Budget-Friendly Generation, Biochemical Analyses, and Lentiviral Transduction of Patient-Derived Colon Organoids
Emilie Rigaux, Emilie Rigaux, Jia-Wei Chen, Jia-Wei Chen, Fabienne George, Fabienne George, Julien Lemaire, Julien Lemaire, Claude Bertrand, Claude Bertrand, Laurence Faugeras, Laurence Faugeras, Antoine Fattaccioli, Antoine Fattaccioli, Quentin Gilliaux, Quentin Gilliaux, Lionel D'Hondt, Lionel D'Hondt, Carine Michiels, Carine Michiels, Henri-François Renard, Natacha Zanin
cancer
high-resolution microscopy
immunoblotting
lentiviral transduction
patient-derived organoids
RT-qPCR
Abstract
For the past decade, three-dimensional (3D) culture models have been emerging as powerful tools in translational research to overcome the limitations of two-dimensional cell culture models. Thanks to their ability to recapitulate the phenotypic and molecular heterogeneity found in numerous organs, organoids have been used to model a broad range of tumors, such as colorectal cancer. Several approaches to generate organoids exist, with protocols using either pluripotent stem cells, embryonic stem cells, or organ-restricted adult stem cells found in primary tissues, such as surgical resections as starting material. The latter, so-called patient-derived organoids (PDOs), have shown their robustness in predicting patient drug responses compared to other models. Because of their origin, PDOs are natural offspring of the patient tumor or healthy surrounding tissue, and therefore, have been increasingly used to develop targeted drugs and personalized therapies. Here, we present a new protocol to generate patient-derived colon organoids (PDCOs) from tumor and healthy tissue biopsies. We emphasize budget-friendly and reproducible techniques, which are often limiting factors in this line of research that restrict the development of this 3D-culture model to a small number of laboratories worldwide. Accordingly, we describe efficient and cost-effective techniques to achieve immunoblot and high-resolution microscopy on PDCOs. Finally, a novel strategy of lentiviral transduction of PDCOs, which could be applied to all organoid models, is detailed in this article. © 2023 The Authors. Current Protocols published by Wiley Periodicals LLC.
Basic Protocol 1 : Establishment of PDCOs from biopsies
Basic Protocol 2 : Long-term maintenance and expansion of PDCOs in BME domes
Basic Protocol 3 : Cryopreservation and thawing of PDCOs
Basic Protocol 4 : Lentiviral transduction of PDCOs
Basic Protocol 5 : Immunoblot and evaluation of variability between donors
Basic Protocol 6 : Immunofluorescence labeling and high-resolution microscopy of PDCOs
Basic Protocol 7 : Transcriptomic analyses of PDCOs by RT-qPCR
INTRODUCTION
This article describes protocols aiming at developing, routinely in the laboratory, the use of patient-derived colon organoids (PDCOs) under the scope of both a tight budget and reproducibility, which are very well-known limitations in the field. Indeed, the major goal of this article is to detail step-by-step robust protocols to isolate intestinal crypts from patient biopsies using both an enzyme-free reagent and mechanical disruptive approaches. Of note, these crypts are architectural subunits of the intestinal epithelium including adult stem cells enabling the self-renewal of the colon tissue previously fragmented, and consequently, the formation of organoids. Then the intestinal crypts are embedded in domes made of Cultrex, a basement membrane extract, and fed with IntestiCult, a commercially available conditioned medium. This ensures a potent establishment of the dome-organoid cultures, and their subsequent propagation passages after passages. On the contrary to most protocols in literature, it is crucial to notice that the PDCO cultures we developed originate not only from the patient tumor tissue but also from the patient healthy tissue. Growing both types of organoids is of high interest in the cancer research field to identify novel biomarkers. To reach this objective, this article also describes immunoblot, immunofluorescence, and RT-qPCR protocols. These three different downstream assays are relevant to study the levels of expression of proteins or mRNA, as well as the spatial localization of proteins within the organoids assessed by confocal microscopy. Finally, this work investigates an unresolved question in the organoid field, which is the efficiency of lentiviral transduction of organoids, to generate silenced tumor patient-derived organoids and identify target genes leading to translation in therapeutics.
This article presents comprehensive and detailed protocols for the production, culture, and analysis of patient-derived colon organoids (PDCOs). Firstly, we provide a protocol for the production of PDCOs (Basic Protocol 1) and their long-term maintenance and expansion in culture (Basic Protocol 2). We also describe all the steps for cryopreserving and thawing patient-derived organoids (Basic Protocol 3), facilitating the establishment of a biobank. Furthermore, we offer multiple protocols for the analysis of these PDCOs. Firstly, we propose a lentiviral transduction protocol for gene knockdown (Basic Protocol 4). Secondly, we describe two different protocols for the analysis of protein expression in PDCOs, including an immunoblot protocol (Basic Protocol 5) and an immunofluorescence protocol (Basic Protocol 6). Finally, we conclude with a protocol for the transcriptomic analysis of PDCOs using RT-qPCR (Basic Protocol 7). Importantly, we propose budget-friendly notes in each protocol. We provide a cost comparison of budget-friendly notes vs conventional procedures in Table 1.
Dome number | Budget-friendly notes | Conventional procedures | ||||
---|---|---|---|---|---|---|
Tumor | Healthy | Solution/reagent | Price (€) | Solution/reagent | Price (€) | |
Basic Protocol 1 | 6 | 6 | Cultrex | 40.00 | Geltrex | 74.00 |
DMEM/F-12 | 0.22 | Intesticult | 5.00 | |||
Murine (M-) Wtn3a | 2.60 | Human (H-) Wtn3a | 4.68 | |||
(Refresh) IntestiCult | 300.00 | (Refresh) IntestiCult | 360.00 | |||
(Refresh) M-Wtn3a | 20.40 | (Refresh) H-Wtn3a | 56.16 | |||
Passage 1:2 Cultrex | 79.90 | Passage 1:2 Geltrex | 148.00 | |||
DMEM/F-12 | 0.22 | Intesticult | 5.00 | |||
Basic Protocol 2 | 12 | 12 | M-Wtn3a | 5.10 | H-Wtn3a | 9.36 |
(Refresh) IntestiCult | 600.00 | (Refresh) IntestiCult | 720.00 | |||
(Refresh) M-Wtn3a | 10.20 | (Refresh) H-Wtn3a | 18.72 | |||
Total cost (TC) (€) | 1058.60 | 1400.90 | ||||
BP4 | Plasmids to produce lentivirus particles | 240.00 | Commercial lentivirus | 1700.00 | ||
CaCl2 | <0.50 | Transduction reagent | 180.00 | |||
TC (€) |
240.50 | 1880.00 | ||||
BP5 | Homemade gel | <1.00 | (Bio-Rad) precast gel | 15.00 | ||
Wet-transfer buffer | <1.00 | Trans-Blot Turbo kit | 264.00 | |||
TC (€) |
<1.00 | 279.00 |
- a In the first (top) part of this table, we compare the cost difference for generating 6 healthy and 6 tumor organoid-domes over 2 months, with or without the budget-friendly notes included in both Basic Protocol 1 (culturing) and Basic Protocol 2 (passaging and continued culture). Without the application of budget-friendly notes, these steps of both protocols would be 1.3 times more expensive.
- b In the second (bottom) part of this table, we compare the cost difference between budget-friendly notes and conventional procedures for the lentiviral transduction of PDCOs (Basic Protocol 4) and immunobloting on PDCOs (Basic protocol 5). Without the application of budget-friendly notes, Basic Protocol 4 and 5 would be ∼7.8 and 279 times more expensive, respectively.
STRATEGIC PLANNING
Before performing any of the Basic Protocols detailed hereafter, ensure to understand every single step, then make sure to have all the reagents ready and prepared in agreement with the safety data sheet (SDS). Aseptic culture conditions under an adequate biosafety cabinet (BSC) must be applied according to the protocol followed (Basic Protocols 1 to 3), as well as wearing personal protective equipment (PPE). Good laboratory practices must be followed at any time. In addition, work with lentiviruses must be performed in a BSL2+ environment with adapted PPE (Basic Protocol 4). Finally, confocal fluorescent microscopy involves laser handling, so work according to health and safety rules and avoid exposing your eyes directly to the laser beam.
NOTE : We obtained colorectal biopsies of patients from the CHU UCL Namur Mont-Godinne hospital (Belgium), following ethical approval and upon informed consent of each patient. Informed consent must be obtained from all subjects before the collection of the primary human tissue material.
Basic Protocol 1: ESTABLISHMENT OF PDCOS FROM BIOPSIES
This protocol aims to establish colon organoid cultures from patient biopsies. The tumor and healthy tissues are processed in parallel, with a separate set of sterile material, and in a BSC to work under aseptic culture conditions. Once extracted from patients, the biopsies are put in ice-cold DMEM/F-12 supplemented with antibiotic-antimycotic and kept at 4°C for transport. Then, in a BSC, the biopsies are extensively washed and minced into small pieces in a solution of gentle cell dissociation reagent (GCDR). Biopsies are incubated at 37°C on a shaker to induce tissue dissociation resulting in a turbid solution containing small chunks and isolated intestinal crypts. This solution is filtered to mechanically fragment the chunks and obtain a higher yield of isolated intestinal crypts, which will enable the growth and the maturation of colon organoids. The intestinal crypt solution is centrifuged, and the pellet is resuspended in Cultrex, which is basement membrane extract (BME). Then, domes containing Cultrex-intestinal crypts are seeded in a 24-well plate and dried before the incubation in IntestiCult to establish and propagate colon organoids derived from patients. An overview of this workflow is presented in Figure 1.
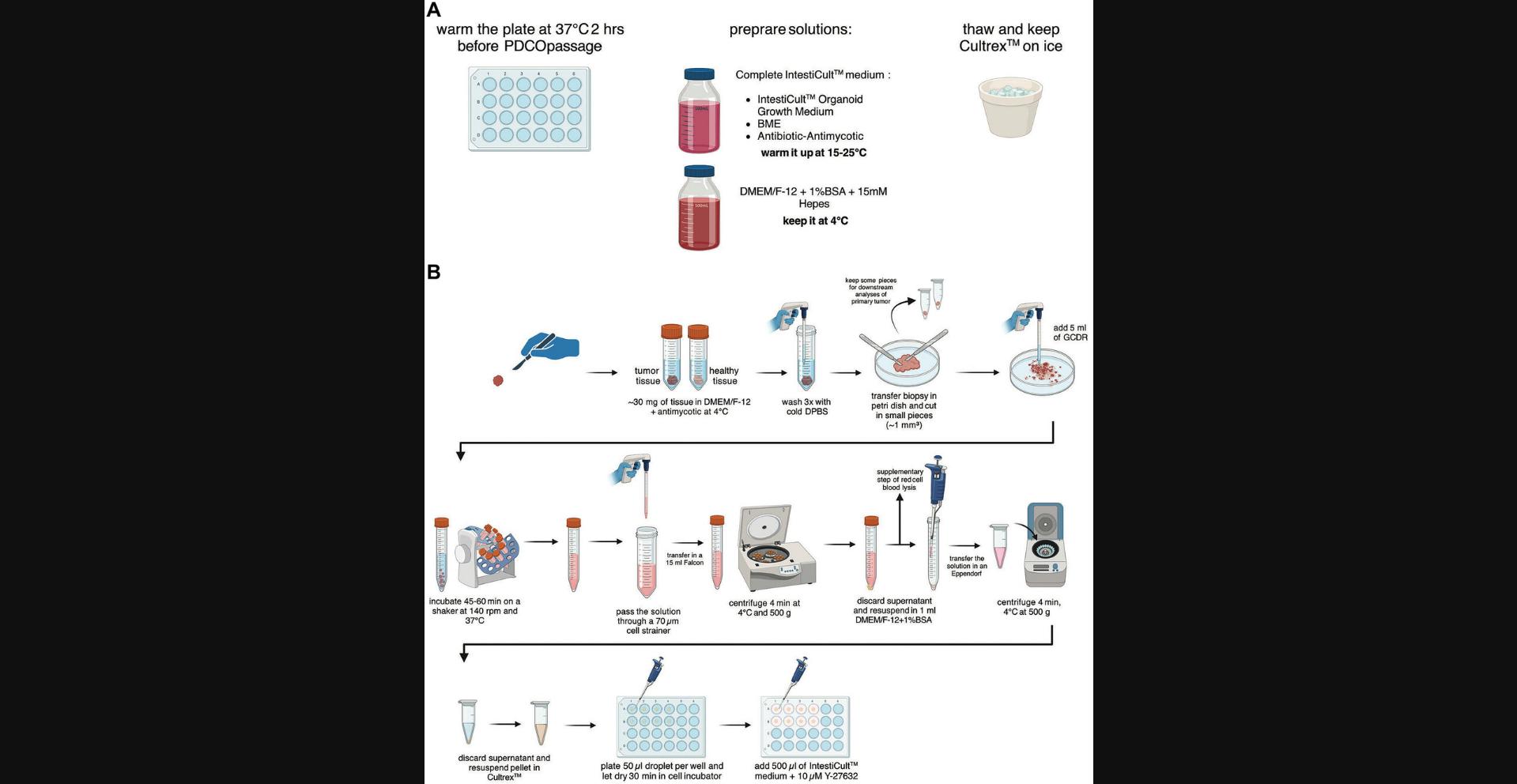
Materials
-
IntestiCult organoid growth medium (human) (StemCell Technologies, cat. no. 06010)
-
Antibiotic-antimycotic (100×) (ThermoFisher Scientific, cat. no. 15240062)
-
DMEM/F-12 (ThermoFisher Scientific, cat. no. 11320033)
-
HEPES, 1 M (ThermoFisher Scientific, cat. no. 15630056)
-
Bovine serum albumin (BSA) fraction, protease-free, TSE-/BSE-free (Carl Roth, cat. no. T844.3)
-
Cultrex UltiMatrix reduced growth factor basement membrane extract (RGF BME) (Bio-techne R&D Systems, cat. no. BME001-05)
-
Geltrex LDEV-free reduced growth factor basement membrane matrix (ThermoFisher Scientific, cat. no. A1413201)
-
DPBS, no calcium, no magnesium (ThermoFisher Scientific, cat. no. 14190144)
-
Liquid nitrogen, or dry ice and methyl-2 butane (isopentane), ≥99%, GPR RECTAPUR (VWR, cat. no. 24872)
-
4% PFA (see recipe)
-
Sucrose (ThermoFisher Scientific, cat. no. S0389)
-
OCT embedding matrix (Carl Roth, cat. no. 6478.2)
-
Gentle cell dissociation reagent (GCDR) (StemCell Technologies, cat. no. 100-0485)
-
1× RBC lysis buffer, eBioscience (ThermoFisher Scientific, cat. no. 00-4333-57)
-
Y-27632, ROCK inhibitor (see recipe)
-
Recombinant human Wnt-3a protein (Bio-techne R&D Systems, cat. no. 5036-WN)
-
Wnt-3a, murine (see recipe)
-
24-well clear multiple well plates, flat bottom with lid, sterile, Corning CellBIND (Corning, cat. no. 3337)
-
Incubator for cell culture, CellXpert C170 (Eppendorf, cat. no. 6734000011)
-
Sterile disposable scalpels, shape 10 (Laborimpex, cat. no. AGAR_T5217-10)
-
10-cm Petri dish, glass (Merck, cat. no. BR455742-10EA)
-
Cryogenic vials (VWR, cat. no. 479-1220)
-
15-ml centrifuge tube (Corning, cat. no. 430791)
-
Tube revolver rotator (ThermoFisher Scientific, cat. no. 88881001)
-
10-ml pipette, Stripette (Corning, cat. no. 4488)
-
70-µm reversible strainers, large (StemCell Technologies, cat. no. 27260)
-
50-ml centrifuge tube (Corning, cat. no. 430829)
-
Stripettor ultra pipet controller (Corning)
-
Assorted pipettor and tips
-
Centrifuges, 5702 and 5425 (Eppendorf, cat. nos. 5702000010 and 5405000310)
-
1.5-ml Eppendorf microtubes (Merck, cat. no. Z606340)
Preparation
1.Warm an empty tissue-culture treated 24-well plate at 37°C, at least 2 hr before the beginning of the experiment.
2.Prepare complete IntestiCult organoid growth medium (human) following the supplier guidelines, and warm it up to room temperature (15° to 25°C).
3.Prepare 12 ml of sterile DMEM/F-12 with 15 mM HEPES and 1% (w/v) BSA (or a larger volume for storage at 4°C for up to 3 months) and keep it on ice.
4.Thaw Cultrex UltiMatrix RGF BME on ice and keep it on ice during all the following steps of the protocol.
Biopsy mechanical processing
5.Weigh the biopsies that are in 10 ml DMEM/F-12 supplemented with antimycotic. Keep the biopsies on ice. Process the two biopsies (tumor tissue and healthy tissue) side-by-side and at the same time. Make sure to either rinse or change the scalpel set between each tissue to prevent cross-contamination.
6.Wash the biopsy 3 times with 5 ml of cold DPBS. Between each washing step, aspirate the supernatant carefully and discard it.
7.Add 3 ml of fresh DPBS and transfer the biopsy into a 10-cm Petri dish.
8.Using 2 sterile scalpels to create a neat scission, split the biopsy into 2 pieces accounting for 1/3 and 2/3 in size of the original biopsy, respectively. The smallest part can be cut into 2 pieces, placed in cryogenic vials, snap-frozen in liquid nitrogen, and kept at −80°C for future downstream experiments (protein or RNA extraction for example). The larger piece is processed as follows.
9.Use the scalpels to mince tissues into the smallest pieces you can generate. Add 5 ml of GCDR to start the dissociation of the tissue pieces and transfer the solution into a 15-ml centrifuge tube.
10.Rinse the 10-cm dish with 5 ml of fresh GCDR and transfer the solution with the remaining tissue chunks to the 15-ml tube.
Biopsy disruption to isolate intestinal crypts
11.Incubate the GCDR solution with the tissue pieces for 45 to 60 min at 37°C, on a tube rotator at 140 rpm.
12.Transfer the GCDR solution, containing chunks and isolated intestinal crypts, with a 10-ml pipette onto a 70-μm cell strainer placed on a 50-ml centrifuge tube. In the event of a hindered filtration, pipette-mediated scratching of the top of the strainer should help to resume the process.
13.To maximize material collection, rinse the strainer with 3 ml of DMEM/F-12 with 15 mM HEPES and 1% BSA. The collected medium is then transferred to the 50-ml tube containing the rest of the filtered solution (total of ∼13 ml).
14.Transfer the filtrated solution of intestinal crypts into a 15-ml tube.
15.Centrifuge once for 4 min at 500 × g , 4°C. Carefully remove the supernatant. If a red coloration of the pellet is observed due to the presence of blood cells, proceed to step 16, otherwise go to step 17.
16.Resuspend the pellet in 3 ml of red blood cell lysis buffer and incubate for 5 min at room temperature. Then, add 5 ml of DMEM/F-12 with 15 mM HEPES and 1% BSA medium to filtrated solution. Centrifuge 4 min at 500 × g , 4°C, and aspirate the supernatant.
17.Wash the pellet 2 times with 3 ml of DMEM/F-12 with 15 mM HEPES and 1% BSA. Each time, spin 4 min at 500 × g , 4°C, and carefully remove the supernatant.
Dome-organoid seeding in 24-well plate
18.Resuspend the pellet in 1 ml DMEM/F-12 with 15 mM HEPES and 1% BSA and transfer the solution containing intestinal crypts into a 1.5-ml Eppendorf tube. Spin for 4 min at 500 × g , room temperature.
19.Resuspend the final pellet (+ thin layer of DMEM/F-12 with 15 mM HEPES and 1% BSA) in undiluted Cultrex. Avoiding bubble formation. A dome of 50 μl of Cultrex-intestinal crypt solution is plated per well of a warmed 24-well plate.
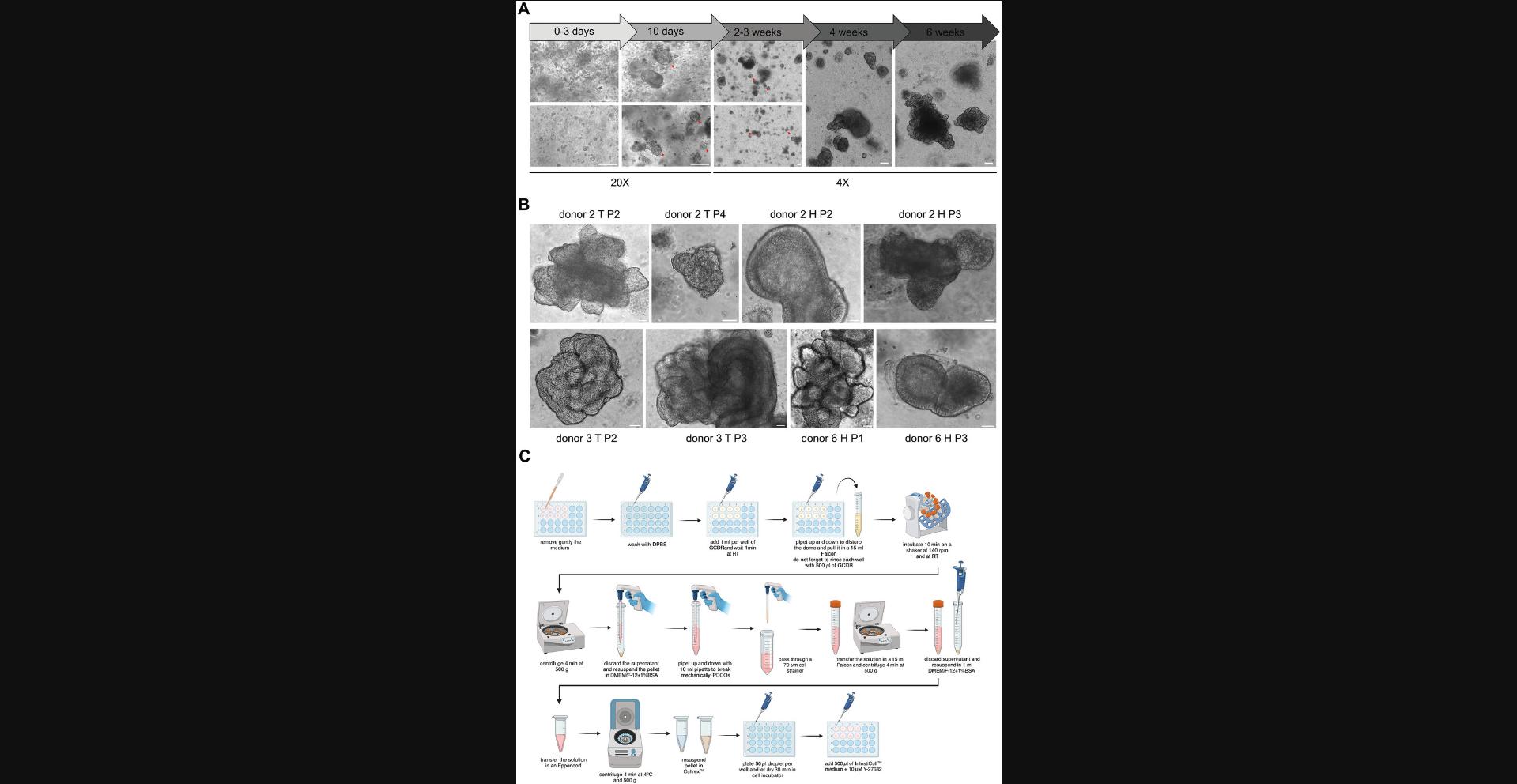
20.Incubate the plate for 30 min at 37°C under 5% CO2 to allow dome solidification.
21.Once dome solidification is achieved, remove the 24-well plate from the incubator and add 500 μl of IntestiCult medium supplemented with 10 μM of the ROCK inhibitor Y-27632 to each single well, as well as 50 ng/ml of Wnt3a for healthy tissue exclusively.
22.Incubate the plate at 37°C under 5% CO2 for 2 weeks until the first passage. Refresh the medium every two days with 500 μl of IntestiCult only for tumor organoids and of IntestiCult supplemented with Wtn3a for healthy organoids.
Basic Protocol 2: LONG-TERM MAINTENANCE AND EXPANSION OF PDCOS IN BME DOMES
This protocol aims to maintain and expand PDCO cultures in BME domes, here Cultrex-domes. The goal is to perform different downstream assays, such as lentiviral transduction, immunoblotting, immunofluorescence, or RT-qPCR. Cultrex-organoid dome cultures are passaged every 1 to 2 weeks depending on the confluency and the morphology of the organoids. Indeed, large doughnut-like organoids and budding ones have been observed to produce robust and fertile fragments leading to a sustained expansion of PDCOs (Fig. 2A and B). On the contrary, small organoid (<50 µm) structures or mature ones (>250 µm) are hardly expandable due to their lack of intestinal crypts containing Lgr5+ stem cells. Briefly, domes to be passaged are mechanically disrupted by flushing them with GCDR and then filtered to obtain a solution rich in small fragments containing intestinal crypts that will self-assemble to generate novel organoids. IntestiCult is used for the maintenance and expansion of PDCOs. An overview of this workflow is presented in Figure 2C.
Materials
-
IntestiCult organoid growth medium (human) (StemCell Technologies, cat. no. 06010)
-
Antibiotic-antimycotic (100×) (ThermoFisher Scientific, cat. no. 15240062)
-
DMEM/F-12 (ThermoFisher Scientific, cat. no. 11320033)
-
HEPES, 1 M (ThermoFisher Scientific, cat. no. 15630056)
-
Bovine serum albumin (BSA) fraction, protease-free, TSE-/BSE-free (Carl Roth, cat. no. T844.3)
-
Cultrex UltiMatrix reduced growth factor basement membrane extract (RGF BME) (Bio-techne R&D Systems, cat. no. BME001-05)
-
Geltrex LDEV-free reduced growth factor basement membrane matrix (ThermoFisher Scientific, cat. no. A1413201)
-
DPBS, no calcium, no magnesium (ThermoFisher Scientific, cat. no. 14190144)
-
Gentle cell dissociation reagent (GCDR) (StemCell Technologies, cat. no. 100-0485)
-
Y-27632, ROCK inhibitor (see recipe)
-
Recombinant human Wnt-3a protein (Bio-techne R&D Systems, cat. no. 5036-WN)
-
Wnt-3a, murine (see recipe)
-
24-well clear multiple well plates, flat bottom with lid, sterile, Corning CellBIND (Corning, cat. no. 3337)
-
Incubator for cell culture, CellXpert C170 (Eppendorf, cat. no. 6734000011)
-
Assorted pipettor and tips
-
15-ml centrifuge tube (Corning, cat. no. 430791)
-
Tube revolver rotator (ThermoFisher Scientific, cat. no. 88881001)
-
50-ml centrifuge tube (Corning, cat. no. 430829)
-
Centrifuges, 5702 and 5425 (Eppendorf, cat. nos. 5702000010 and 5405000310)
-
10-ml pipette, Stripette (Corning, cat. no. 4488)
-
70-µm reversible strainers, large (StemCell Technologies, cat. no. 27260)
-
Stripettor ultra pipet controller (Corning)
-
1.5-ml Eppendorf microtubes (Merck, cat. no. Z606340)
Preparation
1.Warm an empty tissue-culture treated 24-well plate at 37°C, at least 2 hr before the beginning of the experiment.
2.Prepare complete IntestiCult organoid growth medium (human) following the supplier guidelines, and warm it up to room temperature (15° to 25°C).
3.Prepare sterile DMEM/F-12 with 15 mM HEPES and 1% (w/v) BSA and keep it on ice. The final volume depends on the number of domes that need to be passaged.
4.Thaw Cultrex on ice and keep it on ice during all the following steps of the protocol.
Mechanical disruption of domes
5.Identify the domes to be passaged and remove gently the medium in each well, without disturbing the integrity of the Cultrex-dome.
6.Gently wash the exposed domes with DPBS at room temperature and then discard the DPBS.
7.Add 1 ml of GCDR on top of each dome and incubate for 1 min at room temperature.
8.Mechanically break down each dome using a P1000 pipette tip, and pipet GCDR up and down above the dome to create a disruptive flow.
9.Transfer the resulting solution of dome clumps in a 15-ml centrifuge tube.
10.Add 500 μl per dome of GCDR to the newly emptied well to rinse it. Then, transfer the 500 μl solution in the 15-ml tube from step 8.Incubate it for 10 min on a tube rotator at ∼160 rpm, at room temperature.
11.Centrifuge the tube 4 min at 500 × g , room temperature. Discard the supernatant.
12.Add 1 ml per passaged dome of ice-cold DMEM/F-12 with 15 mM HEPES and 1% BSA in the 15-ml centrifuge tube. Proceed to a mechanical disruption of the dome clumps to get organoid chunks, by performing vigorous up and down 15 times using a 10-ml pipette.
13.Using the same 10-ml pipet, transfer the solution onto a 70-μm cell strainer placed on a 50-ml centrifuge tube. In the event of a hindered filtration, pipette-mediated scratching of the top of the strainer should help to resume the process.
14.To collect a maximum of material, rinse the strainer with 3 ml of DMEM/F-12 with 15 mM HEPES and 1% BSA. The collected medium is then transferred to the 50-ml centrifuge tube containing the rest of the filtered solution.
15.Transfer the filtrated solution into a 15-ml centrifuge tube.
16.Centrifuge once for 4 min at 500 × g , 4°C. Aspirate the supernatant.
Dome-organoid cultures seeding in 24-well plate
17.Resuspend the pellet in 1 ml DMEM/F-12 with 15 mM HEPES and 1% BSA and transfer the solution into a 1.5-ml Eppendorf tube. Spin 4 min at 500 × g , room temperature.
18.Partially remove the supernatant. Leave a thin layer of medium (∼30 µl) in which the pellet will be resuspended carefully. Then, add Cultrex and resuspend the residual 30 µl carefully, avoiding bubbles. The final volume of Cultrex depends on the number of passaged domes and the final dilution.
19.Plate 50 μl of Cultrex-intestinal crypt solution in drop shape into each well in a pre-warmed 24-well plate.
20.Incubate the plate for 30 min at 37°C under 5% CO2 to allow dome solidification.
21.Once dome solidification is achieved, remove the 24-well plate from the incubator and add 500 μl of IntestiCult medium supplemented with 10 μM of the ROCK inhibitor Y-27632 to each single well, as well as 50 ng/ml of Wnt3a for healthy tissue exclusively.
22.Incubate the plate at 37°C under 5% CO2 for 1 to 2 weeks until the next passage. Refresh the medium every two days with 500 µl of IntestiCult only for tumor organoids and of IntestiCult supplemented with Wtn3a for healthy organoids.
Basic Protocol 3: CRYOPRESERVATION AND THAWING OF PDCOS
Cryopreservation of PDCOs is essential for biobanking, which is crucial in any pre-clinical study. The first step of this protocol is to select domes featuring most of the organoids in a doughnut-like shape (shown in Fig. 2A). Then, these PDCOs are passaged. Of note, it is essential to cryopreserve the organoids 4 days after the passaging step to ensure that PDCOs are in an exponential growth phase, resulting in an optimal viability yield after thawing. Then, newly passaged Cultrex-dome organoid cultures are mechanically disrupted by flushing and incubating them with GCDR. The solution containing organoid fragments from 2 domes pooled together is then washed at 4°C, centrifuged, and the corresponding pellet is resuspended in 1 ml of ice-cold CryoStor CS10 and transferred into a cryovial. The cryovials are stored overnight at −80°C and the next morning transferred into a liquid nitrogen tank. Finally, a fast-thawing protocol at 37°C involving a water-bath is described.
Materials
-
DMEM/F-12 (ThermoFisher Scientific, cat. no. 11320033)
-
HEPES, 1 M (ThermoFisher Scientific, cat. no. 15630056)
-
Bovine serum albumin (BSA) fraction, protease-free, TSE-/BSE-free (Carl Roth, cat. no. T844.3)
-
CryoStor CS10 (StemCell Technologies, cat. no. 100-1061)
-
DPBS, no calcium, no magnesium (ThermoFisher Scientific, cat. no. 14190144)
-
Gentle cell dissociation reagent (GCDR) (StemCell Technologies, cat. no. 100-0485)
-
IntestiCult organoid growth medium (human) (StemCell Technologies, cat. no. 06010) with antibiotic-antimycotic (1×) (ThermoFisher Scientific, cat. no. 15240062)
-
Ethanol, absolute, molecular biology grade (ThermoFisher Scientific, cat. no. 16685992)
-
Assorted pipettor and tips
-
15-ml centrifuge tube (Corning, cat. no. 430791)
-
Tube revolver rotator (ThermoFisher Scientific, cat. no. 88881001)
-
50-ml centrifuge tube (Corning, cat. no. 430829)
-
1.5-ml Eppendorf microtubes (Merck, cat. no. Z606340)
-
Cryogenic vials (VWR, cat. no. 479-1220)
-
Cell freezing container, Corning CoolCell LX (Merck, cat. no. CLS432002)
-
Incubator for cell culture, CellXpert C170 (Eppendorf, cat. no. 6734000011)
-
Centrifuges, 5702 and 5425 (Eppendorf, cat. nos. 5702000010 and 5405000310)
-
37°C water bath
-
Class II biological safety cabinet (BSC)
-
10-ml pipette, Stripette (Corning, cat. no. 4488)
Cryopreservation
1.Place DMEM/F-12 with 15 mM HEPES and 1% (w/v) BSA(1 ml needed) and CryoStor CS10 on ice.
2.Organoid domes selected for freezing are passaged 4 days prior to this step (see Basic Protocol 2) to maximize post-thaw recovery. This ensures that the organoids are in the logarithmic growth phase at the time of cryopreservation. The shape and size of the organoids at this stage are illustrated in Figure 2A.
3.Gently wash the domes once with DPBS at room temperature and then discard the DPBS.
4.Add 1 ml of GCDR on top of each dome and incubate for 1 min at room temperature.
5.Mechanically break down each dome using a P1000 pipette tip and pipet GCDR up and down above the dome to create a disruptive flow.
6.Transfer the resulting solution containing dome chunks in a 15-ml centrifuge tube. Pool all the domes that need to be passaged altogether (i.e., domes from a same donor and same tissue subtype normal versus tumor).
7.Add 500 μl per dome of GCDR to the newly emptied well to rinse it. Then, transfer the 500 μl solution in the 15-ml centrifuge tube of step 6.Incubate the 15-ml tube for 10 min on a tube rotator at ∼160 rpm, at room temperature.
8.Centrifuge the tube 4 min 500 × g , room temperature. Discard the supernatant.
9.Resuspend the pellet in 1 ml of ice-cold DMEM/F-12 with 15 mM HEPES and 1% BSA and transfer it in a 1.5-ml Eppendorf tube.
10.Centrifuge the tube 4 min at 500 × g , 4°C, and discard the supernatant.
11.Resuspend the pellet in 1 ml of ice-cold CryoStor CS10 per 2 domes and transfer each 1 ml of the solution into a well-labeled cryovial kept on ice.
12.Transfer the cryovials overnight in a freezing container at −80°C for controlled temperature decrease.
13.The next morning, transfer the cryovials to a liquid nitrogen container for long-term storage.
Thawing
14.Prepare a 15-ml centrifuge tube with 5 ml of pre-warmed IntestiCult.
15.Remove a cryovial from the liquid nitrogen tank.
16.Plunge the cryovial below the cap in a 37°C water bath to enable fast thawing.
17.Remove the cryovial from the water bath and spray it extensively with ethanol, before opening it in a BSC and transferring the cells into the centrifuge tube containing 5 ml pre-warmed IntestiCult.
18.Perform Basic Protocol 2 beginning at step 16.
Basic Protocol 4: LENTIVIRAL TRANSDUCTION OF PDCOS
This protocol aims to generate tumor or healthy PDCOs in which genes are silenced using lentiviral transduction to investigate their function. It has been established with a GFP lentiviral vector but can be applicable with any shRNA plasmid. Lentivirus manipulation requires to work under aseptic culture conditions with adapted BSC (BSL2+) and PPE. This protocol is divided into several major steps, as depicted in the workflow presented in Figure 3.The first step is the production of lentiviral particles containing the plasmid of interest by HEK293T cells. The concentration of lentiviral particles is quantified using the Lenti-X RT-qPCR Titration kit from Takara, which is a crucial step to determine the multiplicity of infection (MOI). Then, PDCOs are disrupted into a single-cell suspension solution by TrypLE, after which they are transduced with TransDux MAX reagent for 72 hr in InstestiCult (Fig. 4A). A notable transduction has been achieved at an MOI of 4000 (Fig. 4B for MOIs of 1000 and 4000; other MOIs are shown in supplementary Figure 1; lower magnification for MOIs 1000 to 6000 is shown in supplementary Figure 2; see Supporting Information). Finally, the single-cell suspension is pelleted and resuspended in Cultrex to enable the seeding of Cultrex-domes containing transduced intestinal crypts. After 8 days, the antibiotic selection starts as shown in Figure 4C. Given the length and complexity of Basic Protocol 4, detailed time considerations are provided directly within the respective protocol sections.
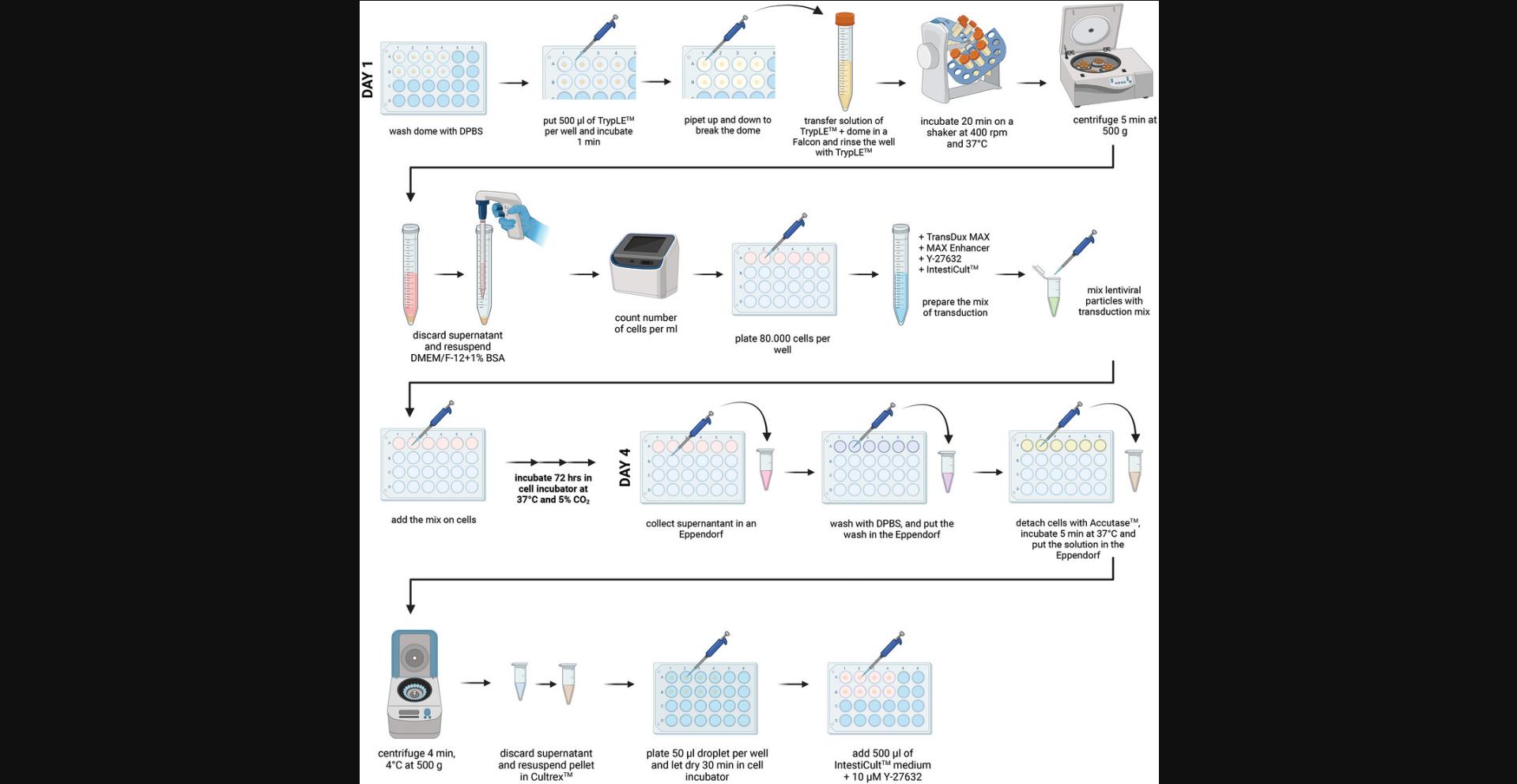
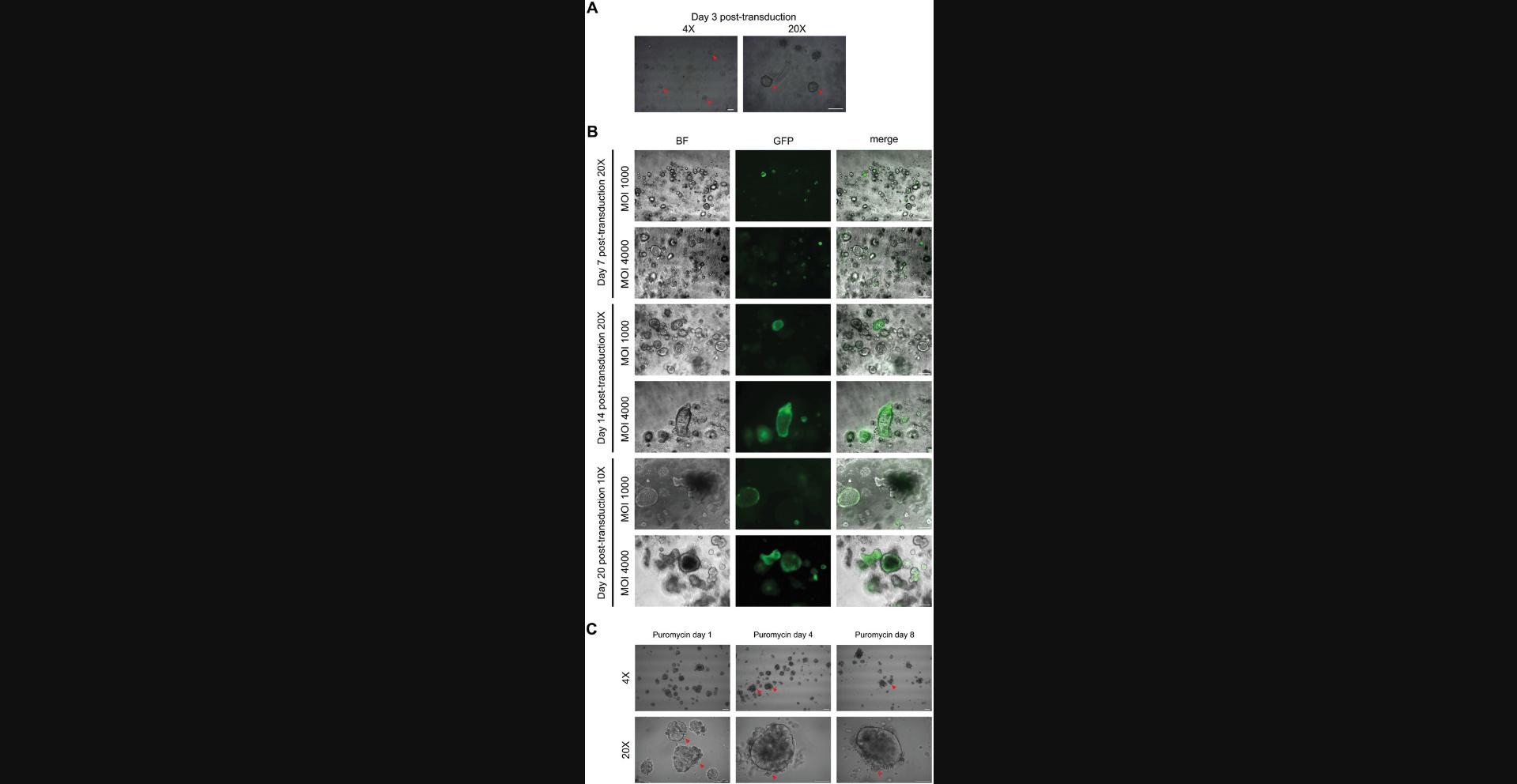
Materials
-
HEK293T cells (ATCC, cat. no. CRL-3216)
-
DMEM, low glucose, pyruvate (ThermoFisher Scientific, cat. no. 31885023)
-
Fetal bovine serum (FBS) (ThermoFisher Scientific, cat. no. 26140079)
-
Penicillin-streptomycin, Gibco (ThermoFisher Scientific, cat. no. 15140122)
-
H2O, milliQ
-
pLV-eGFP (AddGene, cat. no. 36083)
-
pCMV-DR8.91 (Delta 8.9) (AddGene, cat. no. 8455)
-
VSV-G plasmid (AddGene, cat. no. 8454)
-
CaCl2, 2 M solution (see recipe)
-
HEPES-buffered saline (HeBS), 2× (see recipe)
-
NucleoSpin RNA virus, mini kit for viral RNA from cell-free fluids (Macherey-Nagel, cat. no. 740956.50)
-
Lenti-X RT-qPCR titration kit (Takara Bio, cat. no. 631235)
-
DPBS (see recipe)
-
TrypLE Express enzyme, 1×, no phenol red (ThermoFisher Scientific, cat. no. 12604039)
-
DMEM/F-12 (ThermoFisher Scientific, cat. no. 11320033)
-
HEPES, 1 M (ThermoFisher Scientific, cat. no. 15630056)
-
Bovine serum albumin (BSA) fraction, protease-free, TSE-/BSE-free (Carl Roth, cat. no. T844.3)
-
TransDux MAX lentivirus transduction enhancer (SanBio, cat. no. LV860A-1)
-
Y-27632, ROCK inhibitor (see recipe)
-
IntestiCult organoid growth medium (human) (StemCell Technologies, cat. no. 06010)
-
Antibiotic-antimycotic (100×) (ThermoFisher Scientific, cat. no. 15240062)
-
Cultrex UltiMatrix reduced growth factor basement membrane extract (RGF BME) (Bio-techne R&D Systems, cat. no. BME001-05)
-
Geltrex LDEV-free reduced growth factor basement membrane matrix (ThermoFisher Scientific, cat. no. A1413201)
-
Accutase (StemCell Technologies, cat. no. 07920)
-
Recombinant human Wnt-3a protein (Bio-techne R&D Systems, cat. no. 5036-WN)
-
Wnt-3a, murine (see recipe)
-
Puromycin, sterile, ≥98%, HPLC, 10 mg/ml, liquid (InvivoGen, cat. no. ant-pr-1)
-
T150 flask (Corning, cat. no. 734-1719)
-
Assorted pipettor and tips
-
15-ml centrifuge tube (Corning, cat. no. 430791)
-
Vortex
-
Incubator for cell culture, CellXpert C170 (Eppendorf, cat. no. 6734000011)
-
0.2-µm filter (Sarstedt AG&Co, cat. no. 83.1826.001)
-
Cryogenic vials (VWR, cat. no. 479-1220)
-
Tube revolver rotator (ThermoFisher Scientific, cat. no. 88881001)
-
Centrifuges, 5702 and 5425 (Eppendorf, cat. nos. 5702000010 and 5405000310)
-
24-well clear multiple well plates, flat bottom with lid, sterile, Corning CellBIND (Corning, cat. no. 3337)
-
1.5-ml Eppendorf microtubes (Merck, cat. no. Z606340)
Homemade production of lentiviral particles
Day 1: Seed HEK293T cells (∼20 min)
1.Seed one T150 flask of HEK293T per condition. The next day, cells must reach 50% of confluency. The T150 is filled up with 30 ml of DMEM containing 10% of FBS and penicillin-streptomycin (pen-strep, 100 U/ml).
Day 2 (end of the day): Calcium phosphate transfection of HEK293T cells with plasmids allowing the production of lentiviral particles (∼1 hr)
2.Prepare the transfection mix in a 15-ml conical tube per condition (i.e., per T150 flask):
- 1100 μl milliQ H2O
- 8 μg of plasmid of interest (shRNA or lentiviral GFP as a control)
- 6 μg of pCMV-dR8.91(Delta 8.9) packaging expression vector for lentivirus production encoding the gag , pol , and rev genes
- 4 μg of VSV-G plasmid expressing the viral envelope
- 170 μl of 2 M CaCl2
3.Add dropwise 1200 μl of 2× HEPES-buffered saline (HeBS) to the mix; vortex immediately.
4.Incubate for 20 min at room temperature.
5.Add the mix dropwise to the T150 flask. Gently shake the flask to homogenize the solution.
6.Incubate overnight in the incubator at 37°C under 5% CO2.
Day 3 (morning): Medium refresh (∼10 min)
Manipulation of lentivirus requires to perform experiments below a dedicated safety cabinet (BSL2+) and to be properly equipped and protected while handling lentivirus (days 3, 4 and 5).
7.Change the medium of HEK293T cells, with 30 ml fresh complete DMEM medium complemented with 10% FBS and pen-strep, to prevent toxicity due to the transfection reagent.
Day 4 (morning): Collection of lentiviral particles at ∼40 hr post-transfection (∼2 hr)
8.Collect the supernatant of HEK293T containing the lentiviral particles and filter it using a 0.2-μm membrane.
9.Divide the lentivirus-containing medium into 500 µl aliquots labeled beforehand and kept on ice.
10.Freeze the cryovials at −80°C.
11.Add 30 ml of fresh medium to HEK293T cells (DMEM with 10% FBS and pen-strep)
Day 5 (morning): Collection of lentiviral particles at 72 hr post-transfection (∼2 hr)
Lentiviral particles harvested at the different times post-transfection originate from the same HEK293T flask; only the medium is changed at step 11.
12.Repeat the same steps on day 4 above (steps 8 to 10).
Titration of lentiviral particles with the Lenti-X RT-qPCR titration kit (0.5 day)
The titration of lentiviral particles is a prerequisite to the calculation of the MOI value, which is the number of particles that can potentially infect a single cell, essential to the transduction process. First, RNA will be extracted and purified from the lentiviral particles. Then, the subsequent lentiviral genomic RNA will be amplified and quantified by qRT-PCR.
Extraction and purification of lentiviral genomic RNA
13.Follow the guidelines of NucleoSpin RNA virus kit.
Titration of lentiviral genomic RNA by RT-qPCR
14.Follow the guidelines of Lenti-X RT-qPCR Titration kit.
Lentiviral transduction and culture of colon organoids
Day 1: Generation of a single cell suspension of colon organoids (∼1.5 hr)
This step aims to gently break the maturing colon organoids (7 to 14 days old) into a single cell suspension in order to monitor with accuracy the number of cells/ml, leading to the establishment of an accurate MOI.
15.Depending on your experimental design (i.e., the number of conditions), select the appropriate number of domes to be passaged.
16.Wash the selected domes twice with DPBS.
17.Add 500 μl of TrypLE on top of the exposed dome in each well. Pipet the TrypLE in the well up and down 3 to 4 times to break up the dome and the organoids. Using the same pipette tip, transfer the organoid mixture to a 15-ml conical tube.
18.Repeat the previous step with 150 μl of TrypLE to ensure all pieces of BME have been recovered from the plate.
19.Incubate for 20 min at 37°C on an orbital tube rotator (∼400 rpm).
20.Centrifuge the cells 4 min at 500 × g , room temperature. Gently pour off and discard the supernatant.
21.Add 1 ml/dome passed of DMEM/F-12 + 15 mM HEPES + 1% BSA to each 15-ml conical tube and count the cells.
22.In a 24-well plate, seed in IntestiCult, 80,000 cells/well for a total volume of 200 μl in each condition (i.e., for each batch of lentiviral particles and/or MOI).
23.Prepare the mix of transduction in an Eppendorf tube, or a 15-ml conical tube (depending on the final volume), as shown in Table 2.
Reagents | 1 well (ml) | 6 well (ml) |
TransDux MAX | 2.5 | 15 |
MAX enhancer | 100 | 600 |
Y-27632 (10 mM) | 0.25 | 1.5 |
IntestiCult | 200 | 1200 |
Volume of the mix/well | ∼300 ml | |
Total volume/well | ∼500 ml |
24.Add the proper number of lentiviral particles.
25.Incubate the single-cell suspension with the lentiviral particles for 72 hr at 37°C (Fig. 4 A). Gently tap with your finger on the 24-well plate to homogenize the solution.
Day 4: Back to 3D-dome culture (∼1 hr)
At this step, the single-cell suspension is very heterogeneous with some of the transduced cells in suspension and others adherent. Most of them present clusters of cells with the formation of a lumen (Fig. 4A).
a. Preparation
26.Warm at 37°C, at least 2 hr prior the beginning of the experiment, a tissue-culture treated 24-well plate.
27.Prepare complete IntestiCult organoid growth medium (human) following the supplier guidelines, and warm it up to room temperature (15° to 25°C).
28.Prepare DMEM/F-12 with 15 mM HEPES and 1% BSA and keep it on ice.
29.Thaw on ice Cultrex, for each well of a 24-well plate.
b. Dome seeding
30.Transfer the supernatant of each well in a sterile Eppendorf tube, to collect the cells in suspension.
31.Rinse each well with 200 μl of DPBS at room temperature and transfer the supernatant to the corresponding Eppendorf tube.
32.Add 150 μl of Accutase in each well to detach gently the adherent cells. Incubate 5 min at 37°C. Under the microscope, double-check that all cells are detached and transfer the supernatant to the corresponding Eppendorf tubes.
33.Centrifuge the cells 4 min at 500 × g, room temperature. Gently pour off and discard the supernatant.
34.Add 60 μl of Cultrex per condition. Gently homogenize the cell pellet by doing up and down with the pipette.
35.Seed each dome in a well of 24-well plate.
36.Incubate the plate for 30 min at 37°C under 5% CO2 to allow dome solidification.
37.Once dome solidification is achieved, remove the 24-well plate from the incubator and add 500 μl of IntestiCult medium supplemented with 10 μM of the ROCK inhibitor Y-27632 to each single well, as well as 50 ng/ml of Wnt3a for healthy tissue exclusively.
38.Refresh the medium as described previously in Basic Protocol 2:
-
750 μl of IntestiCult from Monday to Friday.
-
500 μl of IntestiCult from Friday to Monday.
Day 12: Selection (∼15 min)
The selection process was performed at day 8 post-seeding, to allow organoid recovery before starting antibiotic treatment.
39.Start the selection by adding puromycin at 5 µg/ml in IntestiCult.
40.Refresh the medium as described above with puromycin at 5 µg/ml:
-
750 μl of IntestiCult + puromycin from Monday to Friday.
-
500 μl of IntestiCult + puromycin from Friday to Monday.
Basic Protocol 5: IMMUNOBLOT AND EVALUATION OF VARIABILITY BETWEEN DONORS
Originally, this immunoblotting protocol was developed to be able to extract and detect proteins from a single Cultrex-organoid dome as shown in Figure 5A. However, our first experiments revealed that the abundance of clathrin (CHC) varies from one dome to another. Since clathrin is a ubiquitously expressed protein involved in a canonic endocytic mechanism which ensures housekeeping cellular functions, its protein abundance generally correlates with the amount of cellular material present in a sample. In theory, CHC abundance should thus remain constant from dome to dome, given that the same volume of crypt solution has been seeded in each dome. Thus, the observed variations in the CHC abundance between domes likely reflect some variability in the number of organoids which effectively implanted in each dome and in their growth speed. Therefore, we eventually propose an alternative protocol in which 3 domes are pooled together to compensate this inter-dome variability and reach more homogeneous protein concentrations as shown in Figure 5B. This protocol can be applied to cancer biomarker discovery studies. Briefly, PDCO-domes are disrupted using TrypLE, washed and then incubated with a lysis buffer in reducing conditions to enable protein extraction. Then, proteins from the lysates are separated by SDS-PAGE and transferred onto a membrane using a Trans-Blot Turbo transfer system. Finally, the proteins of interest are detected by western blot and revealed by chemiluminescence.
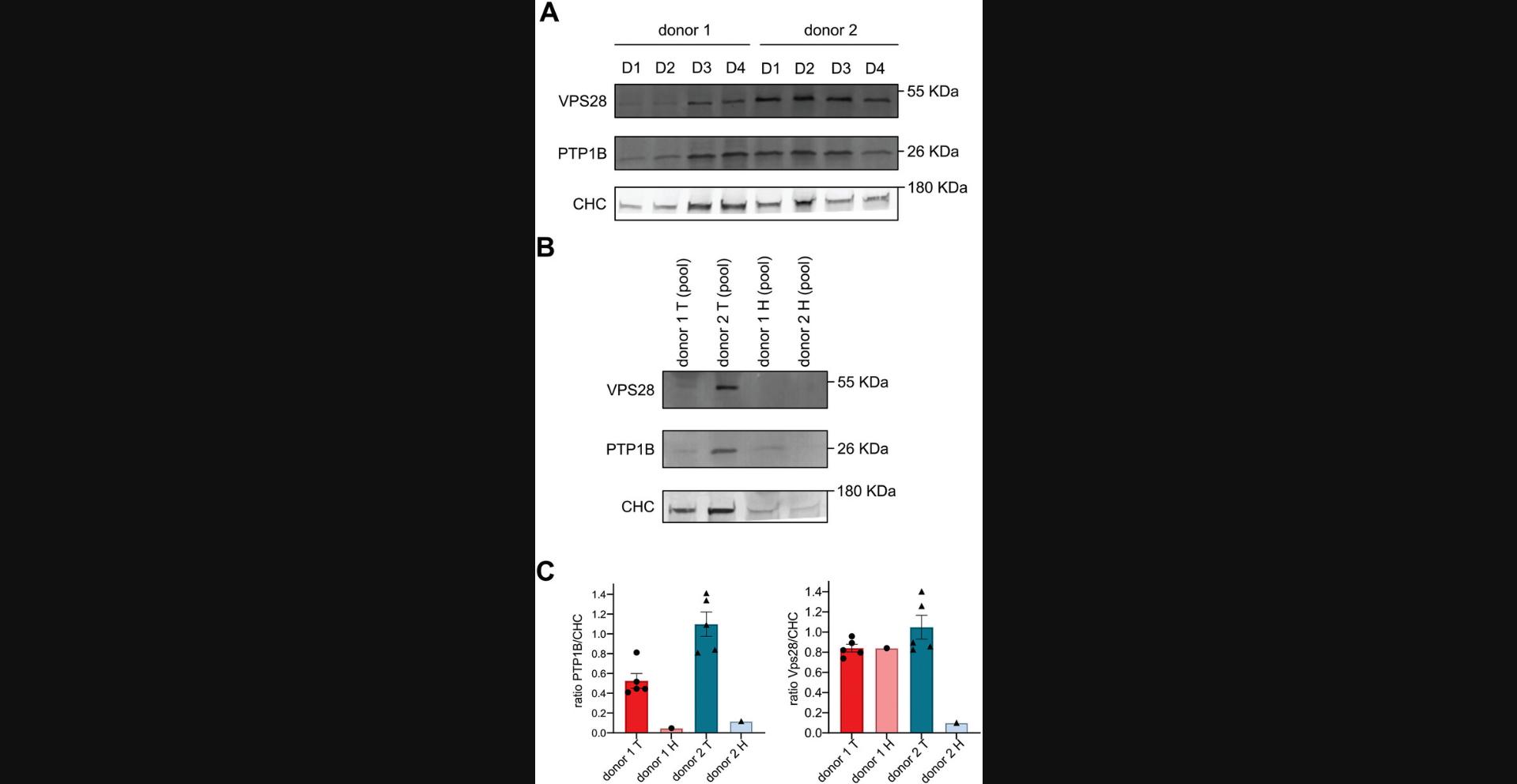
Materials
-
DPBS (see recipe)
-
TrypLE Express enzyme, 1×, no phenol red (ThermoFisher Scientific, cat. no. 12604039)
-
RIPA lysis buffer, 10× (Merck, cat. no. 20-188)
-
Loading buffer 5× (see recipe)
-
Migration buffer (see recipe)
-
4% to 15% Mini-PROTEAN TGX precast protein gels, 10-well, 50 µl (Bio-Rad, cat. no. 4561084)
-
Color pre-stained protein standard, broad range, 10 to 250 kDa (New Englands BioLabs Inc, cat. no. P7719S)
-
Trans-Blot Turbo RTA Mini LF PVDF transfer kit, 40 blots (Bio-Rad, cat. no. 1704272)
-
Wet-transfer buffer (see recipe)
-
Bovine serum albumin (BSA) fraction, protease-free, TSE-/BSE-free (Carl Roth, cat. no. T844.3)
-
Tween 20 (Carl Roth, cat. no. 9127.1)
-
Milk powder (Régilait 0.8 MG)
-
Pierce ECL western blotting substrate (ThermoFisher Scientific, cat. no. 32109)
-
SuperSignal West Femto maximum sensitivity substrate (ThermoFisher Scientific, cat. no. 34095)
-
Purified mouse anti-clathrin heavy chain clone 23 (BD Transduction Laboratories, cat. no. 610500)
-
Anti-VPS28 rabbit polyclonal antibody (Proteintech, cat. no. 15478-1-AP)
-
Anti-PTP1B antibody [EP1841Y] (Abcam, cat. no. ab75856)
-
Goat anti-mouse immunoglobulins/HRP (Agilent Technologies, cat. no. P044701-2)
-
Goat anti-rabbit immunoglobulins/HRP (Agilent Technologies, cat. no. P044801-2)
-
1.5-ml Eppendorf microtubes (Merck, cat. no. Z606340)
-
15-ml centrifuge tube (Corning, cat. no. 430791)
-
Tube revolver rotator (ThermoFisher Scientific, cat. no. 88881001)
-
Centrifuges, 5702 and 5425 (Eppendorf, cat. nos. 5702000010 and 5405000310)
-
Criterion vertical electrophoresis cell (Bio-Rad, cat. no. 1656001)
-
SureLock tandem midi blot module (Thermo Scientific, cat. no. S2001)
-
Western blotting filter paper, 0.83-mm thick, 8-cm × 13.5-cm (Thermo Scientific, cat. no. 84784)
-
Immobilon-FL PVDF, 0.45 µm, 8.5-cm × 10-m roll (Merck, cat. no. IPFL85R)
-
Trans-Blot Turbo transfer system (Bio-Rad, cat. no. 1704150)
-
Chemiluminescence imaging system
Protein extraction
1.Wash the domes of interest once with cold DPBS.
2.Add 500 μl of TrypLE on top of the dome in each well. Pipet the TrypLE in the well up and down 3 to 4 times to break up the dome and generate a single-cell suspension. Using the same pipette tip, transfer the organoid mixture to a 1.5-ml Eppendorf tube.
3.Repeat the previous step with 150 μl of TrypLE to rinse it and to ensure all pieces of organoids embedded in the dome of Cultrex have been recovered from the well.
4.Incubate for 20 min at 37°C on a tube rotator at ∼400 rpm.
5.Centrifuge the cells 4 min at 500 × g , 4°C. Carefully discard the supernatant.
6.Resuspend the cell pellet in 1 ml of ice-cold DPBS within the same Eppendorf tube.
7.Repeat step 5.
8.Resuspend the cell pellet in 20 μl of lysis buffer.
9.Incubate for 15 to 30 min on a shaker at 4°C.
10.Spin 10 min at 14,000 × g , 4°C.
11.Transfer the supernatant to a new Eppendorf tube.
Electrophoresis separation and semi-dry transfer
12.Add the loading buffer (1× final) to each sample to denature the proteins in reductive conditions and heat at 95°C for 10 min.
13.Fill up the electrophoresis tank with migration buffer (1×) containing SDS to allow proteins to migrate according to their molecular weight.
14.Load the samples on 4% to 15% Mini-PROTEAN TGX precast protein gels using color pre-stained protein standard ladder as a molecular weight marker.
15.Allow the migration at 160 to 180 V for 35 to 50 min depending on the size of the proteins of interest.
16.Perform semi-dry transfer using the Trans-Blot Turbo transfer system and following Bio-Rad guidelines (3 to 10 min).
17.Incubate the membranes in a blocking buffer containing 5% BSA with 0.05% of Tween 20 in DPBS (referred to DPBST 5% BSA), for at least 45 min at room temperature, on an orbital shaker.
18.Dilute the primary antibody (anti-clathrin heavy chain, anti-VPS28 and anti-PTP1B) in DPBST 5% BSA at the concentration recommended by the company.
19.Incubate the primary antibody on the membrane overnight at 4°C.
20.Wash the membrane 3 times for 5 min, at room temperature in DPBST 5% BSA.
21.Repeat steps 17 to 19 with a secondary antibody (goat anti-mouse or anti-rabbit) coupled to HRP and incubate the membrane for 30 min to 45 min at room temperature.
Chemiluminescence reaction and revelation
22.Use Pierce ECL western blotting substrate for revelation in chemiluminescence, if your proteins are well expressed and you expect a strong signal, such as for clathrin or tubulin. Alternatively, use SuperSignal West Femto substrate for revelation if your proteins have a weaker expression or if you expect a weak signal. Follow the supplier guidelines.
23.Monitor chemiluminescence signals with any western blot imager commercially available.
Basic Protocol 6: IMMUNOFLUORESCENCE LABELING AND HIGH-RESOLUTION MICROSCOPY OF PDCOS
This protocol aims to easily achieve high resolution confocal microscopy on PDCOs in order, for instance, to assess their maturation status or determine the localization of specific markers at the subcellular level (Fig. 6A-B). An important step in this protocol is to use the adapted devices developed for microscopy. Consequently, after passage, Cultrex-organoid dome cultures are seeded in Lab-Tek chambers (Fig. 6C), which have a glass bottom adapted to imaging by confocal and high-resolution microscopy devices displaying an inverted configuration. Once PDCOs grown in IntestiCult in the Lab-Tek containers have reached maturation, they are fixed with 4% PFA at 4°C, residues of PFA are quenched with NH4Cl, and cells are permeabilized with 0.5% Triton X-100. Then, primary and secondary antibodies are incubated with the Cultrex-domes to label the PDCOs. After extensive washing steps, PDCOs are ready for imaging.
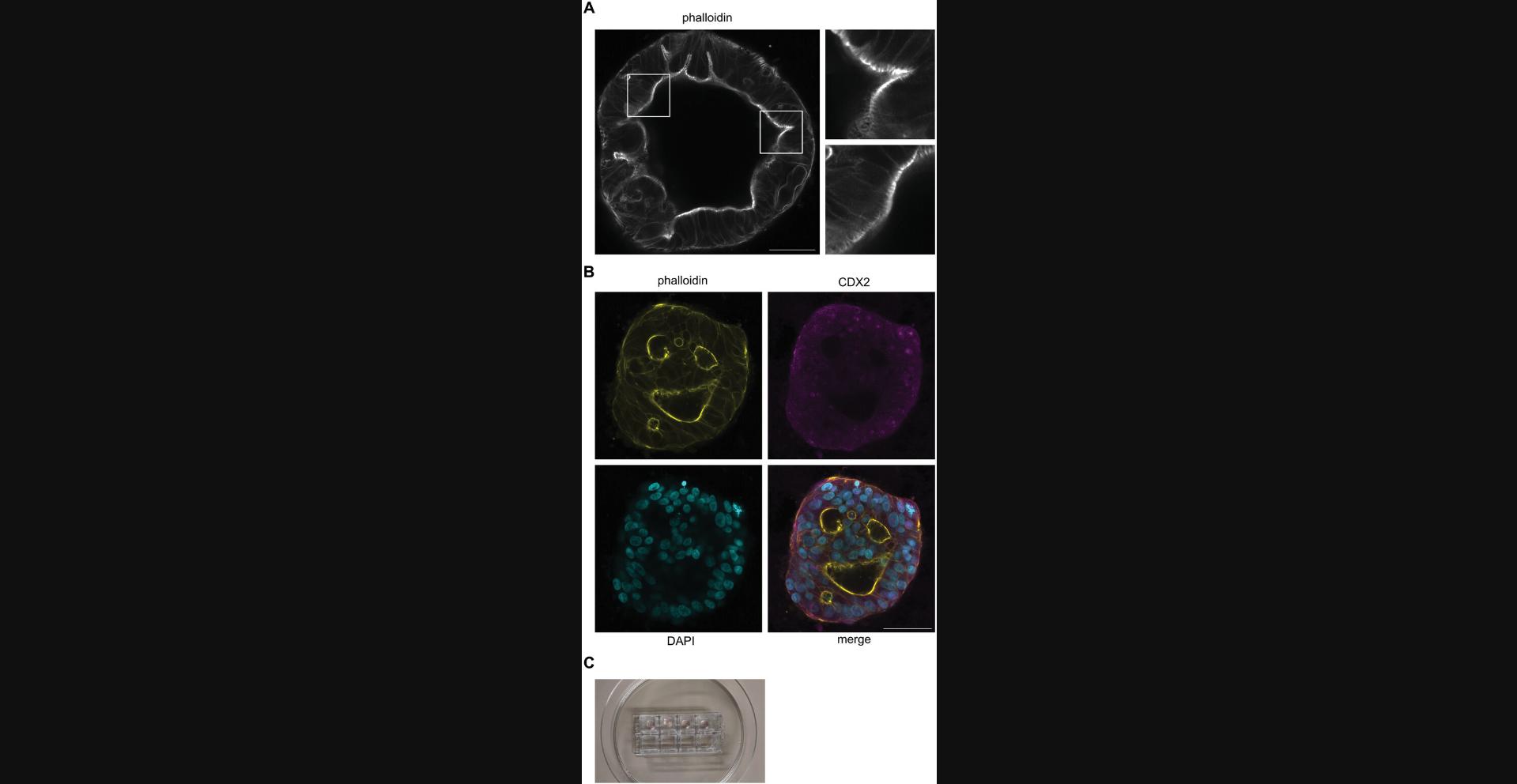
Materials
-
Cultrex UltiMatrix reduced growth factor basement membrane extract (RGF BME) (Bio-techne R&D Systems, cat. no. BME001-05)
-
IntestiCult organoid growth medium (human) (StemCell Technologies, cat. no. 06010) with antibiotic-antimycotic (1×) (ThermoFisher Scientific, cat. no. 15240062)
-
DPBS, no calcium, no magnesium (ThermoFisher Scientific, cat. no. 14190144)
-
4% PFA (see recipe)
-
NH4Cl (ammonium chloride) (Merck, cat. no. A9434)
-
Triton X-100 (Merck, cat. no. 9036-19-5)
-
Blocking buffer (see recipe)
-
Bovine serum albumin (BSA) fraction, protease-free, TSE-/BSE-free (Carl Roth, cat. no. T844.3)
-
Fetal bovine serum (FBS) (ThermoFisher Scientific, cat. no. 26140079)
-
Invitrogen Alexa Fluor 633 phalloidin (ThermoFisher Scientific, cat. no. A22284)
-
Anti-CDX2 mouse monoclonal antibody, clone 5A4E6 (Proteintech, cat. no. 60243-1-IG)
-
Anti-MUC2 rabbit polyclonal antibody (Proteintech, cat. no. 27675-1-AP)
-
Anti-Sox9 mouse monoclonal antibody (Proteintech, cat. no. 67439-1-IG)
-
Anti-CGA rabbit polyclonal antibody (Proteintech, cat. no. 10529-1-AP)
-
Anti-ZO-1 rabbit monoclonal antibody, D6L1E (Bioke, cat. no. 13663)
-
Anti-EPCAM rabbit polyclonal antibody (Proteintech, cat. no. 21050-1-AP)
-
DAPI (Merck, cat. no. D9542)
-
Appropriate secondary antibodies conjugated to Alexa Fluor 488/568 dyes (Invitrogen)
-
Nunc Lab-Tek chambered coverglass, #1.5 borosilicate glass (ThermoFisher Scientific, cat. no. 155411)
-
Zeiss LSM900 confocal microscope equipped with Airyscan 2 (or equivalent technology)
-
Cell culture dish, polystyrene, 100/20 mm (Greiner Bio-one, cat. no. 664160)
-
Corning Pasteur pipettes, non-sterile (Merck, cat. no. CLS7095B5X)
-
Plan-Apochromat 20×/NA 0.8 objective (Zeiss, cat. no. 440640-9903-000)
PDCO seeding in Lab-Tek chambered slides
1.Passage PDCOs following Basic Protocol 2 and seed 30 μl of the Cultrex-organoid suspension per well of a Lab-Tek chambered coverglass, #1.5 borosilicate glass.
2.Maintain the Cultrex-organoid domes from 2 to 4 weeks until you reach PDCO maturation, that can be assessed by monitoring organoid morphology (see Fig. 6A-B). Refresh the medium every other day with 250 μl of complete IntestiCult.
PDCO fixation
3.On the day of the experiment, carefully wash the dome cultures once with DPBS at room temperature.
4.Carefully discard DPBS using a Pasteur pipette as mentioned above.
5.Fix with 4% PFA 30 min at 4°C.
6.Quench PFA remnants with a 15 min incubation in 50 mM NH4Cl solution at room temperature.
PDCO permeabilization and blocking
7.Then, permeabilize the Cultrex-organoid domes with a solution of DPBS containing 0.5% Triton X-100 for 1 hr at room temperature.
8.Carefully discard the Triton X-100 solution.
9.Incubate the Cultrex-organoid domes with blocking buffer for 1.5 hr at room temperature. The blocking buffer is composed of 10% FBS, 1% BSA, and 0.1% Triton X-100 in DPBS.
PDCO fluorescence labeling
10.Carefully discard the blocking buffer solution without disturbing the domes.
11.Incubate primary antibodies diluted in the blocking buffer on top of the Cultrex-organoid domes for 16 hr at 4°C on a flat and still shelf.
12.Carefully discard the primary antibody solution without disturbing the domes. Gently wash once with 350 μl per well of blocking buffer.
13.Incubate Cultrex-organoid domes with DAPI and the secondary antibodies diluted in the blocking buffer for 2 hr at room temperature on a flat and still shelf, protected from light.
14.Carefully discard the secondary antibody solution without disturbing the domes. Carefully, wash three times with 350 μl per well of blocking buffer.
PDCOs high-resolution imaging with Zeiss LSM900 Airyscan 2
15.Perform the focus settings with DAPI staining through the objectives.
Basic Protocol 7: TRANSCRIPTOMIC ANALYSES OF PDCOS BY RT-qPCR
This protocol enables the extraction of mRNA from organoid-domes and the subsequent analysis of target gene expression through RT-qPCR. It allows for the comparison of gene expression under various conditions, such as tumor organoids vs healthy organoids after a specific treatment (Figure 7). In summary, PDCO-domes are disrupted using TrypLE, washed, and then incubated with a lysis buffer to facilitate mRNA extraction. Starting from mRNA, cDNA is generated using reverse transcriptase and specific primers. The cDNA is then analyzed using qPCR. For this type of analysis, it is recommended to pool at least three domes together and perform the extraction on the pooled sample. This approach ensures there is sufficient material and reduces variability between domes derived from the same donor, as previously mentioned in Basic Protocol 5.
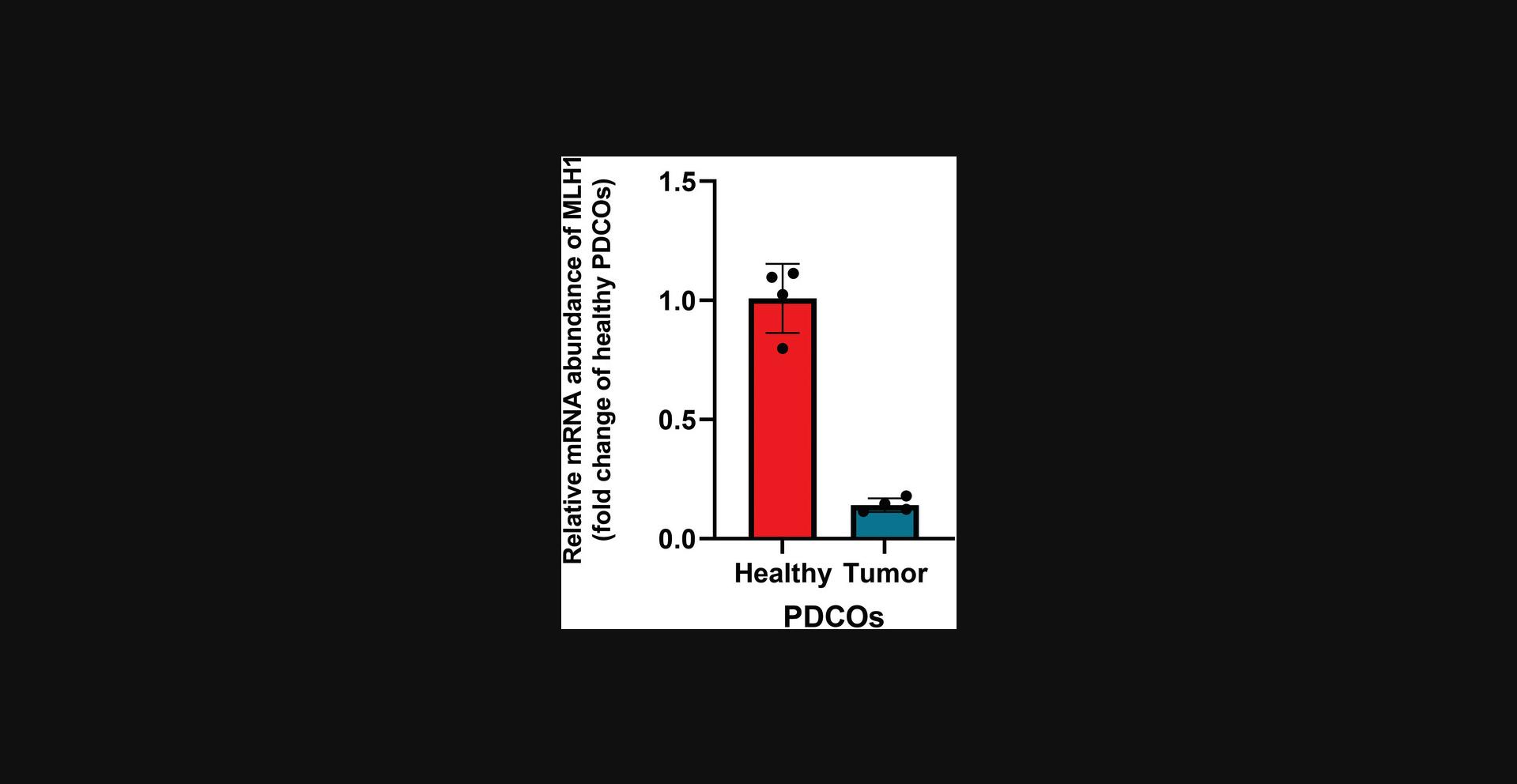
Materials
-
10% SDS solution, ultrapure (VWR, cat. no. J77504)
-
DPBS (see recipe)
-
TrypLE Express enzyme, 1×, no phenol red (ThermoFisher Scientific, cat. no. 12604039)
-
ReliaPrep RNA tissue miniprep system (Promega, cat. no. Z6111)
-
GoScript reverse transcriptase mix + random primer (Promega, cat. no. A2801)
-
GoScript reverse transcriptase mix + oligo(dT) (Promega, cat. no. A2791)
-
GoTaq qPCR and RT-qPCR systems (Promega, cat. no. A6001)
-
H2O, milliQ
-
Assorted pipettors and RNase-free filter tips
-
15-ml centrifuge tube (Corning, cat. no. 430791)
-
Tube revolver rotator (ThermoFisher Scientific, cat. no. 88881001)
-
Centrifuges, 5702 and 5425 (Eppendorf, cat. nos. 5702000010 and 5405000310)
-
1.5-ml Eppendorf microtubes (Merck, cat. no. Z606340)
-
NanoPhotometer N50 (Westburg Life sciences, cat. no. IM N50)
-
8-tube strips and caps, PCR, polypropylene, 0.2-ml (Greiner Bio-One, cat. no. 60827)
-
Savant SpeedVac DNA 130 integrated vacuum concentrator system (ThermoFisher Scientific, cat. no. DNA130-230)
-
MyBlock HL Mini dry bath with heated lid (Merck, cat. no. Z742495)
-
SimpliAmp thermal cycler (ThermoFisher Scientific, cat. no. A24811)
-
Vortex ZX3 (VWR, cat. no. 444-2000)
-
Applied Biosystems MicroAmp Fast optical 96-well reaction plate, 0.1-ml (Fisher Scientific, cat. no. 43-469-07)
-
MicroAmp optical adhesive film (Fisher Scientific, cat. no. 43-119-71)
-
ViiA 7 real-time PCR system with 96-well block (ThermoFisher Scientific, cat. no. 4453534)
NOTE : For this protocol, it is important to work in an RNase-free environment. To achieve this, clean the bench and all materials with a 1% SDS solution diluted in deionized water. Use appropriate gloves and RNase-free filter tips.
mRNA extraction
1.Wash the domes of interest once with cold DPBS.
2.Add 500 μl of TrypLE on top of the dome in each well. Pipette up and down with the TrypLE in the well 3 to 4 times to break up the dome and generate a single-cell suspension. Using the same pipette tip, transfer the organoid mixture of each dome in a common 15-ml centrifuge tube.
3.Repeat the previous step with 150 μl of TrypLE to rinse the well and to ensure all pieces of organoids embedded in the dome of Cultrex have been recovered from the well. Add the washing solution to the 15-ml centrifuge tube.
4.Incubate for 20 min at 37°C on a tube rotator at ∼400 rpm.
5.Centrifuge the cells 4 min at 500 × g , 4°C. Carefully discard the supernatant.
6.Resuspend the cell pellet in 1 ml of ice-cold DPBS and transfer it into a 1.5-ml Eppendorf tube.
7.Repeat step 5 to have a dry pellet at the end.
8.Follow the guidelines of ReliaPrep RNA tissue miniprep system.
9.After RNA extraction, keep the samples on ice.
10.Measure the mRNA concentration using a nanophotometer.
Reverse transcription
In this part of the protocol, it is crucial to work on ice, as mRNA is less stable compared to cDNA.
11.Dilute mRNA in 0.2-ml microtubes to reach 2 µg per condition in a maximum volume of 12 µl.
12.Heat the microtubes containing mRNA in a dry bath for 5 min at 70°C.
13.Prepare the Reverse Transcription Mix in an Eppendorf tube on ice, as shown in Table 3.Gentle vortex the mix and add 8 µl of the mix in each 0.2 ml microtube on ice.
Reagents | 1 sample (µl) | n sample + 1 |
H2O, RNase free | 2 | 2 × (n + 1) |
Buffer random primers | 2 | 2 × (n + 1) |
Buffer oligo dT | 2 | 2 × (n + 1) |
Enzyme mix | 2 | 2 × (n + 1) |
Volume of the mix/microtube | 8 µl | 8 × (n + 1) µl |
14.Spin the microtubes briefly at maximum speed, room temperature, and start the reverse transcription reaction in a controlled-temperature block or in a programmed thermal cycler.
15.After reverse transcription, spin the 0.2-ml microtubes briefly at maximum speed, room temperature. cDNA can be stored at 4°C for direct analyses or at −20°C for long-term storage.
Real-time PCR
For the real-time PCR, it is important to not forget a housekeeping gene (e.g., GAPDH) and a blank condition corresponding to PCR mix + H2O (without cDNA). Moreover, each sample needs to be done in triplicate. For the RT-PCR, we designed and validated our own primers, however validated commercial primers can be used.
16.Dilute cDNA 100-fold with miliQ H2O and dilute the qPCR primers at a concentration of 3 µM.
17.Prepare the PCR mix in an Eppendorf tube, as shown in Table 4.Vortex the PCR Mix.
Reagents | For each gene (µl) | n sample + 1 |
H2O, milliQ | 2 | 2 × (n + 1) |
Forward primer 3 µM | 2 | 2 × (n + 1) |
Reverse primer 3 µM | 2 | 2 × (n + 1) |
GoTaq qPCR master mix (2×) | 10 | 10 × (n + 1) |
Volume of the mix/gene | 16 µl | 16 × (n + 1) µl |
18.In a 96-well plate designed for real-time PCR, transfer 16 µl of PCR mix in each well (depending on the number of genes and conditions to test). Add 4 µl of cDNA diluted 100-fold in the corresponding wells, or 4 µl of milliQ H2O for the blank condition.
19.Seal the 96-well plate with an adhesive film and centrifuge the plate 1 min at 250 × g , room temperature.
20.Run the real-time PCR using the ViiA 7 real-time PCR system with standard cycling conditions.
REAGENTS AND SOLUTIONS
Blocking buffer
- 5 ml FBS
- 0.5 g BSA
- 50 µl Triton X-100
- 45.950 ml of DPBS (see recipe)
- Store up to 1 month at 4°C
CaCl2, 2 M
Add 14.7 g of CaCl2·2H2O in 50 ml of ultrapure H2O. Make fifty 1 ml aliquots into Eppendorf tubes and store indefinitely at −20°C. CaCl2 is in a liquid form at this temperature.
DPBS, 1×
- 9 g NaCl
- 20 ml 0.5 M phosphate buffer, pH 7.4
- Bring up to 1 L with deionized H2O
- Store indefinitely at 4°C
HEPES-buffered saline (HeBS), 2×
- 8 g NaCl (274 mM)
- 0.37 g KCl (10 mM)
- 0.1065 g Na2HPO4·2H2O (1.5 mM)
- 1 g dextrose (12 mM)
- 5 g HEPES (50 mM)
- 450 ml deionized H2O
- Adjust to pH 7 with NaOH
- Bring up to 500 ml with deionized H2O
- Store up to several years at 4°C
Loading buffer, 5×
- 10 ml SDS 20% (6%)
- 5 ml β-mercaptoethanol
- 10 ml 85% glycerol (24.3%)
- 17.5 mg bromophenol blue
- 35 ml H2O
- Store indefinitely at −20°C
Migration buffer, 10×
- For 1 L:
- 30.03 g Tris (250 mM)
- 143 g glycine (1.9 M)
- 100 ml 10% SDS
- Bring up to 750 ml with H2O
- Adjust pH to between 8 and 9 with NaOH or HCl
- Bring up to 1 L with H2O
- Store up to 6 months at 4°C
PFA, 4%
Break the 10 ml ampule of 16% formaldehyde solution, methanol-free (ThermoFisher Scientific, cat. no. 28908) and transfer the solution to a 50-ml centrifuge tube. Add 30 ml DPBS (see recipe). Due to PFA instability, aliquot PFA in 10-ml centrifuge tubes and store up to 6 months at −20°C. Alternatively store up to 3 weeks at 4°C. Avoid freeze/thaw cycles.
RIPA buffer
- 50 mM Tris·HCl
- 50 mM NaCl
- 0.25% sodium deoxycholate
- 1% Triton X-100
- 1 mM EDTA
- 0.1% SDS
- Dilute in deionized H2O
- Adjust to pH 7.4 using NaOH or HCl
- Store up to 1 year at 4°C
Wet-transfer buffer, 10×
- For 1 L:
- 30.03 g Tris (250 mM)
- 143 g glycine (1.92 M)
- Bring up to 750 ml with deionized H2O
- Adjust pH to 8.4 with NaOH or HCl
- Bring up to 1 L with deionized H2O
- Store up to 6 months at 4°C
Wnt3a, murine
Prepare a solution of DPBS (see recipe) with 0.2% BSA and filter it using a 0.2-µm filter. Reconstitute recombinant murine Wnt-3a (PeproTech, cat. no. 315-20) stock solution at 10 μg/ml by adding 1 ml of sterile DPBS containg 0.2% BSA in the vial containing 10 μg of Wnt3a. Use a working concentration of 50 ng/ml (dilution 1:200). Once reconstituted, make 50 μl aliquots and store up to 1 year at −80°C.
Y-27632, ROCK inhibitor
The Y-27632 (dihydrochloride) (StemCell Technologies, cat. no. 72304) stock solution is 20 mM and used at 10 μM. Add 0.5 μl to prepare 1 ml of IntestiCult supplemented in Y-27632. Once reconstituted store up to 1 year at −20°C protected from light. Make small aliquots of 50 μl to avoid thaw/freeze cycles.
COMMENTARY
Background Information
For decades, much of the knowledge we have accumulated in human biology has been from 2D culture and animal models. However, these models suffer from limitations: 2D-culture models, while being technically convenient, remain a simplistic view of cells growing independently to physiological parameters, which are crucial for integrating all the complexity of the human body. This level of complexity is inherent to animal models, being subsequently the clinical model of predilection. However, it is facing recurrent ethical issues and constant questioning about whether its discoveries are 100% transferrable and consequently relevant to human biology, based on the failure of a significant number of clinical trials (Harrison, 2016; Seruga et al., 2015). In 2009, Sato and colleagues revolutionized the disease model field with their hallmark study revealing how to grow a “mini-gut” from stem cells (Sato et al., 2009). Two years later, they published another breakthrough work reporting the generation of 3D organoids from patient biopsies, paving the way for personalized medicine therapy (Sato et al., 2011). These PDCOs are grown from colon-restricted adult stem cells resulting from healthy or diseased tissue resections. They are able to self-organize in vitro, self-renew, and proliferate. In addition, they display the features of the tumor or the healthy surrounding tissue, such as the complex cell hierarchy and spatial distribution highlighting their ability to recapitulate at best the phenotypic and molecular heterogeneity found in the colon. As such, they represent a growing interest in pre-clinical trial phases and personalized therapies (Chew et al., 2021; Gonzalez-Exposito et al., 2019; Pamarthy & Sabaawy, 2021). However, their generation, maintenance and study in the laboratory are confronted with budget contstraints and reproducibility issues. Moreover, the number of protocols for some techniques to study these PDCOs (including immunoblot, high-resolution confocal microscopy, and lentiviral transduction) is limited.
Critical Parameters
Access to a fresh source of biopsies near the lab
Considering that PDCOs must be generated from fresh biopsies, the most critical parameter that should be considered while starting any patient-derived organoid project, is the access to a hospital linked to a biobank located within a close perimeter from the laboratory. For instance, the authors were 30 min away from the biobank providing the biopsies.
Primary tissues used in this study were obtained from the Biobank of the CHU UCL Namur (coordinated by Dr. Fabienne George). The Biobank organized and supervised the controlled transit of the samples from the hands of the surgeons directly to the hands of the researchers under strict standard operating procedures.
BME selection
Basic Protocol 1 and 2 describe the establishment, maintenance, and expansion of PDCOs embedded in a dome of BME. The choice of BME is crucial to maintain a constant growth of the organoids. In addition, it is crucial to use BME showing consistency among batches and resistance over days in culture on different plastic- and glassware. That is the reason why Cultrex UltiMatrix RGF BME, and alternatively Geltrex LDEV-free reduced growth factor basement membrane matrix, have been chosen.
PDCO culture work with InstestiCult and cryopreservation with CryoStor CS10
In literature, several homemade conditioned media are described for the culture of patient-derived organoids. However, given the number of reagents composing these media and the associated risk accountable to variations between batches, in addition to the complexity to make them, the authors decided to choose InstestiCult. This medium is commercially available and ensures reproducibility. Moreover, InstestiCult has been developed by the Dutch biotech company HUB Organoid Technology to set up optimal culture conditions for efficient PDCO growth. It is crucial to use this culture medium for experimental reproducibility and to secure an efficient PDCO culture.
Another crucial medium is the one used for cryopreservation, as all patient-derived organoids are intended for biobanking. CryoStor CS10 offers the advantage of excellent recovery post-thaw, a crucial factor for organoid regeneration and growth.
Accurate titration of lentivirus and efficient transduction
The crucial step of PDCO lentiviral transduction is to define the MOI resulting in the most efficient transduction of PDCOs. Lentiviral particle titration must be extremely accurate, which can be achieved with Lenti-X RT-qPCR titration kit from Takara, as described in Basic Protocol 4. The operator must be extremely cautious to work under RNAse-free conditions. Moreover, it is vital to perform the titration on an aliquoted sample that is thawed to remain in the same condition (1 freeze/thaw cycle) as the lentiviral particles that will be used to transduce PDCOs.
Antibody specificity
Before their use, it is crucial to validate the specificity of each antibody. An siRNA approach targeting the protein of interest can be adopted, and the specificity of the antibody monitored by immunoblot or immunofluorescence. The antibodies mentioned in Basic Protocols 5 and 6 have been validated.
In addition to antibody specificity, it is essential to assess whether the antibody of interest can be used for different assays, such as immunofluorescence labeling or immunoblot. It is not guaranteed that an antibody working for immunofluorescence labeling will work also for immunoblot, and vice versa.
Adapted plastic- and glassware to each single downstream experiment
Before seeding Cultrex-domes containing organoid fragments, it is crucial to take time to consider whether the plastic- or glassware the users want to employ is adapted for the downstream analysis. For instance, these authors have reported in Basic Protocol 6 that utilizing Lab-Tek #1.5 borosilicate glass is optimal for confocal microscopy with LSM900 Airyscan 2.
Troubleshooting
Each step of the protocols has been troubleshooted to address the most frequently encountered issues during the process. Detailed troubleshooting information can be found in Tables 5 to 10. These tables primarily address common problems related to organoid-dome handling. For certain protocols, particularly Basic Protocol 7 that involves the use of commercial kits, it is advisable to consult the troubleshooting tables provided with those kits.
Problem | Possible cause | Solution |
---|---|---|
Biopsy cannot be digested into small pieces | The texture of the biopsy is extremely crunchy due to the tumor | Mince the biopsy to the smallest pieces that can be generated and continue the protocol (it will be less efficient) |
Biopsy has been cut into too big chunks | Mince the biopsy into the smallest pieces that can be generated | |
Healthy tissue organoid culture domes are not growing | Wnt3a forgotten | Add Wnt3a at 50 ng/ml |
Fragments extracted from the biopsy are not growing into organoids | Absence of intestinal crypts due to an over-digestion of the tissue or, on the contrary, not enough digestion | Adapt accordingly to the time of digestion |
Domes detach from the culture support | Presence of numerous bubbles | Leave a slim layer of medium above the pellet and resuspend it in this liquid phase before adding the Cultrex, mix intestinal crypts gently with Cultrex to avoid bubbles |
Too harsh flushing | Be gentle while refreshing the medium; do not use vacuum aspiration, but only pipet with a Pasteur pipette or with a tip | |
Absence of organoid fragments in domes freshly seeded after passaging | Loss of material during centrifugation steps | Increase the time of centrifugation steps to get an optimal pellet |
Do not disturb the pellet and do not discard the whole supernatant; always leave a slim layer of liquid above the pellet | ||
Generation of several organoid fragments after passaging but no growth induction | Organoid culture was not in an exponential phase | Carefully select the domes to be passaged; most organoids must display a doughnut-like shape |
Too high concentration of intestinal crypts | Increase the dilution of intestinal crypts | |
Organoids are growing extremely slowly after thawing | Organoids were not in an exponential phase when seeded | Passage organoid cultures before cryopreservation; do not wait >1 week between passage and cryopreservation |
Not enough intestinal crypts | Cryopreserved organoids should come from doughnut-like organoid cultures and not from too young or too mature cultures, which will result in the absence of Lgr5+ stem cells allowing the regeneration of organoids | |
Organoids do not recover after passaging | ROCK inhibitor forgotten | Add Y-27632 at 10 mM in the culture medium after dome seeding |
Problem | Possible cause | Solution |
---|---|---|
Cryopreserved organoids do not recover after thawing | Toxicity due to too slow freezing and/or thawing steps | Label cryovials in advance and put them on ice |
After 1 min in the water bath, add pre-warmed medium on top of the half-frozen cryovial supernatant and pipet it up and down to induce faster thawing | ||
ROCK inhibitor forgotten | Add Y-27632 at 10 mM in the culture medium after dome seeding | |
No cells after thawing | Loss of material during centrifugation steps | Increase the time of centrifugation steps to get an optimal pellet |
Do not disturb the pellet; do not discard the whole supernatant; always leave a slim layer of liquid above the pellet | ||
Organoids are growing extremely slowly after thawing | Organoids were not in exponential phase when seeded | Passage organoid cultures maximum 1 week before cryopreservation |
Not enough intestinal crypts | Cryopreserved organoids should come from doughnut-like organoids cultures and not from too young or too mature cultures, which will result in the absence of Lgr5+ stem cells allowing the regeneration of organoids |
Problem | Possible cause | Solution |
---|---|---|
Poor production of lentiviral particles | Too old HEK293T cells | Do not use HEK293T at a passage higher than 15 |
Failed transfection of HEK293T with CaCl2 | Double-check to add all the needed lentiviral plasmids in the mix | |
Add HeBS 2× quickly to the mix, process one sample at a time | ||
Poor recovery of lentiviral particles after thawing | Failed cryopreservation | Cryopreservation must be performed with CryoStor CS10 quickly on ice, cells must be kept in a freezing container at −80°C and be transferred the next day in liquid nitrogen |
Not enough cells seeded after the single cell suspension solution | Loss of cells | Be cautious during the recovery of solutions (PBS wash, Accutase steps) and optimize the centrifugation steps |
Cells remained adherent | Increase the incubation time with Accutase at 37°C, assess under a bright-field microscope the number of cells detached before transferring the cell solution and discard the plate | |
Poor transduction | Transduction reagent | Use TransDux MAX |
Single-cell incubation time too short | It is extremely important to let the transduction mix incubate on the single cell suspension of organoids for 72 hr; 24 and 48 hr have been assessed and failed | |
Non-optimal MOI | Depending on the plasmid used, adapt the MOI | |
Organoids dying after transduction | Apoptosis due to stress | Do not forget to add Rock inhibitor Y-27632 at 10 µM |
Problem | Possible cause | Solution |
---|---|---|
Poor extraction: no protein detected after lysate titration | Lysis failed | Increase the incubation time with the lysis buffer (be careful to work at 4°C) |
Low number of organoids per dome | Pool several domes belonging to the same condition altogether | |
Signal variability in the loading control | Absence of protein titration | Titrate protein levels with a range of BSA |
Selection of a wrong protein as loading control | Use ubiquitous proteins, e.g., cytoskeleton proteins, which exhibit constancy over time and treatments | |
No migration/transfer | Buffer problem | Double check on the buffer composition, and pay attention to work with the 1× |
Weak transfer with Turbo-Transblot | Technical issue with the device | Clean the electrodes of Turbo-Transblot with a solution of acetic acid 20% (in H2O) and wash several times with water before use |
Be sure that the magnets of Turbo-Transblot are still in place at the back of the cassette | ||
Transfer time not adequate | Choose the optimal program depending on the size of the proteins of interest: low <25 kDa, mixed for proteins between 25 and 150 kDa, high >150 kDa | |
Non-specific signal | Problem with blocking buffer/antibody | To be more stringent, use PBST with 5% milk to block instead of PBST with 5% BSA |
Test the antibody specificity using a lysate from cells in which the protein of interest has been depleted by RNA interference (siRNA, shRNA, etc.) |
Problem | Possible cause | Solution |
---|---|---|
Destruction of Cultrex-domes and loss of the organoids | 4% PFA fixation step | Decrease the time of incubation with 4% PFA from 30 min to 20 min at 4°C; if the problem persists, work with 2% PFA at room temperature for 10 min |
Detachment and aspiration of Cultrex-domes | Abrupt movement leading to harsh flushing of the dome | Be extremely cautious during washing steps; if using the transfer pipette induces too harsh flows, work with P200 tips or a Pasteur pipette |
Autofluorescence | PFA is not pure enough | Use PFA adapted to microscopy |
High noise compared to the signal | Non-optimal final washing step | Increase the incubation time of the washing steps |
Add one more washing step | ||
Faint signal | Non-optimal antibody concentration/problem with permeabilization | Increase antibody concentration and/or the incubation time with the permeabilization buffer |
No signal under the confocal microscope | Magnification/focus plan problems | Due to the thickness of the domes, objectives with high magnification (≥40×) or small working distance are not usable; prefer the use of lower magnification objectives (e.g., dry objectives ≤20×) or objectives with larger working distance to reveal signal; focus is done using DAPI signal |
Problem | Possible cause | Solution |
---|---|---|
Poor extraction of mRNA, concentration of mRNA is low | Lysis failed | Add the right volume of lysis buffer (recommended in Promega kit) |
Elution problem | If the first elution gave a too low concentration of mRNA, a second elution is possible (but mRNA will be more diluted); it is possible to concentrate the mRNA using a vacuum concentrator system | |
Low number of organoids per dome | Pool several domes belonging to the same condition altogether | |
Problem with 260/280 and 260/230 ratios | Poor sample wash | PBS wash after incubation with TrypLE is crucial; take care to eliminate any trace of TrypLE |
Understanding Results
This article described a step-by-step workflow to generate PDCOs from biopsies as presented in Figure 1. This results in the establishment of PDCO cultures that undergo morphological and size changes over the course of weeks, which is inherent in their growth as shown in Figure 2A. Indeed, after 2 to 3 days, small doughnut-like organoids appear in the dome and grow to become larger spheres/ovals containing a lumen after 1 to 2 weeks. Then, some buds become visible and the PDCOs start their complex folding to reach their mature morphology ∼4 to 6 weeks. Figure 2B displays the different PDCOs coming from tumor or healthy tissues, generated by the authors following Basic Protocols 1 and 2. It is important to highlight that depending on the donor and the nature of the tissue used to establish the organoid culture, the growth rate will vary in speed. Therefore, the proposed timeline offers ranges and not defined values (Fig. 2A).
Basic Protocol 4 aims to tackle the technical challenge of establishing silenced PDCO cultures. As reported in Figure 3, PDCOs are first dissociated to generate a single cell culture suspension solution. The latter is transduced with lentiviral particles containing the shRNA of interest, here a GFP lentiviral vector to visualize the efficiency of the proposed strategy. After 72 hr (Fig. 4A), cells are seeded back in domes and their growth and GFP expression are monitored over 21 days using inverted epifluorescence microscope (Fig. 4B). Green fluorescence can be noticed already 7 days post-transduction for an MOI of 1000, 4000 and 6000. The reader may think that these MOI are very high, but it must be considered that all the particles will not necessarily infect the cells due to the complex medium and the interference it can induce. Remarkably, it was reported that after 21 days in culture, the transduced PDCOs with the 3 different MOI display mature morphology. An MOI of 4000 reveals a PDCO population expressing medium levels of GFP compared to an MOI of 1000 (low) and an MOI of 6000 (high). These data led the authors to conclude that an MOI of 4000 seems to be a relevant MOI to trigger efficient silencing in PDCOs. Of note, it is important to adjust this MOI to the different lentiviral vectors used, investigating different titrations, and following the same example as the ones tested in this article. Finally, the antibiotic selection was addressed and showed notable death of the PDCOs with 5 μg/ml of puromycin (Fig. 4C).
In the literature, the study of potential therapeutic target genes often begins with classical biochemistry investigations. This is exactly what the authors encourage to do in PDCOs with the description of Basic Protocols 5 to 7. First, the immunoblot approach was under the spotlight revealing a variation in protein abundance between domes of the same donor (Fig. 5A). This can be explained by the various number of organoids inside each dome. That is the reason why it was suggested to pool together 3 domes to tackle this variability and generate representative and reproducible data. In addition, the results highlighted an expected heterogeneity of protein levels between different donors, and between the different types of tissues (tumor versus healthy) (Fig. 5B). The variability between donors is the perfect illustration of what happens in clinical trials. Although it will involve increasing the number of donors to achieve statistically significant data, it strongly supports the fact that PDCOs is a relevant pre-clinical model. Then, the differentially expressed protein levels observed between healthy PDCOs vs tumor PDCOs is an important argument to use this protocol to identify novel colorectal cancer biomarkers. For instance, the immunoblot presented in the article may suggest that PTP1B could be considered as such, although it would require further investigations to be confirmed. Another way to analyze the differences between tumor and healthy tissues is to look at the transcriptomic data. Thanks to RT-qPCR, we can analyze the expression of target-genes depending on several conditions. To conclude, the other biochemical assay investigated is the immunofluorescence labeling monitored by inverted high resolution confocal microscopy. In this article, immunofluorescence labeling has been used to assess PDCO maturation status, but this assay is not restricted to this aspect. Indeed, it could be used to monitor the spatial distribution of different molecular players including the potential therapeutic targets. Confocal imaging microscopy reveals microvilli brush borders of enterocyte cells as well as intestinal progenitor marker (CDX2), showing the cellular complexity of the PDCOs that have been generated (Fig. 6A-B).
Time Considerations
In addition to the timeline described in each basic protocol listed hereabove, the reader must also consider one's level of technical expertise. Indeed, this point is extremely relevant for Basic Protocol 1, dealing with the establishment of PDCOs from biopsies, since someone with expertise in cellular biology techniques will be more efficient due to some automatism acquired with experience than someone starting from scratch. Besides this crucial aspect, the particularity of this protocol is to work with fresh biopsies, meaning that the operator must arrange for the transport of the biopsies and take into consideration this extra time that will define the starting point of Basic Protocol 1. Consequently, it is necessary to work with a biobank located at close range from the laboratory. Once the 2 biopsies (tumor tissue and healthy tissue) arrive under the BSC, the whole steps of Basic Protocol 1 can be completed between 3.5 hr and 5 hr depending on the operator's level of expertise. Then, the timeline of the maintenance of PDCO cultures, as referred in Basic Protocol 2, is detailed in Figure 2A, with the obtention of mature PDCOs after 4 to 6 weeks, which is dependent on each patient and piece of biopsy received. Of note, healthy tissue with the addition of Wnt3a tends to grow a bit faster than tumor PDCOs generated from the same patient.
Each PDCO passage should take <1 hr, with an average of 8 domes to passage. Of course, this time consideration can vary depending on the number of domes to be passaged and then to be seeded.
Considering that when reaching Basic Protocol 3 to perform cryopreservation and/or thawing of PDCOs, the operator would have acquired expertise in PDCO culture through Basic Protocol 1 thus the cryopreservation step should take between 1 hr to 1.5 hr, depending on the number of domes and consequently of samples that will be cryopreserved. The thawing of 4 to 6 cryopreserved PDCO vials should take between 45 min to 1 hr.
Due to the length and complexity of Basic Protocol 4, detailed time considerations are directly given in the different protocol sections. However, for an overview, the generation of lentiviral particles leading to the freezing can be performed in 5 days (usually from Monday to Friday). Then, allow half a day for lentiviral titration, which is usually in the morning prior to performing PDCO transduction in the afternoon. The generation of single cell suspension from dome-organoid culture and transduction take 1.5 hr. Finally, allow between 45 min and 1 hr to generate dome-organoid culture starting from transduced cells.
For Basic Protocol 5, depending on the number of samples, allow between 1 hr and 1.5 hr to perform the protein extraction steps. Then, consider 1.5 hr for both sample preparation and SDS-PAGE (add 1 hr to this timeline if casting homemade gels). The transfer step will last between 5 and 10 min, plus 10 min of preparation. Finally allow 45 min for the blocking step, overnight incubation for primary antibodies, 1 hr for secondary antibodies (including washes), and an average of 15 to 30 min for the acquisition of the chemiluminescence data (can vary depending on the specificity of the antibody and the level of expression of the protein of interest).
For Basic Protocol 6, the seeding of PDCOs on Lab-Tek takes the same time as the standard passage described in Basic Protocol 2 (<1 hr). Allow one afternoon to fix, permeabilize, and block overnight the PDCOs, allowing 1 hr for the fixation and the permeabilization, respectively. Then allow the next morning to perform the rest of the experiment. Finally, no time consideration can be provided regarding the imaging acquisition step since it depends on the number and the quality of the samples, on the microscope used, as well as on the resolution and the outcomes sought. Finally, for Basic Protocol 7, the extraction of mRNA and the reverse transcription can be done in a half-day and real-time PCR take ∼3 hr (depending on the number of conditions).
A summary of time considerations for each basic protocol is presented in Supplementary Figure 3 (see Supporting Information).
Acknowledgements
We would like to acknowledge the following people or entities for providing experimental and technical help: Hortence Paré and the CHU UCL Mont-Godinne, especially Morgane Canonne. J.-W.C. is supported by ProtherWal project funded by the Walloon Region (Belgium). H.-F.R. is supported by a Start-Up Grant Collen-Francqui from Francqui Foundation (Belgium) and an Incentive Grant for Scientific Research from the Fonds de la Recherche Scientifique (FNRS, MIS-F.4540.21). N.Z. received a grant from “Covid impact: support for postdocs (2021-2022) from the Wallonia-Brussels Federation” (Belgium). Workflow diagrams were produced with BioRender software. Microscopy images were treated and/or quantified using Fiji software (fji-win64). Bar graphs were generated with GraphPad Prism software (Prism9).
Author Contributions
Emilie Rigaux : Data curation; formal analysis; investigation; validation; visualization; writing original draft; writing review and editing. Jia-Wei Chen : Data curation; methodology; validation; visualization; writing review and editing. Fabienne George : Resources; writing review and editing. Julien Lemaire : Resources. Claude Bertrand : Resources. Laurence Faugeras : Resources. Antoine Fattaccioli : Investigation. Quentin Gilliaux : Resources. Lionel D'Hondt : Resources; supervision. Carine Michiels : Funding acquisition; supervision; writing review and editing. Henri-François Renard : Conceptualization; funding acquisition; project administration; supervision; validation; visualization; writing review and editing. Natacha Zanin : Conceptualization; data curation; formal analysis; funding acquisition; investigation; methodology; project administration; supervision; validation; visualization; writing original draft; writing review and editing.
Conflict of Interest
The authors declare no competing interests.
Open Research
Data Availability Statement
The authors declare that the main data are available within the manuscript. Extra data are available from the corresponding authors on reasonable request.
Supporting Information
Filename | Description |
---|---|
cpz1943-sup-0001-FigureS1.pdf926 KB | Supplementary Figure 1: Lentiviral transduction of PDCOs. Representative images of PDCO growth and expression of GFP from the lentiviral vector in additional conditions, complementary to Figure 4B. (A) After 7 days post-transduction, MOIs of 100 and 500 (20× objective). Of note, the efficiency of transduction appears lower at these MOIs. (B) After 7-, 14- and 20-days post-transduction, MOI of 6000. Scale bars, 100 µm for 20×, 200 µm for 10×. |
cpz1943-sup-0002-FigureS2.pdf2.8 MB | Supplementary Figure 2: Overview of lentiviral transduction of PDCOs observed at lower magnification (4× objective). Complementary to Figure 4B. Representative images of PDCO growth and expression of GFP from the lentiviral vector after 14- and 20-days post-transduction with MOIs of 1000, 4000 and 6000. Of note, the lower magnification allows us to observe a homogeneous expression of GFP at MOIs of 4000 and 6000. Scale bar, 500 µm. |
cpz1943-sup-0003-FigureS3.pdf94.2 KB | Supplementary Figure 3: Summary of time considerations for each Basic Protocol. |
Please note: The publisher is not responsible for the content or functionality of any supporting information supplied by the authors. Any queries (other than missing content) should be directed to the corresponding author for the article.
Literature Cited
- Chew, N. J., Lim Kam Sian, T. C. C., Nguyen, E. V., Shin, S. Y., Yang, J., Hui, M. N., Deng, N., McLean, C. A., Welm, A. L., Lim, E., Gregory, P., Nottle, T., Lang, T., Vereker, M., Richardson, G., Kerr, G., Micati, D., Jardé, T., Abud, H. E., … Daly, R. J. (2021). Evaluation of FGFR targeting in breast cancer through interrogation of patient-derived models. Breast Cancer Research , 23(1), 82. https://doi.org/10.1186/s13058-021-01461-4
- Gonzalez-Exposito, R., Semiannikova, M., Griffiths, B., Khan, K., Barber, L. J., Woolston, A., Spain, G., von Loga, K., Challoner, B., Patel, R., Ranes, M., Swain, A., Thomas, J., Bryant, A., Saffery, C., Fotiadis, N., Guettler, S., Mansfield, D., Melcher, A., … Gerlinger, M. (2019). CEA expression heterogeneity and plasticity confer resistance to the CEA-targeting bispecific immunotherapy antibody cibisatamab (CEA-TCB) in patient-derived colorectal cancer organoids. Journal for Immunotherapy of Cancer , 7(1), 101. https://doi.org/10.1186/s40425-019-0575-3
- Harrison, R. K. (2016). Phase II and phase III failures: 2013–2015. Nature Reviews Drug Discovery , 15(12), 817–818. https://doi.org/10.1038/nrd.2016.184
- Pamarthy, S., & Sabaawy, H. E. (2021). Patient derived organoids in prostate cancer: Improving therapeutic efficacy in precision medicine. Molecular Cancer , 20(1), 125. https://doi.org/10.1186/s12943-021-01426-3
- Sato, T., Stange, D. E., Ferrante, M., Vries, R. G., van Es, J. H., van den Brink, S., van Houdt, W. J., Pronk, A., van Gorp, J., Siersema, P. D., & Clevers, H. (2011). Long-term expansion of epithelial organoids from human colon, adenoma, adenocarcinoma, and Barrett's epithelium. Gastroenterology , 141(5), 1762–1772. https://doi.org/10.1053/j.gastro.2011.07.050
- Sato, T., Vries, R. G., Snippert, H. J., van De Wetering, M., Barker, N., Stange, D. E., van Es, J. H., Abo, A., Kujala, P., Peters, P. J., & Clevers, H. (2009). Single Lgr5 stem cells build crypt-villus structures in vitro without a mesenchymal niche. Nature , 459(7244), 262–265. https://doi.org/10.1038/nature07935
- Seruga, B., Ocana, A., Amir, E., & Tannock, I. (2015). Failures in Phase III: Causes and consequences. Clinical Cancer Research , 21(20), 4552–4560. https://doi.org/10.1158/1078-0432.CCR-15-0124