Preparation and Compositional Analysis of Lignocellulosic Plant Biomass as a Precursor for Food Production During Food Crises
Tara N. Mather, Tara N. Mather, Niroshan Siva, Niroshan Siva, Marjorie Jauregui, Marjorie Jauregui, Hannah Klatte, Hannah Klatte, Joshua D. Lambert, Joshua D. Lambert, Charles T. Anderson, Charles T. Anderson
compositional analysis
emergency foods
lignocellulosic biomass
plant cell wall
post-catastrophic foods
resilient foods
Abstract
In the event of a sunlight-blocking, temperature-lowering global catastrophe, such as a global nuclear war, super-volcano eruption or large asteroid strike, normal agricultural practices would be severely disrupted with a devastating impact on the global food supply. Despite the improbability of such an occurrence, it is prudent to consider how to sustain the surviving population following a global catastrophe until normal weather and climate patterns resume. Additionally, the ongoing challenges posed by climate change, droughts, flooding, soil salinization, and famine highlight the importance of developing food systems with resilient inputs such as lignocellulosic biomass. With its high proportion of cellulose, the abundant lignocellulosic biomass found across the Earth's land surfaces could be a source of energy and nutrition, but it would first need to be converted into foods. To understand the potential of lignocellulosic biomass to provide energy and nutrition to humans in post-catastrophic and other food crisis scenarios, compositional analyses should be completed to gauge the amount of energy (soluble sugars) and other macronutrients (protein and lipids) that might be available and the level of difficulty in extracting them. Suitable preparation of the lignocellulosic biomass is critical to achieve consistent and comparable results from these analyses. Here we describe a compilation of protocols to prepare lignocellulosic biomass and analyze its composition to understand its potential as a precursor to produce post-catastrophic foods which are those that could be foraged, grown, or produced under the new climate conditions to supplement reduced availability of traditional foods. These foods have sometimes been referred to in the literature as emergency, alternate, or resilient foods. © 2024 The Authors. Current Protocols published by Wiley Periodicals LLC.
Basic Protocol 1 : Convection oven drying (1 to 2 days)
Alternate Protocol 1 : Air-drying (2 to 3 days)
Alternate Protocol 2 : Lyophilization (1 to 4 days)
Support Protocol 1 : Milling plant biomass
Support Protocol 2 : Measuring moisture content
Basic Protocol 2 : Cellulose determination
Basic Protocol 3 : Lignin determination
Basic Protocol 4 : Crude protein content by total nitrogen
Basic Protocol 5 : Crude fat determination via soxtec extraction system
Basic Protocol 6 : Sugars by HPLC
Basic Protocol 7 : Ash content
INTRODUCTION
The following protocols detail the steps involved in the preparation and analysis of lignocellulosic biomass (hereafter, biomass) for cell wall polysaccharides and other components. Although versions of several of these protocols have been previously documented in the literature, this compilation provides a streamlined approach to processing and evaluating biomass through the lens of potential for conversion to food, particularly in a global, post-catastrophic scenario, such as a nuclear winter with conditions of diminished sunlight, temperature, and humidity. While such a scenario is improbable, it remains prudent to consider how we could feed the surviving population without relying on conventional agriculture, instead harvesting nutrition from existing biological resources. Plant biomass is plentiful across the Earth's land surfaces, translating into ∼450 Gt of carbon (Bar-On et al., 2018). If about half of the carbon in this biomass is embodied in the form of cellulose, which is composed of glucose, as part of the plant cell wall (Bengtsson et al., 2020), then there would be sufficient lignocellulosic biomass available for conversion to foods to sustain humans for many decades, even at a conservative conversion efficiency of 30% for all nutrients, assuming that the cellulose, which cannot be digested by humans, can be deconstructed to simple sugars (Siva & Anderson, 2023). It should be noted that lignocellulosic biomass-derived foods would also have utility in a variety of other challenging scenarios, such as climate change, famine, floods, droughts, regional wars, and mass migrations, that create food crises.
To evaluate and compare the potential of different lignocellulosic precursors to generate food, their macromolecular compositions should be determined for later correlation with the nutrient content of the derived foods. To allow meaningful evaluation of lignocellulosic precursors, the first step is to prepare samples in a uniform, consistent manner so that subsequent analyses will yield comparable results. Critical parameters for prepared lignocellulosic biomass are water content and particle size distribution. Basic Protocol 1 describes the steps required to convert lignocellulosic biomass, which could be in the initial form of wood chips, grasses, leaves, etc., to a powdered material with <10% moisture content and a particle size distribution ranging from ∼170 to 840 µm (80 to 20 mesh). Basic Protocols 2 and 3 detail how to determine the cellulose and lignin contents of lignocellulosic biomass, respectively. Basic Protocol 4 describes the estimation of crude protein in lignocellulosic biomass by first determining the total nitrogen content. Basic Protocol 5 details how to determine lipid content in a biomass sample through a Soxhlet extraction process. Basic Protocol 6 describes analysis of individual sugars by high performance liquid chromatography (HPLC), and Basic Protocol 7 details the procedure for determining ash content in lignocellulosic biomass as a proxy for mineral content.
STRATEGIC PLANNING
Preparation of Lignocellulosic Biomass for Compositional Analysis
Before most compositional analyses can be performed, biomass particle size must be reduced to allow for handling and to increase surface area to promote the pertinent chemical reactions. Because the preparation method can influence subsequent analytical results, it is important to prepare biomass samples in a consistent manner so that results are comparable.
Collection and fragmentation of plant biomass
In a post-catastrophic scenario, resources would be highly limited in some areas, and supply chains and transportation would be disrupted. Collecting biomass from fields could be accomplished using agricultural harvesting equipment if available. In that case, it would likely be converted to chips or fragments that would be suitable for further particle size reduction. If typical agricultural equipment were unavailable, hand-gathering using available vehicles, tools, and collection containers would be necessary. From a research perspective, lignocellulosic biomass, e.g., willow chips, can be obtained through agricultural research plots where plants are grown at large scales (Montes et al., 2021). Other potential sources of lignocellulosic biomass for research include harvesting (with permission) from public or private lands.
Drying plant biomass
Proper sample preparation is important to convert a variety of plant biomass types into a uniform material suitable for chemical analysis. This preparation also allows plant biomass to be stored for a year or longer without microbial degradation and allows the material to be milled efficiently to further reduce particle size. The drying protocols discussed here are developed according to the National Renewable Energy Laboratory report (NREL/TP-510-42620) (Hames et al., 2008) and include three drying methods that can be used according to the sample size and type. From a research perspective, we prefer convection oven drying because it allows for faster drying than air-drying or lyophilization. Also, convection ovens are broadly available in research settings.
In much of our research utilizing these protocols, willow wood was used as a source of lignocellulosic biomass. Regardless of substrate type, record details such as the species and strain (if applicable) of biomass, as well as the location at which the biomass was grown. It is also important to note any relevant growth conditions, such as unusual weather patterns including abnormal heat or drought. Lastly, consider the time of year during which the biomass was harvested, as the composition of plant biomass may vary seasonally. (Roni et al., 2023)
Basic Protocol 1: CONVECTION OVEN DRYING (1 TO 2 DAYS)
This procedure can be used for very wet samples. It is well-suited for a variety of sample quantities depending on the number and sizes of ovens available. Advantages of this method are faster drying than air drying or lyophilization and the broad availability of convention ovens in research settings. Biomass pieces should be roughly <2-cm long, <0.5-cm wide, and <0.5-cm thick to allow for complete drying.
Materials
- Convection oven, 45° to 60° ± 3°C
- Moisture can (aluminum pan with tight-fitting lid), 51-mm to 89-mm diameter (e.g., Humboldt, cat. no. H-4927)
- Desiccator (e.g., VWR, cat. no. 75871-662)
- Desiccant (e.g., Drierite Indicating, cat. no. 470300-992)
- Balance, sensitive to 0.1 g
- Airtight container (e.g., Ziploc bag or Tri-Seal containers, Electron Microscopy Sciences, cat. no. 64235)
1.Turn on the oven and set to a temperature in the range of 45° to 60°C.
2.Select a heat-resistant, open container (e.g., an aluminum moisture can without its lid) and keep it inside the oven for at least 3 hr.
3.Place the container in a desiccator containing desiccant and allow it to cool to room temperature.
4.Weigh the container to the nearest 0.1 g and record this weight as Wt.
5.Place the biomass material into the dried container to a maximum depth of 1 cm.
6.Weigh the container plus biomass to the nearest 0.1 g and record this weight as Wi.
7.Place the container and biomass in a drying oven, maintaining the desired temperature, and dry for 24 hr.
8.Remove the container plus biomass from the drying oven, place in a desiccator and allow the sample to cool to room temperature.
9.Weigh the container and biomass to the nearest 0.1 g and record this weight as Wf.
10.Return the container and biomass to the drying oven at the desired temperature for 1 hr.
11.Remove the container and biomass from the drying oven, place in a desiccator and allow the sample to cool to room temperature.
12.Weigh container and biomass to the nearest 0.1 g and record this weight.
13.Repeat steps 10 to 12 until the change in the weight of the sample is <1% in 1 hr.
14.Store the samples in an airtight container.
Alternate Protocol 1: AIR-DRYING (2 TO 3 DAYS)
This procedure is well-suited for drying larger quantities of biomass (>20 g). Ambient humidity must be low enough to allow the samples to dry to a constant weight in a reasonable amount of time without spoiling. While there are no definitive ambient humidity criteria, ≤40% relative humidity should allow for air-drying of biomass samples. Use Basic Protocol 1 if unsure whether ambient humidity conditions will allow sufficient air-drying.
Additional Materials (also see Basic Protocol 1)
- Large table or drying rack
1.Cut the samples to dimensions less than 5 × 5 × 0.6–cm. Stems or twigs should not exceed 0.6 cm in diameter to allow for efficient grinding later.
2.Spread out the biomass on a surface, e.g., large table or drying rack, that allows for airflow over the biomass. Do not pile the material deeper than 15 cm.
3.Turn the material at least once per day to ensure even drying and prevent microbial growth.
4.Allow the material to air dry until the change in the weight of the sample is <1% in 24 hr.
5.Store the samples in an airtight container.
Alternate Protocol 2: LYOPHILIZATION (1 TO 4 DAYS)
This procedure can be used for very wet biomass that is at risk for microbial growth during drying, wet pretreated biomass, and samples that would not be stable during prolonged exposure to ambient conditions. This procedure is also suitable for materials that are heat sensitive and would degrade if subjected to Basic Protocol 1.This drying method is suitable for small samples of biomass (<20 g).
Additional Materials (also see Basic Protocol 1)
- Containers for freeze-drying (e.g., zipper pouches, Uline, cat. no. S-22820, or 50-ml Falcon tubes, Fisher Scientific, cat. no. 14-432-22)
- Parafilm
- Balance, sensitive to 0.01 g
- Freeze-dryer system with vacuum chamber and pump capable of maintaining a pressure of <133 Pa (0.02 psi) and a cold finger in the chamber capable of maintaining a temperature of −50°C (biomass drying procedure 3)
1.Weigh a suitable freeze-dryer container to the nearest 0.1 g and record this weight as Wt.
2.Place the biomass in the container. Do not fill the container more than half full.
3.Weigh the container and biomass to the nearest 0.1 g and record this weight as Wi.
4.Cover the sample container with parafilm to prevent biomass from being sucked into the freeze-dryer chamber.
5.Freeze the sample in its container overnight.
6.The next day, quickly transfer the frozen sample to the freeze-dryer. The collector should be set to (or achieve) −45°C and the vacuum should be set to 276 Pa (0.04 psi).
7.Allow the biomass to dry until all visible traces of ice and frost are gone from the sample.
8.Remove the sample from the freeze-dryer, quickly weigh it the nearest 0.01 g, and return it to the freeze-dryer.
9.Allow the material to air dry until the change in the weight of the sample is <1% in 24 hr.
10.Once the sample has reached a constant weight, allow it to warm to room temperature and then store in an airtight container.
Support Protocol 1: MILLING PLANT BIOMASS
Milling or grinding plant biomass after drying has many benefits for chemical analysis. Grinding decreases particle size, hence increasing surface area, which improves chemical extraction efficiency. Additionally, grinding increases homogeneity; however, it can also lead to contamination, increased moisture, and evaporation/alteration of volatile/labile constituents. This procedure is developed according to the National Renewable Energy Laboratory report (NREL/TP-510-42620) (Hames et al., 2008).
Materials
- Standard laboratory knife mill with 1- to 2-mm screens (e.g., Thomas Scientific Wiley Model 4 or Wiley Mini-Mill), or equivalent
- Sieve set [e.g., sNo. 20 (850 µm) and 04-881-10W No. 80 (180 µm)]
- Sieve shaker that provides motion in both horizontal and vertical axes for optional sieving step, optional
- Airtight container (e.g., Ziploc bag or Tri-Seal containers, Electron Microscopy Sciences, cat. no. 64235)
1.Select an appropriate knife mill.
2.Feed the dried biomass into a knife mill such that the mill does not slow down or become jammed.
3.Sieving is an optional step that can be used either to separate particles of different sizes for further processing or to analyze the particle size distribution for a given biomass sample. If homogeneous particle size is critical for an experiment, use a desired screen size and sieve your sample.
4.Store milled and sieved samples in an airtight container (e.g., sealable polyethylene bag) and keep at −20°C until needed.
Figure 1 shows willow chips before and after grinding.
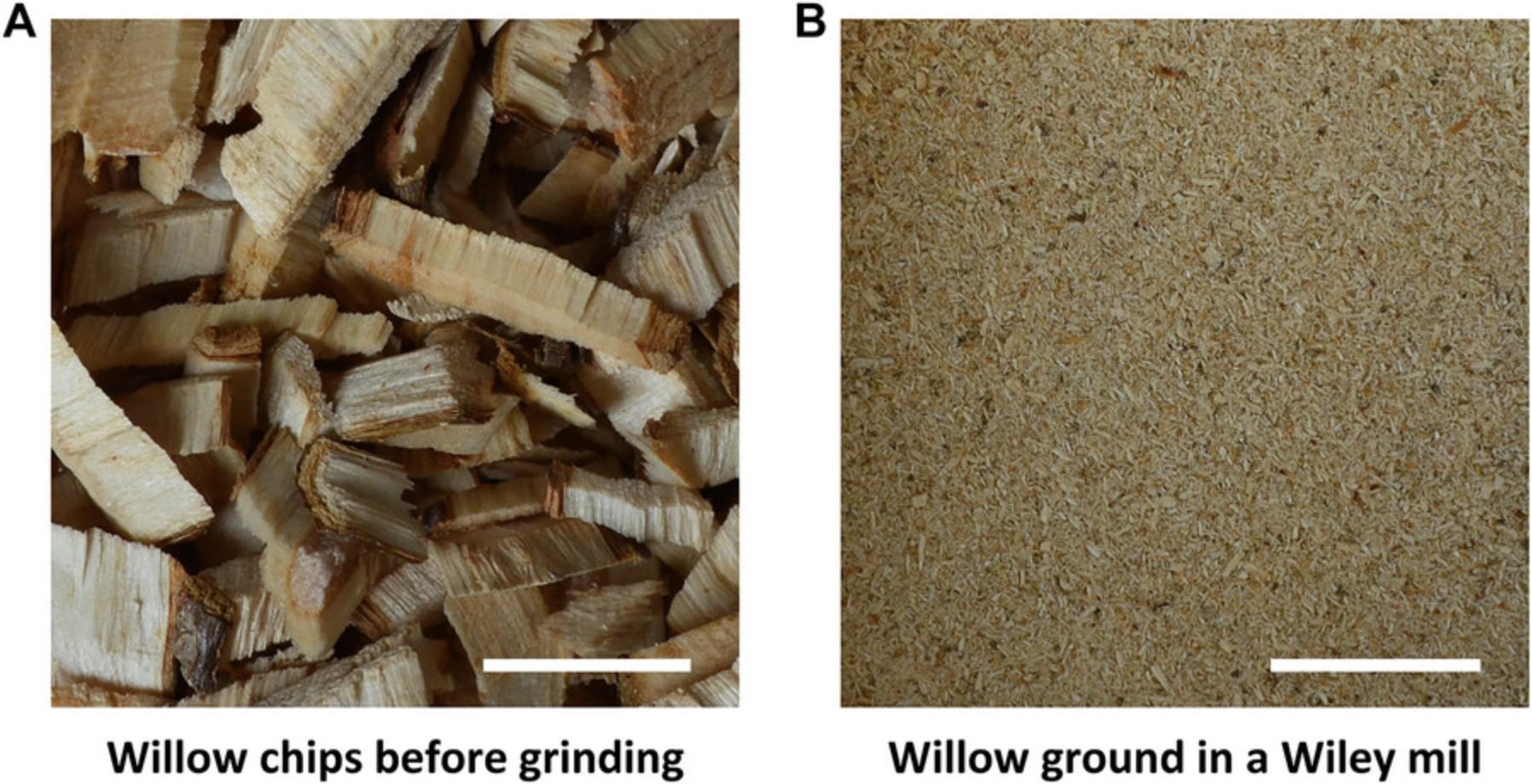
Support Protocol 2: MEASURING MOISTURE CONTENT
The moisture content of plant biomass refers to how much water is present in the biomass. Moisture content can be calculated on a dry basis (db) or wet basis (wb) and is typically expressed as a percentage. Measuring moisture content is useful to normalize the solid biomass of the samples and compare the concentrations of nutrients or other chemical compounds among different biomass types (Fontana & Carter, 2020).
Additional Materials (also see Basic Protocol 1)
- Lab tissue wipers (e.g., VWR, cat. no. 82003-820)
- Isotropic oven, 100° to 150°C
1.Clean a moisture can using lab tissue.
2.Measure the weight of the moisture can to the nearest 0.01 g (Wc).
3.In triplicate, transfer 3 to 5 g of ground biomass to a moisture can and record the weight in grams to 0.01 g (Wi).
4.Dry the sample in a preheated oven at 105°C for 1 hr (Sluiter et al., 2008).
5.Transfer the sample to a desiccator to cool to room temperature.
6.Measure the weight of the dried sample plus the moisture can in grams to the nearest 0.01 g (Wc+d).
7.Repeat Steps 4 to 6 at 1 hr intervals until the Wc+d does not change >1% between sequential measurements.
8.Calculate the moisture content as follows:
Where:
- MCdb % = percent moisture content on a dry basis
- Wc = tare weight of container
- Wi = initial weight of biomass
- Wc+d = final weight of container and dry biomass.
A sample graph showing dry weight changes over time is given below in Figure 2.
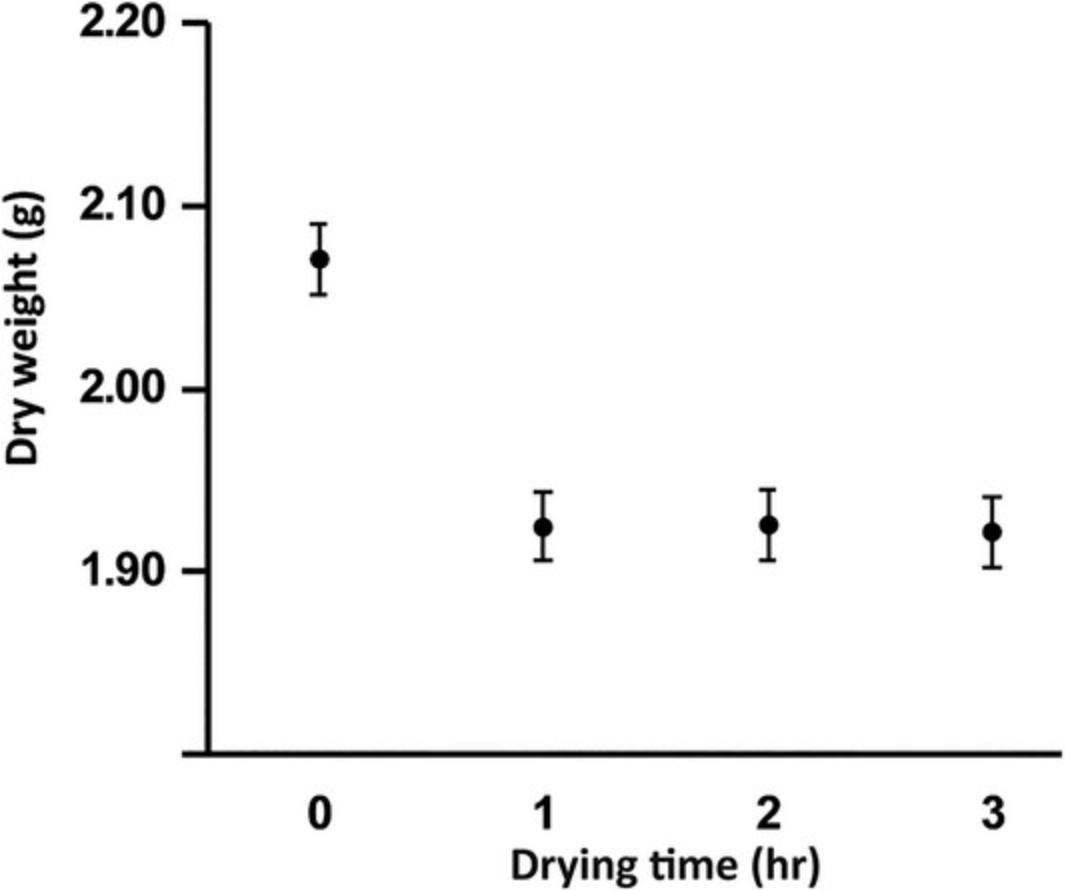
Basic Protocol 2: CELLULOSE DETERMINATION
Cellulose contains much of the glucose in lignocellulosic biomass. Together with hemicellulose and lignin, cellulose forms a complex structural assemblage of polymers that is naturally recalcitrant to degradation, but due to its partially crystalline structure it represents a particularly recalcitrant fraction of that biomass. This procedure, based on a standard protocol for measuring crystalline cellulose content (Updegraff, 1969), is described here for woody biomass or grass samples. Dried, ground biomass is obtained, or prepared according to the protocols above, and digested with a mixture of acetic and nitric acid to extract hemicellulose and lignin. In the same tube, concentrated sulfuric acid is then added to hydrolyze the remaining crystalline cellulose into glucose monomers. Standard solutions of known glucose concentration are prepared and measured via a colorimetric anthrone assay to yield a regression line of glucose concentration as a function of absorbance at 620 nm. The absorbance values of hydrolyzed samples are then measured, and the regression line is used to calculate the glucose concentration. Finally, the weight percent of cellulose in the original biomass can be calculated on a dry weight basis from the glucose concentration of the hydrolyzed samples.
Materials
-
Lignocellulosic biomass or cell wall preparation of choice
-
Cellulose positive control (e.g., Avicel, Sigma-Aldrich, cat. no. 11365-1KG)
-
Acetic acid/water/nitric acid mixture (see recipe)
-
H2O, ultrapure, 18.2 MΩ.cm (e.g., Milli-Q water, Millipore)
-
Sulfuric acid, 72% w/w aqueous solution (Thermo Fisher Scientific, cat. no. 045596.K2)
-
1 mg/ml glucose stock solution (see recipe)
-
Anthrone reagent (see recipe)
-
Analytical balance (Mettler Toledo, Excellence Series)
-
9-ml round bottom glass centrifuge tubes, 13-mm × 100-mm, with screwcaps (Pyrex, cat. no. 9826-13)
-
Personal protective equipment (PPE) including safety glasses, lab coat, and gloves
-
Water bath for boiling samples
-
Timer
-
Vortex mixer
-
Ice bucket
-
Centrifuge (Eppendorf 5804R with rotor, VWR cat. no. 97007-370, or equivalent)
-
Glass pipette
-
10-ml volumetric flask
-
NanoDrop 2000C spectrophotometer (Thermo Fisher Scientific, model ND-2000C-PC) or other UV-Vis spectrophotometer
-
2-ml centrifuge tubes with screwcaps (e.g., Sarstedt, cat. no. 72.693)
-
Quartz cuvette, 0.7-ml volume (Sigma-Aldrich, cat. no. Z600199)
CAUTION : Sulfuric acid is a strong acid; wear appropriate PPE and work in a fume hood.
Preparation and digestion of samples
1.Measure 30 mg biomass or 15 mg Avicel into a 9-ml round bottom glass centrifuge tube with screw cap (3 to 5 replicates for each sample type).
2.Complete an initial digestion of the sample by adding 3 ml acetic acid/water/nitric acid mixture.
3.Heat the suspension in a boiling water bath for 30 min with mixing every 5 to 10 min. Start the timer when you place the tubes in the water bath. Every 5 to 10 min, pause the timer, remove each tube and vortex for 3 s. Resume the timer after you place the tubes back in the water bath.
4.Cool the sample to room temperature using an ice bath.
5.Centrifuge the sample 3 min at 2500 × g , room temperature.
6.Using a glass pipette, carefully transfer the supernatant into a chemical waste container.
7.Resuspend the pellet thoroughly in 5 ml of ultrapure water by vortexing, centrifuge 3 min at 2500 × g , room temperature, and carefully discard the supernatant.
8.Repeat Step 7 to further wash the pellet.
9.Hydrolyze the pellet by adding 2.5 ml of 72% sulfuric acid and incubating at 22° to 25°C for 1 hr with vortexing every 5 to 10 min.
10.Cool the sample in an ice bath, transfer the clear solution into a 10-ml volumetric flask, and fill to the mark with ultrapure water. Keep the dilution in an ice bath while proceeding with the next steps.
Anthrone method to determine glucose concentration
Basic Protocol 6 can be performed in lieu of the anthrone method if appropriate equipment (Dionex HPAEC-PAD or equivalent) is available.
11.Generate a standard curve by preparing and measuring the absorbance of glucose standards. Combine glucose stock solution (1 mg/ml), anthrone reagent and ultrapure water according to Table 1.Process the standards as described in steps 13 to 19 for the samples, measuring absorbance at 620 nm. Plot absorbance vs glucose concentration (mg/ml) as illustrated in Figure 3.
Standard | 1 mg/ml glucose stock (µl) | Ultrapure water (µl) | Final [glucose] (mg/ml) |
---|---|---|---|
Blank | 0 | 500 | 0 |
1 | 5 | 495 | 0.01 |
2 | 10 | 490 | 0.02 |
3 | 25 | 475 | 0.05 |
4 | 50 | 450 | 0.1 |
5 | 100 | 400 | 0.2 |
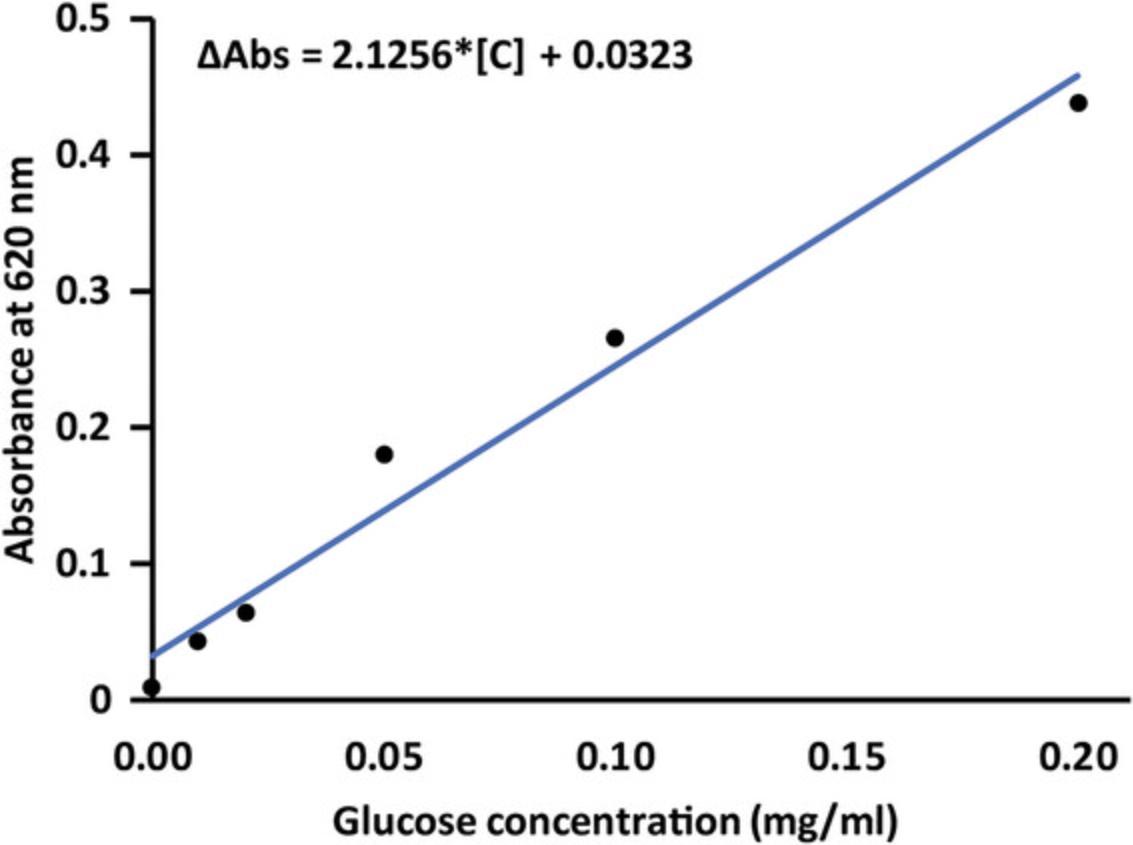
12.Dilute 100 µl of digested solution from step 10 to 400 µl total volume with ultrapure water in a 2-ml round bottom, glass screw-cap tube and put on ice.
13.Add 1 ml of freshly prepared, ice-cold anthrone reagent to sample and standards.
14.Vortex immediately for 3 s and return to ice for 5 min to remove the heat of solution generated from adding acid to the aqueous sample.
15.Allow the sample and standard series to acclimate at ambient temperature for 5 min.
16.Heat the sample and standard series for 15 min in a boiling water bath.
17.Cool sample and standard series on ice for 2 min and then incubate at room temperature for 5 min.
18.Transfer the sample or standard to a quartz cuvette.
19.Measure absorbance at 620 nm against a reagent blank consisting of 1 ml anthrone reagent dissolved in 0.5 ml ultrapure water.
20.Fit the measured absorbance of the sample to the standard curve to determine the glucose content and then calculate the cellulose content according to the equations below.
Glucose concentration:
Where:
- [C] = glucose concentration (mg/ml) in the sample
- Δabs = blank corrected absorbance of sample at 620 nm
- a = intercept of the standard curve derived from glucose standards
- m = slope of the standard curve derived from glucose standards.
% Cellulose:
Where:
- [C] = glucose concentration (mg/ml) derived from standard curve
- D = 150, the combined dilution factor from steps 10, 12 and 13
Further adjustment to D is required if additional dilution is needed per the annotation in Step 19.
- W = dry weight (mg) of initial biomass
- 0.9 = conversion factor of glucose to cellulose.
Basic Protocol 3: LIGNIN DETERMINATION
Lignin is a polyphenolic compound that increases the resistance of biomass to degradation. Measuring lignin content provides information about the composition, structure, and degradation resistance of plant biomass, e.g., in bioenergy and biomaterials production and can also indicate whether the biomass will be suitable for further processing into foods. Below we include a version of the acetyl bromide soluble lignin (ABSL) assay for total lignin quantification from plant biomass (Barnes & Anderson, 2017), tailored for lignocellulosic biomass with potential for conversion to post-catastrophic foods.
Materials
-
Lignocellulosic biomass of choice
-
70% ethanol (see recipe)
-
1:1 chloroform:methanol (see recipe)
-
Acetone, ≥99.5% (EMD Millipore, cat. no. AX0120)
-
90% DMSO (see recipe)
-
Lugol's iodine staining solution (Sigma-Aldrich, cat. no. 32922)
-
H2O, deionized
-
25% acetyl bromide, diluted in glacial acetic acid (see recipe)
-
Glacial acetic acid, ≥99.7% (EMD Millipore, cat. no. AX0073)
-
1.5 N sodium hydroxide (NaOH) (see recipe)
-
0.5 M hydroxylamine hydrochloride (see recipe)
-
Liquid nitrogen
-
PPE including safety glasses, lab coat, and gloves
-
Cryogenic-compatible containers
-
Stainless steel grinding balls for cryo ball mill (e.g., Retsch, sizes 5-, 9-, and 20-mm, cat. nos. 05.368.0034, 05.368.0036, and 05.368.0062, respectively)
-
Stainless steel grinding jars for cryo ball mill (e.g., Retsch, sizes 10-, 25-, and 50-ml, cat. nos. 01.462.0236, 01.462.0213, and 01.462.0216, respectively)
-
Cryo ball mill (e.g., Retsch, cat. no. 20.749.0001)
-
Reacti-Therm heating and stirring module (Thermo Fisher Scientific, cat. no. TS-18823), optional
-
Glass beads (Kimble Chase Life Science and Research Products, cat. no. 13500-4), optional
-
Chemical fume hood
-
Analytical balance (e.g., Mettler Toledo, Excellence Series)
-
2-ml Sarstedt tubes (Sarstedt, cat. no. 72.694.007)
-
Vortex mixer (e.g., VWR, cat. no. 58816-123)
-
Microcentrifuge capable of 10,000 × g (e.g., Eppendorf, cat. no. 5424)
-
Platform rocker (e.g., VWR, cat. no. 12620-906)
-
7-ml glass screw-cap vial, thread for 15-425 (Sigma-Aldrich, cat. no. 27151)
-
Solid cap with PTFE liner, 15-mm, for 7-ml glass screw-cap vial (Sigma-Aldrich, cat. no. 27152)
-
Water bath or incubator, 70°C (e.g., VWR, cat. no. 89032-216)
-
Ice bucket
-
NanoDrop 2000C spectrophotometer, or other UV-Vis spectrophotometer (Thermo Fisher Scientific, model no. ND-2000C-PC)
-
Quartz cuvette, 0.7-ml volume (Sigma-Aldrich, cat. no. Z600199)
-
10-, 200-, and 1000-µl pipettes and tips
CAUTION : PPE should be worn at all times when handling flammable chemicals and concentrated acids and bases, such as methanol, ethanol, acetyl bromide, glacial acetic acid, and NaOH. Wear safety glasses, a lab coat and gloves when handling these materials.
Biomass preparation (1 day per sample series)
1.Transfer 2 to 3 g of ground biomass samples (see Support Protocol 1) to cryogenic-compatible containers with steel balls, leaving 1/3 of the container empty for air space, and mill for 3 min using a Retsch CryoMill ball mill, or equivalent, with a pre-cooling cryo setting at 5 Hz, followed by grinding for 5 to 10 min at 30 Hz.
Alcohol insoluble residue (AIR) preparation (2 to 3 days per sample series)
The cell wall components comprise the AIR. All steps of AIR preparation are performed at room temperature, with the option of drying at 35°C under a stream of air at steps 8 and 12.Use of a Reacti-Therm module is not required, but samples will dry faster and more evenly with this module due to the supply of gentle airflow and heat. Drying in the Reacti-Therm module usually takes <1 hr, and the pellet will appear cracked when it is completely dry. This method, as opposed to allowing samples to dry in a chemical hood overnight, accounts for the differences in estimated time given for each sub-heading of the protocol.
2.Weigh roughly 70 mg of finely ground biomass into a 2-ml Sarstedt tube.
3.Add 1.5 ml of 70% ethanol, vortex thoroughly, centrifuge 10 min at ≥10,000 × g , room temperature, to pellet the residue, and remove as much supernatant as possible without disturbing the pellet.
4.Wash with 1.5 ml of 1:1 chloroform:methanol, vortex to resuspend the pellet, then centrifuge and remove supernatant as described in step 3.
5.Add 1.5 ml acetone, vortex, centrifuge, and remove supernatant as described in step 3.Allow material suspended in residual acetone to air dry in a chemical hood overnight with the tube cap removed, or dry under a stream of air at 35°C on a Reacti-Therm apparatus until completely dry. The remaining material is AIR.
6.To destarch the AIR, add 1.5 ml of 90% DMSO to the pellet, vortex thoroughly, and allow to shake overnight on a platform rocker at a speed of at least 50 rpm at the highest angle to facilitate mixing. The following day, centrifuge and remove supernatant as previously described in step 3.
7.Wash once more in 1.5 ml of 90% DMSO, then vortex, centrifuge, and remove supernatant as described above in step 3.
8.Wash six times in 1.5 ml of 70% ethanol. Vortex, centrifuge, and remove supernatant each time as described in step 3.
9.Add 1.5 ml acetone, vortex, centrifuge, and remove supernatant as described in step 3. Allow material suspended in residual acetone to air dry in a chemical hood overnight, or dry under a stream of air at 35°C on a Reacti-Therm apparatus. The remaining material is de-starched AIR.
10.Verify the absence of starch by staining a small portion of destarched AIR with Lugol's iodine solution and washing with water once as follows:
-
Use at least 2 mg AIR for ease of visualizing stained starch.
-
Add 500 µl Lugol's iodine solution to the AIR.
-
Vortex, incubate at room temperature for 5 min, centrifuge and remove supernatant as described in Step 3.
-
Wash with 1 ml water, centrifuge and remove supernatant as described in step 3.
-
Observe the color of the AIR. If starch is present, the AIR will appear dark in color and the destarching protocol should be repeated.
Lugol's iodine staining is recommended to verify the presence or absence of starch due to the facile nature of staining and noticeably darker coloration of the AIR containing starch after staining. Lugol's iodine staining is commonly used to stain starch granules in intact plant tissues, with sensitivity of detection largely limited to visual constraints (Ovecka et al.,2012). Removal of starch is necessary for accurate determination of lignin content because the ABSL quantification method quantifies lignin content with respect to the mass of cell wall material (AIR). If the AIR contains starch, starch will contribute to the overall weight of the AIR in the acetyl bromide digestion and result in a lower and variable weight of cell wall material in each sample, thus diminishing the accuracy of lignin determination.
Acetyl bromide soluble lignin determination (1 to 2 days per sample series)
11.Weigh ∼5 mg of destarched AIR into a 7-ml glass screw-cap vial and record the exact mass. For each biological sample, perform technical replicates in triplicate.
12.In a chemical hood, prepare 25% acetyl bromide by diluting in glacial acetic acid.
13.Working in a chemical hood, to each glass tube containing destarched AIR, gently add 1 ml of 25% acetyl bromide and swirl gently to mix the AIR into the 25% acetyl bromide. Also add 1 ml of 25% acetyl bromide to an empty glass tube to serve as a blank.
14.Incubate all samples at 70°C for 1 hr with gentle swirling every 10 min.
15.Immediately put samples on ice to cool. While samples are on ice, add 5 ml glacial acetic acid and vortex thoroughly in the chemical hood.
16.Allow any residual AIR to settle to the bottom of the glass vials for at least 1 hr to overnight at room temperature.
17.Once settled, gently transfer 300 µl from the top of each acetyl bromide solution to a quartz cuvette while avoiding resuspending any residual AIR. Add 400 µl of 1.5 N NaOH, then 300 µl of 0.5 M freshly made hydroxylamine hydrochloride to the cuvette. Pipette the solution gently in the cuvette until fully mixed after addition of the hydroxylamine hydrochloride.
18.Immediately after mixing is complete, measure absorbance at 280 nm (A280) against a blank on a spectrophotometer. The blank consists of the blank acetyl bromide digestion sample mixed with 1.5 N NaOH and hydroxylamine hydrochloride in the same ratio as described in step 17.See Figure 4 for a representative absorbance spectrum showing a lignin peak at 280 nm.
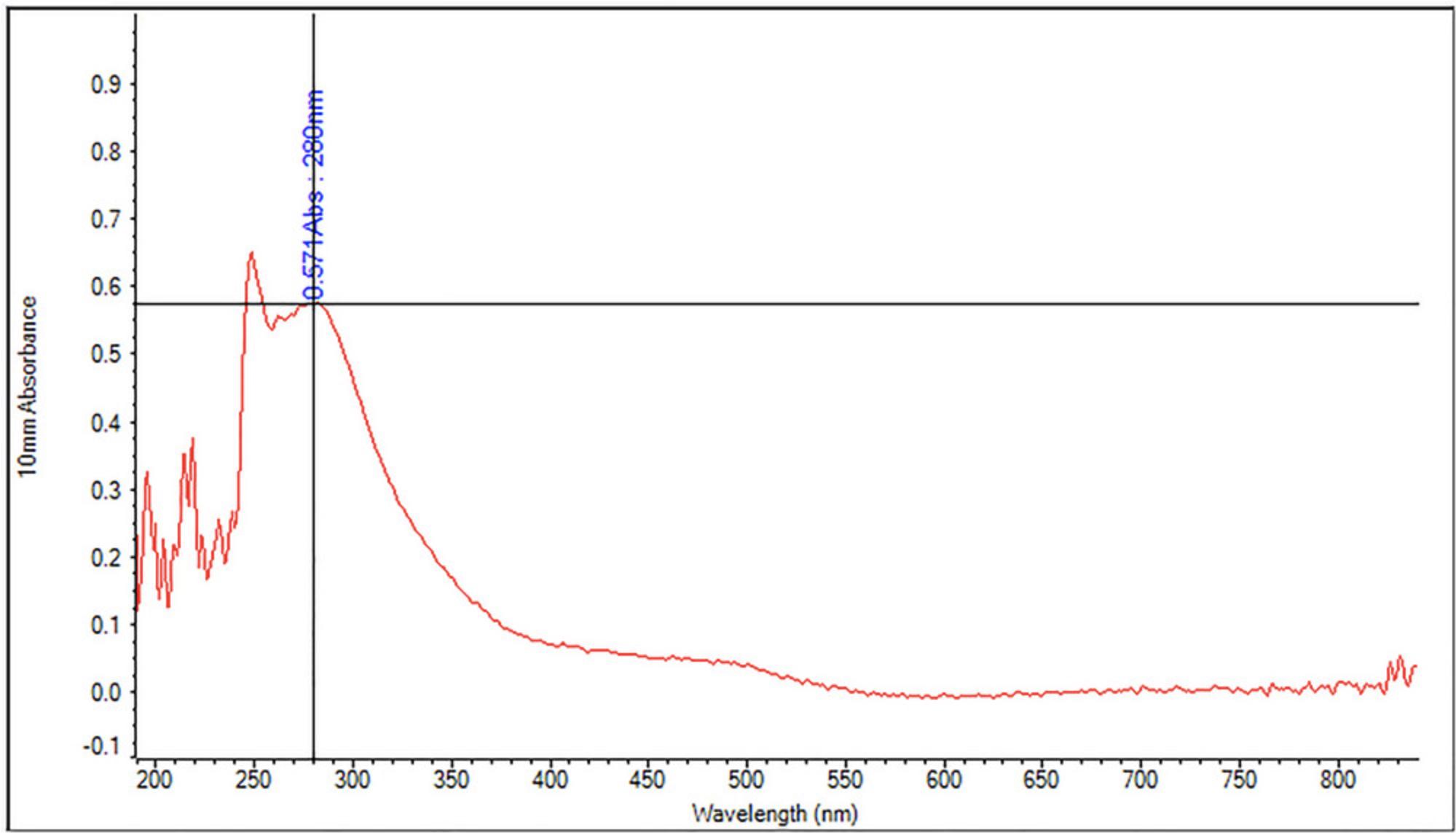
19.Between samples, wash the inside of the cuvette with glacial acetic acid and wipe the outside of the cuvette clean with 70% ethanol.
20.Use Beer's Law to calculate the percentage of acetyl bromide soluble lignin with the proper extinction coefficient. See Table 2.
Specimen |
Extinction coefficient (g-1 L cm-1) |
Reference |
---|---|---|
Arabidopsis thaliana, various ecotypes, stem tissues | 23.35 | Chang et al. (2008) |
Poplar (Populus sp.) | 18.21 | Foster et al. (2010) |
Arabidopsis thaliana, alternative | 15.69 | Foster et al. (2010) |
Grasses | 17.75 | Foster et al. (2010) |
Brachypodium distachyon | 18.126 | Fukushima and Hatfield (2004) |
Zea mays | 17.747 | Fukushima and Hatfield (2004) |
Medicago sativa, whole plant mean | 14.23 | Fukushima and Hatfield (2004) |
Trifolium pretense L., red clover | 14.49 | Fukushima and Hatfield (2004) |
Bromus inermis Leyss., bromegrass boot stage; anthesis; postseed stems | 17.11; 16.73; 17.40, respectively | Fukushima and Hatfield (2004) |
Avena sativa L., oat straw leaf; stem | 20.10; 18.91, respectively | Fukushima and Hatfield (2004) |
Triticum aestivum L., wheat straw leaf; stem | 19.81; 17.54, respectively | Fukushima and Hatfield (2004) |
Calculations
21.The following formula can be used to determine the mass percentage of the AIR that is ABSL, and can be converted to µg/mg AIR:
Where:
- A280 = Absorbance at 280 nm (blank corrected)
- ε = extinction coefficient (g-1 L cm-1)
- L = spectrophotometer path length (cm)
- D = dilution factor from digested AIR
- m = mass of destarched AIR (mg).
Basic Protocol 4: CRUDE PROTEIN CONTENT BY TOTAL NITROGEN
Crude protein content in biomass is typically calculated by measuring total nitrogen and multiplying by a nitrogen-to-protein conversion factor appropriate for the type of biomass. Before measuring the total nitrogen content, the samples should be dried and screened to remove large particles. The protocol below is designed according to the LECO operator instruction manual.
Materials
-
EDTA reference standard, LCRM class, certified reference material (LECO Corporation, cat. no. 502-896)
-
Tin foil cups (e.g., LECO, cat. no. 502-186)
-
Balance
-
Spatula (e.g., LECO, cat. no. 501-614)
-
Nitrogen analyzer (e.g., LECO nitrogen analyzer, model FP828p)
1.Before analyzing samples, verify equipment operational conditions by checking gas supplies and conducting leak checks according to the instrument manual.
2.Run 10 to 15 blanks until the values start to bottom out and then show a slight increase.
3.For system calibration, use tin foil cups. Weigh the cups and run at least five standards containing 0.2 g EDTA. Record the weight of each standard for documentation.
4.Assess repeatability in the standards by analyzing the standard deviation of EDTA samples. Compare this with the standard deviation reference value from the EDTA bottle and run the standards again if necessary to achieve consistency.
5.In triplicate, use a spatula to weigh ∼0.05 to 0.20 g (depending on the density of the sample) of finely ground biomass into a tin foil cup. Seal the tin foil in a drop-like shape to prevent air entrapment.
6.Run the samples on the nitrogen analyzer and obtain the percent nitrogen values from the chromatograms generated by the instrument. If necessary, convert the nitrogen values to a dry weight basis.
7.Multiply by 6.25 (or appropriate conversion factor) to calculate the crude protein content. See Table 3 for representative data for alfalfa biomass.
Sample | Weight (g; dry basis) | Nitrogen (%) |
Crude protein (%) (6.25 × nitrogen %) |
---|---|---|---|
Alfalfa 1 | 0.0507 | 3.59 | 22.42 |
Alfalfa 2 | 0.0501 | 3.51 | 21.91 |
Alfalfa 3 | 0.0508 | 3.63 | 22.69 |
8.Analyze a standard at the end of sample set to validate initial calibration.
Basic Protocol 5: CRUDE FAT DETERMINATION VIA SOXTEC EXTRACTION SYSTEM
Fats/lipids are an essential component of the human diet, thus, analyzing fat content in food products is important to assess their nutritional quality. Although these are not abundant in many types of lignocellulosic biomass, they can still be present in the form of oils and waxes. One widely used method for crude fat determination is the Soxhlet extraction, which involves the extraction of crude fat using either petroleum ether or diethyl ether as a solvent. Following the extraction at temperatures between 100° and 125°C, the solvent is volatized leaving behind a residue which is later quantified as fat mass. This protocol is modified from AACC International Method 30-25.01.
Materials
-
Petroleum ether, boiling point 30° to 50°C (Millipore Corporation cat. no. 64742-49-0)
-
No. 60 sieve (Thermo Fisher Scientific, cat. no. 041200.ON)
-
Electric blade coffee grinder (Shardor, cat. no. CG7255-UL)
-
Agate mortar and pestle, standard form, 95-mm O.D. (Millipore-Sigma, cat. no. Z112526)
-
Extraction thimbles, 25-mm × 60-mm (Whatman, cat. no. 10350215)
-
Sand, CAS 14808-60-7 (Ward's Science, cat. no. 470302-336)
-
Balance
-
Spatula
-
Glass wool
-
Thimble adapter (Foss, cat. no. 1000 7524)
-
Soxtec solvent extraction system (e)
-
Glass beads, anti-bumping granules, 5-mm (Sigma-Aldrich, cat. no. 18406)
-
Oven, 100°C
-
Desiccator (e.g., VWR, cat. no. 75871-662)
Sample preparation (dry sample)
1.Grind a dry sample of pre-milled biomass (see Suppport Protocol 1) into a fine powder that can pass through a No. 60 sieve. A coffee grinder can be used together with a mortar and a pestle. A cryo-mill can also be used (see Basic Protocol 3).
Using a Soxtec solvent extraction system
2.Depending on the density of the material, weigh ∼1 to 2 g of biomass into a pre-weighed extraction thimble containing 0.5 to 1 g sand, and record the mass to the nearest 0.01 g.
3.Mix sand and sample with a spatula. Wipe the spatula with a piece of glass wool and place the glass wool in the top of the extraction thimble.
4.Attach a thimble adapter to the extraction thimble containing the pre-dried sample covered with glass wool.
5.Place thimbles into the thimble supports attached to the thimble support holder.
6.Insert the thimbles into the condensers and raise the holder into the condenser. Attach the magnetic clasp to the thimble adapter by moving the extraction knob toward the boiling position.
7.When the magnetic clasp engages, raise the thimble to the rinsing position.
8.Remove the thimble supports.
9.Weigh extraction cups and record the weights to the nearest 0.01 g. Add 3 to 4 glass beads and 50 ml petroleum ether into each cup.
10.Insert the extraction cups into the Soxtec using the cup holder. Lower the safety lever ensuring that each cup is secured to the condenser. Move the extraction knob to the boiling position. The thimbles are now immersed in the boiling solvent.
11.Boil with the thimbles immersed for 25 min.
12.After 25 min, move the extraction knob to the rinsing position. The thimble should now be above the level of the solvent. Extract in the rinsing position for 30 min.
13.Close the condenser valve by turning one-quarter turn (horizontal position). When almost all the solvent is collected in the condenser, press the air button on the service unit and open the evaporation valve. The last traces of solvent will now be collected in the condenser.
14.Close the evaporation valve after 2 min or when there is no visible ether evaporation.
15.Release the extraction cups by raising the safety lever and remove the cups with the cup holder. Place the cups in an oven at 100°C for 30 min to evaporate the last traces of solvent prior to determination of the mass of the residue which contains the lipids from the biomass.
16.Cool in a desiccator for ∼5 min.
17.Weigh the extraction cups and record values to the nearest 0.01 g.
18.Place the thimble supports in the holder and place them on the hot plate beneath the thimbles. Lower the safety lever and, using the extraction knobs, lower the thimbles into the holders. Raise the safety lever and remove the thimbles from the Soxtec.
Calculation of lipid content
19.Calculate lipid content.
Fat weight (FW):
Percent crude fat (% crude fat):
Where:
- FW = fat weight
- FCW = final cup weight
- ICW = initial cup weight
- SW = sample weight.
Basic Protocol 6: SUGARS BY HPLC
HPLC in the form of high-performance anion exchange chromatography with pulsed amperometric detection (HPAEC-PAD) can be used to separate and detect individual sugars derived from the saccharification of lignocellulosic biomass. In most types of this biomass, the predominant sugars will be glucose (derived from cellulose) and xylose (derived from xylan and xyloglucan) with smaller amounts of arabinose, galactose, mannose, fucose, galacturonic acid, and glucuronic acid. The latter two of these, which are acidic sugars, can be underestimated when hydrolyzing biomass using trifluoroacetic acid (TFA) alone, and are better quantified using a TFA-methanolysis protocol (De Ruiter et al., 1992; Willför et al., 2009). This protocol can be used to measure sugars from a biomass sample after performing an appropriate digestion/hydrolization/extraction technique. For example, in Basic Protocol 2, steps 1 to 10, biomass is converted by digestion, acid hydrolysis, and extraction to a solution containing unknown amounts of sugars (Du et al., 2020).
Materials
-
H2O, ultrapure (18.2 MΩ.cm) (e.g., Milli-Q water, Millipore)
-
200 mM NaOH (see recipe)
-
100 mg/L sugar mix stock solution (see recipe)
-
0.45-µm syringe filter, 13 mm-wide, hydrophobic PTFE membrane (VWR International, cat. no. 76479-008)
-
MicroSolv screw top vial kit, with write-on patch, 2-ml vials (VWR International, cat. no. 97052-544)
-
High-performance anion-exchange chromatography system (e.g., Thermo Fisher Scientific Dionex ICS-6000)
-
Dionex CarboPac PA20 analytical column, 3-mm × 150-mm (Thermo Fisher Scientific, cat. no. 060142)
-
Dionex CarboPac PA20 guard column, 3-mm × 30-mm (Thermo Fisher Scientific, cat. no. 0601144)
-
Pulsed amperometric detector for Dionex ICS-6000 (Thermo Fisher Scientific, cat. no. 072044)
-
Dionex standard carbohydrate gold on PTFE electrochemical detector disposable electrode (Thermo Fisher Scientific, cat. no. 066480)
-
Silver-silver chloride (Ag/AgCl) reference electrode at 2.0 µA (Thermo Fisher Scientific, cat. no. 061879)
1.Using a suitable protocol (e.g., Basic Protocol 2, steps 1 to 10), digest and hydrolyze biomass samples to prepare solutions containing unknown quantities of sugars for analysis.
2.Filter the sample through a 13 mm–wide, 0.45-µm syringe filter with PTFE membrane prior to glucose analysis and transfer a minimum of 0.5 ml to a 2-ml vial suitable for analysis in the HPLC.
3.Make sure the HPLC instrument has appropriate columns and detector in place for analyzing sugars. If necessary, install the following (or equivalent):
- Dionex CarboPac PA20 analytical column connected to a Dionex CarboPac PA20 guard column
- Pulsed amperometric detector
- Dionex standard carbohydrate gold on PTFE electrochemical detector disposable electrode
- Ag/AgCl reference electrode at 2.0 µA.
4.For separating sugars on the HPLC, prepare supplies of ultrapure water for solvent A and 200 mM NaOH for solvent B.
5.Create a program using ultrapure water as solvent A and 200 mM NaOH as solvent B with the mobile phases at a total flow rate of 0.4 ml/min. Set the following parameters for solvent B: 1.2% for 18 min, increase to 50% by 20 min, hold at 50% for 10 min, then reduce to 1.2% for 5 min. (total run time of 35 min). See Figure 5 for a schematic of the HPLC program.
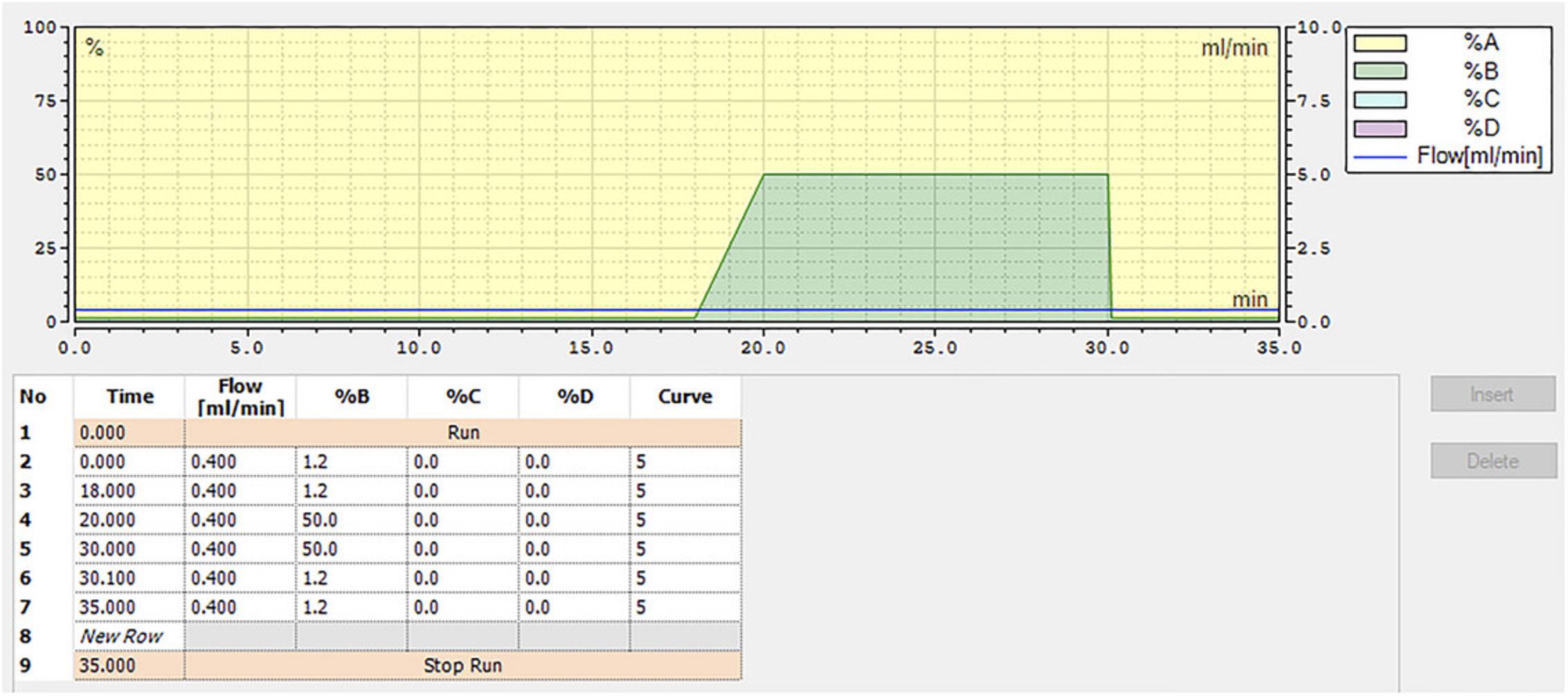
6.Prepare 1 L of a 100 mg/L sugar mix stock solution.
7.Using your sugar stock solution from step 6, prepare standards in the 0 to 10 mg/L sugar range as outlined in the Table 4.
Standard | 100 mg/L sugars stock (ml) | Ultrapure water (ml) | Final [sugar] (mg/L) |
---|---|---|---|
Blank | 0 | 10 | 0 |
1 | 0.1 | 9.9 | 1 |
2 | 0.25 | 9.75 | 2.5 |
3 | 0.5 | 9.5 | 5 |
4 | 0.75 | 9.25 | 7.5 |
5 | 1 | 9 | 10 |
8.Run the standards on the HPLC using the program created in step 5 and generate a best-fit equation for each sugar in mg/ml vs the area of the corresponding sugar peak. See Figure 6 for a representative chromatogram of the 6 sugars in the sugar mix at 1 mg/L and 10 mg/L.
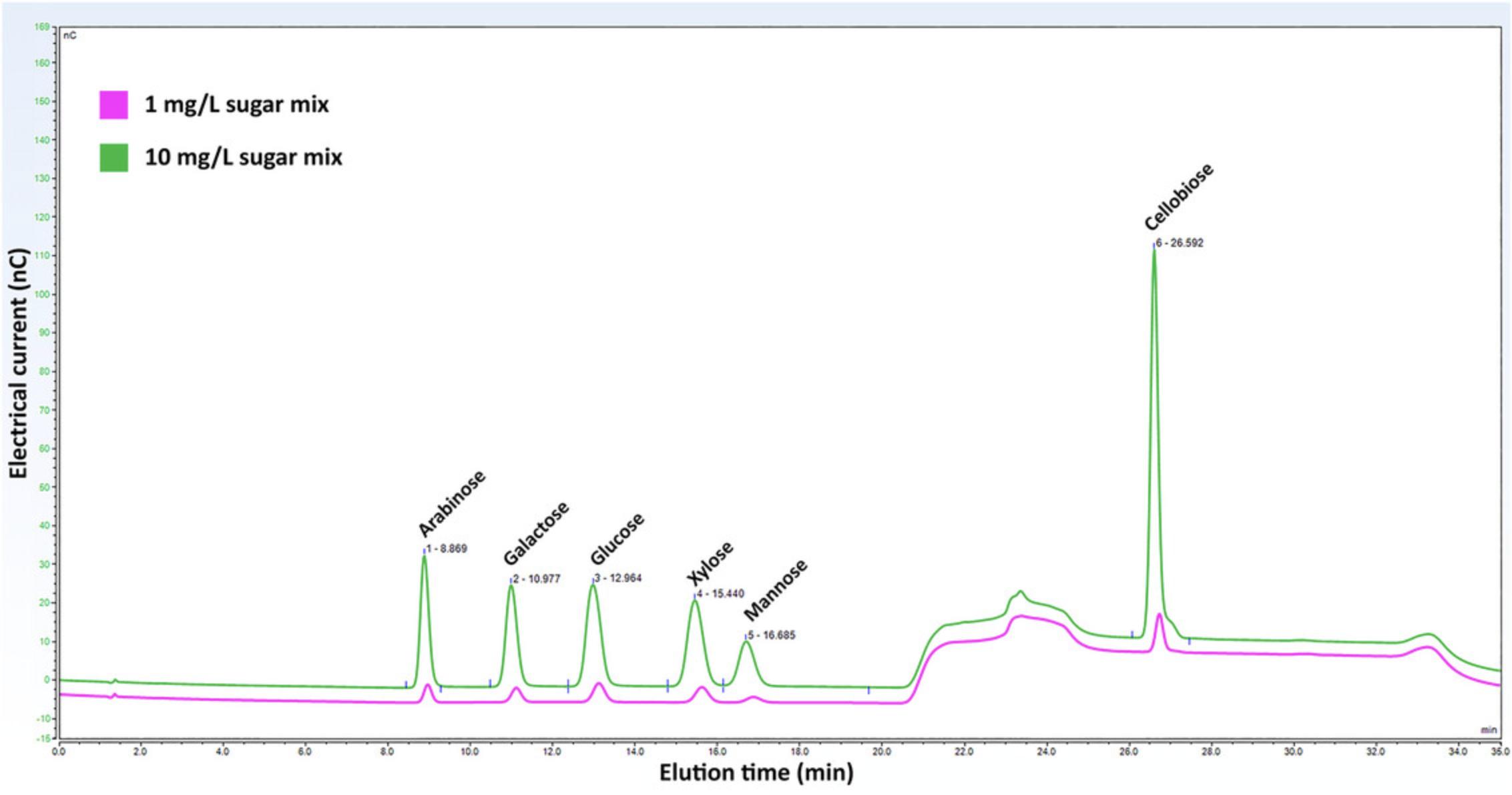
9.Run your sample on the HPLC using the same program.
10.Using the corresponding sugar peak area for your sample and the best-fit equation from running your standards, calculate the concentration of sugar in mg/ml for your filtrate from step 1.
Where:
- % sugar (w/w) = amount of glucose in the biomass
- ΔPA = blank corrected area of sugar peak
- a = intercept of the standard curve derived from corresponding sugar standards
- m = slop of the standard curve derived from corresponding sugar standards
- D = dilution factor
- V = total volume
- W = weight of the biomass (dry basis).
Basic Protocol 7: ASH CONTENT
Because certain minerals are critical to human health and nutrition, it is important to understand the potential of lignocellulosic biomass to contribute to the mineral content of foods subsequently produced from it. Ash content can be used as a proxy for total mineral content, and therefore it is of interest to determine ash content of lignocellulosic biomass. Ash comprises the inorganic material remaining after all organic matter in a biomass sample is combusted. While it typically includes other inorganics in addition to dietary minerals, it is still of interest to determine the ash content in context of analyzing the overall composition of lignocellulosic biomass. However, ash is only an estimation of total minerals. Individual minerals can be quantified using ICP (inductively coupled plasma) spectroscopy.
Materials
- Ashing crucibles and lids, 50-ml, porcelain, silica, or platinum
- Crucible marking pen, high temperature (Thermo Fisher Scientific, cat. no. NC9254302)
- Analytical balance, accurate to 1 mg
- Ashing burner and ignition source
- Muffle furnace, equipped with a thermostat, set to 500°C
- Desiccator (e.g., VWR, cat. no. 75871-662)
- Desiccant (e.g., Drierite Indicating, cat. no. 470300-992)
NOTE : When handling crucibles containing biomass, protect the sample from drafts to avoid mechanical loss of sample. When the organic material is calcined by the high temperatures in the furnace, the sample loses density. There is a significant chance of losing some of the sample due to static generated when removing samples from the furnace if the crucibles are not covered. It is always better to keep them covered.
1.Dry lignocellulosic biomass using the procedure described in Basic Protocol 1.
2.Label the crucible with a porcelain marker or a pen with ink resistant to high temperature. Record the weight to 1 mg (Wc).
3.Weigh ∼5 g dry biomass into a dried and tared crucible and record the weight to 1 mg (Wi).
4.Heat the biomass in the crucible over low flame to remove residual moisture.
5.Increase the flame and heat the sample for 15 to 30 min to obtain a gray ash.
6.Transfer the sample to a muffle furnace and burn for 6 hr once the temperature reaches 500°C.
7.Allow the crucible to cool to 70° to 80°C and transfer it to a desiccator containing desiccant.
8.Weigh the crucible at room temperature (Wc+d) and calculate the percent ash of the sample on dry weight basis per the equation below.
Where:
- Wc = tare weight of crucible
- Wi = initial weight of biomass
- Wc+d = final weight of crucible and ash.
REAGENTS AND SOLUTIONS
Scale all recipes in accordance with total volume needs based on the number of samples to be prepared.
Acetic acid/water/nitric acid mixture
Mix 150 ml of 80% acetic acid and 15 ml concentrated nitric acid (8:2:1, v/v/v, acetic acid/water/nitric acid). Store up to 6 months at room temperature.
Acetyl bromide (25%), diluted in glacial acetic acid
Mix 1:3 (v/v) acetyl bromide:glacial acetic acid (acetyl bromide, 99%, Sigma-Aldrich, cat. no. 135968; glacial acetic acid, ≥99.7%, EMD Millipore, cat. no. AX0073). Store up to 1 month at room temperature. When diluting acetyl bromide to 25% in glacial acetic acid in Baic Protocol 3, step 16, be sure to measure fuming acetyl bromide using only glass containers, e.g., a glass graduated cylinder, glass Pasteur pipette, and glass tubes. Using a micropipette will result in damage to the micropipette and the need for repair. Be cautious acetyl bromide is a strong acid that reacts violently with water.
Anthrone reagent
Dissolve 0.2 g anthrone (Sigma-Aldrich, cat. no. 319899) in 100 ml concentrated sulfuric acid [Thermo Fisher Scientific, cat. no. A300-212, certified ACS Plus, 95% to 98% (w/w)]. Prepare fresh daily. Chill for 2 hr in a refrigerator prior to use. The color of the anthrone reagent should be light yellow. While it will darken over time, it should be stable for at least a few hours (Kumar & Turner, 2015).
Chloroform:methanol, 1:1
Mix 1:1 (v/v) chloroform:methanol (chloroform, ≥ 99.5%, Sigma-Aldrich, cat. no. C2432; methanol, ≥ 99.8%, Fisher Scientific, cat. no. A412P). Store up to 2 years.
DMSO, 90%
Mix 1:9 (v/v) double distilled water:DMSO (dimethyl sulfoxide, ≥99.9%, Sigma-Aldrich, cat. no. 276855). Store up to 1 year at room temperature.
Ethanol, 70%
Mix 7:3 (v/v) 100% ethanol:double distilled water (Decon Labs, cat. no. V1001). Store up to 6 months at room temperature.
Glucose stock solution, 1 mg/ml
- 100 mg glucose (Sigma-Aldrich, cat. no. 49139)
- 100 ml H2O, ultrapure, 18.2 MΩ.cm (e.g., Milli-Q water, Millipore)
- Store up to 1 week at 4°C
Hydroxylamine hydrochloride, 0.5 M
- 347.45 mg hydroxylamine hydrochloride, 98% (Sigma-Aldrich, cat. no. 255580)
- 10 ml H2O, ultrapure, 18.2 MΩ.cm (e.g., Milli-Q water, Millipore)
- Mix well
- Prepare fresh before each use
Hydroxylamine hydrochloride is a strong reducing agent; store in a desiccator with desiccant.
NaOH (sodium hydroxide), 1.5 N
- 60.00 g NaOH pellets, ≥97% (Thermo Fisher Scientific, cat. no. BP359-500)
- 500 ml H2O, ultrapure (e.g., Milli-Q water, Millipore)
- Dissolve in a 1 L volumetric flask
- Mix, cool, and dilute to volume
- Store up to 6 months at room temperature
NaOH is a strong base. Work in a fume hood and handle with appropriate PPE.
NaOH, 200 mM
- To a 1 L volumetric flask, add:
- 16.00 g NaOH 50% (w/w) solution (Sigma-Aldrich, cat. no. 415413)
- Fill to the mark with ultrapure water (e.g., Milli-Q water, Millipore)
- Carefully mix the solution until it is fully dissolved
- Store up to 1 month at room temperature
Always use 50% (w/w) NaOH solution. Do not pipette the required aliquot from the top of the bottle where sodium carbonate may have been formed. Do not use NaOH pellets since they are coated with a layer of carbonate. The HPLC tubing and column are highly sensitive to particulate contaminates as might be introduced from the carbonate coating on pellets or from carbonate formed at the solution/air interface of the bottle. NaOH is a strong base. Work in a fume hood and handle with appropriate PPE.
Sugar mix stock solution, 100 mg/L
- To a 1 L glass volumetric flask, add:
- 100 mg glucose (Sigma-Aldrich, cat. no. 49139)
- 100 mg arabinose (Sigma-Aldrich, cat. no. A3256)
- 100 mg galactose (Sigma-Aldrich, cat. no. G0625)
- 100 mg xylose (Sigma-Aldrich, cat. no. 95729)
- 100 mg mannose (Sigma-Aldrich, cat. no. M2069)
- 100 mg cellobiose (Sigma-Aldrich, cat. no. C7252)
- Fill to the mark with ultrapure water, 18.2 MΩ.cm (e.g., Milli-Q water, Millipore)
- Mix until fully dissolved
- Store up to 1 month at 4°C
The individual solid sugar standards should be stored in a desiccator with desiccant to keep them dry.
COMMENTARY
Background Information
There is a great variety of lignocellulosic biomass types, and a large body of research is focused on its conversion to biofuels or suitability for animal feed. Less research is available on the conversion of biomass to food for humans. There is much to be studied, and we hope these protocols will be a useful guide as research evolves in this area.
In a post-catastrophic scenario, regions would be disconnected from normal communication and transportation channels. For this reason, local or regional lignocellulosic biomass resources might be of interest to researchers. Most of our work was completed using willow as the lignocellulosic biomass. Other sources may handle and process differently.
Critical Parameters
Preparation of lignocellulosic biomass for compositional analysis (Basic Protocol 1, Alternate Protocols 1 and 2, Support Protocols 1 and 2)
Temperature is critical during oven drying. If it is too high, the biomass will burn. For oven and air drying, the biomass pieces must be sized for easy turning and mixing during the drying process to allow all the water to evaporate. If there are large chunks of biomass, they may not dry uniformly inhibiting overall drying and potentially resulting in inconsistent moisture content throughout the sample. Regular mixing of biomass to redistribute pieces is necessary for uniform drying. Consistent moisture content is important to allow accurate normalization of samples on a dry weight basis for comparison of components of interest. Furthermore, if the biomass is not thoroughly dry, it may decompose during storage. For lyophilization, cover the sample containers with parafilm or plug them with cotton to prevent biomass from being sucked into the vacuum chamber during vacuum pull and release. This will prevent loss of sample mass which would cause erroneous weight measurements and will prevent damage to the vacuum system of the freeze-dryer. Ensuring that your biomass is thoroughly dry will allow more efficient processing in the milling step.
When milling lignocellulosic biomass, the goal is to process the material until it passes through the screen of selected size on the output of the grinding chamber. The dimensions of the biomass feedstock and its moisture content will impact how it processes through the knife mill. The biomass should flow through the knife mill without causing it to slow or jam. Smooth processing will minimize heat build-up. While lignocellulosic biomass is not highly heat sensitive, it is still important to minimize heat generation during processing to avoid damage to the biomass and/or loss of volatile components.
Moisture content, while simple and straightforward to measure, it an important parameter for subsequent calculations. Care should be taken to make accurate weight measurements. Once dried in the oven, samples must be kept dry in a desiccator to avoid reabsorption of moisture. Verify that the oven used is set to the correct temperature of 105°C and check periodically that the interior is maintained at the set temperature.
Basic Protocol 2: Cellulose determination
The initial digestion of the lignocellulosic biomass is the critical first step to remove hemicellulose and lignin from the plant cell walls. Errors in this step will translate directly to errors in the cellulose determination. The digestion incubation time and temperature and the mixing time and frequency should be carefully monitored and kept consistent across samples designated for comparative analysis. Take care not to lose any of the pellet that contains the cellulose when discarding the supernatant after the digestion and through all washing steps. Pipet out the supernatant very slowly to avoid removing any of the pellet. If pellet material is discarded with the supernatant, the cellulose determination will be falsely low. The hydrolysis step to convert cellulose to glucose is the next critical step. Again, incubation time and temperature and mixing time and frequency should be carefully monitored across samples designated for comparative analysis. Visually inspect the tubes to see if the pellet is completely dissolved, indicating complete hydrolysis. If you can see particulate matter, the incubation time must be increased. If the hydrolysis is incomplete due to shorter time, lower temperature or inadequate mixing, cellulose will not be fully converted to glucose and cellulose determination will be falsely low.
When measuring the glucose content of the dilution with the anthrone method, there are two critical parameters. First, because the anthrone reagent is highly unstable, it must be freshly prepared and chilled for at least two hours before use. Also, a new set of glucose standards must be prepared and measured for each batch of anthrone reagent. If old or degraded anthrone reagent is used, the reaction with glucose will be incomplete and a falsely low glucose content will be calculated. To generate comparative results, make sure to be consistent with time and temperature of the boiling water bath step after the anthrone reagent is added to the samples.
Basic Protocol 3: Lignin determination
The lignocellulosic biomass sample should be finely ground before beginning the lignin determination protocol. If it is not, the alcohol soluble compounds will not be effectively removed, inflating the AIR, and causing a falsely low lignin determination. Similarly, thorough removal of starch from the AIR is necessary or the mass of the destarched AIR will be falsely high translating to a falsely low lignin determination. Aggregates of particulate in AIR, if larger than ∼0.5-mm in diameter, could contribute to incomplete digestion of lignin leading to a falsely low lignin result. Suspended particles of AIR will interfere with A280 measurements. Wait for any AIR residual particulates to settle, and then very carefully pipette the 300 µl slowly to prevent any AIR particles from transferring to the cuvette. Waiting for settling will not impact the absorbance at 280 nm (A280) measurements because the samples are stable one glacial acetic acid has been added. NaOH and hydroxylamine hydrochloride must be well mixed in the cuvette. Make sure there is no cloudy, immiscible material in the bottom of the cuvette.
Basic Protocol 4: Crude protein content by total nitrogen
Before measuring total nitrogen, make sure biomass samples are finely ground, homogeneous, and screened to remove large particles. This will ensure that samples will fully combust in the nitrogen analyzer and yield an accurate total nitrogen value. Consider the biomass source and choose an appropriate conversion factor to calculate crude protein from total nitrogen. While a conversion factor of 6.25 is appropriate for many types of biomasses, lignocellulose derived from certain sources, such as fungi and algae, may require different conversion factors.
Basic Protocol 5: Crude fat determination via Soxtec extraction system
The fat obtained after completing step 14 of the Soxtec method must be transparent (it may have a light-yellow color) and devoid of any particulate matter. If it is not, repeat the analysis with a fresh sample, taking care to prevent contamination of the extraction cup with unextracted biomass from the initial sample. Make sure the sample is contained in the thimble by the glass wool that is placed on top in step 3. If the fat is burned after completing step 14 of the Soxtec method, the sample should be discarded without further analysis to avoid inaccuracies during quantification. Instead, the protocol should be repeated from the beginning with fresh biomass. The temperature setting of the service unit that heats the solvent in the Soxtec system and the time required for fat extraction depend on the type of sample being analyzed. For example, plant biomass requires a lower temperature and less time for fat extraction compared to meat. As mentioned, glass wool keeps samples contained in the thimble. This is particularly important for the fine powered samples typically obtained when preparing plant biomass for crude fat analysis. Water flow to the Soxtec system is critical for temperature control. If there is insufficient water flow, the solvent temperature may become too high leading to burned fat. On the other hand, too much water flow in any extraction unit can cause too low of an extraction temperature and incomplete fat extraction. Make sure to check that the water valve is open and there is an appropriate flow to provide temperature control for the system. Monitor the solvent in the extraction cups. Ensure that is it boiling and that conditions are equivalent in all units.
Basic Protocol 6: Sugars by HPLC
Aqueous NaOH should be used to prepare mobile solvents. Do not use solid NaOH as residual sodium carbonate may block the HPLC tubing. Sample solutions should be filtered before transferring to measurement vials to prevent residual particles from blocking the HPLC tubing. The temperature of the column and detector should be kept at 30°C for optimal function. To identify deterioration of the disposable gold electrode, it is critical to run a 10 mg/ml glucose standard regularly (every 15 samples) and check the peak value. If a peak area reduction is observed for the standard, replace the gold electrode.
Basic Protocol 7: Ash content
Properly prepared (clean, heated, and cooled in desiccator) crucibles are required for accurate ash content measurement. Make sure to use biomass that has already been oven dried at 105°C for 1 hr to remove moisture to facilitate ashing in the furnace and so that ash content is calculated on a dry weight basis.
Troubleshooting
See Table 5 for troubleshooting tips.
Basic Protocol (no.) | Problem | Possible cause | Solution |
---|---|---|---|
1 | Inconsistent moisture content in dried biomass | Too large pieces or chunks that did not dry at the same rate as remaining material | Cut the biomass into smaller pieces; stir more frequently and thoroughly; increase oven temperature (60°C max) |
1 | Biomass decomposed during storage | Biomass was not dried sufficiently prior to storage | Fragment biomass into uniform small pieces and dry thoroughly with regular turning/mixing until constant weight |
1 | Biomass sticks to walls of knife mill hopper and grinding chamber | Biomass was not sufficiently dry before grinding | Fragment biomass into uniform small pieces and dry thoroughly with regular turning/mixing until constant weight |
1 | Knife mills slows and jams | Biomass was not sufficiently fragmented into small enough pieces before grinding | Fragment biomass into uniform small pieces and dry thoroughly with regular turning/mixing until constant weight |
2 | Cellulose determination seems too low | Some of the pellet was removed after the digestion step |
Use more care when discarding the supernatant in steps 6 to 8 |
2 | Cellulose determination seems too low | Incomplete hydrolysis | Verify the time and temperature of the hydrolysis step; increase the time and/or temperature; mix more frequently |
2 | Sample A620 is >1 in step 19 | Glucose content of sample is high and reaction with anthrone has saturated the color | Increase the dilution factor in step 11 |
2 | Cellulose determination seems too low | Anthrone reagent not freshly prepared or kept chilled; anthrone reaction efficiency compromised and does not react with all available lignin | Prepare anthrone reagent fresh each time you measure absorbance of samples; chill anthrone reagent on ice for at least 2 hr prior to use |
2 | Calculated glucose values are variable and/or unexpected | Time, temperature, and mixing were not standardized for the anthrone steps among batches of samples | Carefully follow protocol and make sure to be consistent for all batches of samples |
3 | No/no expected absorbance value | Vial contents are not properly mixed | Mix the contents in cuvette very well |
4 | No nitrogen detected | Nitrogen in the samples is below detection limit | Increase the sample mass and reanalyze |
4 | Unexpected protein value | Conversion factor might differ | Select the appropriate conversion factor instead of 6.25 |
5 | Unexpected lipid value | Incomplete fat extraction | Increase the extraction time |
5 | Fat residue in the extraction cup contains particulate matter | Cup was dirty or contaminated; a deteriorated or previously used thimble can allow non-fat matter into cup | Ensure cups are clean and dry; use new thimbles for each extraction |
Fat residue in the extraction cup is burned | Extraction temperature too high, or extraction time too long; continued drying in oven after solvent is gone | Lower the temperature in the service unit or decrease the extraction time | |
6 | No peak area is detected |
Dilution is too high, thus, sugar level is below the detection limit or working electrode is deteriorated |
Reduce the dilution factor and rerun the sample or change the disposable working electrode |
6 | Sample peak area is beyond the standard curve range | Dilution is too high or low | Change the dilution factor and rerun the sample |
6 | Sugar peak area could not be determined due to other peaks | Samples might have other sugars or impurities that are not interested | Spike the sample vial with corresponding sugar standard and rerun it and observe a peak increase |
6 | Peak area is reduced between standard runs | Working electrode is deteriorated | Change the disposable working electrode |
7 | Ash content is unexpectedly high | Biomass was not thoroughly dried before weighing into the crucible | Dry biomass at 105°C for 1 hr before analyzing ash content |
Understanding Results
Collection and fragmentation of biomass
Initial size reduction is critical to thorough drying of the lignocellulosic biomass which sets the stage for successful and accurate downstream processing and analysis. The biomass should be reduced to pieces that are small enough to dry to constant weight in a reasonable amount of time using the drying method of choice. Because there is a great variety of biomass types and research in this area is in early stages, some ingenuity and experimentation may be required to procure biomass from sources of interest and design initial processing methods to reduce the biomass to pieces that are sized and shaped such that it can be dried successfully. This might entail using a woodchipper, hedge trimmers, scissors, etc.
Basic Protocol 1 and Alternate Protocols 1 and 2
Lignocellulosic biomass can be dried in a variety of ways. Characteristics of the biomass (e.g., quantity available and moisture content) and space and equipment available are factors that will guide the choice of drying method. In all cases, the desired outcome is to dry the biomass to the point of constant weight as indicated by a weight change of <1% after an appropriate time/temperature increment. See Table 6 for guidance on time required for drying using different methods. If the biomass is not dry, it may decompose if storage is necessary before testing. Furthermore, it may stick to surfaces in the knife mill hopper and grinding chamber making it difficult to process into a homogeneous material for compositional analyses.
Drying method | Temperature | Time (hr) |
---|---|---|
Convection oven drying | 45° to 60°C | 1 |
Air drying | Ambient temperature | 24 |
Lyophilization | −45° to −50°C | 24-96 |
Support Protocol 1: Milling plant biomass
Sufficient grinding through a knife mill is achieved by processing biomass that has been fragmented and thoroughly dried and selecting an appropriate screen size for the exit of the grinding chamber. Optionally, the ground biomass may be sieved, either by hand or using an automated sieve shaker, to further refine the particle size distribution. A screen size of 0.5 mm on the mill exit should provide a ground material suitable for cellulose, lignin, protein, lipid, sugar, and ash determination according to the basic protocols described above. It should be reiterated that before milling, the biomass should be chopped or cut into small pieces, <1-cm in size, so that they can be processed easily through the mill without jamming it and causing heat build-up. If the mill overheats, the biomass may be damaged.
Support Protocol 2: Measuring moisture content
While measuring moisture content is a straightforward protocol, it is critical that measurements are made accurately because the value is used to determine the dry weight of the biomass to which other components are typically normalized for comparison. Weigh containers and samples carefully and make sure the oven is set correctly and maintains the expected temperature consistently.
Basic Protocol 2: Cellulose determination
This protocol details steps to digest lignocellulosic biomass to remove hemicellulose and lignin, and then hydrolyze the remaining crystalline cellulose to glucose monomers that can be measured by absorbance after reacting with an anthrone reagent. The absorbance values as a function of glucose content are the key data derived from the protocol. From the absorbance values, glucose content is calculated based on a standard curve generated during the protocol as shown in Figure 3. Finally, the percent cellulose is calculated from the glucose content, the initial weight of the lignocellulosic biomass, the combined dilution factor from the entire protocol, and a conversion factor for glucose to cellulose.
Basic Protocol 3: Lignin determination
This complex protocol involves many steps which must be completed carefully to achieve accurate, consistent results. The AIR portion of the biomass is prepared and destarched, then the lignin in the AIR is solubilized by digestion with acetyl bromide reagent. The solubilized lignin is mixed well with hydroxylamine hydrochloride in a cuvette to generate colored reaction products that absorb at 280 nm. The absorbance values as a function of lignin content are the key data derived from the protocol (see Fig. 4). The % ABSL is then calculated from absorbance, the extinction coefficient for the biomass type analyzed, the spectrophotometer path length, the dilution factor, and the mass of the destarched AIR.
Basic Protocol 4: Crude protein from total nitrogen
Most researchers have access to a nitrogen analyzer that utilizes the combustion method first documented by Dumas (1831) to yield total nitrogen content. The instrument returns the total percent nitrogen which is then converted to crude protein content using an appropriate conversion factor and reported on a dry weight basis of the initial sample.
Basic Protocol 5: Crude fat determination via Soxtec extraction system
Using an automated Soxtec solvent extraction system, total crude fat can be extracted from multiple plant biomass samples at once speeding the data collection process compared to traditional Soxhlet extraction on which the system is based. The biomass is weighed into an extraction thimble and is boiled in petroleum ether with refluxing. The fat is solubilized into the boiling ether and retained in the extraction cups of the system after the sample is rinsed and the solvent is recovered. Once the extracted fat is dried in an oven to remove residual solvent, the mass of the extracted fat is determined, and the % crude fat in the original sample is calculated and adjusted for dry weight basis as needed. See Table 7 for a representative presentation of results.
Crude (total) fat | |
---|---|
Mass (g, dry weight) | Fat (%) |
0.936 | 3.00 |
0.919 | 2.86 |
0.927 | 2.83 |
0.952 | 2.90 |
0.926 | 2.63 |
0.962 | 2.20 |
0.905 | 2.26 |
0.912 | 2.56 |
0.914 | 1.50 |
0.901 | 1.30 |
Basic Protocol 6: Sugars by HPLC
This method uses an anion-exchange chromatography technique for the separation of sugars. Sugars are weak acids and can become anions in high-pH media, such as NaOH. Subsequently, sugar anions can be effectively separated through a column containing positively charged functional groups of an anion-exchange resin. The isolated sugars, including glucose, are directly identifiable via pulsed amperometry technique which measures the electrical current produced by the oxidation of the sugars at the surface of the gold working electrode. The peak area, determined as the product of the current generated by the sugar and the time required for complete elution of the sugar, correlates directly with the concentration of sugar in the eluate. By plotting a graph for the concentration of glucose against the peak area, the glucose concentration of unknown samples can be measured.
Basic Protocol 7: Ash content
Ash content is calculated from the weight of material remaining after a biomass sample is combusted in a muffle furnace at high temperature for a specified period. The weight of the ash is then expressed as a percentage on a dry weight basis for the biomass.
Time Considerations
Strategic planning
Collecting and fragmenting biomass: 1 to 2 days.
Basic Protocol 1
Convection oven drying: 1 to 2 days.
Alternate Protocol 1
Air-drying: 2 to 3 days.
Alternate Protocol 2
Lyophilization: 3 to 4 days.
Support Protocol 1
Milling plant biomass: 1 day.
Support Protocol 2
Measuring moisture content: 1 day.
Basic Protocol 2
Cellulose determination. Allow 1 day to cryo ball mill samples, weigh them into tubes, digest, rinse and isolate the pellet, and let the pellet dry overnight. Allow a second day to hydrolyze the pellets and complete the anthrone colorimetric absorbance measurements.
Basic Protocol 3
Lignin determination. About 1 day will be required to cryo ball mill ground samples, 2 to 3 days for AIR preparation and destarching, and 1 to 2 days for the acetyl bromide soluble lignin determination.
Basic Protocol 4
Crude Protein Content by total nitrogen. Preparing the nitrogen analyzer including running bland and standards takes ∼90 min. Weighing samples into the tin foil cups and twisting them into a drop shape takes ∼2 min per sample, and running each sample in the analyzer takes ∼3 min. With samples run in triplicate, allow 15 min per sample.
Basic Protocol 5
Crude fat determination via Soxtec extraction system. Preparing finely ground samples prior to extraction takes ∼10 min per sample. The Soxtec system can process 6 samples at a time. The extraction, rinsing, and oven drying steps along with weighing samples before and after the extraction require ∼2 hr for 6 samples.
Basic Protocol 6
Sugars by HPLC. Diluting and filtering samples can be accomplished quickly. 10 to 20 samples can be prepared for analysis in 1 to 2 hr. The program for analyzing sugars requires 35 min to complete. Based on this time and the number of samples to be analyzed, the total time can be calculated. Running the required standards before and during a series of samples must also be factored in. It is also a good idea to analyze a ultrapure water sample periodically to check the state of the column.
Basic Protocol 7
Ash content. If the biomass has already been dried, then ≤1 day should be sufficient for ashing and weighing samples. Allow 2 to 3 additional days if the biomass needs to be dried.
Acknowledgments
This work was supported by Open Philanthropy. Thanks to members of the Penn State Emergency Food Resilience team for helpful input.
Author Contributions
Tara N. Mather : Conceptualization; methodology; project administration; writing—original draft; writing—review and editing. Niroshan Siva : Methodology; writing—review and editing. Marjorie Jauregui : Methodology; writing—review and editing. Hannah Klatte : Methodology; writing—review and editing. Joshua D. Lambert : Methodology; writing—review and editing. Charles T. Anderson : Conceptualization; methodology; writing—review and editing.
Conflict of Interest
The authors declare no conflict of interest.
Open Research
Data Availability Statement
Data available on request from the authors.
Literature Cited
- AACC Approved Methods of Analysis, 11th ed. Method 30-25.01. Crude fat in wheat, corn, and soy flour, feeds, and mixed feeds. Approved (1961, April 16). Cereals & Grains Association.
- Anderson, S. (2019). Soxtec: Its principles and applications. In Oil extraction and analysis (pp. 11–24). AOCS Publishing. https://doi.org/10.1201/9780429104527-2
- Barnes, W., & Anderson, C. (2017). Acetyl Bromide Soluble Lignin (ABSL) assay for total lignin quantification from plant biomass. Bio-Protocol , 7(5), 1–11. https://doi.org/10.21769/bioprotoc.2149
- Bar-On, Y. M., Phillips, R., & Milo, R. (2018). The biomass distribution on Earth. Proceedings of the National Academy of Sciences of the United States of America , 115(25), 6506–6511. https://doi.org/10.1073/pnas.1711842115
- Bengtsson, A., Hecht, P., Sommertune, J., Ek, M., Sedin, M., & Sjöholm, E. (2020). Carbon fibers from lignin–cellulose precursors: Effect of carbonization conditions. ACS Sustainable Chemistry & Engineering, 8(17), 6826–6833. https://doi.org/10.1021/acssuschemeng.0c01734
- Chang, X. F., Chandra, R., Berleth, T., & Beatson, R. P. (2008). Rapid, microscale, acetyl bromide-based method for high-throughput determination of lignin content in Arabidopsis thaliana. Journal of Agricultural and Food Chemistry , 56(16), 6825–6834. https://doi.org/10.1021/JF800775F
- De Ruiter, G. A., Schols, H. A., Voragen, A. G., & Rombouts, F. M. (1992). Carbohydrate analysis of water-soluble uronic acid-containing polysaccharides with high-performance anion-exchange chromatography using methanolysis combined with TFA hydrolysis is superior to four other methods. Analytical Biochemistry , 207(1), 176–185. https://doi.org/10.1016/0003-2697(92)90520-h
- Du, J., Kirui, A., Huang, S., Wang, L., Barnes, W. J., Kiemle, S. N., Zheng, Y., Rui, Y., Ruan, M., Qi, S., Kim, S. H., Wang, T., Cosgrove, D. J., Anderson, C. T., & Xiao, C. (2020). Mutations in the pectin methyltransferase QUASIMODO2 influence cellulose biosynthesis and wall integrity in arabidopsis. Plant Cell , 32(11), 3576–3597. https://doi.org/10.1105/TPC.20.00252
- Dumas, J. B. A. (1831). Procédés de l’analyse organique. Annals of Chemistry and of Physics , 47, 198–205.
- Fontana, A. J., & Carter, B. P. (2020). Measurement of water activity, moisture sorption isotherm, and moisture content of foods. Water Activity in Foods: Fundamentals and Applications , 207–226. https://doi.org/10.1002/9781118765982.ch8
- Foster, C. E., Martin, T. M., & Pauly, M. (2010). Comprehensive compositional analysis of plant cell walls (Lignocellulosic biomass) part I: Lignin. Journal of Visualized Experiments : JoVE , (37), 1745. https://doi.org/10.3791/1745
- Fukushima, R. S., & Hatfield, R. D. (2004). Comparison of the acetyl bromide spectrophotometric method with other analytical lignin methods for determining lignin concentration in forage samples. Journal of Agricultural and Food Chemistry , 52(12), 3713–3720. https://doi.org/10.1021/JF035497L
- Hames, B., Ruiz, R., Scarlata, C., Sluiter, A., Sluiter, J., & Templeton, D. (2008). Preparation of Samples for Compositional Analysis: Laboratory Analytical Procedure (LAP). National Renewable Energy Laboratory (NREL). Issue Date 08/08/2008. Technical Report NREL/TP-510-42620. https://www.nrel.gov/docs/gen/fy08/42620.pdf
- Hatfield, R. D., Grabber, J., Ralph, J., & Brei, K. (1999). Using the acetyl bromide assay to determine lignin concentrations in herbaceous plants: Some cautionary notes. Journal of Agricultural and Food Chemistry , 47(2), 628–632. https://doi.org/10.1021/JF9808776
- Hatfield, R. D., Marita, J. M., Frost, K., Grabber, J., Ralph, J., Lu, F., & Kim, H. (2009). Grass lignin acylation: P-coumaroyl transferase activity and cell wall characteristics of C3 and C4 grasses. Planta , 229(6), 1253–1267. https://doi.org/10.1007/S00425-009-0900-Z
- Johnson, D. B., Moore, W. E., & Zank, L. C. (1961). The spectrophotometric determination of lignin in small wood samples. Tappi , 44, 793–798.
- Kapp, N., Barnes, W. J., Richard, T. L., & Anderson, C. T. (2015). Imaging with the fluorogenic dye Basic Fuchsin reveals subcellular patterning and ecotype variation of lignification in Brachypodium distachyon. Journal of Experimental Botany , 66(14), 4295–4304. https://doi.org/10.1093/JXB/ERV158
- Kumar, M., & Turner, S. (2015). Protocol: A medium-throughput method for determination of cellulose content from single stem pieces of Arabidopsis thaliana. Plant Methods , 11(1), 1–8. https://doi.org/10.1186/s13007-015-0090-6
- Montes, F., Fabio, E. S., Smart, L. B., Richard, T. L., Rodrigo Masip, A., & Kemanian, A. R. (2021). A semi-commercial case study of willow biomass production in the northeastern United States. Agronomy Journal , 113(2), 1287–1302. https://doi.org/10.1002/agj2.20603
- Ovecka, M., Bahaji, A., Muñoz, F. J., Almagro, G., Ezquer, I., Baroja-Fernández, E., Li, J., & Pozueta-Romero, J. (2012). A sensitive method for confocal fluorescence microscopic visualization of starch granules in iodine stained samples. Plant Signaling & Behavior, 7(9), 1146–1150. https://doi.org/10.4161/PSB.21370
- Roni, M. S., Lin, Y., Hartley, D. S., Thompson, D. N., Hoover, A. N., & Emerson, R. M. (2023). Importance of incorporating spatial and temporal variability of biomass yield and quality in bioenergy supply chain. Scientific Reports , 13(1), 6813. https://doi.org/10.1038/s41598-023-28671-4
- Siva, N., & Anderson, C. T. (2023). Assessing lignocellulosic biomass as a source of emergency foods. Current Research in Food Science , 7(July), 100586. https://doi.org/10.1016/j.crfs.2023.100586
- Sluiter, A., Hames, B., Hyman, D., Payne, C., Ruiz, R., Scarlata, C., Sluiter, J., Templeton, D., & Nrel, J. W. (2008). Determination of total solids in biomass and total dissolved solids in liquid process samples. National Renewable Energy Laboratory (NREL)__, March , 3–5.
- Thiex, N. J., Anderson, S., Gildemeister, B., & Collaborators. (2003). Crude fat, diethyl ether extraction, in feed, cereal grain, and forage (Randall/Soxtec/Submersion Method): Collaborative study. Journal of AOAC International , 86(5), 888–898. https://doi.org/10.1093/jaoac/86.5.888
- Updegraff, D. M. (1969). Semimicro determination of cellulose in biological materials. Analytical Biochemistry , 32(3), 420–424. https://doi.org/10.1016/S0003-2697(69)80009-6
- Willför, S., Pranovich, A., Tamminen, T., Puls, J., Laine, C., Suurnäkki, A., Saake, B., Uotila, K., Simolin, H., Hemming, J., & Holmbom, B. (2009). Carbohydrate analysis of plant materials with uronic acid-containing polysaccharides–A comparison between different hydrolysis and subsequent chromatographic analytical techniques. Industrial Crops and Products , 29(2–3), 571–580. https://doi.org/10.1016/J.INDCROP.2008.11.003
- Xiao, C., Barnes, W. J., Zamil, M. S., Yi, H., Puri, V. M., & Anderson, C. T. (2017). Activation tagging of arabidopsis polygalacturonase involved in expansion2 promotes hypocotyl elongation, leaf expansion, stem lignification, mechanical stiffening, and lodging. The Plant Journal , 89(6), 1159–1173. https://doi.org/10.1111/tpj.13453
Citing Literature
Number of times cited according to CrossRef: 1
- Tara Mather, Niroshan Siva, Marjorie Jauregui, Pradip Poudel, Maria Julia Lima Brossi, Joshua D. Lambert, Francesco Di Gioia, Erin L. Connolly, Charles T. Anderson, Nutritional Composition of Post‐Catastrophic Foods, Current Protocols, 10.1002/cpz1.1110, 4 , 8, (2024).