Generation of Bispecific Antibodies by Functionalized Poly-ADP-Ribose Polymers
Hyo Sun Kim, Hyo Sun Kim, Yong Zhang, Yong Zhang
antibody
bispecific antibody
chemical conjugation
immunotherapy
PARP1
poly-ADP-ribose
post-translational modification
Abstract
Bispecific antibodies have drawn considerate research interests for therapeutic development. Numerous genetic and chemical methods are established to produce bispecific antibodies with varied formats. This protocol describes a novel approach to the synthesis of bispecific antibodies by utilizing chemically functionalized poly-ADP-ribose polymers derived from post-translational poly-ADP-ribosylation. Basic Protocol 1 includes experimental procedures for expressing and purifying recombinant full-length human poly-ADP-ribose polymerase 1 (PARP1) as well as monoclonal antibodies targeting T-cell CD3 and breast cancer tumor-associated human epidermal growth factor receptor 2 (HER2) molecules. Basic Protocol 2 details methods for enzymatic preparation of functionalized poly-ADP-ribose polymers by PARP1 and chemical conjugation of antibody molecules for bispecific antibody production. © 2023 The Authors. Current Protocols published by Wiley Periodicals LLC.
Basic Protocol 1 : Expression and purification of PARP1 and antibodies
Basic Protocol 2 : PARP1 auto-modification and antibody conjugation
INTRODUCTION
Simultaneously engaging with two distinct types of antigen molecules, bispecific antibodies possess unique and strong potential for treatment of human diseases, especially in cancer immunotherapy (Li et al., 2020; Wei et al., 2022; Yang et al., 2017). To date, nine bispecific antibodies are approved for clinical use by the FDA, many of which function through recruiting immune effector cells to tumors and activating potent cancer-specific immunity (Esfandiari et al., 2022; Krishnamurthy & Jimeno, 2018). Using different technologies (e.g., quadroma that somatically fuses two types of hybridoma cells, chemical conjugation, and genetic fusion), bispecific antibodies with varied sizes, formats, and pharmacological properties have been generated (Brinkmann & Kontermann, 2017; Dimasi et al., 2021; Klausner, 1987).
Protein ADP-ribosylation is an enzymatic post-translational modification featuring additions of ADP-ribose monomer or poly-ADP-ribose (PAR) polymers onto target proteins (Gibson et al., 2016; Morales et al., 2014). Poly-ADP-ribose polymerase 1 (PARP1) is characterized by robust catalytic activity for cellular protein poly-ADP-ribosylation (PARylation) in response to DNA damage (Beck et al., 2014; Bianchi et al., 2016; De Vos et al., 2012; Jeggo, 1998; Lindahl et al., 1995; Tan et al., 2012). The resulting linear and branched PAR polymers are highly soluble, providing a biocompatible and polymeric scaffold for biomolecule conjugations and therapeutic applications (Cheng et al., 2023; Cheng et al., 2022; Shi et al., 2020). As nicotinamide adenine dinucleotide (NAD+) molecule participates in the protein ADP-ribosylation by donating the ADP-ribose, use of NAD+ analogues with clickable groups that can serve as strong substrates of PARylation could enable functionalization of PAR polymers for facile bioconjugations (Lam et al., 2021; Zhang et al., 2019; Zhang et al., 2022). An NAD+ analogue with a nicotinamide riboside (NR) 3′-azido was developed with high PARylation activity for human PARP1 enzyme, providing a valuable tool for producing functionalized PAR polymers (Cheng et al., 2023; Zhang et al., 2019). In this protocol, we describe a functionalized PAR polymer-based method for generating bispecific antibodies. By coupling the 3′-azido NAD+ analogue with recombinant full-length human PARP1, clickable PAR polymers derived from auto-PARylated PARP1 were synthesized, allowing subsequent conjugation with bicyclo[6.1.0]nonyne (BCN)-functionalized antibody IgGs specific for human CD3 and human epidermal growth factor receptor 2 (HER2) molecules (Fig. 1). The functionalized PAR polymer-mediated antibody conjugation represents a new approach for synthesizing bispecific antibodies.
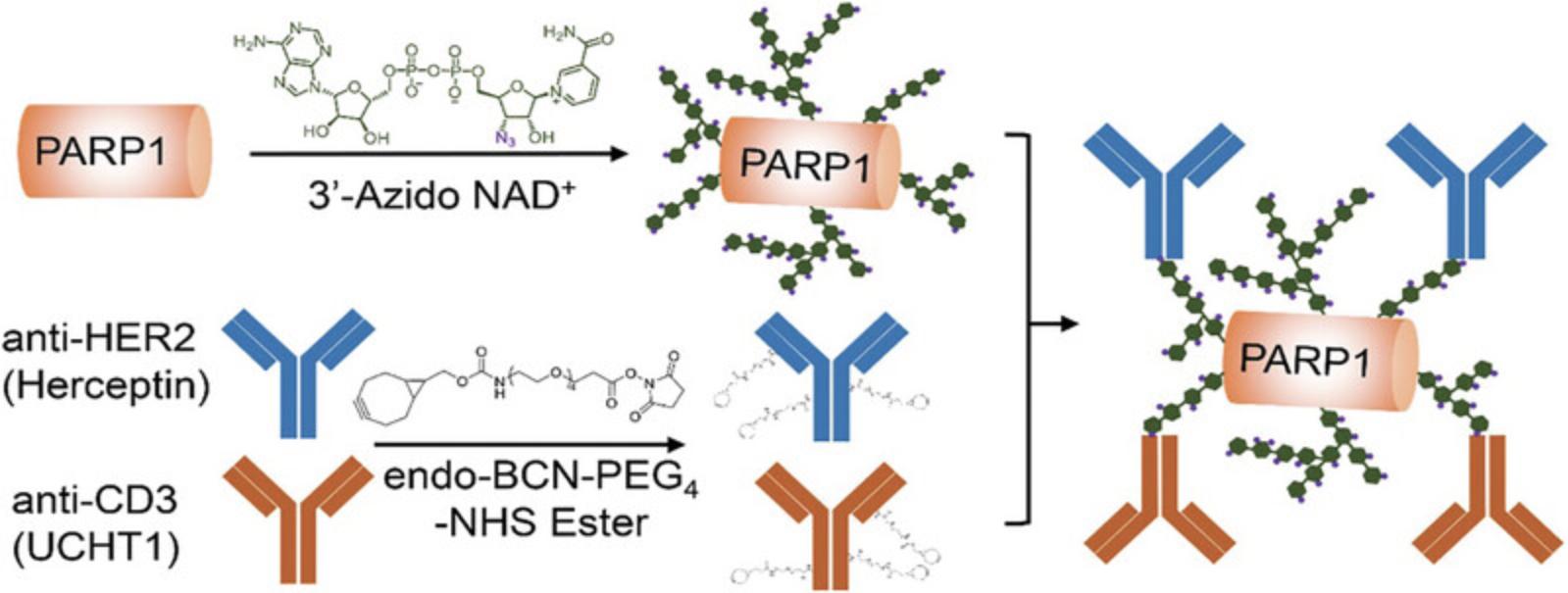
Basic Protocol 1: EXPRESSION AND PURIFICATION OF PARP1 AND ANTIBODIES
In this protocol, plasmids expressing full-length human PARP1 with a C-terminal His6-tag, anti-human CD3 monoclonal antibody IgG (clone: UCHT1), and anti-human HER2 monoclonal antibody IgG (clone: trastuzumab; brand name: Herceptin) are used to express the proteins. cDNA of human PARP1 (GE Healthcare Dharmacon) was cloned into the pET-28a(+) vector (Milipore Sigma, cat. no. 69864) for bacterial expression. DNA fragments encoding heavy and light chains of UCHT1 and Herceptin antibodies were cloned into the pFuse vector (Invivogen, cat. no. pfuse-hg1fc2) for mammalian cell expression. Please refer to previous studies for molecular cloning and plasmid preparation (Cheng et al., 2023; Zhang et al., 2019). Full-length human PARP1 is expressed via a bacterial expression system, while the antibodies are transiently expressed in mammalian cells. Expressed PARP1 and antibody IgGs are then purified using different chromatographic methods.
Materials
-
Bacterial expression of PARP1 reagents:
- BL21(DE3) bacterial cells (Invitrogen, cat. no. C600003)
- LB broth, Miller (Fisher Bioreagents, cat. no. BP1426-2)
- Kanamycin sulfate (VWR, cat. no. 0408-25G)
- ZnSO4 (Thermo Fisher Scientific Chemicals, cat. no. 33399)
- Isopropyl-β-D-thiogalactopyranoside (IPTG), dioxane free (Chem-IMPEX INT'L INC., cat. no. 00194)
-
Purification of PARP1 reagents:
- PARP1 purification buffers (see recipe)
- HisPur Ni-NTA resin (Thermo Fisher Scientific, cat. no. 88221)
- Heparin sepharose 6 fast flow (Cytiva, cat. no. 17099801)
- Superdex 200 Increase 10/300 GL (Cytiva, cat. no. 28990944)
- Pierce LAL chromogenic endotoxin quantitation kits (Fisher Scientific, cat. no. PI88282)
-
Antibody expression and purification from mammalian cells reagents:
- ZymoPure maxiprep kit (Zymo Research, cat. no. D4203)
- Expi293F cell line (Gibco, cat. no. A14527)
- BalanCD HEK293 medium (Fuji Film, cat. no. 91165)
- L-Glutamine (Corning, cat. no. 25-005-CI)
- Trypan blue solution, 0.4% (Gibco, cat. no. 15250-061)
- Opti-MEM (Gibco, cat. no. 31985-070)
- PEI MAX, transfection grade linear polyethylenimine-hydrochloride (Polysciences Inc., cat. no. 24765-1)
- Protein G resin (GenScript, cat. no. L00209)
- Phosphate-buffered saline (PBS) (Current Protocols, 2006)
- Protein G column elution buffer (see recipe)
- 1 M Tris, pH 8.0 (Current Protocols, 2006)
- Dialysis tubing, 10-ft (Science First, cat. no. 2852)
-
Electroporator with 1-mm gap cuvettes (Bio-Rad)
-
Incubator shaker (Series 25, New Brunswick Scientific)
-
Nanodrop 2000c (Thermo Fisher Scientific)
-
Erlenmeyer flasks (Nest Biotechnology, cat. no. 783011)
-
Centrifuge (Beckman J6B Centrifuge with JS-4.2 rotor & JA-17 rotor)
-
French Press G-M (Fisher Scientific, cat. no. NC0355680)
-
0.45-μm cellulose acetate membranes (Advantec, cat. no. C045A047A)
-
Gravity flow column (Bio-Rad, cat. no. 7321010)
-
AKTA Pure chromatography system (Cytiva)
-
Amicon centrifugal filters, 30 kDa (EMD Milipore, cat. no. UFC803008)
-
Acrodisc unit with a mustang E membrane (VWR, cat. no. 28140-521)
-
Vortex (VWR)
-
Standard analog shaker (VWR)
-
Megafuge 16 (Thermo Fisher Scientific)
-
Additional materials for SDS-PAGE (Gallagher, 2008)
Bacterial expression of full-length human PARP1
1.Electroporate BL21(DE3) cells with the constructed pET-28a(+) vector encoding full-length human PARP1.
2.Inoculate the transformed bacterial cells in 10 ml LB broth medium containing 50 μg/ml kanamycin overnight.
3.Transfer the 10 ml LB broth medium into 1 L LB broth medium with 50 μg/ml of kanamycin.
4.Grow the bacterial cells until a nanodrop OD600 measurement reaches 0.6 to 0.8.
5.Add ZnSO4 with a final concentration of 0.1 mM into the cell medium.
6.Keep growing the bacterial cells until OD600 reaches 0.8 to 1.0.
7.Incubate the flask on ice for 1 hr.
8.Add IPTG of a final concentration of 0.5 mM in order to induce the protein expression, followed by overnight incubation at 16 ° C.
Purification of full-length human PARP1
9.Centrifuge the bacterial cell culture using a JS-4.2 rotor for 30 min at 4550 × g , 4°C.
10.Discard the supernatant and resuspend the cells in lysis buffer.
11.Lyse the cells by passing through a French Press 3 times at 25,000 psi.
12.Centrifuge the lysates using a JA-17 rotor for 100 min at 27,000 × g , at 4 ° C.
13.Collect the supernatant and filter it through a 0.45-μm cellulose acetate membrane.
14.Pack a gravity flow column with 5 ml of Ni-NTA resin.
15.Add 10 ml of filtered water, followed by 15 ml of equilibrium buffer to equilibrate the column.
16.Load the filtered solution from step 13 onto the column.
17.Wash the column with 50 ml high-salt wash buffer.
18.Elute the protein with 25 ml elution buffer.
19.Add 25 ml no-salt buffer to the eluted proteins then load the mixed solution to a 5-ml heparin column by a low-pressure peristaltic pump at a flow rate of 3 ml/min.
20.Place the heparin column on an AKTA Pure chromatography system to elute PARP1 using a gradient of 0% to 100% buffer B in a buffer A at a flow rate of 1 ml/min.
21.Combine the fractions with eluted PARP1 and spin it down to 500 μl using centrifugal filters with 30 kDa molecular weight cut-off.
22.Inject the concentrated PARP1 into a Superdex 200 Increase 10/300 GL size-exclusion chromatography column and elute it using gel filtration buffer.
23.Pass the purified protein through an Acrodisc unit with a mustang E membrane.
24.Determine the final endotoxin levels using Pierce LAL chromogenic endotoxin quantitation kits.
25.Use SDS-PAGE gels to evaluate the purified human PARP1 (Fig. 2A).
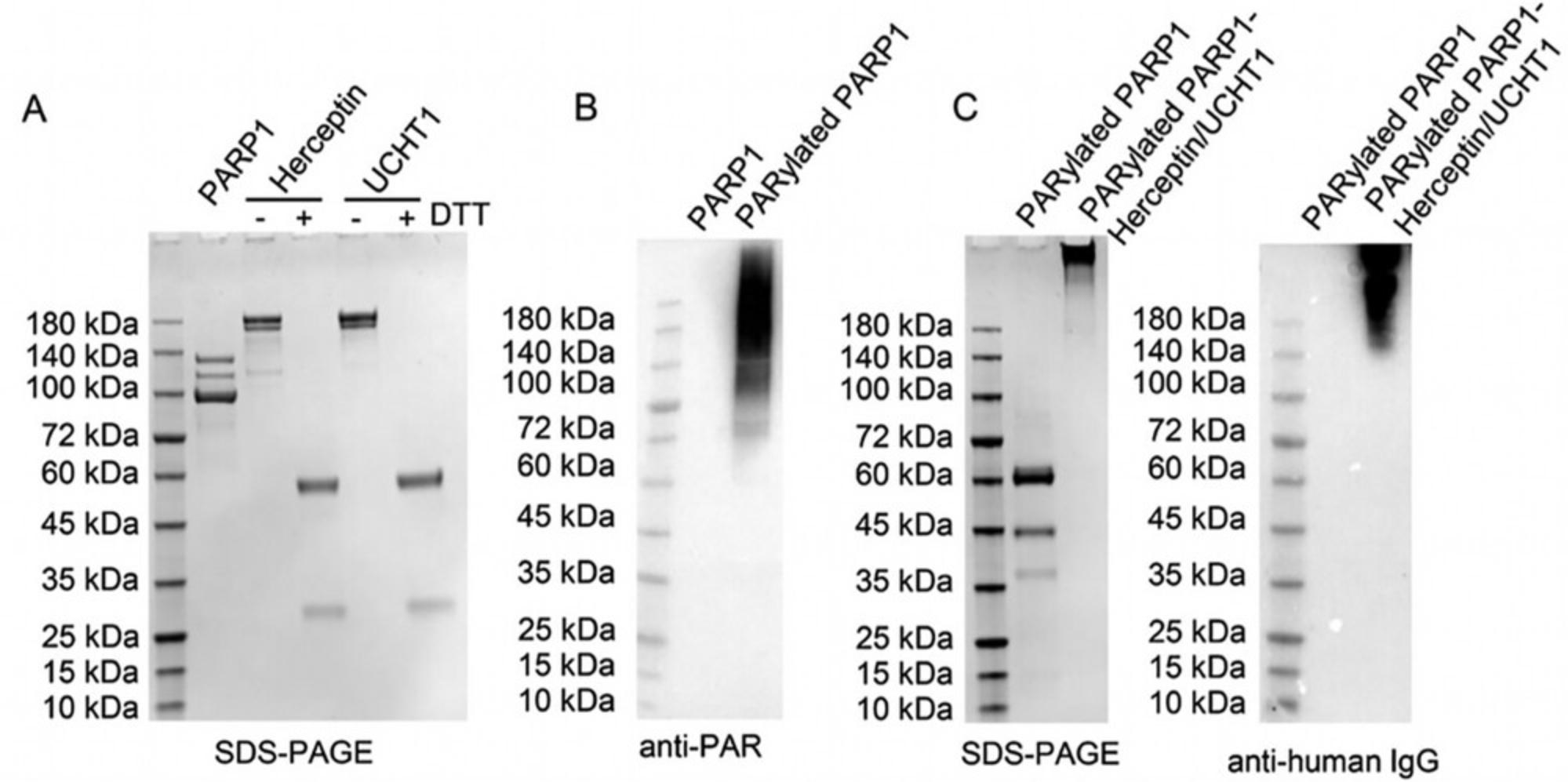
Transient transfection of mammalian cells for antibody expression and purification
26.Amplify the sequence-verified plasmids using maxiprep kits.
27.Grow Expi293F cells in BalanCD HEK293 medium supplemented with L-glutamine for >3 passages in a 37 ° C incubator with 5% CO2.
28.Count the cells using trypan blue stain and passage them to reach at the final density of 5 million cells/ml in 120 ml medium.
29.Prepare the transfection reagent by mixing 12 ml Opti-MEM medium, 120 μg plasmid encoding antibody IgG heavy chain, 120 μg plasmid encoding antibody IgG light chain, and 960 μl transfection grade PEI-MAX (1 mg/ml).
30.Incubate the solution at room temperature for 20 min.
31.Add the transfection complexes to the cell medium and incubate the cell medium in the incubator for 2 hr at 37°C with 5% CO2.
32.Add an additional 120 ml BalanCD HEK293 medium into the cell flasks for a final density of 2.5 million cells/ml in 240 ml medium total.
33.Incubate the cells in the incubator for 5 days.
34.On day 5, centrifuge the cells 10 min at 100 × g , room temperature, to collect the supernatant with secreted antibodies.
35.Centrifuge the collected supernatant 30 min at 4000 × g , 4°C, to further remove cell debris or impurities, then collect the supernatant.
36.Pack a gravity flow column with 2 ml protein G resin and equilibrate the column with 10 ml PBS.
37.Load the supernatant collected in step 34 onto the column.
38.Wash the column with 15 ml PBS and elute the protein with Protein G column elution buffer (100 mM glycine, pH 2.7).
39.Neutralize the fractions with significant protein concentrations with 100 μl of 1 M Tris buffer (pH 8.0).
40.Dialyze the collected fractions against PBS two times (one overnight and one 6-hr dialysis) using 10 kDa molecular weight cutoff dialysis bags at 4 ° C.
41.Use SDS-PAGE gels to evaluate each of the purified antibodies (Fig. 2A).
Basic Protocol 2: PARP1 AUTO-MODIFICATION AND ANTIBODY CONJUGATION
In this protocol, purified PARP1 from Basic Protocol 1 undergoes auto-modification with 3′-azido NAD+ in order to generate azido-functionalized PAR polymers. Please refer to a previous study for the synthesis protocol of 3′-azido NAD+ (Zhang et al., 2019). Purified antibodies from Basic Protocol 1 are functionalized with BCN groups for conjugation with auto-PARylated PARP1 by 3′-azido NAD+ via copper-free click chemistry to form bispecific antibodies.
Additional Materials (also see Basic Protocol 1)
-
Endo-BCN-PEG4-N-hydroxysuccinimide (NHS) ester linker (BroadPharm, cat. no. BP-22851)
-
DMSO
-
PARP1 auto-modification reaction buffer (see recipe)
-
Additional materials and reagents for immunoblotting (Ni et al., 2016)
Antibody NHS-BCN linker conjugation
1.Add endo-BCN-PEG4-NHS ester linker to purified Herceptin or UCHT1 antibody suspended in PBS at a molar ratio of 20 linkers to 1 protein.
2.Gently mix the solution and incubate at room temperature for 2 hr.
3.Buffer exchange the mixture against PBS using 30 kDa molecular weight cut-off Amicon centrifugal filters.
PARP1 auto-modification
4.Prepare the reaction buffer.
5.Incubate the purified human PARP1 (3 μM) in the reaction buffer for 20 min for activation.
6.Add 150 μM of 3′-azido NAD+ in the reaction buffer and incubate the mixture at 30 ° C for 8 hr.
7.Buffer exchange the mixture against PBS using 30 kDa molecular weight cut-off Amicon centrifugal filters.
8.Perform immunoblot analysis with an anti-PAR antibody (clone: 10H) to evaluate the formation of PAR polymers following auto-modification (Fig. 2B).
Generation of bispecific antibodies
9.Mix Herceptin-BCN and UCHT1-BCN antibodies and PARylated PARP1 with a molar ratio of 3:3:1, respectively, in PBS.
10.Incubate the reaction mixture at room temperature for 3 days.
11.Load the reaction mixture to a Superdex 200 Increase 10/300 GL column for size exclusion chromatography purification.
12.Elute the fractions with PBS.
13.Collect the first peak of eluate and concentrate it using Amicon centrifugal filters with 30 kDa molecular weight cut-off.
14.Evaluate antibody conjugation using both Coomassie-stained SDS-PAGE gels and immunoblotting (Fig. 2C).
REAGENTS AND SOLUTIONS
Use ddH2O for all solutions. Unless otherwise stated, all buffers can be stored up to 6 months at room temperature. For commonly used reagents, please see Current Protocols (2006).
PARP1 auto-modification reaction buffer
- 30 mM HEPES pH 8.0
- 5 mM MgCl2
- 5 mM CaCl2
- 250 mM NaCl
- 1 mM DTT
- 100 ng/μl activated DNA (Sigma-Aldrich, D4522)
- Prepare fresh prior to each reaction
PARP1 purification buffers
- _ Lysis buffer_
- 25 mM HEPES pH 8.0
- 500 mM NaCl
- 1 mM phenylmethylsulfonyl fluoride (PMSF)
- Store up to 6 months at 4°C
- Add PMSF immediately prior to use
- Equilibrium buffer
- 25 mM HEPES pH 8.0
- 500 mM NaCl
- 20 mM imidazole
- High-salt wash buffer
- 25 mM HEPES pH 8.0
- 1 M NaCl
- 20 mM imidazole
- Elution buffer
- 25 mM HEPES pH 8.0
- 500 mM NaCl
- 400 mM imidazole
- No-salt buffer
- 50 mM Tris pH 7.0
- 1 mM EDTA
- 0.1 mM DTT
- Buffer A
- 50 mM Tris pH 7.0
- 1 mM EDTA
- 0.1 mM DTT
- 250 mM NaCl
- Buffer B
- 50 mM Tris pH 7.0
- 1 mM EDTA
- 0.1 mM DTT
- 1 M NaCl
- Gel filtration buffer
- 25 mM HEPES pH 8.0
- 150 mM NaCl
- 1 mM EDTA
- 0.1 mM DTT
Protein G column elution buffer
- 100 mM glycine
- Adjust to pH 2.7 using HCl or NaOH
- Prepare fresh prior to each purification
COMMENTARY
Background Information
Protein PARylation is involved in a variety of cellular events (Beck et al., 2014; Gibson et al., 2016; Jeggo, 1998). The resulting ADP-ribose-based polymers provide a valuable scaffold with high solubility and biocompatibility for therapeutic conjugation. Human PARP1 is characterized by robust auto-PARylation (Bianchi et al., 2016; Mendoza-Alvarez & Alvarez-Gonzalez, 1993) and facilitates the preparation of functionalized PAR polymers via its catalyzed auto-modification with NAD+ analogues. PARylation reactions with 3′-azido NAD+, an excellent substrate for PARP1, lead to rapid generation of azido-functionalized PAR polymers. Through copper-free click chemistry, different types of monoclonal antibodies could then be conjugated to generate bispecific antibodies. The PAR polymer-based bispecific antibodies feature high molecular weights and increased valency, which may result in improved pharmacological properties, e.g., extended half-lives and enhanced binding affinity. Despite abundant conjugation sites on functionalized PAR polymers, the molar ratio for conjugation is set at 3:3:1 for anti-CD3 antibody, anti-HER2 antibody, and PARylated PARP1, respectively, to avoid potential steric hindrance between IgG molecules. Given the nature of the functionalized PAR polymers, additional types of antibodies or a different format of antibodies could be included for production.
Critical Parameters and Troubleshooting
PARP1 expression and purification
To ensure proper folding of full-length human PARP1 in bacteria, IPTG-induced expression of PARP1 needs to be conducted at 16°C. As PARP1 is sensitive to proteolysis during purification, freshly prepared PMSF should be added prior to the lysis (Table 1). The French Press apparatus needs to be kept at 4°C before use. Buffers, cell lysates, and the collected supernatants for Ni-NTA affinity chromatography column should be stored on ice.
Problem | Possible cause | Solution |
---|---|---|
Low yield or catalytic activity of PARP1 | Proteolysis during purification | Use freshly prepared PMSF inhibitor, keep all reagents and samples at 4°C, and minimize the time for all purification steps |
Low yield of antibodies | Impurity in the plasmids | Avoid the use of overgrown bacterial culture for maxiprep, ensure complete lysis and neutralization for maxiprep, and sterilize plasmids using 0.2-μm syringe filters before use |
Weak signals on immunoblots after PARP1 auto-modification | Incomplete auto-modification | Increase PARP1 concentration, extend the incubation time to overnight, and ensure 20-min activation time |
Failure of antibody conjugation | Free BCN linkers in the samples | Perform extensive dialysis or buffer exchange to remove free linkers |
Expression and purification of antibodies
The purity of the plasmids used for transient transfection of Expi293F cell is critical for antibody expression in mammalian cells. Make sure to sterilize the plasmids by filtering them through 0.2-μm filters before use. Purified antibodies need to be buffer exchanged against PBS for long-term storage at −80°C.
PARP1 auto-modification
Additions of activated DNA is critical for PARP1 catalytic activity. Concentration of PARP1 is also important for PARP1-catalyzed auto-modification (Table 1). Eight hours or overnight reactions are needed to ensure generation of high levels of functionalized PAR polymers on PARP1. To prevent antibody conjugation with unreacted 3′-azido NAD+ molecules, the reaction mixtures should be buffer exchanged into PBS using 30 kDa molecular weight cut-off centrifugal concentrators following completion of the reactions.
Antibody NHS-BCN linker conjugation
The removal of the unreacted linkers in reactions is critical (Table 1). Excess amounts of unreacted BCN linkers can react with the azide groups on PAR polymers, reducing antibody conjugation efficiency. Make sure to extensively dialyze or buffer exchange the reactions against PBS to remove unreacted linkers.
Generation of bispecific antibodies
The molar ratio of each antibody to PARP1 can be critical to the production of bispecific antibodies. Even though PARylated PARP1 has abundant azido groups for conjugation, a large number of antibody molecules conjugated to each PARylated PARP1 may result in steric hindrance to each other, reducing binding affinity.
Understanding Results
Basic Protocol 1 describes protein expression and purification. Following this protocol, human full-length PARP1, anti-CD3 antibody (UCHT1), and anti-HER2 antibody (Herceptin) are expected to be purified, which can be examined with Coomassie stained SDS-PAGE gels. As shown in Figure 2A, purified full-length human PARP1 migrates slightly below 140 kDa together with two cleaved fragments due to proteolysis (Chaitanya et al., 2010; Gobeil et al., 2001). The two purified antibodies, Herceptin and UCHT1, migrate as intact bands above 150 kDa in the absence of DTT and are reduced to heavy and light chains at 50 kDa and 25 kDa, respectively, in the presence of DTT.
Basic Protocol 2 covers antibody BCN linker conjugation, PARP1 auto-PARylation, and bispecific antibody generation. Following auto-modification reactions with 3′-azido NAD+, the resulting PARylated PARP1 is anticipated to carry functionalized PAR polymers that can be specifically detected by an anti-PAR monoclonal antibody via immunoblots as shown in Figure 2B. The formed PARylated PARP1 feature smeared bands across the regions for a size of ≥100 kDa, while unmodified PARP1 has no detectable signals. The azido-functionalized PAR polymers mediate generation of bispecific antibodies through copper-free click chemistry, resulting in smeared signals at ∼140 kDa and higher as revealed by immunoblots using an anti-human IgG antibody (Fig. 2C). Coomassie stained SDS-PAGE gels also show the generated bispecific antibodies at the size >180 kDa, but little signals for PARylated PARP1, likely lower than the detection threshold of Coomassie stain.
The bispecific antibodies generated from this protocol are expected to display specific binding for T-cell CD3 as well as cancer cell-associated HER2 antigens. As shown in Figure 3, the resulting bispecific antibodies can tightly bind to both HER2-positive HCC1954 breast cancer cells and CD3-positive Jurkat cells but show little binding to MDA-MB-468 cancer cells that lack HER2 and CD3 expression. In vitro cytotoxicity assays indicate that in the presence of non-activated human peripheral blood mononuclear cells (PBMCs), the generated bispecific antibodies induce potent killing of HER2-positive HCC1954 breast cancer cells in a dose-dependent manner (Fig. 4). No significant cytotoxicity is observed for HER2-negative MDA-MB-468 cells treated with human PBMCs and bispecific antibodies.
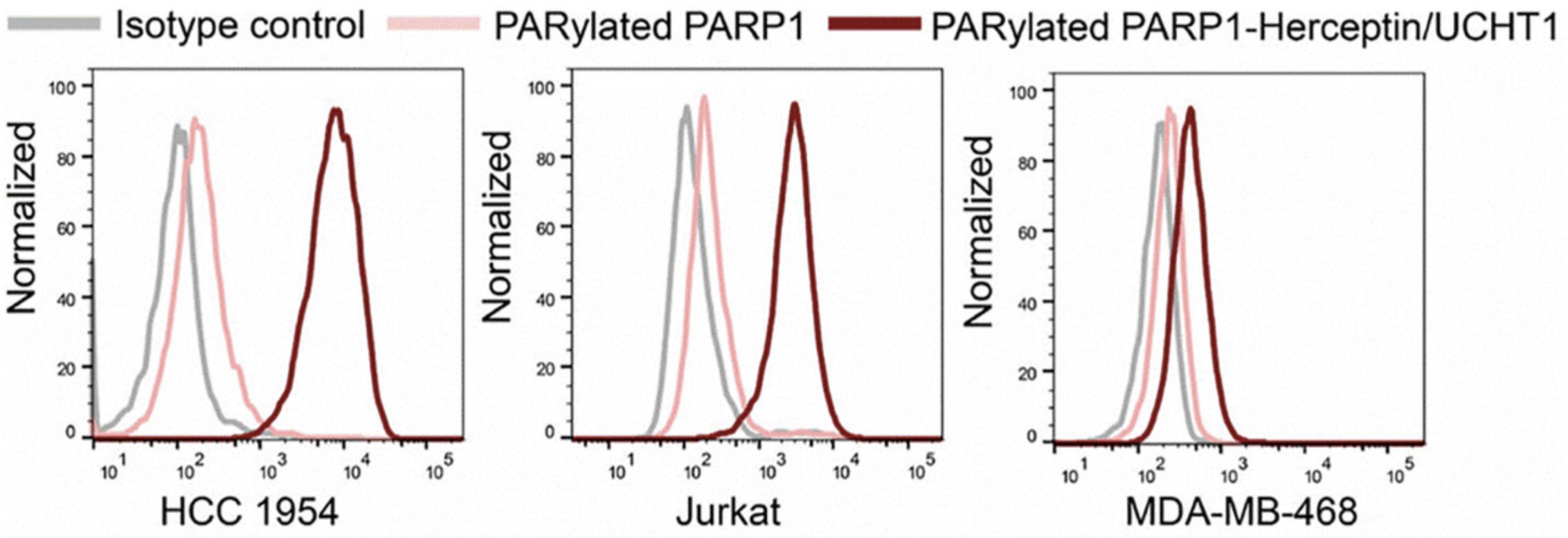
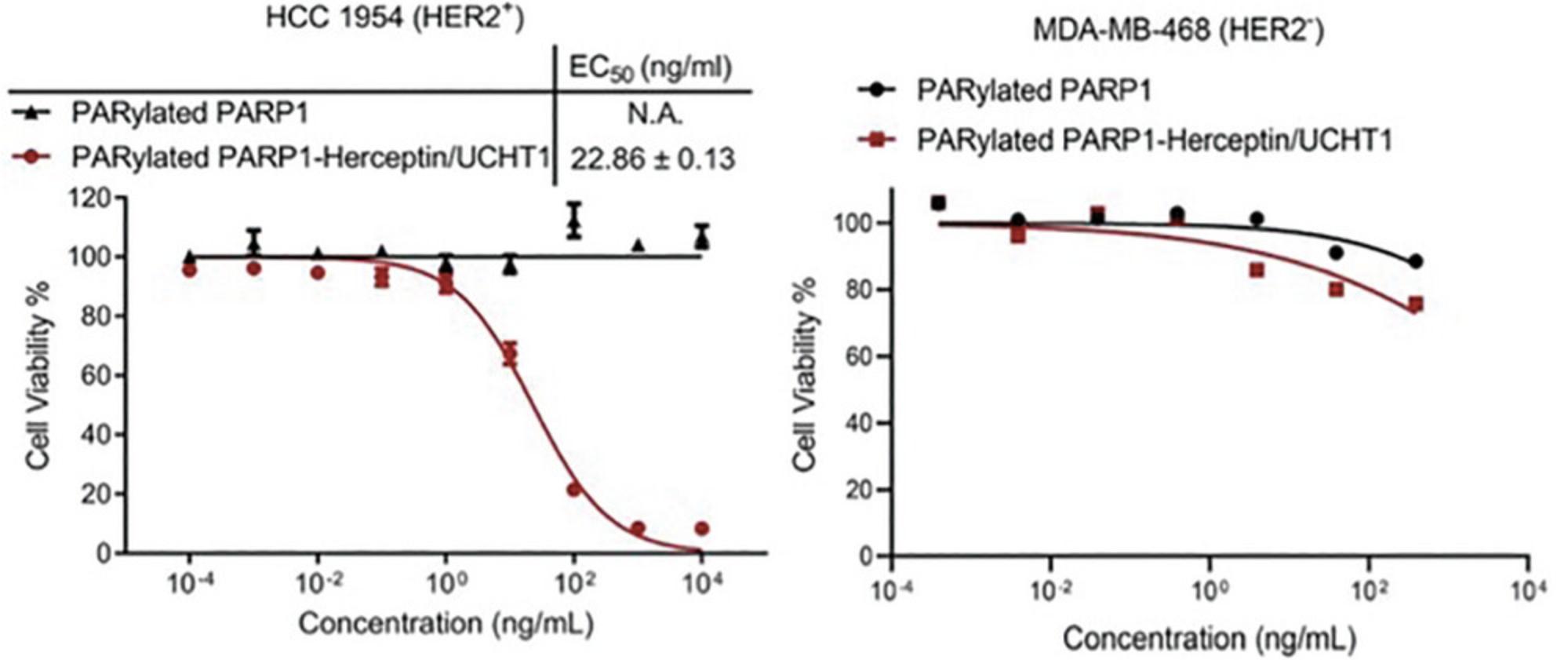
Time Considerations
Bacterial expression of full-length human PARP1 takes 2 days and subsequent three-step chromatographic purification requires 2 to 3 days. Expression of antibody IgG molecules in mammalian cells needs 6 days following transient transfection. To ensure production of antibodies in high yields, it is recommended to use Expi293F cells in passages of 3 to 8, which adds ∼1 week to thaw and culture the cells from frozen stocks. Antibody purification can be completed within 1 to 2 days. Reactions for human PARP1-catalyzed auto-modification are incubated for 8 hr or overnight. Functionalization of antibodies with BCN groups is a 2-hr reaction, followed by a 2-hr buffer exchange process. 3′-azido PARylated PARP1 is then incubated with Herceptin-BCN and UCHT1-BCN for 3 days to generate bispecific antibodies that can be purified by size exclusion chromatography within 4 to 6 hr. In the case that the expression and purification of human PARP1 and the antibody IgGs can be carried out simultaneously, the total time needed to generate bispecific antibodies is anticipated to be ∼3 weeks.
Acknowledgments
This work was supported in part by Sharon L. Cockrell Cancer Research Fund, National Institute of General Medical Sciences (NIGMS) of the National Institutes of Health (NIH) grant R35GM137901 (to Y.Z.), National Institute of Biomedical Imaging and Bioengineering (NIBIB) of the NIH grant R01EB031830 (to Y.Z.), National Cancer Institute (NCI) of NIH grant R01CA276240 (to Y.Z.), and National Institute of Diabetes and Digestive and Kidney Diseases (NIDDK) of the NIH grant P30DK048522 (to USC Research Center for Liver Diseases).
Author Contributions
Hyo Sun Kim : Formal analysis; investigation; writing original draft. Yong Zhang : Conceptualization; formal analysis; funding acquisition; project administration; supervision; writing review and editing.
Conflict of Interest
The authors declare no competing interests to disclose.
Open Research
Data Availability Statement
The data that support the findings of this study are available from the corresponding author upon reasonable request.
Literature Cited
- Beck, C., Robert, I., Reina-San-Martin, B., Schreiber, V., & Dantzer, F. (2014). Poly(ADP-ribose) polymerases in double-strand break repair: Focus on PARP1, PARP2 and PARP3. Experimental Cell Research , 329(1), 18–25. https://doi.org/10.1016/j.yexcr.2014.07.003
- Bianchi, A. R., Ferreri, C., Ruggiero, S., Deplano, S., Sunda, V., Galloro, G., Formisano, C., & Mennella, M. R. (2016). Automodification of PARP and fatty acid-based membrane lipidome as a promising integrated biomarker panel in molecular medicine. Biomarkers in Medicine , 10(3), 229–242. https://doi.org/10.2217/bmm.16.3
- Brinkmann, U., & Kontermann, R. E. (2017). The making of bispecific antibodies. MAbs , 9(2), 182–212. https://doi.org/10.1080/19420862.2016.1268307
- Chaitanya, G. V., Alexander, J. S., & Babu, P. P. (2010). PARP-1 cleavage fragments: Signatures of cell-death proteases in neurodegeneration. Cell Communication and Signaling , 8(1), 31–31. https://doi.org/10.1186/1478-811X-8-31
- Cheng, Q., Zhang, X.-N., Li, J., Chen, J., Wang, Y., & Zhang, Y. (2023). Synthesis of bispecific antibody conjugates using functionalized poly-ADP-ribose polymers. Biochemistry , 62(6), 1138–1144. https://doi.org/10.1021/acs.biochem.2c00718
- Cheng, Q., Zhang, X.-N., Zhang, L., Chen, J., Wang, Y., & Zhang, Y. (2022). A poly-ADP-ribose polymer–GCSF conjugate. Biomacromolecules , 23(12), 5267–5272. https://doi.org/10.1021/acs.biomac.2c01090
- Current Protocols. (2006). Commonly Used Reagents. Current Protocols in Microbiology , 00, A.2A.1–A.2A.15. https://doi.org/10.1002/9780471729259.mca02as00
- De Vos, M., Schreiber, V., & Dantzer, F. (2012). The diverse roles and clinical relevance of PARPs in DNA damage repair: Current state of the art. Biochemical Pharmacology , 84(2), 137–146. https://doi.org/10.1016/j.bcp.2012.03.018
- Dimasi, N., Kumar, A., & Gao, C. (2021). Generation of bispecific antibodies using chemical conjugation methods. Drug Discovery Today. Technologies , 40, 13–24. https://doi.org/10.1016/j.ddtec.2021.08.006
- Esfandiari, A., Cassidy, S., & Webster, R. M. (2022). Bispecific antibodies in oncology. Nature Reviews Drug Discovery , 21(6), 411–412.
- Gallagher, S. R. (2008). SDS-Polyacrylamide Gel Electrophoresis (SDS-PAGE). Current Protocols Essential Laboratory Techniques , 00(1), 7.3.1–7.3.25. https://doi.org/10.1002/9780470089941.et0703s00
- Gibson, B. A., Zhang, Y., Jiang, H., Hussey, K. M., Shrimp, J. H., Lin, H., Schwede, F., Yu, Y., & Kraus, W. L. (2016). Chemical genetic discovery of PARP targets reveals a role for PARP-1 in transcription elongation. Science (New York, N.Y.) , 353(6294), 45–50. https://doi.org/10.1126/science.aaf7865
- Gobeil, S., Boucher, C. C., Nadeau, D., & Poirier, G. G. (2001). Characterization of the necrotic cleavage of poly (ADP-ribose) polymerase (PARP-1): Implication of lysosomal proteases. Cell Death and Differentiation , 8(6), 588–594. https://doi.org/10.1038/sj.cdd.4400851
- Jeggo, P. A. (1998). DNA repair: PARP – another guardian angel? Current Biology , 8(2), R49–R51. https://doi.org/10.1016/S0960-9822(98)70032-6
- Klausner, A. (1987). ‘Quadromas’ yield bispecific antibodies. Bio/Technology , 5(3), 195–196. https://doi.org/10.1038/nbt0387-195
- Krishnamurthy, A., & Jimeno, A. (2018). Bispecific antibodies for cancer therapy: A review. Pharmacology & Therapeutics, 185, 122–134.
- Lam, A. T., Zhang, X. N., Courouble, V. V., Strutzenberg, T. S., Pei, H., Stiles, B. L., Louie, S. G., Griffin, P. R., & Zhang, Y. (2021). A Bifunctional NAD(+) for Profiling Poly-ADP-Ribosylation-Dependent Interacting Proteins. ACS Chemical Biology , 16(2), 389–396. https://doi.org/10.1021/acschembio.0c00937
- Li, H., Er Saw, P., & Song, E. (2020). Challenges and strategies for next-generation bispecific antibody-based antitumor therapeutics. Cellular & Molecular Immunology, 17(5), 451–461. https://doi.org/10.1038/s41423-020-0417-8
- Lindahl, T., Satoh, M. S., Poirier, G. G., & Klungland, A. (1995). Post-translational modification of poly(ADP-ribose) polymerase induced by DNA strand breaks. Trends in Biochemical Sciences , 20(10), 405–411. https://doi.org/10.1016/S0968-0004(00)89089-1
- Mendoza-Alvarez, H., & Alvarez-Gonzalez, R. (1993). Poly(ADP-ribose) polymerase is a catalytic dimer and the automodification reaction is intermolecular. The Journal of Biological Chemistry , 268(30), 22575–22580. https://doi.org/10.1016/S0021-9258(18)41568-2
- Morales, J., Li, L., Fattah, F. J., Dong, Y., Bey, E. A., Patel, M., Gao, J., & Boothman, D. A. (2014). Review of poly (ADP-ribose) polymerase (PARP) mechanisms of action and rationale for targeting in cancer and other diseases. Critical Reviews in Eukaryotic Gene Expression , 24(1), 15–28. https://doi.org/10.1615/CritRevEukaryotGeneExpr.2013006875
- Ni, D., Xu, P., Sabanayagam, D., & Gallagher, S. R. (2016). Protein Blotting: Immunoblotting. Current Protocols Essential Laboratory Techniques , 12(1), 8.3.1–8.3.40. https://doi.org/10.1002/9780470089941.et0803s12
- Shi, X., Zhang, X. N., Chen, J., Cheng, Q., Pei, H., Louie, S. G., & Zhang, Y. (2020). A poly-ADP-ribose polymer-based antibody-drug conjugate. Chemical Science , 11(34), 9303–9308. https://doi.org/10.1039/d0sc01795g
- Tan, E. S., Krukenberg, K. A., & Mitchison, T. J. (2012). Large-scale preparation and characterization of poly(ADP-ribose) and defined length polymers. Analytical Biochemistry , 428(2), 126–136. https://doi.org/10.1016/j.ab.2012.06.015
- Wei, J., Yang, Y., Wang, G., & Liu, M. (2022). Current landscape and future directions of bispecific antibodies in cancer immunotherapy. Frontiers in Immunology , 13, 1035276–1035276. https://doi.org/10.3389/fimmu.2022.1035276
- Yang, F., Wen, W., & Qin, W. (2017). Bispecific antibodies as a development platform for new concepts and treatment strategies. International Journal of Molecular Sciences , 18(1), 48–48. https://doi.org/10.3390/ijms18010048
- Zhang, X. N., Cheng, Q., Chen, J., Lam, A. T., Lu, Y., Dai, Z., Pei, H., Evdokimov, N. M., Louie, S. G., & Zhang, Y. (2019). A ribose-functionalized NAD(+) with unexpected high activity and selectivity for protein poly-ADP-ribosylation. Nature Communications , 10(1), 4196. https://doi.org/10.1038/s41467-019-12215-4
- Zhang, X. N., Lam, A. T., Cheng, Q., Courouble, V. V., Strutzenberg, T. S., Li, J., Wang, Y., Pei, H., Stiles, B. L., Louie, S. G., Griffin, P. R., & Zhang, Y. (2022). Discovery of an NAD(+) analogue with enhanced specificity for PARP1. Chemical Science , 13(7), 1982–1991. https://doi.org/10.1039/d1sc06256e