High-Dimensional Methods of Single-Cell Microglial Profiling to Enhance Understanding of Neuropathological Disease
Katherine R. Pilkington, Katherine R. Pilkington, Alanna G. Spiteri, Alanna G. Spiteri, Claire L. Wishart, Claire L. Wishart, Laurence Macia, Laurence Macia, Nicholas J.C. King, Nicholas J.C. King
autofluorescence
cell sorting
CNS pathology
cytometry
microglia
myeloid
neuroinflammation
single-cell
spectral cytometry
Abstract
Microglia are the innate myeloid cells of the central nervous system (CNS) parenchyma, functionally implicated in almost every defined neuroinflammatory and neurodegenerative disorder. Current understanding of disease pathogenesis for many neuropathologies is limited and/or lacks reliable diagnostic markers, vaccines, and treatments. With the increasing aging of society and rise in neurogenerative diseases, improving our understanding of their pathogenesis is essential. Analysis of microglia from murine disease models provides an investigative tool to unravel disease processes. In many neuropathologies, bone-marrow-derived monocytes are recruited to the CNS, adopting a phenotype similar to that of microglia. This significantly confounds the accurate identification of cell-type-specific functions and downstream therapeutic targeting. The increased capacity to analyze more phenotypic markers using spectral-cytometry-based technologies allows improved separation of microglia from monocyte-derived cells. Full-spectrum profiling enables enhanced marker resolution, time-efficient analysis of >40 fluorescence parameters, and extraction of cellular autofluorescence parameters. Coupling this system with additional cytometric technologies, including cell sorting and high-parameter imaging, can improve the understanding of microglial phenotypes in disease. To this end, we provide detailed, step-by-step protocols for the analysis of murine brain tissue by high-parameter ex vivo cytometric analysis using the Aurora spectral cytometer (Cytek), including best practices for unmixing and autofluorescence extraction, cell sorting for single-cell RNA analysis, and imaging mass cytometry. Together, this provides a toolkit for researchers to comprehensively investigate microglial disease processes at protein, RNA, and spatial levels for the identification of therapeutic targets in neuropathology. © 2024 The Authors. Current Protocols published by Wiley Periodicals LLC.
Basic Protocol 1 : Processing the mouse brain into a single-cell suspension for microglia isolation
Basic Protocol 2 : Staining single-cell mouse brain suspensions for microglial phenotyping by spectral cytometry
Basic Protocol 3 : Flow cytometric sorting of mouse microglia for ex vivo analysis
Basic Protocol 4 : Processing the mouse brain for imaging mass cytometry for spatial microglia analysis
INTRODUCTION
Diseases affecting the central nervous system (CNS) are collectively the leading cause of disability and second leading cause of death worldwide (Bassetti et al., 2022; Feigin et al., 2020). Often prominent in the pathogenesis of CNS disease are microglia, a remarkable innate myeloid immune cell population that tiles the entire CNS parenchyma, projecting cytoplasmic extensions that constantly survey the microenvironment (Nimmerjahn et al., 2005). Upon perturbation, these cells may proliferate, increase the cross-sectional area of their somata, retract cytoplasmic processes, and express a range of cytokines and signaling molecules. Microglia have been implicated in protective and pathological processes contributing to neuroinflammatory, neurodegenerative, and mental health disorders (McQuade & Blurton-Jones, 2019). As such, they represent an important potential target for immunotherapeutics in CNS disease. Historically, elucidating the specific roles of microglia in pathology has been extremely challenging due to the limited research tools available to identify and target these cells behind the blood-brain barrier (Spiteri, Wishart et al., 2022). In some diseases, identifying microglia becomes further challenged by the infiltration of phenotypically similar myeloid cells from the bone marrow (Getts et al., 2008, 2012; Greter et al., 2015; Lewis et al., 2014; Werner et al., 2020). This has significantly impeded understanding of disease pathogenesis in many neuropathologies and hampered the development of reliable diagnostic markers, vaccines, and treatments.
In recent years, a surge in the development of new experimental tools has enabled more accurate microglia identification and targeting. These include microglia-specific identification markers such as P2RY12 and TMEM119 (Bennett et al., 2016; Butovsky et al., 2014) and the CSF1R inhibitor and microglia-depletion drug PLX5622 (Spangenberg et al., 2019). Although these represent significant advances in the field, subsequent work has shown that microglia-specific markers are often downregulated during inflammation, while PLX5622 can also target other cells in the periphery (Depaula-Silva et al., 2019; Greter et al., 2015; Lei et al., 2020; Lewis et al., 2014; Spiteri & King, 2023; Spiteri, Ni et al., 2022; Spiteri et al., 2021; Spiteri, Wishart et al., 2023; Spiteri et al., 2020; Vankriekelsvenne et al., 2022). Moreover, isolated and cultured microglia undergo considerable change from their in vivo state (Bennett et al., 2018; Bohlen et al., 2017; Gosselin et al., 2017), significantly limiting the translatability of in vitro studies. Thus, examination of microglia from untreated and undepleted brain under minimal experimental intervention is crucial to determine their precise role(s) during the pathogenesis of disease. The prompt ex vivo analysis of surface and intracellular protein expression and proliferative status using bromodeoxyuridine (BrdU) or Ki-67, as well as morphology and RNA profile, at various timepoints can together inform detailed understanding of microglial processes.
Advances in spectral cytometry, imaging mass cytometry, and single-cell RNA (scRNA) technology have substantially increased the number of parameters that can be analyzed in a single sample. Pairing these with computational analysis approaches allow better discrimination between microglia and other myeloid subsets. This is important to accurately identify their relative contributions to disease pathogenesis. Although the use of single-cell technologies has increased in neuroscience research, consensus on the best cytometric approach for multiple modalities with validated panels remains scant (Sharp et al., 2023). Thus, we detail here single-cell methods of microglia phenotyping to provide a toolkit for researchers to use to examine these cells in molecular detail for the identification of therapeutic targets in murine models of neuropathology (Fig. 1). Specifically, Basic Protocol 1 describes methods to process whole brain tissue into single-cell suspensions. Basic Protocol 2 details surface and intracellular staining steps to phenotype microglia by spectral cytometry using the Aurora. Basic Protocol 3 describes steps to stain and sort microglia for ex vivo analysis such as single-cell sequencing. Basic Protocol 4 describes brain-processing methods to obtain high-quality histological images.
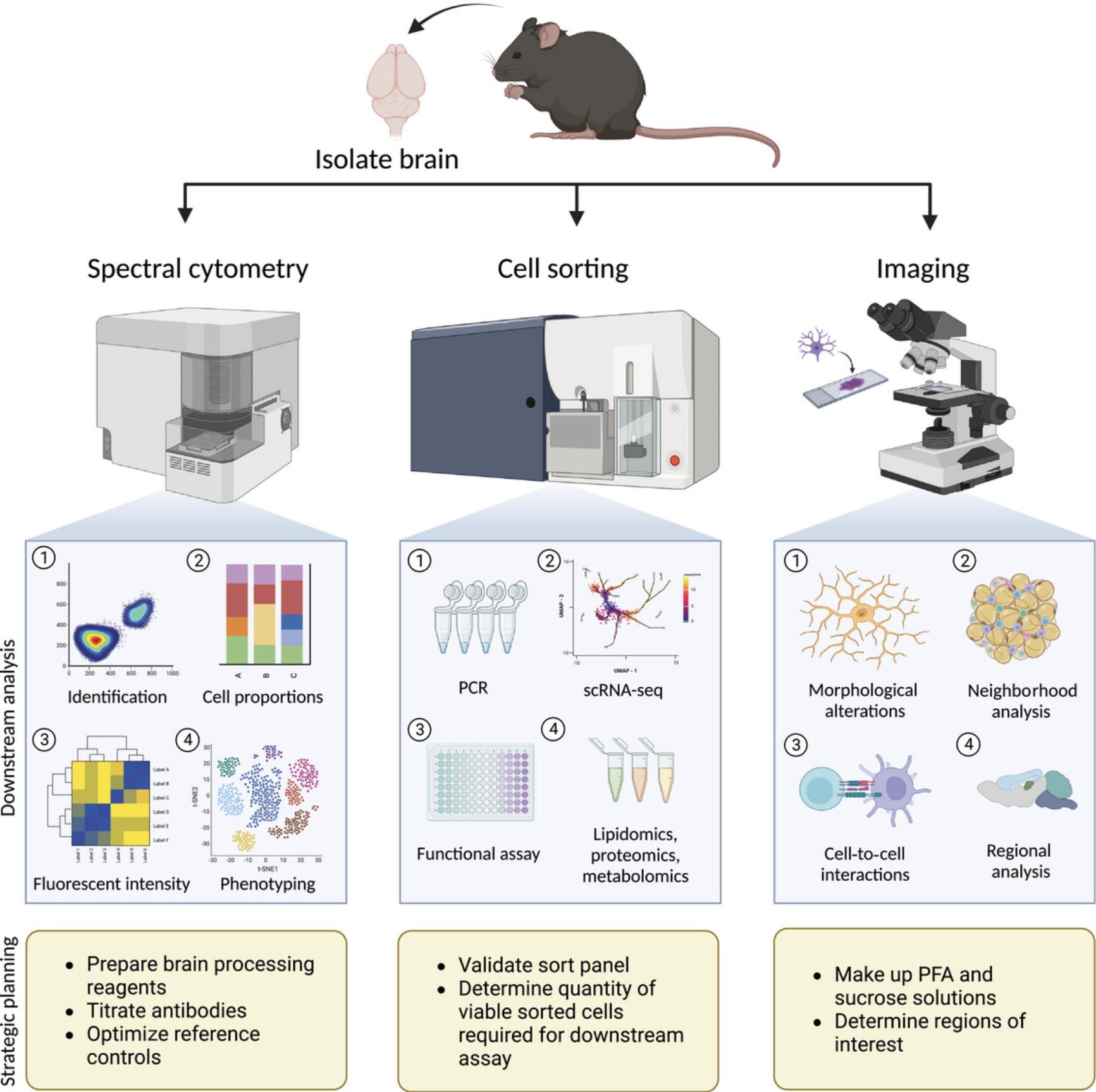
NOTE : All protocols involving animals must be reviewed and approved by the appropriate Animal Care and Use Committee and must follow regulations for the care and use of laboratory animals.
Basic Protocol 1: PROCESSING THE MOUSE BRAIN INTO A SINGLE-CELL SUSPENSION FOR MICROGLIA ISOLATION
Preparing a single-cell suspension is a critical step for single-cell analysis of whole tissues, including for conventional and spectral flow cytometry, cytometry by time of flight (CyTOF), cell sorting, and scRNA analysis. Poor-quality tissue processing impacts cell viability, staining resolution, and the ease of counting, filtering, and running samples on instruments. Conducting the protocol below should yield high-quality data with 85%-90% viability of brain cells.
Various protocols have served as a starting point for the optimized methods described here (Ashhurst et al., 2019; Bohlen et al., 2019; Garcia et al., 2014; Getts et al., 2008; Manglani et al., 2018; Niewold et al., 2020). In this protocol, mice are injected 3 hr before sacrifice with BrdU, an analog of thymidine that is incorporated into DNA during synthesis. Using a fluorochrome-conjugated antibody to label BrdU, it can be determined whether microglia (or other cells) are proliferating in situ. Euthanasia procedures should minimize brain anoxia prior to tissue dissociation. The carefully removed brain is cut up into pieces of 0.3-cm diameter or less. Individual cells are dissociated from the whole brain using the GentleMACS (Miltenyi Biotec) dissociator in a cocktail of DNase I and collagenase type IV, and leukocytes are enriched on a Percoll density gradient (Fig. 2). This protocol should be performed efficiently without interruption to enhance yield and viability.
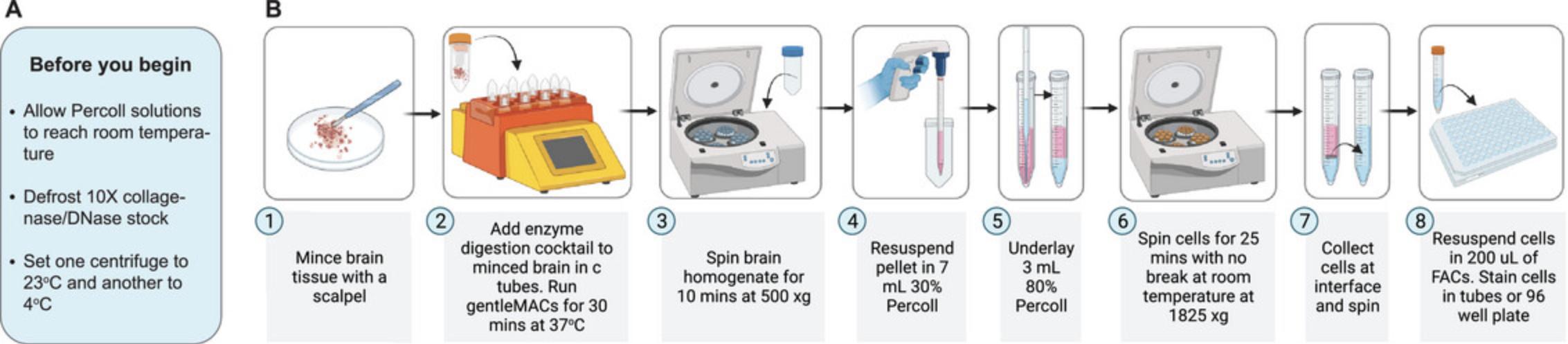
A disadvantage of this enzymatic method is the potential to cleave cell surface markers. From our work, we have shown reductions only in TMEM119 and CD44 expression when using DNase I and collagenase IV (Spiteri et al., 2021). We recommend performing parallel experiments without digestive enzymes to ensure that all surface markers of interest are present. Experiments without enzymes will still yield high-quality data; however, reduced viability and increased debris, should be expected. Digestion with DNase I and collagenase IV, however, is strongly recommended for microglia isolation as it produces high live cell yields (∼5 × 105 cells from the healthy homeostatic brain). Nevertheless, it should be noted that alternative commercial methods are also available, including the neural tissue dissociation kit from Miltenyi Biotec (Garcia et al., 2014). Additionally, if a GentleMACS dissociator is not available, enzyme-digested brain tissue can be gently mashed through a strainer using a pestle (Manglani et al., 2018).
Materials
-
10 mg/ml (2×) BrdU stock solution (see recipe; 1 mg per 18-g mouse)
-
Mice
-
Isoflurane or equivalent for mouse euthanasia, depending on permitted animal ethics protocols
-
Ice-cold PBS (∼25 ml per sample; see recipe)
-
70% (v/v) ethanol
-
10× DNase/collagenase digestion mixture (see recipe; 0.5 ml per sample)
-
Staining buffer (see recipe; ∼12 ml per sample)
-
26% (v/v) Percoll solution (see recipe; 7 ml per sample)
-
73% (v/v) Percoll solution (see recipe; 3 ml per sample)
-
Trypan blue (Thermo Fischer Scientific, cat. no. 15250061)
-
Laminar-flow hood (or, if working with PC2 agents, BSC Class II cabinet or equivalent)
-
Dissection tools: forceps, scissors, and scalpels
-
10-ml syringe
-
21-G needle (1 per mouse)
-
Petri dishes (1 per experimental group)
-
C tubes (Miltenyi Biotec, cat. no. 130-093-237)
-
GentleMACS Octo Dissociator with Heaters (Miltenyi Biotec, cat. no. 130-096-427)
-
50-ml Falcon tube lids (1 per sample)
-
15-ml tubes (2 per sample)
-
Centrifuge
-
Glass pipets
-
Serological pipets: 1 × 5 ml for each brain, 1 × 25 ml to dispense 26% Percoll, and 1 × 5 ml to dispense 73% Percoll
-
1-ml and 10-µl micropipets
-
50-µl multichannel micropipets
-
96-well round-bottom cell-culture-treated plate (Sigma Aldrich, cat. no. CLS3799)
-
Hemocytometer
-
Cell counter
-
Light microscope
-
NOTE: If you are not assessing cell proliferation, start from step 4.
1.Defrost 10 mg/ml BrdU stock solution at room temperature and dilute with sterile PBS to make a 5 mg/ml solution.
2.Inject mice intraperitoneally with 55.6 mg/kg BrdU (1 mg per 18-g mouse; 200 μl of a 5 mg/ml solution).
3.After 3 hr, anesthetize animals in the order in which you injected them with BrdU.
4.Surgically expose the heart and perfuse mice via the left ventricle with 10 ml ice-cold PBS, using a 21-G needle and a 10-ml syringe, snipping the inferior vena cava to enable complete flushing of the brain vasculature (see Current Protocols, Miller et al., 2010).
5.Decapitate mice with large surgical scissors.
6.Cut the skin sagittally from the base of the neck to the eyes using finer scissors.
7.Peel back the skin laterally to expose the skull.
8.Use finer scissors to gently cut through the midline of the skull from the foramen magnum to the eyes.
9.Make an incision in the nasal process.
10.Using forceps gently pull each side of the skull laterally to expose the brain.
11.Pry the brain out with forceps onto a petri dish containing ∼500 μl PBS.
12.Cut the brain into pieces of 2- to 3-mm diameter or less using a scalpel.
13.Transfer brain pieces into a C tube containing 4.5 ml ice-cold PBS.
14.Place C tube on ice.
15.Collect a spleen and/or bone marrow from one animal for extra cell staining controls for Basic Protocol 2.
16.Add 0.5 ml of 10× collagenase/DNase mixture into C tubes once all brains have been collected.
17.Load C tubes and heaters onto GentleMACS dissociator.
18.Select brain-processing program and run (i.e., 30 min at 37°C, as per Supporting Information 1).
19.Top up with staining buffer and seal with 50-ml Falcon tube lid.
20.Place tubes on ice until ready to process.
21.Spin tubes 10 min at 500 × g , 4°C.
22.Carefully pour out supernatant.
23.Resuspend brain samples in 7 ml of 26% Percoll solution using a 5-ml serological pipet and transfer to a 15-ml tube.
24.Place a glass pipet in the center of the tube and pipet 3 ml of 73% Percoll solution into the glass pipet using a 5-ml serological pipet. This should create a sharply defined 26%/73% Percoll fluid interface.
25.Carefully place the tubes in the centrifuge, without disturbing the Percoll interface.
26.Spin tubes for 25 min at 1825 × g , room temperature, no brake.
27.Remove upper fat layer using a pipet tip attached to a vacuum aspirator or 1-ml micropipet.
28.Collect leukocytes from the interface layer between the 26% top (pink) and 73% bottom (clear) layers using a glass pipet or a 1-ml pipet until there are no cells left (∼3 ml).
29.Transfer collected leukocytes to a 15-ml tube containing 5 ml staining buffer.
30.Spin tubes 5 min at 500 × g , 4°C.
31.Discard supernatant and resuspend cells in 200 μl staining buffer.
32.Transfer samples into a 96-well plate, or leave in tubes if preferred. Remeasure each sample volume while transferring samples from tubes to wells or resuspending cells in tubes.
33.Transfer 10 μl of each sample into another tube or plate for counting (leave in fridge).
34.Pre-dilute counting samples in staining buffer according to Table 1.Add 10 μl trypan blue to 10 μl of pre-diluted sample and load on hemocytometer for counting.
Brain sample | Expected cell count | Staining buffer volume to predilute sample | Total dilution factor |
---|---|---|---|
Homeostatic (full brain) | 3.5-7.5 × 105 | 0 μl | 2 |
Moderate chronic neuroinflammationa | 1 × 106 | 0 μl | 2 |
Severe acute neuroinflammationb | 4.5 × 106 | 40 μl | 10 |
- a
Cerebral interferonopathy modelled in transgenic mice with CNS-targeted chronic production of IFN-α (GFAP-IFN; West et al., 2022) or demyelination modeled in mice treated with cuprizone (Song et al., 2021).
- b
West Nile virus encephalitis (Spiteri, Ni et al., 2022; Spiteri et al., 2021; Spiteri, Wishart et al., 2023; Spiteri, Van Vreden et al., 2023; Wishart et al., 2022).
Basic Protocol 2: STAINING SINGLE-CELL MOUSE BRAIN SUSPENSIONS FOR MICROGLIAL PHENOTYPING BY SPECTRAL CYTOMETRY
Depending on the extent of CNS perturbation, microglia upregulate nominal activation markers and produce an array of cytokines and signaling molecules that can dampen or exacerbate pathology. Identifying microglial phenotypes can inform functional processes. However, this must be coupled with additional techniques such as in vivo imaging, scRNA analysis, and proteomics to identify their precise contribution to disease processes.
Here we provide a framework for detailed microglial phenotyping involving surface, intracellular, and intranuclear staining and acquisition on a spectral cytometer (5-laser Cytek Aurora). These include myeloid cell markers (CD45, CD11b, F4/80), antigen-presenting and co-stimulation markers (CD86, MHC-II), migration and phagocytosis-related markers (CD11c, CD64, CD68, CD206), the ‘inflammatory macrophage’ marker Ly6C, markers highly expressed on microglia (P2RY12, CX3CR1), and markers to identify and quantify neutrophils (Ly6G), NK cells (NK1.1), and T cells (CD3ε, CD4, CD8α). To measure proliferation and nitric oxide production, we use BrdU and DAF-FM diacetate, respectively. Production of nitric oxide can enhance CNS pathology and can be targeted therapeutically (Getts et al., 2012). To measure other functional markers, such as intracellular cytokines, monensin and/or brefeldin A should be added to staining buffers as well as all reagents until cell fixation, including PBS used for perfusion and brain processing reagents, to enhance staining.
During neuroinflammation, monocytes from the bone marrow are recruited to the brain, adopting a similar phenotype to responding microglia. To demonstrate this, we use a mouse model of severe and acute neuroinflammation as seen in West Nile virus (WNV) encephalitis. In this model, there is a 10-fold increase in the number of cells isolated from the brain, with the majority being monocyte-derived cells. To distinguish microglia from infiltrating myeloid cells in the inflamed brain, we used the differential profiles of P2RY12 and CD45.The gating strategy is shown in Figure 3 and can be applied to other inflammatory mouse models as well as the homeostatic brain (Supporting Information 2). Although multiple RNA studies, including our own, have shown that microglia downregulate the expression of the nominal homeostatic microglia-specific markers P2ry12 and Tmem119 during neurodegeneration and neuroinflammation (Jordão et al., 2019; Keren-Shaul et al., 2017; Krasemann et al., 2017; Spiteri, Wishart et al., 2023; Wishart et al., 2022), on a protein level, microglia do not downregulate P2RY12 expression in WNE (Spiteri et al., 2021) or alpha-herpesvirus CNS infection (Fekete et al., 2018). As TMEM119 expression is downregulated by microglia in certain inflammatory diseases, such as WNE, P2RY12 enables the separation of microglia from infiltrating myeloid populations when used in combination with CD45.This strategy has been validated with adoptive transfers, monoclonal antibody blocking, and injection of dye to track infiltrating myeloid populations, which could be distinguished from microglia that were depleted with PLX5622 (Spiteri et al., 2021).
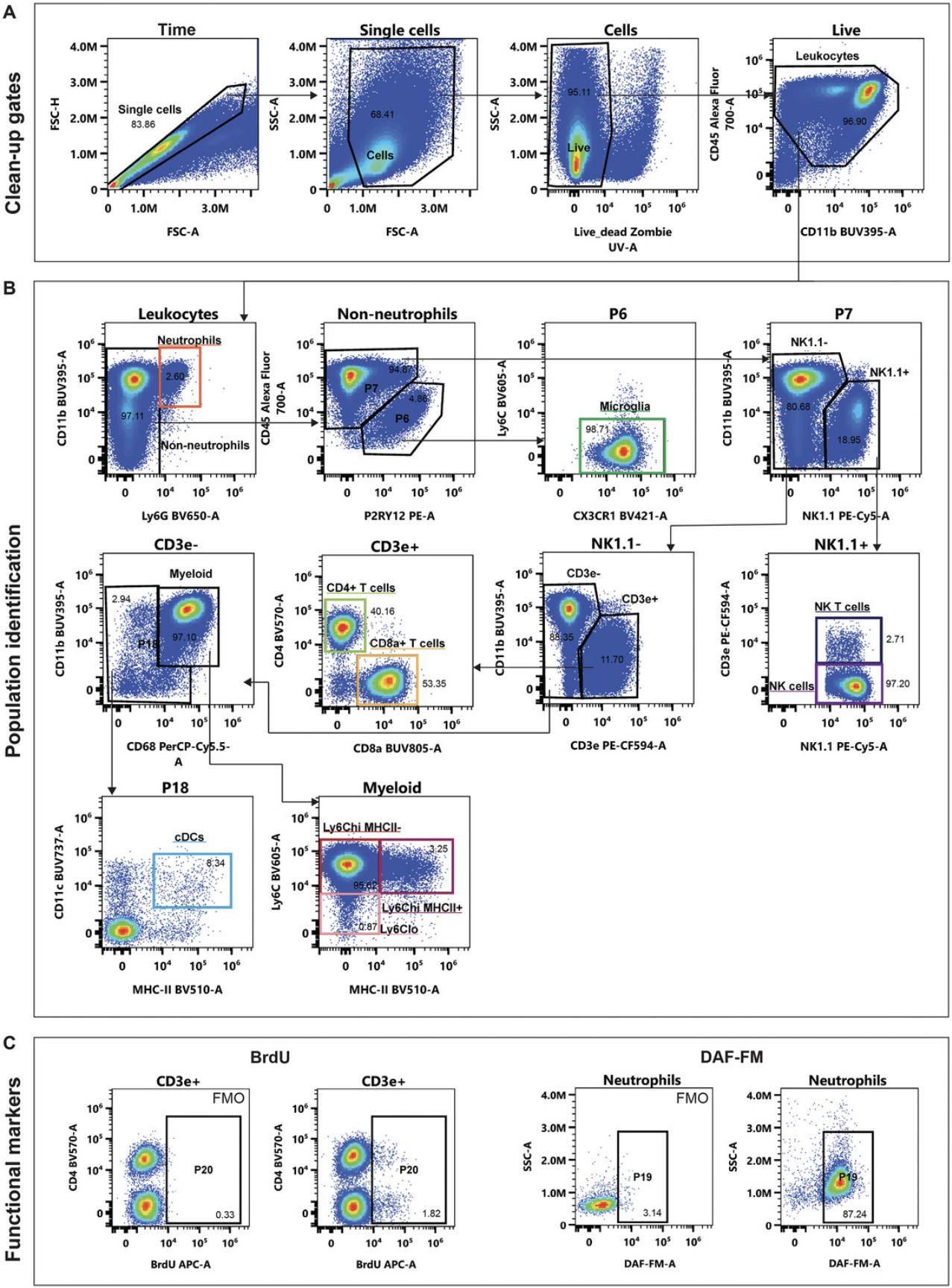
All reagents, including those for the controls (see Table 2), should be titrated and tested on the specific tissue in advance of experiments, taking into consideration that increasing the panel size can impact staining resolution and gating accuracy. For panel expansion, a significant portion of the spectrum between 400 and 730 nm is available for UV-excitable dyes, and there are a number of vacant positions from other laser modules. As brain cells are highly autofluorescent in the UV and violet channels, autofluorescence extraction can substantially improve staining resolution in these channels (Supporting Information 3 and 4). Extracting multiple autofluorescent populations from each unstained control per experimental group will further reduce unmixing errors (Supporting Information 3 and 4). Thus, we recommend spectrally unmixing multiple autofluorescence populations from group-specific unstained controls in Spectroflo Software (Fig. 4). Additionally, as only a limited number of cells can be isolated from the healthy brain, we recommend collecting one alternative tissue (spleen or bone marrow) to use for single-color reference controls (see Table 2). More detailed information about flow cytometry staining, acquisition, autofluorescence, staining indices, and spectral cytometry analysis has been extensively published elsewhere (Ashhurst et al., 2019, 2021; Brummelman et al., 2019; Ferrer-Font et al., 2021, 2023; Fox et al., 2020; Kharraz et al., 2022; Maciorowski et al., 2017; Mahnke & Roederer, 2007; Niewold et al., 2020; Nolan & Condello, 2013).
Control | Purpose |
---|---|
Unstained control from each treatment group |
Autofluorescence properties of cells can change with treatment. Thus, it is important to pool cells only from the same treatment group (e.g., with two groups, healthy vs. disease, pool only healthy with healthy samples and disease with disease samples; this also applies to cells from wild-type vs. knockout, treated vs. untreated, etc.). If all mice have been injected with a dye/fluorochrome, cells from a non-injected animal will need to be obtained. |
Viability control |
Any mixture of cells will be sufficient as long as they are unstained. If a sufficient number of brain cells is not available, consider taking spleen or bone marrow cells. Cells can be heat-shocked for 3 min at 60°C to obtain dead cells: divide the heat-shocked tube of cells in half and stain half with viability dye, while keeping the rest as a matched, unstained cell control. However, if using a tissue digest, you should obtain enough dead cells to begin with. |
Fluorescence minus one (FMO) |
Pool cells only from the same treatment group. If you are performing the protocols for the first time, make FMO controls for each treatment group because staining patterns may differ across groups, with potentially more or less background in the channel of interest, making it difficult to distinguish background from positive signal. This is vital for uncharacterized proteins and/or proteins with a tertiary staining pattern, i.e., a smear with no clear positive and negative population, but is also important for other markers to help identify unexpected antibody interactions that can occur in a multicolor cocktail. |
Positive controls | In the healthy brain, BrdU and DAF-FM will be very weakly detected. Therefore, we recommend processing a bone marrow or spleen to provide a positive single-color control for BrdU and DAF-FM staining. These can also serve as reference controls. However, brain samples from inflammatory conditions should be used for reference controls if they show a higher staining intensity for DAF-FM and BrdU compared to the spleen or bone marrow. |
Reference controls or single-color controls |
Use cell or bead controls, depending on which gives the best unmixing outcome. As only limited cells can be isolated from the brain, consider taking spleens for reference controls where cells provide the best unmixing outcome. See Table 3 for recommendations. However, this must be tested on user samples, as antigen expression will change with disease and treatment conditions. The following points are important for reference controls: (i) They must have the negative and positive populations well separated, while being as bright as, or brighter than, any staining observed in any samples; (ii) the negative and positive particles must have identical autofluorescence characteristics; (iii) the fluorescence spectrum of the reference control must be unique and identical to that of the multicolor sample (some fluorochromes may exhibit spectral shifting when bound to bead controls); and (iv) there must be sufficient events. Control optimization is discussed in detail elsewhere (Ferrer-Font et al., 2021). |
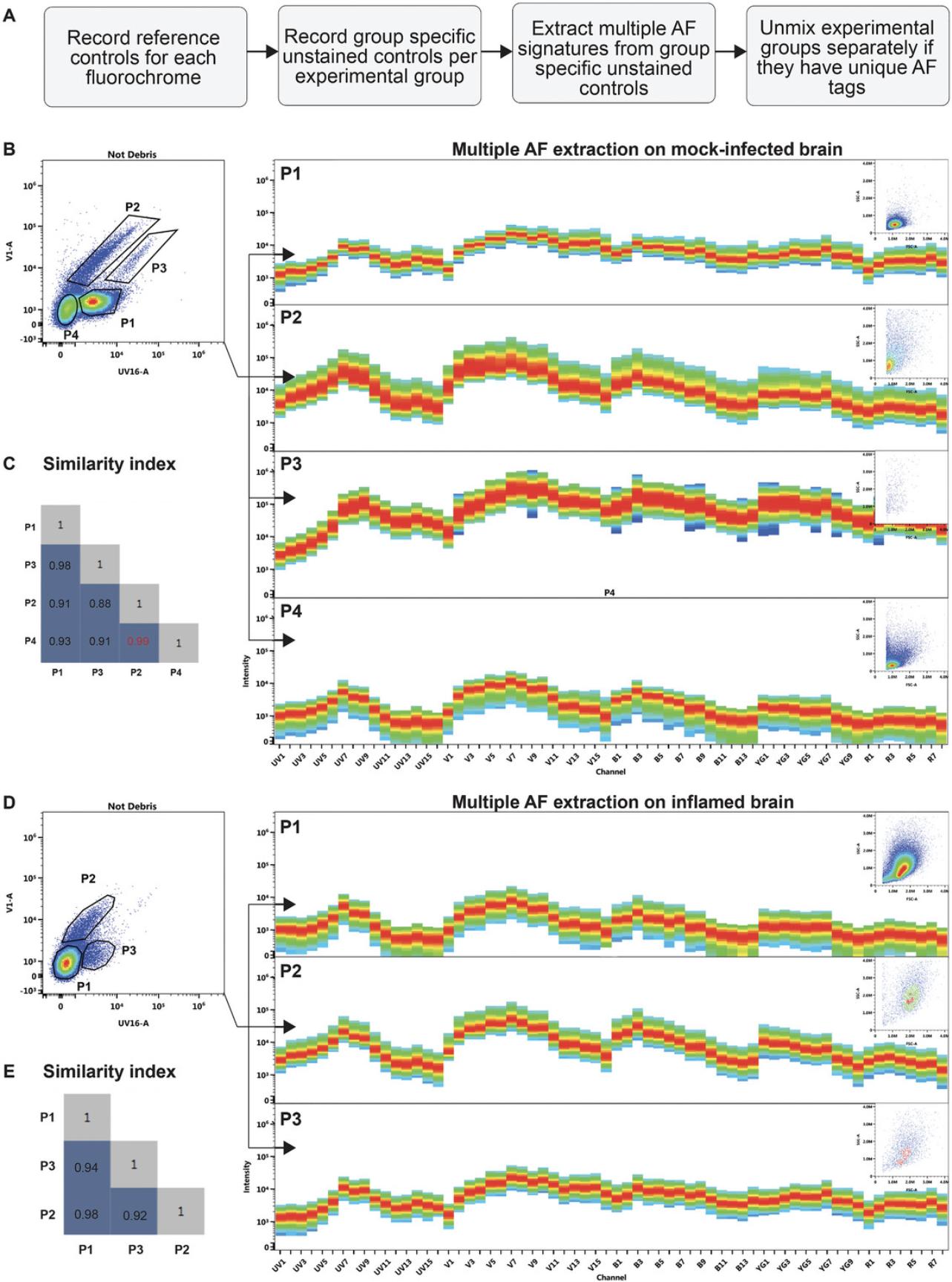
Materials
- Single-cell mouse brain samples and staining controls prepared in Basic Protocol 1 (see Table 2 for staining controls)
- PBS
- Viability/Fc blocking solution (see Table 3) or PBS with Fc block
- DAF-FM solution (see Table 3)
- Staining buffer (see recipe)
- Surface antigen stain mixture (see Table 3)
- UltraComp eBeads Compensation Beads (Thermo Fisher Scientific, cat. no. 01-2222-42), if using these as single-color reference controls
- BD Cytofix™ Fixation Buffer (BD Biosciences, cat. no. 554655) or 4% (w/v) paraformaldehyde (PFA) in PBS (see recipe)
- BD Cytofix/Cytoperm™ Fixation and Permeabilization Solution (BD Biosciences, cat. no. 554722)
- BD Perm/WashTM Buffer (BD Biosciences, cat. no. 554723)
- BD Cytoperm™ Permeabilization Buffer Plus (BD Biosciences, cat. no. 561651)
- 3 mg/ml (3×) DNase stock solution (see recipe)
Viability/Fc block solution | |||||||
Reagent | Marker | Clone | Company | Cat. no. | Mfr. conc. (mg/ml) | Dilution | Reference control type |
Fc block | N/A | 93 | BioLegend | 101302 | 0.5 | 100 | N/A |
Zombie UV™ a | Viability | N/A | BioLegend | 423108 | N/A | 500 | Spleen |
Defrost viability dye immediately before use and add to Fc block/PBS solution. Vortex, spin, and add 50 μl of solution per sample. | |||||||
DAF-FM staining solution | |||||||
Reagent | Marker | Clone | Company | Cat. no. | Mfr. conc. (mM) | Final conc. (μM) | Reference control |
DAF-FM stock | DAF-FM | N/A | Sigma Aldrich | D2321 | 5 | 0.5 | Spleen |
Defrost DAF-FM DMSO stock (see recipe) immediately before use and dissolve in PBS. Vortex, spin, and add 50 μl of solution per sample. | |||||||
Surface antigen stain mixture | |||||||
Fluorophore | Marker | Clone | Company | Cat. no. | Mfr. conc. (mg/ml) | Dilution | Reference control |
BUV395 | CD11b | M1/70 | BD Bioscience | 563553 | 0.2 | 400 | WNV-infected brainb |
BUV737 | CD11c | HL3 | BD Bioscience | 612796 | 0.2 | 100 | Spleen |
BUV805 | CD8α | 53-6.7 | BD Bioscience | 612898 | 0.2 | 300 | Spleen |
BV421 | CX3CR1 | SA011F11 | BioLegend | 149023 | 0.2 | 300 | Mock-infected brain |
BV510 | MHC-II | M5/114.15.2 | BioLegend | 107635 | 0.1 | 300 | Spleen |
BV570 | CD4 | RM4-5 | BioLegend | 100542 | 0.2 | 300 | Beads |
BV605 | Ly6C | HK1.4 | BioLegend | 128036 | 0.05 | 400 | WNV-infected brainb |
BV650 | Ly6G | 1A8 | BioLegend | 127641 | 0.2 | 200 | Beads |
BV711 | F4/80 | BM8 | BioLegend | 123147 | 0.2 | 200 | Beads |
PE | P2RY12 | S16007D | BioLegend | 848004 | 0.2 | 300 | Beads |
PE/CF594 | CD3ε | 145-2C11 | BD Bioscience | 562286 | 0.2 | 200 | Beads |
PE/Cy5 | NK1.1 | PK136 | BioLegend | 108716 | 0.2 | 300 | Beads |
PE/Cy7 | CD64 | X54-5/7.1 | BioLegend | 139314 | 0.2 | 500 | Beads |
AF700 | CD45 | 30-F11 | BioLegend | 103128 | 0.5 | 300 | Spleen |
APC/Cy7 | CD86 | GL-1 | BioLegend | 105030 | 0.2 | 100 | Beads |
Centrifuge antibodies for 5 min at 10,000 × g, 4°C (to sediment aggregates), before combining the required antibodies as a mixture in BD HorizonTM Brilliant Stain Buffer Plus (10 μl per sample). Top the remaining volume up with staining buffer (see recipe). Add 50 μl of solution per sample. | |||||||
Intranuclear and intracellular staining mixture | |||||||
Reagent | Marker | Clone | Company | Cat. no. | Mfr. conc. (mg/ml) | Dilution | Reference control |
BV785 | CD206 | C068C2 | BioLegend | 141729 | 0.2 | 200 | Beads |
PerCP/Cy5.5 | CD68 | FA-11 | BioLegend | 137010 | 0.2 | 300 | Spleen |
APC | BrdU | N/A | BD Bioscience | 51-23619L | 0.2 | 100 | Spleen |
Centrifuge antibodies for 5 min at 10,000 × g, 4°C (to sediment aggregates), before combining the required antibodies as a mixture in 1× BD Perm/WashTM Buffer. |
- a
Zombie UV Fixable Viability Kit (reconstitute as per manufacturer instructions and store at −30°C).
- b
Spleen cells can be used instead of WNV brain cells. The reference type will be user specific and depend on the specific experimental model being used, as single-color reference controls must be as bright or brighter than the fluorescence intensity observed in multicolor brain sample.
- Laminar-flow hood (BSC Class II or equivalent), if working with PC2 agents
- Centrifuge
- 10- and 200-µl pipets
- 50- and 200-µl multichannel pipets
- Aluminum foil
- 5- or 1.2-ml polypropylene tubes
- Falcon® Tube with Cell Strainer Cap (Corning, cat. no. 352235) or equivalent 35 μm nylon mesh
- 5-laser Cytek® Aurora (or equivalent)
- SpectroFlo® Software (or equivalent)
Preparation of controls (beginning after step 34 of Basic Protocol 1)
1.Take an aliquot of each brain sample prepared in Basic Protocol 1 and set aside for brain cell controls, including unstained brain cells, single-color reference controls, and fluorescence minus one (FMO) staining controls.
2.Distribute, label, and spin all samples and staining controls in tubes or a plate (whichever is preferred) for 5 min at 500 × g , 4°C.
Viability staining and blocking
3.Resuspend samples in 50 μl of viability/Fc blocking solution (see Table 3 for recipe) or 50 μl of PBS with Fc block (for single-color or unstained controls).
4.Incubate for 30 min at 4°C.
5.Top up with 150 μl PBS and spin 5 min at 500 × g , 4°C.
DAF-FM staining for nitric oxide production
6.Resuspend cells in 50 μl of DAF-FM DA solution (see Table 3 for recipe; for fully stained samples and single-color DAF-FM control) or PBS (for unstained and viability controls and DAF-FM FMO).
7.Incubate for 30 min at 4°C.
8.Top up with 150 μl PBS.
9.Spin 5 min at 500 × g , 4°C.
10.Resuspend cells in 250 μl PBS.
11.Incubate for 15 min at 4°C.
12.Spin 5 min at 500 × g , 4°C.
13.Vortex compensation beads and aliquot into tubes or plate for single-color reference controls.
Surface staining
14.Flick off supernatant and resuspend samples in 50 μl surface antigen stain mixture (see Table 3 for recipe; for fully stained samples), staining buffer (for unstained controls and single-color controls), and FMO mixtures (for FMO controls).
15.Incubate for 30 min at 4°C.
16.Top wells up with 150 μl staining buffer and spin 5 min at 500 × g , 4°C.
17.Wash twice with 250 μl staining buffer.
Fixation
18.Fix cells in 50 μl of either BD Cytofix™ Fixation Buffer or 4% PFA in PBS for 30 min at room temperature.
19.Top up with 150 μl staining buffer.
20.Spin 3 min at 800 × g , 4°C.
21.Resuspend cells in 200 μl staining buffer.
Next day: Fixation and permeabilization for BrdU detection and intracellular staining
22.Spin 5 min at 800 × g , 4°C.
23.Resuspend cells in 50 μl of BD Cytofix/Cytoperm™ buffer.
24.Incubate for 20 min at room temperature in the dark.
25.Top up with 150 μl of 1× BD Perm/WashTM buffer.
26.Spin 3 min at 800 × g , 4°C.
Permeabilization for BrdU detection
27.Resuspend cells in 100 μl of BD Cytoperm™ Permeabilization Buffer Plus.
28.Cover samples with aluminum foil and incubate for 10 min on ice.
29.Top up with 150 μl of 1× BD Perm/WashTM buffer.
30.Spin 3 min at 800 × g , 4°C.
Re-fixation
31.Resuspend cells in 50 μl of BD Cytofix/Cytoperm™ buffer.
32.Incubate for 5 min at room temperature in the dark.
33.Top up with 150 μl of 1× BD Perm/WashTM buffer.
34.Spin 3 min at 800 × g , room temperature.
DNase digestion
35.Defrost 3 mg/ml DNase stock solution at room temperature and add PBS to make a 1 mg/ml solution.
36.Resuspend all samples in 100 μl DNase solution.
37.Incubate for 1 hr at 37°C.
38.Top up with 150 μl of 1× BD Perm/WashTM buffer.
39.Spin 3 min 800 × g , room temperature.
Intracellular staining
40.Resuspend cells in 50 μl of intracellular antibody mixture (as per Table 3).
41.Incubate for 45 min at room temperature in the dark.
42.Top up with 150 μl of 1× BD Perm/WashTM buffer.
43.Spin 3 min at 800 × g , room temperature.
44.Resuspend samples in 250 μl of 1× BD Perm/WashTM buffer.
45.Spin 3 min at 800 × g , room temperature.
46.Resuspend samples in 250 μl of 1× BD Perm/WashTM buffer.
47.Spin 3 min at 800 × g , room temperature.
48.Resuspend samples in 150 μl of staining buffer.
Analysis of samples on the spectral cytometer
NOTE: This protocol was developed on a 5-laser Cytek® Aurora; as such, this portion of the method is explicitly described for this instrument and software. If using a different analyzer, use the manufacturer-recommended process for setting up the experiment and optimizing the instrument settings; the controls required will remain the same.
49.Set up experiment in SpectroFlo® by adding fluorescent tags, samples, groups, and marker names in the “Edit experiment” portal. Use CytekAssaySettings for fluorescence detector gains (this does not require alteration if recommended antibody concentrations are used) and optimize FSC and SSC settings as required.
50.Add group-specific unstained reference controls for each treatment group in Spectroflo under the “Groups” in the “Edit experiment” portal. Right click the group folder and select “Unstained control.” This is to ensure that you are unmixing with matched autofluorescence properties.
51.Add 50 μl staining buffer to a sample from each treatment group and run at a low flow rate to examine signal intensity to ensure that everything is on scale.
52.Record reference controls, and all unstained cell controls.
53.Spectrally unmix by extracting multiple autofluorescent populations from each group-specific unstained controls (see Fig. 4). Right click the group-specific unstained control and follow prompts in SpectroFlo® to identify the unique autofluorescent populations.
54.Create an unmixed workspace for each experimental group.
55.Record fully stained samples in the appropriate unmixed workspace.
Basic Protocol 3: FLOW CYTOMETRIC SORTING OF MOUSE MICROGLIA FOR EX VIVO ANALYSIS
Isolating microglia is required for downstream assays requiring a pure population, including bulk RNA and scRNA analysis, proteomics, lipidomics, and functional assays. In this protocol, brain leukocytes are enriched using Basic Protocol 1, i.e., stained with surface markers, and sorted on the 7-laser BD InfluxTM cell sorter. It is imperative to work efficiently and continuously (without interruption) to enhance yield and viability of cells. The viability of microglia also substantially decreases after cell sorting; thus, samples must be kept on ice in FBS. Due to the limited number of microglia that can be sorted from a brain, we recommend pooling samples before staining (see Critical Parameters and Troubleshooting for further recommendations). From a single mouse brain, expect to sort ∼8 × 104 according to instrument sort count. However, after spinning and counting cells with trypan blue, this usually equates to ∼2.5 × 104 viable cells.
Other microglial isolation processes, including magnetic separation and immunopanning, have also been described (Bohlen et al., 2019). Although these may be effective for the healthy brain, we do not recommend them for the inflamed brain, as microglia and infiltrating myeloid cells have overlapping expression of many proteins. Thus, it is important to assess the differential expression profiles via flow cytometry to accurately gate each population (see Fig. 3 for gating strategy).
We recommend sorting cells directly into the reagents required for the next steps to avoid cell loss from centrifuging and resuspending cells. For instance, for RNA extraction and lipidomics, we recommend sorting cells directly into TRIzolTM or methanol, respectively. We do not recommend culturing microglia after cell sorting, particularly from adult mice. As well as downregulating genes that inform their in vivo state, too few viable cells will remain after culturing. Previously published protocols recommend using juvenile rats for culturing sorted microglia (Bohlen et al., 2019).
If performing scRNA cell capture after sorting, we recommend barcoding microglia and monocyte populations with separate sample tags to ensure that these populations can be accurately identified during analysis. This is because microglia can downregulate microglia-specific genes that clearly distinguish them from non-resident myeloid cells during inflammation, significantly confounding cell type identification (Spiteri, Wishart et al., 2023). Although other scRNA cell capture systems are available, we recommend using the BD RhapsodyTM system and provide modifications to manufacturer protocols prior to cell capture to deal with the low cell numbers obtained from mouse brains.
Materials
- Viability/Fc blocking solution (see Table 3)
- PBS (see recipe)
- Fc block
- UltraComp eBeads Compensation Beads (Thermo Fisher Scientific, cat. no. 01-2222-42), if using these as single-color reference controls
- Staining buffer (see recipe)
- Surface antigen stain mixture (see Table 4)
- Fetal bovine serum (FBS), 4°C
- BD Mouse Immune Single-Cell Multiplexing Kit (BD Bioscience, cat. no. 633793)
- Trypan blue
- Calcein AM (Thermo Fisher Scientific, cat. no. C1430)
- Draq7™ (BD Bioscience, cat. no. 564904)
- BD Rhapsody™ Cartridge Reagent Kit (BD Bioscience, cat. no. 633731)
Surface antigen stain | ||||||
---|---|---|---|---|---|---|
Fluorophore | Marker | Clone | Company | Cat. no. | Mfr. conc. (mg/ml) | Dilution |
BUV395 | CD11b | M1/70 | BD Bioscience | 563553 | 0.2 | 400 |
BV421 | CX3CR1 | SA011F11 | BioLegend | 149023 | 0.2 | 300 |
BV605 | Ly6C | HK1.4 | BioLegend | 128036 | 0.05 | 400 |
BV650 | Ly6G | 1A8 | BioLegend | 127641 | 0.2 | 200 |
PE | P2RY12 | S16007D | BioLegend | 848004 | 0.2 | 300 |
PE/Cy5 | CD3ε | 145-2C11 | BioLegend | 100310 | 0.2 | 200 |
NK1.1 | PK136 | BioLegend | 108716 | 0.2 | 300 | |
PE/Cy7 | CD64 | X54-5/7.1 | BioLegend | 139314 | 0.2 | 500 |
AF700 | CD45 | 30-F11 | BioLegend | 103128 | 0.5 | 300 |
Centrifuge antibodies for 5 min at 10,000 × g, 4°C (to sediment aggregates), before combining the required antibodies as a mixture in BD HorizonTM Brilliant Stain Buffer Plus (10 μl per sample). Top the remaining volume up with staining buffer (see recipe). Add 50 μl of solution per sample. |
- Falcon® Tube with Cell Strainer Cap (Corning, cat. no. 352235) or equivalent 35-μm nylon mesh
- 5-ml sort tubes: Round-bottom polypropylene tubes (Corning cat. no. 352063)
- 7-laser BD InfluxTM cell sorter (or equivalent)
- INCYTO™ disposable hemocytometer (INCYTO, cat. no. DHC-N01-5)
- BD Rhapsody™ Cartridge Kit (BD Bioscience, cat. no. 633733)
- BD Rhapsody™ Scanner
Isolation of microglia
1.Perform steps 4-31 of Basic Protocol 1.
2.Take an aliquot of each sample for staining controls (i.e., unstained brain cells and FMO controls; see Table 2).
Viability staining and blocking
3.Resuspend samples in 50 μl of viability/Fc blocking solution (see Table 3 for recipe) or 50 μl of Fc block in PBS (dilution 1/100; for single-color or unstained controls).
4.Incubate 30 min at 4°C.
5.Top up with 150 μl PBS.
6.Spin 5 min at 500 × g , 4°C.
7.If using compensation beads as single-color reference controls, vortex beads and distribute the relevant aliquots into tubes or plate.
Surface staining
8.Flick off supernatant and resuspend samples in 50 μl surface antigen stain mixture (for fully stained samples), staining buffer (for unstained controls and single color controls), or FMO mixtures (for FMO controls).
9.Incubate for 30 min at 4°C.
10.Top wells up with 150 μl staining buffer.
11.Spin 5 min at 500 × g , 4°C.
12.Resuspend cells in 250 μl staining buffer.
13.Spin 5 min at 500 × g , 4°C.
14.Resuspend cells in 250 μl staining buffer.
15.Filter samples into a 5-ml sort tube through nylon mesh or Falcon® Tube with Cell Strainer Cap.
16.Add 250 μl staining buffer to well plate or tube and transfer remaining cells into 5-ml sort tubes through nylon mesh or strainer cap.
17.Spin sort tubes 5 min at 500 × g , 4°C.
18.Resuspend cells in 250 μl staining buffer.
19.Set up cell sorter instrument, record compensation controls, compensate, and set up gating strategy.
20.Collect sorted cells in 500 μl 4°C 100% FBS.
21.Place samples on ice.
Single-cell barcoding with single-cell multiplexing kit
If performing scRNA-seq analysis using the BD RhapsodyTM System : Note that the manufacturer's protocol for single-cell multiplexing and staining with viability dyes (steps before cell capture) has been altered to solve the problem of the low cell numbers obtained from brain samples. Please refer to manufacturer documents for further details (Single Cell Labelling with the BD™ Single-Cell Multiplexing Kit and Single Cell Capture and cDNA Synthesis with the BD Rhapsody™ Single-Cell Analysis System).
22.Briefly spin sample tag tubes from BD Mouse Immune Single-Cell Multiplexing Kit.
23.Resuspend sample tags in 180 μl staining buffer.
24.Top up tubes containing sorted cells with 1 ml PBS to dilute FBS, and spin tubes 5 min at 500 × g , 4°C.
25.Aspirate supernatant carefully with a pipet or a vacuum on low.
26.Resuspend cells in 0.5 volume of sample tag buffer (∼90 μl) and transfer cells to a 96-well plate. Add the rest of the sample tag buffer (∼90 μl) to wash and transfer the remaining cells out of the tube and into the well plate.
27.Incubate at room temperature for 20 min.
28.Top up with 150 μl staining buffer.
29.Spin samples 5 min at 500 × g , 4°C.
30.Top up with 250 μl staining buffer.
31.Spin samples 5 min at 500 × g , 4°C.
32.Resuspend cells in 110 μl staining buffer.
33.Take 10 μl from each sample and perform a trypan blue count.
34.Pool cells into a single tube in the desired ratio with an excess of the required cells.
Staining cells with viability markers
35.Add Calcein AM (final concentration 10 μM) and Draq7 (final concentration 1.5 μM) to pooled cells and mix with a micropipet.
36.Incubate in the dark for 5 min at 37°C.
37.Filter samples with nylon mesh or Falcon® Tube with Cell Strainer Cap.
38.Count cells using the Rhapsody scanner by pipetting 10 μl of sample into an INCYTO disposable hemocytometer.
39.Dilute or concentrate pooled cells for cartridge loading.
40.Proceed with manufacturer's instructions for cell capture. Details of sample processing for sequencing can be found in Spiteri, Wishart et al. (2023).
Basic Protocol 4: PROCESSING THE MOUSE BRAIN FOR IMAGING MASS CYTOMETRY FOR SPATIAL MICROGLIA ANALYSIS
The protocols described above involve dissociating the mouse brain into single-cell suspensions, disrupting the tissue microenvironment, and selecting mostly for leukocytes. After CNS perturbation, microglia undergo morphological alterations, including the adoption of a hypertrophied cell soma and shortened and thickened cytoplasmic projections. In addition, these cells can migrate and interact with other cells. Analysis of cell-type-specific interactions, co-localization of markers, and morphological changes, as well as regional analysis from tissue sections, can provide information on additional functional processes that cannot be determined from a single-cell suspension. Thus, below we describe methods to analyze the brain histologically.
The quality of a histological image in a tissue section is dependent on the preservation of cellular morphology, whereas the quality of immunohistochemical labeling depends on the retained or retrievable antigenicity of a tissue section. Satisfactory preservation of cellular morphology can be achieved by fixing tissues in formalin and embedding them in paraffin wax. However, this can significantly challenge the ability to visualize >40 epitopes simultaneously at imaging mass cytometry because this requires antigen-retrieval methods to be optimal for all markers (Ijsselsteijn et al., 2019). Thus, here we suggest freezing tissue to avoid the need for antigen-retrieval processes. We have performed a series of experiments to optimize preparation and fixation conditions on cryosections obtained from frozen mouse brains. The conditions included:
- 1.The use of PBS versus 2% versus 4% PFA perfused transcardially prior to tissue harvest.
- 2.The use of cryoprotection (10%, 20%, or 30% sucrose in PBS).
- 3.The use of a post-fixative (methanol, acetone, or 4% PFA) after cryosectioning.
- 4.Post-fixation of tissue in methanol/acetone/4% PFA immediately after cutting sections and/or after storing slides in the freezer before staining.
Overall, we found that 2% or 4% PFA perfusion with cryopreservation and section fixation with methanol results in the best morphology and antigenicity compared to all other conditions, although high-quality images can also be obtained without PFA perfusion or overnight fixation but with cryopreservation. The use of cryoprotection substantially increased the tissue quality, reducing the occurrence of holes or a “Swiss cheese” appearance in the brain tissue, as well as preserving the integrity of the cell nuclei. Thus, in instances where other assays need to be performed on the same animal, PFA perfusion can be omitted.
In this protocol, tissue sections are stained with fluorochrome-conjugated antibodies and subsequently labeled with metal-conjugated antibodies (Fig. 5). As such, sections can initially be imaged on a fluorescence microscope to identify regions of interest, before assessment by imaging mass cytometry. The panel of metal-conjugated antibodies described below is simple and intended to be expanded for inclusion of user-specific markers (Table 5).
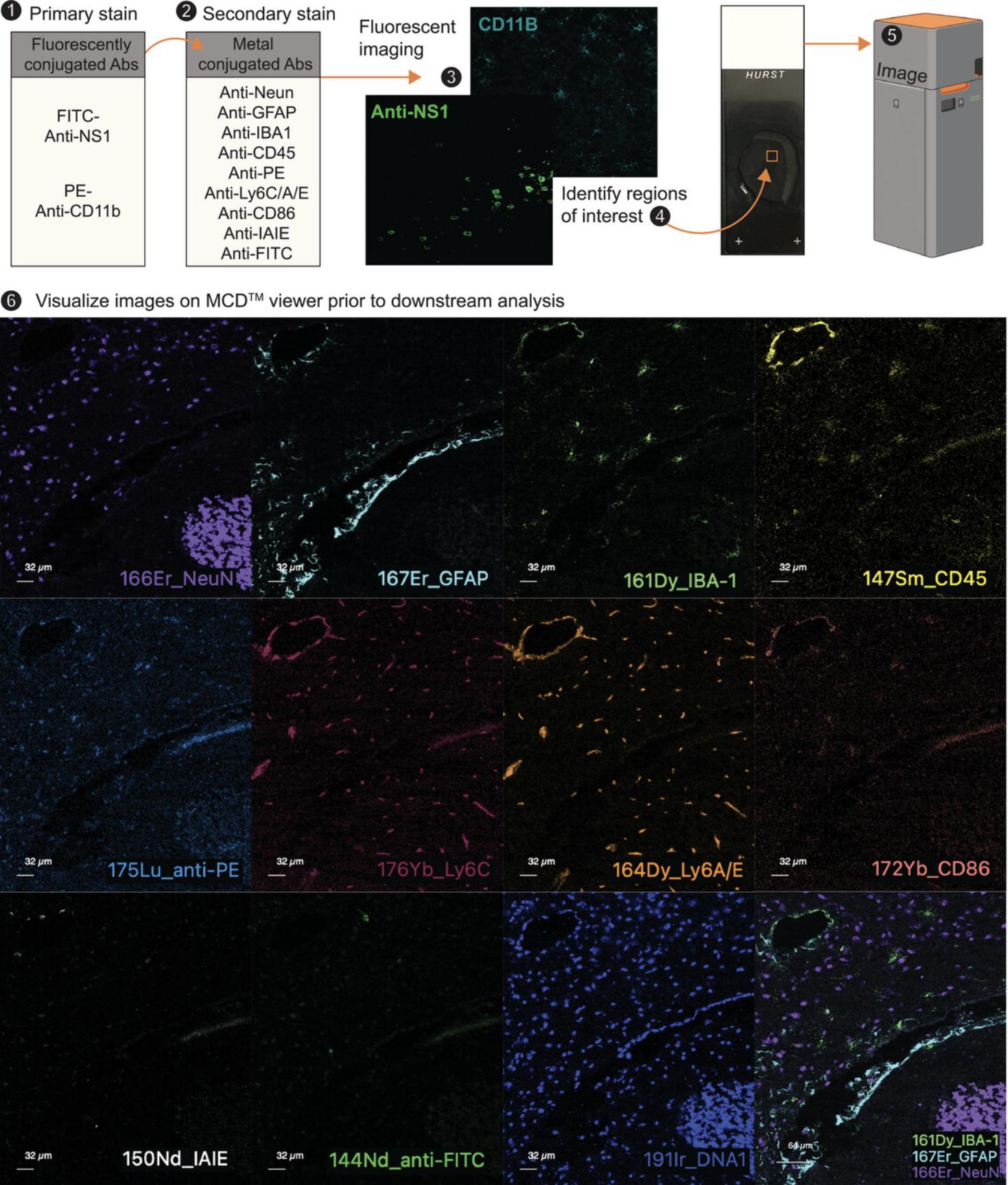
Primary fluorescent antibody mixture | ||||||
Fluorophore | Marker | Clone | Company | Cat. no. | Mfr. conc. (mg/ml) | Dilution |
PE | CD11b | M1/70 | BioLegend | 101208 | 0.2 | 200 |
FITCa | NS1a | N/A | N/A | N/A | N/A | 100 |
Centrifuge antibodies for 5 min at 10,000 × g, 4°C (to sediment aggregates), before preparing aliquots of these in blocking buffer (see recipe). Add 150 μl of solution per tissue section. | ||||||
Metal-conjugated antibody mixtureb | ||||||
Metal | Target | Clone | Company | Cat. no. | Mfr. conc. (mg/ml) | Amount per test (µl) |
144Nd | FITC | FIT-22 | BioLegend | 408302 | 0.5 | 0.5 |
150Nd | MHC-II | M5/114.15.2 | BD Bioscience | 556999 | 0.5 | 0.5 |
153Eu | MAP2 | SMI 52 | BioLegend | 801801 | 1 | 2 |
147Sm | CD45 | 30-F11 | BD Bioscience | 553076 | 0.5 | 0.5 |
161Dy | IBA-1 | EPR16588 | Abcam | AB178846 | 1.27 | 2 |
164Dy | Ly6A/E | D7 | BioLegend | 108102 | 0.5 | 0.5 |
166Er | NeuN | 1B7 | BioLegend | 834501 | 1 | 2 |
167Er | GFAP | 2E1.E9 | BioLegend | 644702 | 0.5 | 1 |
172Yb | CD86 | GL1 | BD Bioscience | 553689 | 0.5 | 1 |
175Lu | Anti-PE | PE001 | BioLegend | 408102 | 0.5 | 1.3 |
176Yb | Ly6C | HK1.4 | BioLegend | 128002 | 0.5 | 0.5 |
Centrifuge antibodies for 5 min at 10,000 × g, 4°C (to sediment aggregates), before combining the required antibodies in blocking buffer (see recipe). Add 150 μl of solution per sample. |
- a
Primary antibodies will be user specific. In this instance, NS1 was used to detect WNV in infected mice.
- b
Sydney Cytometry core facility conjugated antibodies to metal tags and titrated these as part of the Ramaciotti reagent bank service.
Materials
-
Mice
-
PBS, ice cold
-
4% (w/v) PFA in PBS (see recipe), ice cold
-
10%, 20%, and 30% (w/v) sucrose solutions (see recipe), ice cold
-
Liquid nitrogen
-
Hexane (Sigma Aldrich, cat. no. 270504-2L)
-
O.C.T. compound (Tissue-Tek, cat. no. IA018)
-
Methanol, prechilled to −30°C
-
Tris-buffered saline (TBS) solution (see recipe)
-
TBS/0.05% Tween-20 (TBST) solution (see recipe)
-
Blocking buffer: TBST (see recipe) with 10% fetal bovine serum (FBS)
-
Primary staining solution (see Table 5)
-
Secondary staining solution (see Table 5)
-
ProLong Gold Antifade Mountant with DAPI (Thermo Fisher Scientific, cat. no. P36931)
-
Forceps and dissection tools
-
5-ml tubes (1 per brain, for collection)
-
50-ml Falcon tube (3 per brain for each sucrose gradient)
-
Personal protective equipment for procedures
-
Liquid nitrogen canister
-
Cryomolds (mold with a well dimension of 25 × 25 × 5 mm for 2 brain sagittal sections and 17 × 17 × 5 mm for 1 sagittal section; Hurst Scientific, cat. no. 31052525 and 31051717)
-
Aluminum foil
-
Dry ice
-
Cryostat
-
Cryostat blades
-
Positively charged microscope slides
-
Slide rack
-
Hydrophobic pen (Sigma Aldrich, cat. no. Z377821-1EA)
-
Microscope (Olympus BX-51 microscope or equivalent)
-
Dark box
-
Cell-IDTM Iridium DNA intercalator (Standard BioTools, cat. no. 201192A)
-
Hyperion imaging system (Standard BioTools)
Isolating and fixing tissue
1.Anesthetize mice and then transcardially perfuse each mouse with 10 ml ice-cold PBS.
2.Perfuse mice with ice-cold 4% PFA in PBS.
3.Isolate brain as described in Basic Protocol 1 (steps 5-10).
4.Cut the brain sagittally in the median plane, and place both halves in ice-cold 4% PFA in a 5-ml tube. Leave at 4°C overnight.
Next day: Cryopreservation
5.Remove any excess 4% PFA by dabbing the brain tissue on a paper towel and transfer it into 15 ml ice-cold 10% sucrose solution.
6.Allow the tissue to sink to the bottom of the tube before transferring it into 15-ml ice-cold 20% sucrose solution.
7.Repeat step 6, but transfer the brain into 15 ml ice-cold 30% sucrose solution.
Next day: Snap-freezing tissue
8.Fill the metal canister with liquid nitrogen.
9.Half fill a plastic beaker with hexane and place in liquid nitrogen. Allow time for hexane to equilibrate to liquid nitrogen temperature (∼4 min).
10.Spread O.C.T. medium in a cryomold.
11.Blot tissue on a paper towel to remove excess liquid before placing it in the center of the cryomold containing O.C.T.
12.Using forceps at room temperature, grasp the cryomold and plunge it into the hexane. Once the block is completely white, remove it.
13.Remove the frozen tissue block from the cryomold, wrap the tissue in foil, and place on dry ice.
14.Store tissue in a −80°C freezer. The tissue should not lose quality for at least 6 months at this temperature.
Sectioning
15.Attach the tissue block to the cryostat chuck by adding a small amount of O.C.T. to the chuck and placing the tissue on top.
16.Place tissue block under the weights on cryobar and allow it to equilibrate to chamber temperature (−30°C; 15 min).
17.Attach the chuck to the cutting head and blade to the blade holder.
18.Section brains in cryostat at 8-10 μm (−16°C tissue head, −30°C cryobar), placing sections on the positively charged slides.
19.Allow the sections to air-dry for 30 min at room temperature.
20.Store sections on the slides in the −80°C freezer or proceed with step 21.
Staining for immunohistochemistry
21.Place the slides containing the tissue sections in cold methanol for 10 min in –30°C freezer.
22.Rinse tissue slides in TBST three times for 3 min per wash.
23.Wipe any excess liquid from slide and around tissue section.
24.Circle the tissue section on the slide with a hydrophobic marker.
25.Add 150 μl blocking buffer within the hydrophobic circle to cover the enclosed section and let it sit for 30 min at room temperature.
26.Shake off excess solution.
27.Add 150 μl of primary fluorescent antibody mixture and let sit for 1 hr at room temperature (Table 5).
28.Rinse in TBST three times for 3 min per wash.
29.Coverslip the section using DAPI antifade mounting medium.
30.Check the slide under microscope—for best images, take micrographs on the day of staining.
31.Store slides in the refrigerator in a dark box.
Staining for IMC
32.Proceed from step 28 if you are using fluorescently conjugated antibodies to define regions of interest for IMC acquisition.
33.Check slides under fluorescence microscope to ensure staining is visible.
34.Add 150 μl of secondary metal-tagged antibody mixture (Table 5) and let sit for 1 hr at room temperature.
35.Rinse in TBST three times for 3 min per each wash.
36.Add 150 µl of iridium DNA intercalator (1/2000) in TBST and let sit for 30 min at room temperature.
37.Gently dip tissue slides in Milli-Q water. Repeat.
38.Examine slides under a fluorescence microscope to determine regions of interest.
39.Air dry slide for 20 min at room temperature.
40.Image on the Hyperion or store in a sealed container at −20°C or room temperature for up to a month.
REAGENTS AND SOLUTIONS
BrdU stock solution, 2× (10 mg/ml)
Dissolve 250 mg 5-bromo-2′-deoxyuridine (BrdU) powder (Sigma Aldrich, cat. no. B5002-250MG) in 25 ml phosphate-buffered saline (PBS). Filter sterilize and prepare 0.5-ml aliquots in 1.5-ml tubes. Store up to 12 months at −80°C.
DNase and collagenase stock, 10×
- Dissolve in 50 ml PBS :
- 0.05 g DNase I (Sigma Aldrich, cat. no. DN25)
- 0.5 g collagenase, type IV, from Clostridium histolyticum (Sigma Aldrich, cat. no. C5138)
- Filter sterilize and prepare 5-ml aliquots in 5-ml tubes
- Store up to 12 months at −30°C
DNase stock solution, 3× (3 mg/ml)
Dissolve 75 g DNase I powder (Sigma Aldrich, cat. no. DN25; 684.69 U/mg) in 25 ml PBS. Prepare 0.5-ml aliquots in 1.5-ml tubes. Store up to 12 months at −80°C.
DAF-FM DA stock solution
5 mM DAF-FM DA solution in DMSO (Sigma Aldrich, cat. no. D2321). Prepare aliquots. Prepare 5-µl aliquots in 0.2-ml tubes. Store up to 12 months at −30°C.
Paraformaldehyde (PFA) in PBS, 4% (w/v; phosphate-buffered paraformaldehyde)
Weigh out 8 g paraformaldehyde (Sigma Aldrich, cat. no. P6148) in a fume hood. Using a hotplate with integrated magnetic stirrer, combine paraformaldehyde and 100 ml Milli-Q water with a stirring bar and heat to 60°C. Add small pellets of sodium hydroxide (NaOH; Sigma Aldrich, cat. no. 221465), allowing each to dissolve before adding another, until solution clears. Alternatively, using a pipet, add 1 N NaOH dropwise until the solution clears. Cool the mixture and then combine with 100 ml 2× PBS, pH 7.4. Return the pH to 6.9 if necessary, using HCl added dropwise with a pipet. Store up to 2 weeks at 4°C or up to 12 months at −30°C.
Percoll solution, 26%
- 78.9 ml Percoll (Cytiva, cat. no. 17-0891-01)
- 30 ml 1.5 M NaCl (Sigma Aldrich, cat. no. 746398)
- 191.1 ml DMEM (Lonza Australia Pty Ltd, cat. no. 12-604F)
- Keep sterile
- Store up to 12 months at 4°C
Makes 300 ml, sufficient for 42 brains.
To prepare 1.5 M NaCl, dissolve 87.66 g NaCl in 1 L of Milli-Q water, filter, and keep sterile.
Percoll solution, 73%
- 73.1 ml Percoll (Cytiva, cat. no. 17-0891-01)
- 10 ml 1.5 M NaCl
- 16.9 ml Milli-Q water
- Keep sterile
- Store up to 12 months at 4°C
Makes 100 ml, sufficient for 33 brains.
To prepare 1.5 M NaCl, dissolve 87.66 g NaCl in 1 L of Milli-Q water, filter, and keep sterile.
Phosphate-buffered saline solution (PBS)
- Combine 48 g Dulbecco's Phosphate Buffered-Saline (Sigma Aldrich, cat. no. D5652) with 5 liters Milli-Q water, mix a magnetic stirrer, and filter sterilize. Store up to 12 months at room temperature in 1-liter bottles.
Staining buffer
- 100 ml EDTA (ChemSupply, cat. no. EL026-2.5l-P)
- 50 ml fetal bovine serum
- 850 ml PBS
Sucrose solutions, 10%/20%/30%
- 10, 20, or 30 g sucrose (Sigma Aldrich, cat. no. S0389)
- 100 ml PBS
TBST (1× TBS with 0.05% Tween 20)
- 100 ml 10× TBS (see recipe)
- 900 ml Milli-Q water
- 500 μl Tween 20 (Sigma Aldrich, cat. no. P1379)
Tris-buffered saline (TBS), 10×
- 13.9 g Trizma® Base (Sigma Aldrich, cat. no. T1503)
- 60.6 g Trizma® hydrochloride (Sigma Aldrich, cat. no. T3253)
- 60.6 g NaCl
- Make up to 1 L with Milli-Q water
Store up to 12 months at 4°C, and dilute to 1× on the day of staining.
COMMENTARY
Background Information
To elucidate the functions of cells in a disease setting, it is important to examine them using multiple modalities. This is because protein and RNA expression do not always correlate, and analysis of morphological data alone does not necessarily reveal precise cellular functions. We hope that the protocols detailed here will serve as a starting point for future experiments to enhance the understanding of microglial biology in disease.
The phenotypic convergence of microglia and monocyte-derived cells in CNS disease (Getts et al., 2008) has historically impeded the ability to accurately discriminate these populations for functional analysis. Advances in cytometry and computational analysis platforms have better elucidated the identities of these cell types (Ashhurst et al., 2021). This has enabled the development of more accurate gating strategies to phenotype and sort microglia and infiltrating myeloid cells (Spiteri, Wishart et al., 2023).
Advances in cell culture protocols that maintain microglial survival, viability, and in vivo status post isolation will provide powerful tools to address more mechanistic questions. Until then, other techniques not described here, including intravital imaging, spatial molecular imaging to transcriptionally profile cells in situ , and the use of transgenic mice or tissue culture, should also be explored to investigate specific aspects of microglia in disease.
Critical Parameters
Basic Protocol 1
Tissue processing preparation
To work as quickly as possible to prevent tissue degradation, all processing reagents and tubes should be prepared in advance of experiments. This includes preparing the 10× digestion cocktail, Percoll solutions, staining buffer, and PBS. Reagents should be prepared in separate aliquots in bottles/tubes to keep remaining stocks sterile. For every brain, label one C tube and two 15-ml tubes. The day before the experiment, pipet 4.5 ml of PBS into the C tubes and 5 ml of staining buffer into one 15-ml tube. Store these in the refrigerator at 4°C. Leave the other 15-ml tube at room temperature. On the morning of animal euthanasia, remove Percoll solutions from the refrigerator and set one centrifuge to 23°C and another to 4°C. Percoll gradient centrifugation is best performed at room temperature. The 10× enzyme digestion stock should also be thawed and left on ice until use.
Tissue processing
Excepting the Percoll separation centrifugation at room temperature, ensure that tissue and cells are kept on ice during all other processing steps.
Cell counts
It is important to remeasure the volume of staining buffer in which the brain cells are resuspended to get an accurate cell count. Residual volume can affect the final volume and confound cell counts, which are critical for data interpretation.
Basic Protocol 2
Titrating antibodies and optimizing reference controls
Antibodies should be titrated on the exact tissue of interest (including treatment or infection) before the experiment. The cell types infiltrating the brain and their expression profiles will determine the concentration of antibodies required as well as the type of reference control to use (i.e., cells or beads). The age and lot of an antibody may also impact antibody titrations. Although the dilution factors provided here can be used as a starting point, we recommend re-titrating your antibodies on the exact tissue you intend to analyze to achieve saturation labeling, as this enables more accurate quantitative evaluation of antigen expression. Best practices for antibody titration can be found in a number of publications (Brummelman et al., 2019; Ferrer-Font et al., 2021; Maciorowski et al., 2017; Mahnke & Roederer, 2007). As well as titrating antibodies, it is important to determine the type of reference controls to use (i.e., cells or beads) to get the best unmixing result. If you are working on the homeostatic brain and have very limited numbers of cells, consider processing extra brain tissue, collecting alternative tissue(s), or storing good-quality reference controls in reference libraries in the SpectroFlo® Software for use in unmixing future experiments.
Staining mixtures
Prepare staining solutions the day before. For viability/Fc block mixture, prepare PBS and Fc block the day before and add viability dye immediately before staining. To minimize antibody aggregates in premade cocktails, on the day of staining, spin the cocktail staining solution for 5 min at 10,000 rcf and be sure to retrieve the solution from the supernatant carefully, avoiding the bottom of the vial where aggregates could have pelleted.
Staining controls
Accurate assessment of cytometry data requires high-quality controls. Each time a new panel is to be implemented, it is vital to optimize the controls under the specific experimental conditions to be used. If you have not profiled cells from each experimental condition (i.e., untreated vs. treated, disease vs. healthy), we recommend recording unstained and FMO controls for each. Autofluorescence properties can change between experimental conditions, and thus it is important to record an unstained control for each using a group-specific unstained reference control tube. We also recommend using group-specific staining controls such as FMOs, as the staining background can also differ between experimental groups, making it difficult to interpret positive signals for proteins expressed at low levels. If you find no differences between experimental groups in your initial validation experiment, then a single representative of these cells can be used for future experiments.
Unmixing and autofluorescence extraction
Your unmixing results will depend on the quality of reference controls. We recommend control optimization to achieve the best unmixing outcome. Ferrer-Font et al. (2021) contains extensive details and user-friendly videos on troubleshooting and optimizing unmixing. In brief, cleanliness, accuracy of fluorochrome reference spectra, and correct gating in the unmixing wizard are all critical for optimal unmixing. To evaluate unmixing accuracy, always visualize NxN plots on cleaned data using the gating sequence: time > singlet > non-debris > viable (dead cells will show compensation errors) > leukocytes, and look to ensure orthogonal staining and confirm that no hyper-negative events can be observed in your data (hyper-negative events are the result of over-unmixing from one fluorophore into other fluorophore[s]). If any unmixing errors are found, they must be resolved before you proceed with data analysis pipelines.
Unmixing with autofluorescence extraction substantially improves staining resolution in channels where brain cells are highly autofluorescent. This includes a significant portion of the UV and violet channels (Supporting Information 3 and 4). Although unmixing with group-specific unstained controls will improve population resolution, the best unmixing result with minimal unmixing errors (hyper-negatives, odd-shaped negatives, and compressed populations) can be achieved by extracting the multiple autofluorescent populations from each group-specific unstained control and saving a separate unmixed file for each experimental group (Fig. 4). It is important to note that autofluorescent tags from each brain sample (mock-infected vs. infected) are unique and cannot be used to unmix the non-corresponding sample type (Supporting Information 5). Lastly, although multiple autofluorescence extraction will substantially increase the complexity index of the panel (Supporting Information 5), it will nonetheless improve staining resolution and reduce unmixing errors (Supporting Information 3 and 4). As both mock-infected and infected brains were unmixed with the same number of autofluorescent tags, samples can be exported directly into the desired flow cytometry analysis program for downstream data analysis, including comparison of median fluorescence intensities and dimensionality reduction. If a different number of tags are used for each experimental group, you will need to use a parameter-removal tool after multi-autofluorescence unmixing to ensure files have the same numbers of parameters.
Basic Protocol 3
There are two critical parameters that should be considered prior to sorting cells from the brain: (1) microglia will begin to die as a result of the stresses experienced during sorting and (2) there will be a limited number of cells that can be sorted from a brain.
As such, we recommend pooling brain samples before staining to ensure that you have enough viable sorted cells for downstream assays. Tissue processing, staining, and downstream sorting processes must also be performed quickly and efficiently to maximize acquisition of data from viable cells. In our experience, using a U-bottom 96-well plate and flicking off the supernatant from the cell pellet (i.e., quickly tipping the plate upside down) in the washing and staining steps before and after the sort is the best procedure for reducing cell loss. You can also sort cells directly into a plate for labeling to avoid the extra centrifugation step required to transfer cells from a tube to a plate. If this is not possible because of the sample volume, use a polypropylene tube (5 ml or 1.2 ml) and aspirate the supernatant, ensuring that you leave sufficient residual volume to prevent pellet disturbance. Removing unnecessary steps, including tube changes, is critical to reducing cell loss after sorting. Lastly, we recommend using FBS or BSA in staining buffers before and after the sort. This will improve cell retention and viability.
Basic Protocol 4
Relative to the other protocols, Basic Protocol 4 has fewer critical parameters, as the tissue is fixed and sections from the same block can be recut to optimize staining controls. Following the above protocols should allow robust results. Always include isotype and unstained controls for accurate interpretation of positive signals.
Troubleshooting
See Table 6 for a list of common problems with the protocols, their causes, and potential solutions.
Problem | Possible cause | Solution |
---|---|---|
No clear Percoll interface | The Percoll interface has been disturbed |
Collect liquid near the Percoll interface, and a clearer delineation between the two layers will eventually become visible. Alternatively, if you gently tilt the tube from side to side, you will see where the interface has formed. Collect the entire 26% Percoll layer. |
73% Percoll does not pass through glass pipet | Percoll is stuck in the glass pipet | Gently lift up glass pipet slightly, by ∼0.5 mm; or, without lifting the pipet, move the pipet around the tube. |
Not enough viable sorted cells | Cells were lost during staining and washing steps |
Use a U-bottom 96-well plate and a flicking technique to wash and stain. Ensure that you are using staining buffer with FBS or BSA. After processing brain samples, pool the cells into a single well before staining. The more cells in a well, the fewer cells will be lost during staining. Alternatively, to save processing time, process two brains together in a single C tube on the GentleMACS in 9 ml PBS and 1 ml collagenase/DNase mixture. Process all samples in the same way. Minimize time between sorting, staining, and downstream processes. Work as quickly as possible and keep all samples on ice. |
Not enough brain cells to generate staining controls | Not enough brain cells were processed |
Use cells isolated from the bone marrow or spleen as single-color compensation/reference controls if they are as bright as, or brighter than, staining observed in multicolor brain samples. For unstained controls, FMOs, and isotype controls, you will need to process extra brain tissue. |
Collagenase/DNase perturbs expression of specific proteins of interest | Enzymes cleave surface proteins |
We advise testing your full panel on enzyme-digested and non-digested tissue before your experiment. We have previously shown that TMEM119 and CD44 detection is reduced with this enzyme cocktail; however, the use of enzymatic dispersion gave us better viability, cell numbers, and staining. Alternatively, other brain processing kits are available from Miltenyi Biotec, including the Neural Tissue Dissociation kit. |
Unmixing/compensation error | Inappropriate reference controls were used | Use reference controls (i.e., beads or cells) that provide antigen expression that is brighter than or equal to that of fully stained samples.Use cells for fluorochromes that exhibit spectral shifting when bound to bead controls.Reference controls must have:
|
Poor resolution between populations | Antibodies have not been adequately titrated | All reagents should be titrated and tested on the specific tissue in advance of experiments. |
Understanding Results
Expected tissue processing cell counts and viability
Processing healthy murine brains using the protocol detailed here will provide ∼500,000 cells with ∼95% viability (as determined by trypan blue count immediately after processing). However, the viability will decrease during the staining process. You will typically identify 75%-90% live cells in the analyzed sample as determined with a viability dye. Moreover, cell counts may vary depending on the disease model used.
Interpreting spectral cytometry results
Compensation
Data should be unmixed in SpectroFlo®, checked for unmixing error in NxN plots on cleaned data, and exported for analysis in the preferred cytometry program. Firstly, as shown in Figure 3, all samples are cleaned up using a time, singlet cell, and non-debris gate. The non-debris gate is then used to examine the compensation matrix. The live leukocyte gate can also be used to inspect the compensation matrix, as dead cells will show errors. The values in the compensation matrix should all be at zero. If you used appropriate reference controls, the unmixing process should be sufficient. However, you may observe compensation errors. Increase matrix numbers when data are under-compensated and reduce numbers when data are over-compensated (see more details at Brummelman et al., 2019; Maciorowski et al., 2017; Mahnke & Roederer, 2007), ensuring that you do not introduce biological error by incorrect spillover correction.
Gating
Once data have been compensated, return to samples and gate data down to populations of interest. Identification of microglia in the homeostatic brain is straight forward: these are “CD45loCD11b+P2RY12+CX3CR1hiF4/80+CD64+CD68+.” They can be identified in multiple ways as they have marker profiles that definitively distinguish them from other myeloid cells in the brain (CD206+ border-associated macrophages) and lymphocytes (Supporting Information 2). If you are working on an acute and severe inflammatory model, the gating strategy shown in Figure 3 may work for you. However, in neurogenerative conditions, infiltrating myeloid cells may differentiate further with more time spent in the brain, whereas microglia may upregulate so-called “activation markers” (Spiteri, Wishart et al., 2022). We recommend running dimensionality reduction on your data using t-distributed stochastic neighbor embedding (tSNE; van der Maaten & Hinton, 2008) or uniform manifold approximation and projection (UMAP; Mcinnes et al., 2018) visualization and overlaying final gated populations over these to determine if these gates are defined by a cluster. Usually in moderately inflamed conditions, microglia and monocyte-derived cells can be clearly distinguished. Gating strategy accuracy can be validated by (i) using a transgenic animal expressing fluorescent molecules under microglia- and/or monocyte-specific genes (Chen et al., 2020; Kim et al., 2021), (ii) blocking myeloid cell infiltration into the brain, (iii) performing an adoptive transfer of sorted cells, or (iv) injecting a cell tracker dye into the bloodstream to track recently infiltrating cells (Spiteri et al., 2021). To gate BrdU, DAF-FM, or other tertiary proteins of interest, always base this on the FMO for the specific experimental group and gate down to the specific population of interest.
Analysis
There are many great tools to analyze cytometric data, enabling population identification and/or statistics and/or dimensionality reduction in a single package. Firstly, we recommend gating your populations of interest and tabulating cell numbers, cell proportions, and median fluorescence intensity. Secondly, for visualization purposes, we recommend running dimensionality reduction and overlaying gated populations onto these to ensure that they align with your expert gating approach. You can also start with dimensionality reduction and run analysis on the FlowSOM clusters identified; however, we would strongly recommend validating these with expert gating to ensure you are not assessing artefacts. Other visualization approaches include, but are not limited to, heatmaps, bar graphs, bubble plots, and pie charts. High-dimensional analysis can be performed in R with packages such as Spectre (Ashhurst et al., 2021) or Seurat (Reyes et al., 2023) or using browser-based platforms such as the OMIC software from Dotmatics (www.omiq.ai, www.dotmatics.com) and Cytobank (Kotecha et al., 2010).
Cell sorting
One can expect to sort ∼80,000 cells from a single brain of a C57BL/6 mouse, according to instrument sort count. Due to potential inaccuracies with sorting machines and cell loss following centrifuging and counting cells, this is generally reduced by approximately two-thirds (i.e., down to ∼25,000 cells). If more cells are required, we recommend pooling samples, as well as having a spare sample to determine the sort accuracy. These sorted cells will need to be recorded to determine the percentage of cells that fall into your selected gate. If you are performing lipidomics, metabolomics, or qPCR, we recommend collecting cells directly into the medium required for cell storage or processing to prevent further cell loss from cell centrifugation. For scRNA-seq, cell sorting accuracy can be determined by analyzing scRNA-seq data computationally.
Imaging
When imaging brain sections, examine isotype and unstained control samples first to determine whether you are visualizing true staining on fully-stained slides. Decide on the regions of interest and image accordingly. Merging of fluorescence images can be done with a number of programs, including ImageJ or FIJI (Rueden et al., 2017; Schindelin et al., 2012). For high-parameter analysis with multiple markers, particularly if using the Hyperion, cells need to be segmented. This can be done using Elastic (Berg et al., 2019) or Cell Profiler (Stirling et al., 2021). For a detailed review of these processes see (Milosevic, 2023).
Time Considerations
Basic Protocol 1
Preparation of tissue processing reagents and tubes can take some time; thus, it is recommended that reagents, labeled tubes, and antibody-staining cocktails are prepared in advance of experiments. The time required to process brain tissue following dissection will be a minimum of 1.5 hr; however, this will increase with increasing sample numbers and user inexperience. For a sample-number-extrapolated calculation, timing from an experienced technician is as follows: For each brain, it will take 30 min for tissue dissociation on the GentleMACS (max of 8 brains can be processed at a time), 2 min for Percoll layering, ∼50 min for centrifugation without brake, 1 min to isolate brains cells per tube, and 15 min for additional spins throughout protocol. Cell counts will take ∼3 min per sample, including mixing with trypan blue, loading, and cleaning hemocytometers.
Basic Protocol 2
Surface staining for spectral cytometry or cell sorting will take a minimum of 2 hr; however, this will also depend on the number of samples you are staining. If you are performing intracellular and/or intranuclear staining, that can be done the following day and will take an additional 1 and 3 hr, respectively. Obviously, intracellular and/or intranuclear staining are not compatible with live cell sorting.
To set up your experiment in SpectroFlo® Software, run your reference controls, and unmix will take ∼45-60 min. From here, depending on the number of cells you are recording and the volume in which they are resuspended, it will take anywhere from 30 s to a few minutes to record a single sample. We recommend setting up your experiment file in SpectroFlo® Software or Cytek® Cloud in advance to enable prompt sample acquisition.
Basic Protocol 4
In general, we recommend scheduling at least 1.5 hr to set up your sorter, record compensation controls, compensate, and draw your gating strategy. However, increasing the number of fluorochromes and complexity of the gating strategy will add substantially more time. Actual sorting of each sample will take <5 min.
Basic Protocol 5
This protocol is the longest, spanning at least 3 days. On the first day, you will dissect the tissue and leave it overnight in PFA. On the second day, you will submerge the tissue in different concentrations of sucrose: 10% for 10 min, 20% for a few hours, and then 30% brain overnight. The next day (day 3), you will freeze the tissue in liquid nitrogen. You can cryosection the tissue on the same day or store it at −80°C. The efficiency of cryosectioning depends on the user's experience level and the number of sections required, with a minimum of 30 min per block for ∼5-10 sections. Staining will take ∼3.5 hr (for fixation, blocking, primary and/or secondary staining, and washing), and the time for imaging depends on the number of regions of interest and the fluorochromes.
Acknowledgments
NJCK was supported by National Health and Medical Research Council Project Grant 1088242 and by the Merridew Foundation. AGS was supported by an Australian Government Research Training Stipend Scholarship and The University of Sydney Postgraduate Merit Award. We would also like to acknowledge the Sydney Cytometry Core Research Facility and the University of Sydney's laboratory animal services, as well as Thomas Ashhurst and Steven Allen for assistance with cytometry applications.
Open access publishing facilitated by The University of Sydney, as part of the Wiley - The University of Sydney agreement via the Council of Australian University Librarians.
Author Contributions
Alanna G. Spiteri : Conceptualization; data curation; formal analysis; investigation; methodology; project administration; validation; visualization; writing—original draft; writing—review and editing. Katherine R. Pilkington : Formal analysis; investigation; validation; visualization; writing—review and editing. Claire L. Wishart : Conceptualization; writing—review and editing. Laurence Macia : Supervision; writing—review and editing. Nicholas J.C. King : Conceptualization; funding acquisition; project administration; resources; supervision; writing—review and editing.
Conflict of Interest
Kate Pilkington is an employee of Cytek Biosciences, Inc., the manufacturer of the Aurora full-spectrum flow cytometer used in these studies.
Open Research
Data Availability Statement
The datasets used and/or analyzed during the current study are available from the corresponding author upon reasonable request.
Supporting Information
Filename | Description |
---|---|
cpz1985-sup-0001-SuppMat.pdf28.6 MB | The Supporting Information consists of the following five parts. Supporting Information 1 Brain processing program for GentleMacs dissociation. Supporting Information 2 Gating strategy used to identify immune cell populations in the homeostatic brain for analysis and cell sorting. (A) and (B) Quality control gates, including time, single cells, non-debris, and live cell gates, were applied before analyzing cell subsets. (B) Identification of neutrophils, microglia, NK cells, T cells, classic dendritic cells (cDC), and border-associated macrophages (BAMs) in the homeostatic brain. Single-cell brain suspensions from a healthy animal were analyzed on the 5-laser Cytek Aurora. Supporting Information 3 Spectral unmixing with multiple autofluorescence extraction substantially improves staining resolution and reduces unmixing error in infected brain samples. (A)-(C) Dot plots showing data spectrally unmixed without autofluorescence (AF) extraction (A), with AF extraction using group-specific unstained controls (B), and with multiple AF extraction (C). Arrows show unmixing error. Supporting Information 4 Spectral unmixing with multiple autofluorescence extraction substantially improves staining resolution and reduces unmixing error in healthy brain samples. (A)-(C) Dot plots showing data spectrally unmixed without autofluorescence (AF) extraction (A), with AF extraction using group-specific unstained controls (B), and with multiple AF extraction (C). Arrows show unmixing error. Supporting Information 5 Similarity index for a 19- to 20-color brain panel with and without multiple autofluorescence extraction. (A) Normalized median florescence intensity (MFI) for three distinct autofluorescent (AF) tags in the mock-infected and infected brain. (B)-(E) Similarity and complexity indexes with and without multiple AF extraction and intranuclear staining with BrdU. |
Please note: The publisher is not responsible for the content or functionality of any supporting information supplied by the authors. Any queries (other than missing content) should be directed to the corresponding author for the article.
Literature Cited
- Ashhurst, T. M., Cox, D. A., Smith, A. L., & King, N. J. C. (2019). Analysis of the murine bone marrow hematopoietic system using mass and flow cytometry. Methods in Molecular Biology , 1989, 159–192. https://doi.org/10.1007/978-1-4939-9454-0_12
- Ashhurst, T. M., Marsh-Wakefield, F., Putri, G. H., Spiteri, A. G., Shinko, D., Read, M. N., Smith, A. L., & King, N. J. C. (2021). Integration, exploration, and analysis of high-dimensional single-cell cytometry data using Spectre. Cytometry Part A: The Journal of the International Society for Analytical Cytology , 101(3), 237–253. https://doi.org/10.1002/cyto.a.24350
- Bassetti, C. L. A., Endres, M., Sander, A., Crean, M., Subramaniam, S., Carvalho, V., Di Liberto, G., Franco, O. H., Pijnenburg, Y., Leonardi, M., & Boon, P. (2022). The European Academy of Neurology Brain Health Strategy: One brain, one life, one approach. European Journal of Neurology , 29, 2559–2566. https://doi.org/10.1111/ene.15391
- Bennett, F. C., Bennett, M. L., Yaqoob, F., Mulinyawe, S. B., Grant, G. A., Hayden Gephart, M., Plowey, E. D., & Barres, B. A. (2018). A combination of ontogeny and CNS environment establishes microglial identity. Neuron , 98, 1170–1183.e8. https://doi.org/10.1016/j.neuron.2018.05.014
- Bennett, M. L., Bennett, F. C., Liddelow, S. A., Ajami, B., Zamanian, J. L., Fernhoff, N. B., Mulinyawe, S. B., Bohlen, C. J., Adil, A., Tucker, A., Weissman, I. L., Chang, E. F., Li, G., Grant, G. A., Hayden Gephart, M. G., & Barres, B. A. (2016). New tools for studying microglia in the mouse and human CNS. Proceedings of the National Academy of Sciences of the United States of America , 113, E1738–E1746.
- Berg, S., Kutra, D., Kroeger, T., Straehle, C. N., Kausler, B. X., Haubold, C., Schiegg, M., Ales, J., Beier, T., Rudy, M., Eren, K., Cervantes, J. I., Xu, B., Beuttenmueller, F., Wolny, A., Zhang, C., Koethe, U., Hamprecht, F. A., & Kreshuk, A. (2019). ilastik: Interactive machine learning for (bio)image analysis. Nature Methods , 16, 1226–1232. https://doi.org/10.1038/s41592-019-0582-9
- Bohlen, C. J., Bennett, F. C., & Bennett, M. L. (2019). Isolation and culture of microglia. Current Protocols in Immunology , 125, e70. https://doi.org/10.1002/cpim.70
- Bohlen, C. J., Bennett, F. C., Tucker, A. F., Collins, H. Y., Mulinyawe, S. B., & Barres, B. A. (2017). Diverse requirements for microglial survival, specification, and function revealed by defined-medium cultures. Neuron , 94, 759–773.e8. https://doi.org/10.1016/j.neuron.2017.04.043
- Brummelman, J., Haftmann, C., Nunez, N. G., Alvisi, G., Mazza, E. M. C., Becher, B., & Lugli, E. (2019). Development, application and computational analysis of high-dimensional fluorescent antibody panels for single-cell flow cytometry. Nature Protocols , 14, 1946–1969. https://doi.org/10.1038/s41596-019-0166-2
- Butovsky, O., Jedrychowski, M. P., Moore, C. S., Cialic, R., Lanser, A. J., Gabriely, G., Koeglsperger, T., Dake, B., Wu, P. M., Doykan, C. E., Fanek, Z., Liu, L., Chen, Z., Rothstein, J. D., Ransohoff, R. M., Gygi, S. P., Antel, J. P., & Weiner, H. L. (2014). Identification of a unique TGF-β-dependent molecular and functional signature in microglia. Nature Neuroscience , 17, 131–143. https://doi.org/10.1038/nn.3599
- Chen, H.-R., Sun, Y.-Y., Chen, C.-W., Kuo, Y.-M., Kuan, I. S., Tiger Li, Z. R., Short-Miller, J. C., Smucker, M. R., & Kuan, C. Y. (2020). Fate mapping via CCR2-CreER mice reveals monocyte-to-microglia transition in development and neonatal stroke. Science Advances , 6, eabb2119. https://doi.org/10.1126/sciadv.abb2119
- Depaula-Silva, A. B., Gorbea, C., Doty, D. J., Libbey, J. E., Sanchez, J. M. S., Hanak, T. J., Cazalla, D., & Fujinami, R. S. (2019). Differential transcriptional profiles identify microglial- and macrophage-specific gene markers expressed during virus-induced neuroinflammation. Journal of Neuroinflammation , 16, 152. https://doi.org/10.1186/s12974-019-1545-x
- Feigin, V. L., Vos, T., Nichols, E., Owolabi, M. O., Carroll, W. M., Dichgans, M., Deuschl, G., Parmar, P., Brainin, M., & Murray, C. (2020). The global burden of neurological disorders: Translating evidence into policy. Lancet Neurology , 19, 255–265. https://doi.org/10.1016/S1474-4422(19)30411-9
- Fekete, R., Cserep, C., Lenart, N., Toth, K., Orsolits, B., Martinecz, B., Mehes, E., Szabo, B., Nemeth, V., Gonci, B., Sperlagh, B., Boldogkoi, Z., Kittel, A., Baranyi, M., Ferenczi, S., Kovacs, K., Szalay, G., Rozsa, B., Webb, C., … Denes, A. (2018). Microglia control the spread of neurotropic virus infection via P2Y12 signalling and recruit monocytes through P2Y12-independent mechanisms. Acta Neuropathologica , 136, 461–482. https://doi.org/10.1007/s00401-018-1885-0
- Ferrer-Font, L., Kraker, G., Hally, K. E., & Price, K. M. (2023). Ensuring full spectrum flow cytometry data quality for high-dimensional data analysis. Current Protocols , 3, e657. https://doi.org/10.1002/cpz1.657
- Ferrer-Font, L., Small, S. J., Lewer, B., Pilkington, K. R., Johnston, L. K., Park, L. M., Lannigan, J., Jaimes, M. C., & Price, K. M. (2021). Panel optimization for high-dimensional immunophenotyping assays using full-spectrum flow cytometry. Current Protocols , 1, e222. https://doi.org/10.1002/cpz1.222
- Fox, A., Dutt, T. S., Karger, B., Obregon-Henao, A., Anderson, G. B., & Henao-Tamayo, M. (2020). Acquisition of high-quality spectral flow cytometry data. Current Protocols in Cytometry , 93, e74. https://doi.org/10.1002/cpcy.74
- Garcia, J. A., Cardona, S. M., & Cardona, A. E. (2014). Isolation and analysis of mouse microglial cells. Current Protocols in Immunology , 104, 14.35.1–14.35.15. https://doi.org/10.1002/0471142735.im1435s104
- Getts, D. R., Terry, R. L., Getts, M. T., Müller, M., Rana, S., Deffrasnes, C., Ashhurst, T. M., Radford, J., Hofer, M., & Thomas, S. (2012). Targeted blockade in lethal West Nile virus encephalitis indicates a crucial role for very late antigen (VLA)-4-dependent recruitment of nitric oxide-producing macrophages. Journal of Neuroinflammation , 9, 246. https://doi.org/10.1186/1742-2094-9-246
- Getts, D. R., Terry, R. L., Getts, M. T., Müller, M., Rana, S., Shrestha, B., Radford, J., Van Rooijen, N., Campbell, I. L., & King, N. J. C. (2008). Ly6c+ “inflammatory monocytes” are microglial precursors recruited in a pathogenic manner in West Nile virus encephalitis. The Journal of Experimental Medicine , 205, 2319–2337. https://doi.org/10.1084/jem.20080421
- Gosselin, D., Skola, D., Coufal, N. G., Holtman, I. R., Schlachetzki, J. C. M., Sajti, E., Jaeger, B. N., O'Connor, C., Fitzpatrick, C., Pasillas, M. P., Pena, M., Adair, A., Gonda, D. D., Levy, M. L., Ransohoff, R. M., Gage, F. H., & Glass, C. K. (2017). An environment-dependent transcriptional network specifies human microglia identity. Science , 356, eaal3222. https://doi.org/10.1126/science.aal3222
- Greter, M., Lelios, I., & Croxford, A. L. (2015). Microglia versus myeloid cell nomenclature during brain inflammation. Frontiers in Immunology , 6, 249. https://doi.org/10.3389/fimmu.2015.00249
- Ijsselsteijn, M. E., Van Der Breggen, R., Farina Sarasqueta, A., Koning, F., & De Miranda, N. (2019). A 40-marker panel for high dimensional characterization of cancer immune microenvironments by imaging mass cytometry. Frontiers in Immunology , 10, 2534. https://doi.org/10.3389/fimmu.2019.02534
- Jordão, M. J. C., Sankowski, R., Brendecke, S. M., Sagar, Locatelli, G., Tai, Y. H., Tay, T. L., Schramm, E., Armbruster, S., Hagemeyer, N., Groß, O., Mai, D., Çiçek, Ö., Falk, T., Kerschensteiner, M., Grün, D., & Prinz, M. (2019). Single-cell profiling identifies myeloid cell subsets with distinct fates during neuroinflammation. Science , 363, eaat7554. https://doi.org/10.1126/science.aat7554
- Keren-Shaul, H., Spinrad, A., Weiner, A., Matcovitch-Natan, O., Dvir-Szternfeld, R., Ulland, T. K., David, E., Baruch, K., Lara-Astaiso, D., Toth, B., Itzkovitz, S., Colonna, M., Schwartz, M., & Amit, I. (2017). A unique microglia type associated with restricting development of Alzheimer's disease. Cell , 169, 1276–1290.e17. https://doi.org/10.1016/j.cell.2017.05.018
- Kharraz, Y., Lukesova, V., Serrano, A. L., Davison, A., & Munoz-Canoves, P. (2022). Full spectrum cytometry improves the resolution of highly autofluorescent biological samples: Identification of myeloid cells in regenerating skeletal muscles. Cytometry Part A: The journal of the International Society for Analytical Cytology , 101, 862–876. https://doi.org/10.1002/cyto.a.24568
- Kim, J. S., Kolesnikov, M., Peled-Hajaj, S., Scheyltjens, I., Xia, Y., Trzebanski, S., Haimon, Z., Shemer, A., Lubart, A., Van Hove, H., Chappell-Maor, L., Boura-Halfon, S., Movahedi, K., Blinder, P., & Jung, S. (2021). A binary cre transgenic approach dissects microglia and CNS border-associated macrophages. Immunity , 54, 176–190.e7. https://doi.org/10.1016/j.immuni.2020.11.007
- Kotecha, N., Krutzik, P. O., & Irish, J. M. (2010). Web-based analysis and publication of flow cytometry experiments. Current Protocols in Cytometry , 53, 10.17.1–10.17.24. https://doi.org/10.1002/0471142956.cy1017s53
- Krasemann, S., Madore, C., Cialic, R., Baufeld, C., Calcagno, N., El Fatimy, R., Beckers, L., O'Loughlin, E., Xu, Y., Fanek, Z., Greco, D. J., Smith, S. T., Tweet, G., Humulock, Z., Zrzavy, T., Conde-Sanroman, P., Gacias, M., Weng, Z., Chen, H., … Butovsky, O. (2017). The TREM2-APOE pathway drives the transcriptional phenotype of dysfunctional microglia in neurodegenerative diseases. Immunity , 47, 566–581.e9. https://doi.org/10.1016/j.immuni.2017.08.008
- Lei, F., Cui, N., Zhou, C., Chodosh, J., Vavvas, D. G., & Paschalis, E. I. (2020). CSF1R inhibition by a small-molecule inhibitor is not microglia specific; affecting hematopoiesis and the function of macrophages. Proceedings of the National Academy of Sciences of the United States of America , 117, 23336–23338. https://doi.org/10.1073/pnas.1922788117
- Lewis, N. D., Hill, J. D., Juchem, K. W., Stefanopoulos, D. E., & Modis, L. K. (2014). RNA sequencing of microglia and monocyte-derived macrophages from mice with experimental autoimmune encephalomyelitis illustrates a changing phenotype with disease course. Journal of Neuroimmunology , 277, 26–38. https://doi.org/10.1016/j.jneuroim.2014.09.014
- Look, T., Meister, H., Weller, M., & Weiss, T. (2023). Protocol for the expansion of mouse immune effector cells for in vitro and in vivo studies. STAR Protocols , 4, 102700. https://doi.org/10.1016/j.xpro.2023.102700
- Maciorowski, Z., Chattopadhyay, P. K., & Jain, P. (2017). Basic multicolor flow cytometry. Current Protocols in Immunology , 117, 5.4.1–5.4.38. https://doi.org/10.1002/cpim.26
- Mahnke, Y. D., & Roederer, M. (2007). Optimizing a multicolor immunophenotyping assay. Clinics in Laboratory Medicine , 27, 469–+. https://doi.org/10.1016/j.cll.2007.05.002
- Manglani, M., Gossa, S., & McGavern, D. B. (2018). Leukocyte isolation from brain, spinal cord, and meninges for flow cytometric analysis. Current Protocols in Immunology , 121, e44. https://doi.org/10.1002/cpim.44
- Mcinnes, L., Healy, J., & Melville, J. (2018). UMAP: Uniform manifold approximation and projection for dimension reduction [Preprint]. arXiv , 1802.03426. https://doi.org/10.48550/arXiv.1802.03426
- Mcquade, A., & Blurton-Jones, M. (2019). Microglia in Alzheimer's disease: Exploring how genetics and phenotype influence risk. Journal of Molecular Biology , 431, 1805–1817. https://doi.org/10.1016/j.jmb.2019.01.045
- Miller, S. D., Karpus, W. J., & Davidson, T. S. (2010). Experimental autoimmune encephalomyelitis in the mouse. Current Protocols in Immunology , Chapter 15, 15.1.1–15.1.20.
- Milosevic, V. (2023). Different approaches to imaging mass cytometry data analysis. Bioinformatics Advances , 3, vbad046. https://doi.org/10.1093/bioadv/vbad046
- Niewold, P., Ashhurst, T. M., Smith, A. L., & King, N. J. C. (2020). Evaluating spectral cytometry for immune profiling in viral disease. Cytometry Part A: The Journal of the International Society for Analytical Cytology , 97, 1165–1179. https://doi.org/10.1002/cyto.a.24211
- Nimmerjahn, A., Kirchhoff, F., & Helmchen, F. (2005). Resting microglial cells are highly dynamic surveillants of brain parenchyma in vivo. Science , 308, 1314–1318. https://doi.org/10.1126/science.1110647
- Nolan, J. P., & Condello, D. (2013). Spectral flow cytometry. Current Protocols in Cytometry , 63, 1.27.1–1.27.13. https://doi.org/10.1002/0471142956.cy0127s63
- Reyes, J. G. A., Ni, D., Santner-Nanan, B., Pinget, G. V., Kraftova, L., Ashhurst, T. M., Marsh-Wakefield, F., Wishart, C. L., Tan, J., Hsu, P., King, N. J. C., Macia, L., & Nanan, R. (2023). A unique human cord blood CD8+CD45RA+CD27+CD161+ T cell subset identified by flow cytometric data analysis using Seurat [Preprint]. BioRxiv , 2023.08.01.549954. https://doi.org/10.1101/2023.08.01.549954
- Rueden, C. T., Schindelin, J., Hiner, M. C., Dezonia, B. E., Walter, A. E., Arena, E. T., & Eliceiri, K. W. (2017). ImageJ2: ImageJ for the next generation of scientific image data. BMC Bioinformatics , 18, 529. https://doi.org/10.1186/s12859-017-1934-z
- Schindelin, J., Arganda-Carreras, I., Frise, E., Kaynig, V., Longair, M., Pietzsch, T., Preibisch, S., Rueden, C., Saalfeld, S., Schmid, B., Tinevez, J. Y., White, D. J., Hartenstein, V., Eliceiri, K., Tomancak, P., & Cardona, A. (2012). Fiji: An open-source platform for biological-image analysis. Nature Methods , 9, 676–682. https://doi.org/10.1038/nmeth.2019
- Sharp, R. C., Guenther, D. T., & Farrer, M. J. (2023). Experimental procedures for flow cytometry of wild-type mouse brain: A systematic review. Frontiers in Immunology , 14, 1281705. https://doi.org/10.3389/fimmu.2023.1281705
- Song, H., McEwen, H. P., Duncan, T., Lee, J. Y., Teo, J. D., & Don, A. S. (2021). Sphingosine kinase 2 is essential for remyelination following cuprizone intoxication. Glia , 69, 2863–2881. https://doi.org/10.1002/glia.24074
- Spangenberg, E., Severson, P. L., Hohsfield, L. A., Crapser, J., Zhang, J., Burton, E. A., Zhang, Y., Spevak, W., Lin, J., Phan, N. Y., Habets, G., Rymar, A., Tsang, G., Walters, J., Nespi, M., Singh, P., Broome, S., Ibrahim, P., Zhang, C., … Green, K. N. (2019). Sustained microglial depletion with CSF1R inhibitor impairs parenchymal plaque development in an Alzheimer's disease model. Nature Communications , 10, 3758. https://doi.org/10.1038/s41467-019-11674-z
- Spiteri, A. G., & King, N. J. C. (2023). Putting PLX5622 into perspective: Microglia in central nervous system viral infection. Neural Regeneration Research , 18, 1269–1270.
- Spiteri, A. G., Ni, D., Ling, Z. L., Macia, L., Campbell, I. L., Hofer, M. J., & King, N. J. C. (2022). PLX5622 reduces disease severity in lethal CNS infection by off-target inhibition of peripheral inflammatory monocyte production. Frontiers in Immunology , 13, 851556. https://doi.org/10.3389/fimmu.2022.851556
- Spiteri, A. G., Terry, R. L., Wishart, C. L., Ashhurst, T. M., Campbell, I. L., Hofer, M. J., & King, N. J. C. (2021). High-parameter cytometry unmasks microglial cell spatio-temporal response kinetics in severe neuroinflammatory disease. Journal of Neuroinflammation , 18, 166. https://doi.org/10.1186/s12974-021-02214-y
- Spiteri, A. G., Van Vreden, C., Ashhurst, T. M., Niewold, P., & King, N. J. C. (2023). Clodronate is not protective in lethal viral encephalitis despite substantially reducing inflammatory monocyte infiltration in the CNS. Frontiers in immunology , 14, 1203561. https://doi.org/10.3389/fimmu.2023.1203561
- Spiteri, A. G., Wishart, C. L., & King, N. J. C. (2020). Immovable object meets unstoppable force? Dialogue between resident and peripheral myeloid cells in the inflamed brain. Frontiers in Immunology , 11, 600822. https://doi.org/10.3389/fimmu.2020.600822
- Spiteri, A. G., Wishart, C. L., Ni, D., Viengkhou, B., Macia, L., Hofer, M. J., & King, N. J. C. (2023). Temporal tracking of microglial and monocyte single-cell transcriptomics in lethal flavivirus infection. Acta Neuropathologica Communications , 11, 60. https://doi.org/10.1186/s40478-023-01547-4
- Spiteri, A. G., Wishart, C. L., Pamphlett, R., Locatelli, G., & King, N. J. C. (2022). Microglia and monocytes in inflammatory CNS disease: Integrating phenotype and function. Acta Neuropathologica , 143, 179–224. https://doi.org/10.1007/s00401-021-02384-2
- Stirling, D. R., Swain-Bowden, M. J., Lucas, A. M., Carpenter, A. E., Cimini, B. A., & Goodman, A. (2021). CellProfiler 4: Improvements in speed, utility and usability. BMC Bioinformatics , 22, 433. https://doi.org/10.1186/s12859-021-04344-9
- Van Der Maaten, L., & Hinton, G. (2008). Visualizing data using t-SNE. Journal of Machine Learning Research , 9, 2579–2605.
- Vankriekelsvenne, E., Chrzanowski, U., Manzhula, K., Greiner, T., Wree, A., Hawlitschka, A., Llovera, G., Zhan, J., Joost, S., Schmitz, C., Ponsaerts, P., Amor, S., Nutma, E., Kipp, M., & Kaddatz, H. (2022). Transmembrane protein 119 is neither a specific nor a reliable marker for microglia. Glia , 70, 1170–1190. https://doi.org/10.1002/glia.24164
- Werner, Y., Mass, E., Ashok Kumar, P., Ulas, T., Handler, K., Horne, A., Klee, K., Lupp, A., Schutz, D., Saaber, F., Redecker, C., Schultze, J. L., Geissmann, F., & Stumm, R. (2020). Cxcr4 distinguishes HSC-derived monocytes from microglia and reveals monocyte immune responses to experimental stroke. Nature Neuroscience , 23, 351–362. https://doi.org/10.1038/s41593-020-0585-y
- West, P. K., Mccorkindale, A. N., Guennewig, B., Ashhurst, T. M., Viengkhou, B., Hayashida, E., Jung, S. R., Butovsky, O., Campbell, I. L., & Hofer, M. J. (2022). The cytokines interleukin-6 and interferon-alpha induce distinct microglia phenotypes. Journal of Neuroinflammation , 19, 96. https://doi.org/10.1186/s12974-022-02441-x
- Wishart, C. L., Spiteri, A. G., Locatelli, G., & King, N. J. C. (2022). Integrating transcriptomic datasets across neurological disease identifies unique myeloid subpopulations driving disease-specific signatures. Glia , 71(4), 904–925. https://doi.org/10.1002/glia.24314
Citing Literature
Number of times cited according to CrossRef: 1
- Paula Niewold, High‐dimensional flow cytometry data: goldmine or fool's gold?, Cytometry Part A, 10.1002/cyto.a.24849, 105 , 6, (425-427), (2024).