dTBI2: A Calibrated, Tunable Device for Administering Traumatic Brain Injury in Drosophila
Stephen W. Ratner, Stephen W. Ratner, Michael Fetchko, Michael Fetchko, Akanksha S. Mathivanan, Akanksha S. Mathivanan, Seanna E. Kelly, Seanna E. Kelly, Shambhavi Gupta, Shambhavi Gupta, Annika F. Barber, Annika F. Barber
Abstract
The second-generation Drosophila traumatic brain injury (TBI) device dTBI2 improves Drosophila TBI administration by providing a moderate-throughput, tunable, head-specific injury. Our updated device design improves user-friendliness, eliminates inconsistencies in injury timing, and has an updated circuit design to extend the longevity of delicate electronic components. dTBI2 improves reproducibility across users and runs, and results in more consistent post-injury phenotypes. This protocol describes the construction, calibration, and use of the dTBI2 device, which uses an Arduino-controlled piezoelectric actuator to deliver a force that compresses a fly head against a metal collar. The duration and depth of head compression is tunable, allowing calibration of injury severity. All device components are commercially available, and the entire device can be constructed in under a week for less than $1000. The dTBI2 design will enable any lab to build a highly reliable, low-cost device for Drosophila TBI, facilitating increased adoption and ease of exploration of closed-head TBI in Drosophila for forward genetic screens. We describe below the three protocols necessary for constructing a dTBI2 device. © 2024 The Authors. Current Protocols published by Wiley Periodicals LLC.
Basic Protocol 1 : Construction of the dTBI2 control device
Basic Protocol 2 : Construction of the piezoelectric actuator housing
Basic Protocol 3 : Administration of dTBI2 injuries
INTRODUCTION
Traumatic brain injury (TBI) results in death and disability for thousands of people annually, and increases risk for long-term neurological and neuropsychiatric disorders, including chronic traumatic encephalopathy, post-traumatic stress disorder, and neurodegenerative diseases (CDC, 2019; Meng et al., 2017). An external traumatic force causes a primary injury, followed by complex secondary responses that initially serve protective roles, but in some cases trigger progressive, chronic pathologies. The secondary injury phase may last days to weeks, and remodeling of transcriptional profiles and biochemical signaling cascades may persist for years after injury, contributing to the elevated long-term risks of neurological and neuropsychiatric disorders in TBI survivors. While improved treatments have resulted in decreased mortality after TBI, there have been no major breakthroughs that address the chronic impairments experienced by survivors (Bragge et al., 2016). A mechanistic understanding of how inflammatory signaling after TBI alters sleep and circadian function is necessary to develop interventions that improve quality of life for TBI patients.
In the last decade, Drosophila models of TBI have identified genes that contribute to TBI-induced mortality, motor defects, and neurodegeneration (Barekat et al., 2016; Behnke et al., 2021; Katzenberger et al., 2013; Saikumar et al., 2020; van Alphen et al., 2022). Several of these genes also show changes in expression or function after TBI in humans, underscoring the utility of fly TBI models to discover genes and molecular pathways relevant to post-TBI pathology (Anderson et al., 2021; Byrns et al., 2021).
To facilitate further development of Drosophila genetic models for post-TBI outcomes, injury paradigms must optimize four key parameters: throughput, injury severity, reproducibility, and ease of use. To perform genetic screens, moderate to high throughput is required to injure a sufficient number of flies. To model aspects of human TBI, the ability to precisely modulate the severity of injury and consequent post-injury outcomes is necessary. To generate interpretable data, injury characteristics must be reproducible within and between injury trials. Finally, to facilitate broad adoption within the fly community, any injury paradigm must be easy to build and robust to changes in injury characteristics between users. The most widely used Drosophila TBI paradigm is the high-impact trauma (HIT) assay (Katzenberger et al., 2013). The HIT assay delivers whole-animal trauma by grouped injury of flies in a vial affixed to a spring that is bent back, permitting impact of the vial on a soft surface. Although throughput is high, reproducibility of injury is low, and damage to the abdomen, legs, and wings can affect downstream assays. Other grouped fly TBI paradigms using a mixer mill and weight drop offer similar limitations in injury reproducibility (Barekat et al., 2016; Behnke et al., 2021). Two piezo-based methods delivery highly calibrated injuries with low (van Alphen et al., 2022) or moderate (Saikumar et al., 2020) throughput.
The dTBI device is a head-specific, tunable Drosophila injury paradigm that delivers head-specific compression via a piezo-actuated plate with moderate throughput and tunable injury severity (Byrns et al., 2021; Saikumar et al., 2020). However, limitations in the original design result in variable injury characteristics between runs. Although the percent compression of individual fly heads is reasonably consistent between runs, the speed of piezo movement is not (Fig. 1). The time it takes for the piezo plate to go from resting to fully deflected (crush time; Fig. 1B), the time the piezo plate pauses at maximum deflection (pause time: Fig. 1C), and the time for piezo return from maximum deflection to resting position (return time; Fig. 1D) all vary from run to run even with no change in device settings. This variability in injury duration results in variable injury outcomes between runs, including variable mortality, sleep, and circadian rhythm strength. Our second-generation device, the dTBI2, improves on the original design through changes in hardware, circuit design, and Arduino code. Improvements in device timing are reflected in reduced variability of crush, pause, and return times between runs (Fig. 1B-D).
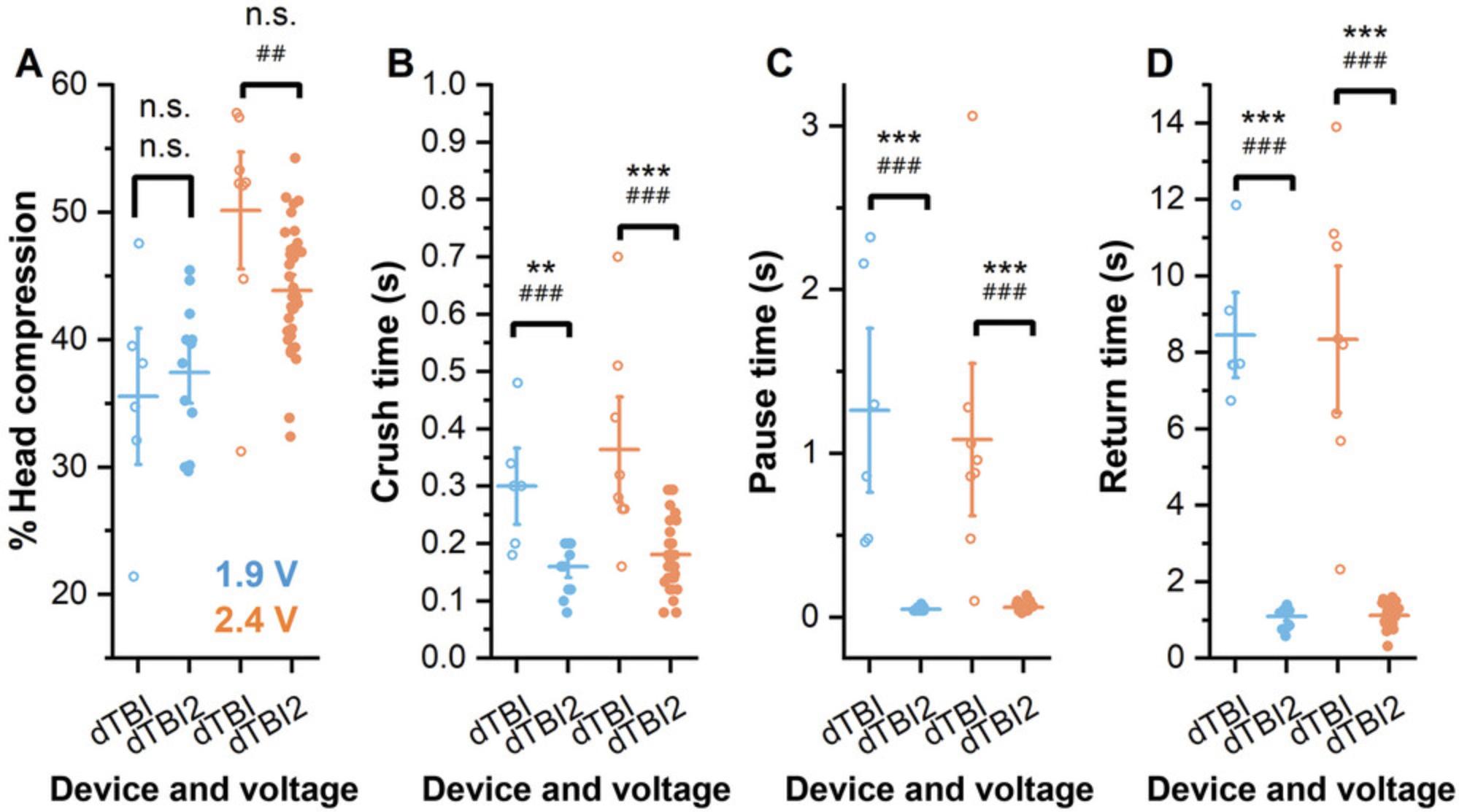
Improvements in reliability of device timing also improve the modulation of injury severity. As shown in Figure 2, the severity of injury can be adjusted both by altering the maximal piezo plate deflection and by controlling the pause time via adjustment of the pulse time in line 24 of CODE FILE 1. We note, however, that increasing pause time (Fig. 3A) also increases crush time, although with a different slope, while return time is unaffected (Fig. 3B,C). Additionally, although pause time increases linearly with changes in the set value in CODE FILE 1, the pause time does not match the set time in milliseconds (Fig. 3B). Changes in crush time when the voltage is held constant do not significantly affect the depth of head compression (Fig. 2A), but increased time at maximum compression increases mortality (Fig. 2B). This finding underscores the importance of the improved control of injury timing using the dTBI2 as essential to reproducibility of injury itself and consequent post-TBI outcomes. Supporting Information Videos 1-4 show dTBI2 injury under different pulse conditions.
Basic Protocol 1: CONSTRUCTION OF THE dTBI2 CONTROL DEVICE
The dTBI2 device is an Arduino-controlled piezo actuator for calibrated compression of fly heads. dTBI2 consists of two hardware components—the control device and the piezo actuator—as well as the software necessary to operate the device. The control device consists of the necessary circuitry to power the piezo and control it via the Arduino. The dTBI2 device has a modified circuit design that protects the Arduino from potentially damaging back-currents and extends device life by replacing the mechanical relay with a solid-state relay. The dTBI2 device also grounds the piezo through a resistor, allowing for reliable control of injury duration. In addition, the Arduino code has been optimized to eliminate uncontrolled triggering of the Arduino, triggering of multiple electrical pulses with a single button depression, and relay chatter during the Arduino boot process. This protocol describes the construction of the control device and the upload and modification of the Arduino code necessary to operate the piezo. Basic soldering skills are required. The complete control device and uploaded code must be paired with the piezo actuator constructed in Basic Protocol 2 to drive calibrated piezo deflection.
Materials
- 8″ × 8″ × 4″ PVC electrical junction box (device housing)
- Electric drill with metric drill bit set
- Arduino Rev3 microcontroller (Arduino, A000066)
- LM2596 DC-DC buck converter step-down module, input range 3-40 V, output range 1.5-35 V (RioRand, 3-01-0076)
- Helping hands soldering tool
- Soldering station
- 60/40 rosin-core solder (Grainger, #1UYH4)
- Flat-head screwdriver
- Two non-solderable, 400-contact breadboards (CircuitSpecialists, model WB-801)
- 22-gauge insulated copper wire (Amazon, ASIN B00B4ZQ3L0)
- Electrical wire strippers (McMaster Carr, model 7294K14)
- PCB-mount, 60 V 750 mA solid-state relay (SSR, Mouser, ID 849-CPC1019N)
- 680-Ω and 1-MΩ resistors, 0.5-W tolerance minimum (Allecin resistor kit, Amazon, ASIN B0BTP63DGQ)
- Pushbutton switch, SPST, normally open (Amazon, ASIN B08SQHRRDH)
- Electrical tape
- 10-kΩ linear variable potentiometer (Uxcell, a15082600ux0077)
- Power adapter, 3-12 V, 12 W (SoulBay, UC02U)
- 5.5 × 2.5–mm female panel mount jack with pre-soldered 16 AWG wires (BixPower, model CNT-W4)
- DC 0-30 V digital voltmeter display (Amazon, ASIN B00YALUXH0)
- Two-pin electrical terminal connector (Amazon, ASIN B01A6LTK44)
- M2.5 nylon hex standoff female kit (Amazon, ASIN B08HS7694T)
- Phillips-head screwdriver kit
- Proportional voltage booster: output 2 mA, 0-250 V DC; input 0-12 V DC, 85 mA; linear output (Gamma High Voltage model SM-2.5)
- Computer
- dTBI Arduino code (doi.org/10.5281/zenodo.10471222)
- USB A–to–USB B cable (USB A/B)
- Arduino integrated development environment (IDE) software application (https://www.arduino.cc; download the most recent version that works on your computer)
- 5-pin male single-row header connector PC mount, 0.1″ (Vetco Electronics, model VET-HEAD-SR-5), pack of 5 (will be snapped apart to single pins)
NOTE : Part numbers are listed when possible, but most parts can be substituted with an equivalent from another vendor. The two exceptions that must be purchased exactly as specified are the Arduino Rev3 microcontroller and the SM-2.5 voltage booster.
Assemble device housing/armature
1.Place the 8″ × 8″ × 4″ PVC electrical junction box (the device housing) on the bench.
2.Use a drill to bore a 10-mm-diameter hole in the back left side of the housing, towards the corner.
3.Place the Arduino microcontroller in the top right quadrant of the housing, orienting the USB port on the microcontroller towards the back wall of the housing (side A) and touching the USB port to the wall. Using the Arduino as a guide, mark the four mounting holes for the microcontroller on the housing, as well as the opening (14 × 14 mm) needed for the USB port.
4.Remove the Arduino and drill holes for M2.5 screws into the base of the housing for the mounting holes. Then drill a hole into the back panel of the housing (side A) for USB port access, leaving enough room for a USB cable to be inserted into the Arduino USB port.
5.Drill a hole in the lid of the housing, in the lower left quadrant between sides C and D (Fig. 4C), such that the potentiometer can be mounted comfortably within the hole.
6.Drill a hole in the lid of the housing between sides A and D for the voltmeter digital display (Fig. 4C), such that only the wiring can descend into the device.
7.Drill a hole in the lid of the housing between sides A and B, such that the pushbutton can be mounted comfortably within the hole (Fig. 4C).
Prepare buck converter
The buck converter has a blue potentiometer attached to it, which is notable for a screw on the top that is used to adjust voltage. This potentiometer must be removed as described here.
8.Place the buck converter and the helping hands soldering tool on the bench.
9.Carefully mount the buck converter in the helping hands tool, leaving the bottom exposed but the top available for manipulation.
10.Remove the blue potentiometer by touching the bottom three pins underneath it with a soldering iron to melt the solder while using a small flat-head screwdriver on the front side of the board to gently pry the potentiometer from the device.
11.Set the buck converter aside.
Preload breadboards
The complete wiring of the device is illustrated in Figure 4A. Wire colors do not affect functionality, but make assembly and troubleshooting easier. Breadboard positions are named by numerical row and alphabetical column as marked on the boards. The ± rails are not labeled. To indicate position, we refer to them by row number as counted from the top (row 1) to the bottom (row 25).
12.Place two non-solderable breadboards on the bench and label them breadboard #1 and #2.
13.Cut wires to the following lengths and then strip ∼1 cm from both ends of each wire.
- six black wires ∼15.2 cm long
- one black wire ∼20.3 cm long
- three red wires ∼20.3 cm long
- one red wire ∼10.2 cm long
- two green wires ∼20.3 cm long
- two orange wires ∼7.6 cm long
14.On breadboard #1, solder the SSR onto each end of a header pin (Fig. 4A) using the helping hands tool to hold each piece in place and clay to assist.
15.On breadboard #1:
- Push the SSR into breadboard #1, inserting the rectangle-demarcated pin into 13f and the adjacent pin into 12f. The pin across from the rectangle pin should be able to sit in 13e with the pin diagonal to the rectangle pin in 12e.
If there is any difficulty inserting the SSR into the breadboard, bend the header pins of the SSR through their soldered joint so that it can fit on the breadboard.
-
Cut the leads of a 680-Ω resistor such that they can be placed easily into the breadboard while preventing the leads from sticking out too far.
-
Place one lead of the 680-Ω resistor into 30h and the other into 28h.
-
Solder a header pin onto each end of the ∼10.2-cm red wire (step 13). Place one end of the wire into 13h and the other into 28j.
-
Solder a header pin onto each end of an ∼15.2-cm black wire (step 13). Place one end into 12j and the other into the right (–) negative rail circuit ground 20 down from the top.
16.On breadboard #2:
- Cut the leads of a 1-MΩ resistor such that it can be placed easily into the breadboard while preventing the leads from sticking out too far.
This is the bleeder resistor (shown in blue in Fig.4A). It can be replaced with another resistor of at least 100 kΩ . Changing the resistor will modulate the return time: the higher the resistivity, the longer it will take for the piezoelectric actuator to return to its resting position.
-
Insert one end of the 1-MΩ resistor into 3c and the other into 5c.
-
Solder a header pin onto each end of an ∼7.6-cm orange wire (step 13), leaving one header pin wire solder joint perpendicular. Place the perpendicular end into 10h such that the wire is flush with the breadboard. Place the other end into 3d.
-
Solder a header pin onto each end of the other ∼7.6-cm orange wire. Insert one end into 5b and the other into the left (–) negative rail of breadboard #2, two down from the top.
-
Solder a header pin onto each end of an ∼15.2-cm black wire (step 13), leaving one header pin wire joint perpendicular. Place the perpendicular end into 20f such that it is flush with the breadboard. Place the other end into the left (–) negative rail of breadboard #2, four down from the top.
17.Set the buck converter face up on the bench.
-
Push one end of an ∼20.3-cm red wire (step 13) into the “IN +” hole of the buck converter and solder it in place. Solder a header pin to the other end of the wire.
-
Push one end of an ∼20.3-cm black wire (step 13) into the “IN –” hole of the buck converter and solder it in place. Solder a header pin to the other end of the wire.
-
Push one end of an ∼20.3-cm green wire (step 13) into the “OUT +” hole of the buck converter and solder it in place. Solder a header pin to the other end of the wire.
-
Push one end of an ∼15.2-cm black wire (step 13) into the “OUT –” hole of the buck converter and solder it in place. Solder a header pin to the other end of the wire so that it is perpendicular to the wire.
The header pins soldered to the free ends of these wires will be used later to connect the converter to constituent parts of the device.
Install pushbutton
18.Strip the bottom end of both wires on the pushbutton, leaving only enough room for soldering. Using the header pins, pushbutton, and helping hands soldering tool, solder one header pin onto each wire extending from the button.
19.Wrap the ends of the button wires with electrical tape such that only the header pins have exposed metal.
20.Mount the pushbutton into the hole between sides A and B of the lid (Fig. 4C). Allow the wires to dangle below into the housing.
Prepare potentiometer
21.Place the potentiometer on the workbench.
22.Cut three wires (one green, one red, and one black) to ∼25.5 to 35.5 cm long. Strip 1 cm from each end of each wire.
23.Insert one end of the green wire through the middle (#2) terminal of the potentiometer (Fig. 4A) and wrap the metal over itself to form a loop locking the wire onto the potentiometer. Solder the looped wire in place.
24.Repeat step 23 to attach the black wire to the right (#1) terminal and the red wire to the left (#3) terminal (Fig. 4A).
Install power adapter
25.Place the power adapter unit on the bench. Using a flat-head screwdriver, turn the red dial to an output setting of 12 V.
26.From the set of plugs provided with the power adapter, select the 5.5-mm plug and attach it to the adapter.
27.Take the female panel mount jack for the power supply and remove the mounting nut (if it is still attached).
28.From the inside the device housing, push the female connecter of the panel mount through the hole that was drilled into side D of the device (step 2; Fig. 4A) and then re-attach the nut on the outside of the housing. Secure it in place by tightening the connecter.
29.Strip the black and red wires of the female panel mount to expose ∼7.5 mm of the covering from each wire.
30.Solder one header pin to each on the exposed ends of the red and black wires of the panel mount such that the pins can be angled perpendicular to the wires.
Install voltmeter
31.Solder a header pin onto the end of each attached wire descending from the voltmeter.
32.Carefully insert the voltmeter into the top of the housing lid (Fig. 4C), such that all three wires descend into the device housing.
Install piezoelectric actuator plug
33.Place the two-pin electrical terminal connector on the bench.
34.Cut and strip 1 cm from the end of each of the wires attached to the female half of the electrical terminal connector, and solder a header pin to the end of each such that the header pins are perpendicular to the length of the wire.
35.Drill a hole large enough to fit the two wires and header pins of the electrical terminal connector on side C of the device housing, towards the bottom right (Fig. 4).
36.Push the wires and header pins inside the device such that the electrical terminal connector head is outside the device. Tie the wires into a small loop inside the device near the drilled hole such that tugging on the outside of the device does not move the wires inside.
Solder potentiometer wires to buck converter
37.Before soldering the buck converter to the potentiometer, ensure that your hands are steady and that you have a second individual who is familiar with the protocol to assist by holding the solder wire.
38.Place the buck converter on the bench such that the “IN +” hole is on the top left side.
39.Place the potentiometer on the bench. Solder a header pin to the end of each of the three wires for ease of manipulation when soldering to the buck converter. Be sure the solder has cooled before proceeding to the next steps.
40.Carefully place the green wire (terminal 2 of the potentiometer) into the central hole of the buck converter (where the old potentiometer was previously) and solder it in place (Fig. 4A).
41.Repeat step 40 to attach the red and black wires (terminals 3 and 1 of the potentiometer) to the left and right holes of the buck converter, respectively.
42.Rest the potentiometer and buck converter on the bench, being careful not to damage the soldered connections.
Install breadboards into housing
43.Wipe the device housing clean using a dry, lint-free cloth to minimize dust.
44.Remove the sticky wrap from the back of breadboard #1 and place it in the device housing in the top left quadrant between sides A and D (Fig. 4A). Be careful not to disturb any preinstalled parts.
45.Repeat to place breadboard #2 in the bottom right quadrant between sides B and C (Fig. 4A).
Install buck converter into housing
46.Carefully move the buck converter/potentiometer to the center of the bench, being careful not to damage any of the soldered attachments.
47.Place the buck converter in the device housing in the bottom left quadrant between sides C and D, such that the “IN +” hole is on the upper left (Fig. 4A).
48.The buck converter has two mounting holes: one next to the “IN +” and one next to the “OUT –”. Mark the bottom of the housing using a marker in the center of these mounting holes. Remove the buck converter and drill two pilot holes compatible with M2.5 screws.
49.Use the M2.5 nylon hex standoff kit to mount the buck converter in the pilot holes using two 6-mm M2.5 spacers with two 12-mm M2.5 nylon screws. Use a Phillips-head screwdriver and be careful not to strip the screws.
50.Mount the potentiometer using the hole drilled in the lid of the enclosure (step 5; Fig. 4C). Be careful not to damage the connections to the buck converter.
Install Arduino into housing
51.Place the Arduino microcontroller on the bench next to the device housing.
52.Place the microcontroller in the area that was previously demarcated (steps 3-4).
53.Use two 6-mm M2.5 hex nylon spacers and two M2.5 nylon 12-mm screws to attach the Arduino microcontroller to the housing closest to side A, with the critical USB port facing the hole on side A (Fig. 4A).
54.Finish mounting the Arduino microcontroller by screwing in another two M2.5 nylon 12-mm screws farthest from side A.
Add connecting wires between parts
To aid with organization, steps for connecting wires are listed in a counterclockwise fashion, starting from the Arduino microcontroller and ending at breadboard #2.Refer to Figure 4A for the wiring diagram.
55.Attach pushbutton.
-
Place the pushbutton's red wire header pin (red in Fig.4A) into Arduino digital pinhole #7. When placing the wire, apply force only to the metal pin, not the plastic brace. Wiggle the connection to ensure it is secure, but do not tug, as this could loosen the connection.
-
Place the pushbutton's black wire header pin (black in Fig.4A) into the second Arduino GND pin from the top (Fig.4).
56.Assemble the control circuit from the Arduino device.
-
Solder a header pin to each end of an ∼20.3-cm red wire (see step 13). Insert one end into Arduino digital pin #12 and the other into 30j of breadboard #1.
-
Solder a header pin to each end of an ∼15.2-cm black wire (see step 13). Insert one end into the Arduino GND one slot above the GND used for the pushbutton and insert the other end into the right (–) negative slot 17 down from the top of breadboard #1.
-
Solder a header pin to each end of the last ∼20.3-cm red wire (see step 13). Insert one end into the Arduino Vinpin and the other into the left (+) positive rail slot 21 down from the top of breadboard #1.
57.Assemble the control circuit from breadboard #1.
-
Attach the power supply panel mount by inserting the header pin of the black wire into the top right (–) negative rail slot of breadboard #1 and inserting the header pin of the red wire into the top left (+) positive rail slot.
-
Attach the voltmeter by inserting the black wire into the right (–) negative rail slot 2 down from the top of breadboard #1, inserting the green wire into 12a, and inserting the red wire into the left (+) positive rail slot 8 down from the top.
If any of the wires have too much exposed conductive surface, electrical tape can be used to insulate them.
-
Solder a header pin to each end of a ∼15.2-cm black wire (see step 13). Place one end into the right (–) negative rail slot 5 down from the top of breadboard #1 and the other end into the top left (–) negative rail slot of breadboard #2.
-
Solder a header pin to each end of the last ∼15.2-cm black wire (see step 13), leaving one header pin perpendicular to the length of the wire. Insert the non-perpendicular header pin into the right (–) negative rail slot 6 down from the top of breadboard #1 and the perpendicular header pin into 12a of breadboard #2 such that the length of the wire is flush with breadboard #2.
-
Using the black wire attached to the “IN –” terminal of the buck converter, insert the free header pin into the right (–) negative rail slot 11 down from the top of breadboard #1.
-
Using the green wire attached to the “OUT +” terminal, insert the free header pin into 13c of breadboard #1.
-
Solder a header pin to each end of the last ∼20.3-cm green wire (see step 13), leaving one header pin perpendicular to the length of the wire. Insert the non-perpendicular header pin into 12b of breadboard #1 and the perpendicular header pin into 18b of breadboard #2 such that the length of wire is flush with breadboard #2.
If there is risk of electrical contact between this wire and the wire in 13c, it is imperative that the end of the wire in 12b be electrically insulated with electrical tape.
-
Using the red wire attached to the “IN +” terminal of the buck converter, insert the free header pin into the left (+) positive rail slot 11 down from the top of breadboard #1.
58.Assemble the power circuit from the buck converter.
-
Using the black wire attached to the “OUT –” terminal of the buck converter, insert the free perpendicular header pin into 12b of breadboard #2 such that the length of wire is flush with breadboard #2.
59.Assemble the power circuit from breadboard #2.
-
Using the red wire from the female electrical terminal connector, place the perpendicular header pin into 10j of breadboard #2 such that the length of wire is flush with the breadboard.
-
Using the black wire from the female electrical terminal connector, place the perpendicular header pin into 20j of breadboard #2 such that the length of wire is flush with the breadboard.
Install voltage booster
60.Place the SM-2.5 high-voltage booster on the bench such that the pins are facing upwards.
61.Turn the voltage booster upside down and orient the “IN” pins to the left and the “OUT” pins to the right. Insert the “– IN” pin into 12c of breadboard #2, the “+ IN” pin into 18c, the “+ OUT” pin into 10i, and the “– OUT” pin into 20i.
Upload and modify Arduino code
To upload code into the newly constructed dTBI2 device, you will need a computer, the provided code CODE FILE 1, and a USB A/B cable. If your computer does not have a USB B port, use a USB A wire that can connect to whichever port you need to use to communicate with the Arduino microcontroller. While uploading code to the Arduino, do not plug in the dTBI2 controller to a power outlet. The Arduino will be able to power itself through the USB A/B cable.
62.Go to https://www.arduino.cc/en/software and download the latest Arduino IDE for your computer.
63.Open the file and follow the installation instructions provided.
64.Download CODE FILE 1.
65.With the Arduino IDE open, copy the contents of CODE FILE 1 and save the program by placing it in a folder with the same name as your file.
66.Plug the Arduino into the computer using the USB A/B cable.
67.Open the program you saved with CODE FILE 1 in it.
68.On the top of the menu is a drop-down menu that either says Select Board or will have the correct board already named. If it says Select Board, click the drop-down menu and select the only visible board.
69.Check the code again to ensure it looks like CODE FILE 1.
To change the injury duration (pause + crush time), alter code line 24, which reads delay(250). Adjusting this value changes the pulse signal duration, which is depicted in milliseconds.
70.Press the green checkmark in the top left corner of the IDE that when hovered over says verify. Wait for the IDE to respond.
71.Once the IDE has responded, press the green arrow that when hovered over says upload. Wait for the IDE to respond.
72.Go to computer settings and disconnect the device before unplugging the Arduino.
73.Proceed to Basic Protocol 2 to construct the piezoelectric activator, which must be connected to the controller to administer injury.
Basic Protocol 2: CONSTRUCTION OF THE PIEZOELECTRIC ACTUATOR PLATFORM
The piezoelectric actuator platform is a separable component of the dTBI2 device, which allows it to be placed at a distance from the control device so that it can be positioned under a dissecting microscope when administering injury and stored in an airtight container to protect delicate components when not in use. The piezo is mounted to a polycarbonate base such that the height of the actuator can be adjusted using washers to allow collared flies to fit beneath it without making contact. After assembling the piezoelectric actuator housing and connecting it to the control box described in Basic Protocol 1, depressing the button on the control box will result in visible piezo actuator deflection.
Materials
- 15.24 × 15.24 × 1.27–cm polycarbonate sheet (McMaster Carr, cat. no. 8574K321)
- Piezoelectric actuator (Piezo.com, cat. no. Q220-A4BR2513YB)
- Electric drill
- Drill bit set (US imperial)
- Two-pin electrical terminal connector (Amazon, ASIN B01A6LTK44)
- Electrical wire strippers (McMaster Carr, model no. 7294K14)
- 22-gauge wire (Amazon, ASIN B00B4ZQ3L0)
- Heat-shrink tubing
- Heat gun or hair dryer
- Non-insulated block spade terminals (Vetco Electronics, cat. no. SR-SPA-1N)
- Soldering station
- 60/40 rosin-core solder (Grainger, cat. no. 1UYH4)
- Helping hands soldering tool (optional)
- 4-40 × 3/8″ and 4-40 × 5/8″ mounting screws (McMaster Carr, cat. nos. 92196A108 and 92196A112 )
- Washers for no. 4 screws (McMaster Carr, model 92141A005)
- M2.5 male-female nylon hex standoff kit (Amazon, ASIN B08HS7694T)
- Phillips-head screwdriver set
- 24 × 16 × 8-cm airtight plastic storage bin
NOTE : Part numbers are listed when possible, but most parts can be substituted with an equivalent from another vendor. The piezoelectric actuator must be purchased exactly as specified.
NOTE : Wear nitrile gloves when handing the piezoelectric actuator.
1.Mark hole locations for piezo mounting on a 15.24 × 15.24 × 1.27–cm polycarbonate sheet. Place the piezo on the polycarbonate sheet, using the thin packaging paper that comes with the piezo to protect the piezo surface from the polycarbonate. Center the piezo, wire-side down, along one side of the polycarbonate sheet and mark the mounting holes ∼3 cm from the edge using a fine-tipped marker (Fig. 5A).
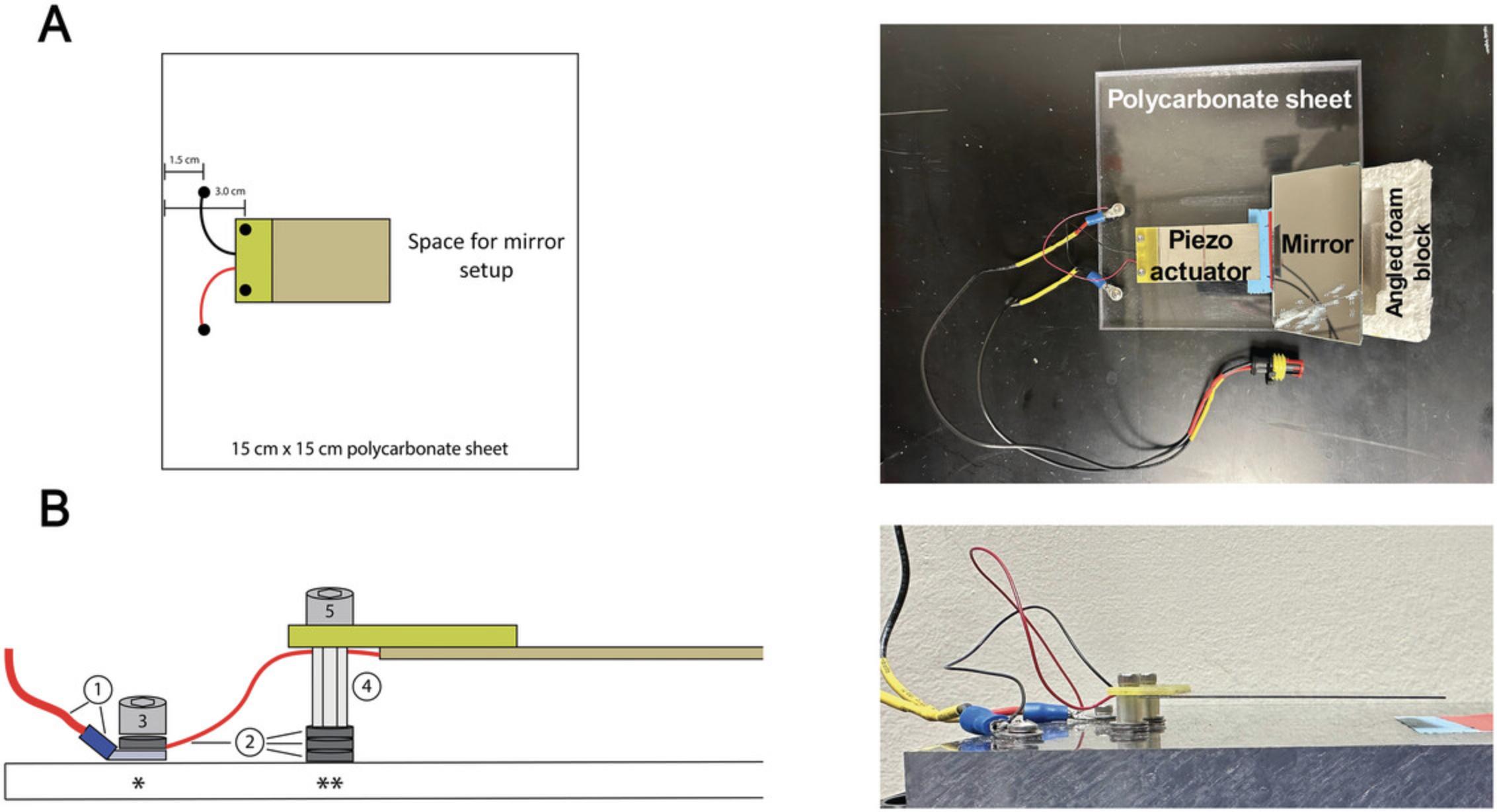
2.Mark holes for spade terminal connections on the polycarbonate sheet. Make two marks between the edge of the polycarbonate sheet and the piezo mounting marks, ∼1.5 cm from the edge of the polycarbonate sheet (Fig. 5A).
3.Using a 3/32″ bit, drill completely through the polycarbonate at each mark, making sure the holes are perpendicular to the surface of the sheet.
4.Cut and strip the ends of the black and red wires extending from the male half of a two-pin electrical terminal connector.
5.Solder an additional 20 cm of wire to both the red and black leads and insulate the wire connections using heat-shrink tubing. Solder a spade terminal to the end of each wire extension (Fig. 5B).
6.Secure spade terminals to the polycarbonate sheet. Lay one of the soldered spade terminals flat over a spade terminal connection hole and use a 4-40 × 3/8″ screw and a thin metal washer to secure the spade terminal to the polycarbonate sheet. Repeat for the other soldered spade terminal.
7.Mount the piezo electric actuator.
-
To prevent arcing from the piezo to the metal mounting screws, use a 1-cm female nylon threaded nut from the M2.5 kit to wrap the longer 4-40 × 5/8″ mounting screws.
-
Thread the mounting screw through the 1-cm female nylon threaded nut to ensure that the screw can pass through the nut.
-
Pass the mounting screw through the plastic mount of the piezo electric actuator and rethread the 1-cm nut onto the mounting screw.
-
Repeat for the second 4-40 × 5/8″ mounting screw.
-
Place thin metal washers between the nuts and the surface of the polycarbonate sheet to adjust the height of the piezo while inserting the mounting screws into the piezo mounting holes. Set the piezo at a height that allows a loaded Heisenberg collar to pass under the actuator without contacting the flies (Fig.5B).
8.Solder the red and black wires from the mounted piezo actuator to their respective spade terminal mounts on the polycarbonate sheet. Make sure to connect black to black and red to red.
9.Plug the male two-pin electrical connector from the piezo actuator platform to the female connector attached to the voltage booster of the dTBI2 control device in Basic Protocol 1.Plug the dTBI2 device to a wall outlet and set the voltage to a value below 4.5 V and push the button.
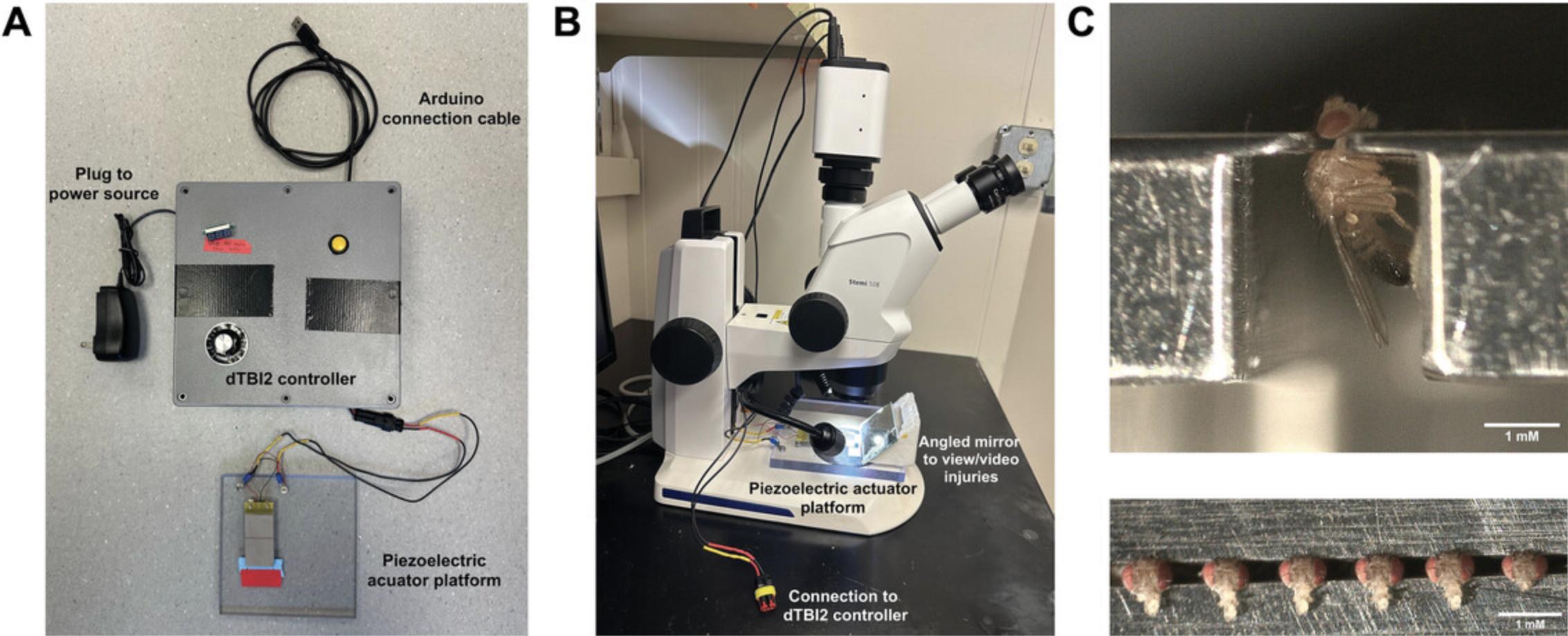
10.When the dTBI2 is not in use, store the piezo actuator platform apparatus in an airtight plastic bin to protect the piezo from moisture.
Basic Protocol 3: ADMINISTRATION OF dTBI2 INJURIES
To use the dTBI2 device, six anesthetized flies are loaded into a Heisenberg collar, then carefully placed under the piezoelectric actuator (Basic Protocol 2) and injured by piezo actuator deflection with a depth and time set by the control device (Basic Protocol 1). Flies are then removed from the collar and ready for use in downstream assays. Sham controls consist of flies loaded into the Heisenberg collar without head compression. To calibrate injury severity, injuries can be videoed and analyzed in ImageJ to calculate percent head compression and piezo actuator crush, pause, and return duration. These data should be combined with gross measures of fly health (here, we use mortality) to determine the appropriate injury severity for downstream experimental applications. Successful injury administration will show increasing head compression with increasing voltage, and crush and pause times that increase linearly with increases in electrical pulse length. Figure 6 shows the dTBI2 device fully assembled and in its active use state. dTBI2 administration remains ostensibly the same as in Saikumar et al. (2021). The primary difference between is the inclusion of injury duration in calibrating the device. A tutorial for injury administration is provided in Video 5.
For uniform head compression within a group of flies collared together, all heads must be of uniform size. This requires careful attention to fly housing density to prevent overcrowding (which leads to size heterogeneity) and requires injuring cohorts of flies that are the same sex and within 1-2 days of the same age. If experimental genotypes or diets substantially alter head size compared to controls, additional calibration of head compression depth will be necessary.
Materials
- Flies to be injured (experimental, control, sham)
- Carbon dioxide and anesthesia pad (Genesee, 59-114)
- Dissecting microscope (Zeiss Stemi or equivalent)
- Paintbrush, angled makeup brush, and/or feathers for fly manipulation
- Classic Heisenberg fly collar (4M Instrument & Tool, http://4mprototype.com, model 10731, custom order)
- Dumont no. 2 forceps (Fine Science Tools, 11223-20) or other dull forceps
- Piezoelectric actuator housing (see Basic Protocol 2)
- dTBI2 device controller (see Basic Protocol 1)
- Computer
- USB-A/B cable
- CODE FILE 1
- Standard fly food vials containing yeast-cornmeal-molasses food
- Mirror (McMaster Carr, 1017T316, cut to size 9 × 5.5 cm)
- Styrofoam wedge (10 × 10 × 10–cm block cut at a 45° angle)
- Super glue
- Camera and associated software for dissecting microscope
- ImageJ image analysis software (https://fiji.sc/)
Prepare flies
1.Anesthetize flies using CO2 and place them on an empty fly pad with continued CO2 flow under a dissecting microscope.
2.Place the Heisenberg collar on an empty portion of the fly pad with the opening for the flies to the right.
3.Using a pair of Dumont no. 2 forceps or dull forceps, grasp a fly by the wings while viewing under the microscope.
4.Bring the fly close to the entrance of the Heisenberg collar such that the head is above the lip of the collar's groove and the thorax is below the lip of the collar. Move the fly into the groove such that the head will no longer fall beneath the collar, and carefully release the fly from the forceps (see collaring video in Saikumar et al., 2021 and Fig. 6C).
5.Use a brush to carefully push the fly into the collar along the groove toward the end of the collar, but before the narrowing of the groove.
6.Load five more flies into the collar, leaving space between them (Fig. 6C).
7.Remove the collar from the CO2 pad.
Administer injury
We recommend administering injury with a partner, with one person collaring and unloading flies while the other administers the injury.
8.Using the dissecting microscope, place the Heisenberg collar under the piezo actuator, being careful to avoid contact between the fly heads and actuator (Fig. 6B).
9.Plug the piezoelectric actuator housing into the dTBI2 controller, and plug the dTBI2 device into the wall outlet.
10.To adjust pulse signal prior to injury, upload the Arduino code at this time (see Basic Protocol 1, steps 62-72).
11.Adjust the voltage using the potentiometer on the dTBI2 device.
12.Push the button to administer the dTBI2 injury. Wait at least 10 s for the device to return to its base state.
13.Remove the Heisenberg collar from the piezo actuator, being careful not to move it upwards, as this may induce a second injury. Once the collar is out from under the actuator, lift it up and place it on the fly pad.
14.Using Dumont no. 2 or dull forceps, carefully remove the flies from the collar (see collaring video in Saikumar et al., 2021).
15.Transfer injured flies to a standard food vial positioned horizontally to allow the flies to recover. Each vial for injured flies should house exactly twelve flies. Once flies are awake, turn the vials upright.
Image or video injury (optional)
16.To take videos and photographs during injury, use super glue to mount a 9 × 5.5–cm mirror to a 10 × 10 × 10–cm Styrofoam wedge cut at a 45° angle.
17.Align the mirror underneath the microscope, parallel to the Heisenberg collar, to visualize the injury as it is performed (Fig. 5A).
18.During injury, use the camera and associated software for the dissecting microscope to record videos at a time resolution of at least 25 frames per second.
Calibrate device
The dTBI2 device is calibrated using metrics of mortality, percent head compression, and injury duration.
19.Collect injured flies and perform a mortality assay. Place a maximum of twelve flies per vial at 25°C at 50% humidity and score the number of surviving flies every 2 days.
20.Calibrate dTBI2 head compression.
-
Open videos in ImageJ and set scale by normalizing the pixel count to the width of the piezoelectric actuator (501 μm).
-
Measure each fly head size before and at maximal compression by drawing a line from the base of the head to the top of the head, not including antennae.
-
Calculate percent head compression.
21.Determine the crush, pause, and return times for your device to validate that it is working appropriately by recording the video timestamp between frames with ImageJ.
-
Analyze crush time from the frame where the piezoelectric actuator begins to descend until the frame of maximal crush (where fly heads are maximally compressed).
-
Analyze pause time from the frame where the piezoelectric actuator reaches maximal crush to the frame where it begins to return by going upward.
-
Analyze return time from the frame where the piezoelectric actuator begins to return to the frame where it stops moving and begins to rest.
Once these steps are completed, the dTBI2 device should be fully calibrated and ready for use in experiments.
COMMENTARY
Critical Parameters
Circuit construction
All circuit contacts must be fully connected and cross-wire contacts must be prevented. Exposed, stripped wires can be insulated with electrical tape or heat-shrink tubing after soldering. Check that pins are fully inserted into breadboards. If reconnecting a component to a breadboard, insert the wire by gripping it with needle-nosed pliers. Do not grip the plastic box at the end of the pins, as the pins can slide and create faulty connections. Careful soldering is required to (1) ensure that all solder joints are fully connected; (2) avoid solder overflow, which could create cross-wire contacts; and (3) prevent melting of any other delicate metal circuit components. The buck converter is particularly sensitive to cross-soldering; if solder cannot be removed, starting over with a new converter may be necessary.
Piezo setup and use
The piezo actuator is the most expensive and delicate dTBI2 component. Careful handling is required to prevent damage and ensure successful installation. Always handle the piezo actuator with gloved hands to protect both the device and the user. Ensure that the correct side of the piezo is facing upward during installation to allow downward deflection (see Fig. 5B). After installation, check that the piezo is level so that injury is consistent across all flies in a collar. If the piezo is not level, adjust the screws anchoring the piezo to level it. Voltage as displayed on the voltmeter can never exceed 4.5 V or the piezoelectric actuator will break. The potentiometer is sensitive to movement, so always check the display before delivering an injury.
Collaring and injury administration
Practice and careful handling are necessary to prevent fly injury during Heisenberg collar loading and unloading. We recommend several practice sessions of collar loading and unloading using the dTBI video collaring tutorial (see collaring video in Saikumar et al., 2021). Flies injured during collaring should not be used, as this will generate inconsistent results.
The collar must be placed precisely under the piezo actuator in every run to ensure consistent injury across trials and users. Tape can be used to mark collar placement on the piezo polycarbonate sheet. When placing and removing the collar, it is critical to slide it so that fly heads do not make contact with the piezo and cause additional injury. If flies do make contact with the piezo during collar placement or removal, they should not be used for downstream assays.
Modifications
A multi-turn potentiometer could be used instead of the current single-turn potentiometer design to better fine-tune injury duration and prevent accidental voltage increases. If users anticipate opening the dTBI2 control device frequently, the control elements wired to the lid can be moved to side C to limit tugging on soldered components.
Troubleshooting
See Table 1 for possible problems, causes, and solutions.
Problem | Cause(s) | Possible solution |
---|---|---|
Inconsistent voltage reading on voltmeter display | Buck converter/potentiometer interface has loose leads or electrical contact between leads | Check soldered connections from the potentiometer to the buck converter. Ensure that all three leads are secure and that they are appropriately insulated from one another. |
Inconsistent head compression duration | Drainage (1 MΩ) resistor is not making electrical contact with the breadboard | Ensure good electrical contact between the 1-MΩ resistor and breadboard |
Loose wires throughout the control circuit | If the 1-MΩ resistor is in place and the problem persists, examine all other wires in the circuit to ensure they are making appropriate electrical connections | |
No deflection of piezoelectric actuator | Loose or improper connections in the dTBI2 device | Ensure that device is wired exactly as in Figure 4 and there are no loose wires |
Piezoelectric actuator appears lopsided over collared fly heads | Piezoelectric actuator is mounted improperly | Adjust piezoelectric actuator by tightening/loosening mounting screws |
Flies are decapitated during collaring | Rough handling during collaring; the entire fly is not being pushed by the forceps | Ensure forceps are inside the groove of the collar before pushing flies out of collar |
Heisenberg collar is sharp | Carefully file the edges of the collar. Maintain collars by occasionally cleaning off debris with 100% ethanol. | |
High sham mortality | Rough handling during collaring or positioning of collar under piezo | Be careful sliding flies into and out of collars and sliding or collar under piezo. Flies should not be experiencing any injury. |
Understanding Results
The dTBI2 device is a second-generation fly TBI procedure that builds on the dTBI design (Saikumar et al., 2021). Our hardware, circuit design, and code changes address improve control of injury timing (Fig. 1) and protect critical device components. Both the depth of head compression and the duration of injury affect fly mortality after TBI (Fig. 2), so tight control of these parameters (Fig. 3) is critical to reproducibility of injury phenotypes. Here, we provide instruction and design schematics (Figs. 4 and 5) for the dTBI2 device, which provides a tunable, moderate-throughput injury paradigm for head-specific Drosophila injury. Users must first build the Arduino-based device controller (Basic Protocol 1) and the piezoelectric actuator (Basic Protocol 2). After building and connecting these device components, injuries must be administered and videoed for calibration of timing and head compression depth (Basic Protocol 3). We also recommend calibrating injury severity by examining mortality after injury, as this varies with fly background strain and age. After calibration by video and mortality assays, users can begin to examine genetic, molecular, or behavioral phenotypes of interest. The dTBI2 device is suitable for large-scale forward genetic screens and for genomic approaches. The tight control of injury severity by both injury timing and depth of head compression will also permit users to identify how multiple injuries or injuries of varying severity affect TBI outcomes of interest from gene expression to behavior.
Time Considerations
Building the dTBI2 device can be accomplished in 8-16 hr by two users with available equipment and necessary soldering skill. Loading and injuring one collar of six flies takes 3-4 min with two skilled users, though practice is required to do this smoothly.
Acknowledgments
We would like to acknowledge Dr. Nancy Bonini for valuable conversations, Mr. Kevin Eric Wine from the Rutgers Department of Electrical and Computer Engineering for input on electrical engineering aspects of the device, and Dr. Ian Oldenburg for use of an oscilloscope for device testing. This work was funded in part by the New Jersey Commission on Brain Injury Research (CBIR22PIL026 to A.F.B.) and the National Institute for Neurological Disorders and Stroke (R21 NS135530 to A.F.B.).
Author Contributions
Stephen W. Ratner : Formal analysis; methodology; software; visualization; writing—original draft; writing—review and editing. Michael Fetchko : Formal analysis; methodology; supervision; validation; writing—review and editing. Akanksha S. Mathivanan : Formal analysis; validation; visualization; writing—review and editing. Seanna E. Kelly : Formal analysis; validation; visualization; writing—review and editing. Shambhavi Gupta : Formal analysis; validation; visualization; writing—review and editing. Annika F. Barber : Conceptualization; formal analysis; funding acquisition; supervision; writing—original draft; writing—review and editing.
Conflict of Interest
The authors declare no conflict of interest.
Open Research
Data Availability Statement
Data sharing is not applicable to this article as no new data were created or analyzed in this study.
Literature Cited
- Anderson, E. N., Morera, A. A., Kour, S., Cherry, J. D., Ramesh, N., Gleixner, A., Schwartz, J. C., Ebmeier, C., Old, W., Donnelly, C. J., Cheng, J. P., Kline, A. E., Kofler, J., Stein, T. D., & Pandey, U. B. (2021). Traumatic injury compromises nucleocytoplasmic transport and leads to TDP-43 pathology. eLife , 10, e67587. https://doi.org/10.7554/eLife.67587
- Barekat, A., Gonzalez, A., Mauntz, R. E., Kotzebue, R. W., Molina, B., El-Mecharrafie, N., Conner, C. J., Garza, S., Melkani, G. C., Joiner, W. J., Lipinski, M. M., Finley, K. D., & Ratliff, E. P. (2016). Using Drosophila as an integrated model to study mild repetitive traumatic brain injury. Scientific Reports , 6, 25252. https://doi.org/10.1038/srep25252
- Behnke, J. A., Ye, C., Setty, A., Moberg, K. H., & Zheng, J. Q. (2021). Repetitive mild head trauma induces activity mediated lifelong brain deficits in a novel Drosophila model. Scientific Reports , 11, 9738. https://doi.org/10.1038/s41598-021-89121-7
- Bragge, P., Synnot, A., Maas, A. I., Menon, D. K., Cooper, D. J., Rosenfeld, J. V., & Gruen, R. L. (2016). A state-of-the-science overview of randomized controlled trials evaluating acute management of moderate-to-severe traumatic brain injury. Journal of Neurotrauma , 33, 1461–1478. https://doi.org/10.1089/neu.2015.4233
- Byrns, C. N., Saikumar, J., & Bonini, N. M. (2021). Glial AP1 is activated with aging and accelerated by traumatic brain injury. Nature Aging , 1, 585–597. https://doi.org/10.1038/s43587-021-00072-0
- Centers for Disease Control and Prevention (CDC). (2019). Surveillance report of traumatic brain injury-related emergency department visits, hospitalizations, and deaths—United States, 2014. Centers for Disease Control and Prevention, U.S. Department of Health and Human Services , 24.
- Katzenberger, R. J., Loewen, C. A., Wassarman, D. R., Petersen, A. J., Ganetzky, B., & Wassarman, D. A. (2013). A Drosophila model of closed head traumatic brain injury. Proceedings of the National Academy of Sciences , 110, E4152–E4159. https://doi.org/10.1073/pnas.1316895110
- Meng, Q., Zhuang, Y., Ying, Z., Agrawal, R., Yang, X., & Gomez-Pinilla, F. (2017). Traumatic brain injury induces genome-wide transcriptomic, methylomic, and network perturbations in brain and blood predicting neurological disorders. EBioMedicine , 16, 184–194. https://doi.org/10.1016/j.ebiom.2017.01.046
- Saikumar, J., Byrns, C. N., Hemphill, M., Meaney, D. F., & Bonini, N. M. (2020). Dynamic neural and glial responses of a head-specific model for traumatic brain injury in Drosophila. Proceedings of the National Academy of Sciences of the United States of America , 117, 17269–17277. https://doi.org/10.1073/pnas.2003909117
- Saikumar, J., Kim, J., Byrns, C. N., Hemphill, M., Meaney, D. F., & Bonini, N. M. (2021). Inducing different severities of traumatic brain injury in Drosophila using a piezoelectric actuator. Nature Protocols , 16, 263–282. https://doi.org/10.1038/s41596-020-00415-y
- van Alphen, B., Stewart, S., Iwanaszko, M., Xu, F., Li, K., Rozenfeld, S., Ramakrishnan, A., Itoh, T. Q., Sisobhan, S., Qin, Z., Lear, B. C., & Allada, R. (2022). Glial immune-related pathways mediate effects of closed head traumatic brain injury on behavior and lethality in Drosophila. PLoS Biology , 20, e3001456. https://doi.org/10.1371/journal.pbio.3001456