From Hair to iPSCs—A Guide on How to Reprogram Keratinocytes and Why
Stefanie Klingenstein, Stefanie Klingenstein, Moritz Klingenstein, Moritz Klingenstein, Alexander Kleger, Alexander Kleger, Stefan Liebau, Stefan Liebau
Abstract
Keratinocytes, as a primary somatic cell source, offer exceptional advantages compared to fibroblasts, which are commonly used for reprogramming. Keratinocytes can beat fibroblasts in reprogramming efficiency and reprogramming time and, in addition, can be easily and non-invasively harvested from human hair roots. However, there is still much to know about acquiring keratinocytes and maintaining them in cell culture. In this article, we want to offer readers the profound knowledge that we have gained since our initial use of keratinocytes for reprogramming more than 10 years ago. Here, all hints and tricks, from plucking the hair roots to growing and maintaining keratinocytes, are described in detail. Additionally, an overview of the currently used reprogramming methods, viral and non-viral, is included, with a special focus on their applicability to keratinocytes. This overview is intended to provide a brief but comprehensive insight into the field of keratinocytes and their use for reprogramming into induced pluripotent stem cells (iPSCs). © 2020 The Authors.
INTRODUCTION
Reprogramming somatic cells into induced pluripotent stem cells (iPSCs) is a widely used technique in molecular biology labs. This approach has opened up a huge field of research with unlimited application possibilities. For the successful generation of iPSCs, the selection of the proper primary cell source is a crucial task. Since the first publication (Takahashi et al., 2007) reporting the preparation of iPSCs from human skin fibroblasts in 2006 by means of the transduction of four reprogramming factors (Oct3/4, Sox2, Klf4, c-Myc) in the Yamanaka lab (rewarded with the Nobel prize for Yamanaka and Takahashi), countless publications have arisen using primary cell sources other than fibroblasts. These have included peripheral blood−derived mononuclear cells and sheared cells from urine, as well as more exotic primary cell sources like third molar teeth, milk teeth, umbilical cord blood, and even post-mitotic primary neurons, all of which have been reprogrammed to human iPSCs. This article will highlight keratinocytes as a primary cell line.
Human hair−derived keratinocytes could be of major interest in the field of starting material for reprogramming in view of their numerous advantages in comparison to other cell types. Plucking hair is non-invasive, no medical personnel are needed, and hair samples can be obtained from almost any volunteer or patient. Moreover, plucked hair can be shipped at room temperature in simple media in which it can be kept for days. Although several publications are available applying keratinocytes for reprogramming (Aasen et al., 2008; Re et al., 2018), this cell source is still uncommon.
This article will describe the technique of plucking and transporting hair in detail. In addition, we provide an overview of the commercially available culture media and coatings specifically developed for keratinocytes, and explain how to deal with keratinocytes in routine laboratory work. Furthermore, the currently used reprogramming techniques are compared, and their advantages and disadvantages when using keratinocytes are discussed. For more insight into successfully carrying out procedures like transportation, cultivation, and reprogramming, tips and tricks are added to the protocols. With this guidance, it should be possible to immediately enter into the field of reprogramming human hair−derived keratinocytes successfully into iPSCs.
WHY USE HAIR?
Before we go into every step of the procedure in depth, we should discuss why to use hair in the first place. There are many advantages, but also some obstacles to overcome.
Not all available reprogramming methods can be applied to primary keratinocytes. Most of the media commonly used to dilute viruses or vectors to be transferred to somatic cells are incompatible with the requirements of keratinocytes. Here, the high calcium sensitivity of keratinocytes plays an important and crucial role. Keratinocytes should be cultivated in a low-calcium medium because they do not tolerate high concentrations of CaCl2, which will cause them to differentiate and no longer be reprogrammable. In addition, reprogramming with mRNA remains difficult, as the mRNA has to be present for several passages and keratinocytes are likely to go into senescence before the pluripotent state is reached. However, knowing these drawbacks, there are many ways to reprogram keratinocytes successfully.
The most convincing advantages are the availability of hair (from people of all ages) and the non-invasive and nearly painless harvesting procedure that involves no long-term consequences. Additionally, for the action of plucking hair, no medical personnel are needed, and this can therefore be done anywhere (Raab, Klingenstein, Liebau, & Linta, 2014).
Furthermore, the choice of keratinocytes over fibroblasts results in an impressively high reprogramming efficiency. Reprogramming of primary keratinocytes from children has shown 100-fold higher efficiency compared to fibroblasts (Aasen et al., 2008; Linta et al., 2012). A possible mechanism may involve the high level of hypermethylated CpG islands, which are not found in fibroblasts; in this respect keratinocyte-derived iPSCs are more similar to ESCs than fibroblast-derived iPSCs (Barrero et al., 2012). The reprogramming time is also faster in comparison to fibroblasts (Piao, Hung, Lim, Wong, & Ko, 2014).
These advantages show that keratinocytes as a primary cell line offer a very simple and easy-to-use technique even for scientists who are new to this field.
ACQUISITION OF HAIR SAMPLES
Plucking hair
One of the huge advantages of using keratinocytes for reprogramming is the simple acquisition of hair from donors. The plucking of hair is relatively easy, and by far not as painful and invasive compared to other methods such as skin biopsies used to obtain fibroblasts. Even though the method is quite simple, there are still some aspects requiring attention.
Before plucking hair from volunteers or patients, gloves and sterilized tweezers are mandatory to minimize the contamination risk. Although it is possible to use hair from almost any region of the body, scalp hair from the top and back of the subject's head works best. Nevertheless, beard hair has showed good outcomes in our hands, but plucking from this region is much more painful for the person. The most important aspect is the awareness of “good” and “bad” hair roots (see Supporting Information video). Perfect roots are in the anagen phase of growing and have a visible white outer root sheath (ORS) (Raab et al., 2014). Catagen or telogen hair roots should not be used, as the hair is in an inactive state and does not grow; thus, no keratinocytes can be isolated (Hung, Pebay, & Wong, 2015) (Fig. 1).
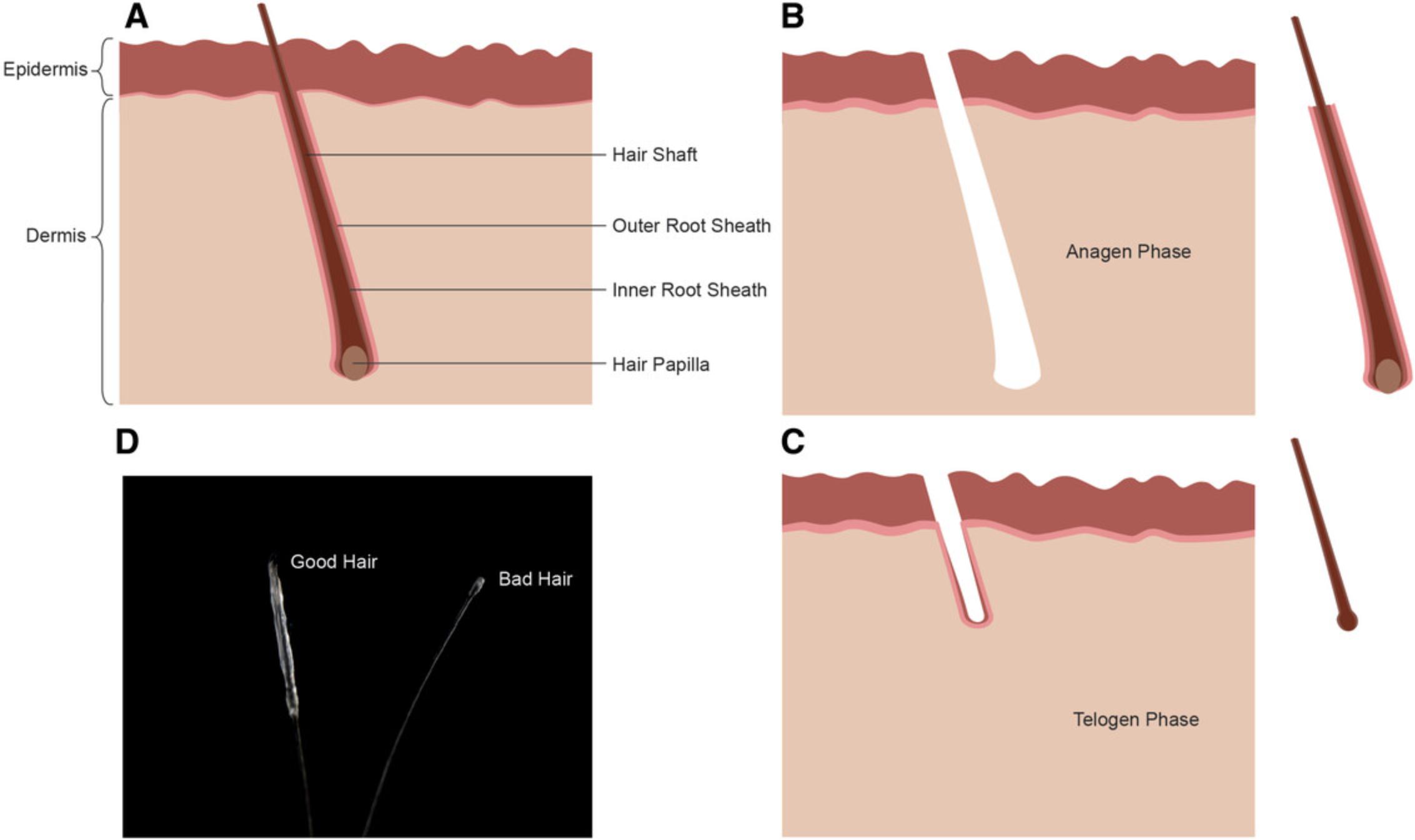
Troubleshooting Tip 1: Pull fast. One should not be too cautious when extracting hair roots; otherwise, the outer root sheath may remain in the skin or be partially destroyed.
Transport of plucked hair
Work quickly to put the hair root into the medium. If the hair is exposed to the air for too long, it can wither to the degree of uselessness. Be sure to completely cover the hair with medium. It is advisable that the chosen transport vessel (e.g., 50-ml Falcon tube) be completely filled with medium (see Supporting Information video). Use of antibiotics and antimycotics in the medium is not obligatory, but can minimize contamination. The vessels can be stored and shipped with the hair over time periods up to 48 hr (best timeframe) or even longer at room temperature. Best results can be achieved when the hair roots are plated in the cell culture as quickly as possible.
Troubleshooting Tip 2: DMEM with or without serum is mostly used as a transport medium.
KERATINOCYTES IN CULTURE
Cultivation media and supplements
A high calcium concentration is necessary for physiological keratinocyte differentiation (Bikle, Xie, & Tu, 2012). In healthy skin development, keratinocytes arise from the stratum basale, a basal layer in the epidermis of the skin. In this microenvironment, a very low calcium concentration is present, which allows the keratinocytes to proliferate. Due to the ongoing differentiation, the cells migrate through increasing ion concentrations toward the outer epidermal barrier, where a very high calcium concentration is present (Elsholz, Harteneck, Muller, & Friedland, 2014). This leads directly to the most important piece of information about the cultivation of keratinocytes in vitro: that a low-CaCl2 medium should be used.
Several companies offer special media for cultivation of keratinocytes. What most media have in common is a defined concentration of 0.06 mM CaCl2 in the prepared medium. In addition to the calcium-reduced basal medium, keratinocytes also require supplements that, depending on the manufacturer, have already been added to the basic medium or must be added separately by the user. Manufacturers of the basal media provide corresponding supplements at standardized concentrations, but be aware of possible variations in the concentrations between different companies.
One such supplement is bovine pituitary extract (BPE). Some special formulations, for more defined media, are available without BPE. BPE is derived from the bovine pituitary gland and comprises different types of growth hormones and mitogens, such as TSH, FSH, ACTH, and GH. These hormones are responsible for growth and for the maintenance of various body functions, like the development of sex hormones (Kent & Bomser, 2003). In addition to BPE, glucocorticoids like hydrocortisone and growth factors are added. Together with insulin and transferrin, they regulate various signaling pathways, e.g., stress response, energy mobilization, and regulation of cell proliferation and differentiation (Keenan, Pearson, O'Driscoll, Gammell, & Clynes, 2006; Shen et al., 2001). The addition of antibiotics to primary cell lines is not necessary, and in some cases even inhibits the outgrowth of keratinocytes (Nygaard et al., 2015).
Plating human plucked hair
To cultivate the plucked hair roots, a crucial preparation step needs to be followed thoroughly. For this purpose, the hair shaft is cut, slightly above the visible ORS. Only the part with the ORS will be used. This reduces the shear forces when changing medium; the hair stays anchored in its position in the coating medium and the keratinocytes can grow out more easily. Subsequently, the cut hair is placed directly on coating medium (see Supporting Information video). Commonly, Matrigel or collagen I is used for coating (Ernst et al., 2013).
Troubleshooting Tip 3: In order to improve the attachment of the hair to the coating, it is advisable to place a drop of the coating medium directly onto the hair root. This has the additional benefit that the hair roots do not dry out and that they stay in place.
Troubleshooting Tip 4: If this method is unsuccessful, the cells of the outer root sheath can be removed by an enzymatic digestion step prior to the plating step. For this purpose, the hair is predigested with enzymes, usually dispase, collagenase, or trypsin-EDTA (for more detail on digestion of outer hair roots, see Chan, Fan, Wang, Mu, & Lin, 2015; Ernst et al. , 2013; Re et al. , 2018; Wagner et al. , 2018 ). The isolated cells are plated onto the coated surface.
As already mentioned, the next step would be carefully adding a low-calcium medium with the appropriate supplementation for the keratinocytes.
Troubleshooting Tip 5: Do not add the medium until the hair roots are firmly fixed to the coating material (approximately after 1-2 hr); otherwise, the roots will detach after adding medium.
Depending on the hair donor, the first keratinocytes will grow out after 1-2 days. However, it is also possible that the first cells will appear after 1 week or later (Fig. 2).
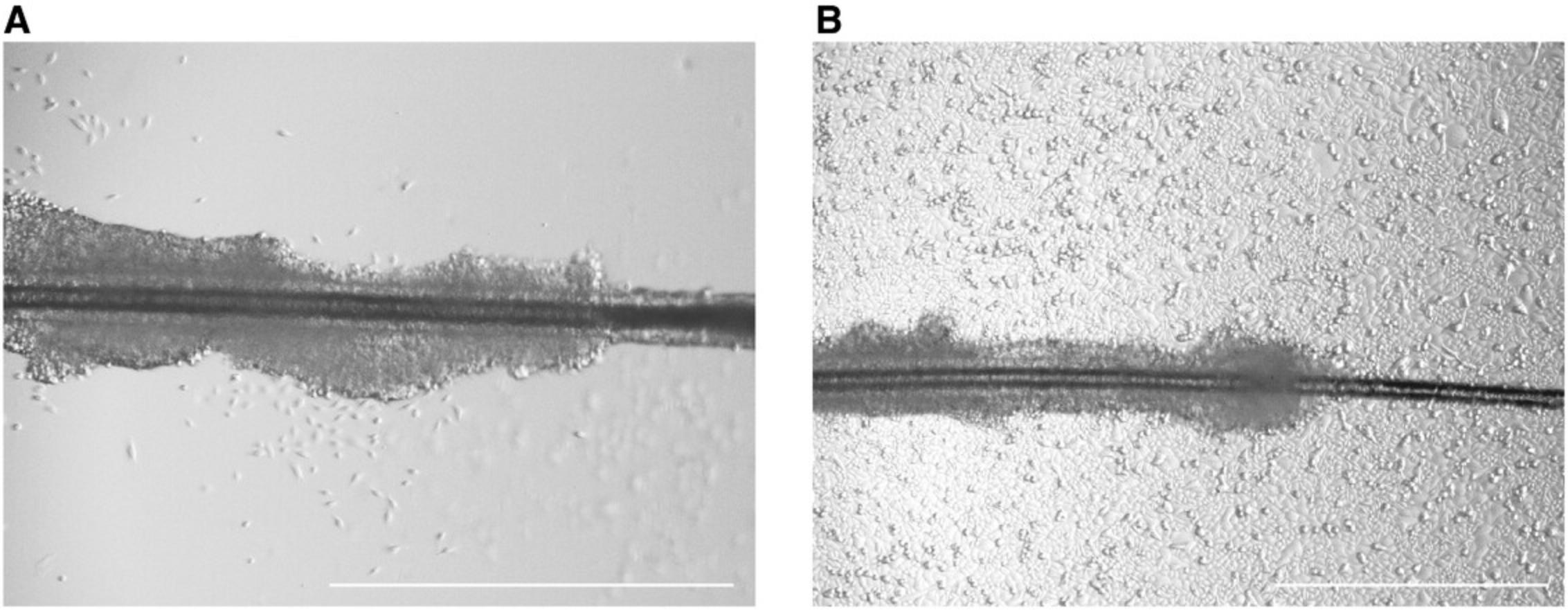
Troubleshooting Tip 6: For enhanced outgrowth stimulation, the freshly plated hair roots may be initially cultivated in conditioned MEF medium. This conditioned medium contains cytokines and growth factors that are secreted into the culture medium by mouse embryonic fibroblast (MEF) cells. As soon as the first keratinocytes are fully grown out, switch to a keratinocyte-specific defined medium.
Troubleshooting Tip 7: The hair roots are usually cultivated in well format, but cultivation in T-25 flasks offers a big advantage, because flasks undergo much lower evaporation compared to well format. As a positive effect, the hair roots will not dry out as easily. Use three to five hair roots per T-25 flask to achieve the best results
Passaging and cryopreservation
If the culture has reached around 70% confluence, cells must be passaged. For this purpose, they are detached enzymatically from the coating surface using TrypLE Express and passaged onto new coated wells in the appropriate culture medium. Since the primary keratinocytes mature directly from the hair roots, they only have a short passaging capacity until they fully differentiate and are no longer able to proliferate. They usually reach this stage after four to five passages. Of note, most primary cells should not be passaged repeatedly (meaning not more than four to five passages for keratinocytes; may differ for other primary cells), as chromosomal aberrations can occur!
Troubleshooting Tip 8: A very early passage should be used for the subsequent reprogramming, since at this early stage the keratinocytes still proliferate and are easy to infect. It is recommended to start the reprogramming process with passage 1, but it is possible to achieve results with passage-2 or -3 keratinocytes. After that, most keratinocytes are fully differentiated and will no longer proliferate properly.
If the cells are to be used at a later time point, they can be easily cryopreserved. For this purpose, the keratinocytes are detached enzymatically from the coating surface using TrypLE Express and taken up in a freezing medium specially produced for keratinocytes. The cells can easily be stored in liquid nitrogen and thawed again when required. Keratinocyte-specific culture medium with 20% DMSO can also be used for cryopreservation.
REPROGRAMMING
Reprogramming is the molecular technique to restore nearly any fully differentiated cell to the state of pluripotency. Pluripotent cells have the capability to differentiate into all cells of the three germ layers: ectoderm, mesoderm, and endoderm.
To achieve this state of pluripotency, reprogramming factors, e.g., Oct4, Sox2, and Klf4, among others, have to be transferred into the primary, somatic cell lines. After several days of reprogramming, the molecular composition of the nucleus rearranges and the cells return to an embryonic, initial state. There are several techniques, viral and non-viral, to induce pluripotency in somatic cells. In the following chapter, these methods are described briefly, and pros and cons are listed.
Which virus to choose for reprogramming?
Retrovirus
In the first publication describing the successful reprogramming of human foreskin fibroblasts (HFF) to iPSCs, retrovirus particles were used (Takahashi et al., 2007). Retroviruses are single-stranded RNA viruses, and infect only dividing cells (Hotta & Ellis, 2008). The major advantage of retroviral transfection is the high reprogramming efficiency. However, retroviruses have the ability to integrate permanently into the genome of the host cell. Uncontrolled viral genome integration can cause mutagenesis and genome instability (Nakagawa et al., 2008), reducing the applicability of retroviruses for, e.g., potential transplant attempts, and they are therefore not the first choice in the current field of research.
Advantages (+) and disadvantages (−) of retrovirus :
+ High reprogramming efficiency + Cloning of large DNA sequences − Stable integration into host genome − No transplantation approaches possible
Lentivirus
Lentiviruses belong, together with adenoviruses, to the first generation of reprogramming viruses. They are single-stranded RNA viruses, which make up a genus among the retrovirus family. This kind of virus can stay lifelong in the host genome due to the ability to bypass the defense mechanisms of the immune system. They transduce dividing and non-dividing eukaryotic cells (Fleury et al., 2003). Lentiviral infection is commonly performed with a commercially available single constitutive polycistronic vector containing all four reprogramming factors (e.g., STEMCCA-OKSM; Sommer et al., 2009). Here, the advantage is the use of one lentiviral vector, which improves the reprogramming efficiency, instead of using a pool of multiple retroviruses. The integrated virus RNA can be eliminated by Cre-enzymatic treatment.
Advantages (+) and disadvantages (−) of lentivirus :
+ High reprogramming efficiency + Only one virus is needed − Stable integration into host genome, but excisable − No transplantation approaches possible; not safe enough
Sendaivirus
After further optimization steps, negative-sense, non-integrating RNA viruses are currently being used. Sendaiviruses (SeV) show a high reprogramming efficiency and do not (or should not) integrate into the host genome by entering the nucleus (Bernloehr et al., 2004). During passaging of the generated iPSCs, the virus will be diluted out of the cells at around 10-20 passages after infection (Fusaki, Ban, Nishiyama, Saeki, & Hasegawa, 2009).
Advantages (+) and disadvantages (−) of sendaivirus :
+ High reprogramming efficiency + No integration into host genome (or at very low chance) + Little technical effort − Expensive
Non-viral reprogramming methods
mRNA
A virus-independent method is the use of modified mRNA. However, a time-consuming approach, with multiple successive infections of the primary cell line, is necessary for successful reprogramming. This entails a high level of personnel effort and expense. However, the generated iPSCs are transgene-free and have no obvious risk of mutation (Badieyan & Evans, 2019). One publication describing the use of mRNA for the reprogramming of keratinocytes observed high dose-dependent cytotoxicity in their experimental setup, which was a serious complication for achieving a stable status of pluripotency. In addition, the use of feeder cells was crucial to this procedure (Warren et al., 2010). For other cell types, this method has been optimized with regard to reducing the number of infections needed, eliminating feeder culture, and shortening the timeframe for the whole procedure. Even if this method is not recommended for keratinocytes at this time, it can become a possible alternative after further improvements and adjustments.
Advantages (+) and disadvantages (−) of mRNA :
+ Applicable in clinical research + Rapid elimination of residual mRNA − Low reprogramming efficiency − Daily transfections of four different mRNAs, 14 days − Currently not recommended for keratinocytes
Episomal DNA
In the second generation of reprogramming techniques, viruses are replaced by small circular DNA to introduce the reprogramming factors. Non-integrating (or partially integrating) episomal DNA plasmids encode the reprogramming factors. In this case, episomal means that the DNA is present in the cytoplasm and, in principle, is not built into the host genome. Since the genetic information for reprogramming also remains in the cytoplasm with this approach, it disappears from the iPSCs over the course of cell division. However, studies have shown that fragments of the episomal vectors are recognized as part of the cell's own DNA and incorporated into the genome (Okita, Nakagawa, Hyenjong, Ichisaka, &Yamanaka 2008; Yu et al., 2009; Schlaeger et al., 2015).
Advantages (+) and disadvantages (−) of episomal DNA plasmid :
+ No need for virus + Applicable in clinical research + Not expensive + Little technical effort − Partial integration into host genome
Synthetic self-replicating RNA
Synthetic self-replicating RNA molecules offer further possibilities for optimization in reprogramming techniques. The commercially available single-stranded RNA possesses the four reprogramming factors and leads to a very high reprogramming efficiency with only one transfection. It is a virus-free, polycistronic RNA replicon that combines all four factors in one RNA strand (Steinle et al., 2019). In addition, the RNA molecules show no integration into the host genome and the remaining molecules can be removed from the cell culture medium simply by removing the interferon γ (IFN-γ) inhibitor, B18R (Kim et al., 2017). Applicability for keratinocytes is currently tested in our lab.
Advantages (+) and disadvantages (−) of RNA replicon :
+ 1 day of infection + High reprogramming efficiency + No integration into host genome − Established for only few primary cell lines − Not possible?/not yet published for keratinocytes
Reprogramming methods for keratinocytes
Since the discovery of the reprogramming mechanism, several approaches have appeared for restoring primary cell lines to the status of pluripotent stem cells. The first experiments to transfer the pluripotency factors into adult primary cell lines were performed using retroviral transduction. The results showed a high reprogramming efficiency; however, the virus genome remained in the host cell, rendering the generated cells unusable for possible clinical applications such as transplantation attempts. In the following generations of virus-based reprogramming methods, more secure systems such as the use of Sendai viruses were established. Additionally, non-virus-based systems were developed to reprogram the somatic cells. Use of episomal DNA and of self-replicating RNA are currently the most promising methods, and also enable possible use of the generated pluripotent cells and their differentiation products in clinical studies.
Troubleshooting Tip 8: Not all methods can be used for reprogramming keratinocytes due to the calcium sensitivity and the short passaging ability of these cells. Table 1 lists the reprogramming methods currently suitable for keratinocytes, together with example publications.
Reprogramming method | Publication |
---|---|
Retrovirus | Lim et al. (2016) |
Lentivirus | Raab et al. (2017) |
Sendai virus | Re et al. (2018) |
Episomal DNA plasmid | Hung et al. (2015) |
KERATINOCYTE CULTURE AND REPROGRAMMING TECHNIQUE USED IN OUR LAB
Materials
Cell culture media:
Transport medium for plucked hair
DMEM (Thermo Fisher # 41965-029) 1% Antibiotic-Antimycotic (Thermo Fisher # 15240-062)
Medium for keratinocyte preparation
1:10 diluted Matrigel (Corning, cat. no. 354234) in EpiLife (Thermo Fisher, cat. no. M-EPI-500-CA) for coating 1:5 diluted Matrigel (Corning, cat. no. 354234) in EpiLife (Thermo Fisher, cat. no. M-EPI-500-CA) for single droplets
Keratinocyte medium
EpiLife (Thermo Fisher, cat. no. M-EPI-500-CA) 1% Human Keratinocyte Growth Supplement (Thermo Fisher, cat. no. S-001-5) 10 nM Y-27632 2HCl (Selleckchem, cat. no. S1049)
Keratinocyte freezing medium
Cryostem (Biological Industries, cat. no. 05-710-1E)
Mouse fibroblast medium
DMEM (Thermo Fisher, cat. no. 41965-039) 10% fetal bovine serum (FBS; Thermo Fisher, cat. no. 10500-064) 1% Antibiotic-Antimycotic (Thermo Fisher, cat. no. 15240-062) 1% GlutaMAX™ (Thermo Fisher, cat. no. 35050-038) 1% Non-essential amino acids solution (Thermo Fisher, cat. no. 11140-050)
Reprogramming medium
Knockout DMEM (Thermo Fisher, cat. no. 10829-018) 20% Knockout Serum Replacement (Thermo Fisher, cat. no. 10828-028) 1% GlutaMAX (Thermo Fisher, cat. no. 35050-038) 1% Non-essential amino acids solution (Thermo Fisher, cat. no. 11140-050) 1% Antibiotic-Antimycotic (Thermo Fisher, cat. no. 15240-062) 50 mM β-mercaptoethanol (Thermo Fisher, cat. no. 31350-010) 50 μg/ml ascorbic acid (Carl Roth, cat. no. 3525.1) 10 nM Y-27632-2HCl (Selleckchem, cat. no. S1049) 10 ng/ml FGF2 (Cell Guidance Systems, cat. no. GFH146)
iPSC Medium
PeproGrowth hESC medium (PeproTech, cat. no. BM-HESC-500) 1:100 Antibiotic-Antimycotic (Thermo Fisher, cat. no. 15240-062)
Chemicals:
Dispase 5 U/ml (Stem Cell technologies, cat. no. 07913) TrypLE Express (Thermo Fisher, cat. no. 12604039) Collagen IV (Sigma, cat. no. 9007-34-5) iPSC Matrigel (Corning, cat. no. 354277) Hexadimethrine bromide (Merck, cat. no. H9268-5G) Polyethylenimine, 25 kDa (Merck, cat. no. 9002-98-6) Lenti-X Concentrator (Takara Clontech, cat. no. 631232)
Plasticware, cells and specific equipment:
Cell culture dish, 10 cm Cell culture flask, 25 cm2 Cell culture 6-well plates Lenti-X 293T (Takara, cat. no. 632180) CD1 E14.5 (Stem cell Technologies, cat. no. 00322) Tweezers (F.S.T., cat. no. 11000-16) Scissors (F.S.T., cat. no. 15100-09)
Vectors used for viral production and reprogramming:
psPAX2 (Addgene, Cambridge, USA) pMD2.6 (Addgene, Cambridge, USA) pRRL.PPT.SF.hOKSMco.idTom.pre FRT (Warlich et al., 2011)
Methods
Preparation and cultivation of keratinocytes
As previously described (see ”Plucking hair” under Acquisition of Hair Samples and the Supporting Information video), human hair is plucked from top or back of the subject's head with large tweezers. Visible hair roots are transferred quickly into a 50-ml Falcon conical tube completely filled with DMEM, preferably containing antibiotics, and transported at room temperature into the lab. The transport time should not exceed 3 days. In the lab, the hair follicles are placed in a petri dish with DMEM, and the roots are cut with small scissors directly above the visible white outer root sheath while holding the hair shaft with tweezers. The short hair roots remain in DMEM until the T-25 flasks are prepared. The T-25 flask is coated for 1 hr with 1:10 diluted Matrigel. After that, a drop of 1:5 diluted Matrigel is added on top of the prior coating and the hair root is inserted into this drop for 1-2 hr in a humidified incubator. 1 ml of conditioned MEF medium has to be added, and changed every day until first keratinocytes appear. From now on, 2 ml of the keratinocyte medium EpiLife is added every second day. Keratinocytes can either be frozen or directly used for further reprogramming (Fig. 3).
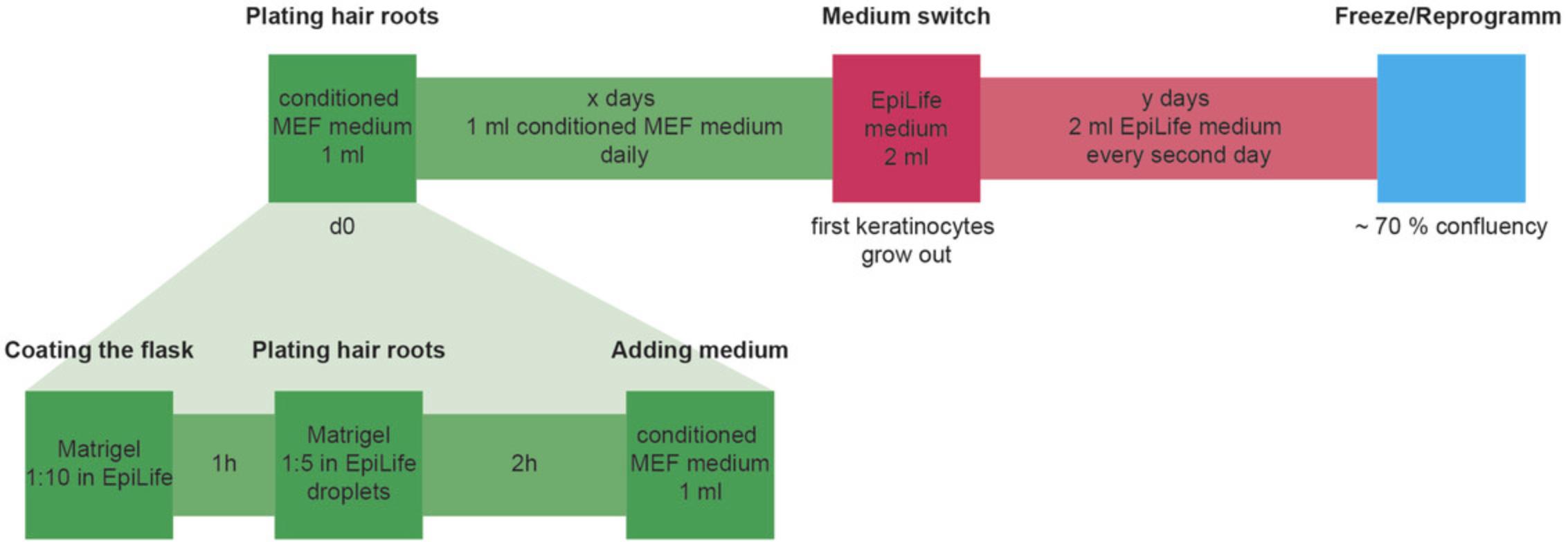
Preparation of conditioned MEF medium
CD1 E14.5 mouse fibroblast cells are cultivated in mouse fibroblast medium under humidified conditions. At 2 and 4 days after passaging the cells, the spent medium will be collected and filtered. The freshly prepared medium has to be enriched with 5 ng/ml FGF2, 10 nM Y-27632-2HCl, and 50 μg/ml ascorbic acid.
Production of viral particles
Viral particles can be obtained from Lenti-X 293T cells. Therefore, the cells are transfected for 4 hr in serum-free MEF medium with 8 µg lentiviral pRRL.PPT.SF.hOKSMco.idTom.pre FRT plasmid (Warlich et al., 2011) together with 5.5 µg psPAX2 and 2 µg pMD2.6 plasmid using polyethylenimine. After changing to serum-containing MEF medium, the supernatant can be collected 2 and 4 days after transfection. To concentrate the viral particles, a viral concentrator must be used according to the manufacturer's protocol. The viral particles can be suspended in EpiLife and stored for further reprogramming in –80°C.
Reprogramming of keratinocytes
Keratinocytes grow on collagen IV−coated culture plates. On 2 successive days, viral particles together with 8 µg/ml hexadimethrine bromide are added directly to the keratinocytes. Infected cells are plated on inactivated feeder cells and kept in reprogramming medium (for more details on inactivation of feeder cells, see Linta et al., 2012). After 3-4 weeks, the first stem cell colonies appear. Colonies can be picked mechanically using a pipet and transferred onto iPSC Matrigel−coated culture plates (6-well-plates) in 1.5 ml iPSC medium. The medium has to be changed every day with 1.5 ml of fresh iPSC medium.
Verification of iPSCs
To confirm the pluripotency of the generated iPSCs, differentiation into all three germ layers must be verified, and pluripotency assays and alkaline phosphate staining have to be performed (for more detail on iPSC characterization, see Klingenstein et al., 2016).
CONCLUSION
In summary, the use of keratinocytes has obvious advantages over the commonly used fibroblasts. In addition, almost all established reprogramming methods can be applied to keratinocytes. In the future, use of keratinocytes as a primary somatic cell line may increase.
Supporting Information
Filename | Description |
---|---|
cpsc121-sup-0001-video.avi180.8 MB | Video 1. Plucking and Plating Hair. This video provides helpful technical information for plucking, transport, and cultivation of human hair roots. In the first section, the plucking of hair from human scalp and transport of the hair roots is explained. Next, the video shows cutting hair roots to the correct length and plating the roots in Matrigel in T-25 flasks. The last section gives instructions how to proceed when keratinocytes first appear. |
Please note: The publisher is not responsible for the content or functionality of any supporting information supplied by the authors. Any queries (other than missing content) should be directed to the corresponding author for the article.
LITERATURE CITED
- Aasen, T., Raya, A., Barrero, M. J., Garreta, E., Consiglio, A., Gonzalez, F., … Izpisua Belmonte, J. C. (2008). Efficient and rapid generation of induced pluripotent stem cells from human keratinocytes. Nature Biotechnology , 26(11), 1276–1284. doi: 10.1038/nbt.1503.
- Badieyan, Z. S., & Evans, T. (2019). Concise review: Application of chemically modified mRNA in cell fate conversion and tissue engineering. Stem Cells Translational Medicine , 8(8), 833–843. doi: 10.1002/sctm.18-0259.
- Barrero, M. J., Berdasco, M., Paramonov, I., Bilic, J., Vitaloni, M., Esteller, M., & Izpisua Belmonte, J. C. (2012). DNA hypermethylation in somatic cells correlates with higher reprogramming efficiency. Stem Cells , 30(8), 1696–1702. doi: 10.1002/stem.1138.
- Bernloehr, C., Bossow, S., Ungerechts, G., Armeanu, S., Neubert, W. J., Lauer, U. M., & Bitzer, M. (2004). Efficient propagation of single gene deleted recombinant Sendai virus vectors. Virus Research , 99(2), 193–197. doi: 10.1016/j.virusres.2003.11.005.
- Bikle, D. D., Xie, Z., & Tu, C. L. (2012). Calcium regulation of keratinocyte differentiation. Expert Review of Endocrinology & Metabolism, 7(4), 461–472. doi: 10.1586/eem.12.34.
- Chan, C. C., Fan, S. M., Wang, W. H., Mu, Y. F., & Lin, S. J. (2015). A two-stepped culture method for efficient production of trichogenic keratinocytes. Tissue Engineering. Part C, Methods , 21(10), 1070–1079. doi: 10.1089/ten.TEC.2015.0033.
- Elsholz, F., Harteneck, C., Muller, W., & Friedland, K. (2014). Calcium—a central regulator of keratinocyte differentiation in health and disease. European Journal of Dermatology , 24(6), 650–661. doi: 10.1684/ejd.2014.2452.
- Ernst, N., Yay, A., Biro, T., Tiede, S., Humphries, M., Paus, R., & Kloepper, J. E. (2013). Beta1 integrin signaling maintains human epithelial progenitor cell survival in situ and controls proliferation, apoptosis and migration of their progeny. PloS One , 8(12), e84356. doi: 10.1371/journal.pone.0084356.
- Fleury, S., Simeoni, E., Zuppinger, C., Deglon, N., von Segesser, L. K., Kappenberger, L., & Vassalli, G. (2003). Multiply attenuated, self-inactivating lentiviral vectors efficiently deliver and express genes for extended periods of time in adult rat cardiomyocytes in vivo. Circulation , 107(18), 2375–2382. doi: 10.1161/01.CIR.0000065598.46411.EF.
- Fusaki, N., Ban, H., Nishiyama, A., Saeki, K., & Hasegawa, M. (2009). Efficient induction of transgene-free human pluripotent stem cells using a vector based on Sendai virus, an RNA virus that does not integrate into the host genome. Proceedings of the Japan Academy. Series B, Physical and Biological Sciences , 85(8), 348–362. doi: 10.2183/pjab.85.348.
- Hotta, A., & Ellis, J. (2008). Retroviral vector silencing during iPS cell induction: An epigenetic beacon that signals distinct pluripotent states. Journal of Cellular Biochemistry , 105(4), 940–948. doi: 10.1002/jcb.21912.
- Hung, S. S., Pebay, A., & Wong, R. C. (2015). Generation of integration-free human induced pluripotent stem cells using hair-derived keratinocytes. Journal of Visualized Experiments , 102, e53174. doi: 10.3791/53174.
- Keenan, J., Pearson, D., O'Driscoll, L., Gammell, P., & Clynes, M. (2006). Evaluation of recombinant human transferrin (DeltaFerrinTM) as an iron chelator in serum-free media for mammalian cell culture. Cytotechnology , 51(1), 29–37. doi: 10.1007/s10616-006-9011-x.
- Kent, K. D., & Bomser, J. A. (2003). Bovine pituitary extract provides remarkable protection against oxidative stress in human prostate epithelial cells. In Vitro Cellular & Developmental Biology Animal, 39(8-9), 388–394. doi: 10.1290/1543-706X(2003)039<0388:BPEPRP>2.0.CO;2.
- Kim, Y. G., Baltabekova, A. Z., Zhiyenbay, E. E., Aksambayeva, A. S., Shagyrova, Z. S., Khannanov, R., … Shustov, A. V. (2017). Recombinant Vaccinia virus−coded interferon inhibitor B18R: Expression, refolding and a use in a mammalian expression system with a RNA-vector. PloS One , 12(12), e0189308. doi: 10.1371/journal.pone.0189308.
- Klingenstein, M., Raab, S., Achberger, K., Kleger, A., Liebau, S., & Linta, L. (2016). TBX3 knockdown decreases reprogramming efficiency of human cells. Stem Cells International , 2016, 6759343. doi: 10.1155/2016/6759343.
- Lim, S. J., Ho, S. C., Mok, P. L., Tan, K. L., Ong, A. H., & Gan, S. C. (2016). Induced pluripotent stem cells from human hair follicle keratinocytes as a potential source for in vitro hair follicle cloning. PeerJ , 4, e2695. doi: 10.7717/peerj.2695.
- Linta, L., Stockmann, M., Kleinhans, K. N., Bockers, A., Storch, A., Zaehres, H., … Liebau, S. (2012). Rat embryonic fibroblasts improve reprogramming of human keratinocytes into induced pluripotent stem cells. Stem Cells and Development , 21(6), 965–976. doi: 10.1089/scd.2011.0026.
- Nakagawa, M., Koyanagi, M., Tanabe, K., Takahashi, K., Ichisaka, T., Aoi, T., … Yamanaka, S. (2008). Generation of induced pluripotent stem cells without Myc from mouse and human fibroblasts. Nature Biotechnology , 26(1), 101–106. doi: 10.1038/nbt1374.
- Nygaard, U. H., Niehues, H., Rikken, G., Rodijk-Olthuis, D., Schalkwijk, J., & van den Bogaard, E. H. (2015). Antibiotics in cell culture: Friend or foe? Suppression of keratinocyte growth and differentiation in monolayer cultures and 3D skin models. Experimental Dermatology , 24(12), 964–965. doi: 10.1111/exd.12834.
- Okita, K., Nakagawa, M., Hyenjong, H., Ichisaka, T., &Yamanaka, S. (2008). Generation of mouse induced pluripotent stem cells without viral vectors. Science , 322(5903), 949–953. doi: 10.1126/science.1164270.
- Piao, Y., Hung, S. S., Lim, S. Y., Wong, R. C., & Ko, M. S. (2014). Efficient generation of integration-free human induced pluripotent stem cells from keratinocytes by simple transfection of episomal vectors. Stem Cells Translational Medicine , 3(7), 787–791. doi: 10.5966/sctm.2013-0036.
- Raab, S., Klingenstein, M., Liebau, S., & Linta, L. (2014). A comparative view on human somatic cell sources for iPSC generation. Stem Cells International , 2014, 768391. doi: 10.1155/2014/768391.
- Raab, S., Klingenstein, M., Moller, A., Illing, A., Tosic, J., Breunig, M., … Liebau, S. (2017). Reprogramming to pluripotency does not require transition through a primitive streak−like state. Scientific Reports , 7(1), 16543. doi: 10.1038/s41598-017-15187-x.
- Re, S., Dogan, A. A., Ben-Shachar, D., Berger, G., Werling, A. M., Walitza, S., & Grunblatt, E. (2018). Improved generation of induced pluripotent stem cells from hair derived keratinocytes—a tool to study neurodevelopmental disorders as ADHD. Frontiers in Cellular Neuroscience , 12, 321. doi: 10.3389/fncel.2018.00321.
- Schlaeger, T. M., Daheron, L., Brickler, T. R., Entwisle, S., Chan, K., Cianci, A., … Daley, G. Q. (2015). A comparison of non-integrating reprogramming methods. Nature Biotechnology , 33(1), 58–63. doi: 10.1038/nbt.3070.
- Shen, S., Alt, A., Wertheimer, E., Gartsbein, M., Kuroki, T., Ohba, M., … Tennenbaum, T. (2001). PKCdelta activation: A divergence point in the signaling of insulin and IGF-1-induced proliferation of skin keratinocytes. Diabetes , 50(2), 255–264. doi: 10.2337/diabetes.50.2.255.
- Sommer, C. A., Stadtfeld, M., Murphy, G. J., Hochedlinger, K., Kotton, D. N., & Mostoslavsky, G. (2009). Induced pluripotent stem cell generation using a single lentiviral stem cell cassette. Stem Cells , 27(3), 543–549. doi: 10.1634/stemcells.2008-1075.
- Steinle, H., Weber, M., Behring, A., Mau-Holzmann, U., Schlensak, C., Wendel, H. P., & Avci-Adali, M. (2019). Generation of iPSCs by nonintegrative RNA-based reprogramming techniques: Benefits of self-replicating RNA versus synthetic mRNA. Stem Cells International , 2019, 7641767. doi: 10.1155/2019/7641767.
- Takahashi, K., Tanabe, K., Ohnuki, M., Narita, M., Ichisaka, T., Tomoda, K., & Yamanaka, S. (2007). Induction of pluripotent stem cells from adult human fibroblasts by defined factors. Cell , 131(5), 861–872. doi: 10.1016/j.cell.2007.11.019.
- Wagner, T., Gschwandtner, M., Strajeriu, A., Elbe-Burger, A., Grillari, J., Grillari-Voglauer, R., … Mildner, M. (2018). Establishment of keratinocyte cell lines from human hair follicles. Scientific Reports , 8(1), 13434. doi: 10.1038/s41598-018-31829-0.
- Warlich, E., Kuehle, J., Cantz, T., Brugman, M. H., Maetzig, T., Galla, M., … Schambach, A. (2011). Lentiviral vector design and imaging approaches to visualize the early stages of cellular reprogramming. Molecular Therapy , 19(4), 782–789. doi: 10.1038/mt.2010.314.
- Warren, L., Manos, P. D., Ahfeldt, T., Loh, Y. H., Li, H., Lau, F., … Rossi, D. J. (2010). Highly efficient reprogramming to pluripotency and directed differentiation of human cells with synthetic modified mRNA. Cell Stem Cell , 7(5), 618–630. doi: 10.1016/j.stem.2010.08.012.
- Yu, J, , Hu, K., Smuga-Otto, K., Tian, S., Stewart, R., Slukvin, I. I., & Thomson, J. A. (2009) Human induced pluripotent stem cells free of vector and transgene sequences. Science , 324(5928), 797–801. doi: 10.1126/science.1172482.
Citing Literature
Number of times cited according to CrossRef: 8
- Iara Pastor Martins Nogueira, Guilherme Mattos Jardim Costa, Samyra Maria dos Santos Nassif Lacerda, Avian iPSC Derivation to Recover Threatened Wild Species: A Comprehensive Review in Light of Well-Established Protocols, Animals, 10.3390/ani14020220, 14 , 2, (220), (2024).
- Ida Derish, Renzo Cecere, Multifaceted Role of Induced Pluripotent Stem Cells in Preclinical Cardiac Regeneration Research, Handbook of Stem Cell Applications, 10.1007/978-981-99-7119-0_33, (787-847), (2024).
- Yuchen Tian, Xinyu Chen, High-Frequency Terahertz Waves Regulate the Dynamics of Mitochondrial Network in Human Pluripotent Stem Cells, Induced Neuron Stem Cells and Motor Neurons, Proceedings of the 5th China and International Young Scientist Terahertz Conference, Volume 1, 10.1007/978-981-97-3786-4_37, (189-194), (2024).
- Ida Derish, Renzo Cecere, Multifaceted Role of Induced Pluripotent Stem Cells in Preclinical Cardiac Regeneration Research, Handbook of Stem Cell Applications, 10.1007/978-981-99-0846-2_33-1, (1-61), (2023).
- Lisa S. Wüstner, Moritz Klingenstein, Karl G. Frey, Mohammad R. Nikbin, Alfio Milazzo, Alexander Kleger, Stefan Liebau, Stefanie Klingenstein, Generating iPSCs with a High-Efficient, Non-Invasive Method—An Improved Way to Cultivate Keratinocytes from Plucked Hair for Reprogramming, Cells, 10.3390/cells11121955, 11 , 12, (1955), (2022).
- Lay Shuen Tan, Juin Ting Chen, Lillian Yuxian Lim, Adrian Kee Keong Teo, Manufacturing clinical‐grade human induced pluripotent stem cell‐derived beta cells for diabetes treatment, Cell Proliferation, 10.1111/cpr.13232, 55 , 8, (2022).
- Arnab Ray, Jahnavy Madhukar Joshi, Pradeep Kumar Sundaravadivelu, Khyati Raina, Nibedita Lenka, Vishwas Kaveeshwar, Rajkumar P Thummer, An Overview on Promising Somatic Cell Sources Utilized for the Efficient Generation of Induced Pluripotent Stem Cells, Stem Cell Reviews and Reports, 10.1007/s12015-021-10200-3, 17 , 6, (1954-1974), (2021).
- Daniel Besser, Advancing Stem Cell Technologies and Applications: A Special Collection from the PluriCore Network in the German Stem Cell Network (GSCN), Current Protocols in Stem Cell Biology, 10.1002/cpsc.129, 55 , 1, (2020).