Establishment of Transgene-Free Porcine Induced Pluripotent Stem Cells
J. Vanessa Conrad, J. Vanessa Conrad, Jaime A. Neira, Jaime A. Neira, Margaret Rusteika, Margaret Rusteika, Susanne Meyer, Susanne Meyer, Dennis O. Clegg, Dennis O. Clegg, Li-Fang Chu, Li-Fang Chu
cellular reprogramming
episomal plasmid
porcine induced pluripotent stem cell
species-specific pluripotency
transgene-free
Abstract
Although protocols to generate authentic transgene-free mouse and human induced pluripotent stem cells (iPSCs) are now well established, standard methods for reprogramming porcine somatic cells still suffer from low efficiency and transgene retention. The Basic Protocol describes reprogramming procedures to establish transgene-free porcine iPSCs (PiPSCs) from porcine fibroblasts. This method uses episomal plasmids encoding POU5F1 , SOX2 , NANOG , KLF4 , SV40LT , c-MYC , LIN28A , and microRNA-302/367 , combined with an optimized medium, to establish PiPSC lines. Support protocols describe the establishment and characterization of clonal PiPSC lines, as well as the preparation of feeder cells and EBNA1 mRNA. This optimized, step-by-step approach tailored to this species enables the efficient derivation of PiPSCs in ∼4 weeks. The establishment of transgene-free PiPSCs provides a new and valuable model for studies of larger mammalian species’ development, disease, and regenerative biology. © 2024 The Authors. Current Protocols published by Wiley Periodicals LLC.
Basic Protocol : Reprogramming of porcine fibroblasts with episomal plasmids
Support Protocol 1 : Preparation of mouse embryonic fibroblasts for feeder layer
Support Protocol 2 : Preparation of in vitro –transcribed EBNA1 mRNA
Support Protocol 3 : Establishment of clonal porcine induced pluripotent stem cell (PiPSC) lines
Support Protocol 4 : PiPSC characterization: Genomic DNA PCR and RT-PCR
Support Protocol 5 : PiPSC characterization: Immunostaining
INTRODUCTION
The derivation of transgene-free induced pluripotent stem cells (iPSCs) enables the generation of a valuable tool for regenerative biology and its medical applications (Okita et al., 2011; Takahashi et al., 2007; Takahashi & Yamanaka, 2006; Yu et al., 2007, 2009). As Sus domesticus (pig) has comparable physiology, immunology, and organ size to humans, it is a befitting large mammalian model for biomedical studies (Bertho & Meurens, 2021; Duran-Struuck et al., 2019; Henze et al., 2019; Lunney et al., 2021). However, the direct application of established murine or primate reprogramming methods to the pig species has not been straightforward in successfully generating true and stable porcine iPSCs (PiPSCs). Numerous efforts in making PiPSCs have used retroviral, lentiviral, and transposon-based vectors, which results in the permanent integration of reprogramming vectors into the genome (Ezashi et al., 2009; Kues et al., 2013; Wu et al., 2009). However, these methods can compromise the genome and permit undesired reactivation of transgenes, making the cells unsuitable for further experiments (Fan et al., 2013; Galat et al., 2016; Kang et al., 2015; Popp et al., 2018). To prevent this, non-integrating reprogramming methods based on the transient, episomal expression of reprogramming factors have been attempted in porcine cells. Despite this non-integrative approach, transgene retention remains a challenge (Aravalli et al., 2012; Du et al., 2015; Montserrat et al., 2011; Park et al., 2013; Telugu et al., 2010). Recently, several studies have reported the generation of transgene-free PiPSCs (Conrad et al., 2023; Li et al., 2018; Yoshimatsu et al., 2021; Zhu et al., 2023). However, variabilities in reprogramming efficiency and episomal plasmid retention remain an obstacle in many methods.
This article describes an optimized method (Conrad et al., 2023) for the efficient derivation of transgene-free PiPSC colonies from porcine fibroblasts using episomal plasmids encoding human POU5F1 , SOX2 , NANOG , KLF4 , SV40LT , c-MYC , and LIN28A , along with microRNA-302/367 (Fig. 1; Basic Protocol). The reprogramming process takes ∼4 weeks from the electroporation of primary fibroblasts to the generation of primary PiPSC colonies. Using this method, we detected the expression of endogenous pluripotency factors without detectable episomal plasmids in clonal lines. Transgene-free PiPSCs can be amplified for many passages and used in further experiments. Additionally, support protocols (Fig. 1) describe the preparation of mouse embryonic fibroblasts (MEFs) used as a feeder layer during reprogramming and the maintenance of PiPSCs (Support Protocol 1), the preparation of EBNA1 mRNA (Support Protocol 2), the establishment of clonal lines (Support Protocol 3), the use of genomic DNA PCR to characterize the transgene status of PiPSCs, and the use of RT-PCR as well as immunostaining to verify the endogenous expression of key pluripotency factors (Support Protocols 4 and 5). Transgene-free PiPSCs can provide new insight into both the regulation of mammalian pluripotency and developmental timing mechanisms (Choi et al., 2019; Conrad et al., 2023; Gao et al., 2019; Jiao et al., 2022; Kinoshita et al., 2021; MacCarthy et al., 2024; Xu et al., 2020; Yoshimatsu et al., 2021; Zhi et al., 2022). Additionally, PiPSCs can serve as powerful models for a better understanding of development, disease, and regenerative biology.
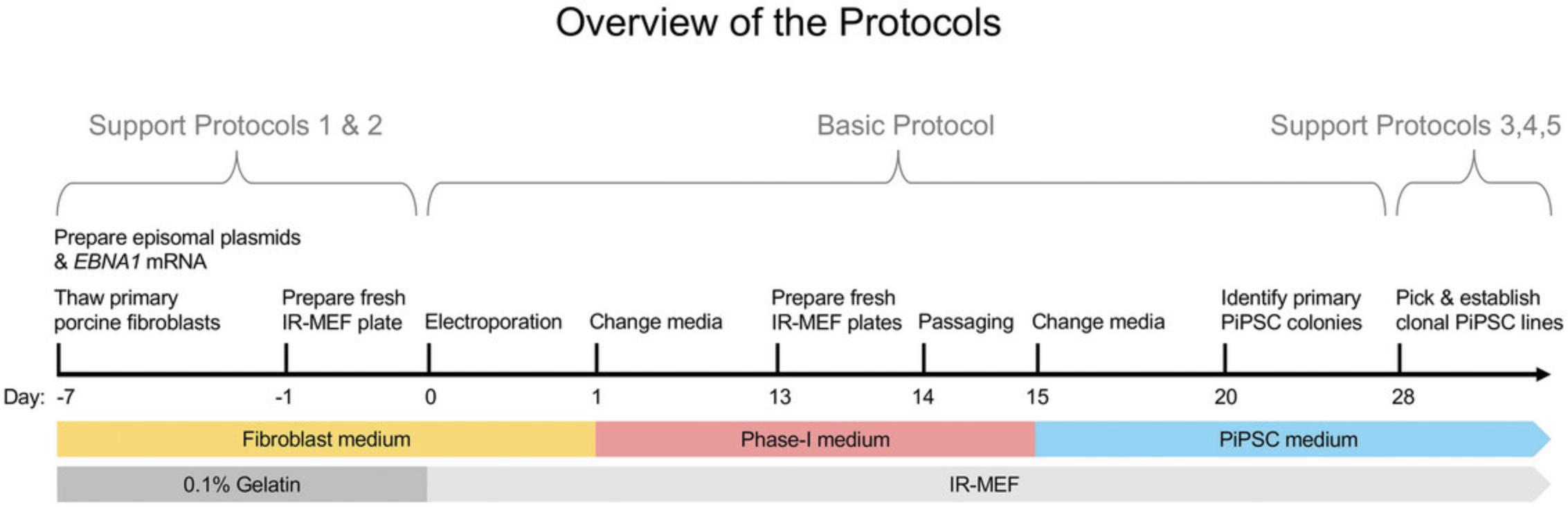
NOTE : All solutions and equipment coming in contact with cells must be sterile. All work with cells should be performed in a biosafety cabinet (Level 2 Biosafety Cabinet, NuAire, model no. NU-425-400). The surface of materials should be sprayed with 70% (v/v) ethanol (in distilled water) before being introduced into the biosafety cabinet.
NOTE : All cell culture should be maintained in an incubator with 37°C, 5% CO2, 20% O2 settings (Thermo Fisher Scientific, cat. no. 51026410).
NOTE : Experiments involving PCR and RNA require extremely careful technique to prevent contamination and RNA degradation.
NOTE : Coat all the necessary tissue culture flasks and plates with 0.1% (w/v) gelatin ≥1 hr prior to plating fibroblasts or irradiated MEFs. The approximate volumes of gelatin to be used are as follows: T75 flask, 12 ml; 4-well plate, 250 µl per well; 6-well plate, 2 ml per well; 12-well plate, 1 ml per well. Plates can be left at room temperature or in the incubator until use.
STRATEGIC PLANNING
Before commencing the reprogramming experiment (Basic Protocol), the preparation of irradiated MEFs (IR-MEFs; described in Support Protocol 1) and episomal plasmids and the in vitro transcription of EBNA1 mRNA (Support Protocol 2) must be completed, and these may be stored for future use. If amplifying primary MEFs, more advanced preparation and amplification may be required than those shown in Figure 1. The thawing of primary porcine fibroblasts must be completed close to the start of the reprogramming experiment. Fresh IR-MEF plates must be prepared within a few days before the start of reprogramming and the passaging of reprogrammed cells on day 14. Appropriate media should be prepared fresh as required. Colonies will be suitable for picking after ∼1 month and can then be amplified (Support Protocol 3) for cryopreservation and to analyze their genomic DNA and RNA. Characterization of these samples (Support Protocols 4 and 5) can be done following sample collection at one's convenience.
Basic Protocol: REPROGRAMMING OF PORCINE FIBROBLASTS WITH EPISOMAL PLASMIDS
This protocol describes the step-by-step reprogramming of porcine fibroblasts into PiPSCs using episomal plasmids encoding human POU5F1 , SOX2 , NANOG , KLF4 , SV40LT , c-MYC , LIN28 A, and microRNA-302/367.In vitro –transcribed (IVT) EBNA1 mRNA is also co-delivered. Fibroblasts are electroporated with these plasmids, grown in Phase-I medium for 2 weeks, passaged, and then grown in PiPSC medium adapted from the porcine embryonic stem cell medium (Choi et al., 2019). Using this optimized protocol tailored to this species enables the efficient derivation of primary PiPSC colonies. The reprogramming protocol takes ∼4 weeks from the electroporation of primary fibroblasts to the generation of primary PiPSC colonies. Before beginning this protocol, MEF feeder (Support Protocol 1), episomal plasmid mix (see recipe), and IVT EBNA1 mRNA (Support Protocol 2) must be prepared.
Materials
-
Primary porcine fibroblasts
-
Phosphate-buffered saline (PBS; Thermo Fisher Scientific, cat. no. 14190250)
-
TrypLE Express Enzyme (Thermo Fisher Scientific, cat. no.12605028)
-
PBS/FBS (see recipe)
-
0.4% (w/v) trypan blue solution (Thermo Fisher Scientific, cat. no.15250061)
-
Episomal plasmid mix (mixed at 1:1:1 ratio; see recipe)
-
pSimple-miRNA-302/367 (Addgene plasmid # 98748)
-
EBNA1 mRNA (see Support Protocol 2)
-
12-well IR-MEF plates (containing irradiated CD1 MEFs; see Support Protocol 1, step 14)
-
Phase-I medium (see recipe)
-
ROCK inhibitor (ROCKi; Y-27632, Bio-Techne, cat. no. 3532)
-
PiPSC medium (see recipe)
-
50-ml tubes (VWR, cat. no. 10025-698)
-
Hemocytometer (Hausser Scientific Bright-Line™ Phase Hemacytometer, Thermo Fisher Scientific, cat. no. 02-671-6)
-
Tissue culture microscope
-
Neon Transfection System:
- Neon Transfection System Pipette Station (Thermo Fisher Scientific, MPS100)
- Neon Transfection System Device (Thermo Fisher Scientific, MPK5000)
- Neon Transfection System Pipette (Thermo Fisher Scientific, MPP100)
-
Neon Transfection System 10 µl Kit (Thermo Fisher Scientific, MPK1096), containing electrolytic buffer, Neon electroporation tubes, and Neon tips
-
1.7-ml microcentrifuge tubes (VWR, cat. no. 10011-722)
-
15-ml tubes (VWR, cat. no. 10025-686)
-
5-ml serological pipets (VWR, cat. no. 76320-286)
-
Additional reagents and equipment for thawing cells into 0.1% gelatinized T75 flasks and for preparing 6-well cell culture plates (Thermo Fisher Scientific, cat. no. 08-772-1B) with IR-MEFs (see Support Protocol 1)
Day -7 to day 0: Preparation for electroporation of episomal plasmids to initiate cellular reprogramming
1.Thaw one vial of primary porcine fibroblasts into a 0.1% gelatinized T75 flask (∼1 × 106 cells per T75) containing fibroblast medium following the procedure from Support Protocol 1, steps 1 to 6.
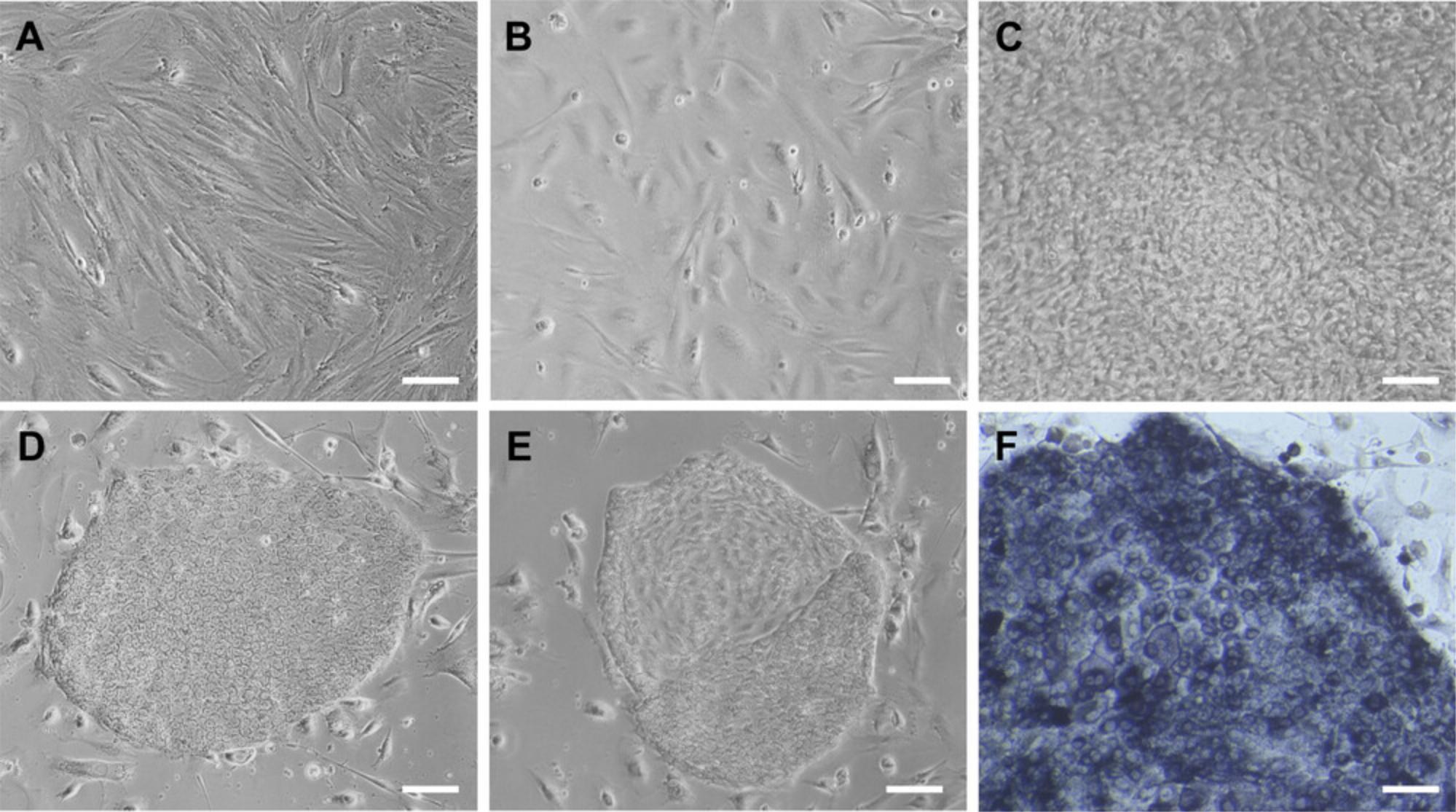
2.When ready for passaging, aspirate medium from plate and wash cells with 10 ml PBS twice.
3.Add 5 ml TrypLE Express Enzyme to dissociate cells and place plate in the incubator for 10 min.
4.Wash cells using PBS/FBS and collect into a 50-ml tube.
5.Centrifuge 5 min at 270 × g.
6.Remove supernatant, flick tube to break apart cell pellet, and resuspend cells in PBS/FBS.
7.Count cells with hemocytometer and tissue culture microscope, diluting cells in 0.4% trypan blue solution (1:1 ratio). Decide how many cells can be passaged or cryopreserved if there are more than necessary for electroporation.
8.Centrifuge cells again for 5 min at 270 × g , remove supernatant, and resuspend in PBS at the concentration calculated for the reprogramming experiment. Determine volumes of cell and plasmid/RNA solutions needed for each electroporation.
9.During centrifugation, set up the Neon Transfection System inside the hood. Add 3 ml electrolytic buffer from the Neon Transfection System 10 μl Kit to the Neon electroporation tube and place the tube inside the Neon Transfection System Pipette Station. Turn on the power for the Neon Transfection System Device. Set electroporation to program #20 (Pulse voltage: 1150, Pulse width: 30, Pulse number: 2) for electroporation.
10.In a 1.7-ml microcentrifuge tube, gently mix the episomal plasmid mix, pSimple-miRNA-302/367, EBNA1 mRNA, and concentrated cells according to predetermined volumes (see step 8).
11.Pipet 10 μl of the mixture from step 10 using Neon Transfection System Pipette and Neon tip and place into station.
12.Electroporate cells.
13.After each electroporation, pool cells into a 15-ml tube containing fibroblast medium (1 ml per electroporation) to mitigate possible variations between the individual electroporations.
14.After all electroporations are completed, distribute the cell-containing medium from step 13 equally between wells of a prepared 12-well IR-MEF plate (1 ml per well of a 12-well plate = 1 × 105 cells per well) and place into incubator.
15.The next day, switch to feeding with Phase-I medium every other day (2 ml per well). Monitor cell proliferation over the next 2 weeks (Fig. 2).
16.At least 1 day before passaging (see steps 17 to 25), prepare an appropriate number of 6-well cell culture plates with IR-MEFs at 2.5 × 106 cells per plate according to Support Protocol 1.
Day 14: Passaging
17.Aspirate medium from reprogramming plate and wash wells twice with PBS, 1 ml per well.
18.Add 500 μl TrypLE per well and incubate for approximately 10 to 15 min.
19.Check cells under microscope to see if cells are dissociating into single cells.
20.Draw up a few milliliters of PBS/FBS into 5-ml serological pipet and use this pipet to collect the cells and TrypLE into a 15- or 50-ml tube, pipetting up and down to singularize cells.
21.Rinse plate with PBS/FBS and collect this in the same tube.
22.Centrifuge cells for 5 min at 270 × g.
23.Remove supernatant and flick pellet.
24.Resuspend in Phase-I medium and add 5 μM ROCKi.
25.Passage cells into a fresh 6-well plate (see step 16) using a 1:8 split ratio to prepared 6-well plates of IR-MEFs.
26.The next day, remove the medium and replace it with PiPSC medium (2 ml per well). Feed every other day.
27.Between days 20 and 28 of the reprogramming experiment, identify distinct primary PiPSC colonies (Fig. 2D) and plan to pick individual colonies to establish clonal lines (see Support Protocol 3).
Support Protocol 1: PREPARATION OF MOUSE EMBRYONIC FIBROBLASTS FOR FEEDER LAYER
This protocol describes the preparation of MEFs used as a feeder layer during reprogramming and maintenance of PiPSCs (see Basic Protocol and Support Protocol 3). Using feeder layers supports the growth and survival of PiPSCs (Ezashi et al., 2009; Gao et al., 2014). MEFs are first amplified to a sufficient quantity and then irradiated to prevent further proliferation. These irradiated fibroblasts (IR-MEFs) can be frozen for later use or plated immediately for use the next day. Plated IR-MEFs are good for 2 weeks. Although it is preferable to use feeder-free conditions, our attempts at applying the Basic Protocol using Matrigel as the matrix were unsuccessful (unpub. observ.). Future studies will be required to optimize the protocol and medium to allow for feeder-free conditions.
NOTE : It is recommended to prepare the feeder plates ≥1 day before use and to use the feeder plates within 2 weeks of plating the IR-MEFs.
Materials
-
Primary CD1 MEFs (from Embryonic Stem Cell and Transgenic Core Services, University of Calgary)
-
Fibroblast medium (see recipe)
-
0.1% (w/v) gelatinized T75 flasks [T75 flasks (VWR, cat. no. 10062-860) pre-coated with 0.1% (w/v) gelatin (autoclaved, Sigma, cat. no. G1890-1KG)]
-
TrypLE Express Enzyme (Thermo Fisher Scientific, cat. no.12605028)
-
PBS/FBS (see recipe)
-
0.4% (w/v) trypan blue solution (Thermo Fisher Scientific, cat. no.15250061)
-
0.1% (w/v) gelatinized 12-well cell culture plates [12-well cell culture plates (Thermo Fisher Scientific, cat. no. 08-772-29) pre-coated with 0.1% (w/v) gelatin (autoclaved, Sigma, cat. no. G1890-1KG)]
-
Fibroblast freezing medium (see recipe)
-
37°C water bath
-
15-ml tubes (VWR, cat. no. 10025-686)
-
Standard tabletop centrifuge
-
50-ml tubes (VWR, cat. no. 10025-698)
-
Hemocytometer (Hausser Scientific Bright-Line™ Phase Hemacytometer, Thermo Fisher Scientific, cat. no. 02-671-6)
-
Tissue culture microscope
-
Irradiator (Gammacell 1000 Elite)
-
1.5-ml cryogenic tubes (Thermo Fisher Scientific, cat. no. 033377Y)
-
Mr. Frosty freezing container (Thermo Fisher Scientific, cat. no. 15-350-50)
-
Liquid nitrogen tank (Locator 6 Plus Liquid Nitrogen tank, Thermo Fisher Scientific, cat. no. CY50985-70)
Thawing
1.Thaw one vial of primary CD1 MEFs (∼1 × 106 cells per T75 flask) by placing the cryogenic tube in a 37°C water bath until thawed (1 to 2 min).
2.Transfer cells into a 15-ml tube containing 8 ml fibroblast medium.
3.Rinse cryogenic tube with 1 ml fibroblast medium and transfer wash to the same 15-ml tube.
4.Centrifuge 5 min at 270 × g.
5.Aspirate supernatant and flick pellet.
6.Add ∼15 ml fibroblast medium and transfer cells to 0.1% gelatinized T75 flask.
Amplification
7.Aspirate medium and rinse twice with PBS (∼10 ml each wash).
8.Add 5 ml TrypLE Express Enzyme to T75 flask and incubate for 10 min at 37°C.
9.Collect cells by adding ∼5 ml PBS/FBS to TrypLE and pipetting into 50-ml tube. Rinse T75 with ∼10 ml PBS/FBS once or twice to collect all cells.
10.Centrifuge 5 min at 270 × g.
11.Aspirate supernatant and resuspend pellet in fibroblast medium. If proceeding to irradiation, count cells with hemocytometer and tissue culture microscope, diluting cell sample in 0.4% trypan blue solution (1:1 ratio).
12.Amplify cells (1:3 or 1:6 split) or proceed to irradiation (see step 13).
Irradiation and freezing
13.Irradiate MEFs with a total dosage of 80 Gy irradiation using an irradiator.
14.After irradiation, plate cells immediately (2.5 × 106 cells per one 0.1% gelatinized 12-well cell culture plate or equivalent; see Fig. 2B) or cryopreserve for later use by centrifuging 5 min at 270 × g , removing supernatant, resuspending in fibroblast freezing medium, and transferring to 1.5-ml cryogenic tubes.
15.Place cryogenic tubes in a Mr. Frosty freezing container at −80°C for ≥2 hr. Then, transfer to liquid nitrogen tank for long-term storage.
Support Protocol 2: PREPARATION OF IN VITRO–TRANSCRIBED EBNA1 mRNA
This protocol describes the preparation of IVT EBNA1 mRNA for use alongside episomal plasmids in pig cell reprogramming (Basic Protocol). Co-delivering EBNA1 mRNA can increase the efficiency of reprogramming (Chen et al., 2011; Howden et al., 2006, 2015). SP6-EBNA1 plasmid is linearized with a restriction enzyme, purified after gel electrophoresis, and then subjected to in vitro transcription, at which point it is ready for use in reprogramming experiments (Basic Protocol).
Materials
-
EcoRI-HF (NEB, cat. no. R3101S)
-
Sp6-EBNA1 plasmid (Addgene plasmid # 98749)
-
10× rCutSmart buffer (NEB, cat. no. R3101S)
-
Nuclease-free water (Thermo Fisher Scientific, cat. no. AM9930)
-
6× gel-loading dye (NEB, cat. no. B7025S)
-
SYBRTM Safe DNA Gel Stain (Thermo Fisher Scientific, cat. no. S33102)
-
DNA ladder (NEB, cat. no. N0550S)
-
QIAQuick Gel Extraction Kit (Qiagen, cat. no. 28706)
-
mMACHINE™ SP6 Transcription Kit (Thermo Fisher Scientific, cat. no. AM1340)
-
1.7-ml microcentrifuge tubes (VWR, cat. no. 10011-722)
-
37°C water bath
-
Electrophoresis comb
-
Lab tape (Thermo Fisher Scientific, cat. no. 15901R)
-
UV light box
-
Balance
-
Plastic wrap
-
Razor blade (VWR, cat. no. 554111-055)
-
Additional reagents and equipment for 1% agarose gel electrophoresis [e.g., agarose (VWR, cat. no. 97064-258), 50× TAE electrophoresis buffer (Thermo Fisher Scientific, cat. no. J63931.K7), and electrophoresis system (Thermo Fisher Scientific, cat. no. FB-SBR-1316); see Current Protocols article: Voytas, 2000]
Preparation of template DNA
1.Combine the following mixture in a 1.7-ml microcentrifuge tube:
Reagent | Volume |
EcoRI-HF | 1 μl |
Sp6-EBNA1 plasmid | 50 μg |
10× rCutSmart buffer | 10 μl |
Nuclease-free water | to 100 μl |
Total reaction volume | 100 μl |
2.Incubate overnight in a 37°C water bath.
3.Stop the reaction by adding 10 μl of 6× gel-loading dye to 50-μl reaction.
Gel extraction and purification
4.Prepare a large-well electrophoresis comb by wrapping lab tape tightly around several teeth of the comb and use this to prepare a 1% agarose gel containing SYBRTM Safe DNA Gel Stain.
5.Run the sample and DNA ladder on 1% agarose gel for ≥40 min.
6.Verify size of band by viewing gel on UV light box (expected size 4.4 kb).
7.Pre-weigh a 1.7-ml microcentrifuge tube.
8.Extract DNA sample from gel by first placing a layer of plastic wrap on top of UV light box and then cutting the gel with razor blade as close to the sample as possible.
9.Place gel sample in the pre-weighed microcentrifuge tube from step 7.
10.Weigh the sample in the microcentrifuge tube and calculate the weight of the sample only.
11.Use a QIAQuick Gel Extraction Kit according to the manufacturer's instructions.
In vitro transcription of EBNA1
12.Use the mMACHINE™ SP6 Transcription Kit according to the manufacturer's protocol.
Support Protocol 3: ESTABLISHMENT OF CLONAL PORCINE INDUCED PLURIPOTENT STEM CELL (PiPSC) LINES
This protocol describes the process of picking colonies for further amplification and establishing clonal lines. Colonies observed to have good morphology are manually cut into smaller pieces, dissociated with Accutase, and then plated onto a fresh well pre-coated with IR-MEF feeder. These clonal lines can be amplified, cryopreserved, characterized to ensure they are fully reprogrammed, and then used for further experiments.
Materials
-
4-well cell culture plates (Thermo Fisher Scientific, cat. no. 144444) and 12-well cell culture plates (Thermo Fisher Scientific, cat. no. 08-772-29) with IR-MEFs (see Support Protocol 1)
-
PiPSC medium (see recipe)
-
5 μM ROCKi (Y-27632, Bio-Techne, cat. no. 3532)
-
Accutase (Thermo Fisher Scientific, cat. no. A1110501)
-
Plates of PiPSC colonies (see Basic Protocol)
-
PBS (Thermo Fisher Scientific, cat. no. 14190250)
-
TrypLE Express Enzyme (Thermo Fisher Scientific, cat. no.12605028; optional)
-
PBS/FBS (see recipe)
-
0.4% (w/v) trypan blue solution (Thermo Fisher Scientific, cat. no.15250061)
-
PiPSC freezing medium (see recipe)
-
96-well U-bottom microplates (Thermo Fisher Scientific, cat. no. 174925)
-
Tissue culture microscope
-
15-ml tubes (VWR, cat. no. 10025-686)
-
Standard tabletop centrifuge
-
Hemocytometer (Hausser Scientific Bright-Line™ Phase Hemacytometer, Thermo Fisher Scientific, cat. no. 02-671-6)
-
Additional reagents and equipment for cryopreserving cells (see Support Protocol 1, steps 14 and 15)
Picking colonies
1.Prepare a 4-well cell culture plate with IR-MEFs by adding 500 μl PiPSC medium and 5 μM ROCKi per well. Warm the medium by placing plate inside the incubator.
2.Determine the number of colonies to be picked from the plate of PiPSC colonies and then add 30 μl Accutase per well of a 96-well U-bottom microplate for each colony to be picked.
3.Aspirate medium from the wells of the plate to be picked and add PBS to each well (∼2 ml per well in a 6-well plate).
4.With a 10-μl pipet tip, carefully cut around the colony, separating it from feeder layer (the colony should still be attached to the plate). Using the edge of a new pipet tip, make cuts vertically and horizontally to cut the colony into small pieces.
5.Using a new pipet tip, transfer colony pieces into a well of the 96-well U-bottom microplate with Accutase (see step 2). Repeat steps 4 and 4 for additional colonies and then place plate in the incubator for 10 min.
6.Check under the tissue culture microscope to confirm cell dissociation. Then, add 70 μl PiPSC medium to each well and pipet up and down several times to further break down the colony, being careful not to introduce air bubbles.
7.Transfer each dissociated colony into one well each of the prepared 4-well plate from step 1.Use additional PiPSC medium to rinse the 96-well U-bottom microplate wells and transfer this medium to the 4-well plate to ensure transfer of all cells.
8.Carefully monitor the growth of picked clonal lines through daily observation under the microscope and check for confluency, signs of differentiation, and the absence of contamination. Amplify once colonies have grown sufficiently or are becoming confluent (70% to 90% confluent and before colonies grow into each other).
Colony maintenance and passaging
9.Aspirate medium. Rinse with PBS once (500 µl per well in a 4-well plate; 1 ml per well in a 12-well plate) and aspirate solution.
10.Incubate with TrypLE Express Enzyme or Accutase (250 µl per well in a 4-well plate; 500 µl per well in a 12-well plate) for 5 to 10 min at 37°C.
11.Neutralize the enzyme with PBS/FBS (∼500 µl per well in a 4-well plate; ∼1 ml per well in a 12-well plate) and collect solution in a 15-ml tube.
12.Centrifuge cells for 5 min at 270 × g.
13.Aspirate supernatant. Flick the bottom of 15-ml tube to break apart cell pellet.
14.Count cells if necessary (with hemocytometer and tissue culture microscope, diluting cells in 0.4% trypan blue solution at a 1:1 ratio) and passage cells at a 1:6 to 1:12 ratio on fresh IR-MEF feeder plates (usually 12-well plates for earlier passages) in PiPSC medium and 5 μM ROCKi overnight.
15.The next day, begin daily feeding with PiPSC medium only (without ROCKi).
16.Cryopreserve clonal PiPSC lines in PiPSC freezing medium according to Support Protocol 1, steps 14 and 15, as soon as possible to avoid loss of established clonal lines.
Support Protocol 4: PiPSC CHARACTERIZATION: GENOMIC DNA PCR AND RT-PCR
This protocol describes steps for genomic DNA PCR to determine the presence of episomal plasmids and steps for RT-PCR to determine the endogenous expression of pluripotency factors. This is necessary to ensure colonies are fully reprogrammed before using them in new experiments. Cell samples can be purified using the DNeasy Blood and Tissue Kit (Qiagen, cat. no. 69506), RNeasy Kit (Qiagen, cat. no. 74104), or Allprep DNA/RNA Mini Kit (Qiagen, cat. no. 80204) beforehand.
Materials
- GoTaq 2× Master Mix (Promega, cat. no. M7123)
- DNA oligos (all synthesized by Integrated DNA Technologies; Table 1)
- Genomic DNA/RNA (from purified DNA/RNA PiPSC and fibroblast samples)
- SuperScript IV VILO Master Mix (Thermo Fisher Scientific, cat. no. 11756050)
- Nuclease-free water (Thermo Fisher Scientific, cat. no. AM9930)
Oligo name | Oligo sequence 5′-3′ | Product size | Source |
---|---|---|---|
pig GAPDH qPCR and RT-PCR - forward | TGCTCCTCCCCGTTCGAC | 100 | Choi et al., 2019, Synthesized by IDT |
pig GAPDH qPCR and RT-PCR - reverse | ATGCGGCCAAATCCGTTC | 100 | Choi et al., 2019, Synthesized by IDT |
pig POU5F1 qPCR and RT-PCR - forward | CTTGGAGAGCCCTGGTTTTACT | 159 | Choi et al., 2019, Synthesized by IDT |
pig POU5F1 qPCR and RT-PCR - reverse | GCCAGGTCCGAGGATCAAC | 159 | Choi et al., 2019, Synthesized by IDT |
pig NANOG qPCR and RT-PCR - forward | CATCTGCTGAGACCCTCGAC | 195 | Choi et al., 2019, Synthesized by IDT |
pig NANOG qPCR and RT-PCR - reverse | GGGTCTGCGAGAACACAGTT | 195 | Choi et al., 2019, Synthesized by IDT |
pig SOX2 qPCR and RT-PCR - forward | CGGCGGTGGCAACTCTAC | 100 | Choi et al., 2019, Synthesized by IDT |
pig SOX2 qPCR and RT-PCR - reverse | TCGGGACCACACCATGAAAG | 100 | Choi et al., 2019, Synthesized by IDT |
Episomal EBNA1- PCR - forward | ATCGTCAAAGCTGCACACAG | 666 | Yu et al., 2009, Synthesized by IDT |
Episomal EBNA1- PCR - reverse | CCCAGGAGTCCCAGTAGTCA | 666 | Yu et al., 2009, Synthesized by IDT |
Episomal OriP - PCR - forward | TTCCACGAGGGTAGTGAACC | 544 | Yu et al., 2009, Synthesized by IDT |
Episomal OriP - PCR - reverse | TCGGGGGTGTTAGAGACAAC | 544 | Yu et al., 2009, Synthesized by IDT |
-
PCR strip tubes and caps (VWR, cat. no. 76318-802)
-
Mini centrifuge
-
Thermocycler (Eppendorf, cat. no. 6331000025)
-
Gel imager
-
Additional reagents and equipment for 1% agarose gel electrophoresis (see Support Protocol 2; see Current Protocols article: Voytas, 2000)
Genomic DNA PCR
1.Prepare PCR reactions following manufacturer's instructions for GoTaq 2× Master Mix and using the appropriate DNA oligos. Prepare master mixes with each primer set, accounting for the number of samples, the positive and negative controls, and volume loss.
2.Dispense master mixes into PCR strip tubes.
3.Add 50 ng genomic DNA sample as template to the master mix. Cap tubes.
4.Mix gently by flicking tube strips and then centrifuge briefly in a mini centrifuge to ensure all contents are at the bottom of the tubes.
5.Amplify with thermocycler with the following settings:
Initial step: | 2 min | 95°C | (initial denaturation) |
35 cycles: | 30 s | 95°C | (denaturation) |
30 s | 55°C | (annealing) | |
30 s | 72°C | (extension) | |
Additional step: | 15 min | 72°C | (final extension) |
Final step: | indefinitely | 10°C | (hold). |
6.Run 10 μl PCR product on a prepared 1% agarose gel at 120 V for 30 min with an appropriate DNA ladder.
7.Image the gel using a gel imager and verify that the bands are at the correct position according to the predicted size.
RT-PCR
8.Prepare RNA samples for reverse transcription according to the manufacturer's instructions for SuperScript IV VILO Master Mix.
9.After combining nuclease-free water, master mix, and RNA samples in PCR strip tubes, mix gently by flicking tube strips and centrifuge briefly.
10.Run RT-PCR on a thermocycler with the following settings:
Initial step: | 10 min | 25°C | (primer annealing) |
Second step: | 10 min | 50°C | (DNA polymerization) |
Third step: | 5 min | 85°C | (enzyme deactivation) |
Final step: | indefinitely | 10°C. | (hold) |
11.Dilute cDNA sample 1:5 with nuclease-free water (add 40 μl water to 10 μl sample).
12.Using the cDNA samples, follow the same steps as for genomic DNA PCR above (see steps 1 to 7) to prepare master mixes and perform electrophoresis.
Support Protocol 5: PiPSC CHARACTERIZATION: IMMUNOSTAINING
This protocol describes the process of immunostaining to verify protein expression of pluripotency factors POU5F1, SOX2, and NANOG.
Materials
-
PiPSCs (see Support Protocol 3)
-
Glass-bottom chambered culture dishes (Nunc Lab-TekTM II Chambered Coverglass, Thermo Fisher Scientific, cat. no. 155409) with IR-MEFs (see Support Protocol 1)
-
PBS (Thermo Fisher Scientific, cat. no. 14190250)
-
BD Cytofix (Thermo Fisher Scientific, cat. no. BD554714)
-
0.2% (v/v) TritonX-100 (Sigma, cat. no. X100) in PBS (Thermo Fisher Scientific, cat. no. 14190250)
-
Blocking buffer (see recipe)
-
Primary antibodies:
-
Anti-POU5F1/OCT4 (Santa Cruz, cat. no. sc-5279)
-
Anti-SOX2 (eBioscience, cat. no. 14-9811-82)
-
Anti-NANOG (PeproTech, cat. no. 500-P236)
-
AlexaFluor-labeled secondary antibodies:
-
AlexaFluor 488 anti-Mouse IgG (Thermo Fisher Scientific, cat. no. A-21200)
-
AlexaFluor 568 anti-Rabbit IgG (Thermo Fisher Scientific, cat. no. A-10042)
-
AlexaFluor 647 anti-Rat IgG (Thermo Fisher Scientific, cat. no. A-21472)
-
Hoechst 33342 (Sigma, cat. no. B2261)
-
Inverted fluorescence microscope (Observer.Z1, Zeiss, cat. no. 38340003367)
Fixing
1.Seed PiPSCs onto glass-bottom chambered culture dishes with IR-MEFs (∼0.05 × 106 cells per well).
2.Once cells have grown sufficiently, aspirate medium and wash cells with PBS once.
3.Fix cells by adding BD Cytofix (250 μl per well) and placing dish at 4°C for 15 min.
4.Remove the fixative and wash the samples three times with PBS.
Immunostaining
5.Permeabilize cells with 0.2% TritonX-100 in PBS (250 μl per well) at room temperature for 30 min.
6.Remove permeabilization solution and block cells with blocking buffer for 1 hr at room temperature.
7.Combine the following primary antibodies in blocking buffer at the following dilutions: anti-POU5F1/OCT4, 1:100; anti-SOX2, 1:500; and anti-Nanog, 1:400.
8.Remove blocking buffer and stain cells by adding primary antibody solution (250 μl per well) and leaving at 4°C overnight.
9.The next day, remove antibodies and wash cells three times with blocking buffer (∼250 μl per wash).
10.Add appropriate AlexaFluor-labeled secondary antibodies (1:1000 dilution) and Hoechst 33342 (1:1000) in 1 ml blocking buffer.
11.Add secondary antibody solution (250 μl per well) to cells and leave for 2 hr at room temperature covered from light.
12.Remove secondary antibody solution and wash wells by adding blocking buffer for 10 min, removing wash, and repeating twice more.
13.Image samples with an inverted fluorescence microscope or store at 4°C.
REAGENTS AND SOLUTIONS
Blocking buffer
- 500 ml PBS (Thermo Fisher Scientific, cat. no. 14190250) [97% (v/v) final]
- 10 ml bovine serum albumin (BSA; Sigma, cat. no. A7030-100G) [2% (v/v) final]
- 5 ml fetal bovine serum (FBS), heat inactivated (Thermo Fisher Scientific, cat. no. SH30396.03) [1% (v/v) final]
- Store ≤6 months at 4°C
Episomal plasmid mix
- pEP4-E02SEN2K (Addgene plasmid # 20925)
- pCEP4-M2L (Addgene plasmid # 20926)
- pEP4-E02SET2K (Addgene plasmid # 20927)
- Precipitate and concentrate at 1:1:1 ratio, aiming for final concentration of 1.0 μg/μl
- Store at 4°C if in use or freeze at −20°C for long-term storage
Fibroblast freezing medium
- 5 ml dimethyl sulfoxide (DMSO; Sigma, cat. no. D2650-100ML) [10% (v/v) final]
- 20 ml fibroblast medium (see recipe) [40% (v/v) final]
- 25 ml FBS, heat inactivated (Thermo Fisher Scientific, cat. no. SH30396.03) [50% (v/v) final]
- Prepare in biosafety cabinet and filter (0.22 μm; 250- and 500-ml 0.22-μm filters, Sigma, cat. no. S2GPU02RE and S2GPU05RE) before use
- Store ≤1 month at 4°C
Fibroblast medium
- 25 ml FBS, heat inactivated (Thermo Fisher Scientific, cat. no. SH30396.03) [10% (v/v) final]
- 225 ml DMEM (Thermo Fisher Scientific, cat. no. 11965118) [90% (v/v) final]
- Prepare in biosafety cabinet and filter (0.22 μm; 250- and 500-ml 0.22-μm filters, Sigma, cat. no. S2GPU02RE and S2GPU05RE) before use
- Store ≤1 month at 4°C
Phase-I medium
- 225 ml DMEM/F12 (Thermo Fisher Scientific, cat. no. 11330057) [90% (v/v)]
- 25 ml FBS, stem cell qualified (Sigma, cat. no. ES-009-B) [10% (v/v)]
- 100 ng/ml FGF2-G3 (Recombinant Protein Production Core, Northwestern University)
- 3 μM CHIR99021 (Bio-Techne, cat. no. 4423)
- 5 μg/ml insulin (Gibco, cat. no. A11382II)
- 1 μg/ml transferrin (Invitria, cat. no. 777TRF029)
- 20 ng/ml sodium selenite (Sigma, cat. no. S5261)
- Prepare in biosafety cabinet and filter (0.22 μm; 250- and 500-ml 0.22-μm filters, Sigma, cat. no. S2GPU02RE and S2GPU05RE) before use
- Store ≤1 month at 4°C
PiPSC freezing medium
- 5 ml DMSO (Sigma, cat. no. D2650-100ML) [10% (v/v) final]
- 20 ml PiPSC medium (see recipe) [40% (v/v) final]
- 25 ml FBS, stem cell qualified (Sigma, cat. no. ES-009-B) [50% (v/v) final]
- Prepare in biosafety cabinet and filter (0.22 μm; 250- and 500-ml 0.22-μm filters, Sigma, cat. no. S2GPU02RE and S2GPU05RE) before use
- Store ≤1 month at 4°C
PiPSC medium
- 207.5 ml KO-DMEM (Thermo Fisher Scientific, cat. no. 10829018) [85% (v/v) final]
- 37.5 ml KnockOut Serum Replacement (KOSR; Thermo Fisher Scientific, cat. no. 10828028) [15% (v/v) final]
- 2.5 ml non-essential amino acids (Thermo Fisher Scientific, cat. no. 11140050) [1× final]
- 2.5 ml GlutaMAX (Thermo Fisher Scientific, cat. no. 35050061) [1× final]
- 250 μl Chemically Defined Lipid Concentrate (CDL; Thermo Fisher Scientific, cat. no. 11905031) [0.1% (v/v) final]
- 20 ng/ml FGF2-G3 (Recombinant Protein Production Core, Northwestern University)
- 5 ng/ml Activin A (Bio-Techne, cat. no. 338-AC-500)
- 1.5 μM CHIR99021 (Bio-Techne, cat. no. 4423)
- 2.5 μM Endo-IWR1 (Bio-Techne, cat. no. 3532)
- 0.1 mM β-ME (Sigma, cat. no. M3148)
- Prepare in biosafety cabinet and filter (0.22 μm; 250- and 500-ml 0.22-μm filters, Sigma, cat. no. S2GPU02RE and S2GPU05RE) before use
- Store ≤1 month at 4°C
PBS/FBS
- 500 ml PBS (Thermo Fisher Scientific, cat. no. 14190250) [99% (v/v) final]
- 5 ml FBS, stem cell qualified (Sigma, cat. no. ES-009-B) [1% (v/v) final]
- Prepare in biosafety cabinet and filter (0.22 μm; 250- and 500-ml 0.22-μm filters, Sigma, cat. no. S2GPU02RE and S2GPU05RE) before use
- Store ≤2 months at 4°C
COMMENTARY
Background Information
The genesis of porcine reprogramming can be traced back to 2009, when multiple groups reported the successful reprogramming of porcine somatic cells by integrating exogenous reprogramming factors into the host cell genome using viral vectors (Esteban et al., 2009; Ezashi et al., 2009; Wu et al., 2009). Concurrently, progress was also being made with non-integrative reprogramming methods based on the transient, episomal expression of reprogramming factors in humans (Okita et al., 2011; Yu et al., 2009). These techniques were soon extended to the porcine model, with the first report of episomal porcine reprogramming emerging the following year (Telugu et al., 2010). However, transgene retention in this and other early reports underscored a persistent challenge for the field (Aravalli et al., 2012; Du et al., 2015; Montserrat et al., 2011; Park et al., 2013). The potential benefits of non-integrative (transgene-free) reprogramming were already well established, as integrative methods can suffer from persistent transgene expression or re-activation, which can influence differentiation (Yu et al., 2007) and raise the risk of tumorigenesis (Okita et al., 2007) or immunogenicity (Zhao et al., 2011). However, despite efforts over the following years (Aravalli et al., 2012; Bui et al., 2012; Congras et al., 2016; Juhasova et al., 2015; Park et al., 2013; Strnadel et al., 2018), most reprogramming reports continued to be performed using integrative strategies, such as retrovirus- (as in Wu et al., 2009), lentivirus- (as in Ezashi et al., 2009), or transposon-mediated (as in Kues et al., 2013) reprogramming, rather than episomal plasmids. The lingering challenges of low reprogramming efficiency and episomal plasmid retention (Okita et al., 2008; Du et al., 2015; Telugu et al., 2010) remained persistent obstacles. A major step forward emerged with the adoption of episomal delivery of the miRNA cluster miRNA-302/367 , which resulted in a pronounced improvement in reprogramming efficiency (Anokye-Danso et al., 2011; Du et al., 2015; Howden et al., 2015; Lin et al., 2008; Miyoshi et al., 2011). Recently, several studies have reported the generation of transgene-free PiPSCs (Conrad et al., 2023; Li et al., 2018; Yoshimatsu et al., 2021; Zhu et al., 2023). However, a detailed, step-by-step reprogramming protocol tailored for swine has not yet been reported. Thus, we hope to bridge this gap by establishing a contemporary technique that incorporates well-established episomal reprogramming plasmids along with updated methods to successfully and efficiently establish transgene-free PiPSCs, as we have recently demonstrated (Conrad et al., 2023). This detailed, step-by-step approach will enable researchers to establish integration-free PiPSCs, harnessing the practical advantages of modern techniques and the translational advantages of true transgene-free reprogramming.
Critical Parameters
Optimal and proper starting materials are essential for the successful generation of PiPSCs (Basic Protocol). The preparation of nucleic acids and EBNA1 mRNA (Support Protocol 2) must be completed properly, and these should be stored appropriately (the IVT EBNA1 mRNA is sensitive and should be kept on ice when in use). Irradiated feeder cells must be plated at an appropriate density to support correct PiPSC morphology and survival (Support Protocol 1; Fig. 2B). Low densities could be detrimental to PiPSC growth. The starting porcine fibroblasts should exemplify a healthy morphology and proliferation; if the passage number is too high, earlier-passage-number fibroblasts should be used instead. Please note that the origin of the primary fibroblasts (passage number, age, sex) may cause the cells to respond differently to the Basic Protocol. The batch-specific reprogramming efficiency will need to be determined experimentally. Our medium system is optimized for pig reprogramming and should result in the production of primary PiPSC colonies. Readers interested in other conditions tested during optimization can find more details in the primary research paper associated with this basic protocol (Conrad et al., 2023).
Troubleshooting
Problems that may arise at different steps and their possible solutions are listed in Table 2.
Problem | Possible cause | Solution |
---|---|---|
Difficult to singularize passaged cells when passaging (Basic Protocol, step 20) |
Over-confluency of cells | • Use 1000-μl pipet tip to break down the large cell clumps by pipetting up and down several times. |
Primary PiPSC colony too heterogenous (Fig. 2D) (Basic Protocol, step 27) |
Colonies were not picked early enough, well was over-confluent, or medium was not changed frequently enough |
• Identify the primary colonies earlier than day 28. • Avoid waiting until colonies are too large, which could trigger spontaneous differentiation. • Change PiPSC medium feeding schedule from every other day to daily feeding. |
MEFs slow to amplify (Support Protocol 1) |
MEFs were seeded at too low of a density or MEFs are of a high passage number |
• Seed cells at a higher density. • If fibroblasts are of a higher passage, use lower-passage cells. |
IR-MEF density too low (Support Protocol 1) |
Cell death, inaccurate cell count |
• Adjust cell density. It is critical to have optimal feeder layer density to support PiPSC reprogramming and maintenance. |
Picked colonies have low survival (Support Protocol 3) | Colonies are picked too early and/or when they are too small |
• Pick sufficiently sized colonies to enhance survival. We estimate that a colony that contains less than 100 cells is too early for picking. • Ensure IR-MEF feeder is adequate and that ROCKi is added to the medium overnight. • It is normal for some colonies not to survive after picking. |
Understanding Results
Reprogramming of porcine fibroblasts (Basic Protocol)
Following this protocol, primary PiPSC colonies can be obtained around the third week (about 20th to 25th day post-electroporation) of reprogramming experiments (Fig. 2C). The number of primary colonies generated per experiment highly depends on the condition of each batch of starting material, including the primary porcine fibroblasts and IR-MEF feeder conditions. During the first 2 weeks of reprogramming, cells are highly proliferative and there are usually no distinct colonies visible. We observed that including miRNA-302/367 plasmids in the episomal electroporation greatly increased the number of colonies formed (Conrad et al., 2023).
Preparation of MEFs for feeder layer (Support Protocol 1)
The IR-MEF feeder should be at a density sufficient to support correct PiPSC morphology and survival (Fig. 2B). During the amplification of MEFs, cells should be able to be split at a ratio of around 1:3 to 1:6 and continue to be amplified over several passages. Proliferation may decrease as the passage number increases beyond P6. At this point, earlier-passage MEFs should be used.
Preparation of in vitro–transcribed EBNA1 mRNA (Support Protocol 2)
After following the protocol for linearization of template DNA, gel purification, and in vitro transcription, a sufficient quantity (500 to 600 μg) of EBNA1 mRNA should be acquired for use in future reprogramming experiments.
Establishment of clonal PiPSC lines (Support Protocol 3)
Through this protocol, several clonal lines can be established. Within a few days after picking, small colonies should be visible. Depending on their growth rate, they could be large enough to amplify in 1 week and can be cryopreserved after approaching confluency.
Characterization of the pluripotency of PiPSCs (Support Protocols 4 and 5)
It is prudent to carefully establish and characterize clonal PiPSC lines free of episomal plasmids. Genomic PCR is used to detect the presence of episomal EBNA1 and OriP sequences in the PiPSC lines (Support Protocol 4; Fig. 3A), which are anticipated to be negative beyond P10 to P20. If PiPSCs are positive for these transgenes, it may indicate a continued reliance on the episomal plasmids. Early-passage colonies are more likely to contain these sequences but should ultimately be negative if they are fully reprogrammed. An RT-PCR assay detects porcine endogenous gene expression of pluripotency markers such as POU5F1 and NANOG (Support Protocol 4; Fig. 3B). All PiPSC lines should be positive for these markers; sometimes, fibroblasts may also be very weakly positive by RT-PCR. The absence of endogenous pluripotency marker expression could be another indicator of incomplete reprogramming. An immunofluorescence assay for POU5F1, NANOG, and SOX2 (Support Protocol 5; Fig. 3C) also verifies the expression of these genes at the protein level. Established clonal lines should be tested for their capacity to differentiate into derivatives representing the primary germ layers in vitro and to form teratomas in immunocompromised mice (Conrad et al., 2023).
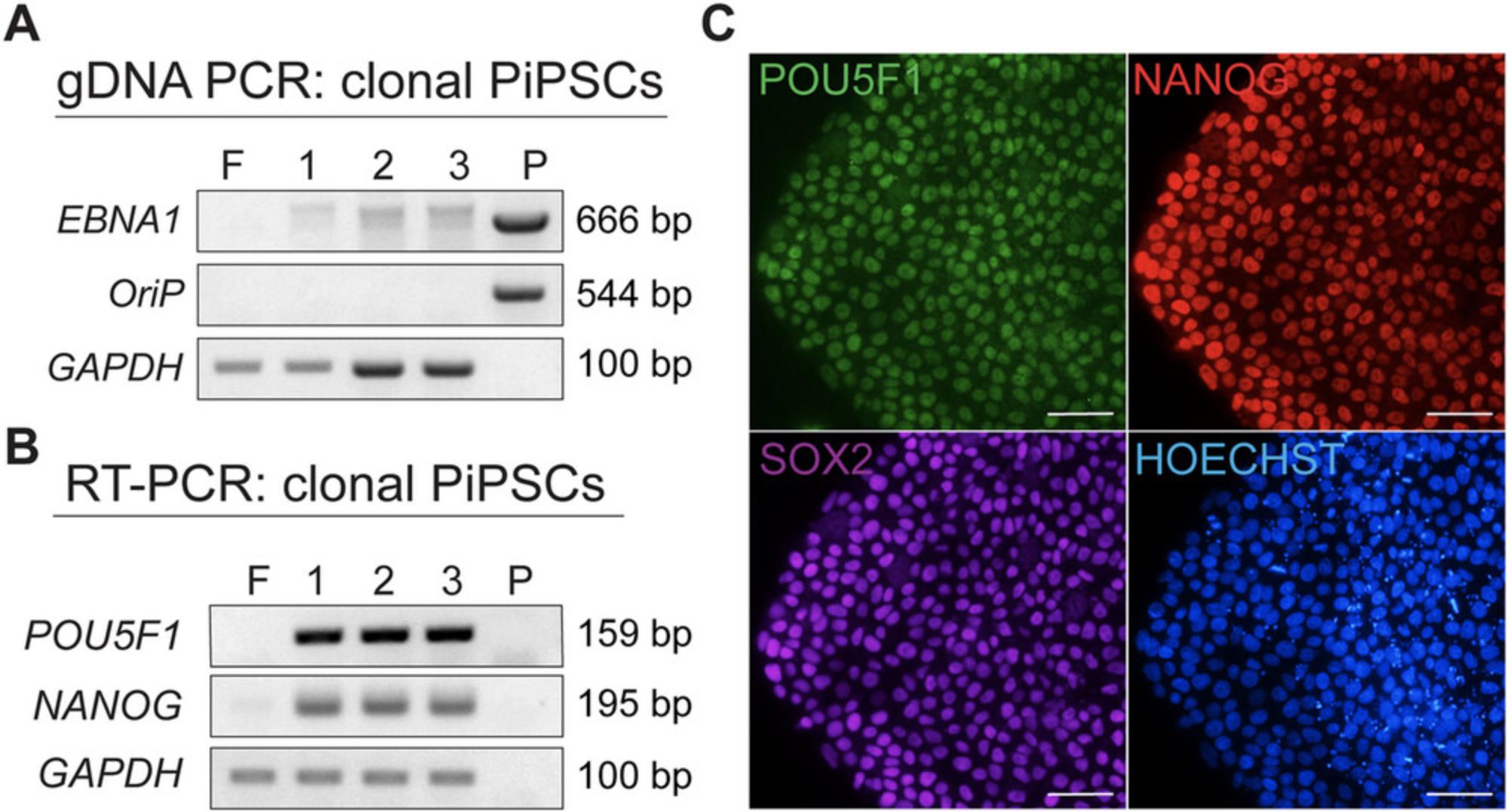
Time Considerations
Basic Protocol
One round of reprogramming experiment to obtain primary colonies: ∼30 days
Initial electroporation: 1 hr
Day 14 passaging: 1 hr
Support Protocol 1
Thawing primary MEFs: 30 min
Time to confluency: 5 to 7 days
Passaging MEFs: 30 to 60 min
MEF irradiation: 30 min
Cryopreservation: 30 min
Support Protocol 2
Preparation of template DNA: 10 min setup, left overnight
Gel extraction and purification: 2 hr
In vitro transcription: 5 hr
Support Protocol 3
Picking PiPSC colonies: 30 to 60 min
Passaging PiPSCs: 30 min
Support Protocol 4
PCR: 2.5 hr
Electrophoresis: 45 min
Support Protocol 5
Immunostaining: 2 days (∼2 hr each day)
Acknowledgments
We thank all members of the Chu Laboratory for their helpful comments on this study. This research was undertaken thanks to funding support from the Faculty of Veterinary Medicine, University of Calgary (UCVM); Alberta Children's Hospital Research Institute (ACHRI); Canada Research Chairs Program (CRC, L-FC, 950-232985); Natural Sciences and Engineering Research Council of Canada (NSERC, L-FC, RGPIN-2021-02580); and Government of Canada's New Frontiers in Research Fund (NFRFE-2020-00446, NFRFE-2023-00170).
Author Contributions
J. Vanessa Conrad : Conceptualization; data curation; formal analysis; investigation; methodology; project administration; validation; visualization; writing—original draft; writing—review and editing. Jaime A. Neira : Conceptualization; investigation; methodology; validation; writing—original draft; writing—review and editing. Margaret Rusteika : writing—critical review and commentary on revised manuscript. Susanne Meyer : Verification—method replication; writing—critical review and commentary on revised manuscript. Dennis O. Clegg : writing—critical review and commentary on revised manuscript. Li-Fang Chu : Conceptualization; data curation; formal analysis; funding acquisition; investigation; methodology; project administration; resources; software; supervision; validation; visualization; writing—original draft; writing—review and editing.
Conflict of Interest
We declare no conflict of interest.
Open Research
Data Availability Statement
Data sharing is not applicable to this article as no new data were created or analyzed in this study.
Literature Cited
- Anokye-Danso, F., Trivedi, C. M., Juhr, D., Gupta, M., Cui, Z., Tian, Y., Zhang, Y., Yang, W., Gruber, P. J., Epstein, J. A., & Morrisey, E. E. (2011). Highly efficient miRNA-mediated reprogramming of mouse and human somatic cells to pluripotency. Cell Stem Cell , 8, 376–388. https://doi.org/10.1016/j.stem.2011.03.001
- Aravalli, R. N., Cressman, E. N. K., & Steer, C. J. (2012). Hepatic differentiation of porcine induced pluripotent stem cells in vitro. The Veterinary Journal , 194, 369–374. https://doi.org/10.1016/j.tvjl.2012.05.013
- Bertho, N., & Meurens, F. (2021). The pig as a medical model for acquired respiratory diseases and dysfunctions: An immunological perspective. Molecular Immunology , 135, 254–267. https://doi.org/10.1016/j.molimm.2021.03.014
- Bui, H.-T., Kwon, D.-N., Kang, M.-H., Oh, M.-H., Park, M.-R., Park, W.-J., Paik, S.-S., Van Thuan, N., & Kim , J.-H. (2012). Epigenetic reprogramming in somatic cells induced by extract from germinal vesicle stage pig oocytes. Development , 139(23), 4330–4340. https://doi.org/10.1242/dev.086116
- Chen, G., Gulbranson, D. R., Hou, Z., Bolin, J. M., Ruotti, V., Probasco, M. D., Smuga-Otto, K., Howden, S. E., Diol, N. R., Propson, N. E., Wagner, R., Lee, G. O., Antosiewicz-Bourget, J., Teng, J. M. C., & Thomson, J. A. (2011). Chemically defined conditions for human iPSC derivation and culture. Nature Methods , 8, 424–429. https://doi.org/10.1038/nmeth.1593
- Choi, K. H., Lee, D. K., Kim, S. W., Woo, S. H., Kim, D. Y., & Lee, C. K. (2019). Chemically defined media can maintain pig pluripotency network in vitro. Stem Cell Reports , 13, 221–234. https://doi.org/10.1016/j.stemcr.2019.05.028
- Congras, A., Barasc, H., Canale-Tabet, K., Plisson-Petit, F., Delcros, C., Feraud, O., Oudrhiri, N., Hadadi, E., Griscelli, F., Bennaceur-Griscelli, A., Turhan, A., Afanassieff, M., Ferchaud, S., Pinton, A., Yerle-Bouissou, M., & Acloque, H. (2016). Non integrative strategy decreases chromosome instability and improves endogenous pluripotency genes reactivation in porcine induced pluripotent-like stem cells. Scientific Reports , 6, 27059. https://doi.org/10.1038/srep27059
- Conrad, J. V., Meyer, S., Ramesh, P. S., Neira, J. A., Rusteika, M., Mamott, D., Duffin, B., Bautista, M., Zhang, J., Hiles, E., Higgins, E. M., Steill, J., Freeman, J., Ni, Z., Liu, S., Ungrin, M., Rancourt, D., Clegg, D. O., Stewart, R., … Chu, L. F. (2023). Efficient derivation of transgene-free porcine induced pluripotent stem cells enables in vitro modeling of species-specific developmental timing. Stem Cell Reports , 18(12), 2328–2343. https://doi.org/10.1016/j.stemcr.2023.10.009
- Du, X., Feng, T., Yu, D., Wu, Y., Zou, H., Ma, S., Feng, C., Huang, Y., Ouyang, H., Hu, X., Pan, D., Li, N., & Wu, S. (2015). Barriers for deriving transgene-free pig iPS cells with episomal vectors. Stem Cells , 33, 3228–3238. https://doi.org/10.1002/stem.2089
- Duran-Struuck, R., Huang, C. A., & Matar, A. J. (2019). Cellular therapies for the treatment of hematological malignancies; Swine are an ideal preclinical model. Frontiers in Oncology , 9, 418. https://doi.org/10.3389/fonc.2019.00418
- Esteban, M. A., Xu, J., Yang, J., Peng, M., Qin, D., Li, W., Jiang, Z., Chen, J., Deng, K., Zhong, M., Cai, J., Lai, L., & Pei, D. (2009). Generation of induced pluripotent stem cell lines from Tibetan miniature pig. Journal of Biological Chemistry , 284, 17634–17640. https://doi.org/10.1074/jbc.M109.008938
- Ezashi, T., Telugu, B. P. V. L., Alexenko, A. P., Sachdev, S., Sinha, S., & Roberts, R. M. (2009). Derivation of induced pluripotent stem cells from pig somatic cells. Proceedings of the National Academy of Sciences , 106, 10993–10998. https://doi.org/10.1073/pnas.0905284106
- Fan, N., Chen, J., Shang, Z., Dou, H., Ji, G., Zou, Q., Wu, L., He, L., Wang, F., Liu, K., Liu, N., Han, J., Zhou, Q., Pan, D., Yang, D., Zhao, B., Ouyang, Z., Liu, Z., Zhao, Y., … Lai, L. (2013). Piglets cloned from induced pluripotent stem cells. Cell Research , 23, 162–166. https://doi.org/10.1038/cr.2012.176
- Galat, V., Galat, Y., Perepitchka, M., Jennings, L. J., Iannaccone, P. M., & Hendrix, M. J. C. (2016). Transgene reactivation in induced pluripotent stem cell derivatives and reversion to pluripotency of induced pluripotent stem cell-derived mesenchymal stem cells. Stem Cells and Development , 25, 1060–1072. https://doi.org/10.1089/scd.2015.0366
- Gao, X., Nowak-Imialek, M., Chen, X., Chen, D., Herrmann, D., Ruan, D., Chen, A. C. H., Eckersley-Maslin, M. A., Ahmad, S., Lee, Y. L., Kobayashi, T., Ryan, D., Zhong, J., Zhu, J., Wu, J., Lan, G., Petkov, S., Yang, J., Antunes, L., … Liu, P. (2019). Establishment of porcine and human expanded potential stem cells. Nature Cell Biology , 21, 687–699. https://doi.org/10.1038/s41556-019-0333-2
- Gao, Y., Guo, Y., Duan, A., Cheng, D., Zhang, S., & Wang, H. (2014). Optimization of culture conditions for maintaining porcine induced pluripotent stem cells. DNA and Cell Biology , 33, 1–11. https://doi.org/10.1089/dna.2013.2095
- Henze, L. J., Koehl, N. J., O'Shea, J. P., Kostewicz, E. S., Holm, R., & Griffin, B. T. (2019). The pig as a preclinical model for predicting oral bioavailability and in vivo performance of pharmaceutical oral dosage forms: A PEARRL review. Journal of Pharmacy and Pharmacology , 71(4), 581–602. https://doi.org/10.1111/jphp.12912
- Howden, S. E., Wardan, H., Voullaire, L., Mclenachan, S., Williamson, R., Ioannou, P., & Vadolas, J. (2006). Chromatin-binding regions of EBNA1 protein facilitate the enhanced transfection of Epstein–Barr virus-based vectors. Human Gene Therapy , 17, 833–844. https://doi.org/10.1089/hum.2006.17.833
- Howden, S. E., Maufort, J. P., Duffin, B. M., Elefanty, A. G., Stanley, E. G., & Thomson, J. A. (2015). Simultaneous reprogramming and gene correction of patient fibroblasts. Stem Cell Reports , 5, 1109–1118. https://doi.org/10.1016/j.stemcr.2015.10.009
- Jiao, H., Lee, M. S., Sivapatham, A., Leiferman, E. M., & Li, W. J. (2022). Epigenetic regulation of BAF60A determines efficiency of miniature swine iPSC generation. Scientific Reports , 12, 9039. https://doi.org/10.1038/s41598-022-12919-6
- Juhasova, J., Juhas, S., Hruska-Plochan, M., Dolezalova, D., Holubova, M., Strnadel, J., Marsala, S., Motlik, J., & Marsala, M. (2015). Time course of spinal doublecortin expression in developing rat and porcine spinal cord: Implication in in vivo neural precursor grafting studies. Cellular and Molecular Neurobiology , 35(1), 57–70. https://doi.org/10.1007/s10571-014-0145-7
- Kang, X., Yu, Q., Huang, Y., Song, B., Chen, Y., Gao, X., He, W., Sun, X., & Fan, Y. (2015). Effects of integrating and non-integrating reprogramming methods on copy number variation and genomic stability of human induced pluripotent stem cells. PLOS ONE , 10, e0131128. https://doi.org/10.1371/journal.pone.0131128
- Kinoshita, M., Kobayashi, T., Planells, B., Klisch, D., Spindlow, D., Masaki, H., Bornelöv, S., Stirparo, G. G., Matsunari, H., Uchikura, A., Lamas-Toranzo, I., Nichols, J., Nakauchi, H., Nagashima, H., Alberio, R., & Smith, A. (2021). Pluripotent stem cells related to embryonic disc exhibit common self-renewal requirements in diverse livestock species. Development , 148, dev199901. https://doi.org/10.1242/dev.199901
- Kues, W. A., Herrmann, D., Barg-Kues, B., Haridoss, S., Nowak-Imialek, M., Buchholz, T., Streeck, M., Grebe, A., Grabundzija, I., Merkert, S., Martin, U., Hall, V. J., Rasmussen, M. A., Ivics, Z., Hyttel, P., & Niemann, H. (2013). Derivation and characterization of sleeping beauty transposon-mediated porcine induced pluripotent stem cells. Stem Cells and Development , 22, 124–135. https://doi.org/10.1089/scd.2012.0382
- Li, D., Secher, J., Hyttel, P., Ivask, M., Kolko, M., Hall, V. J., & Freude, K. K. (2018). Generation of transgene-free porcine intermediate type induced pluripotent stem cells. Cell Cycle , 17, 2547–2563. https://doi.org/10.1080/15384101.2018.1548790
- Lin, S. L., Chang, D. C., Chang-Lin, S., Lin, C. H., Wu, D. T. S., Chen, D. T., & Ying, S. Y. (2008). Mir-302 reprograms human skin cancer cells into a pluripotent ES-cell-like state. RNA , 14, 2115–2124. https://doi.org/10.1261/rna.1162708
- Lunney, J. K., van Goor, A., Walker, K. E., Hailstock, T., Franklin, J., & Dai, C. (2021). Importance of the pig as a human biomedical model. Science Translational Medicine , 13, eabd5758. https://doi.org/10.1126/scitranslmed.abd5758
- MacCarthy, C. M., Wu, G., Malik, V., Menuchin-Lasowski, Y., Velychko, T., Keshet, G., Fan, R., Bedzhov, I., Church, G. M., Jauch, R., Cojocaru, V., Schöler, H. R., & Velychko, S. (2024). Highly cooperative chimeric super-SOX induces naive pluripotency across species. Cell Stem Cell , 31, 127–147. e9. https://doi.org/10.1016/j.stem.2023.11.010
- Miyoshi, N., Ishii, H., Nagano, H., Haraguchi, N., Dewi, D. L., Kano, Y., Nishikawa, S., Tanemura, M., Mimori, K., Tanaka, F., Saito, T., Nishimura, J., Takemasa, I., Mizushima, T., Ikeda, M., Yamamoto, H., Sekimoto, M., Doki, Y., & Mori, M. (2011). Reprogramming of mouse and human cells to pluripotency using mature microRNAs. Cell Stem Cell , 8, 633–638. https://doi.org/10.1016/j.stem.2011.05.001
- Montserrat, N., Bahima, E. G., Batlle, L., Häfner, S., Rodrigues, A. M. C., González, F., & Belmonte, J. C. I. (2011). Generation of pig iPS cells: A model for cell therapy. Journal of Cardiovascular Translational Research , 4, 121–130. https://doi.org/10.1007/s12265-010-9233-3
- Okita, K., Matsumura, Y., Sato, Y., Okada, A., Morizane, A., Okamoto, S., Hong, H., Nakagawa, M., Tanabe, K., Tezuka, K. I., Shibata, T., Kunisada, T., Takahashi, M., Takahashi, J., Saji, H., & Yamanaka, S. (2011). A more efficient method to generate integration-free human iPS cells. Nature Methods , 8, 409–412. https://doi.org/10.1038/nmeth.1591
- Okita, K., Ichisaka, T., & Yamanaka, S. (2007). Generation of germline-competent induced pluripotent stem cells. Nature , 448, 313–317. https://doi.org/10.1038/nature05934
- Okita, K., Nakagawa, M., Hyenjong, H., Ichisaka, T., & Yamanaka, S. (2008). Generation of mouse induced pluripotent stem cells without viral vectors. Science , 322, 949–953. https://doi.org/10.1126/science.1164270
- Park, J. K., Kim, H. S., Uh, K. J., Choi, K. H., Kim, H. M., Lee, T., Yang, B. C., Kim, H. J., Ka, H. H., Kim, H., & Lee, C. K. (2013). Primed pluripotent cell lines derived from various embryonic origins and somatic cells in pig. PLOS ONE , 8, e52481. https://doi.org/10.1371/journal.pone.0052481
- Popp, B., Krumbiegel, M., Grosch, J., Sommer, A., Uebe, S., Kohl, Z., Plötz, S., Farrell, M., Trautmann, U., Kraus, C., Ekici, A. B., Asadollahi, R., Regensburger, M., Günther, K., Rauch, A., Edenhofer, F., Winkler, J., Winner, B., & Reis, A. (2018). Need for high-resolution genetic analysis in iPSC: Results and lessons from the ForIPS consortium. Scientific Reports , 8, 17201. https://doi.org/10.1038/s41598-018-35506-0
- Strnadel, J., Carromeu, C., Bardy, C., Navarro, M., Platoshyn, O., Glud, A. N., Marsala, S., Kafka, J., Miyanohara, A., Kato Jr, T., Tadokoro, T., Hefferan, M. P., Kamizato, K., Yoshizumi, T., Juhas, S., Juhasova, J., Ho, C.-S., Kheradmand, T., Chen, P. X., … Marsala, M. (2018). Survival of syngeneic and allogeneic iPSC-derived neural precursors after spinal grafting in minipigs. Science Translational Medicine , 10(440), eaam6651. https://doi.org/10.1126/scitranslmed.aam6651
- Takahashi, K., Tanabe, K., Ohnuki, M., Narita, M., Ichisaka, T., Tomoda, K., & Yamanaka, S. (2007). Induction of pluripotent stem cells from adult human fibroblasts by defined factors. Cell , 131, 861–872. https://doi.org/10.1016/j.cell.2007.11.019
- Takahashi, K., & Yamanaka, S. (2006). Induction of pluripotent stem cells from mouse embryonic and adult fibroblast cultures by defined factors. Cell , 126, 663–676. https://doi.org/10.1016/j.cell.2006.07.024
- Telugu, B. P. V. L., Ezashi, T., & Roberts, R. M. (2010). Porcine induced pluripotent stem cells analogous to naive and primed embryonic stem cells of the mouse. International Journal of Developmental Biology , 54, 1703–1711. https://doi.org/10.1387/ijdb.103200bt
- Voytas, D. (2000). Agarose gel electrophoresis. Current Protocols in Molecular Biology , 51(1), 2.5A.1–2.5A.9. https://doi.org/10.1002/0471142727.mb0205as51
- Wu, Z., Chen, J., Ren, J., Bao, L., Liao, J., Cui, C., Rao, L., Li, H., Gu, Y., Dai, H., Zhu, H., Teng, X., Cheng, L., & Xiao, L. (2009). Generation of pig induced pluripotent stem cells with a drug-inducible system. Journal of Molecular Cell Biology , 1, 46–54. https://doi.org/10.1093/jmcb/mjp003
- Xu, J., Zheng, Z., Du, X., Shi, B., Wang, J., Gao, D., Zhu, Q., Chen, X., & Han, J. (2020). A cytokine screen using CRISPR-Cas9 knock-in reporter pig iPS cells reveals that Activin A regulates NANOG. Stem Cell Research & Therapy, 11, 1–16. https://doi.org/10.1186/s13287-020-1588-z
- Yoshimatsu, S., Nakajima, M., Iguchi, A., Sanosaka, T., Sato, T., Nakamura, M., Nakajima, R., Arai, E., Ishikawa, M., Imaizumi, K., Watanabe, H., Okahara, J., Noce, T., Takeda, Y., Sasaki, E., Behr, R., Edamura, K., Shiozawa, S., & Okano, H. (2021). Non-viral induction of transgene-free iPSCs from somatic fibroblasts of multiple mammalian species. Stem Cell Reports , 16, 754–770. https://doi.org/10.1016/j.stemcr.2021.03.002
- Yu, J., Vodyanik, M. A., Smuga-Otto, K., Antosiewicz-Bourget, J., Frane, J. L., Tian, S., Nie, J., Jonsdottir, G. A., Ruotti, V., Stewart, R., Slukvin, I. I., & Thomson, J. A. (2007). Induced pluripotent stem cell lines derived from human somatic cells. Science , 318, 1917–1920. https://doi.org/10.1126/science.1151526
- Yu, J., Hu, K., Smuga-Otto, K., Tian, S., Stewart, R., Slukvin, I. I., & Thomson, J. A. (2009). Human induced pluripotent stem cells free of vector and transgene sequences. Science , 324, 797–801. https://doi.org/10.1126/science.1172482
- Zhao, T., Zhang, Z. N., Rong, Z., & Xu, Y. (2011). Immunogenicity of induced pluripotent stem cells. Nature , 474, 212–215. https://doi.org/10.1038/nature10135
- Zhi, M., Zhang, J., Tang, Q., Yu, D., Gao, S., Gao, D., Liu, P., Guo, J., Hai, T., Gao, J., Cao, S., Zhao, Z., Li, C., Weng, X., He, M., Chen, T., Wang, Y., Long, K., Jiao, D., … Han, J. (2022). Generation and characterization of stable pig pregastrulation epiblast stem cell lines. Cell Research , 32, 383–400. https://doi.org/10.1038/s41422-021-00592-9
- Zhu, Q., Wang, F., Gao, D., Gao, J., Li, G., Jiao, D., Zhu, G., Xu, K., Guo, J., Chen, T., Cao, S., Zhi, M., Zhang, J., Wang, Y., Zhang, X., Zhang, D., Yao, Y., Song, J., Wei, H. - J., & Han, J. (2023). Generation of stable integration-free pig induced pluripotent stem cells under chemically defined culture condition. Cell Proliferation , 56, e13487. https://doi.org/10.1111/cpr.13487