Isolation of Extracellular Vesicles from Phloem Sap by Size Exclusion Chromatography
Elisa Garzo, Elisa Garzo, Christian M. Sánchez-López, Christian M. Sánchez-López, Alberto Fereres, Alberto Fereres, Carla Soler, Carla Soler, Antonio Marcilla, Antonio Marcilla, Pedro Pérez-Bermúdez, Pedro Pérez-Bermúdez
Abstract
Extracellular vesicles (EVs) are nanoparticles that are released by cells and participate in the transfer of information. It is now known that EVs from mammalian cells are involved in different physiological and pathophysiological processes (antigen presentation, tissue regeneration, cancer, inflammation, diabetes, etc.). In the past few years, several studies on plants have demonstrated that EVs are also key tools for plant intercellular and cross-kingdom communications, suggesting that these nanostructures may contribute to distinct aspects of plant physiology such as development, defense, reproduction, symbiotic relationships, etc. These findings are challenging the traditional view of signaling in plants.
EVs are probably involved in the phloem's transport system, since this vascular tissue plays a crucial role in translocating nutrients, defensive compounds, and informational signals throughout the plant. The collection of phloem is experimentally challenging because sap is under high turgor pressure inside the sieve elements, which have a small diameter and are hidden within the plant organs. The goals of this work are to develop new protocols that allow us to detect EVs for the first time in the phloem of the plants, and to isolate these nanovesicles for in-depth analysis and characterization.
Our protocols describe two distinct methods to collect the phloem sap from rice and melon. The first method (Basic Protocol 1) involves ‘Aphid stylectomy by radiofrequency microcautery’ using rice plants and the aphid Sitobion avenae. This is considered the least invasive method for collecting phloem sap. The second method, ‘Stem incision’, involves cutting the stem of melon plants for collecting the exuded sap. Phloem sap EVs are then isolated by size exclusion chromatography. The results obtained in this study represent the first report on typical EVs isolated from in vivo –collected phloem sap. © 2023 The Authors. Current Protocols published by Wiley Periodicals LLC.
Basic Protocol 1 : Isolation of EVs from phloem sap: Aphid stylectomy by radiofrequency microcautery
Basic Protocol 2 : Isolation of EVs from phloem sap: Stem incision method
INTRODUCTION
Plants have evolved two major vascular tissues, phloem and xylem, that allow long-distance transport. Phloem translocation is driven by mass flow between source and sink tissues, which are connected by conductive specialized cells, the sieve elements (SEs). This transport route distributes photosynthates and small compounds (sugars, amino acids, inorganic ions, organic acids, etc.), but also signaling molecules such as phytohormones, proteins, and RNAs (Buhtz et al., 2008; Lough & Lucas, 2006; Kehr & Buhtz, 2008), which could act as long-distance signals regulating important plant physiological processes such as growth and development, microbe symbiosis, defense, and stress responses.
Since SEs lose most of their organelles, including nuclei and ribosomes, during their differentiation, these cells are not able to synthesize complex molecules which might be produced in source parenchyma cells, in immature SEs, or in the specialized companion cells (CCs) of SEs. It has been suggested that macromolecules such as proteins and transcripts could be loaded into the phloem vessels through the specialized plasmodesmata (PD) connecting CCs to SEs, and these PD might constitute a symplastic channel for transfer of extracellular vesicles/exosomes between cells (Kehr & Kragler, 2018).
Extracellular vesicles (EVs) are nanometer-sized structures, released by all living cells and delimited by lipid bilayer membranes, that can be classified into subtypes based on cell origin, morphology, and size (Margolis & Sadovsky, 2019; Théry et al., 2018). Originally, it was thought that EVs functioned as a waste disposal system, but it has been demonstrated that EVs act as safe vehicles for short- or long-distance transport of signaling molecules (proteins, lipids, nucleic acids, and other metabolites) within one organism, or play prominent roles in communication between interacting organisms (interkingdom communication; He et al., 2021).
A major limitation in the study of EVs is the lack of standardization for the already challenging techniques that have been used to isolate them. For this reason, the International Society for Extracellular Vesicles (ISEV) has published guidelines trying to standardize EV isolation and characterization techniques (Lötvall et al., 2014; Théry et al., 2018). In this sense, size exclusion chromatography (SEC) is a promising approach for the isolation of EVs that has been successfully used with a large variety of biological fluids. Moreover, compared to other procedures, SEC has increased the purity and functionality of the isolated nanovesicles (Benedikter et al., 2017; Sidhom et al., 2020), although this technique has been scarcely used for the isolation of plant-derived EVs (Bokka et al., 2020; Chen et al., 2022; Kim & Rhee, 2021; Sánchez-López et al., 2022; You et al., 2021).
The aim of this study is to provide the tools to demonstrate the presence of EVs in phloem sap. Here we describe the sap collection methods and the further isolation and purification of phloem sap EVs (PhlEVs) by SEC. Phloem sap is under high hydrostatic pressure inside SEs, and it is difficult to collect because SEs have a small diameter and are hidden within the plant organs. Two different sap-collection strategies have been used to demonstrate, for the first time, that EVs are present in phloem sap of different plant species, as described in the following paragraphs.
Aphid stylectomy by radiofrequency microcautery (RFM; Basic Protocol 1)
The phloem sap obtained using these insects is pure, although the small quantities of collected exudates (∼0.5-2.0 μl/plant) permit EV visualization by transmission electron microscopy (TEM) and nanoparticle tracking analysis (NTA), but do not allow further EV characterization. We have applied aphid stylectomy to rice plants.
Stem incision method (Basic Protocol 2)
This method is based on spontaneous phloem bleeding produced by cutting the stem, or fruits, of several plant species (i.e., cucurbits). In the present research, this method was applied to stems of melon plants, and PhlEVs were isolated and purified from sap by SEC.
Basic Protocol 1: ISOLATION OF EVs FROM PHLOEM SAP: APHID STYLECTOMY BY RADIOFREQUENCY MICROCAUTERY
This protocol describes a recommended process for collecting pure phloem sap from rice plants (Oryza sativa L.; Fig. 1). The Electrical Penetration Graph (EPG) technique (Tjallingii, 1978, 1985) is used to monitor the feeding behavior of the aphids. When the aphids show phloem activities—salivation followed by passive phloem sap ingestion—the stylets of the aphids are amputated by RFM stylectomy (Downing & Unwin, 1977). Sap containing pure SEs is collected as it exudes from the stump of aphid stylets. The samples are analyzed by TEM and NTA.
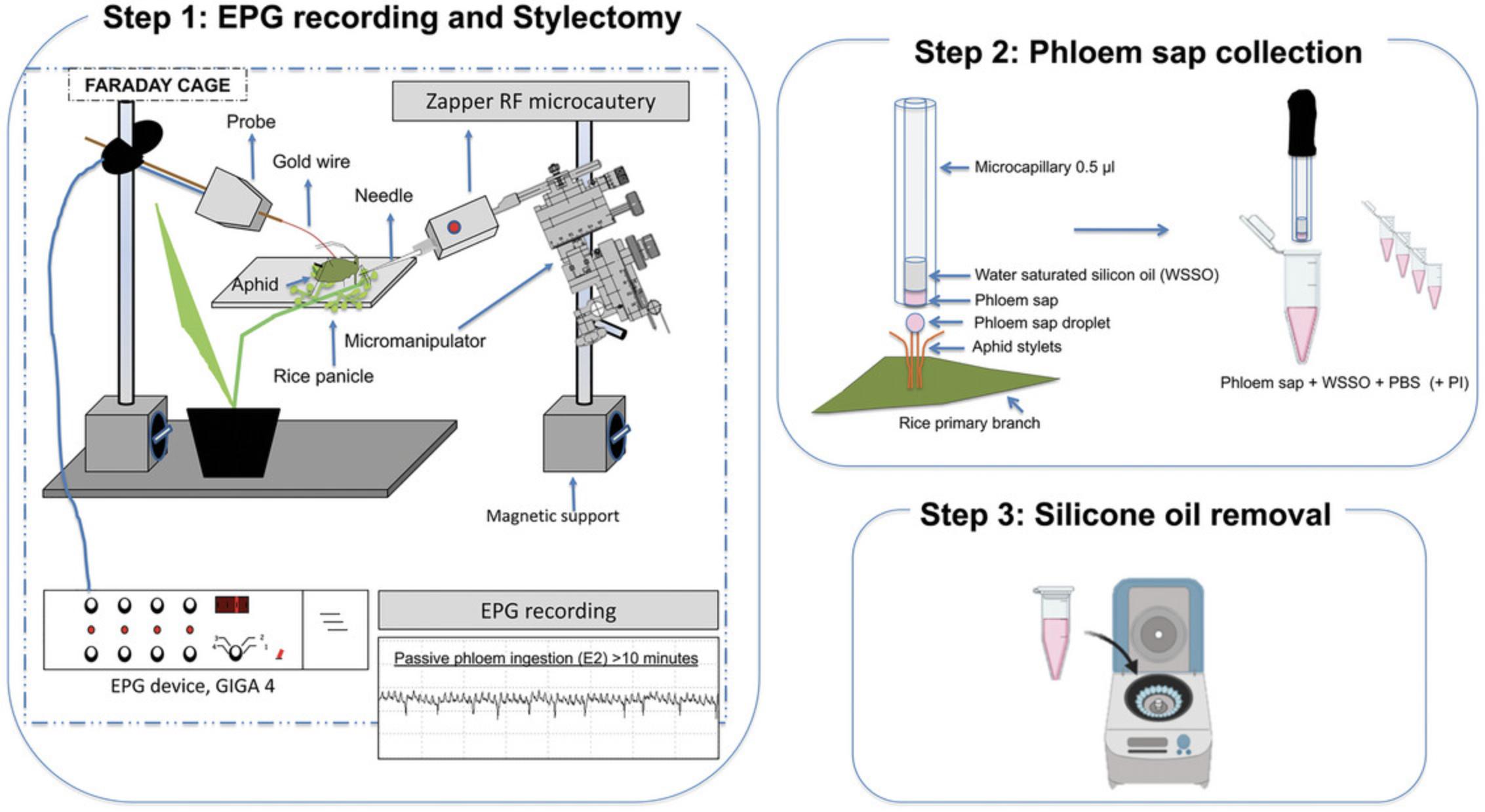
Materials
-
Rice plants (Oryza sativa L., cv. Sendra)
-
Barley plants (Hordeum vulgare L., cv. Shakira)
-
Soil:vermiculite mixture: (2:1soil (Jiffy Tref GO V4) and vermiculite no. 3
-
Plant fertilizer (20-20-20 NPK Nutrichem, Miller Chemical & Fertilizer Corp., Pennsylvania)
-
Aphids (Sitobion avenae F.; Hemiptera: Aphididae); population was supplied by Koppert Biological Systems in 2013)
-
Silicone oil (Sigma-Aldrich, St. Louis, USA)
-
Filtered (0.22 µm) 10 mM phosphate buffered saline (PBS), pH = 7.4 (see recipe)
-
Protease inhibitor (PI, cOmplete™ Mini, EDTA-free Protease Inhibitor Cocktail, Roche)
-
Petri dishes
-
Plastic pots (8 × 8 cm)
-
Plastic pots (13-cm diameter)
-
Walk-in environmental growth chamber
-
Electrical Penetration Graph (EPG) device (https://www.epgsystems.eu)
-
Vacuum pump Dymax 5 (Charles Austen Pumps Ltd, UK)
-
Gold wire, 18.5 μm in diameter, copper wire 2 cm length, and silver conductive paint to attach the gold wire to the aphid dorsum (Manual-Giga-8d.pdf; http://www.epgsystems.eu/downloads, section Manuals)
-
Zapper Radio Frequency Microcautery (RFM) unit (https://www.aphidzapper.com)
-
Microcapillary tubes, 32 mm length and 0.5 μl volume (Drummond Microcaps)
-
Micromanipulator (M3301R from World Precision Instruments)
-
Binocular microscope
-
USB analog/digital converter card (DI-158U; DATAQ Instruments, Akron, Ohio)
-
Stylet+ software (EPG Systems) for Windows
-
GVS nylon syringe filters, 0.22 µm (Thermo Fisher Scientific)
-
200-µl microtubes (Bio-Rad)
Rice and barley plants growth
1.Germinate 10 rice seeds (O. sativa cv. Sendra) in Petri dishes and transplant the plantlets into small pots (8 × 8 cm) with a mixture of soil and vermiculite. Maintain the plants in an insect-free walk-in environmental growth chamber under controlled conditions of temperature [24:20°C day:night (D:N)], relative humidity (80% RH), and photoperiod [16:8 hr light:darkness (L:D)].
2.Germinate 30 barley seeds in pots (13 cm in diameter) under the same conditions as described above.
3.Water seeds and plantlets three times a week using a fertilizer (dose of 0.25 g/L) rich in nitrogen, phosphorus, and potassium (20:20:20).
4.Grow rice plants up to phenological stage of seed development, when they are used to obtain pure phloem sap by stylectomy.
5.Grow barley plants to be used to rear and maintain the aphid colonies.
Aphid culture
6.Establish cultures of the English grain aphid, Sitobion avenae , which are reared on barley plants and maintained in growth chambers with a 70% RH at 23:18°C and 14:10 hr photoperiod (L:D).
7.Use only one adult apterous aphid (9-10 days old) to collect phloem sap from each rice plant as described below.
Phloem sap collection from rice plants: Aphid stylectomy by radiofrequency microcautery
8.Immobilize an adult aphid with a suction device. Attach a gold wire to the dorsum of the aphid using water-based silver glue. Attach the other end of the gold wire to a copper wire and connect to the Electrical Penetration Graph (EPG) device.
9.Place the aphid on the primary branch of a rice panicle (rice plant in the phenological stage of seed development).
10.Monitor the feeding behavior of the young aphids using the EPG technique.
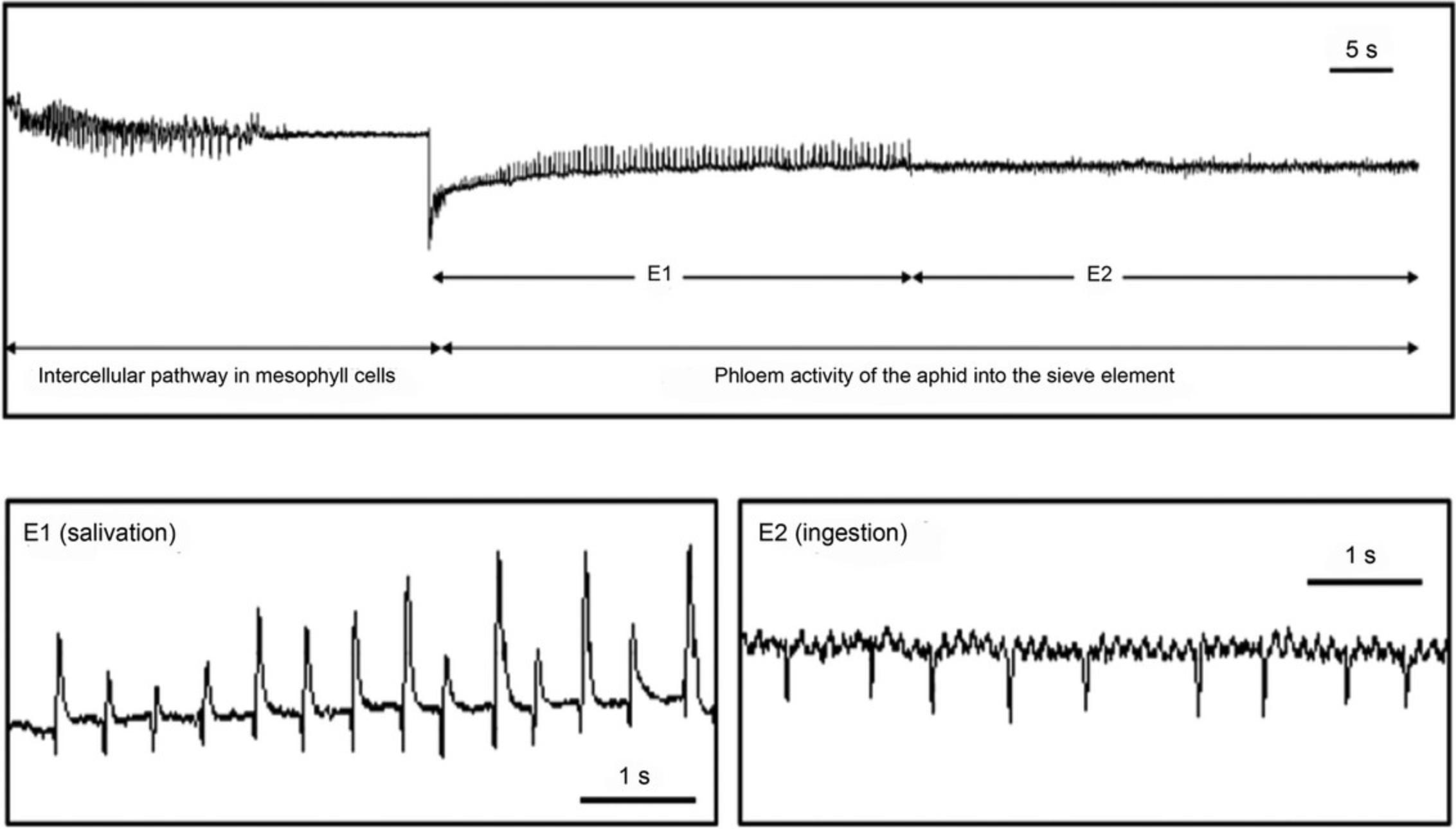
11.Proceed to amputation of stylets using the tungsten needle of the Zapper RFM unit mounted on a micromanipulator and moved under observation by a binocular microscope. After confirming that the aphid shows passive phloem sap ingestion (E2 waveform) using the EPG technique, move the tip of the needle until it touches the labium of the aphid (Fig. 3A). Immediately trigger the RFM unit pulse using the foot switch (see Downing & Unwin, 1977).
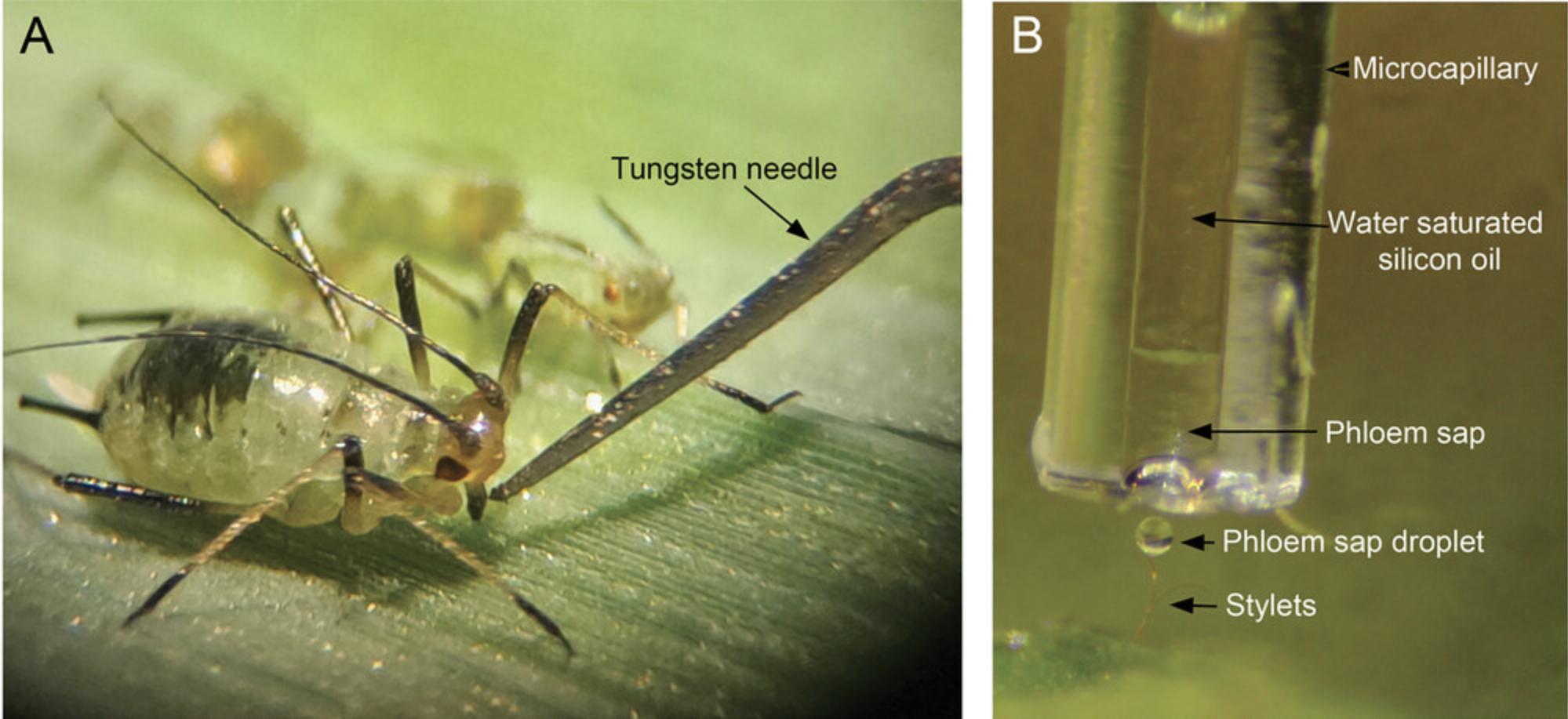
12.Collect sap droplets as they exude from the stump of the amputated aphid stylets. Collect sap samples into the bottom part of the microcapillary tube held in a micromanipulator (Fig. 3B).
13.Place each sap sample (∼0.6-1.9 μl/plant) in a separate 200-µl microtube; dilute each sample with 8 µl of filtered PBS (10 mM, pH = 7.4), with or without proteinase inhibitor (PI).
Basic Protocol 2: ISOLATION OF EVs FROM PHLOEM SAP: STEM INCISION METHOD
Basic Protocol 1 leads to the collection of pure phloem sap obtained directly from the single sieve element in rice plants. Nevertheless, stylectomy is a complicated method and only a small volume of phloem sap is obtained. The following Basic Protocol 2 describes the stem incision method (Fig. 4), which is easier and faster than stylectomy. This method is applied to melon plants, which spontaneously exude abundant phloem sap after cutting of the phloem vessels. PhlEVs are isolated and purified from sap by SEC and analyzed by NTA and TEM.
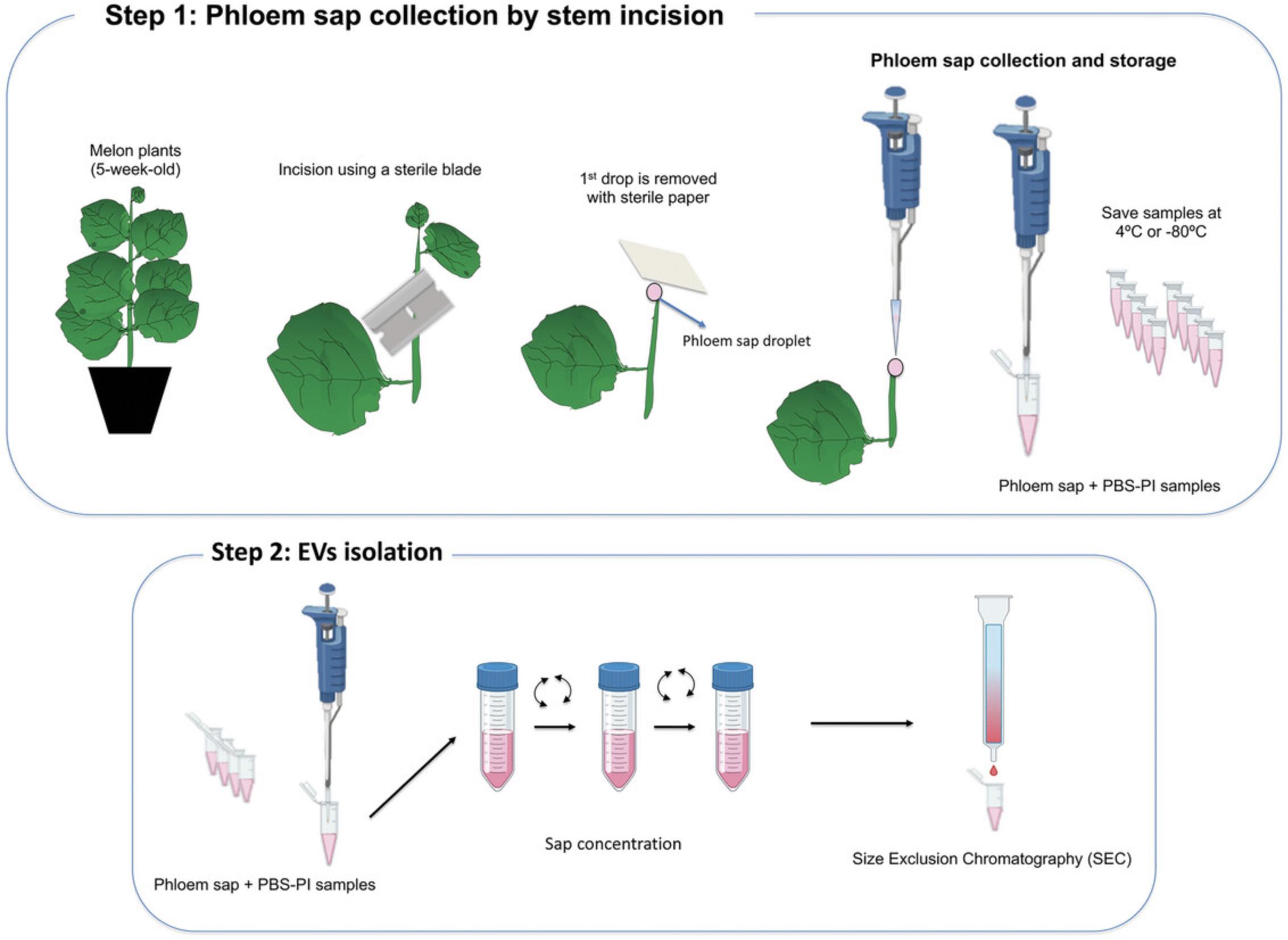
Materials
-
Melon seeds and plants (Cucumis melo L., cv. Bazan, or other cultivars)
-
Soil:vermiculite mixture (2:1)
-
Plant fertilizer (20-20-20 NPK Nutrichem, Miller Chemical & Fertilizer Corp., Pennsylvania)
-
Filtered (0.22 µm) 10 mM phosphate buffered saline (PBS), pH = 7.4 (see recipe)
-
Protease inhibitor (PI, cOmplete™ Mini, EDTA-free Protease Inhibitor Cocktail, Roche)
-
Sepharose-CL2B (Sigma-Aldrich)
-
Plastic pots (15 cm diameter)
-
Walk-in environmental growth chambers for plants
-
GVS nylon syringe filters, 0.22 µm (Thermo Fisher Scientific)
-
Sterile razor blades
-
Sterile filter paper
-
Set of micropipettes
-
Sterile micropipette tips (100-200 μl)
-
Permanent marker
-
Racks for 1.5-ml microtubes
-
1.5-ml polypropylene tubes (Eppendorf)
-
5-10 ml syringes for membrane filtration
-
SEC polypropylene column with porous filter at bottom (MilliporeSigma-Supelco)
-
Laboratory stand
-
Beaker
-
Plastic Pasteur pipettesAmicon Ultra 4 ml (10 kDa) centrifugal filters (Millipore-Sigma-Aldrich)
-
SPE columns, 1 ml, 54220-U (Supelco)
-
Centrifuge (Sorvall)
Melon plants growth
1.Germinate melon seeds (Cucumis melo L., cv. Bazan) in pots with a mixture of soil:vermiculite (2:1). The seeds and plants are grown in a walk-in environmental growth chamber under day:night controlled conditions: 24:20°C, 60%-80% RH and 16:8 hr (L:D) photoperiod.
2.Water plants three times a week using a fertilizer (dose of 0.25 g/L) rich in nitrogen, phosphorus, and potassium.
3.Grow melon plants for 5 weeks and use them to collect phloem sap using the stem incision method described in the steps below.
Phloem sap collection
4.Prepare a rack with labeled 1.5-ml sample tubes. Add to each tube 50 μl of filtered PBS (10 mM, pH = 7.4) mixed with PI at the dose suggested by the manufacturer.
5.Cut the stem of the melon plant, between the first and second expanded leaves from the apex, with a sterile razor blade. Blot the cut surface and first droplet with sterile filter paper and, immediately, collect phloem exudates (which can take up to 1-2 min per each plant) using a micropipette with sterile tips. Measure, approximately, exudate volumes (Fig. 5).
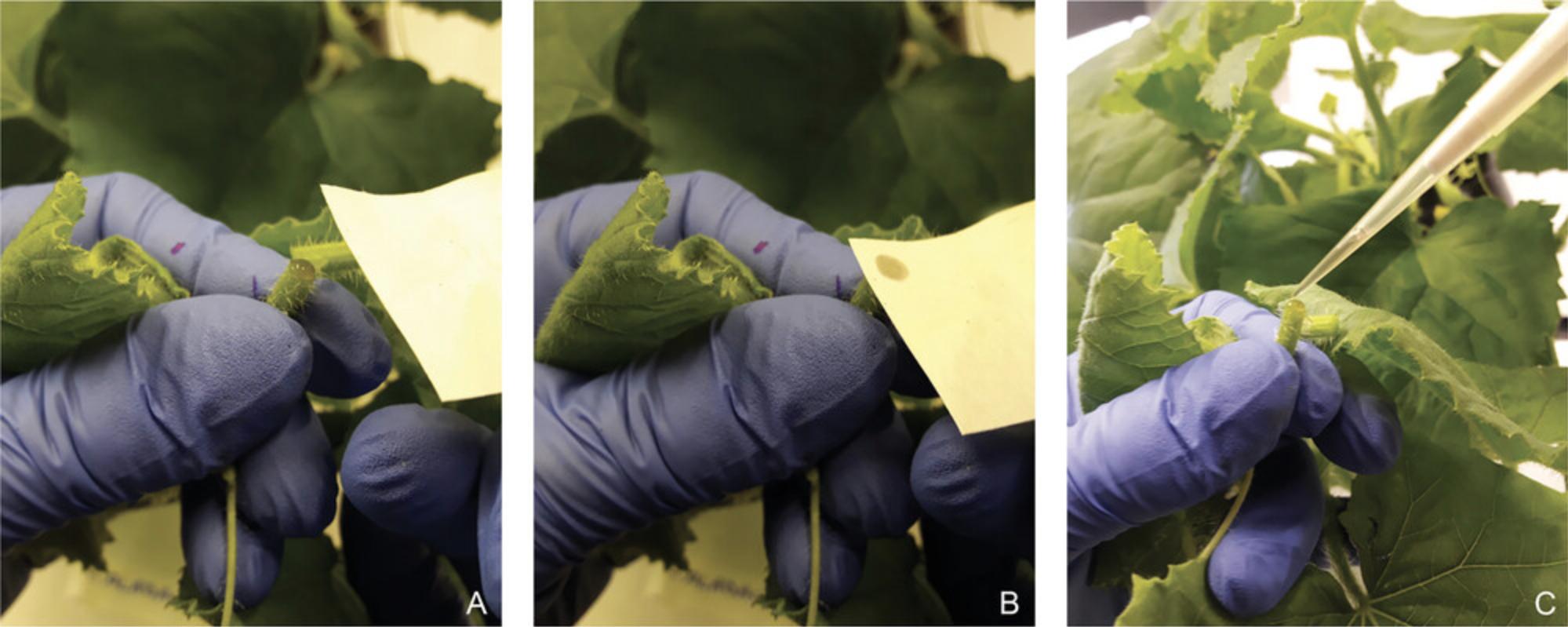
6.Deposit each exudate in a 1.5-ml sample tube with the initial 50 μl PBS+PI, and mix well with the micropipette.
7.Quickly add the required volume of fresh PBS+PI, according to sap volume collected, to make the final sap dilution 1/10 (v/v). Mix the sap sample with a vortex mixer and keep on ice until EV isolation by SEC (step 8). If needed, store sap samples at 4°C for 1-2 days, or at –20°C/–80°C for downstream analyses.
Prepare the column for size exclusion chromatography (SEC)
8.Place a syringe, or a SEC polypropylene column, with a porous filter at the bottom, on a stand. Use a beaker, placed just below the column, to collect the PBS used to clean and elute the column.
9.Wash the column with 1 ml of filtered 10 mM PBS.
10.Add 1 ml (packed volume) of Sepharose-CL2B to the column using a plastic Pasteur pipette or a micropipette (with a cut tip). Add Sepharose slowly and allow to settle down in the column, alternating with more PBS.
11.Wash the Sepharose three times, each time with 1 ml of filtered PBS (×3).
Phloem sap EV (PhlEV) isolation by SEC
12.Concentrate the sample using an Amicon 4 ml centrifugal filter. Deposit the diluted sap in the filter and centrifuge at 3000 × g , 4°C, until the concentrated sample reaches the proper volume for SEC purification. The final volume of the concentrated sap sample should be between 50 and 200 μl.
13.Label twelve 1.5-ml microcentrifuge tubes to collect 12 eluted fractions (∼0.1 ml) from the SEC. Place microtubes in a rack.
14.Before loading sap samples, wait until complete elution of the buffer used to rinse the resin. Then, remove the beaker and place the microtube rack under the column.
15.Load the SEC column with the concentrated phloem sap sample (50-200 μl). Immediately, once the sample penetrates the resin, start to collect the first fractions. Prior to adding more PBS, wait until the column stops dripping. Add more filtered PBS to the column for a complete elution of the sample.
16.Collect 12 consecutive fractions of ∼0.1 ml in the microtubes from each sample.
REAGENTS AND SOLUTIONS
Phosphate buffered saline (PBS), 10 mM (1×), pH = 7.4
g/L | mmol/L | |
NaCl | 8.0 | 137 |
KCl | 0.2 | 2.7 |
Na2HPO4 | 1.44 | 10 |
KH2PO4 | 0.24 | 1.8 |
Add 800 ml Milli-Q water; adjust pH to 7.4 with HCl; bring up to 1 L with water.
COMMENTARY
Background Information
Extracellular vesicles are membrane-enclosed nanostructures released from a cell into the surrounding environment. EVs are key tools, in all domains of life, for intercellular communication, protecting signal molecules for short- or long-distance transport. The first reports of mammalian and plant EVs appeared in the late 1960s, but for the next five decades, the research was mostly limited to animal cells. Scientists working with animal EVs isolated vesicles from distinct biological fluids or culture media and rapidly expanded the studies to analyze their composition and determine the different types of EVs, as well as their origins and release mechanisms. In contrast, EVs from plants were first isolated from apoplast and visualized by TEM in 2009 (Regente et al., 2009). There was a lapse of almost a decade until new studies reported conclusive results on the existence of plant EVs and their involvement in plant-pathogen interactions (Cai et al., 2018; Pérez-Bermúdez et al., 2017; Regente et al., 2017; Rutter & Innes, 2017).
Currently, as clearly stated by Pinedo et al. (2021) in their call for rigor in this field, additional work must be done to better define what are called plant EVs, to standardize procedures for their purification and characterization, to identify specific markers, and to know about the origin and functions of plant EVs. These authors proposed to use the term “plant EVs” only for those nanovesicles isolated from apoplastic fluids, and suggested the term “plant-derived nanovesicles” (PDNV) when the isolation procedures involve the rupture of cellular membranes by pressing or grinding organs or tissues (fruits, leaves, roots, etc.).
Regarding this, if EVs are extracellular vesicles naturally released from cells, it is necessary to remember that in plants there exist two pathways allowing short- or long-distance communication between plant organs or cells: apoplastic and symplastic. Transport in the apoplast occurs mainly via cell walls and the xylem (empty death cells), whereas symplastic movement occurs via plasmodesmata interconnecting the cellular protoplasts (parenchyma, phloem, etc.). Since xylem is formed by tracheary elements, when EVs are isolated from the apoplast it must be assumed that fusion events with the plasma membrane of neighboring living cells participate in their extracellular secretion. In contrast, phloem SEs are living cells that have lost the capability for transcription and translation; therefore, the presence of EVs in phloem sap suggests that these “extracellular nanovesicles” may have arrived symplastically from the companion cells.
Phloem is essential for the transport of nutrients, water, and signals in plants. To study the chemical composition of phloem sap, several methods have been used for its collection including EDTA-facilitated exudation, stem incision, and aphid stylectomy. Unfortunately, there is no ideal method that can be used for all plants, or to collect ultrapure phloem sap (Killiny, 2019). To prove the presence of extracellular vesicles in the phloem of different plant species, we have selected two different sap-collection methods, aphid stylectomy and stem incision.
The stylectomy method is laborious and allows the collection of very small amounts of the phloem sap (in our samples, 0.6-1.9 μl/plant), insufficient for exhaustive analyses (Killiny, 2019). Moreover, this method cannot be used with all insect and plant species, and is limited to young plants (Dinant et al., 2010). However, there are several indications that aphid stylectomy is probably the least invasive method to collect phloem sap, and the fluid obtained is probably the most authentic source of phloem sap (Lohaus, 2022). On the contrary, stem incision is an easy and fast method to collect higher (in our samples, 10-40 μl/plant) volumes of phloem sap, but it is only applicable to species that bleed freely from severed vascular bundles, for example cucurbits (Lohaus, 2022). In most plants, it is difficult to obtain enough sap since incised SEs are rapidly sealed by callose or P-protein deposition in the wounded area.
Sap samples obtained by stylectomy can be directly used for TEM or NTA analyses to show the presence of nanovesicles and visualize their morphology and sizes. In contrast, when the stem incision method is used, after recovery of sap samples various methods could be applied for EV separation, ultracentrifugation (UC) being the most widely used method in the EV field. UC is used alone or combined with other methods such as immunoaffinity, density gradients, etc. Despite this, a worldwide survey performed by the ISEV reported that the use of SEC has significantly increased since 2016 (Royo et al., 2020).
SEC uses the starting fluid as a mobile phase and a porous gel polymer as the stationary phase. EVs move through the SEC column faster, and elute earlier, than other smaller particles such as free proteins. SEC was first used for EV isolation by Böing et al. (2014), who designed a one-step method applied to human fluid samples, and currently it is a real alternative since it is easier and cheaper than other isolation procedures. Moreover, SEC is a less time-consuming method that efficiently removes co-purifying matrix components, including proteins and small molecular species, and produces a high yield of purified vesicles (Benedikter et al., 2017). On the other hand, a comparison between UC and SEC has demonstrated that EVs isolated by SEC showed a higher functionality performance, since this technique favored EVs to preserve their morphological characteristics and integrity (Mol et al., 2017). Basic Protocol 2 in this article describes a simple method based on SEC that allows the efficient and reproducible isolation of pure nanovesicles from phloem sap in sufficient quantities to be characterized.
Critical Parameters
The main goal of a successful experimental procedure focused on isolation of EVs is to obtain pure and intact EVs. These vesicles can be used to determine their size, morphology, and population density by combining NTA and TEM. Further characterization to elucidate EV contents includes protein quantification, proteomic, transcriptomic, lipidomic, and/or metabolic analyses, western blotting, etc.
General critical parameters
- The yield of EVs basically depends on the number of plants used to carry out the isolation. Therefore, according to the objectives and assays planned in the research, the starting number of plants to be grown must be scaled.
- Protocols must be executed in the shortest possible time to reduce alteration of EVs. It is advisable that the experiments be executed by more than one person, particularly when many plants and samples are processed.
- EV samples must be used as soon as possible after isolation. Nevertheless, if needed, samples can be stored at 4°C up to 1-2 days for morphology studies, or at –80°C for prolonged periods before EV functional analyses.
- Depending on the purpose of the research, it is not mandatory to include protease inhibitors during the process of EV isolation and purification. This protective product should be added to PBS at the initial phases of EV isolation when the objectives of the research include functional analyses of the isolated vesicles.
Basic Protocol 1
A critical parameter in this methodology is the moment of stylet amputation. A young adult aphid is wired for EPG recording (Manual-old Giga48.pdf; http://epgsystems.eu/downloads-install-files-manuals/category/7-hard-software) and placed on the primary branch of a rice panicle. EPG recording is monitored using the Stylet+ software (EPG Systems, Wageningen, The Netherlands) until the aphid shows phloem activities: salivation (E1) and passive phloem sap ingestion (E2) for more than 10 min (see Fig. 2). At this moment, the probing is terminated by stylet amputation using the tungsten needle of a Zapper RFM unit following the methodology proposed by Downing & Unwin (1977).
Stylet exudation is rather unpredictable in both success rate and duration. In agreement with Fisher and Frame (1984), the plant-insect system may vary substantially; although the species of plant used is the most relevant element for success, the selected insect is also important since, usually, larger aphids have advantages in terms of easier stylet cutting, leaving longer stylet stumps, and providing larger exudate volumes.
In this technique, the drops of sap exuding from the stylets are directly collected into a microcapillary tube held in a micromanipulator. To prevent evaporation and contamination of the exudates, it is advisable to fill the microcapillary tubes with water-saturated silicone oil (see Figs. 1 and 3B), although in this case, sap samples already diluted with PBS must be centrifuged (10,000 × g , 15 min at 4°C) and oil removed.
Basic Protocol 2
It is very important to use well-developed and healthy plants, with no external damage, to minimize the possible impact of diseases or mechanical wounding on the isolated EV components. Moreover, since the duration of exudation is often quite brief, it is important to use well-watered plants to ensure that all tissues are hydrated and phloem is under a high hydrostatic pressure.
Phloem exudate contains high concentrations of sugars and proteins and, to avoid sap coagulation, it is necessary to proceed to sap dilution immediately after sampling. For this reason, we measure sap volume and deposit sap samples on tubes already containing 50 μl PBS+PI. According to sap volume collected, we quickly add the required buffer and gently mix to make the final dilution. Although different dilution factors can be used, we recommend using a final 1/10 (v/v) dilution or higher.
The yield of EVs depends on the number of plants used to carry out the isolation, and the initial amount of plants should be calculated to meet the objectives planned. A minimum of 3-4 replicates is recommended, where a replication unit consists of the total sap collected from 15-20 plants (∼400-600 μl). This sap volume must be enough to perform characterization of PhlEVs by NTA and TEM, and even to perform one analysis of the EV protein content (i.e., ELISA, western blot, or LC/MSS proteomic assay).
NTA and TEM are used to confirm that fractions 4-7 are highly enriched in PhlEVs. In a first characterization of the SEC fractions, these four fractions can be analyzed separately. In further experiments, since EV concentration is low, we recommend pooling all four fractions in a single sample, or combining only fractions 5-6 when a more enriched sample is needed.
SEC columns can be used to perform at least two fractionation processes on similar samples. For this, the column should be washed with PBS (3 column volumes), plugged with a stopper or with parafilm, and refilled with PBS or Milli-Q water with 10% ethanol. The column is stored at 4°C and rinsed three times with PBS before use.
Troubleshooting
For troubleshooting tips, see Tables 1 and 2.
Problem | Possible cause | Solution |
---|---|---|
No EPG signal | Wrong wire fixations | Check wire connections with the aphid and with EPG device |
No exudation | No passive ingestion | Check passive ingestion for a longer period with another aphid |
Poor exudation | Low pressure in phloem | Use well-watered plants |
Sap coagulation at the stylet tip | Delay in collecting the exuding sap drop with the microcapillary tube | Position the microcapillary tube very close to the aphid to facilitate and speed up sap collection |
Problem | Possible cause | Solution |
---|---|---|
Poor exudation | Low pressure in phloem | Use well-watered plants |
Sap coagulation | High concentration of sap biomolecules | Make a higher sap dilution with PBS |
SEC column: Drop formation is too slow | Poor permeabilization of the filter | Help filter permeabilization with a micropipette |
No PhlEVs detected | Wrong fractions collected; wrong volume collection of each fraction | Perform a full characterization (NTA, TEM, others) of every fraction collected. If no EVs are detected, check that sample collection was done correctly. |
Understanding Results
Basic Protocol 1
Phloem sap collection from rice by aphid stylectomy and PhlEV isolation. This method is time-consuming and only applicable to selected phloem-feeding insects and plant species (Fisher & Frame, 1984). Although stylectomy does not produce much data, when this method is accurately reproduced, it allows the collection of pure phloem sap, and the user will obtain intact PhlEVs. The phloem sap is not altered by the aphids because it is only exposed to the inert internal surfaces of the stylets. Briefly, the feeding behavior of the aphids is monitored as described by Garzo et al. (2018). When the insect shows passive phloem sap ingestion from the SEs (E2 waveform >10 min), the stylet is amputated using the needle of a microcautery unit (see Fig. 4A). Stylets of the insects are used to collect phloem sap into the microcapillary tube (see Fig. 4B) using a micromanipulator. After dilution in PBS, sap samples can be directly used for TEM and NTA analyses to demonstrate the presence of nanovesicles, visualize the morphology, and determine size and number of phloem EVs (PhlEVs). NTA and TEM analyses were carried out according to the protocols described by Sánchez-López et al. (2022). Rice PhlEVs collected with Basic Protocol 1 showed an average size of 100-200 nm, with ∼3 × 108 particles/ml (Fig. 6). NTA devices in general produced peaks at substantially higher sizes and broader size distributions as compared to TEM imaging. This discrepancy might at least in part result from the physical principle of size determination of the NTA devices (Bachurski et al., 2021). Therefore, TEM is required to confirm cleanness. If the collection has not gone well, you will not detect typical EVs (size and morphology).
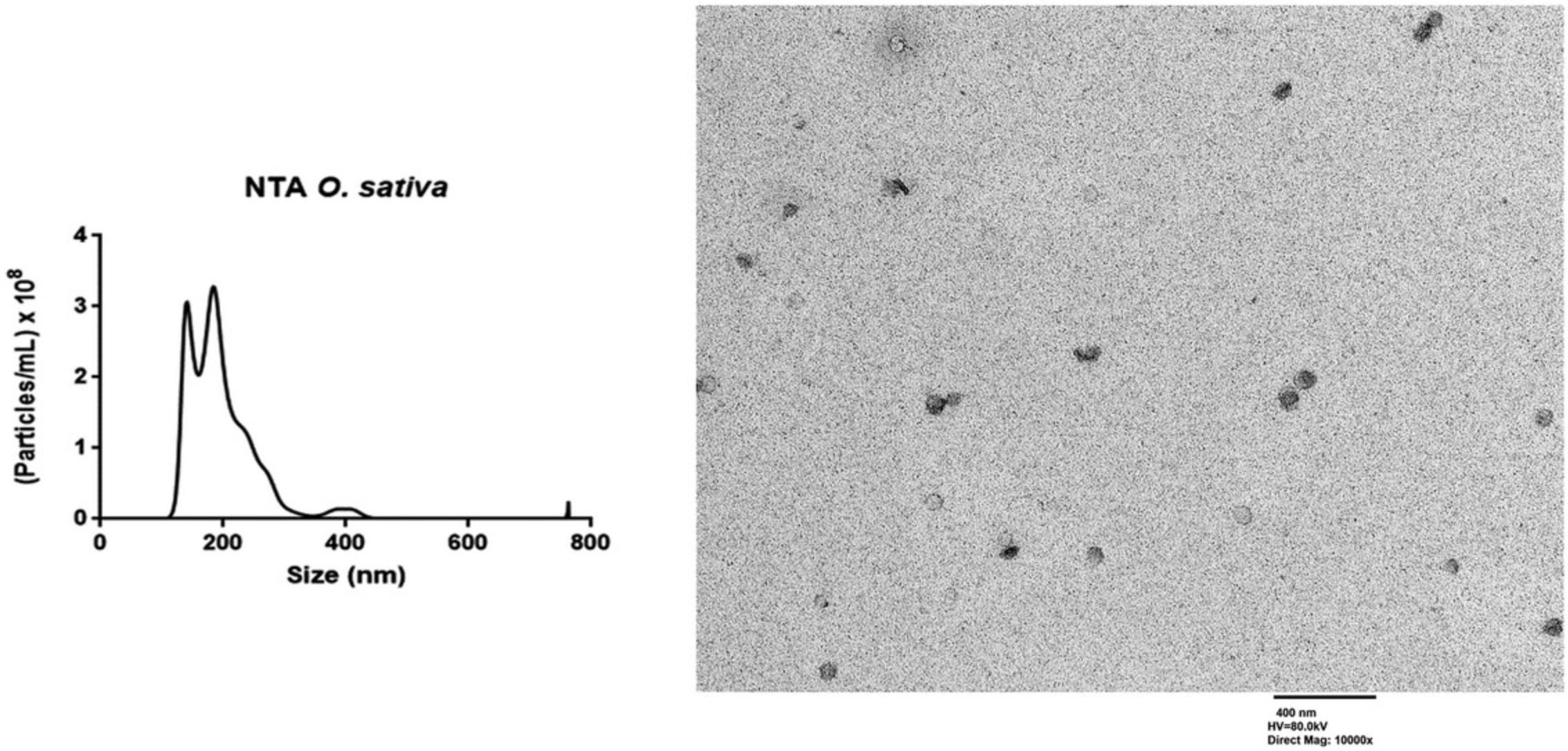
Basic Protocol 2
Phloem sap collection from melon by the stem incision method and PhlEV isolation and purification by SEC. Stem incision is an easy method to collect phloem sap from plant species that exhibit spontaneous phloem bleeding upon cutting the stem, in our case melon plants. Once the cut surface and the first sap drop are blotted with sterile filter paper, sap is collected with a micropipette (see Fig. 5).
Samples are diluted with PBS to avoid sap coagulation, and immediately used for PhlEV purification by SEC or stored at –80°C for posterior analysis. Chromatography columns of different volumes can be prepared according to the sample volume and, according to this, different numbers of fractions must be collected. In our case we load 50-200 μl of concentrated phloem sap into 1-ml columns and collect 12 fractions.
Fractions enriched in vesicles are pooled and PhlEVs characterized (see Sánchez-López et al., 2022) by at least the following basic techniques: (a) protein quantification; (b) TEM; (c) NTA. Melon PhlEVs collected with Basic Protocol 2 showed an average size of 70-150 nm with ∼4.7 × 108 particles/ml (Fig. 7).
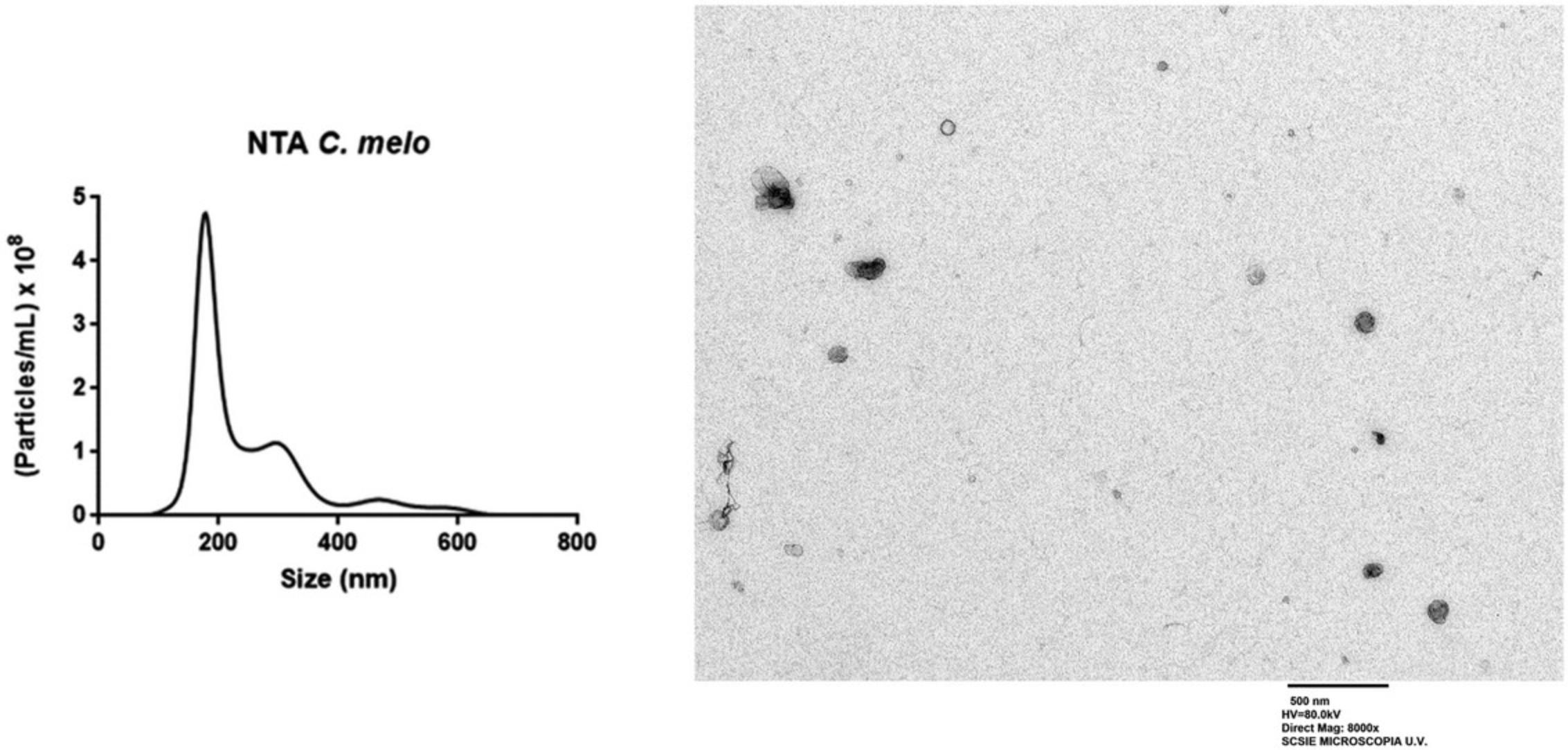
Time Considerations
Basic Protocol 1
Rice plants are grown up to the ripe phenological stage, which takes about 12-15 weeks. Barley plants are grown for 7 days and then used to rear and maintain the aphids’ colonies. The aphids are maintained for 9-10 days on barley plants until they reach the stage of young adults with a suitable size to be used for stylectomy.
Preparing the aphid and EPG connections and recording the aphid feeding behavior (salivation E1 + ingestion E2) takes around 60-120 min. Amputation of the stylet and sap collection can take up to 30-60 min. Therefore, the estimated mean time for a whole successful process is 100-180 min per sample (using one insect per plant).
Basic Protocol 2
Melon plants are grown for 5 weeks. To calculate the time for the whole collection process, which will depend on the starting number of plants, we estimate that stem cutting and sap manipulation (collection, volume measurement, and dilution) can take up to 7-10 min per each plant. We consider that a two-person team will be better to handle high amounts of pots, soil, and plants.
For SEC, we recommend carrying out column preparation and sap sample concentration simultaneously, and immediately proceeding to PhlEV purification. We estimate that 90-120 min is time enough to prepare three columns and purify three sap samples. We recommend a two-person team for the first attempt. Also, the starting volume of sap utilized to perform EV isolation will have an impact on the time, as the time needed to concentrate the sample will increase with the starting sap volume selected.
Acknowledgments
This work was supported by the following grants: PID2019-105713 GB-I00/AEI/10.13039/501100011033 Agencia Estatal de Investigación; and PROMETEO/2020/071-Generalitat Valenciana, and CSIC Intramural Grant 201440E016. C.M.S-L is the recipient of a predoctoral fellowship (PRE2020-092458/AEI /10.13039/501100011033). Plants and aphid cultures and phloem sap collection were carried out in the Institute of Agricultural Sciences of the Spanish National Research Council (ICA-CSIC, Madrid, Spain). TEM and NTA analyses were carried out at SCSIE-UV (Servicio Central de Soporte a la Investigación Experimental-Universitat de València). Some figures were created with BioRender.com.
Author Contributions
Elisa Garzo : Conceptualization, investigation, methodology, writing—original draft, writing—review and editing; Christian M. Sánchez-López : Investigation, methodology, writing—review and editing; Alberto Fereres : Funding acquisition, writing—review and editing; Carla Soler : Methodology, writing—review and editing; Antonio Marcilla : Conceptualization, funding acquisition, methodology, project administration, supervision; Pedro Pérez-Bermúdez : Conceptualization, methodology, supervision, writing—original draft, writing—review and editing.
Conflict of Interest
The authors declare no conflict of interest.
Open Research
Data Availability Statement
Data sharing not applicable—no new data generated.
Literature Cited
- Bachurski, D., Schuldner, M., Nguyen, P. H., Malz, A., Reiners, K. S., Grenzi, P. C., Babatz, F., Schauss, A. C., Hansen, H. P., Hallek, M., & Pogge von Strandmann, E. (2021). Extracellular vesicle measurements with nanoparticle tracking analysis—An accuracy and repeatability comparison between NanoSight NS300 and ZetaView. Journal of Extracellular Vesicles , 8(1), 1596016. https://doi.org/10.1080/20013078.2019.1596016
- Benedikter, B. J., Bouwman, F. G., Vajen, T., Heinzmann, A. C. A., Grauls, G., Mariman, E. C., Wouters, E. F. M., Savelkoul, P. H., Lopez-Iglesias, C., Koenen, R. R., Rohde, G. G. U., & Stassen, F. R. M. (2017). Ultrafiltration combined with size exclusion chromatography efficiently isolates extracellular vesicles from cell culture media for compositional and functional studies. Science Reports , 7, 15297. https://doi.org/10.1038/s41598-017-15717-7
- Böing, A. N., van der Pol, E., Grootemaat, A. E., Coumans, F. A., Sturk, A., & Nieuwland, R. (2014). Single-step isolation of extracellular vesicles by size-exclusion chromatography. Journal of Extracellular Vesicles , 3, 10. https://doi.org/10.3402/jev.v3.23430
- Bokka, R., Ramos, A. P., Fiume, I., Manno, M., Raccosta, S., Turiák, L., Sugár, S., Adamo, G., Csizmadia, T., & Pocsfalvi, G. (2020). Biomanufacturing of tomato-derived nanovesicles. Foods , 9, 1852. https://doi.org/10.3390/foods9121852
- Buhtz, A., Springer, F., Chappell, L., Baulcombe, D. C., & Kehr, J. (2008). Identification and characterization of small RNAs from the phloem of Brassica napus. The Plant Journal , 53, 739–749. https://doi.org/10.1111/j.1365-313X.2007.03368.x
- Cai, Q., Qiao, L., Wang, M., He, B., Lin, F. M., Palmquist, J., Huang, S.-D., & Jin, H. (2018). Plants send small RNAs in extracellular vesicles to fungal pathogen to silence virulence genes. Science , 360, 1126–1129. https://doi.org/10.1126/science.aar4142
- Chen, A., He, B., & Jin, H. (2022). Isolation of extracellular vesicles from Arabidopsis. Current Protocols , 2, e352. https://doi.org/10.1002/cpz1.352
- Dinant, S., Bonnemain, J. L., Girouisse, C., & Kehr, J. (2010). Phloem sap intricacy and interplay with aphid feeding. Comptes Rendus Biologies , 333, 504–515. https://doi.org/10.1016/j.crvi.2010.03.008
- Downing, N., & Unwin, D. M. (1977). A new method for cutting the mouth-parts of feeding aphids, and for collecting plant sap. Physiological Entomology , 2(4), 275–277. https://doi.org/10.1111/j.1365-3032.1977.tb00116.x
- Fisher, D. B., & Frame, J. M. (1984). A guide to the use of the exuding stylet technique in phloem physiology. Planta , 161, 385–393. https://doi.org/10.1007/bf00394567
- Garzo, E., Fernández-Pascual, M., Morcillo, C., Fereres, A., Gómez-Guillamón, M. L., & Tjallingii, W. F. (2018). Ultrastructure of compatible and incompatible interactions in phloem sieve elements during the stylet penetration by cotton aphids in melon. Insect Science , 26, 631–642. https://doi.org/10.1111/1744-7917.12447
- He, B., Hamby, R., & Jin, H. (2021). Plant extracellular vesicles: Trojan horses of cross-kingdom warfare. FASEB BioAdvances , 3(9), 657–664. https://doi.org/10.1096/fba.2021-00040
- Kehr, J., & Buhtz, A. (2008). Long distance transport and movement of RNA through the phloem. Journal of Experimental Botany , 59(1), 85–92. https://doi.org/10.1093/jxb/erm176
- Kehr, J., & Kragler, F. (2018). Long distance RNA movement. New Phytologist , 218, 29–40. https://doi.org/10.1111/nph.15025
- Killiny, N. (2019). Collection of the phloem sap, pros and cons. Plant Signaling and Behavior , 14(8), e1618181. https://doi.org/10.1111/j.1365-3032.1977.tb00116.x
- Kim, D. K., & Rhee, W. J. (2021). Antioxidative effects of carrot-derived nanovesicles in cardiomyoblast and neuroblastoma cells. Pharmaceutics , 13, 1203. https://doi.org/10.3390/pharmaceutics13081203
- Lohaus, G. (2022). Review primary and secondary metabolites in phloem sap collected with aphid stylectomy. Journal of Plant Physiology , 271, 153645. https://doi.org/10.1016/j.jplph.2022.153645
- Lough, T. J., & Lucas, W. J. (2006). Integrative plant biology: Role of phloem long-distance macromolecular trafficking. Annual Review of Plant Biology , 57, 203–232. https://doi.org/10.1146/annurev.arplant.56.032604.144145
- Lötvall, J., Hill, A. F., Hochberg, F., Buzás, E. I., Di Vizio, D., Gardiner, C., Gho, Y. S., Kurochkin, I. V., Mathivanan, S., Quesenberry, P., Sahoo, S., Tahara, H., Wauben, M. H., Witwer, K. W., & Thèry, C. (2014). Minimal experimental requirements for definition of extracellular vesicles and their functions: A position statement from the international society for extracellular vesicles. Journal of Extracellular Vesicles , 3, 26913. https://doi.org/10.3402/jev.v3.26913
- Margolis, L., & Sadovsky, Y. (2019). The biology of extracellular vesicles: The known unknowns. PLoS Biology , 17(7), e3000363. https://doi.org/10.1371/journal.pbio.3000363
- Mol, E. A., Goumans, M. J., Doevendans, P. A., Sluijter, J. P. G., & Vader, P. (2017). Higher functionality of extracellular vesicles isolated using size-exclusion chromatography compared to ultracentrifugation. Nanomedicine , 13(6), 2061–2065. https://doi.org/10.1016/j.nano.2017.03.011
- Pérez-Bermúdez, P., Blesa, J., Soriano, J. M., & Marcilla, A. (2017). Extracellular vesicles in food: Experimental evidence of their secretion in grape fruits. European Journal of Pharmaceutical Science , 98, 40–50. https://doi.org/10.1016/j.ejps.2016.09.022
- Pinedo, M., de la Canal, L., & de Marcos Lousa, C. (2021). A call for Rigor and standardization in plant extracellular vesicle research. Journal of Extracellular Vesicles , 10(6), e12048. https://doi.org/10.1002/jev2.12048
- Regente, M., Corti-Monzón, G., Maldonado, A. M., Pinedo, M., Jorrín, J., & de la Canal, L. (2009). Vesicular fractions of sunflower apoplastic fluids are associated with potential exosome marker proteins. FEBS Letters , 583, 3363–3366. https://doi.org/10.1016/j.febslet.2009.09.041
- Regente, M., Pinedo, M., San Clemente, H., Balliau, T., Jamet, E., & de la Canal, L. (2017). Plant extracellular vesicles are incorporated by a fungal pathogen and inhibit its growth. Journal of Experimental Botany , 68, 5485–5495. https://doi.org/10.1093/jxb/erx355
- Royo, F., Théry, C., Falcón-Pérez, J. M., Nieuwland, R., & Witwer, K. W. (2020). Methods for separation and characterization of extracellular vesicles: Results of a worldwide survey performed by the ISEV rigor and standardization subcommittee. Cells , 9(9), 1955. https://doi.org/10.3390/cells9091955
- Rutter, B. D., & Innes, R. W. (2017). Extracellular vesicles isolated from the leaf apoplast carry stress-response proteins. Plant Physiology , 173(1), 728–741. https://doi.org/10.1104/pp.16.01253
- Sánchez-López, C. M., Manzaneque-López, M. C., Pérez-Bermúdez, P., Soler, C., & Marcilla, A. (2022). Characterization and bioactivity of extracellular vesicles isolated from pomegranate. Food and Function , 13, 12870. https://doi.org/10.1039/d2fo01806c
- Sidhom, K., Obi, P. O., & Saleem, A. (2020). A review of exosomal isolation methods: Is size exclusion chromatography the best option? International Journal of Molecular Science , 21, 6466. https://doi.org/10.3390/ijms21186466
- Théry, C., Witwer, K. W., Aikawa, E., Alcaraz, M. J., Anderson, J. D., Andriantsitohaina, R., Antoniou, A., Arab, T., Archer, F., Atkin-Smith, G. K., Ayre, D. C., Bach, J. -. M., Bachurski, D., Baharvand, H., Balaj, L., Baldacchino, S., Bauer, N. N., Baxter, A. A., Bebawy, M., … Zuba-Surma, E. K. (2018). Minimal information for studies of extracellular vesicles 2018 (MISEV2018): A position statement of the international society for extracellular vesicles and update of the MISEV2014 guidelines. Journal of Extracellular Vesicles , 7(1), 1535750. https://doi.org/10.1080/20013078.2018.1535750
- Tjallingii, W. F. (1978). Electronic recording of penetration behaviour by aphids. Entomologia Experimentalis et Applicata , 24, 721–730. https://doi.org/10.1111/j.1570-7458.1985.tb03516.x
- Tjallingii, W. F. (1985). Electrical nature of recorded signals during stylet penetration by aphids. Entomologia Experimentalis et Applicata , 38, 177–186. https://doi.org/10.1111/j.1570-7458.1985.tb03516.x
- You, J. Y., Kang, S. J., & Rhee, W. J. (2021). Isolation of cabbage exosome-like nanovesicles and investigation of their biological activities in human cells. Bioactive Materials , 6, 4321. https://doi.org/10.1016/j.bioactmat.2021.04.023