In Vitro Generation of Posterior Motor Neurons from Human Pluripotent Stem Cells
Anestis Tsakiridis, Anestis Tsakiridis, Matt Wind, Matt Wind
Abstract
The ability to generate spinal cord motor neurons from human pluripotent stem cells (hPSCs) is of great use for modelling motor neuron–based diseases and cell-replacement therapies. A key step in the design of hPSC differentiation strategies aiming to produce motor neurons involves induction of the appropriate anteroposterior (A-P) axial identity, an important factor influencing motor neuron subtype specification, functionality, and disease vulnerability. Most current protocols for induction of motor neurons from hPSCs produce predominantly cells of a mixed hindbrain/cervical axial identity marked by expression of Hox paralogous group (PG) members 1-5, but are inefficient in generating high numbers of more posterior thoracic/lumbosacral Hox PG(8-13)+ spinal cord motor neurons. Here, we describe a protocol for efficient generation of thoracic spinal cord cells and motor neurons from hPSCs. This step-wise protocol relies on the initial generation of a neuromesodermal-potent axial progenitor population, which is differentiated first to produce posterior ventral spinal cord progenitors and subsequently to produce posterior motor neurons exhibiting a predominantly thoracic axial identity. © 2021 The Authors. Current Protocols published by Wiley Periodicals LLC.
Basic Protocol 1 : Differentiation of neuromesodermal progenitors
Basic Protocol 2 : Posterior ventral spinal cord progenitor differentiation
Basic Protocol 3 : Posterior motor neuron differentiation
INTRODUCTION
During embryonic development, motor neurons (MNs) arise from a set of progenitors within the ventral spinal cord, from which they mature and project axons to innervate target muscles. Multiple MN subtypes are specified across the anterior-posterior (A-P) axis of the spinal cord, allowing for innervation of the diverse axial-level-dependent muscle targets (reviewed in Sagner & Briscoe, 2019). The specification of MNs across the A-P axis of the spinal cord is largely regulated by a family of homeobox genes known as HOX genes (Dasen, Liu, & Jessell, 2003; Dasen, Tice, Brenner-Morton, & Jessell, 2005, 2008; Jung et al., 2010). Hox genes are arranged as paralogous groups (PG) 1-13 across four distinct chromosomal clusters (A-D) and are expressed along the post-cranial A-P axis in a strict spatiotemporal manner reflecting their 3′-to-5′ genomic order: hindbrain/cervical MNs are marked by Hox PG(1-5), whereas more posterior thoracic and lumbosacral MNs are marked by Hox PG(6-9) and Hox PG(10-13), respectively.
Damage or degeneration of MNs results in a large array of movement-based disorders. In vitro production of MNs from human pluripotent stem cells (hPSCs) has provided a useful tool for in vitro modelling of these neurodegenerative diseases as well as cell therapy applications, e.g., for treatment of spinal cord injuries. Most conventional hPSC differentiation protocols for generation of MNs rely predominantly on initial induction of an anterior neural identity that is successively patterned to a ventral spinal cord/MN fate through exposure to sonic hedgehog (SHH) and retinoic acid signals (Amoroso et al., 2013; Lee et al., 2007; Li et al., 2008; Peljto, Dasen, Mazzoni, Jessell, & Wichterle, 2010; Wichterle, Lieberam, Porter, & Jessell, 2002). However, MNs produced in these protocols predominantly exhibit a Hox PG(1-5)+ hindbrain/cervical character, with low yields of the more posterior Hox PG(8-13)+ thoracic/lumbosacral MNs.
We recently described an efficient protocol for generation of posterior motor neurons that mainly display a thoracic axial identity (Wind et al., 2021). Our strategy is based on initial induction of a neuromesodermal progenitor (NMP)–like population from hPSCs following treatment with WNT and FGF signaling pathway agonists (Frith et al., 2018; Gouti et al., 2014; Lippman et al., 2015). In vivo, NMPs are posteriorly located bipotent progenitors that give rise to neural and mesodermal cells of the post-cranial axis during embryonic development (Tzouanacou, Wegener, Wymeersch, Wilson, & Nicolas, 2009; Wymeersch et al., 2016; reviewed in Wymeersch, Wilson, & Tsakiridis, 2021). These are subsequently steered toward a ventral spinal cord progenitor state via active suppression of the pro-dorsal TGF-β and BMP signaling pathways in addition to SHH/retinoic acid signaling stimulation, which eventually gives rise to posterior MNs following culture in neurotrophic medium (Wind et al., 2021). Here we provide an in depth step-by-step description of this approach.
Basic Protocol 1: DIFFERENTIATION OF NEUROMESODERMAL PROGENITORS
The initial step of the differentiation protocol depends upon the high yield generation of NMP-like cells from hPSCs driven by simultaneous stimulation of the WNT and FGF signaling pathways and inhibition of BMP activity (Fig. 1A) to limit induction of neural crest progenitors (Frith et al., 2018). These are marked by the co-expression of TBXT and SOX2, transcriptional factors indicative of a mesodermal and neural identity, respectively. Moreover, NMP cultures exhibit expression of other posteriorly expressed genes, such as NKX1-2, CDX2 and Hox family members.
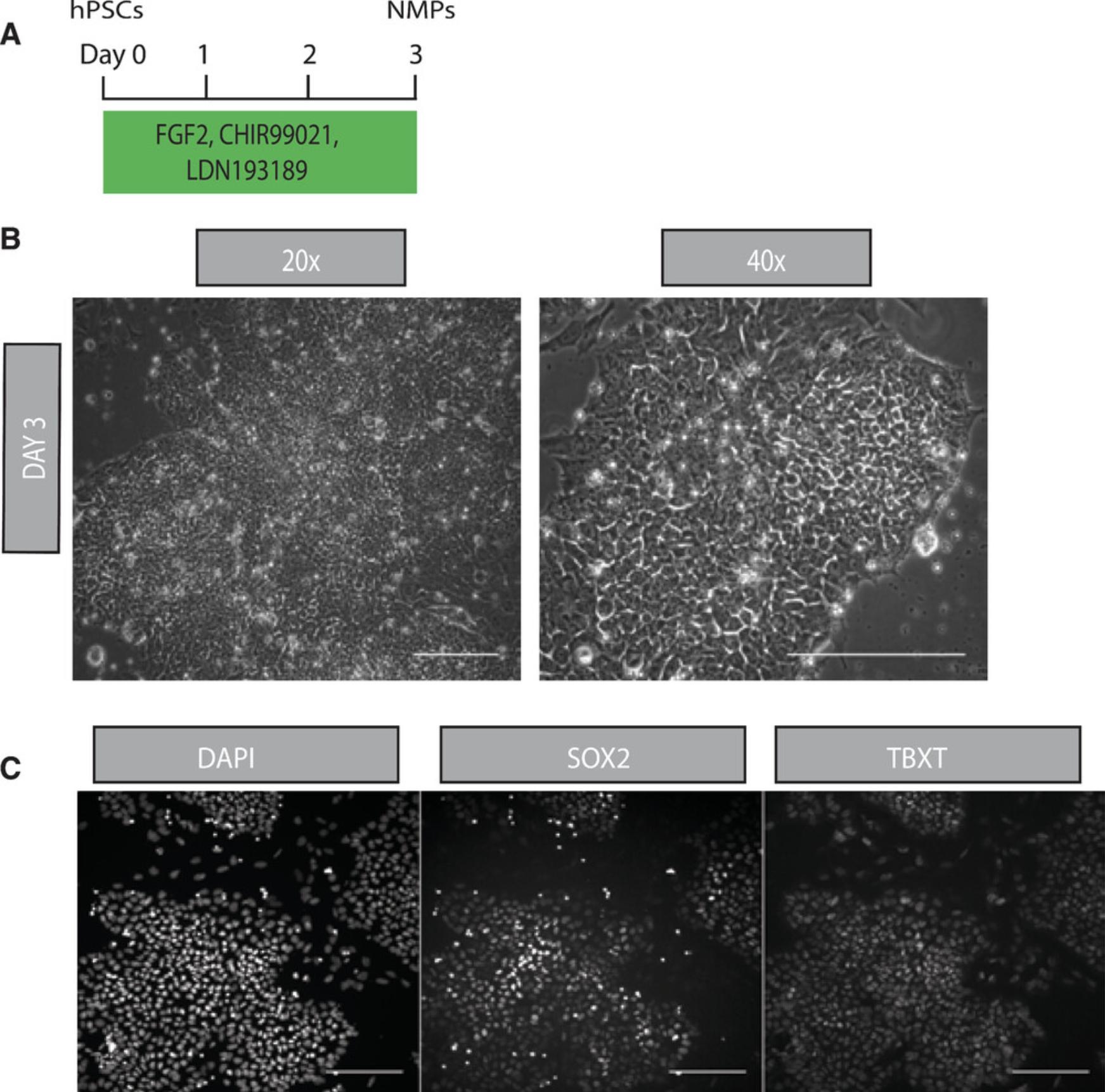
Materials
-
0.6 mg/ml vitronectin (Thermo Fisher Scientific, cat. no. A31804), stored in 200-μl aliquots at −80°C
-
Sterile PBS
-
Human pluripotent stem cells (hPSCs) grown to 60%-80% confluency
-
5 mM UltraPure EDTA, pH 8.0 (Thermo Fisher Scientific, cat. no. 15575020) in sterile PBS, stored at 4°C
-
DMEM F-12 medium with 15 mM HEPES, without l-glutamine (Sigma, cat. no. D6421)
-
N2B27 medium (see recipe), freshly prepared
-
Antibodies for confirming cell identity:
- Anti-SOX2 (Abcam, cat. no. ab92494, RRID AB_10585428)
- Anti-TBXT (R&D, cat. no. AF2085, RRID AB_2200235)
-
Multiwell cell culture plates
-
37°C/5% CO2 incubator
-
15-ml conical tubes (Falcon)
-
Hemocytometer
-
Additional reagents and solutions for standard antibody staining protocols
Day 0: Prepare vitronectin-coated plates 30 min before use
1.Thaw an aliquot of 0.6 mg/ml vitronectin stock at room temperature.
2.Dilute 1:100 with sterile PBS (final 6 µg/ml).
3.Cover the entire surface of multiwell plates with vitronectin (150 µl/cm2) and incubate 1 hr at room temperature or overnight at 37°C/5% CO2.
Day 0: Seed hPSCs
4.Aspirate medium from hPSCs with a confluency of 60%-80%.
5.Add enough 5 mM EDTA to cover the cells (150 µl/cm2) and incubate 5 min at 37°C/5% CO2.
6.Aspirate EDTA and apply DMEM-F12 medium (150 µl/cm2) to lift cells as small clumps.
7.Transfer suspended cells to a 15-ml Falcon tube and spin 4 min at 200 × g.
8.Resuspend cell pellet in 1 ml freshly prepared N2B27 medium supplemented with 10 μM Y-27632.
9.Count cells in a 10-μl aliquot using a hemocytometer.
10.Aspirate vitronectin from the culture plates and add 150 µl/cm2 N2B27 medium with 10 μM Y-27632 to each well.
11.Plate cells at a density of 60,000 cells/cm2.
12.Rock gently to distribute the cells evenly across the wells and incubate overnight at 37°C/5% CO2.
Day 1: Replenish medium
13.Aspirate medium and replace with freshly prepared N2B27 medium without Y-27632.
14.Incubate until day 3 at 37°C/5% CO2.
Day 3: Assess differentiation
15.On day 3, confirm the NMP cell type by antibody staining.
Basic Protocol 2: DIFFERENTIATION OF POSTERIOR VENTRAL SPINAL CORD PROGENITORS
This protocol encompasses the directed differentiation of human NMPs to a posterior ventral spinal cord identity. It relies on combined BMP and TGF-β inhibition and supplementation by retinoic acid and SHH agonist signals (Figs. 2A, 3A) to first promote generation of HOXC9+/SOX1+/PAX6+ posterior neurectoderm cells and subsequently induce differentiation of ventral spinal cord progenitors.
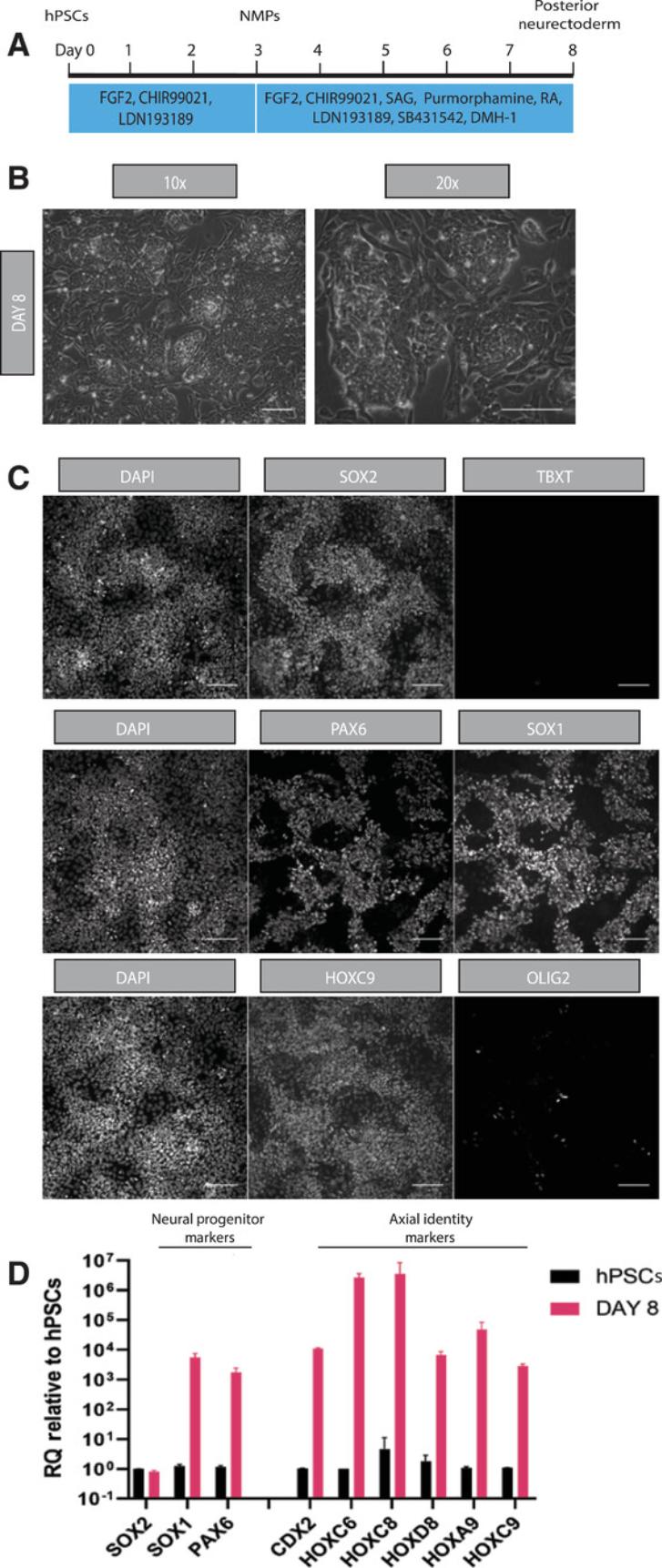
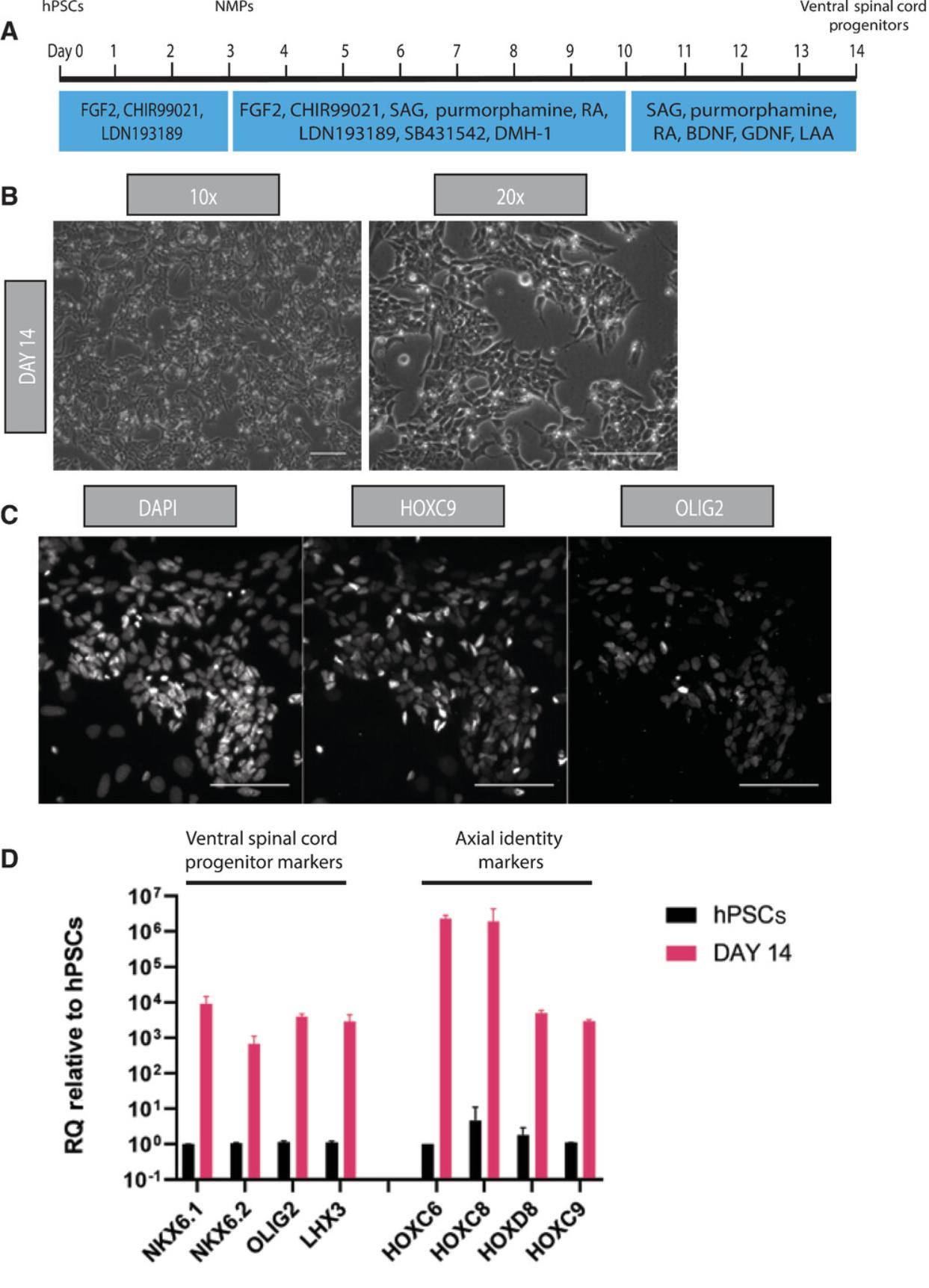
Materials
-
NMPs (see Basic Protocol 1)
-
Geltrex LDEV-Free Reduced Growth Factor Basement Membrane Matrix (Thermo Fisher Scientific, cat. no. A1413201), stored in 100-μl aliquots at −80°C
-
DMEM F-12 medium with 15 mM HEPES, without l-glutamine (Sigma, cat. no. D6421)
-
Accutase (Sigma, cat. no. A6964)
-
Posterior neurectoderm induction medium (see recipe), freshly prepared
-
Posterior ventral spinal cord progenitor induction medium (see recipe), freshly prepared
-
Antibodies for confirming cell identity:
- Anti-SOX2 (Abcam, cat. no. ab92494, RRID AB_10585428)
- Anti-TBXT (R&D, cat. no. AF2085, RRID AB_2200235)
- Anti-PAX6 (Biolegend, cat. no. 901302, RRID AB_2749901)
- Anti-SOX1 (R&D, cat. no. AF3369, RRID AB_2239879)
- Anti-HOXC9 (Abcam, cat. no. ab50839, RRID AB_880494)
- Anti-OLIG2 (R&D, cat. no. AF2418, RRID AB_2157554)
-
Primers for confirming cell identity (see Table 1)
-
Multiwell cell culture plates
-
37°C/5% CO2 incubator
-
15-ml conical tubes (Falcon)
-
Hemocytometer
-
Additional reagents and solutions for standard antibody staining and qPCR protocols
Primer | Forward | Reverse | |
---|---|---|---|
For posterior neuroectoderm: | |||
SOX2 | ttgctgcctctttaagactagga | Taagcctggggctcaaact | |
SOX1 | gaagcccagatggaaatacg | ggacaaggaagggtgttgag | |
PAX6 | gcacacacacattaacacacttg | ggtgtgtgagagcaattctcag | |
CDX2 | atcaccatccggaggaaag | tgcggttctgaaaccagatt | |
HOXC6 | tgaattcctacttcactaacccttc | atcataggcggtggaattga | |
HOXC8 | tcccagcctcatgtttcc | tgataccggctgtaagtttgc | |
HOXD8 | cccttgtaatcgcctgaaat | ctactgaaaataacggaacacagc | |
HOXA9 | ccccatcgatcccaataa | caccgctttttccgagtg | |
HOXC9 | tcctagcgtccaggtttcc | gctacagtccggcaccaa | |
For ventral spinal cord progenitors: | |||
NKX6.1 | gagatgaagaccccgctgta | gacgacgacgaggacgag | |
NKX6.2 | agcacaaaccctcgaacttg | cccggattctgcaaaaatag | |
OLIG2 | agctcctcaaatcgcatcc | atagtcgtcgcagctttcg | |
LHX3 | gttcaggaggggcaggac | ctcccgtagaggccattg | |
HOXC6 | tgaattcctacttcactaacccttc | atcataggcggtggaattga | |
HOXC8 | tcccagcctcatgtttcc | tgataccggctgtaagtttgc | |
HOXD8 | cccttgtaatcgcctgaaat | ctactgaaaataacggaacacagc | |
HOXC9 | tcctagcgtccaggtttcc | gctacagtccggcaccaa | |
For posterior motor neurons: | |||
ISLET1 | aaacaggagctccagcaaaa | aaaggactctttcagccaagg | |
MNX1 | ttacctgacttatgaaacttgaaacc | cccagagacgtaagcataaacc | |
CDX2 | atcaccatccggaggaaag | tgcggttctgaaaccagatt | |
HOXC6 | tgaattcctacttcactaacccttc | atcataggcggtggaattga | |
HOXC8 | tcccagcctcatgtttcc | tgataccggctgtaagtttgc | |
HOXD8 | cccttgtaatcgcctgaaat | ctactgaaaataacggaacacagc | |
HOXC9 | tcctagcgtccaggtttcc | gctacagtccggcaccaa |
Day 3: Prepare Geltrex-coated plates
1.Thaw an aliquot of Geltrex by resuspending 1:100 with cold (4°C) sterile DMEM-F12 medium.
2.Add Geltrex to cover the entire surface of the multiwell culture plates (150 µl/cm2) and incubate 1 hr at 37°C/5% CO2.
Days 3-7: Perform posterior neuroectoderm differentiation
3.Aspirate medium from NMPs and add Accutase to cover the cells (150 µl/cm2). Incubate 5 min at 37°C/5% CO2.
4.Add excess DMEM-F12 (at least 2 vol. relative to Accutase) and triturate gently to create a single-cell suspension.
5.Transfer cell suspension to a 15-ml Falcon tube and centrifuge 4 min at 200 × g.
6.Resuspend cell pellet in 1 ml of freshly prepared posterior neurectoderm induction medium supplemented with 10 μM Y-27632.
7.Count cells in a 10-μl aliquot using a hemocytometer.
8.Aspirate Geltrex from the culture plates and add 150 µl/cm2 of posterior neurectoderm induction medium with 10 μM Y-27632 to each well.
9.Plate cells at a density of 60,000 cells/cm2.
10.Rock gently to distribute the cells evenly across the wells and incubate overnight at 37°C/5% CO2.
11.On days 4 and 6, aspirate medium and replace with fresh medium without Y-27632 (150 µl/cm2).
Days 7-14: Perform posterior ventral spinal cord progenitor differentiation
12.On day 7, repeat steps 1-11 to replate cells at a density of 80,000 cells/cm2.
13.On day 8, aspirate medium and replace with fresh medium without Y-27632 (150 µl/cm2).
14.Also on day 8, confirm posterior neuroectoderm cell type by antibody staining and qPCR.
15.On day 9, aspirate medium and replace with fresh medium without Y-27632 (150 µl/cm2).
16.On day 10, aspirate medium and switch to fresh posterior ventral spinal cord progenitor induction medium (150 µl/cm2).
17.On day 12, aspirate medium and replace with fresh medium posterior ventral spinal cord progenitor induction medium (150 µl/cm2).
Day 14: Assess differentiation
18.On day 14, confirm the posterior ventral spinal cord progenitor cell type by antibody staining and qPCR.
Basic Protocol 3: DIFFERENTIATION OF POSTERIOR MOTOR NEURONS
The final step of the procedure allows for promotion of a post-mitotic posterior motor neuron identity. Supplementation with the γ-secretase inhibitor DAPT promotes exit from a progenitor state, and the presence of neurotrophins promotes neuronal survival and axonal outgrowth (Fig. 4A).
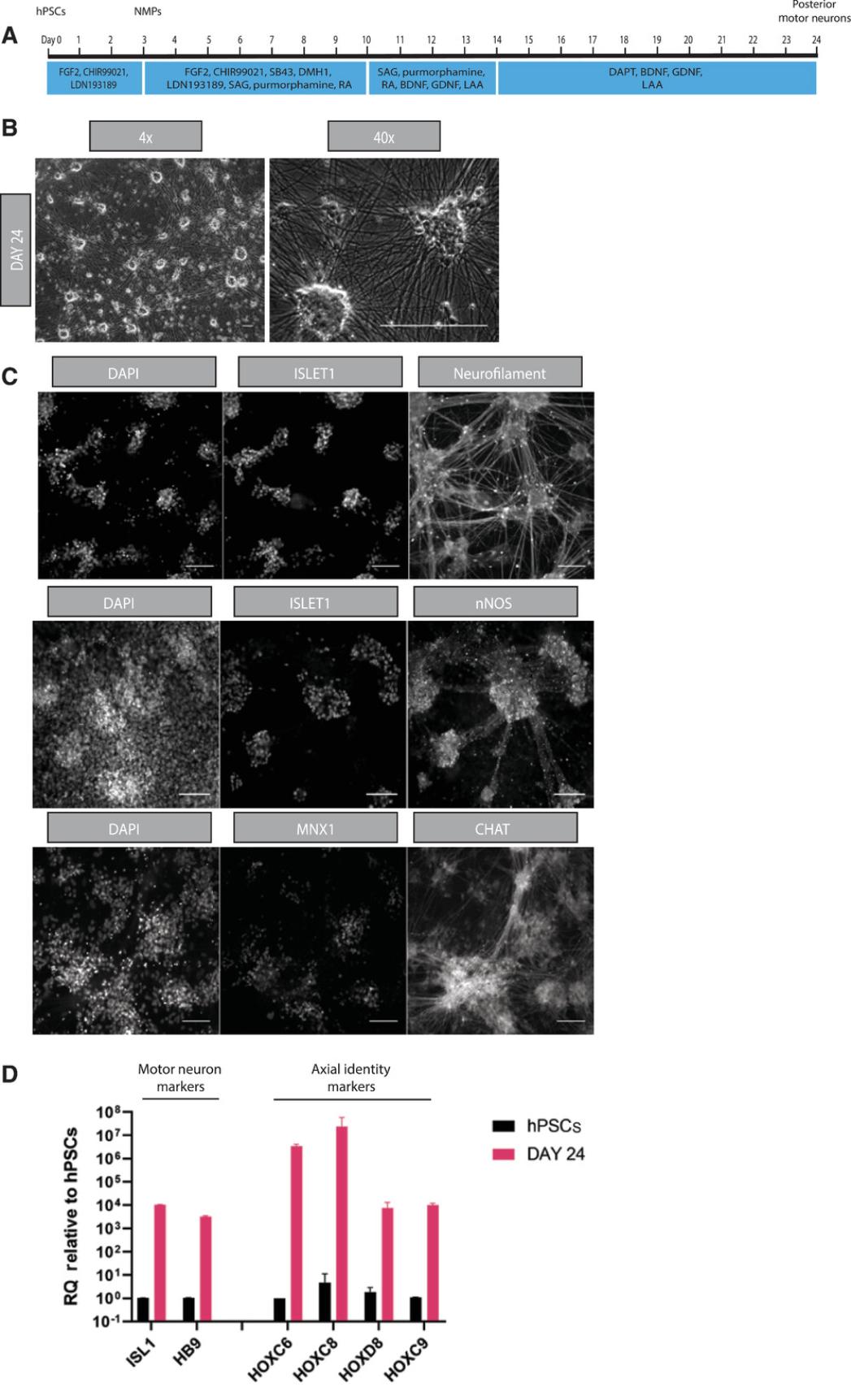
Materials
-
Geltrex LDEV-Free Reduced Growth Factor Basement Membrane Matrix (Thermo Fisher Scientific, cat. no. A1413201), stored in 100-μl aliquots at −80°C
-
DMEM F-12 medium with 15 mM HEPES, without l-glutamine (Sigma, cat. no. D6421)
-
Posterior ventral spinal cord progenitors (see Basic Protocol 2)
-
Accutase (Sigma, cat. no. A6964)
-
Motor neuron maturation medium (see recipe), freshly prepared
-
Antibodies for confirming cell identity:
- Anti-ISELT1 (R&D, cat. no. AF1837, RRID AB_2126324)
- Anti-ISLET1/2 (DSHB, cat. no. 39.4D5, RRID AB_2314683)
- Anti-neurofilament (Abcam, cat. no. ab8135, RRID AB_306298)
- Anti-nNOS (Thermo Fisher Scientific, cat. no. 37-2800, RRID AB_2533308)
- Anti-MNX1 (DSHB, cat. no. 81.5C10, RRID AB_2145209)
- Anti-cholinergic acetyltransferase (Abcam, cat. no. ab144P, RRID AB_90661)
-
Primers for confirming cell identity (see Table 1)
-
Multiwell cell culture plates
-
37°C/5% CO2 incubator
-
15-ml conical tubes (Falcon)
-
Hemocytometer
-
Additional reagents and equipment for standard antibody staining, qPCR, and patch-clamp recording protocols
Day 14: Perform posterior motor neuron differentiation
1.On day 14, prepare fresh Geltrex-coated plates as described (see Basic Protocol 2, steps 1-2).
2.Aspirate medium from ventral cord progenitor cells and add Accutase (150 µl/cm2) to cover the cells. Incubate 5 min at 37°C/5% CO2.
3.Add excess DMEM-F12 (at least 2 vol. relative to Accutase) and triturate gently to create a single-cell suspension.
4.Transfer cell suspension to a 15-ml Falcon tube and centrifuge 4 min at 200 × g.
5.Resuspend cell pellet in 1 ml of freshly prepared motor neuron maturation medium supplemented with 10 μM Y-27632.
6.Count cells in a 10-μl aliquot using a hemocytometer.
7.Aspirate Geltrex from the culture plates and add 150 µl/cm2 of motor neuron maturation medium with 10 μM Y-27632 to each well.
8.Plate cells at a density of 100,000 cells/cm2.
9.Rock gently to distribute the cells evenly across the wells and incubate 48 hr at 37°C/5% CO2.
10.On days 16, 18, 20, and 22, aspirate medium and replace with fresh motor neuron maturation medium without Y-27632 (150 µl/cm2).
Day 24: Assess differentiation
11.On day 24, confirm posterior motor neuron differentiation by antibody staining, qPCR, and electrophysiology.
REAGENTS AND SOLUTIONS
Motor neuron maturation medium
- 49.7 ml 1× N2B27 medium (see recipe)
- 20 ng/ml recombinant BDNF
- 20 ng/ml recombinant GDNF
- 200 µM l-ascorbic acid
- 10 µM γ-secretase inhibitor DAPT
- 10 µM Y-27632 (on day 14 only)
- Prepare fresh each day
Y-27632 is used only for replating ventral spinal cord progenitors.
N2B27 medium
- 94 ml 1× DMEM F-12 with 15 mM HEPES and no l-glutamine (Sigma, cat. no. D6421)
- 94 ml 1× Neurobasal Medium (Thermo Fisher Scientific, cat. no. 21103-049)
- 2 ml 100× N-2 Supplement (Thermo Fisher Scientific, cat. no. 17502-001; final 1×)
- 4 ml 50× B-27 Serum-Free Supplement (Thermo Fisher Scientific, cat. no. 17504-001; final 1×)
- 2 ml 100× MEM Non-Essential Amino Acids (Thermo Fisher Scientific, cat. no. 11140-035; final 1×)
- 2 ml 100× Glutamax (Thermo Fisher Scientific, cat. no. 35050-038; final 1×)
- 200 µl 50 mM 2-mercaptoethanol (Thermo Fisher Scientific, cat. no. 31350-010; final 100 µM)
- 2 ml 100× penicillin-streptomycin (final 1×; optional)
- Store up to 3 weeks at 4°C
- On the day of use add:
- 20 ng/ml recombinant basic FGF
- 3 µM CHIR99021
- 100 nM LDN193189
- 10 μM Y-27632 (on days 1, 3, 7 only)
CHIR99021 is a GSK-3 inhibitor, LDN193189 is an ALK2/ALK3 inhibitor, and Y-27632 is a Rho-kinase inhibitor. Y-27632 is used only for seeding hPSCs and replating NMPs.
Posterior neurectoderm induction medium
- 49.4 ml 1× N2B27 medium (see recipe)
- 100 ng/ml FGF2
- 3 µM CHIR99021
- 100 nM LDN193189
- 10 µM SB431542
- 1 µM DMH1
- 500 nM SAG
- 1 µM purmorphamine
- 100 nM all-trans retinoic acid
- Prepare fresh each day
Posterior ventral spinal cord progenitor induction medium
- 49.7 ml 1× N2B27 medium (see recipe)
- 500 nM SAG
- 1 µM purmorphamine
- 100 nM all-trans retinoic acid
- 20 ng/ml recombinant BDNF
- 20 ng/ml recombinant GDNF
- 200 µM l-ascorbic acid
- Prepare fresh each day
Small molecule and protein stock solutions
- All-trans retinoic acid (Tocris, cat. no. 0695), 1 mM in DMSO, stored in 200-μl aliquots at −20°C
- L-Ascorbic acid 2-phosphate sesquimagnesium salt hydrate (Sigma, cat. no. A8960), 200 mM in sterile H2O, stored in 200-μl aliquots at −20°C
- CHIR99021 (Tocris, cat. no. 4423): 10 mM in DMSO, stored in 200-μl aliquots at −20°C
- DAPT (Sigma, cat. no. D5942), 10 mM in DMSO, stored in 100-μl aliquots at −20°C
- DMH1 (Tocris cat. no. 4126), 10 mM in DMSO, stored in 50-μl aliquots at −20°C
- LDN193189 dihydrochloride (Tocris, cat. no. 6053): 1 mM in DMSO, stored in 50-μl aliquots at −20°C
- Purmorphamine (Sigma cat. no. SML0868), 10 mM in DMSO, stored in 50-μl aliquots at −20°C
- Recombinant human/murine/rat BDNF (Peprotech, cat. no. 450-02), 10 μg/ml in PBS, stored in 200-μl aliquots at −20°C
- Recombinant human FGF-basic (154 a.a.; Peprotech, cat. no. 100-18B): 10 μg/ml in PBS, stored in 200-μl aliquots at −20°C
- Recombinant human GDNF (Peprotech, cat. no. 450-10), 10 μg/ml in PBS, stored in 200-μl aliquots at −20°C
- SAG (Tocris cat. no. 4366), 10 mM in DMSO, stored in 50-μl aliquots at −20°C
- SB431542 (Tocris cat. no. 1614), 10 mM in DMSO, stored in 50-μl aliquots at −20°C
- Y-27632 dihydrochloride (Adooq Biosciences, cat. no. A11001): 10 mM in DMSO, stored 200-μl aliquots at −20°C
For maximum storage times, refer to manufacturer's instructions.
COMMENTARY
Background Information
Because damage or degeneration of MNs occurs in a wide array of movement-based disorders, the in vitro generation of MNs of defined A-P identities from hPSCs has been the focus of intense research.
Conventional MN differentiation protocols typically rely on induction of an early anterior neural identity through exposure to TGF-β and BMP inhibitors (Amoroso et al., 2013; Chambers et al., 2009). Subsequent treatment with SHH agonists has been globally utilized to promote a ventral character, whereas simultaneous exposure to retinoic acid is designed to promote more caudal identities. However, MNs produced through this approach exhibit a predominantly hindbrain/cervical/upper brachial character marked by expression of HOX PG(1-6) members and innervating the anterior musculature upon in vivo transplantation (Amoroso et al., 2013; Soundararajan, Miles, Rubin, Brownstone, & Rafuse, 2006).
During amniote embryonic development, posteriorly located neuromesodermal progenitors have been shown to give rise to cell lineages of the post-cranial axis (Cambray & Wilson, 2007; Tzouanacou et al., 2009; Wymeersch et al., 2016). Similarly, NMP-like cells generated in vitro from hPSCs can also be steered towards posterior neural, mesodermal, and neural crest lineages (Cooper et al., 2021; Frith et al., 2018; Gouti et al., 2014; Lippmann et al., 2015; Mouilleau et al., 2021; Turner, Rué, Mackenzie, Davies, & Martinez Arias, 2014; Verrier, Davidson, Gierliński, Dady, & Storey, 2018). Moreover, we have recently shown that the efficient generation of posterior thoracic HOX PG(6-9) motor neurons is best achieved by differentiating through a neuromesodermal progenitor intermediary state (Wind et al., 2021). This strategy relies on exposure of hPSC-derived NMPs to TGF-β and BMP inhibitors to promote a posterior neural tube identity, similar to that observed by Verrier et al. (2018). Further differentiation in the presence of Shh signals and TGF-β and BMP inhibitors coordinate more efficient ventralization to promote a MN identity, while continued high levels of FGF and Wnt signals are required to promote a posterior axial identity (Mouilleau et al., 2021; Wind et al., 2021).
Our differentiation strategy gives rise to cultures predominantly marked by high levels of HOX PG(8-9) transcripts and large numbers of HOXC9-ISLET1-nNOS co-expressing/MNX1-negative MNs, thus demonstrating a thoracic preganglionic columnar character. However, we have also detected the presence of more anterior (PG4-6) and posterior (PG10) HOX transcripts, indicative of potential co-emergence of MN subpopulations of a brachial and lumbar axial identity, respectively (Wind et al., 2021). Moreover, MNX1 positivity may reflect the induction of MNs of lateral, medial, or hypaxial motor column character.
Critical Parameters
Quality of hPSC cultures
Prior to use in differentiation protocols, it is essential to ensure that the hPSCs have retained pluripotency, which can be observed by screening for pluripotency markers using immunofluorescence and flow cytometry. A reduction in pluripotency and observed spontaneous differentiation in hPSC cultures can result in inefficient differentiation, and cultures with high levels of spontaneous differentiation or reduced pluripotency marker expression should be disposed. Screening for genetic abnormalities is also important, as specific aberrations can impair hPSC differentiation capabilities. Good practices such as regular culture medium changes, passaging cells once they reach 70%-80% confluency, and discarding cells that have a high passage number can ensure better hPSC quality. The protocol described here has been optimized using hPSCs cultured on vitronectin- and laminin-521-coated surfaces in Essential 8 medium. It is important to note that culturing hPSCs on different substrates and in different media can impact downstream differentiation efficiencies, and therefore further optimization may be required.
Titration of small molecule concentrations
We have found that levels of WNT, TGF-β, and BMP signals must be tightly regulated to allow both efficient neural induction and subsequent ventralization. Titration of TGF-β and BMP inhibitor and CHIRON concentrations is essential when using different cell lines, likely due to differences in endogenous signaling levels.
Cell density
It is important to ensure that the optimum number of cells is seeded at the indicated time points to ensure successful differentiation. The cells are highly proliferative between days 3 and 10 of differentiation, so it is important to plate cells at the correct densities on days 3 and 7 to prevent over-confluency and cell death. We have recommended optimal densities at each stage, but these should be fully optimized by each user and for individual hPSC lines.
Troubleshooting
For troubleshooting guidelines, see Table 2.
Problem | Possible solution |
---|---|
Low hPSC quality | Do not grown hPSCs past 80% confluency |
Change medium daily | |
Send cells for routine genetic screening and discard if any genetic aberrations are found | |
Discard hPSCs once they reach a high passage number (e.g., >20 passages) or if spontaneous differentiation is observed | |
Contamination in cell culture | Maintain a sterile working environment at all times when working with cells |
Clean incubators, tissue culture hoods, and water baths regularly | |
During long-term differentiation, include pen-strep or an equivalent antibiotic in the N2B27 basal medium to prevent bacterial contamination | |
Low cell survival following plating or replating | When lifting cells for replating, ensure that Accutase is not left on cells for an extended period of time (e.g., >5 min) |
Do not be handle cells harshly or abrasively when lifting or resuspending them | |
Be sure to seed cells in medium containing 10 μM Y-27632 for at least 24 hr | |
Ensure that plates are coated with vitronectin or Geltrex long enough for full surface coverage; do not allow plates to dry out | |
Poor induction of PAX6+/SOX1+ at posterior neurectoderm stage | Titrate concentrations of TGF-β and BMP inhibitors to identify optimal concentrations for neural induction (levels of endogenous TGF-β and BMP can vary between cell lines) |
Aliquot small molecules in small volumes to prevent repeated freezing and thawing | |
Low induction yield of ISLET1+ posterior motor neurons | Optimize cell density when replating on day 14 of differentiation |
Ensure that cells replated on day 14 are enriched in expression of ventral spinal cord progenitor markers | |
Increase exposure to Y-27632 from 24 hr to 48 hr to enhance formation of axonal projections | |
Difficulty lifting/detaching neurons at later stages of differentiation | At later stages of differentiations (day 18 onwards), the neurons will form foci with bundled axons, which can be fragile and prone to movement. To address this: |
Always apply medium gently to the wall of the plate wells; avoid applying it harshly or directly onto the cells | |
Conduct half medium changes by gently aspirating only half of the medium at a time, as this can be gentler for the cells | |
Supplement media with additional Geltrex during medium replacement to enhance neuron adhesion |
Acknowledgments
M.W. is supported by a University of Sheffield, Biomedical Science Departmental PhD studentship. A.T. is supported by funding from the BBSRC (New Investigator Research Grant BB/P000444/1), H2020-EU.1.2.2 (Grant agreement ID 824070), and the Medical Research Council (MRC; MR/V002163/1).
Author Contributions
Matt Wind : Conceptualization, Data curation, Formal analysis, Investigation, Methodology, Validation, Visualization, Writing-original draft, Writing-review & editing; Anestis Tsakiridis : Conceptualization, Formal analysis, Funding acquisition, Investigation, Project administration, Supervision, Validation, Visualization, Writing-original draft, Writing-review & editing.
Conflict of Interest
The authors declare no conflict of interest.
Open Research
Data Availability Statement
The data that support the findings of this study are available from the corresponding author upon reasonable request.
Literature Cited
- Amoroso, M. W., Croft, G. F., Williams, D. J., O'Keeffe, S., Carrasco, M. A., Davis, A. R., … Wichterle, H. (2013). Accelerated high-yield generation of limb-innervating motor neurons from human stem cells. Journal of Neuroscience , 33(2), 574–586. doi: 10.1523/jneurosci.0906-12.2013.
- Cambray, N., & Wilson, V. (2007). Two distinct sources for a population of maturing axial progenitors. Development , 134(15), 2829–2840. doi: 10.1242/dev.02877.
- Chambers, S. M., Fasano, C. A., Papapetrou, E. P., Tomishima, M., Sadelain, M., & Studer, L. (2009). Highly efficient neural conversion of human ES and iPS cells by dual inhibition of SMAD signaling. Nature Biotechnology , 27(3), 275–280. doi: 10.1038/nbt.1529.
- Cooper, F., Gentsch, G. E., Mitter, R., Bouissou, C., Healy, L., Hernandez-Rodriguez, A., … Bernardo, A. S. (2021). Rostrocaudal patterning and neural crest differentiation of human pre-neural spinal cord progenitors in vitro. bioRxiv , 2020.06.16.155564. doi: 10.1101/2020.06.16.155564.
- Dasen, J. S., De Camilli, A., Wang, B., Tucker, P. W., & Jessell, T. M. (2008). Hox repertoires for motor neuron diversity and connectivity gated by a single accessory factor, FoxP1. Cell , 134(2), 304–316. doi: 10.1016/j.cell.2008.06.019.
- Dasen, J. S., Liu, J. P., & Jessell, T. M. (2003). Motor neuron columnar fate imposed by sequential phases of Hox-c activity. Nature , 425(6961), 926–933. doi: 10.1038/nature02051.
- Dasen, J. S., Tice, B. C., Brenner-Morton, S., & Jessell, T. M. (2005). A Hox regulatory network establishes motor neuron pool identity and target-muscle connectivity. Cell , 123(3), 477–491. doi: 10.1016/j.cell.2005.09.009.
- Frith, T. J. R., Granata, I., Wind, M., Stout, E., Thompson, O., Neumann, K., … Tsakiridis, A. (2018). Human axial progenitors generate trunk neural crest cells in vitro. eLife , 7, e35786. doi: 10.7554/eLife.35786.
- Gouti, M., Tsakiridis, A., Wymeersch, F. J., Huang, Y., Kleinjung, J., Wilson, V., & Briscoe, J. (2014). In vitro generation of neuromesodermal progenitors reveals distinct roles for wnt signalling in the specification of spinal cord and paraxial mesoderm identity. PLoS Biology , 12(8), e1001937. doi: 10.1371/journal.pbio.1001937.
- Jung, H., Lacombe, J., Mazzoni, E. O., Liem, K. F., Grinstein, J., Mahony, S., … Dasen, S. (2010). Global control of motor neuron topography mediated by the repressive actions of a single Hox gene. Neuron , 67(5), 781–796. doi: 10.1016/j.neuron.2010.08.008.
- Lee, H., Al Shamy, G., Elkabetz, Y., Schofield, C. M., Harrsion, N. L., Panagiotakos, G., … Studer, L. (2007). Directed differentiation and transplantation of human embryonic stem cell-derived motoneurons. Stem Cells , 25(8), 1931–1939. doi: 10.1634/stemcells.2007-0097.
- Li, X. J., Hu, B. Y., Jones, S. A., Zhang, Y. S., Lavaute, T., Du, Z. W., & Zhang, S. C. (2008). Directed differentiation of ventral spinal progenitors and motor neurons from human embryonic stem cells by small molecules. Stem Cells , 26(4), 886–893. doi: 10.1634/stemcells.2007-0620.
- Lippmann, E. S., Williams, C. E., Ruhl, D. A., Estevez-Silva, M. C., Chapman, E. R., Coon, J. J., & Ashton, R. S. (2015). Deterministic HOX patterning in human pluripotent stem cell-derived neuroectoderm. Stem Cell Reports , 4(4), 632–644. doi: 10.1016/j.stemcr.2015.02.018.
- Mouilleau, V., Vaslin, C., Robert, R., Gribaudo, S., Nicolas, N., Jarrige, M., … Nedelec, S. (2021). Dynamic extrinsic pacing of the HOX clock in human axial progenitors controls motor neuron subtype specification. Development , 148(6), dev194514. doi: 10.1242/dev.194514.
- Peljto, M., Dasen, J. S., Mazzoni, E. O., Jessell, T. M., & Wichterle, H. (2010). Functional diversity of ESC-derived motor neuron subtypes revealed through intraspinal transplantation. Cell Stem Cell , 7(3), 355–366. doi: 10.1016/j.stem.2010.07.013.
- Sagner, A., & Briscoe, J. (2019). Establishing neuronal diversity in the spinal cord: A time and a place. Development , 146(22), dev182154. doi: 10.1242/dev.182154.
- Soundararajan, P., Miles, G. B., Rubin, L. L., Brownstone, R. M., & Rafuse, V. F. (2006). Motoneurons derived from embryonic stem cells express transcription factors and develop phenotypes characteristic of medial motor column neurons. Journal of Neuroscience , 26(12), 3256–3268. doi: 10.1523/JNEUROSCI.5537-05.2006.
- Turner, D. A., Rué, P., Mackenzie, J. P., Davies, E., & Martinez Arias, A. (2014). Brachyury cooperates with Wnt/β-catenin signalling to elicit primitive-streak-like behaviour in differentiating mouse embryonic stem cells. BMC Biology , 12(1), 63. doi: 10.1186/s12915-014-0063-7.
- Tzouanacou, E., Wegener, A., Wymeersch, F. J., Wilson, V., & Nicolas, J. F. (2009). Redefining the progression of lineage segregations during mammalian embryogenesis by clonal analysis. Developmental Cell , 17(3), 365–376. doi: 10.1016/j.devcel.2009.08.002.
- Verrier, L., Davidson, L., Gierliński, M., Dady, A., & Storey, K. G. (2018). Neural differentiation, selection and transcriptomic profiling of human neuromesodermal progenitor-like cells in vitro. Development , 145(16), dEv166215. doi: 10.1242/dev.166215.
- Wichterle, H., Lieberam, I., Porter, J. A., & Jessell, T. M. (2002). Directed differentiation of embryonic stem cells into motor neurons. Cell , 110(3), 385–397. doi: 10.1016/S0092-8674(02)00835-8.
- Wind, M., Gogolou, A., Manipur, I., Granata, I., Butler, L., Andrews, P. W., … Tsakiridis, A. (2021). Defining the signalling determinants of a posterior ventral spinal cord identity in human neuromesodermal progenitor derivatives. Development , 148(6), dev194415. doi: 10.1242/dev.194415.
- Wymeersch, F. J., Huang, Y., Blin, G., Cambray, N., Wilkie, R., Wong, F. C. K, & Wilson, V. (2016). Position-dependent plasticity of distinct progenitor types in the primitive streak. eLife , 5, e10042. doi: 10.7554/eLife.10042.
- Wymeersch, F. J., Wilson, V., & Tsakiridis, A. (2021). Understanding axial progenitor biology in vivo and in vitro. Development , 148(4), dev180612. doi: 10.1242/dev.180612.
Citing Literature
Number of times cited according to CrossRef: 4
- He Jax Xu, Yao Yao, Fenyong Yao, Jiehui Chen, Meishi Li, Xianfa Yang, Sheng Li, Fangru Lu, Ping Hu, Shuijin He, Guangdun Peng, Naihe Jing, Generation of functional posterior spinal motor neurons from hPSCs-derived human spinal cord neural progenitor cells, Cell Regeneration, 10.1186/s13619-023-00159-6, 12 , 1, (2023).
- Martta Häkli, Satu Jäntti, Tiina Joki, Lassi Sukki, Kaisa Tornberg, Katriina Aalto-Setälä, Pasi Kallio, Mari Pekkanen-Mattila, Susanna Narkilahti, Human Neurons Form Axon-Mediated Functional Connections with Human Cardiomyocytes in Compartmentalized Microfluidic Chip, International Journal of Molecular Sciences, 10.3390/ijms23063148, 23 , 6, (3148), (2022).
- Fay Cooper, Anestis Tsakiridis, Towards clinical applications of in vitro-derived axial progenitors, Developmental Biology, 10.1016/j.ydbio.2022.06.006, 489 , (110-117), (2022).
- Dosh Whye, Delaney Wood, Kristina H. Kim, Cidi Chen, Nina Makhortova, Mustafa Sahin, Elizabeth D. Buttermore, Dynamic 3D Combinatorial Generation of hPSC‐Derived Neuromesodermal Organoids With Diverse Regional and Cellular Identities, Current Protocols, 10.1002/cpz1.568, 2 , 10, (2022).