Dissecting the Biology of the Fungal Wheat Pathogen Zymoseptoria tritici: A Laboratory Workflow
Wagner C. Fagundes, Wagner C. Fagundes, Janine Haueisen, Janine Haueisen, Eva H. Stukenbrock, Eva H. Stukenbrock
Abstract
The fungus Zymoseptoria tritici is one of the most devastating pathogens of wheat. Aside from its importance as a disease-causing agent, this species has emerged as a powerful model system for evolutionary genetic studies of crop-infecting fungal pathogens. Z. tritici exhibits exceptionally high levels of genetic and phenotypic diversity as well as morphological plasticity, which can make experimental studies and comparability of results obtained in different laboratories, e.g., from infection assays, challenging. Therefore, standardized experimental methods are crucial for research on Z. tritici biology and the interaction of this fungus with its wheat host. Here, we describe a suite of well-tested and optimized protocols ranging from isolation of Z. tritici field specimens to analyses of virulence assays under controlled conditions. Several biological and technical aspects of working with Z. tritici under laboratory conditions are considered and carefully described in each protocol. © 2020 The Authors.
Basic Protocol 1 : Purification of Z. tritici field isolates from leaf material
Basic Protocol 2 : Molecular identification of Z. tritici isolates
Support Protocol 1 : Rapid extraction of Z. tritici genomic DNA
Support Protocol 2 : Extraction of high-quality Z. tritici genomic DNA
Basic Protocol 3 : In vitro culture and long-term storage of Z. tritici isolates
Basic Protocol 4 : Analysis of Z. tritici virulence in wheat
Support Protocol 3 : Preparation of Z. tritici inoculum
INTRODUCTION
The ascomycete fungus Zymoseptoria tritici (synonym: Mycosphaerella graminicola) is the causal agent of the foliar disease Septoria tritici blotch (STB), one of the most devastating diseases of wheat. This plant pathogenic fungus infects wheat worldwide and can cause up to 50% yield losses per year in the major European Union wheat-growing countries (Fones & Gurr, 2015). Z. tritici is a member of the Dothideomycetes, a class of fungi comprising several thousand species, including many plant pathogens (Goodwin, Waalwijk, & Kema, 2004, 2011). Members of this order are generally heterothallic, with sexual (teleomorph) and asexual (anamorph) stages, and plant-infecting species are found on a wide range of monocot and dicot hosts (Goodwin et al., 2004). Z. tritici has a hemibiotrophic lifestyle, with a prolonged biotrophic phase followed by a necrotic phase where plant cell death occurs and necrotic lesions develop (Ponomarenko, Goodwin, & Kema, 2011).
Population genetic and genomic studies based on different types of genetic and genomic markers have documented high levels of genetic variation in populations of Z. tritici , even within single fields and individual lesions (Banke, Peschon, & McDonald, 2004; Hartmann, McDonald, & Croll, 2018; Jürgens, Linde, & McDonald, 2006; Linde, Zhan, & McDonald, 2002; Zhan, Pettway, & McDonald, 2003). Moreover, the species history has been well documented, and it was shown that speciation of the wheat pathogen coincided with domestication of the wheat host (Stukenbrock, Banke, Javan-Nikkhah, & McDonald, 2007). The high-quality genome sequence of the reference isolate IPO323 comprises 13 core and eight accessory chromosomes that are fully assembled from telomere to telomere (Goodwin et al., 2011). This reference genome has provided a unique resource for several comparative genome studies as well as transcriptomic and epigenomic analyses (Badet, Oggenfuss, Abraham, McDonald, & Croll, 2020; Haueisen et al., 2019; Möller et al., 2019).
Given the economic importance of Z. tritici , there is an urgent need to better understand the biology of this fungus. Here, we present some considerations for field collections and experimental work with Z. tritici , and we discuss the need for standardized protocols for research on this important pathogen. First of all, experimental work with Z. tritici has to account for the excessive amounts of genetic and phenotypic diversity. The high genetic diversity found among and within Z. tritici populations is reflected in phenotypic diversity. Virulence of Z. tritici is quantitative and determined by the extent of host damage and the density of asexual fruiting structures, or so-called pycnidia. Necrotic leaf area and pycnidia density on susceptible wheat cultivars can vary significantly between isolates including progenies originating from the same sexual cross (Abrinbana, 2017; Habig, Quade, & Stukenbrock, 2017; Stewart & McDonald, 2014; Zhan et al., 2005). Moreover, variation among replicate leaves can be substantial. Comparative analyses of virulence phenotypes between Z. tritici isolates or mutant strains therefore rely on robust methods to quantify virulence traits. Automated leaf image–based analyses have been established as a more precise measure for virulence quantification that ideally should be applied for in planta studies of Z. tritici (Karisto et al., 2018; Stewart & McDonald, 2014; Stewart et al., 2016).
Typical STB symptoms can appear on leaves of seedling to adult wheat plants and are usually easy to spot. However, other wheat diseases with similar symptoms can co-occur in the same field, including the second major Septoria disease, Septoria nodorum blotch, caused by the Dothideomycete fungus Parastagonospora nodorum (synonyms: S. nodorum , Phaeosphaeria nodorum) (Fig. 1A). Co-occurring pycnidia of the two species have been reported, and P. nodorum may occasionally be confounded with Z. tritici (Fig. 1B) in the field (Eyal, Scharen, Prescott, & van Ginkel, 1987). To this end, collections of Z. tritici in the field should ideally be accompanied by species confirmation of the retrieved isolates.
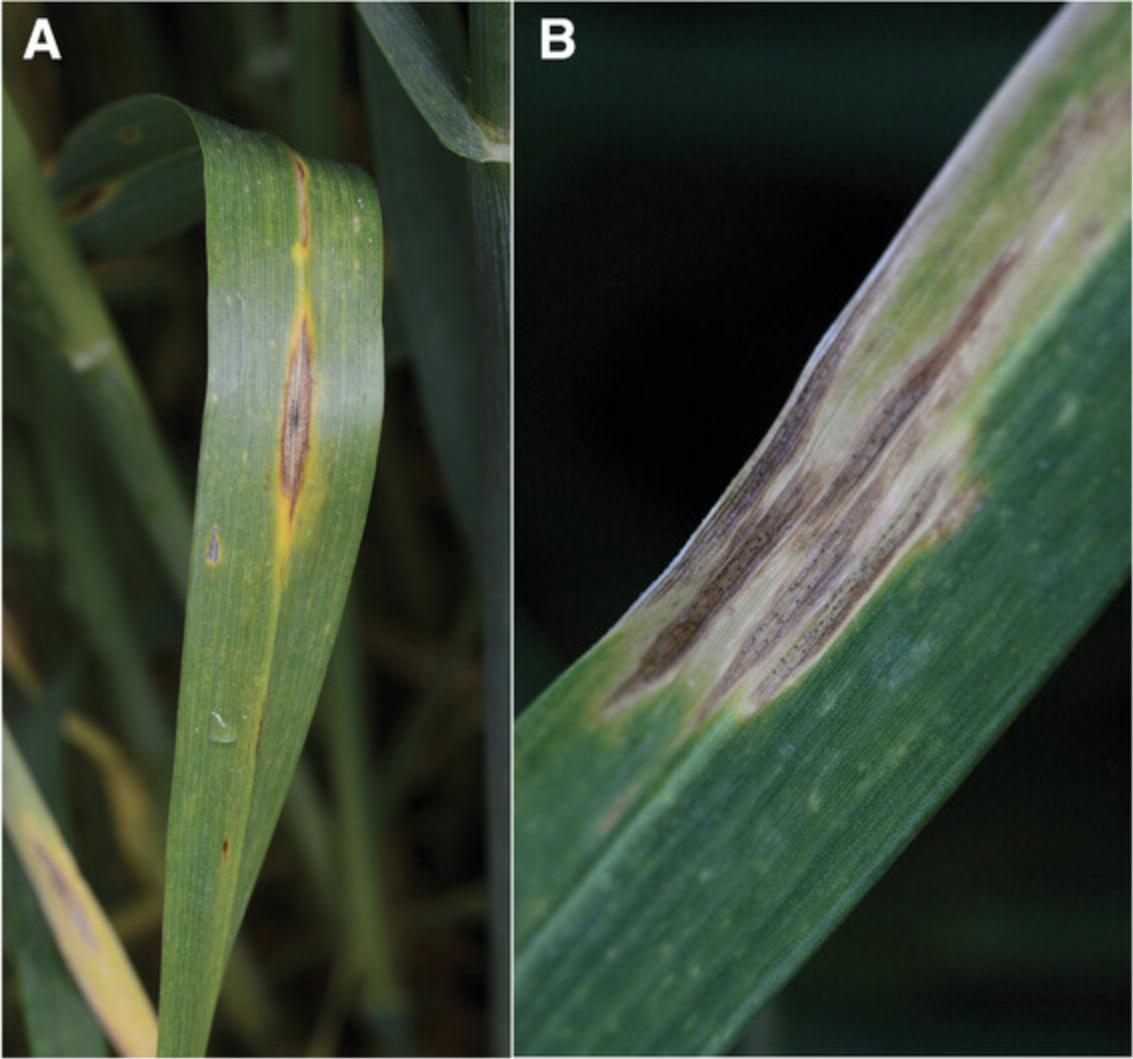
Although Z. tritici is amenable to laboratory conditions, appropriate growth conditions and maintenance of fungal cultures are extremely important for reproducible experiments. Z. tritici is a pleomorphic fungus that can grow as yeast-like blastospores, filamentous hyphae, or chlamydospores (Francisco, Ma, Zwyssig, McDonald, & Palma-Guerrero, 2019). The underlying molecular and genetic bases for this variation in growth morphology are so far not fully understood; however, specific culture conditions may induce the shift from one to another morphotype. As mentioned above, the genome of Z. tritici comprises a set of accessory chromosomes that can be readily lost during mitotic cell divisions in vitro as well as in planta (Möller, Habig, Freitag, & Stukenbrock, 2018). Given this chromosome instability, it is important to maintain Z. tritici isolates under the right conditions for long-term storage and thereby avoid continuous and unnecessary mitotic growth, which may result in a population of aneuploid cells (cells with aberrant chromosome sets).
Considering the above-mentioned aspects and the use of Z. tritici as a model organism, the protocols described here outline important details for practical handling of Z. tritici under laboratory and greenhouse/phytochamber conditions. These protocols can serve either as a baseline for researchers initiating work with Z. tritici or as a reference to make experiments more comparable across different research groups. The protocols included here present a workflow “from the field to the laboratory bench” and describe isolation and confirmation of Z. tritici specimens from wheat samples (Basic Protocols 1 and 2 as well as Support Protocols 1 and 2), culture and long-term storage of Z. tritici isolates (Basic Protocol 3), and inoculation and analysis of Z. tritici virulence on wheat plants (Basic Protocol 4 and Support Protocol 3).
Basic Protocol 1: PURIFICATION OF Z. tritici FIELD ISOLATES FROM LEAF MATERIAL
Population genetic analyses of Z. tritici at different spatial scales have revealed high levels of genetic variation even within single lesions (Linde et al., 2002). Depending on the specific research objectives, this variation should already be considered when sampling Z. tritici in the field. For a representative sampling of genetic variation, a hierarchical sampling design can be applied, in which infected wheat leaves are collected from individual sub-plots along a transect (McDonald, 1997). Most importantly, samples should be properly labeled with a code that allows derivation of the origin of the isolate and that considers the different levels of sampling (field, plot, sub-plot, plant, leaf, lesion).
Fresh leaves can immediately be used for Z. tritici isolation, or leaves can be mounted on paper sheets and stored for later isolations. Such “herbarium” specimens should be well dried and stored in a dry and dark place at room temperature, where they can be kept up to several months until isolations are performed. Due to the destructive nature of isolation, we advise recording information about the leaf by taking a photograph before it is used for isolation, numbering each leaf as well as each lesion on each leaf separately (Fig. 2A). In this way, it will later be possible to trace back to the original virulence phenotype, e.g., as lesion size, pycnidia density, and overall appearance of the leaf.
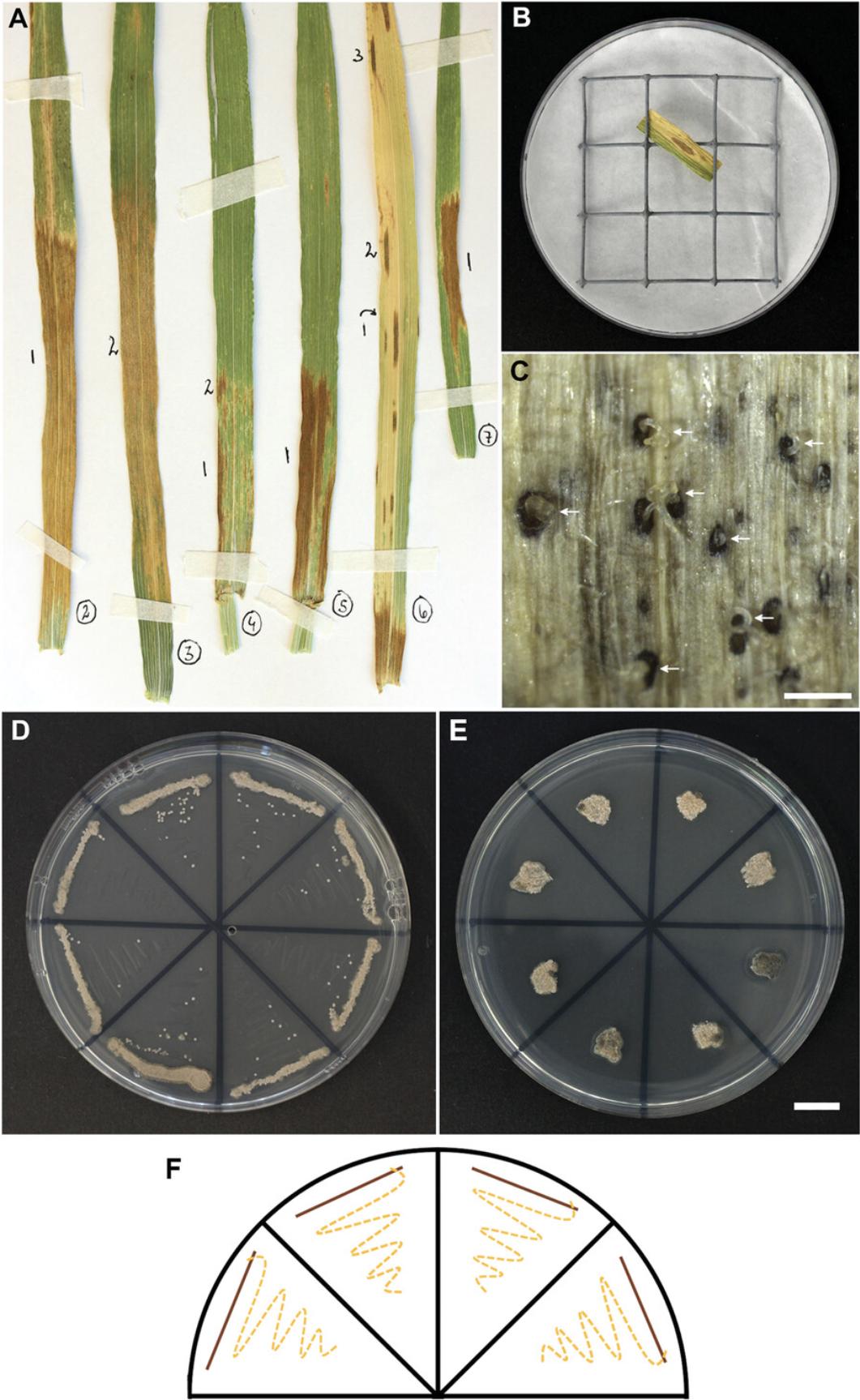
NOTE : We recommend performing the following method of isolation using aseptic techniques under a laminar flow hood and/or close to a Bunsen burner.
Materials
-
Sterile water
-
1.2% (w/v) sodium hypochlorite in aqueous solution
-
Tween® 20 (e.g., Roth, cat. no. 9127.1)
-
70% (v/v) ethanol
-
Leaf samples with necrotic lesions containing pycnidia
-
YMS agar plates with and without 50 µg/ml kanamycin (see recipe)
-
Sterile tweezers
-
Sterile Whatman paper or filter paper (disc-shaped to fit in petri dish)
-
Sterile petri dishes
-
Sterile metal grid or equivalent
-
50-ml Falcon tubes
-
Sterile scissors
-
Parafilm
-
Microbiological incubator with temperature control, 18°C
-
Dissecting microscope
-
Sterilized fine dissecting needles or fine syringe needles (e.g., insulin needles, Gr. 14, Braun, cat. no. 4657640) (see step 9)
-
Sterile toothpicks
-
Sterile 1000-µl pipet tips (optional)
1.Using sterile tweezers, put a sterile Whatman or filter paper inside bottom of a sterile petri dish for each sample. Place a sterile metal grid or equivalent on top of it and wet paper with 2 to 3 ml sterile water.
2.For each sample, prepare the following for leaf surface sterilization in 50-ml Falcon tubes: 1.2% sodium hypochlorite in aqueous solution with a few drops of Tween® 20, 70% ethanol with a few drops Tween® 20, and two tubes of sterile water.
3.Excise 3- to 4-cm sections from leaf material (leaf samples with necrotic lesions containing pycnidia) using sterile scissors and select sections with lesions and pycnidia.
4.Submerge excised leaf sections in the 1.2% sodium hypochlorite solution and wash for 3 min by gently inverting each tube.
5.Transfer leaves with sterile tweezers into 70% ethanol and wash for 15 s by gently inverting each tube.
6.Transfer leaves with sterile tweezers into the first tube of sterile water and wash leaves for ≥30 s by gently inverting each tube. Repeat wash step in the second tube of sterile water.
7.Place surface-sterilized leaves onto the metal grid inside a petri dish (see step 1) using sterile tweezers, as shown in Figure 2B. Prepare one petri dish per leaf section.
8.Seal dishes with Parafilm and incubate at 20°C with a 16-hr light period until pycnidiospores are released in cirrhi (a mucilage that groups spores together; Fig. 2C), usually after 5 days.
9.Under a dissecting microscope, pick pycnidiospores (emerging from one pycnidium in one cirrhus) with a sterilized fine dissecting needle or fine syringe needle.
10.Transfer pycnidiospores to YMS agar plates with 50 µg/ml kanamycin by gently dipping the needle with the picked spores into the agar.
11.Seal plates with Parafilm and incubate at 18°C for 3 to 7 days, until small colonies are visible.
12.Pick cells from a colony with a sterile toothpick and streak out onto a new YMS agar plate with 50 µg/ml kanamycin.
13.On the same new YMS agar plate from step 12, use another sterile toothpick to streak out a second continuous line in “zig-zag” (Fig. 2D and 2F). Seal plates and incubate at 18°C for 5 to 7 days, until single colonies appear around “zig-zag” line.
14.Repeat steps 12 and 13 on YMS agar plates without kanamycin using cells from a well-separated single colony.
15.Pick a single colony from this last round of isolation and spread it onto a small surface area (∼4 mm2; Fig. 2E) on a fresh YMS agar plate without kanamycin using a sterile 1000-µl pipet tip or toothpick. Incubate plates for 5 days at 18°C. Use cells grown in this colony for any downstream procedures, such as species confirmation (see Basic Protocol 2) and inoculation of cultures for long-term storage (see Basic Protocol 3).
Basic Protocol 2: MOLECULAR IDENTIFICATION OF Z. tritici ISOLATES
Z. tritici species identification solely based on disease symptoms and in vitro phenotypes is challenging, so we recommend verifying the species identity of isolates obtained from field collections. To this end, polymerase chain reaction (PCR) followed by amplicon sequencing of a conserved nuclear locus (e.g., internal transcribed spacer rDNA, beta-tubulin, actin) represents a well-established procedure to discriminate Z. tritici specimens from other plant-associated fungi (Crous, Aptroot, Kang, Braun, & Wingfield, 2000; Fraaije, Lovell, Rohel, & Hollomon, 1999; Quaedvlieg et al., 2011). Based on the following protocol, isolates can be readily confirmed by PCR and amplicon sequencing using only small amounts of cells from the last single-colony isolation step in Basic Protocol 1 and a “rapid DNA extraction” method (Support Protocol 1). Once PCR and amplicon sequencing are done, online databases, alignment tools, and software such as NCBI (https://www.ncbi.nlm.nih.gov), BLAST (https://blast.ncbi.nlm.nih.gov), Geneious (https://www.geneious.com), and Blast2GO (https://www.blast2go.com) can be used to align the sequences and support species identification by DNA sequence homology. The following PCR protocol uses crude template genomic DNA (gDNA) from a rapid extraction method (Support Protocol 1) and primer pairs for two loci, ITS and beta-tubulin (TUB2), that have been described previously (Glass & Donaldson, 1995; O'Donnell & Cigelnik, 1997; Weir, Johnston, & Damm, 2012; White, Bruns, Lee, & Taylor, 1990) (Table 1).
Locus | Primer | Orientation | Sequence (5′-3′) | Length (bp) | Reference |
---|---|---|---|---|---|
ITS | ITS5 | Forward | GGAAGTAAAAGTCGTAACAAGG | 22 | White et al., 1990 |
ITS | ITS4 | Reverse | TCCTCCGCTTATTGATATGC | 20 | White et al., 1990 |
TUB2 | T1 | Forward | AACATGCGTGAGATTGTAAGT | 21 | O'Donnell & Cigelnik, 1997 |
TUB2 | Bt2b | Reverse | ACCCTCAGTGTAGTGACCCTTGGC | 24 | Glass & Donaldson, 1995 |
NOTE : Experiments involving PCR require extremely careful technique to prevent contamination.
Materials
-
Template gDNA (see Support Protocol 1)
-
5× Phusion High Fidelity (HF) Buffer (e.g., NEB, cat. no. B0518S)
-
50 mM magnesium chloride (MgCl2; e.g., NEB, cat. no. B0510A)
-
100% dimethylsulfoxide (DMSO; e.g., NEB, cat. no. B0515)
-
5 M betaine (e.g., Sigma, cat. no. B0300-1VL)
-
10 mM deoxynucleotide (dNTP) mix
-
100 pmol/µl forward and reverse oligonucleotide primers
-
2 U/µl Phusion DNA polymerase (e.g., NEB, cat. no. M0530S)
-
Sterile water
-
2% (w/v) agarose gel with nucleic acid gel stain in 1× TAE buffer (from 50× TAE buffer; see recipe)
-
1× TAE buffer (from 50× TAE buffer; see recipe)
-
1-kb or 50-bp DNA ladder (e.g., GeneRuler 1 kb Plus or 50 bp, Thermo Fisher, cat. no. SM1331 or SM0371)
-
PCR cleanup kit (e.g., Wizard SV Gel and PCR Clean-Up System, Promega, cat. no. A9282; optional; alternatively, use cleanup services at commercial sequencing facility)
-
PCR tubes or plates
-
Thermocycler
-
Electrophoresis apparatus
-
Additional reagents and equipment for agarose gel electrophoresis (see Current Protocols article; Armstrong & Schulz, 2015) and Sanger sequencing at sequencing facility
1.Maintain all reagents and template gDNA on ice. Mix the following reagents in a PCR tube or plate well to a final reaction volume of 30 µl for each reaction:
- 15 µl template gDNA
- 6 µl 5× Phusion HF Buffer (final concentration: 1×)
- 0.75 µl 50 mM MgCl2 (final concentration: 1.25 mM)
- 1 µl DMSO (final concentration: 3.3%)
- 6 µl 5 M betaine (final concentration: 1 M)
- 0.5 µl 10 mM dNTP mix (final concentration of each dNTP: 0.17 mM)
- 0.1 µl 100 pmol/µl forward oligonucleotide primer (final concentration: 0.3 pmol/µl)
- 0.1 µl 100 pmol/µl reverse oligonucleotide primer (final concentration: 0.3 pmol/µl)
- 0.5 µl 2 U/µl Phusion DNA polymerase (final concentration: 0.03 U/µl)
- 0.05 µl sterile water
2.Preheat thermocycler to 98°C prior to entering the reaction tubes/plates and set lid temperature to 98°C to avoid condensation in lids. For the PCR reactions to amplify the target DNA, use the following program:
Initial step: | 30 s | 98°C (initial denaturation) |
35 cycles: | 8 s | 98°C (denaturation) |
20 s | x°C (annealing) | |
30 s | 72°C (extension) | |
8 min | 72°C (final extension). |
3.Separate PCR products on a 2% agarose gel with nucleic acid gel stain in 1× TAE buffer in an electrophoresis apparatus containing 1× TAE buffer. Set electrophoresis run to 120 V for 1 hr. Include controls and a 1-kb or 50-bp DNA ladder.
4.Clean up PCR products or gel fragments prior to sequencing using a PCR cleanup kit following the manufacturer's recommendations or cleanup services at a commercial sequencing facility.
5.Submit PCR products for Sanger sequencing using the same primers as used for the PCR reactions.
6.Use ITS and beta-tubulin sequences for alignments and similarity searches.
Support Protocol 1: RAPID EXTRACTION OF Z. tritici GENOMIC DNA
In order to facilitate the molecular identification of newly isolated fungal specimens (Basic Protocol 2), here we describe an easy method for PCR-quality gDNA extraction that is inexpensive and much faster compared to traditional protocols. It is a modified version of the previously published “HotSHOT” DNA extraction protocol (Truett et al., 2000). Besides being short, this protocol does not use potentially harmful organic compounds like chloroform and phenol and requires a low number of cells. These cells can easily be retrieved from the last step of single-colony isolation (Basic Protocol 1, step 15). It is important to note that the gDNA extract is crude and only suitable for amplification of products <1 kb. This protocol is not appropriate to isolate gDNA for whole-genome sequencing or for more delicate PCR-based analyses (e.g., amplification of genetic markers like inter-simple sequence repeats). For such applications, isolation of high-quality DNA as described in Support Protocol 2 is recommended.
NOTE : Considering the chemicals used in this protocol, all the steps can be performed on the laboratory bench using regular latex gloves.
Materials
-
Blastospores from axenic fungal cultures (e.g., from YMS agar plates with Z. tritici single colonies; see Basic Protocol 1, step 15)
-
25 mM sodium hydroxide (NaOH)
-
40 mM Tris·HCl, pH 5.5
-
Sterile toothpicks
-
PCR tubes or plates
-
98°C thermocycler or heat block
1.Pick a small number of cells (blastospores from axenic fungal cultures), approximately one-eighth of a single colony grown on YMS (see Fig. 2E), with a sterile toothpick and resuspend in 50 µl of 25 mM NaOH in a PCR tube or plate.
2.Incubate samples at 98°C for 10 min in a thermocycler or heat block.
3.Add 50 µl of 40 mM Tris·HCl (pH 5.5) to each sample.
4.Store samples at −20°C until further use.
5.Thaw DNA extracts on ice prior to use in Basic Protocol 2.
Support Protocol 2: EXTRACTION OF HIGH-QUALITY Z. tritici GENOMIC DNA
The following protocol describes a cetyltrimethylammonium bromide (CTAB)-based method to isolate high-quality, high-molecular-weight gDNA from Z. tritici blastospores (Fig. 3A; Basic Protocol 3, step 2). This DNA can be used as input for genome sequencing libraries for short-read (e.g., Illumina) and long-read (e.g., SMRT sequencing by PacBio) technologies as well as for a broad range of molecular biology techniques, including PCR-based genotyping and cloning. We observe that DNA extracted using this protocol results in better overall sequencing quality compared to sequencing of DNA, e.g., extracted using a phenol/chloroform-based protocol. This protocol is a modified version of the CTAB method described by Allen, Flores-Vergara, Krasynanski, Kumar, & Thompson (2006).
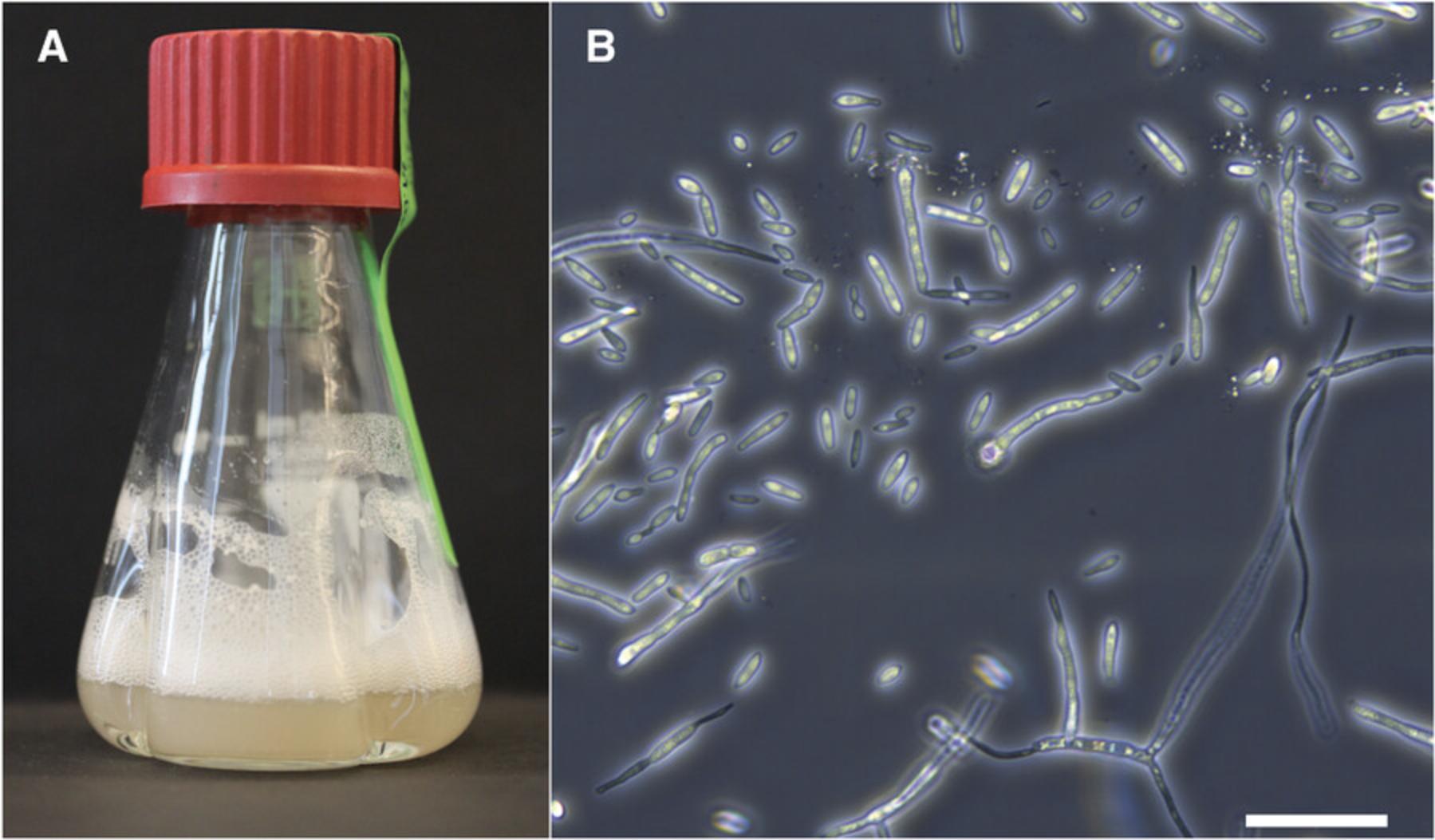
CAUTION : Considering the handling of toxic organic compounds and liquid nitrogen, appropriate safety precautions, use of protective equipment (laboratory coat, goggles, and phenol-proof gloves), and working inside a chemical fume hood are extremely important.
Materials
-
Densely grown liquid Z. tritici cultures (OD600nm = 2.8 to 3.2; see Basic Protocol 3, step 2)
-
Liquid nitrogen
-
1:1 (v/v) phenol/chloroform
-
Isopropanol, −20°C
-
1× TE buffer, pH 8 (see recipe)
-
10 mg/ml RNase A
-
3 M sodium acetate (NaOAc)
-
100% ethanol, −20°C
-
75% (v/v) ethanol
-
0.8% (w/v) agarose gel with nucleic acid gel stain in 1× TAE buffer (from 50× TAE buffer; see recipe)
-
1× TAE buffer (from 50× TAE buffer; see recipe)
-
1-kb DNA ladder (e.g., GeneRuler 1 kb Plus, Thermo Fisher, cat. no. SM1331)
-
Fluorometer DNA assay kit (broad range, measuring nucleic acid concentrations from 100 pg/µl to 1000 ng/µl; e.g., Qubit dsDNA BR Assay Kit, Thermo Fisher, cat. no. Q32853)
-
Water bath with temperature control
-
50-ml Falcon tubes
-
Thermo Scientific Heraeus Biofuge Stratos (cat. no. 10305002) with a fixed-angle rotor (Thermo Scientific HIGHConic, cat. no. 10203802)
-
Mortar and pestle (DNA- and DNase-free by sterilization at 180°C for 6 hr or chemical treatment)
-
Sterile 2-ml microcentrifuge tubes
-
Small spatulas (that can fit into 2-ml microcentrifuge tube)
-
Styrofoam box
-
Vortex (e.g., Vortex-Genie 2, Scientific Industries, cat. no. SI-0236)
-
Microcentrifuge
-
Nutator shaker platform (e.g., Grant-bio Sunflower Mini-Shaker, model PS-3D)
-
37°C thermomixer (for 2-ml microcentrifuge tubes; e.g., Eppendorf Thermomixer, Fisher Scientific, cat. no. 15346551)
-
Electrophoresis apparatus
-
Fluorometer (e.g., Qubit 4, Thermo Fisher, cat. no. Q33226)
-
Additional reagents and equipment for preparing DNA extraction buffer (see recipe) and for agarose gel electrophoresis (see Current Protocols article; Armstrong & Schulz, 2015)
1.Set temperature of the water bath to 65°C.
2.Prepare fresh DNA extraction buffer (see recipe) and pre-warm to 65°C in water bath.
3.Transfer densely grown liquid Z. tritici culture (∼20 ml) to a 50-ml Falcon tube. Centrifuge 10 min at 4000 rpm in a Thermo Scientific Heraeus Biofuge Stratos with a fixed-angle rotor and remove supernatant.
4.Grind cells in liquid nitrogen using a pre-cooled mortar and pestle. Ensure that cells are ground to a fine powder and that no frozen clumps remain.
5.Transfer 100 to 200 mg cell powder (approximately until the 400-µl volume mark) into a pre-cooled, sterile 2-ml microcentrifuge tube using a pre-cooled small spatula. Use a different spatula for different samples. Close microcentrifuge tube and quickly place into a Styrofoam box containing liquid nitrogen.
6.Add 1.2 ml pre-warmed DNA extraction buffer (see step 2) to each sample. Vortex briefly.
7.Incubate sample at 65°C for 1 hr in the water bath (see step 1). Mix tube every 10 min by inversion.
8.Centrifuge sample for 10 min at 16,200 × g at room temperature.
9.Transfer supernatant (approximately 700 to 800 µl) to a new sterile 2-ml microcentrifuge tube containing 800 µl of 1:1 phenol/chloroform.
10.Place sample on a nutator shaker platform and gently shake for 20 min.
11.Centrifuge sample for 10 min at 16,200 × g at room temperature.
12.Transfer aqueous phase (top phase) to a new sterile 2-ml microcentrifuge tube and repeat phenol/chloroform extraction (steps 9 to 11).
13.Transfer aqueous phase (top phase) to a new sterile 2-ml microcentrifuge tube containing 800 µl cold isopropanol (−20°C). Mix several times by inversion (no shaking).
14.Incubate sample for 15 min at −20°C followed by 10 min at room temperature for DNA precipitation.
15.Centrifuge sample for 10 min at 16,200 × g at room temperature.
16.Gently remove supernatant and resuspend pellet in 250 µl of 1× TE buffer (pH 8).
17.Add 25 µl of 10 mg/ml RNase A to each sample and incubate at 37°C for 30 min.
18.Add 25 µl of 3 M NaOAc and mix briefly by inversion. Add 600 µl of cold 100% ethanol (–20°C), mix, and incubate sample for ≥1 hr at −20°C to precipitate DNA.
19.Centrifuge sample for 10 min at 16,200 × g at room temperature.
20.Gently remove supernatant and wash pellet two times with 500 µl of 75% ethanol.
21.Centrifuge sample for 5 min at 16,200 × g at room temperature.
22.Remove supernatant and air-dry pellet for ≥20 min at room temperature.
23.Dissolve DNA pellet in 50 to 100 µl of 1× TE buffer (pH 8) by incubating the sample in the thermomixer at 37°C and 300 rpm for 45 min.
24.Check DNA purity by gel electrophoresis using a 0.8% agarose gel with nucleic acid gel stain in 1× TAE buffer in an electrophoresis apparatus containing 1× TAE buffer. Run gel at 100 V for 45 min. Include a 1-kb DNA ladder.
25.Quantify DNA concentration using a fluorometer and fluorometer DNA assay kit following the manufacturer's recommendations.
26.Keep DNA sample at 4°C for short-term storage or at −20°C for long-term storage prior to use in any analysis that requires high-quality DNA (e.g., cloning, preparation of genome sequencing libraries).
Basic Protocol 3: IN VITRO CULTURE AND LONG-TERM STORAGE OF Z. tritici ISOLATES
Proper storage and maintenance of new Z. tritici isolates (Basic Protocol 1) are important to keep isolates viable and to ensure their genomic integrity. Cells of isolates that are stored under cryogenic conditions can be preserved for decades and therefore serve as valuable resources to answer different research questions throughout history (Hartmann, Sánchez-Vallet, McDonald, & Croll, 2017; Oggenfuss et al., 2020). The Dutch Z. tritici isolate IPO323 was originally isolated in 1981 and has been used as a reference in several studies worldwide since then (Kema & Van Silfhout, 1997). For most experiments, including preparation of long-term stocks, blastospore cultures (“yeast-like” morphology) are used as input. For many isolates, these cells can be obtained from cultures grown in nutrient-rich medium (e.g., yeast malt sucrose, or YMS, medium) at 18°C (Fig. 3).
This protocol describes cultivation and long-term cryopreservation of Z. tritici blastospores and can be initiated using a small number of cells from single-spore colonies immediately after isolation (Basic Protocol 1). Considering the importance of long-term storage stocks, we recommend preparing the cultures using aseptic techniques in a laminar flow hood. Additionally, we propose preparing two distinct sets of cryo-stocks: a safe “backup” stock that will only be used in cases of great necessity and an “in use” stock, which will be used routinely to cultivate cells for experiments. Digital records of the stored isolates can be combined with different types of metadata (e.g., location and date of isolation, original wheat cultivar).
Materials
-
YMS agar plate with Z. tritici single colony (see Basic Protocol 1, step 15)
-
Liquid YMS medium (see recipe)
-
Sterile silica (e.g., Sigma-Aldrich, cat. no. 60741; sterilized at 180°C for 6 hr)
-
Liquid YMS-glycerol medium (see recipe)
-
Liquid nitrogen
-
Sterile toothpicks
-
Sterile culture tubes or sterile Erlenmeyer flasks with baffles
-
Orbital shaker with adapter for culture tubes or flasks and temperature control, 18°C
-
Spectrophotometer
-
Sterile 2-ml cryovials
-
Small spatula (that can fit inside 2-ml cryovials)
-
Shaker with adapter for cryovials (e.g., IKA VXR Basic Vibrax, cat. no. 0002819000 & 0020018016)
1.Pick a small number of cells (from a YMS agar plate with Z. tritici single colony), e.g., approximately one-fourth of a single colony grown on YMS (Fig. 2E), using a sterile toothpick and inoculate ≥5 ml liquid YMS medium in a sterile culture tube or a sterile Erlenmeyer flask with baffles. Label tube with the isolate ID.
2.Incubate cultures using an orbital shaker with an adapter for culture tubes or flasks at 18°C and 200 rpm for 5 days or until the cultures are densely grown (OD600nm = 2.8 to 3.2 via a spectrophotometer) (Fig. 3A).
3.Prepare two or more sterile 2-ml cryovials for each isolate, with vials for both “backup” and “in use” stocks, as follows:
-
Silica stocks: Scoop sterile silica into cryovials using a small spatula until the vials are filled to the 300-µl mark.
-
Glycerol stocks: Add 900 µl liquid YMS-glycerol medium to the cryovials.
Glycerol stocks are easier to handle and are recommended for “in use” stocks.
4.Add 50 and 900 µl of densely grown culture from step 2 to the cryovials containing silica and YMS-glycerol, respectively. Close cryovial caps tightly.
5.Mix liquid culture and YMS-glycerol for the glycerol stocks thoroughly by inversion. Likewise, mix culture and silica for the silica stocks for ≥5 min on a shaker with an adapter for cryovials.
6.Snap-freeze stock cultures using liquid nitrogen and store tubes at −80°C.
7.Keep cryo-stocks on ice or in a cooled rack during use.
8.Remake “in use” stocks before these stocks run too low to avoid samples loss, preferentially retrieving material for culture inoculation from the safe “back up” stocks.
Basic Protocol 4: ANALYSIS OF Z. tritici VIRULENCE IN WHEAT
Ranging from virulence quantification to mutant phenotyping, in planta infection assays are an important component of many Z. tritici studies. The infection outcome, namely wheat susceptibility or qualitative or quantitative resistance, can provide information about the presence or absence of avirulence factors in Z. tritici isolates as well as about the relevance of candidate gene products during host infection (Brown, Chartrain, Lasserre-Zuber, & Saintenac, 2015). For example, the wheat cultivar Chinese Spring harbors the resistance gene Stb6 , which confers resistance against isolates carrying the avirulence factor AvrStb6 , like the Z. tritici reference isolate IPO323 (Kema et al., 2018; Saintenac et al., 2018; Zhong et al., 2017). Hence, Chinese Spring can be used to study the molecular and physiological mechanisms of wheat resistance against Z. tritici AvrStb6 genotypes. Infection of other highly susceptible wheat cultivars can be used to compare the virulence of different Z. tritici genotypes. The cultivars Obelisk, Riband, and Drifter are highly susceptible to IPO323 and to a large number of other isolates and are therefore commonly used in pathogenicity assays to quantify and compare virulence (Chartrain, Brading, & Brown, 2005; Habig et al., 2017; Haueisen et al., 2019; Karisto, Dora, & Mikaberidze, 2019; Stewart & McDonald, 2014; Zhong et al., 2017).
Previous studies revealed that quantitative virulence of Z. tritici can be highly variable within and between experiments, generally indicated by a broad data distribution for quantitative measures like pycnidia density (Habig et al., 2017; Stewart & McDonald, 2014). Thus, technical and experimental replicates are extremely important to be able to discern treatment effects from background variation. We recommend using a minimum of two biological replicates (e.g., two independent deletion mutants) and two technical replicates (e.g., two independent experiments) to obtain at least 30 leaves per treatment and replicate (Z. tritici isolates or mock). Randomization within experiments (randomization of pot placement and randomization of treatment order) is required to minimize confounding effects of environmental factors and experimental procedures. Furthermore, cross-contaminations or other abiotic and biotic biological aspects (e.g., senescence) that may influence the final results must be monitored by the inclusion of a negative control (mock treatment). In addition, observer's bias can be avoided by making the experimenter unaware of the treatment type throughout the whole experiment.
The following protocol describes a method to quantitatively evaluate Z. tritici virulence in wheat hosts under controlled greenhouse or growth chamber conditions. This protocol can be used whenever phenotyping in planta is required. The complete workflow is summarized in Figure 4.
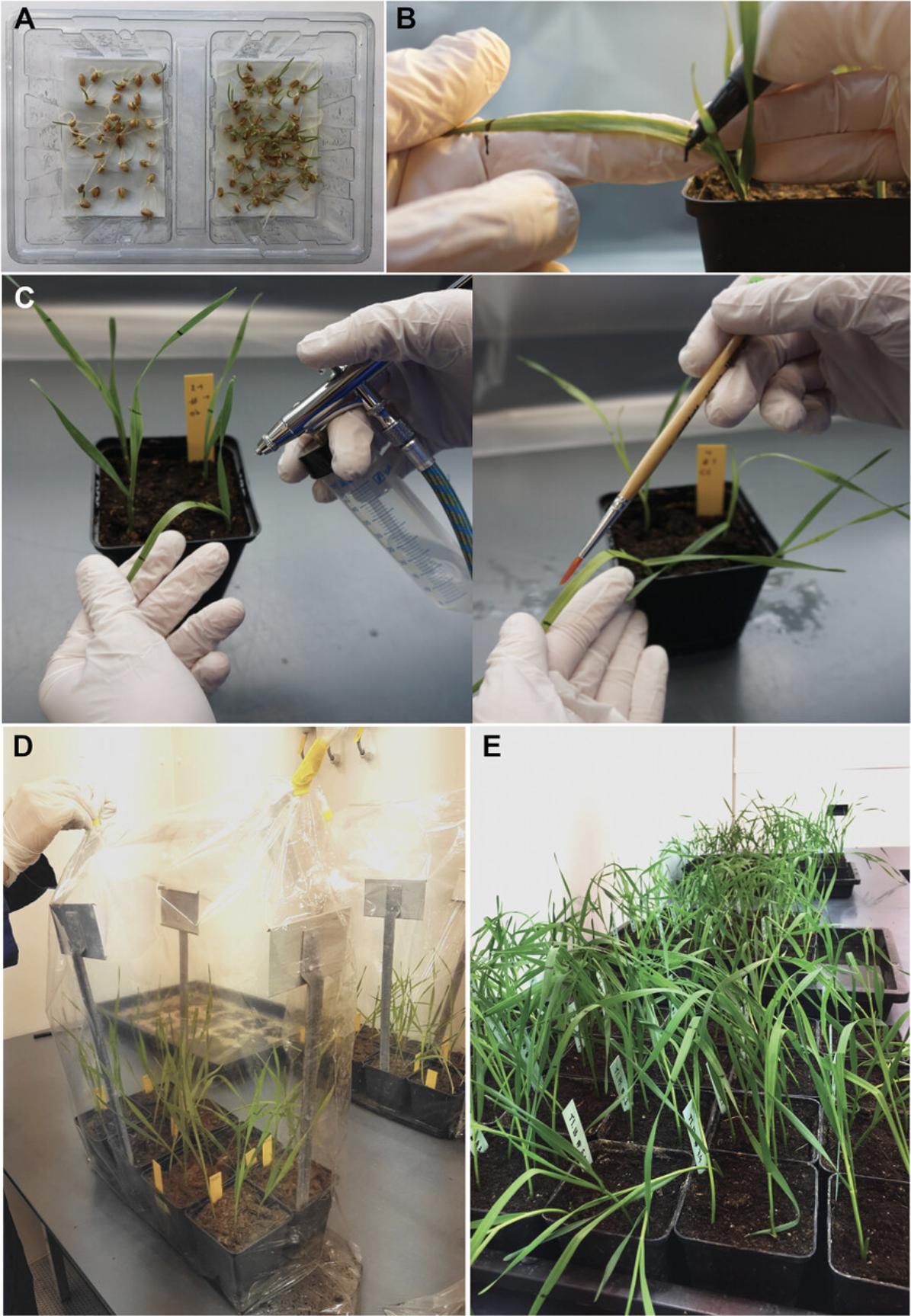
NOTE : Use of latex gloves and goggles is recommended during the inoculation procedures.
Materials
-
Sterile water
-
Wheat seeds
-
Peat potting soil (e.g., Fruhstorfer Erde Typ T, Hawita, cat. no. 01020)
-
70% (v/v) ethanol
-
Z. tritici inoculum (see Support Protocol 3)
-
0.1% (v/v) Tween® 20 (e.g., Roth, cat. no. 9127.1) in sterile water
-
Whatman paper
-
Plastic box (for seed germination; e.g., size 11 × 7 cm)
-
Growth chamber and/or greenhouse
-
Pots and trays (for plant growth; e.g., 9 × 9–cm pots; 60 × 40–cm trays)
-
Plastic tags
-
Small dibble (optional)
-
Permanent black pen with soft tip (e.g., Lumocolor permanent, Staedtler, cat. no. 317-9)
-
Spray gun (from airbrush spray set; 0.5-mm needle, 0.5-mm nozzle, and air hose and compressor; e.g., Timbertech set, cat. no. ABPST06)
-
Large plastic bags (e.g., size 60 × 80 cm)
-
Tape or plastic locking clips
-
White paper sheets (A4 or letter format, depending on scanner)
-
White regular tape
-
Scissors
-
Flatbed scanner (e.g., HP Photosmart C4580; HP for A4 format)
-
ImageJ software
1.Initiate germination of wheat seeds: Place Whatman paper at bottom of a plastic box and add sterile water to moisten it (∼12.5 ml is appropriate for an 11 × 7–cm box). Place wheat seeds inside box and close it. Germinate seeds for 4 days under plant growth conditions (20°C and a 16-hr light period) in a growth chamber (Fig. 4A).
2.Fill pots with peat potting soil and organize them on trays. Add a plastic tag with information about wheat cultivar, treatment (e.g., anonymized isolate and replicate ID), and date to each pot.
3.To plant wheat seedlings, first wet soil in the pots. Make four holes in soil (∼1 cm in depth), e.g., by using a small dibble or a finger. Transplant 4-day old seedlings (see step 1) into holes and cover roots with soil.
4.Grow seedlings for 7 days in soil prior to inoculation. Water plants regularly by pouring water in the trays instead of overhead watering.
5.One day before inoculation, mark a distinct section (e.g., 5 to 10 cm) on adaxial side of the second leaf of each plant using a permanent black pen with a soft tip (Fig. 4B).
6.On the inoculation day, organize pots for each treatment and ensure that all pots are properly labeled.
7.To clean the spray gun, spray 70% ethanol 20 to 30 times for 2 to 3 s each followed by spraying sterile water 20 to 30 times for 2 to 3 s each using 2.0 bar of pressure.
8.To inoculate the plants for each treatment, use cleaned spray gun and a pressure of 2.0 bar to inoculate the marked leaf sections of each plant with the Z. tritici inoculum by evenly spraying until runoff (Fig. 4C). Clean spray gun after each treatment as described in step 7.Follow same procedure for the mock treatment by spraying 0.1% Tween® 20 in sterile water onto the marked leaf sections.
9.Let inoculum dry on leaf surface (∼15 min) and place pots (sorted by treatment) in large plastic bags containing water (∼1 L). Seal bags using tape or plastic locking clips to generate an environment with maximal relative humidity (Fig. 4D). Incubate plants in the bags at ∼20°C (day)/∼12°C (night) and a 16-hr day/8-hr night cycle under controlled greenhouse conditions. Alternatively, in growth chambers, use constant conditions of 20°C and 16 hr of light (∼200 µmol/m2/s).
10.After 48 hr, remove pots from the bags and place plants back onto the trays (Fig. 4E). Ensure randomized placement. Water regularly and maintain a relative humidity of 70% to 90%. Grow wheat for 21 days post-inoculation (dpi) at the same temperature and light conditions as described in step 9.
11.At 21 dpi, harvest inoculated leaf sections by cutting ∼1 cm above and below the pen marks using scissors and mount leaves to white paper sheets using white regular tape.
12.Scan paper sheets using a flatbed scanner, scanning each leaf individually. Use an image resolution of 2400 dots per inch.
13.Analyze scanned images using ImageJ software (Schneider, Rasband, & Eliceiri, 2012) and the published macros for Z. tritici virulence quantification (Karisto et al., 2018; Stewart & McDonald, 2014; Stewart et al., 2016).
14.Compare virulence readouts (e.g., PLACL, normalized pycnidia count) within and between treatments using statistical tests in the R environment (R Core Team, 2013) or Open Office Calc (https://www.openoffice.org/product/calc.html).
Support Protocol 3: PREPARATION OF Z. tritici INOCULUM
In addition to their use in long-term cryo-stocks (Basic Protocol 3), Z. tritici blastospore cultures (“yeast-like” form) are routinely required to prepare inoculum for virulence assays (Basic Protocol 4). The following protocol describes a method to initiate blastospore culture, collect the cells, and adjust their density for plant infections. This protocol should be started 5 days prior to plant inoculation (Basic Protocol 4).
Materials
-
Z. tritici glycerol stock of Z. tritici isolate to be inoculated (see Basic Protocol 3)
-
YMS agar plate without kanamycin (see recipe)
-
Sterile water
-
70% (v/v) ethanol
-
0.1% Tween® 20 (e.g., Roth, cat. no. 9127.1) in sterile water
-
Sterile pipet tips and/or inoculation loops
-
Microbiological incubator with temperature control, 18°C
-
Sterile 2-ml microcentrifuge tubes
-
Vortex (e.g., Vortex-Genie 2, Scientific Industries, cat. no. SI-0236)
-
Hemocytometer and coverslip (e.g., Neubauer improved chamber, 0.100 mm, Marienfeld Superior, cat. no. 0640010)
-
Manual counter (i.e., tally counter)
-
Compound microscope
-
Sterile 50-ml Falcon tube
1.Retrieve Z. tritici glycerol stock of Z. tritici isolates to be inoculated from the −80°C freezer.
2.Dip a sterile pipet tip in soft (not entirely thawed) Z. tritici glycerol culture and transfer cells to a YMS agar plate without kanamycin. Using the pipet tip or a sterile inoculation loop, spread glycerol culture onto a small surface area (∼4 mm2).
3.Incubate plate for 5 days at 18°C and constant darkness.
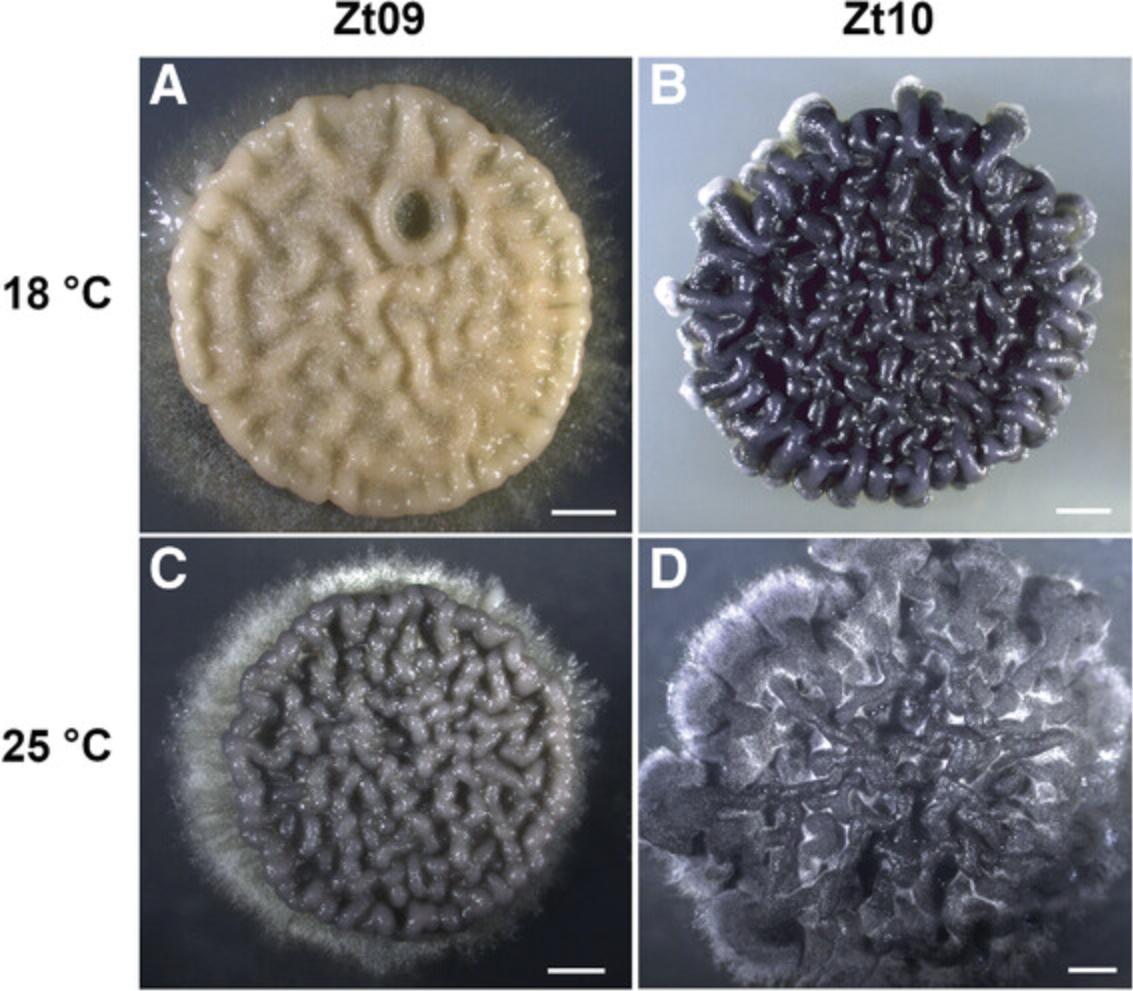
4.Scrape cells from the colony surface using a sterile pipet tip and resuspend them in a sterile 2-ml microcentrifuge tube containing 1 ml sterile water. Swirl pipet tip to release the cells and ensure that the suspension is homogeneous (no cell clumps).
5.Make at least two serial dilutions (1:10 and 1:100) of blastospore suspension in additional sterile 2-ml microcentrifuge tubes. Vortex tubes briefly between dilutions.
6.Carefully clean hemocytometer and coverslip with 70% ethanol before use and position coverslip correctly (indicated by the appearance of Newton's rings). Select appropriate dilution, briefly vortex blastospore suspension, and pipet 10 µl into each compartment of hemocytometer.
7.Observe cells using a compound microscope (e.g., with a 10× objective lens).
8.Count number of cells using a manual counter. Record cell number.
9.Calculate cell density considering the average number of counted cells, the specifications of the hemocytometer in use, and the dilution of the cell suspension that was counted.
10.Adjust inoculum to the required cell density (e.g., 106 or 107 cells/ml) in 0.1% Tween® 20 in sterile water. Keep final inoculum in a sterile 50-ml Falcon tube and use within a few hours.
REAGENTS AND SOLUTIONS
DNA extraction buffer
For 10 ml buffer, mix 1 ml 1 M Tris·HCl (Roth, cat. no. 9090.3), pH 8.0; 2.8 ml 5 M NaCl (Roth, cat. no. 3957.2); and 400 µl 0.5 M EDTA (Roth, cat. no. 8043.2), pH 8.0, using a stir bar. Completely dissolve 0.2 g cetyltrimethylammonium bromide (CTAB; Roth, cat. no. 9161.1) in 3 ml deionized water. Add CTAB solution to the buffer, mix well, and bring up volume to 10 ml with deionized water. Add 50 µl β-mercaptoethanol (Sigma-Aldrich, cat. no. M3148) before use. Prepare fresh immediately before use.
Liquid YMS-glycerol medium
For 1 L medium, dissolve 4 g yeast extract (BD, cat. no. 212720), 4 g malt extract (Roth, cat. no. AE68.1), and 4 g sucrose (Roth, cat. no. 4621.2) in ∼200 ml deionized water. Add 894.85 g of 98% (v/v) glycerol (Roth, cat. no. 7530.4) and stir well. Bring up volume to 1000 ml using deionized water. Aliquot in 100-ml autoclavable glass bottles and autoclave for 5 min at 121°C. Store ≤3 months at room temperature.
Liquid YMS medium
For 1 L liquid YMS medium, solve 4 g yeast extract (BD, cat. no. 212720), 4 g malt extract (Roth, cat. no. AE68.1), and 4 g sucrose (Roth, cat. no. 4621.2) in ∼200 ml deionized water. Stir well and bring up volume to 1000 ml using deionized water. Aliquot in autoclavable glass bottles and autoclave for 5 min at 121°C. Store ≤3 months at room temperature.
TAE buffer (Tris-acetate-EDTA), 50×
For 1 L buffer, add 242 g Tris (Roth, cat. no. 4855.3), 57.1 ml pure acetic acid (Roth, cat. no. 3738.4), and 100 ml 0.5 M EDTA (Roth, cat. no. 8043.2), pH 8.0, to 500 ml deionized water. Adjust pH to 8.3 with NaOH. Mix well using a stir bar and bring up volume to 1000 ml using deionized water.
Dilute to a working concentration of 1×, e.g., by diluting 40 ml 50× TAE buffer in 1960 ml deionized water. Store ≤1 year at room temperature.
TE buffer (Tris-EDTA) (pH 8), 1×
For 100 ml buffer, mix 200 µl 0.5 M EDTA (Roth, cat. no. 8043.2), pH 8.0, and 1 ml 1 M Tris·HCl (Roth, cat. no. 9090.3), pH 8.0, in 50 ml deionized water. Adjust pH to 8.0 with HCl if necessary and bring up volume to 100 ml with deionized water. Autoclave buffer for 5 min at 121°C. Store ≤1 year at room temperature.
YMS agar plates with and without 50 µg/ml kanamycin
For YMS solid medium, add 16 g agar (BD, cat. no. 214030) to an autoclavable 1-L glass bottle and add liquid YMS medium (see recipe) to 800 ml total volume. Add a stir bar and autoclave for 5 min at 121°C. Let cool down while stirring and pour plates under sterile conditions inside a laminar flow hood. Store ≤3 months at 4°C.
For YMS solid medium with 50 µg/ml kanamycin, cool down medium to ∼50°C and add appropriate volume of kanamycin stock. Stir well and pour plates immediately. Store ≤1 month at 4°C.
COMMENTARY
Background Information
Isolates of Z. tritici can switch their growth morphology when environmental conditions change. The fungus grows as blastospores (a “yeast-like” form), as filamentous hyphae, or as chlamydospores, depending on nutrient availability and temperature (Francisco et al., 2019). The molecular pathways that induce these morphological transitions are still largely unknown. Optimal culture conditions, i.e., nutrient-rich medium and an incubation temperature of 18°C, generally promote the formation of blastospores. Stress conditions such as nutrient-limited medium and temperatures between 25°C and 28°C induce filamentous hyphal growth in the Z. tritici reference strain IPO323 (Francisco et al., 2019; Motteram et al., 2011). It is possible that different morphotypes of the same isolate or mutant show different phenotypes in experimental assays. Hence, it is necessary to use standardized growth conditions to obtain consistent phenotypic results. Most laboratory approaches and infection experiments require yeast-like Z. tritici cells. Above, we have outlined protocols that produce such blastospore cultures for long-term storage (Basic Protocol 3) and as inoculum (Support Protocol 3) for in planta virulence assays (Basic Protocol 4).
In vitro growth and storage conditions influence the phenotype but can also impact the genome architecture of Z. tritici isolates. Exceptionally high rates of chromosome losses have been reported for Z. tritici after in vitro growth for only 3 weeks, and chromosome instability increased even more when the temperature was elevated to 28°C (Möller et al., 2018). These observations are biologically fascinating but clearly underline the importance of appropriate growth and storage conditions wherein karyotypes are maintained intact. Not only can chromosomes be lost, but spontaneous mutations may also occur. We observed this in a derived lineage of IPO323: the Z. tritici isolate Zt09, shown in Figure 5, has lost chromosome 18 as well as having partially lost the white collar complex (WCC) transcription factor gene homolog wc-1 during in vitro propagation (Habig et al., 2019; Kellner et al., 2014; Möller et al., 2018).
The phenotypic plasticity that we observe in Z. tritici (Fig. 5) can explain the high level of variability between replicates in experiments and can bias comparative analyses of mutant and wild-type isolates. Phenotypic changes may be caused by small fluctuations in the local environment of the fungus. Abiotic factors like foliar temperature and moisture have been shown to affect infection outcomes and overall STB disease dynamics (Bernard, Sache, Suffert, & Chelle, 2013; Boixel, Gélisse, Marcel, & Suffert, 2019). Biotic factors like host senescence, or the natural yellowing of leaves triggered by stress or aging-related factors, can also interfere with the quantification of Z. tritici virulence (Suffert, Sache, & Lannou, 2013). Hence, virulence phenotyping based on pycnidia densities has become more meaningful than leaf color–based readouts like PLACL and provides an indirect estimate of Z. tritici fitness. Experimental factors like wheat cultivar, inoculum cell density, and inoculum suspension time can further influence infection success (Fones, Steinberg, & Gurr, 2015; Kay, Fones, & Gurr, 2019; Suffert et al., 2013). Although many environmental and technical aspects can influence Z. tritici quantitative virulence, the method of inoculation (spray inoculation or paint-brush inoculation; Basic Protocol 4) seems to have little or no impact. We find that both methods can be used equivalently. However, we recommend that the two methods are not used in combination within one experiment. When establishing Z. tritici experimental infections, we recommend performing test infection experiments to select and adapt the inoculation method based on the Z. tritici isolates and the plant growth conditions that are available.
Although widely used as a model organism, many aspects of the biology of Z. tritici and its interaction with wheat are still largely unknown. Hence, the use of standardized experimental protocols is crucial for Z. tritici research. We hope that the suite of protocols, from field to laboratory, presented here can serve to improve comparability of experimental results between research groups and may provide a valuable guide for researchers new to Z. tritici research.
Critical Parameters and Troubleshooting
As emphasized throughout this article, a range of biological and technical aspects can have significant impacts on Z. tritici data and their interpretation. Genetic and phenotypic diversity, morphological plasticity, and large variation in virulence responses are common characteristics of Z. tritici and can bias the interpretation of results if not accounted for.
Chromosome losses and mutations are possible during prolonged periods of mitotic cell divisions in Z. tritici (Habig et al., 2019; Möller et al., 2018). Thus, strict temporal limitation of in vitro cultivation and proper long-term storage of Z. tritici , as shown in Basic Protocol 3, are essential to avoid the occurrence of aneuploidy and mutation accumulation in laboratory strains. Together with these measures, karyotype analyses using pulsed-field gel electrophoresis (PFGE) (Habig et al., 2017; Haueisen et al., 2019) and qualitative PCR assays using specific primer pairs for each accessory chromosome (Habig et al., 2017) can be applied to assess changes in chromosome composition.
In virulence assays of Z. tritici , quantitative disease measures like pycnidia density and lesion size can vary greatly between isolates (Habig et al., 2017; Stewart & McDonald, 2014). As discussed in Basic Protocol 4, experimental replicates and randomization of treatments are important to reduce both variability within Z. tritici treatments and “background noise” caused by abiotic and biotic factors in the experiment. Additionally, the use of experimental controls is extremely important. Negative-control treatments, i.e., “mock” controls, compensate for possible artifacts due to abiotic or technical influences and may help to spot possible cross-contaminations between treatments, mainly when using spray inoculation methods. A control for reduced virulence together with a positive control for disease can also be helpful, for example the Z. tritici Mg3LysM deletion mutant, which is impaired in leaf colonization and pycnidia production compared to a wild-type strain (Lee, Rudd, Hammond-Kosack, & Kanyuka, 2014; Marshall et al., 2011).
Apart from technical and experimental aspects, robust virulence quantification methods are essential for Z. tritici pathogenicity assays (Basic Protocol 4). High-throughput analysis of scanned leaf images provides a robust and reliable method (Karisto et al., 2018; Stewart & McDonald, 2014; Stewart et al., 2016). This yields readouts of disease symptoms (based on PLACL, for example) and an indirect measure of the potential degree of Z. tritici reproduction in host tissues (i.e., based on pycnidia density per leaf area) that are more accurate and precise than manual visual methods. Image analysis has two main advantages over visual estimates: (i) objectivity and reproducibility independent from the observer/experimenter and (ii) quantitative readouts that allow for more powerful statistical analysis methods. As for any other automated analyses, few adjustments (e.g., healthy leaf and lesion color thresholds) are necessary depending on the conditions of the scanned samples. Additionally, the results should always be double-checked for any spurious outcomes. Soil particles in scanned leaves, in particular, can introduce bias, as they can be counted as pycnidia and give an overestimation of the normalized pycnidia density values.
Understanding Results
Please see the introductions of the individual protocols and the Critical Parameters and Troubleshooting section for this information.
Time Considerations
Please see the time considerations in the individual protocols, including potential stopping points.
Acknowledgments
The authors would like to thank Michael Habig and Mareike Möller for helpful comments on the previous versions of the protocols and Marcello Zara for support in optimizing the CTAB DNA extraction protocol. This work was supported by the Canadian Institute for Advanced Research (CIFAR) and a Max Planck fellowship to EHS.
Open access funding enabled and organized by Projekt DEAL.
Author Contributions
Wagner C. Fagundes : Conceptualization; investigation; methodology; validation; visualization; writing-original draft; writing-review & editing. Janine Haueisen : Conceptualization; formal analysis; investigation; methodology; supervision; writing-review & editing. Eva H. Stukenbrock : Conceptualization; funding acquisition; investigation; methodology; project administration; resources; supervision; writing-review & editing.
Literature Cited
- Abrinbana, M. (2017). Variation in aggressiveness components of Zymoseptoria tritici populations in Iran. Journal of Phytopathology , 166(1), 10–17. doi: 10.1111/jph.12654.
- Allen, G. C., Flores-Vergara, M. A., Krasynanski, S., Kumar, S., & Thompson, W. F. (2006). A modified protocol for rapid DNA isolation from plant tissues using cetyltrimethylammonium bromide. Nature Protocols , 1(5), 2320–2325. doi: 10.1038/nprot.2006.384.
- Altman, D., & Bland, J. (1995). Statistics notes: The normal distribution. BMJ , 310, 298. doi: 10.1136/bmj.310.6975.298.
- Armstrong, J. A., and Schulz, J. R. (2015). Agarose gel electrophoresis. Current Protocols Essential Laboratory Techniques , 10, 7.2.1–7.2.22. doi: 10.1002/9780470089941.et0702s10.
- Badet, T., Oggenfuss, U., Abraham, L., McDonald, B. A., & Croll, D. (2020). A 19-isolate reference-quality global pangenome for the fungal wheat pathogen Zymoseptoria tritici. BMC Biology , 18(1), 1–18. doi: 10.1186/s12915-020-0744-3.
- Banke, S., Peschon, A., & McDonald, B. A. (2004). Phylogenetic analysis of globally distributed Mycosphaerella graminicola populations based on three DNA sequence loci. Fungal Genetics and Biology , 41(2), 226–238. doi: 10.1016/j.fgb.2003.09.006.
- Bernard, F., Sache, I., Suffert, F., & Chelle, M. (2013). The development of a foliar fungal pathogen does react to leaf temperature! New Phytologist , 198(1), 232–240. doi: 10.1111/nph.12134.
- Boixel, A., Gélisse, S., Marcel, T. C., & Suffert, F. (2019). Differential tolerance to changes in moisture regime during early infection stages in the fungal pathogen Zymoseptoria tritici. bioRxiv. doi: 10.1101/867572.
- Brown, J. K. M., Chartrain, L., Lasserre-Zuber, P., & Saintenac, C. (2015). Genetics of resistance to Zymoseptoria tritici and applications to wheat breeding. Fungal Genetics and Biology , 79, 33–41. doi: 10.1016/j.fgb.2015.04.017.
- Chartrain, L., Brading, P. A., & Brown, J. K. M. (2005). Presence of the Stb6 gene for resistance to Septoria tritici blotch (Mycosphaerella graminicola) in cultivars used in wheat-breeding programmes worldwide. Plant Pathology , 54(2), 134–143. doi: 10.1111/j.1365-3059.2005.01164.x.
- Conover, W. J., & Iman, R. L. (1981). Rank transformations as a bridge between parametric and nonparametric statistics. The American Statistician , 35(3), 124–129. doi: 10.1080/00031305.1981.10479327.
- Crous, P. W., Aptroot, A., Kang, J. C., Braun, U., & Wingfield, M. J. (2000). The genus Mycosphaerella and its anamorphs. Studies in Mycology , 2000(45), 107–121.
- Eyal, Z., Scharen, A. L., Prescott, J. M., & van Ginkel, M. (1987). The septoria diseases of wheat: Concepts and methods of disease management. Mexico: CIMMYT.
- Fones, H., & Gurr, S. (2015). The impact of Septoria tritici Blotch disease on wheat: An EU perspective. Fungal Genetics and Biology , 79, 3–7. doi: 10.1016/j.fgb.2015.04.004.
- Fones, H. N., Steinberg, G., & Gurr, S. J. (2015). Measurement of virulence in Zymoseptoria tritici through low inoculum-density assays. Fungal Genetics and Biology , 79, 89–93. doi: 10.1016/j.fgb.2015.03.020.
- Fraaije, B. A., Lovell, D. J., Rohel, E. A., & Hollomon, D. W. (1999). Rapid detection and diagnosis of Septoria tritici epidemics in wheat using a polymerase chain reaction/PicoGreen assay. Journal of Applied Microbiology , 86(4), 701–708. doi: 10.1046/j.1365-2672.1999.00716.x.
- Francisco, C. S., Ma, X., Zwyssig, M. M., McDonald, B. A., & Palma-Guerrero, J. (2019). Morphological changes in response to environmental stresses in the fungal plant pathogen Zymoseptoria tritici. Scientific Reports , 9(1), 1–18. doi: 10.1038/s41598-019-45994-3.
- Glass, N. L., & Donaldson, G. C. (1995). Development of primer sets designed for use with the PCR to amplify conserved genes from filamentous ascomycetes. Applied and Environmental Microbiology , 61(4), 1323–1330. doi: 10.1128/aem.61.4.1323-1330.1995.
- Goodwin, S. B., M'Barek, S. B., Dhillon, B., Wittenberg, A. H. J., Crane, C. F., Hane, J. K., … Kema, G. H. J. (2011). Finished genome of the fungal wheat pathogen Mycosphaerella graminicola reveals dispensome structure, chromosome plasticity, and stealth pathogenesis. PLOS Genetics , 7(6), e1002070. doi: 10.1371/journal.pgen.1002070.
- Goodwin, S. B., Waalwijk, C., & Kema, G. H. J. (2004). Genetics and genomics of Mycosphaerella graminicola, a model for the Dothideales. In D. K. Arora, & G. G. Khachatourians (Eds.), Applied Mycology and Biotechnology. Volume 4: Fungal Genomics (pp. 315–330). Elsevier. doi: 10.1016/S1874-5334(04)80016-9.
- Habig, M., Bahena-Garrido, S. M., Barkmann, F., Haueisen, J., & Stukenbrock, E. H. (2019). The transcription factor Zt107320 affects the dimorphic switch, growth and virulence of the fungal wheat pathogen Zymoseptoria tritici. Molecular Plant Pathology , 21(1), 124–138. doi: 10.1111/mpp.12886.
- Habig, M., Quade, J., & Stukenbrock, E. H. (2017). Forward genetics approach reveals host genotype-dependent importance of accessory chromosomes in the fungal wheat pathogen Zymoseptoria tritici. mBio , 8(6), 1–16. doi: 10.1128/mBio.01919-17.
- Hartmann, F. E., McDonald, B. A., & Croll, D. (2018). Genome-wide evidence for divergent selection between populations of a major agricultural pathogen. Molecular Ecology , 27(12), 2725–2741. doi: 10.1111/mec.14711.
- Hartmann, F. E., Sánchez-Vallet, A., McDonald, B. A., & Croll, D. (2017). A fungal wheat pathogen evolved host specialization by extensive chromosomal rearrangements. ISME Journal , 11(5), 1189–1204. doi: 10.1038/ismej.2016.196.
- Haueisen, J., Möller, M., Eschenbrenner, C. J., Grandaubert, J., Seybold, H., Adamiak, H., & Stukenbrock, E. H. (2019). Highly flexible infection programs in a specialized wheat pathogen. Ecology and Evolution , 9(1), 275–294. doi: 10.1002/ece3.4724.
- Jürgens, T., Linde, C. C., & McDonald, B. A. (2006). Genetic structure of Mycosphaerella graminicola populations from Iran, Argentina and Australia. European Journal of Plant Pathology , 115(2), 223–233. doi: 10.1007/s10658-006-9000-0.
- Karisto, P., Dora, S., & Mikaberidze, A. (2019). Measurement of infection efficiency of a major wheat pathogen using time-resolved imaging of disease progress. Plant Pathology , 68(1), 163–172. doi: 10.1111/ppa.12932.
- Karisto, P., Hund, A., Yu, K., Anderegg, J., Walter, A., Mascher, F., … Mikaberidze, A. (2018). Ranking quantitative resistance to Septoria tritici blotch in elite wheat cultivars using automated image analysis. Phytopathology , 108(5), 568–581. doi: 10.1094/PHYTO-04-17-0163-R.
- Kay, W. T., Fones, H. N., & Gurr, S. J. (2019). Rapid loss of virulence during submergence of Z. tritici asexual spores. Fungal Genetics and Biology , 128, 14–19. doi: 10.1016/j.fgb.2019.03.004.
- Kellner, R., Bhattacharyya, A., Poppe, S., Hsu, T. Y., Brem, R. B., & Stukenbrock, E. H. (2014). Expression profiling of the wheat pathogen Zymoseptoria tritici reveals genomic patterns of transcription and host-specific regulatory programs. Genome Biology and Evolution , 6(6), 1353–1365. doi: 10.1093/gbe/evu101.
- Kema, G. H. J., Mirzadi Gohari, A., Aouini, L., Gibriel, H. A. Y., Ware, S. B., van Den Bosch, F., … Seidl, M. F. (2018). Stress and sexual reproduction affect the dynamics of the wheat pathogen effector AvrStb6 and strobilurin resistance. Nature Genetics , 50(March), 1–6. doi: 10.1038/s41588-018-0052-9.
- Kema, Gert H. J., & van Silfhout, C. H. (1997). Genetic variation for virulence and resistance in the wheat-Mycosphaerella graminicola pathosystem III. Comparative seedling and adult plant experiments. Phytopathology , 87(3), 266–272. doi: 10.1094/PHYTO.1997.87.3.266.
- Large, E. C. (1954). Growth stages in cereals illustration of the Feekes scale. Plant Pathology , 3(4), 128–129. doi: 10.1111/j.1365-3059.1954.tb00716.x.
- Lee, W. S., Rudd, J. J., Hammond-Kosack, K. E., & Kanyuka, K. (2014). Mycosphaerella graminicola LysM effector-mediated stealth pathogenesis subverts recognition through both cerk1 and cebip homologues in wheat. Molecular Plant-Microbe Interactions , 27(3), 236–243. doi: 10.1094/MPMI-07-13-0201-R.
- Levene, H. (1961). Robust tests for equality of variances. In I. Olkin et al. (Eds.), Contributions to probability and statistics: Essays in honor of Harold Hotelling (pp. 279–292). Palo Alto, CA: Stanford University Press.
- Linde, C. C., Zhan, J., & McDonald, B. A. (2002). Population structure of Mycosphaerella graminicola : From lesions to continents. Phytopathology , 92(9), 946–955. doi: 10.1094/phyto.2002.92.9.946.
- Marshall, R., Kombrink, A., Motteram, J., Loza-Reyes, E., Lucas, J., Hammond-Kosack, K. E., … Rudd, J. J. (2011). Analysis of two in planta expressed LysM effector homologs from the fungus Mycosphaerella graminicola reveals novel functional properties and varying contributions to virulence on wheat. Plant Physiology , 156(2), 756–769. doi: 10.1104/pp.111.176347.
- McDonald, B. A. (1997). The population genetics of fungi: Tools and techniques. Phytopathology , 87, 448–453. doi: 10.1094/PHYTO.1997.87.4.448.
- Möller, M., Habig, M., Freitag, M., & Stukenbrock, E. H. (2018). Extraordinary genome instability and widespread chromosome rearrangements during vegetative growth. Genetics , 210(2), 517–529. doi: 10.1534/genetics.118.301050.
- Möller, M., Schotanus, K., Soyer, J. L., Haueisen, J., Happ, K., Stralucke, M., … Stukenbrock, E. H. (2019). Destabilization of chromosome structure by histone H3 lysine 27 methylation. PLOS Genetics , 15(4), e1008093. doi: 10.1371/journal.pgen.1008093.
- Motteram, J., Lovegrove, A., Pirie, E., Marsh, J., Devonshire, J., van de Meene, A., … Rudd, J. J. (2011). Aberrant protein N-glycosylation impacts upon infection-related growth transitions of the haploid plant-pathogenic fungus Mycosphaerella graminicola. Molecular Microbiology , 81(2), 415–433. doi: 10.1111/j.1365-2958.2011.07701.x.
- O'Donnell, K., & Cigelnik, E. (1997). Two divergent intragenomic rDNA ITS2 types within a monophyletic lineage of the fungus Fusarium are nonorthologous. Molecular Phylogenetics and Evolution , 7(1), 103–116. doi: 10.1006/mpev.1996.0376.
- Oggenfuss, U., Badet, T., Wicker, T., Hartmann, F. E., Singh, N. K., Abraham, N., … Biology, M. (2020). A population-level invasion by transposable elements in a fungal pathogen. bioRxiv , doi: 10.1101/2020.02.11.944652.
- Ponomarenko, A., Goodwin, S. B., & Kema, G. H. J. (2011). Septoria tritici blotch (STB) of wheat. Plant Health Instructor , 1–7. doi: 10.1094/PHI-I-2011-0407-01.
- Poppe, S., Dorsheimer, L., Happel, P., & Stukenbrock, E. H. (2015). Rapidly evolving genes are key players in host specialization and virulence of the fungal wheat pathogen Zymoseptoria tritici (Mycosphaerella graminicola). PLOS Pathogens , 11(7), 1–21. doi: 10.1371/journal.ppat.1005055.
- Quaedvlieg, W., Kema, G. H. J., Groenewald, J. Z., Verkley, G. J. M., Seifbarghi, S., Razavi, M., … Crous, P. W. (2011). Zymoseptoria gen. nov.: A new genus to accommodate Septoria -like species occurring on graminicolous hosts. Persoonia: Molecular Phylogeny and Evolution of Fungi , 26, 57–69. doi: 10.3767/003158511X571841.
- R Core Team (2013). R: A language and environment for statistical computing.
- Saintenac, C., Lee, W. S., Cambon, F., Rudd, J. J., King, R. C., Marande, W., … Kanyuka, K. (2018). Wheat receptor-kinase-like protein Stb6 controls gene-for-gene resistance to fungal pathogen Zymoseptoria tritici. Nature Genetics , 50, 1–7. doi: 10.1038/s41588-018-0051-x.
- Schneider, C. A., Rasband, W. S., & Eliceiri, K. W. (2012). NIH Image to ImageJ: 25 years of image analysis. Nature Methods , 9(7), 671–675. doi: 10.1038/nmeth.2089.
- Shapiro, S. S., & Wilk, M. B. (1965). An analysis of variance test for normality (complete samples). Biometrika , 52(3/4), 591. doi: 10.2307/2333709.
- Stewart, E. L., Hagerty, C. H., Mikaberidze, A., Mundt, C. C., Zhong, Z., & McDonald, B. A. (2016). An improved method for measuring quantitative resistance to the wheat pathogen Zymoseptoria tritici using high-throughput automated image analysis. Phytopathology , 106(7), 782–788. doi: 10.1094/PHYTO-01-16-0018-R.
- Stewart, E. L., & McDonald, B. A. (2014). Measuring quantitative virulence in the wheat pathogen Zymoseptoria tritici using high-throughput automated image analysis. Phytopathology , 104(9), 985–992. doi: 10.1094/PHYTO-11-13-0328-R.
- Stukenbrock, E. H., Banke, S., Javan-Nikkhah, M., & McDonald, B. A. (2007). Origin and domestication of the fungal wheat pathogen Mycosphaerella graminicola via sympatric speciation. Molecular Biology and Evolution , 24(2), 398–411. doi: 10.1093/molbev/msl169.
- Suffert, F., Sache, I., & Lannou, C. (2013). Assessment of quantitative traits of aggressiveness in Mycosphaerella graminicola on adult wheat plants. Plant Pathology , 62(6), 1330–1341. doi: 10.1111/ppa.12050.
- Truett, G. E., Heeger, P., Mynatt, R. L., Truett, A. A., Walker, J. A., & Warman, M. L. (2000). Preparation of PCR-quality mouse genomic DNA with hot sodium hydroxide and Tris (HotSHOT). BioTechniques , 29(1), 52–54. doi: 10.2144/00291bm09.
- Tukey, J. W. (1949). Comparing individual means in the analysis of variance. Biometrics , 5(2), 99. doi: 10.2307/3001913.
- Weir, B. S., Johnston, P. R., & Damm, U. (2012). The Colletotrichum gloeosporioides species complex. Studies in Mycology , 73, 115–180. doi: 10.3114/sim0011.
- White, T. J., Bruns, T., Lee, S., & Taylor, J. (1990). Amplification and direct sequencing of fungal ribosomal RNA genes for phylogenetics. PCR Protocols: A Guide to Methods and Applications , 18(1), 315–322.
- Zhan, J., Linde, C. C., Jürgens, T., Merz, U., Steinebrunner, F., & McDonald, B. A. (2005). Variation for neutral markers is correlated with variation for quantitative traits in the plant pathogenic fungus Mycosphaerella graminicola. Molecular Ecology , 14(9), 2683–2693. doi: 10.1111/j.1365-294X.2005.02638.x.
- Zhan, J., Pettway, R. E., & McDonald, B. A. (2003). The global genetic structure of the wheat pathogen Mycosphaerella graminicola is characterized by high nuclear diversity, low mitochondrial diversity, regular recombination, and gene flow. Fungal Genetics and Biology , 38(3), 286–297. doi: 10.1016/S1087-1845(02)00538-8.
- Zhong, Z., Marcel, T. C., Hartmann, F. E., Ma, X., Plissonneau, C., Zala, M., … Palma-Guerrero, J. (2017). A small secreted protein in Zymoseptoria tritici is responsible for avirulence on wheat cultivars carrying the Stb6 resistance gene. New Phytologist , 214(2), 619–631. doi: 10.1111/nph.14434.
Citing Literature
Number of times cited according to CrossRef: 9
- Alma Kokhmetova, Ardak Bolatbekova, Yuliya Zeleneva, Angelina Malysheva, Sholpan Bastaubayeva, Kanat Bakhytuly, Yerlan Dutbayev, Vladimir Tsygankov, Identification of Wheat Septoria tritici Resistance Genes in Wheat Germplasm Using Molecular Markers, Plants, 10.3390/plants13081113, 13 , 8, (1113), (2024).
- Graham Thomas, William T. Kay, Helen N. Fones, Life on a leaf: the epiphyte to pathogen continuum and interplay in the phyllosphere, BMC Biology, 10.1186/s12915-024-01967-1, 22 , 1, (2024).
- Reda Amezrou, Aurélie Ducasse, Jérôme Compain, Nicolas Lapalu, Anais Pitarch, Laetitia Dupont, Johann Confais, Henriette Goyeau, Gert H. J. Kema, Daniel Croll, Joëlle Amselem, Andrea Sanchez-Vallet, Thierry C. Marcel, Quantitative pathogenicity and host adaptation in a fungal plant pathogen revealed by whole-genome sequencing, Nature Communications, 10.1038/s41467-024-46191-1, 15 , 1, (2024).
- Pabitra Joshi, Karansher S. Sandhu, Guriqbal Singh Dhillon, Jianli Chen, Kailash Bohara, Detection and monitoring wheat diseases using unmanned aerial vehicles (UAVs), Computers and Electronics in Agriculture, 10.1016/j.compag.2024.109158, 224 , (109158), (2024).
- Camilla Langlands-Perry, Anaïs Pitarch, Nicolas Lapalu, Murielle Cuenin, Christophe Bergez, Alicia Noly, Reda Amezrou, Sandrine Gélisse, Célia Barrachina, Hugues Parrinello, Frédéric Suffert, Romain Valade, Thierry C. Marcel, Quantitative and qualitative plant-pathogen interactions call upon similar pathogenicity genes with a spectrum of effects, Frontiers in Plant Science, 10.3389/fpls.2023.1128546, 14 , (2023).
- Hadjer Bellah, Nicolas F. Seiler, Daniel Croll, Divergent Outcomes of Direct Conspecific Pathogen Strain Interaction and Plant Co-Infection Suggest Consequences for Disease Dynamics, Microbiology Spectrum, 10.1128/spectrum.04443-22, 11 , 2, (2023).
- M. Amine Hassani, Ernest Oppong-Danquah, Alice Feurtey, Deniz Tasdemir, Eva H. Stukenbrock, Differential Regulation and Production of Secondary Metabolites among Isolates of the Fungal Wheat Pathogen Zymoseptoria tritici, Applied and Environmental Microbiology, 10.1128/aem.02296-21, 88 , 6, (2022).
- Idalia C. Rojas Barrera, Wagner C. Fagundes, Eva H. Stukenbrock, Species of Zymoseptoria (Dothideomycetes) as a Model System to Study Plant Pathogen Genome Evolution, Plant Relationships, 10.1007/978-3-031-16503-0_15, (349-370), (2022).
- Camilla Langlands-Perry, Murielle Cuenin, Christophe Bergez, Safa Ben Krima, Sandrine Gélisse, Pierre Sourdille, Romain Valade, Thierry C. Marcel, Resistance of the Wheat Cultivar ‘Renan’ to Septoria Leaf Blotch Explained by a Combination of Strain Specific and Strain Non-Specific QTL Mapped on an Ultra-Dense Genetic Map, Genes, 10.3390/genes13010100, 13 , 1, (100), (2021).