Mirror-Image Phage Display for the Selection of D-Amino Acid Peptide Ligands as Potential Therapeutics
Marwa Malhis, Marwa Malhis, Susanne Aileen Funke, Susanne Aileen Funke
Abstract
In neurodegenerative diseases like Alzheimer's disease (AD), endogenous proteins or peptides aggregate with themselves. These proteins may lose their function or aggregates and/or oligomers can obtain toxicity, causing injury or death to cells. Aggregation of two major proteins characterizes AD. Amyloid-β peptide (Aβ) is deposited in amyloid plaques within the extracellular space of the brain and Tau in so-called neurofibrillary tangles in neurons. Finding peptide ligands to halt protein aggregation is a promising therapeutical approach. Using mirror-image phage display with a commercially available, randomized 12-mer peptide library, we have selected D-amino acid peptides, which bind to the Tau protein and modulate its aggregation in vitro. Peptides can bind specifically and selectively to a target molecule, but natural L-amino acid peptides may have crucial disadvantages for in vivo applications, as they are sensitive to protease degradation and may elicit immune responses. One strategy to circumvent these disadvantages is the use of non-naturally occurring D-amino acid peptides as they exhibit increased protease resistance and generally do not activate the immune system. To perform mirror-image phage display, the target protein needs to be synthesized as D-amino acid version. If the target protein sequence is too long to be synthesized properly, smaller peptides derived from the full length protein can be used for the selection process. This also offers the possibility to influence the binding region of the selected D-peptides in the full-length target protein. Here we provide the protocols for mirror-image phage display selection on the PHF6* peptide of Tau, based on the commercially available Ph.D.™-12 Phage Display Peptide Library Kit, leading to D-peptides that also bind the full length Tau protein (Tau441), next to PHF6*. In addition, we provide protocols and data for the first characterization of those D-peptides that inhibit Tau aggregation in vitro. © 2024 The Authors. Current Protocols published by Wiley Periodicals LLC.
Basic Protocol 1 : Mirror image phage display selection against D-PHF6* fibrils
Support Protocol 1 : Single phage ELISA
Basic Protocol 2 : Sequencing and D-peptide generation
Basic Protocol 3 : Thioflavin-T (ThT) test to control inhibition of Tau aggregation
Support Protocol 2 : Purification of full-length Tau protein
Basic Protocol 4 : ELISA to demonstrate the binding of the generated D-peptides to PHF6* and full-length Tau fibrils
INTRODUCTION
The purpose of this method is to select D-amino acid peptide ligands for aggregation prone proteins playing a role in neurodegenerative diseases using a recombinant phage library in a procedure termed mirror-image phage display. Mirror-image phage display, a simple and quick option to select peptide ligands, has already been used for a variety of target proteins or peptides (Funke & Willbold, 2009; Schumacher et al., 1996; Wiesehan et al., 2003). D-amino acid proteins are used as targets. These will be bound by phage-presented peptides having the natural L-amino acid configuration, see Figure 1. Subsequently, the selected peptides are synthesized in D-configuration. Those D-amino acid peptides bind to the target protein in the natural L-amino acid orientation.
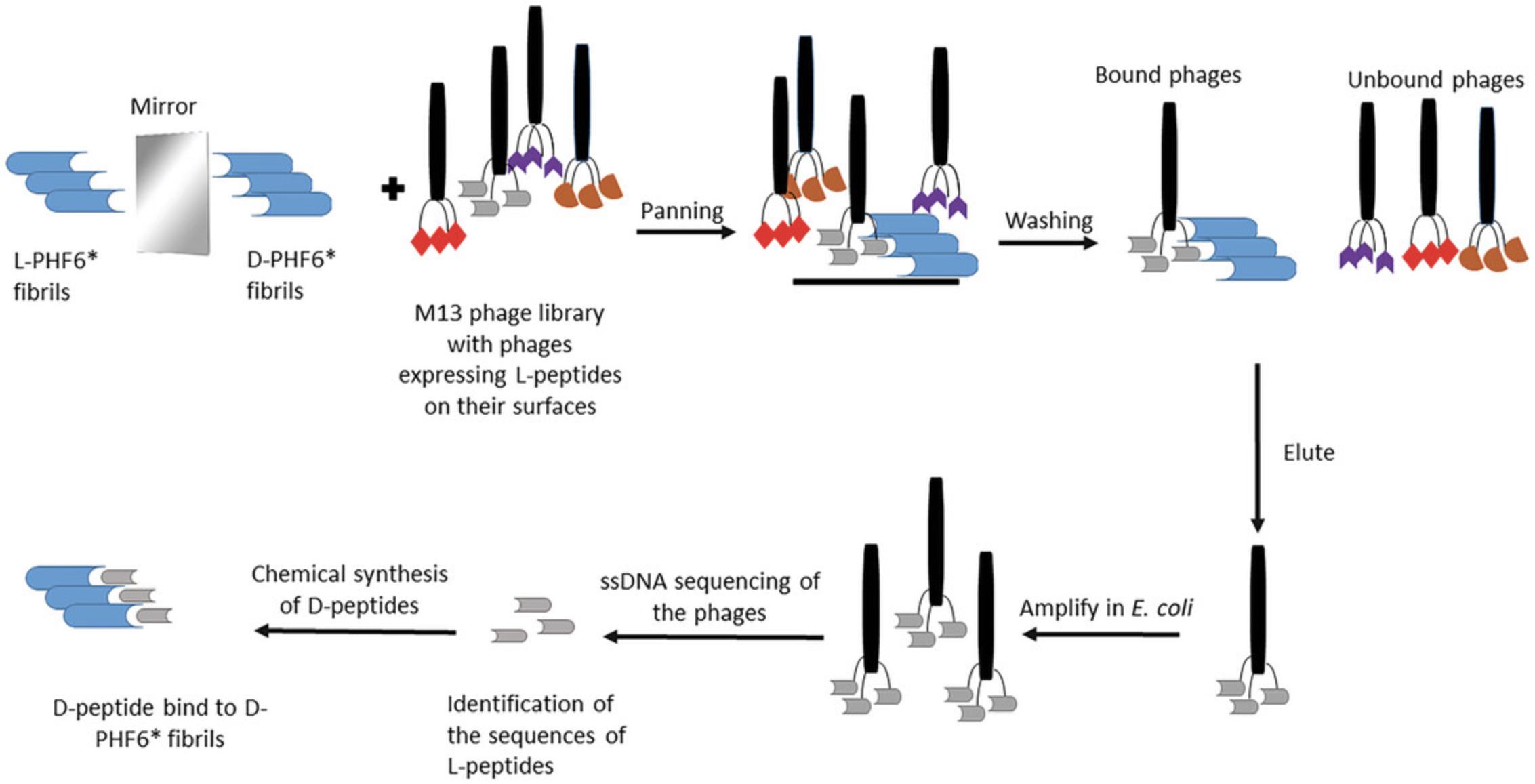
D-amino acid peptides can be alternatives to antibodies or chemical molecules. Permeability of cells and the blood-brain barrier are possible advantages of peptides over therapeutic antibodies. The latter proteins might have higher target affinity, but, in general, cross membranes often poorly (Matsson et al., 2016). As peptides can be produced synthetically, they can be optimized to fit the demands of therapeutic applications (Eustache et al., 2016; Funke & Willbold, 2012; Klein et al., 2016). D-peptides are proven to be protease stable and less immunogenic than L-peptides what makes them suitable for in vivo applications. Some have been shown to cross the blood-brain barrier in combination with high bioavailability and drug exposure to the brain (Funke et al., 2010; Leithold et al., 2016; Pappenheimer et al., 1997).
In Basic Protocol 1, we describe a mirror-image phage display selection process against D-PHF6* fibrils to obtain D-amino acid peptides binding to a critical region of the full-length Tau protein, see also Malhis et al. (2021). Tau aggregation into fibrils and filaments is driven by two hexapeptide fragments within Tau, PHF6* (275-VQIINK-280) and PHF6 (306-VQIVYK-311). These hexapeptide motifs have the highest predicted β-structure potential within the 441 amino acids of Tau, whereas point mutations in these regions lead to an in- or decrease of aggregation (Barghorn et al., 2004; von Bergen et al., 2000, 2001). Support Protocol 1 describes single-phage ELISA; this is needed to select for enriched recombinant phages whose surface-bound random peptides bind to the target. Basic Protocol 2 provides information on the subsequent phage sequencing and D-peptide synthesis. Basic Protocol 4 describes Thioflavin-T tests to control inhibition of Tau aggregation by the selected D-peptides. For those experiments, recombinant Tau protein needs to be expressed and purified; see Support Protocol 2: Purification of full length Tau protein. Basic Protocol 4 provides information on the ELISA to demonstrate the binding of the synthesized D-peptide to PHF6* and full-length Tau fibrils. For additional protocols to characterize D-peptides and the Tau interaction see Aillaud et al. (2022), Dammers et al. (2016), Malhis et al. (2021).
STRATEGIC PLANNING
We have decided to perform mirror-image phage display using a commercially available Ph.D.™-12 Phage Display Peptide Library Kit against D-PHF6* fibrils in order to obtain D-amino acid peptides binding to a critical region of the full length Tau protein. This target consists of 441 amino acid and could not be synthesized as full-length D-amino acid version (a problem discussed above). Another option to generate D-amino acid ligands by phage display is to perform regular phage display on the natural, L-amino acid targets and later synthesize and characterize retro-inverso peptides of the selected binders (Aillaud et al. 2022; Eberle et al., 2023).
Optional phage display peptide library kits would have been available by NEB. e.g., the linear heptapeptide (Ph.D.-7) or loop-constrained heptapeptide (Ph.D.-C7C) libraries, all of them having complexities on the order of 109 independent clones. The Ph.D.™ Peptide Display Cloning System allows the user to design own libraries; see Internet Resources for link. Different premade phage display peptide libraries are also available by Creative Biolabs; see Internet Resources for link.
In our panning, we decided to immobilize D-PHF6*-fibrils by direct coating on the plastic surface of the microtiter plates, using non-specific hydrophobic and electrostatic interactions. If short peptides are used as targets, coating on the plastic surface could result in inaccessible binding sites or denaturation of parts of the target. NEB provides alternative protocols, e.g., affinity-tagged targets bound to the phages in solution, followed by affinity capture of the phage-target complexes. Alternatively, the target can be captured using an affinity tag before adding the phages; see NEB instruction manual (Internet Resources for link). Here, optimization steps for the peptide binding interactions are described with respect to detergents, salts, target concentration and number of selection rounds; see Internet Resources for link. In our process, we performed single-phage ELISA and phage sequencing to obtain sequences of possible target-binding phages. Alternatively, single-stranded phage DNA can be analyzed by next generation sequencing (Santur et al. 2021).
Basic Protocol 1: MIRROR-IMAGE PHAGE DISPLAY SELECTION AGAINST D-PHF6* FIBRILS
This protocol describes mirror-image phage display selection, which was used to obtain 12-mer peptides-binders to the mirror-image form of the target protein. In our selection, the target were fibrils of the D-amino acid form of PHF6*. The protocol includes the incubation of the target-coated plate with phage library to select the phages binding via their surface-presented peptide (termed biopanning), the amplification of the binders, and after several steps of purification and precipitation, the determination of the input titer to perform another panning round (Fig. 1). The Ph.D.™-12 Phage Display Peptide Library Kit (New England BioLabs) was used according to the manufacturer's instructions; see Internet Resources. After performing four panning rounds of the selection, an ELISA was conducted to assess the success of the selection.
Materials
-
D-PHF6* (D-VQIINK, N-terminally acetylated) HPLC-purified lyophilisates with a stated purity of >95% (JPT Peptide Technologies)
-
PHF6* stock solution: 1.5 M in hexafluoro-2-propanol (HFIP; Carl Roth)
-
Coating buffer: 0.1 M NaHCO3. pH 8.6 (Carl Roth)
-
Blocking buffer: 5 mg/ml bovine serum albumin in 0.1 M NaHCO3, pH 8.6; filter through 0.22-µM filter (Carl Roth)
-
TBS: Tris-buffered saline buffer: 50 mM Tris·Cl, pH 7.5 and 150 mM NaCl; autoclave the solution (Carl Roth)
-
Ph.D.™-12 Phage Display Peptide Library Kit (New England BioLabs)
-
Elution buffer: 0.2 M Glycine-HCl (pH 2.2) +1 mg/ml BSA, filter through 0.22-µM filter (Carl Roth)
-
Neutralization buffer: 1 M Tris·Cl, pH 9.1, filter (Carl Roth)
-
LB medium (Lennox): dissolve 20 g in 1 L distilled water and autoclave (Carl Roth)
-
E. coli ER2738:
- F´ proA+ B+ lacIq Δ(lacZ)M15 zzf::Tn10(TetR)/fhuA2 glnV Δ(lac-proAB) thi-1 Δ(hsdS-mcrB)5
-
LB-Agar:1,5% (w/v) Agar-Agar in LB medium (Carl Roth)
-
LB/Tet plates: 1.5% (w/v) Agar-Agar in LB medium (autoclave the solution, cool it to below 60°C, add 0.1% (v/v) tetracycline stock, pour into 150-mm plates, and store then in dark at 4°C)
-
Top agar: 7 g/L Agar-Agar in LB-medium and autoclave (Carl Roth)
-
IPTG/Xgal stock solution: 5 g/L IPTG (isopropyl β-D-1-thiogalactopyranoside) and 4 g/L Xgal in dimethylformamide (DMF) (Carl Roth)
-
LB/IPTG/Xgal/ plates: 1.5% (w/v) Agar-Agar in LB medium, autoclave the solution then cool it to below 60°C, add 0.1 % (v/v) IPTG/X-gal, pour into 100-mm plates, and store them in dark at 4°C.
-
Tetracycline stock solution: 20 mg/ml in 1:1 ethanol:water (Carl Roth)
-
PEG/NaCl: 20% (w/v) polyethylene glycol–8000 in 2.5 M NaCl, autoclaved (Carl Roth)
-
Washing buffer TBST in the first selection round: sterile TBS + 0.1% v/v sterile filtrated Tween 20 (Carl Roth)
-
Washing buffer TBST in the following selection rounds: sterile TBS + 0.5% v/v sterile filtrated Tween 20 (Carl Roth)
-
Polystyrene 96-well microtiter plates (Greiner Bio-One, cat. no. 655101)
-
Gas permanent sealing film (Greiner Bio-One GmbH)
-
Thermomixer Comfort 5355 (Eppendorf)
-
Incubator MaxQ SHKE6000 (Thermo Fisher Scientific)
-
1.5-ml and 2-ml microcentrifuge tubes
-
100-ml and 200-ml flasks
-
Vortex mixer
-
50-ml conical tube
-
Centrifuge
Biopanning on 96-microtiterplate against PHF6* fibrils
1.Coat one well of a microtiter plate with 100 µg/ml D-PHF6* fibrils diluted in NaHCO3, cover the plate with gas permanent sealing film and incubate overnight at 4°C with 300 rpm agitation.
2.The next day, pour off the coating solution by tapping the plate face-down onto a fresh paper towel and block the well with 300 µl blocking solution and incubate for 1 hr at 4°C and 300 rpm.
3.Discard the blocking solution and wash the well six times, each time with 300 µl TBST [TBS + 0.1 (v/v) Tween-20) tapping the plate face-down onto a fresh paper towel.
4.Dilute 10 µl of Ph.D.-12TM Phage Display Library (equivalent to 1 × 1011 phages) with 100 µl TBST and pipette the phage solution onto the coated well, cover the plate with a sealing film, and incubate for 60 min at 22°C and 300 rpm.
5.Discard the unbound phages by pouring off the phage solution and slapping the plate face down on a clean paper towel and wash the well 10 times with TBST as mentioned above.
6.Elute the bound phages by reducing the pH using 100 µl elution buffer and incubate the plate for 20 min at 22°C with a shaking speed 300 rpm.
7.Pipette the bound phages into a 1.5-ml microcentrifuge tube and immediately neutralize the solution by adding 15 µl neutralization buffer.
Determination of the output titer
8.Determine the phage titer after the elution by preparing 10- to 104-fold serial dilutions of the eluted phages in LB medium.
9.In a 100 ml-flask, inoculate 10 ml LB medium with one colony E. coli ER2738 and incubate at 37°C until the culture reaches an OD600 of 0.5.
10.Prepare 10- to 104-fold serial dilutions of the eluted phages in LB medium, by mixing 10 µl from the eluted phages with 90 µl LB medium.
11.Dispense 100 µl of the E. coli ER2738 culture into 1.5-ml microcentrifuge tubes (each tube contains a different phage dilution).
12.Carry out the infection by adding 5 µl from each phage dilution to each bacterial culture tubes, vortex the tube quickly, and incubate for 5 min at RT.
13.Transfer the infected cells into sterile tubes that contain melted Top-Agar. Swiftly vortex these tubes and then pour their contents onto preheated IPTG/Xgal/LB plates.
14.Allow the plates to cool down for 5 min at RT. After that, invert them and incubate overnight at 37°C.
15.On the following day, count the blue plaques visible on the plates and calculate the resulting output titer of the phages, expressing this in plaque-forming units (pfu) per ml.
16.Use the following formula to determine the number of output phages:
Amplification of the eluate
17.In a 200-ml flask, use one colony E.coli ER2738 to inoculate 20 ml LB medium mixed with 20 µl of 20 μg/ml tetracycline and incubate at 37°C with shaking at 140 rpm until the culture reaches the early-log phase with OD600 (0.01–0.05).
18.Add the remaining eluted phages and incubate for 4.5 hr at 37°C and 130 rpm.
19.Discard the pellet and transfer the supernatant into a 50-ml conical tube. Centrifuge for 10 min at 5000 × g , 4°C, transfer the supernatant into a fresh tube, and repeat the centrifugation process under the same conditions for an additional 10 min.
20.Transfer the upper 80% from the supernatant to a new conical tube. Add 1/6 volume of PEG/NaCl, mix several times by inverting the tube, and allow the phages to precipitate overnight at 4°C.
21.On the following day, spin the tube for 50 min at 5000 × g at 4°C.
22.Decant the supernatant and suspend the fingerprint-sized phage pellet with 1 ml TBS.
23.Pipette the suspension into a 1.5-ml microcentrifuge tube and centrifuge for 5 min at 23,000 × g (14,000 rpm), 4°C, to pellet any remaining cells.
24.Pipette the supernatant into a new 1.5-ml microcentrifuge tube, add PEG/NaCl solution in a volume of 1/6 of the supernatant's volume, and incubate on ice for 60 min.
25.Spin the tube for 10 min at 23,000 × g (14,000 rpm), 4°C, to pellet the bacteriophages.
26.Suspend the phage pellet in 200 µl TBS.
27.Centrifuge the phage suspension for 1 min at 23,000 × g (14,000 rpm), 4°C.
28.Transfer the supernatant, which is the amplified eluate, into a new 1.5-ml microcentrifuge tube.
Determination of the input titer
29.Prepare 10-fold serial dilution from the amplified eluate in LB medium (from 10 to 1011).
30.Perform the input titer as previously mentioned in the output titer, plate the dilutions ranging from 108 to 1011 on plates containing IPTG/Xgal (one plate for each dilution) and allow them to incubate overnight at 37°C.
31.Count the colonies on the plates and use the plates that contain several hundred colonies to calculate the output titer.
32.Use the same formula used in the input titer to calculate the output titer in plaque-forming units (pfu) for each ml.
Further selection rounds from 2 to 4
33.Perform additional panning rounds by taking the appropriate amount of the amplified eluate from the previous round, which is equivalent to the number of phages utilized during the first round of panning (1011 pfu).
34.For the subsequent panning round, increase the Tween20 concentration in the washing buffer to 0.5%.
Support Protocol 1: SINGLE-PHAGE ELISA
A single-phage ELISA was utilized to test if a chosen phage clone bound to the target protein; see also NEB Manual (Internet Resources). In this procedure, serial dilutions were prepared from the third and fourth rounds of panning and dilutions from 106 to 109 were plated on IPTG/Xgal plates. Randomly selected blue phage clones were picked from the incubated plates and amplified in E. coli. After several precipitation and centrifugation steps, purified phage solutions were obtained and then used in single phage ELISA. The binding properties of about 96 phage clones were analyzed using this method. Figure 2 shows an example for the examination of 28 phages.
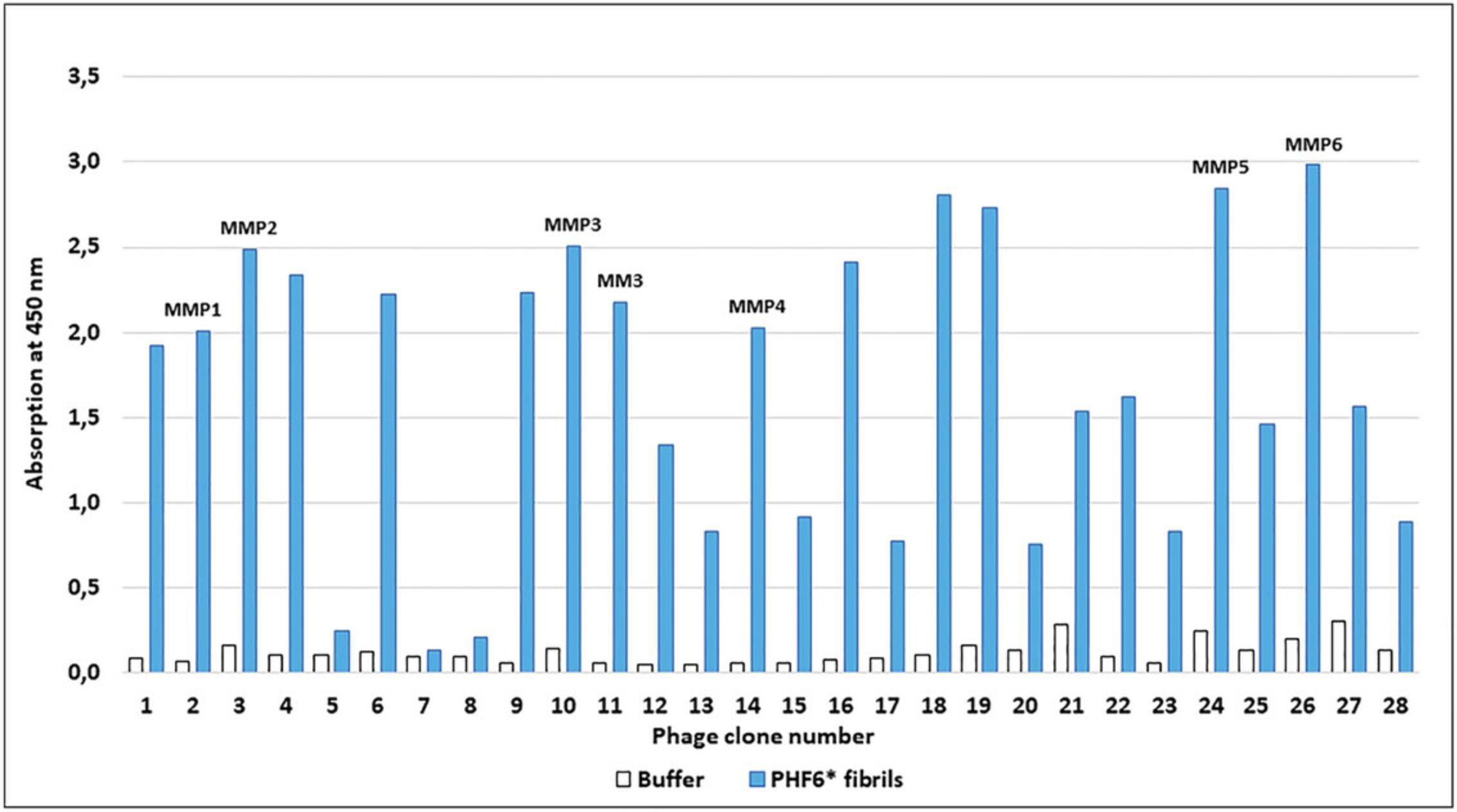
Additional Materials (also see Basic Protocol 1)
-
Amplified eluate of the third and fourth panning round (see Basic Protocol 1)
-
E. coli ER2738:
- F´ proA+ B+ lacIq Δ(lacZ)M15 zzf::Tn10(TetR)/fhuA2 glnV Δ(lac-proAB) thi-1 Δ(hsdS-mcrB)5
-
Washing buffer TBST: sterile TBS + 0.5% v/v sterile-filtrated Tween 20 (Carl Roth)
-
Anti-M13 monoclonal, HRP conjugate primary antibody (GE Healthcare, cat. no. 27-9421-01)
-
TMB Peroxidase EIA Substrate Kit (Bio-Rad Laboratories)
-
Glycerol, autoclaved (Carl Roth)
-
Plate reader Multiscan Go (Thermo Fisher Scientific, Darmstadt)
-
100-mm dish (Greiner Bio-One GmbH)
-
150-mm dish (Greiner Bio-One GmbH)
-
100- ml 200-ml and 2-L flasks
Preparation of purified phage clone solutions
1.Take 10 µl from the amplified eluate of the third and fourth panning round and perform serial dilutions in LB medium (from 101 to 109).
2.Plate the dilutions 106 to 109 on IPTG/Xgal (see determination of output titer in Basic Protocol 1, steps 8-16).
3.The next morning, inoculate 300 ml LB medium with one colony E.coli ER2738 and incubate at 37°C with 150 rpm shaking until the OD600 is between 0.1 and 0.6.
4.Dispense 15 ml from the grown culture into 50-ml conical tubes. Pick well-separated blue phage plaques from IPTG/Xgal plate using pipette tips and transfer into the culture tubes.
5.Incubate for 4.5 hr at 37°C and 150 rpm, perform steps 19-28 from Basic Protocol 1 and obtain purified phage clone solutions for single-phage ELISA.
Single-phage ELISA
6.Coat 16 wells of a 96-well plate with PHF6* fibrils in concentration of 100 µg/ml in NaHCO3, 150 µl per well. As a negative control, fill another 16 wells with only the coating buffer.
7.Cover the plate with gas permeable film and incubate in a thermomixer overnight at 4°C and 300 rpm.
8.Next day, discard the solution and block the coated wells by adding 300 µl blocking buffer and incubate for 2 hr at 4°C and 300 rpm.
9.In a separate plate, pipette 80 µl from the amplified eluate phage clone, add 80 µl from the blocking buffer to each well and pipette up and down to mix, and incubate for 20 min at 22°C and 300 rpm.
10.Discard the solutions from the first plate, and wash six times, each time with 300 µl 0.5% TBST.
11.Transfer the diluted amplified phage eluate from the second plate to the corresponding wells in the PHF6*-coated plate and incubate for 1 hr at 22°C and 300 rpm.
12.After incubation, discard the amplified phage eluates and wash the plate with TBST for 6 times.
13.Add 200 µl from HRP-conjugated anti-M13 antibody (dilution 1:5000) in blocking buffer to the wells and incubate for 1 hr at 22°C.
14.Discard the antibody solution and wash the plate six times, each time with 300 µl TBST.
15.Add 100 µl of TMB substrate solution to the wells and incubate with agitation for 15–30 min at room temperature, until the TMB produces a stable blue color.
16.Stop the enzymatic reaction by adding 100 µl of 20% H2SO4, which changes the blue color to yellow, place the plate in the plate reader, and measure the absorbance at 450 nm.
17.After performing the ELISA, store the purified solution of promising phages by adding an equal amount of sterile glycerol and store at –20°C.
Basic Protocol 2: SEQUENCING AND D-PEPTIDE GENERATION
Before sending the DNA for sequencing, it is required to isolate the DNA from the chosen phages. We used the QIAprep spin M13 Kit for the extraction of the phage DNA.
To allow proper characterization of the selected peptides, D-peptides must be synthesized to perform detailed binding and inhibition assays with controllable D-peptide concentration. This also avoids avidity artifacts associated with pentavalent display on a phage; see also NEB manual (Internet Resources) While the N-terminus of the displayed sequence was free during panning, the C-terminus was connected to the phage. D-peptides should therefore be synthesized N-terminally free and C-terminally amidated.
Additional Materials (also see Basic Protocol 1 and Support Protocol 1)
-
Phage clone eluates (see Support Protocol 1)
-
QIAprep spin M13 Kit (Qiagen)
-
Sterile water
-
-96 gIII sequencing primer, 5´–GTATGGGATTTTGCTAAACAAC–3´(Sigma-Aldrich)
-
200-ml shaking flasks
-
13-ml cell culture tubes (Sarstedt)
-
Nanodrop Multiscan Go (Thermo Fisher Scientific)
DNA extraction
1.For four phage clones, use 200-ml flask to inoculate 20 ml LB medium with one colony E. coli ER2738 and incubate at 37°C, 130 rpm until OD600 reaches 0.01-0.05.
2.Transfer 3 ml from the grown culture into 13-ml culture tubes and add 5 µl from phage clone glycerol stock and incubate for 4 hr at 37°C and 130 rpm.
3.Extract the phage DNA using QIAprep spin M13 Kit according to the instructions of the manufacturer.
4.Elute the DNA with 70 µl sterile water.
5.Measure the concentration of the ssDNA using Nanodrop Multiscan Go.
6.Send the DNA samples and -96 gIII sequencing primer in concentration of 10 pmol.
Basic Protocol 3: THIOFLAVIN-T (ThT) TEST TO CONTROL INHIBITION OF TAU AGGREGATION
To study the effect of the selected peptides on the aggregation kinetics of Tau protein, ThT test was performed. ThT is a fluorescent dye that exhibits a strong increase in fluorescence upon binding to β-sheet fibrils (Naiki et al., 1989). The measurement of the relative fluorescence indicates the amount of bound dye to amyloid fibrils. Prepared Tau solutions were mixed with ThT solution in the presence and absence of the selected peptides and the relative fluorescence was measured. Before beginning with ThT test, the conditions of the assay should be established. Tau protein does not aggregate independently in physiological conditions, so the polyanion heparin is added to the protein to induce the fibrillization (Friedhoff et al., 1998). Only D-peptide MMD3 showed a significant inhibition of PHF6* as well as Tau aggregation (Fig. 3).
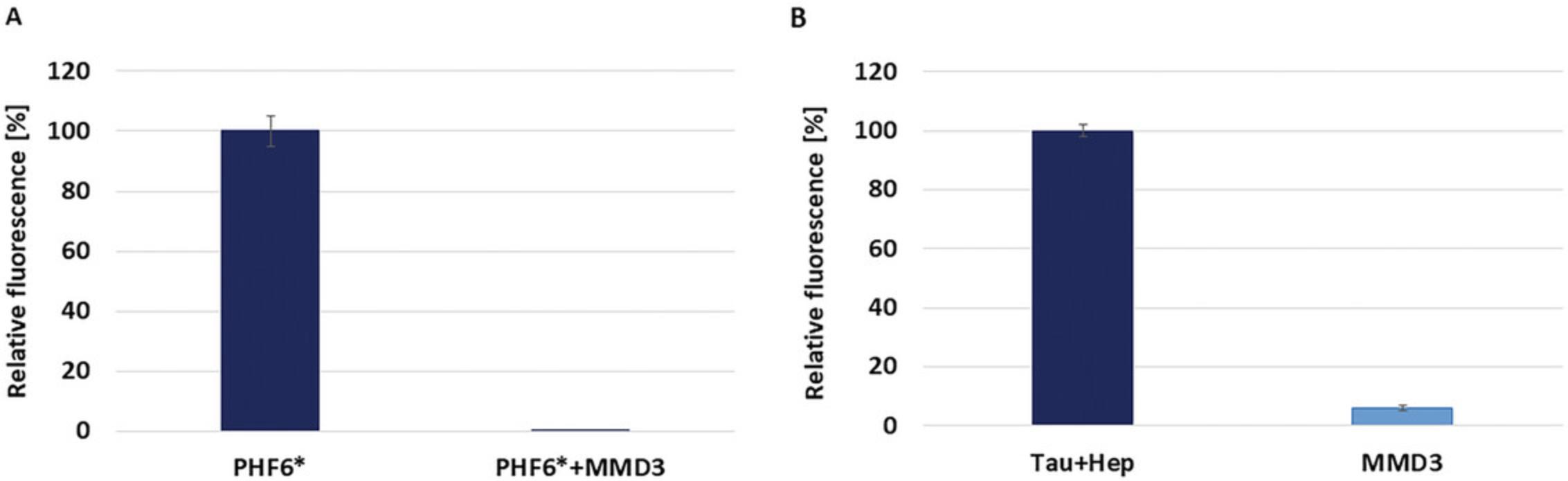
Materials
-
HEPES buffer: 20 mM HEPES, pH 6.7 (Carl Roth)
-
Full-length Tau protein (prepared using Support Protocol 2)
-
Heparin stock solution: 100 mM heparin solution (Thermo Fisher Scientific)
-
Thioflavin T stock solution: 1 mM thioflavin T in ddH2O (Merck)
-
0.5 M NaPi-buffer (sodium phosphate): mix 0.5 M Na2HPO4 (pH 9) solution with 0.5 M H2NaPO4 (pH 4) solution to final pH 7 (Carl Roth)
-
L-PHF6* (VQIINK, acetylated) HPLC-purified lyophilisates with a stated purity of >95% (JPT Peptide Technologies)
-
PHF6* stock solution: 1.5 M PHF6* in hexafluoro-2-propanol (HFIP; Carl Roth)
-
MMD3 (dplkarhtsvwy-NH2) HPLC-purified lyophilisates with a stated purity of >95% (JPT Peptide Technologies)
-
Black 96-well half area clear flat-bottom plate (Greiner Bio-One International GmbH)
-
Sealing film (Greiner Bio-One International GmbH)
-
POLARstar optima microtiter plate reader (BMG-Lab technologies)
-
Microsoft Excel 2013 (Microsoft Corp.)
Fibrillization of full-length Tau protein
1.Mix 24 µM Tau protein, 6 µM heparin, and 10 µM of ThT in HEPES buffer, as negative controls. Mix 24 µM Tau protein with 10 µM ThT in HEPES buffer without heparin and use another solution of HEPES buffer with 10 µM ThT.
2.Pipette the mixture into a black 96-well half area clear flat-bottom plate, three replicates per run. Seal the plate with sealing film and incubate for 72 hr at 37°C.
3.Measure the relative fluorescence intensity at 450/482 nm using POLARstar optima plate reader to quantify the fibrillization.
4.Calculate the mean and the standard deviations of the results using Microsoft Excel 2013.
PHF6* fibrillization inhibition assays
5.Incubate 100 μM PHF6* with 10 µM ThT in NaPi buffer, then add D-peptides MMD3 at a concentration of 1:10 (PHF6*: peptide).
6.As a positive control, incubate 100 µm PHF6* without peptides in NaPi buffer with 10 μM ThT, as a negative control, mix NaPi buffer with 10 µm ThT.
7.Pipette all samples into a 96-well black clear flat-bottom plate, cover the plate with sealing film, and incubate for 30 hr at RT.
8.Measure the relative fluorescence intensity at 450/482 nm on a POLARstar optima plate reader.
9.Calculate the mean of three absorption values, as well as the standard deviation using Microsoft Excel 2013.
10.Replicate on a new plate.
Full-length Tau protein aggregation inhibition assays
11.Mix 24 µM Tau protein with 6 µM heparin and 10 µM ThT in HEPES buffer, and then add D-peptide MMD3 in molar ratio 1:10 (Tau:peptide).
12.Incubate 24 µM Tau with 6 µM heparin and 10 µM ThT to use as positive control, as negative controls, use 24 µM Tau alone with 10 µM ThT, as well as a solution of HEPES buffer with 10 µM ThT.
13.Pipette the samples into a black 96-well clear plate and incubate for 72 hr at 37°C.
14.Measure the relative fluorescence intensity at 450/482 nm at a POLARstar plate reader.
15.Set the relative fluorescence of the positive control to equal 100% and normalize the relative fluorescence of the samples to the positive control.
16.Calculate the mean of three absorption values using Microsoft Excel 2013, as well as the standard deviation.
17.Replicate on a new plate.
Support Protocol 2: PURIFICATION OF FULL-LENGTH TAU PROTEIN
In order to test if the D-peptides inhibited the aggregation of full-length Tau, large quantities of Tau protein were needed. A method of expression and purification of full-length Tau was performed according to (Margittai & Langen, 2004) (Fig. 4).
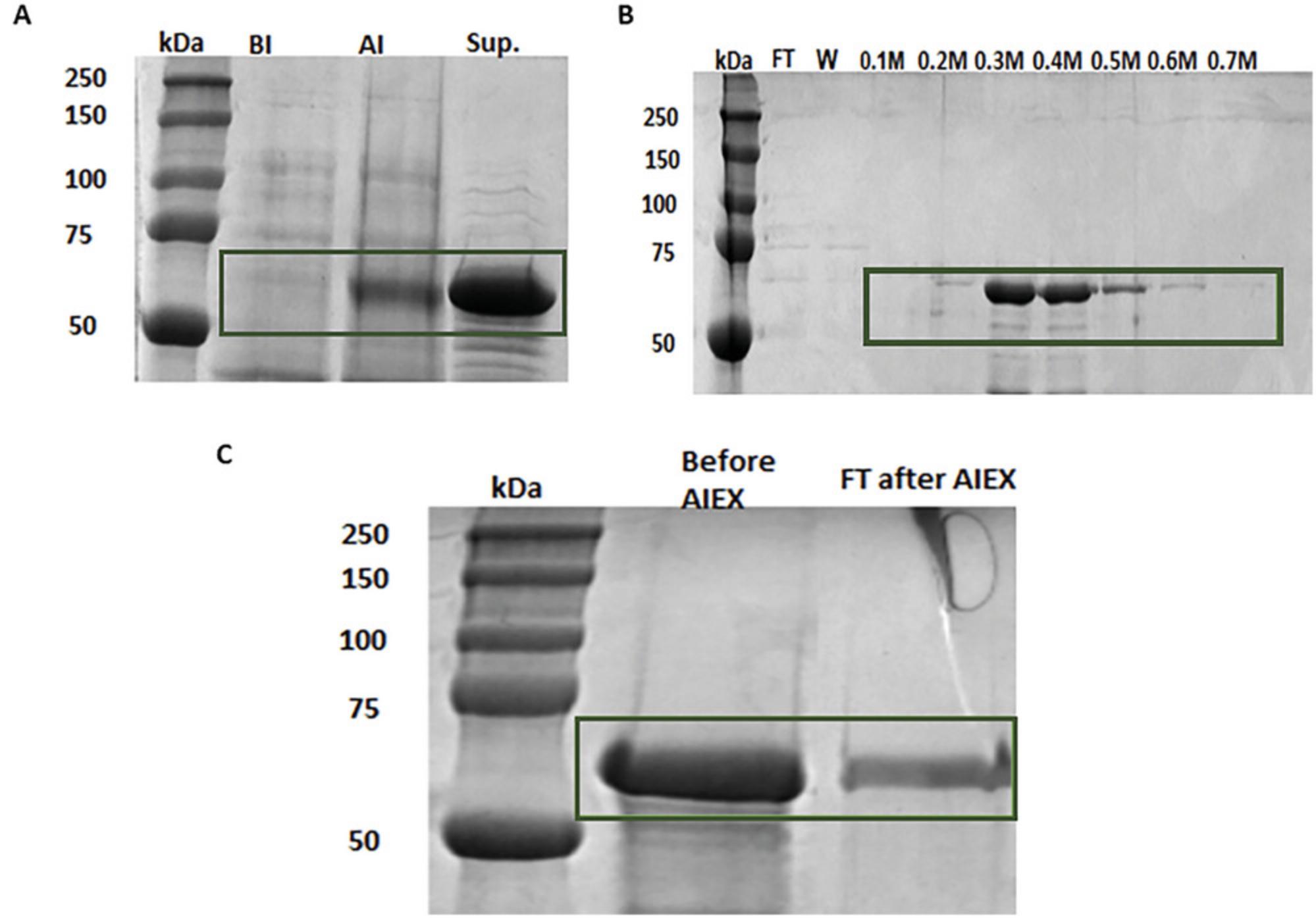
Materials
-
E. coli BL21 (DE3):
- Genotype: F– ompT gal dcm lon hsdSB(rB-mB-) λ(DE3 [lacI lacUV5-T7 gene 1 ind1 sam7 nin5])
-
LB medium (Carl Roth)
-
Calcium chloride (CaCl2) buffer, 50 mM, pH 9.0 (Carl Roth)
-
Glycerol
-
Plasmid DNA: pET28a (+)_Tau 441aa (Genentech); see also (Dammers et al., 2016)
-
Kanamycin stock solution: 50 mg/ml in H2O, filter through 0.22-µM filter (Carl Roth)
-
LB/Kan plates: 1.5% (w/v) Agar-Agar in LB medium
-
Glucose
-
Magnesium chloride (MgCl2; Carl Roth)
-
IPTG stock solution: 500 mM in H2O, filter through 0.22-µM filter (Carl Roth)
-
Lysis buffer (see recipe)
-
Ammonium sulfate (Carl Roth)
-
DTT
-
Prepacked column HiTrap SP FF (GE Healthcare) containing:
- Q Sepharose Fast Flow (GE Healthcare)
-
Buffer A: 20 mM HEPES, pH 6.8 (Carl Roth)
-
1 M NaCl, pH 6.8 (Carl Roth)
-
4× Laemmli sample buffer (BioRad)
-
12% SDS-PAGE gel
-
Coomassie Brilliant Blue G 250 staining solution (Carl Roth)
-
Destaining solution (see recipe)
-
Pierce 660 nm Protein Assay kit (Thermo Fisher Scientific)
-
Precision Plus Protein Standard Dual Color (Bio-Rad)
-
Running buffer: ROTIPHORESE®10× SDS-PAGE (Carl Roth)
-
Shaking incubator
-
Centrifuge
-
42°C water bath
-
2-L shaking flasks
-
50-ml Falcon tube
-
Fritted column
-
Amicon® Ultra-15 centrifugal filter unit with a mass weight cut-off of 10 kDa (Merck)
-
SDS-PAGE equipment
-
GelDoc XR+ Imaging System (Bio-Rad)
-
96-well microtiter plate
-
Mini-Protean® Tetra Handcast System (Bio-Rad)
Preparing of chemically competent cells
1.Inoculate 100 ml LB medium with a single colony of E. coli BL21 (DE3). Incubate the culture overnight at 37°C with gentle shaking at 120 rpm.
2.Next morning, transfer 20 ml of the overnight culture into 200 ml fresh LB medium. Incubate the culture at 37°C with continuous shaking at 140 rpm until the optical density at 600 nm (OD600) ≥0.6.
3.Centrifuge the whole culture for 20 min at 4000 × g , 4°C.
4.Carefully remove the supernatant and then resuspend the cell pellet in 20 ml of ice-cold 50 mM CaCl2 buffer. Allow the suspension to incubate on ice for 30 min.
5.Centrifuge the suspension for 10 min, 4°C.
6.Resuspend the pellet in 10 ml ice-cold 50 mM CaCl2 and allow it to incubate on ice for 15 min.
7.For long-term storage, prepare 200-µl aliquots of bacterial glycerol stocks by adding sterile glycerol to the resuspended cells to achieve a final concentration of 25%. Keep the competent cells at –80°C for future use.
Transformation of DNA into competent cells via heat shock
8.Thaw the competent cells on ice, add 1 µl from the plasmid pET28a(+)_Tau441aa. Incubate the mixture for 15 min on ice.
9.Heat-shock the cells by transferring them to a 42°C water bath for 90 s, and then rapidly cool them on ice for 2 min.
10.Bring the transformed cells up to a total volume of 1 ml using LB medium. Incubate the cells for 1 hr at 37°C and 130 rpm shaking.
11.Plate the cells onto LB/Kanamycin plates in two steps of 200 µl and 800 µl, respectively. Incubate the plates at 37°C overnight.
Expression of recombinant human Tau protein
12.Pick one transformed colony from the LB/Kanamycin plates and use it to inoculate 50 ml of LB containing 50 μg/ml kanamycin. Incubate the culture at 37°C and 130 rpm, overnight.
13.The next day, prepare 1 L of LB medium main containing 50 μg/ml Kanamycin, 0.5% glucose, and 10 mM MgCl2. Inoculate the main medium with 10 ml of the overnight culture and divide it into two 2 L shaking flasks, each containing 500 ml of the culture.
14.Incubate the culture at 37°C and shaking 150 rpm until OD600 ≥ 0.6.
15.Add IPTG solution to a final concentration of 1 mM to induce Tau protein expression. Incubate the induced culture for 3 hr at 37°C with shaking at 150 rpm.
16.After 3 hr, centrifuge the cells for 20 min at 4000 × g ,4°C.
17.Suspend the resulting pellet in 14 ml lysis buffer and store at –20°C for further use.
Extraction of the protein
18.Thaw the frozen cell pellet on ice and transfer the cell lysate into a 50-ml Falcon tube. Boil the lysate for 10 min.
19.Cool the lysate on ice for 10 min, then centrifuge for 1 hr at 5000 × g , 4°C, to remove the denatured proteins.
20.Take the supernatant, which contains Tau protein for further purification steps.
21.Take 20 µl from the supernatant and prepare it for SDS-PAGE analysis.
Ammonium sulfate precipitation
22.Add ammonium sulfate to the protein solution until a saturation level of 50%, perform the precipitation process on ice to avoid protein degradation.
23.Allow the protein to precipitate overnight at 4°C.
24.Centrifuge the solution for 1 hr at 5000 × g , 4°C, to collect the precipitated proteins.
25.Dissolve the pellet in distilled water containing 5 mM DTT and store up to several weeks at 4°C.
Cation exchange chromatography
26.Prepare the HiTrap SP FF column by equilibrating it with buffer A, following the instructions provided with the column.
27.Load the sample into the column with a flow rate of 1 ml/min.
28.Carefully load the sample into the column at a flow rate of 1 ml/min.
29.Employ a stepwise gradient elution method to elute the Tau protein. Increase the salt concentration in buffer A gradually, ranging from 0.1 M to 0.7 M NaCl.
30.During the elution process, collect fractions of 4 ml each. To monitor the elution profile, take 20-µl samples from each fraction for SDS-PAGE analysis.
Anion-exchange chromatography
31.Following the manufacturer's directions, prepare 2 ml of Q Sepharose Fast Flow slurry and transfer it to a fritted column.
32.Wash the slurry with buffer A, load the fractions that include Tau onto the column, and start the run at a flow speed of 1 ml/min.
33.Collect the flow through, which contains Tau protein.
Ultrafiltration
34.Equilibrate Amicon® centrifugal filter unit with buffer A and centrifuge for 10 min at 4000 × g , 8°C.
35.Fill the samples into the column and centrifuge at 4000 × g and 8°C until the target volume is achieved.
SDS-polyacrylamide-gel electrophoresis (SDS-PAGE)
36.Mix protein samples with 4× sample buffer, heat them for 5 min at 95°C, and then perform separation using 12% SDS-PAGE.
37.Prepare the running buffer, load 5 µl from the molecular weight marker (Precision Plus Protein Standard Dual) and 5 µl from each boiled protein sample into the wells of SDS-PAGE gel.
38.Run the gel for a duration of 30-40 min at 200 V, utilizing an electrophoresis power supply (Mini-Protean Tetra Handcast System).
Coomassie brilliant blue staining
39.Stain the gel with Coomassie blue staining solution for 20 min at room temperature (20°C).
40.Incubate the gel in destaining solution overnight to remove excess stain.
41.The next day, analyze the gel using GelDocTM XR+ Imaging System.
Determination of protein concentration
42.Determine the concentration of Tau protein using Pierce 660 nm Protein Assay kit according to the manufacturer's instructions.
43.Use BSA standard in range of 100 μg/ml to 2 mg/ml.
44.Transfer 10 µl from each BSA standard, the unknown sample, and the blank sample into a 96-well microtiter plate.
45.Add 150 µl of the working reagent to each well.
46.Allow the plate to incubate for 5 min at room temperature.
47.Create a standard curve from the absorbance values of the BSA standard and determine the concentration of Tau protein.
Basic Protocol 4: ELISA TO DEMONSTRATE THE BINDING OF THE GENERATED D-PEPTIDES TO PHF6* AND FULL-LENGTH TAU FIBRILS
An ELISA was employed to assess the binding of the MMD3 to both PHF6* fibrils, as well as full-length Tau fibrils. To conduct these experiments, the D-peptide was synthesized with FAM labeling. Following the coating of the plate with the target protein, the labeled D-peptide was pipetted onto the plate and detected with an anti-FAM-HRP. The reaction was stopped using sulfuric acid, and the absorption at a wavelength of 450 nm was measured. The obtained absorption values are then correlated with the binding of the peptide to the target.
Materials
-
PHF6* (VQIINK, acetylated) HPLC-purified lyophilisates with a stated purity of >95% (JPT Peptide Technologies)
-
Tau protein (expressed and purified as in Support Protocol 3
-
Coating buffer: NaHCO3 0.1 M pH 8.6 (Carl Roth)
-
Washing buffer PBST: PBS + 1 % Tween-20 % (v/v) (Carl Roth)
-
Bovine serum albumin (BSA)
-
Phosphate-buffered saline (PBS; see recipe)
-
MMD3-Lys(FAM)-NH2 (d-dplkarhtsvwy -lys(FAM)-NH2) (JPT Peptide Technologies)
-
Horseradish peroxidase-conjugated sheep anti-FAM antibody (Bethyl Lab., Inc., Montgomery)
-
TMB Peroxidase EIA Substrate Kit (Bio-Rad Laboratories)
-
20% H2SO4 solution (Carl Roth)
-
Polystyrene 96-well microtiter plates (Greiner Bio-One, cat. no. 655101)
-
Sealing film
-
Thermomixer Comfort 5355, (Eppendorf)
-
Plate reader Multiscan Go (Thermo Fisher Scientific, Darmstadt)
-
Microsoft Excel 2013 (Microsoft Corp.)
1.Prepare the plate by coating it with 50 μg/ml PHF6* fibrils in NaHCO3 or Tau fibrils at a concentration of 10 μg/ml in NaHCO3, pH 8.3.Use NaHCO3 buffer without the target protein as a negative control and fill the corresponding wells.
2.Cover the plate with a sealing film and incubate for 1 hr at room temperature (RT) with 300 rpm agitation.
3.Wash the plate three times, each time with 300 μl washing buffer, and then block it with 1% BSA in PBS for 1 hr at RT with 300 rpm.
4.Wash the plate three times, each time with 300 μl washing buffer.
5.Add 100 µl of FAM-labeled peptide at progressively higher concentrations (10 μg/ml, 20 μg/ml, and 50 μg/ml for PHF6* fibrils; 1 μg/ml, 5 μg/ml, 10 μg/ml, and 20 μg/ml for Tau fibrils). Incubate the plate for 1 hr at RT with 300 rpm agitation.
6.Wash the plate three times, each time with 300 μl TBST, then detect the bound phages by adding 200 µl of horseradish peroxidase-conjugated sheep anti-FITC antibody.
7.Transfer 100 µl from the TMB substrate solution to the relevant wells.
8.Stop the reaction by adding 100 µl of 20% H2SO4 solution, and then read the plate's absorbance at 450 nm.
9.Calculate the mean of three absorption values and the standard deviation using Microsoft Excel 2013.
10.Replicate as before.
REAGENTS AND SOLUTIONS
Destaining solution
- 9% methanol
- 9% acetic acid
- 9% glycerine
- Store up to several weeks at room remperature in a tightly sealed container and in the dark
Lysis buffer
- 20 mM HEPES
- Adjust the pH to 6.8 using NaOH
- Store up to 4 weeks at 4°C
- Just before use add:
- 1 mM EDTA
- 2 mM Protease inhibitor
- 2 mM of 2-Mercaptoethanol
Phosphate-buffered saline (PBS), pH 7.5
- 1,37 M NaCl
- 27 mM KCl
- 14 mM KH2PO4
- 100 mM Na2HPO4 in ddH2O
- Adjust the pH to 7.5 using HCl
- Store up to 1 month at 4°C
COMMENTARY
Background Information
Phage display is an economical and efficient approach to select target-specific ligands, e.g., for specific peptides or proteins. The molecular biology technique was established by George P. Smith in 1985 (Smith, 1985). Peptides or antibody moieties are expressed as fusion proteins with a phage surface protein on the phage surface and used in a procedure called biopanning to select binding partners. The filamentous bacteriophage M13 is commonly used for phage display because it is stable and comparatively easy to handle in the laboratory. Commonly, a gene encoding a randomized peptide is fused to the gene for coat protein pIII, leading to 5-fold expression of the peptide on the phage surface (Smith, 2023).
Peptides represent a unique class of pharmaceutical compounds and are alternatives to small chemical molecules and antibodies. In the body, they are key regulators of multiple biological pathways and execute high biological activity, specificity but comparable low toxicity (Danho et al., 2009; Lien & Lowman, 2003). While the peptides are synthesized chemically, they can easiliy be modified (e.g. addition of tags, exchange of amino acids) (Liu et al., 2016). In 2019, more than 400 peptide drugs were under clinical investigation, 60 were already approved in the USA, Europe, or Japan (Lee et al., 2019). Peptides might be unstable in the body due to protease degradation, a problem that can be overcome by chemical modification or application of D-amino acid peptides (Lee et al., 2019; Leithold et al., 2016; Liu et al., 2010). D-amino acid peptides have already been shown to be protease resistant and less immunogenic than the respective L-peptides (Chalifour et al., 2003; Sadowski et al., 2004; Schumacher et al., 1996). Peptide drugs might also have disadvantages like membrane or blood-brain barrier impermeability (Henninot et al., 2018), but at least some peptides were demonstrated to cross cell membranes or/and the blood-brain barrier (Aillaud et al., 2022; Dammers et al., 2016; Funke et al., 2010; Malhis et al., 2021; Pappenheimer et al., 1997; Zhang et al., 2020). An Aβ-binding D-peptide, denominated D3, was selected by mirror-image phage display, and reduced plaque load and cerebral inflammation of AD mouse models after oral treatment. The cognitive performance of the treated model mice was significantly increased compared to buffer-treated control mice (Funke et al., 2010). D3-derivatives showed adequate blood-brain barrier permeability and their therapeutic potential could be optimized (Jiang et al., 2015; Klein et al., 2016; Leithold et al., 2016). The D3-derivative RD2 passed a first clinical study in men (Kutzsche et al., 2020), clinical studies of phase two are under way.
Critical Parameters
Phages from the library might bind to other targets than the respective target (e.g., polystyrene, polypropylene or BSA). The D-peptides resulting from the phage display should be tested carefully for unspecific binding.
Troubleshooting
For technical issues, users can refer to the troubleshooting guide provided in Table 1 for potential solutions.
Assay | Problem | Possible cause | Solution |
---|---|---|---|
Phage display | No plaques following M13 titering | Poor bacterial growth or loss of F´ from E. coli K12 ER2738 | Streak new E. coli from new stock |
Plates show a blueish tinge | Cross-contamination or phage dilution is not enough | Use aerosol, sterile pipetting tips and be sure to work in sterile environment | |
No phage pellet after amplification | Loss of F´ from E. coli or a failure in the precipitation step | Streak new E. coli and prepare new PEG/NaCl solution | |
Clear plaques after amplifying elution pool | Contamination from the working solutions or working area | Work in sterile environment and use sterile equipment | |
No detectable ELISA signal despite consensus sequence | The selected clones have weak binding to the target | Perform more single phage ELISA and pick new clones | |
No positive results from phage ELISA | The phage library chosen for the selection is not suitable to the target or the selection conditions were inappropriate | Try another phage library and/or optimize the selection conditions | |
No specific binders | Low stringency |
Adjust the stringency of the selection by: 1. Using a higher concentration of detergent, such as Tween 20, in later rounds to reduce unspecific interactions between the phages and the target In addition, salt concentration can be increased. Nonspecific ionic interactions between surface charged groups on the target and phage, which would lead to high background binding, can be avoided. 2. Employing a lower concentration of the target 3. Increasing the number of panning rounds; usually, 3 to 4 rounds are performed. Too many rounds might lead to the unwanted selection of faster growing wild-type phages, which might contaminate the phage display selection process. Work with care to avoid contamination. |
|
ThT | Artifact formation | Sample preparation may lead to the formation of artifacts that mimic amyloid fibrils; D-peptide might form fibrils | Handle samples carefully, use appropriate controls, and validate results with additional methods to rule out artifacts |
False negatives | ThT may not detect certain amyloid structures, only fibrils are detected | Employ complementary techniques such as electron microscopy | |
Variability in ThT fluorescence | Different amyloid proteins or structures may need varying conditions for ThT; see PHF6+ and full-length Tau | Standardize the Thioflavin test protocol for each specific amyloid protein being studied and use consistent assay conditions | |
ELISA | High background | Non-specific binding of detection reagents or sample components | Block the plate with an appropriate blocking buffer, use optimal washing conditions, and optimize the concentration of detection reagents |
Low sensitivity | Insufficient detection of the target | Optimize the coating conditions and use high-affinity detection antibodies |
- a A detailed troubleshooting for the Ph.D.™-12 Phage Display Peptide Library KIT (New England BioLabs) is given in the manufacturer's manual; see Internet Resources.
Understanding Results
In order to obtain D-peptides, which inhibit the aggregation of PHF6* and Tau, we have performed mirror-image phage display selection against fibrils of D-PHF6*, for a scheme of the selection, please see Figure 1. Subsequently, single-phage ELISA was performed (Fig. 2) and purified single-phage solutions from different phage clones were obtained. The DNA of the “positive” phages, which expressed high absorption value in ELISA compared to the negative control without target, was isolated. The DNA was sent for sequencing, and after receiving the DNA data and converting the DNA sequences to amino acids, the selected peptides were determined. Several peptides were tested by employing ThT assays (Fig. 3); however, only one D-peptide showed inhibition of PHF6* fibrillization, as well as full-length Tau aggregation. MMD3 peptide was synthesized as FAM-labeled D-peptide and its binding properties to PHF6* fibrils and Tau fibrils was tested by ELISA.
Time Considerations
Basic Protocol 1: Mirror image phage display selection against D-PHF6* fibrils, for four panning rounds, 2 weeks
Support Protocol 1: Single-phage ELISA, 5 days
Basic Protocol 2: Sequencing and D-peptide generation, 1 day
Basic Protocol 3:ThT test to control inhibition of Tau aggregation, takes about 2 hr and then in case of PHF6* 35 hr of incubation, in case of Tau 72 hr of incubation
Support Protocol 2: Purification of full length Tau protein, 2 days
Basic Protocol 4: ELISA to demonstrate the binding of the generated D-peptides to PHF6* and full-length Tau fibrils, overnight incubation and then 6 hr of work
Acknowledgments
This work was supported by a grant of TechnologieAllianzOberfranken (TAO) to MM, a scholarship of Landeskonferenz der Frauenbeauftragten, Bavaria, Germany, to MM.
Open access funding enabled and organized by Projekt DEAL.
Author Contributions
Marwa Malhis : Data curation; investigation; methodology; validation; visualization; writing–original draft. Susanne Aileen Funke : Conceptualization; data curation; methodology; project administration; supervision; validation; writing–original draft; writing–review and editing.
Conflict of Interest
None.
Open Research
Data Availability Statement
Data sharing is not applicable to this article as no new data were created or analyzed in this study.
Literature Cited
- Aillaud, I., Kaniyappan, S., Chandupatla, R. R., Ramirez, L. M., Alkhashrom, S., Eichler, J., Horn, A. H., Zweckstetter, M., Mandelkow, E., Sticht, H., & Funke, S. A. (2022). A novel D-amino acid peptide with therapeutic potential, designated ISAD1, inhibits aggregation of disease relevant pro-aggregate mutant Tau and prevents Tau toxicity in vitro. Alzheimer's Research & Therapy, 14, 15. https://doi.org/10.1186/s13195-022-00959-z
- Barghorn, S., Davies, P., & Mandelkow, E. (2004). Tau paired helical filaments from Alzheimer's disease brain and assembled in vitro are based on β-structure in the core domain †. Biochemistry , 43, 1694–1703. https://doi.org/10.1021/bi0357006
- Chalifour, R. J., McLaughlin, R. W., Lavoie, L., Morissette, C., Tremblay, N., Boulé, M., Sarazin, P., Stéa, D., Lacombe, D., Tremblay, P., & Gervais, F. (2003). Stereoselective interactions of peptide inhibitors with the beta-amyloid peptide. Journal of Biological Chemistry , 278, 34874–34881. https://doi.org/10.1074/jbc.M212694200
- Dammers, C., Yolcu, D., Kukuk, L., Willbold, D., Pickhardt, M., Mandelkow, E., Horn, A. H. C., Sticht, H., Malhis, M. N., Will, N., Schuster, J., & Funke, S. A. (2016). Selection and characterization of Tau binding D-enantiomeric peptides with potential for therapy of Alzheimer disease. PLoS ONE , 11, e0167432. https://doi.org/10.1371/journal.pone.0167432
- Danho, W., Swistok, J., Khan, W., Chu, X. J., Cheung, A., Fry, D., Sun, H., Kurylko, G., Rumennik, L., Cefalu, J., Cefalu, G., & Nunn, P. (2009). Opportunities and challenges of developing peptide drugs in the pharmaceutical industry. Advances in Experimental Medicine and Biology , 611, 467–469.
- Eberle, R. J., Sevenich, M., Gering, I., Scharbert, L., Strodel, B., Lakomek, N. A., Santur, K., Mohrlüder, J., Coronado, M. A., & Willbold, D. (2023). Discovery of all-d-peptide inhibitors of SARS-CoV-2 3C-like protease. Acs Chemical Biology , 18, 315–330. https://doi.org/10.1021/acschembio.2c00735
- Eustache, S., Leprince, J., & Tufféry, P. (2016). Progress with peptide scanning to study structure-activity relationships: The implications for drug discovery. Expert Opinion on Drug Discovery , 11, 771–784. https://doi.org/10.1080/17460441.2016.1201058
- Friedhoff, P., von Bergen, M., Mandelkow, E. M., Davies, P., & Mandelkow, E. (1998). A nucleated assembly mechanism of Alzheimer paired helical filaments. Proceedings National Academy of Science USA , 95, 15712–15717.
- Funke, S. A., van Groen, T., Kadish, I., Bartnik, D., Nagel-Steger, L., Brener, O., Sehl, T., Batra-Safferling, R., Moriscot, C., Schoehn, G., Horn, A. H. C., Müller-Schiffmann, A., Korth, C., Sticht, H., & Willbold, D. (2010). Oral treatment with the D-enantiomeric peptide D3 improves the pathology and behavior of Alzheimer's disease transgenic mice. Acs Chemical Neuroscience , 1, 639–648. https://doi.org/10.1021/cn100057j
- Funke, S. A., & Willbold, D. (2009). Mirror image phage display–a method to generate D-peptide ligands for use in diagnostic or therapeutical applications. Molecular Biosystems , 5, 783–786. https://doi.org/10.1039/b904138a
- Funke, S. A., & Willbold, D. (2012). Peptides for therapy and diagnosis of Alzheimer's disease. Current Pharmaceutical Design , 18, 755–767.
- Henninot, A., Collins, J. C., & Nuss, J. M. (2018). The current state of peptide drug discovery: Back to the future? Journal of Medicinal Chemistry , 61, 1382–1414. https://doi.org/10.1021/acs.jmedchem.7b00318
- Huang, J., Ru, B., Li, S., Lin, H., & Guo, F. B. (2010). SAROTUP: Scanner and reporter of target-unrelated peptides. Journal of Biomedicine and Biotechnology , 2010, 101932. https://doi.org/10.1155/2010/101932
- Jiang, N., Leithold, L. H. E., Post, J., Ziehm, T., Mauler, J., Gremer, L., Cremer, M., Schartmann, E., Shah, N. J., Kutzsche, J., Langen, K. J., Breitkreutz, J., Willbold, D., & Willuweit, A. (2015). Preclinical pharmacokinetic studies of the tritium labelled D-enantiomeric peptide D3 developed for the treatment of Alzheimer s disease. PLoS ONE , 10, e0128553. https://doi.org/10.1371/journal.pone.0128553
- Klein, A. N., Ziehm, T., Tusche, M., Buitenhuis, J., Bartnik, D., Boeddrich, A., Wiglenda, T., Wanker, E., Funke, S. A., Brener, O., Gremer, L., Kutzsche, J., & Willbold, D. (2016). Optimization of the All-D peptide D3 for Aβ oligomer elimination. PLoS ONE , 11, e0153035. https://doi.org/10.1371/journal.pone.0153035
- Kutzsche, J., Jürgens, D., Willuweit, A., Adermann, K., Fuchs, C., Simons, S., Windisch, M., Hümpel, M., Rossberg, W., Wolzt, M., & Willbold, D. (2020). Safety and pharmacokinetics of the orally available antiprionic compound PRI-002: A single and multiple ascending dose phase I study. Alzheimer's & dementia (New York, N. Y.), 6, e12001. https://doi.org/10.1002/trc2.12001
- Lee, A. C. L., Harris, J. L., Khanna, K. K., & Hong, J. H. (2019). A comprehensive review on current advances in peptide drug development and design. International Journal of Molecular Sciences , 20, 2383. https://doi.org/10.3390/ijms20102383
- Leithold, L. H. E., Jiang, N., Post, J., Ziehm, T., Schartmann, E., Kutzsche, J., Shah, N. J., Breitkreutz, J., Langen, K. J., Willuweit, A., & Willbold, D. (2016). Pharmacokinetic properties of a novel D-peptide developed to be therapeutically active against toxic beta-Amyloid oligomers. Pharmaceutical Research , 33, 328–336. https://doi.org/10.1007/s11095-015-1791-2
- Lien, S., & Lowman, H. B. (2003). Therapeutic peptides. Trends in Biotechnology , 21, 556–562. https://doi.org/10.1016/j.tibtech.2003.10.005
- Liu, H., Funke, S. A., & Willbold, D. (2010). Transport of Alzheimer disease amyloid-beta-binding D-amino acid peptides across an in vitro blood-brain barrier model. Rejuvenation Research , 13, 210–213. https://doi.org/10.1089/rej.2009.0926
- Liu, M., Li, X., Xie, Z., Xie, C., Zhan, C., Hu, X., Shen, Q., Wei, X., Su, B., Wang, J., & Lu, W. (2016). D-peptides as recognition molecules and therapeutic agents. Chemical Record , 16, 1772–1786. https://doi.org/10.1002/tcr.201600005
- Malhis, M., Kaniyappan, S., Aillaud, I., Chandupatla, R. R., Ramirez, L. M., Zweckstetter, M., Horn, A. H. C., Mandelkow, E., Sticht, H., & Funke, S. A. (2021). Potent Tau aggregation inhibitor D-peptides selected against Tau-repeat 2 using mirror image phage display. Chembiochem , 22, 3049–3059. https://doi.org/10.1002/cbic.202100287
- Margittai, M., & Langen, R. (2004) Template-assisted filament growth by parallel stacking of tau. Proceedings National Academy of Science USA , 101, 10278–10283. https://doi.org/10.1073/pnas.0401911101
- Matsson, P., Doak, B. C., Over, B., & Kihlberg, J. (2016). Cell permeability beyond the rule of 5. Advanced Drug Delivery Reviews , 101, 42–61. https://doi.org/10.1016/j.addr.2016.03.013
- Naiki, H., Higuchi, K., Hosokawa, M., & Takeda, T. (1989). Fluorometric determination of amyloid fibrils in vitro using the fluorescent dye, thioflavin T1. Analytical Biochemistry , 177, 244–249. https://doi.org/10.1016/0003-2697(89)90046-8
- Pappenheimer, J. R., Karnovsky, M. L., & Maggio, J. E. (1997). Absorption and excretion of undegradable peptides: Role of lipid solubility and net charge. Journal of Pharmacology and Experimental Therapeutics , 280, 292–300.
- Sadowski, M., Pankiewicz, J., Scholtzova, H., Ripellino, J. A., Li, Y., Schmidt, S. D., Mathews, P. M., Fryer, J. D., Holtzman, D. M., Sigurdsson, E. M., & Wisniewski, T. (2004). A synthetic peptide blocking the apolipoprotein E/beta-amyloid binding mitigates beta-amyloid toxicity and fibril formation in vitro and reduces beta-amyloid plaques in transgenic mice. American Journal of Pathology , 165, 937–948.
- Santur, K., Reinartz, E., Lien, Y., Tusche, M., Altendorf, T., Sevenich, M., Tamgüney, G., Mohrlüder, J., & Willbold, D. (2021) Ligand-induced stabilization of the native human superoxide dismutase 1. Acs Chemical Neuroscience , 12, 2520–2528. https://doi.org/10.1021/acschemneuro.1c00253
- Schumacher, T. N., Mayr, L. M., Minor, D. L., Milhollen, M. A., Burgess, M. W., & Kim, P. S. (1996). Identification of D-peptide ligands through mirror-image phage display. Science , 271, 1854–1857.
- Smith, G. P. (1985) Filamentous fusion phage: Novel expression vectors that display cloned antigens on the virion surface. Science , 228, 1315–1317. https://doi.org/10.1126/science.4001944
- Smith, G. P. (2023). Principles of affinity selection. Cold Spring Harbor Protocols , https://doi.org/10.1101/pdb.over107894
- von Bergen, M., Barghorn, S., Li, L., Marx, A., Biernat, J., Mandelkow, E. M., & Mandelkow, E. (2001). Mutations of tau protein in frontotemporal dementia promote aggregation of paired helical filaments by enhancing local beta-structure. Journal of Biological Chemistry , 276, 48165–48174. https://doi.org/10.1074/jbc.M105196200
- von Bergen, M., Friedhoff, P., Biernat, J., Heberle, J., Mandelkow, E. M., & Mandelkow, E. (2000). Assembly of tau protein into Alzheimer paired helical filaments depends on a local sequence motif ((306)VQIVYK(311)) forming beta structure. Proceedings National Academy of Science USA , 97, 5129–5134.
- Wiesehan, K., Buder, K., Linke, R. P., Patt, S., Stoldt, M., Unger, E., Schmitt, B., Bucci, E., & Willbold, D. (2003). Selection of D-amino-acid peptides that bind to Alzheimer's disease amyloid peptide Aβ1–42 by mirror image phage display. Chembiochem , 4, 748–753. https://doi.org/10.1002/cbic.200300631
- Zhang, X., Zhang, X., Zhong, M., Zhao, P., Guo, C., Li, Y., Wang, T., & Gao, H. (2020). Selection of a d-enantiomeric peptide specifically binding to PHF6 for inhibiting Tau aggregation in transgenic mice. ACS Chemical Neuroscience , 11, 4240–4253. https://doi.org/10.1021/acschemneuro.0c00518
Internet Resources
This is NEB Homepage.
Phage Display Peptide Library Kit manual.
Homepage for Creative Biolabs.