Microwave-Assisted One-Step Synthesis of 2′,3′-Cyclic Phosphates of Nucleosides
Kollery S. Veena, Kollery S. Veena, Harold A. Cruz, Harold A. Cruz, Ramanarayanan Krishnamurthy, Ramanarayanan Krishnamurthy
Abstract
2′,3′-Cyclic canonical nucleotides are an important class of compounds playing broad roles in regulating biological processes and are investigated in the context of prebiotic chemistry as activated nucleotides for oligonucleotide formation. Despite their growing importance, synthetic access of 2′,3′-cyclic nucleotides is constrained, resulting in their cost-prohibitive commercial prices. Here, we describe a microwave-assisted one-pot synthesis starting from commercially available nucleosides employing an easily available cyclophosphorylating reagent, bis(dimethyldiamino)phosphorodiamidate (BDMDAP). The corresponding 2′,3′-cyclic nucleotides are isolated in good yields (70-91%) by a simple ion-exchange column with no further workup. The nucleosides require no protecting group as the cyclophosphorylation reaction is selective for the 2′,3′-dihydroxyl groups. The experimental protocol is robust and can be run in parallel to provide access to gram quantities of these 2′,3′-cyclic nucleotides within a day. © 2023 The Authors. Current Protocols published by Wiley Periodicals LLC.
Basic Protocol : Procedure for the phosphorylation of nucleoside (2′,3′-cyclic phosphates of nucleosides)
Support Protocol : Synthesis of bis(dimethylamino)phosphorodiamidate sodium salt
INTRODUCTION
The first chemical synthetic access to 2′,3′-cyclic canonical nucleotides was published in 1952 by Todd and colleagues (Brown et al., 1952) and further elaborated by the Khorana group (Tener & Khorana, 1955). Since that time there have been other synthetic approaches which rely mainly on intramolecular cyclization of a 2′- or 3′-phosphate derivative or treatment of the nucleoside with POCl3 resulting in multi-step synthesis and further workup before column purification and isolation (Eto et al., 1978; Mohamady & Taylor, 2016; Simoncsits & Tomasz, 1975; van Boom et al., 1973). Coupled with the drawback of some of the workup procedures, robust access to 2′,3′-cyclic nucleotides have been limited. Herein we report a mild method of a one-step and one-pot selective cyclophosphorylation using bis(dimethyldiamino)phosphorodiamidate (BDMDAP) (Support Protocol; Yadav & Krishnamurthy, 2019) as a reagent and employing microwave heating to accelerate the reaction (Basic Protocol; Fig. 1). Since the cyclophosphorylation reaction is selective for the cis-2′,3′-dihydroxyl groups, it obviates the need to protect the 5′-OH and the functional-groups on the nucleobases. The simplicity of the reaction setup, requiring no workup, potentiates the gram scale synthesis of these precious (canonical) nucleotides that are otherwise quite expensive to procure as sodium salt (∼100 mg for US
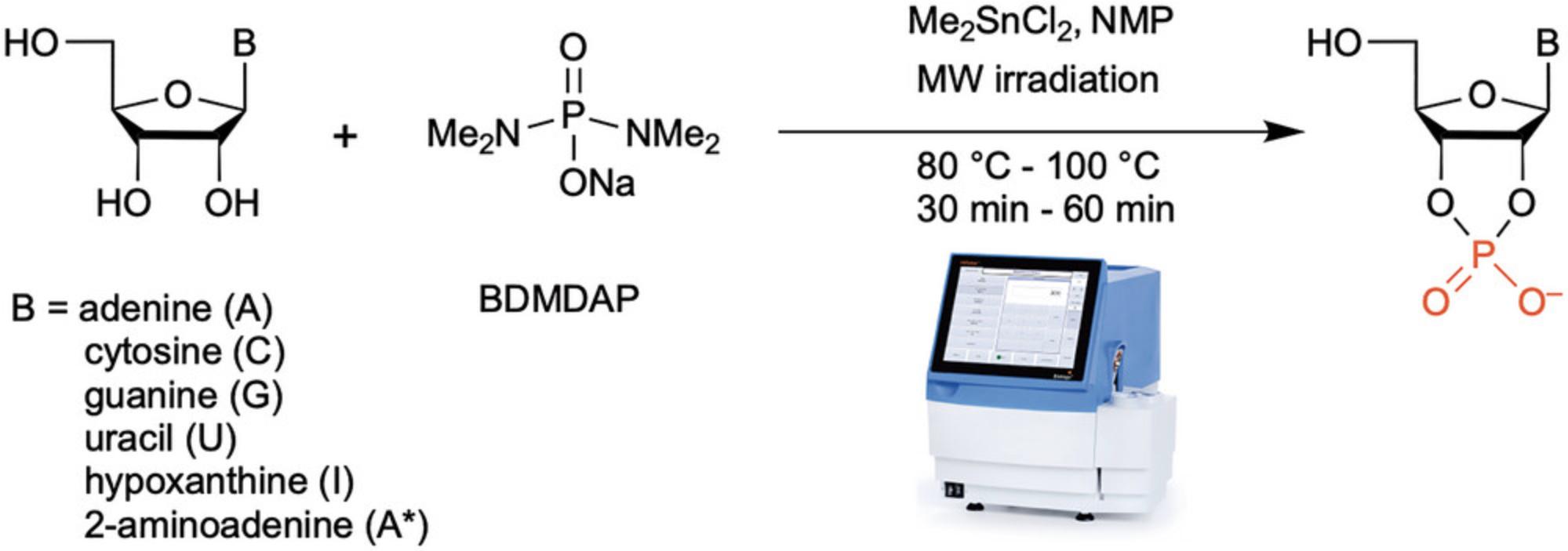
NOTE : Reagents, apparatus and solvents are available from suppliers noted next to each item. Before planning for the reaction check the SDS for the following chemicals: dimethyltin dichloride (Me2SnCl2; CAS number 753-73-1, from Spectrum), and 1-methyl-2-pyrrolidone (NMP; CAS number 872-50-4, from Thermo Scientific).
Basic Protocol: PROCEDURE FOR THE PHOSPHORYLATION OF NUCLEOSIDE (2′,3′-CYCLIC PHOSPHATES OF NUCLEOSIDES)
This protocol uses the synthesized reagent BDMDAP (see Support Protocol) and a desired starting nucleoside to synthesize 2′,3′-cyclic phosphates of nucleosides.
Materials
-
Adenosine (CAS number 58-61-7, from Sigma)
-
2-aminoadenosine (CAS number 3868-33-5, from Berry & Associates)
-
Uridine (CAS number 58-96-8, from Sigma)
-
Guanosine (CAS number 118-00-3, from Fluka Biochemica)
-
Inosine (CAS number 58-63-9, from HD Ark Pharm)
-
Cytidine (CAS number 65-46-3, from Aldrich)
-
Bis(dimethylamino)phosphorodiamidate (BDMDAP) (see Support Protocol)
-
Dimethyltin dichloride (Me2SnCl2) (CAS number 753-73-1, from Spectrum)
-
1-methyl-2-pyrrolidone (NMP) (CAS number 872-50-4, from Thermo Scientific)
-
Deuterium oxide (D2O; CAS number 7789-20-0, from Sigma)
-
Sodium bicarbonate (CAS number 144-55-8, from Fisher Chemicals)
-
Ultra pure H2O
-
Triethylammonium bicarbonate (Et3N) buffer (CAS number 15715-58-9, from Merck)
-
0.5-2 ml microwave reaction tubes (Biotage cat. no. 352016), oven-dried with a magnetic stir bar
-
Balloons (Fisher Science Education BL-5200-144PK)
-
N2 gas
-
1- and 2-ml plastic syringes (Henke-Ject cat. nos. 19G334 and 19G339)
-
22-G × 0.75-inch needles (0.7-mm × 19-mm; BD cat. no. 305142)
-
20-G × 1.5-inch needles (0.9-mm × 40-mm; BD cat. no. 305176)
-
Micropipettes
-
1.5-ml Eppendorf tubes
-
Vortex
-
NMR tubes
-
Metal tube caps with 20-mm PTFE/silicon (blue/white) septa (Biotage cat. no. 352016)
-
Microwave tube crimper and decapper (VWR cat. no. 89041-330)
-
Microwave synthesizer (Initiator Classic, Biotage cat. no. 356007)
-
Ion-exchange resin (Sephadex A-25, chloride form; CAS number 12609-80-2, from Sigma)
-
250-ml Erlenmeyer flasks
-
Plastic chromatography columns
-
pH meter (Fisherbrand accumet AB150 pH)
-
16 × 150-mm test tubes
-
100- and 250-ml round-bottom flasks, oven-dried
-
Rotary evaporator (Buchi cat. no. Z563633) equipped with a vacuum pump (Welch cat. no. 202801)
-
High-vacuum oil pump (Welch cat. no. 3081-01)
-
24/40 vacuum adapter (ChemGlass cat. no. G5885485)
Protocol steps for preparing the reaction mixture in the microwave tube
1.Prepare a 0.5 to 2 ml microwave tube (oven-dried with a magnetic stir bar already inside).
2.Add the following amount of the desired starting nucleoside to a 0.5-2 ml microwave tube.
- 0.1362 g (0.51 mmol) adenosine (1)
- 0.1439 g (0.51 mmol) 2-aminoadenosine (2)
- 0.1245 g (0.51 mmol) uridine (3)
- 0.143 g (0.50 mmol) guanosine (4)
- 0.135 g (0.51 mmol) inosine (5)
- 0.125 g (0.51 mmol) cytidine (6)
3.Add the phosphorylation reagent BDMDAP (7) to the above microwave tube.
- For compounds 1 , 2 , 3 : 0.133 g (0.76 mmol)
- For compounds 4 and 5 : 0.131 g (0.75 mmol)
- For compound 6 : 0.178 g (1.02 mmol)
4.Add the following amount of Me2SnCl2 (8) to the above reaction mixture in the microwave tube.
- For compounds 1 , 2 , 3 : 0.011 g (0.05 mmol)
- For compounds 4 and 5 : 0.016 g (0.07572 mmol)
- For compound 6 : 0.022 g (0.1027 mmol)
5.Draw up the required amount (0.75–0.8 ml) of NMP using 1- or 2-ml syringe (dried; see Critical Parameters section for details) under nitrogen atmosphere using a balloon filled with N2 attached to a syringe equipped with a needle, add to the above reaction mixture in the microwave tube, and dissolve to ensure a homogeneous solution.
6.Withdraw a small amount (∼25 µl) of the above solution using a micropipette, transfer the solution into a 1.5-ml Eppendorf tube and dilute it with 500–600 µl D2O. Mix it well by vortexing and add the solution into an NMR tube and run a 1H NMR spectrum to check state of the solution at beginning of the reaction.
7.Seal the microwave tube with a septum-equipped metal cap and crimp it shut using the microwave tube crimper.
Program microwave synthesizer (see Efthymiou & Krishnamurthy, 2015)
8.Using the touch screen, select “Editor” from the main window to enter the following parameters:
-
Select “Time” and enter the desired reaction duration (60 min for adenosine and cytidine, 30 min for 2-aminoadenosine, uridine, guanosine, and inosine) using the number pad on the screen. Press “Enter” to confirm the reaction time.
-
Select “Temperature” and enter the desired temperature (80°C for adenosine, 100°C for cytidine, 2-aminoadenosine, uridine, guanosine, and inosine) and press “Enter”.
The minimum temperature that can be entered on the Biotage Initiator Classic is 60°C.
- Select “Pre-Stirring” and enter the desired pre-stirring time prior to the irradiation cycle and press “Enter”.
This feature is optional and can be skipped entirely.
-
Select “Vial Type” and choose from the series of volume ranges displayed with each press of the button. Select the option until the desired volume range appears (see step 5).
-
Select “Absorption Level” option until the “Normal” option is highlighted.
Perform microwave-assisted phosphorylation
9.Ensure that the reaction is set up for 60 min at 80°C for adenosine, 60 min at 100°C for cytidine and 30 min at 100°C for 2-aminoadenosine, uridine, guanosine, and inosine with a “Normal” absorbance level.
10.Select “Run” at the bottom of the screen.
11.Choose a “User Profile” from the scroll-down menu.
12.Select “Run” again to initiate the reaction.
Perform workup and analysis
13.Ensure the complete consumption of starting material by monitoring with 1H NMR spectroscopy. After the completion of the programmed time, open the microwave tube using a decapper and withdraw a small amount (∼25 µl) of the reaction mixture using a micropipette. Transfer the reaction mixture into a 1.5-ml Eppendorf tube and dilute it with D2O (500-600 µl). Mix it well by vortexing and add the solution into an NMR tube and run a 1H NMR spectrum to check for the signals of the starting nucleoside. Disappearance of the signals corresponding to the starting nucleoside indicates completion of reaction.
14.If the reaction is not complete, put a new cap on the microwave tube and repeat steps 11 and 12 for the desired time.
15.Once the reaction is complete, use the decapper to remove the metal cap and septum from the microwave tube.
16.Prepare a Sephadex A-25, bicarbonate ion-exchange column (2.5 cm × 5.0 cm for 9 , 10 , 11 and 1.5 cm × 5.0 cm for 12 , 13 , 14): Soak the Sephadex A-25 chloride resin in ∼1.2 M sodium bicarbonate (9.6% w/w) overnight in a 250-ml Erlenmeyer flask. Rinse with plenty of ultra pure H2O (until the pH of the water washings is almost neutral as checked by pH meter).
17.To the reaction mixture in the microwave tube, directly add an equal amount (volume, 800 µl) of H2O.
18.Introduce this diluted reaction mixture solution to the prepared ion-exchange column and allow the solution to become adsorbed on the column-bed (taking care not to let the column-top run dry).
19.Elute as follows and collect fractions in test tubes:
-
For compounds9,10, and11, start eluting the ion-exchange column initially with 50 ml H2O, followed by an increasing concentration of triethylammonium bicarbonate buffer (0.05–0.5 M buffer in 0.05 M increments of 20 ml each).
-
For compounds12,13, and14, start eluting the column initially with 20 ml H2O, followed by an increasing concentration of triethylammonium bicarbonate buffer (20 ml volumes each of 0.005 M, 0.01 M, 0.02 M, 0.03 M, 0.04 M, 0.05 M, 0.1 M, 0.15 M, and 0.2 M).
20.Monitor the fractions using 31P NMR. The cyclophosphate-product has a chemical shift ∼19-21 ppm. Pure fractions of the cyclic phosphate-product are eluted (in ∼ 0.2 M buffer) as the triethylammonium salt.
21.Combine all the fractions containing the cyclophosphate-product in a 100- or 250-ml round bottom flask and evaporate the solvent under vacuum using the rotary evaporator equipped with a vacuum pump to afford pure 2′,3′-cyclic phosphate of nucleoside as triethylammonium salt. To remove any remaining excess triethyl ammonium bicarbonate buffer, add water to the residue and repeat the evaporation under vacuum using the rotary evaporator equipped with a vacuum pump, followed by drying with the high-vacuum oil pump using the 24/40 vacuum adapter until a constant weight is obtained (see Critical Parameters section for details).
22.Characterize the products by 1H-NMR, 13C-NMR, 31P-NMR, and mass spectrometry (see Supporting Information for spectral data) by preparing a sample as described in step 13.
Spectral data (see Supporting Information)
Adenosine-2′,3′-cyclic monophosphate triethylammonium salt (9) (83%)
1.1H-NMR (600 MHz, D2O) δ (ppm): 8.24 (s, 1H), 8.13 (s, 1H), 6.19 (d, J = 4.3 Hz, 1H), 5.40–5.31 (m, 1H), 5.06 (td, J = 7.3, 4.0 Hz, 2H), 4.40 (q, J = 4.1 Hz, 1H), 3.88–3.83 (m, 1H), 3.82–3.76 (m, 1H), 3.11 (qt, J = 7.4, 1.0 Hz, CH2-triethylammonium), 1.19 (tt, J = 7.3, 1.0 Hz, CH3-triethylammonium). 13C NMR (151 MHz, D2O) δ (ppm): 155.5, 152.6, 148.3, 140.6, 118.8, 89.3 (d, J = 3.9 Hz), 85.2 (d, J = 3.9 Hz), 80.2, 77.5, 61.0, 46.4, 8.4.31P NMR (162 MHz, D2O) δ (ppm): 19.93.ESI-MS m/z calculated for C10H11N5O6P- [M-H]- 328.0452, found 328.0502.
2-Aminoadenosine-2′,3′-cyclic monophosphate triethylammonium salt (10) (85%)
- 1H-NMR (600 MHz, D2O) δ (ppm): 7.87 (s, 1H), 6.02 (d, J = 6.3 Hz, 1H), 5.36–5.27 (m, 1H), 5.11–5.00 (m, 1H), 4.35 (t , J = 4.1 Hz, 1H), 3.83 (ddd, J = 12.6, 3.3, 1.7 Hz, 1H), 3.77 (ddd, J = 12.7, 4.4, 1.7 Hz, 1H), 3.10 (qd, J = 7.3, 1.7 Hz, CH2-triethylammonium), 1.18 (td, J = 7.3, 1.8 Hz, CH3-triethylammonium). 13C NMR (151 MHz, D2O) δ (ppm): 159.71, 155.86, 150.53, 138.21, 138.17, 113.20, 89.03 (d, J = 24.2 Hz), 85.11 (dd, J = 9.6, 4.2 Hz), 80.13 (d, J = 14.6 Hz), 77.68 (d, J = 21.7 Hz), 61.29, 61.16, 61.02, 46.75, 46.63, 46.51, 8.21, 8.19, 8.17, 8.16.31P NMR (162 MHz, D2O) δ (ppm): 19.88.ESI-MS m/z calculated for C10H12N6O6P- [M-H]- 343.0561, found 343.0638.
Uridine-2′,3′-cyclic monophosphate triethylammonium salt (11) (91%)
- 1H-NMR (600 MHz, D2O) δ (ppm): 7.64 (d, J = 8.0 Hz, 1H), 5.82 (d , J = 2.9 Hz, 1H), 5.78 (d, J = 8.0 Hz, 1H), 5.09 (td , J = 6.8, 2.9 Hz, 1H), 4.86 (dt, J = 12.1, 6.2 Hz, 2H), 4.22 (q, J = 5.2 Hz, 1H), 3.83 (dd, J = 12.4, 3.6 Hz, 1H), 3.74 (dd, J = 12.4, 5.6 Hz, 1H), 3.12 (q, J = 7.3 Hz, CH2-triethylammonium), 1.20 (d, J = 7.3 Hz, CH3-triethylammonium). 13C NMR (151 MHz, D2O) δ (ppm): 166.33, 151.15, 143.88, 143.81, 102.08 (d, J = 14.3 Hz), 92.87 (d, J = 21.3 Hz), 85.11 (d, J = 18.7 Hz), 80.60 (d, J = 20.5 Hz), 77.05 (d, J = 18.3 Hz)., 60.99, 60.88, 60.76, 46.76, 46.65, 46.54, 8.22, 8.21.31P NMR (162 MHz, D2O) δ (ppm): 20.02.ESI-MS m/z calculated for C9H10N2O8P- [M-H]- 305.0180, found 305.0234.
Guanosine-2′,3′-cyclic monophosphate triethylammonium salt (12) (70%)
- 1H NMR (600 MHz, D2O) δ 7.79 (s, 1H), 5.98 (d, J = 4 Hz, 1H), 5.26 (m, 1H), 5.00 (m, 1H), 4.31 (d, J = 3.9 Hz, 1H), 3.79 (dd, J = 12.5, 3.0 Hz, 1H), 3.74 (dd, J = 12.5, 4.0 Hz, 1H), 3.01 (q, J = 7.3 Hz, CH2-triethylammonium), 1.12 (t, J = 7.4 Hz, CH3-triethylammonium). 13C NMR (151 MHz, D2O) δ 164.5, 158.3, 150.9, 136.9, 117.4, 88.9, 88.8, 84.9, 84.9, 80.1, 80.0, 77.6, 61.1, 46.4, 8.19.31P NMR (162 MHz, D2O) δ 19.93. ESI-MS m/z calculated for C10H12N5O7P [M-H]- 344.0402, found 344.0402.
Inosine-2′,3′-cyclic monophosphate triethylammonium salt (13) (84%)
- 1H NMR (600 MHz, D2O) δ 8.11 (s, 1H), 7.99 (d, J = 0.9 Hz, 1H), 6.09 (d, J = 3.9 Hz, 1H), 5.23 (m, 1H), 4.99 (m, 1H), 4.32 (q, J = 4.3 Hz, 1H), 3.79 (dd, J = 12.5, 3.4 Hz, 1H), 3.73 (dd, J = 12.5, 4.8 Hz, 1H), 3.05 (q, J = 7.3 Hz, CH2-triethylammonium), 1.13 (dd, J = 7.8, 6.9 Hz, CH3-triethylammonium). 13C NMR (151 MHz, D2O) δ 157.92, 147.91, 146.08, 140.31, 140.26, 123.82, 89.45, 89.31, 85.46, 85.43, 85.38, 85.36, 80.74, 80.63, 77.47, 77.34, 60.98, 46.7, 8.12.31P NMR (162 MHz, D2O) δ 19.74. ESI-MS m/z calculated for C10H10N4O7P [M-H]- 329.0293, found 329.0293.
Cytidine-2′,3′-cyclic monophosphate triethylammonium salt (14) (88%)
- 1H NMR (600 MHz, D2O) δ 7.62 (d, J = 7.5 Hz, 1H), 5.95 (d, J = 7.5 Hz, 1H), 5.77 (d, J = 2.8 Hz, 1H), 5.05 (td, J = 6.6, 2.7 Hz, 1H), 4.85 (m, 1H), 4.22 (td, J = 5.6, 3.6 Hz, 1H), 3.83 (dd, J = 12.4, 3.6 Hz, 1H), 3.75 (dd, J = 12.3, 5.7 Hz, 1H), 3.12 (q, J = 7.3 Hz, 1 CH2-triethylammonium), 1.20 (t, J = 7.3 Hz, 2 CH3-triethylammonium). 13C NMR (151 MHz, D2O) δ 165.87, 156.18, 144.06, 143.96, 96.08, 95.91, 93.83, 93.79, 93.68, 85.22, 85.08, 81.00, 80.85, 77.35, 77.22, 61.06, 61.05, 45.95, 8.32. 31P NMR (162 MHz, D2O) δ 20.05.ESI-MS m/z calculated for C9H12N3O7P [M-H]- 304.0340, found 304.0341.
Support Protocol: SYNTHESIS OF BIS(DIMETHYLAMINO)PHOSPHORODIAMIDATE SODIUM SALT
This protocol describes the synthesis of BDMDAP (compound 7), which is used as a key ingredient in the Basic Protocol (see Yadav & Krishnamurthy, 2019).
Additional Materials (also see Basic Protocol)
-
Bis(dimethylamino)phosphorochloridate (CAS number 1605-65-8, from Acros Organics)
-
2 M NaOH (for a 10 M stock solution, see Current Protocols, 2006)
-
95% ethanol
-
Whatman filter paper (VWR, cat. no. 28333-065)
1.Add bis(dimethylamino)phosphorochloridate dropwise (5 mM final; over ∼10 min) to a stirred solution of 5.2 ml 2 M NaOH at 0°C.
2.After complete addition, stir the above reaction mixture at room temperature until all the starting material is completely consumed (monitored by 31P NMR).
3.Adjust the final pH of the reaction mixture to 9.0 using 2 M NaOH.
4.Concentrate the reaction mixture under vacuum using the rotary evaporator equipped with a vacuum pump, followed by drying with the high-vacuum oil pump using the 24/40 vacuum adapter.
5.Dissolve the crude residue in 50 ml 95% ethanol and filter and concentrate the filtrate under vacuum using the rotary evaporator equipped with a vacuum pump, followed by drying with the high-vacuum oil pump using the 24/40 vacuum adapter to afford the pure BDMDAP sodium salt as white powder.
Spectral data (see Supporting Information)
BDMDAP sodium salt (7)
1.1H NMR (600 MHz, D2O) δ 2.40 (d, J = 10.2 Hz, 1H); 13C NMR (151 MHz, D2O) δ 36.51 (d, J = 1.7 Hz); 31P NMR (162 MHz, D2O) δ 18.61.
COMMENTARY
Background Information
2′,3′-Cyclic nucleotides have been shown to be an integral part biology: (a) as intermediates in the ribonuclease mediated hydrolysis of RNA (Bernfield, 1965); (b) as substrates for ribonucleases (Remus et al., 2014); and (c) as regulators of certain biological processes (Bordeleau et al., 2014; Newell et al., 2015). More recently, the 2′,3′-cyclic phosphate containing RNAs themselves have been recognized as “hidden layer of the transcriptome” (Shigematsu et al., 2018). Thus, accessing the 2′,3′-cyclic phosphate moiety of the riboside unit is an important endeavor. Historically, the synthesis of the 2′,3′-cyclic nucleotide has involved multistep synthesis and workup procedures that have hindered the scaleup of these reactions, thus limiting their potential applications. A chance discovery (Gibard et al., 2018) that the reaction of diamidophosphate (DAP) with ribonucleosides produced the corresponding 2′,3′-cyclic nucleotides led us to the development of a ‘cyclophosphorylating’ reagent, BDMDAP, for use in synthetic organic chemistry (Yadav & Krishnamurthy, 2019). The addition of dimethyltin dichloride as a catalyst was found to be essential for the efficient conversion to the cyclic phosphate. Using this combination of reagents, we demonstrated the one-pot synthesis of 2′,3′-cyclic nucleotides starting from nucleosides by thermal activation at 80°C for 8 hr with yields of 88-92%. In order to shorten the duration of the reaction we applied microwave-heating. This idea was inspired by our previous use of microwaves for the preparation of phosphoramidites by phosphitylation of RNA and DNA nucleosides (Efthymiou & Krishnamurthy, 2015; Meher et al., 2014) and synthesis of cyclic-phospholipids by combining microwaves and BDMDAP (Ortuno et al., 2022).
Here we show that the combination of BDMDAP and microwave-heating provides robust access to 2′,3′-cylcic nucleotides starting from the respective nucleosides. The reaction is simple in its setup and does not require any special handling procedures. The post reaction processing involves no workup and consists of a straightforward ion-exchange column that directly provides the 2′,3′-cyclic nucleotides in excellent yields (70% for the guanosine derivative at the lower end and 91% for the uridine derivative at the higher end). The reactions can be run in parallel to access gram quantities of these cyclic nucleotides in a short time (usually within a day).
The simplicity of the microwave cyclophosphorylation method has the potential to expand it to other molecules that have a cis-diol moiety (Yadav & Krishnamurthy, 2019) and even perhaps to RNA oligonucleotides themselves to directly produce 2′,3′-cyclic phosphate of RNA at the 3′-end (Le Vay & Mutschler, 2022; Shigematsu et al., 2018).
Critical Parameters
2′,3′-Cyclic nucleotides can be hydrolyzed to the corresponding 2′- or 3′-monophosphate nucleosides (Rudolph et al., 1971). To avoid this, it is important to check the water content in the NMP solvent (see Internet Resources). A typical low water content value is less than 20 ppm. A high water content will interfere with the cyclophosphorylation reaction and the yield of cyclic nucleotides will be low (also due to hydrolysis).
The time spent on the ion-exchange column should be minimized as the 2′,3′-cyclic nucleotides can also hydrolyze if left on the column for long time leading to deceased yield of the products.
Triethylammonium bicarbonate buffer is the preferred buffer for purification of nucleotides since it is a neutral and volatile buffer (Greenhut & Rudolph, 1985; Kore et al., 2012). Removal of the excess triethylammonium bicarbonate is affected by repeated addition of water and concentration in vacuo; however, care should be taken while monitoring the repeated evaporation process for obtaining a constant weight of the product (Kolodziej et al., 2015).
The proper selection of the microwave tube in relation to the scale of the reaction and the amount of the solvent (NMP) is crucial for the reaction (see Internet Resources).
Time Considerations
The entire sequence of reactions from start to finish can be completed in 1 day for each nucleoside, with final NMR analysis taking additional time (30 min). Basic Protocol, steps 1 to 9 take ∼1–2 h; steps 10 to 16 take ∼2–3 hr (same day) depending on the number of fractions recollected during ion-exchange column and the subsequent evaporation of the water/buffer under vacuum.
Acknowledgments
This work was funded by the Simons Foundation to RK (327124FY19) and a NASA exobiology program grant (80NSSC22K0509).
Author Contributions
Kollery S. Veena : Data curation, formal analysis, investigation, methodology, validation, visualization, writing - original draft; Harold A. Cruz : Data curation, formal analysis, investigation, methodology, validation, visualization, writing - original draft; Ramanarayanan Krishnamurthy : Conceptualization, data curation, formal analysis, funding acquisition, investigation, methodology, project administration, resources, supervision, validation, writing - review and editing.
Conflict of Interest
The authors declare no conflict of interest.
Open Research
Data Availability Statement
No new data were created or analyzed during the generation and the writing of the protocol.
Supporting Information
Filename | Description |
---|---|
cpz1834-sup-0001-SuppMat.docx3.2 MB | Contains 1H, 13C, 31P NMR and mass-spectral data of compounds 9-14 and BDMDAP. |
Please note: The publisher is not responsible for the content or functionality of any supporting information supplied by the authors. Any queries (other than missing content) should be directed to the corresponding author for the article.
Literature Cited
- Bernfield, M. R. (1965). Ribonuclease and oligoribonucleotide synthesis: I. Synthetic activity of bovine pancreatic ribonuclease derivatives. Journal of Biological Chemistry , 240(12), 4753–4762. https://doi.org/10.1016/S0021-9258(18)97020-1
- BioLog, L. S. I. (2023). 2′,3′-cyclic nucleotides. Retrieved from https://www.biolog.de/cyclic-nucleotides/2-3-cyclic-nucleotides
- Bordeleau, E., Oberc, C., Ameen, E., da Silva, A. M., & Yan, H. (2014). Identification of cytidine 2′,3′-cyclic monophosphate and uridine 2′,3′-cyclic monophosphate in Pseudomonas fluorescens pfo-1 culture. Bioorganic & Medicinal Chemistry Letters, 24(18), 4520–4522. https://doi.org/10.1016/j.bmcl.2014.07.080
- Brown, D. M., Magrath, D. I., & Todd, A. R. (1952). 511.Nucleotides. Part XII. The preparation of cyclic 2′: 3′-phosphates of adenosine, cytidine, and uridine. Journal of the Chemical Society (Resumed) , 0, 2708–2714. https://doi.org/10.1039/JR9520002708
- Current Protocols. (2006). Commonly Used Reagents. Current Protocols in Microbiology , 00, A.2A.1–A.2A.15. https://doi.org/10.1002/9780471729259.mca02as00
- Efthymiou, T., & Krishnamurthy, R. (2015). Microwave-assisted phosphitylation of DNA and RNA nucleosides and their analogs. Current Protocols in Nucleic Acid Chemistry , 60(1), 2.19.11–12.19.20. https://doi.org/10.1002/0471142700.nc0219s60
- Eto, M., Iio, M., Omura, H., & Eto, M. (1978). Direct synthesis of ribonucleoside 2′,3′-cyclic phosphate from ribonucleoside. Agricultural and Biological Chemistry , 42(1), 199–200. https://doi.org/10.1080/00021369.1978.10862953
- Gibard, C., Bhowmik, S., Karki, M., Kim, E. K., & Krishnamurthy, R. (2018). Phosphorylation, oligomerization and self-assembly in water under potential prebiotic conditions. Nature Chemistry , 10(2), 212–217. https://doi.org/10.1038/nchem.2878
- Greenhut, J., & Rudolph, F. B. (1985). Preparative separation of nucleotides by high-performance-ion exchange liquid chromatography using a volatile buffer system. Journal of Chromatography A , 319, 461–466. https://doi.org/10.1016/S0021-9673(01)90591-6
- Hoard, D. E., & Ott, D. G. (1965). Conversion of mono- and oligodeoxyribonucleotides to 5′-Triphosphates1. Journal of the American Chemical Society , 87(8), 1785–1788. https://doi.org/10.1021/ja01086a031
- Kolodziej, K., Romanowska, J., Stawinski, J., Kraszewski, A., & Sobkowski, M. (2015). The case of triethylammonium cation loss during purification of certain nucleotide analogues: A cautionary note. Analytical and Bioanalytical Chemistry , 407(6), 1775–1780. https://doi.org/10.1007/s00216-014-8397-0
- Kore, A. R., Shanmugasundaram, M., Senthilvelan, A., & Srinivasan, B. (2012). Gram-scale chemical synthesis of 2′-deoxynucleoside-5′-O-triphosphates. Current Protocols in Nucleic Acid Chemistry , 49(1), 13.10.11–13.10.12. https://doi.org/10.1002/0471142700.nc1310s49
- le Vay, K. K., & Mutschler, H. (2022). Generation of RNA with 2′, 3′-cyclic phosphates by deoxyribozyme cleavage in frozen solutions. In G. Steger, H. Rosenbach, & I. Span (Eds.), DNAzymes: Methods and protocols (pp. 301–309). Springer US.
- Meher, G., Efthymiou, T., Stoop, M., & Krishnamurthy, R. (2014). Microwave-assisted preparation of nucleoside-phosphoramidites. Chemical Communications , 50(56), 7463–7465. https://doi.org/10.1039/c4cc03092c
- Mohamady, S., & Taylor, S. D. (2016). One flask synthesis of 2′,3′-cyclic nucleoside monophosphates from unprotected nucleosides using activated cyclic trimetaphosphate. Tetrahedron Letters , 57(49), 5457–5459. https://doi.org/10.1016/j.tetlet.2016.10.076
- Newell, E. A., Exo, J. L., Verrier, J. D., Jackson, T. C., Gillespie, D. G., Janesko-Feldman, K., Kochanek, P. M., & Jackson, E. K. (2015). 2′,3′-cAMP, 3′-AMP, 2′-AMP and adenosine inhibit TNF-α and CXCL10 production from activated primary murine microglia via A2A receptors. Brain Research , 1594, 27–35. https://doi.org/10.1016/j.brainres.2014.10.059
- Ortuno, V. E., Pulletikurti, S., Veena, K. S., & Krishnamurthy, R. (2022). Synthesis and hydrolytic stability of cyclic phosphatidic acids: Implications for synthetic- and proto-cell studies. Chemical Communications , 58(42), 6231–6234. https://doi.org/10.1039/D2CC00292B
- Remus, B. S., Jacewicz, A., & Shuman, S. (2014). Structure and mechanism of E. coli RNA 2′,3′-cyclic phosphodiesterase. RNA , 20(11), 1697–1705. https://doi.org/10.1261/rna.046797.114
- Rudolph, S. A., Johnson, E. M., & Greengard, P. (1971). The enthalpy of hydrolysis of various 3′,5′- and 2′,3′-cyclic nucleotides. Journal of Biological Chemistry , 246(5), 1271–1273. https://doi.org/10.1016/S0021-9258(19)76969-5
- Shigematsu, M., Kawamura, T., & Kirino, Y. (2018). Generation of 2′,3′-cyclic phosphate-containing RNAs as a hidden layer of the transcriptome. Front Genet , 9(562), 562. https://doi.org/10.3389/fgene.2018.00562
- Simoncsits, A., & Tomasz, J. (1975). A simple one-step synthesis of ribonucleoside 2′,3′-cyclic phosphate 5′-phosphates. Biochimica et Biophysica Acta (BBA) - Nucleic Acids and Protein Synthesis , 395(1), 74–79. https://doi.org/10.1016/0005-2787(75)90235-X
- Tener, G. M., & Khorana, H. G. (1955). Cyclic phosphates. II. Further studies of ribonucleoside 2′:3′-cyclic Phosphates1. Journal of the American Chemical Society , 77(20), 5349–5351. https://doi.org/10.1021/ja01625a043
- van Boom, J. H., de Rooy, J. F. M., & Reese, C. B. (1973). The synthesis of oligoribonucleotides. Part X. Preparation of 2′,3′-cyclic phosphates of ribonucleosides and diribonucleoside phosphates via phosphotriester intermediates. Journal of the Chemical Society, Perkin Transactions , 1(0), 2513–2517. https://doi.org/10.1039/P19730002513
- Yadav, M., & Krishnamurthy, R. (2019). Bis(dimethylamino)phosphorodiamidate: A reagent for the regioselective cyclophosphorylation of cis-Diols enabling one-step access to high-value target cyclophosphates. Organic Letters , 21(18), 7400–7404. https://doi.org/10.1021/acs.orglett.9b02694
Internet Resources
Determining water content in the NMP solvent using a Mettler Toledo C20 Coulometric KF titrator.
Further guidance for the proper selection of microwave tube.