Isolation, In Vitro Differentiation, and Culture of Murine Megakaryocytes From Fetal Liver and Adult Bone Marrow
Kristina Mott, Kristina Mott, Harald Schulze, Harald Schulze
Abstract
Megakaryocytes (MKs) are the source of circulating platelets and are readily recognized by their large size and distinctive morphology. Their poor representation in hematopoietic tissues often requires enrichment or considerable ex vivo expansion to generate cells for biochemical and cell biological studies. These experimental protocols describe the enrichment of primary MKs directly from the murine bone marrow as well as in vitro differentiation of fetal liver– or bone marrow–derived hematopoietic stem cells into MKs. Although in vitro–differentiated MKs are not synchronized in their maturation, they can be enriched over an albumin density gradient, and one-third to one-half of recovered cells will typically elaborate proplatelets. Support protocols describe methods for preparing fetal liver cells, identifying mature rodent MKs by staining for flow cytometry analysis, and immunofluorescence staining of fixed MKs for confocal laser scanning microscopy. © 2023 The Authors. Current Protocols published by Wiley Periodicals LLC.
Basic Protocol 1 : Isolation of mature bone marrow megakaryocytes by magnetic-activated cell sorting
Basic Protocol 2 : Preparation of a megakaryocyte suspension culture from murine fetal livers or lineage-depleted adult bone marrow
Support Protocol 1 : Preparation of a single-cell suspension from murine fetal livers for megakaryocyte culture
Support Protocol 2 : Megakaryocyte culture from lineage-depleted murine bone marrow
Support Protocol 3 : Quality control of megakaryocyte culture with flow cytometry
Support Protocol 4 : Immunofluorescence staining of megakaryocytes for detection with confocal laser scanning microscopy
INTRODUCTION
Megakaryocytes (MKs) are the largest cells of the hematopoietic system and the direct precursors of blood platelets. During maturation MK progenitors duplicate their DNA content without subsequent cytokinesis resulting in polyploid cells, a process referred to as endomitosis. Concomitantly with polyploidization, early MKs increase their inner demarcation membrane system (DMS) and their granule content, which is needed for proper platelet production. Mature MKs have a diameter of 20 to 60 µm and only make up 0.05% of all nucleated bone marrow (BM) cells. This paucity of MKs in adult BM often requires in vitro differentiation and expansion of MK progenitors under the administration of thrombopoietin (TPO) in order to obtain enough cells for biochemical or biological studies or direct isolation of primary MKs, which may impose obstacles due to their large size.
During the last years, new methods have evolved that facilitate the identification of the cells of the MK lineage, mostly by flow cytometry or by immunofluorescence microscopy. Other methods like acetylcholinesterase staining have lost their significance and are not used anymore. We have thus updated this article to reflect state-of-the-art protocols for MK isolation and purification. Basic Protocol 1 describes a method to enrich mature BM MKs by magnetic-activated cell sorting (MACS) for virtually all methods that benefit from a pure cell population (e.g., immunoblot, quantitative PCR). Basic Protocol 2 describes an approach for the cultivation of murine fetal liver cells and lineage-depleted BM in the presence of TPO. This simple method directs differentiation of a majority of the cells toward the MK lineage by using TPO as the exogenous growth factor in the presence of fetal calf serum. One intrinsic drawback of the method is that cells are not synchronized for different steps along the MK differentiation pathway. Therefore, a simple albumin step gradient is included to substantially enrich mature in vitro–differentiated MKs. Support protocols are included for the preparation of single-cell suspensions from fetal mouse livers (Support Protocol 1), depletion of lineage-positive cells from adult murine BM (Support Protocol 2), flow cytometry analysis of MKs (Support Protocol 3), and immunofluorescence staining of fixed cells (Support Protocol 4).
NOTE : All protocols using animals must first be reviewed and approved by an Institutional Animal Care and Use Committee (IACUC) and must follow officially approved procedures for the care and use of laboratory animals.
Basic Protocol 1: ISOLATION OF MATURE BONE MARROW MEGAKARYOCYTES BY MAGNETIC-ACTIVATED CELL SORTING
This protocol describes the isolation of murine BM MKs by MACS with CD61 MicroBeads from Miltenyi Biotec. MKs are rare BM cells that have to be enriched and purified for mRNA or protein studies. There are several hematopoietic compartments that can be used to isolate stem and progenitor cells (hematopoietic stem cells [HSCs]) for in vitro MK differentiation. However, simple suspension culture does not mimic the complex in vivo MK niche leading to differences in, for example, polyploidy or DMS characteristics. Studies that aim to decipher in vivo conditions of MK biology thus require their direct isolation from the BM or spleen. MACS represents a method to harvest cells based on minimal labeling of cells with superparamagnetic beads combined with a strong magnetic field in MACS columns. The MicroBeads used in this protocol are biologically inert and therefore do not interfere with downstream analyses, such as flow cytometry or even in vivo use for HSC transplantation.
Direct positive selection is described in this protocol of MKs from murine BM with CD61 MicroBeads and Large Cell Separation Columns from Miltenyi Biotec. Alternatively, cells can be labeled and isolated by using a sandwich approach with a primary antibody (either unconjugated or fluorophore/biotin conjugated) combined with the respective MicroBeads. The choice of which type of separation column should be used depends on the isolation method (positive selection or depletion), the target cell size, and cell number. Isolation of MKs with Large Cell Columns provides the best yield and purity in our hands, but isolation can also be done with LS columns (Miltenyi Biotec). This protocol can be adapted for equivalent magnetic labeling reagents from other manufacturers.
Materials
-
MACS buffer (see recipe)
-
Adult mice
-
Dulbecco's phosphate-buffered saline (DPBS; e.g., Sigma-Aldrich)
-
Trypan blue (e.g., Thermo Fisher Scientific)
-
Surgical equipment for harvesting bones including forceps and scissors
-
0.5- and 1.5-ml reaction tube
-
18-G cannula
-
Microcentrifuge
-
70-µm cell strainer (e.g., Pluri Select)
-
15- and 50-ml conical tubes
-
Neubauer hemocytometer
-
Centrifuge
-
CD61 mouse and rat MicroBeads (e.g., Miltenyi Biotec)
-
Large Cell Separation Column (e.g., Miltenyi Biotec)
-
Magnetic separator (e.g., MiniMACS Separator, OctoMACS Separator; Miltenyi Biotec)
-
23-G needle
-
Additional reagents and equipment for mouse euthanasia (see Current Protocols article: Donovan & Brown, 2006)
Dissect mice and isolate bone marrow
1.Prepare MACS buffer as described in Reagents and Solutions.
2.Euthanize adult mice, and dissect femurs and tibiae. Clear bones from adherent muscle tissue, and cut open femurs and tibiae at one epiphysis. Punch a hole into the bottom of a 0.5-ml reaction tube using an 18-G cannula, and place bones with the open end down in the tube. Pipette 100 µl DPBS into a 1.5-ml reaction tube, and put 0.5-ml reaction tube into it.
3.Centrifuge 1 min at 2600 × g , room temperature, to spin out BM (Heib et al., 2021), which should be visible as a red pellet. Resuspend BM pellet in 1 ml MACS buffer.
4.Place 70-µm cell strainer on a 50-ml conical tube. Equilibrate strainer with 1 ml MACS buffer. Pass BM suspension through the cell strainer, and wash two times with 1 ml MACS buffer.
Separate MKs by MACS
5.Determine cell number with a Neubauer hemocytometer and trypan blue.
6.Centrifuge cell suspension 5 min at 200 × g , room temperature. Discard supernatant and resuspend BM pellet in 80 µl MACS buffer per 1 × 107 total cells.
7.Add 20 µl MicroBeads per 1 × 107 cells, and incubate 15 min in the dark at 4°C.
8.Adjust total volume to 500 µl per 1 × 107 cells with MACS buffer.
9.Place Miltenyi Large Cell Separation Column in an appropriate MACS separator, and attach 23-G needle as a flow resistor to the column. Place 15-ml conical tube under the column to collect the flow through. Equilibrate column with 500 µl MACS buffer, and discard flow through.
10.Load column with up to 2 × 108 total cells, and let cell suspension run through. Collect unlabeled cells as flow through.
11.Wash column three times with 500 µl MACS buffer, and collect unlabeled cells as flow through.
12.Remove column from magnetic separator and flow resistor. Place column on a new 15-ml conical tube.
13.Pipette 1 ml MACS buffer on the column. Immediately use plunger supplied with the columns to flush out labeled cells.
Basic Protocol 2: PREPARATION OF A MEGAKARYOCYTE SUSPENSION CULTURE FROM MURINE FETAL LIVERS OR LINEAGE-DEPLETED ADULT BONE MARROW
This protocol describes the preparation of an unsynchronized mouse MK suspension culture derived from fetal liver cells. The same protocol can be adapted for BM cultures (see Support Protocol 2).
Both fetal liver and BM-derived MKs can be used for RNA or protein analyses and are suitable for typical MK assays, such as proplatelet formation (Fig. 1) or spreading. However, there are a few differences between both types of MK culture that need to be considered when choosing which one to use.
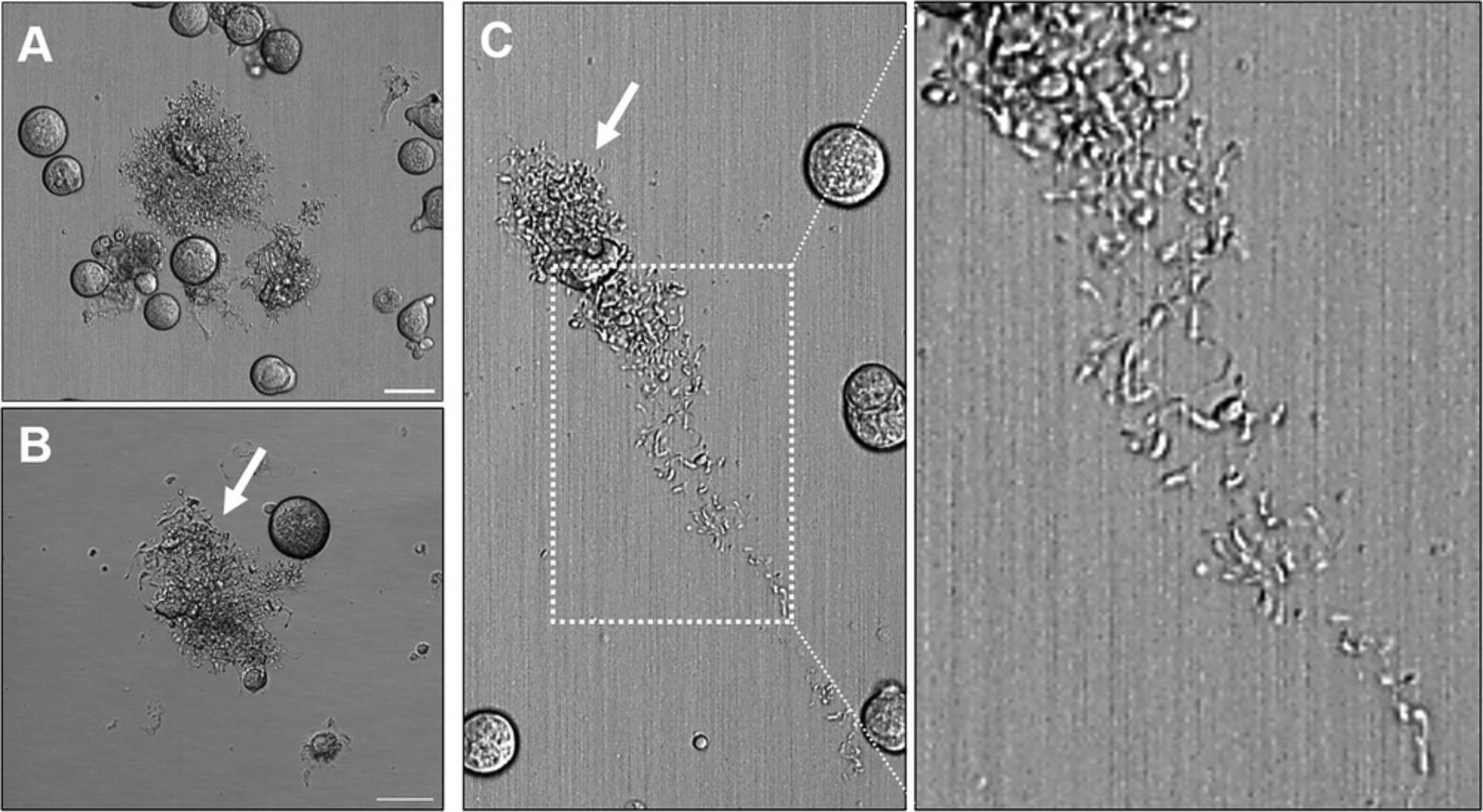
Harvesting fetal livers involves a substantial breeding effort that must be carefully timed. Fetal liver cells can therefore not be readily analyzed at any given time. In addition, these cells represent fetal hematopoiesis, which differs from adult hematopoiesis in several features. Fetal liver–derived MKs, for example, show a reduced degree of polyploidy, have an increased proliferative potential, and release more proplatelets compared with BM-derived MKs under identical conditions. One advantage is the generation of littermate controls, which are not influenced by environmental factors after birth. BM-derived MKs are not dependent on breeding and can therefore be isolated at any desired time, while representing adult hematopoietic cells. However, BM-derived MKs have a much lower capacity to form proplatelets compared with fetal liver–derived MKs and furthermore depend on the addition of hirudin for proplatelet formation.
Materials
-
Single-cell suspension of mouse fetal liver cells (see Support Protocol 1) or lineage-depleted BM (see Support Protocol 2)
-
Dulbecco's modified Eagle medium (DMEM) containing 10% (v/v) fetal bovine serum (FBS), with and without 50 U/ml penicillin and 50 μg/ml streptomycin
-
Recombinant TPO (e.g., R&D Systems)
-
1.5% and 3% (w/v) bovine serum albumin (BSA; e.g., Sigma-Aldrich, Serological) in DPBS, sterile and prewarmed to 37°C
-
10-cm tissue culture dishes or 75-cm2 tissue culture flasks, sterile
-
37°C, 5% CO2 humidified incubator
-
15-ml conical tubes, sterile
-
Inverted microscope
-
Centrifuge
-
Additional reagents and equipment for flow cytometry analysis (see Support Protocol 3) or immunofluorescence staining (see Support Protocol 4)
1.Transfer a single-cell suspension of mouse fetal liver cells or lineage-depleted BM in DMEM supplemented with 10% (v/v) FBS, antibiotics, and 50 ng/ml recombinant TPO at a density of 1–5 × 107 cells/ml to sterile 10-cm tissue culture dishes (10 ml) or 75-cm2 flasks (20 ml). Incubate 2 to 5 days at 37°C in a humidified 5% CO2 incubator.
2.Prepare a two-step density gradient in a 15-ml conical tube: Pipet 1.5 ml prewarmed sterile 3% (w/v) BSA in DPBS first, and then gently overlay 1.5 ml prewarmed sterile 1.5% (w/v) BSA in DPBS.
3.Aspirate cell suspension from culture days 2 to 5 into 15-ml conical tubes using a sterile 5- or 10-ml pipet. Rinse the bottom of each dish with 5 ml fresh DMEM supplemented with 10% (v/v) FBS to include cells that may adhere weakly to the plastic surface. Control the efficiency of this step by monitoring the dish under an inverted microscope.
4.Centrifuge cell suspension 5 min at 200 × g , room temperature, and resuspend cell pellet in 1 ml DMEM supplemented with 10% (v/v) FBS. Overlay this solution on the 1.5% to 3% BSA step gradient with minimal agitation. Incubate 30 to 45 min at room temperature.
5.Remove upper phase consisting of all but the lowest (sedimented) 0.5 to 0.8 ml.
6.Optional : Wash cells from the upper phase in DMEM once, and culture for an additional 3 to 5 days in the presence of TPO to generate a second wave of MKs.
7.Resuspend harvested cells in 10 ml DMEM, and centrifuge again 5 min at 200 × g , room temperature.
8.Analyze cells in the lower phase for MK-specific surface receptor proteins (see Support Protocol 3) or by immunofluorescence staining (see Support Protocol 4).
Support Protocol 1: PREPARATION OF A SINGLE-CELL SUSPENSION FROM MURINE FETAL LIVERS FOR MEGAKARYOCYTE CULTURE
There are several waves of hematopoiesis in the mouse fetus, initially taking place in the yolk sac. During further development, the aorta-gonad mesonephros region is the next site of hematopoietic activity. Beginning from 10 dpc, HSCs colonize and proliferate in the fetal liver, which is therefore a rich source for HSCs during the time frame. This protocol describes the isolation of fetal liver tissue and the generation of MKs from fetal liver–derived HSCs. In order to achieve a maximum MK yield, livers from 14.5 dpc should be used over 12.5 dpc (lower MK yield) or 15.5 dpc (increased red blood cell contamination).
Dissection of pregnant mice and removal of fetal livers do not need to be performed under sterile conditions. However, we recommend subsequently moving to a sterile laminar flow hood for preparation of single-cell suspensions and culture of isolated cells in the presence of antibiotics.
Materials
-
Pregnant mice, 13.5 to 15.5 dpc
-
Hanks’ balanced salt solution (HBSS; e.g., Sigma-Aldrich) without calcium chloride, magnesium chloride, or magnesium sulfate, sterile
-
DMEM containing 10% (v/v) FBS, 50 U/ml penicillin, and 50 μg/ml streptomycin
-
Red blood cell lysis buffer (see recipe)
-
Recombinant TPO (e.g., R&D Systems)
-
Surgical instruments including sharp scissors and no. 5 fine-tip forceps
-
10-cm petri dishes, sterile
-
18-, 21-, and 23-G needles, sterile
-
10-ml syringes, sterile
-
Inverted microscope
-
15- or 50-ml conical tube, sterile
-
Centrifuge
-
Additional reagents and equipment for mouse euthanasia (see Current Protocols article: Donovan & Brown, 2006) and red blood cell lysis (see Current Protocols article: Kruisbeek, 2000; for 15.5 dpc fetal livers only)
Dissect pregnant mice and collect fetuses
1.Euthanize pregnant mice between 13.5 and 15.5 dpc. Use sharp scissors to make a small vertical mid-abdominal incision, and then gently tear the skin to expose the peritoneal and pelvic cavities.
2.Place uterus in a 10-cm petri dish containing sterile HBSS, and use sharp scissors to dissect it open on the side opposite the linear array of placental implantations.
3.Sever umbilical vessels with sharp scissors to isolate individual fetuses. Allow rapid, partial exsanguination of the fetuses, and then move them to a fresh petri dish containing sterile HBSS.
Remove fetal livers
4.Cut open fetal skin from the left side using a gentle tearing motion with two pairs of sterile fine-tip forceps. Using the same fine-tip forceps, isolate liver from any adhering connective tissue or gut segments, and transfer to a new dish containing DMEM supplemented with 10% (v/v) FBS, 50 U/ml penicillin, and 50 μg/ml streptomycin. Collect up to ten livers from 13.5 dpc fetuses or six livers from 14.5 or 15.5 dpc fetuses in 10 ml medium per 10-cm petri dish.
Prepare single-cell suspension
5.Attach a sterile 18-G needle to a sterile 10-ml syringe, and alternately aspirate and expel each tissue eight to ten times until it fragments into small pieces.
6.Replace 18-G needle with a sterile 21-G needle, and aspirate tissue another eight to ten times.
7.Inspect suspension under an inverted microscope to confirm that most of the tissue has been broken up into single cells. If there are still many cell clumps (i.e., tissue fragments), aspirate again using a 23-G needle.
8.Pipet cell suspension into a sterile 15- or 50-ml conical tube, and allow any contaminating connective tissue or residual large cell clumps to sediment for 3 to 5 min. Transfer suspension into a fresh tube, and centrifuge 5 min at 200 × g , room temperature or 4°C.
9.For 15.5 dpc fetal livers, perform lysis of red blood cells. To do so, discard supernatant and resuspend cells in 1× red blood cell lysis buffer. Incubate suspension 10 min at room temperature, and centrifuge suspension 5 min at 200 × g , room temperature.
10.Discard supernatant and resuspend cells in 10 ml DMEM supplemented with 10% (v/v) FBS, appropriate antibiotics, and 50 ng/ml recombinant TPO. Continue immediately with Basic Protocol 2.
Support Protocol 2: MEGAKARYOCYTE CULTURE FROM LINEAGE-DEPLETED MURINE BONE MARROW
MACS cell separation can be performed by positive selection of the target cells based on immunolabeling of a surface antigen or by negative selection of untouched cells through the depletion of unwanted cells. This protocol describes the generation of a BM stem and progenitor cell culture by MACS depletion of lineage-positive cells from a BM single-cell suspension by using a “lineage cocktail” for magnetic labeling.
This protocol describes the isolation of lineage-marker negative cells with the Lineage Cell Depletion Kit for murine BM and LS columns from Miltenyi Biotec. This protocol can be adapted for equivalent magnetic labeling reagents from other manufacturers.
Materials
-
MACS buffer (see recipe)
-
Adult mice
-
DPBS (e.g., Sigma-Aldrich)
-
Trypan blue (e.g., Thermo Fisher Scientific)
-
1 ml biotin-antibody cocktail (i.e., lineage cocktail of biotin-conjugated monoclonal antibodies directed against lineage-specific cell surface markers):
- CD5 (T cells)
- CD45R (B220; B cells)
- CD11b (macrophages)
- Gr-1 (Ly-6G/C; granulocytes)
- Ly6B.2 (neutrophils/monocytes)
- Ter-119 (reticulocytes and erythrocytes)
-
Anti-biotin MicroBeads (clone Bio3-18E7.2, mouse IgG1)
-
DMEM containing 10% (v/v) FBS, 50 U/ml penicillin, 50 μg/ml streptomycin, and 50 ng/ml TPO
-
Surgical equipment for harvesting bones including forceps and scissors
-
0.5- and 1.5-ml reaction tube
-
18-G cannula
-
Microcentrifuge
-
70-µm cell strainer (e.g., Pluri Select)
-
15- and 50-ml conical tubes
-
Neubauer hemocytometer
-
LS columns (e.g., Miltenyi Biotec)
-
Magnetic separator (e.g., MidiMACS separator; Miltenyi Biotec)
Dissect mice and isolate bone marrow
1.Prepare MACS buffer as described in Reagents and Solutions.
2.Euthanize adult mice, and dissect femurs and tibiae. Clear bones from adherent muscle tissue, and cut open femurs and tibiae at one epiphysis. Punch a hole into the bottom of a 0.5-ml reaction tube using an 18-G cannula, and place bones with the open end down in the tube. Pipette 100 µl DPBS into a 1.5-ml reaction tube, and put 0.5-ml reaction tube into it.
3.Centrifuge 1 min at 2600 × g , room temperature, to spin out the BM, which should be visible as a red pellet. Resuspend BM pellet in 1 ml MACS buffer.
4.Place 70-µm cell strainer on a 50-ml conical tube. Equilibrate strainer with 1 ml MACS buffer. Pass BM suspension through the cell strainer, and wash two times with 1 ml MACS buffer.
Separate lineage-positive cells by MACS
5.Determine cell number with a Neubauer hemocytometer and trypan blue.
6.Centrifuge 5 min at 200 × g , room temperature, and discard supernatant.
7.Resuspend cell pellet in 80 µl MACS buffer per 2 × 107 cells.
8.Add 20 µl direct lineage depletion antibody cocktail per 2 × 107 cells.
9.Incubate cells 10 min at 4°C to 8°C.
10.Place Miltenyi LS columns in a suitable MACS separator, and place a 15-ml conical tube underneath. Equilibrate column with 3 ml MACS buffer, and discard flow through.
11.Apply cell suspension to the column, and let cells pass through. Collect lineage-negative cell fraction in a 15-ml conical tube.
12.Wash column three times with 3 ml MACS buffer, and collect flow through in the same tube as in step 11.
13.Centrifuge flow through 5 min at 200 × g , room temperature, and resuspend cells in DMEM with 10% (v/v) FBS, 1% penicillin/streptomycin, and 50 ng/ml TPO. Immediately proceed with Basic Protocol 2.
Support Protocol 3: QUALITY CONTROL OF MEGAKARYOCYTE CULTURE WITH FLOW CYTOMETRY
This protocol describes the assessment of MK culture purity in response to Basic Protocol 1 or comparable purification methods by using flow cytometry.
Cell number and purity are readily controlled by flow cytometry, which requires overall low number of cells. Mature MKs are readily recognized by their large size and high granule content, which can be detected by their scatter properties. MKs are high for both forward scatter and side scatter. The detection is typically set to linear settings, but depending on the type of flow cytometer used, a logarithmic setting might be better. MK progenitors are smaller and cannot be exclusively separated by light scatter properties. At least one lineage-specific marker should be added, which is typically fluorophore conjugated (Fig. 2). We recommend antibodies directed against the fibrinogen receptor complex GPIIb/IIIa (CD41a) or the von Willebrand factor receptor complex GPIb/IX/V (CD42a-d). Of note, antibodies recognizing GPIIIa (CD61) alone are not recommended, as some leukocyte progenitor cells express CD61, in part with CD51.Flow cytometers should thus have at least two lasers, comprising the argon laser at 488 nm and one or more additional lasers (i.e., 640 nm). There might be specific consideration for the selection of new generation (tandem) fluorophores: 405-nm laser or 561-nm laser in case of protein-based fluorophores (e.g., dsTomato, Cherry). Compensation of fluorescence signals should be performed according to the manufacturer's instructions.
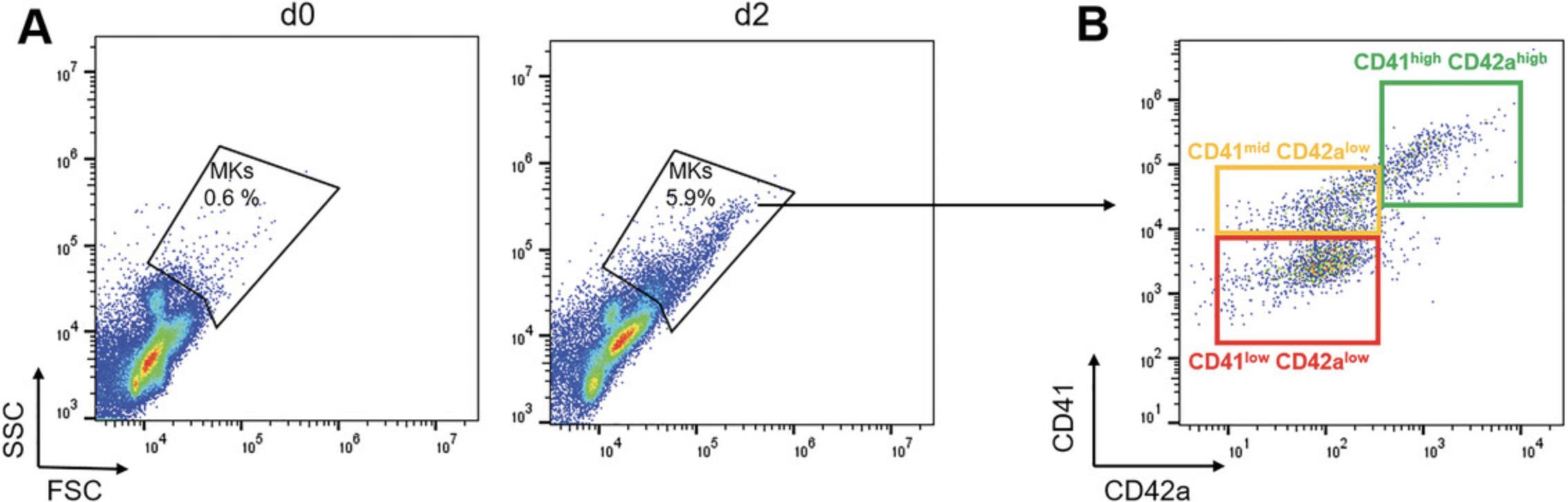
Materials
-
MK sample (see Basic Protocol 1)
-
CATCH buffer (see recipe)
-
FcγR block (clone 2.5G2; e.g., Cell Signaling)
-
Anti-CD41/CD61 antibody, Alexa Fluor 647 conjugated (clone Leo.H4; e.g., Emfret)
-
Anti-CD42a antibody, Alexa Fluor 405 conjugated (clone Xia.B4; e.g., Emfret)
-
5-ml round-bottom FACS tubes (e.g., Corning)
-
Flow cytometer
1.Resuspend MK sample in 250 µl CATCH buffer in 5-ml round-bottom FACS tubes. Add 5 µg FcγR block, and incubate 15 min at room temperature.
2.Add 1 µg anti-CD41/61 fluorophore-conjugated antibody and anti-CD42a fluorophore-conjugated antibody. Incubate 15 min at room temperature in the dark.
3.Centrifuge cells 5 min at 300 × g , room temperature. Discard supernatant and resuspend pellet in 500 µl CATCH buffer.
4.Proceed with flow cytometry.
Support Protocol 4: IMMUNOFLUORESCENCE STAINING OF MEGAKARYOCYTES FOR DETECTION WITH CONFOCAL LASER SCANNING MICROSCOPY
This protocol describes immunofluorescence staining of cultured or directly isolated MKs for confocal laser scanning microscopy. The identification of MKs and MK progenitors has been made by immunohistochemical and immunofluorescence staining of cells or tissue sections. MKs of rodents express acetylcholinesterase, but this staining protocol is time and labor intensive and has nowadays been replaced with antibody-based markers. Surface markers are mostly used (Fig. 3), but other lineage-specific markers (like β1-tubulin) can also be used.
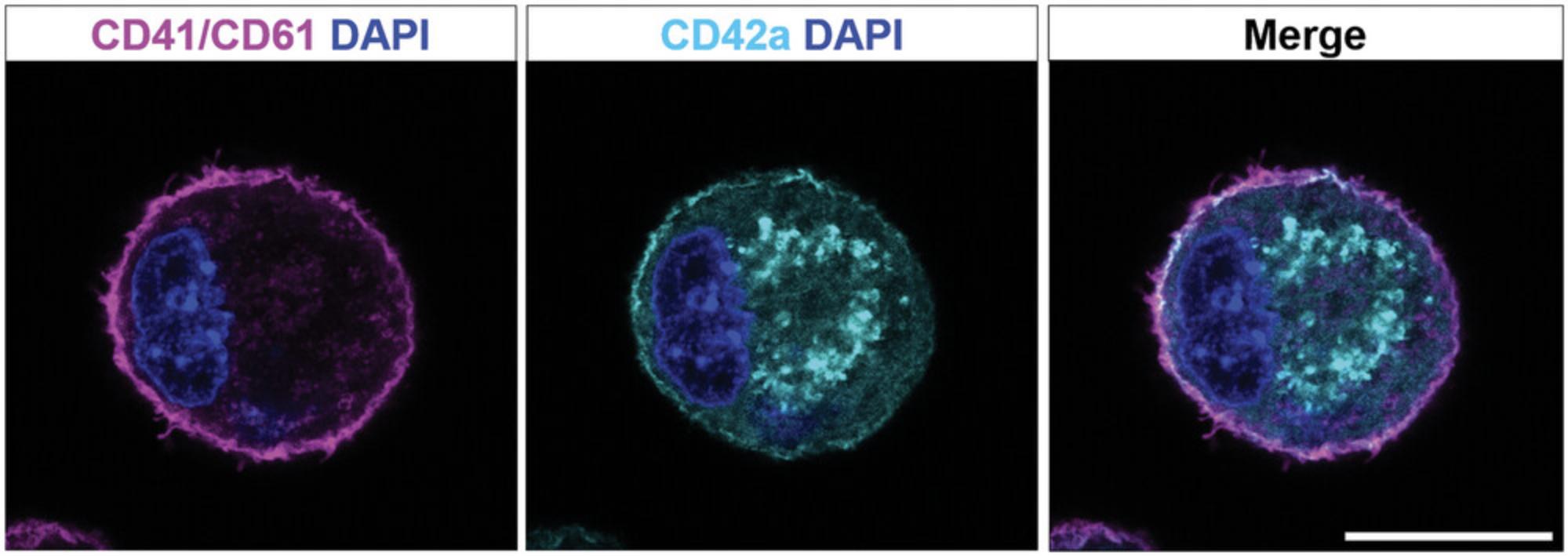
Materials
-
Paraformaldehyde
-
Triton X-100
-
PHEM buffer (see recipe)
-
MKs (see Basic Protocols 1 and 2)
-
DPBS (e.g., Sigma-Aldrich)
-
Anti-CD42a antibody, Alexa Fluor 647 conjugated (clone Xia.B4; e.g., Emfret)
-
Anti-CD41/CD61 antibody, Alexa Fluor 546 conjugated (clone Leo.H4; e.g., Emfret)
-
Phalloidin-Alexa 647 (e.g., Invitrogen)
-
Fluoroshield containing DAPI (e.g., Sigma-Aldrich)
-
Centrifuge
-
Chambered cover slips (e.g., Ibidi)
-
Inverted confocal laser scanning fluorescence microscope (e.g., Leica TCS SP8)
1.Prepare 2% (w/v) paraformaldehyde and 0.1% (v/v) Triton X-100 in PHEM buffer.
2.Resuspend MKs in 500 µl PHEM buffer containing paraformaldehyde and Triton X-100.
3.Fix and permeabilize cells for 20 min at room temperature.
4.Centrifuge samples 5 min at 300 × g , room temperature.
5.Discard supernatant and resuspend cells in 500 µl DPBS.
6.Add 1 µg anti-CD42a and anti-CD41/61 fluorophore-conjugated antibodies to the cells. Add 0.75 U/ml Alexa Fluor 647–conjugated phalloidin, and stain sample for 1 hr at room temperature in the dark.
7.Centrifuge samples 5 min at 300 × g , room temperature, and discard supernatant.
8.Resuspend cells in 100 µl DPBS, and add one drop of Fluoroshield with DAPI to counterstain nuclei.
9.Transfer samples to a chambered cover slip, and image cells with an inverted confocal laser scanning microscope.
REAGENTS AND SOLUTIONS
CATCH buffer
- DPBS (e.g., Sigma-Aldrich, cat. no. D8537)
- 12.5 mM HEPES (e.g., Sigma-Aldrich, cat. no. H3357)
- 1.5 mM EDTA (e.g., AppliChem, cat. no. A4892)
- 1.75% (w/v) BSA fraction V, cell culture grade (e.g., Sigma-Aldrich, cat no. A9418)
- 2.5% (v/v) FBS (e.g., Sigma-Aldrich, cat. no. F7524)
- Store at 4°C for up to 1 year
Freshly add BSA and FBS before use.
MACS buffer
- DPBS (e.g., Sigma-Aldrich, cat. no. D8537)
- 0.5% (w/v) BSA fraction V, cell culture grade (e.g., Sigma-Aldrich, cat no. A9418)
- 2 mM EDTA (e.g., AppliChem, cat. no. A4892)
- Store at 4°C for up to 4 weeks
BSA and EDTA should be freshly added before use.
Keep buffer cold for cell separation.
PHEM buffer
- 60 mM PIPES (e.g., Sigma-Aldrich, cat. no. P6757)
- 25 mM HEPES (e.g., Sigma-Aldrich, cat. no. H3357)
- 10 mM EGTA (e.g., Sigma-Aldrich, cat. no. E3889)
- 2 mM MgCl2
- Adjust pH to 7.0
- 2% (w/v) paraformaldehyde (e.g., Sigma-Aldrich, cat. no. 158127)
- 0.1% (v/v) Triton X-100 (e.g., AppliChem, cat. no. A9779)
- Store at 4°C for up to 4 weeks
Freshly add 2% paraformaldehyde and 0.1% Triton X-100 before use.
Red blood cell lysis buffer, 10×
- 1.5 M NH4Cl
- 1 mM EDTA (e.g., AppliChem, cat. no. A4892)
- 0.14 M NaHCO3
- Adjust pH to 7.3
- Filter sterilize
- Store at 4°C for up to 1 year
COMMENTARY
Background Information
MKs are rare hematopoietic cells that are difficult to isolate and culture due to their (1) large size, (2) frailty, and (3) paucity in the BM niche. Especially low MK numbers pose an obstacle for most experimental analyses, which is usually circumvented by isolation of HSCs and progenitor cells for ex vivo expansion of MKs. MK culture medium can be supplemented with several growth factors, such as stem cell factor or interleukin (IL)-3, especially for human MKs. However, TPO is the preferred cytokine for in vitro differentiation of MKs (Dolzhanskiy et al., 1997; Zeigler et al., 1994).
Since recombinant TPO has become available in 1994, it became possible to extensively study MK biology in vitro. For this, usually the fetal liver was used as the primary source for stem and progenitor cells (Semeniak et al., 2016; Vijey et al., 2018; also see Current Protocols article: Shivdasani & Schulze, 2005). Nonetheless, efforts have been made to improve protocols for the expansion of BM-derived MKs, which yield enough cells for various biochemical analyses (Becker et al., 2022; Bender et al., 2014; Englert et al., 2022; Heib et al., 2021). As an update to the 2016 version of this method (see Current Protocols article: Schulze, 2016), we present a reliable approach for the negative selection of HSCs and progenitor cells from the adult murine BM by depletion of lineage marker–positive cells and subsequent MK expansion with TPO (see Support Protocol 2). Additional supplementation of the culture medium with hirudin enhances proplatelet formation of BM-derived MKs and thus makes them a complementary alternative to fetal liver–derived MKs. At this point, we note that there are also other protocols to cultivate MKs, such as BM explant models (Eckly et al., 2012).
Maturation and enrichment of MKs can be assessed by flow cytometry analysis. During differentiation, several MK-specific surface antigens become highly expressed. Although there is so far no specific set of markers that could be assigned to a defined stage of megakaryopoiesis, immunophenotyping of murine MK progenitors during differentiation has demonstrated that immature MKs are CD41 positive, whereas expression of CD42a or CD42b is higher in more mature MKs (Debili et al., 2004; Matsumura-Takeda et al., 2007). Thus, staining of MK-specific receptors, such as CD41/61 and CD42a, allows for studying MK maturation and quantifying the purity of an MK culture. Besides flow cytometry, improved fluorescence microscopy techniques and advanced multicolor immunofluorescence staining approaches have gradually replaced classical histologic staining methods such as May-Grünwald-Giemsa. These allow for resolving cellular structures in two or three dimensions. A corresponding protocol for immunofluorescence staining of MKs is described in Support Protocol 4. Mature MKs typically have a distinct DMS, which can be visualized by staining CD42a and large polylobulated nuclei.
Critical Parameters and Troubleshooting
Basic Protocol 2 describes a practical method for isolating murine MKs in sufficient numbers for single-cell or biochemical analysis. If MKs are required on a regular basis, a back-up supply of pregnant dams is recommended to avoid delays precipitated by the common occurrence of nonpregnant females. In general, 13.5 or 14.5 dpc fetuses consistently yield adequate cell numbers, with the younger tissue providing fewer cells but greater MK enrichment. Older (15.5 dpc) fetuses may also be used, although in this case a red blood cell lysis procedure is highly recommended to reduce the number of mature erythrocytes. The quality of the culture improves with better fetal liver isolation, and it is recommended that novices, in particular, perform the dissection of 13.5 or 14.5 dpc fetal livers under a dissecting microscope to avoid contaminating the cultures with connective or gut tissue.
Cell density is an important parameter for optimal MK differentiation, which leads to elaboration of proplatelets. Cells plated too sparsely or too densely will differentiate into MKs, as judged by morphology, but functional proplatelet formation may be absent or markedly reduced. Although efficient proplatelet formation can be detected within a wide range of cell densities, it is recommended to use a consistent number of mouse fetal liver cells per culture volume, as described in Basic Protocol 2. If there are not enough cells available, the cell culture volumes and plate sizes can be downsized to 6-well plates (or even smaller diameter, up to 96-well plates) to ensure sufficient cell density for proplatelet formation.
TPO is the only supplementary growth factor in these protocols, although FBS is present at 10%. Therefore, over the culture period there is initial attrition of other lineages and preferential expansion of committed MK progenitors. TPO also functions as an early stem cell factor (Kaushansky, 1995; Sitnicka et al., 1996), however, which accounts for the persistence of all other blood lineages, especially over extended periods. Total MK output may be increased by supplementing the culture medium with recombinant IL-3. However, the higher cell yield is achieved at the expense of lineage enrichment, and the inevitable losses associated with crude gradient separation methods (see Basic Protocol 2) lead to comparable cell samples. Terminal MK differentiation and platelet release seem not to depend on TPO, and there is some suggestion that TPO inhibits or diminishes this process (Choi et al., 1996). Although there is no advantage to withdrawing this cytokine mid-culture to observe optimal proplatelet formation, it is important to titrate the initial amount, which is typically included as a purified recombinant protein. The recommended starting concentration is 50 ng/ml. When using a new lot, the authors recommend plating cells in sterile 12- or 24-well tissue culture plates at different (two-fold) cell densities and TPO concentrations. Suboptimal plating densities or excessive TPO can each influence the degree of MK differentiation that is achieved in vitro (Choi et al., 1996).
The MK cultures described in these protocols are not synchronized, which makes it difficult to isolate pure populations of MKs at distinct stages of differentiation. However, it is at least obvious by morphology that the number of advanced MKs increases over time and peaks between culture days 4 and 5 for fetal livers and BM cultures. Separation of cells over a BSA gradient (see Basic Protocol 2) allows one to obtain two mixed-cell populations with different densities that reflect less- and more-mature MKs. A more homogeneous cell population is obtained when gradient purification is applied on fetal liver culture day 2 and when all cells in the sedimented fraction are cultured for an additional 2 to 3 days in the presence of TPO. The relative depletion of immature MK progenitors and non-MK cells (e.g., neutrophils) in such a step facilitates recovery of more-mature MKs, although the range of maturation remains broad.
Cells that fail to sediment in BSA after 3 to 6 days of culture can be recultured for a second wave of MK differentiation. Wash the cells once, resuspend in medium supplemented with TPO, and resume their culture for an additional 2 to 4 days. This process is more efficient if recultivation occurs in the same 10-cm dishes or 75-cm2 flasks in which the cells were expanded previously, presumably because of support from adherent mesenchymal cells or soluble factors.
Primary MKs can be isolated or enriched using antibodies and magnetic beads, either by positive or negative selection, respectively (see Basic Protocol 1 and Support Protocol 2). Intriguingly, these MKs often display a “ghostly” appearance (a term adapted from so-called erythrocyte ghosts) with readily visible nuclei and textured cytoplasm in bright field microscopy (Osman et al., 2018). However, this does not affect cell quality for downstream analyses.
Understanding Results
MK cultures thrive best if they are maintained at 37°C, and manipulations should be minimized to avoid disrupting the temperature-sensitive, microtubule-based elaboration of proplatelets. The status of the culture can be monitored by inverted phase-contrast microscopy: By day 2, 20% to 30% of the cells should be visibly larger than the starting population of nucleated cells, and by day 4, impressively large cells should dominate the culture. BM produces numbers that are about one order of magnitude lower. For Basic Protocol 2, 10 to 15 fetuses at 13.5 or 14.5 dpc readily yield 1–5 × 108 cells/10 ml culture medium; 10% to 20% of these should show MK differentiation.
If a BSA step gradient is applied between culture days 2 and 4, 20% to 30% of sedimenting cells may go on to demonstrate terminal MK differentiation, as judged by the appearance of proplatelets, which are recognized readily and may be counted under phase-contrast microscopy. They are tortuous, beaded projections (from two to more than ten per MK) that extend >50 μm and occasionally over the full diameter of a high-power microscopic field. Once these procedures are established in a laboratory, they are stable to small changes. BM MKs cultures need to be supplemented with hirudin to boost proplatelet formation. After enrichment of mature MKs by BSA density gradient and subsequent plating at the appropriate cell density, ∼20% of MKs should form proplatelets. Cultures can, however, vary significantly in their proplatelet output from week to week without overt methodologic changes.
MKs have a frequency of around 0.05% to 0.1% of nucleated BM cells (Ebaugh & Bird, 1951; Italiano et al., 1999). The isolation of MKs by MACS may result in a 500-fold enrichment (up to 50%) depending on the selection of MicroBeads and columns for cell separation. Further, primary MKs and in vitro differentiated MKs exhibit several distinct features that should be taken into account during experiment design. As mentioned previously, primary MKs tend to have a “ghostly” appearance. Still, these MKs are larger in size and have a prominently visible DMS and polylobulated nucleus, reflecting higher polyploidy compared with cultured MKs.
Time Considerations
Basic Protocol 1 requires a considerable amount of time to isolate BM, adjust cell counts, perform MACS separation to harvest the primary cells, and plate the cultures. The beginner should schedule at least half a day for this procedure. Subsequent analyses such as flow cytometry or immunofluorescence staining further require 3 to 4 hr. Optionally, cells can be fixed and stored at 4°C for analysis at a later time.
For Basic Protocol 2, the culture period from isolating fetal livers or BM to obtaining mature, proplatelet-forming MKs lasts about 4 to 6 days. The bulk of the labor, however, is concentrated in the first day (day 0), including dissection of tissue and preparation of single-cell suspensions. The beginner should schedule 3 to 4 hr for these steps, depending on his/her skill in dissection and tissue harvest.
If a BSA step gradient is applied to enrich for MKs at advanced differentiation stages, it is advisable to prepare 1.5% and 3% BSA stock solutions in advance (stored at 4°C). Gradient separation and preparation for additional cell culture takes 60 to 90 min; about half this time is hands off while the cells sediment by gravity.
For flow cytometry analysis of MKs (Support Protocol 3), one should consider ∼1 hr for the preparation of CATCH buffer. However, this step is substantially accelerated once a stock solution has been prepared. Depending on the MK yield after isolation/culture measurement, recording of a sufficient number of events may take several minutes per sample.
Immunofluorescence staining of MKs according to Support Protocol 4 takes about 2 to 2.5 hr, but incubation time of antibodies may have to be optimized for each antibody separately. Image acquisition and processing is dependent on the desired image quality and resolution. This usually requires 2 days in addition to the amount of time needed for staining.
Acknowledgments
We thank Margret Droste and Anna Maria Arold for excellent experimental support. This research was funded by the Deutsche Forschungsgemeinschaft (DFG, 284 German Research Foundation) – Projektnummer 374031971 – TRR 240 to HS (A03).
Open access funding enabled and organized by Projekt DEAL.
Author Contributions
Kristina Mott : conceptualization, methodology, writing–original draft; Harald Schulze : conceptualization, funding acquisition, methodology, supervision, writing–review and editing.
Conflict of Interest
The authors declare no conflicts of interest.
Open Research
Data Availability Statement
The data that support the findings of this study are available from the corresponding author upon reasonable request.
Literature Cited
- Becker, I C., Nagy, Z., Manukjan, G., Haffner-Luntzer, M., Englert, M., Heib, T., Vögtle, T., Gross, C., Bharti, R., Dietrich, S., Mott, K., Heck, J., Stegmaier, S., Baranowsky, A., Schinke, T., Schlegel, N., Heckel, T., Stegner, D., Pleines, I., … Nieswandt, B. (2022). G6b-B regulates an essential step in megakaryocyte maturation. Blood Advances , 6(10), 3155–3161. https://doi.org/10.1182/bloodadvances.2021006151
- Bender, M., Stritt, S., Nurden, P., Van Eeuwijk, J. M. M., Zieger, B., Kentouche, K., Schulze, H., Morbach, H., Stegner, D., Heinze, K. G., Dütting, S., Gupta, S., Witke, W., Falet, H., Fischer, A., Hartwig, J. H., & Nieswandt, B. (2014). Megakaryocyte-specific profilin1-deficiency alters microtubule stability and causes a Wiskott-Aldrich syndrome-like platelet defect. Nature Communications , 5, 4746. https://doi.org/10.1038/ncomms5746
- Choi, E. S., Hokom, M. M., Chen, J. L., Skrine, J., Faust, J., Nichol, J., & Hunt, P. (1996). The role of megakaryocyte growth and development factor in terminal stages of thrombopoiesis. British Journal of Haematology , 95(2), 227–233. https://doi.org/10.1046/j.1365-2141.1996.d01-1920.x
- Cortegano, I., Serrano, N., Ruiz, C., Rodríguez, M., Prado, C., Alía, M., Hidalgo, A., Cano, E., De Andrés, B., & Gaspar, M. L. (2019). CD45 expression discriminates waves of embryonic megakaryocytes in the mouse. Haematologica , 104(9), 1853–1865. https://doi.org/10.3324/haematol.2018.192559
- Debili, N., Louache, F., & Vainchenker, W. (2004). Isolation and culture of megakaryocyte precursors. Methods in Molecular Biology , 272, 293–308. https://doi.org/10.1385/1-59259-782-3:293
- Dolzhanskiy, A., Basch, R. S., & Karpatkin, S. (1997). The development of human megakaryocytes: III. Development of mature megakaryocytes from highly purified committed progenitors in synthetic culture media and inhibition of thrombopoietin-induced polyploidization by interleukin-3. Blood , 89(2), 426–434.
- Donovan, J., & Brown, P. (2006). Euthanasia. Current Protocols in Immunology , 73, 1.8.1–1.8.4. https://doi.org/10.1002/0471142735.im0108s73
- Ebaugh, F. G. Jr., & Bird, R. M. (1951). The normal megakaryocyte concentration in aspirated human bone marrow. Blood , 6(1), 75–80. https://doi.org/10.1182/blood.V89.2.426
- Eckly, A., Strassel, C., Cazenave, J. P., Lanza, F., Léon, C., & Gachet, C. (2012). Characterization of megakaryocyte development in the native bone marrow environment. Methods in Molecular Biology , 788, 175–192. https://doi.org/10.1007/978-1-61779-307-3_13
- Englert, M., Aurbach, K., Becker, I. C., Gerber, A., Heib, T., Wackerbarth, L M., Kusch, C., Mott, K., Araujo, G. H. M., Baig, A. A., Dütting, S., Knaus, U. G., Stigloher, C., Schulze, H., Nieswandt, B., Pleines, I., & Nagy, Z. (2022). Impaired microtubule dynamics contribute to microthrombocytopenia in RhoB-deficient mice. Blood Advances , 6(17), 5184–5197. https://10.1182/bloodadvances.2021006545
- Heib, T., Gross, C., Müller, M. L., Stegner, D., & Pleines, I. (2021). Isolation of murine bone marrow by centrifugation or flushing for the analysis of hematopoietic cells - a comparative study. Platelets , 32(5), 601–607. https://doi.org/10.1080/09537104.2020.1797323
- Italiano, J. E. Jr., Lecine, P., Shivdasani, R. A., & Hartwig, J. H. (1999). Blood platelets are assembled principally at the ends of proplatelet processes produced by differentiated megakaryocytes. Journal of Cell Biology , 147(6), 1299–1312. https://doi.org/10.1083/jcb.147.6.1299
- Kaushansky, K. (1995). Thrombopoietin: The primary regulator of platelet production. Blood , 86(2), 419–431. https://doi.org/10.1182/blood.V86.2.419.bloodjournal862419
- Kruisbeek, A. M. (2000). Isolation of mouse mononuclear cells. Current Protocols in Immunology , 39, 3.1.1–3.1.5. https://doi.org/10.1002/0471142735.im0301s39
- Matsumura-Takeda, K., Sogo, S., Isakari, Y., Harada, Y., Nishioka, K., Kawakami, T., Ono, T., & Taki, T. (2007). CD41+/CD45+ cells without acetylcholinesterase activity are immature and a major megakaryocytic population in murine bone marrow. Stem Cells , 25(4), 862–870. https://doi.org/10.1634/stemcells.2006-0363
- Osman, S., Dalmay, D., & Mahaut-Smith, M. (2018). Fluorescence approaches to image and quantify the demarcation membrane system in living megakaryocytes. Methods in Molecular Biology , 1812, 195–215. https://doi.org/10.1007/978-1-4939-8585-2_12
- Schulze, H. (2016). Culture, expansion, and differentiation of murine megakaryocytes from fetal liver, bone marrow, and spleen. Current Protocols in Immunology , 112, 22F.26.21–22F.26.15. https://doi.org/10.1002/0471142735.im22f06s112
- Semeniak, D., Kulawig, R., Stegner, D., Meyer, I., Schwiebert, S., Bösing, H., Eckes, B., Nieswandt, B., & Schulze, H. (2016). Proplatelet formation is selectively inhibited by collagen type I through Syk-independent GPVI signaling. Journal of Cell Science , 129(18), 3473–3484. https://doi.org/10.1242/jcs.187971
- Shivdasani, R. A., & Schulze, H. (2005). Culture, expansion, and differentiation of murine megakaryocytes. Current Protocols in Immunology , 112, 22F.6.1–22F.6.15. https://doi.org/10.1002/0471142735.im22f06s67
- Sitnicka, E., Lin, N., Priestley, G. V., Fox, N., Broudy, V. C., Wolf, N. S., & Kaushansky, K. (1996). The effect of thrombopoietin on the proliferation and differentiation of murine hematopoietic stem cells. Blood , 87(12), 4998–5005. https://doi.org/10.1182/blood.V87.12.4998.bloodjournal87124998
- Vijey, P., Posorske, B., & Machlus, K. R. (2018). In vitro culture of murine megakaryocytes from fetal liver-derived hematopoietic stem cells. Platelets , 29(6), 583–588. https://doi.org/10.1080/09537104.2018.1492107
- Zeigler, F. C., De Sauvage, F., Widmer, H., Keller, G., Donahue, C., Schreiber, R., Malloy, B., Hass, P., Eaton, D., & Matthews, W. (1994). In vitro megakaryocytopoietic and thrombopoietic activity of c-mpl ligand (TPO) on purified murine hematopoietic stem cells. Blood , 84(12), 4045–4052. https://doi.org/10.1182/blood.V84.12.4045.bloodjournal84124045