Production and Thermal Exchange of Conditional Peptide-MHC I Multimers
Jolien J. Luimstra, Jolien J. Luimstra, Kees L. M. C. Franken, Kees L. M. C. Franken, Malgorzata A. Garstka, Malgorzata A. Garstka, Jan W. Drijfhout, Jan W. Drijfhout, Jacques Neefjes, Jacques Neefjes, Huib Ovaa, Huib Ovaa
Abstract
Cytotoxic CD8+ T cells mediate cellular immunity through recognition of specific antigens presented by MHC class I on all nucleated cells. Studying T cell interactions and responses provides invaluable information on infection, autoimmunity and cancer. Fluorescently labeled multimers of MHC I can be used to quantify, characterize, and isolate specific CD8+ T cells by flow cytometry. Here we describe the production and use of conditional MHC I multimers that can be loaded with peptides of choice by incubating them at a defined temperature. Multimers are folded with a template peptide that forms a stable complex at low temperature, but dissociates at a defined elevated temperature. Using this technology multiple MHC I multimers can be generated in parallel, to allow staining and isolation of large sets of antigen-specific CD8+ T cells, especially when combined with barcoding technologies. © 2019 The Authors.
INTRODUCTION
Major histocompatibility class I (MHC I) molecules complexed with antigenic peptides and multimerized on a streptavidin backbone are the classical reagents to visualize, characterize, and isolate antigen-specific CD8+ T cells (Altman et al., 1996). Fluorophore-labeled peptide-MHC I (pMHC I) multimers can be used for flow cytometry analysis and isolation of antigen-specific CD8+ T cells. Many different specificities can be identified in parallel using combinatorial coding, mass cytometry, or DNA-barcoding technologies (Bentzen et al., 2016; Hadrup et al., 2009; Newell et al., 2013). Conventional production of pMHC I complexes is a laborious process: for every T cell specificity a new pMHC I complex with a different peptide has to be produced, as MHCs are unstable without peptide and cannot be folded empty (Ljunggren et al., 1990). We have recently reported a peptide exchange technology that allows generation of a large batch of pMHC I multimers and exchange of the peptide using thermal dissociation (Fig. 1) (Luimstra et al., 2018). We have established exchange conditions for the most common human MHC I allele in the Caucasian population, HLA-A*02:01, and the murine allele H-2Kb. The design of template peptides suitable for thermal exchange on other MHC I alleles requires careful selection of proper peptides that dissociate under low-temperature conditions and the generation of conditional multimers for those alleles is anticipated in the near future.
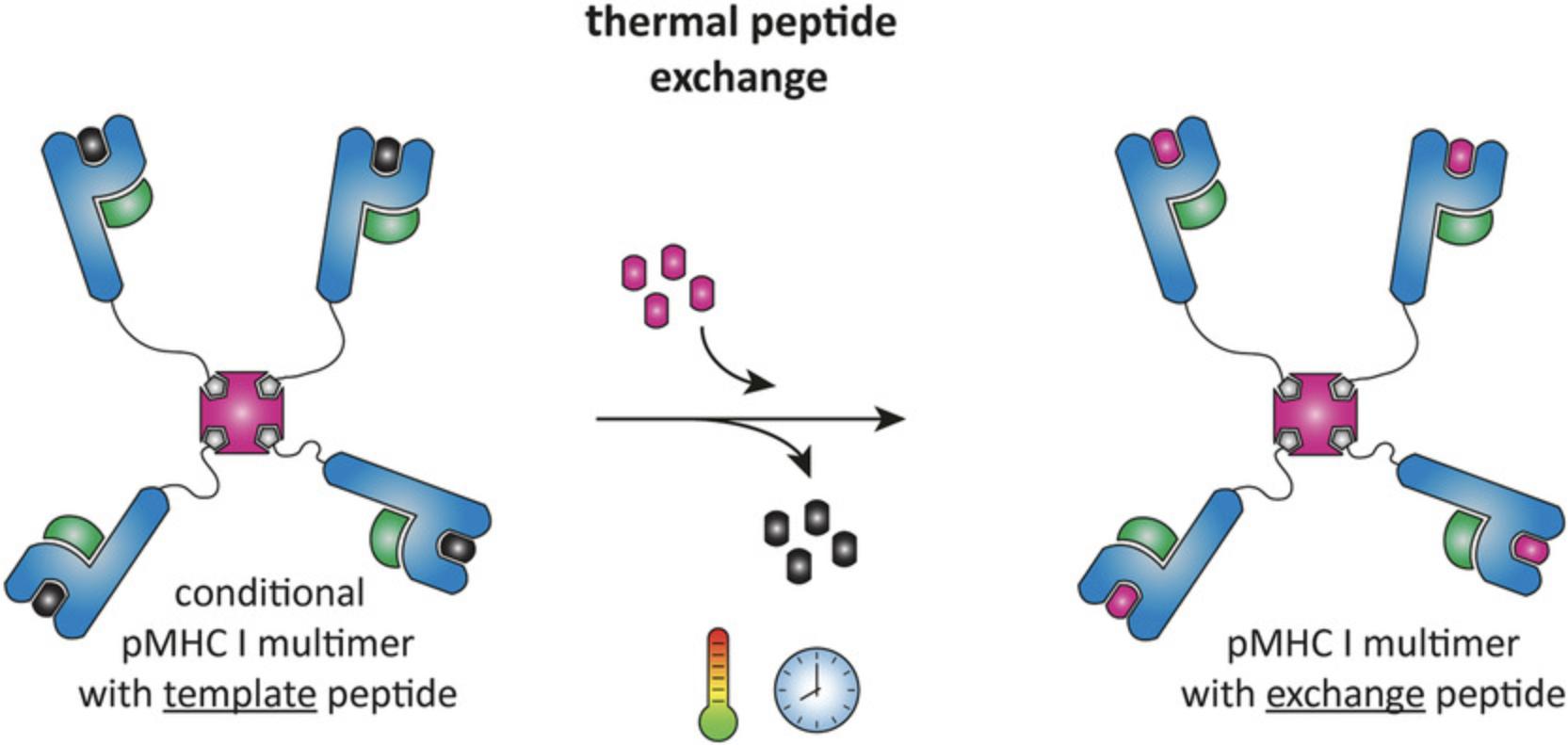
Basic Protocol 1 describes the production of conditional pMHC I monomers and Basic Protocol 2 describes the validation of thermal dissociation and peptide-mediated stabilization. Procedures for multimerization and exchange are described in Basic Protocols 3 and 4. Support protocols describe the expression and purification of MHC I heavy chain and β2m inclusion bodies, folding of β2m, and determination of the biotinylation efficiency.
Basic Protocol 1: FOLDING, BIOTINYLATION AND PURIFICATION OF CONDITIONAL pMHC I MONOMERS
The procedure for folding and biotinylation of pMHC I complexes is based on previously described protocols, with some adaptations (Garboczi, Hung, & Wiley, 1992; Rodenko et al., 2006; Toebes, Rodenko, Ovaa, & Schumacher, 2009). Conditional complexes are produced from MHC I heavy chain inclusion bodies, prefolded β2m (described in Support Protocol 2) and a template peptide (IAKEPVHGV for HLA-A*02:01 or FAPGNAPAL for H-2Kb). The MHC I heavy chain contains a 15-amino acid C-terminal recognition sequence for the BirA biotin ligase, which enzymatically conjugates a biotin molecule to the lysine in that sequence. The degree of biotinylation of pMHC I monomers following Basic Protocol 1 should be (near) complete, but it is recommended to determine the degree of biotinylation for each batch of pMHC I as described in Support Protocol 3.The present protocol describes a 50-ml folding reaction, but can be scaled up as desired. Alternative steps for large-scale folding reactions are mentioned when applicable.
Materials
-
Denaturing buffer (8 M urea/100 mM Tris•Cl, pH 8)
-
MHC I heavy chain inclusion bodies (Support Protocol 1)
-
Folding buffer (see recipe)
-
Template peptide: IAKEPVHGV for HLA-A*02:01; or FAPGNAPAL for H-2Kb (commercial sources or prepared through standard solid-phase peptide synthesis)
-
Dimethyl sulfoxide (DMSO)
-
Prefolded β2m (Support Protocol 2)
-
Milli-Q water
-
MHC buffer (300 mM NaCl/20 mM Tris•Cl, pH 8)
-
Biotinylation solution (see recipe)
-
Glycerol
-
Liquid nitrogen (for freezing)
-
1.5-ml microcentrifuge tubes
-
Rotator
-
50-ml conical tubes
-
Ice bucket with ice
-
Sonicator
-
Microcentrifuge
-
Syringes and 0.22- and 0.45-µm syringe filters
-
Water bath at 10°C
-
30-kDa MWCO centrifugal concentrators, 0.5- and 15-ml (e.g., Amicon Ultra centrifugal filters; Merck Millipore)
-
Illustra NAP-10 column (GE Healthcare)
-
Spin-X centrifuge tube filters 0.22 µm (Corning)
-
FPLC system with gel-filtration column (e.g., Superdex 75 10/300 (GE Healthcare))
-
PCR tubes or 1.5-ml polypropylene screw cap microcentrifuge tubes (Sarstedt)
-
Additional reagents and equipment for SDS gel electrophoresis and staining of proteins (Gallagher, 2006; Sasse & Gallagher, 2003) and gel-filtration chromatography (Hagel, 1998)
Folding of pMHC I complexes
1.Prepare 500 µl fresh denaturing buffer.
2.In a 1.5-ml reaction tube, dissolve ∼2.5 mg of MHC I heavy chain inclusion bodies (Support Protocol 1) in 500 µl denaturing buffer. Rotate at room temperature for at least 2 hr and preferably overnight to ensure complete dissolution.
3.Set up 50 ml folding buffer in a 50-ml conical tube, rotate ∼15 min at RT, and then cool on ice for 1−1.5 hr.
4.In the meantime, dissolve 3 mg of template peptide in ∼500 µl DMSO, and sonicate 10−15 min.
5.Add template peptide solution to the tube containing 50 ml folding buffer (60 µM final concentration).
6.Thaw a 1.2-mg aliquot of prefolded β2m (Support Protocol 2).
7.Microcentrifuge β2m and MHC I heavy chain for 2 min at 16,000 × g , save 1 µl of each supernatant at −20°C as a reference for SDS-PAGE analysis (step 18), and add the remainder of each supernatant to the folding buffer containing template peptide (final concentrations: 6 µM β2m and 3 µM MHC I heavy chain).
8.Filter the folding solution through a 0.22-µm filter using a syringe and leave in a 10°C water bath for 4−5 days.
Biotinylation of pMHC I complexes
NOTE : Folded complexes will dissociate at elevated temperatures, so from this point, keep all solutions and reagents on ice and centrifuges at 4°C!
9.Sediment aggregates in the folding solution by centrifugation for 10 min at 4,000 × g , 4°C to, and filter supernatant through a 0.45-µm filter using a syringe.
10.Wash a 15-ml 30-kDa MWCO centrifugal concentrator first with Milli-Q water and then with MHC buffer by centrifugation for 10 min each at 4,000 × g , 4°C. Add the filtered folding reaction (from step 9) and concentrate to ≤1 ml by centrifugation for 10 min at 4,000 × g , 4°C.
11.In a cold room, recover concentrated sample and exchange the folding buffer for MHC buffer using a NAP-10 column: Wash the column 3 times with 1 ml MHC buffer, apply sample, and elute with 1 ml MHC buffer.
12.Filter concentrated folding reaction through a SpinX centrifuge tube filter by centrifugation for 2 min (or longer if necessary) at 16,000 × g , 4°C.
13.Prepare biotinylation solution (see recipe) on ice, and add 1 ml biotinylation solution to the 1 ml pMHC I solution. Incubate overnight at 4°C, preferably on a rotator.
Purification of biotinylated pMHC I
14.Sediment aggregates in the biotinylation solution by centrifugation for 10 min at 4,000 × g , 4°C.
15.Wash a 0.5-ml 30-kDa MWCO centrifugal concentrator with Milli-Q water and then with MHC buffer. Concentrate biotinylation reaction to ∼500 µl by centrifugation for 10 min at 16,000 × g , 4°C.
16.Filter concentrated solution containing biotinylated pMHC I through a SpinX centrifuge tube filter by centrifugation for 2 min (or longer if necessary) at 16,000 × g , 4°C.
17.Purify biotinylated pMHC I complexes by gel-filtration chromatography at 4°C, for example, using an FPLC system equipped with a Superdex 75 10/300 column (GE Healthcare).
18.Analyze fractions using SDS-PAGE. For reference include a protein standard, such as SeeBlue™ Pre-stained Protein Standard, and the reference MHC I heavy chain and β2m samples (set aside in step 7). Figure 2 shows a typical FPLC chromatogram and corresponding gel.
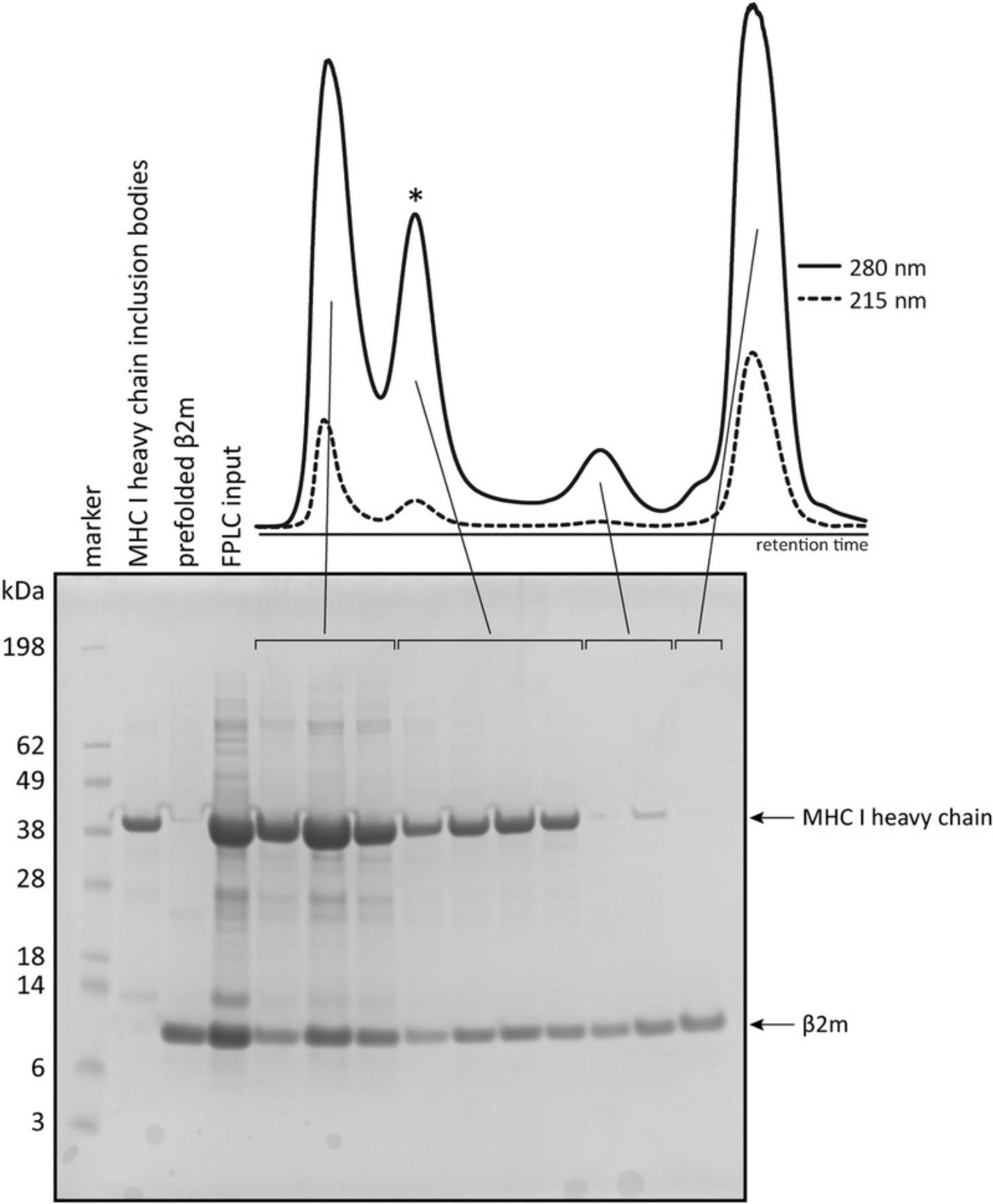
19.Pool fractions that contain pMHC I, and concentrate to 2−5 mg/ml using a 15-ml 30-kDa MWCO centrifugal concentrator (prewashed with Milli-Q water and MHC buffer).
20.Determine the volume of the sample and add glycerol to a final concentration of 15%.
21.Aliquot the sample into PCR tubes or 1.5-ml polypropylene screw cap tubes, depending on the desired volume. Snap-freeze aliquots in liquid nitrogen and store at –80°C.
Basic Protocol 2: CONFIRMING THERMAL EXCHANGE PERFORMANCE OF CONDITIONAL pMHC I MONOMERS BY GEL FILTRATION HPLC
For each batch of conditional pMHC I monomers, the thermal exchange performance should be validated. This protocol describes the use of gel filtration HPLC to confirm exchange at pre-established conditions, but can also be used to test additional exchange times and temperatures. When incubated at a higher temperature without peptide, the pMHC I monomer peak should disappear (Fig. 3, magenta line compared to black line), but in the presence of an exchange peptide the complex is stabilized and the peak should remain visible (Fig. 3, green line). For efficient stabilization the exchange peptide should have a higher affinity for its corresponding MHC I than the template peptide (<4,000 nM for H-2Kb-FAPGNAPAL and <7,288 nM for HLA-A*02:01). A link to an affinity prediction tool is provided in Internet Resources.
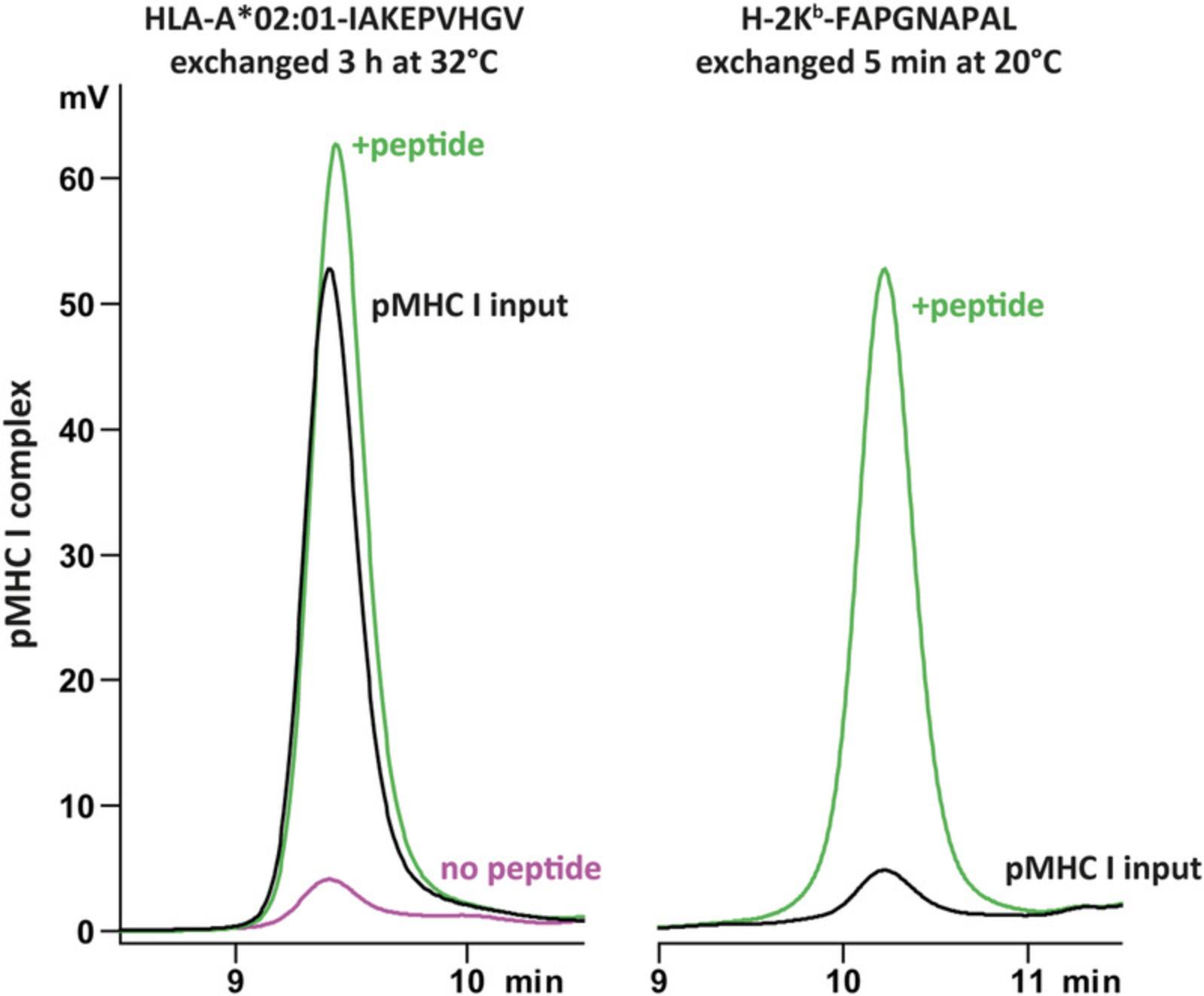
Materials (also see Basic Protocol 1)
-
Conditional pMHC I monomers (Basic Protocol 1)
-
Cold phosphate-buffered saline (PBS, pH 7.4; tablets reconstituted in 500 ml demineralized water; Gibco)
-
10 mM exchange peptide in DMSO, higher affinity than the template peptide (e.g., cytomegalovirus peptide NLVPMVATV for HLA-A*02:01 or ovalbumin peptide SIINFEKL for H-2Kb)
-
HPLC system with gel filtration column (e.g., 300 × 7.8-mm BioSep SEC-s3000; Phenomenex, cat. no. 00H-2146-K0)
-
PCR machine, Thermoblock, or incubator
Testing the stability of pMHC I monomers at room temperature
1.Thaw conditional pMHC I monomers on ice. Typically, a 5-µl aliquot of 2–5 mg/ml pMHC I monomer is enough for four to ten 100-µl injections of 0.5 µM pMHC I monomers.
2.In a 1.5-ml screw-cap microcentrifuge tube, dilute conditional pMHC I monomers to 0.5 µM in PBS.
3.Sediment aggregates by centrifugation for 1 min at 16,000 × g , room temperature.
4.Analyze the sample by HPLC using a gel filtration column, such as a 300 × 7.8-mm BioSep SEC-s3000 column (Phenomenex) with PBS as running buffer.
Analysis of peptide-mediated stabilization of pMHC I monomers post thermal exchange
5.Prepare 0.5 µM pMHC I in PBS containing 50 µM exchange peptide to confirm stabilization of the exchanged complex.
6.Incubate at established exchange conditions in a PCR machine, Thermoblock, or incubator (3 hr at 32°C for HLA-A*02:01; 5 min at room temperature for H-2Kb).
7.Sediment aggregates by centrifugation for 1 min at 16,000 × g , room temperature.
8.Analyze by gel-filtration HPLC.
Thermal dissociation of conditional pMHC I monomers
9.Prepare a 0.5 µM pMHC I solution in PBS.
10.Incubate at established exchange conditions in a PCR machine, Thermoblock, or incubator (3 hr at 32°C for HLA-A*02:01 or 5 min at room temperature for H-2Kb).
11.Sediment aggregates by centrifugation for 1 min at 16,000 × g , room temperature.
12.Analyze by gel-filtration HPLC.
Basic Protocol 3: MULTIMERIZATION OF CONDITIONAL pMHC I MONOMERS
The low affinity of a T cell receptor (TCR) for pMHC I monomers enables sequential activation of multiple T cells by one pMHC in vivo. Through multimeric binding, the avidity of pMHC binding to TCRs becomes sufficiently high to stably label specific CD8+ T cells for visualization and isolation (Davis, Altman, & Newell, 2011). Therefore, pMHC I monomers are biotinylated for multimerization on streptavidin in order to create tetrameric complexes. In addition, labeled streptavidin can be used to incorporate desired fluorophores. Allophycocyanin (APC) and phycoerythrin (PE) are typically used, but other fluorophores have also been successfully used in combination with pMHC I multimers. This protocol describes the preparation of 80 µl pMHC I multimer solution (0.625 µM final), but the reaction can be scaled depending on concentration and volume of pMHC I aliquots.
Materials (also see Basic Protocols 1 and 2)
- Biotinylated conditional pMHC I monomers (Basic Protocol 1)
- 1 mg/ml APC-conjugated streptavidin (SA-APC; Thermo Fisher Scientific, Invitrogen, cat. no. S868) or 1 mg/ml PE-conjugated streptavidin (SA-PE; Thermo Fisher Scientific, Invitrogen, cat. no. S866)
- Cold glycerol
NOTE : Folded complexes will dissociate at elevated temperatures. Keep all solutions and reagents on ice and centrifuges at 4°C!
1.On ice, dilute biotinylated conditional pMHC I monomers in cold PBS to a concentration of 5 µM.
2a. If making APC-labeled multimers: Add 53.6 µl cold PBS to 10 µl of pMHC I monomers.
2b. If making PE-labeled multimers: Add 52.4 µl cold PBS to 10 µl of pMHC I monomers.
3.Add either 1.4 µl SA-APC or 2.6 µl SA-PE. To ensure saturation of all four biotin-binding sites, add streptavidin conjugates stepwise. For example, three 0.47-µl additions of SA-APC or three 0.87-µl additions of SA-PE at 5-min intervals.
4.Add 15 µl cold glycerol and mix well.
5.Aliquot into PCR tubes or 1.5-ml polypropylene screw cap tubes, depending on the desired volume. Snap-freeze aliquots in liquid nitrogen and store at –80°C.
Basic Protocol 4: THERMAL PEPTIDE EXCHANGE ON CONDITIONAL pMHC I MULTIMERS
This protocol describes the thermal exchange of conditional pMHC I multimers for any number of desired peptides in parallel. Conditional multimers are temperature-labile and should be kept on ice until a peptide is added. For efficient stabilization the exchange peptide should have a higher affinity than the template peptide (<4,000 nM for H-2Kb-FAPGNAPAL and <7,288 nM for HLA-A*02:01) for MHC I. One 10 µl aliquot of exchanged multimer is typically enough to stain ten to twenty samples, each containing 1,000,000 peripheral blood mononuclear cells (PBMCs).
Materials (also see Basic Protocols 1 to 3)
- 10 mM exchange peptide(s) stock solution
- Conditional pMHC I multimers (Basic Protocol 3)
1.Dilute 10 mM exchange peptide stock(s) to 250 µM in PBS.
2.Take an 8-µl aliquot of MHC I multimer from freezer and immediately place on ice.
3.Add 2 µl 250 µM exchange peptide solution to frozen 8 µl MHC I multimer. As the mixture thaws, briefly pipette up and down to mix.
4.Briefly spin and incubate exchange reactions in a PCR machine, Thermoblock, or incubator at defined temperature and time to induce exchange.
5.Briefly spin the tubes. The exchanged multimers are now ready for staining of T cells.
Support Protocol 1: BACTERIAL EXPRESSION AND PURIFICATION OF MHC I HEAVY CHAIN AND Β2M INCLUSION BODIES
The procedure for bacterial expression and purification of MHC I heavy chain and β2m inclusion bodies is based on protocols described previously (Garboczi et al., 1992; Rodenko et al., 2006; Toebes et al., 2009). Both proteins can be expressed in parallel following the same steps. This protocol describes expression in 2 L and can be scaled up or down accordingly.
Materials
-
Competent E. coli strain BL21 (DE3) (Novagen, cat. no. 69450)
-
MHC I heavy chain and human β2m expression constructs (see recipe)
-
Liquid LB medium (sterilized, e.g., BD Difco™ LB Broth, cat. no. 244620)
-
Ampicillin (Roche Diagnostics)
-
1 M isopropyl β-D-1-thiogalactopyranoside (IPTG) in de-ionized water
-
Lysis buffer (see recipe)
-
10 mg/ml lysozyme (Roche Diagnostics) in lysis buffer
-
1 M MgCl2 in de-ionized water
-
1 M MnCl2 in de-ionized water
-
10 mg/ml DNase I stock (see recipe)
-
Detergent buffer (see recipe)
-
Wash buffer (see recipe) Triton
-
Incubator (shaking and stationary)
-
LB agar plate containing 50 µg/ml ampicillin
-
Sterile pipette tip
-
Inoculation tube (with foil or cap)
-
Spectrophotometer and cuvettes
-
2-L Erlenmeyer flasks
-
High-speed centrifuge and 250-ml to 1-L buckets
-
Sonicator
Protein expression in E. coli
1.Express MHC I heavy chains and β2m separately. Transform 100 to 200 ng plasmid DNA (MHC I heavy chain or β2m) into 50 to 100 µl competent E. coli cells in a reaction tube for 30 min on ice, 2 min at 42°C, 5 min on ice, respectively.
2.Add 500 µl LB medium and incubate 30 to 60 min at 37°C with shaking.
3.Plate 200 µl of inoculate onto an LB agar plate containing 50 µg/ml ampicillin, and incubate overnight at 37°C.
4.Use sterile pipette tips to select two single colonies from the LB plate, and drop each tip into an inoculation tube containing 10 ml liquid LB medium with 50 µg/ml ampicillin. Cover the tubes loosely with foil or a cap that is not air tight and incubate ∼6 hr at 37°C with shaking to an OD600 of 0.8, and then store at 4°C overnight.
5.Add the 10-ml inoculates to 2 L of liquid LB medium containing 50 µg/ml ampicillin. Divide between four 2-L Erlenmeyer flasks and incubate the cultures at 37°C with shaking to an OD600 of 0.6.
6.Take a 1-ml sample of the culture for SDS-PAGE analysis. Pellet bacteria by centrifugation for 10 min at 12,000 × g , 4°C. Discard supernatant and store pellet at −20°C.
7.Induce protein expression by adding 200 µl 1 M IPTG to each Erlenmeyer flask containing 500 ml E. coli cell culture (final concentration, 0.4 mM IPTG).
8.Incubate ∼4 hr at 37°C with shaking.
9.Take a 0.5-ml sample for SDS-PAGE analysis. Pellet bacteria by centrifugation for 10 min at 12,000 × g , 4°C. Discard supernatant and store pellet at −20°C.
10.Harvest the remainder of the induced bacteria by centrifugation for 15 min at 4,000 × g , 4°C. Suspend the cell pellet(s) in 25 ml lysis buffer per 2-L culture, and transfer the suspension to a 50-ml conical tube. Store suspended cells at −80°C for at least a year or −20°C for a few days.
Isolation and purification of inclusion bodies
11.Thaw the bacteria from 2 L culture.
12.Once the suspension is thawed, add 2.5 ml lysozyme (10 mg/ml in lysis buffer), and incubate 20 min on ice or on a rotator in a cold room.
13.Add the following:
- 275 µl 1 M MgCl2 (10 mM final)
- 27.5 µl 1 M MnCl2 (1 mM final)
- 27.5 µl 10 mg/ml DNase I (10 µg/ml final)
14.Incubate 30 min at room temperature.
15.Sonicate at 50% for 2 min with 20 s on, 20 s off intervals.
16.Centrifuge lysates for 10 min at 12,000 × g , 4°C, and discard the supernatant.
17.Add 25 ml detergent buffer, and sonicate at 30% for 30 s with 10 s on/10 s off intervals.
18.Centrifuge lysate 10 min at 12,000 × g , 4°C and discard the supernatant.
19.Add 20 ml wash buffer, and sonicate at 30% for 30 s with 10 s on/10 s off intervals.
20.Centrifuge lysate 10 min at 12,000 × g , 4°C, and discard the supernatant.
21.Repeat steps 19 and 20 twice.
22.Add 20 ml wash buffer without Triton, and sonicate at 30% for 30 s with 10 s on/10 s off intervals.
23.Centrifuge lysate 10 min at 12,000 × g , 4°C, and discard the supernatant.
24.Repeat steps 22 and 23.
25.Suspend inclusion bodies in 10 ml wash buffer without Triton, and measure the protein concentration, e.g., using the Bradford assay.
26.Prepare desired aliquots, and pellet inclusion bodies by centrifugation for 5 min at 16,000 × g , room temperature, and discard the supernatant.
Support Protocol 2: FOLDING OF HUMAN β2M
Human β2m is used for the production of both human and murine MHC I complexes, because of its higher stability compared to its murine counterpart. Using prefolded β2m for folding of pMHC I ensures stabilization of MHC I and increases folding yields compared to using β2m inclusion bodies.
Materials
- Denaturing buffer (8 M urea/100 mM Tris•Cl, pH 8)
- Purified human β2m inclusion bodies (Support Protocol 1)
- 10 mM Tris•Cl (pH 7) in PBS
- Dialysis tubing (10 kDa MWCO) and large beaker or bucket
1.Suspend pelleted β2m inclusion bodies to 3 mg/ml in freshly-prepared denaturing buffer.
2.Transfer the solution to a dialysis tube, and dialyze overnight against 2 L 10 mM Tris•Cl (pH 7) in PBS at 4°C.
3.The next day, dialyze against two changes of fresh buffer, 4 hr each.
4.Transfer dialyzed, folded β2m to a 1.5-ml reaction tube, and sediment insoluble material by centrifugation for 20 min at 12,000 × g , 4°C.
5.Analyze 10 µl of supernatant and samples from steps 6 and 9 of Support Protocol 1, by SDS-PAGE.
6.Determine the concentration of the folded β2m protein, e.g., using a Bradford assay.
7.Prepare desired aliquots of folded β2m, snap freeze, and store at −80°C.
Support Protocol 3: DETERMINATION OF THE BIOTINYLATION EFFICIENCY
This protocol describes how to use HPLC to determine the degree of biotinylation of MHC I monomers. Each batch of pMHC I monomers and preferably each batch of streptavidin should be tested. MHC I multimers will form by addition of streptavidin, and the height of the monomer peak in the chromatogram will decrease with increasing ratios of streptavidin (Fig. 4). Generally, 90%−95% of pMHC I monomers will be biotinylated. When testing highly unstable complexes, such as H-2Kb-FAPGNAPAL, exchange peptide should be added for stabilization. If samples can be measured on a cooled (4°C) HPLC system, the peptide can be omitted.
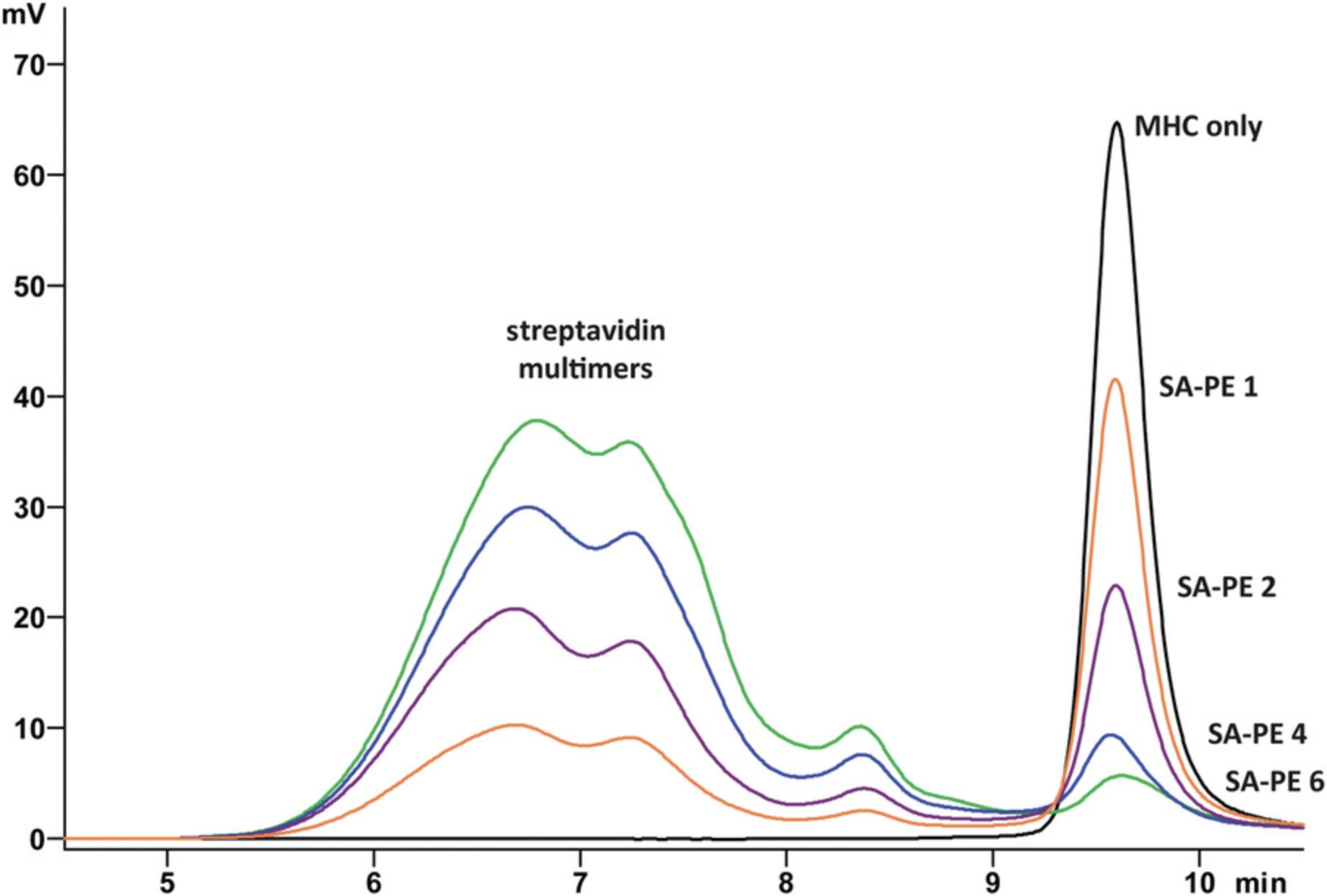
Materials
- Conditional pMHC I monomers (Basic Protocol 1)
- Exchange peptide (of higher affinity than the template peptide; e.g., cytomegalovirus peptide NLVPMVATV for HLA-A*02:01 or ovalbumin peptide SIINFEKL for H-2Kb)
1.Prepare five samples in 1.5-ml Sarstedt tubes to determine the biotinylation efficiency in 100 µl pMHC I monomer solution (0.5 µM). Prepare each sample fresh before analysis.
pMHC I only | SA-PE 1 | SA-PE 2 | SA-PE 4 | SA-PE 6 | |
---|---|---|---|---|---|
PBS | to 100 µl final volume | ||||
exchange peptide | optional; 50 µM final | ||||
pMHC I monomers | 0.5 µM final | ||||
SA-PE (µl) | – | 1 | 2 | 4 | 6 |
2.Incubate the sample on ice in the dark for 5 min to allow all biotinylated monomers to bind.
3.Sediment aggregates by centrifugation for 1 min at 16,000 × g , room temperature.
4.Analyze each sample by HPLC using a gel filtration column (e.g., 300 × 7.8-mm BioSep SEC-s3000 column) with PBS as running buffer.
REAGENTS AND SOLUTIONS
Biotinylation solution (1 ml)
- 40 µl 5 mM D-biotin in 100 mM NaP, pH 7.5 (0.2 mM final concentration)
- 40 µl 0.5 M ATP in 1 M Tris•Cl, pH 9.5 (20 µM final concentration)
- 1.5 µg BirA biotin ligase (commercial sources, such as Avidity)
- 200 µl 10× ligase buffer (50 mM MgCl2 in 0.2 M Tris•Cl, pH 7.5; 10 mM final MgCl2 concentration)
- 80 µl complete EDTA-free Protease Inhibitor Cocktail (1 tablet in 2 ml Milli-Q water)
- 640 µl Milli-Q water
- Prepare fresh
Detergent buffer
- 0.2 M NaCl
- 1% (w/v) sodium deoxycholate monohydrate
- 1% (v/v) Nonidet P-40 substitute
- 20 mM Tris•Cl (pH 7.5)
- 2 mM EDTA
- Store up to one year at room temperature
DNase I stock solution, 10 mg/ml
- 10 mg/ml DNase I (Roche Diagnostics)
- 50% (v/v) glycerol
- 150 mM NaCl
- Store up to 1 year at −20°C
Folding buffer (50 ml)
- 4.2 g L-arginine–HCl (400 mM final concentration)
- 5 ml 1 M Tris•Cl, pH 8 (100 mM final concentration)
- 0.2 ml 0.5 M EDTA (2 mM final concentration)
- 5% (v/v) glycerol
- Adjust to 47 ml with Milli-Q water
- Filter sterilize through a 0.22-µm filter, and store at 4°C for at least a few weeks
Recipe
Immediately before folding reaction, add:
- 76.8 mg or 2.5 ml 100 mM reduced glutathione (5 mM final concentration)
- 16.4 mg or 0.5 ml 50 mM oxidized glutathione (0.5 mM final concentration)
- 0.5 tablet cOmplete EDTA-free Protease Inhibitor Cocktail (Roche Diagnostics)
- Adjust to 50 ml total with Milli-Q water if necessary
Lysis buffer
- 50 mM Tris•Cl (pH 8)
- 25% (w/v) sucrose
- 1 mM EDTA
- Filter sterilize and store at 4°C for up to 1 year
Triton wash buffer
- 50 mM Tris•Cl (pH 8)
- 100 mM NaCl
- 1 mM EDTA (pH 8)
- 0.5% (v/v) Triton X-100
- Filter sterilize, and store at 4°C for up to 1 year
COMMENTARY
Background Information
The ability to distinguish between healthy and infected or mutated cells is crucial for maintaining the balance between immunity and tolerance. This immune recognition is mediated by T cells, the key players of the highly specific adaptive arm of immunity. By displaying peptides derived from intracellular proteins on their surface, all nucleated cells can provide cytotoxic CD8+ T cells with a glimpse of the ongoing processes inside the cell. Upon recognition of a non-self (i.e., viral or mutated) peptide, CD8+ T cells become activated, resulting in proliferation and killing of the target cell. After clearance of the infection or cancer, most CD8+ T cells disappear, but some remain to become memory T cells. The memory response is much faster than the first response and ensures that the infection will be rapidly cleared in case of re-infection with the same pathogen.
The molecules responsible for presentation of intracellular peptides are major histocompatibility complex class I (MHC I) molecules, heterotrimeric complexes that consist of an immunoglobulin (Ig)-like heavy chain, beta-2 microglobulin (β2m) and the peptide that resides in a binding groove formed by two α helices in the heavy chain. MHC I typically binds peptides of 8−10 amino acids that have been processed by proteasomes. These peptides fit in a peptide-binding groove that is closed at two ends, thus fixing the length of peptides. Longer peptides can bind with the two ends of the peptide fixed and the center of the peptide bulging out of the binding groove. Which peptides bind is determined by the interactions between the amino acid side chains of the peptide and the binding pockets present in the MHC's specific peptide-binding groove. The MHC heavy chain is highly polymorphic, which means that many variants exist due to mutation, recombination, and gene conversion. For this reason, MHC I (and MHC II, the other predominant polymorphic protein class) are the major transplantation antigens. Most of the polymorphisms are found in the DNA regions that code for the binding groove and therefore the location and nature of the binding pockets differs between MHC I subtypes. As a consequence each subtype (allele) binds preferred peptide motifs. Every individual has three MHC class I heavy chain genes, named HLA-A, HLA-B, and HLA-C. HLA genes can differ between parents, so each human individual expresses three to six different allotypes that can present different peptide fragments of intracellular pathogens, and thus provide broad protection.
Cytotoxic T cells distinguish between self and non-self peptides through their T cell receptors (TCRs). TCRs are highly diverse and recognize only specific peptide-MHC I (pMHC I) combinations. The frequency of a specific T cell in circulation is typically low if it has never encountered its cognate antigen, and therefore analyzing T cell frequencies in blood or tissue samples provides valuable information on an individual's immune status. In addition, CD8+ T cells are potent targets for immune therapy due to their cytotoxic activity directed only at infected or mutated cells. Characterizing and visualizing CD8+ T cell responses using MHC I multimers enables the study of antigen-specific T cell populations and the efficacy of immune intervention strategies.
To facilitate parallel production of multimers with different specificities a number of peptide exchange technologies have been developed. These methods allow the folding of one large batch of pMHC I monomers and the exchange of the template peptide either by using chemicals or dipeptides as catalysts or by cleaving a UV-labile peptide (Amore et al., 2013; Choo et al., 2014; Rodenko et al., 2009; Saini et al., 2015; Toebes et al., 2006). We have recently developed an exchange technology that does not rely on chemicals or UV, which can damage the protein, but instead uses temperature to induce exchange (Fig. 1; Luimstra et al., 2018). Our method was based on the finding that MHC I on-rates of peptides with various affinities are comparable at a range of temperatures, but off-rates increase with temperature (Garstka et al., 2015). For both HLA-A*02:01 and H-2Kb, we designed template peptides with affinities high enough to promote efficient folding at 4°C, but low enough to dissociate at elevated temperatures. This novel exchange technology is superior to preceding techniques in its potential for peptide exchange on MHC multimers, reducing pre-staining handling time even further.
Critical Parameters and Troubleshooting
The folding buffer is an aqueous solution and therefore template peptides with poor water solubility may precipitate, as may the peptides used for exchange, which is performed in PBS. We therefore recommend to use only peptides from stocks in DMSO, preferably sonicated before use, both for folding and exchange. Filtering the folding buffer removes precipitates, thus increasing folding yields.
The conditional monomers and multimers produced through this protocol are sensitive to elevated temperatures. Thus, folded pMHC I monomers and other reagents should be kept on ice and centrifuges at 4°C. Peptides used for folding should be >99% pure. Since the peptides used for thermal exchange have a low affinity for MHC I, any impurity in the form of a peptide may result in folding of an incorrect complex. Truncated high-affinity peptides may form a stable complex unsuitable for thermal exchange. Undesirable stabilization can be discovered by checking the exchange performance of every batch of pMHC I monomers—prior to multimerization. Likewise, we recommend the degree of biotinylation be determined for every batch of biotinylated pMHC I. Failure to saturate all streptavidin binding sites results in lower order multimers (trimers or even dimers) that may poorly bind TCRs due to lower avidity, which would decrease staining efficiency and increase background signal.
The efficiency of exchange is related to the affinity of the exchange peptide. Lower-affinity peptides are less potent in stabilizing MHC I at elevated temperatures and therefore exchange for low-affinity peptides may be less efficient at the exchange conditions.
Understanding Results
The efficiency of the MHC I folding reaction depends on the folding peptide; in general, higher affinity peptides increase the yield. Since the template peptide should have a low affinity to allow temperature-mediated exchange, folding yields are expected to be low. We have previously observed yields of ∼25%−30% for HLA-A*02:01-IAKEPVHGV (∼800-1000 µg from a 50-ml folding reaction) and ∼2-5% for H-2Kb-FAPGNAPAL (∼70−170 µg from a 50-ml folding reaction).
Time Considerations
Folding, biotinylation, and purification of pMHC I monomers (Basic Protocol 1) takes 5−6 days, of which 3−4 days are merely incubation time and can be spent otherwise. Monomers can be stored in at –80°C until tested (Basic Protocol 2) or multimerized (Basic Protocol 3), which takes little time. This is not different from conventional MHC I multimer production. Peptide exchange (Basic Protocol 4) takes only minutes for multimers of H-2Kb-FAPGNAPAL and 3 hr for multimers of HLA-A*02:01-IAKEPVHGV. Bacterial expression of MHC I heavy chains and β2m (Support Protocol 1) takes about a week, including folding of β2m (Support Protocol 2). These procedures need only be executed occasionally, since large batches can be produced and stored for later use. Determining the biotinylation efficiency (Support Protocol 3) takes a few hours, depending on the HPLC system and column used.
Acknowledgments
The authors would like to thank Veerle M. Luimstra for critical reading of the manuscript. This work was supported by a grant from the Institute for Chemical Immunology (to H. Ovaa and J. Neefjes) with funds from Nederlandse Organisatie voor Wetenschappelijk Onderzoek/Dutch Research Council; and by grants from the Second Affiliated Hospital of Xi’an Jiaotong University and National Natural Science Foundation of China (grant numbers 81700691 and 81870536 to M.A. Garstka). This work is part of the Oncode Institute, which is partly financed by the Dutch Cancer Society.
Conflicts of Interest
J.J. Luimstra, M.A. Garstka, J. Neefjes, and H. Ovaa are listed as inventors on an international patent (WO/2019/083370 A1, Methods for producing a MHC multimer) owned by Stichting Het Nederlands Kanker Instituut-Antoni van Leeuwenhoek Ziekenhuis and Leids Universitair Medisch Centrum.
Literature Cited
- Altman, J. D., Moss, P. A., Goulder, P. J., Barouch, D. H., McHeyzer-Williams, M. G., Bell, J. I., … Davis, M. M. (1996). Phenotypic analysis of antigen-specific T lymphocytes. Science , 274, 5284, 94–96.
- Amore, A., Wals, K., Koekoek, E., Hoppes, R., Toebes, M., Schumacher, T. N., … Ovaa, H. (2013). Development of a hypersensitive periodate-cleavable amino acid that is methionine- and disulfide-compatible and its application in MHC exchange reagents for T cell characterisation. Chembiochem , 14(1), 123–131. doi: 10.1002/cbic.201200540.
- Bentzen, A. K., Marquard, A. M., Lyngaa, R., Saini, S. K., Ramskov, S., Donia, M., … Hadrup, S. R. (2016). Large-scale detection of antigen-specific T cells using peptide-MHC-I multimers labeled with DNA barcodes. Nature Biotechnology , 34(10), 1037–1045. doi: 10.1038/nbt.3662.
- Choo, J. A., Thong, S. Y., Yap, J., van Esch, W. J., Raida, M., Meijers, R., … Grotenbreg, G. M. (2014). Bioorthogonal cleavage and exchange of major histocompatibility complex ligands by employing azobenzene-containing peptides. Angewandte Chemie , 53(49), 13390–13394. doi: 10.1002/anie.201406295.
- Davis, M. M., Altman, J. D., & Newell, E. W. (2011). Interrogating the repertoire: Broadening the scope of peptide-MHC multimer analysis. Nature Reviews Immunology , 11(8), 551–558. doi: 10.1038/nri3020.
- Garboczi, D. N., Hung, D. T., & Wiley, D. C. (1992). HLA-A2-peptide complexes: Refolding and crystallization of molecules expressed in Escherichia coli and complexed with single antigenic peptides. Proceedings of the National Academy of Sciences of the United States of America , 89(8), 3429–3433.
- Garstka, M. A., Fish, A., Celie, P. H. N., Joosten, R. P., Janssen, G. M. C., Berlin, I., … Neefjes, J. (2015). The first step of peptide selection in antigen presentation by MHC class I molecules. Proceedings of the National Academy of Sciences of the United States of America , 112(5), 1505–1510. doi: 10.1073/pnas.1416543112.
- Gallagher, S. R. (2006). One-dimensional SDS gel electrophoresis of proteins. Current Protocols in Immunology , 75, 8.4.1–8.4.37. doi:10.1002/0471142735.im0804s75.
- Hadrup, S. R., Bakker, A. H., Shu, C. J., Andersen, R. S., van Veluw, J., Hombrink, P., … Schumacher, T. N. (2009). Parallel detection of antigen-specific T-cell responses by multidimensional encoding of MHC multimers. Nature Methods , 6(7), 520–526. doi: 10.1038/nmeth.1345.
- Hagel, L. (1998). Gel-filtration chromatography. Current Protocols in Molecular Biology , 44, 10.9.1–10.9.2. doi:10.1002/0471142727.mb1009s44.
- Ljunggren, H. G., Stam, N. J., Ohlen, C., Neefjes, J. J., Hoglund, P., Heemels, M. T., … Ploegh, H. L. (1990). Empty MHC class I molecules come out in the cold. Nature , 346(6283), 476–480. doi: 10.1038/346476a0.
- Luimstra, J. J., Garstka, M. A., Roex, M. C. J., Redeker, A., Janssen, G. M. C., van Veelen, P. A., … Ovaa, H. (2018). A flexible MHC class I multimer loading system for large-scale detection of antigen-specific T cells. Journal of Experimental Medicine , 215(5), 1493−1504. doi: 10.1084/jem.20180156.
- Newell, E. W., Sigal, N., Nair, N., Kidd, B. A., Greenberg, H. B., & Davis, M. M. (2013). Combinatorial tetramer staining and mass cytometry analysis facilitate T-cell epitope mapping and characterization. Nature Biotechnology , 31(7), 623–629. doi: 10.1038/nbt.2593.
- Rodenko, B., Toebes, M., Celie, P. H., Perrakis, A., Schumacher, T. N., & Ovaa, H. (2009). Class I major histocompatibility complexes loaded by a periodate trigger. Journal of the American Chemical Society , 131(34), 12305–12313. doi: 10.1021/ja9037565.
- Rodenko, B., Toebes, M., Hadrup, S. R., van Esch, W. J., Molenaar, A. M., Schumacher, T. N., & Ovaa, H. (2006). Generation of peptide-MHC class I complexes through UV-mediated ligand exchange. Nature Protocols , 1(3), 1120–1132. doi: 10.1038/nprot.2006.121.
- Saini, S. K., Schuster, H., Ramnarayan, V. R., Rammensee, H. G., Stevanovic, S., & Springer, S. (2015). Dipeptides catalyze rapid peptide exchange on MHC class I molecules. Proceedings of the National Academy of Sciences of the United States of America , 112(1), 202–207. doi: 10.1073/pnas.1418690112.
- Sasse, J., & Gallagher, S. R. (2003). Staining Proteins in Gels. Current Protocols in Immunology , 58, 8.9.1–8.9.25. doi:10.1002/0471142735.im0809s58.
- Toebes, M., Coccoris, M., Bins, A., Rodenko, B., Gomez, R., Nieuwkoop, N. J., … Schumacher, T. N. (2006). Design and use of conditional MHC class I ligands. Nature Medicine , 12(2), 246–251. doi: 10.1038/nm1360.
- Toebes, M., Rodenko, B., Ovaa, H., & Schumacher, T. N. (2009). Generation of peptide MHC class I monomers and multimers through ligand exchange. Current Protocols in Immunology , 87(1) 18.16.1−18.16.20. doi: 10.1002/0471142735.im1816s87.
Key Reference
- Luimstra, J. J. et al. (2018). See above.
Describes the development of temperature-mediated peptide exchange on HLA-A*02:01 and H-2Kb.
Internet Resources
Web site used to predict peptide-MHC I binding affinities.
Citing Literature
Number of times cited according to CrossRef: 11
- Marten Meyer, Christina Parpoulas, Titouan Barthélémy, Jonas P. Becker, Pornpimol Charoentong, Yanhong Lyu, Selina Börsig, Nadja Bulbuc, Claudia Tessmer, Lisa Weinacht, David Ibberson, Patrick Schmidt, Rüdiger Pipkorn, Stefan B. Eichmüller, Peter Steinberger, Katharina Lindner, Isabel Poschke, Michael Platten, Stefan Fröhling, Angelika B. Riemer, Jessica C. Hassel, Maria Paula Roberti, Dirk Jäger, Inka Zörnig, Frank Momburg, MediMer: a versatile do-it-yourself peptide-receptive MHC class I multimer platform for tumor neoantigen-specific T cell detection, Frontiers in Immunology, 10.3389/fimmu.2023.1294565, 14 , (2024).
- Lea Weiss, René Classens, Marjolein Schluck, Emilia Grad, Yusuf Dölen, Lieke van der Woude, Dominique van Midden, Lisa Maassen, Kiek Verrijp, Koen van Riessen, Eric van Dinther, Philipp M. Hagemann, Carl G. Figdor, Roel Hammink, Immunofilaments Are Well Tolerated after Local or Systemic Administration in Mice, ACS Pharmacology & Translational Science, 10.1021/acsptsci.4c00180, 7 , 6, (1874-1883), (2024).
- Lea Weiss, Jorieke Weiden, Yusuf Dölen, Emilia M. Grad, Eric A. W. van Dinther, Marjolein Schluck, Loek J. Eggermont, Guido van Mierlo, Uzi Gileadi, Ariadna Bartoló-Ibars, René Raavé, Mark A. J. Gorris, Lisa Maassen, Kiek Verrijp, Michael Valente, Bart Deplancke, Martijn Verdoes, Daniel Benitez-Ribas, Sandra Heskamp, Annemiek B. van Spriel, Carl G. Figdor, Roel Hammink, Direct In Vivo Activation of T Cells with Nanosized Immunofilaments Inhibits Tumor Growth and Metastasis, ACS Nano, 10.1021/acsnano.2c11884, 17 , 13, (12101-12117), (2023).
- Lotte Gerrits, Lea Weiss, Emilia M. Grad, Marjolein Schluck, Lisa Maassen, René Classens, Chadia Archidi, Martijn Verdoes, Carl G. Figdor, Paul H. J. Kouwer, Roel Hammink, Semi‐Flexible Immunobrushes Facilitate Effective and Selective Expansion of Antigen‐Specific T Cells, Advanced Functional Materials, 10.1002/adfm.202307606, 34 , 14, (2023).
- Fiamma Berner, David Bomze, Christa Lichtensteiger, Vincent Walter, Rebekka Niederer, Omar Hasan Ali, Nina Wyss, Jens Bauer, Lena Katharina Freudenmann, Ana Marcu, Eva-Maria Wolfschmitt, Sebastian Haen, Thorben Gross, Marie-Therese Abdou, Stefan Diem, Stella Knöpfli, Tobias Sinnberg, Kathrin Hofmeister, Hung-Wei Cheng, Marieta Toma, Niklas Klümper, Mette-Triin Purde, Oltin Tiberiu Pop, Ann-Kristin Jochum, Steve Pascolo, Markus Joerger, Martin Früh, Wolfram Jochum, Hans-Georg Rammensee, Heinz Läubli, Michael Hölzel, Jacques Neefjes, Juliane Walz, Lukas Flatz, Autoreactive napsin A–specific T cells are enriched in lung tumors and inflammatory lung lesions during immune checkpoint blockade, Science Immunology, 10.1126/sciimmunol.abn9644, 7 , 75, (2022).
- Paula Ruibal, Ian Derksen, Marjolein Wolfswinkel, Linda Voogd, Kees L. M. C. Franken, Angela F. El Hebieshy, Thorbald Hall, Tom A. W. Schoufour, Ruud H. Wijdeven, Tom H. M. Ottenhoff, Ferenc A. Scheeren, Simone A. Joosten, Thermal‐exchange HLA‐E multimers reveal specificity in HLA‐E and NKG2A/CD94 complex interactions, Immunology, 10.1111/imm.13591, 168 , 3, (526-537), (2022).
- Paula Ruibal, Kees L. M. C. Franken, Krista E. van Meijgaarden, Lucy C. Walters, Andrew J. McMichael, Geraldine M. Gillespie, Simone A. Joosten, Tom H. M. Ottenhoff, Discovery of HLA-E-Presented Epitopes: MHC-E/Peptide Binding and T-Cell Recognition, T-Cell Repertoire Characterization, 10.1007/978-1-0716-2712-9_2, (15-30), (2022).
- Juliette Vaurs, Gaël Douchin, Klara Echasserieau, Romain Oger, Nicolas Jouand, Agnès Fortun, Leslie Hesnard, Mikaël Croyal, Frédéric Pecorari, Nadine Gervois, Karine Bernardeau, A novel and efficient approach to high-throughput production of HLA-E/peptide monomer for T-cell epitope screening, Scientific Reports, 10.1038/s41598-021-96560-9, 11 , 1, (2021).
- Muhammed A Rahman, Kenji Murata, Brian D Burt, Naoto Hirano, Changing the landscape of tumor immunology: novel tools to examine T cell specificity, Current Opinion in Immunology, 10.1016/j.coi.2020.11.003, 69 , (1-9), (2021).
- Jackwee Lim, Destabilizing single chain major histocompatibility complex class I protein for repurposed enterokinase proteolysis, Scientific Reports, 10.1038/s41598-020-71785-2, 10 , 1, (2020).
- Edyta Wieczorek, Malgorzata A. Garstka, Recurrent bladder cancer in aging societies: Importance of major histocompatibility complex class I antigen presentation, International Journal of Cancer, 10.1002/ijc.33359, 148 , 8, (1808-1820), (2020).