Multi-Attribute Method (MAM) Analytical Workflow for Biotherapeutic Protein Characterization from Process Development to QC
Silvia Millán-Martín, Silvia Millán-Martín, Craig Jakes, Craig Jakes, Sara Carillo, Sara Carillo, Jonathan Bones, Jonathan Bones
Abstract
The multi-attribute method (MAM) has emerged significantly in recent years to support biotherapeutic protein characterization from process development to the QC environment. MAM is a liquid chromatography mass spectrometry (LC-MS) based peptide mapping approach, which combines the benefits from liquid chromatography coupled to high resolution accurate mass mass spectrometry (LC-HRAM MS), enabling direct assessment of protein sequence and product quality attributes with site specificity. These product quality attributes may impact efficacy, safety, stability, and process robustness. MAM is intended to replace conventional analytical approaches as it offers a more streamlined strategy for parallel monitoring of multiple attributes in a single analysis with high sensitivity and confidence, and ultimately supports more robust Quality by Design (QbD) approaches and faster decision cycles for biotherapeutic development. MAM consists of three main stages. The first stage is sample digestion, which typically entails proteolytic digestion of the protein. The second stage is reversed-phase chromatographic separation of the generated peptides and detection by HRAM MS in two phases. During MAM Phase I (discovery phase), data-dependent acquisition (DDA) MS/MS is performed to enable confident identification of peaks and development of a peptide workbook. During MAM Phase II (monitoring phase), full MS acquisition is only carried out for the monitoring of predefined product quality attributes (PQAs). The third stage is data processing, which entails analysis and reporting for each of the two phases including evaluation of sequence coverage, assessment of PQAs and peptide workbook creation during phase I, and targeted monitoring of predefined product attributes and new peak detection (NPD) during phase II. The latter is a comparative analysis that uses a base peak alignment algorithm to determine any non-monitored differences between the LC-MS chromatograms of a test sample and a reference standard. © 2023 The Authors. Current Protocols published by Wiley Periodicals LLC.
Basic Protocol 1 : In-solution sample digestion
Alternate Protocol : Automated sample digestion
Basic Protocol 2 : Reversed-phase chromatographic separation and detection by HRAM-MS (RPLC-HRAM MS)
Basic Protocol 3 : Data processing and reporting
INTRODUCTION
Biotherapeutics are among the fastest-growing sectors of the pharmaceutical industry. Biotherapeutic products encompass a variety of biologic-based molecules produced by living cells, which are key therapies in the treatment of life-threating diseases, e.g., cancer, autoimmune diseases and infectious diseases (Au et al., 2021; Otsubo & Yasui, 2022; Shim, 2020; Yasunaga, 2020). Biotherapeutics include proteins and hormones, monoclonal antibodies, cytokines, cell and gene therapy products, vaccines, and stem cell therapies. During their development, manufacturing, and storage, they can suffer from structural modifications, known as posttranslational modifications (PTMs), which can ultimately alter their biological activity and safety (Das et al., 2020; Rocamora et al., 2023; Wen & Jawa, 2021). Thus, advancement in their production and their increased complexity force the need for cutting-edge analytical approaches to deliver high-quality products more efficiently. In this regard, high resolution accurate mass mass spectrometry (HRAM MS) has become an indispensable technique for biotherapeutic characterization, batch release, and stability testing (Rathore et al., 2018). The use of MS supports different aspects for the analysis of biotherapeutic products e.g., identification, characterization, comparative analytical assessment (to compare biosimilars vs reference product or to monitor the impact of process changes), surveillance for adulteration and contamination, process improvement, new product knowledge (new critical post-translational modifications or other structural attributes) and pharmacokinetic and pharmacodynamic (PK/PD) measurements (FDA, 2022). MS is a sensitive technique for studying the structure, quality, and stability of therapeutic proteins, such as antibodies during the drug discovery and development phases. The structure-function relationship has been attained by high-resolution native MS to uncover the unique features of complex glycans and to ascertain the correlation between the glycoprotein structure and their cellular functions (Struwe & Robinson, 2019). MS is also an effective tool to understand underlying disease mechanisms and identify putative drug targets, which can also accelerate development timelines and reduce costs (Terracciano et al., 2021). MS can be used to understand absorption, distribution, metabolism, and excretion (ADME) of drug candidates by simultaneously detecting the parent drug and its metabolites (Holm et al., 2022).
It is important to highlight that applying advanced MS methods offers the potential to accelerate product development by shortening process characterization timelines, as the sensitivity and specificity that MS offers is a key advantage in biopharmaceutical analysis. In this regard, the multi-attribute method (MAM) emerged as a new MS-based technology some years ago. MAM was originally developed by scientists at Amgen with the aim of replacing conventional analytical approaches based on chromatographic and electrophoretic techniques or immunoassays, which needed to be used orthogonally, may lack sensitivity or specificity, and are time consuming to perform (Rogers et al., 2015). Conventional methods include size exclusion chromatography (SEC) (Haberger et al., 2016), ion-exchange chromatography (IEX) (Fekete et al., 2015; Trappe et al., 2018), hydrophilic interaction chromatography (HILIC) (Melmer et al., 2010), capillary gel electrophoresis (CGE) (Szabo et al., 2022), imaging isoelectric focusing (iCIEF) (Suba et al., 2015) and enzyme-linked immunosorbent assays (ELISA) (Zhu-Shimoni et al., 2014). They are normally used as purity assays for lot release and stability testing. These methods are predominantly profile-based analyses based on their use of optical detection techniques and are often not capable of identifying and quantifying site-specific critical quality attributes (CQAs). Each conventional assay is designed to primarily monitor one type of CQA and, in general, has limited resolution and specificity.
MAM is an LC-MS-based peptide mapping approach that involves the enzymatic cleavage of the protein followed by the separation and identification of the generated peptide. Depending on the type of biotherapeutic protein characterized, the choice of proteases may change. While trypsin is the protease of choice for standard monoclonal antibodies (Jakes et al., 2021; Wang et al., 2016), more complex molecules e.g., fusion proteins, may require a combination of different enzymes (Puranik et al., 2023; Stavenhagen et al., 2019). In the case of emerging therapies, such as viral vector-based gene therapies, an alternative protease may be required (Guapo et al., 2022). Other frequently used proteases are chymotrypsin (Yang et al., 2010), Lys-C (Li et al., 2022; Xu et al., 2022), Asp-N or Glu-C (Buettner et al., 2020). In all cases, it is important to obtain a reproducible digest with low artificially induced modifications (Jakes et al., 2021).
The MAM approach consists of three main stages, graphically described in Figure 1. First is the sample preparation stage, which typically consists of enzymatic digestion of the protein after diluting the sample to the required concentration, reduction, and alkylation steps to unfold protein structure and facilitate protease action (Fig. 1, steps 1a to 1d). Second is reversed-phase chromatographic separation of the generated peptides and detection by HRAM MS in two phases (Fig. 1- step 2). MAM phase I, also known as the discovery phase, involves MS/MS acquisition for confident sequence coverage assessment and site-specific quality attribute evaluation. MAM phase II, also known as the monitoring phase, normally involves full MS-only acquisition, as it is more applicable in the QC environment due to operational simplicity and the fact that it is based on retention time and accurate mass information. The third and last stage of the MAM approach includes the data processing and reporting steps (Fig. 1, step 3). Data processing is also divided into 2 phases. During the discovery phase the data are interrogated against the specific product sequence to assess sequence coverage and most relevant PQAs/CQAs. It is also possible to evaluate digestion efficiency by assessing missed cleavages or nonspecific generated peptides, which are generated without following protease rules. Chromatographic signals and MS/MS fragmentation should be manually checked for confident peptide identity. A peptide workbook is created at this stage containing both retention time and high-resolution mass data. During phase II, targeted monitoring of predefined quality attributes and new peak detection (NPD) are performed; the first to obtain quantitative information for the targeted features and the second to investigate the presence of product and process-related impurities. The NPD function is a comparative analysis that uses a base peak alignment algorithm to determine any non-monitored differences between the LC-MS chromatogram of a test sample and that of a reference standard. Reporting of the obtained results is also included in this phase. It is important to highlight that in recent years, efforts have focused on the use of MAM implemented within a platform that allows interconnectivity among laboratories for instrument management, software integration, data storage, and seamless data and workflow transfer, which could be server or cloud based and ultimately will result in the preservation of data integrity, accelerated data processing, method transfer, and advanced reporting.
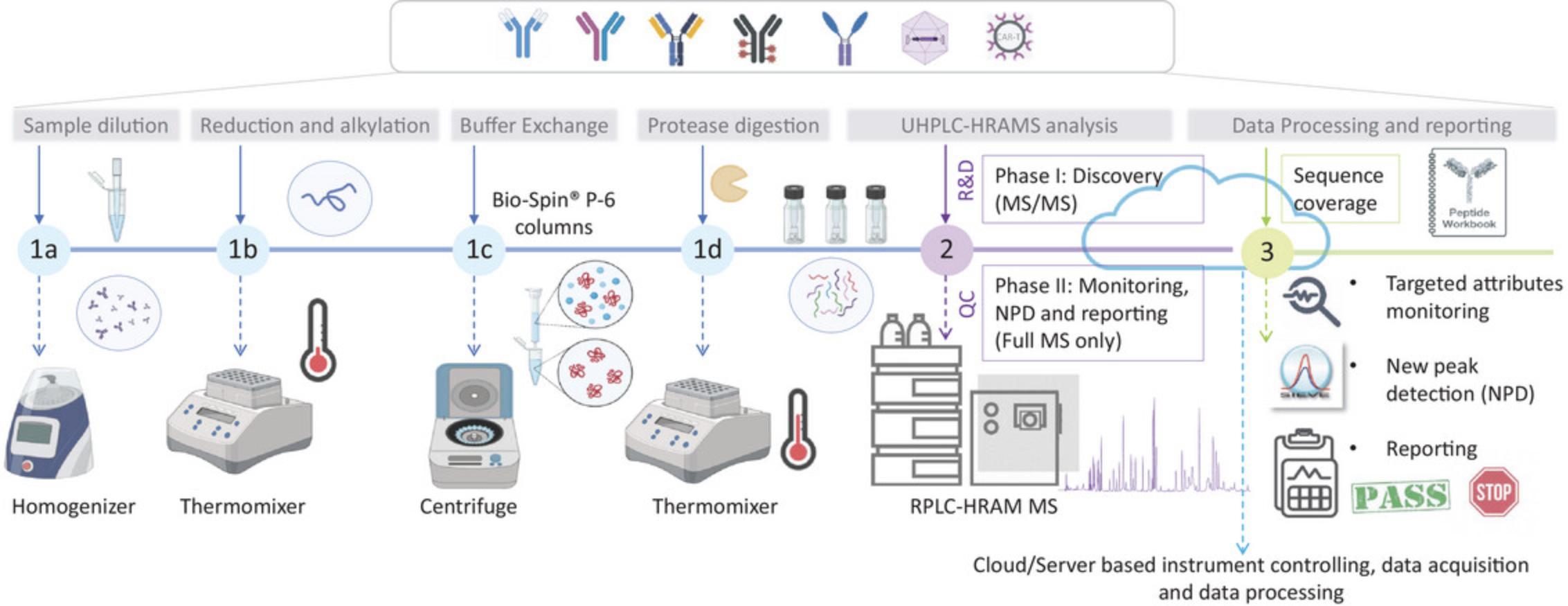
A summary of the different quality attributes that can be measured by the MAM approach compared to conventional methods are highlighted in Table 1. The number of individual tests for specific potential CQAs can be decreased considerably by using an appropriately developed MAM. MAM has the potential to increase usage of MS in quality control in a regulated environment (Rogers et al., 2018). MAM could be employed for diverse applications, e.g., product characterization, sequence variant analysis in bioprocess development (Niu et al., 2023), for tracking the behavior of quality attributes during cell culture experiments (Jakes et al., 2021), and to support comparative analytical assessment for biosimilars (Millan-Martin, Jakes, Carillo, & Bones, 2023). Currently, there are multiple analytical platforms and software available to implement the MAM approach from different vendors (Hao et al., 2021; Mouchahoir et al., 2021; Qian et al., 2021; Rogers, 2019). Over the last 8 years, the scientific community has been working on developing the different aspects of the approach with the aim of demonstrating the reproducibility, resolution, and robustness needed for protein characterization, from process development of upstream and downstream processes to quality control release under current good manufacturing practices (cGMP) as required from regulatory agencies. Numerous sample preparation strategies are available in the literature, with variations in experimental details. Some focus on in-solution manual digestion protocols while there is an increasing interest in automated approaches (Millan-Martin, Jakes, Carillo, Gallagher, et al., 2023). As the technique has been progressing over the years, MAM is now increasingly being used for routine PQA/CQA analysis of mAb molecules. This is being extended to other biotherapeutics modalities, such as bispecific or multispecific antibodies (BsAbs or msAbs) (Evans et al., 2021), Ab fragments (Song et al., 2021), growth factors, and fusion proteins (Guan et al., 2022). Nowadays, the focus is still on the implementation in QC labs and the use of cloud- or server-based platforms, which allow users to operate from any location while maintaining data integrity all the time.
Quality attribute | MAM | SEC | IEX | HILIC | rCE-SDS | nrCE-SDS | ELISA |
---|---|---|---|---|---|---|---|
Aggregates | |||||||
Oxidation | |||||||
Deamidation | |||||||
Succinimide | |||||||
Isomerization | |||||||
C-terminal variants | |||||||
N-terminal pyroglutamate | |||||||
Glycation | |||||||
Glycosylation | |||||||
Host cell proteins (HCPs) | |||||||
Fragments | |||||||
Mutations and misincorporations | |||||||
Disulfide isoforms |
- a Green shading indicates that the technique is suitable to the specific analysis of the selected attribute; gray shading indicates is not suitable and yellow shading indicates it is potentially suitable.
- b MAM, multi-attribute method; SEC, size exclusion chromatography; IEX, ion-exchange chromatography; HILIC, hydrophilic interaction chromatography; rCE-SDS, reduced capillary electrophoresis sodium dodecyl sulfate; nrCE-SDS, non-reduced capillary electrophoresis sodium dodecyl sulfate; ELISA, enzyme-linked immunosorbent assay.
This protocol outlines the basic steps required to prepare samples for MS analysis within the context of monoclonal antibodies, but it could be adapted to the specific sample of interest. Digestion conditions should be optimized in each case in terms of protein to enzyme ratio, digestion temperature, and digestion time, to ensure minimal sample-induced artifacts are generated and efficient and reproducible digestion is achieved. We describe a MAM protocol to monitor and quantify quality attributes on monoclonal antibodies and the use of the NPD tool as a purity test to support the comparative analytical assessment of biosimilars vs the reference product (RP).
This method is divided into three protocols. Basic Protocol 1 describes pre-analytical steps regarding sample preparation by in-solution enzymatic digestion. As an alternative, an optimized workflow using automated trypsin digestion is also described in the Alternate Protocol as developed by Millán-Martín et al. (2020). The benefits of automated immobilized enzyme digestion are increased workflow efficiency and simplicity, and reduced sample handling and time needed for sample preparation. The method of choice will depend on the available resources and the preference of the user. Basic Protocol 2 describes the chromatographic separation of the generated peptides using reversed-phase liquid chromatography (RPLC) and HRAM MS detection on an Orbitrap based mass spectrometer, e.g., the Orbitrap Exploris 240 or Orbitrap Exploris MX (Thermo Fisher Scientific). Basic Protocol 3 describes data processing and reporting of the results in an electronic report format using a server-based system that allows for data integrity and seamless data analysis and reporting.
CAUTION : Safety glasses and reagent-impermeable protective gloves should be worn for sample preparation and manipulation of the analytical platforms.
Basic Protocol 1: IN-SOLUTION SAMPLE DIGESTION
Sample preparation is one of the most critical steps of the MAM approach. The development of a robust and reproducible sample preparation protocol with minimal sample preparation-induced artificial protein modifications is key to design a robust and reliable MAM workflow. Manual sample preparation has been extensively used, although to date there is no consensus on the protocol and different reagents and buffers that are used in this regard. Conventionally, it requires the researcher to denature, reduce, and alkylate the sample followed by a desalting step or buffer exchange into the digestion buffer prior to enzymatic digestion. Overall, trypsin is the most used protease for peptide mapping on mAbs. However, certain more complex mAbs or other biotherapeutic modalities might require other enzymes to achieve full sequence coverage and a reliable PTMs assessment.
Materials
-
Infliximab RP, commercial chimeric IgG1 monoclonal antibody drug product (FDA UNII registry: 4F4 × 42SYQ6)
-
Infliximab biosimilars, commercial chimeric IgG1 biosimilars Flixabi, Inflectra and Zessly (Evidentic GmbH; Flixabi batch no. EC1216, Inflectra batch no. GFS988, Zessly batch no. SUR22)
-
NIST humanized IgG1κ monoclonal antibody, lot 14HB-D-002 (NIST, RM 8671)
-
Guanidine-Tris buffer, pH 8.3 (see recipe)
-
500 mM DTT (see recipe)
-
500 mM iodoacetic acid (IAC) (see recipe)
-
50 mM DTT (see recipe)
-
50 mM Tris, pH 7.9 (see recipe)
-
H2O, LC-MS grade (Thermo Scientific, cat. N0. W8-1)
-
Trypsin protease, MS grade, 100 µg per vial (Thermo Scientific, cat. no. 90058)
-
10% (v/v) formic acid (see recipe)
-
0.5-ml protein LoBind microcentrifuge tubes (Eppendorf, cat. no. 022431064)
-
1000-, 200-, and 20-µl graduated micropipettes (Sarstedt, cat. nos. 70.3050.200, 70.760.502, and 70.1114.200, respectively)
-
Bio-Spin P-6 gel columns, Tris buffer (Bio-Rad, cat. no. 732-6227)
-
2-ml microcentrifuge tube (Eppendorf, cat. no. 022431102)
-
Benchtop refrigerated centrifuge (Eppendorf, cat. no. Z605212) with a fixed-angle rotor (Eppendorf, cat. no. Z666408)
-
1.5-ml microcentrifuge tubes (Eppendorf, cat. no. 022431081)
-
Vortex mixer (Merck, cat. no. CLS6776)
-
Eppendorf ThermoMixer (Eppendorf, cat. no. 5382)
-
pH meter (Thermo Scientific, cat. no. 11651439)
-
Low-binding chromatography glass vials (Fisher Scientific, cat. no. 2-SVWGKST-CPK)
-
Low-binding chromatography glass inserts (Fisher Scientific, cat. no. C4010-630)
CAUTION : DTT causes skin and eye irritation and may cause respiratory irritation. IAC may cause an allergic skin reaction. Formic acid is a flammable liquid and vapor. Wear personal protective equipment when handling.
1.Adjust the sample (infliximab RP, biosimilars or NISTmAb) concentration to 1 mg/ml by diluting with guanidine-Tris buffer. Place 10 µl of sample (10 mg/ml) into a polypropylene 0.5 ml microcentrifuge tube and add 90 µl of guanidine-Tris buffer.
2.Add 2.0 µl of 500 mM DTT and mix by pipetting, yielding a final concentration of ∼10 mM DTT. Incubate the reduction reaction for 30 min at room temperature.
3.Add 4.0 µl of 500 mM IAC and mix by pipetting to give a final concentration of 20 mM IAC. Store the solution in the absence of light for 20 min at room temperature. Arrest alkylation by adding a further 4.0 µl of 50 mM DTT, resulting in a total volume of 110 µl (11 mM DTT).
4.Perform buffer exchange by using Bio-Spin P-6 gel column(s) as follows:
-
Break off the tip and place the column in a 2-ml Eppendorf microcentrifuge tube.
-
After removing the cap, centrifuge the column for 2 min at 1000 ×g, 4°C.
-
Dispose of the liquid collected in the Eppendorf tube and carefully add 500 µl of 50 mM Tris at pH 7.9 to the top of the BioSpin-6 column.
-
Repeat centrifugation for 2 min at 1000 ×g, 4°C. Discard the collected liquid.
-
Repeat this centrifugation step three more times (four times in total) to completely buffer-exchange the BioSpin-6 column.
Before sample preparation, invert the BioSpin-6 columns vigorously several times and allow them to equilibrate at room temperature for 30 min. To save time, prepare the buffer cartridges during the 20 min resting period of the sample during the alkylation (step 3).
5.Place the spin column in a new 1.5-ml microcentrifuge tube and carefully add 110 µl of the alkylated sample to the center of the column bed. Centrifuge the column for 4 min at 1000 × g , 4°C, and collect the eluate.
6.Perform trypsin digestion as follows:
-
Add 100 µl of LC-MS-grade water to a vial of 100 µg of trypsin to give a final concentration of 1 mg/ml.
-
Mix the solution by gentle vortexing.
-
Add this solution at a 1:10 ratio to the protein sample above (e.g., 10 µl of 1 mg/ml trypsin to 100 µl of sample solution).
-
Place the resulting solution in an Eppendorf ThermoMixer and heat at 37°C for 30 min.
-
Stop digestion by adding 10% formic acid (1:10 ratio in volume with the digestion solution).
-
Verify that the pH is acidic (pH ∼1.5) using a pH meter.
-
Transfer the final sample solution to low-binding chromatography glass vials with inserts.
7.Place the sample vials in the sample rack held at 5°C for analysis.
Alternate Protocol: AUTOMATED SAMPLE DIGESTION
As an alternative, an optimized workflow using automated trypsin digestion is also described in this protocol as developed by Millán-Martín et al. (2020). Automation of sample preparation using trypsin magnetic beads allows for high-throughput and improves robustness and consistency. The benefits of automated immobilized enzyme digestion are increased workflow efficiency and simplicity, and reduced sample handling and time needed for sample preparation. Briefly, by using this approach, the protein is unfolded by using heat denaturation; the digestion is performed with a heat-resistant trypsin on a magnetic robotic platform for 30 min at 70°C with the use of MES buffer at pH 6.5.Subsequently, Tris(2-carboxyethyl) phosphine hydrochloride (TCEP), a reducing agent that is active at reduced pH, is added and significantly improves the digestion efficiency, removing the need for an additional reduction step. The addition of TCEP as a reducing agent without the need for detergents and any further alkylation step also simplifies sample preparation and allows for full, or almost full, sequence coverage.
Additional Materials (also see Basic Protocol 1)
-
SMART Digest trypsin kit, magnetic bulk resin option, low pH (Thermo Scientific, cat. no. 60109-101-MB-LPH)
-
H2O, Milli-Q
-
TCEP Bond-Breaker solution neutral pH (Thermo Scientific, cat. no. 77720)
-
10% (v/v) trifluoroacetic acid (TFA) (see recipe)
-
96-well plate, KF deep-well (Thermo Scientific, cat. no. 95040450)
-
12-tip comb, KF Duo (Thermo Scientific, cat. no. 97003500)
-
Purification system, KingFisher (KF) Duo Prime (Thermo Scientific, cat. no. 5400110)
-
BindIt software version 4.0 (Thermo Scientific)
CAUTION : TCEP and TFA cause severe skin burns and eye damage. Wear personal protective equipment when handling.
1.Dilute sample (infliximab RP and biosimilars) by adding 150 µl of SMART Digest buffer to the 50 µl sample (previously diluted if needed, to contain 100 µg of protein). Mix the solution by gentle vortexing and add to row A of a 96-well plate.
2.Prepare wash buffer by adding 50 µl of SMART digest buffer to 150 µl of Milli-Q water in a 1.5-ml microcentrifuge tube. Mix the solution by vortexing and add to row E of the 96-well plate.
3.Prepare magnetic bulk trypsin resin by adding 15 µl of magnetic trypsin beads to 100 µl of SMART digest buffer. Mix the bead solution first by aspiration and then by gentle vortexing. Add the mixed bead solution to row D of the 96-well plate.
4.Place the 12-tip comb in row B of the 96-well plate and add this plate to the KF Duo Prime purification system.
5.In each well containing sample in row A, add 2 µl of TCEP to the 198 µl of solution to give a final concentration of 5 mM TCEP.
6.Begin the digestion by pressing start on the software.
7.Quench after digestion by removing the 96-well deep-well plate from the KF Duo Prime purification system. Add 1 µl of 10% TFA to row A for a final concentration of 0.1% (v/v) TFA. Transfer the final sample solution in row A to a low-binding chromatography glass vial with inserts.
8.Place the sample vials in the sample rack kept at 5°C.
Basic Protocol 2: REVERSED-PHASE CHROMATOGRAPHIC SEPARATION AND DETECTION BY HRAM MS (RPLC-HRAM MS)
After digestion, samples are transferred to chromatography low-binding vials for subsequent RPLC-MS analysis. In this protocol, we describe the RPLC-HRAM MS MAM workflow developed on a Vanquish Flex binary UHPLC system (Thermo Scientific) coupled to an Orbitrap Exploris 240 mass spectrometer (Thermo Scientific) for the MAM discovery phase thus including MS/MS acquisition, or Orbitrap Exploris MX mass detector (Thermo Scientific) with full MS only acquisition for the MAM monitoring phase. Before the analysis of the digested peptides, the LC-MS system was evaluated for optimum performance by using a system performance evaluation test (SET), which consisted of a commercially available bovine serum albumin (BSA) tryptic digest standard. This step is designed to be run during installation and troubleshooting following instrument vendor recommendations. Additionally, sample preparation quality and LC-MS system performance must also be monitored throughout the experiment. To address this, a system check known as system suitability test (SST) is included within each sequence of analysis. NIST monoclonal antibody reference material is used in the present work, but it is not limited to that, and any other well-characterized molecule within each laboratory could also be used.
It is important to highlight that other instrumentation can be used from other vendors; however, the performance of the method outlined can be guaranteed only on an equivalent instrument platform.
Materials
-
FlexMix calibration solution (Thermo Scientific, cat. no. A39239)
-
Formic acid, Optima LC-MS grade (Sigma-Aldrich, cat. no. T-26029)
-
H2O, LC-MS grade (Thermo Scientific, cat. no. W8-1)
-
Acetonitrile, LC-MS grade (Thermo Scientific, cat. no. A956-1)
-
BSA tryptic digest standard (Thermo Scientific, cat. no. 88341)
-
Vanquish Flex binary UHPLC system (Thermo Scientific, cat. no. IQLAAAGABHFAPUMBJC)
-
Orbitrap Exploris 240 mass spectrometer (Thermo Scientific, cat. no. BRE725535)
-
Orbitrap Exploris MX mass detector (Thermo Scientific, cat. no. BRE725536)
-
Ion Max source equipped with the HESI-II-probe (Thermo Scientific, cat. no. IQLAAEGABBFACNMAGY)
-
Hypersil GOLD Vanquish C18 column, 1.9-µm, 2.1 mm × 150 mm (Thermo Scientific, cat. no. 25002-152130-V)
1.Calibrate the Orbitrap Exploris 240 mass spectrometer by using the FlexMix calibration solution and following the manufacturer's instructions.
2.Equilibrate the column as follows:
-
Add solvent lines to their corresponding solvent bottles and flush the system at 50% A (0.1% (v/v) formic acid in water) and 50% B (0.1% (v/v) formic acid in acetonitrile) by opening the purge valve.
-
Close the purge valve, connect the column, and bring it slowly to the operational flow rate in 0.050 ml min−1intervals.
-
Continue until the column pressure is stabilized (within a fluctuation of ±1 bar), which takes ∼30 min.
3.Run a system performance evaluation test (SET) as follows:
-
Add 1 ml of LC-MS grade water to a vial of BSA tryptic digest.
-
Prepare 200 µl aliquots (2 pmol/µl) and freeze them at −20°C.
-
Before running a SET, remove a vial from the freezer and thaw it in the autosampler at 5°C.
-
Run a sequence containing five 5 µl BSA injections and investigate the results by using a report template previously created by the user.
The SET is designed to be run during installation and troubleshooting, but not as a sample prior to each sample analysis.
4.Create an injection sequence for RPLC-HRAM MS analysis of peptides:
- In the Chromeleon console, click ‘Create’ → ‘Sequence.’ The sequence wizard opens. Follow the on-screen instructions to complete each page of the sequence wizard and click ‘Finish.’
The sequence should include replicates of the reference sample, blanks, a reference molecule for system suitability monitoring along the sequence and the sample set under investigation (e.g., drug product, stress study, newly produced batch, and biosimilar comparison).
-
Each injection is defined by a group of variables that characterize it. Provide the injection name and type, position in the autosampler, injection volume and instrument method, as the minimum required.
-
Select the location for the new sequence, enter a name and click ‘Save.’
Reversed phase HRAM MS analysis of peptides
5.For the discovery phase, perform RPLC-HRAM MS analysis by using a full MS/DDA method with the use of settings listed in Table 2.The sequence should include replicates of the reference sample to be characterized, blanks and a well-established standard to be analyzed for system suitability purpose.
Mobile phase gradient for UHPLC separation of peptides | |||
---|---|---|---|
Time (min) | Flow (ml min-1) | % mobile phase B | Curve |
0.0 | 0.25 | 1.0 | 5 |
5.0 | 0.25 | 1.0 | 5 |
6.0 | 0.25 | 10.0 | 5 |
70.0 | 0.25 | 35.0 | 5 |
72.0 | 0.25 | 90.0 | 5 |
77.0 | 0.25 | 90.0 | 5 |
79.0 | 0.25 | 1.0 | 5 |
81.0 | 0.25 | 1.0 | 5 |
83.5 | 0.25 | 10.0 | 5 |
91.5 | 0.25 | 45.0 | 5 |
93.0 | 0.25 | 90.0 | 5 |
99.0 | 0.25 | 90.0 | 5 |
101.0 | 0.25 | 1.0 | 5 |
115.0 | 0.25 | 1.0 | 5 |
MS source and analyzer conditions | |||
MS source parameters | Settings | ||
Source | Ion Max source with HESI II probe | ||
Sheath gas pressure | 30 au | ||
Auxiliary gas flow | 10 au | ||
Sweep gas | 1 au | ||
Ion transfer tube temp | 225°C | ||
Vaporizer temp | 200°C | ||
Source voltage | 3.5 kV | ||
MS method parameters | |||
General | Setting | MS2 parameters | Setting |
Runtime | 0-115 min | Data dependent scans | 5 |
Polarity | Positive | MIPS | Peptide |
Full MS parameters | Setting | Intensity threshold | 5.0E3 |
Full MS mass range | 200-2000 m/z | Charge state | 2-7 |
Resolution settings | 120,000 (at 200 m/z) | Dynamic exclusion | After 1 time 7.0 s Mass tolerance 10 ppm Exclude isotopes |
RF lens | 70% | ddMS2 | Isolation window 1.2 m/z Normalized collision energy Resolution 15,000 |
Normalized AGC target | 300% | ||
Max injection time | 100 ms | ||
Microscans | 1 | ||
MS acquisition | 0-70 min |
- a
On a C18, 1.9-µm, 2.1 mm × 150 mm column; MS source and analyzer conditions for site-specific peptide mapping analysis; au, arbitrary units.
6.For the monitoring phase, RPLC-HRAM MS analysis must be performed by using a full MS-only method. The sequence should include replicates of the reference sample, blanks, a reference molecule for system suitability monitoring along the sequence and the sample set under investigation (e.g., stress study, newly produced batch, and biosimilar comparison).
The SST should contain a general system check and a special system check; the first evaluates retention time and peak area precision, peak width and peak height and mass accuracy, while the second focuses on monitoring some PTM levels to ensure consistency across all the analyses within or between days, operators, etc. NPD analysis is also performed to ensure no contaminants are present in the system rather than the sample. Table 3 summarizes the SST-monitored peptides, evaluated parameters and acceptance criteria defined for the NIST monoclonal antibody tryptic digestion used. An example of SST extracted ion chromatogram plot showing the location of the NISTmAb reference peptides is shown in Figure 2.
Figure 3 shows the total ion chromatogram (TIC) and selected ion chromatograms (SICs) of DTLMISR peptide from the heavy chain in the unmodified and oxidized forms respectively for infliximab RP after RPLC-HRAMS MS analysis using the settings described in Table 2. As it can be observed, oxidation impacts the retention time behavior of the peptides by reducing the retention time based on reversed-phase chromatography. Confident identification is possible also based on MS/MS data analysis searched using the originator sequence, with parameters set to allow for amino acid substitutions.
Evaluated parameters | Acceptance criteria |
---|---|
List of peptides for general check | EEQYN[A2G0F]STYR; EEQYN[A2G1F]STYR; HYNPSLK; ALPAPIEK; LLIYDTSK; NQVSLTCLVK; TTPPVLDSDG; SFFLYSK; VVSVLTVLHQDWLNGK ALEWLADIWWDDKK |
RT % CV | ≤2% |
Peak area % CV | ≤10% |
Peak width at 10% height | ≤10 s |
Peak width at 10% height % CV | ≤10% |
Peak height (min, max) |
Min ≥1.0 × 107 counts Max ≤7.0 × 109 counts |
Composite scoring | Confirmed |
Mass accuracy | ≤5 ppm (absolute) |
List of peptides for special system check | DTLM[oxidation]ISR; SLSLSPG[Lys]; EEQYN[A2G0F]STYR; EEQYN[A2G1F]STYR |
% Oxidation | ≤3% |
% Oxidation % CV | ≤10% |
% C-term Lys | Between 13%-18% |
% C-term Lys % CV | ≤10% |
% EEQYN[A2G0F]STYR % EEQYN[A2G1F]STYR |
Between 35%-45% Between 35%-45% |
% EEQYN[A2G0F]STYR % CV % EEQYN[A2G1F]STYR % CV |
≤10% ≤10% |
- a
Defined for NISTmAb tryptic digest peptide mapping on orbitrap-based LC-MS system.
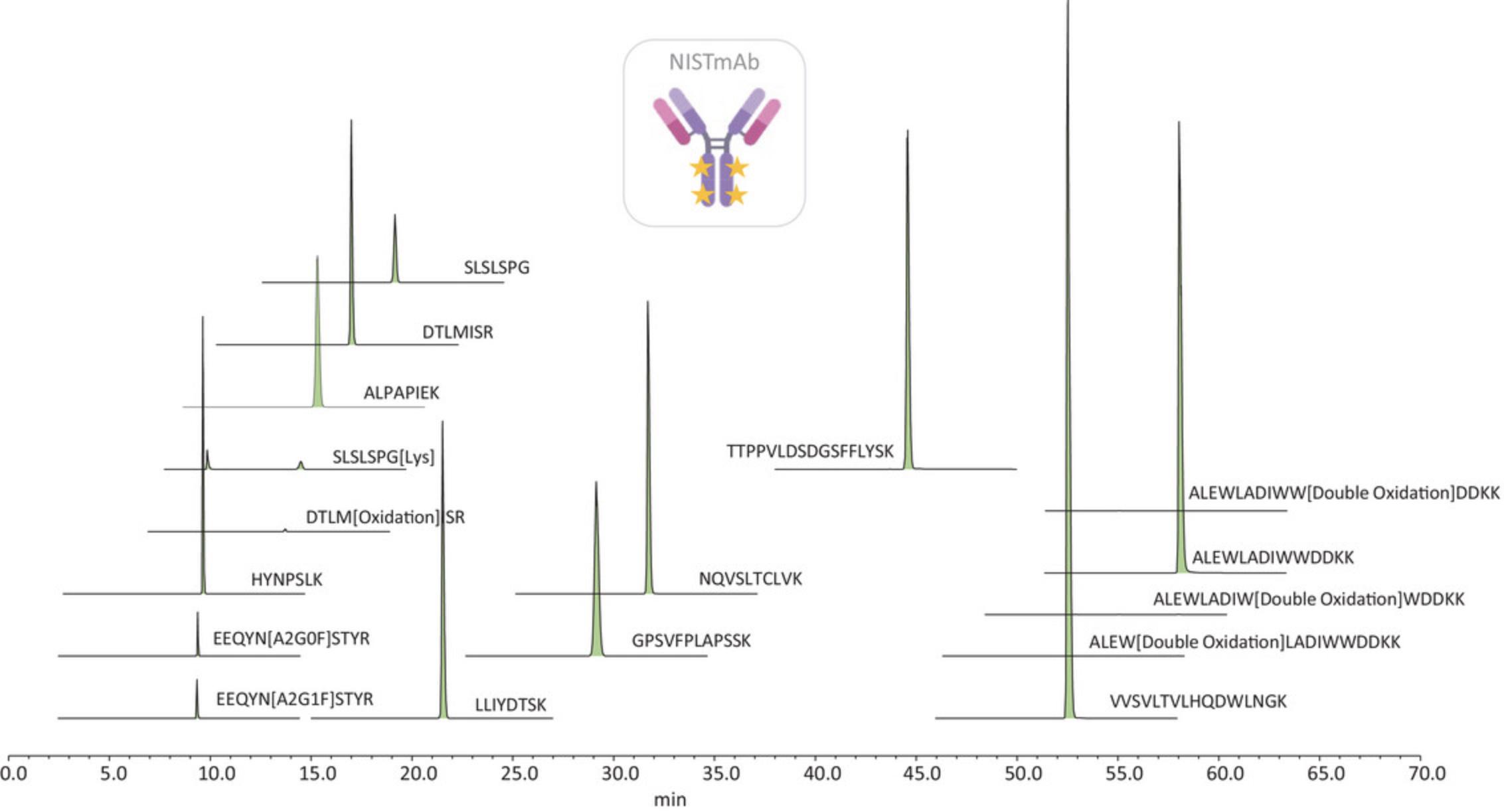
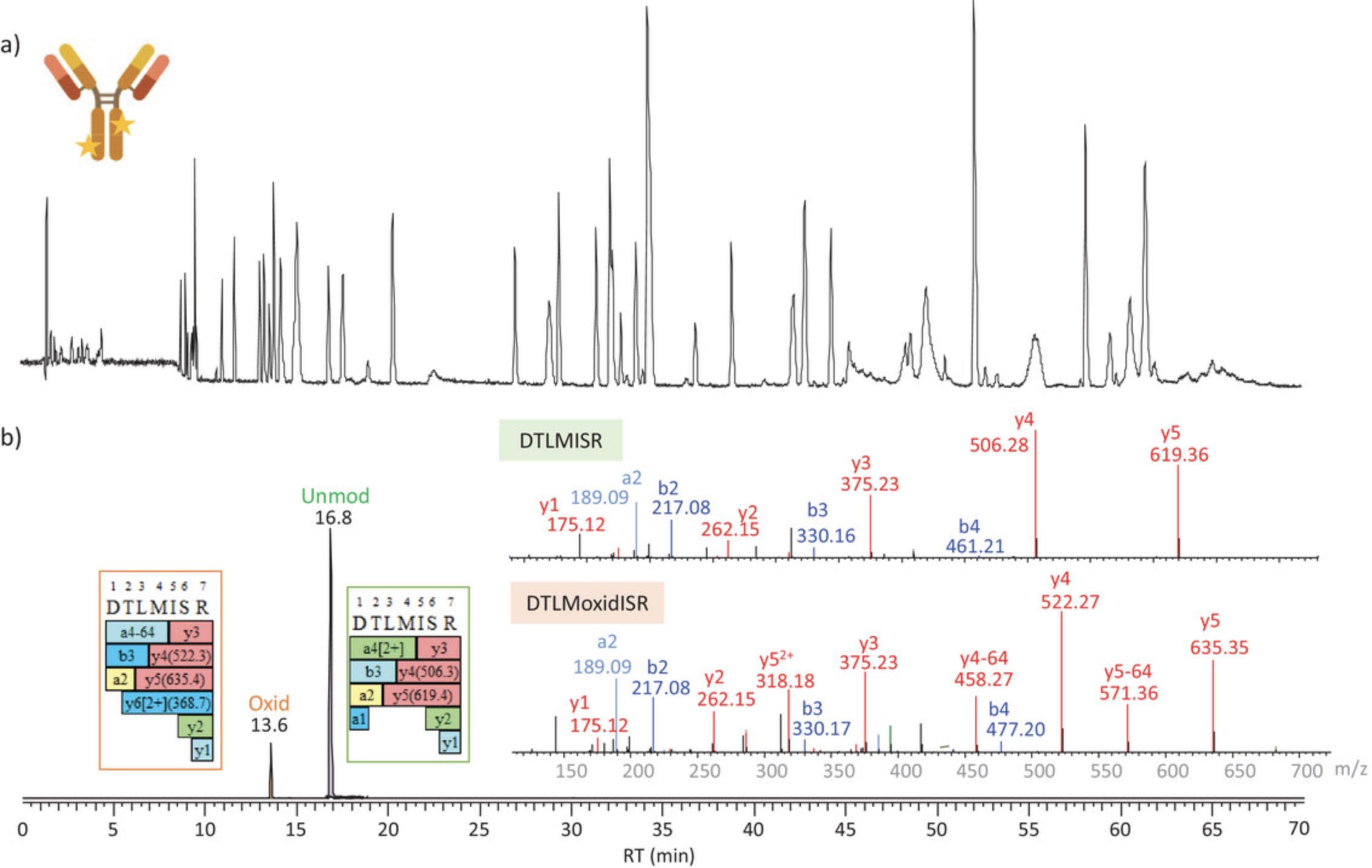
Basic Protocol 3: DATA PROCESSING AND REPORTING
The final stage of the MAM approach is the data processing stage, which is also divided into two phases. During Phase I of MAM, evaluation of protein sequence coverage, assessment of most relevant quality attributes and peptide workbook creation are performed. During Phase II of MAM, targeted monitoring of predefined product attributes and non-targeted MS (NTMS) processing or new peak detection (NPD) are attained, to investigate the presence of product- and process-related impurities or any other difference with noticeable inter-sample difference (e.g., potential sequence variants or differences in any quality attribute). The NPD function is a comparative analysis that uses a base peak alignment algorithm to determine any non-monitored differences between the LC-MS chromatogram of a test sample and that of a reference standard. The reporting of the obtained results is also included in this phase. Default report templates are included within the data processing software, which would be implemented by the user by means of including tables with the analytical conditions, data processing parameters, table results and plots of interests. Once the report is custom designed and created by the user this can be saved and used for future analysis.
In this protocol, server-based software is used to perform data processing and reporting. The server integrates all the instrument platforms available in the lab, together with all the software platforms used for data acquisition and data processing. The server-based software includes features, e.g., seamless workflow between various applications at the platform level, standardized accessibility to algorithms, data and results, and customizable reporting engines and dashboards.
Supplementary Data 1 (see Supporting Information) provides screenshots with a walk-through of all the steps described in this section.
Materials
- Thermo Scientific Chromeleon CDS software version 7.3.1 or equivalent
- Thermo Scientific BioPharma Finder software version 5.1 or equivalent
1.Connect to the server to access the MS/MS raw data from Chromeleon CDS.
2.Open the ‘Application Registration and Management’ to generate a registration code and copy it.
Discovery phase: quality attributes characterization
3.Open BioPharma Finder software by double clicking the BioPharma Finder icon to open the home page window. On the top right corner, click ‘Help,’ select ‘server-based software management’ followed by ‘Registration and connection’ and paste the code obtained in step 2.
4.Introduce the protein sequence and all the information needed for the analysis by selecting the ‘Sequence Manager’ option. Fixed and/or variable modifications, type of protein glycosylation and maximum number of allowed modifications per peptide must be defined.
5.Run the peptide mapping experiment as follows:
-
Select the ‘Peptide Mapping Analysis’ option from the BioPharma Finder home page window to create a new peptide mapping experiment.
-
Enter the experiment name (1).
-
Load the *.raw data file(s) located server data vault (2).
-
Select the protein sequence of interest (3).
-
Select a processing method to start processing (4).
-
A defined condition must be specified for each of the raw files selected and one of them should be selected as the reference.
The BioPharma Finder application contains a default method for basic peptide mapping analysis, which can be edited and adapted to the target protein. ‘Multiconsensus’ is selected as the result format for multiple files. Processing parameters used for peptide identification and PTM assessment are summarized in Table4.
All the default methods contained within the BioPharma Finder software cannot be overwritten, so any modified parameter will require saving the method under a new name.
Component detection | Settings |
---|---|
Absolute MS signal threshold | 2.00E04 |
Typical chromatographic peak width (min) | 0.3 |
Maximum chromatographic peak width (min) | 1.0 |
Relative MS signal threshold (% of base peak) | 1.0 |
Relative analog threshold (% of highest peak) | 1.0 |
Width of Gaussian filter (represented as 1/n of chromatographic peak width) | 3.0 |
Minimum valley to be considered as two chromatographic peaks | 80.0 |
Minimum MS peak width (Da) | 1.20 |
Maximum MS peak width (Da) | 4.20 |
Mass tolerance (ppm for high resolution or Da for low resolution) | 4.0 |
Maximum RT shift (min) | 0.5 |
Maximum mass (Da) | 30,000 |
Mass centroiding cutoff (percentage from base) | 15.0 |
Identification | Settings |
Maximum peptide mass (Da) | 7000 |
Mass accuracy (ppm) | 5.0 |
Minimum confidence | 0.80 |
Maximum number of modifications for a peptide | 1 |
Unspecified modification (Da) | −58 to +162 |
N-glycosylation | CHOa |
Protease | Trypsin |
Protease specificity | High |
- a
CHO, Chinese hamster ovary.
6.View the results for peptide mapping analysis: sequence coverage.
- After the application completes the analysis of an experiment, you can open the results by selecting the experiment and clicking ‘Load Results’ from the top pane within ‘BPF results’ folder.
The ‘Process and Review’ page opens and displays the different types of chromatograms (BPC-Base peak chromatogram, SIC-Single ion chromatogram or TIC-Total ion chromatogram), trend plots, protein sequence, full and MS/MS spectra, the results table, and the real-time optimization pane. The latter allows users to change any of the method or sequence parameters and reprocess the data in real-time again.
-
Filter the results table as summarized in Table5to exclude peptides containing sodium or potassium adducts, as well as those that correspond to nonspecific protease activity, gas phase generated ions or unspecified modifications, and to consider peptides with up to only one missed cleavage. Custom defined filters can be saved for future use as *.cfg file format to be applied to another data set.
-
The resulting component list must be selected to include all the filtered peptides in the sequence coverage map. To view the coverage map, select the ‘Mapping’ tab. By default, the application opens the ‘Coverage’ page, which displays the color-coded chromatogram, the sequence coverage map, and the results.
An example of a sequence coverage map of infliximab RP obtained using in-solution tryptic digestion is shown in Figure4. Full or almost full sequence coverage is attained for light chain (LC) and heavy chain (HC) respectively. The only missing peptide for the LC is EAK, and it is not shown as it can only be detected by full MS and the resulting confidence score is equal to zero, thus it is excluded as does not follow the applied filter rules stated in Table5.
-
Peptide mapping results can be saved in the centralized storage in a report format or downloaded as a pdf or excel file.
Field | Operator | Operand | Comment |
---|---|---|---|
Modification | Does not contain | + | To exclude adducts |
Does not contain | Nonspecific | To exclude nonspecific generated peptides (those that do not follow protease rules) | |
Does not contain | Gas phase | To exclude gas phase ions | |
Does not contain | . | To exclude unknown modifications | |
Delta (ppm) | ≥ | −5 | To include all the peptides within ± 5 ppm accuracy |
≤ | 5 | ||
Confidence score | ≥ | 95 | Experimental MS2 spectrum match with the theoretical spectrum |
Missed cleavages | ≤ | 1 | To include peptides with up 1 missed cleavage |
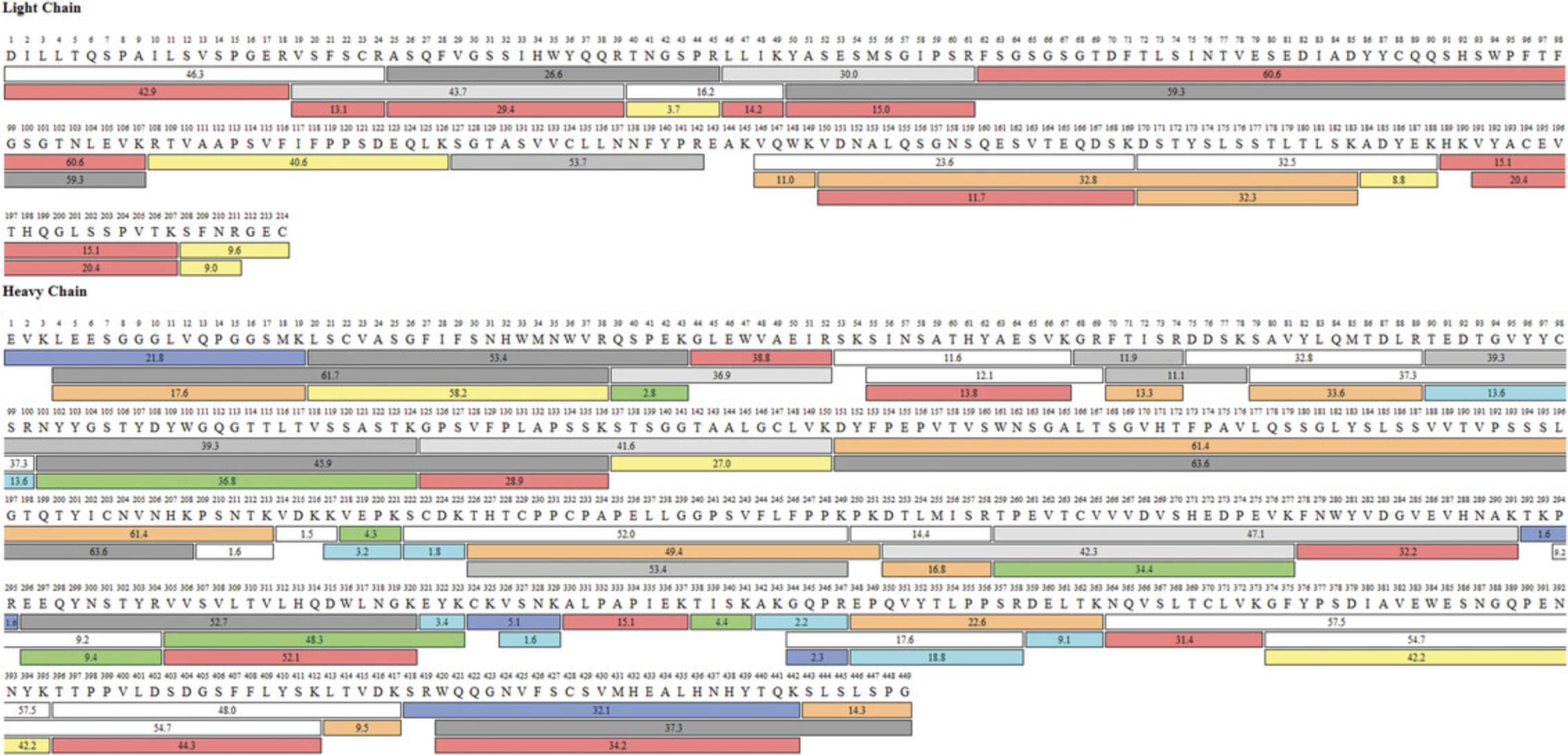
7.Create target peptide workbook:
- a.
Navigate to the ‘Modification Summary’ within the ‘Mapping’ tab.
For each modification, the selection of the component included for the calculation of modification percentage abundance is semi-automated, because components are also filtered following the rules specified in Table 5.For IgG1 type monoclonal antibodies, when evaluating N-glycans, the peptide TKPREEQYNSTYR, although containing two missed cleavages, was also included in the component list because of its significant abundance and its harboring of the Fc N-glycans.
- b.
Once the components to compute the relative quantitation are selected, right-click anywhere in the components table and choose ‘save as peptide workbook’ → checked.
- c.
Save the peptide workbook within the specific folder in the server-based repository. Peptide workbook can be edited any time when needed and it can be overwritten or appended to an existing one.
8.Choose the PQAs/CQAs that are relevant for your protein. The PQAs/CQAs chosen for relative quantitation in this study are glycosylation, deamidation, isomerization, succinimide formation, oxidation, C-terminal lysine content and N-terminal pyroglutamate formation with relative abundances ≥ 0.1%.
9.Export the target peptide workbook. To export the target peptide workbook, click ‘Export’ at the top-right corner above the list of target peptide workbooks. The ‘Save as’ type is set to BioPharma Workbook (.wbpf).
Monitoring phase: targeted quality attributes relative quantitation
10.Open Chromeleon CDS by double clicking the Chromeleon icon to open the home page window:
- a.
Create a new processing method within the Chromeleon console, by clicking ‘Create’ → ‘Processing method’ from the top panel.
- b.
When creating a processing method, you can choose from several predefined method templates.
For this study, the MS quantitative processing method layout template is used and edited accordingly. Method parameters used for the relative PQA/CQA quantitation are summarized in Table 6.
- c.
Once the new method is created and renamed, import the target peptide workbook generated during the previous step into the MS Component Table of the processing method editor by clicking ‘Import’ and selecting the workbook path.
- d.
The imported peptide data contains the list of components, RT, charge state, precursor mass and four isotopes used for MS quantitation and confirmation.
You must create a processing method before importing the peptide workbook in the Chromeleon CDS.
MS detection | Setting |
---|---|
Extracted ion chromatogram | MS default detection settings |
Detection algorithm | ICIS |
Area noise factor | 5 |
Peak noise factor | 10 |
Baseline window | 80 |
Noise method | INCOS |
Min peak width | 3 |
Multiple resolution | 10 |
Area tail extension | 5 |
Area scan window | 0 |
MS component table | Setting |
Table S1 (see Supporting Information) | .wbpf workbook from BPF |
MS settings | Settings |
MS spectra bunching | — |
Peak spectrum bunch | Width 1 (spectra) |
Baseline correction | No |
MS noise reduction | Do not reduce noise |
MS chromatogram settings | — |
Mass precision | 4 decimal places |
Mass tolerance (manually defined) | 5.0 ppm |
Peptide table | Settings |
Imported from BPF software 5.1 peptide workbook | — |
Composite scoring | Settings |
Pass score if at least | 2 criteria passed |
Fail score if less than | 1 criterion passed |
MS criteria | General MS |
Isotopic dot product | ≥ 0.9000 |
Mass accuracy | ≤5.00 ppm |
Peak apex alignment | ≤0.50 min |
11.View detected components from the MS data and adjust processing parameters to achieve consistent peak detection and integration:
-
In the Chromeleon console, click ‘Studio,’ then click ‘Data Processing’ and select ‘Processing Method,’ ‘MS Components’ and ‘Mass Spectra’ in the top pane group. The MS components pane appears next to the mass spectra at the top, while processing method parameters are shown at the bottom by default.
-
In the ‘MS component table’ tab within the processing method table, select each of the components and double-click to adjust retention properties. Unmodified peptides are selected as a reference component, while for modified peptides, a time distance and a proper component match algorithm are selected.
-
Window width must also be adjusted accordingly so that any peak that must be integrated is within the selected RT window.
It may be necessary to adjust the MS detection settings of the processing method, and this will depend on the chromatography and the peak shape of the detected peptides. Some peaks are very sharp, whereas others, normally the ones eluting later in the chromatogram, are broader; thus, increasing the baseline window in the algorithm setting sometimes helps achieve a better integration of the broader peaks. In the present study, a value of 80 was used (50 is the default value, but this could be within 1 and 500).
12.View the isotopic distribution chart. In the ‘Data Processing’ home, select ‘Interactive Charts’ from the top pane group. Click ‘Charts’ from the top pane → ‘All Charts’ → ‘Isotopic Distribution’ and click ‘Insert Chart.’
New peak detection: non-targeted MS (NTMS) processing to monitor inter-sample differences
13.Select NTMS New Peak Detection Algorithm. It is important to select the new peak detection algorithm for data processing once the sequence is created and the RPLC-HRAM MS analysis is performed. In the Chromeleon console select the sequence with the data, right click and go to ‘Properties’ → ‘Features.’ Select BioPharma Finder as the NTMS new peak detection algorithm.
14.Set up NPD parameters and start NPD analysis:
- a.
In the chromatography studio, click ‘Non-Targeted MS processing’ from the navigation panel. Define the processing method parameters according to the approach that you would like to take.
In the present study NPD is used for biosimilarity evaluation with the aim to detect any new or modified peaks with significant intersample differences in abundance compared to the reference product, which is previously characterize during MAM discovery phase. The parameter settings used for the NPD step in this study are outlined in Table 7.
- b.
Once the processing parameters are defined, click ‘Start’ from the top processing pane, if only a single injection is to be analyzed, or ‘Start All,’ to process all the injections within the sequence at a time.
The time required for processing a single file will depend on the parameters selected. If a low signal threshold is selected data processing will take longer.
Property | Value |
---|---|
General | |
Reference (control) injection #, name | Infliximab DP (#2) |
Processing type | Find all ions in the run |
Basic | |
Auto-compute basic parameters | False |
S/N threshold | 100 |
MS noise level | 8000 |
Absolute MS signal threshold | 8.0E05 |
Beginning peak width (min) | 0.20 |
Typical chromatographic peak width (min) | 0.20 |
Ending peak width (min) | 0.20 |
Max chromatographic peak width (min) | 1.0 |
Retention time limit start (min) | 5.0 |
Time limit end (min) | 70.0 |
Mass tolerance (ppm) | 5.00 |
Mass accuracy (ppm) | 5.00 |
Maximum RT shift (min) | 0.50 |
Advanced | |
Relative MS signal threshold | 1.00 |
Relative analog threshold | 1.00 |
Width of Gaussian filter | 3.00 |
Minimum chromatography valley (%) | 80.00 |
Minimum MS peak width (Da) | 1.20 |
Maximum MS peak width (Da) | 4.20 |
Maximum MS (Da) | 30,000 |
Mass centroiding cutoff (% from base) | 15.00 |
Results component filter | Value |
RT tolerance (min) | 0.5 |
m/z tolerance (ppm) | 5 ppm |
m/z range | Is between 220 and 1600 |
Charge state | Is between 2 and 5 |
MS area ratio | Is not between 0.1 and 10 |
15.View NPD results. Once the data analysis is completed ‘Component Results table,’ ‘Component Results plot’ and ‘MS plot’ will be shown.
16.Set up Result Component filters. Results must be filtered with the aim of reducing false negatives/positives, including RT tolerance (min), m/z tolerance (ppm), m/z range, charge state and MS area ratio. The latter allows for an x-fold change, either more or less, in terms of average intensity (Table 7).
17.Save the NPD processing parameters within the processing method and keep the NPD results in case they are needed in the future. The saved parameters can be modified later.
18.Identify the newly detected components. Protein identification software will be needed, as will the use of protein databases to search for the peptide identity. In the present work, BioPharma Finder software is used.
Design of the electronic report
19.In the Chromeleon console, click ‘Create’ → ‘Report Template.’ A few default templates are available in the software. Select ‘Empty Template’ and then customize it by including different sheets with the results that you want to be included. To rename the template, right-click the template name and choose ‘Rename.’ The template with its new name appears in the ‘Report Templates’ section in the ‘Report Designer’ from the navigation pane.
20.Create a sequence overview tab to summarize sequence and injection details:
-
Double-click the sheet 1 tab and name it accordingly.
-
Manually introduce sequence details, e.g., name, instrument and processing method and then choose the formula of interest by clicking ‘Insert’ from the top panel and selecting ‘Sequence,’ ‘Injection’ or ‘Chromatogram’ from the ‘Report Variables’ group on the top panel.
-
Do the same for the MS acquisition details.
-
Select an empty cell to introduce injection details and click ‘Insert’ → ‘All Tables’ → ‘Peak Summary Table’ → ‘Table’ → ‘Sequence Overview.’ The different columns in the table can be edited by the user.
-
It is also possible to add a chromatogram view by selecting ‘Insert’ → ‘Chromatogram’ from the ‘Plots’ group on the top panel. The chromatogram view will change depending on the injection that is selected at any time.
To add more worksheets, select ‘Home’ from the top panel, click ‘Insert’ from the ‘Cells and Sheets’ pane on the top and then select ‘Insert Sheet.’
21.Create PTM tabs for the different monitored attributes, e.g., oxidation, deamidation, N- and C-terminal composition and N-glycosylation:
-
Insert sequence details at the top of the page as described in step 20b.
-
Then, click ‘Insert’ → ‘All Tables’ → ‘Peak Summary Table’ → ‘Summary Table.’ The information contained for each column must be defined by the user, but in general, injection number, injection name and peak area for the detected components could be defined.
-
To enter this information, the application contains a full list of formulas and variables that can be used by just selecting the one of interest at any time.
-
To obtain the value of the relative levels of each of the modifications to be monitored, the formula must be entered manually within a cell.$$\begin{equation*} {\rm{% ;}}Modification = \frac{{XIC{\rm{;}}Area{\rm{;}}of{\rm{;}}modified{\rm{;}}peptide{\rm{;}}}}{{{\rm{SUM;}}\left( {XIC{\rm{;}}Area{\rm{;}}of{\rm{;}}modified{\rm{;}}peptide + XIC{\rm{;}}Area{\rm{;}}unmodified{\rm{;}}peptide} \right)}}\times{\rm{;}}100 \end{equation*}$$
22.Create a non-targeted MS processing tab to summarize the NPD results in a new worksheet renamed as ‘NPD,’ insert the NTMS processing parameters table at the top of the page by clicking ‘Insert’ → ‘All Tables’ → ‘Peptide Results’ → ‘BioPharma Finder Processing Parameters Table.’ Below, in an empty cell, click ‘Insert’ → ‘All Tables’ → ‘Peptide Results’ → ‘BioPharma Finder Component Results Table.’
23.Print the report and save it as a pdf. Select ‘Page Layout’ from the top panel and define page breaks, margins, orientation and autorepeat area. Click ‘Print Preview’ to see the page configuration before generating the final pdf, which could contain all the worksheets or only the ones you would like to include at any time.
REAGENTS AND SOLUTIONS
DTT, 50 mM
- 100 µl 500 mM DTT (see recipe)
- 900 µl of guanidine-Tris buffer (see recipe)
- Mix by vortexing in a 1.5-ml microcentrifuge tube (Eppendorf, cat. no. 022431081)
- Prepare fresh each day
DTT, 500 mM
- 50.0 mg DTT (Sigma-Aldrich, cat. no. D5545-5G)
- 649 µl guanidine-Tris buffer (see recipe)
- Mix by vortexing in a 1.5-ml microcentrifuge tube (Eppendorf, cat. no. 022431081)
- Prepare fresh each day
Formic acid, 10% (v/v)
- 1 ml formic acid, LC-MS grade (Thermo Scientific, cat. no. 28905)
- 9 ml LC-MS-grade H2O (Thermo Scientific, cat. no. W8-1)
- Mix in a 10-ml tube by vortexing
- Store up to 4 weeks at 4°C
Guanidine-Tris buffer
- For 100 ml:
- 87.5 ml 8.0 M guanidine HCl solution (Sigma-Aldrich, cat. no. G7294-100ML) (7.0 M final concentration)
- 10.0 ml 1 M Tris·HCl, pH 7.5, UltraPure (Invitrogen, cat. no. 15567-027) (100 mM final concentration)
- Mix thoroughly by inversion
- Adjust to pH 8.3 with NaOH concentrate (Sigma, cat. no. 43617-1L)
- Bring up to 100 ml with LC-MS-grade H2O (Thermo Scientific, cat. no. W8-1)
- Store up to 2 weeks at 4°C
Preparation should be conducted in a fume hood as sodium hydroxide causes severe skin burns and eye damage. Wear protective equipment when handling.
IAC, 500 mM
- 50.0 mg iodoacetic acid IAC (Sigma-Aldrich, cat. no. I9148-5G)
- 481 µl guanidine-Tris buffer (see recipe)
- Mix by vortexing in a 1.5-ml microcentrifuge tube (Eppendorf, cat. no. 022431081)
- Prepare fresh each day and store tube in the absence of light
Trifluoroacetic acid (TFA), 10% (v/v)
- 1 ml trifluoroacetic acid, eluent additive for LC-MS (Sigma, cat. no. 80457-50ML-B)
- 9 ml LC-MS-grade H2O (Thermo Scientific, cat. no. W8-1)
- Mix by vortexing in a 10-ml tube
Store up to 4 weeks at 4°C
Tris pH 7.9, 50 mM
- 10 ml 1 M Tris·HCl, pH 7.5, UltraPure (Invitrogen, cat. no. 15567-027)
- Bring up to 200 ml with LC-MS-grade H2O (Thermo Scientific, cat. no. W8-1)
- Mix 200-ml flask by inversion
- Adjust pH to 7.9 with HCl or NaOH
- Store up to 2 weeks at 4°C
COMMENTARY
Background Information
MAM is an emerging analytical workflow for biopharmaceutical characterization, batch release and cGMP purity testing at the peptide level. It is a bottom-up approach that involves the digestion of the protein of interests for subsequently reversed-phase chromatographic separation coupled to HRAM MS analysis, which provides high-resolution and high-mass-accuracy data. It is intended to replace conventional methods that are time consuming as they typically use profile-based measurements, which only allow to monitor individual quality attributes. They often lack sensitivity for the detection of impurities, and they are required to be used orthogonally. In contrast, MAM has the potential to provide more detailed information about potential PQAs/CQAs of complex products and is more sensitive than UV methods for the detection of impurities and low level PQAs/CQAs. It was initially developed for the characterization of mAb-based products, and since then it has attracted substantial attention within the biopharma community, now being extended to the application of more complex molecules, e.g., fusion proteins, BsAb or msAbs, Ab fragments, etc.
The main advantage lies in the ability to monitor multiple quality attributes in a single assay, facilitating streamlined biopharmaceutical production, from research and development to the QC environment. In alignment with quality by design (QbD), it helps in advancing a biologic to market and accelerating the process and product development by providing a more complete product profile characterization. MAM is one of the most sensitive approaches providing site-specific identification and quantitation of multiple PTMs in biotherapeutics. Overall, most studies report the application of MAM outside QC environment, while only a few recent publications focus on the application for release and testing in QC laboratories. There is a big interest in developing automated and high-throughput sample preparation to achieve robust digestion efficiency and low levels of protocol induced PTMs.
The application of MAM also requires compliant and fully automated software platforms to help with extensive data acquisition and processing in all stages of the MAM approach. On the other hand, instrument-to-instrument variability needs to be evaluated and optimized. Although LC-MS instruments provide comprehensive information, users require certain specialized training, and a certain level of MS expertise is required for data interpretation. While the MAM discovery phase sits in a less regulated environment and requires more specialized personnel and MS systems with MS/MS capabilities, more QC-friendly MS systems that are easy to operate is a requirement in cGMP environment.
Currently, moving MAM from research and development into QC environments still poses some challenges, and overcoming them would require a tight collaboration between the research community, biopharma industry and instrument/software vendors. Manufacturers are working on the creation of end-to-end solutions that facilitate the implementation in QC environments with the introduction of more QC-friendly mass detectors and a centralized data management system, which provides data security and integrity, increased performance by accelerating data processing, and increased productivity by facilitating data sharing and information connectivity. It is now possible to create eWorkflow procedures that contains all the associated methods and the reporting template required to set up the injection sequence, acquire, process the data, and summarize the results in a report with a visual pass/fail representation. On the other hand, a data lake would allow for software integration, instrument management, data storage, seamless data, and workflow transfer, accelerated data processing and advanced reporting. The first MAM implementation in the cGMP environment was in 2015 by Amgen (Rogers et al., 2015). In 2019, MAM was accepted by FDA as the replacement method for 4 conventional assays including: charge variants (IEX), protein fragments (rCE-SDS), glycan map (HILIC) and identification (ELISA). In 2022, a cooperative agreement with FDA under a funded research grant entitled “Assessment of the performance vs conventional QC methods for evaluation of PQAs of adalimumab and etanercept”, awarded USP (United States Pharmacopeia) a $1.5 million grant to study ways to enhance biosimilar and interchangeable biosimilar product development through use of MAM (USP, 2022).
Critical Parameters
Sample preparation is one of the most critical steps of the MAM approach. The development of a robust and reproducible sample preparation protocol with minimal sample preparation-induced artificial protein modifications is key to design a robust and reliable MAM workflow. The digestion procedure is also a critical point in the full analytical protocol and is a potential source of variability from operator to operator and laboratory to laboratory. As mentioned before, there have been many attempts to optimize the conditions used and there is great interest in moving toward sample automation or semi-automation, but this will also require specialized instruments, e.g., robotic platforms, which may not always be available. Digestion efficiency in terms of missed cleavages and nonspecific modifications must be evaluated for each type of sample. Depending on the complexity of the sample, different enzymes may be needed to achieve full sequence coverage and each of them would require digestion conditions to be optimized. Sample preparation must be assessed throughout the experiment by using a reference standard or a well-known sample within the laboratory (SST).
The choice of reversed-phase chromatographic column is also critical to have the efficiency and selectivity needed to separate some CQAs, e.g., deamidation, as deamidated peptides normally elute very close to the unmodified species. Gradients should be adjusted to the specific column dimension with the aim of keeping optimum chromatographic resolution in any case while keeping the analysis as short as possible to allow for high throughput analysis. In addition, mass spectrometers with high resolution mass analyzers are preferred over the use of low- and unit-resolution mass analyzers for both MS and tandem MS (MS/MS), because high mass resolution can improve mass accuracy and the reliability of peptide sequence identification.
It is important to highlight that to keep variability under control, both sample preparation quality and LC-MS system performance must be assessed. First, a system performance evaluation test (SET) is performed following instrument vendor recommendations any time there is a change or modification within the instrument platform, before the analysis of the digested peptides. Secondly, the SST must be included within the sequence of analysis.
For the data processing stage, NPD is the most critical aspect. It requires the optimization of most critical parameters e.g., Rt window, tolerance (ppm) and peak intensity threshold, to reduce the detection of false positives or negatives, always depending on the question to be addressed.
Troubleshooting
In Table 8, we provide a list of common problems associated with each of the protocols described, and their solutions.
Problem | Possible cause | Solution |
---|---|---|
The bed of the P6 column did not settle in the column | The column was spun at the wrong speed or for too long | Ensure that the centrifuge is running in g or rcf and not rpm; ensure that it is set for 1000 × g and runs for 2 min |
Mass spectrometry is failing to pass calibration | Quality of FlexMix is too poor | Use fresh FlexMix solution; any FlexMix solution that has been added to a syringe should not be added back to the main FlexMix bottle and instead should be stored in a separate Eppendorf tube |
Column pressure is unstable or lower than excepted | There is a leak in the connections | Ensure that the purge valve is closed fully and investigate the solvent lines to and from the column to identify the source of the leak |
Column pressure too high | There is a blockage in the column | Flush the column with a high percentage of B solvent (∼80%); if this does not resolve the issue then the column should be replaced |
Forevacum pressure too low | The ion transfer tube or sweep cone may be blocked | Clean both the ion transfer tube and sweep cone as per the vendors recommendation; CAUTION: ensure the temperature of the ion transfer tube is below 40°C to prevent burn damage |
The total signal intensity over replicates of a single sample is decreasing | Poor-quality vials | Assuming SET was successfully completed, loss of signal over replicate injections could be determined by adsorption of peptides on the walls of the glass/plastic vials; ensure that low-binding materials are used throughout and especially during sample storage in the autosampler over long sequences |
MS/MS data processing returns sequence coverage <80% | Low enzymatic activity; loss of hydrophilic peptides during LC separation | Check enzyme lifetime and replace the digestion buffer; also ensure that digestion tubes are kept at the right temperature and that there is no malfunctioning of the fridge/freezer; if the digestion generates very small hydrophilic peptides, the gradient for the separation should take into consideration a longer hold time or a slower gradient with a low percentage of organic phase |
Excessive deamidation and oxidation levels observed in the analyte or SST sample |
Incorrect sample storage; incorrect sample digestion | Ensure that the sample was stored correctly before and after digestion; replace buffers used during sample preparation and avoid excessive handling |
Understanding Results
To illustrate the applicability of the protocol, the analytical similarity of three different infliximab biosimilars was accomplished in the present study using the described MAM protocol. This allowed for primary sequence assessment and evaluation of multiple quality attributes including deamidation, oxidation, succinimide formation, N- and C- terminal composition and detailed N-glycosylation analysis. In the first step, characterization of the most relevant PTMs of a reference product was attained during the discovery phase of MAM. The second step describes biosimilarity evaluation of predefined quality attributes and new peak detection for the assessment of any new or modified peak compared to the infliximab RP. This study highlights a new perspective of the MAM approach and its underlying power for biotherapeutic comparability exercises in addition to analytical characterization, as previously described by Millán-Martín et al. (2023).
In the present study, infliximab RP was analyzed during the discovery phase of MAM using MS/MS acquisition on an Orbitrap Exploris 240 mass spectrometer. In a second step, infliximab biosimilars were analyzed by full MS-only acquisition during the MAM monitoring phase using an Orbitrap Exploris MX mass detector. Triplicate digests were prepared, and samples were analyzed following the workflow summarized in Figure 1 and as described in Basic Protocol 1. Initially, sequence coverage was evaluated, and results showed that full or almost full sequence coverage is attained for light chain (LC) and heavy chain (HC), respectively, for all the RP replicates, as described previously (Fig. 4 and Table 5). Table S1 lists the selected quality attributes for infliximab RP comprising 39 attributes (see Supporting Information). Only those attributes with relative abundances over 0.1% were considered. The peptide workbook was generated with BioPharma Finder and a summary of all the peptides contained is provided in Supplementary Data 2 (see Supporting Information).
The next step focused on the evaluation of the predefined PQAs/CQAs for three different infliximab biosimilars. Samples were digested in triplicate as described in Basic Protocol 1 and analyzed according to the chromatographic conditions described in Basic Protocol 2. Full MS-only data acquisition was carried out for the monitoring of quality attributes of infliximab biosimilars. The resulting chromatograms are shown in Figure 5, where a mirror plot represents the TICs of the biosimilars compared to infliximab RP (bottom blue trace). Overall, all the biosimilar traces are comparable to the originator with just some small noticeable differences in terms of peak abundances for some of the biosimilars.
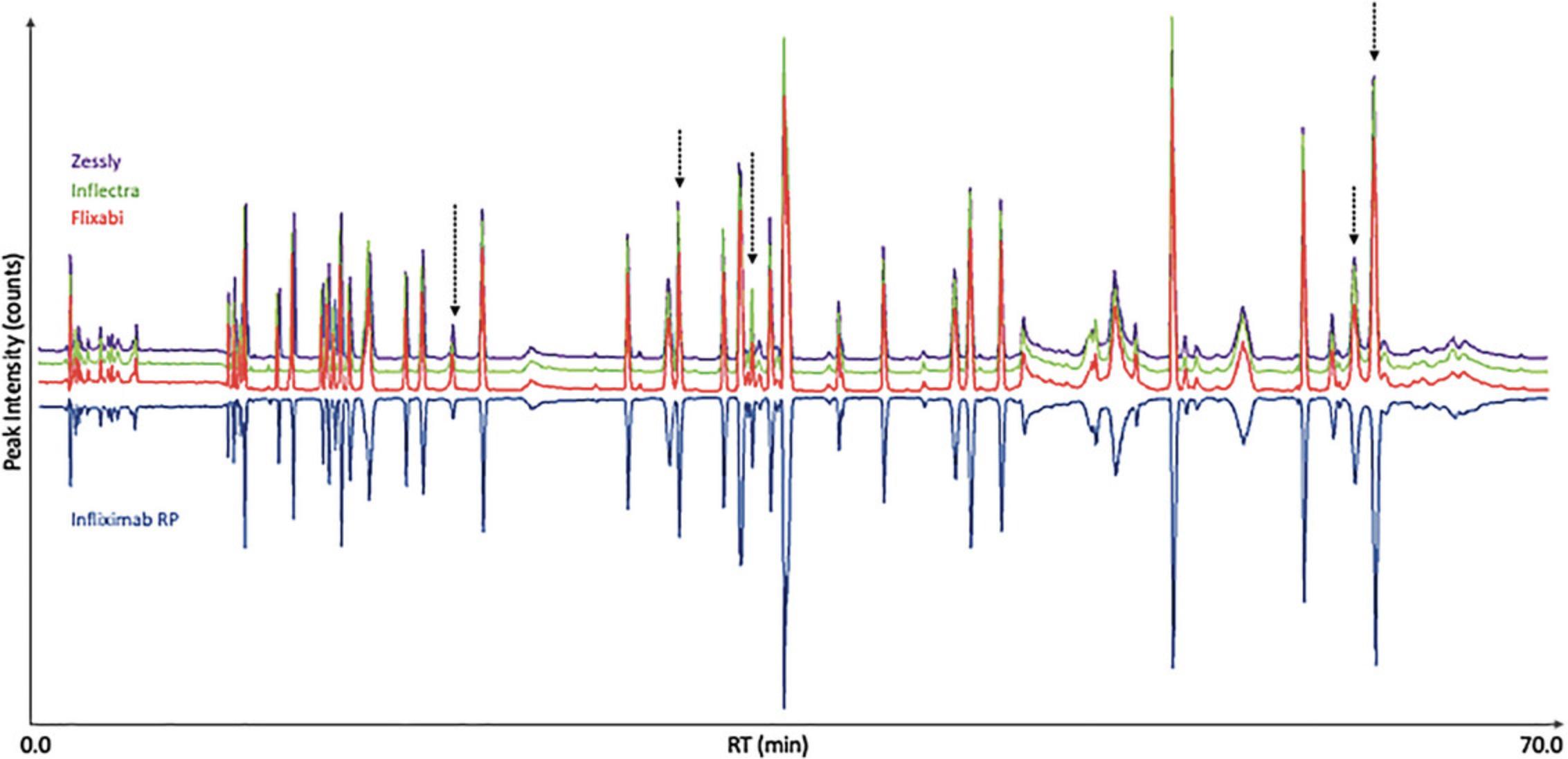
Predefined PQAs/CQAs were then evaluated for all biosimilars compared to the RP using the MAM processing method created during MAM discovery phase. Specific method templates were created to show the results. An example of NISTmab SST report containing all the injections performed during monitoring phase is shown in Supplementary Data 3 (see Supporting Information), where all the evaluated parameters are within the established acceptance criteria. Figures 6 and 7 and Figure S1 show the average relative abundances for the monitored PQAs/CQAs, and the error associated with them expressed as standard deviation of the triplicates (see Supporting Information). Overall, values were comparable for all the studied biosimilars and the RP, e.g., some deamidated, succinimide, oxidized species (i.e., N318, N31, M431, M85, or M55) and glycation. Main differences were observed for some oxidation hotspots e.g., M255 and M34 residues (Yang et al., 2015), with values varying from 1.1% (infliximab RP) to 4.8% (Flixabi) and from 2.4% (infliximab RP) to 6.1% (Zessly), respectively. Deamidation on the PENNYK peptide showed values between 0.6% (Zessly) and 1.1% (infliximab RP), and N57 deamidation between 0.3% (infliximab RP) and 0.8% (Flixabi). C-term lysine (Lys) content was notably different, with values between 5.2% (Flixabi) and 62.9% (infliximab RP). It is important to highlight that the loss of C-terminal Lys in HC (K450) is not expected to be clinically significant and already showed high variability across investigated RP batches (Dick et al., 2008; Millan-Martin, Jakes, Carillo, & Bones, 2023).
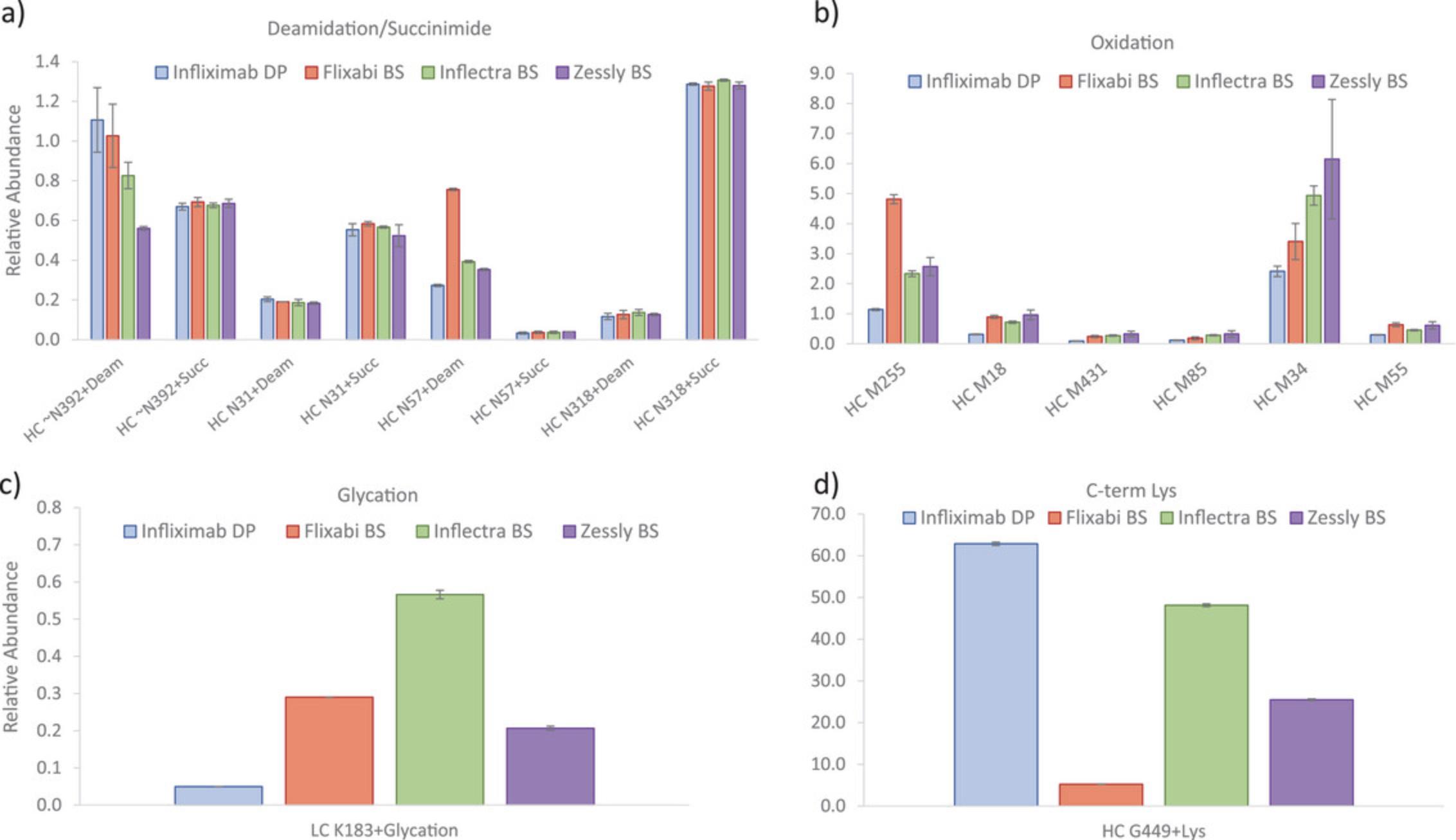
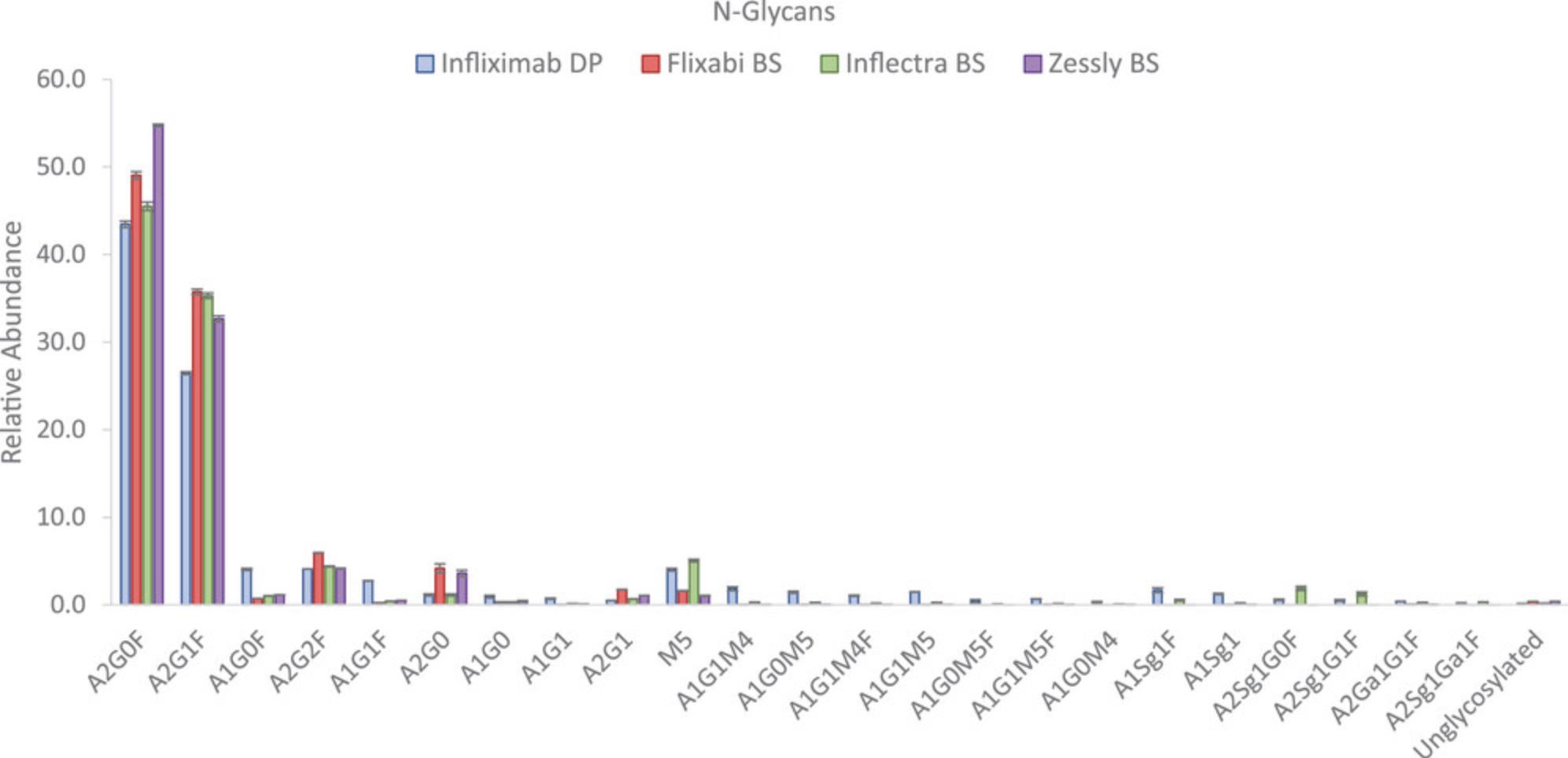
Most abundant glycoforms were identified as core fucosylated structures with different degrees of galactosylation (i.e., A2G0F, A2G1F, A1G0F, A2G2F, A1G1F), afucosylated structures e.g., A2G0, or high mannose structures (i.e., M5). Noticeable differences were detected for A2G0F, with values between 43.4% (infliximab RP) and 54.7% (Zessly); A2G1F, with values between 26.4% (infliximab RP) and 35.8% (Flixabi) or A1G0F, with values between 0.7% (Flixabi) and 4.0% (infliximab RP).
The last step for analytical comparability evaluation of infliximab biosimilars was to apply NPD with the aim of detecting any new or modified peaks with significant inter-sample differences in abundance, as impurity profiling is a prerequisite during biosimilar development. Product-related variants and impurities, including size- or charge-based heterogeneity, and other product modifications correspond to heterogeneities formed during bioprocess manufacturing, handling, and storage. The parameter settings used for the NPD step in this study are outlined in Table 7. The selected parameters were based on a recently published study performed by the group (Millan-Martin, Jakes, Carillo, & Bones, 2023). Results showed some new/modified peaks for the 3 biosimilars evaluated. Tentative identification of the new peaks detected was carried out after further MS/MS analysis of the selected biosimilars and data processing with BioPharma Finder software using the RP protein sequence. Flixabi was the biosimilar exhibiting more differences compared to the RP (Table 9), while Inflectra showed only two components with differences in abundances for a nonspecific generated peptide. Table 9 summarizes all the new detected or modified peaks for all the studied infliximab biosimilars where only those components present in at least two of the three replicates were included in all cases. Overall, most of the components reported by NPD showed changes in peak abundance, mainly for some glycoforms, e.g., sialylated structures (i.e., A1Sg1F detected only for infliximab RP and Inflectra), hybrid structures (i.e., A1G0M5, A1G1M5, A1G1M4, detected mainly for infliximab RP), and other glycoforms, such as A1G1F.
RT (min) | m/z | Charge state | Mono mass experimental | MS area ratio | MS area | Control MS area | Peptide identity (BioPharma Finder) |
---|---|---|---|---|---|---|---|
Flixabi biosimilar | |||||||
8.99 | 769.8342 | 4 | 3074.3008 | 0.05 | 9.93E03 | 1.93E05 | HC:T292-R304 (+A1G1F) |
9.00 | 773.8330 | 4 | 3090.2971 | 0.01 | 1.23E03 | 1.78E05 | HC:T292-R304 (+A1G0M5) |
9.10 | 846.6066 | 4 | 3381.3940 | 0.00 | 2.69E01 | 1.82E05 | HC:T292-R304 (+A1Sg1F) |
9.30 | 919.3616 | 3 | 2754.0583 | 0.03 | 6.08E03 | 1.92E05 | HC:E296-R304 (+A1Sg1) |
9.32 | 865.3441 | 3 | 2592.0071 | 0.07 | 1.84E04 | 2.77E05 | HC:E296-R304 (+A1G1F) |
9.34 | 924.6940 | 3 | 2770.0579 | 0.03 | 7.00E03 | 2.09E05 | HC:E296-R304 (+A1G1M5) |
9.36 | 870.6760 | 3 | 2608.0039 | 0.02 | 5.58E03 | 2.92E05 | HC:E296-R304 (+A1G1M4) |
9.36 | 1305.5125 | 2 | 2608.0059 | 0.02 | 3.71E03 | 1.93E05 | HC:E296-R304 (+A1G1M4) |
9.55 | 967.7094 | 3 | 2899.0994 | 0.00 | 2.61E02 | 1.75E05 | HC:E296-R304 (+A1Sg1F) |
11.44 | 409.7073 | 4 | 1634.8003 | 17.11 | 3.69E05 | 2.16E04 | HC:S55:R69 (+15.9915) |
11.45 | 545.9407 | 3 | 1634.8004 | 17.77 | 1.61E05 | 9.03E03 | HC:S55:R69 (+15.9915) |
13.14 | 711.8459 | 2 | 1421.6772 | 39.22 | 2.34E05 | 5.95E03 | HC:S55-K67 (+15.9949) |
13.14 | 474.8997 | 3 | 1421.6772 | 37.47 | 9.75E05 | 2.60E04 | HC:S55-K67 (+15.9949) |
21.80 | 461.7023 | 2 | 921.3900 | N/A | 1.71E05 | 0.00E00 | not identified |
36.69 | 553.2894 | 3 | 1656.8464 | 45.05 | 8.25E05 | 1.83E04 | HC:Q39-R52 (+W47 oxidation) |
49.08 | 1246.6174 | 5 | 6225.0400 | 0.05 | 9.05E04 | 1.75E06 | HC:T396-G449 (+ Lys) |
61.78 | 1235.2916 | 3 | 3700.8479 | 0.02 | 8.22E03 | 4.81E05 | not detected |
61.89 | 1001.4734 | 4 | 3999.8596 | 21.34 | 5.42E05 | 2.54E04 | HC:L4-R38 (+K39 glycation) |
Inflectra biosimilar | |||||||
23.58 | 947.4473 | 3 | 2838.3169 | 13.08 | 4.91E05 | 3.76E04 | LC:V146-K169 (+K149 glycation) |
32.16 | 758.0319 | 3 | 2270.0708 | 13.4 | 5.99E05 | 4.47E04 | LC:D170-K188 (+K183 glycation) |
Zessly biosimilar | |||||||
9.02 | 773.8342 | 4 | 3090.3010 | 0.02 | 4.00E03 | 1.78E05 | HC:T292-R304 (+A1G0M5) |
9.16 | 846.6089 | 4 | 3381.3940 | 0.00 | 4.44E02 | 1.82E05 | HC:T292-R304 (+A1Sg1F) |
9.41 | 924.6956 | 3 | 2770.0615 | 0.04 | 7.85E03 | 2.09E05 | HC:E296-R304 (+A1G1M5) |
9.43 | 870.6777 | 3 | 2608.0081 | 0.02 | 6.89E03 | 2.92E05 | HC:E296-R304 (+A1G1M4) |
9.43 | 1305.5143 | 2 | 2608.0081 | 0.02 | 4.36E03 | 1.93E05 | HC:E296-R304 (+A1G1M4) |
9.54 | 967.7081 | 3 | 2899.0994 | 0.00 | 0.00E00 | 1.75E05 | HC:E296-R304 (+A1Sg1F) |
10.57 | 615.8097 | 2 | 1229.6049 | 1375.41 | 1.55E05 | 1.12E02 | HC:Y281-K291 (nonspecific) |
10.57 | 410.8757 | 3 | 1229.6053 | 394.15 | 1.00E06 | 2.54E03 | HC:Y281-K291 (nonspecific) |
13.29 | 474.9004 | 3 | 1421.6794 | 13.35 | 3.47E05 | 2.60E04 | HC:S55-K67 (+15.9949) |
14.10 | 251.6837 | 2 | 501.3529 | N/A | 1.04E05 | 0.00E00 | LC:L46-K49 (+15.9952) |
36.66 | 553.2899 | 3 | 1656.8479 | 23.95 | 4.38E05 | 1.83E04 | HC:Q39-R52 (+15.9952) |
- a Using NPD tool for 8.0E05 absolute MS signal threshold within BioPharma finder algorithm.
It is important to highlight 2 new peaks, with m/z 545.941 (z = 3) or m/z 409.707 (z = 4) eluting at 11.40 min for Flixabi (Table 9); and m/z 711.846 (z = 2) or m/z 474.900 (z = 3) eluting at 13.15 min for Flixabi and Zessly, were reported and identified as potential sequence variants (SINSATHYAESVKGR, +15.9915 or SINSATHYAESVK, +15.9949, respectively). For peptides containing a single amino acid substitution, identification was possible also using MS/MS data analysis searched using the RP sequence, with parameters set to allow for amino acid substitutions. Full sequence coverage was attained in all cases. The relative abundances were quantified using LC-MS analysis of the trypsin digested protein as previously described (Millan-Martin, Jakes, Carillo, & Bones, 2023). The potential sequence variant represents the 1.7% (n = 3) for Flixabi biosimilar and 0.3% (n=3) for Zessly biosimilar, in terms of relative abundance and could be the result of the substitution of alanine (A) for serine (S). Amino acid misincorporation is the errant replacement of an amino acid during the translation process, producing unintended protein sequence variants, which inherently occurs at generally low levels depending on the expression system and cell line (Wong et al., 2018). Low levels of alanine to serine sequence variants were identified in an IgG4 monoclonal antibody by ultra/high performance liquid chromatography and tandem mass spectrometry by Fu et al. (2012). Zessly biosimilar also showed a new peak with m/z 251.6837 (z = 2), eluting at 14.10 min and a modified peak with m/z 553.2899 (z =3), eluting at 36.66 min. Based on MS/MS analysis (Figure S2; see Supporting Information) the potential modification was assigned to lysine hydroxylation on the peptide LLIK and QSPEKGLEWVAEIR, respectively. Relative levels were below 2% in both cases. Discovery and characterization of hydroxylysine in recombinant monoclonal antibodies has been previously reported by Xie et al. (2016).
Overall, all evaluated biosimilars showed high similarity compared to the RP. Only two of the analyzed biosimilars contained a new peak when the NPD tool was applied, with the new peak identified as a potential sequence variant. The identity of this potential sequence variant could be confirmed by MS/MS data demonstrating the power of MAM as an approach for comparability assessment.
Time Considerations
The protocols described here can be completed as follows:
Basic Protocol 1. One person can typically prepare a maximum of 12 samples within 8 hr. Timing of 90 to 120 min, depending on the size of the sample set. Alternate Protocol. One person can typically prepare a maximum of 12 samples in one plate using the KF purification system described in the protocol. For larger quantities of samples, additional KF systems are available to allow for the digestion of up to 96 samples, e.g., the KF Flex system, which allows handling up to 24 to 96 samples per run. Timing of 40 to 60 min, depending on the size of the sample set. Basic Protocol 2. Reversed-phase chromatographic separation and detection by HRAM-MS, including instrument set up, calibration, etc. could take from 7 to 72 hr. As mentioned earlier, it is important to ensure that acquisition does not exceed 72 hr to prevent ion transfer tube blockage and to allow for mass calibration to be performed. Consequently, sequences should be kept to a maximum length of 36 injections, but this will also depend on the type of sample and the applied gradient length. Basic Protocol 3. Data processing, analysis and reporting could take from 2 to 40 hr, depending on the number of samples and quality attributes to monitor and report. Discovery phase for detailed quality attribute characterization could take from 2 to 7 hr minimum, including the time needed to create the peptide workbook. This will depend on the number of attributes to be monitored. For the monitoring phase, the initial processing method creation will require from few hours to even days (depending on the number of attributes to be monitored and the number of data files within the sequence), but once the method is created, it will take only a few seconds to minutes to process new samples and obtain a report with the results (depending on the sample set).
Acknowledgments
The authors gratefully acknowledge Thermo Fisher Scientific for instrument access and financial support.
Open access funding provided by IReL.
Author Contributions
Silvia Millán-Martín : Conceptualization; formal analysis; methodology; visualization; writing original draft; writing review and editing. Craig Jakes : Conceptualization; investigation; methodology; writing review and editing. Sara Carillo : Conceptualization; investigation; methodology; supervision; writing review and editing. Jonathan Bones : Conceptualization; funding acquisition; supervision; writing review and editing.
Conflict of Interest
J.B. received funding to support undertaking this study as part of a funded collaboration between NIBRT and Thermo Fisher Scientific. S.C., C.J., and S.M.-M. are employed on this collaborative project. Beyond this, the authors are not aware of any affiliations, memberships, funding, or financial holdings that might be perceived as affecting the objectivity of this article. The authors declare no competing financial interests.
Open Research
Data Availability Statement
The data that support the findings of this protocol are available from the corresponding author upon reasonable request.
Supporting Information
Filename | Description |
---|---|
cpz1927-sup-0001-SuppMat.docx306.1 KB | File contains: Figure S1. Zoomed view from figure 7, excluding most abundant glycans A2G0F and A2G1F, representing the average relative abundance (n = 3) of monitored N-glycans on the Fc region. Error bars represent standard deviation error of mean calculated for triplicates of infliximab biosimilars and RP; Figure S2. MS/MS spectrum with peak assignments and fragment coverage map for parent peptide QSPEKGLEWVAEIR (a) and potential hydroxylated peptide (+15.9952), for Zessly biosimilar (b), obtained with BioPharma Finder; and Table S1. List of the CQAs/PQAs identified through MS/MS analysis of EU-licensed infliximab RP. HC: heavy chain; LC: light chain. |
cpz1927-sup-0002-SuppMat.pdf5.5 MB | Supplementary Data 1: Data processing walk-through. |
cpz1927-sup-0003-SuppMat.xlsx43.8 KB | Supplementary Data 2: Peptide workbook. |
cpz1927-sup-0004-SuppMat.pdf436.9 KB | Supplementary Data 3: SST report. |
Please note: The publisher is not responsible for the content or functionality of any supporting information supplied by the authors. Any queries (other than missing content) should be directed to the corresponding author for the article.
Literature Cited
- Au, H. K. E., Isalan, M., & Mielcarek, M. (2021). Gene therapy advances: A meta-analysis of AAV usage in clinical settings. Frontiers in Medicine (Lausanne) , 8, 809118. https://doi.org/10.3389/fmed.2021.809118
- Buettner, A., Maier, M., Bonnington, L., Bulau, P., & Reusch, D. (2020). Multi-attribute monitoring of complex erythropoetin beta glycosylation by GluC liquid chromatography-mass spectrometry peptide mapping. Analytical Chemistry , 92(11), 7574–7580. https://doi.org/10.1021/acs.analchem.0c00124
- Das, T. K., Narhi, L. O., Sreedhara, A., Menzen, T., Grapentin, C., Chou, D. K., Antochshuk, V., & Filipe, V. (2020). Stress factors in mAb drug substance production processes: Critical assessment of impact on product quality and control strategy. Journal of Pharmaceutical Sciences , 109(1), 116–133. https://doi.org/10.1016/j.xphs.2019.09.023
- Dick, L. W., Jr., Qiu, D., Mahon, D., Adamo, M., & Cheng, K. C. (2008). C-terminal lysine variants in fully human monoclonal antibodies: Investigation of test methods and possible causes. Biotechnology & Bioengineering, 100(6), 1132–1143. https://doi.org/10.1002/bit.21855
- Evans, A. R., Hebert, A. S., Mulholland, J., Lewis, M. J., & Hu, P. (2021). ID-MAM: A validated identity and multi-attribute monitoring method for commercial release and stability testing of a bispecific antibody. Analytical Chemistry , 93(26), 9166–9173. https://doi.org/10.1021/acs.analchem.1c01029
- FDA. (2022). Quality considerations for the Multi-Attribute Method (MAM) for therapeutic proteins. Webcast, October 13, 2022. https://www.fda.gov/science-research/fda-grand-rounds/quality-considerations-multi-attribute-method-mam-therapeutic-proteins-10132022#event-materials
- Fekete, S., Beck, A., Veuthey, J. L., & Guillarme, D. (2015). Ion-exchange chromatography for the characterization of biopharmaceuticals. Journal of Pharmaceutical and Biomedical Analysis , 113, 43–55. https://doi.org/10.1016/j.jpba.2015.02.037
- Fu, J., Bongers, J., Tao, L., Huang, D., Ludwig, R., Huang, Y., Qian, Y., Basch, J., Goldstein, J., Krishnan, R., You, L., Li, Z. J., & Russell, R. J. (2012). Characterization and identification of alanine to serine sequence variants in an IgG4 monoclonal antibody produced in mammalian cell lines. Journal of Chromatography B: Analytical Technologies in the Biomedical and Life Sciences , 908, 1–8. https://doi.org/10.1016/j.jchromb.2012.09.023
- Guan, X., Eris, T., Zhang, L., Ren, D., Ricci, M. S., Thiel, T., & Goudar, C. T. (2022). A high-resolution multi-attribute method for product characterization, process characterization, and quality control of therapeutic proteins. Analytical Biochemistry , 643, 114575. https://doi.org/10.1016/j.ab.2022.114575
- Guapo, F., Strasser, L., Millan-Martin, S., Anderson, I., & Bones, J. (2022). Fast and efficient digestion of adeno associated virus (AAV) capsid proteins for liquid chromatography mass spectrometry (LC-MS) based peptide mapping and post translational modification analysis (PTMs). Journal of Pharmaceutical and Biomedical Analysis , 207, 114427. https://doi.org/10.1016/j.jpba.2021.114427
- Haberger, M., Leiss, M., Heidenreich, A. K., Pester, O., Hafenmair, G., Hook, M., Bonnington, L., Wegele, H., Haindl, M., Reusch, D., & Bulau, P. (2016). Rapid characterization of biotherapeutic proteins by size-exclusion chromatography coupled to native mass spectrometry. MAbs , 8(2), 331–339. https://doi.org/10.1080/19420862.2015.1122150
- Hao, Z., Moore, B., Ren, C., Sadek, M., Macchi, F., Yang, L., Harris, J., Yee, L., Liu, E., Tran, V., Ninonuevo, M., Chen, Y., & Yu, C. (2021). Multi-attribute method performance profile for quality control of monoclonal antibody therapeutics. Journal of Pharmaceutical and Biomedical Analysis , 205, 114330. https://doi.org/10.1016/j.jpba.2021.114330
- Holm, N. B., Deryabina, M., Knudsen, C. B., & Janfelt, C. (2022). Tissue distribution and metabolic profiling of cyclosporine (CsA) in mouse and rat investigated by DESI and MALDI mass spectrometry imaging (MSI) of whole-body and single organ cryo-sections. Analytical and Bioanalytical Chemistry , 414(24), 7167–7177. https://doi.org/10.1007/s00216-022-04269-z
- Jakes, C., Millan-Martin, S., Carillo, S., Scheffler, K., Zaborowska, I., & Bones, J. (2021). Tracking the behavior of monoclonal antibody product quality attributes using a multi-attribute method workflow. Journal of the American Society for Mass Spectrometry , 32(8), 1998–2012. https://doi.org/10.1021/jasms.0c00432
- Li, X., Rawal, B., Rivera, S., Letarte, S., & Richardson, D. D. (2022). Improvements on sample preparation and peptide separation for reduced peptide mapping based multi-attribute method analysis of therapeutic monoclonal antibodies using lysyl endopeptidase digestion. Journal of Chromatography A , 1675, 463161. https://doi.org/10.1016/j.chroma.2022.463161
- Melmer, M., Stangler, T., Schiefermeier, M., Brunner, W., Toll, H., Rupprechter, A., Lindner, W., & Premstaller, A. (2010). HILIC analysis of fluorescence-labeled N-glycans from recombinant biopharmaceuticals. Analytical and Bioanalytical Chemistry , 398(2), 905–914. https://doi.org/10.1007/s00216-010-3988-x
- Millan-Martin, S., Jakes, C., Carillo, S., & Bones, J. (2023). Multi-attribute method (MAM) to assess analytical comparability of adalimumab biosimilars. Journal of Pharmaceutical and Biomedical Analysis , 234, 115543. https://doi.org/10.1016/j.jpba.2023.115543
- Millan-Martin, S., Jakes, C., Carillo, S., Buchanan, T., Guender, M., Kristensen, D. B., Sloth, T. M., Ørgaard, M., Cook, K., & Bones, J. (2020). Inter-laboratory study of an optimised peptide mapping workflow using automated trypsin digestion for monitoring monoclonal antibody product quality attributes. Analytical and Bioanalytical Chemistry , 412(25), 6833–6848. https://doi.org/10.1007/s00216-020-02809-z
- Millán-Martín, S., Jakes, C., Carillo, S., Gallagher, L., Scheffler, K., Broster, K., & Bones, J. (2023). Multi-Attribute Method (MAM): An Emerging Analytical Workflow for Biopharmaceutical Characterization, Batch Release and cGMP Purity Testing at the Peptide and Intact Protein Level. Critical Reviews in Analytical Chemistry , 1–18. Advance online publication. https://doi.org/10.1080/10408347.2023.2238058
- Mouchahoir, T., Schiel, J. E., Rogers, R., Heckert, A., Place, B. J., Ammerman, A., Li, X., Robinson, T., Schmidt, B., Chumsae, C. M., Li, X., Manuilov, A. V., Yan, B., Staples, G. O., Ren, D., Veach, A. J., Wang, D., Yared, W., Sosic, Z., & Yuan, H. (2021). New peak detection performance metrics from the MAM consortium interlaboratory study. Journal of the American Society for Mass Spectrometry , 32(4), 913–928. https://doi.org/10.1021/jasms.0c00415
- Niu, B., Lu, Y., Chen, X., & Xu, W. (2023). Using new peak detection to solve sequence variants analysis challenges in bioprocess development. Journal of the American Society for Mass Spectrometry , 34(3), 401–408. https://doi.org/10.1021/jasms.2c00292
- Otsubo, R., & Yasui, T. (2022). Monoclonal antibody therapeutics for infectious diseases: Beyond normal human immunoglobulin. Pharmacology & Therapeutics, 240, 108233. https://doi.org/10.1016/j.pharmthera.2022.108233
- Puranik, A., Rasam, P., Dandekar, P., & Jain, R. (2023). Development and optimization of a LC-MS based multi-attribute method (MAM) workflow for characterization of therapeutic Fc-fusion protein. Analytical Biochemistry , 660, 114969. https://doi.org/10.1016/j.ab.2022.114969
- Qian, C., Niu, B., Jimenez, R. B., Wang, J., & Albarghouthi, M. (2021). Fully automated peptide mapping multi-attribute method by liquid chromatography-mass spectrometry with robotic liquid handling system. Journal of Pharmaceutical and Biomedical Analysis , 198, 113988. https://doi.org/10.1016/j.jpba.2021.113988
- Rathore, D., Faustino, A., Schiel, J., Pang, E., Boyne, M., & Rogstad, S. (2018). The role of mass spectrometry in the characterization of biologic protein products. Expert Review of Proteomics , 15(5), 431–449. https://doi.org/10.1080/14789450.2018.1469982
- Rocamora, F., Peralta, A. G., Shin, S., Sorrentino, J., Wu, M. Y. M., Toth, E. A., Fuerst, T. R., & Lewis, N. E. (2023). Glycosylation shapes the efficacy and safety of diverse protein, gene and cell therapies. Biotechnology Advances , 67, 108206. https://doi.org/10.1016/j.biotechadv.2023.108206
- Rogers, R. (2019). Case study: Automated software-driven implementation of the Multi-Attribute Method (MAM) for biotherapeutic characterization. Retrieved from https://static.yanyin.tech/literature/current_protocol/10.1002/cpz1.927/attachments/Ex_Just_MAM_19E03.pdf
- Rogers, R. S., Abernathy, M., Richardson, D. D., Rouse, J. C., Sperry, J. B., Swann, P., Wypych, J., Yu, C., Zang, L., & Deshpande, R. (2018). A view on the importance of “multi-attribute method” for measuring purity of biopharmaceuticals and improving overall control strategy. The APPS Journal , 20(1), 7. https://doi.org/10.1208/s12248-017-0168-3
- Rogers, R. S., Nightlinger, N. S., Livingston, B., Campbell, P., Bailey, R., & Balland, A. (2015). Development of a quantitative mass spectrometry multi-attribute method for characterization, quality control testing and disposition of biologics. MAbs , 7(5), 881–890. https://doi.org/10.1080/19420862.2015.1069454
- Shim, H. (2020). Bispecific antibodies and antibody-drug conjugates for cancer therapy: Technological considerations. Biomolecules , 10(3), 360. https://doi.org/10.3390/biom10030360
- Song, Y. E., Dubois, H., Hoffmann, M., Eri, S. D., Fromentin, Y., Wiesner, J., Pfenninger, A., Clavier, S., Pieper, A., Duhau, L., & Roth, U. (2021). Automated mass spectrometry multi-attribute method analyses for process development and characterization of mAbs. Journal of Chromatography B: Analytical Technologies in the Biomedical and Life Sciences , 1166, 122540. https://doi.org/10.1016/j.jchromb.2021.122540
- Stavenhagen, K., Gahoual, R., Dominguez Vega, E., Palmese, A., Ederveen, A. L. H., Cutillo, F., Palinsky, W., Bierau, H., & Wuhrer, M. (2019). Site-specific N- and O-glycosylation analysis of atacicept. MAbs , 11(6), 1053–1063. https://doi.org/10.1080/19420862.2019.1630218
- Struwe, W. B., & Robinson, C. V. (2019). Relating glycoprotein structural heterogeneity to function - insights from native mass spectrometry. Current Opinion in Structural Biology , 58, 241–248. https://doi.org/10.1016/j.sbi.2019.05.019
- Suba, D., Urbanyi, Z., & Salgo, A. (2015). Capillary isoelectric focusing method development and validation for investigation of recombinant therapeutic monoclonal antibody. Journal of Pharmaceutical and Biomedical Analysis , 114, 53–61. https://doi.org/10.1016/j.jpba.2015.04.037
- Szabo, M., Sarkozy, D., Szigeti, M., Farsang, R., Kardos, Z., Kozma, A., Csanky, E., Chung, D. S., Szekanecz, Z., & Guttman, A. (2022). Introduction of a capillary gel electrophoresis-based workflow for biotherapeutics characterization: Size, charge, and N-glycosylation variant analysis of bamlanivimab, an anti-SARS-CoV-2 product. Frontiers in Bioengineering and Biotechnology , 10, 839374. https://doi.org/10.3389/fbioe.2022.839374
- Terracciano, R., Preiano, M., Fregola, A., Pelaia, C., Montalcini, T., & Savino, R. (2021). Mapping the SARS-CoV-2-host protein-protein interactome by affinity purification mass spectrometry and proximity-dependent biotin labeling: A rational and straightforward route to discover host-directed anti-SARS-CoV-2 therapeutics. International Journal of Molecular Sciences , 22(2), 532. https://doi.org/10.3390/ijms22020532
- Trappe, A., Fussl, F., Carillo, S., Zaborowska, I., Meleady, P., & Bones, J. (2018). Rapid charge variant analysis of monoclonal antibodies to support lead candidate biopharmaceutical development. Journal of Chromatography B: Analytical Technologies in the Biomedical and Life Sciences , 1095, 166–176. https://doi.org/10.1016/j.jchromb.2018.07.037
- USP. (2022). U.S. Pharmacopeia awarded FDA funding to create a Multi-Attribute Method (MAM) knowledge base. September 29th, 2022. https://www.usp.org/news/us-pharmacopeia-awarded-fda-funding-to-create-a-multi-attribute-method-knowledge-base
- Wang, Y., Li, X., Liu, Y. H., Richardson, D., Li, H., Shameem, M., & Yang, X. (2016). Simultaneous monitoring of oxidation, deamidation, isomerization, and glycosylation of monoclonal antibodies by liquid chromatography-mass spectrometry method with ultrafast tryptic digestion. MAbs , 8(8), 1477–1486. https://doi.org/10.1080/19420862.2016.1226715
- Wen, Y., & Jawa, V. (2021). The impact of product and process related critical quality attributes on immunogenicity and adverse immunological effects of biotherapeutics. Journal of Pharmaceutical Sciences , 110(3), 1025–1041. https://doi.org/10.1016/j.xphs.2020.12.003
- Wong, H. E., Huang, C. J., & Zhang, Z. (2018). Amino acid misincorporation in recombinant proteins. Biotechnology Advances , 36(1), 168–181. https://doi.org/10.1016/j.biotechadv.2017.10.006
- Xie, Q., Moore, B., & Beardsley, R. L. (2016). Discovery and characterization of hydroxylysine in recombinant monoclonal antibodies. MAbs , 8(2), 371–378. https://doi.org/10.1080/19420862.2015.1122148
- Xu, C., Khanal, S., Pierson, N. A., Quiroz, J., Kochert, B., Yang, X., Wylie, D., & Strulson, C. A. (2022). Development, validation, and implementation of a robust and quality control-friendly focused peptide mapping method for monitoring oxidation of co-formulated monoclonal antibodies. Analytical and Bioanalytical Chemistry , 414(29–30), 8317–8330. https://doi.org/10.1007/s00216-022-04366-z
- Yang, Y., Strahan, A., Li, C., Shen, A., Liu, H., Ouyang, J., Katta, V., Francissen, K., & Zhang, B. (2010). Detecting low level sequence variants in recombinant monoclonal antibodies. MAbs , 2(3), 285–298. https://doi.org/10.4161/mabs.2.3.11718
- Yang, Y., Zhao, J., Geng, S., Hou, C., Li, X., Lang, X., Qiao, C., Li, Y., Feng, J., Lv, M., Shen, B., & Zhang, B. (2015). Improving Trastuzumab's stability profile by removing the two degradation hotspots. Journal of Pharmaceutical Sciences , 104(6), 1960–1970. https://doi.org/10.1002/jps.24435
- Yasunaga, M. (2020). Antibody therapeutics and immunoregulation in cancer and autoimmune disease. Seminars in Cancer Biology , 64, 1–12. https://doi.org/10.1016/j.semcancer.2019.06.001
- Zhu-Shimoni, J., Yu, C., Nishihara, J., Wong, R. M., Gunawan, F., Lin, M., Krawitz, D., Liu, P., Sandoval, W., & Vanderlaan, M. (2014). Host cell protein testing by ELISAs and the use of orthogonal methods. Biotechnology & Bioengineering, 111(12), 2367–2379. https://doi.org/10.1002/bit.25327
Citing Literature
Number of times cited according to CrossRef: 1
- Yosra Helali, Cédric Delporte, Updates of the current strategies of labeling for N-glycan analysis, Journal of Chromatography B, 10.1016/j.jchromb.2024.124068, 1237 , (124068), (2024).