High-Resolution Multiparameter DNA Flow Cytometry for Accurate Ploidy Assessment and the Detection and Sorting of Tumor and Stromal Subpopulations from Paraffin-Embedded Tissues
Willem E. Corver, Willem E. Corver, Natalja T. ter Haar, Natalja T. ter Haar
carcinoma cells
DNA content and cell cycle analysis
DNA-diploid reference
FFPE tissues
molecular genetic analysis
Abstract
This article contains detailed protocols for the simultaneous flow cytometric identification of tumor cells and stromal cells and measurement of DNA content of formalin-fixed, paraffin-embedded (FFPE) tissues. The vimentin-positive stromal cell fraction can be used as an internal reference for accurate DNA content assessments of FFPE carcinoma tissues. This allows clear detection of keratin-positive tumor cells with a DNA index lower than 1.0 (near-haploidy) and of keratin-positive tumor cells with a DNA index close to 1.0 in overall DNA aneuploid samples, thus improving DNA ploidy assessment in FFPE carcinomas. Furthermore, the protocol is useful for studying molecular genetic alterations and intratumor heterogeneity in archival FFPE samples. Keratin-positive tumor cell fractions can be sorted for further molecular genetic analysis, while DNA from the sorted vimentin-positive stromal cells can serve as a reference when normal tissue of the patient is not available. © 2023 The Authors. Current Protocols published by Wiley Periodicals LLC.
Basic Protocol : Multiparameter DNA content analysis of FFPE carcinomas
Alternate Protocol 1 : Immunocytochemistry for keratin and vimentin, and DNA labeling for blue and red excitation
Alternate Protocol 2 : Immunocytochemistry for keratin and vimentin, and DNA labeling for blue excitation
Support Protocol : Sorting cell population from FFPE carcinomas
INTRODUCTION
Accurate DNA ploidy assessments and molecular genetic analysis of formalin-fixed, paraffin-embedded (FFPE) tissue can be hampered by a low percentage of tumor cells in the sample or by lack of normal tissue from the same patient used as reference. The exploitation of the normal stromal cell fraction from the same tissue block would then be favorable and could save precious clinical material.
As an alternative to tryptic or pepsin proteolysis of FFPE tissues after heat-induced antigen-retrieval (HIAR), a method is presented based on dissociation by a mixture of collagenase and dispase (Corver et al., 2005). This procedure allows the simultaneous immunophenotypic identification of tumor stromal cells and carcinoma cells and labeling of DNA for ploidy measurements and sorting of tumor (sub)populations. The method has been successfully applied to study DNA ploidy and molecular genetic alterations in tumor cells derived from archival FFPE gastric (Carvalho et al., 2005), cervical (de Boer et al., 2007; Kloth et al., 2008), colon (Bolognesi et al., 2016; Middeldorp et al., 2008), lung (Bolognesi et al., 2016), breast (Dayal et al., 2013), and thyroid, parathyroid, and adrenocortical cancer samples (Corver et al., 2018; Corver et al., 2012; Corver et al., 2014), and has several advantages:
- 1.The vimentin-positive stromal cell fraction can be used as an internal DNA diploid reference (Corver et al., 2011; Qiu et al., 2008) for ploidy measurements of FFPE carcinomas. For example, this allows plain identification of tumor cells with a DNA index lower than 1.0, e.g., near-haploidy, in FFPE samples without the aid of normal cells from another sample of the same patient (Corver et al., 2018; Corver et al., 2012; Corver et al., 2014).
- 2.By using appropriate anti-keratin antibodies, carcinoma cells can be readily identified, thereby improving S-phase estimates especially in tumors with a DNA index close to 1.0. Even in FFPE breast cancer samples, it was shown that a high S-phase fraction was independently associated with a poor overall survival (Dayal et al., 2013). Furthermore, tumor cells can be detected with a DNA index close to 1.0 in overall aneuploid samples (Bonsing et al., 2000).
- 3.The keratin-positive carcinoma cells and vimentin-positive stromal cells can be sorted for further molecular genetic studies. This is of particular value for studying the clonal relationship between tumor (sub)populations and tumor evolution in a sample by loss of heterozygosity (LOH) and copy number abnormalities (CNA) using SNP arrays (Corver et al., 2008; Corver et al., 2018; van den Tillaart et al., 2013) or other molecular-genetic techniques when a normal sample from the patient is not available (Qiu et al., 2008). In addition, samples can be used with low percentages of tumor cells (Bolognesi et al., 2016). Too often, studies on molecular genetic analysis of tumors are biased since only clinical samples that contain more than 70% tumor cells are selected.
- 4.Vimentin co-expression by carcinoma cells can be quantified in FFPE tissues by flow cytometry, and can be associated with a more aggressive tumor phenotype (Hendrix et al., 1997).
It is highly recommended to first read the Commentary at the end of this article. The simultaneous detection of RPE and PI fluorescence (used for DNA cell cycle analysis) is especially critical and requires dedicated instrumental settings.
STRATEGIC PLANNING
The working up of FFPE tissues to cell suspensions suitable for multiparameter DNA flow cytometry includes different, partially overlapping processing methods. A simplified procedure is presented by pictograms in Figure 1. Tissue cores (punches) of 2 mm can be punched from an area of interest in order to enrich tumor cells. These cores can be embedded, and 60-µm tissue sections can be taken or taken directly from the tissue block. Next, manual dissociation or dissociation with a device can be chosen. Optimal results must be obtained empirically. In our hands, the manual methods using nylon biopsy bags work best for cervical carcinomas, but for colon carcinomas we prefer the gentleMACSTM dissociation device. The working up of the cell suspensions (immunofluorescent labeling) is independent of the selected dissociation method. Finally, cells can be analyzed using a flow cytometer or imager and sorted using a fluorescence activated cell sorter (FACS) or image-based cell sorter when required.
Basic Protocol: MULTIPARAMETER DNA CONTENT ANALYSIS OF FFPE CARCINOMAS
The first part of this protocol describes the generation of cell suspensions from FFPE carcinoma tissue blocks. It is an alternative to earlier HIAR and pepsin- or trypsin-based protocols. Instead, cells are obtained after pretreatment of thick FFPE tissue rolls by HIAR, followed by dissociation with an enzyme mixture composed of collagenase Ia and dispase. An enrichment step by taking FFPE cores of preselected tissue area can be applied to samples with low percentages of tumor cells. Dissociation with collagenase/dispase is relatively mild, and cellular morphological characteristics are maintained. Two dissociation procedures are described: one makes use of nylon biopsy bags allowing the cells to be isolated via a centrifugation step; but a second procedure is described using the gentleMACSTM, allowing mechanical disruption of the predigested tissue followed by cell isolation (see also Strategic Planning and Fig. 1 at the end of this article). In addition to epithelial (carcinoma) cells, stromal cells can be detected after this dissociation procedure. These cells can be used as reference for diverse techniques, including accurate ploidy measurements of FFPE tissues and detection of chromosomal aberrations.
In turn, the second part of the protocol is specifically designed for the simultaneous labeling and measurement of epithelial cells (keratin-positive, FITC fluorescence), stromal cells (vimentin-positive, RPE or APC or Alexa FluorTM 647 (AF647) fluorescence), and DNA content (DAPI or PI fluorescence) using a flow cytometer equipped with ultraviolet (UV; 355 nm), violet (violet; 405 nm), and/or blue (488 nm) and red (633 nm) excitation lasers (see as examples Figs. 4 to 8). It has the advantage over the alternate protocols in that hardly any correction for spectral spillover of the DNA dye (DAPI) is necessary (Fig. 4 and 6). The spectral spillover of PI into the FITC or Alexa FluorTM 488 and APC or AF647 emission spectrum is limited (Fig. 7 and 8). However, other dye combinations can also be used (see Alternate Protocols 1 and 2, and Background Information) depending on the configuration of the flow cytometer, cell sorter, or image-based sorter (Bolognesi et al., 2016).
The third part of this protocol demonstrates how to measure the keratin, vimentin, and DNA-labeled cell suspensions, and how to calculate the DNA index of the samples.
Materials
-
Formalin-fixed, paraffin-embedded tissue sample (carcinoma), H&E-stained: see Hofman & Taylor (2013) and Zeller (1989)
-
100% xylene (e.g., Merck, Sigma-Aldrich, cat. no. 108684, CAS 106-42-3)
-
100% (v/v), 70% (v/v), and 50% (v/v) ethanol in distilled water
-
Milli-Q water (instrument for highly purified water can be obtained from Veolia or Merck)
-
Heat-induced antigen-retrieval (HIAR) buffer (see recipe)
-
Phosphate-buffered saline (PBS; see recipe)
-
RPMI 1640 medium (can be obtained ready-to-use from: e.g., Invitrogen Life Sciences, (http://www.invitrogen.com/site/us/en/home.html; cat. no. 11875093)
-
Dissociation solution (see recipe)
-
Hematoxylin, ready-to-use (e.g., Merck, cat. no. 51275)
-
Crushed ice (ice making machine, MRC Laboratory Instruments, cat. no. XB-30)
-
PBS/BSA/Tween (PBATw buffer; see recipe)
-
Primary reagents (see recipe):
- anti-keratin clones AE1/AE3 IgG1 (ThermoFisher Scientific, cat. no. A500-019A)
- anti-keratin clone MNF116 IgG1 (Agilent, Dako, cat. no. M082101)
- anti-vimentin clone Vim 3B4 IgG2a (Agilent, Dako, cat. no. M702001) or
- anti-vimentin clone V9-2b IgG2b (http://www.abforresearch.nl/new2/catalog/moabs/V9-2B.htm; cat. no. ARA27)
-
Secondary reagents (see recipe):
- goat anti–mouse IgG1-FITC (e.g., SouthernBiotech cat. no. 1070-02) or
- goat anti–mouse IgG1-Alexa FluorTM 488 (ThermoFisher Scientific, cat. no. A-21121
- goat anti–mouse IgG2a-RPE (e.g., SouthernBiotech cat. no. 1082-09 or
- goat anti–mouse IgG2b-RPE, e.g., SouthernBiotech cat. no. 1092-09)
-
DNA staining solution containing 4′,6-diamidino-2-phenylindole (DAPI; Merck cat. no. 508741, CAS 28718-90-3 (see recipe)
-
DNA staining solution containing propidium iodide (PI) and RNase A (see recipe)
-
Nylon biopsy bags EprediaTM (31 × 43–mm, normally used for fixing and dehydration of biopsies; e.g., Fisher Scientific, cat. no. 11932355
-
Pair of tweezers (steel)
-
An ordinary cigarette lighter
-
2.0 mm punch set for the Beecher tissue arrayer, AlphaMetrix Biotech, cat. no. MP20
-
Tissue arrayer, AlphaMetrix Biotech, cat.no. MTA-1
-
gentleMACSTM, Miltenyi Biotec, cat. no. 130-093-235
-
gentleMACSTM C Tubes (Miltenyi Biotec, cat. no. 130-093-237)
-
Microtome, e.g., Leica BioSystems, HistoCore BIOCUT, cat. no. 149BIO000C1, blades cat. no. 3802112
-
pH meter, e.g., HANNA Instruments
-
13 × 100–mm Pyrex (glass) disposable culture tubes, capacity 10.0 ml, e.g., Merck, Corning, cat. no. CLS9944513
-
Tube racks (e.g., FisherbrandTM DelrinTM, cat. no. 15340380, 15340390 and 15340400)
-
Any safety cabinet with an air-controlled hood
-
Nitrile examination gloves, powder free, e.g., FisherbrandTM¸ ThermoFisher Scientific, cat. no. 15642367 (medium, number is glove-size dependent)
-
80°C and 37°C water baths, e.g., PURA 4, VWR, cat. no. 462-0625P
-
Vortex, e.g., MS 3 basic IKA, cat. no. 0003617000
-
1.5-ml microcentrifuge tubes e.g., Merck, Eppendorf Biotech, cat. no. Z606340
-
BrandTM Bürker (or equivalent) cell count chamber, Fisher Scientific, cat. no. 10513451
-
Standard microscope e.g., Zeiss Primovert Tissue Microscope
-
12 × 75–mm (5 ml, FalconTM, e.g., Scientific Lab Supplies, cat. no. 352052)
-
CellTrics 50-μm and 100-μm nylon mesh filters (e.g., Sysmex or equivalent, cat. no. 04-004-2317 and cat. no. 04-004-2318, respectively)
-
Centrifuge with cooling device (maximum g needed: 1000 × g), e.g., Heraeus CryofugeTM 5500i
-
Micropipettes (range 1- to 1000-μl, Eppendorf Bioteck or LabSystems or equivalent)
-
Microtips (1-2 μl, 1-10 μl, 10-200 μl, 100-1000 μl (Eppendorf Bioteck, or LabSystems, or Greiner Bio-ONE or equivalent)
-
Conventional refrigerator (2°C to 8°C)
-
Flow cytometer equipped with UV (355 nm) or violet (405 nm), blue (488 nm) and red (633 nm) excitation and filters for collection of blue (BP 450/50 nm), green (BP 530/30 nm), orange (BP 575/26 nm) or red (610/20 nm), and infra-red (BP 670/14 nm, or >670 nm) fluorescence. Filter configurations can vary between different types of instruments.
-
Imaging device capable of collecting blue (DAPI), green (FITC or Alexa FluorTM 488) and orange (RPE) fluorescence. (e.g., DEPArrayTM PLUS, Menarini Silicon Biosystems (sorting capabilities) or the NucleoCounter® NC3000TM, ChemoMetec (imaging only)
-
Additional reagents and equipment for immunohistochemistry (see Current Protocols articles Hofman & Taylor, 2013; Zeller, 1989)
IMPORTANT NOTE: For imaging cell suspensions stained for DNA, keratin, and vimentin, the following combination of fluorescent dyes is recommended: DAPI (DNA), Alexa FluorTM 488 (keratin), and Alexa FluorTM 647 (vimentin). Labels for keratin and vimentin can be interchanged. The fluorescent dyes FITC and RPE (also accounts for APC) are more sensitive to fading due to the longer exposure times used for acquisition of images compared to flow cytometers.
Prepare cell suspensions from FFPE carcinomas
There are two alternative procedures for doing this, both of which are described in the steps below: one involving use of a nylon biopsy bag for containing the sample during treatment and the other involving the use of the gentleMACSTM instrument and C-Tubes from Miltenyi Biotec. For the gentleMACSTM procedure, skip step 1a and b and carry out the dissociation in C-Tubes as in the instructions below. The following instructions will focus on the nylon bags; see variations for C-Tubes in step 3.
1.Prior to tissue sectioning, prepare a nylon biopsy bag.
- Obtain a nylon biopsy bag.
Biopsy bags are obtainable from different companies. Bags larger than 3 × 5 cm need to be resized (next step of this protocol), or, depending on the size (large bags), cut in half. Keep the width of the bag wider than the diameter of the glass tube. This way, the bag can be fixed at the bottom of the tube, allowing easy handling of fluids (see Fig.2at the end of this article for detailed instructions).
-
If necessary, cut away superfluous nylon using a pair of scissors. With a pair of tweezers, tightly close an open boundary of the nylon bag keeping the legs of the tweezers parallel to the opening. Let a few millimeters of the open boundary stick out. Light the cigarette lighter and melt the nylon with a small flame (Fig.2).
2.Prepare tissue cores prior to sectioning (Fig. 3 at the end of the article).
-
When taking whole sections instead of tissue cores from the tissue block, go to step 3, substep b.
-
To take the core, have an experienced pathologist select the area of interest of the H&E-stained FFPE tissue section with a waterproof marker using a light microscope (Fig.3).
-
Prepare a clean recipient paraffin block and drill 2.0-mm-diameter cores (number of cores depends on the number of tissue cores that will be taken) using the red recipient puncher by simultaneously punching and swinging the puncher sideways.
See Hofman & Taylor (2013) and Zeller (1989) for basic immunohistochemical procedures.
-
Orient the marked-up H&E-stained section in such a way that the section matches the contours of the corresponding tissue in the paraffin tissue block.
-
Copy the boundaries of the marked-up area of H&E-stained section on the tissue block using a permanent marker.
-
Using a tissue arrayer, punch 2.0-mm-diameter tissue cores (blue donor punch, Fig.3) within the marked-up area (number of cores needed will depend on the depth and type of the tissue and must be empirically explored).
-
Transfer the punches to the paraffin recipient block containing the 2.0-mm-diameter pre-drilled empty cores.
-
Store the block overnight at 37°C.
This way the punches will be properly fixed into the recipient paraffin.
3.Cut FFPE tissue sections, collect tissue, and deparaffinize.
-
If using the gentleMACSTM, collect the sections directly into C-Tubes, put the tubes in a tube rack, and go to step 3, substep f. If not, when using nylon biopsy bags, start from substep b, below.
-
If using nylon biopsy bags, carefully collect the 60-μm sections (paraffin rolls) in the nylon bag via the last opening.
-
Close the nylon bag as described in step 1, substep b. (see also Fig.2).
CAUTION: Do not heat up the tissue roll.
-
Put the bag containing the tissue roll(s) at the bottom of a clean 10.0-ml Pyrex glass disposable culture tube(s).
-
Put the tube(s) in a tube rack and place the tube rack containing the tube(s) in a safety cabinet with an air-controlled hood.
-
Add 5.0 ml of 100% xylene to the glass tube(s) until the nylon bag with the tissue roll(s) is completely immersed. If using C-Tubes, add 5.0 ml of 100% xylene to the C-Tube(s) with the section(s).
-
Let stand for 10 min at room temperature in the safety cabinet.
-
Discard the xylene, re-immerse the sections in the tube(s) or the bag completely in fresh xylene, and let stand for another 10 min at room temperature.
-
Repeat step 3, substep h.
CAUTION: Xylene is harmful and can cause irritation, e.g., of the skin and eyes. Long exposure can cause neurological damage. Wear protective nitrile examination gloves. Xylene is also harmful to the environment. It must be collected in special containers for organic solvents and should not be discarded down the sink.
- Discard the 100% xylene and rehydrate the sample by sequential incubation in each of the following:100% ethanol, three 5-min changes70% ethanol, three 5-min changes50% ethanol, three 5-min changes Milli-Q water, once for 5 min.
Do not allow the tissue roll(s) to dry. Always re-immerse the bag completely for each sequential incubation. This also applies for the following steps.
-
Substitute the Milli-Q water with HIAR buffer, pH 6.4, at room temperature. Immerse the bag completely.
-
Refresh the HIAR buffer after 5 min with prewarmed HIAR buffer (80°C) and place the sample tube in an 80°C water bath for 60 min.
-
Take out the tube containing the sample bag from the 80°C water bath and let it cool down to room temperature.
This will take ∼20 to 30 min.
-
Wash the sample twice, each time for 5 min with PBS.
-
Replace the PBS with plain RPMI 1640 medium, pH 7.6.
-
After 5 min, replace RPMI 1640 medium with prewarmed dissociation solution. Ensure that the nylon bag containing the sample is completely immersed in the RPMI-enzyme mixture.
-
Incubate the sample 30 to 120 min in a 37°C water bath.For nylon bags: Monitor the dissociation process by swirling the sample on a vortex every 15 min until the tissue section(s) disintegrate. For C-Tubes: Put the C-Tube(s) in the gentleMACSTMafter 15 min of incubation. Select program 3.2: SPLEEN followed by RUN (16 sec). Repeat the dissociation process every 15 min until the tissue section(s) are disintegrated.
The solution will get cloudy. This is indicative of cell release. Optimal dissociation is tissue- (type-), time-, and temperature-dependent (Corver et al.,2005). Do not completely digest the sample, as this might induce bare nuclei and debris. Some optimization might be needed
-
Monitor dissociation more precisely as follows:Take a small aliquot from the sample (10 μl), put it in a 1.5-ml microcentrifuge tube, and add 40 μl of hematoxylin.Mix well and count the number of cells (nuclei will be stained dark blue) using a Bürker counter chamber and a standard microscope.
-
Stop the dissociation process by putting the sample on ice as soon as the cell numbers of two consecutive 15-min counts are approximately identical.
-
Transfer the cells through a 100-μm mesh nylon filter into a FACS tube (12 × 75–mm) and spin down the cells by centrifuging 5 min at 1000 ×g, using a pre-cooled centrifuge (4°C).
-
Wash the pellet twice with 2 ml ice-cold PBATw (4°C), and spin down 5 min at 1000 ×g, using a pre-cooled centrifuge (4°C). Discard the supernatant after each wash.
-
Add 2 ml of ice-cold PBATw to the cell pellet, vortex, take a 10-μl sample, and put it in a 1.5-ml microcentrifuge tube. Dilute cells 1:5 in hematoxylin and count cells using a Bürker counter chamber and a standard microscope.
Perform immunocytochemistry for keratin and vimentin, and DNA labeling for UV or violet and blue excitation
4.Take four 12 × 75–mm tubes, pipet 1 × 106 cells in each, and spin cells down by centrifuging 5 min at 1000 × g , 4°C.
Tube # | Primary reagent | Secondary reagent | DNA staina |
---|---|---|---|
1 | Staining buffer | GaMIgG1-FITC/GaMIgG2b-RPE | DAPI |
2 | Anti-keratin | GaMIgG1-FITC/GaMIgG2b-RPE | DAPI |
3 | Anti-vimentin | GaMIgG1-FITC/GaMIgG2b-RPE | DAPI |
4 | Anti-keratin/anti-vimentin | GaMIgG1-FITC/GaMIgG2b-RPE | DAPI |
- a
Requires a flow cytometer equipped with UV (355 nm) or violet (405 nm) and blue (488 nm) excitation; for alternative DNA stains, see Alternate Protocols 1 and 2. GaM = Goat anti-mouse; FITC = fluorescein isothiocyanate; RPE = R-phycoerythrin; DAPI = 4′,6-diamidino-2-phenylindole.
5.According to the scheme, add 100 μl of primary reagent (or irrelevant isotype control or buffer), vortex gently until the pellet is completely in solution, and incubate overnight at 4°C in PBATw.
6.The next day, wash twice, each time with 1 ml ice-cold PBATw, centrifuging 5 min at 1000 × g , 4°C, and removing the supernatant after each wash.
7.Then, add 100 μl of secondary reagents (mixture of GaMIgG1-FITC and GaMIgG2b-RPE) to the pellets and gently vortex until the pellet is completely dissolved (± 5 s). Place tubes on ice.
8.After 30 min on ice, wash twice, each time with 1 ml PBATw, centrifuging 5 min at 1000 × g , 4°C, and removing the supernatant after each wash.
9.Add 0.5 ml DNA staining solution containing DAPI to the cell pellets and vortex gently until the pellet is completely dissolved (± 5 s).
10.Store in the dark for at least 2 hr before analysis.
11.To prevent clogging of the flow cytometer, filter all samples over a nylon mesh of 50-μm before acquisition.
Perform multiparameter DNA measurement of cell suspensions from FFPE carcinomas
12.For data acquisition and sorting, design a layout displaying the following bivariate dot plots and single-parameter histograms as follows (see Fig. 4 at the end of this article as an example):
Bivariate dot plots
- a. FSC (lin) vs. SSC (lin).
- b. 355 DNA-DAPI 450/50-A (lin) or Height (lin) vs. 355 DNA-DAPI 450/50-A (lin).
This dot plot is used to gate on single cells.
- c. 355 DNA-DAPI 450/50-A (lin) vs. green (FITC) fluorescence (biexponential).
- d. 355 DNA-DAPI 450/50-A (lin) vs. orange (RPE) fluorescence (biexponential).
- e. Green (FITC) fluorescence (biexponential) vs. orange (RPE) fluorescence (biexponential).
Single-parameter histograms
- f. Green (FITC) fluorescence (biexponential).
- g. Orange (RPE) fluorescence (biexponential).
- h. 2 × 355 DNA-DAPI 450/50-A (lin) for ungated and gated events.
13.Acquire data from the sample tube stained with DAPI alone.
14.Place a threshold on the FSC (i.e., 5000 at 256 × 100 channel resolution) in combination with a threshold on the DAPI detector.
15.Adjust the PMT settings of the DAPI fluorescence detector until the first G0G1 peak is approximately positioned at one fifth of the linear scale (i.e., channel 50,000 at 256 × 100 channel resolution).
16.Create an electronic region/gate R1 around the G0G1 peak and color-code this gate in the FSC–SSC dot plot.
17.Adjust the FSC settings until all colored events show up to the right of the FSC threshold.
18.Draw a second electronic region/gate R2 around the single-cell events of the 355 DNA-DAPI 450/50-A or Height versus 355 DNA-DAPI 450/50-A bivariate dot plot and activate this region/gate R2 in the other dot plots and histograms.
19.Adjust PMT settings. A log-normal distribution must be shown in the first log-decade of the 4-decade FITC (488 530/30-A) or RPE (488 575/26-A) detector.
20.Take the vimentin-positive sample (RPE and DAPI fluorescence) and adjust for RPE spillover into the FITC and DAPI fluorescence detectors, respectively.
21.Repeat the same action for the keratin-positive sample (FITC fluorescence emission) and adjust for FITC spillover into the RPE and DAPI fluorescence detectors, respectively.
22.The flow cytometer is now ready to acquire and store events for multiparameter DNA analysis of samples labeled with DAPI, FITC, and RPE (Fig. 4, M-P).
23.For S-phase estimates, it is recommended to stop acquisition only when >20,000 single keratin-positive events are collected within R2 when using a 1024-channel resolution scale for data analysis (Brown et al., 1996). Higher data analysis resolution settings are not useful.
24.Store the data in the computer.
Perform immunophenotypic analysis of flow cytometry data
25.For data analysis, design a layout displaying the following bivariate dot plots and single-parameter histograms:
Bivariate dot plots
- a. FSC (lin) versus SSC (lin).
- b. 355 DNA-DAPI 450/50-A (lin) or Height (lin) versus 355 DNA-DAPI 450/50-A (lin).
This dot plot is used to gate on single cells.
- c. 355 DNA-DAPI 450/50-A (lin) versus green FITC fluorescence (biexponential) (488 FITC 530/30-A).
- d. 355 DNA-DAPI 450/50 A (lin) versus orange (RPE) fluorescence (biexponential) (488 RPE 575/26-A).
- e. Green (FITC) fluorescence (biexponential) (488 FITC 530/30-A) vs. orange (RPE) fluorescence (biexponential) (488 RPE 575/26-A).Single-parameter histograms:
- f. Green (FITC) fluorescence (biexponential) (488 FITC 530/30-A).
- g. Orange (RPE) fluorescence (biexponential) (488 RPE 575/26-A).
- h. Three 355 DNA-DAPI 450/50 A (lin) histograms [one ungated and two gated histograms for showing the cell cycle distribution of the vimentin-positive stromal cell fraction and of the keratin-positive cell fraction(s), respectively].
26.Open the data file of the DAPI-labeled cells only and create an analysis region R1 around the single events excluding debris and cell aggregates using the 355 DNA-DAPI 450/50 A (lin) or Height (lin) versus 355 DNA-DAPI 450/50 A (lin) bivariate dot plot.
27.Activate R1 in the following bivariate dot plots and single parameter histograms:
-
355 DNA-DAPI 450/50 A (lin) versus green (FITC) fluorescence (biexponential) (488 FITC 530/30-A).
-
355 DNA-DAPI 450/50 A (lin) versus orange (RPE) fluorescence (biexponential) (488 RPE 575/26-A).
-
Green (FITC) fluorescence (biexponential) (488 FITC 530/30-A) versus orange (RPE) fluorescence (biexponential) (488 RPE 575/26-A).
-
Green (FITC) fluorescence (biexponential) (488 FITC 530/30-A).
-
Orange (RPE) fluorescence (biexponential) (488 RPE 575/26-A).
-
Three 355 DNA-DAPI 450/50 A (lin) histograms.
28.Next, open the data file of the vimentin-positive control.
29.Adjust the spectral spillover of RPE fluorescence in the DAPI fluorescence parameter until the vimentin-positive events are parallel to the ordinate of the 355 DNA-DAPI 450/50 A (lin) versus red (RPE) fluorescence (log) dot plot.
30.Create a region R2 around the vimentin-positive events and activate this gate in the first 355 DNA-DAPI 450/50 A (lin) histogram.
31.Open the data file of the keratin-positive control and, when necessary, correct for spectral spillover of FITC into the RPE and DAPI parameter.
32.Create a region R3 around the keratin-positive events and activate this gate in the second 355 DNA-DAPI 450/50 A (lin) histogram.
33.Finally, load the data file containing the triply stained cells and make small final spectral spillover adjustments when required.
34.Create a region R4 around the G0G1 population of the DNA histogram composed of the vimentin-positive events. Calculate the median fluorescence using the software.
35.Create a region R5 around the G0G1 population of the DNA histogram composed of the keratin-positive events. Calculate the median fluorescence using the software.
36.Do the same for other G0G1 populations when present in the keratin-positive population.
WinList script “Edit Remote Link”
- POKE (DATA, VSH)
- POKE (KEYWORDS, VSH, KEYWORDS)
- [SetProperty(ExternalReference={DS1.R4.MedianX},262144)]
When another region is used to define the G0G1 of the DNA-diploid internal vimentin-positive, keratin-negative stromal reference R4 must be changed accordingly.
- [AutoAnalysis()]
- [AutoLinearity()]
- [Update()]
- [SetGraphicSize({HISTOGRAMSIZE})]
- [Copy('Graph')]
- PASTE(DIB)
- [Copy('Results')]
- PASTE(Text)
Alternate Protocol 1: IMMUNOCYTOCHEMISTRY FOR KERATIN AND VIMENTIN, AND DNA LABELING FOR BLUE AND RED EXCITATION
Most modern standard equipped flow cytometers, e.g., FACSCanto, CytoFlex, or NovoCyte, permit violet, blue, and red excitation, and assist the use of DAPI as DNA stain. However, some instruments, especially older models, e.g., FACSCalibur, do not support the use of DAPI. A good alternative for DAPI is propidium iodide (PI). However, PI, in contrast to DAPI, is not base-pair specific and requires RNase treatment in order to reduce binding of PI to dsRNA. A cell population left untreated will show a significantly higher coefficient of variation (CV) of the G0G1 peak as compared to the G0G1 peak of an RNase-treated cell population, stained with PI.
The following protocol is identical to the Basic Protocol, except for steps 7 and 9. GaMIgG2b-RPE or GaMIgG2a-RPE is replaced by GaMIgG2a-APC or GaMIgG2b-APC, DNA is labeled by PI, and cells need to be treated by RNase, respectively.
Additional Materials (also see Basic Protocol)
- Secondary reagents:
- Goat anti–mouse IgG2a-RPE or goat anti–mouse IgG2b-RPE are replaced by:
- goat anti–mouse IgG2a-APC (e.g., SouthernBiotech, cat. no. 1080-11)
- goat anti–mouse IgG2a-Alexa FluorTM 647, ThermoFisher Scientific, cat. no. A-21241
- goat anti–mouse IgG2b-APC (e.g., SouthernBiotech, cat. no. 1090-11)
- goat anti–mouse IgG2b- Alexa FluorTM 647 (e.g., ThermoFisher Scientific, cat. no. A-21242)
- DNA staining solution with PI (see recipe) is used instead of the DAPI staining solution.
- Flow cytometer equipped with a blue 488-nm laser and red 633-nm laser and optical filters for collection of green (FITC or and Alexa FluorTM 488), red (PI), and infra-red (APC or and Alexa FluorTM 647) fluorescence equipped with the following optical filters: BP 530/30 nm, BP 610/20 nm or LP 670 nm, and BP 670/14 nm.
- Imager suitable for the collection of FITC or Alexa FluorTM 488, PI and APC, or Alexa FluorTM 647 fluorescence and corresponding optical filters
Prepare cell suspensions from FFPE tissues
1.Prepare cell suspension from FFPE tissues as described in the Basic Protocol (steps 1 to 3).
Cell staining
2.Follow steps 4 to 11 of the Basic Protocol.
3.Add 100 μl of secondary reagents (mixture of GaMIgG1-FITC or GaMIgG1-Alexa FluorTM 488 and GaMIgG2b-APC) to the pellets and gently vortex until the pellet is completely dissolved (±5 s). Place tubes on ice.
4.After 30 min on ice, wash twice, each time with 1 ml PBATw, centrifuging 5 min at 1000 × g , 4°C, and removing the supernatant after each wash.
5.Add 0.5 ml DNA staining solution with PI to each of the cell pellets and vortex gently. Put the tubes for 30 min in a 37°C water bath.
6.Store in the dark at 2°C to 8°C (refrigerator) for at least 2 hr before analysis.
7.To prevent clogging of the flow cytometer, filter all samples over a 50-μm nylon mesh before acquisition.
Perform multiparameter DNA measurement of cell suspensions from FFPE carcinomas and flow cytometry data analysis
Measurement and data analysis are identical to the Basic Protocol except for the parameters used to analyze the stromal cell fraction (vimentin-positive, APC or fluorescence or Alexa FluorTM 647 fluorescence) and the DNA content (PI fluorescence).
8.Follow steps 12 to 24 of the Basic Protocol, but replace the orange (488 RPE 575/26-A) fluorescence (biexponential) and the 355 DAPI-DNA 450/50-A (lin) parameters by the red (633 APC or Alexa FluorTM 647 670/14-A) fluorescence (biexponential) and the 488 DNA-PI 610/20-A (lin) or 488 DNA-PI 670-A (lin) parameters, respectively.
Alternate Protocol 2: IMMUNOCYTOCHEMISTRY FOR KERATIN AND VIMENTIN, AND DNA LABELING FOR BLUE EXCITATION ONLY
Relatively old standard benchtop flow cytometers, e.g., the Coulter XL or FACSCan, are only equipped with a blue (488 nm) laser. Single-laser excitation limits the choice for optimal dye combinations. However, despite the broad emission spectrum of PI, results comparable to those achievable with UV (DAPI) and blue excitation (FITC and RPE, Basic Protocol) can be obtained (see Fig. 5, 6 at the end of this article). Special detector and compensation settings are required in order to separate the fluorescent signals from RPE and PI (see Commentary and Corver et al., 1994), but the standard optical filters used to detect FITC, RPE, and PI fluorescence do not have to be changed for the FACScan or FACSCalibur flow cytometers. The following protocol is identical to the Basic Protocol, except for step 9 (staining DNA with DAPI). Instead of using DAPI to stain DNA, DNA is labeled by PI and cells need to be treated by RNase.
Additional Materials (also see Basic Protocol)
- DNA staining solution containing PI (see recipe) instead of DAPI
- Flow cytometer equipped with blue (488 nm) excitation and filters for collection of green (FITC), orange (RPE) and red (PI) fluorescence
Prepare cell suspensions from FFPE tissues
1.Prepare cell suspension from FFPE tissues as described in the Basic Protocol (steps 1 to 3).
Cell staining
2.Follow steps 4 to 11 of the Basic Protocol.
3.Add 0.5 ml PI staining solution (containing RNase) to the cell pellets and vortex gently. Put the tubes for 30 min in a 37°C water bath.
4.Store in the dark (refrigerator) for at least 2 hr before analysis.
5.To prevent clogging of the flow cytometer, filter all samples over a 50-μm nylon mesh before acquisition.
Perform multiparameter DNA measurement of cell suspensions from FFPE carcinomas and flow cytometry data analysis
Measurement and data analysis are identical to the Basic Protocol except for the DNA content parameter (PI fluorescence).
6.Follow step 12 to 24 of the Basic Protocol but replace the 355 DAPI-DNA 450/50-A (lin) parameter by the 488 DNA-PI >650-A (lin) or 488 DNA-PI >670-A (lin), depending on flow cytometer parameters.
Support Protocol: SORTING CELL POPULATIONS FROM FFPE CARCINOMAS
Solid tumors are heterogeneous in their composition, and frequently multiple populations with differing cell DNA contents can be identified in the keratin-positive cell fraction. For example, it has been demonstrated that overall DNA aneuploid tumors also harbor tumor cells with a DNA index of 1.0 or close to 1.0 (Bonsing et al., 2000; Corver et al., 2008; Corver et al., 2011). These can be normal epithelial cells contaminating the sample, but are very frequently shown to be pseudo-diploid. These cells have harbored chromosomal alterations in which the total sum of copy number gains and losses shows a DNA content equal to that of normal DNA diploid cells (Abeln et al., 1994; Bonsing et al., 2000; Corver et al., 2008; Corver et al., 2011; see Fig. 7 at the end of this article as example). In addition, tumor subpopulations within a single G0G1 fraction defined by flow cytometry DNA cell content measurements have been identified. These can be based on differences in phenotype (Corver et al., 2000; Koopman et al., 2000; Corver et al., 2000a), but also on a mixture of subpopulations with slightly different genomic make-ups. The differences in DNA content are small and fall within the range of variation of a G0G1-peak. The latter is caused by chromosomal instability and mitotic errors, and has been demonstrated by sorting, copy number, LOH, and FISH analysis of the particular G0G1 population (Corver et al., 2018; Corver et al., 2012).
In order to reduce unintended cell loss during sorting, a four-way sorter is recommended. This allows the simultaneous sorting of the vimentin-positive stromal cell fraction, together with up to three tumor subpopulations, saving precious clinical material. For example, the sorting of two tumor subpopulations and normal stromal cells simultaneously would take more time and sample on a two-way cell sorter as compared to a four-way cell sorter (see as examples Fig. 6 and 7). Cell suspensions prepared from FFPE carcinomas by the presented protocol can also be sorted using a recently introduced imaging and digital-sorting device: the DEPArray (Menarini Silicon Biosystems, S.P.A., Italy). Small tumor samples can be applied (advantage over a cell sorter) and reliably allow detection of DNA variants in samples with a very low percentage of tumor cells, e.g., lung cancer, which would have been missed without sorting (Bolognesi et al., 2016).
The Support Protocol below is typically used to sort tumor subpopulations coexisting in FFPE carcinomas using the Basic Protocol after staining for keratin (FITC), vimentin (RPE), and DNA (DAPI). A sorter with UV (355 nm) and blue (488 nm) excitation lasers is required. Sorting of different cell fractions treated according to Alternate Protocol 1 and Alternate Protocol 2 demand blue (488 nm) and red (635 nm), and only blue (488) excitation, respectively.
Additional Materials (also see the Basic Protocol)
-
Commercial DNA purification kit (e.g., NucleoSpin, Macherey-Nagel, cat. no. 740980.50 or Promega Wizard genomic DNA Purification Kit, cat. no. A1120)
-
or (cheaper):
-
Proteinase K solution (see recipe in Reagents and Solutions)
-
1.5-ml microcentrifuge tubes
-
Flow cytometer instrument equipped with sorting capabilities (i.e., ability to simultaneously sort four different cell populations), e.g., InFlux, FACSAria or CytoFLEX SRT cell sorter, or DEPArray (Bolognesi et al., 2016). DEPArray set-up is outside the scope of this article.
-
Spectral sorters like the BigFoot (ThermoFisher), Aurora CS (Cytek Biosciences) or FACSDiscover S8 are not obligatory for cell purification of cell suspensions prepared by the presented protocol
Cell purification/sorting and DNA extraction
1.Follow the steps described for sample preparation and cell staining in Basic Protocol (steps 1 to 11).
2.Display dot plots and histograms as described in the Basic Protocol and follow steps 12 to 22 of the Basic Protocol.
3.Design a gating strategy to sort the stromal, vimentin-positive cell fraction and keratin-positive cell fraction.
4.Sort cells into 12 × 75–mm tubes or directly into 1.5-ml microcentrifuge tubes.
5.After sorting, spin cells down by centrifuging 10 min at 3000 × g , 4°C.
6.Carefully remove the supernatant.
7.Extract DNA using proteinase K (Abeln et al., 1994) at 56°C overnight or using a commercial kit, of which there are many on the market. For proteinase K digestion, use 1 μl proteinase K buffer per 2000 nuclei. When a commercial kit is used, follow the instructions of the manufacturer.
8.The following day, inactivate proteinase K for 10 min at 100°C.
REAGENTS AND SOLUTIONS
Use Milli-Q water for all recipes and protocol steps.
Dissociation solution
Dissolve 200 mg of dispase (e.g., Worthington, cat. no. LS02100) in 10 ml RPMI 1640 (e.g., ThermoFisher, cat. no. 11875093). Dissolve 200 mg of collagenase Ia (e.g., Merck, cat. no. C9891) in 10 ml RPMI 1640. Mix both solutions and store in 1-ml aliquots in the freezer (–20°C). Thaw a small aliquot before use and dilute to 10 × in RPMI 1640.
DNA staining solution with DAPI
Prepare a stock solution of 1.0 mM DAPI (4′-6-diamidino-2-phenylindole; e.g., Merck, cat. no. 508741, CAS 28718-90-3) in the following buffer:
- 146 mM NaCl (e.g., Merck, cat. no. S9888)
- 10 mM Tris base (e.g., Merck)
- 0.1% NP-40 (Sigma)
- 2 mM Ca (e.g., Merck, Sigma-Aldrich C5670)
- 20 mM Mg (e.g., Merck, Sigma-Aldrich C8266)
- 0.05% BSA, pH 7.41 (fraction V, Merck, cat. no. 107350)
Store the DAPI (100×) stock solution up to 6 months at 2°C to 8°C in the refrigerator. Before use, dilute to 1× in NST buffer [146 mM NaCl, 10 mM Tris base, 0.1% NP40 (Sigma), 2 mM Ca, 20 mM Mg, and 0.05% BSA, pH 7.41] (Glogovac et al., 1996).
DNA staining solution containing PI and RNase A
Propidium iodide (PI) stock solution
Dissolve 66.8 mg of propidium iodide (PI) (e.g., Merck, cat. no. 537059, CAS 25535-16-4, PI molar mass = 668.4) in 100 ml PBS (see recipe). Final concentration 1.0 mM. Divide into 10-ml aliquots and store up to 2 years in the freezer (–20°C). A PI stock solution in use can be stored in the refrigerator at 2°C to 8°C.
RNase stock solution
Dissolve 1.0 g of RNase A, Type I-A (e.g., Merck, Sigma-Aldrich, cat. no. R4875) in 100 ml PBATw (see recipe). Store the 1% stock solution as small aliquots of 1.0 ml in the freezer (–20°C).
PI/RNase working solution
- The following is for 10.0 ml of PI/RNase working solution. Volumes should be adjusted accordingly when another final volume is desired.
- Before use thaw a 1.0-ml aliquot of RNase stock solution (see above) and dilute (final 1/10 (v/v) by adding 8.9 ml PBATw (see recipe) and 100 μl 1.0 mM PI stock solution (see above). The final concentration of PI in the working solution will be 10 µM.
CAUTION: Take precautionary measures when working with DNA intercalating dyes. Wear gloves and use a facemask when weighing.
For optimal results, titrate the concentration of PI.
Heat-induced antigen retrieval (HIAR) buffer
- 0.1 M Na3C6H5O7⋅2H2O (tri-sodium citrate dihydrate stock solution; Merck, cat. no. 1064480)
- 0.1 M C6H8O7.H2O (citric acid monohydrate stock solution; Merck, cat. no. 100224)
Antigen retrieval buffer stock solution (10×): Under constant swirling, add 0.1 M citric acid to 100 ml 0.1 M sodium citrate until pH is 6.4. Store up to 6 months at 4°C. Dilute to 1× with Milli-Q water before use. The final pH should be 6.4. Adjust pH when necessary, with 1 M NaOH (Merck, cat. no. S5881) or 1 M HCl (Merck, cat. no. 110165).
PBATw
Dissolve 1.0 g bovine serum albumin (i.e., fraction V, Merck, cat. no. 107350) in 100 ml washing buffer (see recipe).Weigh the albumin and put it slowly on top of the PBSTw. Let the BSA slowly dissolve without swirling. Store up to 4 weeks at 4°C. Check the pH before use (7.2 - 7.4). Discard when pH is below 7.2 and prepare a new solution.
Tween 20
Dissolve 125 ml Tween 20 (e.g., Merck, cat. no. P1379) in 375 ml Milli-Q water. Store this 25% Tween 20 solution at 4°C
Phosphate-buffered saline (PBS), pH 7.5
NaCl 0.9% (w/v) (e.g., Merck, cat. no. S9888): weigh 18 g of NaCl and dissolve in 1900 ml Mill-Q water. When completely dissolved adjust volume to 2.0 L with Milli-Q water
Dissolve 249 mg Na2HPO4·2H2O (e.g., Merck, cat. no. 137036) in 1 liter of 0.9% (w/v) NaCl
Dissolve 191 mg KH2PO4 (e.g., Merck, cat. no. 105104) in 1 liter of 0.9% (w/v) NaCl
Under constant swirling, add KH2PO4/NaCl solution to 500 ml Na2HPO4 solution until the pH is 7.5
Store up to 1 month at 4°C
Primary reagents (monoclonal antibodies)
Anti-keratin antibodies (all mouse cat. no. IgG1):
- clone MNF116 (Agilent, Dako, cat. no. M082101)
- clones AE1/AE3 (Merck, Sigma-Aldrich cat. no. MAB3412)
Anti-vimentin antibodies (mouse IgG2a or IgG2b):
- clone 3B4 (IgG2a) (Agilent, Dako, cat. no.M702001) or
- clone V9-2b (Antibodies for Research Applications cat. no. ARA27 (available via http://www.abforresearch.nl/)
Antibodies are diluted in PBATw (see recipe)
Working concentrations: MNF116, 2.0 μg/ml; AE1/AE3, 5.0 μg/ml; 3B4, 2.0 μg/ml; V9-2B, 1% culture supernatant.
Proteinase K buffer
Dilute proteinase K (e.g., Promega, cat. no. MC5005, stock 20 mg/ml) 5× in 10 ml buffer containing 10 mM Tris·HCl, pH 8.3, 1.0 mM disodium EDTA, and 0.5% Tween 20. Store up to 6 months at –20°C.
Secondary reagents (polyclonal conjugated antibodies)
- Goat anti–mouse IgG1-FITC (e.g., SouthernBiotech cat. no. 1070-02) or
- Goat anti–mouse IgG1-Alexa FluorTM 488 (ThermoFisher Scientific, cat. no. A-21121)
- Goat anti–mouse IgG2a-RPE (e.g., SouthernBiotech cat. no. 1082-09) or
- Goat anti–mouse IgG2b-RPE (e.g., SouthernBiotech cat. no. 1092-09)
- Goat anti–mouse IgG2a-APC (e.g., SouthernBiotech, cat. no. 1080-11)
- Goat anti–mouse IgG2a-Alexa FluorTM 647 (ThermoFisher Scientific, cat. no. A-21241)
- Goat anti–mouse IgG2b-APC (e.g., SouthernBiotech, cat. no. 1090-11)
- Goat anti–mouse IgG2b- Alexa FluorTM 647 (e.g., ThermoFisher Scientific, cat. no. A-21242)
- Antibodies are diluted in PBATw
- Working concentrations are of 2.5 μg/ml. However, for result optimization antibodies need to be titrated.
Note that that Alexa FluorTM 488 or Alexa FluorTM 647 conjugated antibodies are alternatives for FITC and APC conjugated antibodies, respectively. Optical filter settings of a flow cytometer can be left unchanged. In addition, cell-sorting devices are relatively complex instruments, but fortunately they frequently come with many instrumental options. A wide pallet of fluorescent dyes can be detected by these instruments. It is therefore recommend to select dyes with minimal spectral cross-talk.
Secondary reagents are frequently available as F(ab′)2 fragments. These antibodies lack an Fc tail and frequently show less unwanted background staining than whole IgG antibodies. However, they come at higher costs.
Washing buffer
PBS (see recipe) containing 0.05% Tween 20 (PBSTw). Add 2000 μl of 25% Tween 20 to 1 L of PBS (see recipe). Store up to 1 month at 4°C.
COMMENTARY
Background Information
The measurement of the DNA content of neoplastic cells was one of the earliest applications of flow cytometry. In fact, the first commercial flow cytometer, the ICP 11 from Partec, was solely dedicated for this purpose. In the early 1980s, two major technical improvements for DNA cell content measurements by flow cytometry were published: the Vindelov method for fresh/frozen tissues (Vindelov et al., 1983a, 1983b; Vindelov, Christensen, Jensen et al., 1983; Vindelov, Christensen, Keiding et al., 1983) and the Hedley method for formalin-fixed paraffin-embedded (FFPE) tissues (Hedley et al., 1983).
The Vindelov method is based on a lysing-trypsin procedure that allows isolation and staining of bare nuclei of fresh or frozen samples excluding identification of protein molecules by monoclonal antibodies. Instead of a marker for normal cells, e.g., CD45 (Zarbo et al., 1989; Corver et al., 2000a; Koopman et al., 2000), internal standards with pre-established well-known amounts of DNA (i.e., chicken and/or trout erythrocytes) are used, allowing accurate measurement of the DNA content of tumor cells. With this method, cells with DNA content lower than that of normal cells can also be readily detected. Furthermore, high-quality DNA histograms with accurate S-phase estimates can be generated, encompassing relatively low percentages of debris.
In turn, the Hedley method is based on the isolation of nuclei from archived FFPE tissues after pepsin proteolysis, allowing retrospective studies. Both methods contributed greatly to the study of DNA ploidy and clinical behavior of solid tumors, which resulted in hundreds of papers (Corver & Cornelisse, 2002). However, in 1993 it was concluded that in practice, DNA content measurements had limited impact on clinical outcome and individual patient care (Hedley et al., 1993; Hedley et al., 1993). Despite some more recent publications (i.e., Bagwell et al. (2001), the clinical use of ploidy and S-phase measurements is restricted and for many kinds of tumors, still controversial.
The failure of DNA ploidy measurements as a widely applicable clinical tool was attributed to poor standardization, technical failures, and the very limited number of studies that had used a multi-parameter immuno-phenotyping approach (e.g., the use of anti-keratin and other tumor cell associated antibodies). It should also be noted that by convention carcinoma samples showing a uni-modal DNA histogram with a DNA index of 1.0 or close to 1.0 are annotated as DNA diploid (Hiddemann et al., 1984). However, from cytogenetic analysis it was known that these cells frequently show aneuploidy. Conclusively, these “DNA diploid” cells are pseudo-diploid, showing chromosomal copy number variants with a total sum of DNA identical, within the resolution of DNA content flow cytometry, to that of pure diploid cells.
Anti-keratin antibodies facilitate detection of carcinoma cells with a DNA index close to 1.0 in near-diploid, as well as in overall DNA aneuploid samples, and significantly contributes to improved S-phase estimates. Even in FFPE breast cancers, the S-phase showed to be an independent prognostic parameter measured by multiparameter DNA content flow cytometry and using the preparation procedure described in this article (Dayal et al., 2013). It was clearly demonstrated by cell sorting and subsequent molecular genetic analysis that these pseudo-diploid cells indeed show chromosomal copy-number variants, e.g., loss-of heterozygosity simultaneously with copy number gains (Abeln et al., 1994; Bonsing et al., 2000; Corver et al., 2008; Corver et al., 2011). Strikingly, these chromosomal errors could also frequently be found in the DNA aneuploid fraction of the same tumor. The aneuploid fraction progressed from the pseudo-diploid fraction after a tetraploidization step and including additional chromosomal alterations. This has also been nicely demonstrated in progression of Barett's esophagus (Barrett et al., 2003; Barrett et al., 1999; Merlo et al., 2010). The bimodal distribution of DNA indices in, e.g., breast cancer, also clearly support these observations (Devilee & Cornelisse, 1994).
Finally, an internal standard for FFPE tissues showed itself to be hard to achieve (Schultz & Zarbo, 1992). This was due to the fixation of the tissue by formalin, a protein cross-linker that is extensively used in routine pathology for preserving tissues for archiving. Fixation depends on many factors, e.g., time, temperature, pH, formalin concentration, size of the tissue, etc. Small changes in fixation conditions result in variations in the degree of protein-protein and protein-DNA cross-links. Cross-linking affects the accessibility of DNA to dyes. Due to these small variations during fixation, even simultaneously processed normal tissue from the same patient could not be used as a reliable reference. Because of the lack of an adequate reference, it is frequently assumed that the first G0G1-peak of bi-modal DNA histograms generated by the Hedley method represents normal cells and is per definition DNA diploid. During the past, attempts were made to improve the quality of the DNA histograms obtained from FFPE tissues, and many modifications of the Hedley method were published. A major step forward was the demonstration that heating could reverse the effect of formalin on the binding of PI to DNA (Overton & McCoy, 1994). Later on, the same authors showed that normal lymphoid tissue could be used as a reference for DNA ploidy measurements (Overton et al., 1996) by flow cytometry of FFPE tissues.
Heating of formalin-fixed samples originates from the work of Shi et al. (1991) is a widely accepted technique to improve immunohistochemistry of FFPE tissue. The method became known as heat-induced antigen retrieval (HIAR) and, most frequently, a sodium-citrate buffer is used to reverse fixation. In addition, recent studies have demonstrated that heating cleaves the hydroxymethylene bridges caused by the formalin cross-linking reviewed by Yamashita (2007). Leers and colleagues introduced sodium-citrate HIAR prior to pepsin dissociation of FFPE tissue sections and demonstrated improved cell yield, keratin staining, and DNA histogram resolution (Leers et al., 1999).
Dissociation of FFPE tissues
A few years after the Hedley method was published, others pioneered the detection of nuclear proteins in FFPE tissues by flow cytometry (Morkve & Laerum, 1991; Watson et al., 1985). Despite some additional reports in the literature, these methods have never been widely applied. This might be caused by the dissociation procedure, which is difficult to standardize due to lot-to-lot variation of the enzymes. Only protein molecules that are (partially) resistant to proteolytic activity are suitable for immunocytochemical detection. This can explain why antibodies against keratins, intermediate filaments composed of long chains of polypeptides with different properties, are successfully used for detection of epithelial cells in FFPE tissues after pepsin or trypsin dissociation (Frei & Martinez, 1993; Leers et al., 1995; Nylander et al., 1994)(Glogovac et al., 1996). We pioneered the use of collagenases (matrix metalloproteinases) and dispase (a neutral peptidase) for dissociation of FFPE tissues and found that the detection of vimentin in FFPE tissues was especially delicate and largely dependent on the time and temperature of the antigen retrieval process (Corver et al., 2005), while detection of keratin showed to be less affected. The simultaneous use of vimentin and keratin has the advantage that samples with low percentages of tumor cells are also accessible to ploidy analysis. Furthermore, the vimentin-positive, keratin-negative cell fraction can be used as an internal reference for FFPE tissues, allowing the identification of tumor cell populations with DNA content less than that of normal cells, which used to be possible only with fresh and frozen samples.
We also evaluated our method for detection of nuclear antigens, like Ki67 or the proliferating cell nuclear antigen (PCNA). However, we have never been able to detect these proteins. That also applied to CD45, applying different antibodies. This was somewhat surprising since collagenases and dispases are frequently used to isolate live cells from fresh tissues and, according to the literature, they are relatively mild enzymes. The lack of wide applicability of the collagenase/dispase mixture can be caused by the enzymes themselves, which are crude isolates contaminated by a wide range of proteolytic enzymes like trypsin or chymotrypsin. These contaminating enzymes can affect protein molecules of interest other than keratin and vimentin that become susceptible to proteolysis after reversing the formalin cross-links by antigen retrieval. It might be clear that for wide utilization of FFPE tissues for flow cytometry dissociation procedures, these enzyme reagents need to be further optimized.
Antigen retrieval
In addition to improved detection of certain protein molecules by flow cytometry of FFPE tissues after antigen retrieval, antigen retrieval also strongly improves DNA histogram resolution. Coefficients of variation (CVs) can be obtained comparable to what is achieved by the Vindelov method for fresh and frozen samples. This gets around the preferred use of DAPI as a DNA stain for FFPE samples, since stoichiometric binding of DAPI to DNA is known to be less affected by formalin cross-links as compared to other DNA stains. Thus, antigen retrieval facilitates flow cytometry of FFPE tissues by making the method more accessible to laboratories with standard equipment lacking a flow cytometer with UV excitation, allowing PI as a DNA stain for high-resolution DNA content measurements (see Figs. 5, 7 and 8 as examples). However, it should be anticipated that a low CV does not only depend on antigen retrieval. First, the flow cytometer must be optimally aligned. Poor laser alignment results in poor DNA histogram resolution. Secondly, the hydrodynamics of the flow cytometer contributes to the CV. It is recommended to collect the data at a relatively low differential pressure, contributing to the precision of the measurement. Next, quality of the tissue affects DNA histogram resolution. For example, old samples might not have been fixed properly or were fixed in Bouin's (containing picric acid) or unbuffered formalin. Fourthly, formalin is unstable and degrades slowly into formic acid, which can have dramatic effects on the quality of fixation. Furthermore, for optimal results, the DNA dye must be carefully titrated, keeping all other labeling conditions, including cell counts, constant. Finally, chromosomal instability (CIN; Thompson et al., 2010) contributes to the CV. Tumor cells make mitotic errors, and chromosomes can be unevenly distributed among the two newly formed daughter cells. For example, one copy of chromosome 1 makes up about 8% of the total genome (https://www.ensembl.org/index.html). When an imbalanced mitotic error follows chromosome replication during S phase of the cell cycle, the four copies of chromosome 1 are unevenly distributed: one daughter cell obtains three and the other daughter obtains one copy of chromosome 1. The difference in DNA content between the daughter cells will be 16% (+8%, –8%) which will contribute to the variation in the measurement. This is detected by DNA content flow cytometry especially when these cells have gone through multiple rounds of stable mitosis. This phenomenon has been clearly demonstrated in an in vitro cell line model for thyroid cancer (Corver et al., 2018). This might also suggest a correlation between CV of the G1 population, CIN, and clinical behavior as shown in cervical cancer (Wimberger et al., 2002).
Critical Parameters and Troubleshooting
Dye selection for multiparameter DNA analysis
The Basic Protocol describes the use of FITC, RPE, and DAPI as fluorescent dyes for measuring the intermediate filaments keratin and vimentin and the DNA content of different cell populations derived from FFPE clinical samples, respectively (Fig. 4 and 6). The use of DAPI has the advantage that the spectral spillover of the DNA stain is very limited, while maintaining high-resolution DNA content measurements. Nowadays, standard flow cytometers are frequently equipped with three lasers: a violet (405 nm), a blue (488 nm), and a red (633 nm) laser. The 405 nm violet laser can also be used for excitation of DAPI with good results, despite suboptimal excitation at the end of the absorption spectrum of DAPI. Fortunately, violet lasers are less expensive than UV lasers.
PI can be an alternative for DAPI, and comparable results are obtained. The use of FITC or Alexa FluorTM 488 (AF488) and APC as fluorescent dyes for labeling keratin and vimentin, respectively, is then preferred, since the spectral spillover of PI into the FITC and APC fluorescence detector is limited (Alternate Protocol 1) (Fig. 7 and 8). Also, R-PE is a good alternative to FITC or Alexa FluorTM 488 using a blue 488-nm laser for excitation.
When the flow cytometer is only equipped with a 488-nm single laser (e.g., FACSCan or Coulter XL instruments), the APC-conjugated secondary reagent can be replaced by an RPE-conjugated antibody (Alternate Protocol 2; Fig. 5). The spectral spillover of PI into the RPE fluorescence detector is significant, but can be compensated without changing the original optical filters of the FACScan, FACSCalibur, or LSRII flow cytometer (Becton Dickinson). The detection threshold of the RPE detector is under the instrument settings used, ∼51 × 103 MESF (Corver et al., 1994). This does not hamper the detection of keratin or vimentin, since these proteins are abundantly expressed. However, due to a different optical filter settings of the Beckman Coulter FC500 or Epics XL, the simultaneous detection of RPE and PI fluorescence (used for DNA cell cycle analysis) is more complicated and the spill over of PI fluorescence into the RPE detector cannot be entirely compensated for. Filters need to be adjusted. The Epics XL allows changing optical filters easily. Due to the hardware design of the FC500, changing optical filters is problematic.
During the years, many other DNA dyes came on the market, e.g., 7-AAD (Rabinovitch et al., 1986), TO-PRO-3 (Corver et al., 2000; Hirons et al., 1994) and DRAQ5 (Douwes Dekker et al., 2004). These can also be used for DNA content measurements. We have evaluated several of these new dyes to be used as a DNA stain for DNA content measurements and compared them with PI and DAPI. In our hands, PI and DAPI were shown to be superior in terms of CV, and are far less expensive and, thus, cost saving, especially when high cell concentrations are required in order to sort sufficient numbers of cells for genomic profiling.
Table 2 shows an overview of the most commonly used fluorescent dyes used for multiparameter DNA flow cytometry and, when applied in certain combinations, their spectral limitations in terms of spill-over. This table might function as a guide to select fluorescent dyes for the kind of protocols described in this article. To minimize spectral spill-over, it is recommended to use 2 or 3-laser excitation. The latter allows especially excellent separation of the emission spectra of DAPI, FITC or AF488, APC, or AF647.
Fluorescent dye | ||||||
---|---|---|---|---|---|---|
Laser line(s) (nm) | FITC or AF488 | RPE | APC or AF647a | DAPI | PI | spill-over |
488 | + | + | + | significant | ||
488/635 | + | + | + | Some | ||
405/488 | + | + | + | medium | ||
405/488/635 | + | + | + | minimal | ||
405/488/635 | + | + | + | minimal |
- a There are many fluorescent dyes on the market. Also, secondary reagents conjugated to a plethora of fluorescent dyes are commercially available. Due to their brightness, the use of FITC or AF488, RPE, APC, or AF647 is recommended. In addition, DAPI and PI are relatively cheap reagents with excellent cytochemical staining characteristics, as shown by the high-resolution DNA histograms that can be achieved with these dyes (see Figs. 4-8).
- Also take note of the last column showing the spectral spill-over. This is significant using the 488-nm laser only. Especially the spill-over of PI into RPE fluorescence detector is critical but possible (see Fig. 5) (Corver et al., 1994). Whenever possible it is recommended to use a 2- or 3-laser flow cytometer. Imagers are equipped with multiple excitation capabilities and a different optical lay-out compared to flow cytometers (Bolognesi et al., 2016). FITC = fluorescein isothiocyanate, AF = Alexa FluorTM, RPE = R-Phycoerythrin, APC = allophycocyanin, DAPI = 4′,6-diamidino-2-phenylindole, PI = propidium iodide.
Compensation of spectral spillover of samples stained for multiparameter DNA analysis
Color (fluorescence) compensation of samples prepared for multiparameter DNA analysis requires explanation. For standard immunophenotyping, beads or labeled lymphocytes are used to set up the instrument automatically or manually. However, instrument settings used to measure lymphocytes cannot be superimposed to measure cells present in tissue dissociates or cell mixtures stained for DNA and multiple proteins, since DNA is measured on a linear scale. Furthermore, the high concentration of a DNA stain in a sample, needed to guarantee low G0G1 CVs, can cause some background staining (Corver et al., 1994). Consequently, lower PMT voltages, as compared to the measurement of lymphocytes, must be used. Using a sample stained for DNA only (e.g., PI), PMT voltages and compensation are set in such a way that a log normal distribution of the cell population is shown in the first (or half way down the second) log decade of the 4-log decades of the green (FITC or Alexa FluorTM 488) fluorescence detector. Using this approach, a complete unstained sample is not needed, and precious clinical material can be saved. The procedure results in clear separation of keratin-negative and keratin-positive cells. Thus, for simultaneous measurement of epithelial cells and DNA content only, two tubes are required: one stained for DNA only and one stained for keratin and DNA.
However, more accurate compensation can be achieved using a sample without a DNA stain and with proper negative-control antibodies. This is not possible on (older) instruments using logarithmic (Log) amplifyers, e.g., Becton Dickinson FACSCan, FACSCalibur, or Beckman Coulter Epics XL. The signal of the negative control is out of scale and compensation cannot be set correctly. The negative events can only be visualized on the Lin scale by increasing the PMT voltage, which is unjustifiable for correct compensation. All samples must be acquired using identical instrument settings.
Fortunately, modern flow cytometers are digital, using linear (Lin) amplification. The linear values are converted to Log values during acquisition, but the actual values are still linear. This allows the use of bi-exponential (BiExp) expression (Bagwell, 2005). During acquisition, the ordinate of the parameter (detector) used for acquisition of the DNA histogram scale can be switched from linear to BiExp and vice versa. In BiExp display, events from both the DNA-stained as well and unstained sample will be shown in the window of analysis using the same PMT voltage optimally set to acquire a DNA histogram. The relative values from FITC or Alexa FluorTM 488 and APC or Alexa FluorTM 647 fluorescence are also displayed bi-exponentially. Now, compensation can be set properly. During acquisition of the sample, the parameter used for detection of the DNA content is now switched to Lin. This way, correct compensation can be achieved with samples stained for multiple proteins and DNA. Even with high concentrations of DAPI, we obtained excellent results using this approach.
Understanding Results
When enumerating stromal cells and epithelial cells derived from FFPE tissue blocks by flow cytometry, it is important to study the tissue by an H&E section under the microscope before use. In this way, the expected percentage of tumor cells can be estimated and the quality of the tissue can be examined. In general, high-quality measurements can be obtained, and samples containing low numbers of tumor cells (<5%) are suitable for this technique. Even under such conditions, carcinoma cells will be effectively detected by keratin antibodies (see Fig. 5 as an example). However, factors that can contribute to inferior results are tissue necrosis, poor fixation, and over-digestion.
Tissues containing necrotic areas are not optimal, and poor results must be anticipated. To avoid poor results caused by necrotic tissue, samples can be macro-dissected using surgical knives, or tissue punches can be taken from the vital parts of the tissue block as described in step 2 of the Basic Protocol and Figure 3. As mentioned above (see Antigen retrieval in Background Information), optimal fixation is crucial. Tissues need to be fixed as soon as possible after surgery to prevent autolysis. In contrast to what is found in the literature (Esteban et al., 1991), prolonged fixation in buffered formalin does not affect DNA histogram resolution or keratin and vimentin staining. A clear example is shown in Figure 5, where the sample displayed is one out of a series of tissue blocks used to evaluate the robustness of the method (Corver et al., 2008), which has been used many times. Remains of cervical carcinoma were stored in buffered formalin for about 3 weeks, paraffin-embedded, and processed using Alternate Protocol 2. As illustrated, excellent results were obtained. This may be due to the HIAR procedure used to reverse formalin cross-links, which strongly facilitates binding of dyes to nucleic acids; at the same time it improves immunophenotyping results. In general, tissues should be fixed for 24 hr in buffered formalin. Deficient formalin treatment will result in unbalanced fixation and differences in responsiveness to enzymatic treatment and DNA labeling even after HIAR. Finally, over-digestion of the tissue also contributes to poor results. For example, breast carcinomas frequently contain a high amount of extracellular matrix. In these cases, it is important to monitor the dissociation process more closely and to stop it at the right moment. Otherwise, by the time the matrix is digested, most cells will be destroyed by proteolytic activity, ending with cellular debris and bare nuclei. Importantly, in our hands we also noticed that for colorectal cancer samples, results after dissociation using the gentleMACSTM were superior to the method using the nylon bags. We do not have a direct explanation for this phenomenon, but it clearly indicates that results are procedure dependent and should be explored experimentally.
In addition, prolonged digestion can result in nonspecific binding of the antibodies. This can be observed by an increase in background staining and poor resolution between the carcinoma and the stromal cells. The protocol described was developed typically to identify epithelial and stromal cells in cervical carcinomas. Since cervical carcinomas are highly heterogeneous in terms of chain-specific keratin expression, a mixture of three monoclonal antibodies is used that covers almost all acidic and basic keratins. This allows clear detection of epithelial cell fraction in carcinomas. Fluorescence signals that can be generated are frequently high, and range between the third and the fourth log decade as compared to the controls, which range between the first and halfway to the second log decade of a four decade amplifier (FACSCalibur or Epics XL) or BiExp display (digital flow cytometers). This mixture of keratin antibodies also showed to be useful in detecting carcinoma cells in other types of carcinomas, e.g., breast, colon, and gastric. Stromal cells (e.g., fibroblasts, leucocytes) are negative for keratin expression. A way to monitor keratin antibody specificity is to cut tissue sections from an FFPE sample, mount the sections on glass slides, and treat the sections according to the Basic Protocol. Next, the sections are exposed to the antibodies, washed, covered with a slip, and studied under a fluorescence microscope.
Time Considerations
It might be clear that multiparameter DNA analysis of FFPE tissues is relatively time consuming as compared to standard immunophenotyping of lymphocytes. However, it should be considered that many steps are involved, including HIAR after tissue dewaxing, tissue dissociation, washing steps, and indirect immunocytochemistry. The first part of the Basic Protocol, including cell counting, takes ∼7 hr. The second part, cell labeling, takes 2.5 hr. Finally, measurement takes 15 to 20 min for each sample, including the controls. Two overnight steps, labeling with the primary antibodies and staining DNA, should be taken into account. The labeling with antibodies can be quickened through the usage of shorter incubation periods. Commercial kits are on the market (Miltenyi Biotec or Menarini Silicon Biosystems). However, higher antibody concentrations are needed, thereby increasing the costs. In addition, directly labeled keratin antibodies are commercially available, but this does not account for vimentin, for which a secondary reagent is still required. It should be noted that for optimal results it is highly recommended to titrate all antibodies used for these assays.
Acquisition of samples labeled for DNA content frequently takes longer than most other measurements. For example, for standard immuno-phenotyping, a sufficient number of cells can be collected within a minute. However, DNA content measurements are frequently more time consuming. Not only the number of keratin-positive cells in the sample determines the acquisition duration, but also hydrodynamic stability of the flow cytometer contributes, since fluctuations affect the CV of the G0G1 peak. For most accurate DNA content measurements, it is recommended to use a low sample pressure (low sample rates), allowing optimized hydrodynamic focusing. And of course, the flow cytometer must be optimally aligned.
Author Contributions
Willem E. Corver : conceptualization, data curation, formal analysis, methodology, writing original draft, Writing review and editing; Natalja T. ter Haar : methodology, writing review and editing.
Conflict of Interest
The authors declare no conflict of interest.
Open Research
Data Availability Statement
Data not available due to privacy/ethical restrictions.
Literature Cited
- Abeln, E. C. A., Corver, W. E., Kuipers-Dijkshoorn, N. J., Fleuren, G. J., & Cornelisse, C. J. (1994). Molecular genetic analysis of flow-sorted ovarian tumour cells: Improved detection of loss of heterozygosity. British Journal of Cancer , 70, 255–262. https://doi.org/10.1038/bjc.1994.289
- Bagwell, C. B. (2005). Hyperlog-a flexible log-like transform for negative, zero, and positive valued data. Cytometry Part A , 64(1), 34–42. https://doi.org/10.1002/cyto.a.20114
- Bagwell, C. B., Clark, G. M., Spyratos, F., Chassevent, A., Bendahl, P. O., Stal, O., Killander, D., Jourdan, M. L., Romain, S., Hunsberger, B., Wright, S., & Baldetorp, B. (2001). DNA and cell cycle analysis as prognostic indicators in breast tumors revisited. Clinics in Laboratory Medicine , 21(4), 875–895, x.
- Barrett, M. T., Pritchard, D., Palanca-Wessels, C., Anderson, J., Reid, B. J., & Rabinovitch, P. S. (2003). Molecular phenotype of spontaneously arising 4N (G2-tetraploid) intermediates of neoplastic progression in Barrett's esophagus. Cancer Research , 63(14), 4211–4217.
- Barrett, M. T., Sanchez, C. A., Prevo, L. J., Wong, D. J., Galipeau, P. C., Paulson, T. G., Rabinovitch, P. S., & Reid, B. J. (1999). Evolution of neoplastic cell lineages in Barrett oesophagus. Nature Genetics , 22(1), 106–109. https://doi.org/10.1038/8816
- Bolognesi, C., Forcato, C., Buson, G., Fontana, F., Mangano, C., Doffini, A., Sero, V., Lanzellotto, R., Signorini, G., Calanca, A., Sergio, M., Romano, R., Gianni, S., Medoro, G., Giorgini, G., Morreau, H., Barberis, M., Corver, W. E., & Manaresi, N. (2016). Digital sorting of pure cell populations enables unambiguous genetic analysis of heterogeneous formalin-fixed paraffin-embedded tumors by next generation sequencing. Scientific Reports , 6, 20944. https://doi.org/10.1038/srep20944
- Bonsing, B. A., Corver, W. E., Fleuren, G. J., Cleton-Jansen, A. M., Devilee, P., & Cornelisse, C. J. (2000). Allelotype analysis of flow-sorted breast cancer cells demonstates genetically related diploid and aneuploid subpopulations in primary tumors and lymph node metastasis. Genes, Chromosomes & Cancer, 28, 173–183. Retrieved from https://onlinelibrary.wiley.com/doi/full/10.1002/%28SICI%291098-2264%28200006%2928%3A2%3C173%3A%3AAID-GCC6%3E3.0.CO%3B2-1?sid=nlm%3Apubmed
- Carvalho, R., Milne, A. N., Polak, M., Corver, W. E., Offerhaus, G. J., & Weterman, M. A. (2005). Exclusion of RUNX3 as a tumour-suppressor gene in early-onset gastric carcinomas. Oncogene , 24(56), 8252–8258. https://doi.org/10.1038/sj.onc.1208963
- Corver, W., Demmers, J., Oosting, J., Sahraeian, S., Boot, A., Ruano, D., Wezel, T. V., & Morreau, H. (2018). ROS-induced near-homozygous genomes in thyroid cancer. Endocrine-Related Cancer , 25(1), 15. https://doi.org/10.1530/ERC-17-0288
- Corver, W. E., & Cornelisse, C. J. (2002). Flow cytometry of human solid tumours: Clinical and research applications. Current Diagnostic Pathology , 8, 249–267. https://doi.org/10.1054/cdip.2002.0125
- Corver, W. E., Cornelisse, C. J., & Fleuren, G. J. (1994). Simultaneous measurement of two cellular antigens and DNA using fluorescein-isothiocyanate, R-phycoerythrin, and propidium iodide on a standard FACScan. Cytometry , 15, 117–128. https://doi.org/10.1002/cyto.990150205
- Corver, W. E., Koopman, L. A., Mulder, A., Cornelisse, C. J., & Fleuren, G. J. (2000). Distinction between HLA class I positive and negative cervical tumor subpopulations by multiparameter DNA flow cytometry. Cytometry , 41(1), 73–80. https://doi.org/10.1002/1097-0320(20000901)41:1<73::AID-CYTO10>3.0.CO;2-5
- Corver, W. E., Koopman, L. A., van der Aa, J., Regensburg, M., Fleuren, G. J., & Cornelisse, C. J. (2000). Four-color multiparameter DNA flow cytometric method to study phenotypic intratumor heterogeneity in cervical cancer. Cytometry , 39(2), 96–107. https://doi.org/10.1002/(SICI)1097-0320(20000201)39:2<96::AID-CYTO2>3.0.CO;2-X
- Corver, W. E., Middeldorp, A., Ter Haar, N. T., Jordanova, E. S., van Puijenbroek, M., van Eijk, R., Cornelisse, C. J., Fleuren, G. J., & van Wezel, T. (2008). Genome-wide allelic state analysis on flow-sorted tumor fractions provides an accurate measure of chromosomal aberrations. Cancer Research , 68(24), 10333–10340. https://doi.org/10.1158/0008-5472.CAN-08-2665
- Corver, W. E., Ruano, D., Weijers, K., den Hartog, W. C., van Nieuwenhuizen, M. P., de Miranda, N., van Eijk, R., Middeldorp, A., Jordanova, E. S., Oosting, J., Kapiteijn, E., Hovens, G., Smit, J., van Wezel, T., & Morreau, H. (2012). Genome haploidisation with chromosome 7 retention in oncocytic follicular thyroid carcinoma. PLoS ONE , 7(6), e38287. https://doi.org/10.1371/journal.pone.0038287
- Corver, W. E., Ter Haar, N. T., Dreef, E. J., Miranda, N. F., Prins, F. A., Jordanova, E. S., Cornelisse, C. J., & Fleuren, G. J. (2005). High-resolution multi-parameter DNA flow cytometry enables detection of tumour and stromal cell subpopulations in paraffin-embedded tissues. The Journal of Pathology , 206(2), 233–241. https://doi.org/10.1002/path.1765
- Corver, W. E., Ter Haar, N. T., Fleuren, G. J., & Oosting, J. (2011). Cervical carcinoma-associated fibroblasts are DNA diploid and do not show evidence for somatic genetic alterations. Cellular Oncology , 34(6), 553–563. https://doi.org/10.1007/s13402-011-0061-5
- Corver, W. E., van Wezel, T., Molenaar, K., Schrumpf, M., van den Akker, B., van Eijk, R., Ruano Neto, D., Oosting, J., & Morreau, H. (2014). Near-haploidization significantly associates with oncocytic adrenocortical, thyroid, and parathyroid tumors but not with mitochondrial DNA mutations. Genes, Chromosomes & Cancer, 53(10), 833–844. https://doi.org/10.1002/gcc.22194
- Dayal, J. H., Sales, M. J., Corver, W. E., Purdie, C. A., Jordan, L. B., Quinlan, P. R., Baker, L., ter Haar, N. T., Pratt, N. R., & Thompson, A. M. (2013). Multiparameter DNA content analysis identifies distinct groups in primary breast cancer. British Journal of Cancer , 108, 873–880. https://doi.org/10.1038/bjc.2013.42
- de Boer, M. A., Jordanova, E. S., Kenter, G. G., Peters, A. A., Corver, W. E., Trimbos, J. B., & Fleuren, G. J. (2007). High human papillomavirus oncogene mRNA expression and not viral DNA load is associated with poor prognosis in cervical cancer patients. Clinical Cancer Research , 13(1), 132–138. https://doi.org/10.1158/1078-0432.CCR-06-1568
- Devilee, P., & Cornelisse, C. J. (1994). Somatic genetic changes in human breast cancer. Biochimica Et Biophysica Acta , 1198(2-3), 113–130. Retrieved from https://ac.els-cdn.com/0304419X94900094/1-s2.0-0304419X94900094-main.pdf?_tid=c889ae1c-ddcd-4881-9395-b87db0bf403d&acdnat=1539355619_7f2ec96d8fedbec256092f4e5e8df4eb
- Douwes Dekker, P. B., Corver, W. E., Hogendoorn, P. C., van der Mey, A. G., & Cornelisse, C. J. (2004). Multiparameter DNA flow-sorting demonstrates diploidy and SDHD wild-type gene retention in the sustentacular cell compartment of head and neck paragangliomas: Chief cells are the only neoplastic component. The Journal of Pathology , 202(4), 456–462. https://doi.org/10.1002/path.1535
- Frei, J. V., & Martinez, V. J. (1993). DNA flow cytometry of fresh and paraffin-embedded tissue using cytokeratin staining [published erratum appears in ModernPathology 1994 Jan;7(1):36]. Modern Pathology , 6(5), 599–605.
- Glogovac, J. K., Porter, P. L., Banker, D. E., & Rabinovitch, P. S. (1996). Cytokeratin labeling of breast cancer cells extracted from paraffin-embedded tissue for bivariate flow cytometric analysis. Cytometry , 24, 260–267. https://doi.org/10.1002/(SICI)1097-0320(19960701)24:3<260::AID-CYTO9>3.0.CO;2-L
- Hedley, D. W., Clark, G. M., Cornelisse, C. J., Killander, D., Kute, T., & Merkel, D. (1993). Consensus review of the clinical utility of DNA cytometry in carcinoma of the breast. Cytometry , 14, 482–485. https://doi.org/10.1002/cyto.990140505
- Hedley, D. W., Friedlander, M. L., Taylor, I. W., Rugg, C. A., & Musgrove, E. A. (1983). Method for analysis of cellular DNA content of paraffin-embedded pathological material using flow cytometry. Journal of Histochemistry & Cytochemistry, 31(11), 1333–1335.
- Hedley, D. W., Shankey, T. V., & Wheeless, L. L. (1993). DNA cytometry consensus conference. Cytometry , 14, 471–471. https://doi.org/10.1002/cyto.990140502
- Hiddemann, W., Schumann, J., Andreeff, M., Barlogie, B., Herman, C. J., Leif, R. C., Mayall, B. H., Murphy, R. F., & Sandberg, A. A. (1984). Convention on nomenclature for DNA flow cytometry. Cancer Genetics and Cytogenetics , 13, 181–183. https://doi.org/10.1016/0165-4608(84)90059-1
- Hirons, G. T., Fawcett, J. J., & Crissman, H. A. (1994). TOTO and YOYO: New very bright fluorochromes for DNA content analyses by flow cytometry. Cytometry , 15, 129–140. https://doi.org/10.1002/cyto.990150206
- Hofman, F. M., & Taylor, C. R. (2013). Immunohistochemistry. Currrent Protocols in Immunology , 103, 21.4.1–21.4.26. https://doi.org/10.1002/0471142735.im2104s103
- Kloth, J. N., Gorter, A., Haar, N. T., Corver, W. E., Jordanova, E. S., Kenter, G. G., & Fleuren, G. J. (2008). Lack of TNFalpha mRNA expression in cervical cancer is not associated with loss of heterozygosity at 6p21.3, inactivating mutations or promoter methylation. Molecular Immunology , 45(1), 152–159. https://doi.org/10.1016/j.molimm.2007.04.028
- Koopman, L. A., Corver, W. E., van der Slik, A. R., Giphart, M. J., & Fleuren, G. J. (2000). Multiple genetic alterations cause frequent and heterogeneous human histocompatibility leukocyte antigen class I loss in cervical cancer. Journal of Experimental Medicine , 191(6), 961–975. https://doi.org/10.1084/jem.191.6.961
- Leers, M. P. G., Schutte, B., Theunissen, P. H. M. H., Ramaekers, F. C. S., & Nap, M. (1999). Heat pretreatment increases resolution in DNA flow cytometry of paraffin-embedded tumor tissue. Cytometry , 35(3), 260–266. https://doi.org/10.1002/(SICI)1097-0320(19990301)35:3<260::AID-CYTO9>3.0.CO;2-O
- Leers, M. P. G., Theunissen, P. H. M. H., Schutte, B., & Ramaekers, F. C. S. (1995). Bivariate cytokeratin/DNA flow cytometric analysis of paraffin- embedded samples from colorectal carcinomas. Cytometry , 21, 101–107. https://doi.org/10.1002/cyto.990210118
- Merlo, L. M., Wang, L. S., Pepper, J. W., Rabinovitch, P. S., & Maley, C. C. (2010). Polyploidy, aneuploidy and the evolution of cancer. Advances in Experimental Medicine and Biology , 676, 1–13. https://doi.org/10.1007/978-1-4419-6199-0_1
- Middeldorp, A., van, P. M., Nielsen, M., Corver, W. E., Jordanova, E. S., ter Haar, N., Tops, C. M., Vasen, H. F., Lips, E. H., van Eijk, R., Hes, F. J., Oosting, J., Wijnen, J., van Wezel, T., & Morreau, H. (2008). High frequency of copy-neutral LOH in MUTYH-associated polyposis carcinomas. The Journal of Pathology , 216(1), 25–31. https://doi.org/10.1002/path.2375
- Morkve, O., & Laerum, O. D. (1991). Flow cytometric measurement of p53 protein expression and DNA content in paraffin-embedded tissue from bronchial carcinomas. Cytometry , 12, 438–444. https://doi.org/10.1002/cyto.990120509
- Nylander, K., Stenling, R., Gustafsson, H., & Roos, G. (1994). Application of dual parameter analysis in flow cytometric DNA measurements of paraffin-embedded samples. Journal of Oral Pathology & Medicine, 23, 190–192.
- Overton, W. R., Catalano, E., & McCoy, J. P. Jr. (1996). Method to make paraffin-embedded breast and lymph tissue mimic fresh tissue in DNA analysis. Cytometry , 26, 166–171. https://doi.org/10.1002/(SICI)1097-0320(19960615)26:2<166::AID-CYTO11>3.0.CO;2-L
- Overton, W. R., & McCoy, J. P. Jr. (1994). Reversing the effect of formalin on the binding of propidium iodide to DNA. Cytometry , 16, 351–356. https://doi.org/10.1002/cyto.990160410
- Qiu, W., Hu, M., Sridhar, A., Opeskin, K., Fox, S., Shipitsin, M., Trivett, M., Thompson, E. R., Ramakrishna, M., Gorringe, K. L., Polyak, K., Haviv, I., & Campbell, I. G. (2008). No evidence of clonal somatic genetic alterations in cancer-associated fibroblasts from human breast and ovarian carcinomas. Nature Genetics , 40(5), 650–655. https://doi.org/10.1038/ng.117
- Rabinovitch, P. S. (1993). Practical considerations for DNA content and cell cycle analysis. In K. D. Bauer, R. E. Duque, & T. V. Shankey (Eds.), Clinical Flow Cytometry ( 1 ed., pp. 117–142). Williams & Wilkins. (Reprinted from: In File).
- Rabinovitch, P. S., Torres, R. M., & Engel, D. (1986). Simultaneous cell cycle analysis and two-color surface immunofluorescence using 7-amino-actinomycin D and singel laser excitation. Applications to study of cell activation and the cell cycle of murine Ly-1 B cells. Journal of Immunology , 136, 2769–2777. https://doi.org/10.4049/jimmunol.136.8.2769
- Schultz, D. S., & Zarbo, R. J. (1992). Comparison of eight modifications of Hedley's method for flow cytometric DNA ploidy analysis of paraffin-embedded tissue. American Journal of Clinical Pathology , 98, 291–295. https://doi.org/10.1093/ajcp/98.3.291
- Shankey, T. V., Rabinovitch, P. S., Bagwell, B., Bauer, K. D., Duque, R. E., Hedley, D. W., Mayall, B. H., Wheeless, L., & Wheeless, L. (1993). Guidelines for implementation of clinical DNA cytometry. Cytometry , 14, 472–477. https://doi.org/10.1002/cyto.990140503
- Shi, S. R., Key, M. E., & Kalra, K. L. (1991). Antigen retrieval in formalin-fixed, paraffin-embedded tissues: An enhancement method for immunohistochemical staining based on microwave oven heating of tissue sections. Journal of Histochemistry & Cytochemistry, 39(6), 741–748.
- Sieben, N. L., Ter Haar, N. T., Cornelisse, C. J., Fleuren, G. J., & Cleton-Jansen, A. M. (2000). PCR artifacts in LOH and MSI analysis of microdissected tumor cells. Human Pathology , 31(11), 1414–1419. https://doi.org/10.1016/S0046-8177(00)80013-1
- Thompson, S. L., Bakhoum, S. F., & Compton, D. A. (2010). Mechanisms of chromosomal instability. Current Biology , 20(6), R285–295. https://doi.org/10.1016/j.cub.2010.01.034
- van den Tillaart, S. A., Corver, W. E., Ruano, N. D., Ter Haar, N. T., Goeman, J. J., Trimbos, J. B., Fleuren, G. J., & Oosting, J. (2013). Loss of heterozygosity and copy number alterations in flow-sorted bulky cervical cancer. PLoS One , 8(7), e67414. https://doi.org/10.1371/journal.pone.0067414
- Vindelov, L. L., Christensen, I. J., Jensen, G., & Nissen, N. I. (1983). Limits of detection of nuclear DNA abnormalities by flow cytometric DNA analysis. Results obtained by a set of methods for sample-storage, staining and internal standardization. Cytometry , 3(5), 332–339. https://doi.org/10.1002/cyto.990030505
- Vindelov, L. L., Christensen, I. J., Keiding, N., Spang-Thomsen, M., & Nissen, N. I. (1983). Long-term storage of samples for flow cytometric DNA analysis. Cytometry , 3(5), 317–322. https://doi.org/10.1002/cyto.990030502
- Vindelov, L. L., Christensen, I. J., & Nissen, N. I. (1983a). A detergent-trypsin method for the preparation of nuclei for flow cytometric DNA analysis. Cytometry , 3, 323–327. https://doi.org/10.1002/cyto.990030503
- Vindelov, L. L., Christensen, I. J., & Nissen, N. I. (1983b). Standardization of high-resolution flow cytometric DNA analysis by the simultaneous use of chicken and trout red blood cells as internal reference standards. Cytometry , 3(5), 328–331. https://doi.org/10.1002/cyto.990030504
- Watson, J. V., Sikora, K., & Evan, G. I. (1985). A simultaneous flow cytometric assay for c-myc oncoprotein and DNA in nuclei from paraffin embedded material. Journal of Immunological Methods , 83(1), 179–192. https://doi.org/10.1016/0022-1759(85)90071-7
- Wersto, R. P., Chrest, F. J., Leary, J. F., Morris, C., Stetler-Stevenson, M. A., & Gabrielson, E. (2001). Doublet discrimination in DNA cell-cycle analysis. Cytometry , 46(5), 296–306. https://doi.org/10.1002/cyto.1171
- Wimberger, P., Hillemanns, P., Kapsner, T., Hepp, H., & Kimmig, R. (2002). Evaluation of prognostic factors following flow-cytometric DNA analysis after cytokeratin labelling: II. Cervical and endometrial cancer. Analytical Cellular Pathology , 24(4-5), 147–158. https://doi.org/10.1155/2002/346969
- Yamashita, S. (2007). Heat-induced antigen retrieval: Mechanisms and application to histochemistry. Progress in Histochemistry and Cytochemistry , 41(3), 141–200. https://doi.org/10.1016/j.proghi.2006.09.001
- Zeller, R. (1989). Fixation, embedding, and sectioning of tissues, embryos, and single cells. Current Protocols in Molecular Biology , 7, 14.1.1–14.1.8. https://doi.org/10.1002/0471142727.mb0101s07
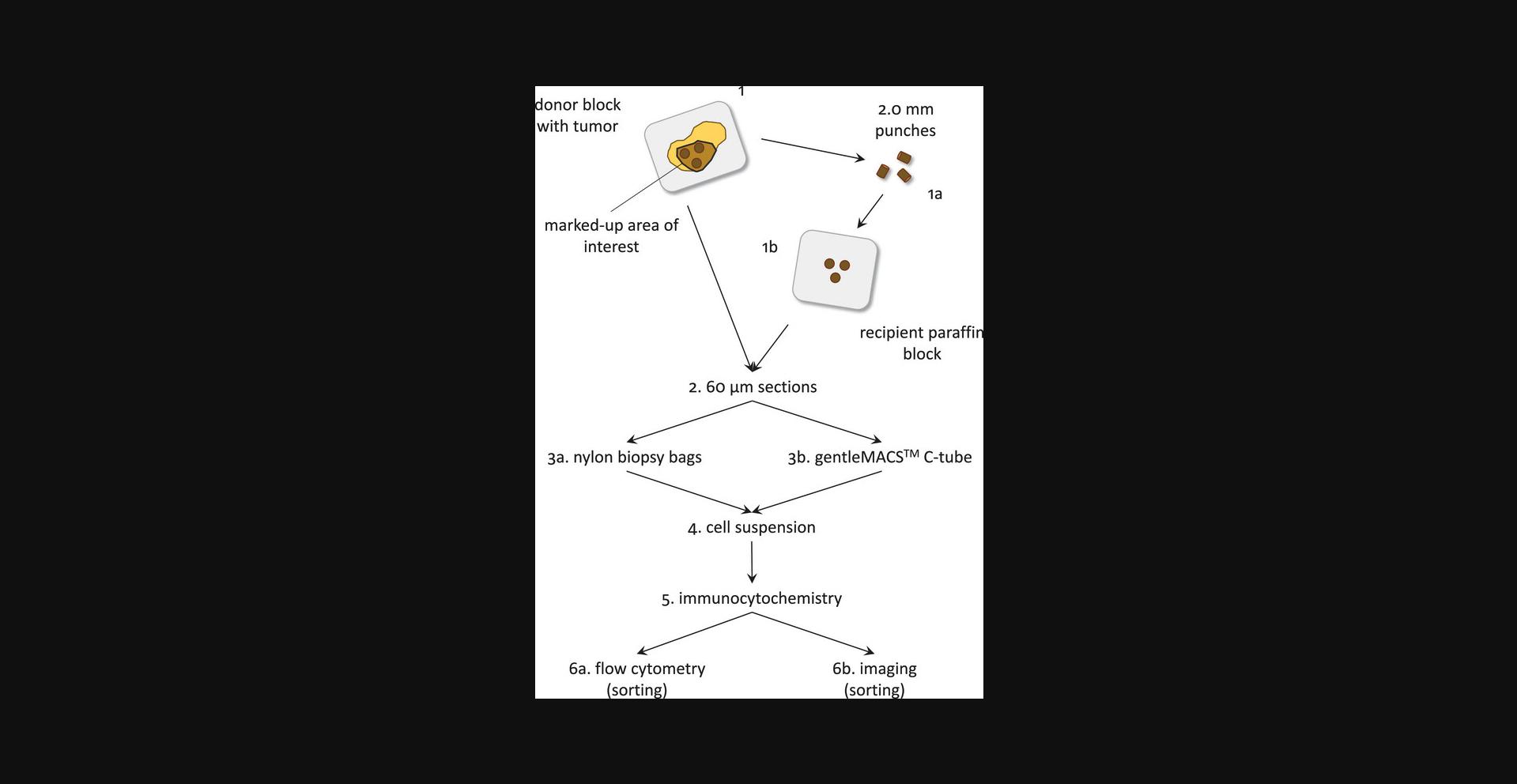
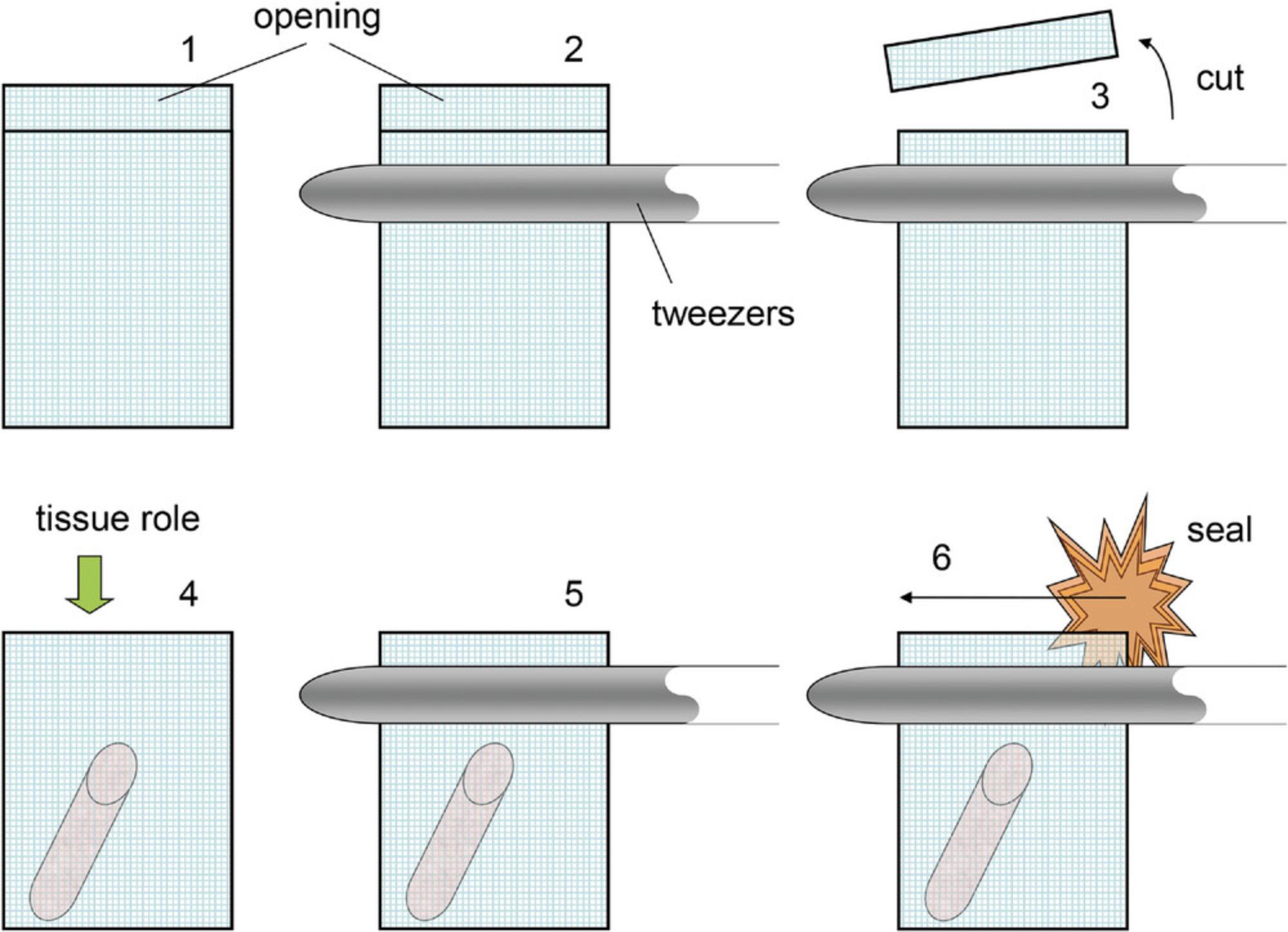
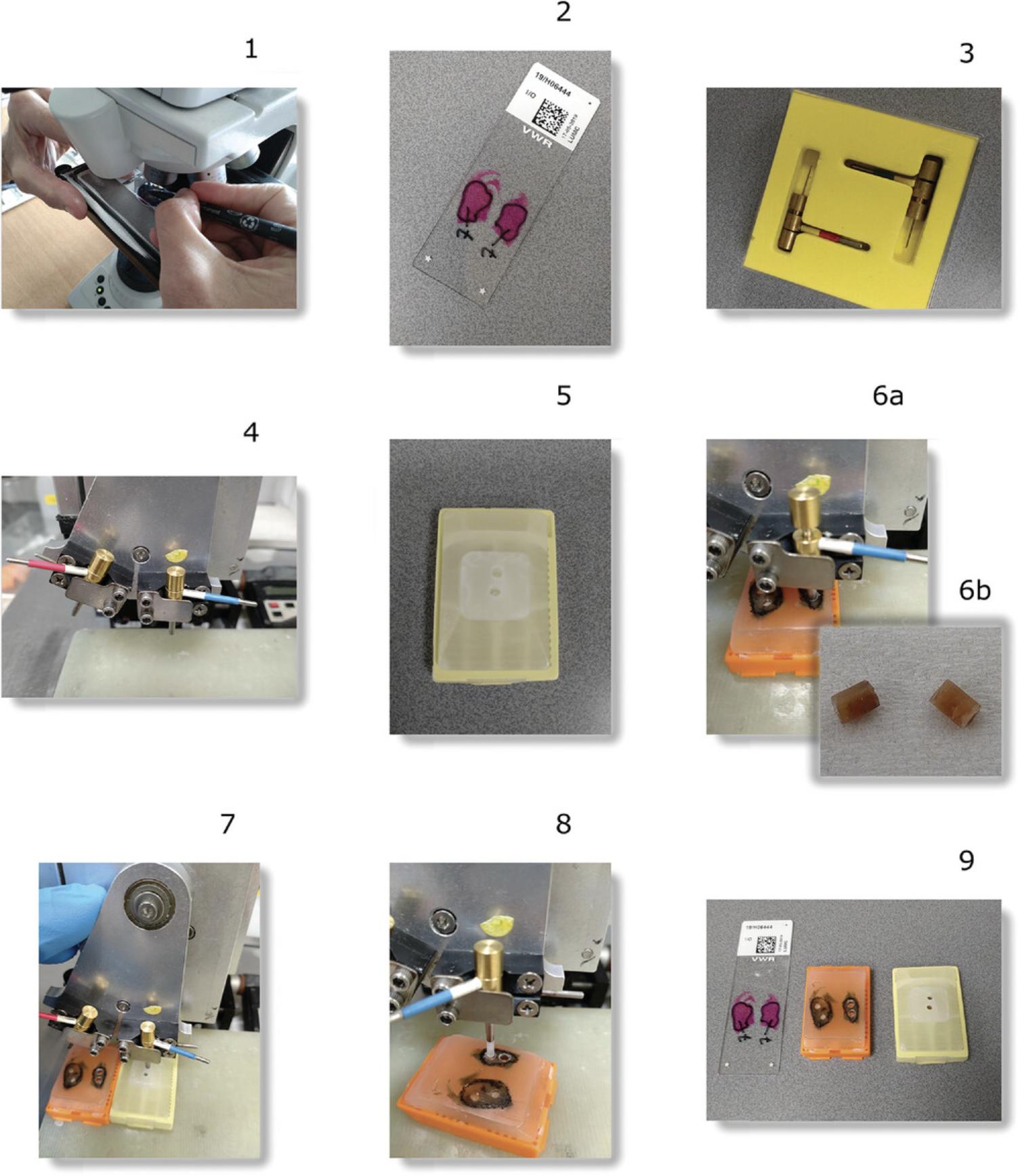
![Details are in the caption following the image Setup of the flow cytometer for multiparameter DNA cell content measurements according to the Basic Protocol. An FFPE squamous cell carcinoma of the uterine cervix was used. The DAPI-stained sample (Tube 1) is used to set up the instrument (LSRII, Becton/Dickinson). (B) First, the PMT voltage of the DAPI detector (355 DNA-DAPI 450/50-A) is adjusted and the G<sub>0</sub>G<sub>1</sub> shows a channel number of ∼50,000 in a 0-2500 × 100 channel scale. A color-coded region (R1) is created to exclude cell debris and multiplets. (A) All events should be to the right of the FSC threshold shown in the FSC vs. SSC dot pot. (C) Then, a second region (R2) is created around the single-cell events in the 355 DNA-DAPI 450/50-A/H versus 355 DNA-DAPI 450/50-A dot plot for doublet discrimination. It should be noted that for some manufacturers’ instrumentation, not width (W) versus area (A) or integral but height (H) versus area (A) is used for doublet discrimination. (D, E, and F) R2 is activated in the other dot plots and single-parameter histograms (panels G and H). (D) shows the relative levels of the background green and deep-orange fluorescence. PMT voltages are adjusted, and Gaussian distributions are shown in the linear part of the bi-exponential scale 488 530/30-A and 488 575/26-A detectors [used for detection of keratin (FITC) and vimentin (RPE), respectively]. Very limited spectral spillover of DAPI into these detectors can be observed. (I and J) Tube 2 is used to correct for spectral spillover of RPE fluorescence into the 488 530/30-A (FITC) and 355 DNA-DAPI 450/50-A detectors, respectively. (K and L) Tube 3 is used to correct for spillover of FITC fluorescence into the BLUE 575/26-A (RPE) and 355 DNA-DAPI 450/50-A detectors, respectively. (M-P) Finally, the triply stained sample is measured (Tube 4). By gating on the vimentin-positive (green) and the keratin-positive populations (red), respectively, and by calculating the relative median fluorescence of the G<sub>0</sub>G1-fractions, the DNA index (DI) can be calculated for the tumor cells (see the Basic Protocol). A more accurate method is by using ModFit software package remotely controlled to WinList (Verity Software House, ME), explained in Figures 7 and 8. Specifications: 355 = 355 nm ultraviolet (UV) laser line excitation; 488 = 488 nm laser line excitation; 450/50, 530/30, or 575/26 bandpass filter (with bandwidth in nanometer (nm)) used to collect fluorescence; A = area. FSC-A = forward scatter-pulse area; SSC-A = side scatter-pulse area; NEGCON = negative control (cells labelled with the DNA stain and secondary reagents only); FITC = fluorescein isothiocyanate; RPE = R-phycoerythrin; DAPI = 4′,6-diamidino-2-phenylindole.](https://static.yanyin.tech/literature_test/cpz1825-fig-0004-m.jpg)
![Details are in the caption following the image Example of a keratin- (FITC-), vimentin- (RPE-), and DNA- (PI-) stained squamous cell carcinoma of the uterine cervix (Alternate Protocol 2) and measured on a FACSCalibur using the single 488-nm laser. (A) A doublet discrimination module was used to enrich for single cell events shown. It should be noted that it is not possible to select for pure single cell events. Cell suspensions prepared from FFPE samples always contain some cell doublets and clumps. Most doublets are correctly identified and “flow” perpendicularly through the laser beam. However, doublets/clumps can disturb the flow cytometer hydrodynamics, and some doublets are positioned in parallel to the laser beam and cannot be identified as such. (see arrow panel A). Doublet discrimination in DNA cell cycle analysis has been extensively discussed by Rabinovitch (1993) and Wersto et al. (2001), and for more information this literature is highly recommended. (B) The negative control stained for PI only is shown. The dot plot shows a mixture of cell populations [lymphocytes, granulocytes, fibroblasts, tumor cells (Corver et al., 2005)] differing in background fluorescence. Region 1 (R1 single cell events) is active. (C-F) This also accounts for the additional panels. (C) The uterine cervix carcinoma sample stained for vimentin (488 VIMENTIN-RPE, 585/42-A) and keratin (488 KERATIN-FITC, 530/30-A) is shown in this panel. High compensation settings are required to correct for spectral spillover of PI fluorescence into the RPE detector. The data shown were generated using the 20 mW 488 blue laser line of a FACSCalibur flow cytometer. The simultaneous use of FITC, RPE and PI fluorescence for cell cycle analysis has been demonstrated (Corver et al., 1994). However, on earlier Beckman Coulter flow cytometer models (Epics XL and FC500) the combination of these dyes gives rise to compensation problems. Optical filters in the Epics XL can be easily adapted, allowing correct compensation. However, due to the hardware of the FC500, this is more problematic for this instrument. (D and E) After gating for the vimentin-positive and keratin-positive cell populations, single-parameter DNA histograms are generated as illustrated in panels D and E, respectively. (D) The vimentin-positive fraction is used as an internal DNA-diploid reference for DNA ploidy assessment. (E) The keratin-positive population is DNA aneuploid with a DI of 1.85 calculated as described under 4.12 of the Basic Protocol. (F) Note that the keratin-positive cell fraction is small and left unnoticed in the combined DNA histogram. Without the use of the keratin and vimentin staining, this sample would likely have been determined as DNA diploid. 488 NEGCON or 488 KERATIN-FITC = fluorescence detector used to collect green fluorescence with a 530/30 nm bandpass filter; 488 NEGCON or VIMENTIN-RPE = fluorescence detector with a 585/42 nm bandpass filter to collect red fluorescence; 488 DNA-PI = fluorescence detector with a 670 nm long-pass filter to collect infra-red fluorescence. A = pulse-area; W = pulse-width; NEGCON = negative control (cells labelled with the DNA stain and secondary reagents only); FITC = fluorescein isothiocyanate; RPE = R-phycoerythrin; PI = propidium iodide.](https://static.yanyin.tech/literature_test/cpz1825-fig-0005-m.jpg)
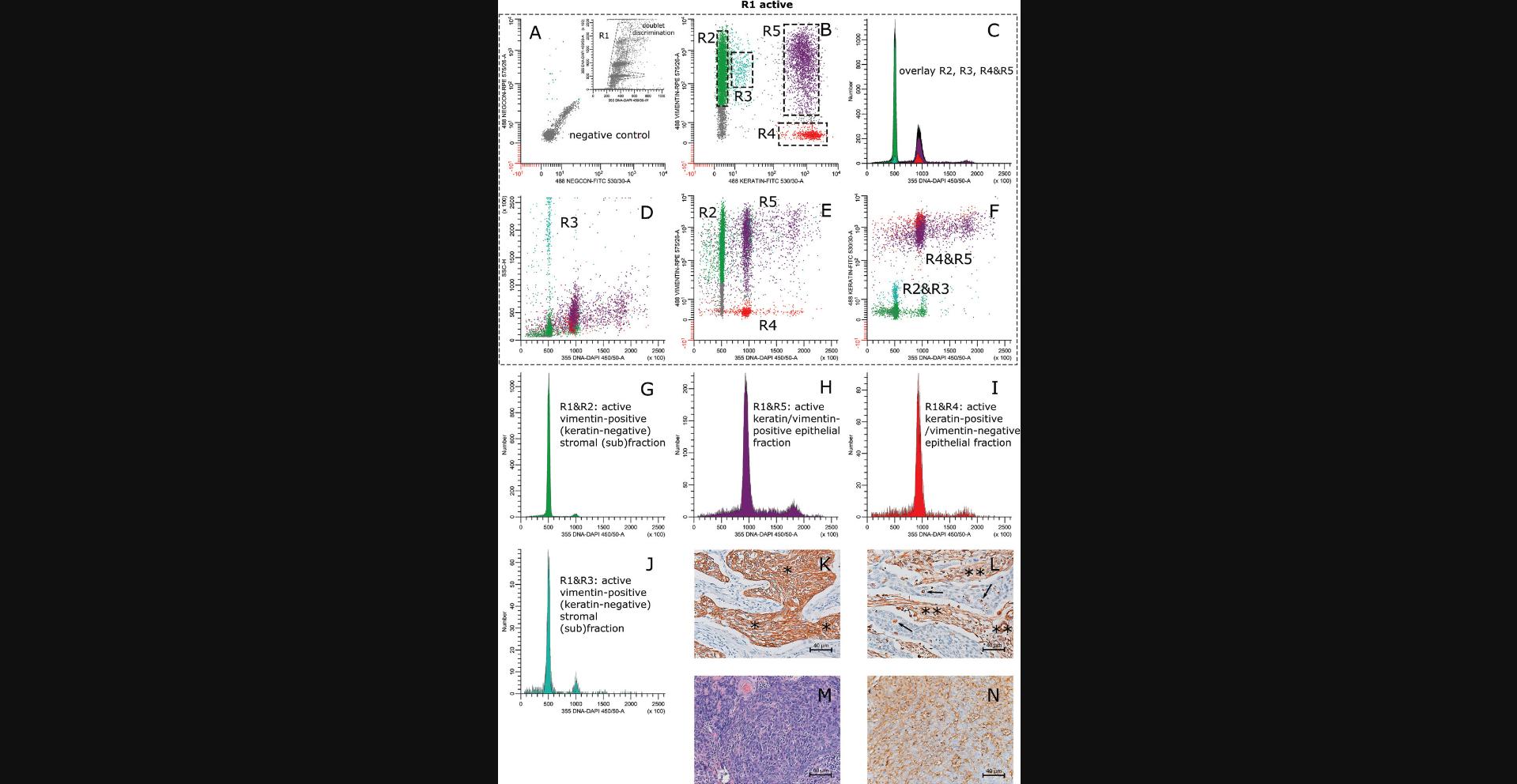
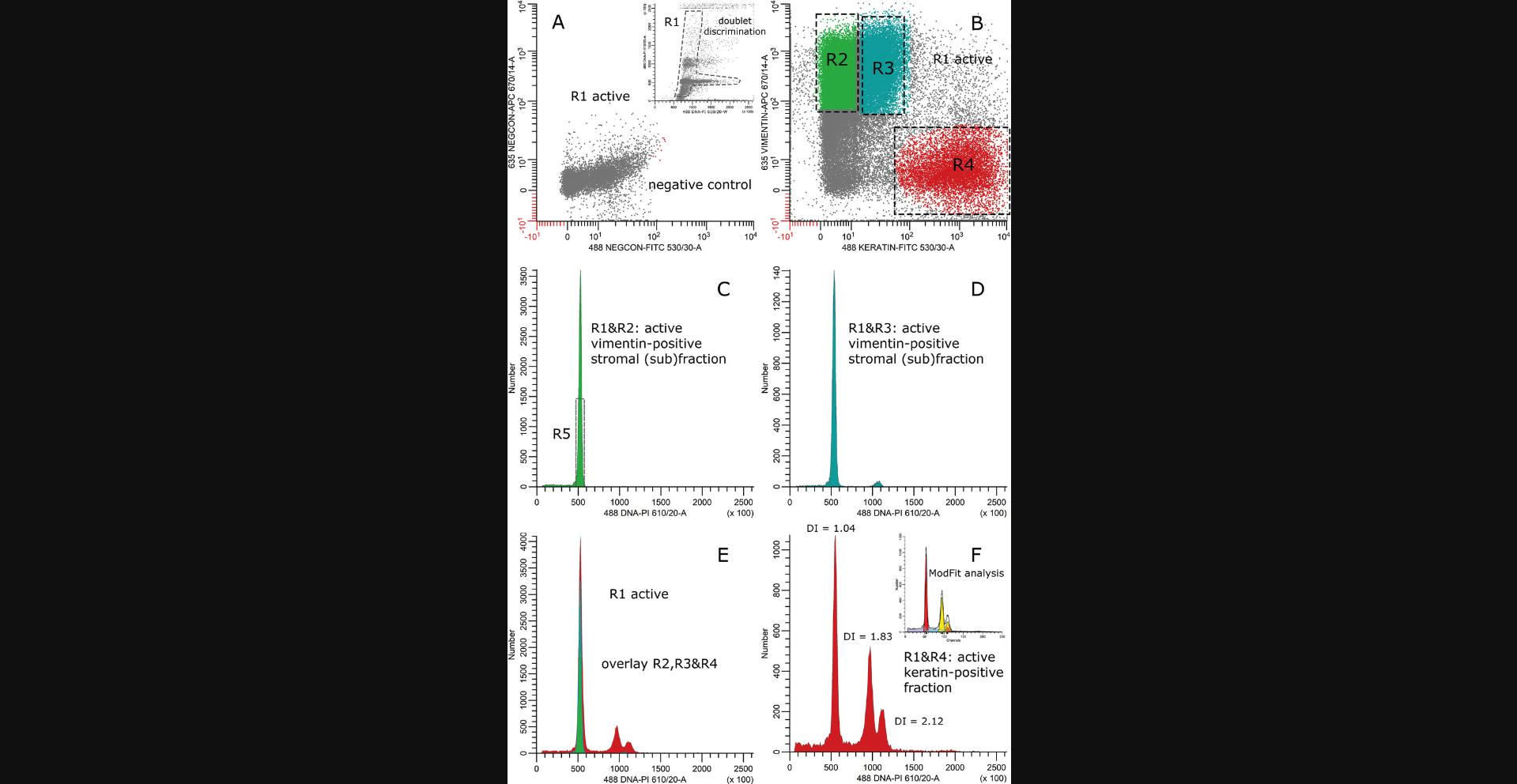
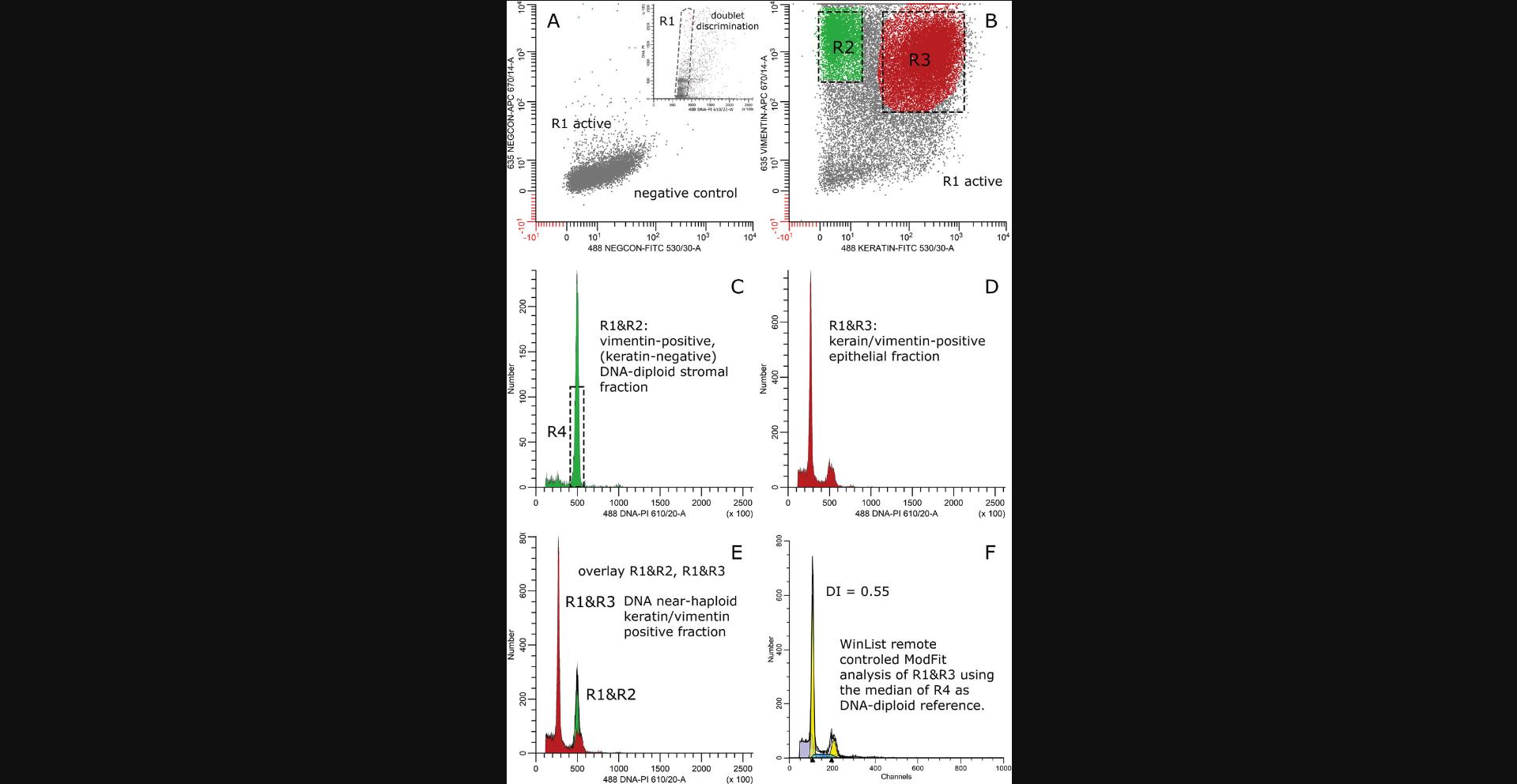