals, cat. no. AX0156-1).
- Store at room temperature in a well-ventilated place for up to 2 months.
Background Information
Most biomarkers measured in GD are nonspecific to the disease, thus limiting their utility (Nagral, 2014). Given the large clinical spectrum and important phenotypic variability associated with GD, other plasma biomarkers should be identified. Lyso-Gb1 has recently emerged as a biomarker of choice for GD monitoring and follow-up (Cozma et al., 2020; Dinur et al., 2021). Some experts argue that this biomarker could be used for diagnosis (Dinur et al., 2022). This is a major departure from the previous dogma, which held that GD biomarkers are only useful for patient monitoring and follow-up. We performed a semi-targeted metabolomic study of plasma specimens obtained from patients with untreated GD and healthy controls (Menkovic et al., 2022a) based on our previous experience with Fabry disease (Auray-Blais et al., 2012; Dupont, Gagnon, Boutin, and Auray-Blais, 2013). Based on the results of this study and the research done by Mirzaian and colleagues (Mirzaian et al., 2015), we developed and validated a UPLC-MS/MS method to quantify lyso-Gb1 and lyso-Gb1 analogs at –28 Da, –12 Da, –2 Da, +14 Da, +16 Da, and +18 Da in addition to N -palmitoyl-O-phosphocholineserine (NPOPCS) and sphingosylphosphorylcholine (SPC) to study the distribution of these markers in treated and untreated patients (Menkovic et al., 2022b). This paper is a detailed protocol for the quantification method.
Critical Parameters
One of the main challenges is the incorporation of SPC due to its limited solubility in the solvents compatible with this method. To address this issue, we added several vortexing (10 s) and sonication (30 s) cycles prior to any step that requires using an SPC solution.
Also, a relative quantification approach was used because standards and internal standards are unavailable for most of the compounds. Analytes for which standards and internal standards are not available are quantified based on the response factor of either lyso-Gb1 (for lyso-Gb1 analogs) or SPC (for NPOPCS). It is important to monitor and document any important changes in signals or retention times because such changes could suggest that the chromatography is affected by interferents and may lead to erroneous results due to matrix effects. Nevertheless, the method described herein has been validated to provide high precision, accuracy, and stability for lyso-Gb1 and SPC.
Understanding the Results
A proxy matrix was used to simulate plasma for the calibration curve point samples because lyso-Gb1 and SPC are found in healthy plasma. It is important to verify whether the chromatography efficiently separates lyso-Gb1 from its structural isomer galactosylsphingosine (psychosine), a biomarker for Krabbe disease. The resolution of these two compounds is important and should be optimized, given that the chromatography might vary due to inter-laboratory differences. In fact, psychosine was found in the surrogate matrix used to prepare the calibration curve points and in healthy reference controls. Split peaks are visible in the chromatogram for the lyso-Gb1 analog at +16 Da. Both peaks are isomers of the lyso-Gb1 analog +16 Da.
Lyso-Gb1 and its analogs are expected to be elevated in patients with Gaucher disease type 1. Lyso-Gb1 is barely detectable (1.22 to 1.44 nM) in healthy controls, and lyso-Gb1 analogs are not detectable. Nevertheless, this method should be used for research purposes, and positive results should be confirmed by established diagnostic assays such as a marked β-glucosidase deficiency in peripheral blood leukocytes and GBA gene sequencing. The levels of lyso-Gb1 and its analogs were higher in patients with severe Gaucher disease type 1 versus those with the attenuated form. Moreover, patients undergoing treatment had a lower concentration of these molecules than naive patients. SPC and NPOPCS were observed in healthy controls and patients with GD type 1, and levels did not correlate with disease severity or treatment status. SPC is also increased in Niemann-Pick types A and B, while NPOPCS is increased in Niemann-Pick type C.
Time Considerations
A technician will need approximately 5 hr to prepare 48 samples, including calibrators and QCs. The UPLC-MS/MS analysis requires 12 min per sample. Data processing generally requires 60 to 90 min for a batch of 48 samples.
Ethics Statement
Patient samples were obtained with signed informed consent under protocols approved by the Research Ethics Board (REB) of the Faculty of Medicine and Health Sciences of the Centre Hospitalier Universitaire de Sherbrooke (CHUS) under project ID MP-31-2017-1414 in accordance with the ethical standards laid down in the World Medical Association Declaration of Helsinki.
Acknowledgments
The authors would like to acknowledge Shire/Takeda Pharmaceutical for funding this research project under a collaborative research agreement. We are grateful to the Faculty of Medicine and Health Sciences at the Université de Sherbrooke for a doctoral student grant for Iskren Menkovic. We would also like to thank Waters Corporation for their technical expertise and continuous support.
Author Contributions
Iskren Menkovic : Formal analysis, methodology, validation, visualization, writing original draft. Michel Boutin : Supervision, writing review and editing. Pamela Lavoie : Visualization, writing review and editing. Christiane Auray-Blais : Conceptualization, data curation, funding acquisition, project administration, resources, supervision, visualization, writing review and editing.
Conflict of Interest
I.M. received a student grant from Shire/Takeda Pharmaceutical. M.B. received a salary from Shire/Takeda Pharmaceutical. C.A.-B. received research grants and honoraria from Shire/Takeda Pharmaceutical.
Open Research
Data Availability Statement
Data sharing is not applicable to this article as no datasets were generated or analyzed during the current study.
Literature Cited
- Andrade-Campos, M., Alfonso, P., Irun, P., Armstrong, J., Calvo, C., Dalmau, J., … Giraldo, P. (2017). Diagnosis features of pediatric Gaucher disease patients in the era of enzymatic therapy, a national-base study from the Spanish Registry of Gaucher Disease. Orphanet Journal of Rare Diseases , 12(1), 84. doi: 10.1186/s13023-017-0627-z
- Arévalo, N. B., Lamaizon, C. M., Cavieres, V. A., Burgos, P. V., Álvarez, A. R., Yañez, M. J., & Zanlungo, S. (2022). Neuronopathic Gaucher disease: Beyond lysosomal dysfunction. Frontiers in Molecular Neuroscience , 15, 934820. doi: 10.3389/fnmol.2022.934820
- Auray-Blais, C., Boutin, M., Gagnon, R., Dupont, F. O., Lavoie, P., & Clarke, J. T. (2012). Urinary globotriaosylsphingosine-related biomarkers for Fabry disease targeted by metabolomics. Analytical Chemistry , 84(6), 2745–2753. doi: 10.1021/ac203433e
- Biegstraaten, M., Cox, T. M., Belmatoug, N., Berger, M. G., Collin-Histed, T., Vom Dahl, S., … Hollak, C. (2018). Management goals for type 1 Gaucher disease: An expert consensus document from the European working group on Gaucher disease. Blood Cells, Molecules & Diseases, 68, 203–208. doi: 10.1016/j.bcmd.2016.10.008
- Charrow, J., & Scott, C. R. (2015). Long-term treatment outcomes in Gaucher disease. American Journal of Hematology , 90(Suppl 1), S19–S24. doi: 10.1002/ajh.24056
- Chis, B. A., Chis, A. F., & Dumitrascu, D. L. (2021). Gaucher disease—bone involvement. Medicine and Pharmacy Reports , 94(Suppl No 1), S61–S63. doi: 10.15386/mpr-2233
- Cozma, C., Cullufi, P., Kramp, G., Hovakimyan, M., Velmishi, V., Gjikopulli, A., … Rolfs, A. (2020). Treatment efficiency in Gaucher patients can reliably be monitored by quantification of Lyso-Gb1 concentrations in dried blood spots. International Journal of Molecular Sciences , 21(13), 4577. doi: 10.3390/ijms21134577
- D'Amore, S., Page, K., Donald, A., Taiyari, K., Tom, B., Deegan, P., … MRC GAUCHERITE Consortium. (2021). In-depth phenotyping for clinical stratification of Gaucher disease. Orphanet Journal of Rare Diseases , 16(1), 431. doi: 10.1186/s13023-021-02034-6
- Dinur, T., Bauer, P., Beetz, C., Kramp, G., Cozma, C., Iurașcu, M. I., … Revel-Vilk, S. (2022). Gaucher disease diagnosis using Lyso-Gb1 on dry blood spot samples: Time to change the paradigm? International Journal of Molecular Sciences , 23(3), 1627. doi: 10.3390/ijms23031627
- Dinur, T., Grittner, U., Revel-Vilk, S., Becker-Cohen, M., Istaiti, M., Cozma, C., … Zimran, A. (2021). Impact of long-term enzyme replacement therapy on glucosylsphingosine (Lyso-Gb1) values in patients with type 1 Gaucher disease: Statistical models for comparing three enzymatic formulations. International Journal of Molecular Sciences , 22(14), 7699. doi: 10.3390/ijms22147699
- Donald, A., Tan, C. Y., Chakrapani, A., Hughes, D. A., Sharma, R., Cole, D., … Schneider, E. (2020). Eye movement biomarkers allow for the definition of phenotypes in Gaucher Disease. Orphanet Journal of Rare Diseases , 15(1), 349. doi: 10.1186/s13023-020-01637-9
- Dupont, F. O., Gagnon, R., Boutin, M., & Auray-Blais, C. (2013). A metabolomic study reveals novel plasma lyso-Gb3 analogs as Fabry disease biomarkers. Current Medicinal Chemistry , 20(2), 280–288. doi: 10.2174/092986713804806685
- Essabar, L., Meskini, T., Lamalmi, N., Ettair, S., Erreimi, N., & Mouane, N. (2015). Gaucher's disease: Report of 11 cases with review of the literature. The Pan African Medical Journal , 20, 18. doi: 10.11604/pamj.2015.20.18.4112
- Gary, S. E., Ryan, E., Steward, A. M., & Sidransky, E. (2018). Recent advances in the diagnosis and management of Gaucher disease. Expert Review of Endocrinology & Metabolism, 13(2), 107–118. doi: 10.1080/17446651.2018.1445524
- Giraldo, P., López de Frutos, L., & Cebolla, J. J. (2018). Biomarker combination is necessary for the assessment of Gaucher disease? Annals of Translational Medicine , 6(Suppl 1), S81. doi: 10.21037/atm.2018.10.69
- Giraldo, P., & Andrade-Campos, M. (2021). Novel management and screening approaches for haematological complications of Gaucher's disease. Journal of Blood Medicine , 12, 1045–1056. doi: 10.2147/JBM.S279756
- Grabowski, G. A., Antommaria, A., Kolodny, E. H., & Mistry, P. K. (2021). Gaucher disease: Basic and translational science needs for more complete therapy and management. Molecular Genetics and Metabolism , 132(2), 59–75. doi: 10.1016/j.ymgme.2020.12.291
- Marshall, J., Sun, Y., Bangari, D. S., Budman, E., Park, H., Nietupski, J. B., … Cheng, S. H. (2016). CNS-accessible inhibitor of glucosylceramide synthase for substrate reduction therapy of neuronopathic Gaucher disease. Molecular therapy: The Journal of The American Society of Gene Therapy , 24(6), 1019–1029. doi: 10.1038/mt.2016.53
- Menkovic, I., Boutin, M., Alayoubi, A., Curado, F., Bauer, P., Mercier, F. E., … Auray-Blais, C. (2022a). Quantitation of a plasma biomarker profile for the early detection of Gaucher disease type 1 patients. Bioanalysis , 14(4), 223–240. doi: 10.4155/bio-2021-0242
- Menkovic, I., Boutin, M., Alayoubi, A., Curado, F., Bauer, P., Mercier, F. E., & Auray-Blais, C. (2022b). Quantitation of a urinary profile of biomarkers in Gaucher disease type 1 patients using tandem mass spectrometry. Diagnostics , 12(6), 1414. doi: 10.3390/diagnostics12061414
- Menkovic, I., Boutin, M., Alayoubi, A., Mercier, F. E., Rivard, G. É., & Auray-Blais, C. (2020). Identification of a reliable biomarker profile for the diagnosis of Gaucher disease type 1 patients using a mass spectrometry-based metabolomic approach. International Journal of Molecular Sciences , 21(21), 7869. doi: 10.3390/ijms21217869
- Mirzaian, M., Wisse, P., Ferraz, M. J., Gold, H., Donker-Koopman, W. E., Verhoek, M., … Aerts, J. M. (2015). Mass spectrometric quantification of glucosylsphingosine in plasma and urine of type 1 Gaucher patients using an isotope standard. Blood Cells, Molecules & Diseases, 54(4), 307–314. doi: 10.1016/j.bcmd.2015.01.006
- Mistry, P., Balwani, M., Barbouth, D., Burrow, T. A., Ginns, E. I., Goker-Alpan, O., … Sidransky, E. (2020). Gaucher disease and SARS-CoV-2 infection: Emerging management challenges. Molecular Genetics and Metabolism , 130(3), 164–169. doi: 10.1016/j.ymgme.2020.05.002
- Mistry, P. K., Deegan, P., Vellodi, A., Cole, J. A., Yeh, M., & Weinreb, N. J. (2009). Timing of initiation of enzyme replacement therapy after diagnosis of type 1 Gaucher disease: Effect on the incidence of avascular necrosis. British Journal of Haematology , 147(4), 561–570. doi: 10.1111/j.1365-2141.2009.07872.x
- Miyamoto, T., Iino, M., Komorizono, Y., Kiguchi, T., Furukawa, N., Otsuka, M., … Nakamura, K. (2021). Screening for Gaucher disease using dried blood spot tests: A Japanese multicenter, cross-sectional survey. Internal Medicine , 60(5), 699–707. doi: 10.2169/internalmedicine.5064-20
- Nagral, A. (2014). Gaucher disease. Journal of Clinical and Experimental Hepatology , 4(1), 37–50. doi: 10.1016/j.jceh.2014.02.005
- Özdemir, G. N., & Gündüz, E. (2022). Gaucher disease for hematologists. Turkish Journal of Haematology , 39(2), 136–139. doi: 10.4274/tjh.galenos.2022.2021.0683
- Revel-Vilk, S., Fuller, M., & Zimran, A. (2020). Value of glucosylsphingosine (Lyso-Gb1) as a biomarker in Gaucher disease: A systematic literature review. International Journal of Molecular Sciences , 21(19), 7159. doi: 10.3390/ijms21197159
- Sam, R., Ryan, E., Daykin, E., & Sidransky, E. (2021). Current and emerging pharmacotherapy for Gaucher disease in pediatric populations. Expert Opinion on Pharmacotherapy , 22(11), 1489–1503. doi: 10.1080/14656566.2021.1902989
- Schiffmann, R., Sevigny, J., Rolfs, A., Davies, E. H., Goker-Alpan, O., Abdelwahab, M., … Zimran, A. (2020). The definition of neuronopathic Gaucher disease. Journal of Inherited Metabolic Disease , 43(5), 1056–1059. doi: 10.1002/jimd.12235
- Silva García, R., de Frutos, L. L., Arreguin, E. Á., González, C. C., Ortiz, J., Ornelas, S. F., … Favela, F. B. (2021). Gaucher Disease: Identification and novel variants in Mexican and Spanish patients. Archives of Medical Research , 52(7), 731–737. doi: 10.1016/j.arcmed.2021.05.001
- Stirnemann, J., Belmatoug, N., Camou, F., Serratrice, C., Froissart, R., Caillaud, C., … Berger, M. G. (2017). A review of Gaucher disease pathophysiology, clinical presentation and treatments. International Journal of Molecular Sciences , 18(2), 441. doi: 10.3390/ijms18020441
- Weinreb, N. J., Camelo, J. S. Jr., Charrow, J., McClain, M. R., Mistry, P., Belmatoug, N., & International Collaborative Gaucher Group (ICGG) Gaucher Registry (NCT00358943) investigators. (2021). Gaucher disease type 1 patients from the ICGG Gaucher Registry sustain initial clinical improvements during twenty years of imiglucerase treatment. Molecular Genetics and Metabolism , 132(2), 100–111. doi: 10.1016/j.ymgme.2020.12.295