Prediction of Acute Hepatotoxicity With Human Pluripotent Stem Cell-Derived Hepatic Organoids
Hyemin Kim, Hyemin Kim, Sang Kyum Kim, Sang Kyum Kim, Michael Oelgeschläger, Michael Oelgeschläger, Han-Jin Park, Han-Jin Park
cytochrome P450
drug testing
hepatic organoid
hepatotoxicity
human pluripotent stem cell differentiation
liver
Abstract
Recent development of hepatic organoids (HOs) derived from human pluripotent stem cells (hPSCs) provides an alternative in vitro model that can mimic the human liver detoxification pathway for drug safety assessment. By recapitulating the high level of maturity and drug-metabolizing capacity of the liver in a three-dimensional organoid culture, HOs may allow researchers to assess drug toxicity and metabolism more accurately than animal models or hepatocellular carcinoma cells. Although this promising potential has contributed to the development of various protocols, only a few protocols are available to generate functional HOs with guaranteed CYP450 enzymatic activity, the key feature driving toxic responses during drug metabolism. Based on previously published protocols, we describe an optimized culture method that can substantially increase the expression and activity of CYP450s, in particular CYP3A4, CYP2C9, and CYP2C19, in HOs. To generate mass-produced and highly reproducible HOs required as models for toxicity evaluation, we first generated hepatic endodermal organoids (HEOs) from hPSCs capable of in vitro proliferation and cryopreservation. The stepwise protocol includes generating HEOs as well as efficient methods to enhance CYP450 expression and activity in terminally differentiated HOs. Furthermore, we present a simple protocol for the assessment of HO cytotoxicity, one of the hallmarks of drug-induced acute hepatotoxicity. The protocols are relatively straightforward and can be successfully used by laboratories with basic experience in culturing hPSCs. © 2024 The Authors. Current Protocols published by Wiley Periodicals LLC.
Basic Protocol 1 : Generation of hepatic endodermal organoids from human pluripotent stem cells
Basic Protocol 2 : Expansion and cryopreservation of hepatic endodermal organoids
Basic Protocol 3 : Differentiation of hepatic organoids from hepatic endodermal organoids
Basic Protocol 4 : Evaluation of hepatotoxicity using hepatic organoids
Support Protocol : Human pluripotent stem cell culture
INTRODUCTION
Drug-induced hepatotoxicity is generally evaluated by administering drugs to animal models or in vitro hepatic cell models, such as primary human hepatocytes (PHHs) and liver cancer cell lines. However, they have various limitations such as inaccuracies in toxicity results owing to species differences between animal models and humans, poor accessibility and large variation among donors of PHHs, and low drug metabolic activity and chromosomal abnormalities of liver cancer cell lines. To overcome such limitations of existing models, new three-dimensional (3D) liver models with guaranteed drug metabolic functions for accurate hepatotoxicity testing are needed. Hepatic organoids (HOs) are 3D cell assemblies composed of the cells that make up liver tissue and recapitulate major functions of the liver, such as drug detoxification. As a toxicity evaluation model, HOs derived from human pluripotent stem cells (hPSCs) have advantages, including high reproducibility of results, mass production, mimicking the human drug metabolism process, and enabling personalized toxicity prediction based on human induced pluripotent stem cells (hiPSCs). In this article, we provide detailed instructions on how to generate expandable hepatic endodermal organoids from hPSCs and differentiate hepatic organoids with high maturity and functionality, especially enhanced cytochrome P450 (CYP450) expression and activities, using an efficient, cost-effective, and reproducible culture system. We also present a protocol for assessing acute drug-induced hepatotoxicity by measuring organoid viability. These protocols should allow researchers with basic experience in hPSC culture to implement 3D hepatic models that mimic human drug metabolism and toxic response in their laboratories.
We have established detailed protocols for the generation of HOs from hPSCs (Kim et al., 2022). In Basic Protocol 1, we present a simple and defined method to generate expandable HEOs from hPSCs. In Basic Protocol 2, we describe the expansion and cryopreservation method of HEOs. Basic Protocol 3 explains the differentiation of HOs from HEOs, and Basic Protocol 4 details the evaluation of hepatotoxicity using HOs (Fig. 1). Support Protocol describes the culture of hPSCs used as the primary component of HOs.
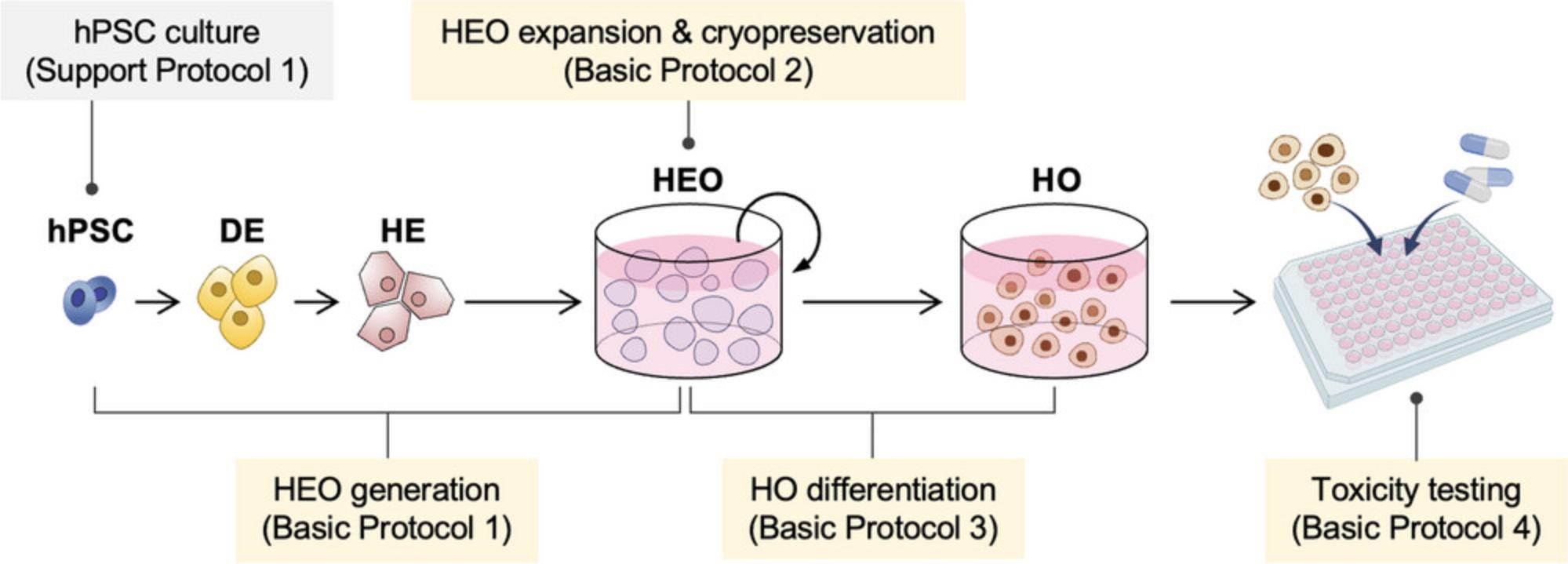
STRATEGIC PLANNING
Before starting the protocol, the user should have hPSCs (e.g., induced pluripotent stem cells, iPSCs; embryonic stem cells, ESCs) in good condition, such as in terms of active self-renewal and pluripotency. These cells can be purchased directly from vendors (e.g., ATCC), obtained from the National Stem Cell Bank, or generated via reprogramming somatic cells. The detailed methods for somatic cell reprogramming have been published by Wu and Tucker groups (Rajarajan et al., 2012; Wiley et al., 2017). Ethics committee approval is required for the use of ESCs and iPSCs. Support Protocol describes hPSC culture prior to hepatic organoid generation.
CAUTION : Human iPSCs are a Biosafety Level 2 (BSL-2) hazard. Follow all appropriate guidelines and regulations for the use and handling of biological hazards.
NOTE : All procedures using human iPSCs must first be reviewed and approved by an institutional review board or must follow local guidelines for the use of human specimens.
NOTE : All procedures pertaining to the culture of stem cells or organoids must be performed using sterile materials and aseptic technique to avoid contamination.
NOTE : All protocols involving animals must be reviewed and approved by the appropriate Animal Care and Use Committee and must follow regulations for the care and use of laboratory animals. Appropriate informed consent is necessary for obtaining and use of human study material.
Basic Protocol 1: GENERATION OF HEPATIC ENDODERMAL ORGANOIDS FROM HUMAN PLURIPOTENT STEM CELLS
In this protocol, we describe an efficient approach to generate expandable hepatic endodermal organoids (HEOs) from hPSCs. This protocol starts with hPSCs in culture and sequentially differentiates them into definitive endoderm (DE) and hepatic endoderm (HE) for 10 days. Successful differentiation of DE and HE cells will result in nearly 100% confluency and homogenous cell morphology at each step. Also, over 90% of CXCR4-positive and EpCAM-positive populations are presented in DE and HE cells using flow cytometry, respectively. After the differentiation of HE cells, a single HE cell can form a cyst-like organoid under the defined organoid culture condition for 14 to 15 days. Successful HEO generation will result in the formation of approximately 100 to 150 HEOs per 10,000 HE cells.
Materials
-
Matrigel solution (see recipe)
-
Human iPSCs (e.g., HDF01 hiPSCs generated by reprogramming human dermal fibroblasts and provided by KAIST, Republic of Korea; see Support Protocol)
-
EDTA solution, 0.5 mM (see recipe)
-
mTeSR1 or mTeSR plus (STEMCELL Technologies, cat. no. 85850 or 100-0276)
-
Y-27632, 20 mM (see recipe)
-
Definitive endoderm (DE) medium 1 (see recipe)
-
DE medium 2 (see recipe)
-
Hepatic endoderm (HE) medium (see recipe)
-
DPBS, with calcium and magnesium (Cytiva, cat. no. SH30264.01 or equivalent)
-
TrypLE express enzyme (Gibco, cat. no. 12604021)
-
Hepatic organoid basal (OB) medium (see recipe)
-
Hepatic endodermal organoid expansion medium (EM) (see recipe)
-
Tissue culture treated 6-well plates
-
Tissue culture treated 24-well plates
-
6-well clear flat bottom ultra-low attachment (ULA) plates (Corning, cat. no. 3471)
-
24-well clear ultra-low attachment (ULA) plates (Corning, cat. no. 3473)
-
15-ml Conical tubes
-
Pipette aid
-
10-ml Serological pipettes
-
1.5-ml Microcentrifuge tubes
-
Biosafety cabinet
-
Inverted microscope
-
37°C, 5% CO2 incubator
-
Centrifuge
-
Ice
Seeding hPSCs (day -10)
1.Prepare a 6-well plate coated with Matrigel (see Support Protocol) and 12 ml of pre-warmed mTeSR medium with 2 μM Y-27632 (1:10,000 [v/v] dilution) in a 15-ml conical tube.
2.When hPSCs are ready to passage, remove the culture medium and add 1 ml of 0.5 mM EDTA per well for a 6-well plate.
3.Incubate for 4 to 5 min at 37°C in a 5% CO2 incubator until the colony edges of the colonies begin to detach and lift from the plate.
4.Carefully remove the 0.5 mM EDTA to avoid dislodging the cells, and harvest with 1 ml of pre-warmed mTeSR medium with 2 μM Y-27632 per well. To inoculate one 6-well plate, collect a total of two wells.
5.Transfer 2 ml of harvested cells to a 15-ml conical tube containing 10 ml of pre-warmed mTeSR medium with 2 μM Y-27632 and mix gently.
6.Remove the used Matrigel solution completely from the Matrigel-coated 6-well plate.
7.Distribute 2 ml of cell suspension to each well of a Matrigel-coated 6-well plate and incubate at 37°C in the presence of 5% CO2 for one day.
DE differentiation from hPSCs (day -9 to day -4)
8.Prepare 12 ml of pre-warmed (37°C) DE medium 1.
9.Remove the culture medium from the hPSCs and add 2 ml of the DE medium 1 to each well.
10.Incubate the cells at 37°C with 5% CO2 for 24 hr.
11.Replace the medium with fresh pre-warmed (37°C) DE medium 2.
12.Incubate the cells for 4 days at 37°C with 5% CO2 and change the fresh DE medium 2 every 24 hr.
13.Perform flow cytometry with the CXCR4 antibody, using at least one well in the 6-well plate.
HE differentiation from DE cells (day -4 to day 0)
14.Prepare 12 ml of pre-warmed (37°C) HE medium.
15.Remove the culture medium of the DE cells and add 2 ml of the HE medium to each well.
16.Incubate the cells for 4 days at 37°C with 5% CO2 and change the fresh HE medium every 24 hr.
17.Perform flow cytometry with the EpCAM antibody, using at least one well in the 6-well plate.
Hepatic endodermal organoid production (day 0 to day 14)
18.After analyzing the EpCAM-positive cells, remove the culture medium and wash with 2 ml of DPBS per well.
19.Add 1 ml of TrypLE Express Enzyme to each well and incubate at 37°C for 10 min.
20.Transfer the individually dissociated cells into a 15-ml conical tube containing 10 ml of prewarmed (room temperature, RT) organoid basal (OB) medium.
21.Centrifuge 3 min at 200 × g , room temperature and remove supernatant without disturbing the pellet.
22.Resuspend the cell pellet in 1 ml of prewarmed (room temperature) OB medium and count cells using your preferred method.
23.Dispense 0.5 ml of cold hepatic endodermal expansion medium (EM) with 2 μM Y-27632 (1: 10,000 [v/v] dilution) into each well of a 24-well ULA plate.
24.Add 10,000 HE cells per well and incubate the plate at 37°C with 5% CO2 for 14 days. When placing the plate in the incubator and before closing the door, carefully spread the cells to ensure even distribution and prevent clumping.
25.Replace EM every 3 days until day 14.
Based on one well of a 24-well ULA plate:
- a) Using a 1000-µl pipette, gently resuspend the organoids and transfer them to a 1.5-ml microcentrifuge tube.
- b) Spin down the organoids with the microcentrifuge (max speed of 5000 rpm, room temperature) and discard the supernatant.
- c) Resuspend the pellet in 0.5 ml of fresh cold EM and transfer it to the plate. Incubate for 3 days more at 37°C with 5% CO2.
Basic Protocol 2: EXPANSION AND CRYOPRESERVATION OF HEPATIC ENDODERMAL ORGANOIDS
This protocol describes the methods for subculturing and freezing HEOs for stable long-term expansion in vitro. Once HEOs are generated, they can be expanded by subculturing under the defined culture conditions. HEOs are composed of hepatic progenitor-like cells that can develop into hepatocytes and cholangiocytes. During early subculture (passages 1 to 10), focus on selecting high-quality HEOs rather than on scale-up. Healthy HEOs are very thin, cyst-like organoids with excellent proliferative capacity. Organoids that are filled, have multiple layers, and are stuck together should be removed from the culture. Successful selection of healthy organoids will result in the exponential and reproducible expansion of HEOs in vitro. If the HEOs used for the next step (Basic Protocol 3) are contaminated with mycoplasma, they may not differentiate into hepatic organoids and die (i.e., the medium turns yellow within 3 days of differentiation).
Materials
-
Hepatic endodermal organoids (HEOs) (see Basic Protocol 1)
-
Hepatic endodermal organoid expansion medium (EM) (see recipe)
-
DPBS, without calcium and magnesium (Cytiva, cat. no. SH30028.02 or equivalent)
-
CryoStar CS10 (STEMCELL Technologies, cat. no. 100-1061)
-
Hepatic organoid basal (OB) medium (see recipe)
-
70% Ethanol
-
Y-27632, 20 mM (see recipe)
-
6-well clear flat bottom ultra-low attachment (ULA) plates (Corning, cat. no. 3471)
-
24-well clear ultra-low attachment (ULA) multiple well plates (Corning, cat. no. 3473)
-
1.5-ml Microcentrifuge tubes
-
15-ml Conical tubes
-
Pipette aid
-
10-ml Serological pipettes
-
1000-µl Pipette
-
Filter tips
-
Ice
-
Centrifuge
-
Microcentrifuge
-
Biosafety cabinet
-
Inverted microscope
-
37°C, 5% CO2 incubator
-
Cryogenic vials
-
Freezing container
-
Liquid nitrogen storage tank
Passage of organoids
1.When the HEOs are ready for passage, gently resuspend and transfer 1 ml of harvested organoids (two wells of a 24-well ULA plate) to a 1.5-ml microcentrifuge tubes.
2.Place the 1.5-ml microcentrifuge tubes on ice for 20 min.
3.Spin down with the microcentrifuge (max speed of 5000 rpm, room temperature).
4.Discard the supernatant, and wash with 1 ml of cold DPBS.
5.Resuspend the organoids in 200 µl of cold EM using filter tips and pipette up and down until the organoids have completely disrupted into fragments.
6.Add 500 µl of cold EM to each well of the 24-well ULA plate.
7.Transfer the 30 to 50 µl of fragmented organoids to each well.
8.Incubate for 6 days at 37°C and 5% CO2.
9.Change the medium every 3 days.
- a) Using a 1000-µl pipette, gently resuspend and transfer 1 ml of harvested organoids (two wells of a 24-well ULA plate) to a 1.5-ml microcentrifuge tube.
- b) Spin down the organoids with the microcentrifuge (max. 5000 rpm, room temperature) and discard the supernatant.
- c) Resuspend the pellet in 0.5 ml of fresh cold EM and transfer it to the plate. Incubate for further 3 days at 37°C with 5% CO2.
Observe HEO under a microscope and remove organoids filled inside.
Cryopreservation of organoids
10.When the HEOs are ready for cryopreservation, harvest the organoids and transfer them to a 1.5-ml microcentrifuge tube.
11.Place the tube on ice for 20 min.
12.Spin down with the microcentrifuge (max speed of 5000 rpm, room temperature) and remove the supernatant.
13.Resuspend the organoids in 200 µl of cold EM using filter tips and pipette up and down until the organoids have completely disrupted into fragments.
14.Wash with 1 ml of cold DPBS.
15.Add 1.5 ml of CryoStor CS10 to a 1.5-ml microcentrifuge tube containing fragmented organoids.
16.Transfer 500 µl aliquots to each labeled cryogenic vial.
17.Transfer cryogenic vials to a freezing container at −80°C, and after 24 hr transfer to a liquid nitrogen tank.
Thawing the organoids
18.Prepare 9.5 ml of pre-warmed (37°C) OB medium in a 15-ml conical tube.
19.Thaw a vial of organoids stock in a 37°C water bath until it is partially thawed, approximately 50% (a small piece of ice remains in the vial).
20.Remove the vial from the water bath and spray 70% ethanol to prevent contamination and wipe dry.
21.Open the cap and carefully add the contents of the vial to the pre-warmed 9.5 ml of OB medium.
22.Centrifuge 3 min at 150 × g , room temperature and discard the supernatant.
23.Resuspend the organoid pellets in 1.5 to 2 ml of cold EM containing 2 μM Y-27632 (1:10,000 [v/v] dilution).
24.Seed into 3 to 4 wells of 24-well ULA plate per cryogenic vial.
25.Incubate for 6 days at 37°C with 5% CO2.
26.Change the medium every 3 days.
Based on one well of a 24-well ULA plate:
- a) Using a 1000-µl pipette, gently resuspend the organoids and transfer them to a 1.5-ml microcentrifuge tube.
- b) Spin down the organoids in the microcentrifuge (max speed of 5000 rpm, room temperature) and discard the supernatant.
- c) Resuspend the pellet in 0.5 ml of fresh cold EM and transfer it to the plate. Incubate for further 3 days at 37°C with 5% CO2.
Basic Protocol 3: DIFFERENTIATION OF HEPATIC ORGANOIDS FROM HEPATIC ENDODERMAL ORGANOIDS
This protocol describes the method for efficient differentiation of hepatic organoids (HOs) from HEOs. Once the HEOs are established to be robust and for reproducible expansion, proceed to Basic Protocol 3 to produce HOs used for hepatotoxicity testing. During the differentiation process, large, thin, and cyst-shaped HEOs are converted into filled and spherical HOs through a self-organization process that gradually shrinks and aggregates. After 15 days of successful differentiation, HOs typically comprise more than 90% of ALB-positive cells and express major hepatic marker genes (ALB , HNF4A , and AAT) and CYP450 genes (CYP3A4 , CYP2C9 , and CYP2C19). Conversely, HOs can be filled with dead cells or fail to self-organize into spherical organoids during differentiation due to a variety of factors, including mycoplasma infection or mechanical stress.
Materials
-
Hepatic endodermal organoids (HEOs) (see Basic Protocol 2)
-
DPBS, without calcium and magnesium (Cytiva, cat. no. SH30028.02 or equivalent)
-
Hepatic endodermal organoid expansion medium (EM) (see recipe)
-
BMP-7, 25 µg/ml (see recipe)
-
Hepatic organoids differentiation medium (DM) (see recipe)
-
6-well flat bottom ultra-low attachment (ULA) plates (Corning, cat. no. 3471)
-
24-well clear ultra-low attachment (ULA) multiple well plates (Corning, cat. no. 3473)
-
1.5-ml Microcentrifuge tubes
-
1000-µl Pipette
-
Filter tips
-
Pipette Aid
-
10-ml Serological pipettes
-
15-ml Conical tubes
-
Ice
-
Biosafety cabinet
-
Inverted microscope
-
37°C, 5% CO2 incubator
-
Microcentrifuge
-
Centrifuge
HEO expansion (day 0 to day 6)
In this protocol, we explain how to make HOs for a 6-well ULA plate.
1.Harvest four wells of HEOs in a 24-well ULA plate and transfer them to two 1.5-ml microcentrifuge tubes.
2.Place the tube on ice for 20 min.
3.Spin down in the microcentrifuge (max speed 5000 rpm, room temperature) and remove the supernatant.
4.Wash with 1 ml of cold DPBS and repeat Step 3.
5.Add 200 µl of cold EM to each 1.5-ml microcentrifuge tube and resuspend the organoids using filter tips. Pipette up and down until the organoids have completely disrupted into fragments. Collect the fragmented HEOs into one tube.
6.Add 2 ml of cold EM with 25 ng/ml BMP-7 to each well of the 6-well ULA plate.
7.Transfer the 60 µl of fragmented organoids to each well.
8.Incubate for 6 days at 37°C with 5% CO2.
9.Change the medium every 3 days.
- a) Harvest the HEOs into a 15-ml conical tube using a 1000-µl pipette.
- b) Centrifuge 1 min at 150 × g , room temperature. Discard the supernatant.
- c) Add 1.5 ml of fresh cold EM containing 25 ng/ml BMP-7 for each well of the culture plate.
- d) Resuspend the HEO pellet in 3 ml of fresh cold EM containing 25 ng/ml BMP-7 and transfer 0.5 ml of HEO suspension to each well.
- e) Incubate for further 3 days at 37°C with 5% CO2.
HO differentiation (day 6 to day 21)
10.On day 6 of the culture, transfer the HEOs into a 15-ml conical tube.
11.Centrifuge 1 min at 150 × g , room temperature. Discard the supernatant.
12.Wash both HEOs and the culture plate with DPBS.
13.Add 1.5 ml of fresh cold DM for each well of the culture plate.
14.Resuspend the HEO pellet in 3 ml of fresh cold DM and aliquot 0.5 ml of HEO suspension per well.
15.Incubate for 15 days at 37°C with 5% CO2.
16.Change the medium every 3 days.
- a) Harvest the organoids into a 15-ml conical tube using a 1000-µl pipette.
- b) Centrifuge 1 min at 150 × g , room temperature and discard the supernatant.
- c) Add 1.5 ml of fresh cold DM for each well of the culture plate.
- d) Resuspend the organoid pellet in 3 ml of fresh DM and transfer 0.5 ml of organoid suspension to each well.
- e) Incubate for further 3 days at 37°C with 5% CO2.
Basic Protocol 4: EVALUATION OF HEPATOTOXICITY USING HEPATIC ORGANOIDS
The response of the liver to drug or chemical exposure can affect the survival of parenchymal cells, the hepatocytes, through cellular stress due to reactive metabolites produced by CYP450 enzymes. This protocol describes a simple method to assess the acute hepatotoxicity of drugs or compounds in HOs through cytotoxicity assay. Here, we detail that HOs were treated with a drug at a series of concentrations based on the maximum blood concentration of the drug (Cmax), the organoid survival rate was analyzed, and a dose-response curve for drug cytotoxicity was constructed. This protocol provides an example treatment of seven representative clinical drugs that cause drug-induced liver injury via CYP450 metabolism.
Materials
-
Hepatic organoids (HOs) (see Basic Protocol 3)
-
DPBS, without calcium and magnesium (Cytiva, cat. no. SH30028.02 or equivalent)
-
Hepatic organoid basal (OB) medium (see recipe)
-
Acetaminophen (see recipe)
-
Benzbromarone (see recipe)
-
Diclofenac (see recipe)
-
Flutamide (see recipe)
-
Ticlopidine (see recipe)
-
Troglitazone (see recipe)
-
Valproic acid (see recipe)
-
CellTiter-Glo® 3D cell viability assay kit (Promega, cat. no. G968A)
-
1000-µl Pipette
-
Filter tips
-
Pipette aid
-
10-ml Serological pipettes
-
15-ml Conical tubes
-
1.5-ml Microcentrifuge tubes
-
Centrifuge
-
Biosafety cabinet
-
96-well flat clear bottom white polystyrene tissue culture-treated microplates
-
37°C, 5% CO2 incubator
-
Reservoirs
-
Multi-channel pipette
-
Microplate mixer or rocking shaker
-
Microplate reader capable of detecting luminescence
-
GraphPad Prism software
When evaluating seven hepatotoxic drugs, two 6-well ULA plates of HOs are required.
1.Transfer the HOs in a 6-well ULA plate to a 15-ml conical tube using a 1000-µl pipette.
2.Centrifuge 1 min at 150 × g , room temperature. Discard the supernatant.
3.Wash the HOs in a conical tube with 5 ml of DPBS.
4.Resuspend the HO pellet in a conical tube with 10 ml of OB medium.
5.Centrifuge 1 min at 150 × g , room temperature. Discard the supernatant.
6.Resuspend the HO pellet in a conical tube with 3 ml of OB medium. Collect the HOs into one tube.
7.Transfer 1 ml aliquot to each 1.5-ml microcentrifuge tube.
8.Prepare a 1.5-ml microcentrifuge tube containing 0.5 ml of each drug diluted in OB medium at a different concentration (see Table 4).
9.Prepare another 1.5-ml microcentrifuge tube for each drug at a different concentration and transfer 100 µl of HOs to each tube via gentle pipetting. Spin down and discard the supernatant.
10.Add 400 µl of each drug diluted in OB medium at a different concentration to a microcentrifuge tube containing HOs.
11.Transfer 100 µl of HO and drug mixture to each well of the 96-well microplates. To use as a blank for viability assay, add 100 µl of only OB medium to the remaining 2 to 3 wells of each microplate.
12.Incubate for 24 hr at 37°C with 5% CO2.
13.Equilibrate the CellTiter-Glo 3D reagent to room temperature for 30 min before use. Mix gently by inverting.
14.Add 100 µl of CellTiter-Glo 3D reagent to each well of the 96-well microplates using a multichannel pipette.
15.Mix the plate with the microplate mixer at 600 rpm for 30 min at room temperature.
16.Measure the luminescence in a plate reader with an integration time of 0.25 to 1 sec per well.
17.Normalize the obtained values relative to the drug's vehicle control, visualize the dose-response relationship as nonlinear regression curves, and calculate the half-maximal inhibitory concentration (IC50) values using GraphPad Prism.
Support Protocol: HUMAN PLURIPOTENT STEM CELL CULTURE
hPSCs are the starting material for the generation of HEOs. The quality of hPSCs and their self-renewal and pluripotency abilities are important to successfully obtain DE cells, HE cells, and HEOs. Indeed, if the hPSCs used are unhealthy and contaminated with mycoplasma, these cells may not differentiate into DE cells and may die. hPSCs can lose their pluripotency during culture, owing to a variety of factors such as cellular stress (e.g., mechanical stress and low temperature) and mycoplasma infection. This protocol describes the cultivation of human induced pluripotent stem cells (iPSCs) using a feeder-free system and emphasizes the crucial aspect of maintaining their pluripotency.
Materials
-
Matrigel solution (see recipe)
-
mTeSR1 or mTeSR plus (STEMCELL Technologies, cat. no. 85850 or 100-0276)
-
Y-27632, 20 mM (see recipe)
-
Human iPSCs (e.g., HDF01 hiPSCs generated by reprogramming human dermal fibroblasts and provided by KAIST, Republic of Korea)
-
70% Ethanol
-
EDTA solution, 0.5 mM (see recipe)
-
CryoStor® CS10 (STEMCELL Technologies, cat. no. 07930)
-
Tissue culture treated 6-well and/or 24-well plates
-
15-ml Conical tubes
-
1.5-ml Microcentrifuge tubes
-
Pipet aid
-
10-ml Serological pipette
-
37°C, 5% CO2 incubator
-
Water bath, 37°C
-
Biosafety cabinet
-
Inverted microscope
-
Centrifuge
-
Cryogenic vials
-
Freezing container
-
Liquid nitrogen storage tank
Matrigel-coated tissue culture plate preparation
1.Add Matrigel solution to the plate (1 ml per well for 6-well plate and 0.5 ml per well for 24-well plate).
2.Incubate the plate at 37°C with 5% CO2 for 30 min for immediate use.
Thawing of hPSCs
3.Prepare a Matrigel-coated 6-well plate and pre-warmed mTeSR medium containing 2 μM Y-27632 (1: 10,000 [v/v] dilution).
4.Thaw a cryogenic vial of hPSCs in a 37°C water bath until a small piece of ice remains in the vial.
5.Spray the cryogenic vial with 70% ethanol and wipe dry.
6.Open the cap and carefully add 1 ml of warm mTeSR medium drop by drop. Mix the contents by gentle pipetting.
7.Carefully transfer the cell suspension to a 15-ml conical tube containing 9 ml of mTeSR medium.
8.Centrifuge 3 min at 200 × g , room temperature. Discard the supernatant.
9.During centrifugation, remove the excess Matrigel solution from a 6-well plate and add 1.5 ml of pre-warmed mTeSR containing 2 μM Y-27632 per well.
10.Resuspend the cell pellet with 1 to 1.5 ml of pre-warmed mTeSR containing 2 μM Y-27632; add 500 µl to each well of a 6-well plate.
11.Incubate overnight at 37°C with 5% CO2.
12.Replace the culture medium with fresh mTeSR medium.
13.Change the medium every day until the cells are ready for passage.
Passaging of hPSCs
14.Prepare a Matrigel-coated 6-well plate and pre-warmed mTeSR medium with 2 μM Y-27632 (1: 10,000 [v/v] dilution).
15.Remove the culture plate from the incubator and transfer it to a clean bench equipped with a microscope.
16.Remove the spent culture medium and add 1 ml of 0.5 mM EDTA per well.
17.Incubate at 37°C with 5% CO2 for 4 to 5 min until the edges of the colonies begin to loosen and lift from the plate.
18.Remove the 0.5 mM EDTA carefully so as not to detach the cells, harvest cells with 1 ml of mTeSR medium containing 2 μM Y-27632 with gentle pipetting, and move the cells to a 1.5-ml microcentrifuge tube.
19.Remove the Matrigel solution from the plate and add 2 ml of mTeSR medium containing 2 μM Y-27632 per well.
20.Plate the cells at the desired density (1:6 ratio) onto each well.
21.Incubate overnight at 37°C with 5% CO2.
22.Replace the culture medium with fresh mTeSR medium without Y-27632.
23.Change the medium every day until the colonies are ready for passage.
Cryopreservation of hPSCs
24.Examine the plate containing hPSCs under an inverted microscope. hPSCs used for cryopreservation should show no sign of microbial contamination.
25.Remove the spent culture medium and add 1 ml of 0.5 mM EDTA.
26.Incubate at 37°C with 5% CO2 for 4 to 5 min until colony edges begin to loosen and lift from the plate.
27.Remove the 0.5 mM EDTA carefully so as not to detach the cells and harvest cells with 1 ml of mTeSR medium via gentle pipetting, and transfer them into a 15-ml conical tube containing 9 ml of mTeSR medium.
28.Centrifuge 3 min at 200 × g , room temperature and carefully discard the supernatant.
29.Finger tap the tube gently to disperse the cell pellet.
30.Resuspend the cell pellet with 2 ml of CryoStor CS10.
31.Transfer 500 µl aliquots into each labeled cryogenic vial.
32.Transfer cryogenic vials to a freezing container at −80°C. After 24 hr, transfer to a liquid nitrogen tank.
REAGENTS AND SOLUTIONS
Prepare and store all reagents and solutions under aseptic conditions. When appropriate, filter sterilize using a 0.22 μm membrane filter (Corning, cat. no. 431098 or equivalent).
A83-01, 50 mM
- 10 mg A83-01 (Tocris Bioscience, cat. no. 2939 or equivalent)
- 475 µl Dimethyl sulfoxide (DMSO) (Sigma-Aldrich, cat. no. D2650 or equivalent)
- Store aliquots at −20°C or below for up to 1 month.
A83-01, 5 mM
- 50 µl A83-01, 50 mM (see recipe)
- 450 µl Dimethyl sulfoxide (DMSO) (Sigma-Aldrich, cat. no. D2650 or equivalent)
- Store aliquots at −20°C or below for up to 1 month.
Acetaminophen, 87.04 mM
- 131.5 mg Acetaminophen (Sigma-Aldrich, cat. no. A7085 or equivalent)
- 10 ml OB medium (see recipe)
- Prepare fresh before use.
Activin A, 100 μg/ml
- 100 μg Recombinant human/murine/rat activin A (PeproTech, cat. no. 120-14E or equivalent)
- 1 ml BSA, 0.1% (w/v) (see recipe)
- Store aliquots at −20°C or below for up to 12 months.
Albumin fraction V in RPMI-1640, 5 mg/ml
- 200 ml RPMI 1640 medium, with L-glutamine (Cytiva, cat. no. SH30027.01 or equivalent)
- 1 g Bovine serum albumin (BSA) (Sigma-Aldrich, cat. no. A7906 or equivalent)
- Filter sterilize; store at 4°C for up to 2 to 3 weeks.
Benzbromarone, 100 mM
- 10 mg Benzbromarone (Sigma-Aldrich, cat. no. Y0000775)
- 235.804 µl Dimethyl sulfoxide (DMSO) (Sigma-Aldrich, cat. no. D2650 or equivalent)
- Store aliquots at −20°C or below for up to 1 month.
BMP-4, 100 μg/ml
- 100 μg Recombinant human BMP-4 (PeproTech, cat. no. 120-05ET or equivalent)
- 0.5 ml 5 mM HCl (Sigma-Aldrich, cat. no. H9892, 1 N or equivalent) diluted in BSA, 0.1% (w/v)
- 0.5 ml BSA, 0.1% (w/v) (see recipe)
- Reconstitute BMP-4 in 5 mM HCl and further dilute to BSA, 0.1% (w/v).
- Store aliquots at −20°C or below for up to 3 months.
BMP-7, 25 μg/ml
- 25 μg Recombinant human BMP-7 (PeproTech, cat. no. 120-03 or equivalent)
- 1 ml BSA, 0.1% (w/v) (see recipe)
- Store aliquots at −20°C or below for up to 7 months.
BSA, 0.1% (w/v)
- 10 mg Bovine serum albumin (BSA) (Sigma-Aldrich, cat. no. A7906 or equivalent)
- 10 ml DPBS, with calcium and magnesium (Cytiva, cat. no. SH30264.01 or equivalent)
- Filter sterilize and store at −20°C for up to 3 months.
CHIR99021, 30 mM
- 5 mg CHIR99021 (Sigma-Aldrich, cat. no. SML1046 or equivalent)
- 358 µl Dimethyl sulfoxide (DMSO) (Sigma-Aldrich, cat. no. D2650 or equivalent)
- Store aliquots at −20°C for up to 3 months.
CHIR99021, 3 mM
- 10 µl CHIR99021, 30 mM (see recipe)
- 90 µl Dimethyl sulfoxide (DMSO) (Sigma-Aldrich, cat. no. D2650 or equivalent)
- Store aliquots at −20°C for up to 3 months.
Complete RPMI-1640 medium
- 440 ml RPMI 1640 medium, with L-glutamine (Cytiva, cat. no. SH30027.01 or equivalent)
- 50 ml 5 mg/ml Albumin fraction V in RPMI-1640 (see recipe)
- 10 ml B-27 supplement, serum-free (Thermo Fisher Scientific, cat. no. 17504001 or equivalent)
- Store at 4°C for up to 2 to 3 weeks.
DAPT, 100 mM
- 5 mg DAPT (Sigma-Aldrich, cat. no. D5942 or equivalent)
- 115.6 µl Dimethyl sulfoxide (DMSO) (Sigma-Aldrich, cat. no. D2650 or equivalent)
- Store aliquots at −20°C for up to 3 months.
Dexamethasone, 30 mM
- 10 mg Dexamethasone (Sigma-Aldrich, cat. no. D4902 or equivalent)
- 849 µl Ethanol (Sigma-Aldrich, cat. no.1009831011 or equivalent)
- Store aliquots at −20°C for up to 3 months.
Definitive endoderm medium 1 (DE medium 1)
- 10 ml Complete RPMI-1640 medium (see recipe)
- 5 µl Activin A, 100 μg/ml (see recipe)
- 10 µl Sodium butyrate, 1 M (see recipe)
- 1 µl CHIR99021, 3 mM (see recipe)
- Prepare fresh before use, final concentrations are as follows: 50 ng/ml activin A, 1 mM sodium butyrate, 3 μM CHIR99021 in complete RPMI-1640 medium.
Definitive endoderm medium 2 (DE medium 2)
- 10 ml Complete RPMI-1640 medium (see recipe)
- 5 µl Activin A, 100 μg/ml (see recipe)
- 1 µl Sodium butyrate, 1 M (see recipe)
- Prepare fresh before use, final concentrations are as follows: 50 ng/ml activin A, 1 mM sodium butyrate in complete RPMI-1640 medium.
Diclofenac, 3.017 mM
- 9.59 mg Diclofenac (Sigma-Aldrich, cat. no. D6899 or equivalent)
- 10 ml OB medium (see recipe)
- Prepare fresh before use.
EDTA solution, 0.5 mM
- 50 ml DPBS, without calcium and magnesium (Cytiva, cat. no. SH30028.02 or equivalent)
- 50 µl EDTA, 0.5 M, pH 8.0 (Promega, cat. no. V4231 or equivalent)
- Store at room temperature for up to 6 months.
FACS buffer
- 500 ml MACS buffer (Miltenyi Biotec, cat. no. 130-091-221)
- 0.5 g BSA (Sigma-Aldrich, cat. no. A7906)
- Filter the buffer into sterile containers with a 0.22 μm membrane filter. Store the buffer for up to 1 month at 4°C.
Allow the BSA to dissolve at room temperature without stirring.
FGF-10, 50 μg/ml
- 50 μg Recombinant human FGF-10 (PeptroTech, cat. no. 100-26 or equivalent)
- 0.1 ml Sodium phosphate, 5 mM, pH 7.4 (Biosesang, Gyeonggi-do, Korea; cat. no. SR2172-050-74 or equivalent)
- 0.9 ml BSA, 0.1% (w/v) (see recipe)
- Reconstitute FGF-10 in 5 mM sodium phosphate and further dilute to BSA, 0.1% (w/v).
- Store aliquots at −20°C or below for up to 3 months.
FGF-19, 100 μg/ml
- 100 μg Recombinant human FGF-19 (e.g., PeproTech, cat. no. 100-32 or equivalent)
- 0.1 ml Sodium phosphate, 10 mM, pH 8.0 (Biosesang, Gyeonggi-do, Korea; cat. no. R2187-550-80 or equivalent)
- 0.9 ml BSA, 0.1% (w/v) (see recipe)
- Reconstitute FGF-19 in 10 mM sodium phosphate and further dilute to BSA, 0.1% (w/v).
- Store aliquots at −20°C or below for up to 12 months.
Flutamide, 1M
- 276.21 mg (Sigma-Aldrich, cat. no. F9397 or equivalent)
- 1 ml Ethanol (Sigma-Aldrich, cat. no. 1009831011 or equivalent)
- Store aliquots at −20°C or below for up to 1 month.
Forskolin, 50 mM
- 50 mg Forskolin (Tocris, cat. no. 1099 or equivalent)
- 2.44 ml Dimethyl sulfoxide (DMSO) (Sigma-Aldrich, cat. no. D2650 or equivalent)
- Store aliquots at −20°C or below for up to 1 month.
[Leu15]-Gastrin I human, 100 μM
- 0.1 mg [Leu15]-Gastrin I human (Sigma-Aldrich, cat. no. G9145)
- 481 µl Water, high-purity and sterile with 25 mM NaOH (Sigma-Aldrich, cat. no. 1091371000 or equivalent)
- Store aliquots at −20°C for up to 2 months.
Hepatic endodermal organoid expansion medium (EM)
- Advanced DMEM/F12 (Thermo Fisher Scientific, cat. no. 12634010 or equivalent)
- HEPES, 1 M (Thermo Fisher Scientific, cat. no. 15630080 or equivalent)
- GlutaMAX supplement (Thermo Fisher Scientific, cat. no. 35050061 or equivalent)
- Penicillin-streptomycin (Thermo Fisher Scientific, cat. no. 15070063 or equivalent)
- BSA (Sigma-Aldrich, cat. no. A7906 or equivalent)
- N-2 supplement (Thermo Fisher Scientific, cat. no. 17502-048 or equivalent)
- B-27 supplement, minus vitamin A (Thermo Fisher Scientific, cat. no. 12587-010 or equivalent)
- Nicotinamide (Sigma-Aldrich, cat. no. N0636 or equivalent)
- N-acetyl-L-cysteine (Sigma-Aldrich, cat. no. A9165 or equivalent)
- FGF-10, 50 μg/ml (see recipe)
- HGF, 50 μg/ml (see recipe)
- [Leu15]-Gastrin I human, 100 μM (see recipe)
- A83-01, 50 mM (see recipe)
- Forskolin, 50 mM (see recipe)
- CHIR99021, 30 mM (see recipe)
- Matrigel (Corning, cat. no. 354230)
- Medium must be made the day before use. See Table 1 for volumes and concentrations. Mix the components except Matrigel. Filter the medium with a 0.22 μm syringe filter. Add 0.32–0.4 mg/ml of Matrigel and vigorously pipette a greater number of times using a 10-ml serological pipette.
Insufficient pipetting causes Matrigel gelation within the medium and settle to the bottom.
- Store at 4°C for up to 2–3 weeks.
Component | Stock concentration | Final concentration | Volume for 50 ml |
---|---|---|---|
Advanced DMEM/F12 | – | – | Up to 50 ml |
HEPES | 1 M | 10 mM | 500 µl |
GlutaMAX™ supplement | 100X | 1X | 500 µl |
Penicillin-Streptomycin |
5000 U/ml Penicillin; 5000 μg/ml Streptomycin |
50 U/ml Penicillin; 50 μg/ml Streptomycin |
500 µl |
BSA | – | 0.1% (w/v) | 50 mg |
N-2 supplement, 100X | 100X | 1X | 500 µl |
B-27 supplement, 50X, minus vitamin A | 50X | 1X | 1 ml |
Nicotinamide | – | 10 mM | 61 mg |
N-acetyl-L-cysteine | 1.25 M | 1.25 mM | 50 µl |
FGF-10 | 50 μg/ml | 50 ng/ml | 50 µl |
HGF | 50 μg/ml | 25 ng/ml | 25 µl |
[Leu15]-Gastrin I human | 100 μM | 10 nM | 5 µl |
A83-01 | 50 mM | 5 μM | 5 µl |
Forskolin | 50 mM | 10 μM | 10 µl |
CHIR99021 | 30 mM | 3 μM | 5 µl |
Matrigela | Lot number dependent | 0.32 – 0.4 mg/ml | Lot number dependent |
- a Aliquot Matrigel according to the recommended dilution factor on the Certificate of Analysis, and then freeze. Always keep Matrigel on ice during thawing and handling, to prevent gelling.
Hepatic endoderm medium (HE medium)
- 10 ml Complete RPMI-1640 medium (see recipe)
- 5 µl BMP-4, 100 μg/ml (see recipe)
- 10 µl SB431542, 10 mM (see recipe)
- Prepare fresh before use.
Hepatic organoids basal medium (OB medium)
- Advanced DMEM/F12 (Thermo Fisher Scientific, cat. no. 12634010 or equivalent)
- HEPES, 1 M (Thermo Fisher Scientific, cat. no. 15630080 or equivalent)
- GlutaMAX supplement (Thermo Fisher Scientific, cat. no. 35050061 or equivalent)
- Penicillin-streptomycin (Thermo Fisher Scientific, cat. no. 15070063 or equivalent)
- Refer to Table 3 for volumes and concentrations. Mix the components and filter the medium with a 0.22 μm syringe filter to sterilize. Store the medium at 4°C for up to 2 to 3 weeks.
Hepatic organoid differentiation medium (DM)
- Advanced DMEM/F12 (Thermo Fisher Scientific, cat. no. 12634010 or equivalent)
- HEPES, 1 M (Thermo Fisher Scientific, cat. no. 15630080 or equivalent)
- GlutaMAX supplement (Thermo Fisher Scientific, cat. no. 35050061 or equivalent)
- Penicillin-streptomycin (Thermo Fisher Scientific, cat. no. 15070063 or equivalent)
- BSA (Sigma-Aldrich, cat. no. A7906 or equivalent)
- B-27 Supplement, minus vitamin A (Thermo Fisher Scientific, cat. no. 12587-010 or equivalent)
- Nicotinamide (Sigma-Aldrich, cat. no.N0636 or equivalent)
- N-acetyl-L-cysteine, (Sigma-Aldrich, cat. no. A9165 or equivalent)
- BMP-7, 25 μg/ml (see recipe)
- FGF-19, 100 μg/ml (see recipe)
- HGF, 50 μg/ml (see recipe)
- A83-01, 5 mM (see recipe)
- DAPT, 100 mM (see recipe)
- Dexamethasone, 10 mM (see recipe)
- Matrigel (Corning, cat. no. 54230)
- Medium must be made the day before use. See Table 2 for volumes and concentrations. Mix the components except Matrigel. Filter the medium with a 0.22 μm syringe filter. Add 0.32 to 0.4 mg/ml of Matrigel and vigorously pipette a great number of times using a 10-ml serological pipette.
Component | Stock concentration | Final concentration | Volume for 50 ml |
---|---|---|---|
Advanced DMEM/F12 | – | – | Up to 50 ml |
HEPES | 1 M | 10 mM | 500 µl |
GlutaMAX™ supplement | 100X | 1X | 500 µl |
Penicillin-Streptomycin |
5000 U/ml Penicillin; 5000 μg/ml Streptomycin |
50 U/ml Penicillin; 50 μg/ml Streptomycin |
500 µl |
BSA | – | 0.1% (w/v) | 50 mg |
B-27 supplement, 50X, minus vitamin A | 50X | 1X | 1 ml |
Nicotinamide | – | 10 mM | 61 mg |
N-acetyl-L-cysteine | 1.25 M | 1.25 mM | 50 µl |
BMP-7 | 25 μg/ml | 25 ng/ml | 50 µl |
FGF-19 | 100 μg/ml | 100 ng/ml | 50 µl |
HGF | 50 μg/ml | 25 ng/ml | 25 µl |
A83-01 | 5 mM | 0.5 μM | 5 µl |
DAPT | 100 mM | 10 μM | 5 µl |
Dexamethasone | 10 mM | 3 μM | 15 µl |
Matrigela | Lot number dependent | 0.32 – 0.4 mg/ml | Lot number dependent |
- a
Aliquot Matrigel according to the recommended dilution factor on the Certificate of Analysis, and then freeze. Always keep Matrigel on ice during thawing and handling, to prevent gelling.
Component | Stock concentration | Final concentration | Volume for 50 ml |
---|---|---|---|
Advanced DMEM/F12 | – | – | Up to 50 ml |
HEPES | 1 M | 10 mM | 500 µl |
GlutaMAX™ supplement | 100X | 1X | 500 µl |
Penicillin-Streptomycin |
5000 U/ml Penicillin; 5000 μg/ml Streptomycin |
50 U/ml Penicillin; 50 μg/ml Streptomycin |
500 µl |
BSA | – | 0.1% (w/v) | 50 mg |
Drug | Cmax (μM) | Conc. of treatment | Vehicle control | Number of plate wells required (includes four replicates) |
---|---|---|---|---|
Acetaminophen | 136 | 0, 1, 5, 10, 20, 40, 80, 160, 320, 640-fold Cmax | OB medium | 40 wells |
Benzbromarone | 23.58 | 0, 1, 2.5, 5, 10, 20, 30-fold Cmax | 0.4% DMSO | 28 wells |
Diclofenac | 9.43 | 0, 1, 5, 10, 20, 40, 80, 160, 320-fold Cmax | OB medium | 36 wells |
Flutamide | 5.43 | 0, 1, 5, 10, 20, 40, 80, 160, 320-fold Cmax | 0.1% Ethanol | 36 wells |
Ticlopidine | 8.07 | 0, 1, 5, 10, 20, 40, 80, 160, 320-fold Cmax | OB medium | 36 wells |
Troglitazone | 6.08 | 0, 1, 5, 10, 20, 40, 80-fold Cmax | 0.4% DMSO | 28 wells |
Valproic acid | 693.43 | 0, 1, 5, 10, 20, 40, 80, 160, 320-fold Cmax | OB medium | 36 wells |
Insufficient pipetting causes Matrigel gelation within the medium and settling to the bottom.
- Store at 4°C for up to 2 to 3 weeks.
HGF, 50 μg/ml
- 50 μg Recombinant human HGF (e.g., PeproTech, cat. no. 100-39H or equivalent)
- 1 ml 0.1% BSA (w/v) (see recipe)
- Store aliquots at −20°C or below for up to 3 months.
Matrigel solution
- 25 ml DMEM/-F12 (Thermo Fisher Scientific, cat. no. 11330032 or equivalent)
- Matrigel, hESC-qualified matrix (Corning, cat. no. 354277)
- Storage at 4°C for up to 2–3 weeks.
Matrigel should be aliquoted according to the recommended dilution factor on the Certificate of Analysis and frozen. Always keep Matrigel on ice during thawing and handling to prevent it from gelling. The dilution factor applies to 25 ml of the medium.
N-acetyl-L-cysteine, 1.25 M
- 2 g N-acetyl-L-cysteine (Sigma-Aldrich, cat. no. A9165 or equivalent)
- 10 ml Water, high-purity and sterile
- Filter the solution with a 0.22 μm syringe filter. Store aliquots at −20°C for up to 1 month.
Permeabilization buffer
- 0.05 ml Triton X-100 (Sigma-Aldrich, cat. no. 93443 or equivalent)
- 50 ml FACS buffer (see recipe)
- Store at 4°C for up to 1 month.
Sodium butyrate, 1 M
- 1.1 g Sodium butyrate (Sigma-Aldrich, cat. no. B5887 or equivalent)
- 10 ml Water, high-purity and sterile
- Store aliquots at −20°C for up to 3 months.
SB431542, 10 mM
- 10 mg SB431542 (Tocris, cat. no. 1614 or equivalent)
- 2.6 ml Dimethyl sulfoxide (DMSO) (Sigma-Aldrich, cat. no. D2650 or equivalent)
- Store aliquots at −20°C or below for up to 1 month.
Ticlopidine, 2.582 M
- 7.75 mg Ticlopidine (Sigma-Aldrich, cat. no. T6654 or equivalent)
- 10 ml OB medium (see recipe)
- Prepare fresh before use.
Troglitazone, 121.6 mM
- 5 mg Troglitazone (Sigma-Aldrich, cat. no. T2573 or equivalent)
- 93.125 µl Dimethyl sulfoxide (DMSO) (Sigma-Aldrich, cat. no. D2650 or equivalent)
- Store aliquots at −20°C or below for up to 1 month.
Valproic acid, 221.897 mM
- 368.76 mg Valproic acid (Sigma-Aldrich, cat. no. P4543 or equivalent)
- 10 ml OB medium (see recipe)
- Prepare fresh before use.
Y-27632, 20 mM
- 10 mg Y-27632 dihydrochloride (Tocris, cat. no. 1254)
- 1.56 ml Water, high-purity and sterile
- Store aliquots at −20°C or below for up to 1 month.
COMMENTARY
Background Information
The human liver is a central organ that manages the endogenous and exogenous metabolism in the body (Tanaka et al., 2011). Most drugs taken orally are metabolized in the liver and then circulated throughout the body. Therefore, the liver is the initial target for drug-induced damage and is one of the most important organs to evaluate safety in the development of new drugs. Drug-induced hepatotoxicity or drug-induced liver injury (DILI) is a major cause of drug failure in the case of clinical trials and post-approval withdrawal (Larrey & Pageaux, 2005; Walker et al., 2020). Animal models used in preclinical trials are often ineffective in predicting human drug toxicity owing to interspecies differences, such as the expression of drug-metabolizing enzymes (Mariotti et al., 2018; Van Norman, 2019). Primary human hepatocytes (PHHs) are the gold standard of in vitro hepatic models (LeCluyse, 2001; Sivaraman et al., 2005); however, their use is restricted by limited accessibility to fresh human liver tissue and unstable functional maintenance in vitro (Castell et al., 2006). These limitations suggest the need to develop more efficient in vitro human hepatic cell models.
Recent advances in human pluripotent stem cell (hPSC) technology and the discovery of three-dimensional (3D) organoids offer novel approaches for generating hepatic models in vitro. hPSCs have the ability to self-renew and differentiate into all types of cells that make up the body. There are embryonic stem cells (ESCs) derived from the inner cell mass of the blastocyst (Thomson et al., 1998) and induced pluripotent stem cells (iPSCs) generated by the reprogramming of somatic cells into a pluripotent stem cell-like status (Yu et al., 2007). There have been many efforts to apply hPSCs for toxicity assessment, since they represent human primary cell types that have not been transformed or derived from unstable tumors, avoiding species differences as well as the non-physiological status of tumor cells. In particular, iPSCs provide unlimited cell supply and high reproducibility, allowing personalized drug testing (Natale et al., 2019). Testing of drugs and environmental chemicals for effects on the liver is of particular importance since DILI and chemical-induced hepatotoxicity are commonly observed adverse effects. In addition, the metabolic activity of the liver defines the toxicokinetic behavior of substances as well as drug interactions. Initial hPSC-based hepatotoxicity models have focused on the development of two-dimensional (2D) hepatocyte-like cell cultures. Although 2D hepatocyte-like cells are simple to culture and scalable for high-throughput screening, they have the disadvantage of a limited differentiation status that is more similar to fetal liver and also provide poor functionality (Baxter et al., 2015; Natale et al., 2019). In particular, the expression and activity of drug-metabolizing enzymes are very low or absent in hPSC-derived 2D hepatocyte-like cells (Park et al., 2015). Although there has been a transition from 2D to 3D spheroid culture to mimic liver physiology, there are still limitations in drug metabolic capability (Yang et al., 2023).
Organoids are 3D cellular structures derived from stem cells that self-renew and self-organize to mimic the structure and function of organs (Marsee et al., 2021). Hepatic organoids (HOs) have the potential to serve as a new model to replace or reduce animal studies and simulate human liver physiology better than current models to facilitate an accurate evaluation and prediction of drug safety. They can be derived from liver tissue-resident stem/progenitor cells, or differentiated from hPSCs, and are capable of proliferation and self-organization (Prior et al., 2019). To establish human liver organoids, bile duct fragments were embedded in an extracellular matrix-rich 3D environment and cultured in a medium containing R-spondin 1, other growth factors, and small molecules (Huch et al., 2015). 3D structures began to appear within a few days and could be serially-passaged for several months. They displayed high levels of genetic stability and were functionalized into hepatocytes and cholangiocytes after the differentiation process. Some groups have generated highly expandable sources of hepatic endodermal or hepatic organoids from hPSCs (Akbari et al., 2019; Mun et al., 2019; Wang et al., 2019). These organoids were ECM-embedded and expanded into epithelial cysts under culture in R-spondin 1-based medium, morphologically resembling the bile duct-derived organoids. Also, they could be matured to perform certain hepatic functions, providing disease modeling, hepatotoxicity, and alcoholic liver injury studies.
However, as in vitro models for drug toxicity and efficacy testing, HOs should ideally be reproducibly generated and exhibit drug metabolism and transport functions comparable to those of the adult liver and PHHs. Hepatocytes, the parenchymal cells of the liver, are primarily responsible for the biotransformation of drugs and lipophilic xenobiotics by cytochrome P450 (CYP450) enzymes, which are mainly involved in drug metabolism and toxicity (Zanger & Schwab, 2013). Therefore, determining whether the HOs can recapitulate CYP450-mediated metabolic processes and toxic responses for use in drug testing is important. In a recent study, we developed an advanced protocol to generate expandable hepatic endodermal organoids (HEOs) from hPSCs and then produce HOs with high CYP450 expression and activity through a differentiation process (Kim et al., 2022). The difference from the previous protocol is the composition of the organoid culture medium. General culture medium for expanding liver or hepatic organoids from adult liver tissue, PHH, or hPSCs contains R-spondin 1 and EGF (Akbari et al., 2019; Hu et al., 2018; Huch et al., 2015; Mun et al., 2019; Wang et al., 2019). In this protocol, we were able to generate and expand HEOs without R-spondin 1 and EGF, which had a significant impact on increasing the expression of hepatic markers and CYP450 genes after HO differentiation. Because the use of recombinant R-spondin 1 greatly increases the cost of organoid culture, the removal of R-spondin 1 is practical for large-scale toxicity testing and drug screening. In addition to ECM embedding, we further established an organoid culture method, which involves culturing HEOs and HOs by suspension in a medium containing ECM (ECM suspension). The HEOs and HOs were reproducibly produced in ECM suspension, which had sufficient proliferation capacities and functionalities for toxicity screening with advantages in organoid handling and massive culture.
Hepatocyte death is one of the main features of drug-induced hepatotoxicity. Apoptosis and necrosis are mainly induced by DILI, and the toxic drugs largely determine the mechanism and extent of cell death (Iorga & Dara, 2019). CYP450-mediated metabolism, which is an important pathway for detoxifying many drugs, can also generate reactive metabolites that accumulate to cytotoxic concentrations, leading to hepatocyte toxicity (Guengerich, 2006). To date, in vitro drug and chemical testing has performed measurements of ATP content to analyze cell viability after exposure to various concentrations, based on the maximum blood concentration of the drug (Cmax) (Cox et al., 2020). Previous studies have shown that 3D hepatic models exhibit higher sensitivity for detecting hepatotoxic chemicals compared to 2D cultures (Gaskell et al., 2016; Lee et al., 2021; Wang et al., 2015; Zhou et al., 2019). The use of 2D cell line systems leads to the misconception that DILI is caused by compounds acting directly on the tested cells, ignoring the effects that the compounds may have through 3D environmental conditions (e.g., cell-cell and cell-ECM interactions) (Yang et al., 2023). Also, in vitro hepatic models aimed at recapitulating liver function and metabolic capacity should ideally be performed in 3D culture (Lee et al., 2021; Ramaiahgari et al., 2017; Zhou et al., 2019). In a recent study, we compared the cytotoxicity of seven hepatotoxic drugs among HOs, PHHs, HepG2 cells, and hPSC-derived 2D hepatocyte-like cells using dose-response curves (Kim et al., 2022). For most drugs, HOs and PHHs showed higher sensitivity at lower concentrations.
HOs still have limitations, including the lack of a protocol to produce uniform-sized organoids containing fully functional hepatocytes, immune environment, oxygen and nutrient supply in culture, and liver zonation. In this protocol, we focused on the optimization of the HO culture protocol to improve the differentiation process and to overcome the problem of partial differentiation into fetal hepatocytes that is associated with low expression of CYP450s in many hPSC-derived hepatic cell models. In a previous study (Kim et al., 2022), the enhanced expression and activities of CYP450s in HOs were significantly correlated with epigenetic changes such as decreased DNA methylation in these transcriptional regulatory regions. In addition, hepatocyte polarity is not typically found in hPSC-derived hepatic cell models because the bile canalicular network is formed at the end of fetal liver development. However, HOs significantly improved the expression and activity of apical drug transporters by recapitulating hepatocyte polarity. Moreover, this protocol has the advantage of producing organoids containing homogeneous hepatocytes through a simple differentiation process; HEOs are like hepatic progenitor cells that can be sub-cultured and cryopreserved. The use of bioreactors can also facilitate the production of scalable and uniformly sized organoids. HOs do not include non-parenchymal cells, such as Kupffer cells, that can mediate hepatotoxicity. Limitations with respect to hepatotoxicity caused by immune responses can be solved by differentiating the same hPSCs in parallel into immune cells and other non-parenchymal cells, and co-culturing them with HEOs or HOs to form a more physiological multicellular organoid. Finally, by combining organoid-on-a-chip technology with this protocol, it will be possible to overcome limitations in respect to oxygen and nutrient supply, liver zonation, and organ-to-organ interactions.
Critical Parameters
It is essential to have good quality starting material. If hESCs or hiPSCs are not growing well, consider thawing a new vial, or changing to new batches of mTeSR. Additionally, mycoplasma contamination of hPSCs must be prevented to ensure efficient and reproducible differentiation into DE and HE cells. After generating HEOs from hPSCs, high-quality organoids must be selected and sub-cultured to ensure HEOs with good proliferative capacity. HEOs in good condition appear as single-cell layered cysts and grow rapidly to over 500 μm in diameter within 5 to 6 days. In addition, mycoplasma contamination of HEOs results in failure of HO differentiation (i.e., dead cells accumulate in the lumen of HOs). At the end of HO differentiation, it is necessary to determine whether it has the morphological features of good Hos before proceeding to the next step. Good HOs with a clear lumen and border are highly correlated with high maturity and functionality. To ensure the relevant and reproducible results in hepatotoxicity testing, HOs should be used within 3 to 4 days after differentiation. Additionally, when HOs are exposed to drugs, an OB medium that excludes additional growth factors and chemicals must be used to avoid interference with the toxic response.
Troubleshooting
See Table 5 for a troubleshooting guide regarding organoid generation, expansion, and differentiation.
Problem | Possible cause | Solution |
---|---|---|
DE cell death at day 1 – 2 of differentiation | Low seeding density of hPSCs | Increase the number of hPSCs by about 1.5-fold |
Mycoplasma contamination | Test mycoplasma contamination and remove it with antibiotic treatment (e.g., BM-Cyclin, Roche, cat. no. 10799050001) during 2 – 3 passages | |
Poor HEO generation efficiency | Poor DE and HE differentiation efficiency | Restart the experiment. First, confirm the best morphological features (100% confluency and homogeneous cell types) of DE and HE cells. Second, check the DE and HE differentiation efficiency by flow cytometry (over 90% of CXCR4-positive cells in DE and EpCAM-positive cells in HE) |
Loss of HEOs when medium changes (i.e., after 3 days of passage) | Insufficient spin down or centrifugation | Matrigel gelation of culture medium interferes with harvesting HEOs. Gently pipette using a 1000-µl pipette before spin down or centrifugation |
Low average number of HEOs | Insufficient mechanical dissociation of the organoids | Pipette a greater number of times using a 200-µl pipette |
Poor expanding HEO size | Inactive forskolin | Use fresh forskolin or change to a new batch |
Poor HO viability | Mycoplasma contamination | Test mycoplasma contamination in HEOs and remove it with antibiotic treatment (e.g., BM-Cyclin, Roche, cat. no. 10799050001) |
Understanding Results
In the DE differentiation stage of Basic Protocol 1, hPSCs begin to differentiate in one day and appear dense with a uniform, flat morphology throughout the culture by day 5 (Fig. 2A). On day 4 of differentiation, the cells should be more than 90% CXCR4-positive (Fig. 2B). If DE differentiation is successful, HE cells with a uniform shape can also be obtained in the next step (Fig. 2A) and the proportion of EpCAM-positive cells is high at over 90% (Fig. 2C). The differentiation efficiency of DE and HE is important for the successful HEO generation in Basic Protocol 1. When organoid culture is performed using HE cells with high differentiation efficiency, cyst-shaped HEOs can be obtained after 14 days (Fig. 2A). Approximately 10,000 HE cells will give rise to 100 to 140 HEOs. They are sub-cultured at a 1:2 ratio according to Basic Protocol 2 and cultured in an expansion medium for 6 days; they will proliferate again into cyst-shaped HEOs (Fig. 3). In the early passage, some HEOs are observed as aggregated, filled, multilayered, and attached together during culture (Fig. 3). They should be picked up and removed during culture using a 200-µl pipette, and the remaining good HEOs are passaged at 1:4 ratio. Successful removal of bad organoids results in exponential and reproducible expansion of HEOs with up to over passage 30 (Fig. 3).
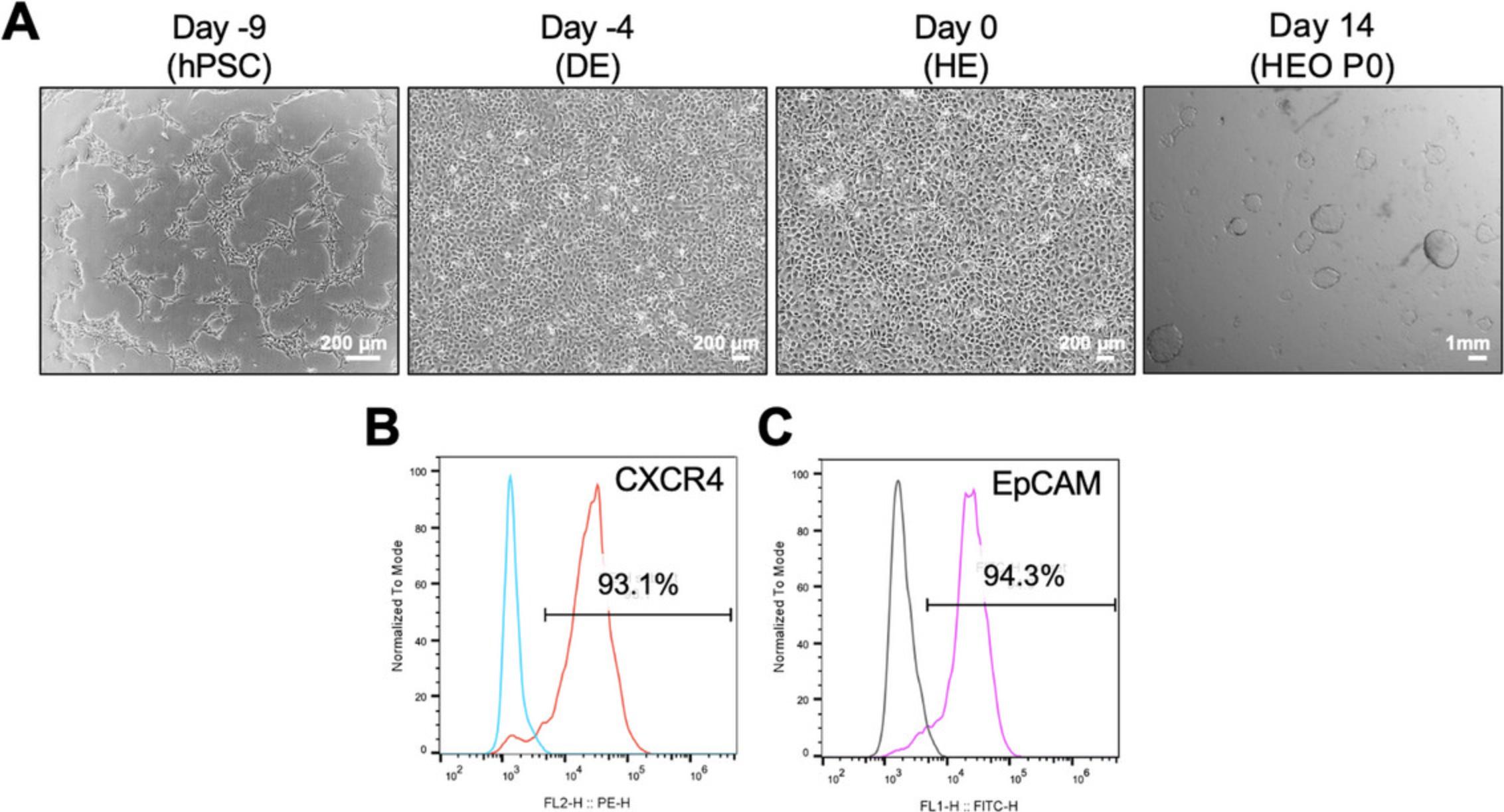
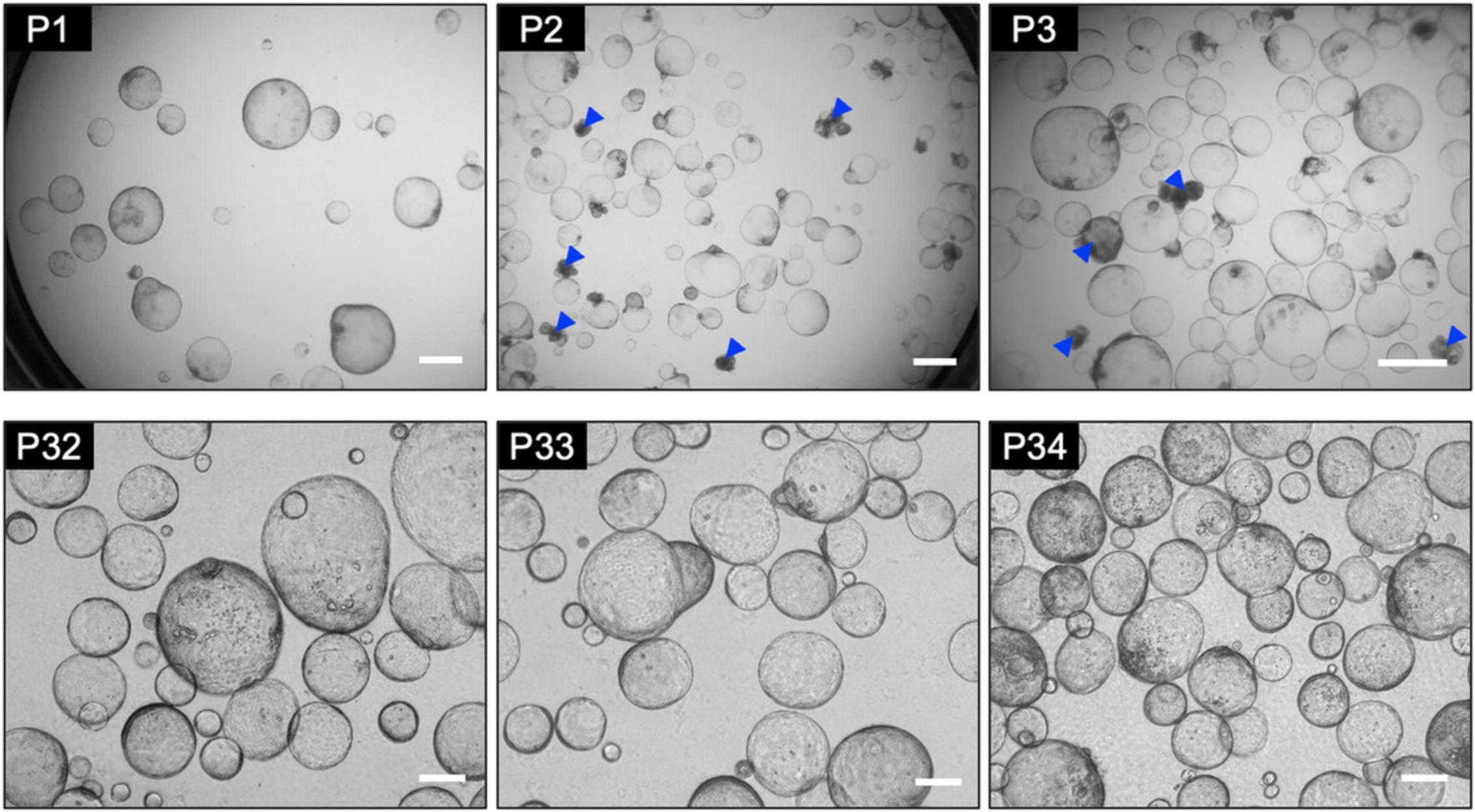
By following Basic Protocol 3, investigators should obtain good HOs similar to the examples shown in Figure 4A. HEOs with a diameter of more than 500 μm undergo a self-organization process as differentiation begins, and the layers gradually contract and thicken, eventually generating HOs that fold into a spherical shape to form a small lumen (Fig. 4A). HOs that form successfully are approximately 200 μm in diameter, have a well-defined border and clear lumen, and should typically be bright when observed under a brightfield microscope (Fig. 4A). In contrast, failure to properly differentiate results in abnormal HOs that are filled with dead cells, are not self-organized, and appear dark (Fig. 4A). Successful HO generation results in organoids comprising over 90% of ALB-positive cells (Fig. 4B). Additionally, HOs should have high expression of major hepatic marker genes (ALB , HNF4A , and AAT) and CYP450 genes (CYP3A4 , CYP2C9 , and CYP2C19) genes (Fig. 4C). The expression levels of these genes might have high variability depending on HO differentiation efficiency. Therefore, these are essential marker genes that must be analyzed when checking for the differentiation status of the HO. In particular, compared to HEOs, HOs should require at least a 10,000-fold increase in ALB and a 1000-fold increase in CYP3A4. HOs can be used for toxicity assessment only if the morphological features of good condition, flow cytometric analysis of ALB, and gene expression results are all satisfied. In a previous study (Kim et al., 2022), we demonstrated that good HOs have the enhanced expression and activities of CYP450s (CYP1A2, CYP2C9, CYP2C19, CYP2D6, and CYP3A4), the functionality of apical drug transporters (MRP2, BSEP, and MDR1), and recapitulating liver metabolism and hepatotoxicity of clinical drugs. Even after HO differentiation, the activities of CYP1A2, CYP2C9, and CYP2D6 were maintained for a week, and CYP3A4 and CYP2C19 were confirmed to be maintained for 3 and 4 days, respectively.
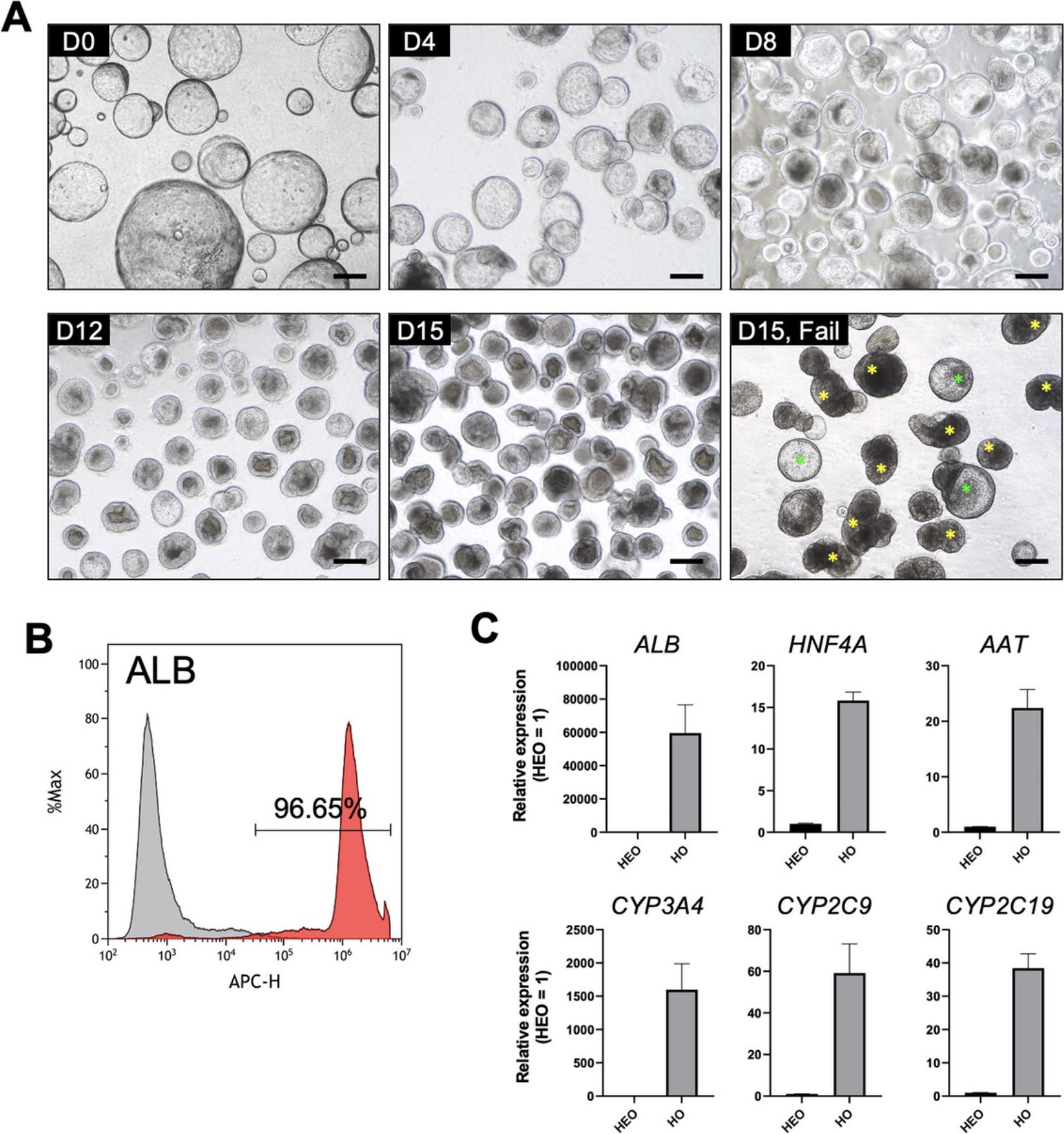
By following Basic Protocol 4, investigators can measure cytotoxic sensitivity to hepatotoxic drugs using HOs. For example, HOs were treated with seven drugs clinically known to cause DILI at a concentration of 7–9 points based on the Cmax concentration, and organoid viability was measured through ATP content. Drug sensitivity for cytotoxicity was also plotted as a dose-response curve (Fig. 5). Compared with the drug responsiveness of PHH and HepG2 (Kim et al., 2022), HOs prepared using this protocol showed similar or higher toxic sensitivity than PHHs for most drugs (Fig. 5).
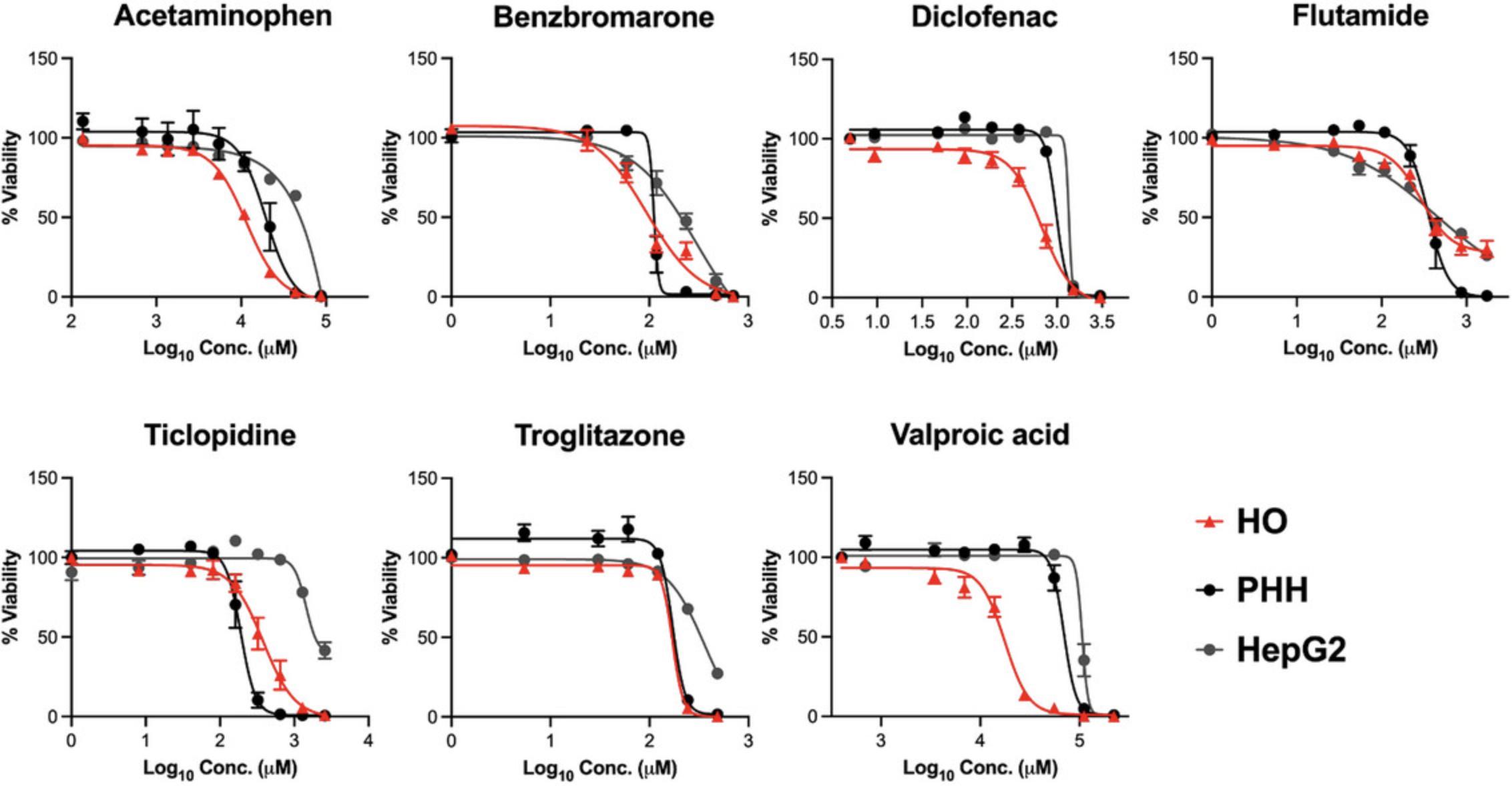
Time Considerations
It takes approximately 24 days to generate HEOs from hPSCs (Basic Protocol 1). After HEO generation, organoids can be passaged multiple times (up to 50 passages) every 6 to 7 days (Basic Protocol 2). For the HO differentiation from HEOs (Basic Protocol 3), it takes about 6 days to expand HEOs and 15 days to complete the HO maturation. For drug testing using HOs (Basic Protocol 4), it takes about 1 to 2 hr to prepare HOs and drug dilutions, 24 hr to expose the hepatotoxic drugs, and 1 hr to measure the organoid viability.
Acknowledgments
This research was supported by the Korea Institute of Toxicology (1711159818) and the Technology Innovation Program funded by the Ministry of Trade, Industry & Energy (20009350).
Author Contributions
Hyemin Kim : Conceptualization; investigation; writing—original draft; writing—review and editing. Sang Kyum Kim : Conceptualization; data curation; investigation; methodology. Michael Oelgeschläger : Writing—review and editing. Han-Jin Park : Conceptualization; funding acquisition; project administration; supervision; writing—review and editing.
Conflict of Interest
The authors declare no conflicts of interest.
Open Research
Data Availability Statement
Data sharing is not applicable to this article as no datasets were generated or analyzed during the current study.
Literature Cited
- Akbari, S., Sevinc, G. G., Ersoy, N., Basak, O., Kaplan, K., Sevinc, K., Ozel, E., Sengun, B., Enustun, E., Ozcimen, B., Bagriyanik, A., Arslan, N., Onder, T. T., & Erdal, E. (2019). Robust, long-term culture of endoderm-derived hepatic organoids for disease modeling. Stem Cell Reports , 13(4), 627–641. https://doi.org/10.1016/j.stemcr.2019.08.007
- Baxter, M., Withey, S., Harrison, S., Segeritz, C. P., Zhang, F., Atkinson-Dell, R., Rowe, C., Gerrard, D. T., Sison-Young, R., Jenkins, R., Henry, J., Berry, A. A., Mohamet, L., Best, M., Fenwick, S. W., Malik, H., Kitteringham, N. R., Goldring, C. E., Piper Hanley, K., … Hanley, N. A. (2015). Phenotypic and functional analyses show stem cell-derived hepatocyte-like cells better mimic fetal rather than adult hepatocytes. Journal of Hepatology , 62(3), 581–589. https://doi.org/10.1016/j.jhep.2014.10.016
- Castell, J. V., Jover, R., Martinez-Jimenez, C. P., & Gomez-Lechon, M. J. (2006). Hepatocyte cell lines: Their use, scope and limitations in drug metabolism studies. Expert Opinion on Drug Metabolism & Toxicology, 2(2), 183–212. https://doi.org/10.1517/17425255.2.2.183
- Cox, C. R., Lynch, S., Goldring, C., & Sharma, P. (2020). Current perspective: 3D spheroid models utilizing human-based cells for investigating metabolism-dependent drug-induced liver injury. Frontiers in Medical Technology , 2, 611913. https://doi.org/10.3389/fmedt.2020.611913
- Gaskell, H., Sharma, P., Colley, H. E., Murdoch, C., Williams, D. P., & Webb, S. D. (2016). Characterization of a functional C3A liver spheroid model. Toxicology Research , 5(4), 1053–1065. https://doi.org/10.1039/c6tx00101g
- Guengerich, F. P. (2006). Cytochrome P450s and other enzymes in drug metabolism and toxicity. The AAPS Journal , 8(1), E101–111. https://doi.org/10.1208/aapsj080112
- Hu, H., Gehart, H., Artegiani, B., C, L. O.-I., Dekkers, F., Basak, O., van Es, J., Lopes, C. d. S., S, M., Begthel, H., Korving, J., van den Born, M., Zou, C., Quirk, C., Chiriboga, L., Rice, C. M., Ma, S., Rios, A., Peters, P. J., … Clevers, H. (2018). Long-term expansion of functional mouse and human hepatocytes as 3D organoids. Cell , 175(6), 1591–1606. e1519. https://doi.org/10.1016/j.cell.2018.11.013
- Huch, M., Gehart, H., van Boxtel, R., Hamer, K., Blokzijl, F., Verstegen, M. M., Ellis, E., van Wenum, M., Fuchs, S. A., de Ligt, J., van de Wetering, M., Sasaki, N., Boers, S. J., Kemperman, H., de Jonge, J., Ijzermans, J. N., Nieuwenhuis, E. E., Hoekstra, R., Strom, S., … Clevers, H. (2015). Long-term culture of genome-stable bipotent stem cells from adult human liver. Cell , 160(1-2), 299–312. https://doi.org/10.1016/j.cell.2014.11.050
- Iorga, A., & Dara, L. (2019). Cell death in drug-induced liver injury. Advances in Pharmacology , 85, 31–74. https://doi.org/10.1016/bs.apha.2019.01.006
- Kim, H., Im, I., Jeon, J. S., Kang, E. H., Lee, H. A., Jo, S., Kim, J. W., Woo, D. H., Choi, Y. J., Kim, H. J., Han, J. S., Lee, B. S., Kim, J. H., Kim, S. K., & Park, H. J. (2022). Development of human pluripotent stem cell-derived hepatic organoids as an alternative model for drug safety assessment. Biomaterials , 286, 121575. https://doi.org/10.1016/j.biomaterials.2022.121575
- Larrey, D., & Pageaux, G. P. (2005). Drug-induced acute liver failure. European Journal of Gastroenterology & Hepatology, 17(2), 141–143. https://doi.org/10.1097/00042737-200502000-00002
- LeCluyse, E. L. (2001). Human hepatocyte culture systems for the in vitro evaluation of cytochrome P450 expression and regulation. European Journal of Pharmaceutical Sciences , 13(4), 343–368. https://doi.org/10.1016/s0928-0987(01)00135-x
- Lee, G., Kim, H., Park, J. Y., Kim, G., Han, J., Chung, S., Yang, J. H., Jeon, J. S., Woo, D. H., Han, C., Kim, S. K., Park, H. J., & Kim, J. H. (2021). Generation of uniform liver spheroids from human pluripotent stem cells for imaging-based drug toxicity analysis. Biomaterials , 269, 120529. https://doi.org/10.1016/j.biomaterials.2020.120529
- Mariotti, V., Strazzabosco, M., Fabris, L., & Calvisi, D. F. (2018). Animal models of biliary injury and altered bile acid metabolism. Biochimica et Biophysica Acta: Molecular Basis of Disease , 1864(4 Pt B), 1254–1261. https://doi.org/10.1016/j.bbadis.2017.06.027
- Marsee, A., Roos, F. J. M., Verstegen, M. M. A., Consortium, H. P. B. O., Gehart, H., de Koning, E., Lemaigre, F., Forbes, S. J., Peng, W. C., Huch, M., Takebe, T., Vallier, L., Clevers, H., van der Laan, L. J. W., & Spee, B. (2021). Building consensus on definition and nomenclature of hepatic, pancreatic, and biliary organoids. Cell Stem Cell , 28(5), 816–832. https://doi.org/10.1016/j.stem.2021.04.005
- Mun, S. J., Ryu, J. S., Lee, M. O., Son, Y. S., Oh, S. J., Cho, H. S., Son, M. Y., Kim, D. S., Kim, S. J., Yoo, H. J., Lee, H. J., Kim, J., Jung, C. R., Chung, K. S., & Son, M. J. (2019). Generation of expandable human pluripotent stem cell-derived hepatocyte-like liver organoids. Journal of Hepatology , 71(5), 970–985. https://doi.org/10.1016/j.jhep.2019.06.030
- Natale, A., Vanmol, K., Arslan, A., van Vlierberghe, S., Dubruel, P., van Erps, J., Thienpont, H., Buzgo, M., Boeckmans, J., de Kock, J., Vanhaecke, T., Rogiers, V., & Rodrigues, R. M. (2019). Technological advancements for the development of stem cell-based models for hepatotoxicity testing. Archives of Toxicology , 93(7), 1789–1805. https://doi.org/10.1007/s00204-019-02465-y
- Park, H. J., Choi, Y. J., Kim, J. W., Chun, H. S., Im, I., Yoon, S., Han, Y. M., Song, C. W., & Kim, H. (2015). Differences in the epigenetic regulation of cytochrome P450 genes between human embryonic stem cell-derived hepatocytes and primary hepatocytes. PLoS One , 10(7), e0132992. https://doi.org/10.1371/journal.pone.0132992
- Prior, N., Inacio, P., & Huch, M. (2019). Liver organoids: From basic research to therapeutic applications. Gut , 68(12), 2228–2237. https://doi.org/10.1136/gutjnl-2019-319256
- Rajarajan, K., Engels, M. C., & Wu, S. M. (2012). Reprogramming of mouse, rat, pig, and human fibroblasts into iPS cells. Current Protocols in Molecular Biology , Chapter 23 , Unit-23.15. https://doi.org/10.1002/0471142727.mb2315s97
- Ramaiahgari, S. C., Waidyanatha, S., Dixon, D., DeVito, M. J., Paules, R. S., & Ferguson, S. S. (2017). Three-dimensional (3D) HepaRG spheroid model with physiologically relevant xenobiotic metabolism competence and hepatocyte functionality for liver toxicity screening. Toxicological Sciences , 160(1), 189–190. https://doi.org/10.1093/toxsci/kfx194
- Sivaraman, A., Leach, J. K., Townsend, S., Iida, T., Hogan, B. J., Stolz, D. B., Fry, R., Samson, L. D., Tannenbaum, S. R., & Griffith, L. G. (2005). A microscale in vitro physiological model of the liver: Predictive screens for drug metabolism and enzyme induction. Current Drug Metabolism , 6(6), 569–591. https://doi.org/10.2174/138920005774832632
- Tanaka, M., Itoh, T., Tanimizu, N., & Miyajima, A. (2011). Liver stem/progenitor cells: Their characteristics and regulatory mechanisms. Journal of Biochemistry , 149(3), 231–239. https://doi.org/10.1093/jb/mvr001
- Thomson, J. A., Itskovitz-Eldor, J., Shapiro, S. S., Waknitz, M. A., Swiergiel, J. J., Marshall, V. S., & Jones, J. M. (1998). Embryonic stem cell lines derived from human blastocysts. Science , 282(5391), 1145–1147. https://doi.org/10.1126/science.282.5391.1145
- van Norman, G. A. (2019). Limitations of animal studies for predicting toxicity in clinical trials: is it time to rethink our current approach? JACC: Basic to Translational Science , 4(7), 845–854. https://doi.org/10.1016/j.jacbts.2019.10.008
- Walker, P. A., Ryder, S., Lavado, A., Dilworth, C., & Riley, R. J. (2020). The evolution of strategies to minimise the risk of human drug-induced liver injury (DILI) in drug discovery and development. Archives of Toxicology , 94(8), 2559–2585. https://doi.org/10.1007/s00204-020-02763-w
- Wang, S., Wang, X., Tan, Z., Su, Y., Liu, J., Chang, M., Yan, F., Chen, J., Chen, T., Li, C., Hu, J., & Wang, Y. (2019). Human ESC-derived expandable hepatic organoids enable therapeutic liver repopulation and pathophysiological modeling of alcoholic liver injury. Cell Research , 29(12), 1009–1026. https://doi.org/10.1038/s41422-019-0242-8
- Wang, Z., Luo, X., Anene-Nzelu, C., Yu, Y., Hong, X., Singh, N. H., Xia, L., Liu, S., & Yu, H. (2015). HepaRG culture in tethered spheroids as an in vitro three-dimensional model for drug safety screening. Journal of Applied Toxicology , 35(8), 909–917. https://doi.org/10.1002/jat.3090
- Wiley, L. A., Anfinson, K. R., Cranston, C. M., Kaalberg, E. E., Collins, M. M., Mullins, R. F., Stone, E. M., & Tucker, B. A. (2017). Generation of Xeno-free, cGMP-compliant patient-specific iPSCs from skin biopsy. Current Protocols in Stem Cell Biology , 42, 4A.12.1–4A.12.14. https://doi.org/10.1002/cpsc.30
- Yang, S., Ooka, M., Margolis, R. J., & Xia, M. (2023). Liver three-dimensional cellular models for high-throughput chemical testing. Cell Reports Methods , 3(3), 100432. https://doi.org/10.1016/j.crmeth.2023.100432
- Yu, J., Vodyanik, M. A., Smuga-Otto, K., Antosiewicz-Bourget, J., Frane, J. L., Tian, S., Nie, J., Jonsdottir, G. A., Ruotti, V., Stewart, R., Slukvin, I. I., & Thomson, J. A. (2007). Induced pluripotent stem cell lines derived from human somatic cells. Science , 318(5858), 1917–1920. https://doi.org/10.1126/science.1151526
- Zanger, U. M., & Schwab, M. (2013). Cytochrome P450 enzymes in drug metabolism: Regulation of gene expression, enzyme activities, and impact of genetic variation. Pharmacology & Therapeutics, 138(1), 103–141. https://doi.org/10.1016/j.pharmthera.2012.12.007
- Zhou, Y., Shen, J. X., & Lauschke, V. M. (2019). Comprehensive evaluation of organotypic and microphysiological liver models for prediction of drug-induced liver injury. Frontiers in Pharmacology , 10, 1093. https://doi.org/10.3389/fphar.2019.01093