Methods to Detect Small Molecule Inhibition of RING E3 Ligase Activity
Ina Rothenaigner, Ina Rothenaigner, Jara Kerstin Brenke, Jara Kerstin Brenke, Kenji Schorpp, Kenji Schorpp, Kamyar Hadian, Kamyar Hadian
Abstract
Protein ubiquitination is an essential post-translational modification that regulates a large number of cellular processes. This reaction is facilitated by the consecutive action of three central enzymes, i.e., E1 activating enzyme, E2 conjugating enzyme, and the E3 ligase. More than 600 E3 enzymes guarantee the specificity and selectivity of these reactions and thus represent an exciting, while highly underrepresented, class of drug targets. Specific methods can be employed to monitor their activity and thus query compound libraries for inhibitory small molecules. Here, we describe two protocols—one high-throughput and one low-throughput method—to detect E3 ligase activity and test small molecule modulation. © 2022 The Authors. Current Protocols published by Wiley Periodicals LLC.
Basic Protocol 1 : AlphaScreen assay to measure TRAF6-Ubc13 interaction
Basic Protocol 2 : Gel-based in vitro ubiquitination assay (K63-linked chains)
INTRODUCTION
Ubiquitination of proteins is a reversible post-translational modification that regulates the function of essential regulators of almost all cellular signaling pathways including protein homeostasis, cell death, transcription, cell cycle, trafficking, DNA damage response, and many more. The enzyme cascade consisting of an ubiquitin-activating E1 enzyme (Schulman & Harper, 2009), an ubiquitin-conjugating E2 enzyme (Ye & Rape, 2009), and an ubiquitin-ligating E3 enzyme is needed to covalently attach ubiquitin to the substrate proteins. This process is reversible through the action of deubiquitinating enzymes (DUBs; Fig. 1A). The E3 ligases contain domains that determine the substrate specificity and/or the localization to specific sites in the cell and mediate the transfer of ubiquitin from the E2 to the specific protein substrate. E3 ligases are the largest and most heterogeneous components in the ubiquitination reaction and are divided according to the catalytic structure into three subfamilies: Really interesting new gene (RING) E3 ligases, HECT E3 ligases, and RBR E3 ligases (Buetow & Huang, 2016; Li et al., 2008). The RING E3 ligases are characterized by the zinc-binding RING domain. Two groups of RING E3 ligases exist: Mono-subunit enzymes (e.g., TRAF6) and the multi-subunit RING ligase complexes (cullin-RING ligases, CRL). The RING domain mediates the binding of the ubiquitin-charged E2 and stimulates the direct ubiquitin transfer from E2 to the substrate (Fig. 1B). Here, the function of the E3 ligase is to bring the E2 protein in close proximity to its substrate protein and thereby guarantee substrate specificity. In contrast, HECT and RBR E3 ligases comprise an internal cysteine to first take up the ubiquitin and subsequently transfer it to the substrate.
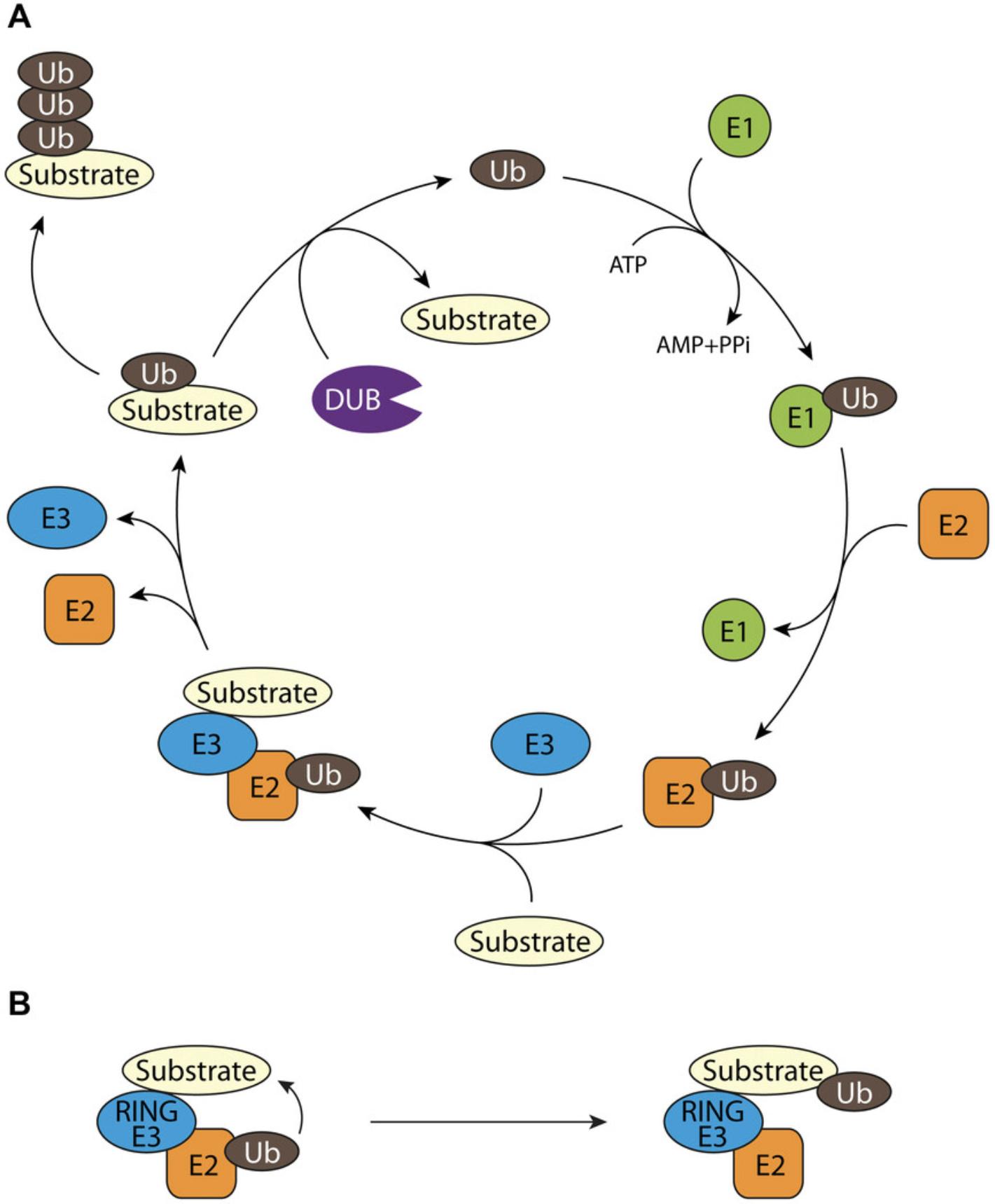
Dysregulation of the ubiquitin system leads to a variety of diseases, including cancer, autoimmune diseases, and neurodegeneration (Popovic, Vucic, & Dikic, 2014). As the ubiquitination via RING E3 ligases critically requires the protein-protein interaction (PPI) with the E2 enzymes, these types of interactions can be utilized to identify small molecule disruptors. So far there are only a few FDA-approved drugs targeting the ubiquitin system and these are mainly modulating the proteasome. As each of the ∼600 E3 ligases regulates a distinct set of targets, these enzymes are getting more attention as valuable drug targets with advanced selectivity and potentially fewer side effects compared to, e.g., proteasome inhibitors. A possible mode of action for small molecules that inhibit E3 ligases is the inhibition of the E3 ligase interaction with its respective E2 conjugating enzyme (Brenke et al., 2018; Scott et al., 2017).
TRAF6 belongs to the RING-type E3 ligases and does not contain an internal cysteine (Yin et al., 2009). Hence, the ubiquitin transfer occurs, upon E2 enzyme (Ubc13 in the case of TRAF6) binding, directly from Ubc13 to the substrate. This is conceptually visualized in Figure 1B. Importantly, as Ubc13 binds to the RING domain, compounds inhibiting TRAF6-Ubc13 binding do not interfere with the TRAF6-substrate binding. The affinity of TRAF6 (RING-Z1)-Ubc13 binding is ∼1.5 µM (Yin et al., 2009); thus, this PPI is favorable for compound interference even at mid-nM concentrations. In line with this, targeting TRAF6-Ubc13 interaction has yielded effective inhibitors with good selectivity across a variety of tested E3 ligases and in vivo activity (Brenke et al., 2018). TRAF6-mediated ubiquitination processes are dependent on activation of receptor signaling processes (e.g., IL-1β or Toll-like receptors) and in turn lead to downstream activation of the NF-κB or MAP kinase pathways (Walsh, Lee, & Choi, 2015).
Here, we describe two protocols that can be used to detect small molecule inhibition of RING E3 ligase activity, exemplified by the inhibition of the E3 ligase TRAF6. The fundamental difference between the two protocols is their throughput capacity.
The first protocol is a high-throughput amendable method—AlphaScreen (amplified luminescent proximity homogeneous assay)—that can be utilized to search for PPI inhibitors (Basic Protocol 1, see Fig. 2). This technology was adapted to measure the TRAF6-Ubc13 PPI and has already been successfully used in a screening campaign for the discovery of a TRAF6 E3 ligase inhibitor (Fig. 3; Brenke et al., 2018).
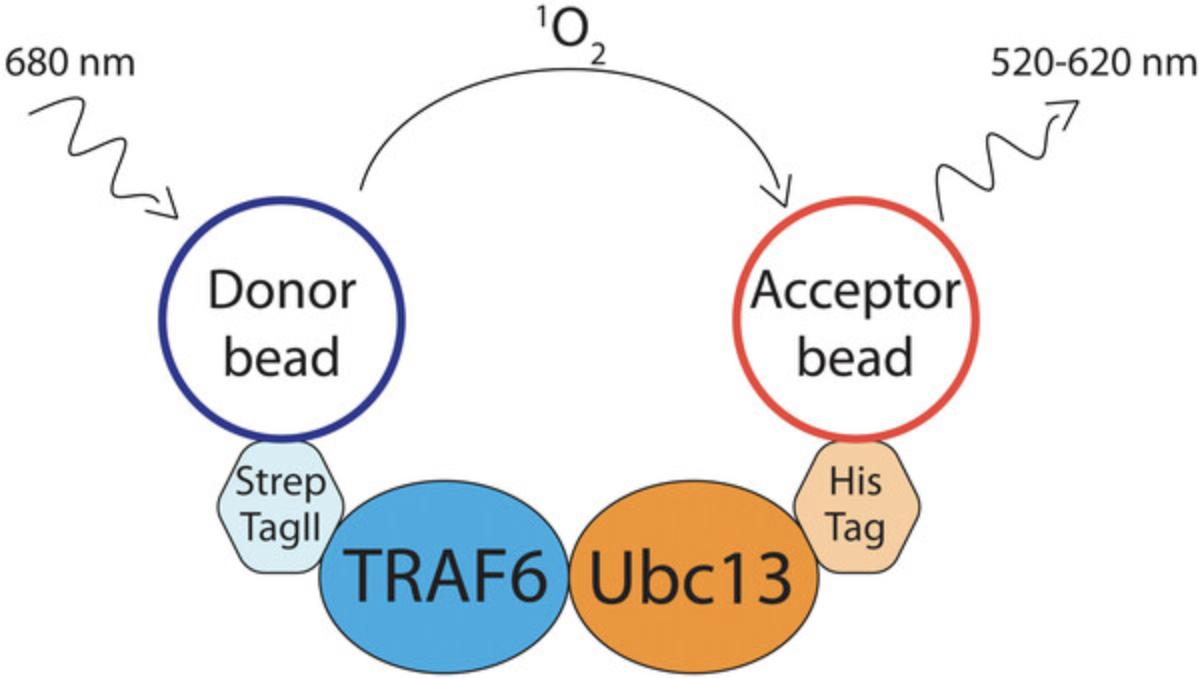
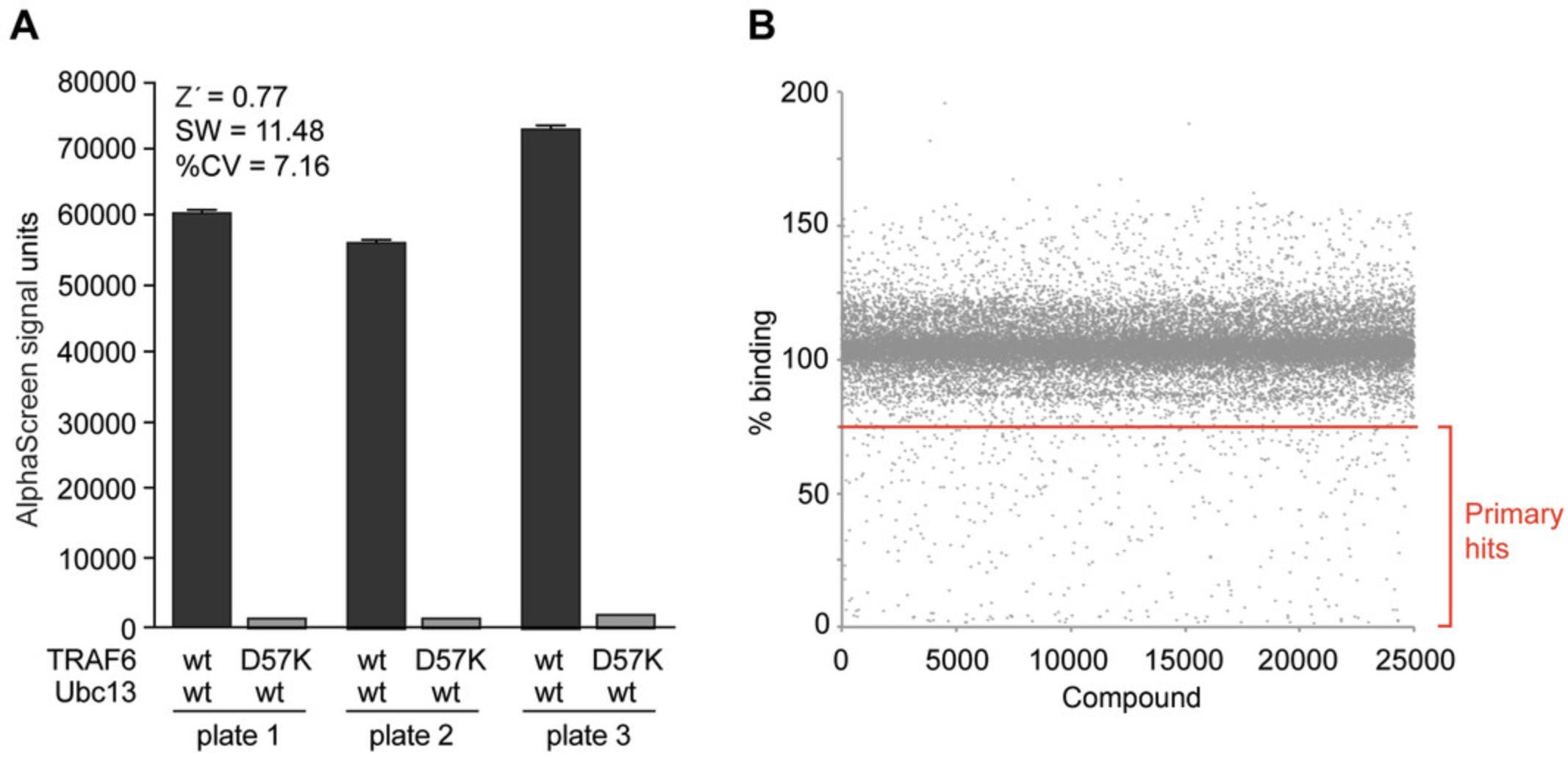
The second tool to identify RING E3 ligase inhibitors is an in vitro ubiquitination assay (Basic Protocol 2), here again described to monitor TRAF6 activity. In a biochemical enzyme reaction with addition of E1 and E2 (Ubc13) enzymes, ubiquitin (Ub), and ATP, the ability of TRAF6 to form ubiquitin chains as well as auto-ubiquitination is analyzed. This assay is suitable to validate identified small molecule inhibitors in a low-throughput, western blot format and has also been demonstrated to be effective and accurate (Fig. 4; Brenke et al., 2018).
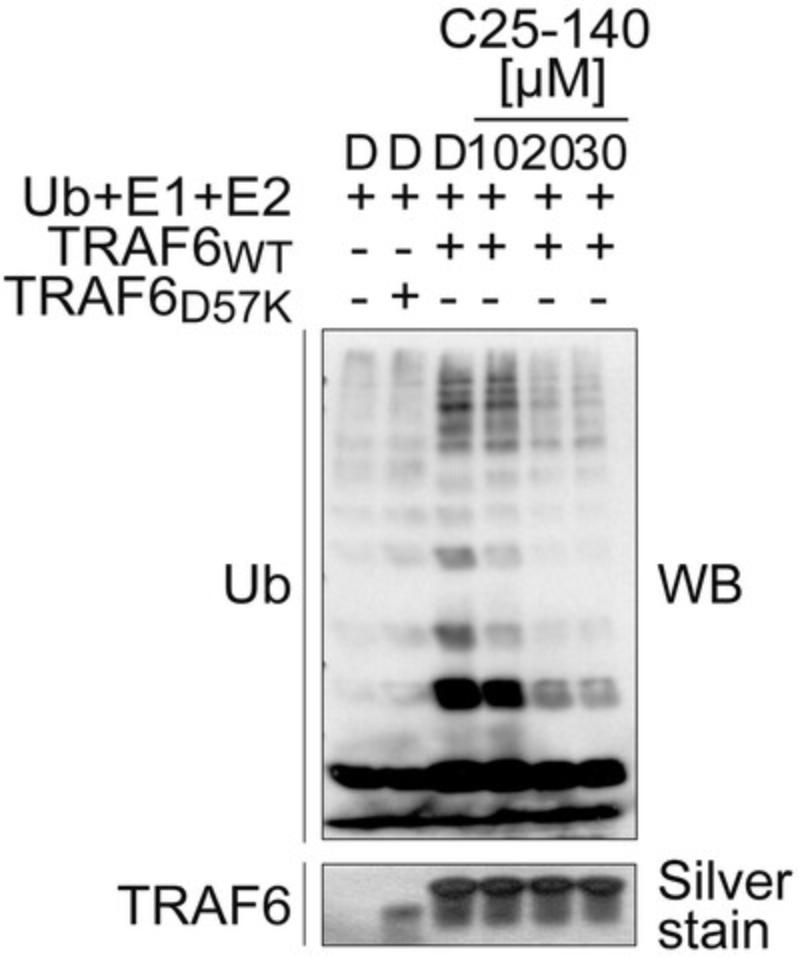
Basic Protocol 1: ALPHASCREEN ASSAY TO MEASURE TRAF6-UBC13 INTERACTION
This protocol is used to identify small molecule inhibitors of TRAF6-Ubc13 interaction. The assay is based on AlphaScreen, which is a bead-based assay for studying biomolecular interactions in a microplate format. Here, Strep-Tactin donor beads bind to StrepII-TRAF6-RZ1 (RZ1 domain; present as dimer) and nickel-chelate acceptor beads bind to Ubc13-FlagHis. Upon interaction of the two protein partners, beads get into close proximity and singlet oxygen is transferred from donor to acceptor beads generating a luminescent signal that can be detected in a suitable microplate reader (Fig. 2). Small molecule inhibitors interrupting this interaction diminish the AlphaScreen signal. We describe the reaction conditions that were used in a screening campaign to identify small molecule inhibitors of TRAF6-Ubc13 (Brenke et al., 2018). Reaction conditions for other E3 RING ligases can be altered accordingly to meet specific requirements. Besides TRAF6-Ubc13, AlphaScreen has also been shown to be suitable to detect, e.g., RNF8-Ubc13 interaction (Weber, Rothenaigner, Brandner, Hadian, & Schorpp, 2017).
Materials
-
Recombinant StrepII-TRAF6-RZ1 protein, only RING-zinc finger1 (RZ1) domain: Wild-type (WT) or D57K mutant (Brenke et al., 2018)
-
Recombinant Ubc13-FlagHis protein
-
AlphaScreen buffer (see recipe)
-
Strep-Tactin Alpha Donor beads (AS106, PerkinElmer)
-
AlphaScreen Histidine (Nickel Chelate) Detection Kit containing nickel chelate (Ni-NTA) AlphaScreen Acceptor beads (6760619C, Perkin Elmer)
-
Small molecules in DMSO (specific to the research question)
-
Dimethyl sulfoxide (DMSO of high purity)
-
384-well white OptiPlates (6007290, Perkin Elmer)
-
Multiplate Dispenser (e.g., Flexdrop or Multidrop)
-
Liquid Handling (e.g., Sciclone G3, PerkinElmer)
-
Cover foil (to seal plate; DMSO resistant)
-
Plate reader (e.g., PerkinElmer Envision)
-
NOTE : The TRAF6 D57K mutant does not bind Ubc13 and acts as a control for the low signal. This mutant is a mimic of full compound inhibition.
1.Purify recombinant proteins in bacterial systems: StrepII-TRAF6-RZ1 (WT or D57K mutant) and Ubc13-FlagHis.
2.Prepare protein stocks: (1) StrepII-TRAF6-RZ1 (WT or D57K mutant) at 200 nM in AlphaScreen buffer and (2) Ubc13-FlagHis at 450 nM in AlphaScreen buffer. For master mixes, calculate 30 µl per sample for StrepII-TRAF6-RZ1 (WT or D57K mutant) and 10 µl per sample for Ubc13-FlagHis.
3.Dispense 30 µl StrepII-TRAF6-RZ1 (WT or D57K mutant; 200 nM in AlphaScreen buffer, final concentration: 100 nM) into each well of a 384-well Optiplate.
4.Add 0.3 µl compound (stock concentration: 1 mM; final concentration: 5 µM in 60 µl) to TRAF6-RZ1 protein (WT or D57K mutant) to each well of the 384-well plate using liquid handling and incubate 30 min at room temperature.
5.Dispense 10 µl Ubc13-FlagHis (450 nM in AlphaScreen buffer, final concentration: 75 nM) into each well of the 384-well plate.
6.Seal the 384-well plate with foil and incubate 1 hr at room temperature.
7.Prepare working solutions for the AlphaScreen beads (stock is 5 mg/ml). Dilute both beads (Donor and Acceptor) separately to 24 µg/ml in the AlphaScreen buffer.
8.Dispense 10 µl Donor beads and 10 µl Acceptor beads into each well of the 384-well plate containing the proteins/compound mix.
9.Seal plate with foil and incubate 1 hr at room temperature.
10.Read out the plate with an AlphaScreen-compatible plate reader (settings: laser excitation at 680 nm, emission at 520-620 nm).
Sample data
See Figure 3.
Basic Protocol 2: GEL-BASED IN VITRO UBIQUITINATION ASSAY (K63-LINKED CHAINS)
This in vitro assay is used to examine the activity of purified E3 ligases to form K63-linked poly-ubiquitin chains after small molecule treatment. This protocol describes how to measure the ability of the E3 ligase TRAF6 in cooperation with the E2 ligase Ubc13 to build free K63-linked ubiquitin chains. Recombinant E1 and E2 enzymes are commercially available. The result of the reaction is checked by western blot analyses using an anti-ubiquitin antibody. This protocol is a low-throughput method that is suitable to validate a shortlisted number of molecules from a screening campaign using, e.g., Basic Protocol 1.
Materials
-
10× K63 assay buffer (see recipe)
-
Recombinant His6-ubiquitin E1 enzyme (UBE1; E-304-050, R&D Systems)
-
Recombinant His6-UBE2N(Ubc13)/Uev1a complex (E2-664, R&D Systems)
-
Recombinant E3-ubiquitin ligase TRAF6, only RING-zinc finger1 (RZ1) domain (Brenke et al., 2018)
-
Recombinant ubiquitin protein (U-100H-10M, R&D Systems)
-
1 mM ZnCl2 in 1× K63 assay buffer
-
Small molecules in DMSO (specific to the research question)
-
Dimethyl sulfoxide (DMSO of high purity)
-
2 mM ATP (MilliporeSigma)
-
SDS loading buffer (4×)
-
10× SDS buffer (see recipe)
-
5× separation gel buffer (1.88 M Tris·HCl, pH 8.8)
-
Separation gel (see recipe)
-
4× stacking gel buffer (0.5 M Tris·HCl, pH 6.8)
-
Stacking gel (see recipe)
-
Pre-stained Protein Marker (Page Ruler)
-
Pierce™ Silver Stain Kit
-
Methanol
-
10× blotting buffer (see recipe)
-
Blocking buffer: 3% BSA (standard quality) in PBST
-
PBST: PBS (Applichem) with 0.1% Tween 20 (Carl Roth)
-
Anti-ubiquitin antibody (P4D1, 1:1,000; Santa Cruz)
-
Secondary HRP-conjugated anti-mouse antibody (1:7,500; Dianova)
-
1.5-ml microcentrifuge tubes
-
SDS-PAGE gel chamber
-
PVDF membrane
-
Whatman paper
-
Semi dry blotter
-
Amersham Hyperfilm ECL
1.Purify recombinant proteins in bacterial systems: Untagged TRAF6-RZ1 (WT or D57K mutant).
2.Thaw reagents and place them on ice: His6-ubiquitin E1 enzyme, His6-UBE2N (Ubc13)/Uev1a complex, purified E3-ubiquitin ligase TRAF6-RZ1, ubiquitin, ZnCl2, and ATP. Compounds and DMSO should be placed at room temperature.
3.Pre-incubate 0.125 µM TRAF6-RZ1 with the compound (amount dependent on concentration to be tested) or DMSO in 1× K63 assay buffer for 30 min. The total reaction volume is 50 µl.
4.Prepare master mix of 0.01 μM His6-ubiquitin E1 enzyme, 0.2 μM His6-UBE2N (Ubc13)/Uev1a complex, 4 μM ubiquitin, 1 mM ZnCl2, and 2 mM ATP in 1× K63 reaction buffer. Add 50 µl master mix to start reaction. Incubate reaction 120 min at 37°C.
5.Stop reaction by adding 25 µl SDS loading buffer (4×), vortex to mix, and boil samples at 95°C for 5 min.
6.Load the input samples on a 12.5% acrylamide gel and the ubiquitination samples on a 10% acrylamide gel for SDS-PAGE electrophoretic separation.
7.The input sample gel will be used directly for silver staining, whereas the ubiquitination sample gel will be used for transfer of the separated proteins onto a PVDF membrane via semi-dry blotting.
8.Block the membrane for 60 min in a blocking buffer at room temperature to cover unspecific binding sites and subsequently incubate the ubiquitination sample membrane with an anti-ubiquitin antibody (P4D1) overnight at 4°C.
9.After washing the membrane three times for 10 min with PBST, add the horseradish-peroxidase-conjugated secondary anti-mouse antibody. Incubate 1 hr at room temperature.
10.Wash membrane again three times with PBST and add enhanced chemiluminescence substrate according to manufacturer's instructions. Detect chemiluminescence using X-ray films for various time points and develop the films in a developer machine.
Sample data
See Figure 4.
REAGENTS AND SOLUTIONS
AlphaScreen buffer
- 1× PBS, pH 7.3 (Thermo Fisher Scientific, 14190-094); store at room temperature
- 0.5% BSA, purity ≥98% (MilliporeSigma, A7030-100G); store at 2°C to 8°C
- 0.01% Tween 20 (Carl Roth, 9127.2); store at room temperature
- Store at 4°C for a few days
Blotting buffer, 10×
- 48 mM Tris (Carl Roth, A411.2); store at room temperature
- 39 mM glycine (Carl Roth, 3908.3); store at room temperature
- 0.03% SDS (Carl Roth, 2326.2); store at room temperature
- 20% methanol (Merck, 1.06009.2500); store at room temperature
- Store at room temperature for several months
K63 assay buffer, 10×
- 250 nM Tris·HCl, pH 7.6 (Carl Roth, A411.2); store at room temperature
- 25 mM MgCl2 (Carl Roth, KK36.2); store at room temperature
- 50 mM creatine phosphate, disodium salt, ≥97% (MilliporeSigma, 2380); store at −20°C
- 3 U/ml inorganic pyrophosphatase
- 3 U/ml creatine phosphokinase
- Use immediately.
SDS buffer, 10×
- 250 mM Tris (Carl Roth, A411.2); store at room temperature
- 2 M glycine (Carl Roth, 3908.3); store at room temperature
- 1% SDS (Carl Roth, 2326.2); store at room temperature
- Store at room temperature for months
Separation gel
- 375 mM Tris·HCl, pH 8.8 (Carl Roth, A411.2); store at room temperature
- 12.5% acrylamide-bisacrylamide (Rothiphorese® Gel 30; Carl Roth, 3029.1); store at 4°C to 8°C
- 0.1% SDS (Carl Roth, 2326.2); store at room temperature
- 0.075% ammonium persulfate (APS; Bio-Rad, 161-0700); store at room temperature
- 0.05% N,N,N′,N′-tetramethyl ethylenediamine (TEMED; Bio-Rad, 161-0800); store at 4°C to 8°C
- Use immediately.
Stacking gel
- 125 mM Tris·HCl, pH 6.8 (Carl Roth, A411.2); store at room temperature
- 5% acrylamide-bisacrylamide (Rothiphorese® Gel 30; Carl Roth, 3029.1); store at 4°C to 8°C
- 0.1% SDS (Carl Roth, 2326.2); store at room temperature
- 0.1% ammonium persulfate (APS; Bio-Rad, 161-0700); store at room temperature
- 0.1% N,N,N′,N′-tetramethyl ethylenediamine (TEMED; Bio-Rad, 161-0800); store at 4°C to 8°C
- Use immediately.
COMMENTARY
Background Information
The hierarchical enzyme cascade orchestrated by the E1, E2, and E3 enzymes facilitates ubiquitination of target proteins during post-translational modification processes. It is important to notice that E3 ligases are subdivided into three main categories, namely RING, HECT, and RBR E3 ligases. Their enzymatic functions differ a lot, as RING ligases do not possess an internal cysteine, while HECT and RBR ligases do. Here, we only concentrate on targeting RING E3 ligases with small molecules for therapeutic interventions.
TRAF6 (a RING E3 ligase) interacts with the heterodimeric E2 enzyme Ubc13/Uev1a to attach K63-linked ubiquitin chains to its target proteins. This process is essential for signal progression in numerous cellular signaling processes, such as (1) T-cell receptor signaling, (2) IL-17 signaling, and (3) innate immune responses (Walsh et al., 2015). Disease onset and progression of distinct autoimmune diseases such as rheumatoid arthritis (RA) are notably dependent on these signaling pathways (McInnes, Buckley, & Isaacs, 2016), thereby making TRAF6 an attractive target for therapeutic interventions. Using a high-throughput small-molecule screening approach, an inhibitor of TRAF6–Ubc13 interaction was discovered that reduces TRAF6 activity both in vitro and in cells (Brenke et al., 2018). Thus, the inhibition of TRAF6 activity by small molecules represents a promising novel strategy for targeting autoimmune and chronic inflammatory diseases.
Importantly, ubiquitin is directly transferred from ubiquitin-charged Ubc13 (E2) to the substrate, both proteins bound by the E3 ligase TRAF6. Thus, targeting TRAF6 E3 activity requires the identification of protein–protein interaction inhibitors, which disrupt the TRAF6–Ubc13 interaction because TRAF6 has no intrinsic catalytic activity. Therefore, we present Basic Protocol 1 that monitors TRAF6-Ubc13 binding and Basic Protocol 2 that detects TRAF6 activity generating poly-ubiquitin chains. The consecutive use of both methods provides a thorough understanding of the compounds’ effects.
Critical Parameters
Critical parameters important for both protocols:
- Expression and purification of E3 ligases in bacterial systems can be very demanding. Thus, purification of the RING domain alone or the RING domain together with an adjacent domain may be much easier to generate. In most cases this will be sufficient to produce robust AlphaScreen binding signals or poly-ubiquitin moieties in in vitro ubiquitination assays.
- Purified proteins should always be snap-frozen in liquid nitrogen prior to long-term storage at −80°C. Importantly, prepare small aliquots to avoid multiple freeze and thaw cycles of recombinant proteins.
- A binding mutant form of the E3 ligase (point mutation in the RING domain, e.g., D57K in the case of TRAF6) should be generated that does not bind to the E2 protein anymore. This will represent the negative control, i.e., the low signal of the AlphaScreen reaction as well as no poly-ubiquitin smears in in vitro ubiquitination reactions.
- It might be necessary to test different tags attached to the respective protein as well as various tag combinations, such as the size of the tag as well as its location on the protein itself (N- versus C-terminus), because these may have an impact on activity in the assay. For example, one tag may cover the site of protein-protein binding or oligomerization of the tag may impact activity of the protein.
Critical parameters for AlphaScreen binding assay (Basic Protocol 1):
- When working with the AlphaScreen technology, it is important to consider the hook effect. As the beads only have a limited capacity of protein binding sites, these can get saturated. Thus, the hook effect describes the fact that extended amounts of either of the proteins, which is beyond the capacity of the beads to bind, will act as inhibitors of the reaction and reduce the signal.
- Both the protein solutions and the AlphaScreen bead mix must be freshly prepared on ice and used immediately to avoid increased background signals. This is a very critical consideration as this will remarkably affect the signal-to-noise ratio.
- AlphaScreen assays should always be carried out in subdued light conditions to ensure no bleaching of the beads due to photosensitivity.
- White opaque plastic plates are required for reading AlphaScreen; it is advisable to use white plates for a better outcome. Black plates will absorb light and lead to greatly reduced signals.
- Buffer choice can be very important. Choose pH, buffering capacity, and salt concentration that will facilitate the desired interactions between the PPI of your assay. In critical instances, a buffer screen might be needed to improve signal output. BSA concentration of 0.1% (w/v) is sufficient to minimize non-specific interactions. A highly pure version of BSA (>98%) is mandatory for good signal output. In non-critical instances, the AlphaScreen buffer can be a buffer of choice and a good starting point.
- Chemiluminescent reactions such as the ones involved in AlphaScreen signal generation are temperature dependent. For the best outcome always perform reactions at constant temperatures around room temperature.
- Always include important controls: (1) both beads without proteins; (2) both beads with either of the protein pairs; (3) one of the beads with both proteins.
Critical parameters for gel-based in vitro ubiquitination assay (Basic Protocol 2):
- Always include important controls: During the assay development phase, always run control reactions (1) without ATP (or if possible, as in our case, an E3 ligase mutant with ATP) and (2) without the E3 ligase.
- Reactions without the E3 ligase will also generate smaller amounts of poly-ubiquitin chains as the E2 enzyme together with the E1 enzyme in a biochemical reaction can assemble ubiquitin chains, although with much less efficiency. It is critical that there is a substantial increase in poly-ubiquitin smears in the presence of the E3 ligase.
- The time window for measuring the compound inhibition is limited and critical. As compounds are usually non-covalent, there will be an equilibrium of on/off rates, which over time, will lead to accumulation of covalent and irreversible ubiquitination. This will dilute the effect.
Troubleshooting
When using the AlphaScreen technology for testing inhibitory compounds, it is important to be aware that many compounds interfere with the underlying chemistry; both inhibitory compounds and interfering compounds may reduce the assay signal. Frequent hitters (i.e., promiscuous compounds) will generate false positive hits that need to be eliminated from the hit list. Chemoinformatics filters can help identify these frequent hitters (Baell & Holloway, 2010; Brenke et al., 2016; Schorpp et al., 2014). Hit evaluation in orthogonal and counter screens is mandatory to confirm activity and specificity (Rothenaigner & Hadian, 2021; Weber et al., 2017).
A counter assay can include the analysis of a linear fusion of StrepII and His to test compound activity in an AlphaScreen assay that is not relying on the PPI. Another strategy of analyzing false positive compounds in an AlphaScreen assay is to apply different PPI pairs and identical tags or the same PPI pairs with different protein tags (e.g., StrepII/His versus GST/His). A genuine TRAF6-Ubc13 inhibitory compound should diminish signals of different tag combinations with the same PPI of interest (here TRAF6 and Ubc13). However, it should not reduce AlphaScreen signals with other PPI pairs or in assays using the linear fusion constructs (Rothenaigner & Hadian, 2021). A study by Brenke et al. (2016) used a GST-His construct to verify GST frequent hitters. This study exemplifies the benefit of using such linear fusions. The gel-based ubiquitination assay is also used as a confirmation assay. Due to the entirely different assay detection methods, AlphaScreen assay artifacts should not have any influence on the formation of poly-ubiquitin chains in the gel-based assay and could therefore be identified and eliminated from the hit list.
Gel-based in vitro ubiquitination reactions are generally straightforward. If enzyme reactions do not work, repeat reactions with fresh enzymes and ATP. Moreover, the percentage of acrylamide of the SDS gels may be an important factor in the visualization of a poly-ubiquitin smear and should be adapted if necessary. When establishing in vitro ubiquitination reactions for other E3 ligases, it might be advantageous to perform a time course first. Taking samples after various time points will help to determine the optimal incubation time for visualization of the poly-ubiquitin smear. In order to analyze the compounds selectivity, various E3 ligase ubiquitination assays could be run in parallel. Several E3 ligases are also commercially available and can be adopted to the described protocol.
Understanding Results
AlphaScreen signals are generally presented as raw signal measurement data that are extracted from plate readers. These numbers may vary from reader to reader. In general, it is important to compare positive and negative controls and aim to achieve a minimum of 5-fold increase of the positive signal over the negative signal as this will guarantee a more robust screening assay. Having these numbers, one can calculate Z′ values, signal windows, and variation of plate-to-plate or day-to-day results (Hughes, Rees, Kalindjian, & Philpott, 2011). During high-throughput screening there will be only one measurement per compound. This limitation is based on the need to maximize throughput.
The output of a gel-based in vitro ubiquitination reaction is the detection of smears in ubiquitin-stained western blot analyses. These smears represent various lengths of ubiquitin linkages (i.e., di-Ub, tri-Ub, tetra-Ub). There are two types of poly-ubiquitin moieties possible: (1) auto-ubiquitination of TRAF6 or (2) free poly-ubiquitin chains. To be able to understand the results, a control without ATP (or if possible, as in our case, an E3 ligase mutant with ATP) as well as a control lacking the E3 ligase is mandatory to be able to visualize the smear.
Time Considerations
Assay development times for AlphaScreen assays are typically very short due to the rapid speed with which one can generate data. Once the purified components are available, the assay can be set up very quickly, typically within a few hours. The set-up experiment using cross-titrations of both binding partners to find the optimal protein concentrations can be performed on 384-well plates in 1 or 2 days, thereby running multiple iterations for refinement. When protein concentrations are optimized and robust signals with low background are available, the assay can be performed in high-throughput format (96- or 384-well plates, or even 1,536-well plates) in <1 day. For AlphaScreen assays, reagents are reasonably stable and the setup of the experiment can tolerate delays.
The in vitro ubiquitination assay takes 2 days to finalize, which is more time consuming than the AlphaScreen assay. On the first day, the enzyme reaction is carried out within 2 to 3 hr. Next, samples are loaded onto SDS gel and subsequently transferred to membranes for western blot analysis. Ubiquitin antibody incubation occurs overnight and the remaining steps of the western blot analysis happen on the second day. Alternatively, the ubiquitination reaction can be stored at –20°C and the SDS PAGE and western blot analyses can be continued whenever possible. For the in vitro ubiquitination assay, the timing of the experiments is crucial. As this setup uses enzymes that will start running the reaction once the ATP is available, it is necessary to prepare all components before starting the reaction.
Author Contribution
Ina Rothenaigner : Formal analysis, investigation, methodology, visualization, writing original draft, writing review and editing; Jara Brenke : Conceptualization, data curation, formal analysis, investigation, methodology, validation, visualization, writing review and editing; Kenji Schorpp : Conceptualization, investigation, methodology, writing review and editing; Kamyar Hadian : Conceptualization, funding acquisition, resources, supervision, visualization, writing original draft, writing review and editing.
Conflict of Interest
The authors have no conflicts of interest.
Open Research
Data Availability Statement
Data sharing not applicable – no new data generated.
Literature Cited
- Baell, J. B., & Holloway, G. A. (2010). New substructure filters for removal of pan assay interference compounds (PAINS) from screening libraries and for their exclusion in bioassays. Journal of Medicinal Chemistry , 53(7), 2719–2740. doi: 10.1021/jm901137j.
- Brenke, J. K., Popowicz, G. M., Schorpp, K., Rothenaigner, I., Roesner, M., Meininger, I., … Hadian, K. (2018). Targeting TRAF6 E3 ligase activity with a small-molecule inhibitor combats autoimmunity. Journal of Biological Chemistry , 293(34), 13191–13203. doi: 10.1074/jbc.RA118.002649.
- Brenke, J. K., Salmina, E. S., Ringelstetter, L., Dornauer, S., Kuzikov, M., Rothenaigner, I., … Hadian, K. (2016). Identification of small-molecule frequent hitters of glutathione S-transferase-glutathione interaction. Journal of Biomolecular Screening , 21(6), 596–607. doi: 10.1177/1087057116639992.
- Buetow, L., & Huang, D. T. (2016). Structural insights into the catalysis and regulation of E3 ubiquitin ligases. Nature Reviews Molecular Cell Biology , 17(10), 626–642. doi: 10.1038/nrm.2016.91.
- Hughes, J. P., Rees, S., Kalindjian, S. B., & Philpott, K. L. (2011). Principles of early drug discovery. British Journal of Pharmacology , 162(6), 1239–1249. doi: 10.1111/j.1476-5381.2010.01127.x.
- Li, W., Bengtson, M. H., Ulbrich, A., Matsuda, A., Reddy, V. A., Orth, A., … Joazeiro, C. A. (2008). Genome-wide and functional annotation of human E3 ubiquitin ligases identifies MULAN, a mitochondrial E3 that regulates the organelle's dynamics and signaling. PLoS One , 3(1), e1487. doi: 10.1371/journal.pone.0001487.
- McInnes, I. B., Buckley, C. D., & Isaacs, J. D. (2016). Cytokines in rheumatoid arthritis - shaping the immunological landscape. Nature Reviews Rheumatology , 12(1), 63–68. doi: 10.1038/nrrheum.2015.171.
- Popovic, D., Vucic, D., & Dikic, I. (2014). Ubiquitination in disease pathogenesis and treatment. Nature Medicine , 20(11), 1242–1253. doi: 10.1038/nm.3739.
- Rothenaigner, I., & Hadian, K. (2021). Brief guide: Experimental strategies for high-quality hit selection from small-molecule screening campaigns. SLAS Discovery , 26(7), 851–854. doi: 10.1177/24725552211008862.
- Schorpp, K., Rothenaigner, I., Salmina, E., Reinshagen, J., Low, T., Brenke, J. K., … Hadian, K. (2014). Identification of small-molecule frequent hitters from alphascreen high-throughput screens. Journal of Biomolecular Screening , 19(5), 715–726. doi: 10.1177/1087057113516861.
- Schulman, B. A., & Harper, J. W. (2009). Ubiquitin-like protein activation by E1 enzymes: The apex for downstream signalling pathways. Nature Reviews Molecular Cell Biology , 10(5), 319–331. doi: 10.1038/nrm2673.
- Scott, D. C., Hammill, J. T., Min, J., Rhee, D. Y., Connelly, M., Sviderskiy, V. O., … Guy, R. K. (2017). Blocking an N-terminal acetylation-dependent protein interaction inhibits an E3 ligase. Nature Chemical Biology , 13(8), 850–857. doi: 10.1038/nchembio.2386.
- Walsh, M. C., Lee, J., & Choi, Y. (2015). Tumor necrosis factor receptor- associated factor 6 (TRAF6) regulation of development, function, and homeostasis of the immune system. Immunological Reviews , 266(1), 72–92. doi: 10.1111/imr.12302.
- Weber, E., Rothenaigner, I., Brandner, S., Hadian, K., & Schorpp, K. (2017). A high-throughput screening strategy for development of RNF8-Ubc13 protein-protein interaction inhibitors. SLAS Discovery , 22(3), 316–323. doi: 10.1177/1087057116681408.
- Ye, Y., & Rape, M. (2009). Building ubiquitin chains: E2 enzymes at work. Nature Reviews Molecular Cell Biology , 10(11), 755–764. doi: 10.1038/nrm2780.
- Yin, Q., Lin, S. C., Lamothe, B., Lu, M., Lo, Y. C., Hura, G., … Wu, H. (2009). E2 interaction and dimerization in the crystal structure of TRAF6. Nature Structural & Molecular Biology, 16(6), 658–666. doi: 10.1038/nsmb.1605.
Key References
- Brenke et al. (2018). See above.
This paper was the first that identified a TRAF6-Ubc13 PPI inhibitor with a screening campaign.