Establishing a Disease-in-a-Dish Model to Study SARS-CoV-2 Infection During Prenatal Development
Ann Song, Ann Song, Jack Ona, Jack Ona, Claudia Osuna, Claudia Osuna, Rattapol Phandthong, Rattapol Phandthong, Prue Talbot, Prue Talbot
Abstract
Mother-to-fetus transmission of the SARS-CoV-2 virus via the placenta has been reported but cannot readily be studied in pregnant women. This protocol describes an in vitro method to investigate SARS-CoV-2 infection of human embryonic stem cells (hESCs), which are similar to epiblast cells in young postimplantation embryos. First, SARS-CoV-2 viral pseudoparticles, which contain the spike protein and a fluorescent reporter, are incorporated into a lentivirus backbone that is expanded in HEK 293T cells. Then, an infection assay based on hESCs is used with the viral pseudoparticles. An application of the infection assay in therapeutic drug screening is provided. This protocol allows infection of hESCs by SARS-CoV-2 pseudoparticles to be studied in vitro and can be used in conjunction with other assays to understand and potentially prevent infection. hESCs could also be differentiated to study infection in the three germ layers and their fetal cell derivatives. This disease-in-a-dish model could be readily applied to other hESC lines, and to other viral infections, that affect human prenatal development. © 2023 The Authors. Current Protocols published by Wiley Periodicals LLC.
Basic Protocol 1 : Preparing HEK 293T cells for lentiviral vector transfection
Support Protocol 1 : Visual inspection of transfected HEK 293T cells
Basic Protocol 2 : Generating viral pseudoparticles
Support Protocol 2 : Determining viral titer with HEK 293T-ACE2 cells
Basic Protocol 3 : Plating hESCs for the infection assay
Support Protocol 3 : Evaluating transduction efficiency
INTRODUCTION
Vertical transmission of the SARS-CoV-2 virus from mother to fetus has been reported in pregnant women who developed COVID-19 in their third trimester (Hosier et al. 2020; Vivanti et al., 2020), and case reports of pregnant women with COVID-19 have been published to inform the public of this potential risk (Young et al. 2022). However, since experiments on pregnant women are seldom done (Talbot & Lin, 2011), an in vitro model is needed to study SARS-CoV-2 infection during human prenatal stages and to test methods to prevent infection.
Embryonic stem cells are derived from embryos and provide a model to address this research problem (Lin et al. 2009, 2010). In vitro assays with human embryonic stem cells (hESCs) simulate development in utero and can be used to evaluate risks during the most sensitive period of the life cycle (Bahl et al., 2012; Lin et al., 2021; Talbot & Lin, 2011). A protocol for an in vitro “disease-in-a-dish model” based on infection of hESCs by SARS-CoV-2 pseudoparticles is presented here. Because hESCs are delicate, conditions have been optimized to carry out the infection assay with these cells. There are three components to our model that can be used to address this research problem: (1) producing SARS-CoV-2 pseudoparticles for infection, (2) optimizing infection of hESCs with the viral pseudoparticles, and (3) applying this infection model to the research problem of interest. Our system is based on H9 hESCs, which are similar to epiblast cells of young postimplantation human embryos (Nichols & Smith, 2009, 2011) and SARS-CoV-2 pseudoparticles, which are accessible to any culture lab and do not present the risks associated with live virus. The protocol could be applied to any line of pluripotent stem cells or their derivatives.
H9 hESCs express both the ACE2 receptor and TMPRSS2 protease, which are components of the SARS-CoV-2 viral infection machinery (Colaco et al., 2021; Weatherbee et al., 2020). hESCs offer tremendous potential, as they can model both the epiblast stage of development and the ectodermal, endodermal, and mesodermal derivatives of the epiblast (Talbot & Lin, 2011). SARS-CoV-2 pseudoparticles are based on a fluorescent viral backbone that allows viral incorporation into hESCs to be observed microscopically and quantified using flow cytometry. This system can be used to screen drugs to better understand the infection mechanism and to identify those that inhibit infection.
This protocol presents a disease-in-a-dish model to study SARS-CoV-2 infection in hESCs and identify drugs that can prevent infection. The overall protocol (Fig. 1) involves first a description of the general procedure for aseptic culturing of HEK 293T cells (Basic Protocol 1), which are used to produce SARS-CoV-2 pseudoparticles (Basic Protocol 2). Then, the virus titer is determined with HEK 293T-ACE2 cells (Support Protocol 2) prior to infecting H9 hESCs with SARS-CoV-2 pseudoparticles (Basic Protocol 3). Multiplicity of infection (MOI) is the ratio of viral particles to cell, which should be precisely calculated for individual virus batches, as transfection and transduction may vary between experiments. Steps to adjust the virus titer are described, such that the desired MOI can be added to the hESC infection assay.
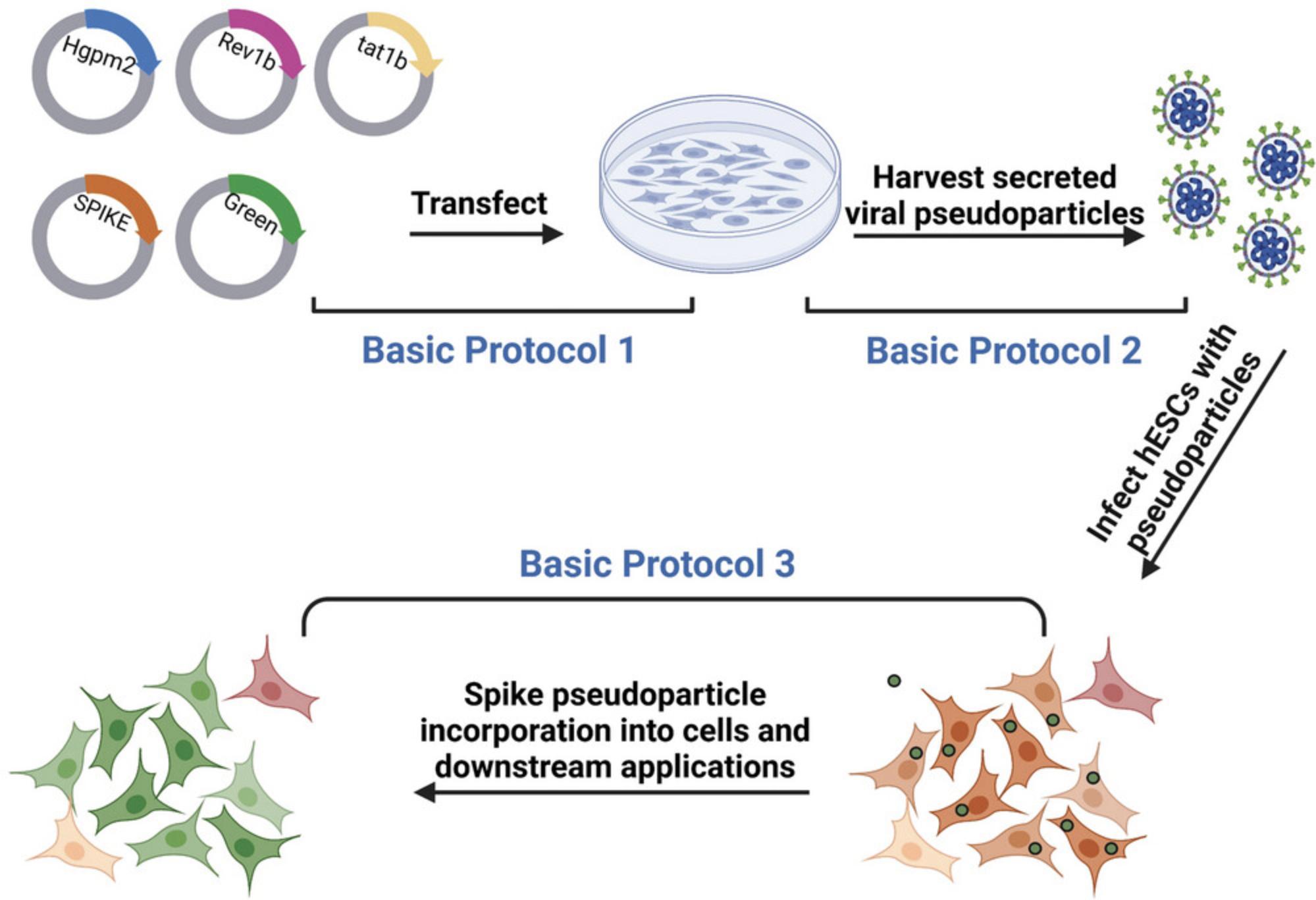
All procedures are performed using sterile technique in a Biosafety cabinet (BSL-2), except viewing cells with a microscope and performing flow cytometry analysis. HEK 293T cells and hESCs are incubated at 37°C with 5% CO2 and 95% relative humidity. hESCs attach overnight and then are exposed to viral pseudoparticles for 48 hr. When testing drugs, treatment is added 2 hr before infection. H9 hESCs and HEK 293T cells are maintained in mTeSR Plus medium with supplement and in DMEM supplemented with 10% FBS and 1% penicillin/streptomycin, respectively.
STRATEGIC PLANNING
Refer to Fig. 1 for a flow chart of the protocols overall. Before beginning, be sure all the components that are needed have been acquired (e.g., plasmids, cell lines, reagents, etc). Be sure that the culture procedure for the cells that will be used is set up and working in the laboratory.
Basic Protocol 1: PREPARING HEK 293T CELLS FOR LENTIVIRAL VECTOR TRANSFECTION
The first step in generating SARS-CoV-2 pseudoparticles involves transfecting third generation lentiviral vectors into HEK 293T cells. A fluorescent viral backbone is used to check infection between the steps microscopically. This protocol was adapted from a previous study (Crawford et al., 2020). Other viral vectors, including new SARS-CoV-2 variants, can be used instead of the NR-52314 spike plasmid to study the disease effects during prenatal development.
This protocol is designed for a 75-cm2 flask, and it is advisable to use this the first time to become familiar with the protocol. It may be desirable in subsequent runs to scale up in order to produce more virus.
Materials
-
HEK 293T cells, growing in 25-cm2 tissue culture flasks (ATCC, cat. no. CRL-3216)
-
Dulbecco's phosphate buffered saline (DPBS), without calcium and magnesium, pH 7.4 (Gibco, cat. no. 14190144)
-
Trypsin-EDTA (0.25%), phenol red (Gibco, cat. no. 25200056)
-
HEK 293T culture medium (see recipe)
-
Trypan blue solution, 0.4%, optional (Gibco, cat. no. 15250061)
-
Plasmids for lentivector transfection (see Table 1, Addgene)
-
Opti-MEM I reduced serum (Gibco, cat. no. 31985062)
-
Lipofectamine 3000 transfection reagent kit (Invitrogen, cat. no. L3000001)
-
Cell culture incubator, operating at 37°C, 5% CO2, and 90 to 95% relative humidity
-
Biosafety cabinet (BSL-2)
-
25-cm2 (T25) tissue culture flasks (Thermo Scientific, cat. no. 156367 or equivalent)
-
Inverted phase contrast/fluorescent microscope, with filters for visualizing green fluorescence
-
Sterile serological pipettes (2-ml, 5-ml, 10-ml)
-
Pipettor (Thermo Scientific, cat. no. 9521 or equivalent)
-
15-ml conical centrifuge tubes (Falcon, cat. no. 352097 or equivalent)
-
Centrifuge (capable of spinning at 335 × g with a rotor for 15-ml centrifuge tubes)
-
Microcentrifuge tubes, sterile
-
Pipette tips, sterile
-
Micropipettes (2.5-µl, 20-µl, 200-µl, 1000-µl)
-
Hemocytometer
-
Microscope cover glasses (Fisher Scientific, cat. no. 12-545A or equivalent)
-
75-cm2 (T75) tissue culture flasks (Thermo Scientific, cat. no.156499 or equivalent)
-
Vortexer
Seeding HEK 293T cells
1.Remove a 25-cm2 tissue culture flask of HEK 293T cells from the incubator and check their confluency with an inverted phase-contrast microscope. If the confluency is 80% to 90%, aspirate the HEK 293T culture medium from the flask.
2.Add 2.5 ml of DPBS along the side of the flask and swirl gently.
3.Remove the DPBS.
4.Add 1.25 ml of 0.25% trypsin-EDTA and place the flask in the incubator for 3 min.
5.Remove the flask from the incubator and gently tap it 3 to 4 times.
6.Add 2.5 ml of HEK 293T culture medium and mix gently so the cells come off the flask.
7.Transfer the cells to a sterile 15-ml conical tube.
8.Centrifuge 3 min at about 335 × g , room temperature.
9.Decant the supernatant gently, taking care that the pellet does not mix with the supernatant.
10.Add 1 ml of medium slowly along the side of the tube and mix to break up the pellet.
11.Mix 10 µl of this suspension with 90 µl of HEK 293T culture medium in a separate tube.
12.Take 10 µl of this suspension and pipet it onto both grids of a clean hemocytometer.
13.Count the number of cells in the four quadrants of each grid using a microscope, taking care to count only the live cells which appear round, versus dead cells which are shriveled.
14.Calculate the average of live cell counts from the four quadrants and obtain the cell concentration in the original suspension using the following formula:
- cells/ml = average count × dilution factor (10-fold) × 10,000
- 10,000 is multiplied to convert 100 nl (volume in each quadrant) to ml.
15.Determine the volume of the cell suspension needed to plate 7 × 106 cells in a 75-cm2 culture flask.
- Volume to add to flask (ml) = 7 × 106 ÷ cell concentration from step 14
16.Add 14 ml of HEK 293T culture medium to a 75-cm2 flask and transfer the calculated volume of cell suspension to the flask.
17.Place the flask inside the incubator at 37°C with 5% CO2 and 95% relative humidity.
18.Check the following day to see if cells have reached about 70% confluency.
Transfecting HEK 293T cells with lentiviral vectors
19.Prepare HEK 293T culture medium without penicillin-streptomycin.
20.Obtain a 75-cm2 flask of HEK 293T cells from Basic Protocol 1.When the confluency is about 70%, aspirate the culture medium from the flask.
21.Add 15 ml of culture medium without penicillin-streptomycin.
22.Place the flask in the incubator while preparing the reagents for transfection.
23.Add 750 µl of Opti-MEM to a 15-ml conical tube and label the tube “A”.
24.Add 60 µl of Lipofectamine 3000 to tube A and gently vortex for 2 sec.
25.Add 750 µl of Opti-MEM to another 15-ml conical tube and label the tube “B”.
26.Add 20 µl of P3000 from the Lipofectamine kit and the plasmids listed in Table 1 and gently vortex for 2 sec.
27.Combine Tube A and B and gently vortex for 2 sec.
28.Incubate the transfectant mix for 10 min at room temperature.
29.Add transfectant complex to cells dropwise over the surface of the culture medium.
30.Place the flask inside the incubator for 24 hr. Proceed to Support Protocol 1.
Support Protocol 1: VISUAL INSPECTION OF TRANSFECTED HEK 293T CELLS
Transfection needs to be verified using fluorescence microscopy. If the transfection efficiency is low, Table 2 offers guidelines for troubleshooting.
Plasmid ID | Plasmid name | Amount (µg) |
---|---|---|
NR-52520 | pHAGE2-CMV-ZsGreen-W | 7.5 |
NR-52517 | HDM-Hgpm2 | 1.65 |
NR-52519 | pRC-CMV Rev1b | 1.65 |
NR-52518 | HDM-tat1b | 1.65 |
NR-52314 | pHDM-SARS-CoV-2 Spike | 2.55 |
Problem | Possible cause | Solution |
---|---|---|
Low transfection efficiency | DNA concentration too low or DNA is degraded | Perform restriction enzyme digest to check the integrity of the plasmids |
Culture medium contains contaminants |
Do not use antibiotics at time of transfection Test cells for mycoplasma or other contaminants |
|
Transfection reagent has been compromised | Store reagents at 4°C since freezing or long-term storage at room temperature can compromise the integrity of these reagents | |
Low cell viability after transfection | Plasmid DNA preparation contains high levels of endotoxin | Use of a midi or a maxi prep kit instead of a mini prep kit is recommended for plasmid DNA purification |
Cationic lipid reagent was oxidized | Excessive vortexing of transfection reagents may form cationic lipid reagent peroxides | |
Low transduction efficiency | Viral vectors can undergo recombination | Perform restriction enzyme digest to check the integrity of the plasmids |
Properly remove packaging cell (293T) debris | Do not skip the filtering (0.45 µm) or centrifuging steps as these steps contribute to the purity of the virus | |
Avoid freeze-thaws of the virus | Take new vials of virus from the freezer for each experiment to maintain the integrity of the virus | |
Low cell viability after transduction | Treatment was toxic | Perform a dose-response of the treatment to optimize the concentration |
Cell density was too low | Wait until the appropriate cell density is reached prior to transduction | |
MOI calculation was incorrect | Recalculate the MOI or check the virus titer to add an appropriate amount of virus to the cells |
- a
Note: Portions of this table were adapted from the Lipofectamine3000 kit.
Materials
-
Transfected HEK 293T cells in 75-cm2 tissue culture flask (Basic Protocol 1)
-
HEK 293T culture medium (see recipe)
-
Bovine albumin (BSA) fraction V, 7.5% solution (Gibco, cat. no. 15260037)
-
Fluorescent microscope with filter set for viewing green fluorescence (495 nm/519 nm)
1.Check green fluorescence of transfected cells with an inverted fluorescence microscope (Fig. 2A, 2B).
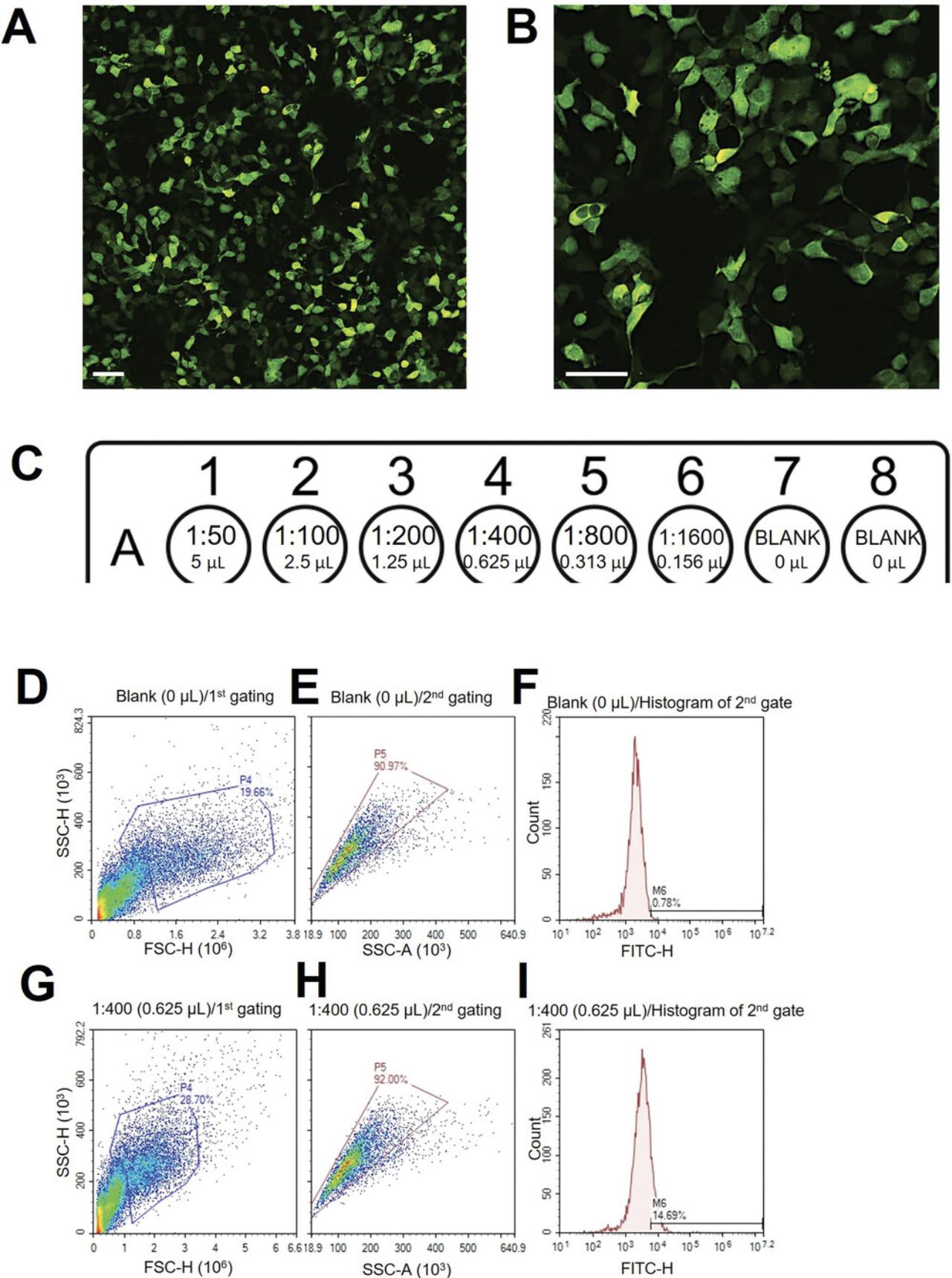
2.Prepare HEK 293T culture medium supplemented with 1% BSA.
3.Aspirate the medium from the flask and add 15 ml of medium supplemented with 1% BSA.
4.Place the flask inside an incubator for 48 hr. Proceed to Basic Protocol 2.
Basic Protocol 2: GENERATING VIRAL PSEUDOPARTICLES
The viral pseudoparticles are secreted into the HEK 293T conditioned medium. Since HEK 293T medium is not compatible with the H9 hESC culture, pseudoparticles must be concentrated using polyethylene glycol (PEG) precipitation and resuspended in a suitable medium. This method also allows for the long-term storage of pseudoparticles at −80°C. Steps described for harvesting pseudoparticles need to be performed cold (4°C) to maintain the integrity of the pseudoparticles.
Materials
-
Transfected HEK 293T cells in 75-cm2 tissue culture flask (Support Protocol 1)
-
PEG virus precipitation kit (Abcam, cat. no. ab102538)
-
DPBS, ice-cold (Invitrogen, cat. no. 14190144)
-
15-ml conical centrifuge tubes (Falcon, cat. no. 352097 or equivalent)
-
Acrodisc 25 mm syringe filter, 0.45-μm pore size (Pall Laboratory, cat. no. 28143-312 or equivalent)
-
10-ml luer loc syringes (BD, cat. no. 309604 or equivalent)
-
50-ml conical centrifuge tube (Falcon, cat. no. 352098 or equivalent)
-
Vortexer
-
Centrifuge (capable of spinning at 2,500 × g with a rotor for 15-ml centrifuge tubes, temperature controllable)
-
Microcentrifuge tubes, sterile
-
-80°C freezer
Precipitating pseudoparticles
1.Using sterile conditions, collect the conditioned medium in a 15-ml conical tube.
2.Attach the 0.45 μm filter to a syringe.
3.Pass the conditioned medium through the syringe-filter and collect filtrate in a 50-ml conical tube.
4.Add 3.75 ml of 5× PEG to the filtered conditioned medium.
5.Vortex at maximum speed for 1 min and incubate at 4°C for 24 hr to precipitate viral pseudoparticles.
Harvesting pseudoparticles
6.Transfer the conditioned medium and PEG mix to two 15-ml conical tubes.
7.Centrifuge 30 min at 2,500 × g , 4°C.
8.Decant the supernatant carefully.
9.Remove any remaining supernatant with a micropipette, taking care not to dislodge the pellet.
10.Resuspend the pellet with 45 µl of ice-cold DPBS. The total volume with the pellet will be approximately 90 µl.
11.Generate 20 µl aliquots of the resuspended viral pellet.
12.Quickly store viral aliquots at −80°C. Proceed to Support Protocol 2.
Support Protocol 2: DETERMINING VIRAL TITER WITH HEK 293T-ACE2 CELLS
Cells can be quickly examined via microscopy to verify that transduction has occurred. This protocol then uses flow cytometry to quantify green fluorescence from the viral backbone, which is an indicator of viral transduction efficiency. Pseudoparticle transduction efficiency is checked by test-infecting HEK 293T-ACE2 cells. HEK 293T-ACE2 cells are engineered to express the ACE2 receptor for SARS-CoV-2.Pseudoparticle transduction efficacy is determined by adding different amounts of pseudoparticles to HEK 293T-ACE2 cells and quantifying the percent that are infected using flow cytometry to determine the relative number of cells expressing green fluorescence. The gating strategy is also described to extrapolate transduction efficiency information. Poisson's formula is used to obtain the MOI of the viral pseudoparticle batch (Crawford et al., 2020).
If studying a different virus, the cell line needs to express the receptor for that virus, which may not be ACE2. This protocol describes the HEK 293T-ACE2 seeding setup and how to carry out a test infection.
Materials
-
HEK 293T-ACE2 cells, growing in 25-cm2 tissue culture flasks
-
Trypsin-EDTA (0.25%), phenol red (Gibco, cat. no. 25200056)
-
Trypan blue solution, 0.4%, optional (Gibco, cat. no. 15250061)
-
HEK 293T culture medium (see recipe)
-
Viral pseudoparticle aliquot (Basic Protocol 2)
-
DPBS (Invitrogen, cat. no. 14190144)
-
BSA fraction V, 7.5% solution (Gibco, cat. no. 15260037)
-
Hemocytometer
-
Microscope cover glasses (Fisher Scientific, cat. no. 12545A)
-
48-well plate, clear flat bottom, tissue culture treated (Falcon, cat. no. 353230 or equivalent)
-
15-ml conical centrifuge tubes (Falcon, cat. no. 352097)
-
Centrifuge (capable of spinning at 335 × g with a rotor for 15-ml centrifuge tubes)
-
Vortexer
-
12 × 75 mm test tube with cell strainer snap cap (Falcon, cat. no. 352235 or equivalent)
-
Flow cytometer
1.Treat a flask of HEK 293T-ACE2 cells that is 80% to 90% confluent with trypsin as described in Basic Protocol 1.
2.Count cells using a hemocytometer as described in Basic Protocol 1.
3.Determine the volume of the cell suspension to plate 20,000 cells/well in a 48-well plate.
4.Plate 8 wells at 20,000 cells/well and incubate for 24 hr in the incubator at 37°C, 5% CO2, and 90 to 95% relative humidity.
Test infection in HEK293T-ACE2 cells
5.Prepare 1 ml of HEK 293T culture medium and mix with 20 µl of a quickly thawed viral pseudoparticle aliquot from Basic Protocol 2.
6.Perform a 2-fold serial dilution of the virus in HEK 293T culture medium covering a range from 1:50 to 1:1600.
7.Aspirate the culture medium from the 48-well plate.
8.Add the serial dilution of the virus to each well. Each well should have a different concentration of virus.
9.Place the plate inside the incubator for 24 hr.
10.Aspirate the virus diluted in culture medium and add fresh culture medium.
11.Place the plate inside the incubator for 24 hr.
Preparation for flow cytometry analysis
12.Treat all wells of HEK 293T-ACE2 cells with trypsin as described in Basic Protocol 1.All reagent volumes should be scaled down 75-fold since the culture vessel is now a 48-well plate.
13.Pellet cells as described in Basic Protocol 1 and resuspend in 250 µl of DPBS.
14.Centrifuge for 2 min at 335 × g, room temperature.
15.Decant the supernatant gently, taking care to not dislodge the pellet.
16.Resuspend the pellet in 250 µl of DPBS and repeat the spin-wash.
17.Resuspend the pellet in 250 µl of 0.5% BSA in DPBS. Vortex to completely break the pellet.
18.Filter cell suspension into cell strainer tubes to completely remove cell clumps.
19.Read the percentage of green fluorescence (FITC channel) of each cell suspension sample using flow cytometry.
Data analysis for fluorescence quantification
20.Start with the no infection control (blank) and plot SSC-H (Side Scatter Height) versus FSC-H (Forward Scatter Height) and gate for low SSC and high FSC (P4) to eliminate cell debris (Fig. 2D).
21.Plot SSC-H versus SSC-A (Area) of cells in P4 from the first gating to select for single cells (P5) (Fig. 2E).
22.Plot a histogram of green fluorescence for the second gating (P5) (Fig. 2F).
23.Apply this gating to all samples to obtain the green fluorescence reading indicative of transduction efficiency (Fig. 2F-I).
24.Subtract the no infection control (Blank) from all raw values to remove autofluorescence.
25.Find a sample that has a final transduction efficiency of ∼10%.
26.Use this value to apply the Poisson formula to obtain the MOI.
- Particle Forming Unit (PFU)/ml = [Number of cells seeded × -Ln {1 - (transduction efficiency ÷ 100)}] ÷ volume of pseudoparticles (ml)
- 13.91% transduction efficiency was obtained with 20,000 cells and 0.625 µl of pseudoparticles:
Basic Protocol 3: PLATING hESCs FOR THE INFECTION ASSAY
The H9 infection assay (Basic Protocol 3) is based on plating 24,000 cells/well in a 48-well plate. This cell number was chosen as the appropriate plating density for H9 hESCs after testing at different MOIs. This protocol involves seeding H9 hESCs using ROCKi to improve single-cell survival (Ohgushi et al., 2010; Watanabe et al., 2007; Weng et al., 2018). Many downstream applications, such as direct differentiation, require accurate counting and plating of single hESCs.
The pseudoparticle infection assay may be used for numerous applications. This protocol uses drug screening as an example. The test drug, Dyngo4a, targets one of the SARS-CoV-2 viral entry pathways; therefore, this treatment requires preincubation of the hESCs with Dyngo4a. Preincubation may not be necessary for some drugs, and thus, the manufacturers’ recommendations for treatments need to be considered.
Materials
-
Matrigel matrix, diluted (Corning, cat. no. 354234) (see recipe)
-
DMEM, high glucose (Gibco, cat. no. 11965092)
-
mTeSR plus (Stem Cell Technologies, cat. no. 100-0276) (see recipe)
-
hESCs (Lin and Talbot, 2011) (WiCell cat. no. WA09)
-
DPBS (Invitrogen, cat. no. 14190144)
-
ReLeSR (Stem Cell Technologies, cat. no. 05872)
-
Gentle cell dissociation reagent (Stem Cell Technologies, cat. no. 100-0485)
-
Y-27632 dihydrochloride (Tocris, cat. no. 1254)
-
Trypan blue solution, 0.4%, optional (Gibco, cat. no. 15250061)
-
Dyngo4a or other test drugs, optional (Selleckchem, cat. no. S7163)
-
Viral pseudoparticle aliquot (Basic Protocol 2)
-
6-well plate, clear flat bottom, tissue culture treated (Falcon cat. no. 353046)
-
Inverted phase contrast microscope
-
15-ml conical centrifuge tubes (Falcon, cat. no. 352097)
-
Centrifuge (capable of spinning at 335 × g with a rotor for 15-ml centrifuge tubes)
-
Hemocytometer
-
Microscope cover glasses (Fisher Scientific, cat. no. 12545A)
-
48-well plate, clear flat bottom, tissue culture treated (Falcon, cat. no. 353230 or equivalent)
Matrigel coating before the experiment
1.Properly prepare Matrigel as described in Reagents and Solutions section, then add diluted Matrigel to the culture vessel and incubate at 37°C for at least 30 min before using the plate.
2.Remove Matrigel from the culture vessel and add mTeSR plus culture medium.
Culturing of H9 hESCs (modified from Lin and Talbot, 2011 and Behar et al. 2012.)
3.Remove a 6-well plate of H9 hESCs from the incubator and check its confluency with an inverted phase-contrast microscope. If the confluency is 60% to 70%, aspirate the mTeSR plus culture medium from the well.
4.Add 1 ml of DPBS dropwise along the wall of the well gently.
5.Remove the DPBS.
6.Add 1 ml of ReLeSR and swirl gently.
7.Remove the ReLeSR and place the plate in the incubator for 4 min.
8.Remove the plate from the incubator and add 1 ml of mTeSR plus medium dropwise along the wall of the well gently.
9.Gently tap the plate 3 to 4 times.
10.Transfer the undifferentiated cells into a sterile 15-ml conical tube.
11.Triturate the cells gently, twice with a 1000-μl pipette
12.Add 100 μl of cells (1:10 dilution) to each well of the 6-well plate prepared in step 2.
13.Place the plate in the incubator overnight.
14.Check the following day and perform a medium change (2 ml of mTeSR plus medium).
15.Culture medium should be changed every other day.
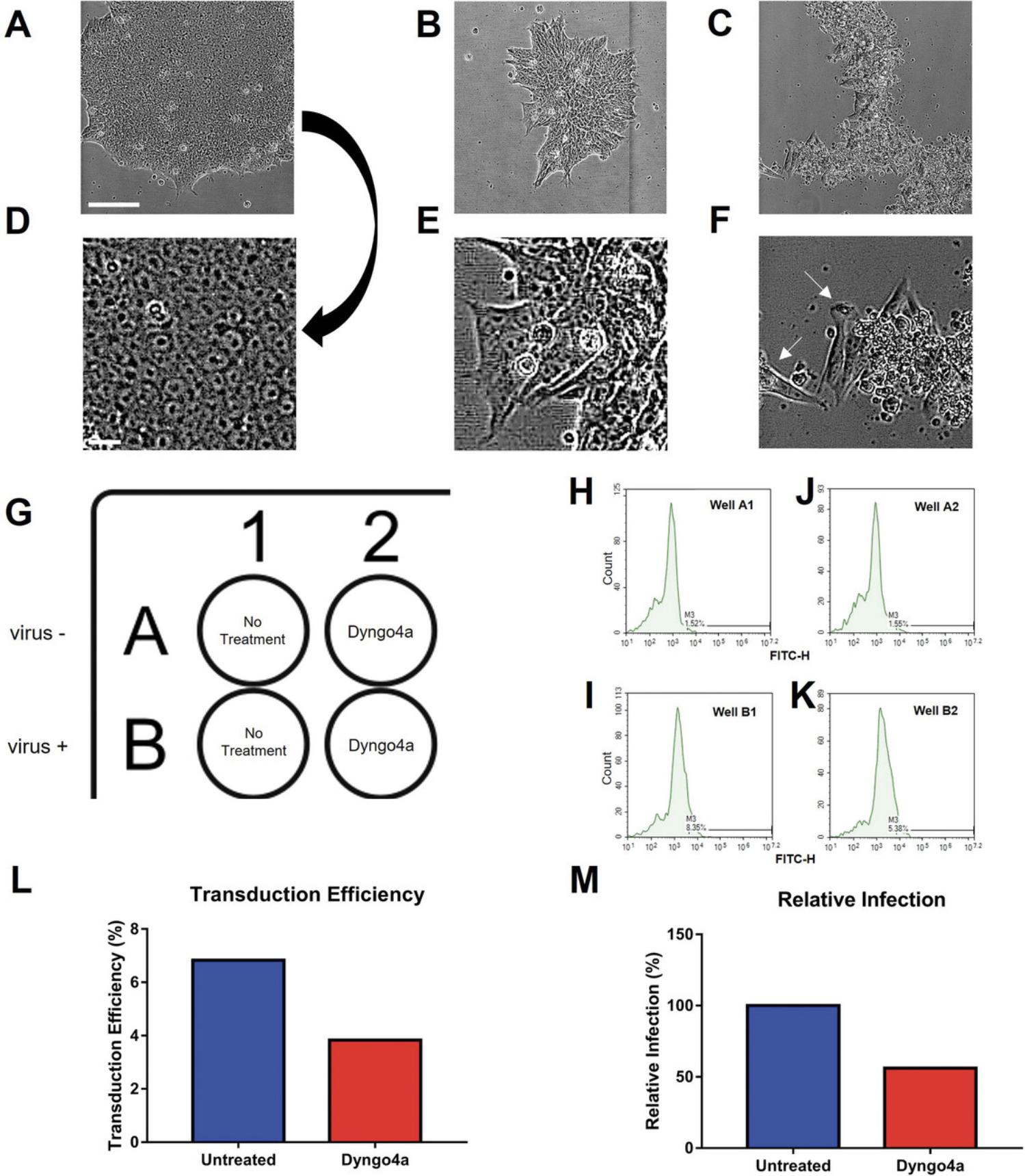
Procedure for ROCK inhibitor treatment
16.Once two wells in a 6-well plate are 60% to 70% confluent, remove mTeSR plus medium from the wells with a 1000-μl pipette.
17.Wash each well with 1 ml of DPBS.
18.Add 1 ml of gentle cell dissociation reagent to each well and incubate for 8 min at 37°C.
19.Add 1 ml of mTeSR plus medium to neutralize gentle cell dissociation reagent.
20.Harvest cells by pipetting up and down with a 1000-μl pipette to ensure a single-cell suspension and transfer cells to a 15-ml conical tube. Rinse wells with an additional 1 ml of DMEM medium and add the rinse to the tube containing the cells.
21.Centrifuge cells at 335 × g for 3 min, room temperature. During the centrifugation, prepare medium with ROCK inhibitor Y-27632 dihydrochloride.
22.Resuspend cells in 1 ml of mTeSR plus medium with ROCK inhibitor.
23.Count cells as described in Basic Protocol 1 to determine the cell concentration.
24.Calculate to find the dilution factor necessary to plate 24,000 cells/well in each well of a 48-well plate.
25.In addition to the wells plated for screening drugs, plate additional control wells with cells to eliminate autofluorescence. These wells should not be infected with pseudoparticles (refer to the plate design in Fig. 3G).
26.Place plate in the incubator for 24 hr to allow cells to attach.
Example of an application: Drug treatment and infection
Steps 27-28 may be skipped if drug testing is not a downstream application of interest. Instead, proper controls must be designed accordingly. For instance, if testing transduction efficiency in different cell lines, then two wells (+/- virus) such as in Figure 3 G should be plated instead of drug treatment wells (see Critical Parameters).
27.Prepare drug(s) to be tested in mTeSR plus medium.
28.Gently remove the culture medium and add 250 µl of the test drug(s) diluted in mTeSR plus medium. Preincubate cells in the drug for 2 hr.
29.Obtain frozen aliquots of viral pseudoparticles from Basic Protocol 2.
30.Add virus to each well. H9 cells typically yield ∼10% transduction efficiency with a MOI of 0.5.
- volume of pseudoparticles (ml) = [Number of cells seeded × -Ln {1 - (transduction efficiency ÷ 100)}] ÷ (PFU/ml)
- If using the same batch, refer to Support Protocol 2 step 26 for PFU/ml:
31.Incubate the plate for 24 hr in the incubator.
32.Gently remove the medium and add 250 µl of the drug(s) diluted in mTeSR plus medium.
33.Incubate the plate for 24 hr in the incubator. Proceed to Support Protocol 3.
Support Protocol 3: EVALUATING TRANSDUCTION EFFICIENCY
This protocol starts with a description of dissociating hESCs adapted from Basic Protocol 3.Then, samples are prepared and analyzed as described in Support Protocol 2.
Materials
-
Cells infected with pseudoparticles in a 48-well plate (Basic Protocol 3)
-
Gentle cell dissociation reagent (Stem Cell Technologies, cat. no. 100-0485)
-
mTeSR plus (Stem Cell Technologies, cat. no. 100-0276) (see recipe)
-
DMEM, high glucose (Gibco, cat. no. 11965092)
-
DPBS (Invitrogen, cat. no. 14190144)
-
BSA fraction V, 7.5% solution (Gibco, cat. no. 15260037)
-
12 × 75 mm test tube with cell strainer snap cap (Falcon, cat. no. 352235 or equivalent)
-
Centrifuge (capable of spinning at 335 × g with a rotor for 15-ml centrifuge tubes)
-
Flow cytometer
-
Centrifuge (capable of spinning at 335 × g with a rotor for 15-ml centrifuge tubes)
-
Inverted phase contrast microscope
-
15-ml conical centrifuge tubes (Falcon, cat. no. 352097)
1.Lift cells with gentle cell dissociation reagent as described in Basic Protocol 3, steps 18 to 19. All reagent volumes should be scaled down 8-fold as the culture vessel is now a 48-well plate.
2.Pellet cells and prepare samples for flow cytometry as described in Support Protocol 2, steps 13 to 18.
3.Run samples using flow cytometry.
4.Analyze the data as described in Support Protocol 2.
5.Graph the results.
REAGENTS AND SOLUTIONS
HEK 293T culture medium (500 ml)
- 440 ml DMEM, high glucose (Gibco, cat. no. 11965092)
- 55 ml of Fetal Bovine Serum (10% final concentration)
- 5 ml of penicillin-streptomycin (1% final concentration)
- Store up to 3 months at 4°C.
Matrigel
- Slowly thaw the stock Matrigel overnight in ice at 4°C.
- Make 1 ml aliquots in prechilled 15-ml tubes to store at −20°C (each aliquot should be 10 mg/ml).
- To prepare a working stock, thaw a frozen aliquot 1 hr to overnight at 4°C.
- Add 14 ml of DMEM (final working concentration of matrigel after dilution should be 666.7 ng/ml).
- Store up to 2 weeks at 4°C.
mTeSR plus culture medium (not to be warmed at 37°C)
- 400 ml of mTeSR plus Basal medium
- 100 ml of mTeSR plus 5× supplement
- Aliquot and store up to 6 months at −20°C and thaw fresh as needed.
COMMENTARY
Background Information
Pseudotyping is used to study binding of the viral protein to the host cell receptors and has been successfully used in previous Coronavirus studies (Hurlburt et al., 2020; Lu et al., 2014; Phandthong et al., 2022a, 2022b). Furthermore, SARS-CoV-2 pseudoparticles can be generated in a BSL-2 biosafety cabinet, which is a major advantage over using live virus. Pseudoparticles cannot replicate, making their use feasible in most labs set up for cell culture. Thus, SARS-CoV-2 pseudoparticle infection is an efficient and safe approach for studying the early stages of viral infection.
When performing an infection, several critical factors need to be considered to obtain reliable results. These include accurate cell counting and selection of the appropriate concentration of drugs. The cell number/well should be the same in all wells in all experiments for fair comparisons. This introduces the need to accurately count cells before plating for infection. hESCs can be seeded accurately as single cells using the ROCK inhibitor, Y27632, which blocks Rho-associated coiled-coil kinase and reduces cell blebbing and apoptosis (Ohgushi et al., 2010; Watanabe et al., 2007; Weng et al., 2018). Moreover, H9 hESCs can differentiate into the three germ layers (ectoderm, endoderm, and mesoderm) making it possible to apply pseudotyping to later stages of prenatal development. The cell seeding scheme can be easily applied to germ layers since most direct differentiation assays require single-cell seeding with ROCK inhibitor.
Once the cell concentration is determined using a hemocytometer, it is necessary to determine the number of cells per well. For the experimental protocols described, the optimal number was determined to be 24,000 cells/well. When working with drugs, a preliminary dose response experiment can be used to determine the final concentration range of the drugs (i.e., those not toxic to hESCs), which is then tested using the plate design in Figure 3G. The data are graphed and analyzed statistically to determine if a drug inhibits infection (Fig. 3L, M).
In some screens, all drugs are used at the same concentrations (10 µM is often used). This is rapid but has the disadvantage that some valuable drugs may be missed when only one concentration is tested.
Critical Parameters
Scaling up the production of viral pseudoparticles
This protocol is based on a single T75 flask. In actual practice, it is probably advisable to scale up to increase the yield of viral pseudoparticles for each batch.
Counting cells with a hemocytometer
It is important to count cells accurately, as MOI depends on the cell count. For a fair comparison, the same number of cells should be plated in each well each day. Automated cell counters may be used instead of hemocytometers. If unfamiliar with the use of a hemocytometer, it may be necessary to develop this skill and confirm that reproducible counts can be made on the same sample. Ideally, very few cells will die, but it is important to count only live cells. If living cells that appear round are difficult to recognize, Trypan blue can be used to stain dead cells and exclude them from the count. If more than 30% of the cells are dead, the integrity of the culture may not be suitable.
Incubation of cells
It is critical that the incubator maintains a temperature of 37°C with 5% CO2 and 95% relative humidity. Sometimes there are zones in incubators that have slightly different conditions; therefore, it is advisable to put plates in the same location in the incubator for each experiment.
Sensitivity of the viral pseudoparticles
Viral pseudoparticles cannot be refrozen. Repeated freeze-thaws will alter the transduction efficiency, and the calculated MOI cannot be applied. Viral pseudoparticles should always be stored at −80°C if not being used for infection.
Viral pseudoparticle titer analysis
When determining the viral pseudoparticle titer (Support Protocol 2), wells that appear around 10% ZsGreen-positive should be selected. The Poisson equation will only be accurate if the percentage of cells that are ZsGreen positive is relatively low (2% to 15%).
Matrigel
Once an aliquot of Matrigel is thawed, it is critical to keep it at 4°C. If it sits at room temperature, it will gel and be unusable.
hESC phenotype
During passaging and maintenance, hESC colonies should appear round with relatively smooth edges and cells tightly packed together as shown in Figure 3A, D. The appearance of gaps between cells is not normal, and the colonies may not be pluripotent (Fig. 3B, E). If colonies deviate from the normal morphology (Fig. 3A, D), there may be a problem with the hESC culture conditions. Cells may also spontaneously differentiate, and this can usually be detected as differentiated cells become spindle-shaped and migrate away from the colony (Fig. 3C, F).
Verification of pluripotency
It is advisable to periodically confirm that hESC colonies express pluripotency markers such as OCT3/4 and SSEA4. Cells can also be periodically karyotyped to confirm that chromosomes are normal.
Contamination
Cultured cells can easily be contaminated by microorganisms. It is advisable to periodically check from mycoplasma using commercially available kits and wear a mask and gloves when working with cells in the biosafety cabinet.
Selection of drug concentration range
Prior to performing infection assays, it is advisable to do an initial screen to determine which concentrations are not toxic to hESCs. After a safe concentration range has been determined, it can be tested using the pseudoparticle infection assay.
Adaptability of various infection assays
This protocol provides infection data with sample treatment (Dyngo4a), and it can be readily adapted to design other infection assays. First, different variants can be examined. This protocol describes infection with the original Wuhan strain of spike protein. However, SARS-CoV-2 has mutated, and additional variants are now available, which are likewise important to study. Second, the infectability of different cell lines can be compared. For instance, various hESC and iPSC lines can be used. Furthermore, an infection assay can be performed in differentiated hESCs. The infectability of undifferentiated hESCs can be compared with that of hESC-derived cells. Lastly, this protocol is easy to modify for testing other viruses, such as Zika, SARS, or Rubella. A third-generation lentiviral vector encoding for the viral protein of interest can replace the spike plasmid in Table 1.
Troubleshooting
Table 2 is included to help troubleshoot problems that may arise during the transfection and transduction steps.
Statistical Analysis
If there is only one treatment group, then a t-test can be used. If there are multiple treatment groups, then a one-way ANOVA can be performed. When the means are significant (p < .05), additional post-hoc analyses may be appropriate depending on the experimental design.
Understanding Results
As shown in Figure 3L and M, a graph can be generated that can be used to determine the level of infection and/or the relative inhibition caused by the drug being screened. The output can be relative infection, which is normalized to the “no treatment” control, or transduction efficiency, which is the percentage of fluorescent cell population.
Time Considerations
Assuming that after the HEK 293T cells are seeded, they would reach proper confluence in 1 day and be ready for transfection, 5 days will be needed to generate pseudoparticles. Another 2 days will be required to check the virus titer in HEK 293T-ACE2 cells prior to infecting hESCs, which takes an additional 2 days.
Acknowledgments
Figure 1 was created with Biorender.com. Micrographs in Figure 3 were contributed by Mohamed Debich and Shabnam Etemadi. We thank the UCR Stem Cell Core for providing access to the Novocyte Flow cytometer and the Nikon BioStation CT. Supported in part by TRDRP Award #R00RG2620, Bridges Fellowships from the California Institute for Regenerative Medicine (CIRM) (Grant #TB1-01185), a fellowship from the CIRM Research Training Program (#EDUC-12752), a Graduate Student Researcher award from the UCR Graduate Division, a Committee on Research grant from the UCR Academic Senate, and the AES Research and Graduate Student Funding Program. The content is solely the authors’ responsibility and does not necessarily represent the official views of the funding agencies.
Author Contributions
Ann Song : Conceptualization, data curation, formal analysis, investigation, methodology, validation, visualization, writing - original draft, and writing - review and editing; Jack Ona : Investigation, methodology, and visualization; Claudia Osuna : Investigation, methodology, and visualization; Rattapol Phandthong : Conceptualization, formal analysis, investigation, and methodology; Prue Talbot : Conceptualization, funding acquisition, investigation, project administration, resources, supervision, validation, visualization, writing - original draft, and writing - review and editing.
Conflict of Interest
The authors have no conflict of interest to declare.
Open Research
Data Availability Statement
Data sharing is not applicable to this article as no new data were created or analyzed in this study.
Literature Cited
- Bahl, V., Lin, S., Xu, N., Davis, B., Wang, Y. H., & Talbot, P. (2012). Comparison of electronic cigarette refill fluid cytotoxicity using embryonic and adult models. Reproductive Toxicology , 34(4), 529–537. https://doi.org/10.1016/j.reprotox.2012.08.001
- Behar, R. Z., Bahl, V., Wang, Y., Weng, J. H., Lin, S. C., & Talbot, P. (2012). Adaptation of stem cells to 96-well plate assays: Use of human embryonic and mouse neural stem cells in the MTT assay. Current Protocols in Stem Cell Biology , Chapter 1. https://doi.org/10.1002/9780470151808.sc01c13s23
- Colaco, S., Chhabria, K., Singh, D., Bhide, A., Singh, N., Singh, A., Husein, A., Mishra, A., Sharma, R., Ashary, N., & Modi, D. (2021). Expression map of entry receptors and infectivity factors for pan-coronaviruses in preimplantation and implantation stage human embryos. Journal of Assisted Reproduction and Genetics , 38(7), 1709–1720. https://doi.org/10.1007/s10815-021-02192-3
- Crawford, K. H. D., Eguia, R., Dingens, A. S., Loes, A. N., Malone, K. D., Wolf, C. R., Chu, H. Y., Tortorici, M. A., Veesler, D., Murphy, M., Pettie, D., King, N. P., Balazs, A. B., & Bloom, J. D. (2020). Protocol and reagents for pseudotyping lentiviral particles with SARS-CoV-2 spike protein for neutralization assays. Viruses , 12(5), 513. https://doi.org/10.3390/v12050513
- Hosier, H., Farhadian, S F., Morotti, R A., Deshmukh, U., Lu-Culligan, A., Campbell, K H., Yasumoto, Y., Vogels, C. B. F., Casanovas-Massana, A., Vijayakumar, P., Geng, B., Odio, C D., Fournier, J., Brito, A F., Fauver, J R., Liu, F., Alpert, T., Tal, R., Szigeti-Buck, K., … Lipkind, H S. (2020). SARS-CoV-2 infection of the placenta. Journal of Clinical Investigation , 130(9), 4947–4953. https://doi.org/10.1172/JCI139569
- Hurlburt, N. K., Seydoux, E., Wan, Yu-H., Edara, V. V., Stuart, A. B., Feng, J., Suthar, M. S., Mcguire, A. T., Stamatatos, L., & Pancera, M. (2020). Structural basis for potent neutralization of SARS-CoV-2 and role of antibody affinity maturation. Nature Communications , 11(1), 5413. https://doi.org/10.1038/s41467-020-19231-9
- Lin, S., Fonteno, S., Weng, J. H., & Talbot, P. (2010). Comparison of the toxicity of smoke from conventional and harm reduction cigarettes using human embryonic stem cells. Toxicological Sciences , 118(1), 202–212. https://doi.org/10.1093/toxsci/kfq241
- Lin, S., & Talbot, P. (2011). Methods for culturing mouse and human embryonic stem cells. Methods in Molecular Biology , 690, 31–56. https://doi.org/10.1007/978-1-60761-962-8_2
- Lin, S., Tran, V., & Talbot, P. (2009). Comparison of toxicity of smoke from traditional and harm-reduction cigarettes using mouse embryonic stem cells as a novel model for preimplantation development. Human Reproduction , 24(2), 386–397. https://doi.org/10.1093/humrep/den419
- Lin, S. C., Loza, A., Antrim, L., & Talbot, P. (2021). Video bioinformatics analysis of human pluripotent stem cell morphology, quality, and cellular dynamics. Stem Cells Translational Medicine , 10(9), 1343–1359. https://doi.org/10.1002/sctm.15-0352
- Lu, L., Liu, Q., Zhu, Y., Chan, K. -. H., Qin, L., Li, Y., Wang, Q., Chan, J. F. - W., Du, L., Yu, F., Ma, C., Ye, S., Yuen, K. -. Y., Zhang, R., & Jiang, S. (2014). Structure-based discovery of Middle East respiratory syndrome coronavirus fusion inhibitor. Nature Communications , 5, 3067. https://doi.org/10.1038/ncomms4067
- Nichols, J., & Smith, A. (2009). Naive and primed pluripotent states. Cell Stem Cell , 4(6), 487–492. https://doi.org/10.1016/j.stem.2009.05.015
- Nichols, J., & Smith, A. (2011). The origin and identity of embryonic stem cells. Development (Cambridge, England) , 138(1), 3–8. https://doi.org/10.1242/dev.050831
- Ohgushi, M., Matsumura, M., Eiraku, M., Murakami, K., Aramaki, T., Nishiyama, A., Muguruma, K., Nakano, T., Suga, H., Ueno, M., Ishizaki, T., Suemori, H., Narumiya, S., Niwa, H., & Sasai, Y. (2010). Molecular pathway and cell state responsible for dissociation-induced apoptosis in human pluripotent stem cells. Cell Stem Cell , 7(2), 225–239. https://doi.org/10.1016/j.stem.2010.06.018
- Phandthong, R., Wong, M., Song, A., Martinez, T., & Talbot, P. (2022a). New insights into how popular electronic cigarette aerosols and aerosol constituents affect SARS-CoV-2 infection of human bronchial epithelial cells. Scientific Reports , 13(1), 5807. https://doi.org/10.1038/s41598-023-31592-x
- Phandthong, R., Wong, M., Song, A., Martinez, T., & Talbot, P. (2022b). Does vaping increase the likelihood of SARS-CoV-2 infection? Paradoxically Yes and No. BioRxiv , 2022.09.09.507373. https://doi.org/10.1101/2022.09.09.507373
- Ricardo, R., & Phelan, K. (2008). Counting and determining the viability of cultured cells. Journal of visualized experiments: JoVE , (16), 752. https://doi.org/10.3791/752
- Talbot, P., & Lin, S. (2011). Mouse and human embryonic stem cells: Can they improve human health by preventing disease? Current Topics in Medicinal Chemistry , 11(13), 1638–1652. https://doi.org/10.2174/156802611796117621
- Vivanti, A. J., Vauloup-Fellous, C., Prevot, S., Zupan, V., Suffee, C., Do Cao, J., Benachi, A., & de Luca, D. (2020). Transplacental transmission of SARS-CoV-2 infection. Nature Communications , 11(1), 3572. https://doi.org/10.1038/s41467-020-17436-6
- Watanabe, K., Ueno, M., Kamiya, D., Nishiyama, A., Matsumura, M., Wataya, T., Takahashi, J. B., Nishikawa, S., Nishikawa, S. -. I., Muguruma, K., & Sasai, Y. (2007). A ROCK inhibitor permits survival of dissociated human embryonic stem cells. Nature Biotechnology , 25(6), 681–686. https://doi.org/10.1038/nbt1310
- Weatherbee, B. A. T., Glover, D. M., & Zernicka-Goetz, M. (2020). Expression of SARS-CoV-2 receptor. Open Biology , 10(8), 200162. https://doi.org/10.1098/rsob.200162
- Weng, N. J., Cheung, C., & Talbot, P. (2018). Dynamic blebbing: A bottleneck to human embryonic stem cell culture that can be overcome by Laminin-Integrin signaling. Stem Cell Research , 33, 233–246. https://doi.org/10.1016/j.scr.2018.10.022
- Young, E. M., Green, O., Stewart, J., King, Y., O'Donoghue, K., Walker, K. F., & Thornton, J. G. (2022). COVID-19 and pregnancy: A comparison of case reports, case series and registry studies. European Journal of Obstetrics, Gynecology, and Reproductive Biology , 268, 135–142. https://doi.org/10.1016/j.ejogrb.2021.12.002