Loading Determination of DMTr-substituted Resins for Large-scale Oligonucleotide Synthesis
Thomas Döring, Thomas Döring, Kevin Weiland, Kevin Weiland, Carolin Plattner, Carolin Plattner, Tobias Huber, Tobias Huber, Dirk Bächle, Dirk Bächle, Daniel Samson, Daniel Samson
DMTr-substituted resins
DMTr determination
oligonucleotide synthesis
substitution of resins
SPOS
solid-phase oligonucleotide synthesis
UV/vis spectroscopy
Abstract
The loading (i.e., substitution) of solid supports for oligonucleotide synthesis is an important parameter in large-scale manufacturing of oligonucleotides. Several key process parameters are dependent on the substitution of the solid support, including the number of phosphoramidite nucleoside equivalents used in the coupling step. For dimethoxytrityl (DMTr)–loaded solid supports, the substitution of the resin is determined by quantitatively cleaving the DMTr protecting group from the resin under acidic conditions and then analyzing the DMTr cation extinction by UV/vis spectroscopy. The spectrometric measurement can be performed at 409 nm or the global extinction maximum of 510 nm. The substitution is then calculated based on the Lambert-Beer law analogously to the substitution determination of Fmoc-substituted resins. Below, the determination of the molar extinction coefficient at 510 nm in a solution of 10% dichloroacetic acid in toluene and subsequent determination of the DMTr loading of DMTr-substituted resins is reported. © 2024 The Authors. Current Protocols published by Wiley Periodicals LLC.
Basic Protocol 1 : Determination of the molar extinction coefficient at 510 nm in DCA Deblock solution
Basic Protocol 2 : Substitution determination of DMTr-substituted resins by cleavage of the DMTr cation
INTRODUCTION
Since the establishment of phosphoramidite-based solid-phase oligonucleotide synthesis by Beaucage and Caruthers (1981), the dimethoxytrityl (DMTr) protecting group has quickly gained importance and is now the dominantly used protecting group for the C5 terminus of phosphoramidite starting materials and solid supports (Beaucage and Caruthers, 1981; Nielsen et al., 1986; Stutz et al., 2000; Krotz et al., 2006; Tram et al., 2011). The DMTr protecting group can be part of the linker moiety of the solid support or can be introduced by coupling a DMTr-protected oligonucleotide onto a functionalized polymeric or glass support. The acid-labile DMTr protecting group is quantitatively cleaved (Fig. 1) by treatment with, for example, 10% dichloroacetic acid in toluene (DCA Deblock), which forms a brightly colored DMTr cation in solution. In DCA Deblock, the cation exhibits two distinct absorption maxima at 409 and 510 nm. Absorption values at either of these maxima can be used to determine the resin substitution by employing the Lambert-Beer law in combination with the respective molar absorption coefficient. Although there is some literature on the molar extinction coefficient of the DMTr cation (ε) in solution (Guzaev and Pon, 2013), there is, to the best of our knowledge, no data for this value in DCA Deblock. The absorption maximum at 510 nm is the global maximum of the absorption spectrum and will henceforth be discussed to calculate the substitution of DMTr-protected resins and glass supports (Fig. 2).
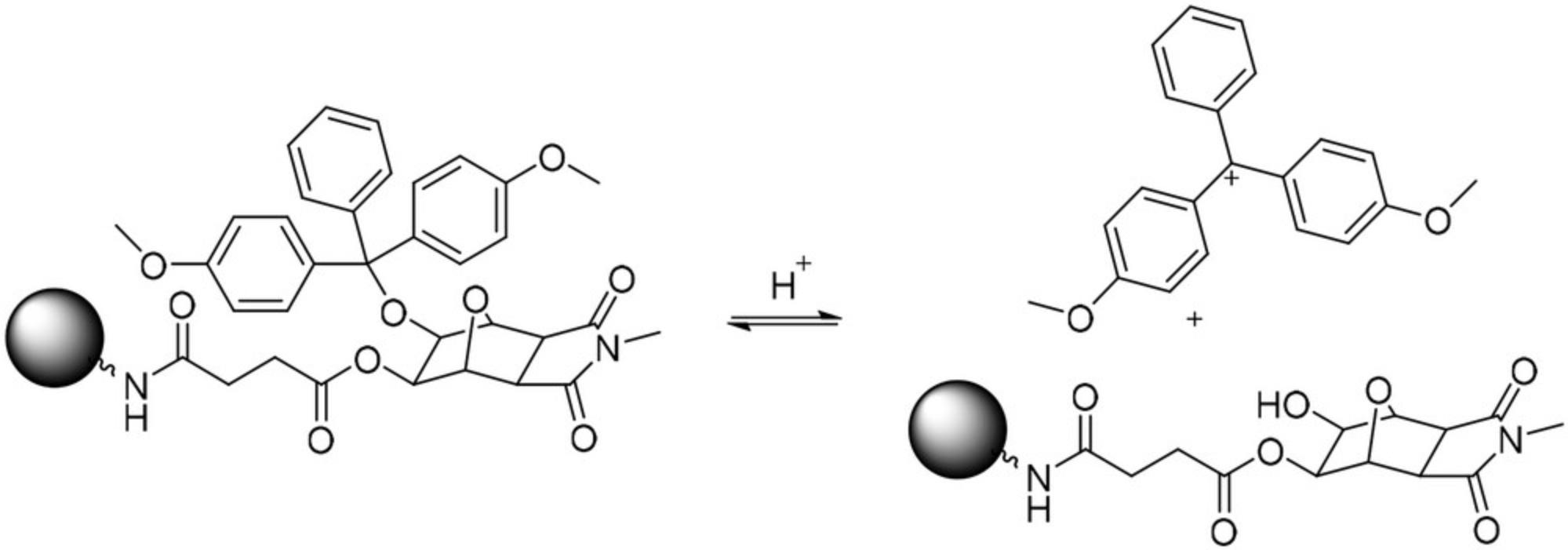
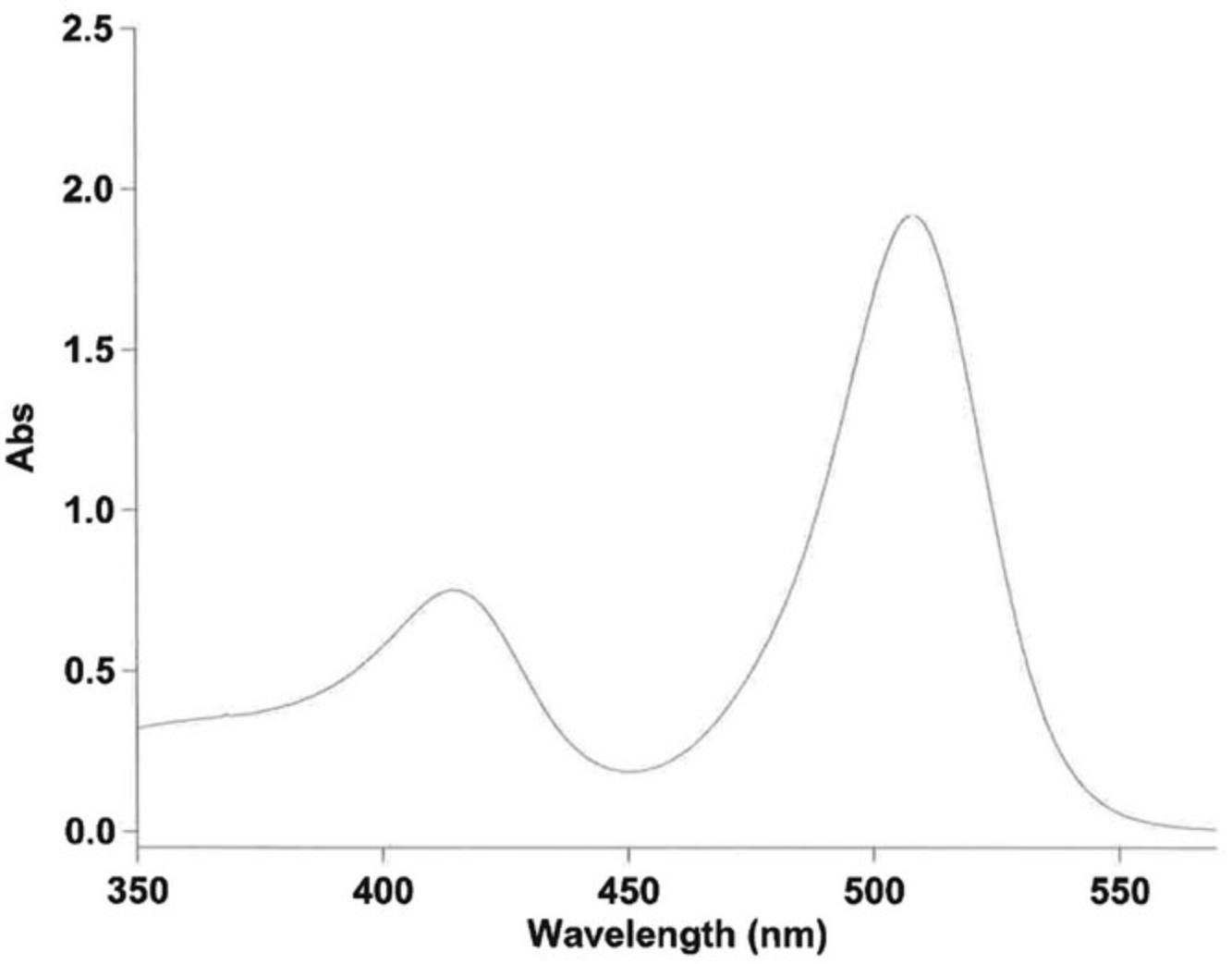
A reliable and robust method for loading determination is of utmost importance, as the determined substitution of a resin defines the batch size and various batch-size-dependent process parameters such as the equivalents of phosphoramidite nucleoside to use, the calculated step yields, and more. Furthermore, resin substitution is an important stability-indicating attribute of the starting resin that must be considered for large-scale oligonucleotide synthesis. The exact and reliable determination of resin substitution directly impacts the economy of the synthesis and potentially the quality of drug substances produced.
Basic Protocol 1: DETERMINATION OF THE MOLAR EXTINCTION COEFFICIENT AT 510 nm IN DCA DEBLOCK SOLUTION
The molar extinction coefficient (ε) can be determined by calculating the slope of a linear regression using the relationship between the absorption of the DMTr cation at 510 nm and different concentration levels. Here, the DMTr cation is released by acid cleavage with 10% DCA in toluene.
Materials
- 4,4′-Dimethoxytriphenylmethyl chloride (DMTr-Cl; Sigma-Aldrich, cat. no. 38827)
- 10% (v/v) dichloroacetic acid (DCA) in toluene (DCA Deblock, BioInnovas, cat. no. NC-0409)
- 100-ml glass volumetric flasks
- Glass volumetric pipettes
- 1-cm-pathlength quartz cuvettes (Hellma Suprasil 100-QS or equivalent)
- UV/vis spectrophotometer (e.g., Shimadzu UV-1800 or equivalent)
NOTE : The indicated DMTr-Cl is a solid pink powder that has a specified purity of ≥ 97.0% (HPLC) by the supplier and a controlled purity of ≥ 98% (HPLC) by Bachem QC-Laboratories.
1.Prepare a stock solution of DMTr cation by dissolving 15 mg DMTr-Cl (338.83 g/mol) in DCA Deblock in a 100-ml volumetric flask.
2.Prepare a dilution series with six different concentration levels by transferring varying amounts (1, 2, 4, 6, 8, and 10 ml) of stock solution to individual 100-ml volumetric flasks, utilizing volumetric pipettes.
3.Fill each flask to the 100-ml mark with DCA Deblock and mix well.
4.Transfer an aliquot of the first solution to a quartz cuvette with an optical path length of 1 cm.
5.Set the slit width of the UV/vis spectrophotometer to 1 nm and the wavelength to 510 nm.
6.Set the spectrophotometer to zero by using DCA Deblock as a blank.
7.Place the cuvette in a UV/vis spectrometer and determine the extinction at 510 nm.
8.Repeat to take measurements from duplicate aliquots at each concentration.
9.Determine the mean for each pair of duplicates.
10.Assess linearity by evaluating the coefficient of determination, the slope of the regression line, the y intercept of the regression line, and the residual sum of squares from the mean value at each concentration by linear regression from the extinction vs. the concentration (in M).
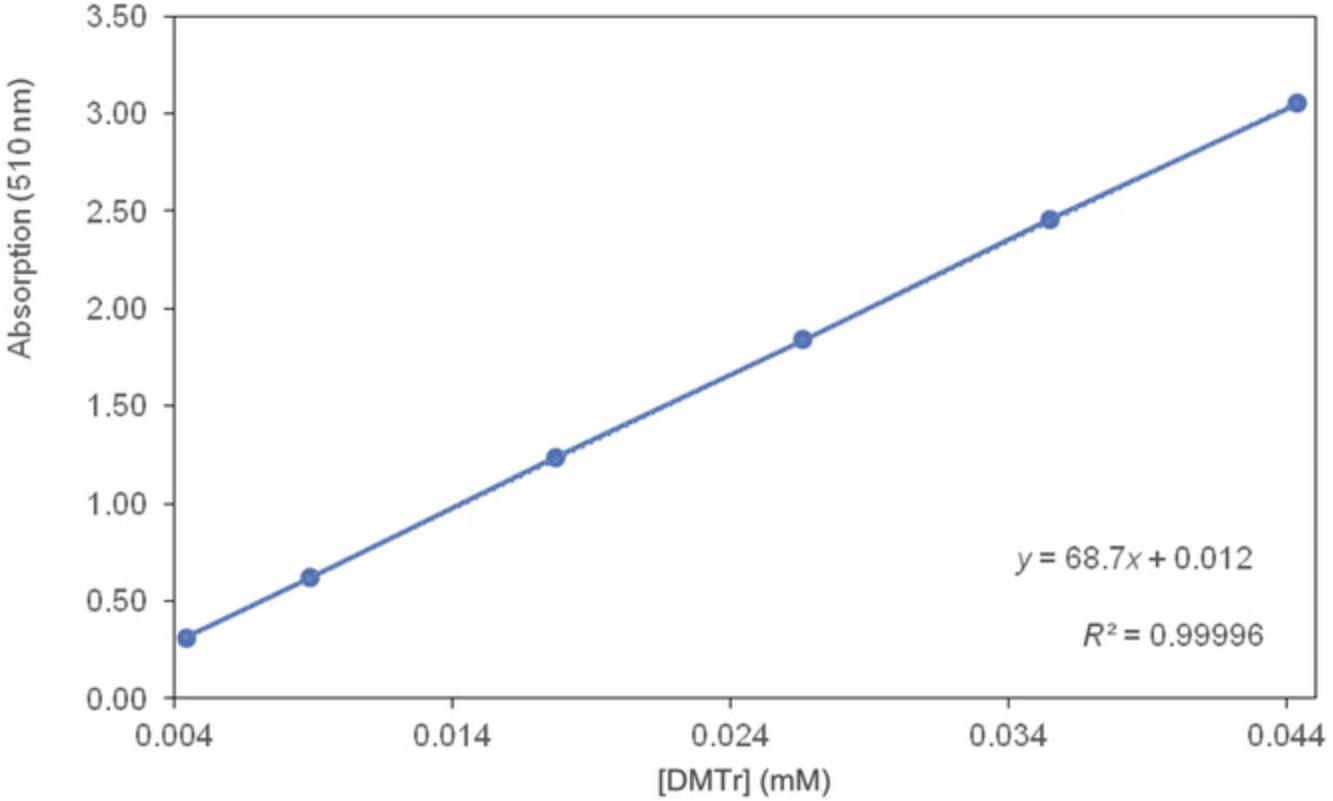
Basic Protocol 2: SUBSTITUTION DETERMINATION OF DMTr-SUBSTITUTED RESINS BY CLEAVAGE OF THE DMTr CATION
The substitution determination of DMTr-substituted resins is based on acidic hydrolyzation and is determined here as previously described by Eissler et al. (2017). Addition of acid to the resin results in an immediate change of the solution to an orange color resulting from release of the DMTr cation. The extinction can be measured by a spectrophotometer at 510 nm. The materials needed are the same as in Basic Protocol 1.
1.Weigh 100 ± 10 mg of DMTr-substituted resin or glass support in a 100-ml glass volumetric flask. Record the weight to an accuracy of 0.1 mg.
2.Add 50 ml DCA Deblock to the flask, seal with a plug, and mix for 40 min on a shaker at low speed (200 rpm).
3.Fill the flask to the mark with DCA Deblock and allow the resin settle for 10 min (without shaking).
4.Transfer 5 ml supernatant (cleave solution) to a 100-ml volumetric flask, fill to the mark with DCA Deblock (20-fold dilution), and shake well by hand.
5.Set the slit width of the UV/vis spectrophotometer to 1 nm and the wavelength to 510 nm.
6.Set the spectrophotometer to zero using DCA Deblock as a blank.
7.Transfer an aliquot of diluted cleavage solution (step 4) to a quartz cuvette and measure the extinction of the solution (E 510nm) .
8.Calculate the DMTr substitution (loading) of the resin (S DMTr in mmol/g) using the formula:
- E 510nm = extinction of diluted cleavage solution in step 8
- V = volume of cleavage solution (0.100 L)
- D = dilution factor (20)
- ε510nm = molar extinction coefficient of DMTr cation at 510 nm (68.7 L mmol–1 cm–1; see Basic Protocol 1)
- m = weight of resin in step 1 (g)
- l = cuvette pathlength (1 cm)
Support | Vendor | Measured value (µmol/g resin) | Specified value (µmol/g resin) |
---|---|---|---|
DMTr-2′-O-Methyl-C(Ac)-CPG (600 Å) | A | 75 | 83 |
DMTr-2′-O-Methyl-C(Ac)-CPG (600 Å) | A | 74 | 84 |
DMTr-2′-O-Methyl-C(Ac)-PS | B | 228 | 247 |
DMTr-2′-O-Methyl-C(Ac)-PS | B | 227 | 255 |
DMTr-2′-O-Methyl-G(iBu)-PS | B | 312 | 357 |
DMTr-2′-O-Methyl-A(Bz)-PS | B | 324 | 351 |
HL C6-Phthalimido 250 | B | 250 | 264 |
HL Unylinker 350 | B | 300 | 348 |
HL Unylinker 300 | B | 270 | 293 |
HL Unylinker 200 | B | 185 | 198 |
- a
Data from material with the same label originates from different batches,
COMMENTARY
Background Information
A reliable and easy-to-use method for determining the loading of DMTr-substituted resins and glass supports is described here. The method operates in quality-control investigations, reviewing data on certificates of analysis from suppliers of DMTr-substituted solid supports. The spectrum of the DMTr cation reveals two maxima. Due to the high purity of the DMTr-Cl standard, we conclude that both peak maxima originate from the 4,4′-dimethoxytriphenylmethyl chloride in the DCA Deblock. The absorption and peak maxima of the DMTr cation depend on the acid and solvent used. Therefore, the molar extinction coefficient cannot be directly compared with the value published by Guzaev and Pon (2013), who used a trifluoroacetic acid/dichloromethane solution.
It is obvious that the measured values presented in Table 1 are systematically lower by a factor of ∼0.9 when compared to certified supplier values. The ratio seems to be independent of support material (polystyrene or controlled-pore glass) and other material specifications. A possible reason is described below (see Troubleshooting).
Critical Parameters
Acid strength, hydrolysis time, and sample amount are critical parameters. No relevant deviation was observed when the acid strength was varied in the range of 6% to 14%. If modifications are necessary, blank corrections must be performed with the same solution. As cleavage by DCA Deblock is a very rapid process, the effect of hydrolysis time is negligible within a range of 20 to 80 min. The sample amount can vary between 50 and 200 mg resin without any influence on the result. The purity of the DMTr-Cl standard used for determination of the molar extinction coefficient must be as high as possible. Analysis by HPLC revealed a high purity (≥98%) for the DMTr-Cl standard, with impurities <0.6% for any detectable impurity.
Troubleshooting
Upon molar extinction coefficient determination, it is noteworthy that the DMTr cation with a molar mass of 303 g/mol is measured, whereas the commercially available DMTr-Cl with a molar mass of 338 g/mol gross is weighted.
Understanding Results
In Figure 3, the extinction is plotted against molar concentration, which is given in mM. The intercept is negligible, and the coefficient of determination is better than 0.99. The method is originally developed for DMTr-substituted solid supports with a specified loading of 350 µmol/g resin. The range of extinction values was therefore chosen from 0.34 to 3.7. To investigate resins with other (e.g., lower) loadings, the sample amount can be varied as described in Basic Protocol 1. The relative standard deviation is smaller than 1%.
Time Considerations
The time required for both protocols is ∼2 hr.
Acknowledgments
We appreciate the valuable discussion and input from Teuta Kukaj, Denis Ackermann, Natascha Knab-Buser, Karen Torres, Dominik Burger, and Pascal Heimer.
Author Contributions
Thomas Doring : Conceptualization; data curation; formal analysis; writing—review and editing. Kevin Weiland : Conceptualization; data curation; writing—original draft. Tobias Huber : Conceptualization; supervision; writing—review and editing. Carolin Plattner : Validation; visualization. Dirk Bächle : Supervision. Daniel Samson : Project administration; writing—review and editing.
Conflict of Interest
The authors declare no conflict of interest.
Open Research
Data Availability Statement
The data that support the findings of this study are available from the corresponding author upon request.
Literature Cited
- Beaucage, S. L., & Caruthers, M. H. (1981). Deoxynucleoside phosphoramidites A new class of key intermediates for deoxypolynucleotide synthesis. Tetrahedron Letters , 22(20), 1859–1862. https://doi.org/10.1016/S0040-4039(01)90461-7
- Eissler, S., Kley, M., Bächle, D., Loidl, G., Meier, T., & Samson, D. (2017). Substitution determination of Fmoc-substituted resins at different wavelengths. Journal of Peptide Science , 23(10), 757–762. https://doi.org/10.1002/psc.3021
- Guzaev, A. P., & Pon, R. T. (2013). Attachment of nucleosides and other linkers to solid-phase supports for oligonucleotide synthesis. Current Protocols in Nucleic Acid Chemistry , 3.2.1–3.2.23. https://doi.org/10.1002/0471142700.nc0302s52
- Krotz, A. H., Cole, D. L., & Ravikumar, V. T. (2006). Dimethoxytrityl removal in organic medium: Efficient oligonucleotide synthesis without chlorinated solvents. Nucleosides, Nucleotides & Nucleic Acids, 18, 1207–1209. https://doi.org/10.1080/07328319908044664
- Nielsen, J., Taagaard, M., Dahl, O., Marugg, J. E., & van Boom, J. H. (1986). Polymer-supported synthesis of deoxyoligonucleotides using in-situ prepared deoxynucleoside 2-cyanoethyl phosphoramidites. Recueil des Travaux Chimiques des Pays-Bas , 105(1), 33–34. https://doi.org/10.1002/recl.19861050106
- Stutz, A., Hörbartner, C., & Pitsch, S. (2000). Novel fluoride-labile nucleobase-protecting groups for the synthesis of 3′(2′)-O -aminoacylated RNA sequences. Helvetica Chemica Acta , 83(9), 2477–2503. https://doi.org/10.1002/1522-2675(20000906)83:9<2477::AID-HLCA2477>3.0.CO;2-9
- Tram, K., Sanghvi, Y. S., & Yan, H. (2011). Further optimization of detritylation in solid-phase oligodeoxyribonucleotide synthesis. Nucleosides, Nucleotides & Nucleic Acids, 30, 12–19. https://doi.org/10.1080/15257770.2010.537291