Overview of Adductomics in Toxicology
Oksana Lockridge, Oksana Lockridge
Abstract
Adductomics is epidemiology at the molecular level. Untargeted adductomics compares levels of chemical adducts on albumin, hemoglobin, and DNA between healthy and exposed individuals. The goal is to determine a cause-and-effect relationship between chemical exposure and illness. Chemical exposures are not necessarily due to synthetic chemicals but are often due to oxidation products of naturally occurring lipids, for example, 4-hydroxynonenal and acrolein produced by lipid peroxidation of arachidonic and linoleic acids. The preferred method used in adductomics is ultra-high pressure liquid chromatography coupled to with nanoelectrospray tandem mass spectrometry. The mass of the adduct indicates its structure and identifies the chemical. The advantages of molecular epidemiology include information about the many toxicants to which a person is exposed over a period of weeks or months and the relative exposure levels. The disadvantage is the absence of information about the mechanism of toxicity. Untargeted adductomics examines albumin and hemoglobin adducts, which serve as biomarkers of exposure but do not identify the proteins and genes responsible for the toxicity. Targeted adductomics is used when the origin of the toxicity is known. This can be either an adducted protein, such as the butyrylcholinesterase protein modified by nerve agents, or a toxicant, such as acetaminophen. Untargeted adductomics methods have identified potential protein adduct biomarkers of breast cancer, colorectal cancer, childhood leukemia, and lung cancer. Adductomics is a new research area that offers structural insights into chemical exposures and a platform for the discovery of disease biomarkers. © 2023 The Authors. Current Protocols published by Wiley Periodicals LLC.
INTRODUCTION
The oldest person ever recorded was a 122-year-old woman who had smoked all her life. Is smoking good for a long life? The statistics are clear that smoking does not promote a long life, rather it causes cancer, stroke, heart failure, and emphysema, thereby shortening the lifespan for most people who smoke. How then has this woman managed to live so long? The answer to the riddle of living a very long life is most likely genetic makeup, with minor roles for diet and other factors. This centenarian is likely missing the genes that convert the chemicals in smoke into toxic metabolites. Unlike the 122-year-old smoker, most people have genes encoding enzymes such as Cytochrome P450, which poison smokers by converting harmless chemicals into toxic metabolites. The toxic metabolites change the ability of proteins to fulfill their functions and maintain life. The toxic metabolites also destroy DNA: they break and bind to DNA, thereby introducing mutations that can lead to cancer.
The fact that most smokers live well beyond adulthood means the body has methods to minimize the destructive effects of chemicals. Innate defense systems capture low levels of chemicals and expel the toxins in urine and feces. But chronic exposure to low levels of poisonous chemicals can cause illness. In addition to those in cigarette smoke, harmful chemical exposures include chemicals in the air from vehicle exhaust and industrial pollution, chemicals in cooked food produced during the cooking process, alcohol, and occupational exposure to benzene and tar, to name a few.
Protein alkylation as a mechanism of chemical toxicity was a novel idea when it was first reported. By now it is an accepted mechanism supported by solid evidence. Inhibition of acetylcholinesterase is one example of protein alkylation that leads to toxicity. The military wanted to understand why nerve agents are lethal; humans died within minutes of exposure. Scientists found that nerve agents, such as sarin and soman, make a covalent bond with acetylcholinesterase, a protein critical for nerve impulse transmission (Millard et al., 1999). The covalent bond with acetylcholinesterase paralyzes the breathing muscles, resulting in death. A nerve agent adduct on a single amino acid, serine in the catalytic triad, completely inhibited acetylcholinesterase activity. Without acetylcholinesterase activity, excess levels of the neurotransmitter, acetylcholine, accumulated at the neuromuscular junction, blocking transmission of nerve signals. Chemists went to work to determine how to reactivate acetylcholinesterase activity. Irwin Wilson found that an oxime restored acetylcholinesterase activity by chemically removing the inhibitor from serine at the active site (Wilson & Ginsburg, 1955). Oximes are now a standard antidote used for treatment of poisoning by nerve agents and organophosphorus pesticides (Thiermann & Worek, 2022).
A second example of protein alkylation is caused by an acetaminophen (Tylenol) overdose, which results in a loss of liver function within a few days and is lethal. The first studies of acetaminophen toxicity assumed that the mechanism of toxicity would follow the pattern established for acetylcholinesterase. It was anticipated that modification of a single protein by acetaminophen would explain liver dysfunction. However, major differences between the mechanisms of nerve agent and acetaminophen toxicity were uncovered (McGill & Hinson, 2020). Acetaminophen is not toxic until it is metabolized to a quinone by cytochrome P450 enzymes. In contrast, nerve agents are active poisons immediately upon exposure. This difference explains why acetaminophen overdose is lethal days after ingestion, but nerve agents are lethal within minutes. The assumption that acetaminophen toxicity could be explained by modification of a single protein was ruled out when it was found that many proteins are irreversibly alkylated by the acetaminophen quinone. A common feature of the acetaminophen-modified proteins was the identity of cysteine as the modified amino acid. The covalent bond was found between the sulfhydryl group of cysteine and the acetaminophen quinone. Prescription level doses of Tylenol are not toxic because low doses of quinones are scavenged by glutathione inside the cell; however, high doses deplete glutathione, allowing the excess acetaminophen quinone to covalently bind to proteins. A parallel process explains why low doses of nerve agents are not toxic. Absence of toxicity is explained by the scavenging activity of butyrylcholinesterase in blood. An understanding of the protective role of glutathione from acetaminophen toxicity, and the fact that toxicity was associated with binding to sulfhydryl groups, led to an antidote for Tylenol overdose (N -acetylcysteine). When administered within hours of Tylenol overdose, N -acetylcysteine protects liver function (McGill & Hinson, 2020). Figure 1 summarizes the mechanisms of toxicity for nerve agents and acetaminophen overdose.
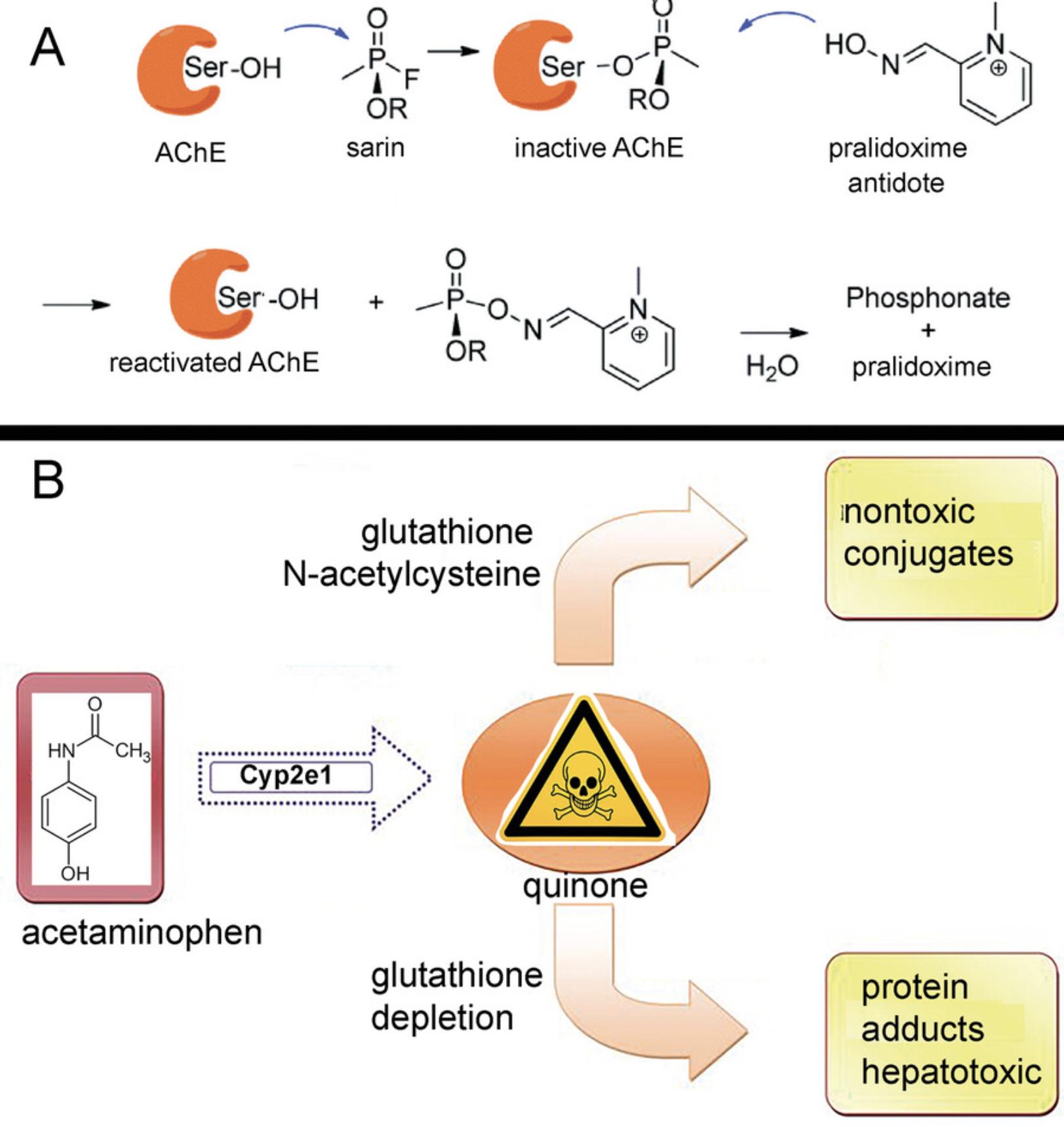
These two examples of protein alkylation show that successful antidotes for specific poisons were developed after the protein adducts had been identified. In the case of acetylcholinesterase, the protein adduct responsible for the toxicity was identified and the adduct could be removed, thereby reversing toxicity. In the case of acetaminophen, the identification of the early steps of protein adduct formation led to a treatment. However, protein adducts themselves are useful only as biomarkers for the diagnosis of exposure. An understanding of the link between protein adducts and organ failure requires years of additional study.
Two centuries before the discovery of DNA, it was known that the high frequency of cancer of the scrotum among chimney sweepers was related to soot exposure. After DNA was shown to be the inherited informational macromolecule, researchers studying mechanisms of chemical carcinogenesis discovered that many known chemical carcinogens are biotransformed to metabolites that covalently bind to DNA (Poirier, 2016). Figure 2 shows the activation of benz(a)pyrene, the carcinogen in soot, into 7β,8α-diol-9α,10α-epoxy-7,8,9,10-tetrahydro-benz(a)pyrene, which then makes a covalent bond with purines in DNA at the exocyclic amino group.
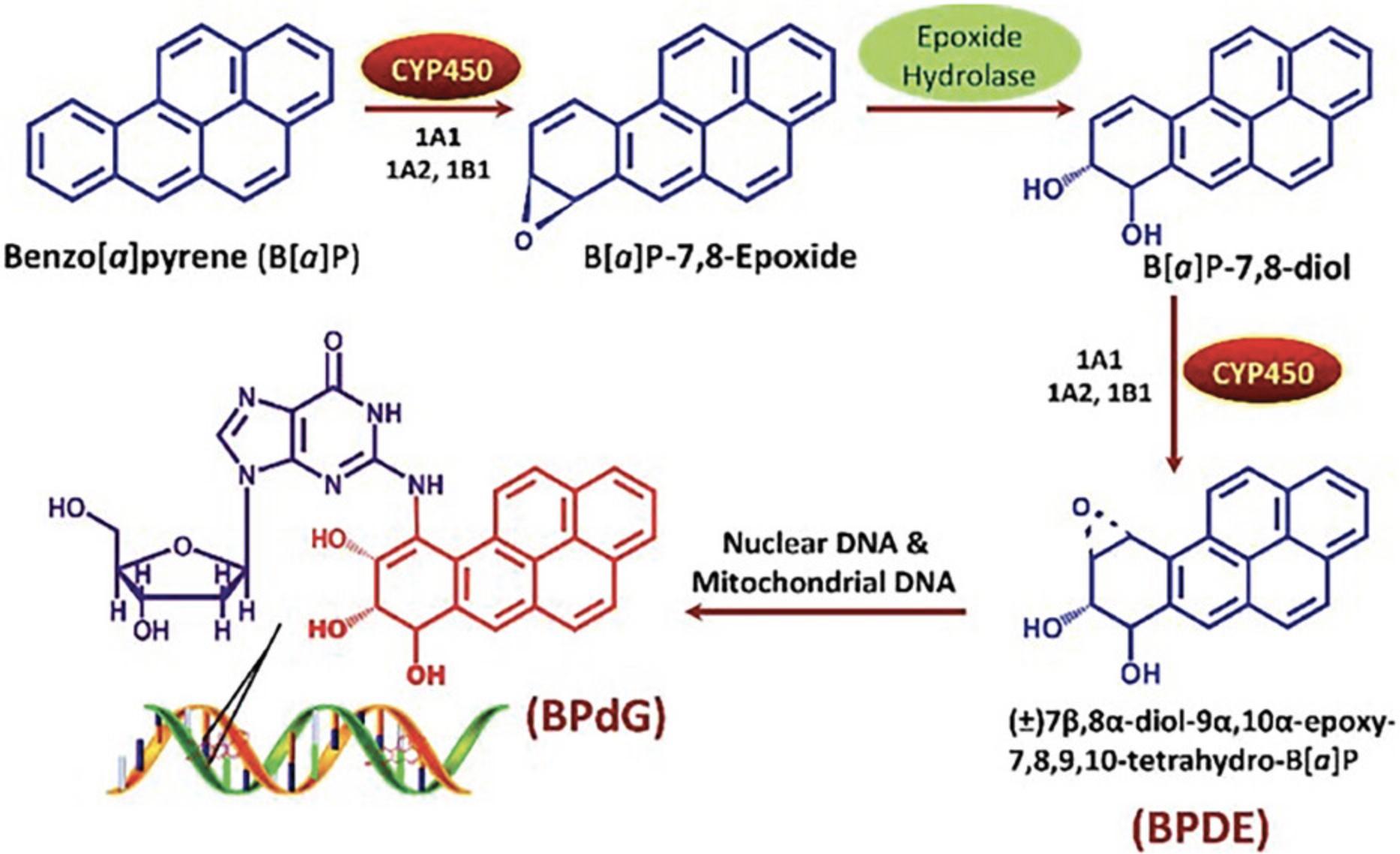
By binding to DNA, chemical carcinogens induce the incorporation of an incorrect base during DNA replication. If the mutation occurs in oncogenes, tumor suppressor genes, and DNA repair genes, the mutation becomes a cancer risk. However, cancer risk is low unless a second strong cancer risk factor is present. For example, chemical exposure to aflatoxin B1 combined with an infection (hepatitis B) increases the risk of liver cancer 60-fold (Poirier, 2016). Chronic exposure to the toxicant and a latent period on the order of years is necessary for sufficient driver mutations to accumulate and tumors to appear (Poirier, 2016).
The search for evidence of chemical exposures is predicated on the hypothesis that some diseases are the consequence of chemical exposure. Lung cancer (tobacco) and cirrhosis (alcohol) are firmly established as diseases triggered by chronic, low level, chemical exposures in combination with a person's genetic makeup, lifestyle, and immune status. It is possible, but not yet clear, that some cases of neurodegenerative and auto-immune diseases may be related to chemical exposure. The disease-inducing chemicals are not exclusively synthetic chemicals but include endogenous chemicals such as those produced by lipid peroxidation during oxidative stress. Researchers hypothesize that knowing the identity and quantity of the chemicals to which a person is exposed will yield biomarkers of disease and provide the missing link between diseases and their environmental causes. This hypothesis led to the field of adductomics.
Adductomics is Epidemiology at the Molecular Level
Untargeted adductomics refers to a search for unknown chemical modifications in specific model proteins. Albumin and hemoglobin are the most popular protein targets of untargeted adductomics because of their high concentrations and long lifetime in human blood (Carlsson, Rappaport, & Tornqvist, 2019). In addition, as blood-proteins, they are readily accessible via minimally invasive procedures. The adducts on albumin and hemoglobin provide a historical record of the chemicals to which a person is exposed over the 20-day lifetime of albumin and the 120-day lifetime of hemoglobin. In 2011, the Rappaport laboratory in Berkeley, California, developed an untargeted strategy for adducts on human albumin (Li et al., 2011) and used untargeted adductomics to compare adducts in pooled plasma obtained from 158 healthy smokers or nonsmokers (Grigoryan et al., 2016). The Tornqvist laboratory in Stockholm, Sweden compared hemoglobin adducts in smokers versus nonsmokers (Carlsson, von Stedingk, Nilsson, & Tornqvist, 2014). Both laboratories agreed that smokers have higher levels of acrylonitrile and ethylene oxide adducts. Tobacco smoke is a known source of acrylonitrile. Both laboratories found dozens of unidentified adducts whose masses do not correspond to known electrophiles. This is consistent with the general observation that humans have a continuous background exposure to electrophiles, both from endogenous and exogenous sources (Carlsson et al., 2019).
Targeted Adductomics
Targeted adductomics refers to a search for covalent adducts formed upon exposure to a specific chemical agent (Nunes et al., 2019). Samples to be searched in a targeted adductomics method are enriched for specific modified proteins before the enriched sample is analyzed by liquid chromatography tandem mass spectrometry. Several strategies have been used to enrich chemically modified proteins: a) Cellular targets of an alkynyl analog of 4-hydroxy-2-nonenal were tagged with biotin using click chemistry (Yang, Tallman, Porter, & Liebler, 2015), followed by binding to immobilized streptavidin; b) Immobilized antibodies specific for diethyl phosphate adducts on tyrosine (Onder et al., 2021) were used to immunopurify proteins modified by chlorpyrifos oxon; c) Histones modified by a carcinogenic furan metabolite were purified by acid precipitation (Nunes et al., 2019); and d) DNA samples were digested to nucleosides. Collision induced dissociation in the mass spectrometer consistently cleaves nucleosides at the glycosidic bond between the 2′-deoxyribose and the nucleobase, regardless of whether the search is for multiple DNA adducts or a specific DNA adduct (Guo & Turesky, 2019). The neutral loss of deoxyribose is a common screening strategy for nucleobases.
Target Tissue Versus Surrogate Tissue
In experimental animal and cell models, one can measure adducts in the target issue (Geib, Moghaddam, Supinski, Golizeh, & Sleno, 2021), but this is not possible in living humans. Autopsy tissues from a fatal poisoning with the nerve agent sarin were studied for sarin adducts on proteins in the bronchus, blood (albumin and butyrylcholinesterase), lung, liver, and kidney of a female victim of chemical warfare in Syria (John et al., 2018). The finding of protein adducts and the detection of sarin hydrolysis products in all tissues including brain, muscle, hair, skin, and heart, provided unambiguous evidence for systemic intoxication and the use of the nerve agent in the conflict (John et al., 2018).
This study of human autopsy tissues supports the conclusion that protein adducts in blood are representative of protein adducts in target tissue for the specific example of the nerve agent sarin. What about other compounds? Aflatoxin in contaminated corn, peanuts, and other foods induces hepatocellular carcinoma. The liver is the primary target organ of aflatoxin adverse effects due to liver proteins CYP1A2 and CYP3A4, which metabolize aflatoxin to aflatoxin-8,9-epoxide (Kensler, Roebuck, Wogan, & Groopman, 2011). The epoxide produces a mutagenic DNA adduct and a long-lived lysine adduct on albumin in blood. Aflatoxin-mercapturic acid detoxification products that are excreted in urine, and aflatoxin-albumin adducts in blood, serve as biomarkers of biologically effective aflatoxin dose (Kensler et al., 2011). The study of acrylamide exposure in factory workers involved in synthesizing acrylamide (Bergmark, Calleman, He, & Costa, 1993; Costa, 2017) provides another example of adducts on the surrogate tissue hemoglobin, which represents adducts on target tissues. Exposure to acrylamide monomers is characterized by peripheral neuropathy including ataxia, skeletal muscle weakness, cognitive impairment, and numbness of the extremities (LoPachin & Gavin, 2012). The most toxicologically relevant targets of acrylamide are the nerve terminal proteins that turnover slowly and are involved in neurotransmitter release, storage, and re-uptake (LoPachin & Gavin, 2012). The peripheral neuropathy in factory workers is accompanied by acrylamide adducts on hemoglobin (Bergmark et al., 1993).
DNA adducts are surrogates only in the sense that the adducted gene is unidentified. The toxic chemical and the modified nucleotides are known. The adduct level is known and correlated with the risk of cancer. For example, depurinating estrogen-DNA adducts are initiators of cancers of the breast, thyroid, ovary, and prostate (Cavalieri & Rogan, 2016).
ADDUCTOMICS METHODS
Ultra-high performance liquid chromatography coupled with high-resolution mass spectrometry is the method of choice both for protein and DNA adductomics (Carlsson et al., 2019; Park et al., 2022). The advantages of liquid chromatography–tandem mass spectrometry over older methods are smaller sample size, higher sensitivity, greater accuracy, and simpler sample preparation procedures. Protein adducts are studied after the protein has been hydrolyzed to peptides. The peptide fragmentation pattern identifies the location and mass of the adduct in a peptide. The peptide sequence identifies the modified protein. DNA adducts are studied after DNA has been digested to individual nucleobases (Park et al., 2022). This strategy identifies the modified nucleobase and the covalently bound chemical but does not identify the modified gene. Studies of chemical carcinogens assume the modified nucleobases are located on a gene frequently mutated in human cancers, such as p53. This assumption was validated by showing that the acrolein-DNA binding pattern is similar to the p53 mutational pattern in human lung cancer (Feng, Hu, Hu, & Tang, 2006). Table 1 summarizes the methods for identifying protein and DNA adducts.
Method | Approach | Reference | Advantages | Disadvantage |
---|---|---|---|---|
32P-postlabeling | DNA hydrolysis, 32P-labeling, and chromatography | (Shibutani, Kim, & Suzuki, 2006) | Measures adduct levels in 1-10 µg DNA; detects 1 adduct in 1010 nt | Adduct identity is unknown; radioactive 32P ATP |
ELISA | Antiserum against DNA or protein adducts | (Dyba et al., 2018; McGill & Hinson, 2020) | Protein adducts in serum; DNA adducts in nucleus | Requires an antibody to each chemical |
Western blot | Antibody detection of protein adducts separated on polyacrylamide gel | (Onder et al., 2021) | High sensitivity; molecular weight of protein adducts | Antibody detects one specific chemical |
Gas chromatography–mass spectrometry | Detach adduct from proteins and derivatize to make the adduct volatile | (John et al., 2018) | High sensitivity; mass identifies the chemical | Derivatization adds a step |
Liquid chromatography–tandem mass spectrometry | Separate peptides and nucleosides by chromatography | (Carlsson et al., 2019; Cavalieri & Rogan, 2016; Park et al., 2022) | Masses of parent and fragment ions identify the adduct | Requires highly skilled scientists |
Older methods for the identification of protein adducts involved detaching the adduct from the proteins followed by liquid chromatography–mass spectrometry or gas chromatography–mass spectrometry with or without derivatization. Older methods for the identification of DNA adducts involved 32P-postlabeling, or high-pressure liquid chromatography with electrochemical or fluorescence detection.
Metabolic Activation
Many chemicals are unreactive with proteins and DNA until they are modified by Cytochrome P450 enzymes into alkynes, aldehydes, quinones, or epoxides. Cytochrome P450 enzymes are located within cells. Activated metabolites produced by Cytochrome P450 enzymes are short-lived. Albumin and DNA, which are located in the intracellular space and the nucleus, respectively, can capture short-lived metabolites, whereas hemoglobin inside red blood cell has limited access. The consequence of this accessibility difference is reflected in adducts of benzene. Cytochrome P450 enzymes convert benzene to the carcinogenic metabolites benzene diol epoxide and benzoquinone. Adducts from these compounds on albumin have been identified by mass spectrometry methods (Smith et al., 2021), but hemoglobin adducts have not. Explanations for the absence of these adducts on hemoglobin include the high concentration of glutathione inside the red blood cell that scavenge incoming electrophiles, and the presence of the red blood cell membrane that blocks electrophile entry (Carlsson et al., 2019).
Patients on certain oral medications, including midazolam, are advised not to drink grapefruit juice for 24 hours before swallowing a pill (Bailey, Malcolm, Arnold, & Spence, 1998). The reason for avoiding grapefruit juice is that components in grapefruit juice inhibit the activity of CYP3A4, a Cytochrome P450 enzyme. In the absence of grapefruit juice, CYP3A4 in the small intestine and colon inactivates midazolam, allowing only 15% of an ingested dose to pass into the circulation. However, in the presence of grapefruit juice, up to 30% of an ingested dose passes into the circulation. The grapefruit juice-induced overdose can cause adverse reactions to the medicine. Similarly, cyclosporin is an oral immunosuppressant for transplant patients. Plasma cyclosporin concentrations must be maintained within a narrow range to achieve adequate immunosuppression without nephrotoxicity (Bailey et al., 1998). Cyclosporin taken with grapefruit juice increases the plasma concentration by 160% compared to when taken with water. The activity of more than 85 drugs is affected by grapefruit juice (Bailey, Dresser, & Arnold, 2013).
Diseases Associated with Protein and DNA Adducts
Diseases associated with protein and DNA adducts have been known for decades. The idea of adductomics was introduced when liquid chromatography coupled with electrospray ionization mass spectrometry became broadly available to research laboratories. The older methods had already established that cancer was caused by DNA-modifying chemicals in tar and smoke, liver failure was caused by acetaminophen overdose (Tylenol), liver cirrhosis was caused by the metabolic products of alcohol, suffocation was caused by protein adducts with chemical warfare nerve agents, and Jamaican vomiting sickness was caused by a toxin in unripe lychee fruit. These diseases were studied because people had symptoms that could be traced to chemical exposure. Adductomics aims to show a cause-and-effect relationship between chemical exposure and a disease before a person has symptoms. The adductomics methods are exquisitely sensitive. Adducts are detected when only 3 albumin molecules out of 100,000, or 5-25 hemoglobin chains out of 10,000,000 are modified (Carlsson et al., 2019). DNA adduct levels are typically detected at 1 per 106 to 1 per 1011 bases (Peterson et al., 2020). Table 2 lists diseases associated with chemical adducts.
Chemical | Disease | Adduct | Reference |
---|---|---|---|
Benz(a)pyrene in soot | Cancer of the scrotum | DNA adducts | (Poirier, 2016) |
Acetaminophen (Tylenol) | Liver failure | Sulfhydryl groups in proteins | (McGill & Hinson, 2020) |
Alcohol | Cirrhosis | Protein and DNA adducts with acetaldehyde | (Setshedi, Wands, & Monte, 2010) |
Alcohol | Hepatocellular carcinoma | Protein and DNA adducts with acetaldehyde | (Setshedi et al., 2010) |
Nerve agents | Suffocation | Covalent adduct on serine at active site of acetylcholinesterase | (John et al., 2018; Noort, Fidder, van der Riet-van Oeveren, Busker, & van der Schans, 2021) |
Hypoglycin in unripe lychee fruit | Jamaican vomiting sickness | Adduct on flavin in acyl-CoA dehydrogenase | (Ghisla, Melde, Zeller, & Boschert, 1990; Grunes et al., 2012) |
Aflatoxin B1 in fungus- infected peanuts | Hepatocellular carcinoma | DNA adduct N7 of deoxyguanosine | (Lin et al., 2014) |
Aristolochic acid in weed that grows in wheat fields | Kidney cancer | DNA adduct N7 of deoxyguanosine | (Stiborova, Arlt, & Schmeiser, 2016) |
Carcinogens in tobacco | Lung cancer | DNA adducts on dA and dG | (Peterson et al., 2020) |
Estrogen metabolites | Breast cancer | Depurinating adducts on DNA | (Cavalieri & Rogan, 2016) |
Polycyclic aromatic hydrocarbons | Breast, skin, and lung cancer | DNA damage | (Barnes, Zubair, John, Poirier, & Martin, 2018) |
Benzene | Leukemia | Sulfhydryl groups in proteins | (Yeowell-O'Connell et al., 1998) |
Cisplatin chemotherapy | Secondary malignancies | DNA adducts | (Poirier, 2016) |
Urushiol in poison ivy | Contact dermatitis | Protein adduct on cytochrome c1 | (Pacheco et al., 2021) |
Environmental chemicals | Auto-immune disease | Protein adducts | (Vojdani, Kharrazian, & Mukherjee, 2015) |
RESULTS FROM UNTARGETED ADDUCTOMICS STUDIES
The review by Carlsson lists adducts on albumin and hemoglobin found in untargeted adductomics studies (Carlsson et al., 2019). A consistent finding is that healthy control groups have a low background level of adducts on Cys34 of albumin and the N-terminal Val of hemoglobin. With few exceptions, the same adducts are found in controls and exposed individuals. Quantitative differences range from 1.11 to 1.20-fold (Grigoryan et al., 2019). Subjects known to have been exposed to various potentially adduct forming compounds have either higher, lower, or identical adduct levels compared to unexposed controls. Of the 34 adducts in a study comparing smokers to nonsmokers (Grigoryan et al., 2016), the levels of 5 adducts differed between smokers and nonsmokers. Levels of ethylene oxide and acrylonitrile adducts on Cys34 of albumin were higher in smokers. Levels of Cys34 sulfinic acid, and S-Cys adducts were lower in smokers. The levels of 29 adducts were statistically the same in smokers and nonsmokers (Grigoryan et al., 2016).
Exposure to tobacco smoke is a risk factor for lung cancer. The albumin and hemoglobin adductomics studies agree that smokers have higher levels of acrylonitrile and ethylene oxide adducts than nonsmokers. These molecules are derived from tobacco smoke. The albumin studies report lower adduct levels of oxidized Cys34 in smokers and attribute this unexpected finding to smoking-induced hypoxia.
Methanethiol is a biomarker of human enteric bacteria. The finding of an increased level of methanethiol adducts on albumin Cys34 in subjects with colon cancer implicates the gut microbiota as a risk factor for colon cancer (Grigoryan et al., 2019).
Occupational exposure to benzene is a risk factor for leukemia. Subjects exposed to benzene have benzene oxide and benzene diolepoxide adducts on Cys34 of albumin (Grigoryan et al., 2018), suggesting these adducts are biomarkers of leukemia risk.
Of the 77 adducts on Cys34 of albumin, 15 are labeled as unknown because their structures have not been determined. Of the 25 adducts on the N-terminal Valine of hemoglobin, 11 are labeled as unknown (Carlsson et al., 2019). The procedure for identifying unknown adducts includes synthesizing potential adducts with the identical molecular weight as the unknown, followed by verification studies to ensure the candidate has the same retention time on the liquid chromatography system and the same fragmentation spectrum in the mass spectrometer as the unknown (Carlsson & Tornqvist, 2016; Degner et al., 2018).
Untargeted adductomics has not revealed exposure to some common chemicals. The herbicide atrazine is an abundant contaminant in ground water. Tylenol is a popular, non-prescription drug for treatment of headache and pain. Malathion pesticide is used to control mosquitoes, lice, ticks, fleas, and insects that attack fruits, vegetables, and shrubs. The added mass for atrazine adducts is 110 and 179 Da (Chu & Letcher, 2021; Dooley et al., 2006), the added mass for Tylenol (acetaminophen) adducts is 151.1 Da (Damsten et al., 2007), and malathion adds 205 and 177 Da to Cys34 of albumin (Yamagishi, Iwase, & Ogra, 2021). No adduct masses corresponding to adducts from atrazine, acetaminophen, or malathion have been found. A 177 Da adduct on Cys34 of albumin that might reflect exposure to malathion is attributed to CysGly (Carlsson et al., 2019), not to malathion.
How to Apply Adductomics Results to an Understanding of Disease
Adduct levels on albumin, hemoglobin and DNA are very low in healthy people. The presence of a biomarker for a particular disease indicates exposure to a toxin, but not the presence of disease. Detoxification systems rid the body of low levels of toxicants. Only when chemical concentrations exceed the body's innate defenses is there a risk for toxic symptoms. Bruce Ames reported that almost all chemicals are carcinogens at high concentrations, but the same chemicals are not toxic at low concentrations (Ames & Gold, 1997).
The path from adduct detection to understanding the underlying mechanism of toxicity requires years of research using a variety of research methods. After 50 years of study, researchers are still searching for a mechanism to explain how an overdose of acetaminophen (Tylenol) damages the liver. Acetaminophen makes adducts on many proteins, but the relevance of acetaminophen adducts to toxicity has only been partially defined (McGill & Hinson, 2020). Acetaminophen becomes toxic after it is activated by Cytochrome P450 enzymes to a quinone. The quinone is detoxified by reaction with glutathione. When high doses deplete glutathione, the quinone binds to protein sulfhydryl groups. Protein binding and toxicity co-localize in hepatocytes high in Cytochrome 2E1. The events leading to hepatocyte necrosis include oxidative stress and mitochondrial dysfunction (McGill & Hinson, 2020), but details of these processes are still under investigation. Figure 3 summarizes the current view of the mechanism of acetaminophen liver toxicity with mitochondrial dysfunction taking center stage.
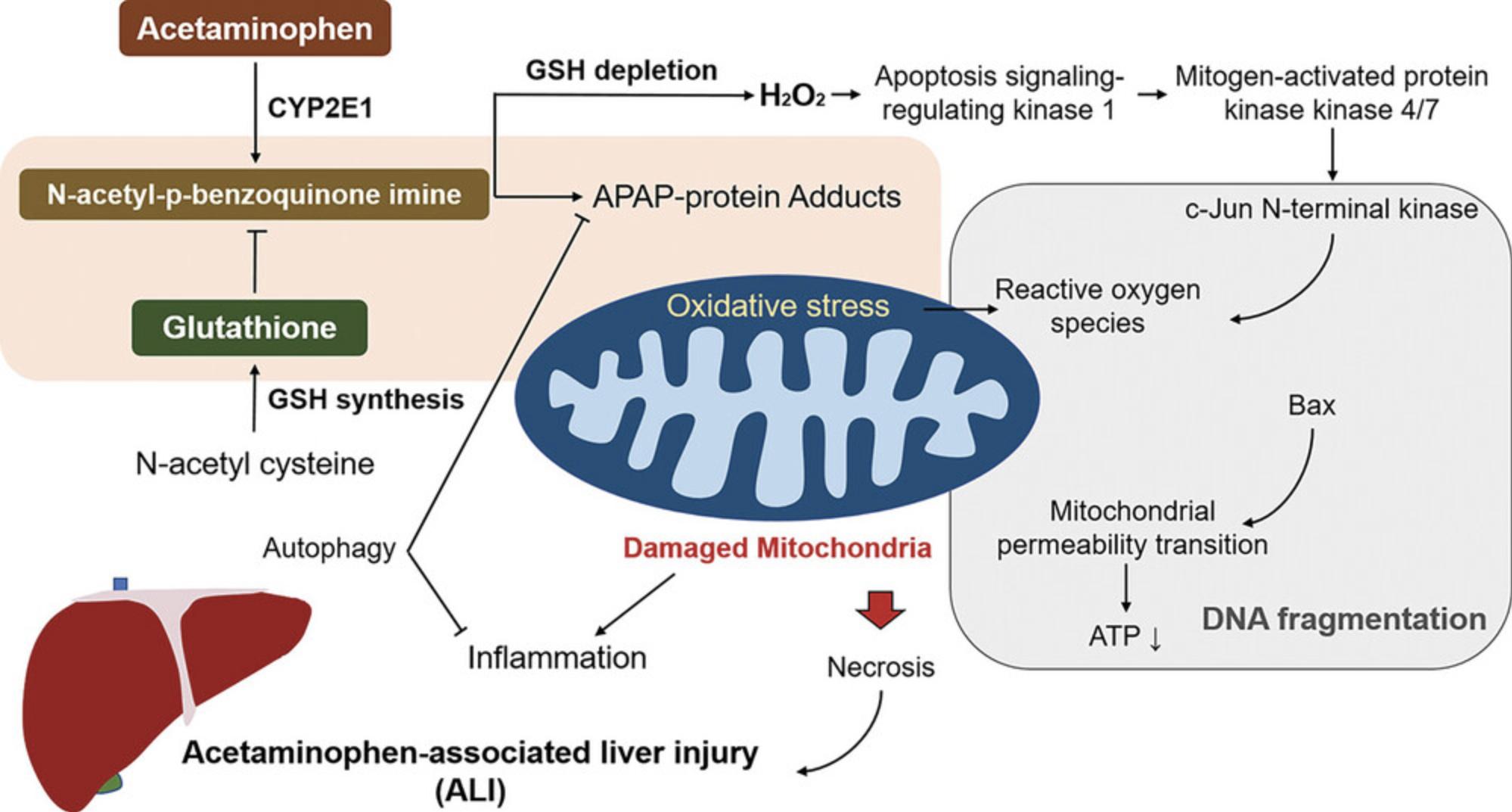
Prediction of Adverse Drug Reactions for New Drug Candidates
Adverse reactions to drugs are often initiated by bioactivation of the drug to an electrophile that modifies cellular proteins. The protein conjugates can disrupt cellular functions and cause an immune response (Stepan et al., 2011). The screening tools for bioactivated metabolites include glutathione trapping and covalent binding assays. The goal is to make a drug that yields minimal or no bioactivated metabolites. The premise in these safety assessments is that a drug candidate that produces no bioactivated metabolites is safe. The principal bioactivation process in safety assessment studies employs liver Cytochrome P450 enzymes. However, as pointed out by Stepan et al. (2011), in vitro assays with Cytochrome P450 enzymes overlook other bioactivation pathways, for example bioactivation to electrophilic acyl-coenzyme A thioester derivatives.
Challenges for Adductomics
To understand organ toxicity, it is important to identify toxicologically relevant protein targets of the bioactivated metabolites. Adductomics can identify many proteins that form covalent links with metabolites, but only a few of these adducts are likely to be critical for toxicity.
An immune response to prescription drugs can cause organ failure. Adductomics methods could be applied to gain information about the nature of the antigenic determinants that trigger an immune response to covalently modified proteins (Stepan et al., 2011)
Where the Field is Going
Targeted covalent inhibitors for various proteins are continually being developed. The initial, reversible association between inhibitor and protein is followed by the formation of a covalent bond between the drug and the protein (Baillie, 2016). New, targeted covalent inhibitors differ from old, reversible inhibitors in three important ways. First, the new, targeted covalent inhibitors are designed to be highly selective for the target protein (Baillie, 2016). High affinity means that low doses are required for efficacy. A low dose reduces interaction with nontargeted proteins. Second, the targeted covalent inhibitors carry a warhead that acts only after the inhibitor is noncovalently bound to its target. Third, metabolic activation is not involved. The warhead in the drug only requires proximity to the nucleophilic target for a reaction to occur.
Osimertinib and carfilzomib are examples of FDA-approved targeted covalent inhibitors (Baillie, 2016). Osimertinib is an oral, epidermal growth factor receptor tyrosine kinase inhibitor indicated for the treatment of metastatic non-small cell lung cancer, where the tumor is positive for the T790M mutation. Carfilzomib is an injectable proteasome inhibitor for the treatment of multiple myeloma.
The pharmaceutical industry hesitated to develop covalent inhibitors because decades of experience warned that unacceptable, serious, adverse effects are associated with drugs that make covalent protein adducts. The standard practice in drug discovery programs is to screen out at an early stage of development those drugs that undergo metabolism to reactive electrophiles that make covalent protein adducts. This standard practice is still in force to exclude the possibility that a targeted covalent inhibitor will be transformed to an electrophile that randomly attacks nonspecific proteins. The eight FDA-approved, targeted, covalent inhibitors have been shown to be specific and cannot be transformed into random electrophiles. Despite the proven effectiveness and generally well tolerated history of these targeted covalent inhibitors, skepticism remains (Baillie, 2021). The concern is the potential of covalent inhibitors to cause immune-mediated adverse reactions triggered by covalent modification of either target or off-target proteins (Baillie, 2021).
Development of covalent drugs for both oncology and non-oncology indications are projected to expand significantly in the coming years. The high-resolution liquid chromatography mass spectrometry methods developed for identification of chemical adducts in adductomics studies are well suited to the study of drug-protein covalent interactions, and for defining the biological consequences, whether beneficial or adverse. (Baillie, 2021)
ACKNOWLEDGMENTS
The helpful editorial comments of Dr. Lucio Costa are gratefully acknowledged. Funded in part by the Fred & Pamela Buffet Cancer Center Support Grant P30CA036727 from the National Institutes of Health.
AUTHOR CONTRIBUTIONS
Oksana Lockridge : Writing original draft
CONFLICT OF INTEREST
The author declares no conflict of interest. The sponsor was not involved in preparation of this review.
Open Research
DATA AVAILABILITY STATEMENT
Data sharing is not applicable to this article as no new data were created or analyzed in this study.
LITERATURE CITED
- Ames, B. N., & Gold, L. S. (1997). The causes and prevention of cancer: Gaining perspective. Environ Health Perspect , 105(Suppl 4), 865–873. doi: 10.1289/ehp.97105s4865
- Bailey, D. G., Dresser, G., & Arnold, J. M. (2013). Grapefruit-medication interactions: Forbidden fruit or avoidable consequences? CMAJ , 185(4), 309–316. doi: 10.1503/cmaj.120951
- Bailey, D. G., Malcolm, J., Arnold, O., & Spence, J. D. (1998). Grapefruit juice-drug interactions. British Journal of Clinical Pharmacology , 46(2), 101–110. doi: 10.1046/j.1365-2125.1998.00764.x
- Baillie, T. A. (2016). Targeted covalent inhibitors for drug design. Angewandte Chemie International Edition England , 55(43), 13408–13421. doi: 10.1002/anie.201601091
- Baillie, T. A. (2021). Approaches to mitigate the risk of serious adverse reactions in covalent drug design. Expert Opinion on Drug Discovery , 16(3), 275–287. doi: 10.1080/17460441.2021.1832079
- Barnes, J. L., Zubair, M., John, K., Poirier, M. C., & Martin, F. L. (2018). Carcinogens and DNA damage. Biochemical Society Transactions , 46(5), 1213–1224. doi: 10.1042/BST20180519
- Bergmark, E., Calleman, C. J., He, F., & Costa, L. G. (1993). Determination of hemoglobin adducts in humans occupationally exposed to acrylamide. Toxicology and Applied Pharmacology , 120(1), 45–54. doi: 10.1006/taap.1993.1085
- Carlsson, H., Rappaport, S. M., & Tornqvist, M. (2019). Protein adductomics: Methodologies for untargeted screening of adducts to serum albumin and hemoglobin in human blood samples. High Throughput , 8(1), 6. doi: 10.3390/ht8010006
- Carlsson, H., & Tornqvist, M. (2016). Strategy for identifying unknown hemoglobin adducts using adductome LC-MS/MS data: Identification of adducts corresponding to acrylic acid, glyoxal, methylglyoxal, and 1-octen-3-one. Food and Chemical Toxicology , 92, 94–103. doi: 10.1016/j.fct.2016.03.028
- Carlsson, H., von Stedingk, H., Nilsson, U., & Tornqvist, M. (2014). LC-MS/MS screening strategy for unknown adducts to N-terminal valine in hemoglobin applied to smokers and nonsmokers. Chemical Research in Toxicology , 27(12), 2062–2070. doi: 10.1021/tx5002749
- Cavalieri, E. L., & Rogan, E. G. (2016). Depurinating estrogen-DNA adducts, generators of cancer initiation: Their minimization leads to cancer prevention. Clinical and Translational Medicine , 5(1), 12. doi: 10.1186/s40169-016-0088-3
- Chang, L., Xu, D., Zhu, J., Ge, G., Kong, X., & Zhou, Y. (2020). Herbal therapy for the treatment of acetaminophen-associated liver injury: Recent advances and future perspectives. Frontiers in Pharmacology , 11, 313. doi: 10.3389/fphar.2020.00313
- Chu, S., & Letcher, R. J. (2021). Identification and characterization of serum albumin covalent adduct formed with atrazine by liquid chromatography mass spectrometry. Journal of Chromatography B: Analytical Technologies in the Biomedical and Life Sciences , 1163, 122503. doi: 10.1016/j.jchromb.2020.122503
- Costa, L. G. (2017). Overview of neurotoxicology. Current Protocols in Toxicology , 74, 11.11.11–11.11.11. doi: 10.1002/cptx.36
- Damsten, M. C., Commandeur, J. N., Fidder, A., Hulst, A. G., Touw, D., Noort, D., & Vermeulen, N. P. (2007). Liquid chromatography/tandem mass spectrometry detection of covalent binding of acetaminophen to human serum albumin. Drug Metabolism & Disposition, 35(8), 1408–1417. doi: 10.1124/dmd.106.014233
- Degner, A., Carlsson, H., Karlsson, I., Eriksson, J., Pujari, S. S., Tretyakova, N. Y., & Tornqvist, M. (2018). Discovery of novel N-(4-Hydroxybenzyl)valine hemoglobin adducts in human blood. Chemical Research in Toxicology , 31(12), 1305–1314. doi: 10.1021/acs.chemrestox.8b00173
- Dooley, G. P., Prenni, J. E., Prentiss, P. L., Cranmer, B. K., Andersen, M. E., & Tessari, J. D. (2006). Identification of a novel hemoglobin adduct in Sprague Dawley rats exposed to atrazine. Chemical Research in Toxicology , 19(5), 692–700. doi: 10.1021/tx060023c
- Dyba, M., da Silva, B., Coia, H., Hou, Y., Noguchi, S., Pan, J., … Chung, F. L. (2018). Monoclonal antibodies for the detection of a specific cyclic DNA adduct derived from omega-6 polyunsaturated fatty acids. Chemical Research in Toxicology , 31(8), 772–783. doi: 10.1021/acs.chemrestox.8b00111
- Feng, Z., Hu, W., Hu, Y., & Tang, M. S. (2006). Acrolein is a major cigarette-related lung cancer agent: Preferential binding at p53 mutational hotspots and inhibition of DNA repair. Proceedings of the National Academy of Sciences USA , 103(42), 15404–15409. doi: 10.1073/pnas.0607031103
- Geib, T., Moghaddam, G., Supinski, A., Golizeh, M., & Sleno, L. (2021). Protein targets of acetaminophen covalent binding in rat and mouse liver studied by LC-MS/MS. Frontiers in Chemistry , 9, 736788. doi: 10.3389/fchem.2021.736788
- Ghisla, S., Melde, K., Zeller, H. D., & Boschert, W. (1990). Mechanisms of enzyme inhibition by hypoglycin, methylenecyclopropylglycine and their metabolites. Progress in Clinical and Biological Research , 321, 185–192. Retrieved from https://www.ncbi.nlm.nih.gov/pubmed/2326291
- Grigoryan, H., Edmands, W., Lu, S. S., Yano, Y., Regazzoni, L., Iavarone, A. T., … Rappaport, S. M. (2016). Adductomics pipeline for untargeted analysis of modifications to Cys34 of human serum albumin. Analytical Chemistry , 88(21), 10504–10512. doi: 10.1021/acs.analchem.6b02553
- Grigoryan, H., Edmands, W. M. B., Lan, Q., Carlsson, H., Vermeulen, R., Zhang, L., … Rappaport, S. M. (2018). Adductomic signatures of benzene exposure provide insights into cancer induction. Carcinogenesis , 39(5), 661–668. doi: 10.1093/carcin/bgy042
- Grigoryan, H., Schiffman, C., Gunter, M. J., Naccarati, A., Polidoro, S., Dagnino, S., … Rappaport, S. M. (2019). Cys34 adductomics links colorectal cancer with the gut microbiota and redox biology. Cancer Research , 79(23), 6024–6031. doi: 10.1158/0008-5472.CAN-19-1529
- Grunes, D. E., Scordi-Bello, I., Suh, M., Florman, S., Yao, J., Fiel, M. I., & Thung, S. N. (2012). Fulminant hepatic failure attributed to ackee fruit ingestion in a patient with sickle cell trait. Case Reports in Transplantation , 2012, 739238. doi: 10.1155/2012/739238
- Guo, J., & Turesky, R. J. (2019). Emerging technologies in mass spectrometry-based DNA adductomics. High Throughput , 8(2), 13. doi: 10.3390/ht8020013
- John, H., van der Schans, M. J., Koller, M., Spruit, H. E. T., Worek, F., Thiermann, H., & Noort, D. (2018). Fatal sarin poisoning in Syria 2013: Forensic verification within an international laboratory network. Forensic Toxicology , 36(1), 61–71. doi: 10.1007/s11419-017-0376-7
- Kensler, T. W., Roebuck, B. D., Wogan, G. N., & Groopman, J. D. (2011). Aflatoxin: A 50-year odyssey of mechanistic and translational toxicology. Toxicological Sciences , 120(Suppl 1), S28–48. doi: 10.1093/toxsci/kfq283
- Li, H., Grigoryan, H., Funk, W. E., Lu, S. S., Rose, S., Williams, E. R., & Rappaport, S. M. (2011). Profiling Cys34 adducts of human serum albumin by fixed-step selected reaction monitoring. Molecular & Cellular Proteomics, 10(3), M110 004606. doi: 10.1074/mcp.M110.004606
- Lin, Y. C., Li, L., Makarova, A. V., Burgers, P. M., Stone, M. P., & Lloyd, R. S. (2014). Error-prone replication bypass of the primary aflatoxin B1 DNA adduct, AFB1-N7-Gua. Journal of Biological Chemistry , 289(26), 18497–18506. doi: 10.1074/jbc.M114.561563
- LoPachin, R. M., & Gavin, T. (2012). Molecular mechanism of acrylamide neurotoxicity: Lessons learned from organic chemistry. Environmental Health Perspectives , 120(12), 1650–1657. doi: 10.1289/ehp.1205432
- McGill, M. R., & Hinson, J. A. (2020). The development and hepatotoxicity of acetaminophen: Reviewing over a century of progress. Drug Metabolism Reviews , 52(4), 472–500. doi: 10.1080/03602532.2020.1832112
- Millard, C. B., Kryger, G., Ordentlich, A., Greenblatt, H. M., Harel, M., Raves, M. L., … Sussman, J. L. (1999). Crystal structures of aged phosphonylated acetylcholinesterase: Nerve agent reaction products at the atomic level. Biochemistry , 38(22), 7032–7039. doi: 10.1021/bi982678l
- Noort, D., Fidder, A., van der Riet-van Oeveren, D., Busker, R., & van der Schans, M. J. (2021). Verification of exposure to novichok nerve agents utilizing a semitargeted human butyrylcholinesterase nonapeptide assay. Chemical Research in Toxicology , 34(8), 1926–1932. doi: 10.1021/acs.chemrestox.1c00198
- Nunes, J., Charneira, C., Morello, J., Rodrigues, J., Pereira, S. A., & Antunes, A. M. M. (2019). Mass spectrometry-based methodologies for targeted and untargeted identification of protein covalent adducts (Adductomics): Current status and challenges. High Throughput , 8(2), 9. doi: 10.3390/ht8020009
- Onder, S., van Grol, M., Fidder, A., Xiao, G., Noort, D., Yerramalla, U., … Lockridge, O. (2021). Rabbit antidiethoxyphosphotyrosine antibody, made by single B cell cloning, detects chlorpyrifos oxon-modified proteins in cultured cells and immunopurifies modified peptides for mass spectrometry. Journal of Proteome Research , 20(10), 4728–4745. doi: 10.1021/acs.jproteome.1c00383
- Pacheco, R., Quezada, S. A., Kalergis, A. M., Becker, M. I., Ferreira, J., & De Ioannes, A. E. (2021). Allergens of the urushiol family promote mitochondrial dysfunction by inhibiting the electron transport at the level of cytochromes B and chemically modify cytochrome c1. Biological Research , 54(1), 35. doi: 10.1186/s40659-021-00357-z
- Park, S. L., Le Marchand, L., Cheng, G., Balbo, S., Chen, M., Carmella, S. G., … Hecht, S. S. (2022). Quantitation of DNA adducts resulting from acrolein exposure and lipid peroxidation in oral cells of cigarette smokers from three Racial/Ethnic groups with differing risks for lung cancer. Chemical Research in Toxicology , 35(10), 1914–1922. doi: 10.1021/acs.chemrestox.2c00171
- Peterson, L. A., Balbo, S., Fujioka, N., Hatsukami, D. K., Hecht, S. S., Murphy, S. E., … Villalta, P. W. (2020). Applying tobacco, environmental, and dietary-related biomarkers to understand cancer etiology and evaluate prevention strategies. Cancer Epidemiology, Biomarkers & Prevention, 29(10), 1904–1919. doi: 10.1158/1055-9965.EPI-19-1356
- Poirier, M. C. (2016). Linking DNA adduct formation and human cancer risk in chemical carcinogenesis. Environmental and Molecular Mutagenesis , 57(7), 499–507. doi: 10.1002/em.22030
- Setshedi, M., Wands, J. R., & Monte, S. M. (2010). Acetaldehyde adducts in alcoholic liver disease. Oxidative Medicine and Cellular Longevity , 3(3), 178–185. doi: 10.4161/oxim.3.3.12288
- Shibutani, S., Kim, S. Y., & Suzuki, N. (2006). 32P-postlabeling DNA damage assays: PAGE, TLC, and HPLC. Methods in Molecular Biology , 314, 307–321. doi: 10.1385/1-59259-973-7:307
- Smith, J. W., O'Meally, R. N., Ng, D. K., Chen, J. G., Kensler, T. W., Cole, R. N., & Groopman, J. D. (2021). Biomonitoring of ambient outdoor air pollutant exposure in humans using targeted serum albumin adductomics. Chemical Research in Toxicology , 34(4), 1183–1196. doi: 10.1021/acs.chemrestox.1c00055
- Stepan, A. F., Walker, D. P., Bauman, J., Price, D. A., Baillie, T. A., Kalgutkar, A. S., & Aleo, M. D. (2011). Structural alert/reactive metabolite concept as applied in medicinal chemistry to mitigate the risk of idiosyncratic drug toxicity: A perspective based on the critical examination of trends in the top 200 drugs marketed in the United States. Chemical Research in Toxicology , 24(9), 1345–1410. doi: 10.1021/tx200168d
- Stiborova, M., Arlt, V. M., & Schmeiser, H. H. (2016). Balkan endemic nephropathy: An update on its aetiology. Archives of Toxicology , 90(11), 2595–2615. doi: 10.1007/s00204-016-1819-3
- Thiermann, H., & Worek, F. (2022). Pro: Oximes should be used routinely in organophosphate poisoning. British Journal of Clinical Pharmacology , 88(12), 5064–5069. doi: 10.1111/bcp.15215
- Vojdani, A., Kharrazian, D., & Mukherjee, P. S. (2015). Elevated levels of antibodies against xenobiotics in a subgroup of healthy subjects. Journal of Applied Toxicology , 35(4), 383–397. doi: 10.1002/jat.3031
- Wilson, I. B., & Ginsburg, B. (1955). A powerful reactivator of alkylphosphate-inhibited acetylcholinesterase. Biochimica et Biophysica Acta , 18(1), 168–170. doi: 10.1016/0006-3002(55)90040-8
- Yamagishi, Y., Iwase, H., & Ogra, Y. (2021). Effects of human serum albumin on post-mortem changes of malathion. Scientific Reports , 11(1), 11573. doi: 10.1038/s41598-021-91145-y
- Yang, J., Tallman, K. A., Porter, N. A., & Liebler, D. C. (2015). Quantitative chemoproteomics for site-specific analysis of protein alkylation by 4-hydroxy-2-nonenal in cells. Analytical Chemistry , 87(5), 2535–2541. doi: 10.1021/ac504685y
- Yeowell-O'Connell, K., Rothman, N., Smith, M. T., Hayes, R. B., Li, G., Waidyanatha, S., … Rappaport, S. M. (1998). Hemoglobin and albumin adducts of benzene oxide among workers exposed to high levels of benzene. Carcinogenesis , 19(9), 1565–1571. doi: 10.1093/carcin/19.9.1565
Citing Literature
Number of times cited according to CrossRef: 1
- Oksana Lockridge, Lawrence M. Schopfer, Review: Organophosphorus toxicants, in addition to inhibiting acetylcholinesterase activity, make covalent adducts on multiple proteins and promote protein crosslinking into high molecular weight aggregates, Chemico-Biological Interactions, 10.1016/j.cbi.2023.110460, 376 , (110460), (2023).