Isolation, Culture, and Transfection of Melanocytes
Philip S. Goff, Philip S. Goff, Joanna T. Castle, Joanna T. Castle, Jaskaren S. Kohli, Jaskaren S. Kohli, Elena V. Sviderskaya, Elena V. Sviderskaya, Dorothy C. Bennett, Dorothy C. Bennett
Abstract
Located in the basal epidermis and hair follicles, melanocytes of the integument are responsible for its coloration through production of melanin pigments. Melanin is produced in a type of lysosome-related-organelle (LRO) called the melanosome. In humans, this skin pigmentation acts as an ultraviolet radiation filter. Abnormalities in the division of melanocytes are quite common, with potentially oncogenic growth usually followed by cell senescence producing benign naevi (moles), or occasionally, melanoma. Therefore, melanocytes are a useful model for studying both cellular senescence and melanoma, as well as many other aspects of biology such as pigmentation, organelle biogenesis and transport, and the diseases affecting these mechanisms. Melanocytes for use in basic research can be obtained from a range of sources, including surplus postoperative skin or from congenic murine skin. Here we describe methods to isolate and culture melanocytes from both human and murine skin (including the preparation of mitotically inactive keratinocytes for use as feeder cells). We also describe a high-throughput transfection protocol for human melanocytes and melanoma cells. © 2023 The Authors. Current Protocols published by Wiley Periodicals LLC.
Basic Protocol 1 : Primary explantation of human melanocytic cells
Basic Protocol 2 : Preparation of keratinocyte feeder cells for use in the primary culture of mouse melanocytes
Basic Protocol 3 : Primary culture of melanocytes from mouse skin
Basic Protocol 4 : Transfection of human melanocytes and melanoma cells
INTRODUCTION
Located in the basal epidermis and hair follicles, melanocytes of the integument are responsible for its coloration through the production of melanin pigments. Melanin is produced in a type of lysosome-related organelle (LRO) called the melanosome, which is transported along dendrites of the melanocyte and transferred into surrounding keratinocytes (reviewed by Wu & Hammer, 2014). In humans, the resulting skin pigmentation acts as an ultraviolet (UV) radiation filter, whereby melanin forms supranuclear caps within basal and suprabasal keratinocytes to reduce both UV irradiation of the nucleus and resulting DNA damage (Kobayashi et al., 1998; reviewed by Brenner & Hearing, 2008). Abnormalities in the division of melanocytes are quite common, with potentially oncogenic growth followed by cell senescence, producing benign naevi (moles) (Michaloglou et al., 2005). A number of signaling pathways regulating proliferation, cell senescence, and apoptosis are affected by common genetic changes in melanoma (reviewed by Bennett, 2016). Culture of melanocytes with and without such genetic changes is therefore useful for elucidating the pathways of the development of melanoma (Sviderskaya et al., 2003) and other cancers.
The differentiation of melanocytes and melanosomes and the development of skin pigmentation are tightly regulated by a number of processes, including signaling pathways and transcription factors. Mutations in key genes affecting these processes may result in a lack of melanocytes, causing hypopigmentation, or in defects or changes in melanin (reviewed by Lamoreux et al., 2010, and Yamaguchi & Hearing, 2014). With over 680 pigmentary mutations described (see Internet Resources), experimentation using melanocytes grown in culture is particularly useful for exploring the underlying genetics and mechanisms of normal variation of pigmentation as well as pigmentary disorders, such as the various forms of albinism (reviewed by Le et al., 2021). Additionally, eumelanin synthesis in the skin and eye are identical; therefore, skin melanocytes can serve as a useful model to understand pigmentation within the eye. We have established immortal murine melanocyte lines of over 40 pigmentary mutant genotypes, which have been widely used for some of the above studies (Sviderskaya et al., 2010; see Internet Resources).
Melanocytes are also useful for studying organelle dynamics as they are adherent cells with dendrites extending up to 100 µm and contain many melanosomes, which are easily visualized using bright-field as well as phase-contrast microscopy, owing to their color (reviewed by Hume & Seabra, 2011). Furthermore, the molecular mechanisms controlling the transport of melanosomes are comparable to those of other LROs; therefore, melanocytes can be used to elucidate the roles of other LROs in inflammation and the immune response (reviewed by Hume & Seabra, 2011). Melanocytes can also be used to provide insight into organelle transport and the further roles of molecular motors (Alzahofi et al., 2020).
For these valuable applications, the successful isolation and culture of melanocytes is paramount. Melanocytes may be derived from patients (for example from postoperative surplus skin) or laboratory mice with specific pigmentary mutations. The methods described here primarily focus on the isolation and culture of both human and murine melanocytes for use in downstream applications, such as transfection (also described). A key feature in the culture and maintenance of melanocytes is the use of a reduced pH compared to that used for most cell types; RPMI 1640 medium is used with 10% CO2, resulting in a pH of 6.9-7.0. This reduces the level of melanin synthesis, allowing for better growth (believed to be because toxic melanin intermediates can escape from melanosomes at high rates of synthesis).
Human melanocytes can be isolated from a small biopsy, as described in Basic Protocol 1. Overnight treatment in dispase separates the dermis and epidermis and is followed by incubation in trypsin-EDTA to give a single-cell suspension. These melanocytes are easily grown in culture using four mitogens: 12-O-tetradecanoylphorbol 13-acetate (TPA), cholera toxin (CT), endothelin 1 (EDN1), and stem cell factor (SCF). More information regarding these mitogens can be found in the Commentary. They do not require the inclusion of feeder cells for successful culture.
Basic Protocol 2 describes the preparation and treatment of mouse keratinocytes for use as feeder cells in Basic Protocol 3. Mouse keratinocytes must be rendered mitotically inactive by treatment with mitomycin C for use as feeder cells. Basic Protocol 3 describes isolation of murine melanocytes from sacrificed newborn mice, which are preferred to older or embryonic mice owing to ease of epidermal separation. The protocol differs slightly from that described for human melanocyte isolation. Overnight dispase treatment is replaced by digestion with concentrated trypsin. There is a reduced need for mitogens, with only TPA and CT being used, but keratinocyte feeder cells are used to allow sustained growth in culture. Melanocytes are readily amenable to genetic modification, and Basic Protocol 4 describes a process for the transfection of melanocytes.
NOTE : All solutions and equipment coming into contact with living cells must be sterile, and aseptic technique should be used accordingly.
NOTE : All cell culture incubations should be carried out in a 37°C, 10% CO2 humidified incubator.
CAUTION : Follow relevant safety guidelines and regulations as some of the reagents used in this article are potentially harmful.
Basic Protocol 1: PRIMARY EXPLANTATION OF HUMAN MELANOCYTIC CELLS
This protocol describes the primary culture of melanocytes from human skin as well as the maintenance of growing cultures. This technique can be used on skin from around the whole body as well as from donors of different ages. However, skin from much older people may result in a lower yield than that from younger donors. Dispase, a neutral protease isolated from Bacillus polymyxa , is used to cleave the basement membrane between the epidermis and dermis as it is more specific than trypsin and its use results in a greater yield of primary cells. For a more detailed explanation regarding the theoretical basis of this protocol, see Commentary.
NOTE : This protocol involves working with human tissue and therefore all local biosafety procedures should be followed. Local ethical approval may be required, and relevant guidelines for using human tissue should be adhered to.
Materials
-
Iodine solution (see recipe)
-
70% ethanol
-
Dulbecco's phosphate-buffered saline without calcium or magnesium (CMF-DPBS; e.g., Thermo Fisher, cat. no. 14190094; see Current Protocols, 2006)
-
10 µg/ml gentamicin (Sigma, cat. no. G1272) in CMF-DPBS
-
Skin sample (surplus postoperative skin or obtained by punch biopsy; >5 mm diameter, best results are obtained with >1 cm2 of skin)
-
10 mg/ml dispase II (Sigma, cat no. D4693) in Hank's balanced salt solution (Sigma, cat no. H6648)
-
500 µg/ml trypsin/EDTA solution (see recipe)
-
RPMI growth medium (see recipe)
-
Mitogen stocks:
- 40 µM TPA (see recipe)
- 40 nM CT (see recipe)
- 5 µM EDN1 (see recipe)
- 5 µg/ml SCF (see recipe)
-
125 µg/ml trypsin/EDTA solution (see recipe)
-
Dimethylsulfoxide (DMSO; Sigma, cat. no. D2650)
-
Phenylthiourea stock (PTU; see recipe)
-
Biological safety cabinet
-
6-well plates (e.g., Thermo Scientific, cat. no. 140675)
-
Automated pipettor with serological pipettes
-
Sterile fine forceps, blunt
-
37°C water bath
-
2.5-ml Combitips (Eppendorf, cat. no. 0030089650) and dispenser
-
Cell culture centrifuge, 4°C
-
Hemocytometer and cover glass
-
Cell culture microscope
-
P200 and P1000 pipettors with tips
-
Tissue culture flasks and dishes
-
10% CO2 incubator, 37°C
-
Ungassed incubator, 37°C
-
30-ml universal tubes (i.e., skirted tube with a conical bottom) (e.g., Elkay Laboratory Products, cat. no. 500-1000-302)
-
2-ml cryotubes
-
Liquid nitrogen storage
Preparation
1.In a 6-well plate, add to 3 wells, respectively, 5 ml iodine solution, 5 ml 70% ethanol, and 5 ml CMF-DPBS. Add 5 ml 10 µg/ml gentamicin to each of two wells.
2.Briefly submerge the skin sample in iodine solution, 70% ethanol, then the CMF-DPBS for a few seconds each, and then submerge in each of the two 10 µg/ml gentamicin solutions, each for 10 min.
3.Add 5 ml 10 mg/ml dispase II in Hank's solution to one well of a new 6-well plate, and submerge skin epidermis-side-down overnight at 4°C.
Separation of epidermis and dermis
4.Using suitable forceps (blunt, not to damage the tissue), gently peel away the epidermis from the dermis and place the epidermis in 5 ml 500 µg/ml trypsin/EDTA solution.
Culturing melanocytes
5.Incubate epidermis in 500 µg/ml trypsin/EDTA solution in a 37°C water bath for ∼10-15 min.
6.Disperse the epidermis into a cell suspension by pipetting up and down using a 2.5-ml Combitip attached to a dispenser.
7.Add 5 ml RPMI growth medium and centrifuge 15 min at 450 × g , 4°C.
8.Remove supernatant and resuspend the pellet in 5 ml fresh medium.
9.Count cells using a hemocytometer.
10.Plate cells at 1.5 × 105 cells/ml (plate 5 ml total volume for a 25-cm2 flask or in general ∼1 ml/5 cm2, depending on the number of cells available), adding 5 µl/ml 40 µM TPA, 5 µl/ml 40 nM CT, 2 µl/ml 5 µM EDN1, and 2 µl/ml 5 µg/ml SCF.
11.Place the culture in a humidified, 37°C, 10% CO2 incubator.
Propagating melanocyte cultures
12.Change RPMI growth medium every 3-4 days, adding the same concentrations of mitogens as in step 10.
13.When cells are confluent, for subculture, remove medium and wash cells in 0.5 vol CMF-DPBS, where 1 vol is the normal culture volume for the flask or plate. Tilt the container gently around to rinse away serum.
14.Add 0.5 vol 125 µg/ml trypsin/EDTA solution. Tilt again briefly then remove most of it to leave 0.1 vol. Place in a 37°C ungassed incubator.
15.When cells are detached, resuspend into fresh medium to give the normal culture volume, with two rinses to remove all cells.
16.Count cells in triplicate using a hemocytometer. Keep the cell suspension on ice to prevent loss, as melanocytes can quickly start to reattach at room temperature.
17.Dilute, mix, and replate cells at the required density in fresh medium with mitogens at the same concentration as in step 10.
Freezing and thawing melanocytes
18.Freeze cells using cultures approaching confluence as follows:
-
Trypsinize as in step 14, and count cells using a hemocytometer.
-
Centrifuge cells 7 min at 200 ×g, 4°C.
-
Label cryotubes before proceeding to minimize the time cells are in DMSO.
-
Resuspend cells in previously mixed 7.5% (v/v) DMSO in RPMI growth medium at a total density of 0.5–1 × 106cells/ml.
-
Dispense 1-ml aliquots into 2-ml cryotubes and freeze at –80°C in an insulated container before moving into liquid nitrogen for long-term storage.
19.Thaw cells quickly as follows:
-
Place the cryotube in a 37°C water bath.
-
Resuspend by pipetting and transfer cells to a 30-ml universal tube.
-
Add 1 ml RPMI growth medium dropwise with swirling over ∼30 s, then add another 1 ml dropwise over 15 s, and finally 18 ml dropwise over 30 s.
-
Centrifuge cells 5 min at 200 ×g, 4°C.
-
Resuspend cells in fresh medium at the desired density (usually 3 × 104cells/ml in 5 ml total volume per 25 cm2culture flask, or in general ∼1 ml/5 cm2) with the same concentration of mitogens as in step 10.
Basic Protocol 2: PREPARATION OF KERATINOCYTE FEEDER CELLS FOR USE IN THE PRIMARY CULTURE OF MOUSE MELANOCYTES
This protocol describes the subculture and preparation of mouse keratinocyte feeder cells. The first technique describes the subculture of XB2 mouse keratinocytes, which can be difficult to subculture as they tend to attach very firmly. The XB2 keratinocytes can be grown and subcultured with a view to treating them with mitomycin C to render them mitotically inactive for use as feeder cells. For a more detailed explanation regarding the theoretical basis of this protocol, see Commentary.
Additional Materials (also see Basic Protocol 1)
- XB2 mouse keratinocytes (available from the Functional Genomics Cell Bank at St. George's, University of London; see Internet Resources) growing in culture
- 500 µM EDTA solution in CMF-DPBS (Sigma, cat. no. E8008)
- 250 µg/ml trypsin/EDTA solution (see recipe)
- Dulbecco's Modified Eagle Medium (DMEM) growth medium (see recipe)
- Mitomycin C stock (see recipe)
Maintenance and subculture of XB2 mouse keratinocytes
1.Thaw XB2 keratinocytes as described in step 19 of Basic Protocol 1 except use DMEM rather than RPMI growth medium.
2.Change DMEM growth medium every 3-4 days.
3.Once ready to subculture, remove medium and wash the cells twice with 500 µM EDTA solution in CMF-DPBS. Use 0.5 vol per wash, where 1 vol is the normal culture volume for the flask or plate, e.g., use 2.5 ml in a 5-ml (25-cm2) flask. Tilt the container gently around to rinse away serum, which inhibits trypsin.
4.Wash the cells once with 0.5 vol of 250 µg/ml trypsin/EDTA solution and tilt around again. Remove most of this to leave 0.1 vol of solution.
5.Incubate at 37°C (in an ungassed incubator rather than with CO2) until the cells have completely detached.
6.Resuspend the cells by pipetting in two rinses of fresh DMEM growth medium into a suitable container (e.g., 30-ml universal tube), to make 1 vol of cell suspension, and count using a hemocytometer.
7.Dilute the required amount of suspension with fresh DMEM growth medium and replate the cells at 3 × 104/ml (plate 5 ml total volume per 25-cm2 flask or in general ∼1 ml/5 cm2 depending on the number of cells available and your requirements, e.g., if preparing feeder cells, plate 35 ml total volume per 175-cm2 flask).
Preparation of feeder cells
In order to prepare feeder cells, the XB2 cells should be ready to subculture, but still growing. They should not be confluent, as this may lead to ineffective treatment with mitomycin C and lead to some of the cells still retaining the capacity to divide. Plate the required volume of cultures (e.g., if you require a large stock of feeder cells, plate cells into 175-cm2 flasks as previously described). It is recommended to make large amounts of vials to allow batch testing. There is no specific limit on passage number for the XB2, but the growing cells should form patches of continuous epithelial pavement. If they start to show a disrupted layer with spindly-looking cells, they should be discarded in favor of a lower passage level.
8.Remove medium and add 0.5 vol fresh DMEM growth medium containing 8 µg/ml mitomycin C. Swirl to mix this with residual old medium.
9.Incubate for 3-3.5 hr in a 37°C, 10% CO2 incubator.
10.Remove medium containing mitomycin C, wash once in 0.5 vol DMEM growth medium and subsequently incubate in DMEM growth medium (no mitomycin C) for 10 min in a 37°C, 10% CO2 incubator.
11.Subculture as previously described (steps 3-7 of this protocol) and either replate 2 ml per 35-mm dish at 3 × 104/ml for use immediately or freeze 1 ml aliquots of 1 × 106 cells/ml and store in liquid nitrogen for future use. For freezing cells, resuspend as detailed above in steps 3-7 of this protocol, then follow step 18 of Basic Protocol 1, except use DMEM rather than RPMI growth medium and use 250 µg/ml trypsin/EDTA instead of 125 µg/ml.
Basic Protocol 3: PRIMARY CULTURE OF MELANOCYTES FROM MOUSE SKIN
This protocol describes the primary culture of melanocytes from mouse skin and their subsequent subculture (Sviderskaya et al., 1997). There is a requirement for feeder cells, and so Basic Protocol 2 should be followed prior to this Protocol. To generate immortal melanocyte lines efficiently, it is recommended to cross the required genotype of mouse with Cdkn2a (Ink4a-Arf) null mice, as Cdkn2a null melanocytes do not senesce at all (Sviderskaya et al., 2002).
This technique can be carried out on either embryonic or newborn skin (of mice up to 3 days old). Older skin is very hard to split because of the hair and contains few melanocytes anyway. It is also hard to split embryonic skin (which is fragile), and this increases the risk of contamination with fibroblasts. Thus, it is preferable to use newborn skin. However, it may be necessary to work with embryonic skin, for example if a mutation you wish to study is lethal to postnatal mice.
In contrast to human melanocytes (which require 4 mitogens; TPA, CT, EDN1, and SCF), successful culture of murine melanocytes only requires TPA and CT. For a more detailed explanation regarding the theoretical basis of this protocol, see Commentary.
NOTE : This protocol involves working with animals and therefore local ethical approval may be required and relevant guidelines for working with animals should be adhered to.
Additional Materials (also see Basic Protocol 1)
-
Keratinocyte feeder cells (see Basic Protocol 2)
-
Mouse pups (up to 3 days old) or pregnant mouse
-
5 mg/ml trypsin (see recipe)
-
250 µg/ml trypsin/EDTA solution (see recipe)
-
1 mg/ml soybean trypsin inhibitor (see recipe)
-
35-mm culture dishes (e.g., Thermo Scientific, cat. no. 153066)
-
Reagents and equipment for dissection of pregnant mouse to obtain embryos or to sacrifice the mouse pups (Limaye et al., 2009)
-
10-cm culture dishes (e.g., Thermo Scientific, cat. no. 150350)
-
Two pairs of fine forceps (for each skin to be processed)
-
Laminar flow hood
-
Dissecting microscope in culture hood (need sterile atmosphere)
-
60-mm (5-ml) culture dishes (e.g., Thermo Scientific, cat. no. 150288)
-
Two curved scalpels (for each skin to be processed)
Skin preparation
1.Plate out keratinocyte feeder cells on a 35-mm dish at 5 × 104/ml in RPMI growth medium (2 ml total volume per 35-mm dish), preferably 1 day before carrying out the remainder of this protocol.
2.Sacrifice the mouse pups (or the pregnant mouse in the case of embryos) by a legal method (depending on the country). If using embryos, dissect the pregnant mouse to obtain the embryos (Limaye et al., 2009).
3.Wash off the ethanol (or wash the embryos) in CMF-DPBS.
4.Dissect off the trunk skin in one piece and keep wet in CMF-DPBS.
5.Transfer to a 10-cm culture dish with enough CMF-DPBS to cover the skin and remove any remaining pieces of muscle using two pairs of fine forceps and a dissecting microscope in a sterile environment.
Splitting the epidermis from the dermis
6.Transfer the skin(s) to individual 30-ml universal tubes containing 5 ml 5 mg/ml trypsin.
7.Incubate at 37°C for ∼1 hr for newborn skin.
8.After the allotted time, transfer the skin to a 10-cm dish containing enough CMF-DPBS to cover the skin.
9.Using a dissecting microscope and two pairs of fine forceps split off the epidermis from the dermis. If it does not come off easily, do not force but place the skin back into 5 mg/ml trypsin and continue incubation. Remove any pieces of dermis still attached to the epidermis.
Culturing melanocytes
10.Wash the epidermis in fresh CMF-DPBS and transfer the 10-cm dish to a laminar flow hood.
11.Add 100 µl 250 µg/ml trypsin/EDTA solution to the middle of a 60-mm dish.
12.Prepare 5 ml RPMI growth medium with 5 µg/ml soybean trypsin inhibitor (add from 1 mg/ml stock).
13.Transfer the epidermis from the CMF-DPBS onto the drop of trypsin/EDTA solution.
14.Using two curved scalpels, chop the epidermis finely.
15.Using a serological pipette and the RPMI growth medium with soybean trypsin inhibitor prepared in step 12, suspend and rinse the chopped pieces of epidermis into a 30-ml universal tube.
16.Mix vigorously three to five times using a 2.5-ml Combitip.
17.Add 5 µl/ml 40 µM TPA and 5 µl/ml 40 nM CT and mix thoroughly.
18.Remove the medium from the mitomycin C-treated XB2 keratinocytes (from step 1), plate the full 5 ml epidermal suspension onto these cells, and place in 10% CO2 incubator at 37°C.
19.Reduce the amount of medium to 2 ml after 1-2 days (or when changing medium). Initially change medium twice a week (using RPMI growth medium with 200 nM TPA and 200 pM CT).
Subculture of mouse melanocytes
The cells should be subcultured at least every 2 weeks (if sparse), and before reaching confluence, onto fresh mitomycin C-treated XB2 keratinocytes until immortal cells take over the culture. At this stage some lines may not require constant culture with CT; this can be determined empirically by the user, i.e., keep at least two separate cultures and remove the CT from one to compare proliferation and differentiation. The XB2 cells are thawed and plated as in Basic Protocol 3, step 1, on the required number of dishes or flasks.
20.Remove medium from the melanocyte culture and wash cells in 0.5 vol CMF-DPBS, where 1 vol is the normal culture volume for the flask or plate. Tilt the container gently around to rinse away serum.
21.Add 0.5 vol 250 µg/ml trypsin/EDTA solution. Tilt again briefly and then remove most of it to leave 0.1 vol. Place in a 37°C ungassed incubator.
22.When cells are detached, resuspend into fresh medium to give the normal culture volume, with two rinses to remove all cells.
23.Count cells in triplicate using a hemocytometer. Keep the cell suspension on ice to prevent loss, as melanocytes can quickly start to reattach at room temperature.
24.Dilute, mix, and replate cells either at 3-5 × 104 cells/ml (5 ml total volume for 25-cm2 flasks or in general ∼1 ml/5 cm2, depending on the amount of cells available), or (for sparse cultures) at a ratio of 1:1, in fresh medium with mitogens (200 nM TPA and 200 pM CT), onto the mitomycin C-treated XB2 cells.
25.For freezing and thawing mouse melanocytes, use the method in Basic Protocol 1, steps 18-19, except use 250 µg/ml trypsin/EDTA solution instead of 125 µg/ml. When initially thawing established mouse cell lines, plate 5 ml total volume in a 25-cm2 flask at 3-10 × 104 cells/ml (depending on viability), adding 200 nM TPA but no CT.
Basic Protocol 4: TRANSFECTION OF HUMAN MELANOCYTES AND MELANOMA CELLS
This protocol describes how to transfect human melanocytes and melanoma cells in 96-well plates. It uses “reverse transfection,” where the nucleic acid is added to wells before the cells, which gives efficient transfection in these cell types. This protocol is based on a high-throughput system designed to screen the activity of tens to hundreds of different siRNAs. However, the volumes used could be scaled up or down depending on the nature of the experiments. Cell and nuclear staining are used to determine the effects of the transfected siRNA on the cells. A sophisticated plate reader, such as the Thermo Scientific Cellomics ArrayScan VTI HCS Reader, is recommended for capturing the outputs in this high-throughput scenario.
Additional Materials (also see Basic Protocol 1)
-
siRNAs:
- Negative control siRNA—Silencer Select negative control (siRNA #2; Invitrogen, cat. no. 4390846)
- Positive control siRNA—CDC2 siRNA gene solution (Qiagen, cat. no. 1027416 gene ID 983)
- Transfection positive control siRNA—AllStars Hs Cell Death Control siRNA (Qiagen, cat. no. 1027298)
- Test siRNAs—Silencer Select validated or predesigned (inventoried) siRNA (Ambion)
-
RNase-free distilled H2O
-
RPMI serum-free medium (see recipe)
-
Lipofectamine 2000 (Invitrogen, cat. no. 11668027)
-
Human melanocyte or melanoma cell suspension (see Basic Protocol 1)
-
RNase/DNase-free tubes (e.g., Invitrogen, cat. no. AM12400)
-
RNase-free filter tips for P20, P200, and P1000 pipettors
-
96-well black optical-bottom tissue culture plates (e.g., Greiner Bio-One, cat. no. 655090)
-
Multichannel pipettors and reservoirs
Preparation and storage of siRNA
1.Resuspend lyophilized siRNAs to 5 µM (5 pmol/µl) in RNase-free water.
2.Prior to storage, aliquot all siRNAs to avoid freeze-thaw cycles and store at −20°C (short-term) and −70°C (long-term).
3.Create an siRNA master plate.
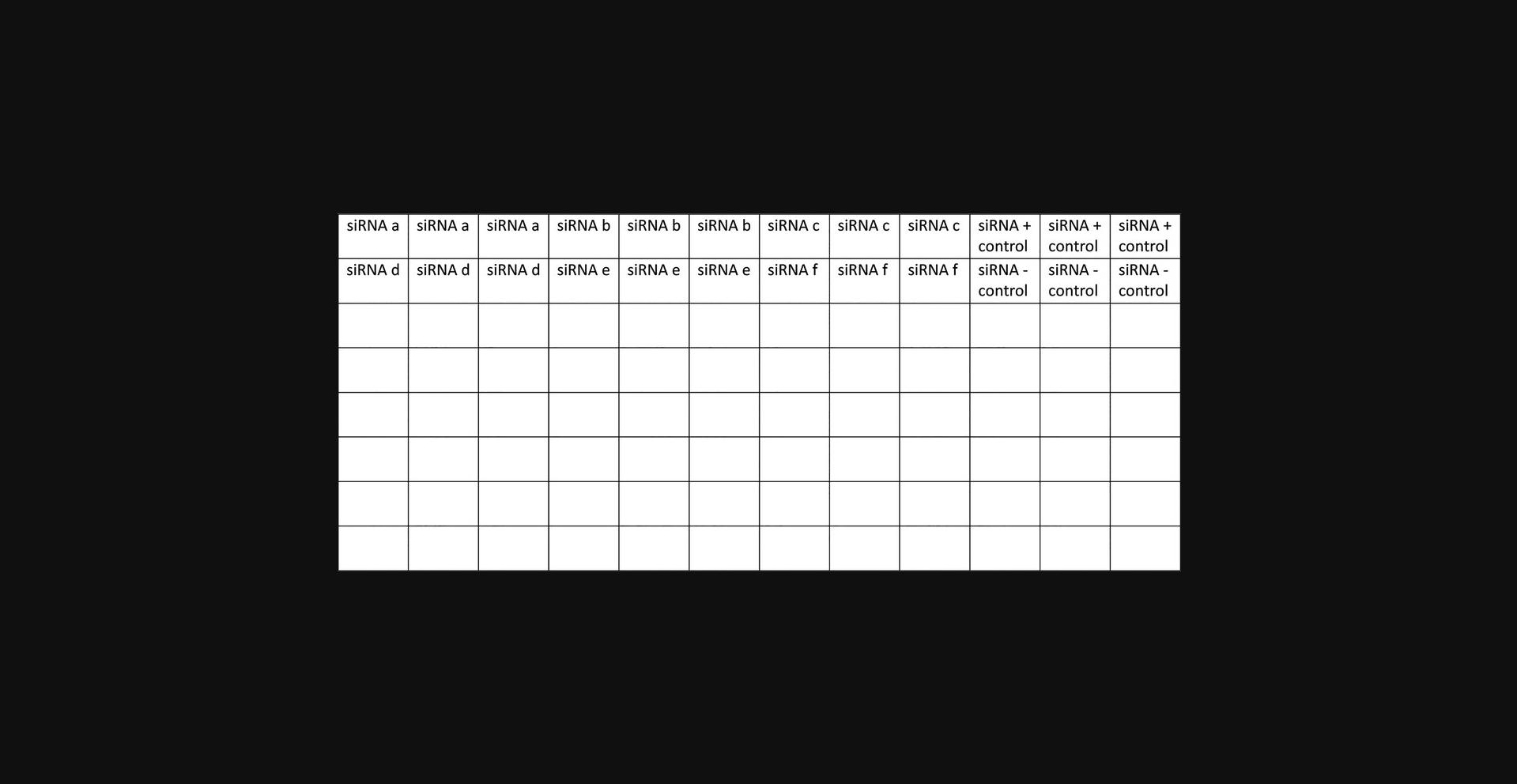
Medium preparation
4.Prepare complete medium (RPMI growth medium with appropriate mitogens).
Transfection
5.Grow cells to ∼80% confluency (i.e., 80% of the culture dish covered and not at saturation density). Trypsinize as for subculture (see Basic Protocol 1) and leave at room temperature.
6.Mix Lipofectamine 2000 with serum-free medium. Prepare a 100 × master mix in an RNase-DNase-free centrifuge tube containing 30 µl Lipofectamine 2000 in 470 µl serum-free medium for each 96-well plate (0.3 µl Lipofectamine 2000 in 4.7 µl serum-free medium per well). Incubate at room temperature for 5 min.
7.Add 8.5 µl serum-free medium to each well of a 96-well black optical bottom plate using a multichannel pipettor.
8.Add 1.5 µl of the required siRNA to each well. Mix using pipettor.
9.Add 5 µl Lipofectamine 2000/serum-free medium to all wells. Mix using pipettor. Incubate for 15 min at room temperature from the last well.
10.During the 15 min incubation, dilute the cell suspension in complete medium (2.22 × 104/ml in 15 ml complete medium (see step 4) per plate to give 3000 cells/well in 135 µl).
11.After 15 min, add 135 µl cell suspension/well.
12.Incubate plates at 37°C, 10% CO2 for the required period (typically around 3-7 days, depending on the research question and assay). For immunostaining, fix and permeabilize cells as required, depending on the assay to be used.
REAGENTS AND SOLUTIONS
Use deionized, distilled water in all recipes and protocol steps.
Bovine serum albumin (BSA), 0.1 mg/ml
Dissolve BSA (e.g., Sigma cat. no. A7906) in CMF-DPBS (e.g., Gibco, cat. no. 14190-094; see Current Protocols, 2006) at 0.1 mg/ml. Filter-sterilize using a 0.22-µm filter. Store for ≤1 month at 4°C.
Cholera toxin (CT)
Dissolve CT (Sigma, cat. no. C8052) in 0.1 mg/ml BSA (see recipe) to make a 2 µM stock solution. Filter-sterilize using a 0.22-µm filter. Aliquot and store indefinitely at –80°C. Thaw one aliquot at a time and dilute with 0.1 mg/ml to make a 40 nM working solution. Store aliquots indefinitely at –80°C or for ≤2 weeks at 4°C.
DMEM growth medium (for keratinocytes)
- 500 ml DMEM (high glucose, 4500 mg/L) without L-glutamine (e.g., Sigma, cat. no. D6546)
- 5.6 ml penicillin/streptomycin stock solution (10,000 U/ml penicillin and 10,000 µg/ml streptomycin) (Gibco, cat. no. 15140-122)
- 5.6 ml 200 mM L-glutamine (Gibco, cat. no. 25030-024)
- 56 ml fetal bovine serum (FBS, Gibco, cat. no. 10270-106)
- Store ≤1 month at 4°C
Endothelin 1 (EDN1)
Dissolve EDN1 (Bachem, cat. no 4040254) in 0.1 mg/ml BSA (see recipe) to make a 50 µM stock solution. Filter-sterilize using a 0.22-µm filter. Store indefinitely at –80°C. Thaw one aliquot at a time and dilute with 0.1 mg/ml BSA to make a 5 µM working solution. Store aliquots indefinitely at –80°C or ≤2 weeks at 4°C.
Iodine solution, 2.5 mg/ml
Dissolve 25 mg iodine crystals in 10 ml sterile distilled H2O. Store indefinitely at 4°C.
Mitomycin C, 500 µg/ml stock
Dissolve 2 mg mitomycin C (Sigma, cat. no. M4287) in 4 ml distilled H2O. Filter-sterilize using a 0.22-µm filter. Store wrapped in foil ≤1 month at 4°C.
Phenol Red, 3 mg/ml
Dissolve 300 mg phenol red (e.g., Sigma, cat. no. P5530) in 100 ml distilled H2O. Filter-sterilize using a 0.22-µm filter. Store indefinitely at room temperature.
Phenylthiourea (PTU), 100 mM
Dissolve PTU (also called phenylthiocarbamide, Sigma, cat. no. P7629) in 100% ethanol to make a 100 mM stock solution. Store indefinitely at −20°C or <1 month at 4°C.
RPMI growth medium
- 500 ml RPMI 1640 with phenol red and L-glutamine (Sigma, cat. no. R8758)
- 1.45 ml 3 mg/ml phenol red solution (see recipe)
- 5.6 ml penicillin/streptomycin stock solution (10,000 U/ml penicillin and 10,000 µg/ml streptomycin) (Gibco, cat. no. 15140-122)
- Gas with 10% CO2 for ∼1 min
- Add 56 ml FBS (Gibco, cat. no. 10270-106)
- Store ≤1 month at 4°C
Extra phenol red is added to the medium to help with monitoring of pH. Serum used for culturing melanocytes should not be heat-inactivated as this destroys its stimulatory activity for melanocytes.
RPMI serum-free medium
- 500 ml RPMI 1640 with phenol red and L-glutamine (Sigma, cat. no. R8758)
- 1.45 ml 3 mg/ml phenol red solution (see recipe)
- 5.6 ml penicillin/streptomycin stock solution (10,000 U/ml penicillin and 10,000 µg/ml streptomycin) (Gibco, cat. no. 15140-122)
- Gas with 10% CO2 for ∼1 min
- Store <1 month at 4°C
Soybean trypsin inhibitor, 5 µg/ml
Dissolve soybean trypsin inhibitor (Sigma, cat. no. T9128) in CMF-DPBS (e.g., Gibco, cat. no. 14190-094; see Current Protocols, 2006) to a concentration of 5 µg/ml. Filter-sterilize using a 0.22-µm filter. Prepare small aliquots and store indefinitely at –20°C.
Stem cell factor (SCF)
Dissolve human SCF (Peprotech, cat. no. 300-07) in 0.1 mg/ml BSA (see recipe) to make a 20 µg/ml stock solution. Store indefinitely at –80°C. Dilute to 5 µg/ml with 0.1 mg/ml BSA (see recipe). Store indefinitely at –80°C or for ≤2 weeks at 4°C after first use.
12-O-Tetradecanoylphorbol 13-acetate (TPA)
Dissolve TPA (Sigma, cat. no. P8139) in 100% ethanol to make a 2 mM stock solution. Store aliquots indefinitely at –80°C. Dilute with 0.1 mg/ml BSA (see recipe) to make a 40 µM working solution. Store in aliquots indefinitely at –80°C or for ≤2 weeks at 4°C after first use.
Trypsin/EDTA solution, 125 µg/ml
- 10 ml 1× trypsin-EDTA solution (Gibco, cat. no. 25300-054; contains 500 µg/ml trypsin and 500 µM EDTA in CMF-DPBS)
- 30 ml 500 µM EDTA solution in CMF-DPBS (Sigma, cat. no. E8008).
- Store ≤2 weeks at 4°C
Trypsin/EDTA solution, 250 µg/ml
- 10 ml 1× trypsin-EDTA solution (Gibco, cat. no. 25300-054; contains 500 µg/ml trypsin and 500 µM EDTA in CMF-DPBS)
- 10 ml 500 µM EDTA solution in CMF-DPBS (Sigma, cat. no. E8008)
- Store ≤2 weeks at 4°C
Trypsin/EDTA solution, 500 µg/ml
- 0.5 ml 10× trypsin-EDTA (Sigma, cat. no. T4174)
- 4.5 ml 500 µM EDTA solution in CMF-DPBS (Sigma, cat. no. E8008)
- Store indefinitely at –20°C
Trypsin, 5 mg/ml
Dissolve trypsin powder (Sigma, cat. no. T4799) in CMF-DPBS (Gibco, cat. no. 14190-094) to 5 mg/ml. Filter-sterilize using a 0.22-µm filter. Prepare small aliquots and store indefinitely at −40°C or −80°C.
COMMENTARY
Background Information
Basic Protocol 1
Human melanocytes are a useful model for studying melanoma, cellular senescence, pigmentation, organelle biogenesis and transport, and related disease mechanisms. An advantage of studying melanocytes is that they are easy to obtain (for example, from elective surgery patients for adult cell lines, and foreskin for neonatal cell lines), and are simple to isolate and maintain in culture.
Four mitogens are used for human melanocyte growth: TPA, also known as phorbol 12-myristate 13-acetate (PMA); CT; SCF; and EDN1. The first reproducible melanocyte culture method was developed in 1982, upon the discovery that use of CT and TPA as mitogens could selectively maintain melanocyte growth in culture (Eisinger & Marko, 1982). CT acts by mimicking the effects of melanocyte stimulating hormone (MSH) by specifically activating adenylate cyclase, thereby causing production of cyclic AMP. This stimulates proliferation, or at higher concentrations cell differentiation and pigment synthesis (O'Keefe & Cuatrecasas, 1974). TPA is a phorbol ester that activates PKC signaling, leading to the phosphorylation of a number of transcription factors, resulting in proliferation and differentiation of melanocytes in culture (reviewed by Yamasaki et al., 2009).
Keratinocytes were traditionally used as feeder cells for melanocyte culture, as a close representation of the epidermal tissue. The release of tumor necrosis factor (TNF)-α and interleukin (IL)-1α by keratinocytes has been shown to induce the production of EDN1 and SCF (Hachiya et al., 2004). It has been established that EDN1 and SCF act synergistically to stimulate the proliferation of melanoblasts and melanocytes in the presence of a cyclic AMP inducer such as CT. In a feeder-free environment, the absence of these mitogens leads to reduced melanocyte proliferation and increased apoptosis (Hirobe et al., 2010; Sviderskaya et al., 2003).
Early melanocyte culture methods also described the use of trypsin to separate the epidermis and dermis. However, dispase has the advantage of low cytotoxicity, and unlike trypsin, the thickness of the skin sample does not affect its efficiency (Kitano & Okada, 1983). Dispase is a neutral protease isolated from the bacterium B. polymyxa , and it acts by specifically cleaving fibronectin and type IV collagen (Stenn et al., 1989), compared to the non-specific action of the serine protease trypsin, prolonged use of which may damage cells and therefore result in lower cell yields.
Basic Protocol 2
Owing to their physiological interaction with melanocytes, keratinocytes make excellent feeder cells for melanocyte primary cultures. There is a requirement for the keratinocytes to be mitotically inactive to avoid contaminating the culture. A well-established method of producing mitotically inactive feeder cells is to treat the cells with the antibiotic mitomycin C. Mitomycin C targets guanine nucleosides in the sequence 5′CpG-3 (Tomasz, 1995), and causes inter-strand cross-links in the DNA, which means the DNA cannot replicate, and leads to cell cycle arrest. This should be an efficient process; however, it is always advisable to check that the treatment with mitomycin C has worked, as even a small percentage of dividing immortal keratinocytes will ruin the melanocyte culture.
The XB2 cell line is an immortal line of mouse keratinocytes and was first described by Rheinwald and Green (1975). XB2 cells are differentiated in culture from teratoma cells and were originally grown on mouse feeder fibroblasts; however, Bennett et al. (1987) selected a variant line that can grow without fibroblasts. These XB2 mouse keratinocytes can be purchased from the Functional Genomics Cell Bank at St. George's, University of London (see Internet Resources).
Basic Protocol 3
Murine melanocytes are an attractive model in which to study the genetics of diseases arising from melanocyte development and pigment production; over 350 genetic loci have been established as required for normal pigmentation alone (see Internet Resources). The mouse and human genomes are of a similar length and are highly conserved. Importantly, mice have a short mating cycle, producing on average 10-15 offspring per litter, with one litter per month. This means that mice are extremely suitable for breeding congenic mutant strains.
During murine development, neural crest cells migrate dorsolaterally between the dermomyotome and epidermis before differentiating into non-pigmented progenitor melanocytes called melanoblasts (reviewed by Kunisada et al., 1996), at around embryonic day 10.5 (E10.5) (Mayer, 1973). By E12, the melanoblasts have reached the limb buds, and at E13/E14 migrate into the epidermis of the lateral trunk (Mayer, 1973), where they differentiate further to become melanocytes before and after birth (reviewed by Kunisada et al., 1996). Melanocytes are therefore present in the late stages of development, and melanoblasts will also differentiate into melanocytes in the culture system described here; however, the fragility of the skin at this stage means it is harder to separate the epidermis from the dermis, increasing the risk of fibroblast contamination. Moreover, the mother mouse has to be killed to obtain the embryos. Thus, newborn mice are more commonly used for isolation of melanocytes.
With the number of pigmentary mutant genotypes available, there is opportunity to study the effects of these mutations at multiple stages of melanocyte development, and also their effects on differentiation, including melanin production and the molecular basis of pigmentary disorders including albinism. Furthermore, murine melanocytes have proved to be extremely useful for the elucidation of the molecular pathways underlying melanoma development and metastasis.
Note the differences between human and murine melanocyte culture methods: feeder keratinocytes are used to support the murine melanocytes, and TPA and CT are the only required mitogens. As mentioned, keratinocytes can release EDN1 and SCF, two mitogens for melanocytes; therefore, the inclusion of feeder cells replaces these extrinsic factors. It has not yet been established whether manufactured mitogens will rescue feeder-free murine melanocyte cultures, but feeder cells are valuable if attempting to immortalize mouse melanocytes, as the melanocytes can become very sparse at this stage. Similarly, we use dispase to split human skin but trypsin for mouse skin, because we have not tested the dispase method on mouse skin.
Basic Protocol 4
Small interfering RNAs (siRNAs) are short sequences of RNA, which, when transfected into cells, are able to silence corresponding genes (Dykxhoorn et al., 2003). Thus, siRNA transfection is a useful tool for studying the role of genes in important cellular processes. Indeed, the technique is very well-known and well-utilized, and many variations exist.
The transfection protocol discussed in this section has several advantages. Since the transfections are transient, the length of the experiment from start to finish is relatively short (versus stable transfections). Therefore, transfections of this type may be performed on a large scale, and data can be produced quickly. The high-throughput nature of the detailed protocol allows many siRNAs to be tested at once.
However, there are disadvantages associated with this protocol. A sophisticated plate reader is required to capture the data if 96-well plates are used; without one, it would be too laborious to process the results. Another limitation is that the ability for effects to be identified is determined by the efficiency of the transfection of any given siRNA. Therefore, if transfection efficiency is low, the effects of the knockdown may be missed and masked by the outgrowth of non-transfected cells. It is preferable to use a number of siRNAs (3-6) to target the same gene. The siRNAs could be pooled or used separately. Triplicates for each experiment are recommended. Finally, the knock-down (and possibly its effects) will be transient.
Critical Parameters
Basic Protocol 1
See the Table 1 troubleshooting guide for details.
Protocol | Problem | Possible cause | Solution |
---|---|---|---|
Relevant to all protocols | Fungal or bacterial contamination | Multiple causes |
This can be prevented by good aseptic technique and conditions, such as the following: a. All reagents and materials included for use in the protocol should be marked as sterile, and only opened in the laminar flow hood. All items should be sprayed with 70% ethanol before placing in the culture hood, and the culture hood should be cleaned thoroughly before use. If performing Basic Protocol 1 or 3, ensure that all forceps are sterilized in either a sterilizing oven or absolute ethanol before use. b. Wear laboratory safety clothing at all times during cell culture, ensuring gloves are pulled up under laboratory coats and no skin is exposed. c. Any individual with a cold or similar respiratory infection should at least wear a face mask or abstain from culturing during this time if possible. d. When transferring liquid from one container to another, ensure that no liquid touches the neck as this can produce a possible contamination pathway if the liquid contacts both interfaces when the container is closed. e. Only autoclaved or distilled water should be used to fill the water pan of the incubator, and this should be changed regularly. An antifungal compound, such as copper sulfate, can be added to the water if necessary. f. Regularly wipe the surface of the flask (do not spray) with 70% ethanol, or if cell growth is slow (e.g., close to senescence), trypsinize and transfer cells to a new flask around every 3 weeks. If diluting the cells less than 1 in 4 upon transfer, it is best to add soybean trypsin inhibitor to the harvested suspension (at 1 μg inhibitor per μg trypsin present) to help neutralize the trypsin. g. If fungal or yeast contamination occurs, treatment (as per the manufacturer's instructions) with an antifungal agent, such as Nystatin (e.g., Sigma, cat. no. N6261) could be tried to save the culture. |
Basic Protocol 1 | Unsatisfactory separation of the epidermis following dispase treatment | Skin sample too large or incubation period too short | Ensure that the skin is cut into pieces of 1 cm2 or smaller before the initial iodine treatment, and that the skin is incubated in the dispase II/Hank's solution for ≥18 hr. |
Basic Protocol 1 | Keratinocyte contamination | Carry over during isolation protocol | Following isolation, diploid keratinocytes may be observed growing in culture as isolated colonies. However, these cells tend to die and detach from the flask before subculturing is required, and any remaining adhered cells will not proliferate after subsequent passaging. |
Basic Protocol 1 | Cells die during protocol | Low density of primary melanocytes following isolation |
Initially, the plating density of primary melanocytes is low in comparison to the number of cells plated: ∼30% of the 1.5 × 105 cells plated will adhere to the flask within 2-3 days. To prevent cell death, several points can be addressed: a. Ensure that any large pieces of peeled epidermis are torn or cut into smaller pieces before trypsin incubation. b. While carrying out step 4, transfer any removed piece of epidermis to the diluted trypsin quickly, to avoid drying of the skin. c. The epidermis should be incubated in trypsin for 15 min only; any longer than this increases the risk of cell death. d. Care should be taken not to shear the cells when creating the cell suspension in step 6; the suspension should be mixed carefully using a slow and steady speed. e. Following step 8, cells should be incubated on ice. Melanocytes are easily able to attach to plastic (even tubes), which is inhibited by low temperatures. The cells can be kept on ice for ∼1 hr. f. During subculture, cells should be kept on ice as much as possible following step 14, to avoid loss and to ensure that the cell counts accurately represent the number of cells in culture. This is especially important should a growth curve graph be required. |
Basic Protocol 1 | Fibroblast contamination | Carry over during isolation protocol or excessive force during separation of dermis from epidermis |
During isolation: two pairs of blunt forceps should be used to separate the dermis and the epidermis; one to grip each layer. The relevant forceps should then be used exclusively for that layer of skin to prevent fibroblasts being transferred to any other solutions or any skin samples (if processing more than one sample). If the epidermis remains ‘stuck’ to the dermis, do not forcibly pull the layers apart, as dermal fibroblasts may adhere to the epidermis. Only a small piece of epidermis is required; one or two pieces of skin 1 cm2 in size are sufficient for a 25-cm2 culture flask. Post-isolation: Fibroblasts grow rapidly and can take over a culture once present but can be removed by the addition of 75-150 µM geneticin (G418) to the culture medium for 3-4 days. Geneticin is not toxic to melanocytes under these conditions, and if the contamination has not subsided after a few more days, the addition can be repeated. If fibroblasts appear whilst culturing human melanocytes, EDN1 can be initially excluded as it can aid fibroblast growth. |
Basic Protocol 2 | XB2 cells retain ability to divide | Ineffective treatment with mitomycin C | Ensure that cells are not confluent and are still growing prior to treatment with mitomycin C. It is recommended to test 106 cells from each batch of feeder cells, as described. If any growing colonies are seen that batch should be discarded and replaced with fresh stocks. |
Basic Protocol 3 | Dermis does not easily separate from epidermis | Inadequate time in trypsin | When trying to split the dermis from the epidermis it is important not to force their separation, as fibroblast contamination may result. If skin does not split easily, place it back in the trypsin at 37°C and leave for a further 10-15 min. Repeat until the two layers split easily. |
Fibroblast contamination | See above advice for Basic Protocol 1 | ||
Basic Protocol 4 | Unreliable data | Initial plating density not determined | Cell density can affect the quality and reliability of the results. This is particularly true when investigating parameters that affect cell growth or death. Select a range of seeding densities, perform the transfections, and then assess the results. For example, in a screen of siRNAs involving growth for 5 further days, the optimized seeding density for melanoma cells was 2000-3000 per well. This ensured that a reasonable density of cells remained in both positive and negative control wells. |
Basic Protocol 2
Owing to XB2 cells attaching very firmly, initial washes in EDTA are required. It is important to adjust the volumes of the washes according to the size of the flask/dish you are using.
Basic Protocol 3
It is critical that feeder cells be used that are mitotically inactive (see Basic Protocol 2). The animal should be hairless, and only newborn skin should be sterilized in 70% ethanol (do not sterilize embryonic skin with ethanol). When removing any muscle that may be left on the mouse skin, take care not to damage the dermis and the epidermis. Throughout the protocol, keep the skin samples wet—unless the protocol says otherwise—e.g., when incubating in trypsin we recommend 5 ml of trypsin as a guide; if you have a particularly large skin sample, make sure there is enough trypsin to cover the skin sample.
Take care when transferring the cultures to the incubator, as the dish is very full, which represents a potential source of contamination. It is recommended to remove the excess medium as soon as possible in this instance, but to leave enough time for any cells to attach.
It is important to check the cultures regularly for contamination with fibroblasts, which can take over the whole culture. (See Table 1 troubleshooting guide for details regarding fibroblast contamination).
The cells should be subcultured either when they are nearly confluent or if they are growing in large colonies. It can take an extended period of time to reach this level (3 or more weeks), but it is recommended to continue, even if you see very little growing. When trypsinizing the cells, it is important to check under the microscope that all the cells have detached, especially if the culture is sparse, in order to maximize the number of cells recovered. Feeders should be used until immortal cells take over the culture. Once this happens, CT can often be omitted (although it is best for the user to determine empirically whether the cells still require CT for routine culture). Immortal cells can be recognized because once the cells have senesced (around 4-6 weeks of culture), many cells fail to reattach at each subculture, and only a small fraction even of surviving melanocytes grows and forms colonies.
Basic Protocol 4
Treatment of cells pre-transfection
Cells should be treated well and remain healthy in the passages before transfection. This will increase the quality and reliability of the results.
Seeding density
It is vital that the seeding density for each cell line be optimized. Ideally, this should be performed before any large-scale transfections are performed.
siRNA master plate
Use an siRNA master plate to ease the transfer of siRNAs to the experimental plates. This will reduce errors and shorten the time it takes to transfect each plate.
siRNAs
It is ideal to use a number of siRNAs (as a pool or separately) to target the same gene. This increases the efficiency of transfection (and the cost of the experiment).
Control siRNAs
At least three control siRNAs are recommended:
Positive control: Choose an siRNA that will induce the required effect (growth arrest, for example). Negative control: Choose a scrambled siRNA. Transfection positive control: Use an siRNA that is toxic for the cell and therefore is a good test of transfection efficiency. A good example of this is AllStars Hs Cell Death Control siRNA from Qiagen.
Pipetting
The use of fresh pipette tips is important to reduce cross-contamination of siRNAs. Thorough mixing of solutions is paramount to the success of the transfections.
Troubleshooting
Table 1 summarizes commonly encountered technical issues along with suggested solutions.
Anticipated Results
Basic Protocol 1
The inclusion of only one to two pieces of adult skin 1 cm2 in size is sufficient to yield 1.5 × 105 cells. The culture will become confluent after 3 weeks. Subsequent passages will take between 10 and 14 days to become confluent; later passages take up to around a month. Fibroblasts and especially keratinocytes are commonly found within this first passage, but growth of these is easily remedied as described in the troubleshooting section. An example of growing human melanocytes can be seen in Figure 2A. Figure 2B shows senescent melanocytes, which are characterized by their large, flat appearance.
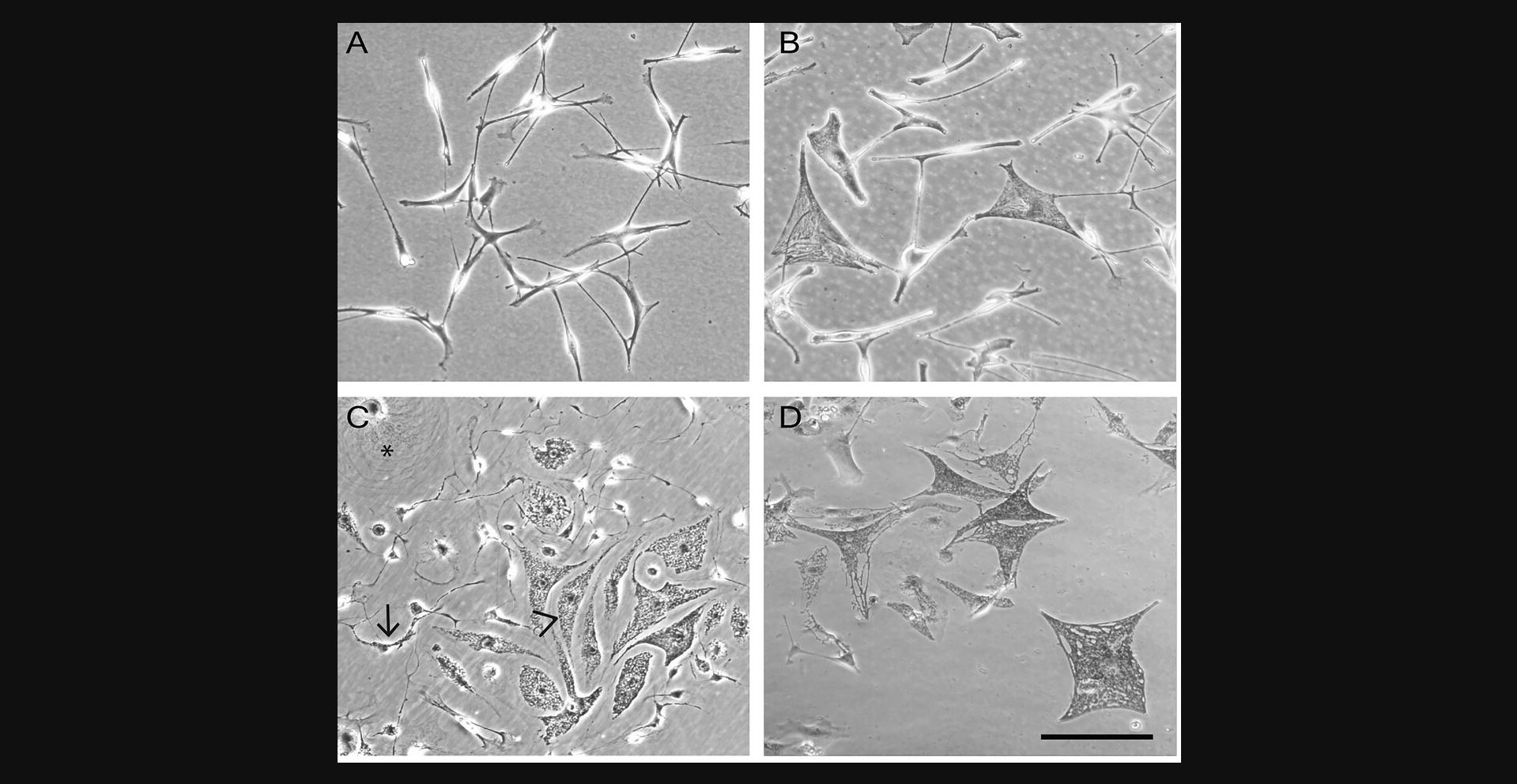
Basic Protocol 2
If using XB2 cells as feeder cells for primary cultures, it is advisable to make a large stock of mitomycin C-treated cells. Once tested to make sure the cells are no longer dividing, stocks can be stored in liquid nitrogen and used when required. From a 175-cm2 flask (35 ml culture), the expected yield of mitomycin C-treated cells is ∼1 × 107 cells, and we store these in liquid nitrogen in aliquots of either 0.5 × 106 or 1 × 106 per vial, giving 10-20 vials of mitomycin C-treated cells. Figure 2C shows a growing primary culture from mouse skin containing melanocytes, melanoblasts, and feeder cells.
Basic Protocol 3
Melanocyte colonies should form after roughly 2-3 weeks; however, this may take longer, and so it is recommended to persevere. The diploid cells will grow for only a few passages under the conditions described. However, ∼1 in 106 normal mouse melanocytes spontaneously becomes immortal, and these form growing colonies of small, less-pigmented cells which should all survive and proliferate after subculture. If any melanocytes are proliferating by 3 months after primary culture, they are almost certainly immortal. It may take up to 6 months to obtain enough of the immortal cells to freeze stocks. Note that this time can be greatly shortened by crossing the required genotype of mouse with Cdkn2a (Ink4a-Arf) null mice, as Cdkn2a null melanocytes do not senesce at all (Sviderskaya et al., 2002). Figure 2D shows a culture of mouse melanocytes, with the appearance of senescent melanocytes, which are larger and highly pigmented.
Basic Protocol 4
Post-transfection, cells can be permeabilized and fixed for subsequent cell and nuclear staining, using established staining protocols (not described here; Soo et al., 2011). If using siRNAs that are anticipated to inhibit growth or induce cell death, for example, a simple pan-nuclear stain such as DAPI could be used. In this case, the following results would be expected:
Positive siRNA control (e.g., inhibits growth): cell number will decrease. Negative siRNA control (e.g., growth unaffected): cell number will increase. Test siRNA: Cell number will increase or decrease depending on the targeted gene.
Time Considerations
Basic Protocol 1
The protocol for the preparation of the skin sample and isolation of melanocytes is described in steps 1-11 and performed over 2 days. The preparatory stages of cleaning and submerging the skin in dispase solution should take ∼30 min, and the second day protocol consisting of the removal of the epidermis and isolation of melanocytes will take 1-2 hr, depending on the number of samples received.
The RPMI growth medium should be changed every 3-4 days, taking no longer than 5 min per flask, and subculturing of cells at subsequent passages as described in steps 13-17 will take ∼30 min. If freezing the cells, allow a further 15-30 min, depending on the number of cryotubes required.
As mentioned previously, the primary melanocyte cell culture at passage 0 will take ∼3 weeks to become confluent, with subsequent passages taking 10-14 days. The time required to reach confluency will increase with age of the cell culture; if the cells are near senescence, this may take as long as 6 weeks. In general, it will take primary melanocyte cultures about 3-12 months to become fully senescent, varying with donor age, among other parameters.
Basic Protocol 2
The subculture of XB2 keratinocytes can be achieved in ∼20-25 min, depending on the time taken for the cells to detach from the flask. When preparing mitomycin C-treated XB2 keratinocytes, the total incubation time is between 3 and 3.5 hr, with subsequent incubation in DMEM growth medium for 10 min to elute any remaining mitomycin C. This is then followed by harvesting and freezing of the treated cells. Batch testing takes ∼2 weeks.
Basic Protocol 3
The plating out of mitomycin C-treated XB2 keratinocytes can be achieved in ∼15-25 min and should be carried out 1-3 days before the rest of the protocol. The number of skin samples determines the time for the explantation protocol. If preparing more than one skin sample, it is recommended to space them out throughout the day, for example, half the samples in the morning and the rest in the afternoon. For one sample, the initial period (steps 2-6) should take 15-20 min. This is then followed by a 1 hr incubation. If the skin is able to be split after this period, then the remaining steps (8-19) should take 15-20 min (if not able to be split, place back into the trypsin and check every 10-15 min to see if skin can be split). After practice and increased familiarity with the technique, ∼8 skin samples can be processed in a day. Subculture should take between 15 and 20 min (depending on how easily the cells detach). The cells start to senesce around 4-6 weeks after explantation. Time for immortalization is discussed above.
Basic Protocol 4
Pre-transfection
It is vital that cells are healthy and growing well before starting the protocol. Do not allow the cells to become 100% confluent in the passages prior to transfection, as this can change the results.
Day 1
Cells are transfected and seeded on the same day. While learning, one 96-well plate per cell line would be advised to ensure efficient transfection. However, with practice, five to ten plates could be transfected in one day.
Acknowledgments
No funding to acknowledge.
Author Contributions
Philip S. Goff : Writing - original draft, writing - review and editing; Joanna T. Castle : Writing - original draft; Jaskaren S. Kohli : Writing - original draft; Elena V. Sviderskaya : Methodology, supervision, writing - review and editing; Dorothy C. Bennett : Methodology, supervision, writing - review and editing.
Conflict of Interest
The authors declare no conflict of interest.
Open Research
Data Availability Statement
Data sharing not applicable to this article as no datasets were generated or analyzed during the current study.
Literature Cited
- Alzahofi, N., Welz, T., Robinson, C. L., Page, E. L., Briggs, D. A., Stainthorp, A. K., Reekes, J., Elbe, D. A., Straub, F., Kallemeijn, W. W., Tate, E. W., Goff, P. S., Sviderskaya, E. V., Cantero, M., Montoliu, L., Nedelec, F., Miles, A. K., Bailly, M., Kerkhoff, E., & Hume, A. N. (2020). Rab27a co-ordinates actin-dependent transport by controlling organelle-associated motors and track assembly proteins. Nature Communications , 11(1), 3495. https://doi.org/10.1038/s41467-020-17212-6
- Bennett, D. C. (2016). Genetics of melanoma progression: The rise and fall of cell senescence. Pigment Cell & Melanoma Research, 29(2), 122–140.
- Bennett, D. C., Cooper, P. J., & Hart, I. R. (1987). A line of non-tumorigenic mouse melanocytes, syngeneic with the B16 melanoma and requiring a tumour promoter for growth. International Journal of Cancer , 39, 414–418. https://doi.org/10.1002/ijc.2910390324
- Brenner, M., & Hearing, V. J. (2008). The protective role of melanin against UV damage in human skin. Photochemistry and Photobiology , 84(3), 539–549. https://doi.org/10.1111/j.1751-1097.2007.00226.x
- Current Protocols. (2006). Commonly Used Reagents. Current Protocols in Microbiology , 00, A.2A.1–A.2A.15. https://doi.org/10.1002/9780471729259.mca02as00
- Dykxhoorn, D. M., Novina, C. D., & Sharp, P. A. (2003). Killing the messenger: Short RNAs that silence gene expression. Nature Reviews Molecular Cell Biology , 4, 457–467. https://doi.org/10.1038/nrm1129
- Eisinger, M., & Marko, O. (1982). Selective proliferation of normal human melanocytes in vitro in the presence of phorbol ester and cholera toxin. Proceedings of the National Academy of Sciences of the United States of America , 79, 2018–2022. https://doi.org/10.1073/pnas.79.6.2018
- Hachiya, A., Kobayashi, A., Yoshida, Y., Kitahara, T., Takema, Y., & Imokawa, G. (2004). Biphasic expression of two paracrine melanogenic cytokines, stem cell factor and endothelin-1, in ultraviolet B-induced human melanogenesis. American Journal of Pathology , 165, 2099–2109. https://doi.org/10.1016/S0002-9440(10)63260-9
- Hirobe, T., Shinpo, T., Higuchi, K., & Sano, T. (2010). Life cycle of human melanocytes is regulated by endothelin-1 and stem cell factor in synergy with cyclic AMP and basic fibroblast growth factor. Journal of Dermatological Science , 57, 123–131. https://doi.org/10.1016/j.jdermsci.2009.11.006
- Hume, A. N., & Seabra, M. C. (2011). Melanosomes on the move: A model to understand organelle dynamics. Biochemical Society Transactions , 39, 1191–1196. https://doi.org/10.1042/BST0391191
- Kitano, Y., & Okada, N. (1983). Separation of the epidermal sheet by dispase. British Journal of Dermatology , 108, 555–560. https://doi.org/10.1111/j.1365-2133.1983.tb01056.x
- Kobayashi, N., Nakagawa, A., Muramatsu, T., Yamashina, Y., Shirai, T., Hashimoto, M., Ishigaki, Y., Ohnishi, T., & Mori, T. (1998). Supranuclear melanin caps reduce ultraviolet induced DNA photoproducts in human epidermis. Journal of Investigative Dermatology , 1998, 806–810. https://doi.org/10.1046/j.1523-1747.1998.00178.x
- Kunisada, T., Yoshida, H., Ogawa, M., Shultz, L. D., & Nishikawa, S. (1996). Characterization and isolation of melanocyte progenitors from mouse embryos. Development Growth and Regeneration , 38, 87–97.
- Lamoreux, M. L., Delmas, V., Larue, L., & Bennett, D. C. (2010). The colors of mice: A model genetic network. Wiley-Blackwell.
- Le, L., Sirés-Campos, J., Raposo, G. G., Delevoye, C., & Marks, M. S. (2021). Melanosome biogenesis in the pigmentation of mammalian skin. Integrative and Comparative Biology , 61(4), 1–67. https://doi.org/10.1093/icb/icab078
- Limaye, A., Hall, B., & Kulkarni, A. B. (2009). Manipulation of mouse embryonic stem cells for knockout mouse production.Current protocols in cell biology , Chapter, 19, Unit–19.13.24. https://doi.org/10.1002/0471143030.cb1913s44
- Mayer, T. C. (1973). The migratory pathway of neural crest cells into the skin of mouse embryos. Developmental Biology , 34, 39–46. https://doi.org/10.1016/0012-1606(73)90337-0
- Michaloglou, C., Vredeveld, L., Soengas, M., Denoyelle, C., Kuilman, T., van der Horst, C., Majoor, D., Shay, J., Mooi, W., & Peeper, D. (2005). BRAF E600-associated senescencelike cell cycle arrest of human naevi. Nature , 436, 720–724. https://doi.org/10.1038/nature03890
- O'Keefe, E., & Cuatrecasas, P. (1974). Cholera toxin mimics melanocyte stimulating hormone in inducing differentiation in melanoma cells. Proceedings of the National Academy of Sciences of the United States of America , 71, 2500–2504. https://doi.org/10.1073/pnas.71.6.2500
- Rheinwald, J. G., & Green, H. (1975). Formation of a keratinizing epithelium in culture by a cloned cell line derived from a teratoma. Cell , 6, 317–330. https://doi.org/10.1016/0092-8674(75)90183-X
- Soo, J. K., Mackenzie Ross, A. D., Kallenberg, D. M., Milagre, C., Heung Chong, W., Chow, J., Hill, L., Hoare, S., Collinson, R. S., Hossain, M., Keith, W. N., Marais, R., & Bennett, D. C. (2011). Malignancy without immortality? Cellular immortalization as a possible late event in melanoma progression. Pigment Cell & Melanoma Research, 24, 490–503.
- Stenn, K. S., Link, R., Moellmann, G., Madri, J., & Kuklinska, E. (1989). Dispase, a neutral protease from Bacillus polymyxa , is a powerful fibronectinase and type IV collagenase. Journal of Investigative Dermatology , 93, 287–290. https://doi.org/10.1111/1523-1747.ep12277593
- Sviderskaya, E. V., Bennett, D. C., Ho, L., Bailin, T., Lee, S. T., & Spritz, R. A. (1997). Complementation of hypopigmentation in p-mutant (pinkeyed dilution) mouse melanocytes by normal human P cDNA, and defective complementation by OCA2 mutant sequences. Journal of Investigative Dermatology , 108, 30–34. https://doi.org/10.1111/1523-1747.ep12285621
- Sviderskaya, E. V., Hill, S. P., Evans-Whipp, T. J., Chin, L., Orlow, S. J., Easty, D. J., Cheong, S. C., Beach, D., DePinho, R. A., & Bennett, D. C. (2002). p16(Ink4a) in melanocyte senescence and differentiation. JNCI: Journal of the National Cancer Institute , 94, 446–454. https://doi.org/10.1093/jnci/94.6.446
- Sviderskaya, E. V., Gray-Schopfer, V. C., Hill, S. P., Smit, N. P., Evans-Whipp, T. J., Bond, J., Hill, L., Bataille, V., Peters, G., Kipling, D., Wynford-Thomas, D., & Bennett, D. C. (2003). P16/cyclin-dependent kinase inhibitor 2A deficiency in human melanocyte senescence, apoptosis and immortalisation: Possible implications for melanoma progression. JNCI: Journal of the National Cancer Institute , 95, 723–732. https://doi.org/10.1093/jnci/95.10.723
- Sviderskaya, E. V., Kallenberg, D. M., & Bennett, D. C. (2010). Resource. The Wellcome Trust Functional Genomics Cell Bank: Holdings. Pigment Cell & Melanoma Research, 23, 147–150.
- Tomasz, M. (1995). Mitomycin C: Small, fast and deadly (but very selective). Chemistry & Biology, 2, 575–579.
- Wu, X., & Hammer, J. A. (2014). Melanosome transfer: It is best to give and receive. Current Opinion in Cell Biology , 29(1), 1–7. https://doi.org/10.1016/j.ceb.2014.02.003
- Yamaguchi, Y., & Hearing, V. J. (2014). Melanocytes and their diseases. Cold Spring Harbor Perspectives in Medicine , 4(5), a017046–a017046. https://doi.org/10.1101/cshperspect.a017046
- Yamasaki, T., Takahashi, A., Pan, J., Yamaguchi, N., & Yokoyama, K. K. (2009). Phosphorylation of activation transcription factor-2 at serine 121 by protein kinase C controls c-Junmediated activation of transcription. Journal of Biological Chemistry , 284, 8567–8581. https://doi.org/10.1074/jbc.M808719200
Key Reference
- Bennett et al. (1987). See above.
The development of the first non-tumorigenic melanocyte mouse line.
- Eisinger and Marko (1982). See above.
A description of the first long-term culture of human melanocytes.
- Sviderskaya et al. (1997). See above.
Includes the current mouse melanocyte culture method.
Internet Resources
Our materials and methods page with further and related details for melanocyte culture methods.
Web site of the Functional Genomics Cell Bank at St. George's, University of London with access to, among others, mouse melanocyte and melanoblast lines carrying a variety of pigmentary mutations.
Information on the mapped color genes in mice, zebrafish, and human models.