Agrobacterium-Mediated Transformation of Setaria viridis, a Model System for Cereals and Bioenergy Crops
Todd Finley, Todd Finley, Hal Chappell, Hal Chappell, Veena Veena, Veena Veena
AGL1
Agrobacterium-mediated transformation
Agrobacterium tumefaciens
bioenergy crops
C4 photosynthesis
cereals
foxtail millet
ME034V-1
model system
Setaria viridis
transformation
Abstract
Setaria viridis is an emerging model system for the genetic and molecular characterization of cereals and bioenergy crops. Here, we describe a detailed procedure for genetic transformation of the S. viridis accession line ME034V-1. This method utilizes callus generated from mature seeds for infection with Agrobacterium tumefaciens strain AGL1 to regenerate hygromycin-resistant stable transgenic plants. It takes approximately 7 weeks to generate callus from mature seeds, 11-17 weeks from infection to the regeneration of transgenic lines, and an additional 3-4 weeks for plant growth in the greenhouse for seed collection. The protocol as presented consistently results in transformation frequency of approximately 25% for the generation of transgenic plants, with fewer escapes and higher survivability in soil for optimal seed collection. © 2021 Donald Danforth Plant Science Center. Current Protocols published by Wiley Periodicals LLC.
Basic Protocol 1 : Generation of S. viridis (Accession ME034V-1) callus from mature seeds
Basic Protocol 2 : Agrobacterium -mediated transformation of callus to generate transgenic plants
Basic Protocol 3 : Plantlet transplantation in soil, plant growth in greenhouse, and seed collection
Support Protocol : Preparation of Agrobacterium culture for infection
INTRODUCTION
Setaria viridis is the wild ancestor of Setaria italica (foxtail millet) and exhibits an evolutionary relationship with various agronomically important plant species such as maize, sorghum, sugarcane, and bioenergy feedstocks such as switchgrass and Miscanthus (Martins et al., 2016; Muthamilarasan et al., 2015). It embodies several desirable traits similar to the most popular dicot model plant, Arabidopsis thaliana. S. viridis has a short stature, small genome size, and rapid life cycle, produces a copious amount of seeds per generation, is amenable to genetic manipulation, and is easy to grow in laboratory settings (Brutnell et al., 2010; Kellogg, 2013; Li & Brutnell, 2011; Pant, Irigoyen, Doust, Scholthof, & Mandadi, 2016). In addition, the availability of a sequenced and annotated genome (Bennetzen et al., 2012; Huang, Shyu, Coelho, Cao, & Brutnell, 2016), a publicly accessible collection of transcriptomes (Anderson et al., 2021; Ermawar et al., 2015; Ferreira et al., 2019; Kikuchi, Shyu, & Brutnell, 2017), and repositories of geographically diverse and ecologically distinct accessions have provided new opportunities to unravel the complexities of various biochemical and physiological pathways, including C4 photosynthesis, towards the goal of developing new crop varieties with improved traits. Crops with a higher yield and beneficial agronomic attributes for sustainable agriculture are among the most significant global challenges of the 21st century. This is, in part, due to the rising global population, economic growth in developing countries, and societal pressure for sustainable energy production while preserving the environment and vital natural resources. Combinatorial approaches, including efficient and sustainable crop production methods and improvement by breeding and genetic engineering, will be required to meet these critical challenges.
Over the years, several methods have been developed for gene delivery methodology in S. viridis (accession A10.1) using Agrobacterium tumefaciens. These include (a) tissue culture−based methods that utilize infection of callus derived from mature seeds and (b) in planta methods that involve infection of floral organs, e.g., the floral-dip method (Martins, Nakayama et al., 2015; Martins, Ribeiro, Cunha, Kobayashi, & Molinari, 2015; Nguyen, Van Eck, Eamens, & Grof, 2020; Plaza-Wüthrich, & Tadele, et al., 2012; Van Eck, 2018; Van Eck & Swartwood, 2015; Van Eck, Swartwood, Pidgeon, & Maxson-Stein, 2017; Wang et al., 2011). Among these, the method utilizing Agrobacterium -mediated transformation of callus derived from mature seeds is the most popular for the recovery of stable transgenic plants. Despite the aforementioned progress, the method is labor-intensive, lacks reproducibility, and suffers from the low transformation efficiency of the accession line A10.1.These bottlenecks have hindered the pace of wider adoption of S. viridis as a model system.
We describe an efficient and reproducible protocol for A. tumefaciens− mediated transformation of the S. viridis accession line ME034V-1 using callus derived from mature seeds (Fig. 1). This method consistently results in approximately 25% transformation efficiency based on the number of infected calli and selection of one transgenic event per callus. The method as described shows lower escape rates and high survivability when plants are transferred to soil. Critical factors for the improved efficiency and reliability in recovery of transgenic plants includes the choice of the S. viridis accession (for example, ME034V-1 that has been screened and selected for its transformability potential; see Background Information for details) and utilization of quality seeds, freshly prepared medium, tightly controlled environmental conditions, and timely subculturing during various stages of transformation. The method outlined in this paper provides descriptive details for the various key processes, including tips on critical parameters and troubleshooting to facilitate the rapid generation of S. viridis transgenic plants.
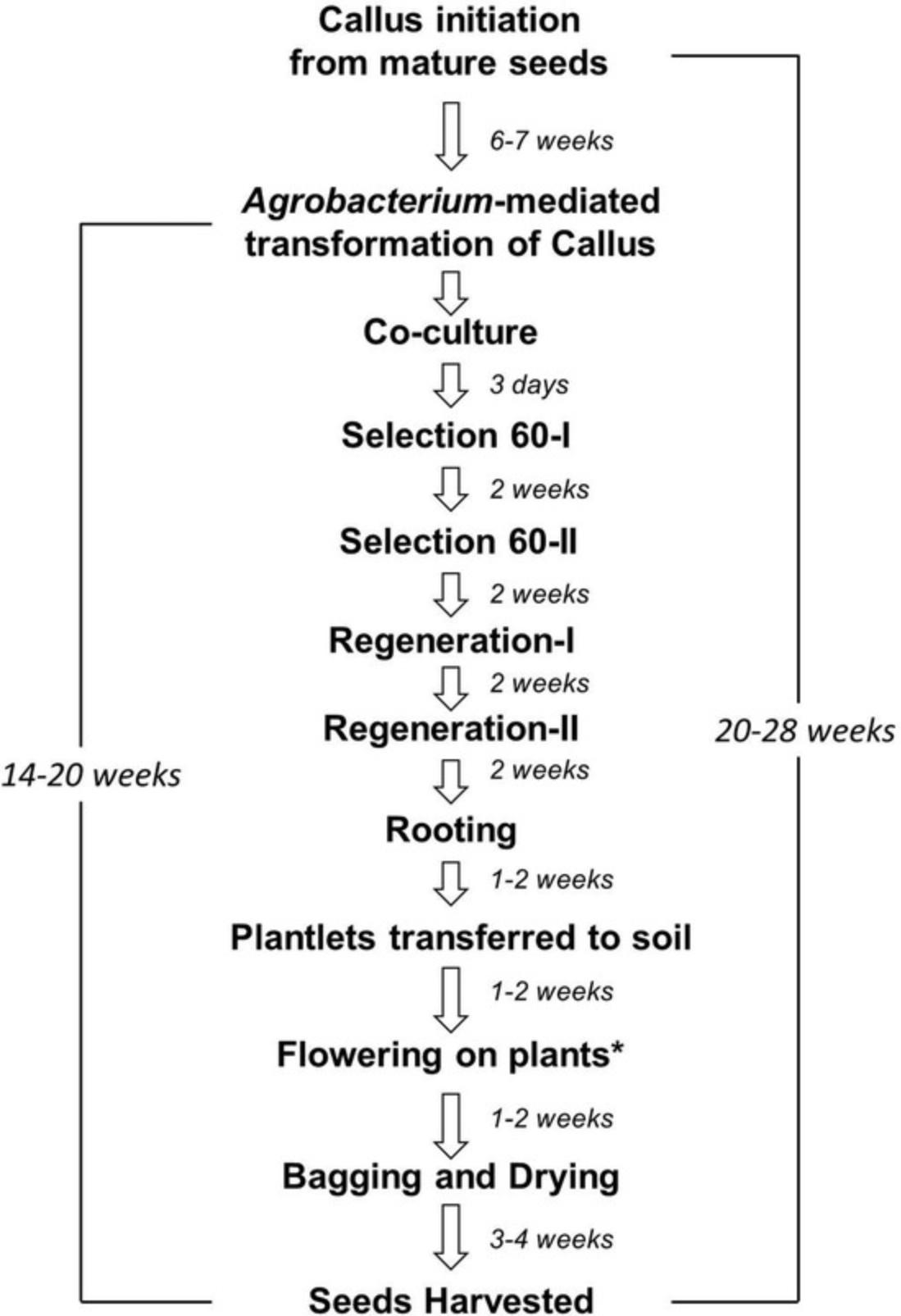
In this article, we describe the method for transformation of S. viridis accession line ME034V-1 using A. tumefactions strain AGL1. This method comprises three stages:
- 1.Initiation of callus from mature seeds.
- 2.Agrobacterium -mediated transformation of callus to generate transgenic plants.
- 3.Transfer of transgenic plants to soil, plant care, and seed harvest.
Basic Protocol 1: GENERATION OF S. VIRIDIS (ACCESSION ME034V-1) CALLUS FROM MATURE SEEDS
In this first section, we describe the protocol to generate callus starting from dry mature seeds. This method utilizes dehusked seeds, followed by sterilization and plating in callus-inducing medium to generate high-quality callus. The resulting callus is amenable to Agrobacterium -mediated transformation when implemented as described below.
Materials
- S. viridis (ME034V-1) seeds
- Note that ME034V-1 = ME034V. Source : https://npgsweb.arsgrin.gov/gringlobal/accessiondetail?id=1918592.
- 6% (v/v) bleach (Clorox)
- 1% (v/v) Tween 20 (Sigma, cat. no. P1379)
- Callus induction medium (CIM) plates (see recipe)
- Seed dehusking device (Fig. 2B)
- The seed dehusking device used in this work was made using wooden pieces and fine-rib corrugated rubber matting assembled in the shape of a float trowel (similar to the tool commonly used to level and smooth concrete) and a base tray. The bottom surface of the float and the base tray inner surface are lined with a corrugated plastic sheet with a ribbed side facing outwards. Rubber matting used in the device was purchased from Amazon (http://www.amazon.com/gp/product/B01DCR5JB2/ref=ppx_yo_dt_b_asin_title_o05_s00?ie=UTF8&th=1). Alternatively, a method described by Van Eck and Swartwood (2015) can be used to dehusk the seeds.
- 50-ml conical tube (Falcon, cat. no. 352070)
- Fine-tip forceps
- Vertical rotating mixer (BenchTop Lab Systems, cat. no. VM-80)
- Rocking platform
- Whatman filter paper (Whatman, cat. no. 1001-085)
- Laminar flow hood
- Plastic wrap (we routinely use plastic wrap from GLAD to wrap Petri plates containing plant tissue during various stages of transformation) or Micropore tape (3M Company)
- Plant growth chambers with following conditions:
- 24°C dark
- 24°C, 16-hr photoperiod, 60 μ/m2/s light
- Stereomicroscope
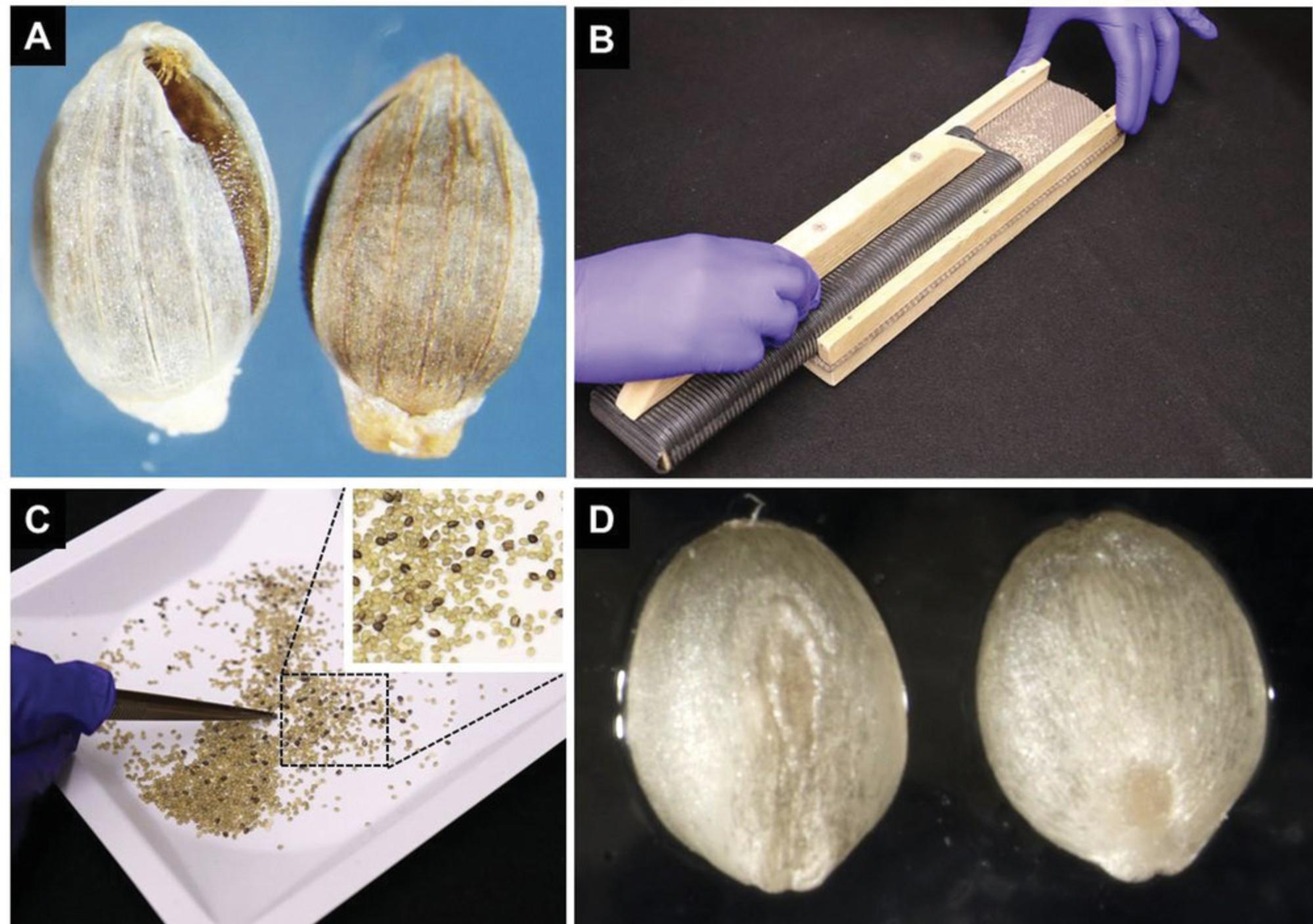
Initiation of callus from S. viridis ME034V-1 seeds
1.Remove seed coats from 2-3 g of dry seeds (dehusk the seeds; Fig. 2A). We use a homemade device shown in Figure 2B for the removal of the seed coat. Avoid applying too much pressure on the seeds, as it could damage the embryos. Remove chaff and seeds that retain their seed coat (Fig. 2C and 2D).
2.Sterilize the dehusked seeds in a 50-ml conical tube filled with 40 ml of sterilization solution made with 10% bleach plus 0.1% Tween-20 for 10 min on rotating mixer at 30 rpm (Fig. 3A).
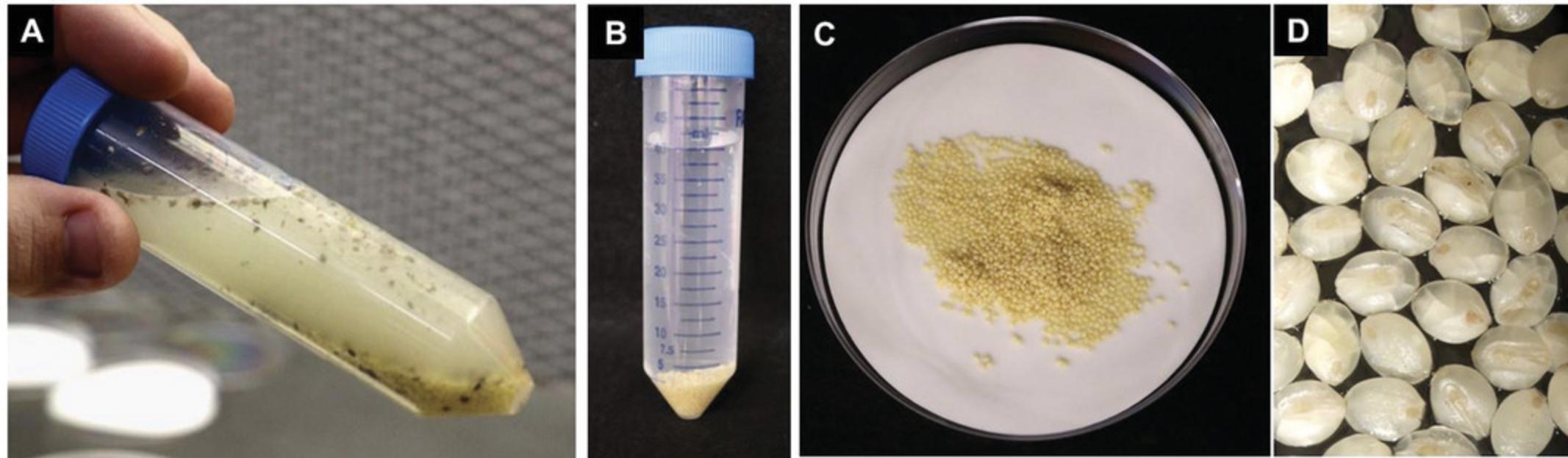
3.Rinse seeds five times with sterile deionized water, gently rocking the tube for 1-2 min during each rinse step (Fig. 3B).
4.After the completion of the final rinsing step, remove the excess water and transfer the sterilized seeds on top of two to three pieces of dry, sterile Whatman paper (Fig. 3C and 3D). Allow seeds to dry in a laminar flow hood for 20-30 min.
5.Plate out ∼25 evenly spaced seeds (Fig. 4A) with the intact embryo facing upwards onto each plate containing CIM medium (Fig. 4B). This orientation is important for the development of high-quality, regenerable callus compared to seeds with the embryo side plated touching the medium surface (Fig. 4C). Wrap plates with plastic wrap or Micropore tape and place plates in a growth chamber maintained at 24°C with a photoperiod of 16 hr for 1 week (Fig. 5A) followed by 4-5 weeks incubation in the dark at 24°C.
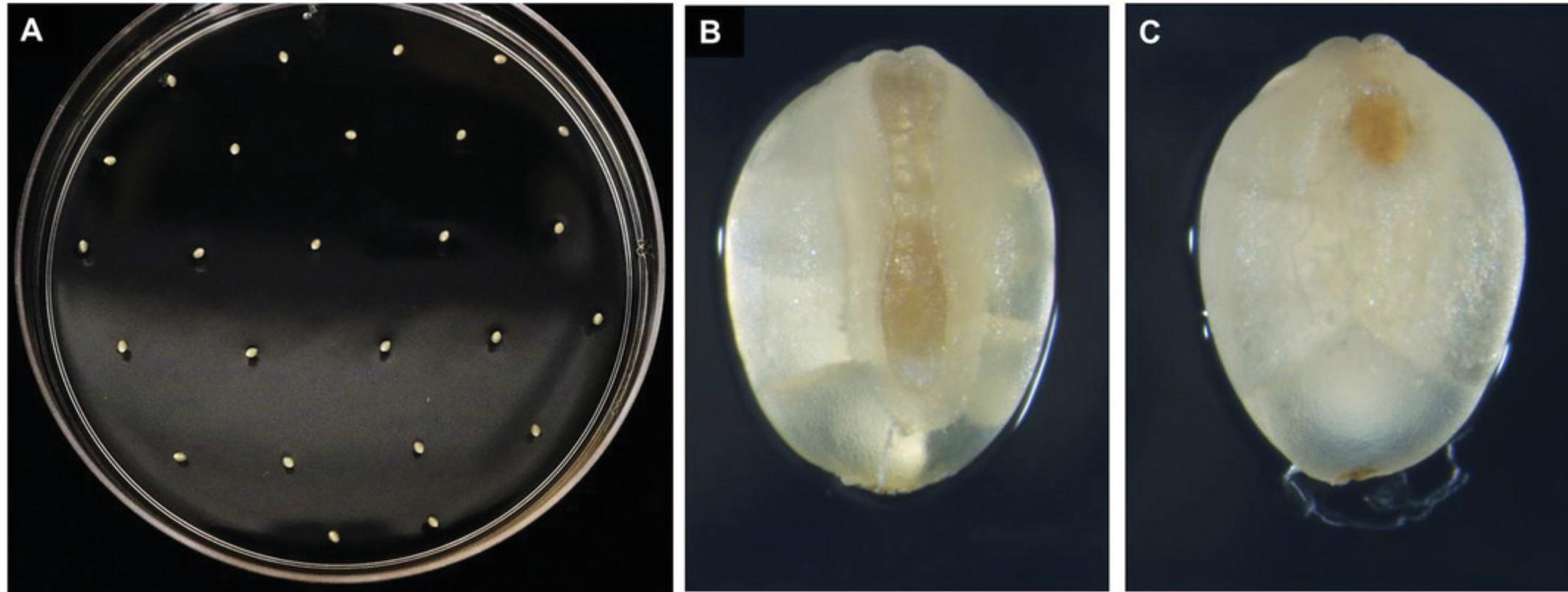
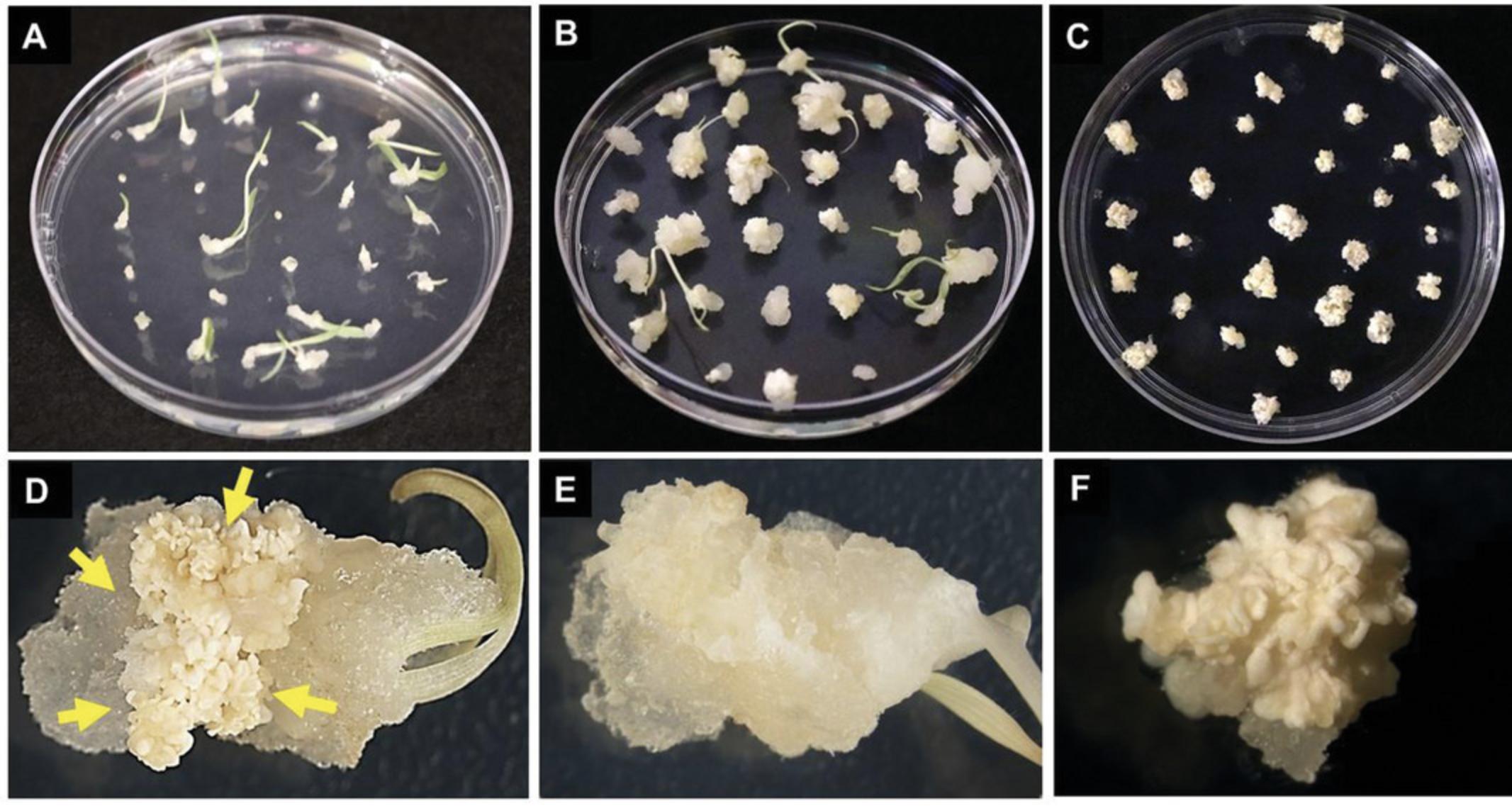
6.Remove any leaves, roots, and gelatinous or dead tissue from the callus (Fig. 5E) and sub-culture calli (Fig. 5B and 5D) onto freshly prepared CIM (Fig. 5C). Incubate newly sub-cultured calli in the dark at 24°C for 1 week (Fig. 5C and 5F) prior to transformation with Agrobacterium (Basic Protocol 2).
Basic Protocol 2: Agrobacterium-MEDIATED TRANSFORMATION OF CALLUS TO GENERATE TRANSGENIC PLANTS
The following protocol describes the generation of transgenic plants via A. tumefaciens -mediated transformation using callus derived from mature seeds, as described in Basic Protocol 1.This basic protocol is divided into three steps: (i) preparation of Agrobacterium for transformation, (ii) inoculation and co-culture of explants derived from Basic Protocol 1 with Agrobacterium strain AGL1 containing binary plasmid, and (iii) selection and regeneration of plants.
Materials
-
A. tumefaciens (AGL1) containing a binary vector that contains a selectable marker gene cassette comprising a ubiquitin promoter driving hygromycin phosphotransferase (hyg) e.g., binary vector pANIC10A (Mann et al., 2012; pANIC10 vector obtained from Addgene); see Support Protocol for culture and preparation
-
LB plates containing appropriate antibiotics (see recipe)
-
Liquid CIM (liquid callus induction medium; see recipe)
-
200 mM acetosyringone (Solution F; see recipe for Stock solutions)
-
Callus generated from mature seeds of S. viridis (ME034V-1) (see Basic Protocol 1)
-
Co-culture medium plates (see recipe)
-
Plant regeneration medium plates (see recipe)
-
Plant rooting medium plates (see recipe)
-
Growth chamber with following condition:
- 24°C light/dark photoperiod (16 hr/8 hr)
- 21°C dark
-
50-ml conical tubes (Falcon, cat. no. 352070)
-
Disposable loops (VWR, cat. no. 30002-090)
-
Spectrophotometer (Thermo Scientific, GENESYS 30)
-
Sterile filter paper, 85 mm diameter (Whatman, cat. no. 1001-085)
-
Petri plates, 20 × 100 mm (VWR, cat. no. 25384-342)
-
Aluminum foil
-
Solo cups, 8 oz, 236 ml; Solo Cup Company, cat. no. SD8) or Magenta boxes
Preparation of Agrobacterium for transformation
1.Three days prior to the initiation of transformation, streak a lawn of Agrobacterium from a “master plate” (see Support Protocol) onto LB plates containing the appropriate selection agents (e.g., carbenicillin and kanamycin for A. tumefaciens AGL1 containing pANIC10A binary vector). Incubate plate at 22°C for 2 days (Fig. 6A).
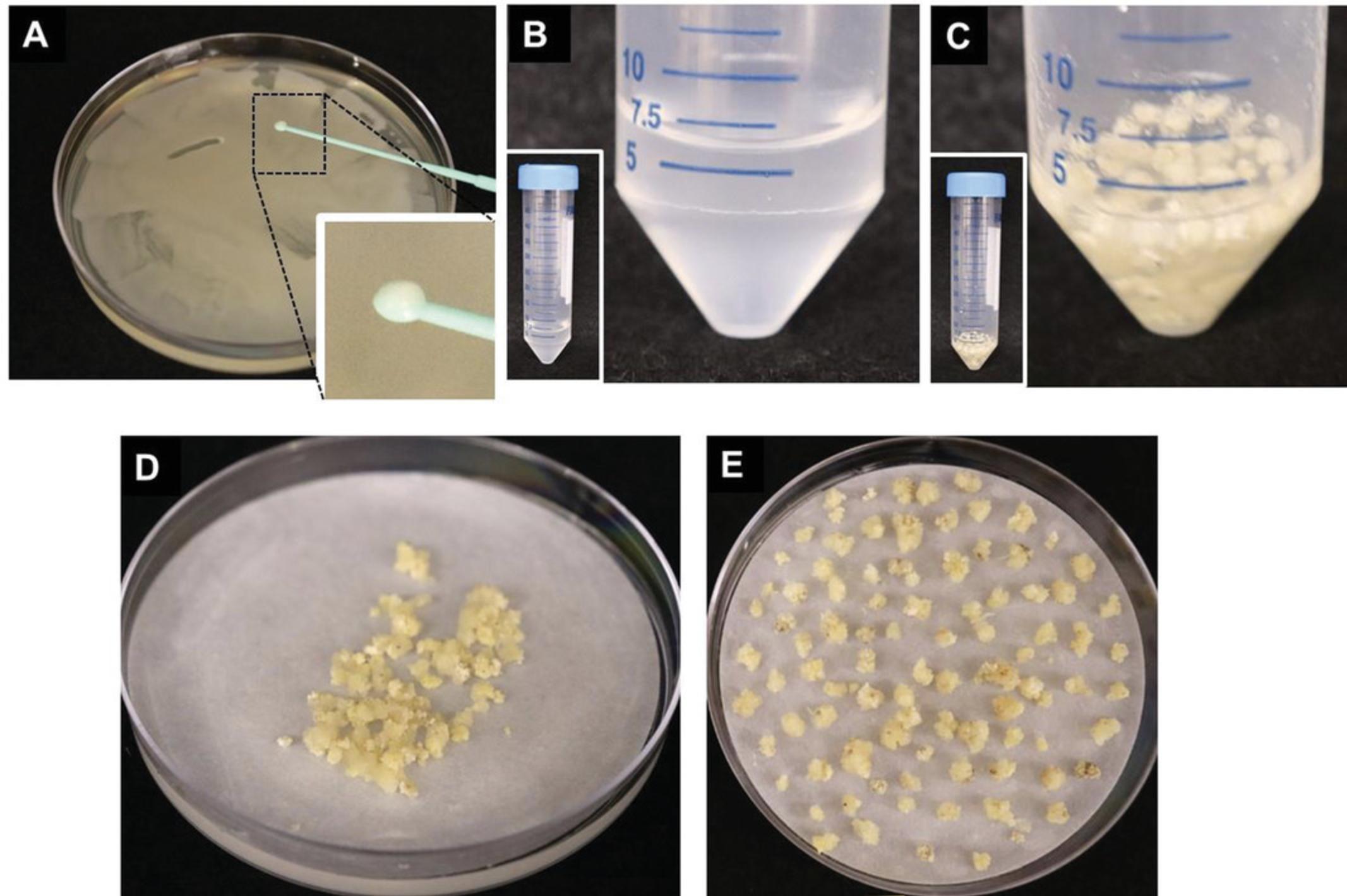
2.Take 2-3 loops of Agrobacterium lawn (using a 1-μl loop) and inoculate into a 50-ml conical tube containing 10 ml of liquid CIM medium supplemented with 200 μM acetosyringone. Gently shake the tube until all Agrobacteria have evenly dispersed in the liquid medium to form a homogeneous suspension (Fig. 6B).
3.Measure OD600 and adjust to 0.25-0.4 with liquid CIM supplemented with 200 μM acetosyringone (Fig. 6B).
Inoculation of explants and co-culture
4.Transfer ∼100 calli from 1-week-old sub-cultured callus (step 6 of Basic Protocol 1) into a sterile 50-ml conical tube.
5.Add liquid CIM containing Agrobacterium suspension (from step 3) so that the callus is completely submerged in the Agrobacterium suspension solution (Fig. 6C).
6.Vortex gently for 10 s and incubate for 5 min.
7.Pour calli and Agrobacterium solution onto a stack of 2-3 sterile sheets of filter paper placed inside an empty Petri plate. Swirl plate until solution has soaked into the filter paper (Fig. 6D).
8.Transfer 100 calli per petri plate from step 7 directly onto a sterile sheet of filter paper that is placed on top of co-culture medium in a Petri plate (Fig. 6E).
9.Wrap plates with aluminum foil and incubate in dark for 3 days at 22°C for co-culture.
Selection and regeneration of plants
10.Transfer callus to CIM Selection 60 medium plates (CIM Selection 60-I; see Fig. 1). Place ∼20 pieces of calli per plate and incubate in dark for 2 weeks at 24°C (Fig. 7A and 7D).
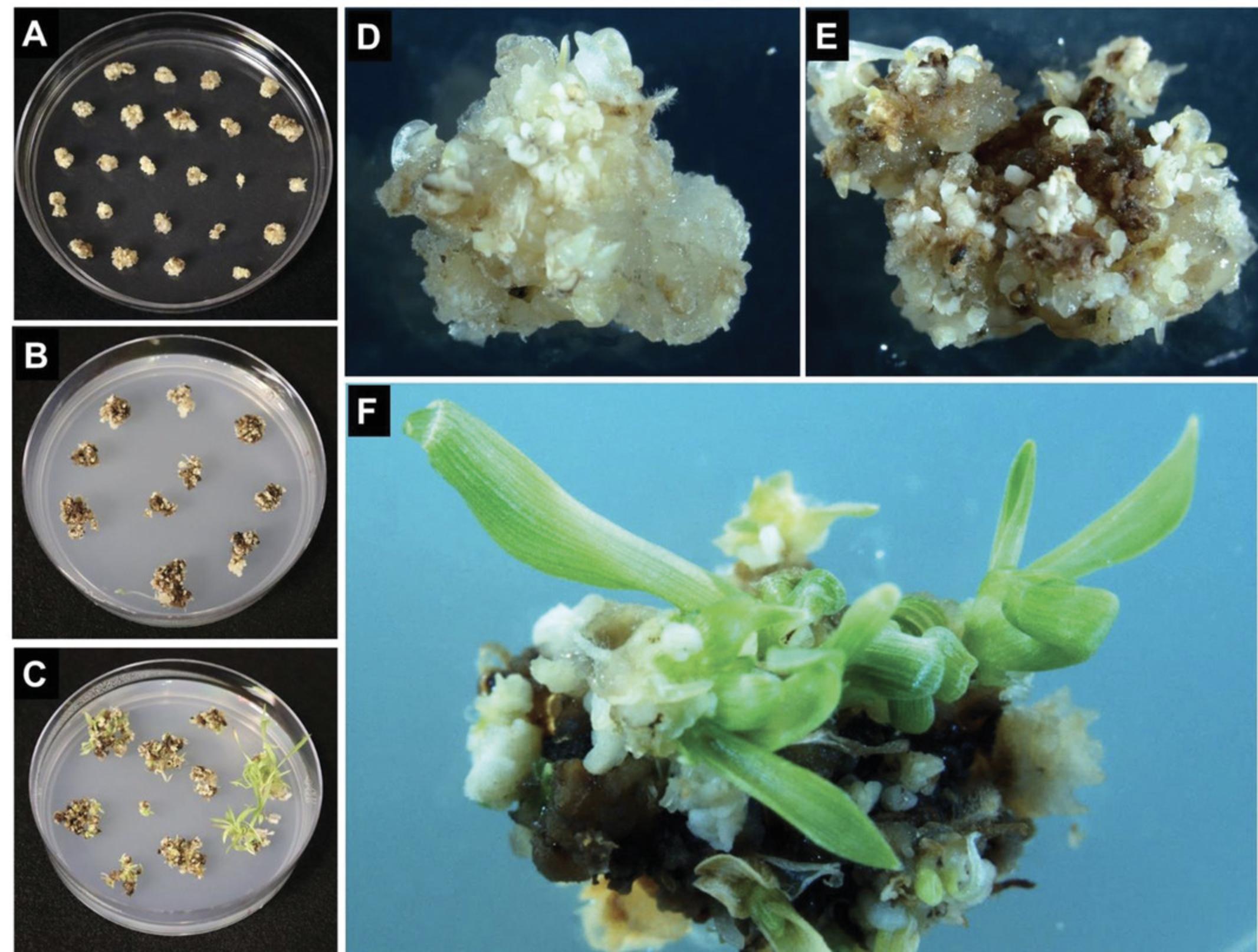
11.Subculture callus to fresh CIM Selection 60 medium plates (CIM Selection 60-II; see Fig. 1). Transfer ∼14 pieces per plate and incubate in dark for 2 weeks at 24°C in the dark (Fig. 7B and 7E).
12.Transfer callus to plant regeneration medium (Regeneration-I; see Fig. 1). Transfer ∼12 callus pieces per fresh regeneration medium plate and incubate at 24°C under a 16-hr photoperiod for 2 weeks. Repeat for additional 2 weeks under same conditions with ∼ 8 callus pieces per medium plate (Regeneration-II; see Fig. 1).
13.Subculture callus onto the fresh plant rooting medium plates (Rooting; see Fig. 1) containing the appropriate selection. Transfer 4 callus pieces per plate and incubate at 24°C under a 16-hr photoperiod for 1-2 weeks until shoots have developed (Fig. 7C and 7F).
14.Once shoots have developed, transfer ∼10 mm tall shoots to the rooting medium plates, typically four independent events/plantlets per plate, with a Solo Cup lid (Fig. 8A-8C), and incubate at 24°C under a 16-hr photoperiod for 1-2 weeks until the plantlet is big enough and formed robust roots system (Fig. 8D).
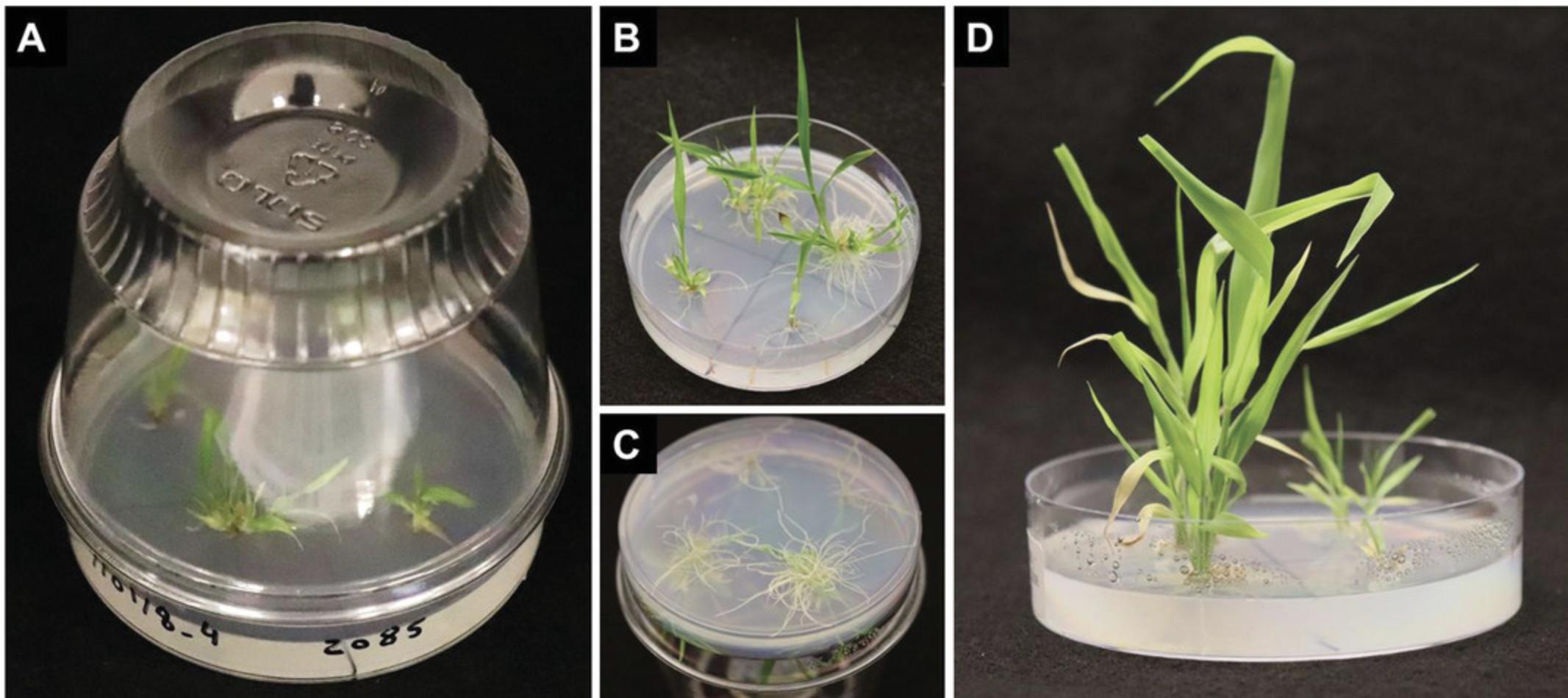
15.When the shoots have well established primary and lateral root systems (Fig. 8C), transplant them into soil (Basic Protocol 3).
Basic Protocol 3: PLANTLET TRANSPLANTATION IN SOIL, PLANT GROWTH IN GREENHOUSE, AND SEED COLLECTION
The shoots from Basic Protocol 2 that have well-developed root systems are transferred to soil for further growth and seed collection. The method outlined below offers the highest survivability potential for putative transgenic plantlets under greenhouse conditions. Routine monitoring and inspection of plantlets is advisable to identify any issues and implement corrective measures in a timely manner to maintain healthy plant growth for optimal seed collection.
Materials
-
S. viridis (accession: ME034V-1) plantlets from Basic Protocol 2
-
Potting soil (Jolly Gardener Products, Inc., Jolly Gardener Potting Soil)
-
Reverse osmosis (RO) water (U.S. Filter, cat. no. ROSLV21602)
-
Fertilizer: Jack's Professional 20-20-20 General Purpose (JR Peters, Inc., cat. no. 77010)
-
Plastic holding trays with holes (Hummert International, Flat T.O. Plastics Standard 1020 Open W/Holes, cat. no. item no. 11300000; see Fig. 9)
-
Flat 1801 Deep individually perforated inserts (Hummert International, Insert T.O. Plastics 1801 Standard, cat. no. 11075000; see Fig. 9)
-
Spray bottle
-
Humi-Dome, Clear Plastic (Hummert International, cat. no. 14385100)
-
Greenhouse, 31°C/16 hr light and 22°C/8 hr dark, 50% humidity, lighting: 250 µmol
-
Optional : Total dissolved solids (TDS) meter (Hanna Instruments, cat. no. HI 9813 - 5)
-
Perforated 6-in × 28-in clear plastic bags (Penn Jersey Paper Company, cat. no. 361001)
-
1/4-in. × 4-in. 1/4-in. × 7-in. twist ties (T and T Industries Inc, cat. no. 35-925-41-3000 and 35-025-07-3000)
-
Plastic stakes (for labeling of transgenic plants)
-
Scissors
-
Weighing boats
-
Coin envelope (Uline, cat. no. S-7798)
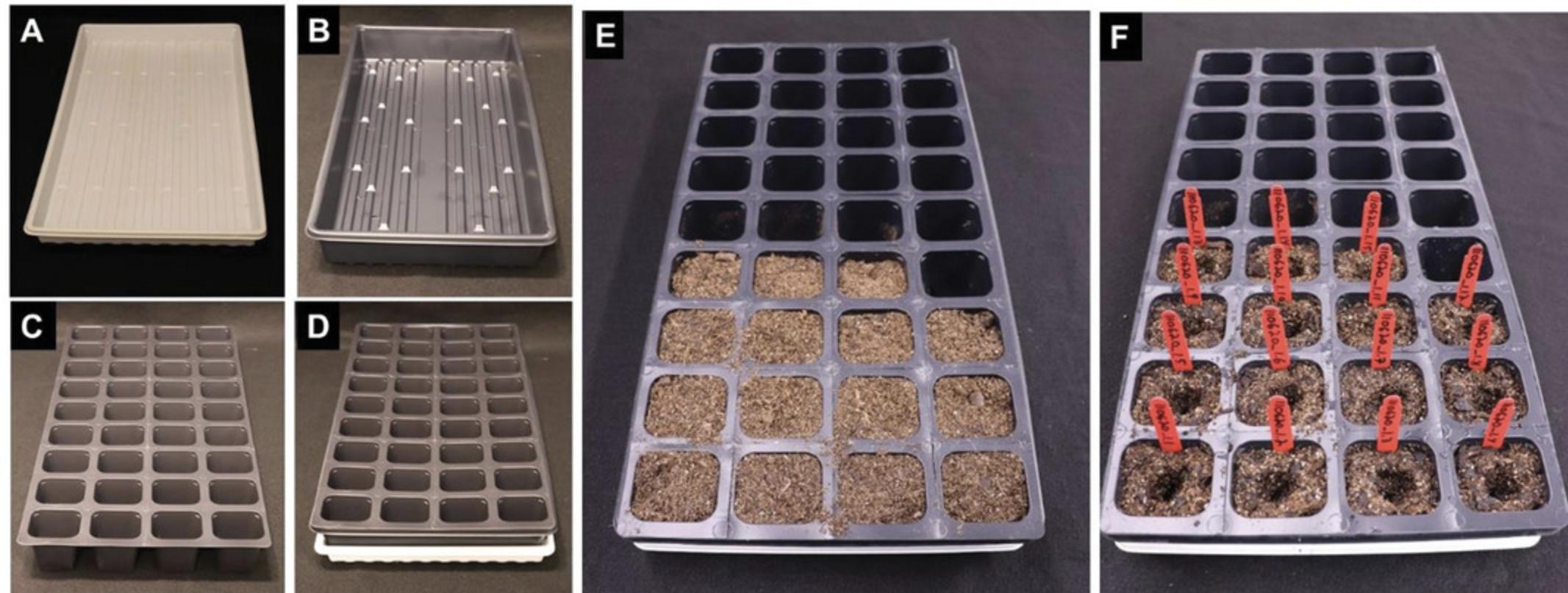
Transplantation of plantlets from tissue culture medium plate to soil
1.Prepare trays by placing 1801 deep inserts into 1020 flats with holes (Fig. 9A-D).
2.Fill 1801 cells with Jolly Gardener potting soil. Do not pack or compact soil. Use RO water to thoroughly saturate the soil (Fig. 9E).
3.Create a dimple in the soil for each cell with your finger for the easy placement of the plantlets (Fig. 9F).
4.Remove plantlets individually from the Petri dish by separating them gently from the callus at the juncture between the shoot and the roots (Fig. 10). Remove all medium that is still attached to the roots by rinsing with RO water before placing the plantlet into the designated cell. Cover the roots with soil by gently tapping the soil around the root mass and stem base. Do not compress the soil excessively, as this can lead to root and plant stunting (Fig. 10).
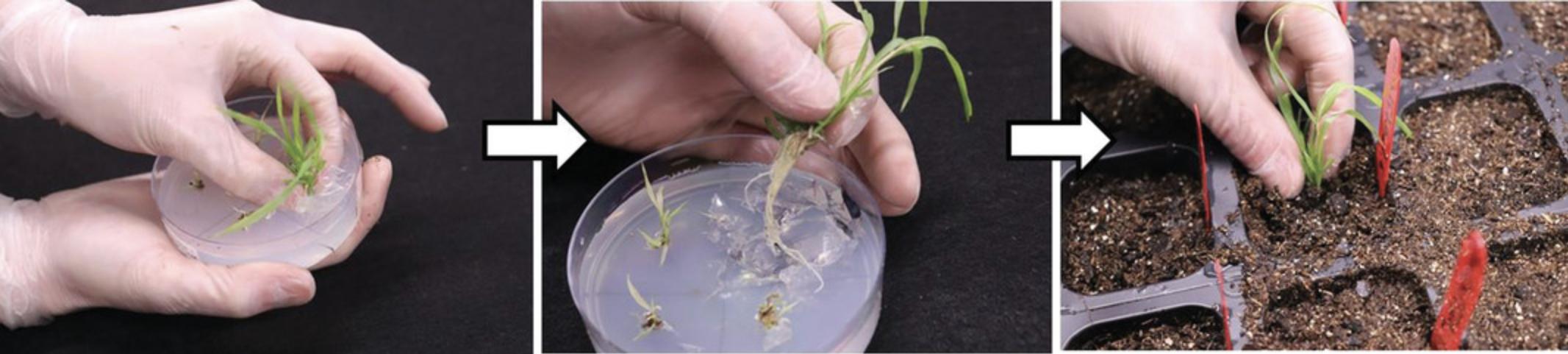
5.Repeat steps 3 and 4 until all plantlets have been transplanted to soil.
6.Place flats with plantlets in the greenhouse with a Humi-Dome and spray with RO water 2-3 times daily for approximately 3 days after which the Humi-Dome is removed.
7.Supplement bottom water as needed 1-2 times per week with Jack's 20-20-20 fertilizer mixed at a concentration of 200 ppm.
8.Bag plants with maturing inflorescence, which have approximately ¼ of their seeds dark in color, using perforated clear plastic bags by gently pulling the bag over the plant to the base where it can then be tied off using a 4-in. twist-tie. Secure the plants around the stake using 7-in. twist-ties (6 plants per stake).
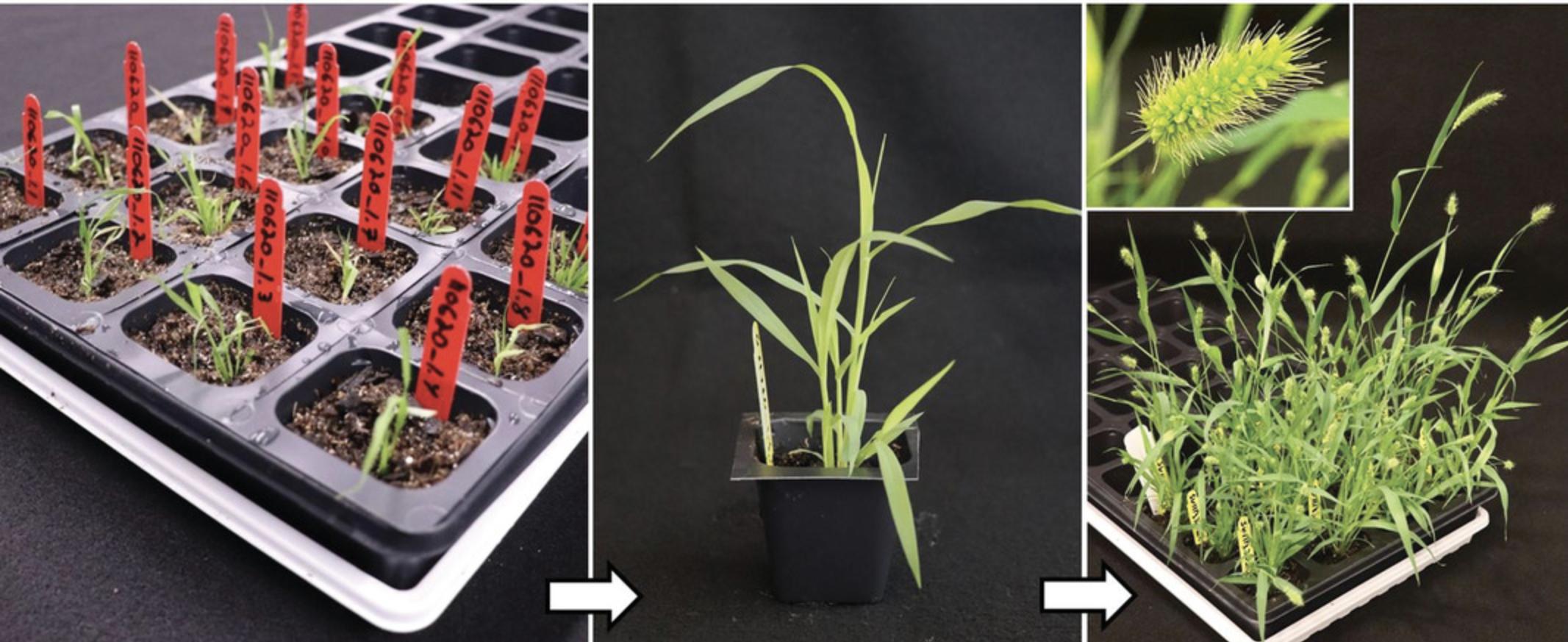
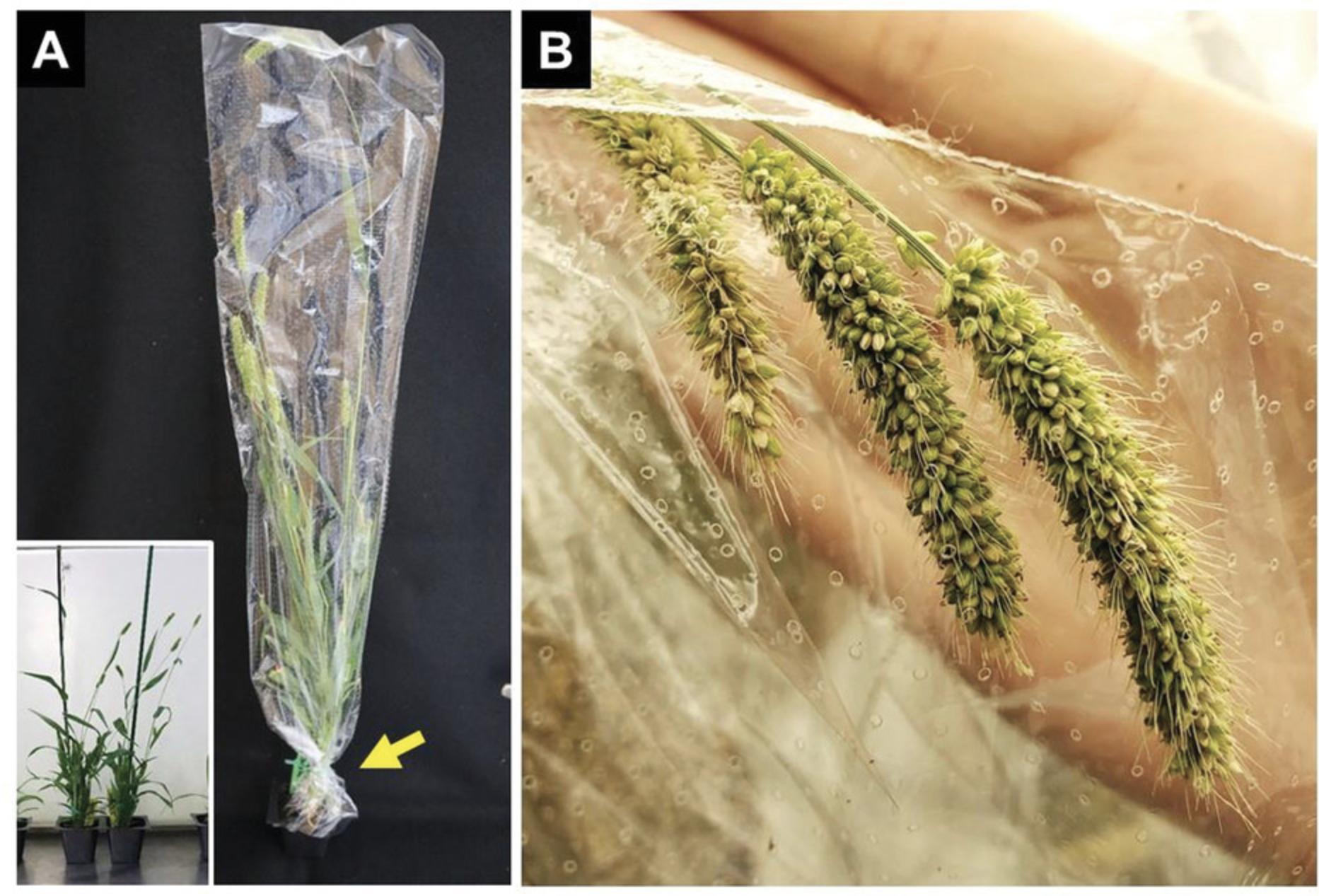
9.Continue monitoring plants for signs of fungus, pests, and condensation near the panicle and address those accordingly.
10.Once approximately 50% of the seeds on the panicle have matured and turned darker in color, stop watering to allow plants to completely dry down prior to harvest (Fig. 12).
11.Harvest seeds from a single plant at a time by cutting off the base of the plant at the soil level using a pair of scissors while keeping the 4-in. twist tie in place to prevent the loss of any seed that has already shattered from the dry inflorescence (Fig. 13A and 13B).
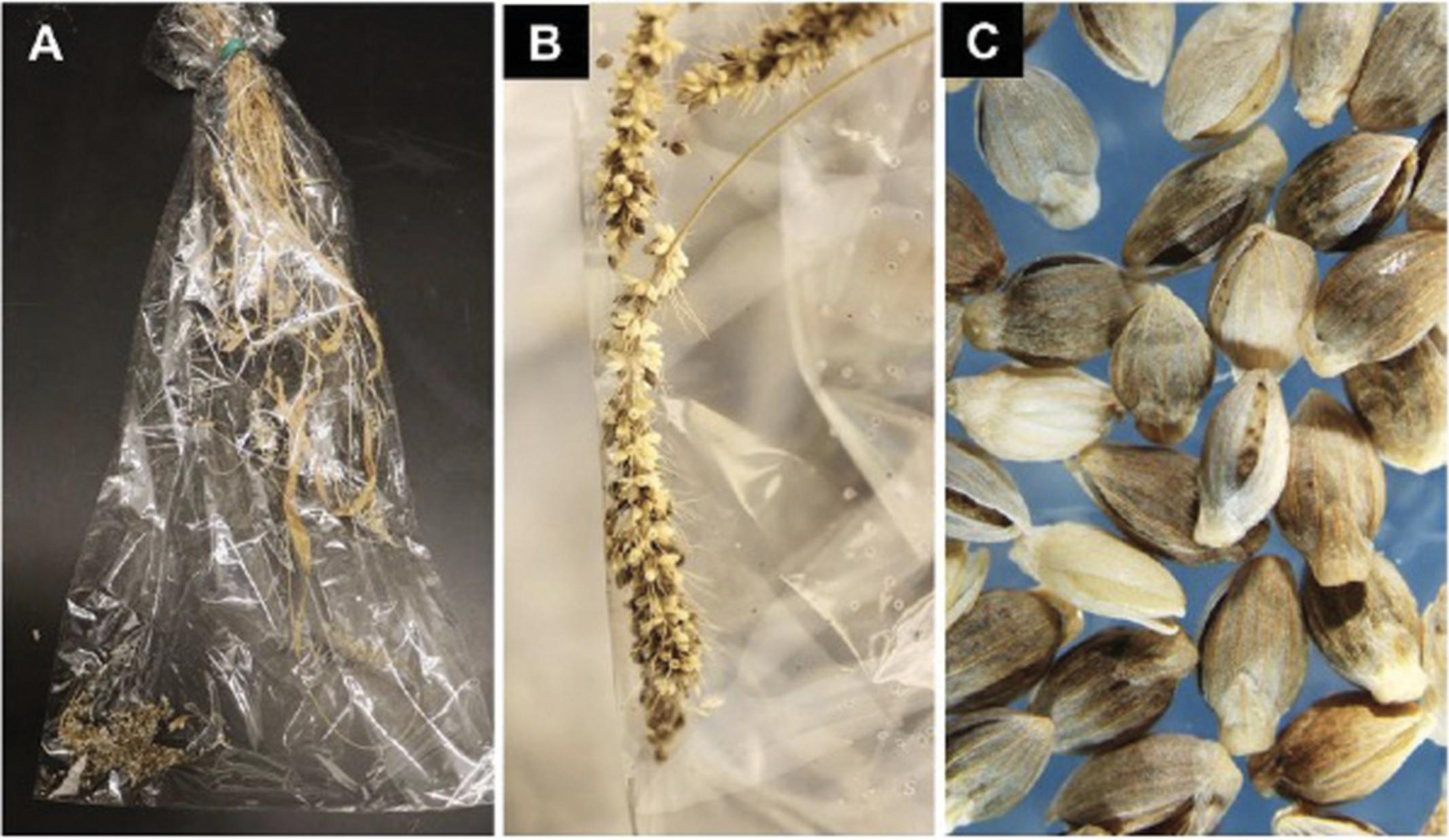
12.Invert the bag containing the plant so that all seed will fall toward the “top” of the bag, and tap the panicles to dislodge the remaining mature seed.
13.Using scissors, snip off a corner of the bag and transfer seeds into a clean weighing boat.
14.Clean the seeds from any plant debris and transfer clean seeds into small coin envelope (Fig. 13C). Label the packet and store at 4°C.
Support Protocol: PREPARATION OF Agrobacterium CULTURE FOR INFECTION
In this Support Protocol, we describe the initiation of master plate from Agrobacterium strain AGL1 harboring a binary plasmid with a gene of interest stored in a −80°C freezer.
Materials
-
Agrobacterium glycerol stock AGL1 containing pANIC10A (pANIC10A plasmid obtained from Addgene). Note that the pANIC10A binary vector is selected due to the presence of a ubiquitin-driven hygromycin phosphotransferase (hyg) cassette as a selectable marker.
-
LB medium plates with appropriate antibiotics (see recipe)
-
Sterile loop (VWR, cat. no. 30002-090)
-
Aluminum foil
-
Permanent marker
-
Gloves
-
−80°C freezer
-
28°C incubator
1.A. tumefaciens strain AGL1 containing the binary vector (pANIC10A) is stored as a glycerol stock at −80°C.
2.As needed, and at least every 3-4 weeks, a “master” plate can be re-initiated from this glycerol stock by streaking the bacteria on LB medium plates with appropriate antibiotics and incubating for 2 days at 28°C.
3.The “master” plate can be stored up to 4 weeks at 4°C and used as a source plate for initiating a fresh Agrobacteria culture, 3 days prior to transformation experiments.
REAGENTS AND SOLUTIONS
Co-culture medium
- 4.3 g MS salt (Phytotech Labs, cat. no. M524)
- 100 mg Myo-inositol (Sigma, cat. no. 17508)
- 40 g Maltose (Phytotech Labs, cat. no. M588)
- 1.2 ml Solution A (see recipe under Stock solutions)
- 1 ml Solution B (see recipe under Stock solutions)
- 2 ml Solution C (see recipe under Stock solutions)
- 0.5 ml Solution D (see recipe under Stock solutions)
- Deionized water to 1000 ml
- Add Solution K (see recipe under Stock solutions) dropwise to achieve pH 5.2
- Add 4 g Gelzan (Sigma, cat. no. G1910)
- Autoclave at 121°C/15 PSI for 20 min and cool to 65°C
- Add post autoclave additives listed below:
- 1 ml Solution E (see recipe under Stock solutions)
- 1 ml Solution F (see recipe under Stock solutions)
- Mix well and pour ∼25 ml into 100 × 15 mm Petri plates
- Once medium is solidified, store plates at 4°C for up to 1 month
CIM medium (callus inducing medium)
- 4.3 g MS salt (Phytotech Labs, cat. no. M524)
- 100 mg Myo-inositol (Sigma, cat. no. 17508)
- 40 g Maltose (Phytotech Labs, cat. no. M588)
- 1.2 ml Solution A (see recipe under Stock solutions)
- 1 ml Solution B (see recipe under Stock solutions)
- 2 ml Solution C (see recipe under Stock solutions)
- 0.5 ml Solution D (see recipe under Stock solutions)
- Deionized water to 1000 ml
- Add Solution K (see recipe under Stock solutions) dropwise to achieve pH 5.8
- Add 4 g Gelzan (Sigma, cat. no. G1910)
- Autoclave at 121°C/15 PSI for 20 min and cool to 65°C
- Add post-autoclave additives listed below:
- 1 ml Solution E (see recipe under Stock solutions)
- Mix well and pour ∼25 ml into 100 × 15 mm Petri plates
- Once medium is solidified, store plates at 4°C for up to 1 month
CIM selection-60 medium plates
- 4.3 g MS salt (Phytotech Labs, cat. no. M524)
- 100 mg Myo-inositol (Sigma, cat. no. 17508)
- 40 g Maltose (Phytotech Labs, cat. no. M588)
- 1.2 ml Solution A (see recipe under Stock solutions)
- 1 ml Solution B (see recipe under Stock solutions)
- 2 ml Solution C (see recipe under Stock solutions)
- 0.5 ml Solution D (see recipe under Stock solutions)
- Deionized water to 1000 ml
- Add Solution K (see recipe under Stock solutions) dropwise to achieve pH 5.8
- Add 4 g Gelzan (Sigma, cat. no. G1910)
- Autoclave at 121°C/15 PSI for 20 min and cool to 65°C
- Add post-autoclave additives listed below:
- 1 ml Solution E (see recipe under Stock solutions)
- 1 ml Solution G (see recipe under Stock solutions)
- 0.6 ml Solution H (see recipe under Stock solutions)
- Mix well and pour ∼25 ml into 100 × 15 mm Petri plates
- Once medium is solidified, store plates at 4°C for up to 1 month
Liquid CIM medium
- 4.3 g MS salt (Phytotech Labs, cat. no. M524)
- 100 mg Myo-inositol (Sigma, cat. no. 17508)
- 40 g Maltose (Phytotech Labs, cat. no. M588)
- 1.2 ml Solution A (see recipe under Stock solutions)
- 1 ml Solution B (see recipe under Stock solutions)
- 1 ml Solution C (see recipe under Stock solutions)
- 0.5 ml Solution D (see recipe under Stock solutions)
- 1 ml Solution E (see recipe under Stock solutions)
- Deionized water to 1000 ml
- Add Solution K (see recipe under Stock solutions) dropwise to achieve pH 5.2 if needed
- Filter sterilize using 0.2-µm filter
- Aliquot 40 ml per 50-ml conical tube and store at −20°C
- Prior to transformation initiation, add 40 L Solution F (see recipe under Stock solutions) to 40 ml of thawed medium and vortex.
LB medium
- 25 g LB broth (Teknova L9130)
- Deionized water to 1000 ml
- 12 g type-A agar (Sigma, cat. no. A4550)
- Autoclave at 121°C/15 PSI for 20 min and cool to 65°C
- Add post-autoclave additives listed below:
- 1 ml Solution I (see recipe under Stock solutions)
- 0.5 ml Solution J (see recipe under Stock solutions)
- Mix well and pour ∼ 25 ml into 100 × 15 mm plates
- Once medium is solidified, store plates at 4°C for up to 1 month
Plant regeneration medium
- 4.3 g MS salt (Phytotech Labs, cat. no. M524)
- 100 mg Myo-inositol (Sigma, cat. no. 17508)
- 20 g Sucrose (Phytotech Labs, cat. no. S391)
- 1.2 ml Solution A (see recipe)
- 1 ml Solution B (see recipe)
- 1 ml Solution C (see recipe)
- 1 ml Solution D (see recipe)
- Deionized water to 1000 ml
- Add Solution K (see recipe) dropwise to achieve pH 5.8
- 7 g Phyotoblend (Caisson Labs, cat. no. PTP01)
- Autoclave at 121°C/15 PSI for 20 min and cool to 65°C
- Add post-autoclave additives listed below:
- 1 ml Solution E (see recipe for Stock solutions)
- 1 ml Solution G (see recipe for Stock solutions)
- 0.2 ml Solution H (see recipe for Stock solutions)
- Mix well and pour ∼40 ml into 100 × 25 mm Petri plates
- Once medium is solidified, store plates at 4°C for up to 1 month
Plant rooting medium
- 2.15 g MS salt (Phytotech Labs, cat. no. M524)
- 100 mg Myo-inositol (Sigma, cat. no. 17508)
- 20 g Sucrose (Phytotech Labs, cat. no. S391)
- Deionized water to 1000 ml
- Add Solution K (see recipe for Stock solutions) dropwise to achieve pH 5.8
- Add 7 g Phyotoblend (Caisson Labs, cat. no. PTP01)
- Autoclave at 121°C/15 PSI for 20 min and cool to 65°C
- Add post-autoclave additives listed below:
- 1 ml Solution E (see recipe for Stock solutions)
- 1 ml Solution G (see recipe for Stock solutions)
- 0.2 ml Solution H (see recipe for Stock solutions)
- Mix well and pour ∼40 ml to 100 × 25 mm Petri plates
- Once medium is solidified, store plates at 4°C for up to 1 month
Stock solutions
- Solution A : Zinc sulfate heptahydrate (29 mg/ml) (Sigma, cat. no. Z0251); per 50 ml (final volume) deionized water: 1.44 g ZnSO4.7H2O
- Solution B : Copper sulfate pentahydrate (0.6 mg/ml) (Sigma C3036); per 50 ml (final volume) deionized water: 30 mg CuSO4.5H2O
- Solution C : 2, 4-D (1 mg/ml) (Phytotech Labs, cat. no. D295)
- Solution D : Kinetin (1 mg/ml) (Sigma, K0753); per 50 ml (final volume) deionized water: 50 mg Kinetin and 6 ml 1 M KOH
- Solution E : MS vitamins (1000×); per 1000 ml (final volume) deionized water:
- 2 g Glycine (Sigma, cat. no. G7403)
- 100 mg Thiamine hydrochloride (Sigma, cat. no. T1270)
- 500 mg Pyridoxine hydrochloride (Sigma, cat. no. P6280)
- 500 mg Nicotinic acid (Sigma, cat. no. N0761)
- Filter sterilize using 0.2-µm filter
- Solution F : Acetosyringone (200 mM) (Phytotech Labs, A104); per 10 ml (final volume) dimethylsulfoxide: 392 mg acetosyringone
- Filter sterilize using 0.2-µm filter
- Solution G : Timentin (150 mg/ml) (Phytotech Labs, T869); per 50 ml (final volume) deionized water: 7.5 g Timentin
- Filter sterilize using 0.2-µm filter
- Solution H : Hygromycin B (100 mg/ml) (Phytotech Labs, cat. no. H395)
- Solution I : Kanamycin (50 mg/ml) (GoldBio K-120-5); per 50 ml (final volume) deionized water: 2.5 g kanamycin
- Filter sterilize 0.2-µm filter
- Solution J : Carbenicillin (100 mg/ml) (Phytotech Labs, C346); per 50 ml (final volume) deionized water: 5 g carbenicillin
- Filter sterilize using 0.2-µm filter
- Solution K : KOH (0.1 M); per 250 ml (final volume) deionized water: 1.4 g KOH
COMMENTARY
Background Information
Since the inception of the idea to utilize S. viridis as a model system for C4 photosynthesis plants (Brutnell et al., 2010; Doust et al., 2009), significant progress has been made towards the generation and accumulation of genetic resources in Setaria (Zhu, Jiani, & Shyu, 2017). Access to these resources has broadened the scope and potential of this model system to accelerate the pace of fundamental research for unraveling important complex physiological traits—including: C4 photosynthesis, developmental biology, and stress tolerance—to speed up development of new varieties of crops with improved traits. Previous attempts to demonstrate the amenability of S. viridis to genetic manipulation have utilized S. viridis accession line A10.1 as a source of explants for transformation purposes. Even though the established method was successful in generating transgenic plants, regenerability potential under tissue culture conditions of S. viridis accession line A10.1 is inconsistent. Lower transformation efficiencies coupled with a labor-intensive methodology that requires highly skilled researchers trained in tissue culture techniques has significantly hindered a broader and successful adoption by the scientific research community. In an effort to address these challenges, the Van Eck group at Boyce Thompson Institute screened approximately 100 genotypes of S. viridis for callus transformation and regenerability potential based on the protocol established for A10.1 (see Van Eck, 2018, and references therein). This effort led to the identification of ME034V-1 genotype that had the highest transformation potential among other genotypes including A10.1.
This manuscript offers details related to Agrobacterium -mediated transformation of callus derived from mature seeds of S. viridis accession line ME034V-1 for generation of transgenic plants. This method provides robust methodology and enhanced transformation efficiency, with minimal rates of escape and higher survivability in soil.
Critical Parameters
- Use of high-quality, mature donor seed is critical to the efficient production of callus. When storing seed at 4°C, place seed packets in airtight containers to prevent the seed from absorbing moisture during refrigeration. Immature, low-quality, and damp seeds are typically more difficult to de-husk without damaging the embryos. Seed husk removal and sterilization must be carried out gently, to minimize any damage to endosperm and embryo. Callus generated from seeds that are stored for 3 months or longer tend to produce higher-quality callus and have higher transformation efficiency (Nguyen et al., 2020). If necessary, freshly harvested seeds can be treated with liquid smoke or GA3 and KNO3 to promote seed germination for callus formation (Sebastian, Wong, Tang, & Dinneny, 2014).
- It is critical to plate sterilized seeds in proper orientation on the medium surface (see Fig. 4B).
- Monitor callus growth frequently to assess the callus for any contamination. Select quality callus at the right stage of development for transformation.
- Use maltose as a carbohydrate source in all callus induction media.
- Managing Agrobacterium regrowth after co-culture and throughout the selection and regeneration processes is important to reduce loss of explants.
- Medium can be stored for 1 month at 4°C or 2 weeks at room temperature. However, for best results, we strongly recommend the use of freshly prepared media.
- Aliquot Timentin stock solutions in one-time-use quantities and store at −20°C, thaw stock at room temperature prior to using and discard away the unused solution.
- Co-culture medium should be prepared fresh on the day of or 1 day prior to transformation initiation. We have found that liquid hygromycin (Phytotech Labs, cat. no. H385) provides a better selection and improved shoot survival when compared to stock solutions prepared from a hygromycin prepared in-house using powder.
- Skills in handling of tissue culture plant materials, timely subcultures, proper storage of solutions, and tightly controlled environmental conditions for humidity, temperature, and lights are important for efficient and reliable outcome of any tissue culture based transformation methods.
Troubleshooting
- Inclusion of experimental controls helps in proper assessment of the observations and troubleshooting. Routine monitoring of the experiments in progress for the overgrowth of bacteria and contamination is essential. If Agrobacterium overgrowth is observed at any stage of culture, uncontaminated explants can be rescued and transferred to a new plate with fresh medium.
- Carefully dissect out the callus from the base of regenerated shoots before transfer to rooting medium, as it can inhibit formation of roots on transformed explants
- If experiencing higher escape rates based on molecular analysis of the events (i.e., regeneration of non-transgenic plants), alternative hygromycin concentrations ranging from 40 to 80 mg/L can be used, which may help in reduction of escape rates. Avoid overcrowding of explants on any plates including selection medium and ensure timely transfer of explants onto proper medium for effective selection and regeneration of transgenic plants.
- Failure to transfer rooted explants to soil in a timely manner will cause plantlets to flower from stress, which can significantly reduce seed yield.
Time Considerations
The following is a recommended general timeline for S. viridis (ME034V-1) transformation:
- Plate seed for callus induction 5-7 weeks prior to anticipated transformation date.
- Co-culture for 3 days in dark. Transfer explants onto CIM Selection-60 medium for 4 weeks followed by transfer to plant regeneration medium for 4 weeks for generation of shoots and 1-2 weeks in rooting medium for root formation.
- Transfer plants with roots to soil for further growth and seed collection. It takes typically take 6-8 weeks for T0 plantlets to reach maturity in soil.
Acknowledgments
We thank Dr. Thomas P. Brutnell (Danforth Plant Science Center) for kind donation of Setaria viridis (accession line ME034V-1) seeds and thoughtful suggestions during the early stages of method establishment; Dr. Joyce Van Eck (Boyce Thompson Institute) for critical comments and review of methodology presented in the manuscript; Preethi Vallal, Saranaya Devi Seemadurai, Jake Vehlewald, Jinxia Wu, and Saranya (Danforth Plant Science Center) for assistance in transformation; Dr. Hochul Yi (Danforth Plant Science Center) for comments on the manuscript; and Danforth Center Plant Growth Facility for access to growth chambers.
Author Contributions
Todd Finley: data curation, formal analysis, investigation, methodology, validation, writing review and editing, Hal Chappell: data curation, formal analysis, investigation, methodology, validation, writing original draft, Veena Veena: conceptualization, data curation, formal analysis, investigation, methodology, project administration, resources, supervision, validation, writing review and editing.
Conflict of Interest
The authors declare no conflict of interest.
Open Research
Data Availability Statement
Data sharing not applicable–no new data generated.
Literature Cited
- Anderson, C. M., Mattoon, E. M., Zhang, N., Becker, E., McHargue, W., Yang, J., … Zhang, R. (2021). High light and high temperature reduce photosynthesis via different mechanisms in the C4 model Setaria viridis. Nature Biotechnology , 30, 555–561. doi: 10.1038/nbt.2196.
- Brutnell, T. P., Wang, L., Swartwood, K., Goldschmidt, A., Jackson, D., Zhu, X. G., … Van Eck, J. (2010). Setaria viridis : A model for C4 photosynthesis. Plant Cell , 22, 2537–2544. doi: 10.1105/tpc.110.075309.
- Ermawar, R. A., Collins, H. M., Byrt, C. S., Henderson, M., O'Donovan, L. A., Shirley, N. J., … Burton, R. A. (2015). Genetics and physiology of cell wall polysaccharides in the model C4 grass, Setaria viridis spp. BMC Plant Biology , 15, 236. doi: 10.1186/s12870-015-0624-0.
- Ferreira, S. S., Simoes, M. S., Carvalho, G. G., De Lima, L. G. A., Svartman, R. M. A., & Cesarino, I. (2019). The lignin toolbox of the model grass Setaria viridis. Plant Molecular Biology , 101, 235–255. doi: 10.1007/s11103-019-00897-9.
- Huang, P., Shyu, C., Coelho, C. P., Cao, Y., & Brutnell, T. P. (2016). Setaria viridis as a model system to advance millet genetics and genomics. Frontiers in Plant Science , 7, 1781. doi: 10.3389/fpls.2016.01781.
- Kellogg, E. A. (2013). C4 photosynthesis. Current Biology , 23, 594–599. doi: 10.1016/j.cub.2013.04.066.
- Kikuchi, K., Shyu, C., & Brutnell, T. P. (2017). Transposon tagging in Setaria viridis. In A. Doust & X. Diao (Eds.), Genetics and genomics of Setaria (Vol. 19, pp. 323–342). Berlin: Springer.
- Li, P., & Brutnell, T. P. (2011). Setaria viridis and Setaria italica , model genetic systems for the panicoid grasses. Journal of Experimental Botany , 62, 3031–3037. doi: 10.1093/jxb/err096.
- Mann, D. G., Lafayette, P. R., Abercrombie, L. L., King, Z. R., Mazarei, M., Halter, M. C., … Neal Stewart, C., Jr. (2012). Gateway-compatible vectors for high-throughput gene functional analysis in switchgrass (Panicum virgatum L.) and other monocot species. Plant Biotechnology Journal , 10, 226–236. doi: 10.1111/j.1467-7652.2011.00658.x.
- Martin, A. P., Palmer, W. M., Brown, C., Abel, C., Lunn, J. E., Furbank, R. T., & Grof, C. P. (2016). A developing Setaria viridis internode: An experimental system for the study of biomass generation in a C4 model species. Biotechnology for Biofuels , 9, 45. doi: 10.1186/s13068-016-0457-6.
- Martins, P. K., Nakayama, T. J., Ribeiro, A. P., Cunha, B., Nepomuceno, A. L., Harmon, F. G., … Molinari, H. (2015). Setaria viridis floral-dip: A simple and rapid Agrobacterium -mediated transformation method. Biotechnology Reports , 6, 61–63. doi: 10.1016/j.btre.2015.02.006.
- Martins, P. K., Ribeiro, A. P., Cunha, B., Kobayashi, A. K., & Molinari, H. B. C. (2015). A simple and highly efficient Agrobacterium -mediated transformation protocol for Setaria viridis. Biotechnology Reports , 6, 41–44. doi: 10.1016/j.btre.2015.02.002.
- Muthamilarasan, M., Khan, Y., Jaishankar, J., Shweta, S., Lata, C., & Prasad, M. (2015). Integrative analysis and expression profiling of secondary cell wall genes in C4 biofuel model Setaria italica reveals targets for lignocellulose bioengineering. Frontiers in Plant Science , 6, 965. doi: 10.3389/fpls.2015.00965.
- Nguyen, D. Q., Van Eck, J., Eamens, A. L., & Grof, C. P. L. (2020). Robust and reproducible Agrobacterium -mediated transformation system of the C4 genetic model species Setaria viridis. Frontiers in Plant Science , 11, 281. doi: 10.3389/fpls.2020.00281.
- Pant, S. R., Irigoyen, S., Doust, A. N., Scholthof, K. B. G., & Mandadi, K. K. (2016). Setaria : A food crop and translational research model for C4 grasses. Frontiers in Plant Science , 7, 1885. doi: 10.3389/fpls.2016.01885.
- Plaza-Wüthrich, S., & Tadele, Z. (2012). Millet improvement through regeneration and transformation. Biotechnology and Molecular Biology Reviews , 7, 48–61. doi: 10.5897/BMBR12.001.
- Sebastian, J., Wong, M. K., Tang, E., & Dinneny, J. R. (2014). Methods to promote germination of dormant Setaria viridis seeds. PLoS One , 9, e0095109. doi: 10.1371/journal.pone.0095109.
- Van Eck, J. (2018). The status of Setaria viridis transformation: Agrobacterium -mediated to floral dip. Frontiers in Plant Science , 9, 652. doi: 10.3389/fpls.2018.00652.
- Van Eck, J., & Swartwood, K. (2015). Setaria viridis. Methods in Molecular Biology , 1223, 57–67. doi: 10.1007/978-1-4939-1695-5_5.
- Van Eck, J., Swartwood, K., Pidgeon, K., & Maxson-Stein, K. (2017). Agrobacterium tumefaciens -mediated transformation of Setaria viridis. In A. Doust & X. Diao (Eds.), Genetics and genomics of Setaria (pp. 343–356). Cham: Springer. doi: 10.1007/978-3-319-45105-3_20.
- Wang, M. Z., Pan, Y. L., Li, C., Liu, C., Zhao, Q., Ao, G. M., & Yu, J. J. (2011). Culturing of immature inflorescences and Agrobacterium -mediated transformation of foxtail millet (Setaria italica). African Journal of Biotechnology , 10, 16466–16479. doi: 10.5897/AJB10.2330.
- Zhu, C., Jiani, Y., & Shyu, C. (2017). Setaria comes of age: meeting report on the second international Setaria genetics conference. Frontiers in Plant Science , 8, 1562. doi: 10.3389/fpls.2017.01562.
Citing Literature
Number of times cited according to CrossRef: 5
- Yi Cao, Yuning Lan, Hongjuan Huang, Shouhui Wei, Xiangju Li, Ying Sun, Ruolin Wang, Zhaofeng Huang, Molecular Characterization of Resistance to Nicosulfuron in Setaria viridis, International Journal of Molecular Sciences, 10.3390/ijms24087105, 24 , 8, (7105), (2023).
- Thomas E. Hughes, Olga Sedelnikova, Mimi Thomas, Jane A. Langdale, Mutations in NAKED-ENDOSPERM IDD genes reveal functional interactions with SCARECROW during leaf patterning in C4 grasses, PLOS Genetics, 10.1371/journal.pgen.1010715, 19 , 4, (e1010715), (2023).
- Leydson Gabriel Alves de Lima, Sávio Siqueira Ferreira, Marcella Siqueira Simões, Lucas Xavier da Cunha, Alisdair R. Fernie, Igor Cesarino, Comprehensive expression analyses of the ABCG subfamily reveal SvABCG17 as a potential transporter of lignin monomers in the model C4 grass Setaria viridis, Journal of Plant Physiology, 10.1016/j.jplph.2022.153900, 280 , (153900), (2023).
- Monoj Sutradhar, Nirmal Mandal, Reasons and riddance of Agrobacterium tumefaciens overgrowth in plant transformation, Transgenic Research, 10.1007/s11248-023-00338-w, 32 , 1-2, (33-52), (2023).
- Hangqin Liu, Xiaojian Fang, Leina Zhou, Yan Li, Can Zhu, Jiacheng Liu, Yang Song, Xing Jian, Min Xu, Li Dong, Zhongwei Lin, Transposon Insertion Drove the Loss of Natural Seed Shattering during Foxtail Millet Domestication, Molecular Biology and Evolution, 10.1093/molbev/msac078, 39 , 6, (2022).