Let's Get Rolling: Precise Control of Microfluidic Assay Conditions to Recapitulate Selectin-Mediated Rolling Interactions of the Leukocyte Adhesion Cascade
Zeinab Amoabediny, Zeinab Amoabediny, Aman Mittal, Aman Mittal, Subham Guin, Subham Guin, Alexander Buffone Jr, Alexander Buffone Jr
Abstract
The leukocyte adhesion cascade governs the recruitment of circulating immune cells from the vasculature to distal sites. The initial adhesive interactions between cell surface ligands displaying sialyl-LewisX (sLeX) and endothelial E- and P-selectins serve to slow the cells down enough to interact more closely with the surface, polarize, and exit into the tissues. Therefore, precise microfluidic assays are critical in modeling how well immune cells can interact and “roll” on selectins to slow down enough to complete further steps of the cascade. Here, we present a systematic protocol for selectin mediated rolling on recombinant surfaces and endothelial cell monolayers on polyacrylamide gels of varying stiffness. We also describe step-by-step the protocol for setting up and performing the experiment and how to analyze and present the data collected. This protocol serves to simplify and detail the procedure needed to investigate the initial selectin-mediated interactions of immune cells with the vasculature. © 2024 The Authors. Current Protocols published by Wiley Periodicals LLC.
Basic Protocol 1 : Preparing dishes for cell rolling experiments
Basic Protocol 2 : Fabrication of polyacrylamide gels for cell rolling experiments
Alternate Protocol 1 : Protein conjugation with N6 linker
Alternate Protocol 2 : HUVEC culturing for monolayers
Basic Protocol 3 : Conducting cell rolling experiments on polyacrylamide gels
Basic Protocol 4 : ImageJ analysis of cell rolling movies
Basic Protocol 5 : Quantification of Fc site density on a surface (e.g., for Fc chimeras)
INTRODUCTION
Immune cell recruitment to sites of inflammation is a hallmark of the innate immune response. Leukocyte recruitment occurs in discrete steps, commonly known as the leukocyte adhesion cascade (Fig. 1). Initially, cells are slowed by selectin-mediated interactions, allowing for chemokine-induced activation of integrins, firm adhesion, and then transmigration across the endothelial layer (Ley et al., 2007; Springer, 1994). Neutrophils are the so-called “first responders” and will traffic to the sites of inflammation immediately upon sensing the inflammatory insult (Jenne et al., 2018). At the outset of the adhesion cascade, human neutrophils bind and roll on P- and L-selectins through a specific sialyl-LewisX (sLeX) decorated O-glycan presented by P-selectin Glycoprotein Ligand-1 (PSGL-1) (Buffone et al., 2013; Malý et al., 1996; Mondal et al., 2014; Wilkins et al., 1996). Afterwards, a variety of sLeX-decorated glycoproteins (Chase et al., 2012; Hidalgo et al., 2007) and glycolipids (Mondal et al., 2016; Nimrichter et al., 2008) are utilized to bind to endothelial E-selectin in order to slow the neutrophil enough to interact with chemokine expressed on both the endothelial surface and in solution. Chemokine stimulation by CXCL12 or CXCL8 (IL-8) leads to intracellular signaling cascades activated trough their cognate cell-surface receptors, CXCR1, 2, or 4 (Eash et al., 2010; Kolaczkowska & Kubes, 2013; Yoshimura, 2007). Chemokine ligation induces activation of three integrins, Macrophage-1 Antigen (Mac-1, αMβ2), Lymphocyte Functional Antigen-1 (LFA-1, αLβ2), and Very Late Antigen-4 (VLA-4, α4β1), to adopt high-affinity states that lead to arrest on the apical surface of the endothelium (Luo et al., 2015). Once adherent, neutrophils migrate along apical endothelium that expresses the cell adhesion molecules (CAMs), ICAM-1 and VCAM-1 (Huttenlocher & Horwitz, 2011), before they crawl through the endothelium to reach the basal lamina and the site of infection (Rowe & Weiss, 2008).
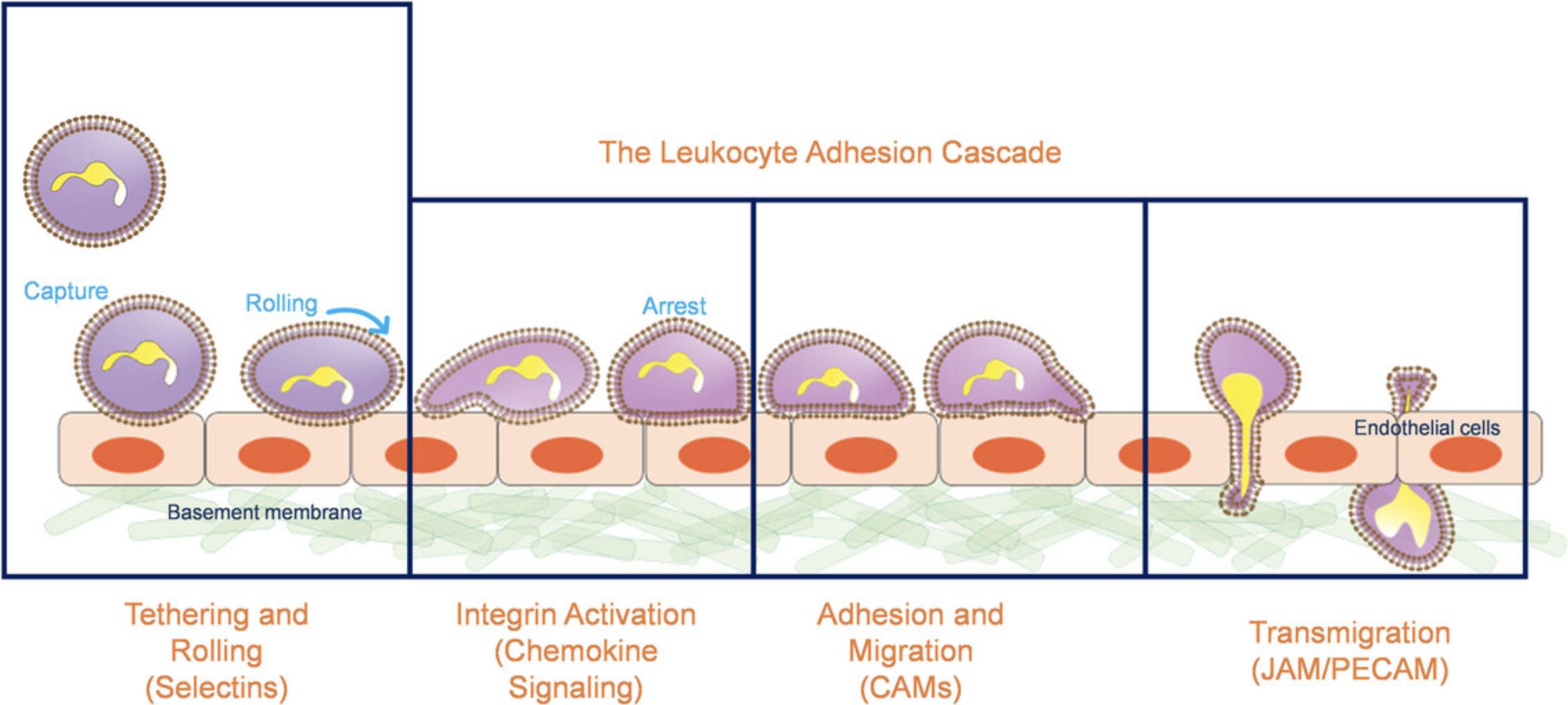
The initial selectin interactions of the cascade are of critical importance, as it determines whether the immune cell can slow enough to elicit the further activation, crawling, and transmigration steps of the cascade. These selectin-mediated interactions are primarily mediated by the interaction between the immune cell borne sLeX glycan and endothelially expressed E- and P-selectin (Buffone et al., 2013, 2017; Lo, Antonopoulos, Dell et al., 2013; Lo, Antonopoulos, Gupta et al., 2013; Lo et al., 2016; Marathe et al., 2010; Mondal et al., 2013, 2014, 2016, 2018; Morikis et al., 2017; Stolfa et al., 2016). Owing to the complexity of the leukocyte adhesion cascade, precisely engineering microfluidic assays that recapitulate this initial selectin mediated interactions are critically important.
Thus, the following protocols delineate methodologies to measure cell rolling by describing the process for Petri dish preparation (Basic Protocol 1), polyacrylamide gel fabrication and functionalization to conduct neutrophil rolling experiments in parallel-plate flow chamber (Basic Protocols 2 and 3, Alternate Protocol 1), and neutrophil tracking and analysis (Basic Protocols 4 and 5). With the capacity to probe other cell types, this protocol is valuable in understanding and analyzing not only neutrophil rolling under shear flow but also the behavior of other leukocytes under similar or slightly modified conditions.
Basic Protocol 1: PREPARING DISHES FOR CELL ROLLING EXPERIMENTS
This protocol explores when immune cells initially slow down, roll, and interact with selectin ligands on the endothelial surface during inflammation. Particularly, these experiments were developed for neutrophil interactions in response to inflammatory cues such as IL-1β. Our experimental analyses employ an optimized in vitro flow chamber assay specifically tailored to study HL-60 and primary neutrophils attachment and rolling behavior on protein-coated plastic dishes. Note this protocol can be similarly adapted to measure immune cell crawling on ICAM-1 and VCAM-1 (Buffone et al., 2018, 2019, 2023).
Materials
-
70% ethanol (Sigma-Aldrich, cat. no. 459836)
-
Recombinant protein A/G (Thermo Fisher Scientific Pierce, cat. no. 21186)
-
Phosphate-buffered saline (PBS) (Cytiva HyClone, cat. no. SH30256.01)
-
Chemokine, optional
-
Recombinant human proteins:
- P-selectin (R&D Systems, cat. no. ADP3-050)
- E-selectin (R&D Systems, cat. no. ADP1-050)
- ICAM-1 (R&D Systems, cat. no. ADP4-050)
- VCAM-1 (R&D Systems, cat. no. ADP5-050)
- PD-1 Fc chimeras (R&D Systems, cat. no.1086-PD-050)
- CXCL12 (ThermoFisher Scientific, cat. no. A42506)
-
1% bovine serum albumin in PBS (Sigma-Aldrich, cat. no. A5611)
-
flexiPERM gaskets (Sarstedt, cat. no. 94.6032.039)
-
50-ml conical tube
-
35-mm Petri dishes (Corning, cat. no. 430588)
-
Tweezers (Fisher Scientific, cat. no. 12-000-122)
-
Refrigerator (Fisher Scientific, cat. no. FBG30RSGA), 4°C
-
Rocking table (Fisher Scientific, cat. no. 02-217-765)
1.Submerge gaskets in 70% ethanol (e.g., within 50-ml conical tube) and sonicate for 5 to 10 min to clean the gaskets.
2.Let gaskets dry in a tissue culture hood.
3.Place each gasket in the middle of a Petri dish firmly to create a seal. On the bottom of the dish, mark the position of the gasket by scraping it with tweezers (Fig. 2).
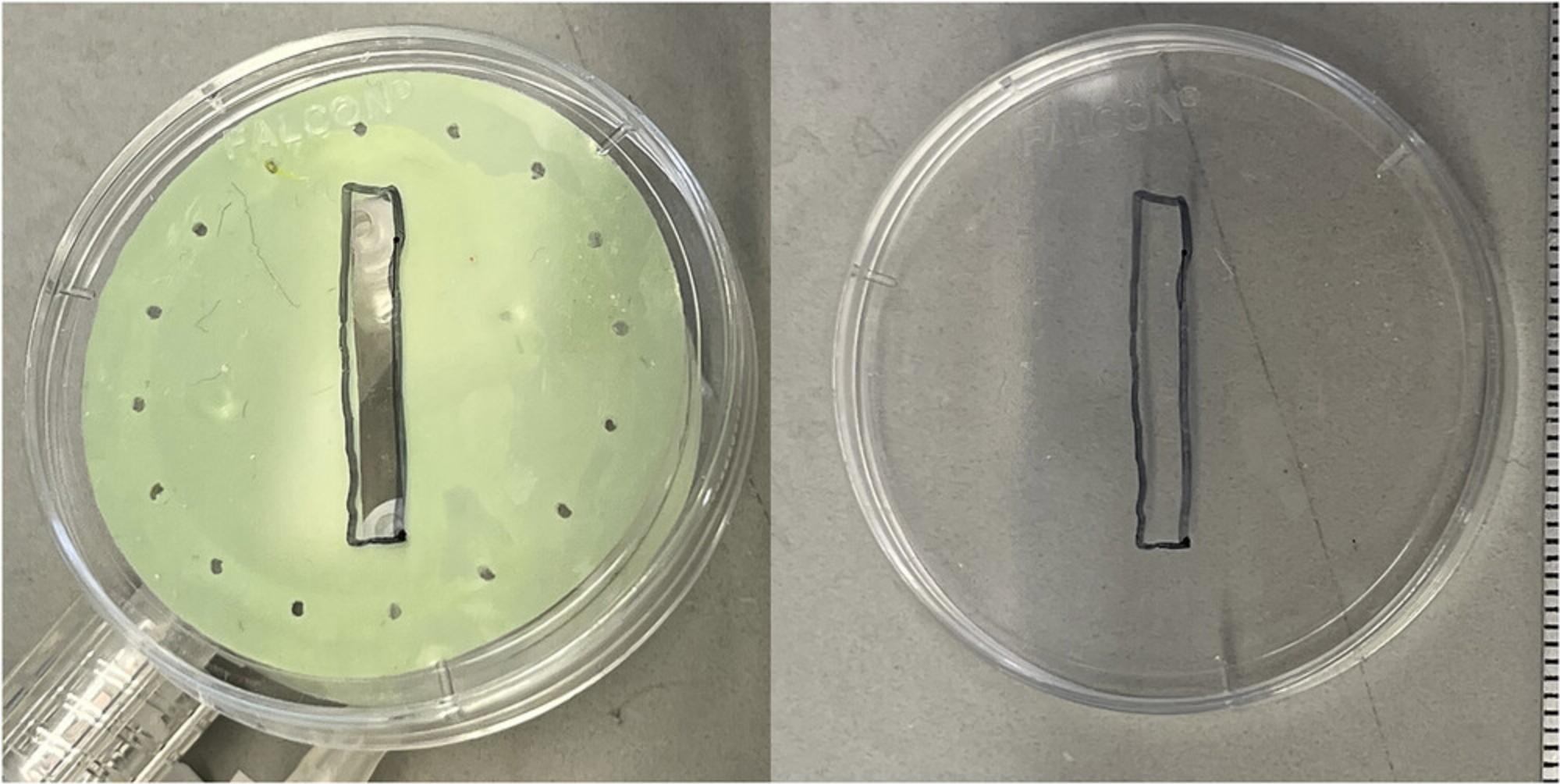
4.Make a solution of protein A/G in PBS with chemokine, if desired, at a concentration of 2 µg/ml protein A/G + 1 µg/ml chemokine of choice.
5.Add 100 µl protein A/G solution to the middle of each gasket. Tap the dish to ensure the surface is covered and avoid touching the bottom.
6.Incubate overnight in the 4°C refrigerator or rocking table in a cold room at 4°C.
7.Wash the surfaces 3× with ∼200 µl PBS per gasket.
8.Make a solution of selectins in PBS. For murine T cells: 0.2 µg/ml P-selectin/Fc or 0.2 µg/ml E-selectin/Fc.
9.Add 100 µl solution with selectins to the middle of each gasket. Tap the dish to make sure the surface is covered.
10.Incubate at room temperature for 3 hr on a rocking table (or at 37°C for 1 hr).
11.Wash the surfaces 3× with ∼200 µl PBS per gasket.
12.Add 100 µl of 1% BSA in PBS to the middle of each gasket. Tap the dish to make sure the surface is covered.
13.Incubate at room temperature for 1 hr on a rocking table.
14.Wash the surfaces 3× with ∼200 µl PBS per gasket.
15.Store dishes on ice or in the fridge. Ideally, use the dishes the same day.
Basic Protocol 2: FABRICATION OF POLYACRYLAMIDE GELS FOR CELL ROLLING EXPERIMENTS
When performing cell rolling experiments on polyacrylamide gels, it is crucial to ensure the gel surface is smooth and uniform. Any irregularities on the gel surface can hinder cell attachment and disrupt fluid flow. Therefore, it is essential to prepare a pristine environment, such as a tissue culture hood, for gel fabrication and use filter-sterilized solutions to eliminate debris. This protocol provides instructions for creating polyacrylamide gels on 25-mm coverslips for use with the Glycotech circular parallel plate flow chamber. The protocol is particularly useful when coating the gels with protein A/G and Fc chimeras. If you need to conjugate proteins directly to the gel surface without relying on protein A/G and Fc chimeras, adjustments to the coating conditions may be necessary, as described at the end of this protocol.
Materials
-
0.2 M HCl (Sigma, cat. no. 13-1720)
-
H2O, deionized
-
0.1 M NaOH (Sigma, cat. no. 1310-73-2)
-
(3-Aminopropyl)trimethoxysilane (Sigma, cat. no. 13822-56-5)
-
Glutaraldehyde (Sigma, cat. no. 111-30-8)
-
PBS, 1× (HyClone, cat. no. SH30258.02)
-
Silica gel, grade 47 desiccant (Fisher Scientific, cat. no. S161-500)
-
Rain‑X glass treatment solution
-
H2O, Milli-Q
-
40% acrylamide solution (Sigma, cat. no. 79-06-1)
-
2% bis-acrylamide solution (Fisher BioReagents, cat. no. BP1404-250)
-
Ammonium persulfate (APS) (Sigma, cat. no. 7727-54-0)
-
TEMED (Thermo Fisher Scientific, cat. no. 15524010)
-
Sulfo-SANPAH (Sigma, cat. no. 803332)
-
Dimethyl sulfoxide (DMSO) (Sigma, cat. no. 5.89569)
-
0.25 M HEPES (Fisher Scientific, cat. no. 15630106)
-
Ethanolamine (Sigma, cat. no. 141-43-5)
-
25-mm glass coverslips (Fisher Scientific, cat. no. 50-121-5161)
-
100-mm dish (Fisherbrand, cat. no. FB012924)
-
Orbital shaker (Heidolph, cat. no. 13-878-521)
-
50-ml conical centrifuge tubes (Falcon, Fisher Scientific, cat. no. 352070)
-
Kimwipes (Kimtech Science, cat. no. 06-666)
-
0.2-µm sterile membrane filter (Sigma, cat. no. RW0304700)
-
1.5-ml microcentrifuge tubes (Fisherbrand, cat. no. 05-408-129)
-
4.7-L vacuum chamber (Thermo Scientific, cat. no. 01-060A)
-
Tissue culture hood with nitrogen gun
-
24-well plates, tissue culture (TC) or non-TC (Sigma, cat. no. CLS3527)
-
6-well non-TC treated plates (Fisher Scientific, cat. no. 08-772-49)
-
Fine-point tweezers (Fisher Scientific, cat. no. 12-000-122)
-
−20°C freezer (Thermo Scientific, cat. no. TSV20FPSAVM)
-
UV lamp spotlight (Fisher Scientific, cat. no. UVP76005501) of UV lamp rectangular (Spectro-UV, cat. no. EN-160)
-
Aluminum Foil (Fisherbrand, cat. no. 01-213-100)
-
Refrigerator (Fisher Scientific, cat. no. FBG30RSGA), 4°C
-
Rocking table (Fisher Scientific, cat. no. 02-217-765)
Prepare activated bottom coverslips
1.Place coverslips in a single 100-mm dish (can activate multiple 25-mm glass coverslips at one time; these can be stored at the end for long periods of time).
2.Add 20 ml (40 to 50 ml for 150-mm dish) of 0.2 M HCl, shake to disperse coverslips, and leave it overnight at room temperature.
3.Decant 0.2 M HCl (can be reused multiple times).
4.Rinse coverslips with several changes (4 to 5) of deionized water for ∼2 min per rinse, shaking on an orbital shaker.
5.Add 20 ml of 0.1 M NaOH and incubate at room temperature on the orbital shaker for 10 min (minimum).
6.Rinse coverslips with several changes (4 to 5) with deionized water for ∼2 min, shaking on orbital shaker.
7.Add 20 ml deionized water and add 100 µl aminopropyl trimethoxysilane (0.5% v/v final).
8.Incubate with shaking on an orbital shaker at room temperature for a minimum of 30 min.
9.Collect aminopropyl trimethoxysilane as hazardous waste. Rinse coverslips with several changes of deionized water, shaking on orbital shaker. Rinse 4 to 5 times quickly at the sink, then shake with 4 to 5 changes of water for several minutes each on an orbital shaker (must remove all traces of aminopropyl trimethoxy silane).
10.Add 0.5% (v/v) glutaraldehyde in 1× PBS (20 ml for 100-mm dish). Incubate on orbital shaker for at least 1 hr.
11.Collect glutaraldehyde solution as hazardous waste. Rinse coverslips with several changes of deionized water shaking on an orbital shaker (rinse 4 to 5 times quickly just at the sink, then shake with 4 to 5 changes of water for several minutes each on an orbital shaker.
12.Let coverslips dry, then store them in a 100-mm dish in a container with desiccant.
Prepare hydrophobic top coverslips
13.Place ∼20 ml Rain-X in a 50-ml centrifuge tube
14.Dunk coverslips in the Rain-X and let dry on a Kimwipe.
15.Wipe off the excess Rain-X on the coverslips with Kimwipes. Rinse the coverslips in filtered Milli-Q water. Let it dry, and wipe again until there is no residual Rain-X or Milli-Q water remaining.
16.You can re-use the coverslips multiple times as long as you wipe them with clean (throw them away if gels start to stick to them).
Gel polymerization
17.Prepare acrylamide/bis-acrylamide stock formulations according to Table 1 and Table 2.It is easiest to first filter the 40% acrylamide and 2% bis-acrylamide solutions with a 0.2-µm sterile filter and then mix the filtered solutions with Milli-Q water. Store stock solutions at 4°C in the dark.
Elastic modulus | 1 kPa | 5 kPa | 10 kPa | 24 kPa | 84 kPa | |
Final % acrylamide | 3% | 5.5% | 7.5% | 7.5% | 12% | |
Final % bis-acrylamide | 0.2% | 0.15% | 0.15% | 0.3% | 0.28% | |
Components | ||||||
40% acrylamide (ml) | 3.98 | 7.29 | 9.94 | 9.94 | 15.9 | |
2% bis-acrylamide (ml) | 5.3 | 3.98 | 3.98 | 7.95 | 7.42 | |
Milli-Q water (ml) | 28.89 | 26.9 | 24.25 | 20.28 | 14.85 | |
0.25 M HEPES (ml) | 6.3 | 6.3 | 6.3 | 6.3 | 6.3 |
- a
From these stock solutions, you will take 840 µl for a final gel solution volume of 1 ml, so the “Final % acrylamide” and “Final % bis-acrylamide” are based on the final volume.
- b
HEPES is added to the solution to achieve pH ∼6, which is necessary when using the N6 linker to limit hydrolysis of the NHS ester. If you only plan to use sulfo-SANPAH as the linker, you can replace this volume with Milli-Q water.
Elastic modulus | 1250 Pa | 2500 Pa | 7900 Pa | 60,000 Pa | ||
Final % acrylamide | 3% | 5% | 7.5% | 10% | ||
Final % bis-acrylamide | 0.2% | 0.2% | 0.07% | 0.5% | ||
Components | ||||||
40% acrylamide (ml) | 1.62 | 2.7 | 4.0 | 5.4 | ||
2% bis-acrylamide (ml) | 2.2 | 2.2 | 0.77 | 5.5 | ||
0.25 M HEPES (ml) | 2.6 | 2.6 | 2.6 | 2.6 | ||
TEMED (µl) | 10 | 10 | 10 | 10 | ||
ddH2O (ml) | 12 | 10.94 | 11.07 | 4.95 |
- a
The recipes in this protocol have TEMED added. You can instead add TEMED immediately before polymerization (1 µl TEMED for 1 ml gel solution).
18.Calculate the volume of gel you need depending on how many gels you plan to make. For 1 ml final gel volume, place 840 µl gel stock solution in a 1.5-ml microcentrifuge tube. Then, add 150 µl water (or dissolved N6 linker in water/ethanol). For 25-mm coverslips, use 50 µl gel solution to achieve a 100-µm gel thickness.
19.Place the tubes in a vacuum chamber with the lids off and pull the vacuum for 30 min.
20.In a tissue culture hood, use the nitrogen gun to blow off any debris from the activated bottom coverslips and lay out the coverslips on 24-well plates with the lids off.
21.In the tissue culture hood, lay out the Rain-X treated coverslips and use clean gloved fingers to wipe the coverslips clean. Be sure to remove any marks or debris.
22.Weigh out ∼50 mg APS in a microcentrifuge tube and add ∼500 µl water to achieve a final concentration of 10% w/v. Mix well.
23.Put 2 ml filtered Milli-Q water into each well of non-TC treated 6-well plates. You will need 1 well per gel.
24.Retrieve gel solutions from the vacuum chamber and retrieve TEMED from the fridge.
25.Polymerize one tube of gel solution at a time by adding 5 µl of 10% APS and 0.5 µl TEMED to 500 µl gel solution (scale as necessary). Immediately mix and pipette 50 µl solution to the center of each activated bottom coverslip. Use tweezers to carefully lay a Rain-X treated coverslip on top.
26.Allow the gels to polymerize for 2 to 10 min (soft gels take longer than stiff gels). You can be sure that the gels have fully polymerized when water has started to evaporate from the edges of the coverslips (leaving behind air gaps).
27.Once polymerized, place each gel/coverslip sandwich into a well in the 6-well plate filed with water from step 23.Swirl the plate to get the edges of the gels wet. Let sit for at least a few minutes.
28.Remove the top coverslip by holding the gel/coverslip sandwich in one gloved hand by the edges, then gently wedge one point of the tweezers in between the two coverslips and push the top coverslip up. Try to remove the top coverslip without scratching the gel.
29.Rinse the gels 3× with 2 ml water and store at 4°C for up to 1 week.
Protein conjugation with sulfo-SANPAH
Sulfo-SANPAH is a bifunctional linker: one end reacts with the polyacrylamide gel when exposed to UV light, and the other has an NHS ester that reacts with primary amines on protein. The NHS reaction is sensitive to pH (reacts at pH 7 to 9), and hydrolysis is a competing, unfavorable reaction. Once you dilute sulfo-SANPAH in water, work quickly to limit hydrolysis.
30.For a new bottle of sulfo-SANPAH:
-
First let the bottle warm to room temperature.
-
Prepare stock aliquots by dissolving the powder in DMSO at a final concentration of 100 mg/ml.
-
Aliquot into microcentrifuge tubes so that you will not need to re-freeze/thaw again (5 to 10 µl aliquots work well).
-
Place the stock aliquots in a bag with desiccant and store them in the −20°C freezer, protected from light.
31.Activate one plate at a time. Depending on how many gels are in your plate, place 200 µl Milli-Q water per gel into a microcentrifuge tube.
32.Retrieve an aliquot of sulfo-SANPAH from the freezer.
33.Place a 1 ml pipette tip onto the end of an aspirating pipette and aspirate all the water from the gels. Use the pipette tip to nudge the coverslip into the middle of the well and completely dry around the coverslip. Carefully aspirate excess water from the surface of the gels.
34.Immediately dilute the sulfo-SANPAH in water (1 µl sulfo-SANPAH for every 200 µl water; final concentration = 0.5 mg/ml). Then, add 195 µl to the surface of each gel.
35.Place the plate under a UV lamp with the lid off and expose it to UV for 8 to 15 min, depending on which lamp you are using:
-
If using the long rectangular lamp expose the plate to UV for 15 min.
-
If using the spotlight lamp, you should have pre-warmed the lamp for ∼10 min. Expose the plate to UV for 8 min (if you expose it longer, the gels will dry out).
36.During the UV incubation, prepare a protein solution in either PBS or 50 mM HEPES, pH 8.5.You will need 200 µl per gel. Keep the solution cold.
37.At the end of the UV incubation, immediately wash the gels 3× with Milli-Q water (2 to 3 ml per gel). Then, aspirate all the remaining water as in step 4.
38.Add 195 µl protein solution to the surface of each gel. Gently rotate the plate to ensure the enture surface of each gel is covered, but do not allow the solution to run off the gels.
39.Wrap the plate in foil and incubate overnight at 4°C.
40.The next day, prepare a solution of ethanolamine: for each gel, dilute 2 µl ethanolamine in 200 µl PBS or 50 mM HEPES.
41.Wash the gels 3× with 2 ml water, then aspirate the remaining water as in step 4.
42.Add 195 µl of the ethanolamine solution to the surface of each gel and incubate for 30 min at room temperature on the rocker plate.
43.Wash the gels 3× with PBS and store them at 4°C if they will not be used immediately.
44.If you need to add Fc chimeras, aspirate all the PBS as in step 4 and incubate the gels with the Fc chimera solution for 1 hr at 37°C. Then wash the gels 3× with PBS and store them at 4°C. Ideally, use the plates on the same day.
Alternate Protocol 1: PROTEIN CONJUGATION WITH N6 LINKER
Protein conjugation with the N6 linker involves covalently attaching the N6 linker molecule to proteins. The N6 linker typically features a reactive group, often an N-hydroxysuccinimide (NHS) ester, facilitating specific conjugation to primary amines on the protein surface. Unlike sulfo-SANPAH, the N6 linker process does not rely on UV light exposure or exhibit sensitivity to pH conditions.
Additional Materials (also see Basic Protocol 2)
-
N6-crosslinker (ThermoFisher Scientific, cat. no. 22105)
-
100% ethanol (Sigma, cat. no. 459836)
-
Growth medium of cell type being assayed
-
Razor blades (Fisherbrand, cat. no. 12-640)
-
500-nm fluorescent beads (FluoSpheres, cat. no. F8813)
-
Sonicator (Fisherbrand, cat. no. FB15337409)
-
Parafilm (Fisher Scientific, cat. no. 13-374-16)
-
Vortex mixer (VWR, cat. no. 10153-838)
-
Slide holder (Fisherbrand, cat. no. 12-588-25)
-
Ziploc bag
-
15-ml centrifuge tubes (Falcon, Fisher Scientific, cat. no. 14-959-53A)
-
Circular parallel-plate flow chamber (Glycotech, cat. no. 31-001) with tubing and gaskets
-
Vacuum grease
-
Incubator
Prepare activated bottom coverslips
1.Spread coverslips in 100-mm tissue culture dish. Coat coverslips with a thin layer of 0.1 M NaOH and let dry.
2.Coat coverslips with 100 µl of 3-aminopropyltrimethoxysilane and spread with a glass pipette.
3.Allow to dry for 5 min (no more than 10 min).
4.Rinse coverslips 3× for 5 min in distilled water in the plastic Petri dishes.
5.Thaw glutaraldehyde for 15 min in a chemical hood before use.
6.Prepare a 0.5% glutaraldehyde solution in PBS and incubate coverslips in ∼2 to 3 ml glutaraldehyde solution per coverslip for 30 min.
7.Rinse coverslips 3× for 10 min in distilled water in 100-mm tissue culture dishes.
8.Remove coverslips from the dishes with a razor blade and place on a Kimwipe to dry (can be left overnight). Store coverslips in a container with desiccant up to 6 months.
Prepare polyacrylamide substrates
You should adjust the pH of all solutions to pH 6.0 with 5 N HCl using pH paper (low pH prevents the N6 from hydrolyzing), although adjusting the pH may not be necessary. Store the solutions at 4°C in the dark.
9.Put 60 µl of 500-nm fluorescent beads in a 1.5-ml Eppendorf tube and sonicate (amplitude 40) for 5 to 10 min (be careful to just turn the time dial and not turn on the heat switch).
10.Place 5.6 mg N6 crosslinker per 1 ml solution in an Eppendorf tube, and then add 70 µl of 100% ethanol. Sonicate for ∼5 s (not longer, or it will heat up) to break up the N6.You can also mechanically break up the larger chunks of crosslinker in the ethanol with a pipet tip. Before returning N6 to the freezer, gently fill the bottle with nitrogen and wrap the cap with parafilm.
11.Add 845 µl gel solution (scale all volumes to mg of crosslinker weighed out; all values used here correspond to 5.6 mg of N6) to the dissolved crosslinker in the ethanol, vortex for up to 10 min at 3200 rpm until the crosslinker is dissolved, then add 40 µl double distilled water and 40 µl fluorescent beads (if adding fewer beads replace that volume with double distilled water to keep the total solution volume the same). Mix with a pipet, and do not vortex once beads are added to prevent beads from clumping.
12.Place Eppendorf tubes in a vacuum chamber with the caps off and degas for 30 min.
13.Coat 2 to 4 coverslips with Rain-X. The size of these coverslips will determine the size of your gels. Initially, apply Rain-X with a cotton swab (or dunk the coverslips in Rain-X), but use your gloved fingertip to smear the solution evenly over the cover glass. Allow Rain-X to dry for ∼5 min and wipe clean with clean, gloved fingertips.
14.Place the activated bottom coverslips on a small plastic Petri dish (or another surface).
15.Add 6 µl of freshly made 10% APS (0.1 g in 1 ml double distilled water) to 1 ml gel solution (do one gel at a time). Mix up and down with a pipette and immediately dispense on top of the activated coverslips (50 µl gel solution for 25-mm coverslips would produce gels that are 100-µm thick; scale as necessary). Immediately place a Rain-X coated top coverslip on top of the gel solution, sandwiching the gel solution between the activated and Rain-X coated coverslips. For traction force gels, immediately flip the coverslip sandwich over so that the beads will fall to the top surface of the gel.
16.Polymerize gels on a slide holder with water in the bottom and in a Ziploc bag purged of O2 with N2. This will allow the gels to polymerize all the way to the edge of the coverslip and help prevent cracks from forming. Let the gels polymerize for 30 to 45 min.
17.Chill 10 ml double distilled water in 4°C for use in steps 19 and 21.
18.Prepare the peptide or protein solution to bind to the polyacrylamide gels. You will need 100 to 300 µl protein solution for each gel. In a 15-ml conical tube, add an appropriate amount of protein solution to PBS or 50 mM HEPES buffer, pH 8.0. Keep the protein solution on ice.
19.Once the gels are polymerized, dunk them in ice-cold double distilled water, and then remove the top coverslip from the gel with a razor blade. Rinse with ice-cold double distilled water and place in a small plastic Petri dish or multi-well plate with the gel side up. Immediately dispense 300 µl of the prepared protein solution onto the gel. Cover the gels and incubate at 4°C for 2 hr or overnight.
20.Prepare a solution of 1/100 parts ethanolamine in 50 mM HEPES buffer, pH 8.0, in the chemical hood and then vortex. You will need 100 to 300 µl for each gel that you made. Place the solution on ice to cool completely (which may not be necessary).
21.After the 2-hr or overnight protein incubation, rinse the gels with chilled HEPES buffer and dispense 100 to 300 µl prepared ethanolamine solution onto each gel. Incubate for 30 min covered at 4°C (or room temperature is acceptable).
22.After the 30-min incubation, rinse the gels with ice-cold double distilled water and place them in cold PBS. Wrap the dishes in parafilm and aluminum foil and store for up to a week at 4°C.
Chamber assembly and cell plating
23.Rinse reusable chambers and plugs with ethanol, wipe them dry with a Kimwipe, and place them in the cell culture hood.
24.Place the plugs on each end of the chamber outlets and apply a thin coat of vacuum grease on the bottom of the chamber around the opening.
25.Shake a gel dry, wipe any excess water on the coverslip around the gel dry with a Kimwipe, and affix it to the vacuum grease on the chamber. The gel side should obviously go into the opening of the chamber.
26.Add 3 ml growth medium into the chamber on top of the gel.
27.Repeat steps 18 and 19 for the remainder of the chambers and sterilize the chambers under a UV lamp for 30 min.
28.Passage your cells. For each gel that you are going to plate, prepare 3 ml growth medium. Prepare cells at a concentration of 3000 cells/ml medium. Mix medium and cells well by pipetting up and down carefully without forming bubbles.
29.Aspirate the growth medium from each chamber and add 3 ml growth medium with cells. Place chambers in the incubator overnight.
Alternate Protocol 2: HUVEC CULTURING FOR MONOLAYERS
This protocol provides the necessary methodology to culture HUVECs and serves as an alternative to protein opsonization by utilizing a more physiologically relevant approach. Unlike protein opsonization, this protocol requires the sterile and systematic culture of HUVECs to passage, maintain, cryopreserve, and thaw HUVECs to be functionalized with the polyacrylamide gels and used for subsequent cell rolling experiments.
Materials
-
HUVECs (ATCC, cat. no. PCS-100-010)
-
HEPES-buffered saline (Cytiva, cat. no. SH3025801)
-
Trypsin/EDTA (Gibco, cat. no. 25200056)
-
Trypsin neutralizing solution (TNS) (ATCC, cat. no. PCS-999-003)
-
EGM-2 basal medium (Lonza, cat. no. CC-3156)
-
EGM-2 endothelial cell growth medium-2 BulletKit (Lonza, cat. no. CC-3162)
-
Liquid nitrogen
-
70% ethanol
-
DMSO (Sigma, cat. no. 5.89569)
-
Tissue culture flasks (Fisher Scientific, cat. no. FB012937)
-
Incubator
-
Microscope
-
15-ml centrifuge tube (Falcon, Fisher Scientific, cat. no. 14-959-53A)
-
Refrigerated centrifuge
-
Hemocytometer (Hauser Scientific, cat. no. 02-671-6)
-
Cryovials
-
37°C water bath
-
Mr. Frosty freezing container (Thermo Scientific, cat. no. 5100-0001)
Passaging cells
1.Passage HUVECs that are ∼80% confluent and have many mitotic figures throughout the flask.
2.Aspirate the cell culture medium.
3.Rinse the cells with 2 to 3 ml of room temperature HEPES-buffered saline.
4.Aspirate the HEPES-buffered saline from the flasks.
5.Cover the cells with 1 ml of 0.25 mg/ml trypsin/EDTA.
6.Allow cells to trypsinize in the incubator for 5 min and then examine under the microscope to ensure that all the cells have balled up or are floating. Rap the flask to release the balled cells. If most cells do not release, then allow them to trypsinize longer.
7.After the cells release, neutralize the trypsin with 2 to 3 ml of room temperature TNS.
8.Transfer the detached cells in the TNS to a 15-ml centrifuge tube.
9.Examine the harvest flasks under the microscope to ensure that 95% of the cells were harvested.
10.Centrifuge the cells at 5 min at 220 × g , 4°C, to pellet the cells.
11.Aspirate most of the supernatant, being careful not to suck up the pellet. Flick the tube to loosen the pellet in the remaining 100 to 200 µl of supernatant.
12.Resuspend the cells in 2 to 3 ml EBM-2 basal medium with EBM-2 BulletKit added (depending on the size of the pellet) and note the total volume of the suspension.
13.Determine the cell count using a hemocytometer after resuspending the cells. If the cells are too dense, you may need to dilute (e.g., 1:5) in a 0.5-ml tube before counting.
14.Seed cells at a density of 5 × 104 cells per T-25 flask in 4 to 5 ml fresh growth medium (EGM-2).
15.Return properly labeled flasks (initials, cell type, passage date, passage #) to the incubator at 37°C and 5% CO2.
Maintenance
16.Change the growth medium every other day on HUVECs. Aspirate off old medium and add fresh, warmed growth medium.
Thawing cells
17.Calculate the number of flasks needed based on the required seeding density and the number of cells in the cryovial.
18.Add the appropriate amount of growth medium to the vessels (1 ml/5 cm2) and allow them to equilibrate in the incubator for 30 min at 37°C and 5% CO2.
19.Wipe cryovial taken from the liquid nitrogen with 70% ethanol. In a sterile field, briefly twist the cap a quarter turn to release the pressure, and then retighten the cap.
20.Quickly thaw in a 37°C water bath. Submerge only the bottom ¾ of the vial and remove the vial as the last sliver of ice melts. Do not wait for more than 2 to 3 min, or the results will not be optimal.
21.Gently resuspend the cells in the cryovial with a micropipette set to less than the total volume of the cryovial to reduce air bubbles.
22.Dispense the cells into the culture flasks prepared earlier. Do not centrifuge the cells before dispensing them since centrifugation is more damaging at this point than the residual DMSO left in the flasks.
23.Return the flasks to the incubator and change the medium the next day to remove the DMSO.
Basic Protocol 3: CONDUCTING CELL ROLLING EXPERIMENTS ON POLYACRYLAMIDE GELS
This protocol examines the cell capture and rolling on polyacrylamide gels using a circular parallel-plate flow chamber. The polyacrylamide gels should be fabricated on 25-mm coverslips in a clean environment (i.e., Class II Biosafety Cabinet), and all reagents used should be sterile filtered to create a smooth and homogeneous gel surface. This protocol is also applicable to plastic or glass surfaces. To use these surfaces, a vacuum pump must be used to keep the flow chamber sealed. For surfaces coated with PDMS (polydimethylsiloxane; or polyacrylamide as described here), a vacuum pump is not required as the surfaces will generate a self-sufficient airtight seal. For polyacrylamide gels, using a vacuum pump could distort the gels and potentially pull soft gels upwards into the flow path.
Materials
-
70% ethanol (Sigma, cat. no. 459836)
-
PBS (Cytiva HyClone, cat. no. SH30256.01)
-
Polyacrylamide gels on 25-mm coverslips, coated with adhesion proteins (see Basic Protocol 2)
-
RPMI 1640 medium (Fisher Scientific, cat. no. 11-875-093)
-
Fetal bovine serum (FBS) (Cytiva, cat. no. SH30071.03)
-
Penicillin-streptomycin solution (penstrep) (Cytiva, cat. no. SV30010)
-
Inverted light microscope with 10× or 20× phase objective and camera (>30 fps) and environment chamber (Nikon or equivalent)
-
Circular parallel-plate flow chamber (Glycotech, cat. no. 31-001) with tubing and gaskets
-
20-ml syringes (Fisherbrand, cat. no. 14-955-460)
-
Kimwipes (Fisher Scientific, cat. no. 06-666)
-
Syringe pump (Harvard Apparatus, cat. no. 70-4500)
-
8-ml round-bottom tubes (Fisher Scientific, cat. no. 14-959-38C)
-
1.5-ml microcentrifuge tubes (Eppendorf, cat. no. 0030123611)
-
Fine point tweezers (Fisher Scientific, cat. no. 12-000-122)
-
35-mm tissue culture dish (Corning, cat. no. 430165)
-
Parafilm (Fisher Scientific, cat. no. 13-374-16)
Preparation ahead of time
1.Heat microscope chamber on inverted light microscope to 37°C.
2.Set the microscope to the following parameters:
- 10× objective lens
- Phase ring PH 1
- Light Intensity 3.9 V
3.Assemble flow chamber on top of the gasket (Fig. 3).
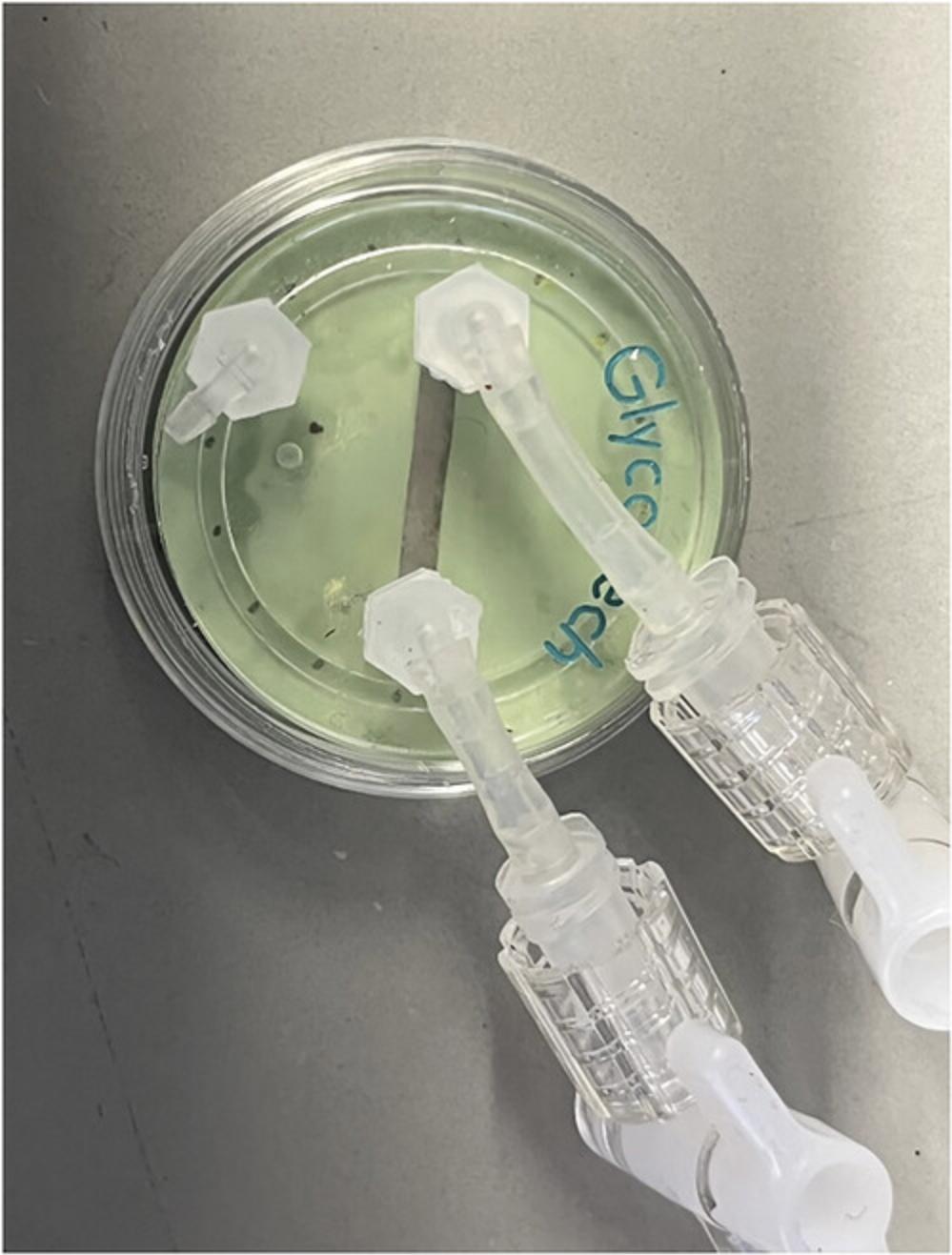
4.Attach a short tube to the inlet port and a long tube to the outlet port.
5.Connect the outlet tubing to a 20-ml syringe with a Luer lock, but do not overtighten.
6.Wipe the gasket with 70% ethanol and let dry.
7.Wipe the bottom of the flow chamber with 70% ethanol and let dry.
8.Place the gasket on a clean Kimwipe and carefully align the flow chamber on top.
9.Place a second 20-ml syringe in the pump.
10.Turn the pump on and enter the syringe diameter and desired flow rate.
11.Fill 8-ml round-bottom polystyrene tubes with 6-ml sterile PBS.
12.Fill 1.5-ml centrifuge tubes with 1 ml medium (RPMI 1640 supplemented with 10% FBS and 1% penstrep) and keep warm.
Running experiment
13.Use tweezers to pick up a gel/coverslip by the edge. Hold the coverslip vertically and wick away almost all excess liquid by touching the edge of the gel to a Kimwipe.
14.Inspect the gel surface and use tweezers to remove any wrinkles or defects in the gel.
15.With the flow chamber upside down (gasket oriented up), carefully place the gel face-down on the gasket so that the flow path is centered on a perfectly smooth region of the gel.
16.Do not move the gel once it is placed since this might ruin the gel surface.
17.Place the flow chamber carefully into the bottom of a 35-mm dish and gently wrap parafilm around the edge (to prevent the chamber from sliding around). Turn the chamber over and make sure there is no liquid outside the area of the coverslip, since this would cause leaking later.
18.Place the flow chamber/dish onto the microscope stage so that the flow path is horizontal in the eyepiece.
19.Insert the inlet tubing into an 8-ml tube of PBS, resting the tube on its side so that the tubing is fully submerged (but do not let PBS spill everywhere). Use the syringe to pull PBS through the device and completely fill the outlet tubing up to the syringe. Do this in quick spurts to remove any trapped air bubbles from within the flow chamber.
20.Remove the tube of PBS and use the syringe to draw up a small amount of air into the inlet tubing (∼¼ inch long), then place the inlet tubing into a 1.5-ml tube of medium, resting the tube on its side. You should have a small air bubble separating the PBS and the medium.
21.Slowly draw the air bubble up to the inlet of the flow chamber, and then, in one quick motion, pull the air bubble through the flow chamber. Continue pulling medium through until only ∼0.5 ml medium is left in the 1.5-ml tube but ensure that the inlet tubing is still fully submerged.
22.Use your fingers to pinch the outlet tubing right before the syringe, then use your other hand to disconnect the outlet tubing and reconnect it to the syringe situated in the pump.
23.Turn on the pump at 40.3 µl/min (100s-1) and examine the inlet and outlet tubing for air bubbles.
24.Get rid of trapped bubbles near the outlet by gently lifting the outlet tubing until the bubbles stick to the larger air bubble (that was placed between the PBS and medium). Do not let any air bubbles stay stuck along the tubing.
25.If there are bubbles in the inlet tubing, switch the outlet tubing back to the free syringe (while pinching the end of the tubing). Draw up another small air bubble, place the tubing in a fresh tube of medium, and quickly pull the air bubble through the flow chamber as before.
26.Stop the pump and retrieve the cell culture solution.
27.Before adding your cell solution, look through the eyepiece to ensure that there is no flow within the flow chamber, which would indicate leaking. If debris is still moving, wait until it stops. If the flow does not stop, then your apparatus is leaking, and you will need to try again with a different gel.
28.Carefully remove the 1.5-ml tube of medium and replace it with the 1.5-ml tube of cells.
29.Turn on the pump and start the timer.
30.If necessary, reposition the field of view to the middle of the flow path and ensure that the flow path is horizontal on the monitor.
31.Start recording when cells approach the field of view (for 40 µl/min the cells will take ∼2.5 min to reach the chamber).
32.Before the cell solution is completely drawn up, look through the inlet tubing and manually scan the entire flow path (to enable a total count of arrested cells later). Then stop recording and stop the pump.
33.Carefully take the flow chamber/dish off the microscope and visually check whether any liquid leaked from between the gel and the gasket. If the apparatus was sealed the entire time, the dish should be as dry as when you started the experiment.
Preparing for a subsequent sample
34.Remove the parafilm from the flow chamber and switch the outlet tubing from the syringe on the pump to the free syringe.
35.Remove the gasket from the flow chamber and wipe both sides with 70% ethanol. Let the gasket dry on a clean Kimwipe.
36.Hold the flow chamber lower than the table and use a Kimwipe to collect some liquid from the outlet tubing (otherwise, it will come out later and make a mess).
37.Remove all liquid from the inlet tubing by gently tapping on a Kimwipe.
38.Wipe the bottom surface of the flow chamber with 70% ethanol and let dry. Also, dry the 35-mm dish.
39.Repeat setup as before.
Basic Protocol 4: ImageJ ANALYSIS OF CELL ROLLING MOVIES
This protocol examines the process of analyzing cell rolling movies captured on an inverted light microscope. The protocols require the installation of software like ImageJ with the MTrack2 plugin. The ImageJ software with the additional MTrack2 plugin is used to track the XY positions of the cells. The protocol also provides a MATLAB script to plot trajectories and manipulate the data acquired from the MTrack2 plugin.
Necessary Resources
Hardware
- At least 8 GB RAM, 256 GB hard drive storage or solid-state drive storage, and Intel i5 processor or AMD Ryzen 5
Software
- Windows OS or LINUX of Mac OS X
- MATLAB (see Internet Resources)
- ImageJ with MTrack2 plugin (see Internet Resources)
Input files
Tiff images of each timepoint acquired from microscope
ImageJ analysis
Shortcut keys, as well as macros, can be created in ImageJ. How to utilize these macros is shown step by step below.
1.F12 → This is a shortcut for “File” > “Import” > “Image Sequence.”
2.Choose the images to import from the inverted light microscope.
3.Ctrl/Command + shift + I → This inverts the LUT (i.e., whether pixel value 0 is black or white)
4.(Optional) If your image needs to be rotated for the flow stream to be horizontal, hit g → This is a shortcut for “Z-project.” Choose the Projection type: “Standard Deviation.” Then hit F3 → this is a shortcut for “Image” > “Transform” > “Rotate…”. Choose a rotation angle as needed (it helps to use the grid lines and preview option).
5.You need a clean image of the background to subtract the image from your movie stack. Either:
- a. Scroll to an image in your movie stack that has no cells and hit.
Ctrl/Command + shift + d → this duplicates the image.
- b. If you cannot find a clean background image or your background changes over time, go to “Image” > “Stacks” > “Tools” > “Make Substack…” (you could also make a shortcut for this). Choose a few frames throughout your movie stack. Hit g , then choose Projection type: “Min Intensity”.
6.F11 → This is a shortcut for “Process” > “Image Calculator…”. Choose your movie stack for Image 1, your background image for Image 2, and Operation: “Subtract”. Check “Create new window.”
7.F10 → This is a shortcut that executes the macro called “RollingMTrack2.jim”. The commands within this macro are shown below. The commands can be edited by going to “Plugins” > “Macros” > “Edit…” and choosing the macro file. You may want to change the value for “setThreshold(40,255)” depending on the size of your cells: perhaps (20,255) for smaller cells and (60,255) for larger cells. The result of the macro should be binary images with a white background and solid black objects representing the cells.
RollingMtrack2.jim:
- setAutoThreshold("Default dark");
- setThreshold(40, 255);
- setOption("BlackBackground", false);
- run("Convert to Mask", "method=Default background=Dark");
- run("Close-", "stack");
- run("Open", "stack");
- run("Watershed", "stack");
8.F9 → This is a shortcut that executes the MTrack2 plugin. In the dialog window, set the minimum object size to 10 pixels2 and the maximum object size to 2000 pixels2 (any objects smaller/larger are not tracked). For a shear rate of 100 s-1, set the maximum velocity to 8 pixels/frame and the minimum track length to 30 frames. Check the first 3 boxes (for “Save Results File,” “Display Path Lengths,” and “Show Labels”). Designate a file name/location for the XY position data.
9.The MTrack2 plugin will take anywhere from 30 s to 30 min to run, depending on how many cells are tracked. When the analysis is complete, a new window will appear, showing the binary movie stack with the objects labeled by track number.
10.F8 → this is a shortcut for “Image” > “Color” > “Merge channels…”. To overlay the track numbers on the original movie stack, choose the “…labels” stack for red and your original movie stack for gray.
11.F6 → this is a shortcut for “File” > “Save as” > “AVI…”. Choose Compression: “JPEG” and Frame Rate: 30 fps.
Further analysis with MATLAB
12.The ImageJ Mtrack2 plugin creates a text file listing the XY positions of every track (Fig. 4). It is best to open these files in Excel. Tracks 1 to 75 will be listed from left to right, then below that will be the next 75 tracks, and so on. We utilize a custom MATLAB script that requires that each track is saved as an individual text file with the first column = frame #, second column = X position, and third column = Y position.
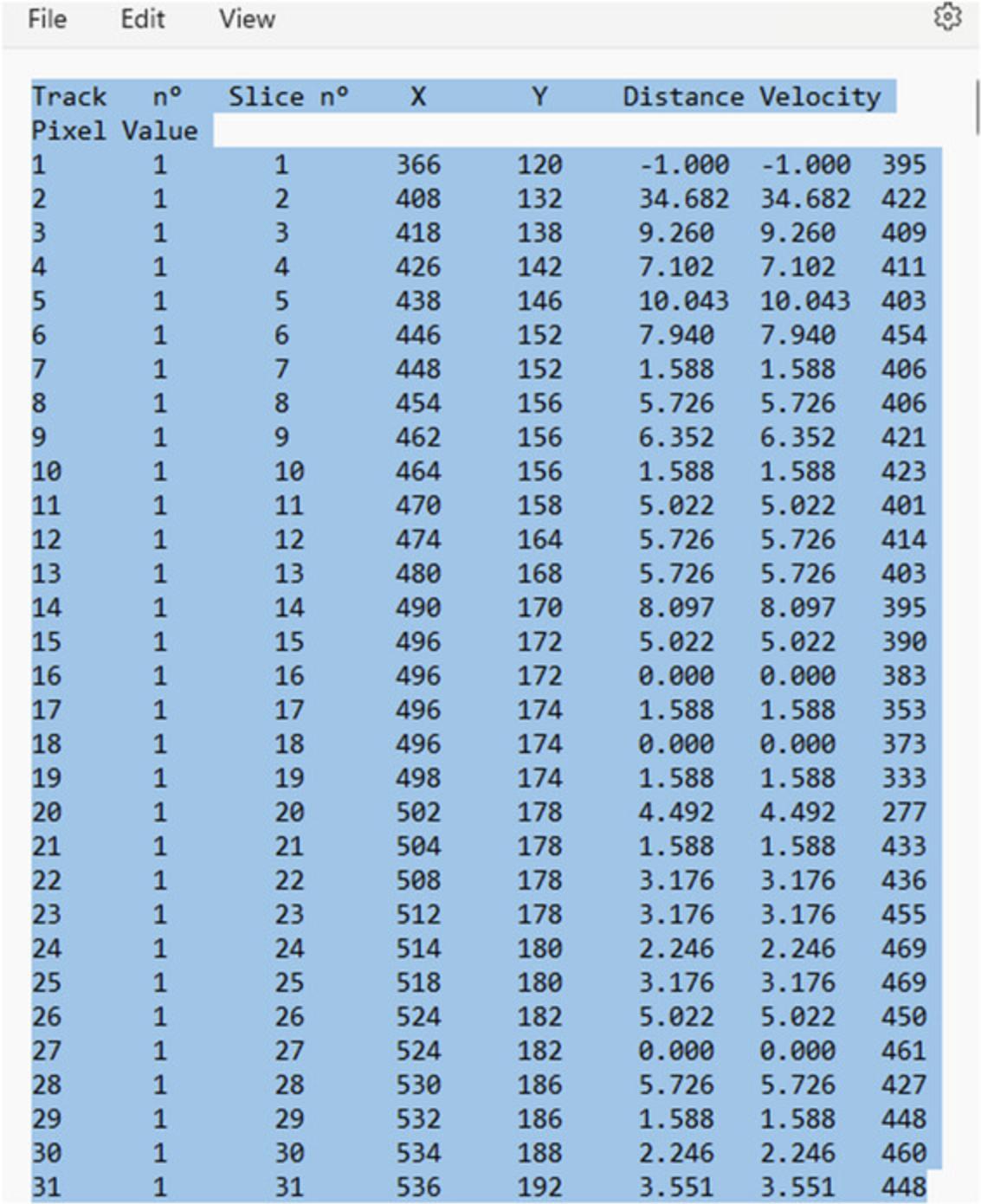
13.Next, we use an ImageJ macro called “FindTrack.jim” to create these individual track files from the MTrack2 output file.
14.The commands are listed below. You will need to edit this macro to designate the location of your MTrack2 output file (“Plugins” > “Macros” > “Edit…”), then run the macro.
15.Designate the beginning and end frames of the track you want to extract, and then save the “results” window that pops up as a .txt file. You can then run the CellTracks script to output MSD, speed, persistence length, directionality, and line plots of the trajectories (Fig. 5).
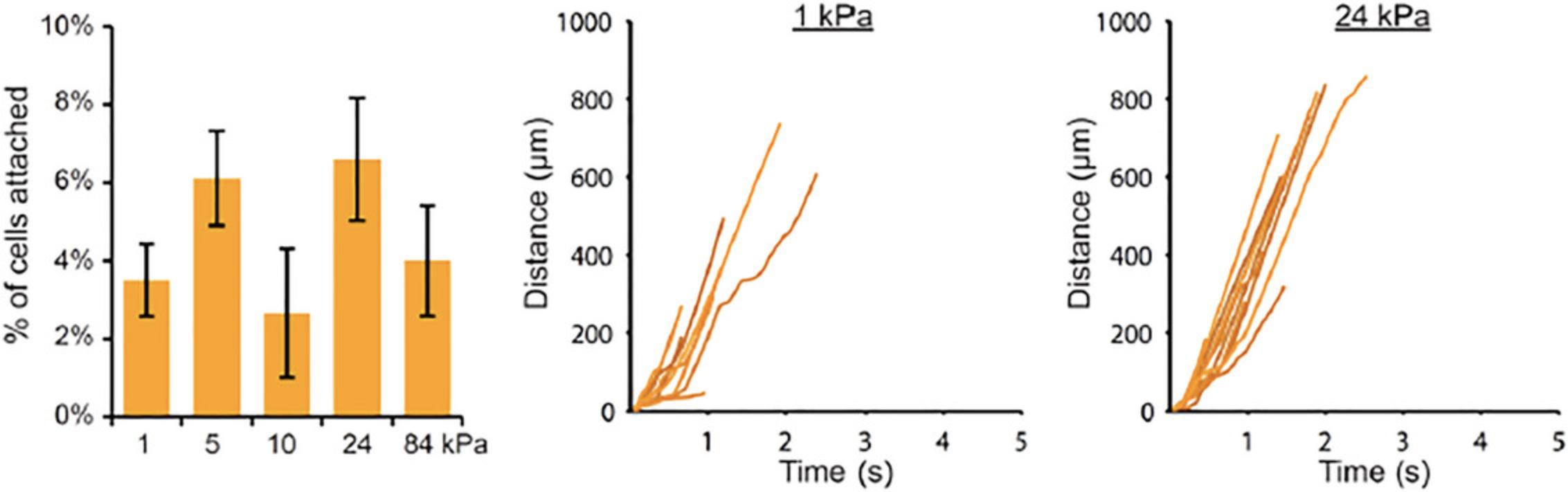
Basic Protocol 5: QUANTIFICATION OF Fc SITE DENSITY ON A SURFACE (e.g., FOR Fc CHIMERAS)
This protocol was designed for quantifying the density of selectin/Fc proteins on stiff polyacrylamide gels, which had first been functionalized with sulfo-SANPAH and coated with protein A/G (Kim & Hammer, 2021; MacKay & Hammer, 2016; Mondal et al., 2014). The assay is sensitive for detecting 2–7 × 1010 molecules per 100 µl of 1-Step Turbo TMB, which equates to a surface density of 20 to 150 molecules/µm2 on polyacrylamide gels with a diameter of 25 mm. This protocol should also work for other Fc fusion proteins and for other surfaces besides polyacrylamide gels, but the following parameters may need to be optimized: concentration of mouse IgG1 isotype control antibody, concentration of HRP-tagged anti-Fc antibody, and the choice of HRP substrate (OPD is less sensitive than TMB, and luminol is more sensitive). Note that this assay does not work well for soft polyacrylamide gels because the background signal (for gels without Fc protein) is very high.
Materials
-
Fluorescently-labeled human IgG1 (Abcam, cat. no. 90283, label with separate kit)
-
1% BSA in PBS (Sigma-Aldrich, cat. no. A5611)
-
Mouse HRP-tagged anti-human IgG1 hinge antibody (Southern Biotech, cat. no. 9052-05)
-
PBS (Cytiva HyClone, cat. no. SH30256.01)
-
1-Step Turbo TMB-ELISA (Thermo Scientific, cat. no. 34022)
-
2 N H2SO4 (Sigma-Aldrich, cat. no. 1.60313)
-
Mouse IgG1 isotype control antibody (any is fine)
-
Nanodrop (Fisher Scientific, cat. no. 840281500)
-
96-well tissue culture treated plates (BD, cat. no. 353072)
-
100- to 200-µl multichannel pipette (Fisher Scientific, cat. no. FBE800010)
-
Humidified incubator, 37°C
-
Plate reader for absorbance and fluorescence
-
Rocking table (Fisher Scientific, cat. no. 02-217-765)
-
Paper towels
-
Microsoft Excel
Preparation of standard curve
A surface coated with Fc protein at a known density is needed to create a standard curve, which converts HRP-mediated color changes into a “number of molecules.” The following procedure uses fluorescently-labeled human IgG1 as the standard. A solution of fluorescent IgG1 at a known concentration is used to coat tissue culture treated plastic 96-well plates. The fluorescence of the solution is measured after coating, and the loss of fluorescence is used to calculate the loss of IgG1 from the solution, which is assumed to adsorb to the plastic plate. This allows the calculation of the number of IgG1 molecules that are adsorbed in each well. Note that you can change the volumes used in this protocol, but always make sure that your solutions completely cover the area of the wells that are coated with fluorescent IgG1.
1.Confirm the stock concentration of fluorescently-labeled IgG1 using the nanodrop, which is sensitive for >0.2 mg/ml.
2.Make serial dilutions of the fluorescent IgG1 in water so that the final volume of each dilution is 240 μl, then distribute each dilution across 6 wells in a 96-well plate (40 μl per well). Place the solution directly in the bottom of the well.
3.Incubate plate in a humidified incubator at 37°C for 30 min (or at room temperature).
4.Take the plate out of the incubator. Place ∼400 μl of 1% BSA in PBS in a fresh column of wells, 1 well per dilution.
5.Use a multichannel pipette to remove exactly 35 μl fluorescent IgG1 solution from each well and combine each dilution together in a fresh column of wells so that the total volume is 210 μl per well.
6.Use the multichannel pipette to remove and discard the remaining fluorescent IgG1 solution from the coated wells. Then, immediately add 50 μl of 1% BSA in PBS to each of the coated wells. Incubate at room temperature for 30 min.
7.During the 30-min incubation:
-
Make fresh serial dilutions of fluorescently labeled IgG1 as in step 2 so that the final volume is 210 μl per well and place it in a fresh column of wells in the same 96-well plate.
-
Measure the fluorescence on a plate reader (for Alexa Fluor 555, using excitation at 555 nm and emission at 580 nm), comparing the recovered solutions from step 5 with these fresh dilutions.
-
Place a plate on a rocker at room temperature to finish the BSA incubation.
8.Add HRP-tagged anti-Fc antibody directly to the wells to achieve a final concentration of 2.5 μg/ml (or the concentration that you choose to use for your samples) and incubate at room temperature for 1 hr.
9.Remove all the solution from the wells by dumping it into the sink and firmly tapping (i.e., banging) onto a pile of paper towels. Use the multichannel pipette to wash the wells several times with 200 μl PBS. Wash 5 times with PBS, incubating each wash for 15 min at room temperature (and removing all solution in between washes).
10.During the washing incubations, take the TMB out of the fridge. In the dark, place 350 μl TMB solution in 2 columns of wells in a new 96-well plate and let the solution reach room temperature. 100 μl of this will be added to each coated well using a multichannel pipette.
11.After the final wash, completely remove all PBS from the wells (by dumping and banging several times on a pile of paper towels). In the dark, use the multichannel pipette to place 100 μl TMB solution in each well coated with IgG1.Incubate for exactly 15 min. The solutions will begin to turn blue.
12.During the 15-min incubation, place 22 μl in each of the 16 wells of 2 N H2SO4.
13.At the end of the 15-min incubation, use the multichannel pipette to transfer the TMB solutions to fresh wells in the other 96-well plate. Then, immediately add 100 μl of 2 N H2SO4 using the multichannel pipette. This will stop the reaction, and the solutions will turn from blue to yellow.
14.Read the absorbance at 450 nm on a plate reader.
Calculations for standard curve (in Excel)
15.Normalize the fluorescence readings from step 7 above (by subtracting the value of the “water only” wells from that of the fluorescent IgG1 wells). Then, make a scatter plot with the concentrations of the fresh IgG1 dilutions on the Y-axis, and the respective fluorescence values on the X-axis. The data points should form a straight line. Add a linear fit trendline and choose to display the equation on the chart.
16.Use the linear fit equation to convert the fluorescence values of the recovered IgG1 solutions into concentration values.
17.Subtract the “recovered” concentration values from the original concentration values (in step 2). This represents the amount of IgG1 that was lost through adsorption to the plastic plate. Multiply the “lost” concentrations by the volume that was originally placed in each well (40 μl) and convert from moles to # of molecules by multiplying by Avogadro's number (6.022 × 1023 molecules/mole). You now have the average number of IgG1 molecules that should have been absorbed to the bottom of each well.
18.Normalize the A450 absorbance values from step 14 above (by subtracting the value of the “water only” wells from the IgG1 coated wells). Average the A450 values for each dilution and plot against the number of IgG1 molecules calculated in the previous step. This is your standard curve (and should be a straight line). Add a linear fit trendline and choose to display the equation on the chart.
19.Repeat this experiment several times to get an accurate standard curve equation.
Procedure for sample surfaces
20.Prepare your surfaces as usual. Also, prepare a control surface without your Fc protein of interest (but include everything else that might be on the surface, such as protein A/G).
21.If your surfaces contain protein A/G (or protein A or protein G), you will need to block any remaining binding sites to prevent the HRP-tagged antibody from binding to the protein A/G through its own Fc domain. Since the isotype of the HRP-tagged antibody is mouse IgG1, incubate your surface with a high concentration of mouse IgG1 isotype control antibody to functionally block the protein A/G.
22.Wash the surfaces with PBS (mostly to remove residual sodium azide).
23.Incubate surfaces in 1% BSA in PBS for 1 hr at room temperature.
24.Add the HRP-tagged anti-human IgG1 antibody in 1% BSA in PBS and incubate for 1 hr at room temperature.
25.Wash the surfaces 5 times with a large volume of PBS (e.g., >2 ml per 25-mm gel), incubating each wash for 15 min at room temperature.
26.During the washing incubations, calculate the amount of TMB solution needed for your surfaces (for 25-mm gels, use 125 μl per gel) and include an additional 1 ml. In the dark, transfer the amount of TMB you need to a tube and let it warm to room temperature.
27.Prepare 2× serial dilutions of the HRP-tagged antibody stock in 5 μl PBS in a 96-well plate, ranging from ∼5 to 50 ng/ml plus a “water only” control.
28.After the final wash, completely remove all PBS from the surfaces. In the dark, add an appropriate volume of TMB to your surfaces and 100 μl TMB to the serially diluted HRP antibody in the 96-well plate. Incubate for 15 min. The solutions will begin to turn blue.
29.At the end of the 15-min incubation, transfer the TMB solutions to fresh wells in the 96-well plate. Then immediately add an equal volume of 2 N H2SO4. This will stop the reactions, and the solutions will turn from blue to yellow. Also add 100 μl of 2 N H2SO4 to the serially diluted HRP antibody.
30.Read the absorbance at 450 nm on a plate reader.
Calculations for samples (in Excel)
31.Normalize the A450 absorbance values from step 10 above (by subtracting the value of the “control surface” wells from the sample wells).
32.Make a scatter plot of the HRP antibody dilution concentrations vs their normalized A450 values.
33.Use your standard curve equation from the fluorescent IgG1 experiments to convert your sample A450 values into the number of HRP antibody molecules per well.
34.Depending on your surface, determine how many HRP-tagged antibody molecules should bind to your Fc protein of interest.
35.Calculate the number of Fc protein molecules that are on your surface and divide by the surface area to give a final site density.
COMMENTARY
Background Information
Polyacrylamide gels
Polyacrylamide gels are multi-faceted materials that provide variegated uses for mechanobiology applications (Denisin & Pruitt, 2016). Specifically, polyacrylamide gels provide versatility by modifying acrylamide and bis-acrylamide concentrations that fine tune stiffness values to physiological and pathological tissue stiffnesses (Paszek et al., 2005). Gels can also be functionalized with ECM proteins (i.e., adhesion ligands, focal adhesion kinases, etc.) to promote and control cell adhesion (Kraning-Rush et al., 2012). For instance, gels have been functionalized with ICAM-1 and VCAM-1, responsible for leukocyte arrest in the leukocyte adhesion cascade, to determine the direction of migration under shear flow when Mac-1 or LFA-1 integrins have been blocked in various types of leukocytes (Buffone et al., 2019; Kim & Hammer, 2021). Therefore, polyacrylamide gels in this protocol provide an ideal substrate to conduct cell rolling experiments with different leukocytes.
Parallel-plate flow chambers
Parallel-plate flow chambers are used to simulate physiological fluid flow and shear stresses on various cell types and, subsequently, their ligands (Yasunaga et al., 2021). These chambers provide modularity in modifying the fluid flow rate to represent different pathological conditions (i.e., turbulent flow due to atherosclerosis, increased blood flow due to hypertension, etc.) (Buffone et al., 2013, 2017; Marathe et al., 2010; Mondal et al., 2014, 2016; Morikis et al., 2017; Stolfa et al., 2016; Wong et al., 2016). The coverslips used in these chambers create the biofunctionalized interface used to mimic and modify the leukocyte adhesion cascade by utilizing polyacrylamide hydrogels functionalized with adhesive ligands (i.e., ICAM-1, VCAM-1) (Buffone et al., 2013, 2017, 2018, 2019; Marathe et al., 2010; Mondal et al., 2014, 2016; Morikis et al., 2017; Stolfa et al., 2016). Thus, parallel-plate flow chambers provide tunability and versatility to be incorporated within our cell rolling experiments.
Critical Parameters
Homogenic polyacrylamide gels
Maintaining smoothness and homogeneity within polyacrylamide gels is critical during the fabrication process. Defects within the polyacrylamide gel may disrupt fluid flow and prevent cell attachment to the substrate. Therefore, gels should be fabricated in a clean environment, and all solutions should be sterile filtered before fabrication.
Sealing of parallel plate flow chamber
The seal of the parallel plate flow chamber must be airtight to ensure laminar flow within the chamber and to maintain a uniform channel thickness. For surfaces incorporating PDMS, a supplemental vacuum pump is not necessary as the PDMS generates a self-sufficient seal. For surfaces utilizing glass coverslips, a vacuum pump is necessary to generate the airtight seal. When aligning the gasket with the chamber, if air bubbles are trapped between this interface, smooth them out.
Concentration of antibody for surface conjugation
A saturating concentration of antibodies is required for surface preparation. For example, 2.5 µg/ml HRP-tagged antibody is sufficient for surface preparation to quantify fragment crystallizable regions. However, a higher concentration of antibodies may give a higher background. Therefore, optimization may be necessary for use with different antibodies.
Control surface
A control surface must be prepared when quantifying Fc density on stiff polyacrylamide gels (Basic Protocol 5). The surface must contain all parameters that are used to generate the Fc site densities, without the Fc protein of interest. In substitution, mouse IgG isotype control antibody can therefore be used when preparing the surfaces. Typically, 20 to 50 µg/ml of IgG isotype control antibody will satisfy the control surface, but higher concentrations may work better.
Optimization of protein coating
If you need to increase the amount of protein conjugated to the gel surface, there are several parameters you can try changing (in addition to simply increasing the protein concentration). First, the spotlight UV lamp (UVP B-100AP) is much stronger than the rectangular lamp (Spectroline EN-160 L) and may increase sulfo-SANPAH conjugation to the gel. If you use this lamp, pre-warm it for ∼10 min and raise your plate as close to the bulb as possible. You should only activate 4 gels at once because of the shape of the bulb. Second, since the NHS ester reaction is pH dependent, the buffer used to dilute the sulfo-SANPAH, the solution used to wash the gels after UV exposure, and the buffer used in the protein solution should be pH 7 to 9. Third, reduce the concentration of sulfo- SANPAH. If unbound sulfo-SANPAH is not removed during the washing step, it could react with the protein and prevent its conjugation to the gel.
Troubleshooting
Potential problems in conducting the Protocols, along with the possible causes and solutions, are shown in Table 3.
Problem | Possible cause | Solution |
---|---|---|
Hard to peel off hydrophobic top coverslip | Incomplete Rain-X treatment | Apply Rain-X with Kimwipe after dunking |
Gel is not completely polymerized | Use longer tweezers to remove coverslip | |
Allow longer time for polymerization | ||
Cells do not survive cryopreservation step | Not enough FBS | Add more FBS (12%-15%) |
Too much DMSO | Lower DMSO concentration to 8% | |
Not enough cells interact with surface | Improper coating with selectins | Increase concentration of selectin coated |
Cells interact with surface non-specifically | Increase Pluronic F-127 concentration to 0.3% (w/v) | |
Flow rate is too high | Reduce shear rate to 100 s−1 |
Understanding Results
Interpreting the data produced from selectin rolling assays involves examining the images produced of a certain area in the microfluidic chamber over a defined length of time (normally ∼5 min) and analyzing the movement of the cells over the course of the frames. A single cell trajectory is calculated by tracking the path of a cell through all the frames acquired using the TrackMate function in ImageJ. This produces X- and Y-coordinates for each cell track at a given time. The entirety of the tracked cells can then be plugged into a MATLAB script to calculate mean squared displacement (MSD), speed, persistence time and length, directionality, and number of interacting cells per area.
Different cell conditions, such as antibody blocking, or knockouts, can be compared as a one-way ANOVA to the wildtype cells.
Time Considerations
Basic Protocols 1 and 3 together can be completed within 4 to 5 hr so long as the set-up of the microfluidic device and preparation of the cells happens simultaneously. Basic Protocol 2 or Alternate Protocols 1 and 2 need to be started the day before and left overnight before performing Basic Protocol 3. Each microfluidic experiment takes ∼10 min to acquire once started. Basic Protocol 4 can be accomplished in a matter of 1 to 2 hr, but it is dependent on the number of samples. Basic protocol 5 takes ∼5 to 6 hr on average.
Acknowledgments
This work was supported by NIH GM143357 to A.B.J.
Author Contributions
Zeinab Amoabediny : Conceptualization; visualization; writing original draft; writing review and editing. Aman Mittal : Conceptualization; visualization; writing original draft; writing review and editing. Subham Guin : Visualization; writing original draft; writing review and editing. Alexander Buffone : Conceptualization; data curation; funding acquisition; project administration; supervision; writing original draft; writing review and editing.
Conflict of Interest
The authors declare that the research was conducted in the absence of any commercial or financial relationships that could be construed as a potential conflict of interest.
Open Research
Data Availability Statement
Data sharing is not applicable to this article as no new data were created or analyzed in this study.
Literature Cited
- Buffone, A., Jr, Anderson, N. R., & Hammer, D. A. (2018). Migration against the direction of flow is LFA-1-dependent in human hematopoietic stem and progenitor cells. Journal of Cell Science , 131(1), jcs205575. https://doi.org/10.1242/jcs.205575
- Buffone, A., Jr, Hammer, D. A., Kim, S. H. J., Anderson, N. R., Mochida, A., Lee, D.-H., & Guin, S. (2023). Not all (cells) who wander are lost: Upstream migration as a pervasive mode of amoeboid cell motility. Frontiers in Cell and Developmental Biology , 11, 1291201. https://doi.org/10.3389/fcell.2023.1291201
- Buffone, A., Jr, Anderson, N. R., & Hammer, D. A. (2019). Human neutrophils will crawl upstream on ICAM-1 if Mac-1 is blocked. Biophysical Journal , 117(8), 1393–1404. https://doi.org/10.1016/j.bpj.2019.08.044
- Buffone, A., Jr, Mondal, N., Gupta, R., McHugh, K. P., Lau, J. T. Y., & Neelamegham, S. (2013). Silencing α1,3-fucosyltransferases in human leukocytes reveals a role for FUT9 enzyme during E-selectin-mediated cell adhesion. Journal of Biological Chemistry , 288(3), 1620–1633. https://doi.org/10.1074/jbc.M112.400929
- Buffone, A., Jr, Nasirikenari, M., Manhardt, C. T., Lugade, A., Bogner, P. N., Sackstein, R., Thanavala, Y., Neelamegham, S., & Lau, J. T. Y. (2017). Leukocyte-borne α(1,3)-fucose is a negative regulator of β2-integrin-dependent recruitment in lung inflammation. Journal of Leukocyte Biology , 101(2), 459–470. https://doi.org/10.1189/jlb.3A0516-215RR
- Chase, S. D., Magnani, J. L., & Simon, S. I. (2012). E-selectin ligands as mechanosensitive receptors on neutrophils in health and disease. Annals of Biomedical Engineering , 40(4), 849–859. https://doi.org/10.1007/s10439-011-0507-y
- Denisin, A. K., & Pruitt, B. L. (2016). Tuning the range of polyacrylamide gel stiffness for mechanobiology applications. ACS Applied Materials & Interfaces, 8(34), 21893–21902. https://doi.org/10.1021/acsami.5b09344
- Eash, K. J., Greenbaum, A. M., Gopalan, P. K., & Link, D. C. (2010). CXCR2 and CXCR4 antagonistically regulate neutrophil trafficking from murine bone marrow. The Journal of Clinical Investigation , 120(7), 2423–2431. https://doi.org/10.1172/JCI41649
- Hidalgo, A., Peired, A. J., Wild, M., Vestweber, D., & Frenette, P. S. (2007). Complete identification of E-selectin ligand activity on neutrophils reveals a dynamic interplay and distinct functions of PSGL-1, ESL-1, and CD44. Immunity , 26(4), 477–489. https://doi.org/10.1016/j.immuni.2007.03.011
- Huttenlocher, A., & Horwitz, A. R. (2011). Integrins in cell migration. Cold Spring Harbor Perspectives in Biology , 3(9), a005074. https://doi.org/10.1101/cshperspect.a005074
- Jenne, C. N., Liao, S., & Singh, B. (2018). Neutrophils: Multitasking first responders of immunity and tissue homeostasis. Cell and Tissue Research , 371(3), 395–397. https://doi.org/10.1007/s00441-018-2802-5
- Kim, S. H. J., & Hammer, D. A. (2021). Integrin cross-talk modulates stiffness-independent motility of CD4+ T lymphocytes. Molecular Biology of the Cell , 32(18), 1749–1757. https://doi.org/10.1091/mbc.E21-03-0131
- Kolaczkowska, E., & Kubes, P. (2013). Neutrophil recruitment and function in health and inflammation. Nature Reviews Immunology , 13, 159. https://doi.org/10.1038/nri3399
- Kraning-Rush, C. M., Califano, J. P., & Reinhart-King, C. A. (2012). Cellular traction stresses increase with increasing metastatic potential. PLoS ONE , 7(2), e32572. https://doi.org/10.1371/journal.pone.0032572
- Ley, K., Laudanna, C., Cybulsky, M. I., & Nourshargh, S. (2007). Getting to the site of inflammation: The leukocyte adhesion cascade updated. Nature Reviews Immunology , 7(9), 678–689. https://doi.org/10.1038/nri2156
- Lo, C. Y., Antonopoulos, A., Dell, A., Haslam, S. M., Lee, T., & Neelamegham, S. (2013). The use of surface immobilization of P-selectin glycoprotein ligand-1 on mesenchymal stem cells to facilitate selectin mediated cell tethering and rolling. Biomaterials , 34(33), 8213–8222. https://doi.org/10.1016/j.biomaterials.2013.07.033
- Lo, C. Y., Antonopoulos, A., Gupta, R., Qu, J., Dell, A., Haslam, S. M., & Neelamegham, S. (2013). Competition between Core-2 GlcNAc-transferase and ST6GalNAc-transferase regulates the synthesis of the leukocyte selectin ligand on human P-selectin glycoprotein ligand-1. Journal of Biological Chemistry , 288(20), 13974–13987. https://doi.org/10.1074/jbc.M113.463653
- Lo, C. Y., Weil, B. R., Palka, B. A., Momeni, A., Canty, J. M. Jr., & Neelamegham, S. (2016). Cell surface glycoengineering improves selectin-mediated adhesion of mesenchymal stem cells (MSCs) and cardiosphere-derived cells (CDCs): Pilot validation in porcine ischemia- reperfusion model. Biomaterials , 74, 19–30. https://doi.org/10.1016/j.biomaterials.2015.09.026
- Luo, D., McGettrick, H. M., Stone, P. C., Rainger, G. E., & Nash, G. B. (2015). The roles of integrins in function of human neutrophils after their migration through endothelium into interstitial matrix. PLoS ONE , 10(2), e0118593. https://doi.org/10.1371/journal.pone.0118593
- MacKay, J. L., & Hammer, D. A. (2016). Stiff substrates enhance monocytic cell capture through E-selectin but not P-selectin. Integrative Biology , 8(1), 62–72. https://doi.org/10.1039/C5IB00199D
- Malý, P., Thall, A. D., Petryniak, B., Rogers, C. E., Smith, P. L., Marks, R. M., Kelly, R. J., Gersten, K. M., Cheng, G., Saunders, T. L., Camper, S. A., Camphausen, R. T., Sullivan, F. X., Isogai, Y., Hindsgaul, O., von Andrian, U. H., & Lowe, J. B. (1996). The α(1,3)Fucosyltransferase Fuc-TVII controls leukocyte trafficking through an essential role in L-, E-, and P-selectin ligand biosynthesis. Cell , 86(4), 643–653. https://doi.org/10.1016/S0092-8674(00)80137-3
- Marathe, D. D., Buffone, A., Chandrasekaran, E. V., Xue, J., Locke, R. D., Nasirikenari, M., Lau, J. T. Y., Matta, K. L., & Neelamegham, S. (2010). Fluorinated per-acetylated GalNAc metabolically alters glycan structures on leukocyte PSGL-1 and reduces cell binding to selectins. Blood , 115(6), 1303–1312. https://doi.org/10.1182/blood-2009-07-231480
- Mondal, N., Buffone, A., Stolfa, G., Antonopoulos, A., Lau, J. T. Y., Haslam, S. M., Dell, A., & Neelamegham, S. (2014). ST3Gal-4 is the primary sialyltransferase regulating the synthesis of E-, P-, and L-selectin ligands on human myeloid leukocytes. Blood , 125(4), 687–696. https://doi.org/10.1182/blood-2014-07-588590
- Mondal, N., Buffone, A., Jr, & Neelamegham, S. (2013). Distinct glycosyltransferases synthesize E-selectin ligands in human vs. mouse leukocytes. Cell Adhesion & Migration, 7(3), 288–292. https://doi.org/10.4161/cam.24714
- Mondal, N., Dykstra, B., Lee, J., Ashline, D., Reinhold, V. N., Rossi, D. J., & Sackstein, R. (2018). Distinct human α(1,3)-fucosyltransferases drive Lewis-X/sialyl Lewis-X assembly in human cells. Journal of Biological Chemistry , 293(19), 7300–7314. https://doi.org/10.1074/jbc.RA117.000775
- Mondal, N., Stolfa, G., Antonopoulos, A., Zhu, Y., Wang, S.-S., Buffone, A., Atilla-Gokcumen, G. E., Haslam, S. M., Dell, A., & Neelamegham, S. (2016). Glycosphingolipids on human myeloid cells stabilize E-selectin–dependent rolling in the multistep leukocyte adhesion cascade. Arteriosclerosis, Thourombosis, and Vascular Biology , 36(4), 718–727. https://doi.org/10.1161/atvbaha.115.306748
- Morikis, V. A., Chase, S., Wun, T., Chaikof, E. L., Magnani, J. L., & Simon, S. I. (2017). Selectin catch-bonds mechanotransduce integrin activation and neutrophil arrest on inflamed endothelium under shear flow. Blood , 130(19), 2101. https://doi.org/10.1182/blood-2017- 05–783027.
- Nimrichter, L., Burdick, M. M., Aoki, K., Laroy, W., Fierro, M. A., Hudson, S. A., Von Seggern, C. E., Cotter, R. J., Bochner, B. S., Tiemeyer, M., Konstantopoulos, K., & Schnaar, R. L. (2008). E-selectin receptors on human leukocytes. Blood , 112(9), 3744. https://doi.org/10.1182/blood-2008-04-149641
- Paszek, M. J., Zahir, N., Johnson, K. R., Lakins, J. N., Rozenberg, G. I., Gefen, A., Reinhart-King, C. A., Margulies, S. S., Dembo, M., Boettiger, D., Hammer, D. A., & Weaver, V. M. (2005). Tensional homeostasis and the malignant phenotype. Cancer Cell , 8(3), 241–254. https://doi.org/10.1016/j.ccr.2005.08.010
- Rowe, R. G., & Weiss, S. J. (2008). Breaching the basement membrane: Who, when and how? Trends in Cell Biology , 18(11), 560–574. https://doi.org/10.1016/j.tcb.2008.08.007
- Springer, T. A. (1994). Traffic signals for lymphocyte recirculation and leukocyte emigration: The multistep paradigm. Cell , 76(2), 301–314. https://doi.org/10.1016/0092-8674(94)90337-9
- Stolfa, G., Mondal, N., Zhu, Y., Yu, X., Buffone, A., & Neelamegham, S. (2016). Using CRISPR- Cas9 to quantify the contributions of O-glycans, N-glycans and glycosphingolipids to human leukocyte-endothelium adhesion. Scientific Reports , 6, 30392. https://doi.org/10.1038/srep30392
- Wilkins, P. P., McEver, R. P., & Cummings, R. D. (1996). Structures of the O-glycans on P- selectin glycoprotein ligand-1 from HL-60 cells. Journal of Biological Chemistry , 271(31), 18732–18742. https://doi.org/10.1074/jbc.271.31.18732
- Wong, A. K., P, L. L., Boroda, N., Rosenberg, S. R., & Rabbany, S. Y. (2016). A parallel-plate flow chamber for mechanical characterization of endothelial cells exposed to laminar shear stress. Cellular and Molecular Bioengineering , 9(1), 127–138. https://doi.org/10.1007/s12195-015-0424-5
- Yasunaga, A. B., Murad, Y., Kapras, V., Menard, F., & Li, I. T. S. (2021). Quantitative interpretation of cell rolling velocity distribution. Biophysical Journal , 120(12), 2511–2520. https://doi.org/10.1016/j.bpj.2021.04.021
- Yoshimura, T. (2007). Chemokine receptors and neutrophil trafficking. In J. K. Harrison & N. W. Lukacs (Eds.), The chemokine receptors (pp. 71–86). Humana Press. https://doi.org/10.1007/978-1-59745-020-1_5
Internet Resources
- http://imagej.nih.gov/ij/
- The ImageJ site contains subsections pertaining to downloading instructions, as well as tutorials for software use. The ImageJ software is compatible with Mac OS X, Linux, and Windows based computer operating systems.
- https://imagej.net/plugins/mtrack2
- The MTrack2 plugin site will direct you to the installation instructions for the MTrack2 plug-in, as well as a testing dialogue to set initial parameters.
- https://www.mathworks.com/products/matlab.html
- The MATLAB website contains instructions for downloading the MATLAB software, as well as creating an account, if necessary. The MATLAB software is compatible with Mac OS X, Linux, and Windows.