AAV Production Everywhere: A Simple, Fast, and Reliable Protocol for In-house AAV Vector Production Based on Chloroform Extraction
Matilde Negrini, Matilde Negrini, Gang Wang, Gang Wang, Andreas Heuer, Andreas Heuer, Tomas Björklund, Tomas Björklund, Marcus Davidsson, Marcus Davidsson
AAV vector
adeno associated virus
chloroform extraction
gene expression
gene therapy
PEI transfection
vector production
Abstract
Recombinant adeno-associated virus (rAAV) is a mammalian virus that has been altered to be used as a gene delivery vehicle. Several changes to the viral genome have made them replication deficient so that this aspect of the viral infection cycle is under full control of the experimenter, while maintaining gene expression machinery. Over the last decades, rAAVs have become the gold standard for studying in vivo gene function and are especially favorable for gene transfer in the central nervous system. AAVs have been proven safe and provide stable gene expression over a long period of time. They are extensively used in preclinical experiments and show great potential for clinical applications. However, the use of AAVs in preclinical settings are often held back due to availability. Waiting lines are long at commercial production facilities, and in-lab production is hindered due to lack of specific laboratory equipment needed. Here we present a novel production method that can be carried out in any molecular biology laboratory using standard laboratory equipment. We provide a simple, fast, and streamlined protocol for production that can result in titers comparable with the more time-consuming iodixanol gradient ultracentrifugation method. The yield using this protocol is high enough for any type of study where AAV is the vector of choice. © 2020 The Authors.
Basic Protocol:
Adeno associated viruses (AAVs) are small, nonpathogenic DNA viruses with a 4.7-kb single-stranded genome belonging to the Dependoviruses. The wild-type AAV genome consists of open reading frames for rep, cap, and aap, flanked by two inverted terminal repeats (ITRs). The rep gene codes for four nonstructural proteins (Rep78, Rep68, Rep52, and Rep40) involved in ITR-dependent viral replication, transcription, site-specific integration, and AAV genome encapsidation (Kotterman, Chalberg, & Schaffer, 2015). The cap gene codes for three capsid proteins (VP1, VP2, and VP3) that are assembled in a 1:1:10 molar ratio (Govindasamy et al., 2013), and aap is involved in the assembly process. AAVs are not linked to any human diseases. They can infect both dividing and nondividing cells, and the viral genome persists episomally, with minimal integration events into the host chromosome (Kotterman et al., 2015; Wu, Asokan, & Samulski, 2006).
In order to use AAVs as gene expression vehicles, they have been rendered replication deficient by several modifications. Recombinant AAVs (rAAVs) are generated by replacing the rep and cap genes with a transgenic cassette. While the flanking ITR sequences that contain cis-acting elements required for vector genome packaging into AAV capsids remain intact, the excised rep and cap genes are provided in trans, either together with an AAV helper plasmid or delivered as separate entities. To date, there are 12 different serotypes described (AAV1 through AAV12; Vance, Mitchell, & Samulski, 2015). Many serotypes use different receptors for infection. For example, AAV2 primarily uses heparin sulfate proteoglycan, AAV5 binds to N-linked sialic acid, and AAV9 binds to N-linked galactose. The different serotypes also have diverse transduction abilities. AAV1, 2, 3, 5, 6, 7, 8, and 9 exhibit high affinity for central nervous system tissue, whereas AAV4 efficiently transduces the eye, and AAV1, 6, and 9 can also infect heart and skeletal muscle (Vance et al., 2015). Even though AAV can efficiently transduce a wide array of tissues and cell types, huge efforts are being put in to generating new and improved viral capsids (Davidsson et al., 2019; Deverman et al., 2016; Ojala et al., 2018). Another way to improve transduction efficiency regardless of serotype used was the development of self-complementary AAVs (scAAV; McCarty, Monahan, & Samulski, 2001). scAAVs are double stranded (contrary to wild-type AAVs that carry a single-stranded genome) and can therefore circumvent the rate-limiting step of DNA replication upon infection. Studies have shown a 5- to 140-fold increase in infectivity using scAAVs compared with ssAAVs. However, increase in transduction of the double-stranded scAAVs is gained at the expense of a halved loading capacity of 2.4 kb (McCarty et al., 2001). There are numerous ways to produce and purify AAVs. Production is often carried out in HEK293T cells (Salganik, Hirsch, & Samulski, 2015), but it can also be done in the baculovirus expression system using an insect cell line such as Sf9 (Kohlbrenner et al., 2005; Ni, Zhou, McCarty, Zolotukhin, & Muzyczka, 1994). The latter has been especially interesting for clinical applications as the use of mammalian products for good manufacturing practice application always bears the risk of introducing xenogeneic disease.
Transfection of viral plasmids into producer cells are most often done using calcium chloride (resulting in a calcium phosphate precipitate; Graham & van der Eb, 1973) by using polyethylenimine (PEI; Boussif et al., 1995) or lipofection (Felgner et al., 1987). For preclinical applications, AAVs have traditionally been purified using cesium chloride (Bachrach & Friedmann, 1971), iodixanol gradients (Strobel, Miller, Rist, & Lamla, 2015; Zolotukhin et al., 1999), or, as described in this protocol, chloroform precipitation (Davidsson et al., 2019). We have used the protocol described here in a previous study, expressing transgenes both in vitro and in vivo (Davidsson et al., 2019). The main advantages of the current protocol over existing ones are the timescale for purification of the AAV vector (5 to 6 hr vs 15 to 16 hr) and the lack of specialized equipment that is needed for the iodixanol precipitation protocol (e.g., ultracentrifuge, special pumps).
Here we present a detailed protocol for the fast and efficient production of AAV vectors (Fig. 1), with a titer yield comparable with that of the gold-standard iodixanol gradient–based protocols. This protocol has a workflow that can be performed in any molecular biology laboratory using standard laboratory equipment. The outlined protocol contains detailed information for cell culture, transfection, cell harvesting, and AAV purification.
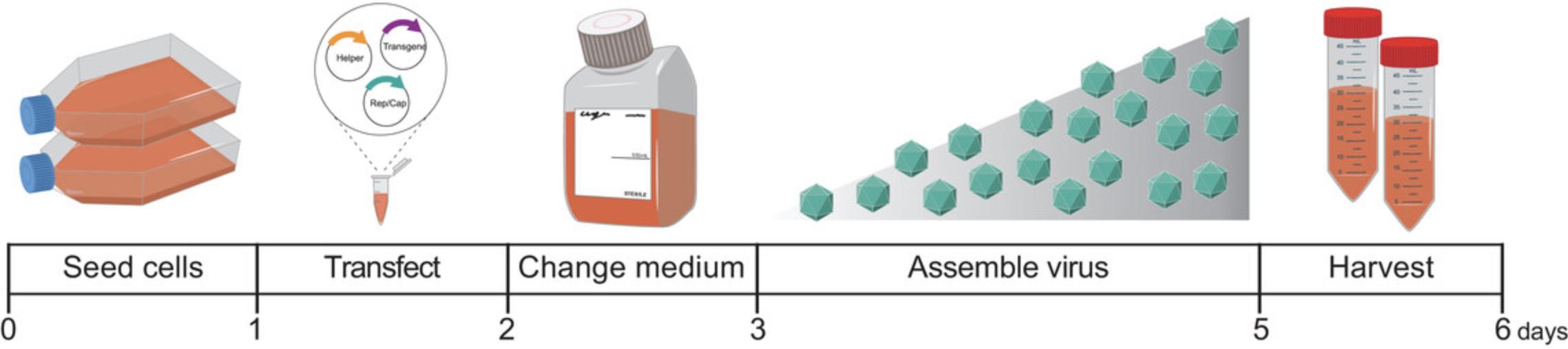
Before starting to produce AAV vectors, the necessary safety and regulatory documents and standard operating procedures have to be established according to national and local guidelines.
This protocol describes the complete process of AAV purification from triple-transfected HEK293T cells. All reagents and consumables used during AAV production should be kept sterile, and sterile technique should be applied throughout the production protocol.
The experimenter can freely decide on the scale of production. Our recommendation is that two 175-cm2 flasks are transfected per virus batch.
Materials
-
HEK293T cells (e.g., ATCC #CRL-11268)
-
Dulbecco's modified Eagle medium (DMEM; e.g., Thermo Fisher Scientific, cat. no. 61965-026)
-
Fetal bovine serum (FBS; e.g., Biological Industries, cat. no. 04-007-19)
-
10,000 U/ml penicillin-streptomycin (P/S; e.g., Thermo Fisher Scientific, cat. no. 15140-122)
-
Dulbecco's phosphate-buffered saline (DPBS) with calcium and magnesium (e.g., Sigma-Aldrich, cat. no. D8662)
-
2.5% (w/v) trypsin (e.g., Thermo Fisher Scientific, cat. no. 15090-046)
-
pHelper (HGTI) plasmid
-
Rep/cap plasmid
-
Transgene of interest
-
1 μg/μl PEI (see recipe)
-
OptiPRO (e.g., Thermo Fisher Scientific, cat. no. 12309-019)
-
Chloroform (e.g., Sigma-Aldrich, cat. no. C2432)
-
5 M NaCl (e.g., Sigma-Aldrich, cat. no. S3014)
-
Polyethylene glycol (PEG-8000; see recipe)
-
HEPES buffer (see recipe)
-
1 M MgCl2 (e.g., Sigma-Aldrich, cat. no. M2670)
-
DNase I (e.g., New England Biolabs, cat. no. M0303S)
-
10 μg/μl RNase A (e.g., Thermo Fisher Scientific, cat. no. EN0531)
-
175-cm2 cell culture flask (e.g., Thermo Fisher Scientific)
-
37°C cell culture incubator
-
50-ml conical tube
-
Centrifuge
-
Hemocytometer
-
Vortex mixer (e.g., Heidolph, cat. no. 5451000000)
-
Cell scraper (e.g., Fisher Scientific, cat. no. 8100241)
-
1.5-ml microcentrifuge tube
-
0.5-ml Amicon Ultra Centrifugal Filter, 100 kDa (e.g., Millipore, cat. no. UFC510024)
-
Microcentrifuge
-
Glass vial or sigma-coated plastic vial
Cell culture
1.Grow HEK293T cells in a 175-cm2 flask using DMEM supplemented with 10% (v/v) FBS and 1% (v/v) P/S. Split cells when they reach 75% to 80% confluency (steps 2 to 11; Fig. 2), normally two to three times per week.
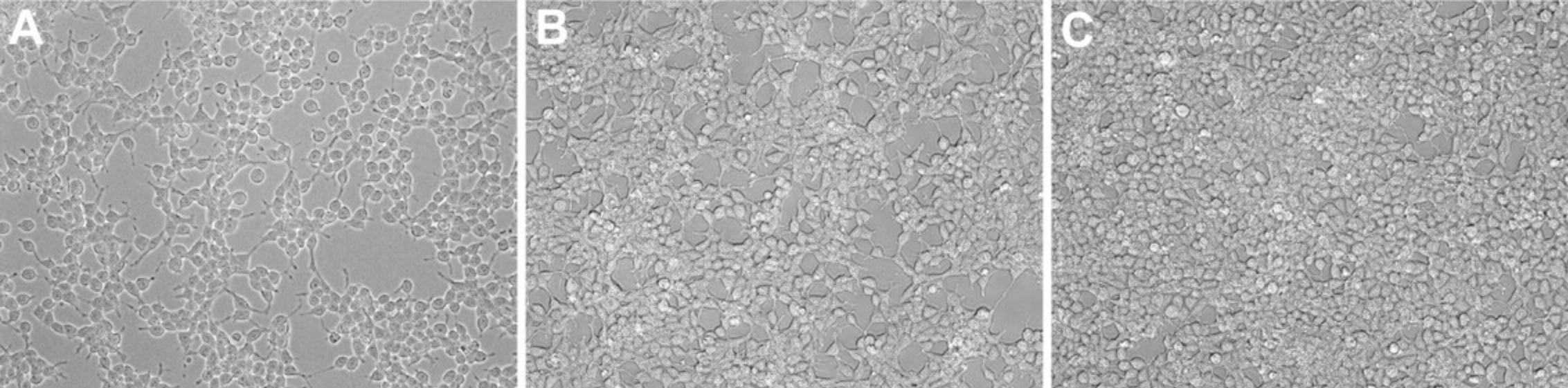
Splitting cells
2.Aspirate medium from flask without touching the cells.
3.Carefully add 10 ml DPBS, and swish over cells to wash off the medium.
4.Aspirate DPBS.
5.Add 3 ml of 2.5% (w/v) trypsin to the flask, and swirl flask to distribute evenly. Then let sit for 3 min.
6.Gently tap flask to detach cells that remain attached to the bottom of the flask.
7.Add 10 ml DMEM supplemented with 10% (v/v) FBS and 1% (v/v) P/S to inactivate trypsin.
8.Rinse multiple times by pipetting up and down.
9.Collect cells and medium in a 50-ml conical tube.
10.Centrifuge cells 5 min at 500 × g , room temperature.
11.Resuspend cells in 5 ml DMEM supplemented with 10% (v/v) FBS and 1% (v/v) P/S, and quantify the number of cells using a hemocytometer.
Seeding cells
12.Seed 11 × 106 HEK293T cells in a 175-cm2 flask using 27 ml DMEM supplemented with 10% (v/v) FBS and 1% (v/v) P/S.
Transfection
13.For the three-plasmid transfection system, prepare a 1:1:1 molar ratio for all plasmids (calculations per 175-cm2 flask):
- 17.7 μg pHelper (HGT1) (17,800 bp)
- 7.9 μg rep/cap (8000 bp)
- 5.9 μg transgene (6000 bp)
- 94.5 μl of 1 μg/μl PEI (3 μg PEI per 1 μg DNA)
- Bring to 3 ml with DMEM supplemented with 1% (v/v) P/S.
14.Warm DNA, PEI, and DMEM supplemented with 1% (v/v) P/S to room temperature before mixing.
15.Add DNA to prewarmed DMEM supplemented with 1% (v/v) P/S.
16.Add 94.5 µl PEI, and mix by vortexing for 10 s.
17.Incubate at room temperature for 15 min.
18.Add the total volume of transfection mix to the cell culture flask, and swirl flask gently in a figure 8 motion three times to mix transfection mix with the medium.
19.Change medium 24 hr post transfection: Keep 3 ml old medium, and add 27 ml OptiPRO supplemented with 1% (v/v) P/S.
20.Harvest cells and purify AAVs 72 hr after the final medium change (step 19).
AAV harvest and purification
21.Use cell scraper to scrape cells from the bottom of the flask.
22.Transfer cells and medium from one flask to a 50-ml conical tube using a 25-ml serological pipette. If needed, rinse flask with extra OptiPRO to make a total volume of 30 ml.
23.Add 3 ml chloroform to each tube.
24.Vortex for 5 min.
25.Add 7.6 ml of 5 M NaCl to each tube.
26.Vortex each tube for 10 s.
27.Place tubes into precooled centrifuge, and centrifuge 5 min at 3000 × g , 4°C.
28.Using a 25-ml serological pipette, carefully collect the aqueous phase into a new 50-ml conical tube (Fig. 3A). Avoid including any chloroform when transferring supernatant.
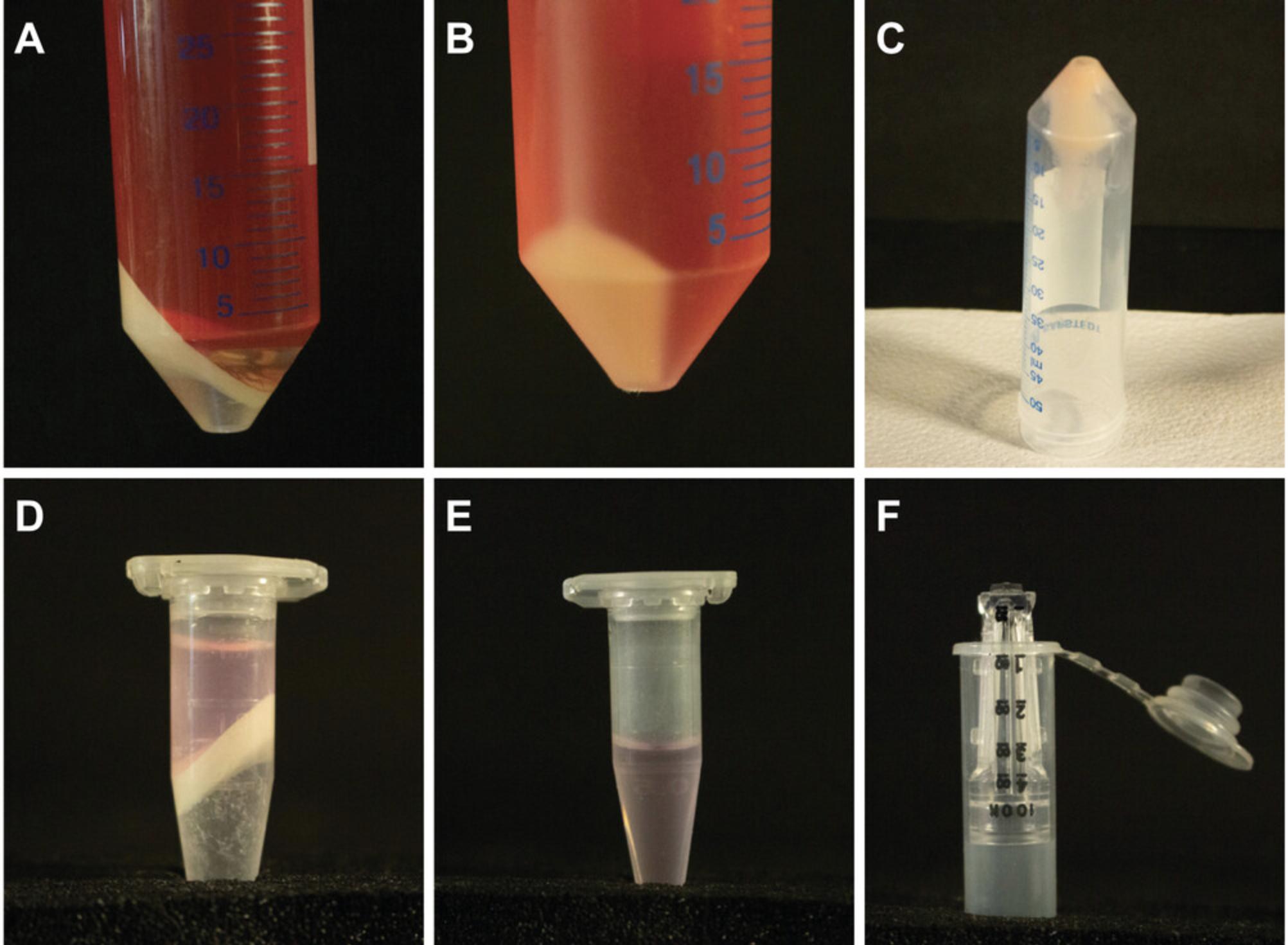
29.Add 9.4 ml of 50% (v/v) PEG 8000 to each tube.
30.Vortex each tube for 10 s.
31.Incubate 1 hr on ice.
32.Centrifuge 30 min at 3000 × g , 4°C (Fig. 3B).
33.Gently pour off supernatant, and place tubes upside down on paper towels for 10 min to dry. Dry residual liquid at the edge of the tube with paper towel if necessary (Fig. 3C).
34.Add 1.4 ml HEPES buffer to tubes, and gently resuspend cell pellet by pipetting using a 1000-ml pipette. Make sure that the pellet is completely resuspended before commencing with the next step.
35.Vortex tubes for 5 min.
36.To each tube add:
- 3.5 µl of 1 M MgCl2
- 14 µl DNase I
- 1.4 µl of 10 μg/μl RNase A.
37.Incubate tubes at 37°C for 20 min.
38.Split resuspended solution of each tube into two 1.5-ml microcentrifuge tubes with ∼750 µl each.
39.Add chloroform at 1:1 (v:v) proportion to each tube.
40.Vortex tubes for 10 s.
41.Centrifuge tubes 5 min at 3000 × g , 4°C.
42.Carefully collect aqueous phase using a 1000-ml pipette (Fig. 3D).
43.Repeat steps 38 to 41 two to three times (until aqueous phase is clear; Fig. 3E).
44.Leave tubes with the lid open in a biosafety cabinet, and evaporate chloroform for 30 min.
45.Add up to 400 μl sample to an Amicon Ultra Centrifugal Filter.
46.Centrifuge tubes 5 min at 14,000 × g , room temperature. Discard flow-through.
47.Repeat until all of the sample has been through the Amicon Ultra Centrifugal Filter.
48.Add 400 µl DPBS, and pipette carefully three to four times to mix both solutions.
49.Centrifuge tubes 5 min at 14,000 × g , room temperature. Discard eluate.
50.Repeat steps 48 and 49 three times.
51.Add 400 µl DPBS, and pipette carefully three to four times to mix both solutions.
52.Centrifuge tubes 7 to 8 minutes at 14,000 × g , room temperature.
53.Place column upside down in a new tube (provided with Amicon Ultra Centrifugal Filter; Fig. 3F).
54.Centrifuge 2 min at 1000 × g , room temperature.
55.Adjust total volume to 25 µl by adding DPBS.
56.Immediately place in glass vial (or sigma-coated plastic tube), and ensure the glass vial is properly sealed. To avoid evaporation, seal vials or tubes with Parafilm.
57.Store AAV at 4°C 6 months.
Titration of AAV
Titration of AAV can be carried out in multiple ways and lies outside the scope of this protocol. The most commonly used methods are quantitative PCR (qPCR; Aurnhammer et al., 2012) or droplet digital PCR (ddPCR; Lock, Alvira, Chen, & Wilson, 2014). The main difference between these methods is that qPCR uses a standard curve based on plasmid dilutions to assess viral titer, whereas ddPCR gives an absolute count of viral particles. Comparisons have been made between the two methods, and both methods give satisfactory results (Sanmiguel, Gao, & Vandenberghe, 2019).
REAGENTS AND SOLUTIONS
HEPES buffer, 50 mM (pH 8.0)
- 238.3 g HEPES (free acid; e.g., Sigma-Aldrich, cat. no. H3375)
- 800 ml Milli-Q water
- Adjust pH to 8.0 with 10 N NaOH
- Bring volume to 1000 ml with Milli-Q water
- Store at room temperature for up to 1 year
The solution prepared is a 1 M HEPES stock solution. Before use, dilute 20× in Milli-Q water to prepare a 50 mM solution.
PEG 8000, 50%
- 250 g PEG 8000 (e.g., Sigma-Aldrich, cat. no. 89510-250G-F)
- 250 ml Milli-Q water
- Heat to 80°C to dissolve PEG 8000
- Allow solution to cool
- Store at room temperature for up to 2 months
PEI, 1 µg/µl
- 1.Dissolve PEI (e.g., Polysciences, cat. no. 23966-1) by swirling using sterile water heated to 80°C and using ∼90% volume required to reach a final concentration of 1 g/L (1 µg/µl).
- 2.Cool to room temperature.
- 3.Adjust pH to 7.0 with HCl.
- 4.Add sterile water to a final concentration of 1 µg/µl.
- 5.Filter using a 0.22-µm membrane filter, and divide into aliquots. Store at −20°C for up to 1 year.
- 6.Thaw in a 37°C water bath until precipitates have fully dissolved.
- 7.Store thawed aliquot at 4°C for up to 1 month.
Redissolve precipitates by incubating at 37°C before use.
COMMENTARY
Background Information
Over the past few decades, several methods of AAV production have been developed, validated, and refined. Traditionally, AAVs have been produced in mammalian cells (e.g., HEK293T cells), but in recent years production in insect cells (e.g., SF9 cells) has become more popular. Transient transfection of mammalian cells will, however, offer a more flexible production system compared with constitutively expressing insect cells. Expressing DNA via transient transfections has also evolved during the years. Popular methods like calcium phosphate precipitation and lipofection have more and more been replaced by cheaper and more reproducible methods like PEI transfection. PEI transfection offers several advantages over already mentioned methods. PEI is well tolerated by HEK293 cells, and transfection efficacy is high. Further, it is an inexpensive method, and it gives reproducible results. Purification of AAVs has evolved from time-consuming cesium chloride gradient centrifugation (Gray et al., 2010) to iodixanol gradients with low toxicity (Zolotukhin et al., 1999). Several different chromatography methods are also available for AAV purification. These can be ion exchange or affinity based (Auricchio, Hildinger, O'Connor, Gao, & Wilson, 2001; O'Riordan, Lachapelle, Vincent, & Wadsworth, 2000). The method of choice is quite often based on personal preference and knowledge available in the laboratory. Here, we have presented a novel, easy-to-perform, and fast method to produce AAVs at a very low cost without using specific and expensive equipment and without sacrificing quality and titers. AAVs produced using this protocol are highly infective both in vivo and in vitro (Fig. 4). In addition, the method has successfully been used to produce a wide variety of AAV serotypes (Davidsson et al., 2019).
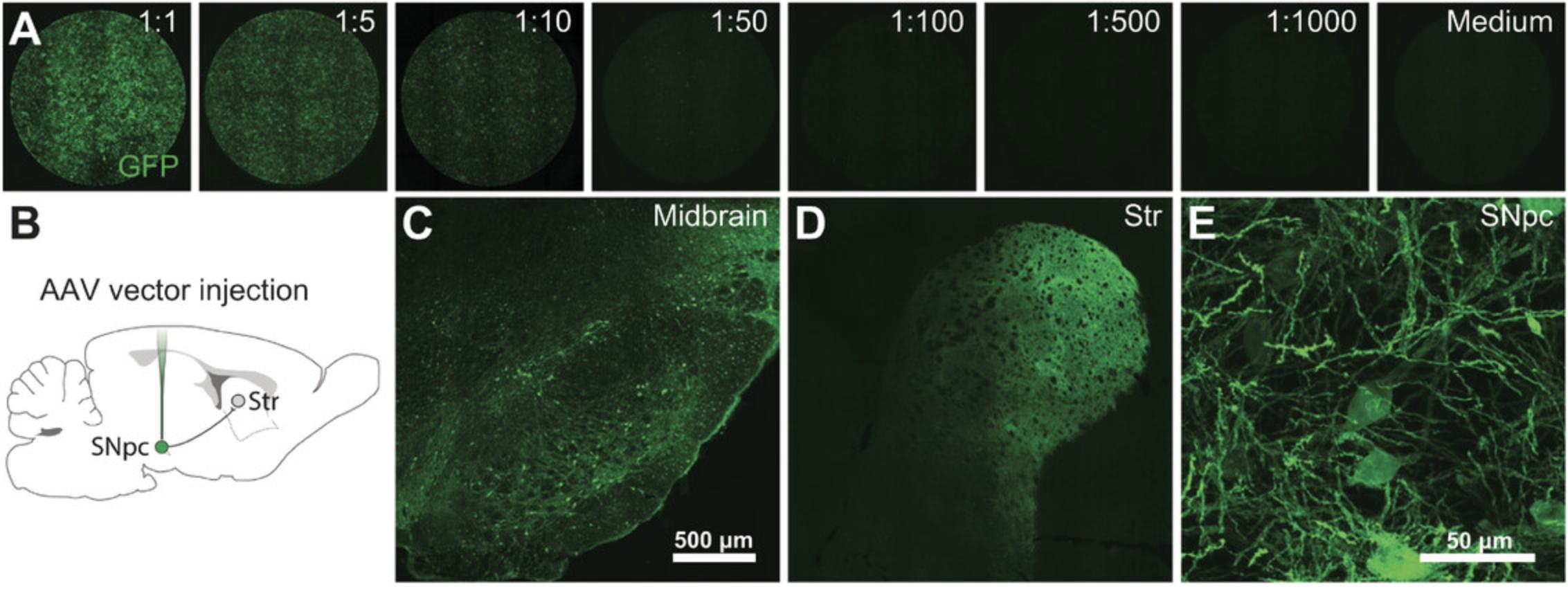
Critical Parameters
The final AAV titer is mainly dependent on three parameters: transfection efficiency, cell state, and, purification. Maximum transfection efficacy is achieved by passaging the cells regularly and maintaining them in exponential growth phase. Most important is to never let the cells reach 90% to 100% confluency. If this should happen, it is advised to thaw a new vial of cells and start over. Further, it is also recommended to keep the passage number as low as possible. Monitoring titers and passage numbers can be a good way to set up a maximum passage number for the cells being used for production. Pilot transfection using a new batch of PEI is also recommended. Using an AAV plasmid with a fluorescent protein is a quick and easy way to validate transfection efficacy. Gene expression can be assessed by fluorescence microscopy. Transfection efficacy should preferably reach 75% of cells 24 hr post transfection.
Troubleshooting
Low yield in AAV production can be caused by low transfection efficacy (inefficient PEI transfection), nonoptimal cells (too many passages), or loss of ITR integrity. Transfection efficacy should always be monitored (i.e., with fluorescence) before continuing with AAV purification if possible. ITR integrity should always be assessed after each new bacterial preparation by restriction enzyme digestion using SmaI. ITR recombination is a major contributing factor of low yield in AAV production.
Understanding Results
With some experience and general molecular biology expertise, it is possible to obtain 25 to 50 µl AAV with a titer of 1012 to 1013 genome copies per ml from two 175-cm2 flasks. Titer yield varies significantly between AAV serotypes, and the purified virus should be validated in the appropriate setting for each study (Fig. 4).
Time Considerations
Splitting cells for productions requires ∼1 hr. Transfection requires ∼30 min. Medium changes take ∼30 min, and harvesting and purification require ∼5 to 6 hr.
Acknowledgments
M.D. is supported by the Physiographic Society in Lund and the Segerfalk Foundation. A.H. is supported by a starting grant from the Swedish Research Council (VR 2016-01789), as well as the Åhlens Foundation, the Crafoord Foundation, and the Physiographic Society in Lund.
Conflicts of Interest
The authors declare no conflicts of interest.
Author Contributions
Matilde Negrini : Investigation; methodology; resources; validation; visualization; writing-original draft. Gang Wang : Conceptualization; formal analysis; investigation; methodology; validation; visualization; writing-original draft. Andreas Heuer : Conceptualization; investigation; methodology; project administration; resources; validation; visualization; writing-original draft. Tomas Björklund : Conceptualization; formal analysis; project administration; visualization; writing-original draft. Marcus Davidsson : Conceptualization; formal analysis; funding acquisition; investigation; methodology; project administration; resources; supervision; validation; visualization; writing-original draft.
Literature Cited
- Auricchio, A., Hildinger, M., O'Connor, E., Gao, G. P., & Wilson, J. M. (2001). Isolation of highly infectious and pure adeno-associated virus type 2 vectors with a single-step gravity-flow column. Human Gene Therapy , 12, 71–76. doi: 10.1089/104303401450988.
- Aurnhammer, C., Haase, M., Muether, N., Hausl, M., Rauschhuber, C., Huber, I., … Baiker, A. (2012). Universal real-time PCR for the detection and quantification of adeno-associated virus serotype 2-derived inverted terminal repeat sequences. Human Gene Therapy Methods , 23, 18–28. doi: 10.1089/hgtb.2011.034.
- Bachrach, U., & Friedmann, A. (1971). Practical procedures for the purification of bacterial viruses. Applied Microbiology , 22, 706–715. doi: 10.1128/AEM.22.4.706-715.1971.
- Boussif, O., Lezoualc'h, F., Zanta, M. A., Mergny, M. D., Scherman, D., Demeneix, B., & Behr, J. P. (1995). A versatile vector for gene and oligonucleotide transfer into cells in culture and in vivo: Polyethylenimine. Proceedings of the National Academy of Sciences of the United States of America , 92, 7297–7301. doi: 10.1073/pnas.92.16.7297.
- Davidsson, M., Wang, G., Aldrin-Kirk, P., Cardoso, T., Nolbrant, S., Hartnor, M., … Bjorklund, T. (2019). A systematic capsid evolution approach performed in vivo for the design of AAV vectors with tailored properties and tropism. Proceedings of the National Academy of Sciences of the United States of America , 116, 27053–27062. doi: 10.1073/pnas.1910061116.
- Deverman, B. E., Pravdo, P. L., Simpson, B. P., Kumar, S. R., Chan, K. Y., Banerjee, A., … Gradinaru, V. (2016). Cre-dependent selection yields AAV variants for widespread gene transfer to the adult brain. Nature Biotechnology , 34, 204–209. doi: 10.1038/nbt.3440.
- Felgner, P. L., Gadek, T. R., Holm, M., Roman, R., Chan, H. W., Wenz, M., … Danielsen, M. (1987). Lipofection: A highly efficient, lipid-mediated DNA-transfection procedure. Proceedings of the National Academy of Sciences of the United States of America , 84, 7413–7417. doi: 10.1073/pnas.84.21.7413.
- Govindasamy, L., DiMattia, M. A., Gurda, B. L., Halder, S., McKenna, R., Chiorini, J. A., … Agbandje-McKenna, M. (2013). Structural insights into adeno-associated virus serotype 5. Journal of Virology , 87, 11187–11199. doi: 10.1128/JVI.00867-13.
- Graham, F. L., & van der Eb, A. J. (1973). A new technique for the assay of infectivity of human adenovirus 5 DNA. Virology , 52, 456–467. doi: 10.1016/0042-6822(73)90341-3.
- Gray, S. J., Blake, B. L., Criswell, H. E., Nicolson, S. C., Samulski, R. J., McCown, T. J., & Li, W. (2010). Directed evolution of a novel adeno-associated virus (AAV) vector that crosses the seizure-compromised blood-brain barrier (BBB). Molecular Therapy , 18, 570–578. doi: 10.1038/mt.2009.292.
- Kohlbrenner, E., Aslanidi, G., Nash, K., Shklyaev, S., Campbell-Thompson, M., Byrne, B. J., … Zolotukhin, S. (2005). Successful production of pseudotyped rAAV vectors using a modified baculovirus expression system. Molecular Therapy , 12, 1217–1225. doi: 10.1016/j.ymthe.2005.08.018.
- Kotterman, M. A., Chalberg, T. W., & Schaffer, D. V. (2015). Viral vectors for gene therapy: Translational and clinical outlook. Annual Review of Biomedical Engineering , 17, 63–89. doi: 10.1146/annurev-bioeng-071813-104938.
- Lock, M., Alvira, M. R., Chen, S. J., & Wilson, J. M. (2014). Absolute determination of single-stranded and self-complementary adeno-associated viral vector genome titers by droplet digital PCR. Human Gene Therapy Methods , 25, 115–125. doi: 10.1089/hgtb.2013.131.
- McCarty, D. M., Monahan, P. E., & Samulski, R. J. (2001). Self-complementary recombinant adeno-associated virus (scAAV) vectors promote efficient transduction independently of DNA synthesis. Gene Therapy , 8, 1248–1254. doi: 10.1038/sj.gt.3301514.
- Ni, T. H., Zhou, X., McCarty, D. M., Zolotukhin, I., & Muzyczka, N. (1994). In vitro replication of adeno-associated virus DNA. Journal of Virology , 68, 1128–1138. doi: 10.1128/JVI.68.2.1128-1138.1994.
- O'Riordan, C. R., Lachapelle, A. L., Vincent, K. A., & Wadsworth, S. C. (2000). Scaleable chromatographic purification process for recombinant adeno-associated virus (rAAV). The Journal of Gene Medicine , 2, 444–454. doi: 10.1002/1521-2254(200011/12)2:6<444::AID-JGM132>3.0.CO;2-1.
- Ojala, D. S., Sun, S., Santiago-Ortiz, J. L., Shapiro, M. G., Romero, P. A., & Schaffer, D. V. (2018). In vivo selection of a computationally designed SCHEMA AAV library yields a novel variant for infection of adult neural stem cells in the SVZ. Molecular Therapy , 26, 304–319. doi: 10.1016/j.ymthe.2017.09.006.
- Salganik, M., Hirsch, M. L., & Samulski, R. J. (2015). Adeno-associated virus as a mammalian DNA vector. Microbiology Spectrum , 3, MDNA3-0052-2014. doi: 10.1128/microbiolspec.MDNA3-0052-2014.
- Sanmiguel, J., Gao, G., & Vandenberghe, L. H. (2019). Quantitative and digital droplet-based AAV genome titration. Methods in Molecular Biology , 1950, 51–83. doi: 10.1007/978-1-4939-9139-6_4.
- Strobel, B., Miller, F., Rist, W., & Lamla, T. (2015). Comparative analysis of cesium chloride- and iodixanol-based purification of recombinant adeno-associated viral vectors for preclinical applications. Human Gene Therapy Methods , 26, 147–157. doi: 10.1089/hgtb.2015.051.
- Vance, M. A., Mitchell, A., & Samulski, R. J. (2015). AAV biology, infectivity and therapeutic use from bench to clinic. In D. Hashad (Ed.) Gene Therapy - Principles and Challenges (pp. 119–143). London: InTech Open. doi: 10.5772/61988.
- Wu, Z., Asokan, A., & Samulski, R. J. (2006). Adeno-associated virus serotypes: Vector toolkit for human gene therapy. Molecular Therapy , 14, 316–327. doi: 10.1016/j.ymthe.2006.05.009.
- Zolotukhin, S., Byrne, B. J., Mason, E., Zolotukhin, I., Potter, M., Chesnut, K., … Muzyczka, N. (1999). Recombinant adeno-associated virus purification using novel methods improves infectious titer and yield. Gene Therapy , 6, 973–985. doi: 10.1038/sj.gt.3300938.
Citing Literature
Number of times cited according to CrossRef: 16
- Danish M. Anwer, Francesco Gubinelli, Yunus A. Kurt, Livija Sarauskyte, Febe Jacobs, Chiara Venuti, Ivette M. Sandoval, Yiyi Yang, Jennifer Stancati, Martina Mazzocchi, Edoardo Brandi, Gerard O’Keeffe, Kathy Steece-Collier, Jia-Yi Li, Tomas Deierborg, Fredric P. Manfredsson, Marcus Davidsson, Andreas Heuer, A comparison of machine learning approaches for the quantification of microglial cells in the brain of mice, rats and non-human primates, PLOS ONE, 10.1371/journal.pone.0284480, 18 , 5, (e0284480), (2023).
- Zoe Whiteley, Giulia Massaro, Georgios Gkogkos, Asterios Gavriilidis, Simon N. Waddington, Ahad A. Rahim, Duncan Q. M. Craig, Microfluidic production of nanogels as alternative triple transfection reagents for the manufacture of adeno-associated virus vectors, Nanoscale, 10.1039/D2NR06401D, 15 , 12, (5865-5876), (2023).
- Ji-Young Park, Sivasankar Chandran, Chamith Hewawaduge, John Hwa Lee, Development and evaluation of a mouse model susceptible to severe fever with thrombocytopenia syndrome virus by rAAV-based exogenous human DC-SIGN expression, Microbial Pathogenesis, 10.1016/j.micpath.2023.106079, 178 , (106079), (2023).
- F. Gubinelli, L. Sarauskyte, C. Venuti, I. Kulacz, G. Cazzolla, M. Negrini, D. Anwer, I. Vecchio, F. Jakobs, F.P. Manfredsson, M. Davidsson, A. Heuer, Characterisation of functional deficits induced by AAV overexpression of alpha-synuclein in rats, Current Research in Neurobiology, 10.1016/j.crneur.2022.100065, 4 , (100065), (2023).
- Marcus Davidsson, Andreas Heuer, Small scale adeno-associated virus-vector production for preclinical gene delivery based on chloroform precipitation, Neural Regeneration Research, 10.4103/1673-5374.314309, 17 , 1, (99), (2022).
- Vijayakumar Jawalagatti, Perumalraja Kirthika, Chamith Hewawaduge, Ji-Young Park, Myeon-Sik Yang, Byungkwan Oh, Mi Young So, Bumseok Kim, John Hwa Lee, A Simplified SARS-CoV-2 Mouse Model Demonstrates Protection by an Oral Replicon-Based mRNA Vaccine, Frontiers in Immunology, 10.3389/fimmu.2022.811802, 13 , (2022).
- Jia-Shiung Guan, Kai Chen, Yingnan Si, Taehyun Kim, Zhuoxin Zhou, Seulhee Kim, Lufang Zhou, Xiaoguang Liu, Process Improvement of Adeno-Associated Virus Production, Frontiers in Chemical Engineering, 10.3389/fceng.2022.830421, 4 , (2022).
- Satoshi Watanabe, Yuta Nihongaki, Kie Itoh, Toru Uyama, Satoshi Toda, Shigeki Watanabe, Takanari Inoue, Defunctionalizing intracellular organelles such as mitochondria and peroxisomes with engineered phospholipase A/acyltransferases, Nature Communications, 10.1038/s41467-022-31946-5, 13 , 1, (2022).
- Patrick Aldrin-Kirk, Malin Åkerblom, Tiago Cardoso, Sara Nolbrant, Andrew F. Adler, Xiaohe Liu, Andreas Heuer, Marcus Davidsson, Malin Parmar, Tomas Björklund, A novel two-factor monosynaptic TRIO tracing method for assessment of circuit integration of hESC-derived dopamine transplants, Stem Cell Reports, 10.1016/j.stemcr.2021.11.014, 17 , 1, (159-172), (2022).
- Luis Quintino, Francesco Gubinelli, Livija Sarauskyte, Elin Arvidsson, Marcus Davidsson, Cecilia Lundberg, Andreas Heuer, Automated quantification of neuronal swellings in a preclinical rodent model of Parkinson’s disease detects region-specific changes in pathology, Journal of Neuroscience Methods, 10.1016/j.jneumeth.2022.109640, 378 , (109640), (2022).
- F. Gubinelli, G. Cazzolla, M. Negrini, I. Kulacz, A. Mehrdadian, G. Tomasello, C. Venuti, L. Sarauskyte, F. Jacobs, F.P. Manfredsson, M. Davidsson, A. Heuer, Lateralized deficits after unilateral AAV-vector based overexpression of alpha-synuclein in the midbrain of rats on drug-free behavioral tests, Behavioural Brain Research, 10.1016/j.bbr.2022.113887, 429 , (113887), (2022).
- Brian Zingg, Hong‐Wei Dong, Huizhong Whit Tao, Li I. Zhang, Application of AAV1 for Anterograde Transsynaptic Circuit Mapping and Input‐Dependent Neuronal Cataloging, Current Protocols, 10.1002/cpz1.339, 2 , 1, (2022).
- Lyubava Belova, Konstantin Kochergin‐Nikitsky, Anastasia Erofeeva, Alexander Lavrov, Svetlana Smirnikhina, Approaches to purification and concentration of rAAV vectors for gene therapy, BioEssays, 10.1002/bies.202200019, 44 , 6, (2022).
- Aitthiphon Chongchai, Sajee Waramit, Tunchanok Wongwichai, Jirawan Kampangtip, Thanyaluck Phitak, Prachya Kongtawelert, Amin Hajitou, Keittisak Suwan, Peraphan Pothacharoen, Targeting Human Osteoarthritic Chondrocytes with Ligand Directed Bacteriophage-Based Particles, Viruses, 10.3390/v13122343, 13 , 12, (2343), (2021).
- Tomas Björklund, Marcus Davidsson, Next-Generation Gene Therapy for Parkinson’s Disease Using Engineered Viral Vectors, Journal of Parkinson's Disease, 10.3233/JPD-212674, 11 , s2, (S209-S217), (2021).
- Marcus Davidsson, Matilde Negrini, Swantje Hauser, Alexander Svanbergsson, Marcus Lockowandt, Giuseppe Tomasello, Fredric P. Manfredsson, Andreas Heuer, A comparison of AAV-vector production methods for gene therapy and preclinical assessment, Scientific Reports, 10.1038/s41598-020-78521-w, 10 , 1, (2020).