Fluorescent Protein Production, Purification, and Coupling to Microspheres
Marija Dramicanin, Marija Dramicanin, Kevin Lim, Kevin Lim, Simon Monard, Simon Monard
Abstract
Fluorescent proteins (FPs) have become an essential tool for biological research. Since the isolation and description of green FP, hundreds of FPs have been discovered and created with various characteristics. The excitation of these proteins ranges from ultraviolet (UV) up to near infrared (NIR). Using conventional cytometry, with each detector assigned to a fluorochrome, great care must be taken when selecting the optimal bandpass filters to minimalize the spectral overlap as the emission spectra of FPs are broad. Full-spectrum flow cytometers eliminate the need to change optical filters for analyzing FPs, which simplifies instrument setup. In experiments where more than one FP is used, single-color controls are required. These can be cells expressing each of the proteins separately. In the case of the confetti system, for instance, when four FPs are used, all these proteins will need to be expressed separately so that compensation or spectral unmixing can be performed, and this can be inconvenient and expensive. An appealing alternative is to produce FPs in Escherichia coli , purify them, and covalently couple them to carboxylate polystyrene microspheres. Such microspheres are ready to use and can be stored at 4°C for months or even years without any deterioration in fluorescence. The same procedure can be used to couple antibodies or other proteins to these particles. Here, we describe how to express and purify FPs, how to couple them to microspheres, and how to evaluate the fluorescent properties of the particles. © 2023 The Authors. Current Protocols published by Wiley Periodicals LLC.
Basic Protocol 1 : Escherichia coli expression and purification of recombinant mPlum
Basic Protocol 2 : Coupling fluorescent proteins to polystyrene microspheres
Support Protocol 1 : Comparing the cell-bound and bead-bound fluorescence signatures
Support Protocol 2 : Comparing spectral signatures via the similarity index, complexity matrix, and spillover spread matrix of fluorescent protein–coupled beads
INTRODUCTION
Fluorescent Proteins
Since the discovery of green fluorescent protein (GFP) (Shimomura et al., 1962), its cloning (Prasher et al., 1992), and demonstration of the utility of GFP (Chalfie et al., 1994), hundreds of fluorescent proteins (FPs) have been discovered and created. An exhaustive list is outside the scope of this article, but a valuable resource is the FP database FPbase (Lambert, 2019). FPs are widely used in flow cytometry and imaging applications, and the applications are varied, from simple experiments where an FP is used to demonstrate the successful transfection or transduction of cells to more complex experiments where several FPs are used to demonstrate cell cycle state (Abe et al., 2013) or various gene activities (Cai et al., 2013). FPs can be excited by ultraviolet (UV) to near-infrared (NIR) light. Some are very bright, whereas others are not. There are many excellent reviews on the applications of FPs in biology (Chudakov et al., 2010; Rodriguez et al., 2017). The emission spectra of FPs are often broad, which has made the use of multiple FPs in flow cytometry challenging. Another obstacle to using several FPs together is the need for single-color controls. For instance, the “brainbow” (Cai et al., 2013) mouse using the confetti system, including GFP, yellow FP (YFP), red FP (RFP), and cyan FP (CFP), would require each of the FPs to be expressed separately to be used for compensation or unmixing. This would necessitate maintaining cell lines or animal colonies just for this purpose, which would add considerable cost to the experiment. A convenient alternative is to express each FP in Escherichia coli , purify the FPs, and then couple the purified proteins to polystyrene microspheres. Such beads can be stored at 4°C for many months or even years without any change to their fluorescent spectral emission (Monard, 2022).
Conventional and Full-Spectrum Flow Cytometry
Flow cytometry is an essential tool for both biological research and clinical diagnostic services. Flow cytometers have been used in research settings for ∼50 years and in diagnostic labs for ∼40 years. Conventional cytometers have a number of optical detectors, which are usually photomultiplier tubes (PMTs), but several instruments use avalanche photodiodes (APDs) or silicon photomultipliers. Generally, the same number of detectors is employed as the number of different dyes used in the experiment, and unused detectors are not applied to data acquisition to reduce the size of the data file. Fluorescence spillover can be corrected for by using color compensation (Nguyen et al., 2013).
With full-spectrum flow cytometry, single-color controls are referred to as reference controls, all the detectors are always used, and each reference control will generate a spectral signature unique to that fluorochrome. Instead of compensation, spectral unmixing is used (Novo et al., 2013). All the work done for this article has been performed using a five-laser Cytek Aurora because that is what is available in our lab. The claimed advantages of spectral instruments are as follows:
- Dyes with similar spectral characteristics can be used together;
- There is less spread, allowing more fluorochromes to be used simultaneously;
- Autofluorescence can be treated as a “color,” preventing its impact on other parameters; and
- No filter changes are ever required.
Coupling Proteins to Carboxylate Microspheres
There are many reasons to couple proteins to microsphere beads. Antibody capture beads can be used as a cell substitute for flow cytometry experiments as a tool for adjusting for spectral overlap, and they are available from many commercial sources. We have previously described making antibody capture beads by coupling polyclonal goat anti-rat IgG or goat anti-mouse IgG to the surface of carboxylate beads (Monard, 2022). The same process can be used to couple FPs to beads. First, the surface carboxyl groups are activated with a soluble carbodiimide such as N -(3-dimethylaminopropyl)-N ′-ethylcarbodiimide hydrochloride (EDAC). Protein can be added immediately or after a brief incubation, or the beads can be washed prior to adding the protein (Bangs Laboratories, 2013; Staros et al., 1986). The protein and beads are incubated for several hours with constant mixing to allow coupling. This results in stable covalent coupling, and the beads can then be washed and stored at 4°C for many months or even years.
Expressing Fluorescent Proteins in Escherichia coli
E. coli is one of the oldest and most used expression systems for recombinant protein production. E. coli is the preferred host due to its fast growth, simple culture procedures, and low related costs. Recombinant FPs have been routinely produced in this expression system, and many studies have shown their functionality in various assays. They are produced as monomers and engineered using structure-guided changes and directed evolution for optimal stability and functionality.
We have previously demonstrated coupling FPs and antibodies to beads (Monard, 2022). In this article, we demonstrate the expression and purification of an additional FP, mPlum (Lambert, 2019; Wang et al., 2004). mPlum is a monomeric red FP derived from Discosoma sp. We used the E. coli expression system to recombinantly produce this protein, and we demonstrate successful coupling of this protein to beads. We use the mPlum expression and purification protocol as an example of recombinant protein production that can be applied to other relevant FPs.
Basic Protocol 1 demonstrates the successful expression and purification of the monomeric RFP mPlum using the E. coli expression system. Basic Protocol 2 describes how to covalently couple FPs (or any other protein) to carboxylate polystyrene microspheres. Microsphere-coupled FPs can have a slightly different spectral signature compared to FP-expressing cells. A similar phenomenon is seen when using polystyrene antibody capture beads. Comparing bead-bound fluorescence and FP fluorescence expressed by cells is described in Support Protocol 1. Support Protocol 2 describes how to determine the likelihood of success of a panel of FPs by looking at the similarity of the fluorochromes and the spread that will be encountered.
Basic Protocol 1: Escherichia coli EXPRESSION AND PURIFICATION OF RECOMBINANT mPlum
mPlum-pBAD FP is expressed in One Shot TOP10 electrocompetent E. coli cells. The mPlum construct used in this article has an N-terminal poly-histidine affinity tag and is purified by two-step purification, including immobilized metal affinity chromatography (IMAC) and size exclusion chromatography (SEC). The purity of the sample is evaluated by SEC and sodium dodecyl sulfate–polyacrylamide gel electrophoresis (SDS-PAGE). A high-quality protein sample shows a predominant protein band at an expected molecular weight of 29.5 kDa on SDS-PAGE and a single, symmetrical peak with an elution volume >50 ml on SEC (Fig. 1). This protocol will allow researchers to produce recombinant mPlum, but it can also be adapted for the production of other FPs of interest.
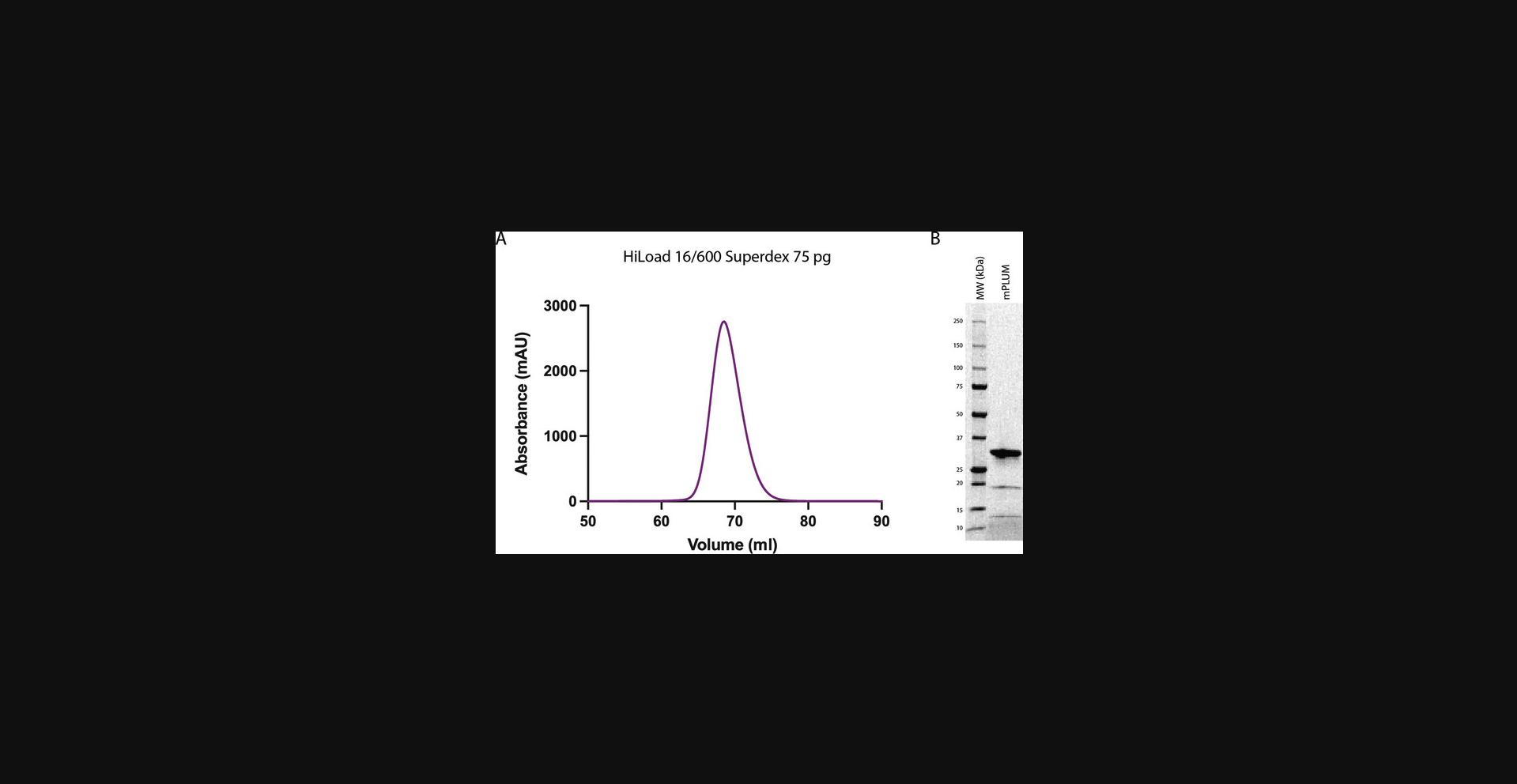
Materials
-
mPlum-pBAD (gift from Michael Davidson and Roger Tsien; Addgene, plasmid cat. no. 54564; http://n2t.net/addgene:54564; RRID: Addgene_54564)
-
Qiagen Plus Maxi Kit (Qiagen, cat. no. 12963)
-
One Shot TOP10 electrocompetent E. coli cells (Invitrogen, cat. no. C404050)
-
LB agar plates (Sterilin Standard 90 mm Petri Dishes, Thermo Scientific, cat. no. 101R20) supplemented with 2% (w/v) glucose (Merck, cat. no. 67021) and 100 µg/ml ampicillin (ampicillin sodium salt, AG Scientific, cat. no. A-1414)
-
LB medium supplemented with 2% (w/v) glucose (Merck, cat. no. 67021) and 100 µg/ml ampicillin (ampicillin sodium salt, AG Scientific, cat. no. A-1414)
-
20% (w/v) L-arabinose (Sigma, cat. no. A91906)
-
cOmplete Protease Inhibitor, EDTA free (Roche, cat. no. 05056489001)
-
Deoxyribonuclease I (DNase I; from bovine pancreas; Merck, cat. no. DN25)
-
Dulbecco's phosphate-buffered saline (DPBS; Gibco, cat. no. 14190144)
-
Glycerol (Univar, cat. no. AJA242)
-
Liquid nitrogen
-
Electroporation cuvettes (Gene Pulser/MicroPulser Electroporation Cuvettes, 0.2-cm gap, Bio-Rad, cat. no. 1652082)
-
Electroporation unit (Bio-Rad Gene Pulser Xcell Main Unit, Bio-Rad, cat. no. 1652666)
-
37°C bacterial incubator
-
2-L baffled flasks
-
37°C shaking incubator
-
Spectrophotometer (NanoDrop One, Thermo Scientific)
-
1000-ml centrifuge bottles (Thermo Scientific, cat. no. 010-1491)
-
Refrigerated superspeed centrifuge (Sorvall Lynx Centrifuge, Thermo Scientific), 4°C
-
50-ml centrifuge tubes (Nalgene, cat. no. 3119-0050)
-
Sonicator (Omni Sonic Ruptor 400 with stepped titanium micro tip)
-
1-L Schott bottles
-
0.22-µm syringe filter (Sartorius, cat. no. 16532)
-
1-ml IMAC column (HisTrap HP IMAC 1 ml Column, Cytiva, cat. no. 29051021)
-
Peristaltic pump (Gilson Minipuls 3)
-
Bio-Rad NGC Quest 10 Plus medium-pressure liquid chromatography system (Bio-Rad, cat. no. 7880003)
-
Bio-Rad NGC Quest 10 Plus software
-
4%-12% Mini-PROTEAN TGX Stain-Free Precast Gel (Bio-Rad, cat. no. 4568096)
-
10-kDa-cutoff centrifuge filters (Merck, cat. no. UFC901024)
-
HiLoad 16/600 Superdex 75 pg SEC column (Cytiva, cat. no. 28989333)
-
5-ml manual load loop
-
Additional reagents and equipment for preparing cell lysis buffer (see recipe) and IMAC elution buffer (see recipe) and for SDS-PAGE
1.Upon receipt of mPlum-pBAD, prepare plasmid DNA using the Qiagen Plus Maxi Kit according to the manufacturer's instructions.
2.Place the One Shot TOP10 electrocompetent E. coli cells in an electroporation cuvette and add ∼100 ng DNA.
3.Activate the electroporation unit and pulse for the designated time.
4.Plate cells on an LB agar plate supplemented with 2% glucose and 100 µg/ml ampicillin and incubate at 37°C for 24 hr to allow for recovery.
5.Pick a single colony from the transformed plate and inoculate into 300 ml LB medium supplemented with 2% glucose and 100 µg/ml ampicillin in a 2-L baffled flask to form a starter culture.
6.Incubate starter culture at 37°C for 18 hr with shaking at 120 rpm.
7.Determine OD600 of starter culture using a spectrophotometer.
8.Using the equation C1V1 = C2V2, calculate how much starter culture is required to inoculate an expression culture to an OD600 of 0.075.
9.Inoculate 1 L LB medium supplemented with 0.2% glucose and 100 µg/ml ampicillin with the required amount of starter culture in a 2-L baffled flask to form an expression culture.
10.Incubate expression culture at 37°C with shaking at 120 rpm until OD600 = 0.6.
11.Induce expression of mPlum by adding 20% (w/v) L-arabinose to a final concentration of 0.2% (v/v) to expression culture.
12.Incubate expression culture overnight at 37°C with shaking at 120 rpm.
13.Pour expression culture into 1-L centrifuge bottles and harvest cells by centrifugation for 15 min at 13,000 × g , 4°C.
14.Pour off supernatant, transfer cell pellets to 50-ml centrifuge tubes, and freeze down cell pellets to −80°C to aid with bacterial lysis.
15.Prepare cold cell lysis buffer (see recipe).
16.Thaw cell pellets on ice and add 40 ml fresh cold cell lysis buffer per liter of expression culture supplemented with one cOmplete Protease Inhibitor tablet and a few grains of DNase I.
17.Mix cell lysate by gently swirling every minute for 10 min at room temperature.
18.Disrupt cells by sonication using 40% amplitude and pulsating for 10 s on and 10 s off for a total of 10 min. Keep lysate cold by sonicating on ice.
19.Clarify lysate by centrifugation for 40 min at 40,000 × g , 4°C.
20.Filter clear lysate into a 1-L Schott bottle using a 0.22-µm syringe filter.
21.Prepare a 1-ml IMAC column according to manufacturer's instructions.
22.Apply cleared filtered lysate from step 20 onto IMAC column at 1 ml/min using a peristaltic pump and collect flow-through in a new 1-L Schott bottle.
23.Apply flow-through onto the same IMAC column at 1 ml/min using the peristaltic pump and collect flow-through in a new 1-L Schott bottle.
24.Prepare IMAC elution buffer (see recipe).
25.Plumb IMAC column onto Bio-Rad NGC Quest 10 Plus medium-pressure liquid chromatography system.
26.Fill Buffer A reservoir with cell lysis buffer (see step 15) and Buffer B reservoir with IMAC elution buffer (see step 24).
27.Wash IMAC column using 15 column volumes of cell lysis buffer.
28.Elute protein of interest using 20 column volumes of gradient elution from 0% IMAC elution buffer to 100% IMAC elution buffer.
29.Collect samples from fractionated peaks displayed on the Bio-Rad NGC Quest 10 Plus software and run on a 4%-12% Mini-PROTEAN TGX Stain-Free Precast Gel under reduced and denatured conditions.
30.Store fractions at 4°C until use.
31.Pool fractions containing protein of interest and concentrate to 5 ml using a 10-kDa-cutoff centrifuge filter according to the manufacturer's protocol.
32.Plumb a HiLoad 16/600 Superdex 75 pg SEC column onto the Bio-Rad NGC Quest 10 Plus system and equilibrate with DPBS.
33.Using a 5-ml manual load loop, load 5 ml concentrated pooled fraction onto the SEC column.
34.Resolve protein of interest on SEC column using 1.2 column volumes.
35.Collect fractionated peaks and run on SDS-PAGE to identify protein of interest.
36.Pool fractions containing protein of interest and add glycerol to a final concentration of 10%.
37.Concentrate protein to between 2 and 4 mg/ml using a 10-kDa-cutoff centrifuge filter. Determine concentration of protein by using a spectrophotometer and dividing A280 by extinction coefficient of the protein.
38.Aliquot protein into 250-µg lots and flash-freeze in liquid nitrogen.
39.Store at −80°C until use (see Basic Protocol 2).
Basic Protocol 2: COUPLING FLUORESCENT PROTEINS TO POLYSTYRENE MICROSPHERES
Carboxylate microspheres can be purchased from several sources. They come in various sizes and can come dyed in different colors or with fluorescent characteristics. The microspheres (also referred to as beads) used in this protocol are composed of 4.5-µm undyed carboxylate polystyrene. Although larger beads can bind more protein, there will be fewer beads per milliliter of suspension, and larger beads settle more quickly. These beads are found to bind enough FPs to compare well with all but the brightest FP-expressing cells.
Figure 2 demonstrates the results of coupling of nine FPs to beads. The relative brightness of each of the FPs coupled in our laboratory is shown in Table 1.The least bright is mPlum.
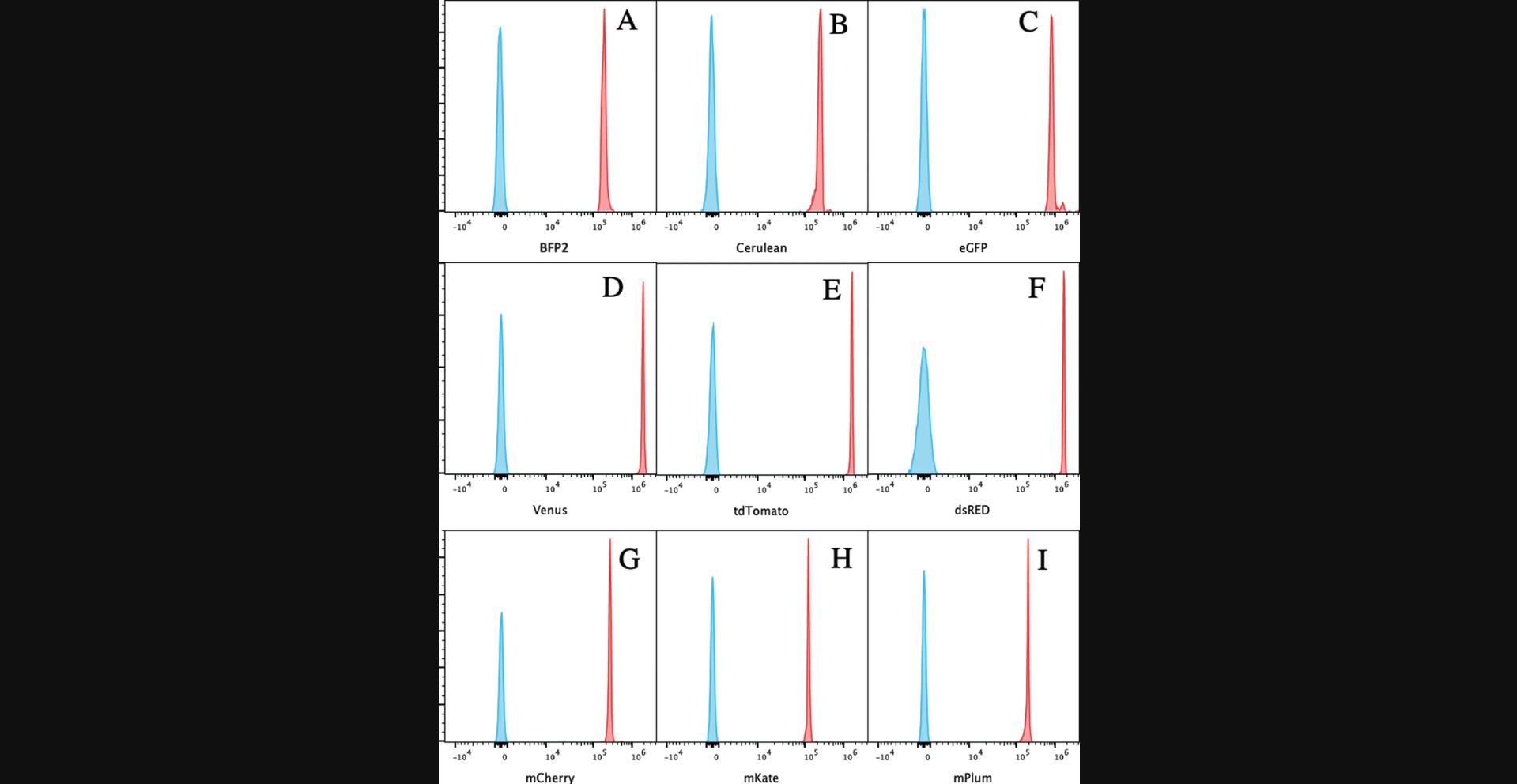
Brightness | Max excitation | Laser wavelength | %max excitation | Relative brightnessa | |
---|---|---|---|---|---|
mPlum | 4.1 | 590 | 561 | 68% | 2.8 |
mKate | 14.85 | 588 | 561 | 68% | 10.1 |
mCherry | 15.84 | 587 | 561 | 64% | 10.1 |
BFP2 | 17.92 | 385 | 405 | 67% | 12.0 |
Cerulean | 26.66 | 433 | 405 | 51% | 13.6 |
Venus | 52.55 | 514 | 488 | 38% | 20.0 |
dsRED | 49.3 | 558 | 561 | 61% | 30.1 |
eGFP | 33.54 | 488 | 488 | 100% | 33.5 |
tdTomato | 95.22 | 554 | 561 | 91% | 86.7 |
- a
The relative brightness corrects for how well each of the FPs is excited by the lasers available.
Materials
-
4.5-µm carboxylate microspheres (Polysciences, cat. no. 17140-5)
-
PolyLink Protein Coupling Kit (Polysciences, cat. no. 24350-1), including PolyLink coupling and storage buffers and EDAC
-
Purified FP suspended in 100 µl DPBS
-
250 µg purified FP (see Basic Protocol 1) suspended in 100 μl of 90% (v/v) DPBS (Gibco, cat. no. 14190144) and 10% (v/v) glycerol (Univar, cat. no. AJA242) (stored at −80°C; ideal concentration 2 to 4 mg/ml)
-
DPBS (Gibco, cat. no. 14190144) with 0.02% (w/v) sodium azide (Merck, cat. no. S2002-25G)
-
DPBS (Gibco, cat. no. 14190144) with 0.02% (w/v) sodium azide (Merck, cat. no. S2002-25G) and 2% (w/v) bovine serum albumin (BSA; Merck, cat. no. A2153-10G)
-
Vortex
-
1.5-ml microcentrifuge tubes (e.g., Merck, cat. no. HS4423)
-
Microcentrifuge (e.g., Corning® LSE™ mini microcentrifuge, Merck, cat. no. CLS6770-1EA)
-
Rotary mixer (e.g., RotoFlex® Plus bench top tube rotator, Merck, cat. no. Z740289)
-
5-ml dropper bottles (e.g., Nalgene™ Dropper Bottles with Control Dispensing Tip, Thermo Fisher, cat. no. NAL2752-9125)
1.Resuspend the 4.5-µm carboxylate microspheres by inverting the bottle multiple times or vortexing vigorously.
2.Pipet 0.5 ml bead suspension into a 1.5-ml microcentrifuge tube.
3.Centrifuge 5 min at 2000 × g.
4.Remove supernatant and resuspend beads in 0.5 ml PolyLink coupling buffer (from the PolyLink protein coupling kit).
5.Centrifuge 5 min at 2000 × g.
6.Remove supernatant and resuspend beads in 0.17 ml PolyLink coupling buffer.
7.Prepare 10 mg EDAC in 50 µl PolyLink coupling buffer. Add 20 µl to the bead suspension and incubate at room temperature for 10 min.
8.Vortex briefly then add 250 µg purified FP suspended in 100 μl of 90% (v/v) DPBS and 10% (v/v) glycerol.
9.Incubate for 4 hr on a rotary mixer at room temperature.
10.Centrifuge 5 min at 2000 × g.
11.Remove supernatant and resuspend beads in 0.5 ml PolyLink storage buffer.
12.Centrifuge 5 min at 2000 × g.
13.Remove supernatant and resuspend beads in 1 ml PolyLink storage buffer. Add 50 µl bead suspension to a 5-ml dropper bottle and then add 4 ml DPBS with 0.02% sodium azide.
14.Prepare blank beads by diluting 25 µl stock beads with 4 ml DPBS with 0.02% sodium azide and 2% BSA.
Support Protocol 1: COMPARING THE CELL-BOUND AND BEAD-BOUND FLUORESCENCE SIGNATURES
FP-coupled beads (Basic Protocol 2) may have a slightly different fluorescent signature compared to cells expressing the same FP. When using the Cytek Aurora, unmixing can be performed using FP-coupled beads. Single FP–expressing cells can then be acquired on the instrument. There may be some “unmixing errors.” This protocol will enable investigators to compare the spectral signature of cells expressing FPs and beads coupled to FPs. These differences are very small with some FPs, whereas with others, the difference is greater. Care must be taken to ensure that the cells not expressing FPs (negative cells) and the FP-expressing cells have the same autofluorescence. Similar disparity can be observed when using polystyrene antibody capture beads. The median fluorescence intensities (MFIs) from all fluorescence channels for blank beads, FP-coupled beads, unstained cells, and FP-expressing cells can be exported from SpectroFlo to Excel™ (Microsoft) or other graphing software. The values from the negative particles can be subtracted from those for the respective positive particles to give the values from the FPs regardless of the autofluorescence of the particles themselves. These fluorescent signatures can be normalized and the differences measured as is described below. The similarity index (SI) described in Support Protocol 2 cannot be used for this, as it only allows comparisons of reference controls.
Materials
-
4.5-µm polystyrene microspheres with no FP (blank beads; see Basic Protocol 2)
-
DPBS (Gibco, cat. no. 14190144)
-
4.5-µm polystyrene microspheres (beads) coupled to FP (see Basic Protocol 2)
-
Non-FP-expressing parental cells (e.g., HEK 293T)
-
FP-expressing cells (e.g., HEK 293T)
-
Vortex (optional)
-
12 × 75–mm polystyrene test tubes (Falcon, Corning, cat. no. 352054)
-
SpectroFlo software, v3.x (Cytek Bio)
-
Cytek Aurora spectral cytometer (Cytek Bio)
-
Microsoft Excel or similar
1.Resuspend the 4.5-µm polystyrene microspheres with no FP (blank beads) by inverting the bottle multiple times or vortexing vigorously.
2.Dispense one drop of blank beads into a 12 × 75–mm polystyrene test tube (∼40 µl). Add 200 µl DPBS.
3.Repeat steps 1 and 2 but with 4.5-µm polystyrene microspheres coupled to FP.
4.Add 200 µl non-FP-expressing parental cells (hereafter referred to as unstained cells) to a 12 × 75–mm test tube (∼50,000 cells).
5.Add 200 µl FP-expressing cells to a 12 × 75–mm test tube (∼50,000 cells).
6.Open SpectroFlo software and choose “default experiment.”
7.Install the tube of blank beads in the Cytek Aurora spectral cytometer and click “acquire.” Adjust forward and side scatter (FSC and SSC) to position the beads in a central position on the FSC and SSC plot. Create a gate around the beads called P1.Set cytometer to acquire 10,000 events within the P1 gate. Click “record.” Repeat the process for the FP-coupled beads.
8.Repeat step 7 for the unstained and FP-expressing cells.
9.Viewing a raw worksheet where all the unmixed channels are present, create a statistics box to include only the MFIs of all fluorescence channels and the parameter name. Right-click the statistics box to save these values as a CSV file.
10.Create a spreadsheet with the parameter names in row 1, the MFI values of each fluorescence channel from the blank beads in row 2, and the MFI values of the FP-coupled beads in row 3.In row 4, calculate the values derived from subtracting the blank-bead MFIs from the FP-bead MFIs, thus giving the median value of the FP in each channel without the autofluorescence contribution of the blank beads (these values can be referred to as corrected MFIs, or CMFIs). Normalize these CMFI values by dividing each value in row 4 by the highest value in row 4, ensuring that highest fluorescence value will be 1. Create a scatter plot in Microsoft Excel or similar, with the fluorescence channel along the x -axis and the normalized CMFI on the y -axis.
11.Repeat step 10 for unstained and FP-expressing cells.
12.Comparing the normalized values of the CMFIs for cells and beads, calculate the differences in these values for each channel between cells and beads. Determine the sum of these values, referred to as the difference index (DI).
13.Normalize the DI by dividing the DI value by the DI value of beads that share little spectral similarity, such as CFP- and mCherry-coupled beads, to produce a metric referred to as the corrected difference index, or CDI.
Support Protocol 2: COMPARING SPECTRAL SIGNATURES VIA THE SIMILARITY INDEX, COMPLEXITY INDEX, AND SPILLOVER SPREAD MATRIX OF FLUORESCENT PROTEIN–COUPLED BEADS
This protocol describes how to use the SI and complexity index (CI) in SpectroFlo software to measure the similarity of the reference controls within an experiment and how to use FlowJo software to look at the spreading of the reference controls. This can be helpful when planning an experiment to determine the likely success of a panel. This protocol uses unmixed data.
Additional Materials (also see Support Protocol 1)
- FlowJo software, v10.x (BD Biosciences)
1.Resuspend the blank beads by inverting the bottle multiple times or vortexing vigorously.
2.Dispense one drop of blank beads into a 12 × 75–mm test tube (∼40 µl). Add 200 µl DPBS.
3.Repeat step 2 but with each of the FP-coupled bead types.
4.Add 200 µl unstained cells to a 12 × 75–mm test tube (∼50,000 cells).
5.Create an experiment in SpectroFlo and select the fluorochromes (FPs) used in the experiment.
6.Run the unstained cells and then each of the reference controls. Select “unmix,” click “next,” and choose the “QC controls” tab. Select the “similarity matrix” button and then select “View Similarity Matrix.” Export the matrix.
7.Open FlowJo software and use “add samples” to import all reference-control FCS files (these will all be unmixed FCS files). Drag the single FP–coupled beads and the blank beads onto the Compensation group.
8.Open the compensation tool. Display the compensation matrix and then click on the “SSM” button and choose “export SSM.”
REAGENTS AND SOLUTIONS
Cell lysis buffer
- 100 mM Na2PO4, pH 7.4 [combine sodium phosphate monobasic monohydrate (Merck, cat. no. S9638) and sodium phosphate dibasic heptahydrate (Merck, cat. no. S9390)]
- 150 mM NaCl (Univar, cat. no. AJA465)
- 20 mM imidazole (Merck, cat. no. 1.04716.100)
- 10% (v/v) glycerol (Univar, cat. no. AJA242)
- Store ≤2 weeks at 4°C
IMAC elution buffer
- 100 mM Na2PO4, pH 7.4 [combine sodium phosphate monobasic monohydrate (Merck, cat. no. S9638) and sodium phosphate dibasic heptahydrate (Merck, cat. no. S9390)]
- 150 mM NaCl (Univar, cat. no. AJA465)
- 500 mM imidazole (Merck, cat. no. 1.04716.100)
- 10% (v/v) glycerol (Univar, cat. no. AJA242)
- Store ≤2 weeks at 4°C
COMMENTARY
Background Information
The first spectral cytometer became commercially available in 2014, when Sony released the SP6800™ instrument. The instrument used a single 32-channel PMT as the detector. In 2017, Cytek Bio released their Aurora™ spectral analyzer, which became an overnight success. So far, over 1000 Auroras have been sold. Sony released a more advanced instrument in 2019: the ID 7000™. Becton Dickinson started offering a spectral version of their flagship high-end analyzer, the BD FACSymphonyA5™, and has recently announced a new instrument, called the FACS Discover™ S8, a cell sorter with some imaging capabilities. Thermo Fisher has also released a cell sorter that uses spectral unmixing, called the BigFoot™. Spectral cytometers, although they have many similar components to conventional cytometers, are used a little differently. They will have more detectors than fluorochromes, but all the fluorescent detectors will be used for every experiment and will produce a spectral signature for each dye.
Monomeric, engineered FPs can be produced in a bacterial host. The advantages of this system are that E. coli cells are readily available in any standard laboratory and that the expression protocol is fast and of a low cost. Recombinant proteins can be fused to different affinity tags, depending on the purification strategy and the final use of the protein. The N- or C- terminal poly-histidine tag enables affinity purification using an IMAC gravity column. This is one of the most standard and economical ways to isolate recombinant proteins of interest from other host-expressed proteins. Another advantage of the poly-histidine affinity tag is that it is short and normally does not interfere with the function of the protein. Isolation of recombinant protein of high quality usually requires additional purification steps. One of the most common final steps of purification is SEC. This is important for quality control of a sample, and it is used to evaluate protein aggregation, purity, solubility, and homogeneity and to estimate the oligomeric state of the protein. The purity of the isolated protein is also routinely evaluated with SDS-PAGE.
Traditionally, either primary or cultured cells are used as single-color or reference controls for FPs in flow cytometry. Maintaining supplies of cells is both time consuming and costly. The coupling of FPs to beads was developed as a convenient tool for compensating or unmixing samples where multiple FPs are used together and where cells expressing each FP alone are not available. FP-coupled beads are also useful when samples have very few cells expressing FPs, making the samples unsuitable as a single-color control or reference control. FP-coupled beads can additionally be used during the panel design phase of planning an experiment to determine the compatibility of a series of FPs and fluorescent antibodies. It is possible to make anti-rat IgG and anti-mouse IgG antibody capture beads from the same particles (Monard, 2022). This allows a single type of blank bead to be used as a negative control for both FPs and antibodies.
An advantage of FP-coupled beads is convenience. The beads are stable for >1 year if kept at 4°C. They require no preparation before use and are inexpensive to make. A disadvantage of FP-coupled beads is that sometimes there may be slight unmixing errors observed when beads have been used as reference controls. The cause of this is unknown; it may be due to interactions between the bead-bound FP and the polystyrene beads. Similar problems occur when using antibody capture beads.
FP-coupled beads can be used as single-color controls for both spectral and conventional cytometry.
Critical Parameters
Basic Protocol 1
A critical parameter for FP production is the selection of optimal expression and purification conditions. For the mPlum-pBAD construct, the cell line must be compatible with L-arabinose-induced protein expression. We recommend using One Shot TOP10 electrocompetent or chemically competent E. coli or, alternatively, the LMG194 E. coli strain. FPs are engineered to be highly stable; however, we recommend executing the two-step purification protocol as fast as possible. Protein quality control includes evaluation of the purity by SDS-PAGE and of protein solubility and homogeneity by SEC. Once the protein of interest is isolated and has passed all quality-control steps, we recommend that the protein be prepared in a single-use aliquot size to avoid freeze-thaw cycles that might affect its performance. To maintain protein structure and function, we recommend flash-freezing the aliquots in liquid nitrogen in the presence of 10% glycerol and storage at −80°C.
Basic Protocol 2
The chemistry of coupling proteins to carboxylate beads is robust and well established. It is recommended that the EDAC is stored in the absence of oxygen and moisture and that the vial of EDAC is flooded with nitrogen after use. However, in our laboratory, we have stored it at −20°C without nitrogen, and it has been stable for >1 year. All FPs and antibodies have coupled well without our changing the coupling protocol. The most critical parameters are the concentration and quality of the protein being coupled. Care should be taken so that the cells expressing no FP (called unstained cells in the SpectroFlo software) have the same autofluorescence as the FP-expressing cells, and ideally, the non-FP-expressing cells should be the parental line for the FP-expressing cells. The blank beads should also be of the same lot number as the FP-coupled beads. The length of the coupling process can be reduced if less bound protein can be tolerated; the majority of the coupling takes place during the first 30 min, but coupling will continue for several hours.
Troubleshooting
The difficulties encountered in our lab when coupling FPs to microspheres are listed in Table 2.
Problem | Possible cause | Solution |
---|---|---|
Staining of beads is heterogenous (a smear rather than one peak) or intensity is poor | FP protein concentration is too low | Add more protein; the recommended protein concentration is 250 µg in 100 µl. Check the concentration of protein. Concentrate the protein using 10-kDa-cutoff centrifuge filters (see Basic Protocol 1). |
Staining intensity is poor | EDAC is too old; old EDAC can absorb water and become less effective | Purchase new EDAC. |
Protein precipitates | Protein was not stored correctly | Proteins should be dissolved in PBS with 10% glycerol and stored at −80°C. |
Understanding Results
Basic Protocol 1
The quality of the produced recombinant FP can be evaluated by SEC and SDS-PAGE. mPlum protein, described in this article, elutes as a single peak on SEC, and the elution volume does not correspond to protein aggregates (Fig. 1A). This indicates that the sample is homogeneous and soluble. The protein is >90% pure and runs at the expected size of 29.5 kDa, as judged by SDS-PAGE (Fig. 1B). Additionally, two unidentified protein bands of lower molecular weight are present in the sample (Fig. 1B). These could be products of mPlum degradation or bacterial protein contamination. Further investigations should be conducted to identify these purification products. According to our coupling experiments, the presence of the additional bands does not affect mPlum's performance. In the case that contaminant bands affect the performance of FPs, additional purification steps (such as ion exchange chromatography) should be introduced into the purification protocol.
Basic Protocol 2
The success of the FP-to-bead coupling process can be measured by running the bead samples on the Aurora instrument and measuring the MFI. If the “use autofluorescence as a fluorescent tag” option is used, the blank beads will have an MFI of approximately 0. The relative intensities vary somewhat; this can be explained by the efficiency of the coupling process (mostly due to FP concentration) and the relative brightness of the FP, which is the brightness multiplied by the percentage of maximum excitation at the wavelength of the laser used (see Table 1). The gains used by the Aurora cytometer will also influence the measured brightness; the Cytek Assay Setting gains are used. The process of coupling FPs to carboxylate microspheres is robust, and the protocol suggested with the PolyLink Coupling Kit works well. A slight modification is used, where the beads are first activated by a 10-min incubation with EDAC before the addition of the protein. Both Polysciences and Bangs Laboratories offer some technical papers, found on their websites, to allow optimization of the procedure. Although mPlum is not a bright FP, with a relative brightness of only 2.8 (see Table 1), the signal from mPlum-coupled beads is comparable to that of Cerulean and BFP2, suggesting that the purified protein is of good quality.
Support Protocol 1
This protocol demonstrates a method to examine the spectral difference between FP-coupled beads and FP-expressing cells. It can also be used to compare the fluorescence from an antibody bound to both beads and cells. After acquisition of data, a statistics window can be made showing the channel names (e.g., V1, V2) and the MFI for each channel. The median fluorescence values for each of these channels can be exported by right-clicking the statistics window. These values will form the basis of the spectral signatures shown in Figure 3. For both beads and cells, the median values of the appropriate negative particles are subtracted. The signature is then normalized to the highest value in any channel. The normalized signatures of the beads and cells are overlaid as line graphs, as shown in Figure 3. A template to aid in this calculation can be found in the Supporting Information. The difference between each normalized CMFI of value for each channel for cells and beads is calculated and summated. This will give a measure of the total difference for two overlaid curves. The correction factor we use is the difference between two spectral signatures with few similarities. All the other values are normalized to the cumulative difference between those two signatures. The metric is referred to as the CDI. Two identical signatures will have a value of 0, and two signatures with very few similarities (in this case, mCherry and Cerulean) will have a value of ∼1. If the CDI is <0.1, the two signatures are very similar, and very little or no unmixing error will be observed. This is an imprecise metric, however, that cannot predict unmixing errors with any precision, but it does give an indication of the differences between the two spectra. A template is included in the Supporting Information to allow this metric to be generated from MFI values from the Aurora. An example of two FPs is shown in Figure 3.
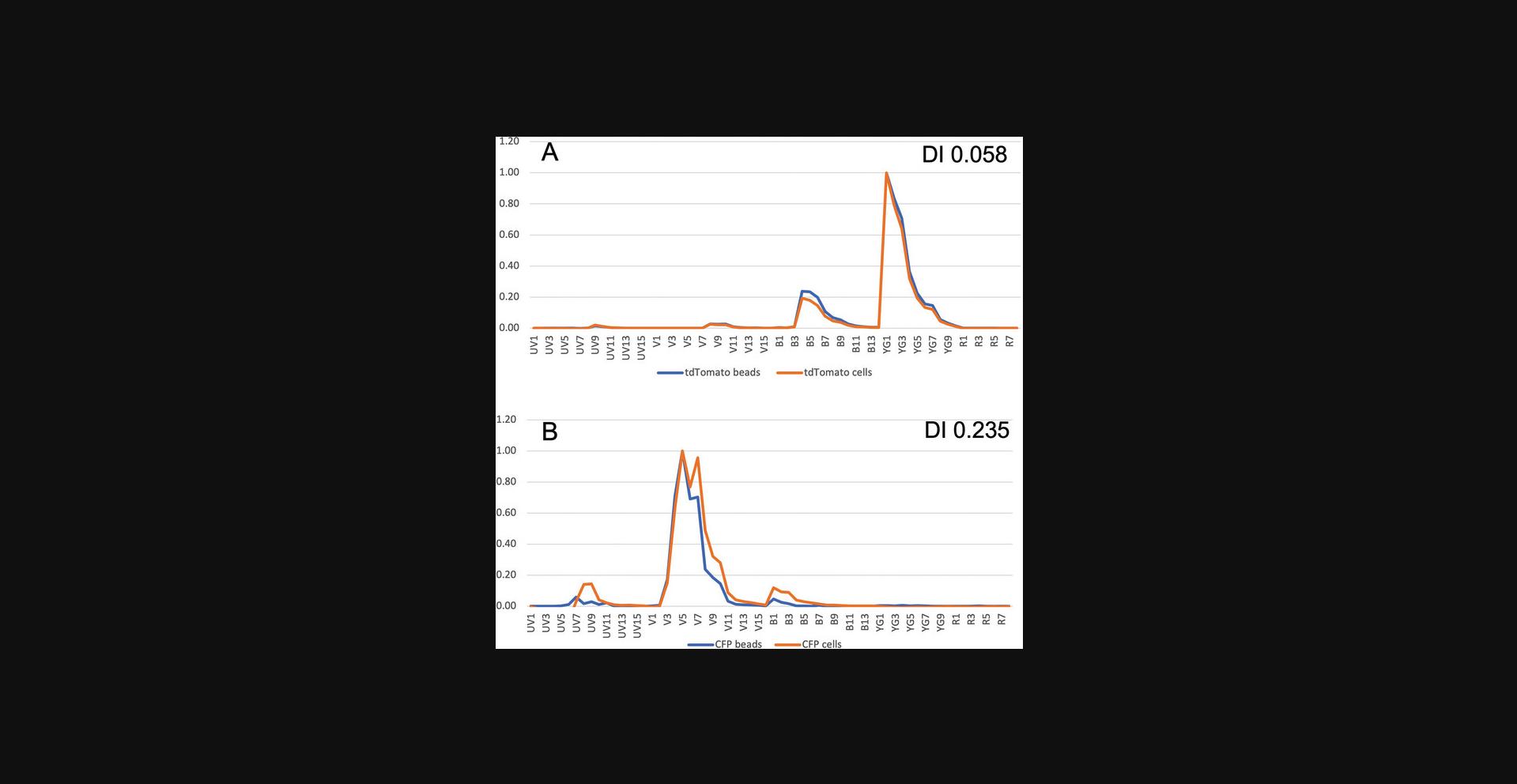
Support Protocol 2
This protocol uses the inbuilt feature of SpectroFlo v3.x software to provide the SI and the CI. These metrics provide a measure of the similarity of different FPs, which indicates whether they can be used together. The CI can be useful for panel design: all reference controls including antibodies and FPs can be viewed in the matrix, and the complexity indicates the quality of the panel. This can be done during the design phase of a panel entirely with beads, that is, both antibody capture and FP-coupled beads. As mentioned in this protocol, all reference controls can be opened in FlowJo v10.x and copied to the compensation group, and the compensation matrix can be viewed; the values should be very small if there are few unmixing errors. More useful is the SSM, which shows the level of spread from one control to another. Unlike the CI, it shows spread of FP1 into FP2 and vice versa, which can be useful. An example showing seven FPs is shown in Table 3. The most similar are mPlum and mCherry, with an SI of 0.78. An example of the SSM of the same FPs is shown in Table 4. Tables 3 and 4 show the SI and the SSM, respectively, for the same samples. The CI suggests that this combination of seven FPs may be used without issues. However, in the SSM, it can be seen that tdTomato spreads significantly into mCherry; the value in the SSM matrix is 21.3, much higher than all the other values. This is demonstrated in the dot plots in Figure 4, where the tdTomato Signal spreads into the mCherry channel. It should be noted that mCherry does not spread into tdTomato to the same degree. This may be relevant in panel design. The SI table (Table 3) identifies the greatest similarity between mPlum and mCherry, whereas the SSM (Table 4) only identifies modest spread between those two FPs.
BFP | Cerulean | GFP | Venus | tdTomato | mCherry | mPlum | |
---|---|---|---|---|---|---|---|
BFP | 1 | ||||||
Cerulean | 0.61 | 1 | |||||
GFP | 0.02 | 0.12 | 1 | ||||
Venus | 0.01 | 0.07 | 0.7 | 1 | |||
tdTomato | 0 | 0.01 | 0.02 | 0.05 | 1 | ||
mCherry | 0 | 0.01 | 0.01 | 0.01 | 0.7 | 1 | |
mPlum | 0 | 0 | 0.02 | 0.02 | 0.38 | 0.78 | 1 |
Complexity index: | 4.51 |
- a The pairs of FPs with the most similarity are CFP-BFP, Venus-GFP, mCherry-tdTomato, and mPlum-mCherry. The higher the SI, the darker the red color.
SSM | BFP | Cerulean | GFP | Venus | tdTomato | mCherry | mPlum |
---|---|---|---|---|---|---|---|
BFP | 0.0 | 2.6 | 0.5 | 0.0 | 0.0 | 0.0 | 0.0 |
Cerulean | 2.2 | 0.0 | 2.6 | 1.1 | 0.0 | 0.0 | 0.0 |
GFP | 0.2 | 4.5 | 0.0 | 7.7 | 0.4 | 0.3 | 0.2 |
Venus | 0.2 | 5.0 | 2.7 | 0.0 | 0.3 | 0.3 | 0.1 |
tdTomato | 0.0 | 2.6 | 5.0 | 5.3 | 0.0 | 21.3 | 5.3 |
mCherry | 0.0 | 1.4 | 0.5 | 0.8 | 2.4 | 0.0 | 2.8 |
mPlum | 0.0 | 1.3 | 1.0 | 0.7 | 1.1 | 2.9 | 0.0 |
- a The higher values in the table have a darker red color. The tdTomato spillover into mCherry has by far the highest value.
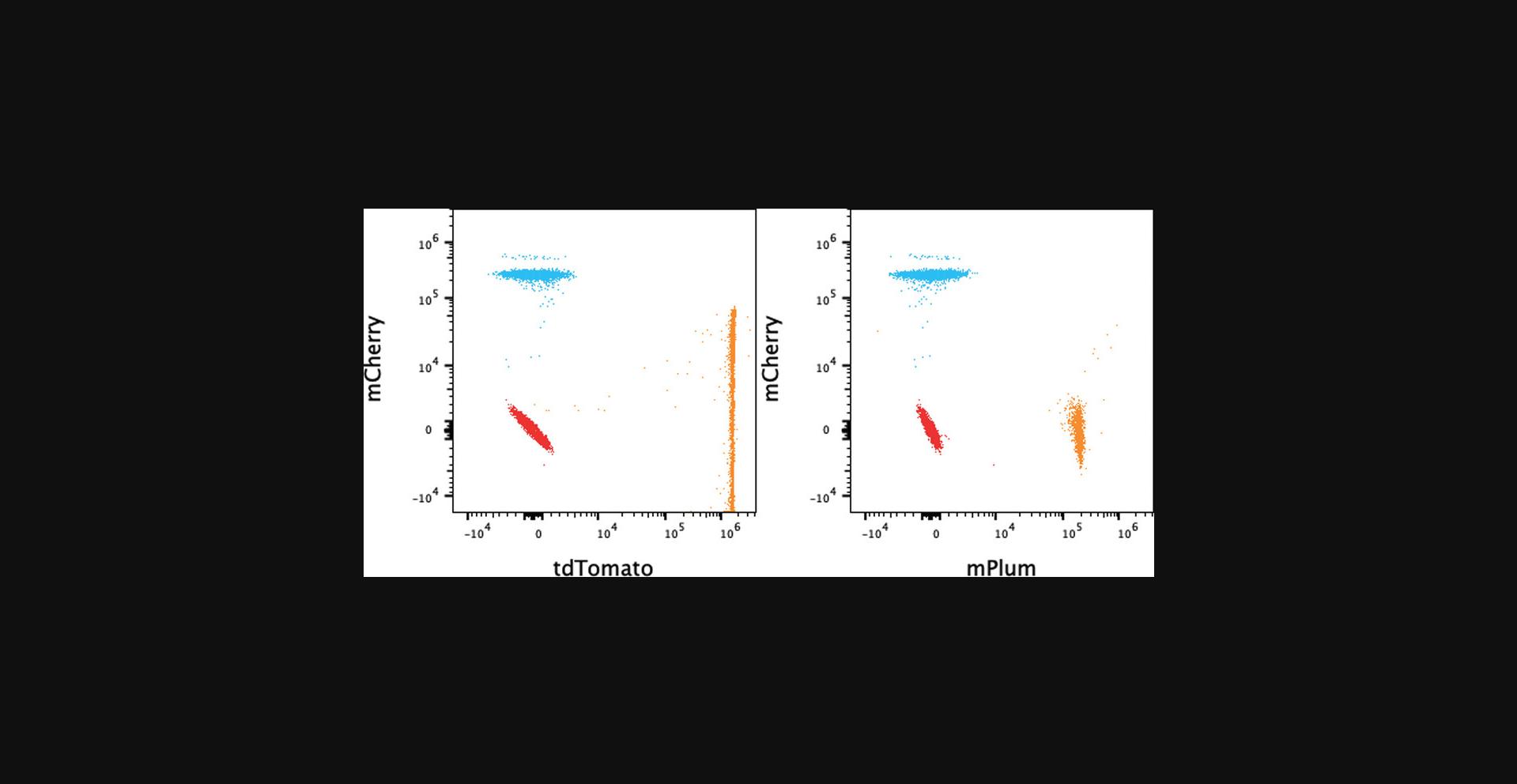
This implies that both the CI and the SSM should both be consulted during panel design and that the SI index values do not always predict spread. Two dot plots are displayed in Figure 4, where mCherry is shown against both mPlum and tdTomato. The high level of spread from tdTomato into mCherry can be seen. It should be noted, however, that the tdTomato spreading into mCherry is exaggerated by the higher tdTomato MFI. The SSM calculation, however, is independent of MFI, but the actual spread increases with the square root of the MFI (Nguyen et al., 2013).
Time Considerations
Basic Protocol 1: The time needed to express 1 L FPs is 5 to 7 hr and to purify these FPs is 7 to 10 hr.
Basic protocol 2: The time needed to couple FPs to beads is 5 hr.
Support Protocol 1: Comparing the FP-coupled beads and FP-expressing cells will take 1 to 2 hr.
Support Protocol 2: Comparing the FP-coupled beads will take 1 to 2 hr.
Acknowledgments
The authors would like to thank the Division of Advanced Technology and Biology at the Walter and Eliza Hall Institute of Medical Research (WEHI) for supporting this research. The authors would also like to thank the Monash Protein Production Facility for expressing and purifying eBFP2, Cerulean, Venus, tdTomato, mCherry, dsRED, and mKate. The authors are grateful to David Miller and the Jacqui Gulbis Structural Biology division, WEHI, for the purified eGFP.
Author Contributions
Marija Dramicanin : Methodology, validation, writing - review and editing; Kevin Lim : Methodology, validation, writing - review and editing; Simon Monard : Conceptualization, formal analysis, investigation, validation, writing - original draft, writing - review and editing.
Conflict of Interest
The authors declare no conflict of interest.
Open Research
Data Availability Statement
The data that support the findings of this study are openly available in Figshare at https://doi.org/10.6084/m9.figshare.21227264 and https://doi.org/10.6084/m9.figshare.21227135.
Supporting Information
Bead cell diff index file
Supporting Information
Filename | Description |
---|---|
cpz1745-sup-0001-bead-cell-diff-index.xlsx164.8 KB | Supporting Information |
Please note: The publisher is not responsible for the content or functionality of any supporting information supplied by the authors. Any queries (other than missing content) should be directed to the corresponding author for the article.
Literature Cited
- Abe, T., Sakaue-Sawano, A., Kiyonari, H., Shioi, G., Inoue, K., Horiuchi, T., Nakao, K., Miyawaki, A., Aizawa, S., & Fujimori, T. (2013). Visualization of cell cycle in mouse embryos with Fucci2 reporter directed by Rosa26 promoter. Development , 140(1), 237–246. https://doi.org/10.1242/dev.084111
- Bangs Laboratories (2013). TechNote 205: Covalent coupling. https://static.yanyin.tech/literature/current_protocol/10.1002/cpz1.745/attachments/TechNote%20205%20Web.pdf
- Cai, D., Cohen, K. B., Luo, T., Lichtman, J. W., & Sanes, J. R. (2013). Improved tools for the Brainbow toolbox. Nature Methods , 10(6), 540–547. https://doi.org/10.1038/nmeth.2450
- Chalfie, M., Tu, Y., Euskirchen, G., Ward, W. W., & Prasher, D. C. (1994). Green fluorescent protein as a marker for gene expression. Science , 263(5148), 802–805. https://doi.org/10.1126/science.8303295
- Chudakov, D. M., Matz, M. V., Lukyanov, S., & Lukyanov, K. A. (2010). Fluorescent proteins and their applications in imaging living cells and tissues. Physiological Reviews , 90(3), 1103–1163. https://doi.org/10.1152/physrev.00038.2009
- Lambert, T. J. (2019). FPbase: A community-editable fluorescent protein database. Nature Methods , 16(4), 277–278. https://doi.org/10.1038/s41592-019-0352-8
- Monard, S. (2022). Building a spectral cytometry toolbox: Coupling fluorescent proteins and antibodies to microspheres. Cytometry Part A: The Journal of the International Society for Analytical Cytology , 101, 846–855. https://doi.org/10.1002/cyto.a.24557
- Nguyen, R., Perfetto, S., Mahnke, Y. D., Chattopadhyay, P., & Roederer, M. (2013). Quantifying spillover spreading for comparing instrument performance and aiding in multicolor panel design. Cytometry Part A: The Journal of the International Society for Analytical Cytology , 83(3), 306–315. https://doi.org/10.1002/cyto.a.22251
- Novo, D., Gregori, G., & Rajwa, B. (2013). Generalized unmixing model for multispectral flow cytometry utilizing nonsquare compensation matrices. Cytometry Part A: The Journal of the International Society for Analytical Cytology , 83(5), 508–520. https://doi.org/10.1002/cyto.a.22272
- Prasher, D. C., Eckenrode, V. K., Ward, W. W., Prendergast, F. G., & Cormier, M. J. (1992). Primary structure of the Aequorea victoria green-fluorescent protein. Gene , 111(2), 229–233. https://doi.org/10.1016/0378-1119(92)90691-h
- Rodriguez, E. A., Campbell, R. E., Lin, J. Y., Lin, M. Z., Miyawaki, A., Palmer, A. E., Shu, X., Zhang, J., & Tsien, R. Y. (2017). The growing and glowing toolbox of fluorescent and photoactive proteins. Trends in Biochemical Sciences , 42(2), 111–129. https://doi.org/10.1016/j.tibs.2016.09.010
- Shimomura, O., Johnson, F. H., & Saiga, Y. (1962). Extraction, purification and properties of aequorin, a bioluminescent protein from the luminous hydromedusan, Aequorea. Journal of Cellular and Comparative Physiology , 59, 223–239. https://doi.org/10.1002/jcp.1030590302
- Staros, J. V., Wright, R. W., & Swingle, D. M. (1986). Enhancement by N-hydroxysulfosuccinimide of water-soluble carbodiimide-mediated coupling reactions. Analytical Biochemistry , 156(1), 220–222. https://doi.org/10.1016/0003-2697(86)90176-4
- Wang, L., Jackson, W. C., Steinbach, P. A., & Tsien, R. Y. (2004). Evolution of new nonantibody proteins via iterative somatic hypermutation. Proceedings of the National Academy of Sciences of the United States of America , 101(48), 16745–16749. https://doi.org/10.1073/pnas.0407752101