Optimized and Scalable Precoating-Free Reprogramming of Human Peripheral Blood Mononuclear Cells into iPSCs
Elisabetta Fiacco, Elisabetta Fiacco, Sara Landi, Sara Landi, Jacopo Zasso, Jacopo Zasso, Chiara Ambrosini, Chiara Ambrosini, Giovanni Faga, Giovanni Faga
coating
extracellular matrix
induced pluripotent stem cells
laminin-511
reprogramming PBMCs
scaling down
Abstract
Human disease modeling has been profoundly transformed by the introduction of human induced pluripotent stem cells (iPSCs), marking the onset of a new era. This ground-breaking development offers a tailored framework for generating pluripotent cells from any individual, effectively enabling the development of cellular models for the study of human physiology and diseases on an unprecedented scale. Although technologies for iPSCs generation have advanced rapidly over the past two decades, protocols for reprogramming patient-derived somatic cells into stem cells still pose a major challenge for the development of automated pipelines capable of generating iPSCs at scales that are cost-effective, reproducible, and easy to implement. Most methods commonly rely on extracellular matrix protein mixtures or synthetic substrates to promote efficient proliferation of iPSCs. Nonetheless, employing these substances entails a laborious and time-consuming process, as the culture surface requires coating treatments before cell seeding. Here we describe a method for reprogramming blood-derived mononucleated cells that eliminates the need to precoat culture surfaces for the entire experimental flow. This procedure is suitable for fresh or frozen purified peripheral blood mononuclear cells (PBMCs) and allows seeding of reprogrammed cells in a culture medium containing a fragment of laminin-511, regardless of the method of reprogramming employed. Our protocol incorporates a streamlined workflow that optimizes key factors, including cell density, culture medium composition, and iPSC culture propagation techniques. Using a precoating-free approach, we eliminate the time-consuming steps, while our optimized subcloning method improves the scalability of the protocol, making it suitable for large-scale applications. Additionally, the automation-friendly nature of our protocol allows for high-throughput processing, reducing the labor and costs associated with manual handling. © 2024 The Authors. Current Protocols published by Wiley Periodicals LLC.
Basic Protocol 1 : Miniaturized and time efficient precoating-free reprogramming of fresh or frozen PBMCs
Alternate Protocol : Erythroid progenitor cells (EPCs) enrichment and reprogramming into iPSCs using Sendai viral vectors
Basic Protocol 2 : Picking and precoating-free optimized expansion of iPSC clones
INTRODUCTION
The advent of technology for generating human induced pluripotent stem cells (iPSCs) has radically revolutionized the approach to disease modeling, accelerating the development of cellular models for studying previously inaccessible tissues and their underlying molecular mechanisms. Protocols for the generation of pluripotent stem cells have seen significant technological advancement since their first introduction. Although the efficiency is currently poor, both chemical and transgenic reprogramming approaches enable the production of high-quality iPSCs within a timeframe of 2 to 4 months (Schlaeger et al., 2015). Following the expression of a cocktail of reprogramming factors (Takahashi et al., 2007), the subsequent isolation of iPSC colonies (colony picking) and the ensuing process of clone expansion and characterization are highly time-consuming and labor-intensive stages.
In human pluripotent stem cell (hPSC) passaging techniques, the culture surfaces are typically coated with recombinant human extracellular matrix (ECM) proteins like vitronectin or laminins, or complex matrices, such as Matrigel. However, the requirement for coating the culture surface in either case presents an additional obstacle to the scalable processing of hPSCs, necessitating meticulous planning. The incorporation of iMatrix-511, a synthetic fragment derived from laminin-511, in culture systems without the need for precoating represents a significant advancement in the progress of fully automated methods for stem cell propagation (Miyazaki et al., 2017). However, at present, this technique is exclusively applicable to the cultivation of established iPSC lines.
Here, we describe a protocol for reprogramming peripheral blood mononuclear cells (PBMCs) using Sendai viral (SEV) vectors that eliminates the need for precoated culture vessels from the first step immediately following transduction. The protocol involves the preparation of SEV-transduced PBMCs, followed by their seeding using iMatrix-511 diluted in culture medium (Basic Protocol 1). This method can be applied starting from frozen PBMCs or after enrichment of erythroid precursors cells (EPCs) (Alternate Protocol). After the emergence, successfully reprogrammed colonies are cherry picked and expanded for subsequent characterization and cryopreservation (Basic Protocol 2).
STRATEGIC PLANNING
Make sure that the laboratory is fully accredited and well-equipped, and that the staff members are adequately trained and qualified to carry out these procedures. To perform the techniques outlined in this article, you can acquire PBMCs from commercial sources (see Materials in Basic Protocol 1). If laboratory staff are unfamiliar with the described protocol, we strongly recommend reprogramming only one sample at a time. Before performing the protocol, please refer to the CytoTune-iPS 2.0 kit manual.
The viability of cryopreserved PBMCs is a crucial determinant for the success of the reprogramming protocol. When using PBMCs obtained from commercial sources or freshly purified from blood samples, it is common to observe the generation of high-quality iPSC colonies. In such instances, Basic Protocol 1 can be confidently employed for pluripotent stem cell production.
To address the problem of low viability in PBMCs (either cryopreserved or freshly purified) and avoid potential reprogramming failures, a set of cell subpopulations, such as CD34+ hematopoietic stem and progenitor cells, as well as EPCs, can be expanded from the PBMCs preparation, representing an attractive mean for reprogramming (Mack et al., 2011; Skorik et al., 2020). However, due to their scarcity in whole blood, these cells must be expanded in vitro to obtain the amount required for reprogramming. The protocol for the expansion and reprogramming of EPCs is described in Alternate Protocol.
NOTE : All the reagents used in these protocols, excluding iMatrix-511, were equilibrated to room temperature prior to use. iMatrix-511 must be kept on ice during the execution of the protocol.
NOTE : Appropriate informed consent is necessary for obtaining and use of human study material.
Basic Protocol 1: MINIATURIZED AND TIME EFFICIENT PRECOATING-FREE REPROGRAMMING OF FRESH OR FROZEN PBMCs
Here we describe a feeder-free and precoating free protocol to reprogram fresh or frozen PBMCs using CytoTune-iPS 2.0 Sendai reprogramming kit. During this phase, PBMCs are prepared in a complete PBMC medium from day −3 to day 0 before they undergo transduction with the three SEV-based reprogramming viral vectors (Fig. 1). After transduction, cells are plated at a clonal seeding density in StemPro-34 SFM, a serum-free medium specially designed to nurture the growth of human hematopoietic cells. The manufacturer's protocol recommends using wells precoated with vitronectin or rh-laminin-521 for this step. However, the detailed method below bypasses the need for precoating plastic surfaces by utilizing a culture medium supplemented with iMatrix-511.This approach enhances the efficiency and convenience of cell seeding post-transduction.
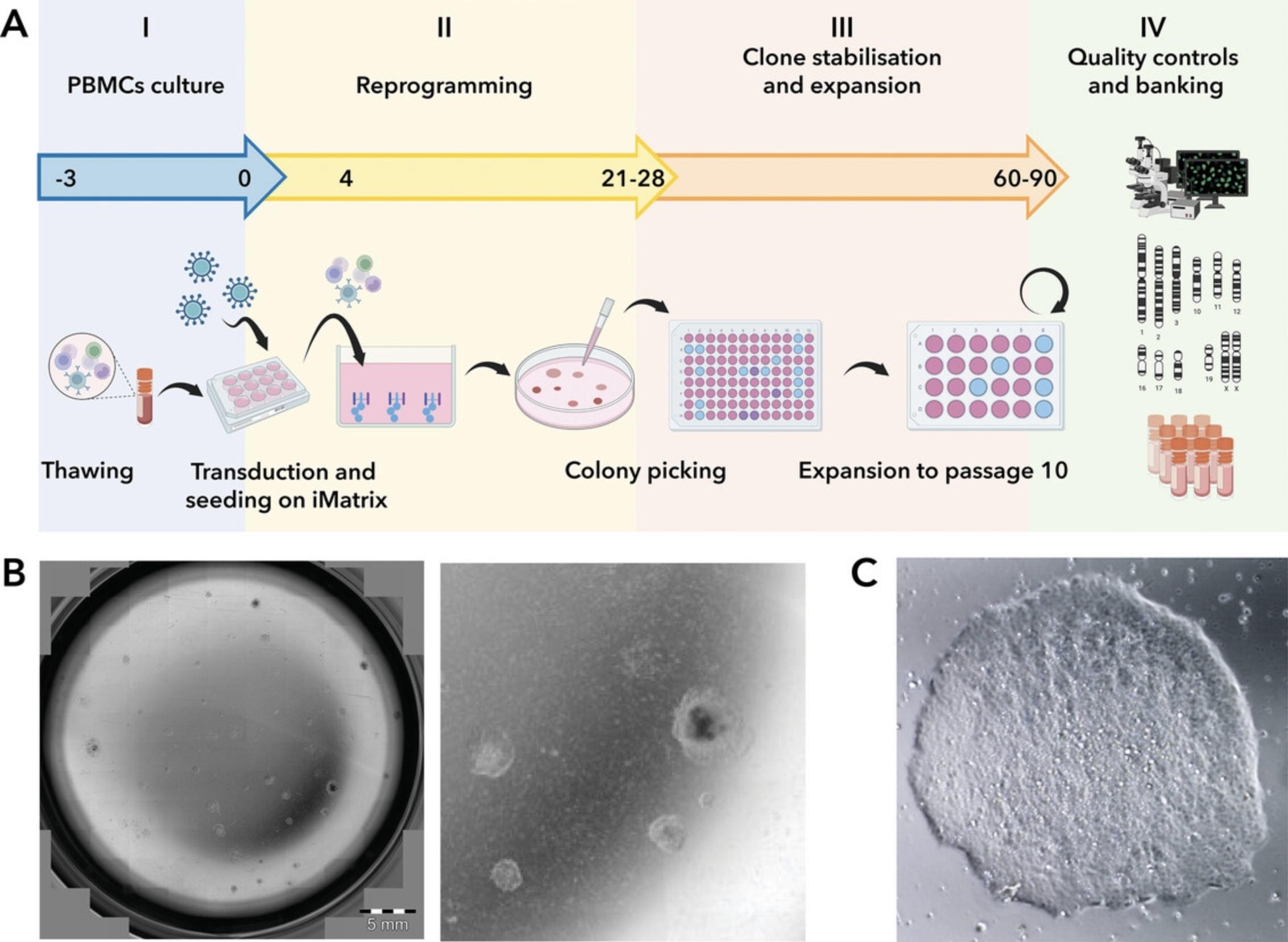
Materials
-
Frozen PBMCs (Stemcell Technologies, cat. no. 70025.1)
-
StemPro-34 complete medium (see recipe)
-
PBMC medium (see recipe)
-
Trypan blue solution, 0.4% (Thermo Fisher Scientific, cat. no. 15250061)
-
Reprogramming virus mixture (see recipe)
-
Bleach
-
BG iMatrix-511 Silk, 350 µg × 6 (1050 µg) (Biogems, cat. no. RL511S-350)
-
Essential 8 complete medium (see recipe)
-
Water bath, 37°C
-
15-ml polystyrene conical tubes, sterile
-
Benchtop centrifuge
-
2-, 5-, and 10-ml serological pipettes, sterile
-
0.5- to 1000-µl micropipettes and tips
-
96-, 12-, and 6-well cell culture plates
-
Incubator, humidified, 37°C, 5% CO2
-
1.5- and 2-ml tubes, sterile
-
Inverted widefield microscope
Thawing and culturing of PBMCs (days −3 to −1)
PBMCs viability can vary greatly from sample to sample. Before transducing the cells (day 0), you may expect up to 90% cell death with respect to the thawing day (day −3). Alternate Protocol addresses the PBMCs low viability issue and may prevent failure of the reprogramming process.
Day −3: Thawing and plating of cryopreserved PBMCs
1.Retrieve PBMCs cryovials from the liquid nitrogen and incubate the cryotube immediately at 37°C in a water bath until 70% of the cell suspension is defrosted.
2.Transfer the thawed PBMCs to a 15-ml tube, slowly dilute with 5 ml StemPro-34 complete medium, and centrifuge 10 min at 200 × g , room temperature.
3.Discard the supernatant and resuspend the cell pellet in PBMC medium at a density of 5 × 105 cells/ml.
4.Plate the cells in the appropriate vessel depending on the volume obtained in step 3 (e.g., 1 to 2 ml in 1 well of a 12-well plate, 2 to 4 ml in 1 well of a 6-well plate, etc.).
5.Transfer the plate to incubator at 37°C, 5% CO2.
Days −2 and −1: Half-medium change with fresh PBMC medium
6.On day −2 gently collect half the medium from the top of the well trying not to disturb the cells remaining in the well and transfer it to a new 15-ml tube.
7.Centrifuge the tube 10 min at 200 × g , room temperature, discard the supernatant, and resuspend the cells in the same volume of fresh PBMC medium before adding them back to the same well.
8.Transfer the plate to incubator at 37°C, 5% CO2.
9.On day −1, Repeat steps 6 to 8.
10.On day 0, cells are ready for transduction.
Transduction of PBMCs using CytoTune-iPS 2.0 Sendai reprogramming kit (days 0 to 3)
Reprogramming efficiency is influenced by the quality of the PBMCs and the PBMC preparation. If PBMCs are derived from immunodepressed individuals or the preparation contains contaminant cells (e.g., red blood cells or platelets), reprogramming efficiency may be dramatically affected. Significant cell death after thawing (>90%) could lead to the failure of the reprogramming protocol.
Day 0: Transduction and plating of PBMCs
11.Transfer PBMCs suspension into a sterile 15-ml tube.
12.Count viable cells using the trypan blue exclusion method (Phelan, 2006), or the desired method.
13.Transfer 1.5 × 105 PBMCs to a 2-ml tube and centrifuge 10 min at 200 × g , room temperature.
14.Warm 1 ml PBMC medium to room temperature.
15.Retrieve a reprogramming virus mixture aliquot from −80°C and thaw it on ice.
16.Combine the thawed virus mixture with 1 ml PBMC medium.
17.Ensure that the solution is mixed by gently pipetting.
18.Discard the supernatant and resuspend the cell pellet in 1 ml reprogramming virus mixture by gently pipetting up and down.
19.Perform spinoculation by centrifuging the tube 30 min at 1000 × g , room temperature.
20.Once the centrifugation is complete, gently resuspend the cells in the supernatant and transfer the cell suspension to a well of a 12-well plate.
21.Add 1 ml PBMC medium and incubate overnight in the incubator at 37°C, 5% CO2. The final volume in the well is 2 ml.
Day 1: Remove the medium containing Sendai viruses
22.Transfer 2 ml of the cell suspension into a new tube.
23.Centrifuge 10 min at 200 × g , room temperature.
24.Add 0.5 ml fresh PBMC medium to the original source well.
25.Discard the supernatant, resuspend the cell pellet in 0.5 ml PBMC medium and transfer the cell suspension to the original well. The final volume in the well is 1 ml.
26.Transfer the plate to the incubator at 37°C, 5% CO2 for 2 days.
Transition to PSC culture conditions and emergence of iPSC colonies (days 4 to 28)
Days 4 to 6: Plating transduced PBMCs under precoating free conditions using iMatrix-511
27.Count viable cells using the trypan blue exclusion method or the desired method.
28.Transfer 12,500 cells/well in a 6-well plate. Add StemPro-34 complete medium up to 2 ml and supplement with 0.25 µg/cm2 iMatrix-511.
29.Transfer the plate to the incubator at 37°C, 5% CO2 for 3 days.
Day 7: Transitioning cells to Essential 8 complete medium
30.Warm 1ml Essential 8 complete medium to room temperature for each well prepared in step 28.
31.Remove 1 ml StemPro-34 medium from the cells and substitute it with 1 ml Essential 8 complete medium to initiate the process of adaptation to the new culture medium.
32.Transfer the plate to the incubator at 37°C, 5% CO2.
Days 8 to 28: Daily medium changes and iPSC colonies emergence
33.Change the entire volume of the medium with fresh Essential 8 complete medium daily.
34.Monitor the well(s) under a microscope for the appearance of cell clumps, indicative of nascent hiPSC-like colonies.
35.When colonies are ready for picking and transfer (Fig. 1B and C), proceed with Basic Protocol 2.
Alternate Protocol: ERYTHROID PROGENITOR CELLS (EPCs) ENRICHMENT AND REPROGRAMMING INTO iPSCs USING SENDAI VIRAL VECTORS
PBMCs are cultured and expanded for 8 days in a serum-free medium that includes a specific combination of recombinant human cytokines for expansion of human hematopoietic cells. This formulation selectively enhances the expansion and differentiation of erythroid progenitor cells derived from CD34+ cells (Fig. 2).
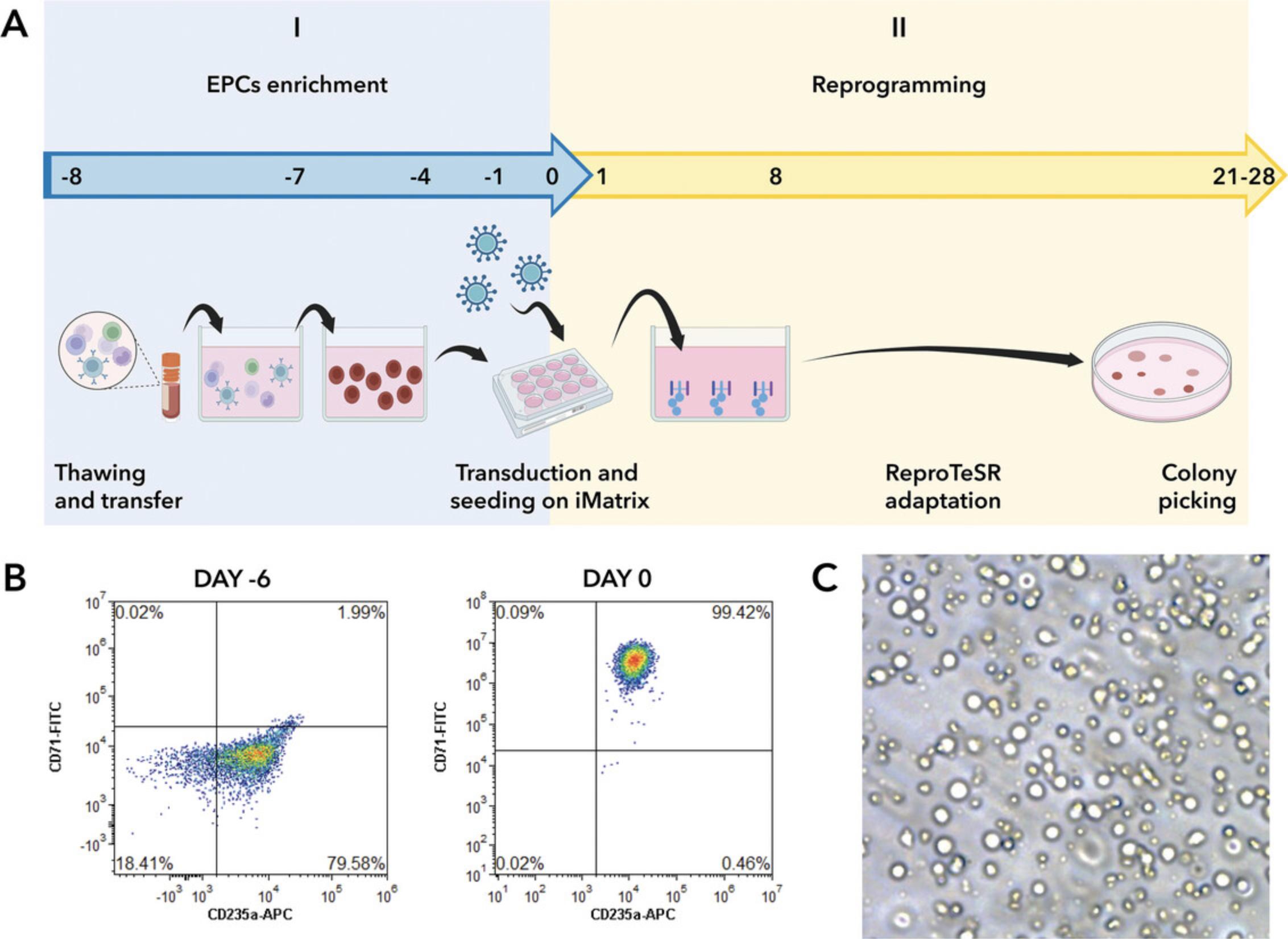
Additional Materials (also see Basic Protocol 1)
- Complete erythroid expansion medium (see recipe)
- ReproTeSR complete medium (see recipe)
Enrichment of EPCs starting from a vial of frozen PBMCs (days −8 to 0)
Day −8: Thawing and plating of cryopreserved PBMCs
1.Retrieve PBMCs cryovials from the liquid nitrogen and incubate the cryotube immediately at 37°C in a water bath until 70% of the cell suspension is defrosted.
2.Transfer the thawed PBMCs to a 15-ml tube, slowly dilute with 5 ml StemPro-34 complete medium, and centrifuge 10 min at 200 × g , room temperature.
3.Discard the supernatant and resuspend the cell pellet into 0.5 ml complete erythroid expansion medium.
4.Count viable cells using the trypan blue exclusion method or the desired method.
5.Dilute the cell suspension in complete erythroid expansion medium to a final density 1.5-2.5 × 105 cells/ml.
6.Add 2 ml cell suspension to 1 well of a 6-well plate, yielding a final cell density of 300,000 to 500,000 cells/well.
7.Transfer the plate to the incubator at 37°C, 5% CO2.
Day −7: Transfer of non-adherent PBMCs
8.Transfer the cell suspension to a new well (6-well plate) without replacing the medium.
Days −6, −4, and −1: Medium change and cell plating at optimal density
9.Transfer the cell suspension to a 15-ml conical tube.
10.Centrifuge the tube 5 min at 300 × g , room temperature.
11.Discard the supernatant and resuspend the cell pellet in 1 ml fresh complete erythroid expansion medium.
12.Count viable cells using the trypan blue exclusion method or the desired method.
13.Dilute the cell suspension in complete erythroid expansion medium to a final density 1.5–2.5 × 105 cells/ml.
14.Add complete erythroid expansion medium to a final volume of 2 ml.
15.Transfer the plate to the incubator at 37°C and 5% CO2.
Reprogramming of EPCs into iPSCs using Sendai virus vectors without precoating (days 0 to 28)
Day 0: Transduction and plating of EPCs
16.Transfer the cell suspension to a 15-ml conical tube.
17.Centrifuge 5 min at 300 × g , room temperature.
18.Discard the supernatant and resuspend the cell pellet in fresh complete erythroid expansion medium.
19.Count viable cells using the trypan blue exclusion method or the desired method.
20.Transfer 1.5 × 105 live EPCs to a 2-ml tube and centrifuge 10 min at 200 × g , room temperature.
21.Warm 1 ml of complete erythroid expansion medium to room temperature.
22.Retrieve a reprogramming virus mixture aliquot from −80°C and thaw it on ice.
23.Combine the thawed virus mixture with 1 ml complete erythroid expansion medium.
24.Ensure that the solution is mixed by gently pipetting.
25.Discard the supernatant and resuspend the cell pellet in 1 ml complete erythroid expansion medium supplemented with the reprogramming virus mixture. Gently pipette up and down.
26.Perform spinoculation by centrifuging the tube 30 min at 1000 × g , room temperature.
27.Once the centrifugation is complete, gently resuspend the cells in the supernatant and transfer the cell suspension to a well of a 12-well plate.
28.Add 1 ml complete erythroid expansion medium and transfer the plate to the incubator at 37°C, 5% CO2. The final volume in the well is 2 ml.
Day 1: Remove the medium containing Sendai virus vectors
29.Transfer 2 ml of the cell suspension to a clean, sterile 15-ml tube.
30.Centrifuge 10 min at 200 × g , room temperature.
31.Add 1.5 ml fresh complete erythroid expansion medium to the original source well.
32.Discard the supernatant, resuspend the cell pellet in 0.5 ml complete erythroid expansion medium, and transfer the cell suspension to the original well.
33.Transfer the plate to the incubator at 37°C, 5% CO2.
Day 2: Plating of transduced EPCs under precoating free conditions using iMatrix-511
34.Transfer the cell suspension to a 15 ml-tube.
35.Centrifuge the tube 5 min at 300 × g , room temperature.
36.Discard the supernatant and resuspend the cell pellet in 1 ml complete erythroid expansion medium.
37.Count viable cells using the trypan blue exclusion method or the desired method.
38.Transfer a quantity of cells ranging from 7–15 × 105 into each of three separate wells of a 6-well plate, and supplement each well with 1.5 ml fresh complete erythroid expansion medium.
39.Add 0.25 µg/cm2 iMatrix-511 in each well (5 µl stock solution/well) to favor cell adhesion and transfer the plate to the incubator at 37°C, 5% CO2.
Day 3: Addition of fresh complete erythroid expansion medium
40.Add 1 ml fresh complete erythroid expansion medium to each well.
41.Transfer the plate to the incubator at 37°C, 5% CO2.
Days 4 and 7: Addition of ReproTeSR
42.On day 4, add 1 ml ReproTeSR complete medium to each well.
43.Incubate the plate in the incubator at 37°C, 5% CO2 until day 7.
44.On day 7, add 1 ml ReproTeSR medium to each well.
45.Transfer the plate to the incubator at 37°C, 5% CO2.
Days 8 to 20: Daily medium change
46.Carefully aspirate the supernatant from each well and add 1.5 ml ReproTeSR medium.
47.Incubate the plate in the incubator at 37°C, 5% CO2.
48.Between day 9 and day 20, execute a daily change of the medium. Aspirate the medium from each well and add 1.5 ml ReproTeSR medium.
49.Transfer the plate to the incubator at 37°C, 5% CO2.
Days 21 to 28: Transitioning to Essential 8 medium and iPSC colonies emergence
50.On day 21, aspirate the supernatant from each well and add 0.375 ml Essential 8 complete medium and 1.125 ml ReproTeSR medium.
51.Incubate the plate overnight in the incubator at 37°C, 5% CO2.
52.On day 22, aspirate the supernatant from each well and add 0.75 ml Essential 8 complete medium and 0.75 ml ReproTeSR medium.
53.Incubate the plate overnight in the incubator at 37°C, 5% CO2.
54.On day 23, aspirate the supernatant from each well and add 1.125 ml Essential 8 complete medium and 0.375 ml ReproTeSR medium.
55.Transfer the plate to the incubator at 37°C, 5% CO2.
56.On day 24, aspirate the supernatant from each well and add 1.5 ml Essential 8 complete medium.
57.Incubate the plate overnight in the incubator at 37°C, 5% CO2.
58.Between day 25 and day 28, execute a daily change of the medium. Aspirate the medium from each well and add 1.5 ml Essential 8 complete medium.
59.Monitor the well(s) under a microscope for the appearance of cell clumps, indicative of nascent iPSC-like colonies.
60.When colonies are ready for picking and transfer (Fig. 1B and C), continue with Basic Protocol 2.
Basic Protocol 2: PICKING AND PRECOATING-FREE OPTIMIZED EXPANSION OF iPSC CLONES
This protocol outlines the procedure for isolating and expanding hiPSC clones generated with Basic Protocol 1 or Alternate Protocol. Partially reprogrammed colonies may become evident as early as day 12 post-transduction. By day 21, the cell colonies are expected to have undergone further growth and maturation, displaying a more substantial and compact appearance. However, only a fraction of these colonies will consist of iPSCs, which are recognizable by their homogeneous and tightly packed cells with clear borders and well-defined edges (see Understanding Results). Allowing the colonies to grow larger than required for picking offers no benefit. Overly large colonies tend to develop differentiated regions or might merge with neighboring colonies.
Mature hiPSC colonies can be manually picked by mechanical dissection into small pieces and transferred into a 96-well plate. The commonly employed protocols advise placing harvested colonies in wells precoated with extracellular matrix extracts or recombinant proteins. The approach outlined in this protocol removes the requirement for precoating plastic surfaces by utilizing a culture medium supplemented with iMatrix-511. This adjustment enhances the efficiency and convenience of cell seeding post-transduction (see Commentary).
The colonies initially moved to 96-well plates can be subsequently expanded by sequential transfer to culture plates with larger surface areas, such as 24- and 6-well plates. This expansion process is a preparatory step for allowing the maturation and stabilization of the iPSC line and ensures the clearance of residual reprogramming transgenes (i.e., the Sendai virus RNA).
Following a minimum of eight passages in 24-well plates, we recommend selection of no fewer than six clones for a comprehensive analysis of genome integrity and confirmation of Sendai virus clearance. Among these, 50% to 80% of clones should test negative for the presence of Sendai virus transgenes and exhibit no signs of abnormal karyotype or acquired copy number variations (CNVs). These selected clones should be expanded in 6-well plates and frozen once they reach passage 10.
Picking reprogrammed iPSC colonies
This protocol allows for the selection and picking of iPSC colonies by employing a transmitted light imaging system positioned on the work area of a class II laminar flow hood. This integration enables seamless coupling between the imaging and picking steps. Alternatively, a standard inverted microscope can be used to identify and label the iPSC colonies for picking. Subsequently, the culture plate can be transferred into the biosafety cabinet, where the manual picking takes place in a sterile environment.
A successful reprogramming experiment enables the picking of 30 to 60 colonies (10 to 20 colonies per well). Whenever possible, isolate a minimum of 20 colonies.
NOTE : We recommend performing maximum two picking sessions per sample to avoid cross contamination between clones, waiting 2 to 3 days between them to reach 40 to 50 individual iPSC-picked colonies per sample.
REAGENTS AND SOLUTIONS
CEPT mixture
The CEPT (Chroman-1, Emricasan, Polyamine, Trans-ISRIB) is a combination of small-molecule compounds that effectively enhance cytoprotective cell survival in hPSCs by shielding them from various stress mechanisms (Chen et al., 2021). CEPT can be used as an alternative to ROCK inhibitors like Y-27632, thiazovivin, and blebbistatin, ultimately leading to improved cell survival. CEPT is composed of the following reagents (Table 1 indicates the final concentration for each reagent):
- Chroman-1 (MedChemExpress, cat. no. HY-15392)
- Emricasan (Sigma-Aldrich, cat. no. SML2227-5MG)
- Trans-ISRIB (Tocris, cat. no. 5284)
- Polyamine supplement (Sigma-Aldrich, cat. no. P8483-5ML)
Reagent | 1× concentration |
---|---|
Chroman-1 | 50 nM |
Emricasan | 1.5 µM |
Polyamine supplement (1000×) | 1× |
Trans-ISRIB | 0.7 µM |
Combine 200 µM Chroman-1, 6 mM Emricasan, and 2.8 mM trans-ISRIB to create a final stock concentration of 1333×, referred to as the CET 1333× mix. This mix can be safely stored up to 1 year at −80°C. The polyamine supplement is stored until the expiry date at 4°C and should be added at the time of use by diluting it at a ratio of 1:1000.
Complete erythroid expansion medium
- Prepare complete erythroid expansion medium:
- Thaw the StemSpan erythroid expansion supplement (STEMCELL Technologies, cat. no. 02692) and the StemSpan SFEM II medium (STEMCELL Technologies, cat. no. 09605) on ice or at 4°C
- Aliquot both the StemSpan erythroid expansion supplement (100 µl aliquots) and the StemSpan SFEM II (10 ml aliquots) preserving the sterility of all the components
- Store at −20°C until the date of expiration of the components
- To generate an aliquot of erythroid expansion complete medium, thaw 1 aliquot of StemSpan erythroid expansion supplement and 1 aliquot of StemSpan SFEM II
- Using sterile technique add 100 µl StemSpan erythroid expansion supplement to 10 ml StemSpan SFEM II and mix gently
- Store the complete medium up to 15 days at 4°C
For detailed instructions refer to the StemSpan SFEM II and StemSpan erythroid expansion supplement manuals.
EDTA, 0.5 mM working stock
Prepare 10 to 50 ml working stock solution by diluting UltraPure 0.5 M EDTA, pH 8.0 (Thermo Fisher Scientific, cat. no 15575-020) to 0.5 mM in PBS, pH 7.2 w/o Ca and Mg (Thermo Fisher Scientific, cat. no. 20012027) in sterile conditions. Store up to 2 weeks at room temperature.
Essential 8 complete medium
- Essential 8 medium (Thermo Fisher Scientific, cat. no. A1517001) contains:
- 500 ml Essential 8 basal medium (store at 2° to 8°C protected from light)
- 10 ml of 50× Essential 8 supplement (store –20° to −5°C protected from light)
- Prepare Essential 8 complete medium:
- Thaw Essential 8 Supplement at room temperature
- Mix the supplement by gently inverting the vial
- Remove 10 ml from the bottle of Essential 8 basal medium, then transfer 10 ml Essential 8 supplement to the bottle of Essential 8 basal medium
- Store protected from light up to 2 weeks at 4°C or up to 6 months at −20°C
- Optional: add 1% penicillin-streptomycin (10,000 U/ml; Thermo Fisher Scientific cat. no. 15140122)
For detailed instructions refer to the Essential 8 Medium manual.
Essential 8 complete medium supplemented with CEPT and iMatrix-511
Prepare the Essential 8 complete medium (see recipe), incorporating CEPT components (see recipe) at the concentrations outlined in Table 1. For iMatrix-511 (500 µg/cm² stock solution; Biogems, cat. no. 348RL511S), add it at a final working concentration of 0.25 µg/cm² immediately prior to cell seeding. The appropriate quantity of iMatrix-511 to be used, as detailed in Table 2, varies based on plate format and well area.
Cell culture plate format | Well area (cm2) | Amount of iMatrix-511 stock/well |
---|---|---|
96-well | 0.32 | 0.2 µl |
24-well | 1.9 | 1 µl |
6-well | 9.5 | 5 µl |
PBMC medium
- StemPro-34 complete medium (see recipe) supplemented with:
- 100 ng/ml SCF (Peprotech, cat. no. AF-300-07)
- 100 ng/ml FLT-3 (Peprotech, cat. no. AF-300-19)
- 20 ng/ml IL-3 (Peprotech, cat. no. AF-200-03)
- 20 ng/ml IL-6 (Peprotech, cat. no. AF-200-06)
- Prepare fresh
Reprogramming virus mixture
Required reagents for this mixture are included in the CytoTune-iPS 2.0 Sendai reprogramming kit (Thermo Fisher Scientific, cat. no. A16518). In accordance with the standard manufacturer's instructions, it is advisable to use a multiplicity of infection (MOI) of 5-5-3 for KOS, cMYC, and KLF4 Sendai viral particles. Creating individual, single-use aliquots that combine all three Sendai particles can greatly facilitate the protocol's execution. These aliquots can then be safely stored at −80°C until they are required. Calculate the volume of viral particles to obtain the 5-5-3 MOI according to the following formula, where CIU is cell infectious units, as reported on the manufacturer's recommendations:
- Volume of virus (µl) = [MOI (CIU/cell) × number of cells]/[titer of virus (CIU/ml) × 10-3 (ml/µl)]
The following specifications can be used as a starting point to prepare single-use aliquots of virus mixture: cMYC titer, 1.5 × 108; KOS titer, 1.5 × 108; KLF4 titer: 1.4 × 108. Follow the outlined steps to create individual-use aliquots, each suitable for transducing 1.5 × 105 cells (the aliquots should consist of a mixture of the three Sendai particles in the specified ratios: 5 µl cMYC, 5 µl KOS, and 3.2 µl KLF4):
- Cool down sterile 200-µl tips, 10-µl tips, and 1.5-ml tubes at −20°C, overnight
- Thaw all three tubes of Sendai viral particles on ice and resuspend them using a micropipette
- Prepare a mix of the three viruses to obtain the 5-5-3 MOI on ice in a pre-chilled 1.5-ml tube
- Flash-freeze the virus mixture on dry ice
- Store the aliquots immediately at −80°C up to the expiration date reported on the manufacturers' Certificate of Analysis
The titer of the CytoTune 2.0 reprogramming vector varies depending on the lot. To find the exact titer of your vectors, visit thermofisher.com/cytotune and search for the Certificate of Analysis (CoA) using the product lot number, which is indicated on the vial.
It is advisable to avoid repeated freezing and thawing of the reprogramming vectors as viral titers can significantly decrease with each cycle.
ReproTeSR complete medium
- ReproTeSR complete medium (STEMCELL Technologies, cat. No. 05926) contains:
- 480 ml TeSR-E7/ReproTeSR basal medium (store at 2° to 8°C protected from light)
- 20 ml ReproTeSR 25× supplement (store at –20°C protected from light)
- Prepare ReproTeSR complete medium:
- Thaw ReproTeSR 25× supplement at room temperature
- Mix the supplement by gently inverting the vial
- Transfer 20 ml ReproTeSR 25× supplement to the bottle of TeSR-E7/ReproTeSR basal medium
- Store protected from light up to 2 weeks at 4°C or up to 6 months at −20°C
- Optional: add 1% penicillin-streptomycin (10,000 U/ml; Thermo Fisher Scientific, cat. no. 15140122).
For detailed instructions refer to the ReproTeSR medium manual.
StemPro-34 complete medium
- StemPro-34 SFM kit (Thermo Fisher Scientific, cat. no. 10639011) contains:
- 500 ml of 1× StemPro-34 SFM (store at 2° to 8°C protected from light)
- 13 ml of 40× StemPro-34 nutrient supplement (store at –20° to –5°C protected from light)
- Prepare StemPro-34 complete medium:
- Thaw StemPro-34 nutrient supplement overnight at 4°C
- Add 13 ml StemPro-34 nutrient supplement directly to the 500 ml bottle of StemPro-34 SFM basal medium and rotate to mix
- Add GlutaMAX supplement (Thermo Fisher Scientific, cat. no. 35050061) to a final concentration of 1×
- Store protected from light up to 1 month at 4°C
- Optional: add 1% penicillin-streptomycin (10,000 U/ml; Thermo Fisher Scientific, cat. no. 15140122).
For detailed instructions refer to the StemPro-34 SFM (1×) medium manual.
COMMENTARY
Background Information
The ability to derive potentially pluripotent stem cells from any individual was the cornerstone of a new era in stem cell biology. For decades, the study of the molecular and cellular mechanisms underlying human disease has relied on the use of a variety of models, both in vitro and in vivo, whose intrinsic limitations have slowed the discovery of potential therapeutic targets and the introduction of tailored treatments for patients. Immortalized human cell lines do not represent a faithful physiological model of the disease. Animal models, in which inter- and intra-species differences are the main obstacle to the development of effective therapies in humans, are expensive and have limited scalability. Primary cells, obtained either from the patient or from an animal model, are often the sole available source but are rarely readily accessible and do not allow for broad validations due to the complexity of culture maintenance techniques. iPSCs and their potential to differentiate in different cell types, represent an almost inexhaustible source of biological material, allowing the study of the mechanism underlying complex pathologies that have remained inaccessible up to now.
Currently, there are various approaches available for generating iPSCs through the reprogramming of somatic cells derived from skin biopsies (fibroblasts; Poleganov et al., 2015) or blood samples (PBMCs; Churko et al., 2013). Although these methods still face challenges in terms of low efficiency, they do enable the isolation and expansion of bona fide pluripotent stem cell clones.
Following clone isolation, the primary methods employed to sustain long-term hPSCs cultures involve coating the culture surfaces with ECM extracts (e.g., Matrigel and Geltrex) (Xu et al., 2001). However, these substances show variable properties between different batches and their interaction with hPSCs is not well-defined (reviewed in Aisenbrey & Murphy, 2020). Numerous efforts have been made to identify viable alternatives to the use of complex ECMs. One particularly promising approach involves replacing Matrigel with its core components, such as recombinant proteins like laminins (Miyazaki et al., 2012; Rodin et al., 2010, 2014) or vitronectin (Braam et al., 2008).
Laminin-511 and laminin-521 both permit clonal survival and long-term self-renewal of hESCs under xeno-free conditions, as demonstrated by Rodin et al. (2010, 2014). The finding that laminin-E8 fragments (LM-E8s) enable reliable replication of hESCs and hiPSCs in an undifferentiated state represented a further development (Miyazaki et al., 2012).
Regardless of the selected substrate, feeder-free hPSC passage protocols invariably involve surface coating. This passage must be carefully planned, anticipating the next steps, and introducing an additional hurdle in the sequential high-throughput processing of cultured stem cells. Moreover, coating the culture surface with Matrigel requires careful temperature control to avoid the undesirable phenomenon of matrix gelation. Laborious and inefficient manual preparation of culture surfaces is an important limiting factor for the implementation of high-throughput and robotic platform compatible workflows for iPSC generation and manipulation, affecting the development and optimization of scalable and cost-effective stem cell propagation systems.
The utilization of a recombinant laminin-511 fragment, known as iMatrix-511, and its application in coating-free conditions for stem cell culture propagation marked a notable breakthrough in the advancement of protocols suitable for highly automated processes that involve liquid handlers and reagent dispensers (Miyazaki et al., 2017). The authors investigated the effectiveness of substrate proteins (iMatrix-511, laminin-521, and rhVTN-N, which is a truncated form of human vitronectin) when added directly to the culture medium during cell seeding, without the need for precoating the culture surface. Remarkably, the inclusion of iMatrix-511 as a medium supplement shows to be an excellent method of supporting iPSCs proliferation.
Our objective was to create and refine a protocol that integrates iMatrix-511 as a medium supplement throughout the entire process of generating iPSCs. As depicted in Basic Protocol 1, iMatrix-511 is introduced into the culture medium as an additive starting from the initial clonal seeding phase, immediately after the application of reprogramming factors (colony emergence days 4 to 21). By omitting the coating step, the reprogramming process remains unaffected, resulting in the formation of stem cell colonies exhibiting morphological features comparable to those produced by the traditional method (Fig. 1).
The protocol can be successfully executed by incorporating iMatrix-511 dilutions into the culture media provided for subsequent passages, eliminating de facto the need for coating plastic surfaces. This enables the seamless progression of subsequent steps and ultimately facilitates the generation of iPSCs. In addition, uncoated iMatrix-511 promotes cell attachment and long-term growth at lower doses (0.25 µg/cm2) than the precoated protocol (1 µg/cm2), thereby improving cost-effectiveness in the generation and expansion of hPSCs.
The ability to complete the entire experimental flow of reprogramming somatic cells into iPSCs without relying on complex extracellular matrices and omitting the tedious and labor-intensive method of coating the culture surfaces, is an essential piece in the quest to establish automated disease modeling platforms with high efficiency and capacity.
Critical Parameters
The process of reprogramming PBMCs into iPSCs is prone to variability and is heavily influenced by factors, such as the user's level of expertise, proper storage and handling of reagents, quality of the starting biological material, and the availability of suitable equipment for conducting key steps distributed throughout the experimental workflow. Addressing the critical parameter that significantly influences the success rate of the entire reprogramming protocol is beyond the scope of this study. Detailed discussions regarding this matter can be found elsewhere (González & Huangfu, 2016; Meir & Li, 2021; Rao & Malik, 2012). Here, we focus only on two fundamental benchmarks: quality of the starting material, and optimal density for clonal seeding.
Quality of PBMCs
The effectiveness of the reprogramming protocol is closely tied to the quality of cryopreserved PBMCs. The viability of cells during the thawing process significantly influences the number of target cells and their quality during the transduction step using the CytoTune-iPS 2.0 Sendai reprogramming kit. PBMCs stored for a long time under suboptimal conditions may show <20% viability after seeding, a condition that could significantly diminish the success rate of the reprogramming process.
In cases where PBMC quality is poor or it is not possible to assess the viability of the available biological material in advance, the Alternate Protocol can be considered a viable solution. Expansion of the erythroid compartment, through the proliferation of rare progenitor cells in the sample, can circumvent the limitation imposed by the scarcity of starting material (Chou et al., 2011; Park et al., 2020; Singh et al., 2022).
Clonal seeding
Following the transduction of PBMCs (or EPCs in Alternate Protocol) with Sendai virus cocktails containing reprogramming factors, it is crucial to carefully consider the cell number for clonal seeding. Using iMatrix-511 as a supplement in culture medium, our protocol is designed to optimize the seeding of 12,500 cells in a single well of a 12-well plate. However, it is recommended for each user to optimize the ideal seeding density based on their specific experimental conditions, standardizing counting procedures before reprogramming multiple subjects simultaneously. After their emergence, iPSC colonies can be manually transferred to a 96-well plate and expanded.
Troubleshooting
See Table 3 for a list of possible problems, causes and solutions.
Problem | Possible cause | Solution |
---|---|---|
>90% cell death before transduction | Improper i. medium formulation, or ii. growth factors handling | Repeat medium preparation, keep growth factors on ice, use StemPro-34 complete medium within 2 weeks from preparation, prepare fresh PBMC medium daily |
>90% cell death before transduction | Improper PBMCs isolation | Repeat blood draw and PBMC isolation |
Contaminant cells (RBCs and platelets) in PBMC preparation | Improper PBMCs isolation | Repeat blood draw and PBMC isolation if reprogramming is affected |
>50% iPSC colonies differentiation before picking | Cell density too high | Repeat transduction seeding at a lower cell density |
>50% iPSC colonies differentiation before picking | Improper Essential 8 complete medium handling | Repeat medium preparation, use within 2 weeks, never warm to 37°C |
Sendai genome persistence after 10 passages | Improper subcloning during passaging | Perform subcloning selecting fewer colonies during passaging |
iPSC clones differentiation | Improper Essential 8 complete medium handling | Repeat medium preparation, use within 2 weeks, never warm to 37°C |
iPSC clones differentiation | Improper passaging technique | Pipette gently, adjust EDTA incubation time |
Understanding Results
Following transduction with Sendai viral vectors, PBMCs or expanded EPCs are seeded at clonal density in one or more wells of a 6-well plate. The emergence of partially reprogrammed colonies is evident as early as day 15 post-transduction (see Fig. 1B and C). While the recommended seeding densities are 12,500 (Basic Protocol 1) or 7–15 × 105 (Alternate Protocol), it is noteworthy that these cell densities do not consistently ensure the formation of adequately spaced colonies suitable for picking and establishing stable iPSC lines. The optimal colony density for proceeding to the picking phase is illustrated in Figure 1B. Users, particularly those with limited reprogramming experience, may choose to seed cells at varying densities post-transduction to acquaint themselves with the protocol.
By day 21, the cell colonies are anticipated to have proliferated, displaying a compact appearance. Figure 4 provides a visual representation of an ideal iPSC colony. Parameters, such as the number of nucleoli, nucleolus-nucleolus ratio, cell size, nucleus-cytoplasm ratio, and the presence of well-defined borders are critical for distinguishing bona fide iPSC colonies.
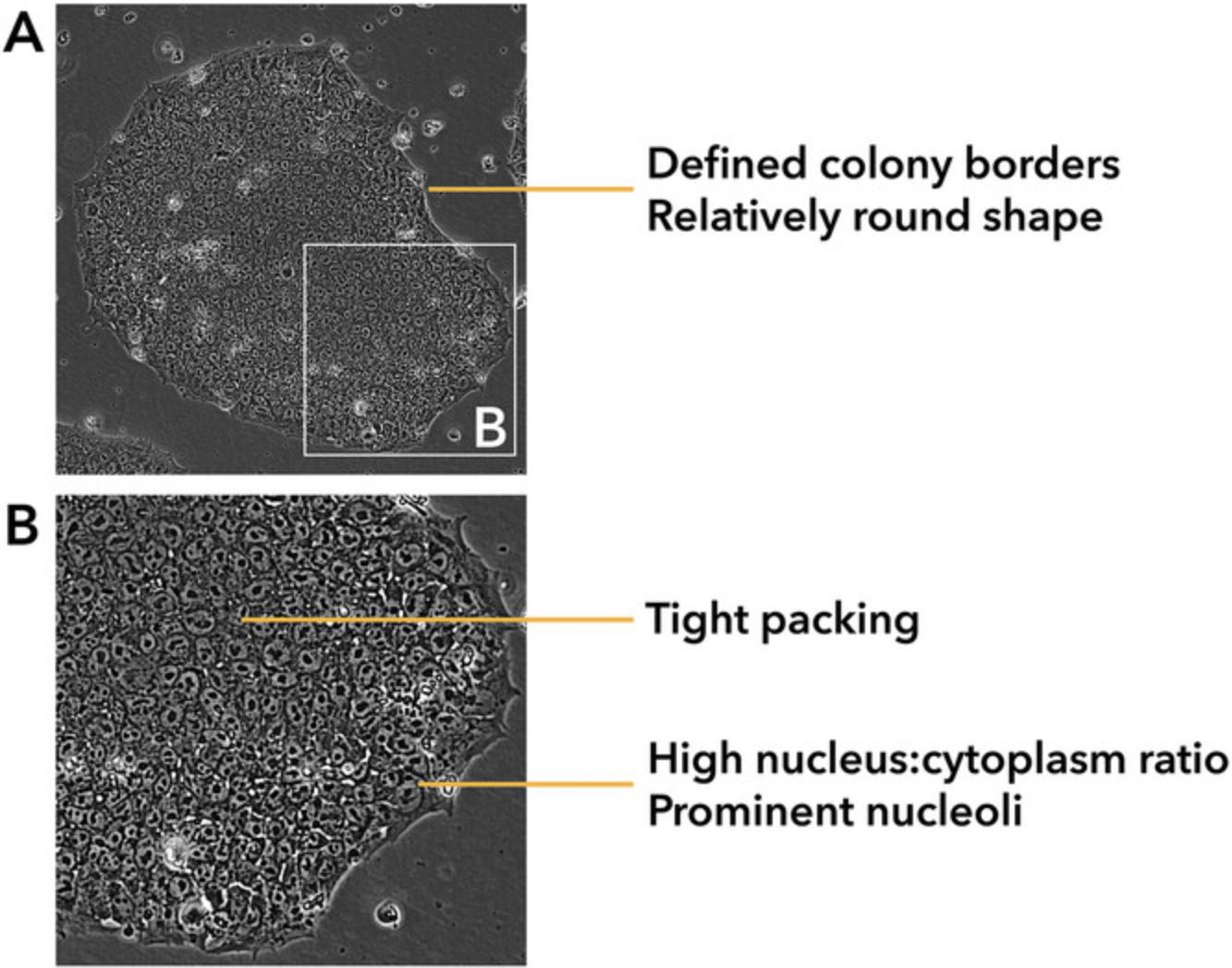
It is crucial to emphasize that the isolation of iPSC colonies marks the conclusion of a multifaceted cell fate rewiring process, influenced by a variety of intrinsic and extrinsic factor (Mack et al., 2011; Skorik et al., 2020). Consequently, the ongoing expansion of stem cell aggregates in culture following isolation can frequently yield unpredictable outcomes. Typically, hiPSC colonies at early passages experience alterations in various characteristics, including their visual appearance, growth patterns, and proclivity for spontaneous differentiation. In summary, the continued growth of cultured stem cell aggregates post-isolation is likely to result in either the culture's demise or the formation of viable colonies displaying the characteristic morphological features associated with a successful reprogramming procedure (Ban et al., 2011; Skorik et al., 2020; Ye & Wang, 2018).
Particular attention must be paid to the method of passaging iPSC. Prolonged expansion of hPSC cultures has been linked to genetic and epigenetic alterations (Halliwell et al., 2020; Kuijk et al., 2020; Närvä et al., 2010). Both enzymatic and mechanical passaging methods for colonies have been associated with the promotion of genome aberrations, with a more pronounced association found in single cell enzymatic passaging (Bai et al., 2015; Garitaonandia et al., 2015; Tosca et al., 2015). To address these concerns, our recommendation is to utilize 0.5 mM EDTA for the chemical dissociation of colonies. This approach offers precise control over the size of cell clumps and minimizes the potential for excessive mechanical stress during pipetting (also see Skorik et al., 2020).
Time Considerations
A typical reprogramming experiment spans a duration of 2 to 3 months. Following the picking phase, iPSC clones will exhibit varying growth rates, leading to asynchronous attainment of passage 10 and consequently influencing the overall completion time of the protocol.
Acknowledgments
Figures 1A and 2A were generated using Biorender. We thank Giuseppe Testa, Nicolò Caporale, and Emanuele Villa for helpful discussions. The authors are grateful to Nicolò Panini, Silvia Bombelli, and Alessio Palini of the Flow Cytometry Facility at Human Technopole for the outstanding support provided.
Author Contributions
Elisabetta Fiacco : Conceptualization; investigation; methodology; resources; validation; visualization; writing original draft; writing review and editing. Sara Landi : Conceptualization; investigation; methodology; resources; validation; visualization; writing original draft; writing review and editing. Jacopo Zasso : Conceptualization; investigation; methodology; resources; validation; visualization; writing original draft; writing review and editing. Chiara Ambrosini : Investigation; methodology; resources; validation; writing review and editing. Giovanni Faga : Conceptualization; methodology; project administration; resources; supervision; validation; visualization; writing original draft; writing review and editing.
Conflict of Interest
The authors declare no conflict of interest.
Open Research
Data Availability Statement
Data sharing not applicable to this article as no datasets were generated or analyzed during the current study.
Literature Cited
- Aisenbrey, E. A., & Murphy, W. L. (2020). Synthetic alternatives to Matrigel. Nature Reviews. Materials , 5(7), 539–551. https://doi.org/10.1038/s41578-020-0199-8
- Bai, Q., Ramirez, J.-M., Becker, F., Pantesco, V., Lavabre-Bertrand, T., Hovatta, O., Lemaître, J.-M., Pellestor, F., & De Vos, J. (2015). Temporal analysis of genome alterations induced by single-cell passaging in human embryonic stem cells. Stem Cells and Development , 24(5), 653–662. https://doi.org/10.1089/scd.2014.0292
- Ban, H., Nishishita, N., Fusaki, N., Tabata, T., Saeki, K., Shikamura, M., Takada, N., Inoue, M., Hasegawa, M., Kawamata, S., & Nishikawa, S.-I. (2011). Efficient generation of transgene-free human induced pluripotent stem cells (iPSCs) by temperature-sensitive Sendai virus vectors. Proceedings of the National Academy of Sciences , 108(34), 14234–14239. https://doi.org/10.1073/pnas.1103509108
- Bluhmki, T., Traub, S., Müller, A.-K., Bitzer, S., Schruf, E., Bammert, M.-T., Leist, M., Gantner, F., Garnett, J. P., & Heilker, R. (2021). Functional human iPSC-derived alveolar-like cells cultured in a miniaturized 96‑Transwell air–liquid interface model. Scientific Reports , 11(1), 1. https://doi.org/10.1038/s41598-021-96565-4
- Braam, S. R., Zeinstra, L., Litjens, S., Ward-van Oostwaard, D., van den Brink, S., van Laake, L., Lebrin, F., Kats, P., Hochstenbach, R., Passier, R., Sonnenberg, A., & Mummery, C. L. (2008). Recombinant vitronectin is a functionally defined substrate that supports human embryonic stem cell self-renewal via αVβ5 integrin. Stem Cells , 26(9), 2257–2265. https://doi.org/10.1634/stemcells.2008-0291
- Chen, Y., Tristan, C. A., Chen, L., Jovanovic, V. M., Malley, C., Chu, P.-H., Ryu, S., Deng, T., Ormanoglu, P., Tao, D., Fang, Y., Slamecka, J., Hong, H., LeClair, C. A., Michael, S., Austin, C. P., Simeonov, A., & Singeç, I. (2021). A versatile polypharmacology platform promotes cytoprotection and viability of human pluripotent and differentiated cells. Nature Methods , 18(5), 528–541. https://doi.org/10.1038/s41592-021-01126-2
- Chou, B.-K., Mali, P., Huang, X., Ye, Z., Dowey, S. N., Resar, L. M., Zou, C., Zhang, Y. A., Tong, J., & Cheng, L. (2011). Efficient human iPS cell derivation by a non-integrating plasmid from blood cells with unique epigenetic and gene expression signatures. Cell Research , 21(3), 3. https://doi.org/10.1038/cr.2011.12
- Churko, J. M., Burridge, P. W., & Wu, J. C. (2013). Generation of human iPSCs from human peripheral blood mononuclear cells using non-integrative sendai virus in chemically defined conditions. In R. L. Kao (Ed.), Cellular Cardiomyoplasty: Methods and Protocols (pp. 81–88). Humana Press. https://doi.org/10.1007/978-1-62703-511-8_7
- Cohen, R. I., Thompson, M. L., Schryver, B., & Ehrhardt, R. O. (2015). Standardized cryopreservation of pluripotent stem cells. Current Protocols in Stem Cell Biology , 35(1), 1C.14.1–1C.14.14. https://doi.org/10.1002/9780470151808.sc01c14s35
- Garitaonandia, I., Amir, H., Boscolo, F. S., Wambua, G. K., Schultheisz, H. L., Sabatini, K., Morey, R., Waltz, S., Wang, Y.-C., Tran, H., Leonardo, T. R., Nazor, K., Slavin, I., Lynch, C., Li, Y., Coleman, R., Romero, I. G., Altun, G., Reynolds, D., … Laurent, L. C. (2015). Increased risk of genetic and epigenetic instability in human embryonic stem cells associated with specific culture conditions. PLOS ONE , 10(2), e0118307. https://doi.org/10.1371/journal.pone.0118307
- González, F., & Huangfu, D. (2016). Mechanisms underlying the formation of induced pluripotent stem cells. WIREs Developmental Biology , 5(1), 39–65. https://doi.org/10.1002/wdev.206
- Halliwell, J., Barbaric, I., & Andrews, P. W. (2020). Acquired genetic changes in human pluripotent stem cells: Origins and consequences. Nature Reviews Molecular Cell Biology , 21(12), 12. https://doi.org/10.1038/s41580-020-00292-z
- Josephson, R., & Auerbach, J. (2009). Authentication and banking of human pluripotent stem cells. Current Protocols in Stem Cell Biology , 11(1), 1C.9.1–1C.9.11. https://doi.org/10.1002/9780470151808.sc01c09s11
- Kuijk, E., Jager, M., van der Roest, B., Locati, M. D., Van Hoeck, A., Korzelius, J., Janssen, R., Besselink, N., Boymans, S., van Boxtel, R., & Cuppen, E. (2020). The mutational impact of culturing human pluripotent and adult stem cells. Nature Communications , 11(1), 1. https://doi.org/10.1038/s41467-020-16323-4
- Ludwig, T. E., Andrews, P. W., Barbaric, I., Benvenisty, N., Bhattacharyya, A., Crook, J. M., Daheron, L. M., Draper, J. S., Healy, L. E., Huch, M., Inamdar, M. S., Jensen, K. B., Kurtz, A., Lancaster, M. A., Liberali, P., Lutolf, M. P., Mummery, C. L., Pera, M. F., Sato, Y., … Mosher, J. T. (2023). ISSCR standards for the use of human stem cells in basic research. Stem Cell Reports , 18(9), 1744–1752. https://doi.org/10.1016/j.stemcr.2023.08.003
- Mack, A. A., Kroboth, S., Rajesh, D., & Wang, W. B. (2011). Generation of induced pluripotent stem cells from CD34+ cells across blood drawn from multiple donors with non-integrating episomal vectors. PLOS ONE , 6(11), e27956. https://doi.org/10.1371/journal.pone.0027956
- Meir, Y.-J. J., & Li, G. (2021). Somatic reprogramming—Above and beyond pluripotency. Cells , 10(11), 11. https://doi.org/10.3390/cells10112888
- Miyazaki, T., Futaki, S., Suemori, H., Taniguchi, Y., Yamada, M., Kawasaki, M., Hayashi, M., Kumagai, H., Nakatsuji, N., Sekiguchi, K., & Kawase, E. (2012). Laminin E8 fragments support efficient adhesion and expansion of dissociated human pluripotent stem cells. Nature Communications , 3(1), 1. https://doi.org/10.1038/ncomms2231
- Miyazaki, T., Isobe, T., Nakatsuji, N., & Suemori, H. (2017). Efficient adhesion culture of human pluripotent stem cells using laminin fragments in an uncoated manner. Scientific Reports , 7(1), 1. https://doi.org/10.1038/srep41165
- Närvä, E., Autio, R., Rahkonen, N., Kong, L., Harrison, N., Kitsberg, D., Borghese, L., Itskovitz-Eldor, J., Rasool, O., Dvorak, P., Hovatta, O., Otonkoski, T., Tuuri, T., Cui, W., Brüstle, O., Baker, D., Maltby, E., Moore, H. D., Benvenisty, N., … Lahesmaa, R. (2010). High-resolution DNA analysis of human embryonic stem cell lines reveals culture-induced copy number changes and loss of heterozygosity. Nature Biotechnology , 28(4), 4. https://doi.org/10.1038/nbt.1615
- Park, Y. J., Jeon, S.-H., Kim, H.-K., Suh, E. J., Choi, S. J., Kim, S., & Kim, H. O. (2020). Human induced pluripotent stem cell line banking for the production of rare blood type erythrocytes. Journal of Translational Medicine , 18(1), 236. https://doi.org/10.1186/s12967-020-02403-y
- Phelan, M. C. (2006). Techniques for mammalian cell tissue culture. Current Protocols in Protein Science , 46(1), A.3C.1–A.3C.18. https://doi.org/10.1002/0471140864.psa03cs46
- Poleganov, M. A., Eminli, S., Beissert, T., Herz, S., Moon, J.-I., Goldmann, J., Beyer, A., Heck, R., Burkhart, I., Barea Roldan, D., Türeci, Ö., Yi, K., Hamilton, B., & Sahin, U. (2015). Efficient reprogramming of human fibroblasts and blood-derived endothelial progenitor cells using nonmodified RNA for reprogramming and immune evasion. Human Gene Therapy , 26(11), 751–766. https://doi.org/10.1089/hum.2015.045
- Rao, M. S., & Malik, N. (2012). Assessing iPSC reprogramming methods for their suitability in translational medicine. Journal of Cellular Biochemistry , 113(10), 3061–3068. https://doi.org/10.1002/jcb.24183
- Rodin, S., Antonsson, L., Niaudet, C., Simonson, O. E., Salmela, E., Hansson, E. M., Domogatskaya, A., Xiao, Z., Damdimopoulou, P., Sheikhi, M., Inzunza, J., Nilsson, A.-S., Baker, D., Kuiper, R., Sun, Y., Blennow, E., Nordenskjöld, M., Grinnemo, K.-H., Kere, J., … Tryggvason, K. (2014). Clonal culturing of human embryonic stem cells on laminin-521/E-cadherin matrix in defined and xeno-free environment. Nature Communications , 5(1), 1. https://doi.org/10.1038/ncomms4195
- Rodin, S., Domogatskaya, A., Ström, S., Hansson, E. M., Chien, K. R., Inzunza, J., Hovatta, O., & Tryggvason, K. (2010). Long-term self-renewal of human pluripotent stem cells on human recombinant laminin-511. Nature Biotechnology , 28(6), 6. https://doi.org/10.1038/nbt.1620
- Schlaeger, T. M., Daheron, L., Brickler, T. R., Entwisle, S., Chan, K., Cianci, A., DeVine, A., Ettenger, A., Fitzgerald, K., Godfrey, M., Gupta, D., McPherson, J., Malwadkar, P., Gupta, M., Bell, B., Doi, A., Jung, N., Li, X., Lynes, M. S., … Daley, G. Q. (2015). A comparison of non-integrating reprogramming methods. Nature Biotechnology , 33(1), 58–63. https://doi.org/10.1038/nbt.3070
- Singh, G., Manian, K. V., Premkumar, C., Srivastava, A., Daniel, D., & Velayudhan, S. R. (2022). Derivation of clinical-grade induced pluripotent stem cell lines from erythroid progenitor cells in xenofree conditions. In A. Nagy & K. Turksen (Eds.), Induced Pluripotent Stem (iPS) Cells: Methods and Protocols (pp. 775–789). Springer US. https://doi.org/10.1007/7651_2021_349
- Skorik, C., Mullin, N. K., Shi, M., Zhang, Y., Hunter, P., Tang, Y., Hilton, B., & Schlaeger, T. M. (2020). Xeno-Free Reprogramming of peripheral blood mononuclear erythroblasts on Laminin-521. Current Protocols in Stem Cell Biology , 52(1), e103. https://doi.org/10.1002/cpsc.103
- Takahashi, K., Tanabe, K., Ohnuki, M., Narita, M., Ichisaka, T., Tomoda, K., & Yamanaka, S. (2007). Induction of pluripotent stem cells from adult human fibroblasts by defined factors. Cell , 131(5), 861–872. https://doi.org/10.1016/j.cell.2007.11.019
- Tosca, L., Feraud, O., Magniez, A., Bas, C., Griscelli, F., Bennaceur-Griscelli, A., & Tachdjian, G. (2015). Genomic instability of human embryonic stem cell lines using different passaging culture methods. Molecular Cytogenetics , 8(1), 30. https://doi.org/10.1186/s13039-015-0133-8
- Xu, C., Inokuma, M. S., Denham, J., Golds, K., Kundu, P., Gold, J. D., & Carpenter, M. K. (2001). Feeder-free growth of undifferentiated human embryonic stem cells. Nature Biotechnology , 19(10), 10. https://doi.org/10.1038/nbt1001-971
- Ye, H., & Wang, Q. (2018). Efficient generation of non-integration and feeder-free induced pluripotent stem cells from human peripheral blood cells by Sendai Virus. Cellular Physiology and Biochemistry , 50(4), 1318–1331. https://doi.org/10.1159/000494589