Differentiation Protocol for 3D Retinal Organoids, Immunostaining and Signal Quantitation
Hannah Döpper, Hannah Döpper, Julia Menges, Julia Menges, Morgane Bozet, Morgane Bozet, Alexandra Brenzel, Alexandra Brenzel, Dietmar Lohmann, Dietmar Lohmann, Laura Steenpass, Laura Steenpass, Deniz Kanber, Deniz Kanber
Abstract
Structures resembling whole organs, called organoids, are generated using pluripotent stem cells and 3D culturing methods. This relies on the ability of cells to self-reorganize after dissociation. In combination with certain supplemented factors, differentiation can be directed toward the formation of several organ-like structures. Here, a protocol for the generation of retinal organoids containing all seven retinal cell types is described. This protocol does not depend on Matrigel, and by keeping the organoids single and independent at all times, fusion is prevented and monitoring of differentiation is improved. Comprehensive phenotypic characterization of the in vitro–generated retinal organoids is achieved by the protocol for immunostaining outlined here. By comparing different stages of retinal organoids, the decrease and increase of certain cell populations can be determined. In order to be able to detect even small differences, it is necessary to quantify the immunofluorescent signals, for which we have provided a detailed protocol describing signal quantitation using the image-processing program Fiji. © 2020 The Authors.
Basic Protocol 1 : Differentiation protocol for 3D retinal organoids
Basic Protocol 2 : Immunostaining protocol for cryosections of retinal organoids
Support Protocol : Embedding and sectioning protocol for 3D retinal organoids
Basic Protocol 3 : Quantitation protocol using Fiji
INTRODUCTION
Human stem cell–based retina models have become popular, as they have opened the road to studying human retinogenesis in development and disease. These models generate retinal organoids that not only contain all seven retinal cell types, but also show distinct layering similar to the in vivo retina, and thus, at present, are the best in vitro model for retinogenesis. Moreover, 3D culturing in combination with CRISPR/Cas technology enable modeling of genetic diseases affecting cells of the retina, and therefore allow drug testing. Several protocols for 3D differentiation toward neural retina have been published (Llonch, Carido, & Ader, 2018). In principle, the protocols are based on the ability of the pluripotent stem cells to self-reorganize after dissociation, which, in combination with certain supplemented factors, can be specifically driven toward neural retina differentiation. Basic Protocol 1 below is based on a combination of Kuwahara et al. (2015) and Browne et al. (2017) with some modifications, and results in the generation of retinal organoids containing all seven retinal cell types arising in their natural order during differentiation. For phenotypic characterization, an immunostaining protocol (Basic Protocol 2), and for a detailed analysis of cell number, a quantitation protocol using Fiji (Basic Protocol 3) is provided.
Basic Protocol 1: DIFFERENTIATION PROTOCOL FOR 3D RETINAL ORGANOIDS
In this protocol, the stable and reproducible generation of 3D retinal organoids is described. The human embryonic stem cell line H9 is differentiated to 3D retinal organoids using a combination of two already published protocols (Browne et al., 2017; Kuwahara et al., 2015) with some modifications. This protocol describes the procedure for one 70%-80% confluent well of a 6-well plate. Briefly, 12,000 single cells are seeded into each well of a 96-well U-bottom plate (low-adhesion). The formed organoids are transferred to a 48-well flat-bottom plate coated with agarose at day 21 (d21), keeping one organoid per well during long-term culturing (>22 weeks), which prevents fusion of organoids and improves medium supply and monitoring of organoids. Using this protocol, retinal organoids with all seven retinal cell types are obtained. One has to be aware that bipolar and Müller glia cells are late-born cell types, and therefore will appear after d126 of culturing. However, at this time there will be no ganglion cells left, as they disappear over time, probably because of malnutrition (diffusion of the medium into the inner organoid decreases with time). Figures 1 and 2 illustrate the different stages of the differentiation process.
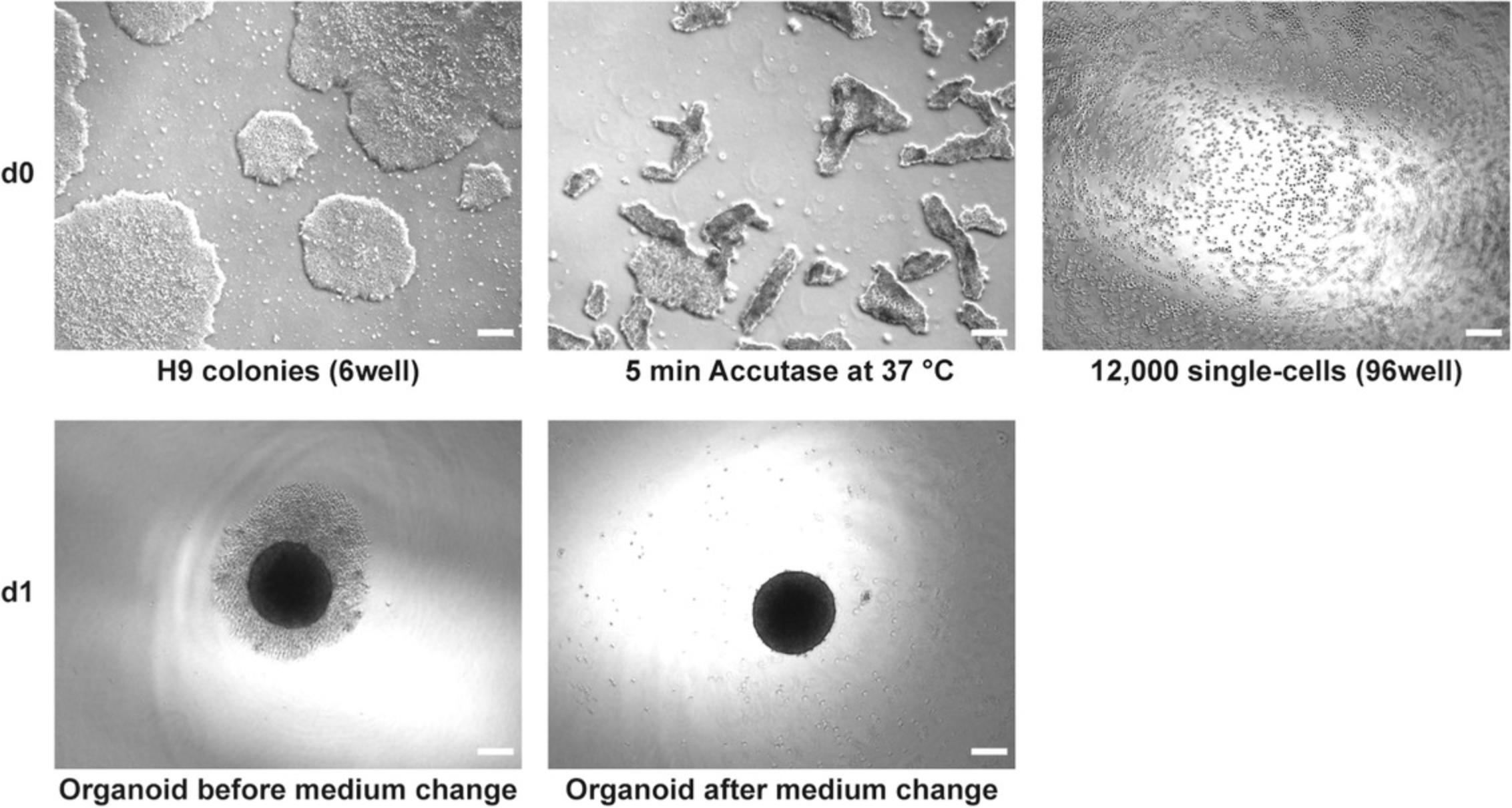

NOTE : All cell culture work is performed under sterile conditions in a laminar flow hood.
NOTE : All solutions and equipment that come into contact with living cells must be sterile.
NOTE : Cells and organoids should be incubated in a humidified tissue culture incubator at 37°C with 5% CO2 under normoxic conditions.
NOTE : This protocol is described for cells from one well of a 6-well plate, resulting in one 96-well U-bottom plate (low-adhesion) and later in two 48-well flat-bottom plates.
NOTE : Start on a Wednesday for weekend-free culturing.
NOTE : Before performing a medium change, the corresponding medium should be at room temperature.
Materials
-
Embryonic stem cell line H9 (WiCell, WA09; please note that restrictions on importation and use of hESCs might apply in some countries, e.g., Germany)
-
For reagents and consumables used in this protocol, see Tables 1 and 2, respectively
-
Additional reagents and equipment for basic cell culture techniques including counting cells with a hemocytometer and mycoplasma testing (see Current Protocols article: Phelan & May, 2017)
Item | Cat. no. | Supplier | Stock conc. | Final conc. |
---|---|---|---|---|
DMEM/F12-GlutaMAXTM Supplement | 31331-093 | ThermoFisher Scientific | – | – |
Y-27632 ROCK inhibitor | S1049 | Selleckchem | 10 mM | 20 µM |
Medium M0 | see recipe in Reagents and Solutions (Table 8) | |||
AggreWell medium | 5893 | StemCell Technologies | – | – |
D-PBS (1×) | 14190-169 | ThermoFisher Scientific | – | – |
StemPro Accutase | A11105-01 | ThermoFisher Scientific | – | – |
IWR1-endo | S7086 | Selleckchem | 10 mM | 3 µM |
SB-431542 | S1067 | Selleckchem | 20 mM | 10 µM |
LDN-193189 | S2618 | Selleckchem | 1 mM | 100 nM |
Medium M1 | see recipe in Reagents and Solutions (Table 8) | |||
Recombinant Human BMP4 | 314-BP/CF | R&D Systems | 50 µg/ml | 55 ng/ml |
Medium M2 | see recipe in Reagents and Solutions (Table 8) | |||
CHIR99021 | S1263 | Selleckchem | 5 mM | 3 µM |
SU5402 | SML0443-5MG | Sigma-Aldrich | 10 mM | 5 µM |
peqGOLD Universal Agarose | 732-2789 | VWR | 1% | 1% |
Water | 1.153.332.500 | Merck Millipore | – | – |
Medium M3 | see recipe in Reagents and Solutions (Table 8) | |||
Retinoic acid | R-2625-50 mg | Sigma-Aldrich | 100 µM | 0.5 µM |
Item | Cat. no. | Company |
---|---|---|
Nunclon-Sphera 96-well U-bottom plate, sterile, with lid | 174925 | ThermoFisher Scientific |
48-well flat-bottom suspension culture plate, sterile, with lid | 677102 | Greiner |
Reagent reservoir, sterile | 4-0016 | neoLab |
DISTRIMAN repetitive pipette | F164001 | Gilson |
DISTRITIP Maxi syringe tips, 12.5 ml | F164150 | Gilson |
d0
1.Prepare 4 ml DMEM/F12-GlutaMAXTM Supplement with 20 µM Y-27632 ROCK inhibitor.
2.Prepare 3 ml AggreWell medium with 20 µM Y-27632 ROCK inhibitor.
3.Prepare 10 ml of medium M0 as described in Reagents and Solutions (Table 8).
4.Wash the cells (one well of a 6-well plate) with 2 ml D-PBS (room temperature), aspirate, and add 1 ml Accutase to the well.
5.Incubate for 5-10 min at 37°C (in a humidified 5% CO2 incubator).
6.Place 2 ml of DMEM/F12-GlutaMAXTM Supplement with 20 µM Y-27632 ROCK inhibitor (prepared in step 1) in a 15-ml tube during the incubation in step 5.
7.Confirm under the microscope that all cells are detached (Fig. 1). After 10 min, all cells should be detached—do not incubate for longer.
8.Transfer cell suspension (1 ml) into the prepared tube (step 6) and rinse the well with 1 ml of the rest of DMEM/F12-GlutaMAXTM Supplement containing 20 µM Y-27632 ROCK inhibitor (the 15-ml tube contains now 4 ml). Avoid too much pipetting, as cells are very sensitive—transfer cells all at once and rinse one to two times.
9.Centrifuge 5 min at 300 × g , room temperature.
10.Aspirate supernatant and resuspend the cell pellet in 1 ml AggreWell medium containing 20 µM Y-27632 ROCK inhibitor (prepared in step 2). Do not pipette too much, as cells are very sensitive (this depends on the cell line used).
11.Count viable cells using a hemocytometer (see Current Protocols article: Phelan & May, 2017).
12.Prepare 8 ml cell suspension with 1.6 × 105 cells/ml using medium M0 (prepared in step 3).
13.Count viable cells again using a hemocytometer (optional).
14.Pipette 75 µl (12,000 cells) of cell suspension into each well of a 96-well U-bottom plate (Fig. 1) and place the plate in the incubator.
d1
15.Prepare 60 ml of medium M1 as described in Reagents and Solutions (Table 8). Add IWR1-endo, SB-431542, and LDN-193189 to 10 ml of M1 at final concentrations of 3 µM, 10 µM, and 100 nM, respectively, and perform a complete medium change on the cells from step 14.To do that, aspirate the medium carefully without destroying the built organoid and carefully add 100 µl of the prepared medium to each well using a repetitive pipette (Fig. 1).
16.Place the plate in the incubator until d6.
d6
17.Add BMP4 to 20 ml of M1 for a final concentration of 55 ng/ml and perform a complete medium change on the cells. Aspirate the medium carefully without destroying the organoid, and carefully add 200 µl of the prepared medium to each well using a repetitive pipette.
18.Place the plate in the incubator until d9.
d9 and d12
19.Perform a half-medium change on d9 as well as on d12 (on each day a total of 10 ml of medium M1 is needed). Carefully take off 100 µl medium without disturbing the organoid using a 200-µl pipette and carefully add 100 µl of medium M1 using a multichannel pipette and a reagent reservoir (add 10 ml of medium to the reagent reservoir).
20.Place the plate in the incubator until d12 and d15, respectively.
d15
21.Prepare 60 ml of medium M2 as described in Reagents and Solutions (Table 8).
22.Add CHIR99021 and SU5402 to 20 ml of M2 at final concentrations of 3 µM and 5 µM, respectively, and perform a complete medium change on the cells. Aspirate almost all medium carefully without disturbing the organoid, and carefully add 200 µl of the prepared medium to each well using a repetitive pipette.
23.Place the plate in the incubator until d16.
d16
24.Add CHIR99021 and SU5402 to 10 ml of M2 at final concentrations of 3 µM and 5 µM, respectively, and perform a half-medium change on the cells. Take off 100 µl of the medium carefully, without disturbing the organoid, using a 200-µl pipette, and carefully add 100 µl of the prepared medium to each well using a multichannel pipette and a reagent reservoir (add 10 ml of medium to the reagent reservoir).
25.Place the plate in the incubator until d19.
d19
26.Add CHIR99021 and SU5402 to 20 ml of M2 for final concentrations of 3 µM and 5 µM, respectively, and perform a complete medium change on the cells. Carefully aspirate as much as possible of the medium without destroying the organoid, and gently add 200 µl of the prepared medium to each well using a repetitive pipette.
27.Place the plate in the incubator until d21.
d21
28.Prepare 380 ml medium M3 as described in Reagents and Solutions (Table 8).
29.Coat two 48-well flat-bottom plates with 1% agarose dissolved in ultra-pure water. Weigh 1 g agarose and dissolve in 100 ml ultra-pure water, then boil in the microwave until agarose is completely dissolved. Let it cool down a bit and pipette 300 µl into each well. Add retinoic acid (RA) to 50 ml of medium M3 for a final concentration of 0.5 µM, and add 400 µl of the prepared medium to each well using a repetitive pipette when agarose has hardened (the rest of the prepared medium is needed in step 30).
30.Perform a complete medium change. Aspirate the medium carefully without destroying the organoid, and carefully add 100 µl of medium M3 containing freshly added 0.5 µM retinoic acid to each well of the 96-well U-bottom plate using a repetitive pipette (use 10 ml of the medium prepared in step 29).
31.Transfer each organoid to a well of the 48-well flat-bottom plates (step 29)—each organoid is kept separately. If you started with a full 96-well U-bottom plate at d0, you will need two 48-well flat-bottom plates at this step. For the transfer use a 200 µl pipette with tips that were cut (sterile; size of opening should be ∼3 mm as the organoids at this point have a size of ∼1-1.5 mm) so that the organoid will not be destroyed.
32.Place the plate in the incubator until d23.
d23
33.Add retinoic acid to 60 ml of medium M3 for a final concentration of 0.5 µM and perform a complete medium change every Monday, Wednesday, and Friday for long-term culturing until d152 or beyond. Aspirate the medium carefully without destroying the organoid and carefully add 600 µl of the prepared medium to each well using a repetitive pipette. The last addition of retinoic acid is on d119.
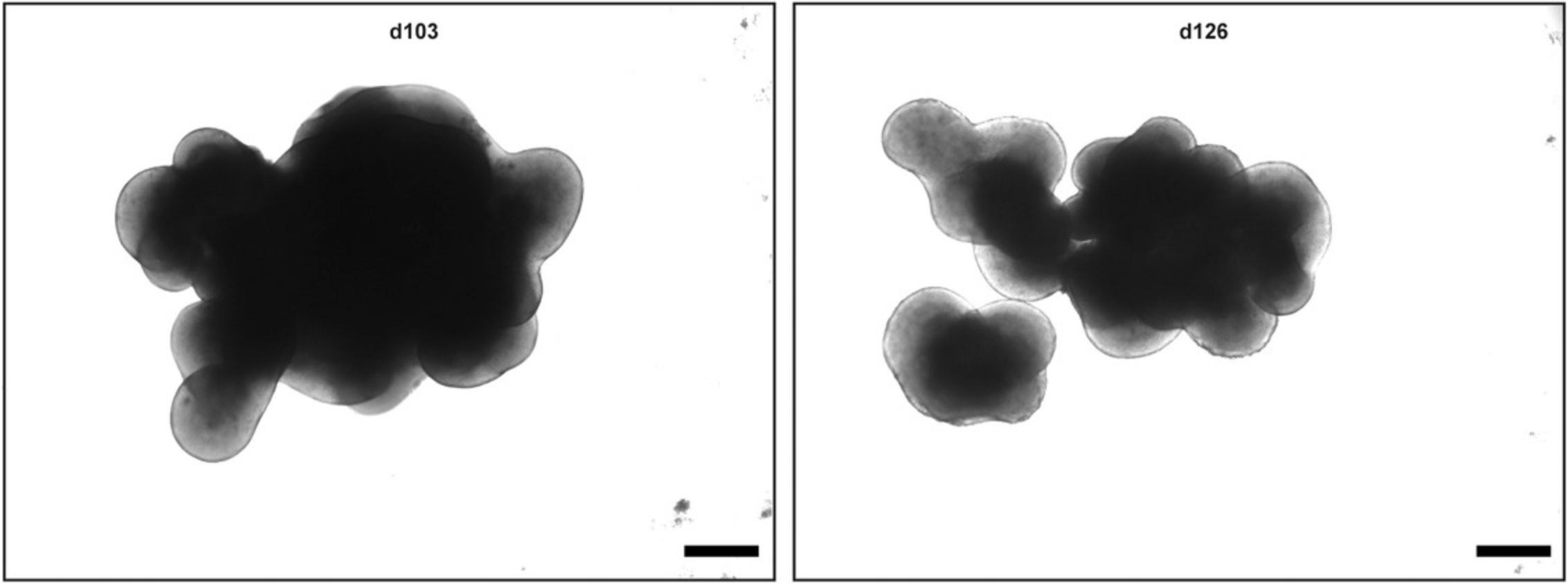
34.Harvest organoids for RNA and DNA analysis and embedding/immunofluorescent staining at desired time points during differentiation. Embed and section as in Support Protocol, then proceed to Basic Protocol 2.
Basic Protocol 2: IMMUNOSTAINING PROTOCOL FOR CRYOSECTIONS OF RETINAL ORGANOIDS
Detailed analysis of, e.g., cell composition, spatial cell organization, and cell proliferation in the in vitro–generated retinal organoids is performed by immunostaining of cryosections (which generally result in better detection of antigens compared to paraffin sections) of the organoids (Fischer, Jacobson, Rose, & Zeller, 2008; Hira et al., 2019). Presence, absence, localization, and distribution of certain cell types can be determined by using specific antibodies for marker proteins (Fig. 4). This protocol is based on Browne et al. (2017).
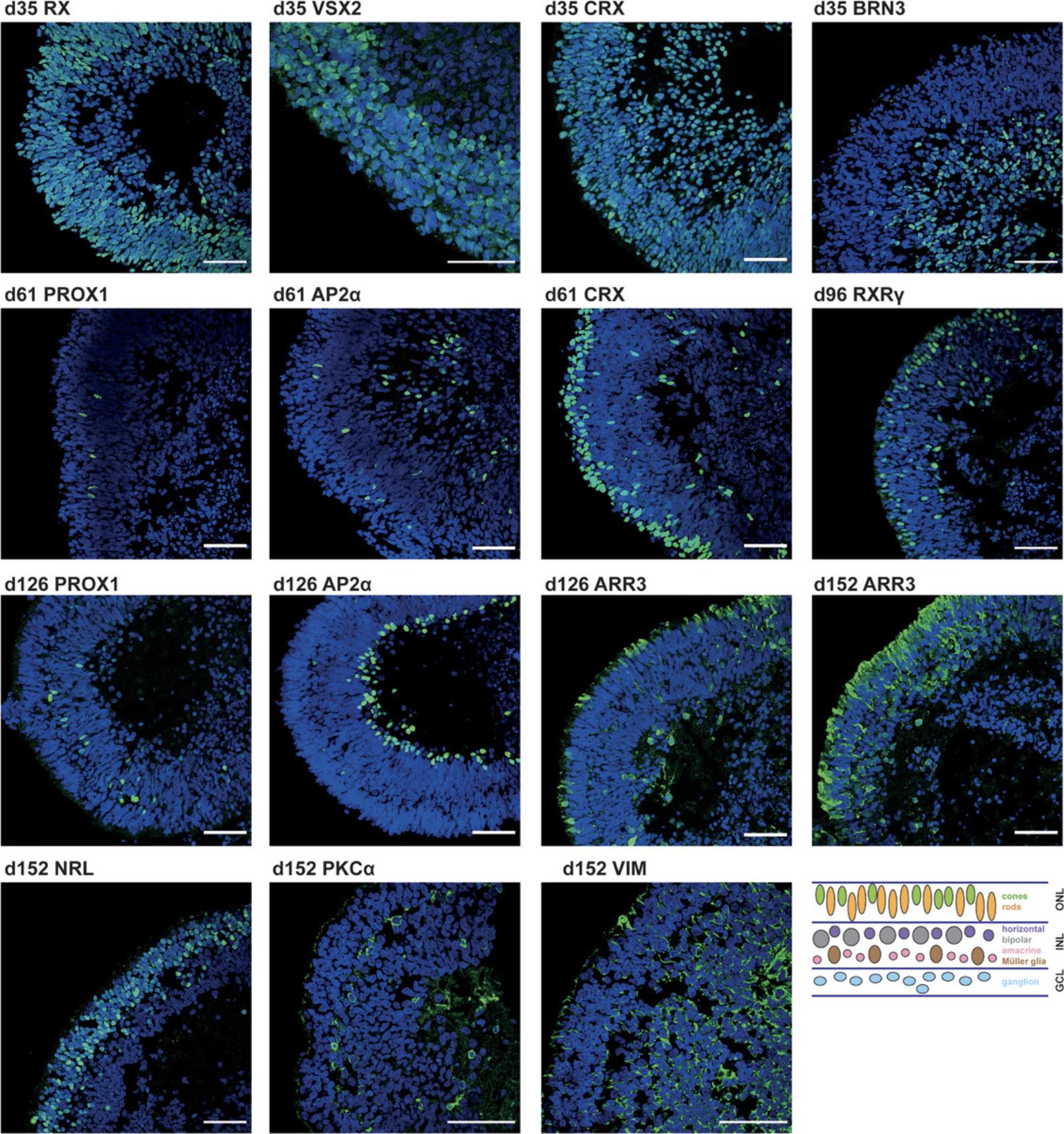
Materials
- Organoids, embedded and sectioned, on slides (Support Protocol)
- Primary antibodies (Table 3)
- Secondary antibodies (Table 4)
- For reagents and consumables used in this protocol, see Tables 5 and 6, respectively
Marker | Cell type | Antibody | Dilution | Host | |||
---|---|---|---|---|---|---|---|
RX (G-12) | Retinal progenitors | Santa Cruz Biotechnologies | sc-271889 | mc | RRID:AB_10708730 | 1:200 | mouse |
VSX2 | Retinal progenitors | Exalpha Biologicals | X1179P | pc | Not provided | 1:300 | sheep |
CRX (4G11) | PR progenitors, immature PRs | Abnova | H00001406-M02 | mc | RRID:AB_606098 | 1:1000 | mouse |
BRN3 (C-13) | Ganglion cells | Santa Cruz Biotechnologies | sc-6026a | pc | RRID:AB_673441 | 1:100 | goat |
PROX1 | Horizontal cells | Merck Millipore | AB5475 | pc | RRID:AB_177485 | 1:2000 | rabbit |
AP2α | Amacrine cells | DSHB | 3B5-s | mc | Not provided | 2 µg/ml | mouse |
RXRγ | Cones (early marker) | SpringBioscience | E4332 | pc | RRID:AB_11218714 | 1:500 | rabbit |
ARR3 | Cones | LifeSpan Biosciences | LS-C382134-100 | pc | Not provided | 1:250 | rabbit |
NRL | Rods (early marker) | R&D Systems | AF2945 | pc | RRID:AB_2155098 | 1:100 | goat |
PRKCA (H-7) | Bipolar cells | Santa Cruz Biotechnologies | sc-8393 | mc | RRID:AB_628142 | 1:100 | mouse |
VIM (E-5) | Müller glial cells | Santa Cruz Biotechnologies | sc-373717 | mc | RRID:AB_10917747 | 1:100 | mouse |
- a
Discontinued, but sc-390780 works also fine (monoclonal; clone A4; 1:100; mouse).
-
mc, monoclonal; pc, polyclonal; PR, photoreceptor.
Secondary antibody | Cat. no. | Company | Dilution | |
---|---|---|---|---|
Rabbit anti-Goat IgG (H+L), Alexa Fluor® 488 conjugate | A-11078 | RRID:AB_2534122 | ThermoFisher Scientific | 1:1000 |
Donkey anti-Sheep IgG (H+L), Alexa Fluor® 555 conjugate | A-21436 | RRID:AB_2535857 | ThermoFisher Scientific | 1:1000 |
Anti-mouse IgG (H+L), F(ab′)2 Fragment (Alexa Fluor® 488 Conjugate) | 4408S | RRID:AB_10694704 | Cell Signaling | 1:1000 |
Anti-Rabbit IgG (H+L), F(ab′)2 Fragment (Alexa Fluor® 488 Conjugate) | 4412S | RRID:AB_1904025 | Cell Signaling | 1:1000 |
Item | Cat. no. | Company |
---|---|---|
IMMEdge hydrophobic barrier PAP pen | H-4000 | VECTOR Laboratories |
4% paraformaldehyde (PFA) | see recipe in Reagents and Solutions (Table 8) | |
TBS-T | see recipe in Reagents and Solutions (Table 8) | |
Blocking buffer 1 | see recipe in Reagents and Solutions (Table 8) | |
Blocking buffer 2 | see recipe in Reagents and Solutions (Table 8) | |
DAPI (1 mg/ml in water) | 62248 | ThermoFisher Scientific |
TrueBlack Lipofuscin Autofluorescence Quencher, 20× in dimethylformamide (DMF) | B-23007 | Biotium |
Ethanol | 1.00983.2500 | Merck Millipore |
D-PBS (1×) | 14190-169 | ThermoFisher Scientific |
VECTASHIELD Mounting Medium | VEC-H-1000 | VECTOR Laboratories |
CoverGripTM Coverslip Sealant | 23005 | Biotium |
Article | Cat. no. | Company |
---|---|---|
Metal or plastic box with lid | – | – |
DISTRIMAN repetitive pipette | F164001 | Gilson |
DISTRITIP Maxi syringe tips, 12.5 ml | F164150 | Gilson |
1.Take the slides out of the −80°C freezer and place them on a 37°C heating plate for 15 min.
2.Prepare humid chamber in the meantime (metal or plastic box with lid, lined with tissues that are wetted with water).
3.Outline the sections with a pap pen and label the slides (three staining areas on a slide, Fig. 5A).
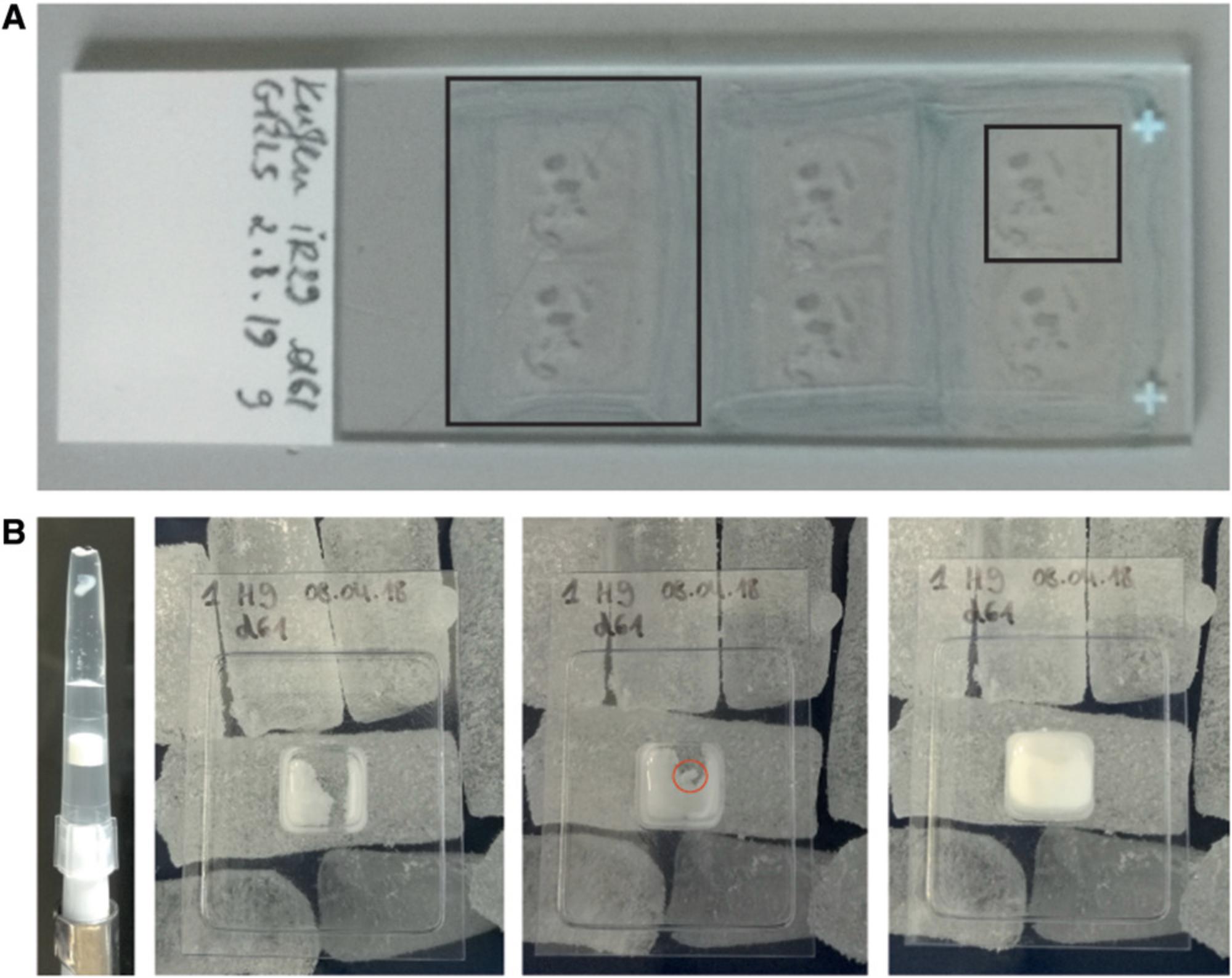
4.Post fix with 4% PFA for 5 min at room temperature (80 µl per section). Add the 4% PFA solution directly onto the organoids on the slide (Fig. 5A small black rectangle) and aspirate after 5 min.
5.Wash three times with TBS-T, each time for 5 min with 100 µl per staining area; use repetitive pipette; aspirate solution between washes.
6.Block for 1 hr with blocking buffer 1 at room temperature in the humid chamber (100 µl per staining area; use repetitive pipette).
7.Incubate overnight with primary antibody diluted in blocking buffer 1 at 4°C in the humid chamber (80 µl per staining area).
8.Wash three times with TBS-T, each time for 5 min with 100 µl per staining area; use repetitive pipette; aspirate solution between washes.
9.Incubate 1 hr with secondary antibody diluted in blocking buffer 2 at room temperature in the humid chamber (keep dark, 80 µl per staining area).
10.Wash twice with TBS-T, each time for 5 min with 100 µl per staining area protected from light; use repetitive pipette; aspirate solution between washes.
11.Wash once with TBS-T containing DAPI (1:1000 or final conc. 1 ng/µl) for 5 min protected from light (100 µl per staining area, use repetitive pipette, aspirate).
12.Treat with TrueBlack (dilute stock to 1× in ethanol; use 100 µl per staining area) for 30 s to reduce autofluorescence; aspirate and immediately wash with D-PBS (100 µl per staining area for 5 min, protected from light, do NOT use repetitive pipette).
13.Repeat wash step with D-PBS twice, protected from light (100 µl per staining area, use repetitive pipette, aspirate).
14.Coverslip the slide using VectaShield (∼5 µl per staining area) and seal with coverslip sealant.
15.Keep the slides at −20°C and in the dark until and after microscopic analysis.
Support Protocol: EMBEDDING AND SECTIONING PROTOCOL FOR 3D RETINAL ORGANOIDS
Good embedding is essential for good sectioning. If embedding is poorly executed, you will have trouble at the cryotome while sectioning the cryoblocks, as several or a whole series of sections can be torn, resulting in loss of information. This Support Protocol describes the individual steps to improve embedding and thus immunostaining.
Materials
- Organoids in wells of 48-well plate (Basic Protocol 1)
- Cryotome
- For reagents and consumables used in this protocol, see Table 7
Article | Cat. no. | Company |
---|---|---|
D-PBS (1×) | 14190-169 | ThermoFisher Scientific |
4% PFA | see recipe in Reagents and Solutions (Table 8) | |
Sucrose | 84097-1KG | Sigma-Aldrich |
Tissue-Tek O.C.T. compound | 4584 | Sakura |
Tissue-Tek cryomold (10 mm × 10 mm × 5 mm) | 4564 | Sakura |
Superfrost Plus microscope slides | J1800AMNZ | ThermoFisher Scientific |
1.Transfer organoid together with medium (100-200 µl) from the 48-well flat-bottom-plate to a 1.5-ml tube using cut 200-µl pipette tips to avoid destruction of the organoid (see Basic Protocol 1, step 31).
2.Let the organoid sink, and remove supernatant (medium) carefully. Wash once for 3 min with 1 ml D-PBS at room temperature. Let the organoid sink and remove D-PBS carefully.
3.Fix for 15 min at room temperature in 400 µl 4% PFA.
4.Wash three times, each time for 10 min with D-PBS.
5.Incubate in 1 ml of 30% sucrose in D-PBS at 4°C for ∼1 hr.
6.Aspirate supernatant, add ∼500 µl O.C.T. compound, and incubate for 30 min at room temperature.
7.Transfer the organoid into a fresh tube with 1 ml fresh O.C.T. compound. Avoid transfer of too much “old” O.C.T. compound. Use cut 200-µl pipette tips to avoid destroying the organoid (see Basic Protocol 1, step 31).
8.Label a tissue mold (10 mm × 10 mm), pipette ∼100 µl O.C.T. compound into it at room temperature, and place it on dry ice. As soon as the O.C.T. compound starts to freeze, transfer the organoid into the mold using a cut 200-µl pipette tip to avoid destroying the organoid (see Basic Protocol 1, step 31). Avoid air bubbles. Immediately fill up the rest of the tissue mold with fresh O.C.T. compound and let it freeze on dry ice (∼15-30 min; Fig. 5B).
9.Wrap up the tissue mold in aluminum foil that has been labeled, and keep the cryo-block at −80°C overnight.
10.Section the cryoblock using a cryotome (prepare 10-µm sections). As soon as you have reached the organoid, you will see white/yellowish tissue in the cryoblock and on the test slide. From this point on, place six sections on a labeled Superfrost slide, as shown in Figure 5A. Two sections will be one staining area (Fig. 5A). Store slides at −80°C until used for immunostaining.
Basic Protocol 3: QUANTITATION PROTOCOL USING FIJI
In order to use immunostaining to analyze possible differences regarding specific cell types, it is necessary to quantify either cell number or the area of specifically labeled fluorescence signals. This protocol describes how to quantify cell numbers using macros in Fiji (Schindelin et al., 2012). For quantitation, TIFF images are acquired on a confocal microscope. In this case, a Leica SP8 confocal laser scanning microscope is used, and according to experience, a pixel size of 150 nm is set as default. However, this may vary depending on the microscope used. To increase precision, at least four pictures are taken per organoid, with three organoids per differentiation and three biological replicates of differentiations (36 images in total). As the described macro is based on a DAPI mask, it is mandatory to take an image of the DAPI channel for each picture to make sure that only nucleated cells are included into the results.
On the DAPI channel image, a watershed algorithm will be performed which is only sufficient to separate sparsely touching cell-cell events in a single cell layer derived from, e.g., cell culture slides. However, in thicker tissue slices, more than one layer of cells will be present, and the density of nuclei will be too high for precise quantitation of single cells. The DAPI area will be eroded by a fixed factor to mimic the true size of a cell. Subsequently, a mask for the fluorescent signal of the immunostaining will be created by a fixed threshold value that is to be tested by the user for each condition. It is also important to adapt the macro for different cellular localizations of the fluorescence staining. In the next step, the fluorescence marker label will be compared with the previously created nuclei mask. Only signals within the DAPI mask will be used for final outputs. After thresholds have been determined for a staining, all images can be processed as a batch. The results table of the macro script will show absolute cell numbers and area values (integrated density). Depending on the type of slides/samples used—monolayer cell culture slides or tissue sections—the respective number can be used for calculations. Calculation of the ratio between the fluorescence area values and the DAPI area values results in cell quantitation on a percentage basis.
Materials
- Macro for cell quantitation (Supporting Information Macro S1)
1.Install the Fiji software including the Biovoxxel toolbox (Brocher, 2014, 2015).
2.Because the macro is provided as a .docx file, it needs to be converted to a .ijm file, which can be processed by Fiji. Open Fiji, then open the macro editing window by clicking on the tab “Plugins” in the task bar. Next, choose the tab “New” from the drop-down menu, and finally the tab “Macro.” Copy the text from the .docx file into the macro editing window. Click on the tab “Language,”select “IJ1 Macro,” and save the macro.
3.For automated cell counting, it is necessary to find the best threshold for each staining. Drag and drop the converted macro into the Fiji taskbar to open it in editing mode. Adjust the percentage for the threshold of your staining (line 91 of the macro). Start, for example, with a threshold of 95.The macro will hereby take only the brightest 5% of the signal into account. Run the macro and select a destination folder in which to save the results.
4.Make sure that the threshold suits the staining as exactly as possible by taking a close look at the resulting pictures. In the resulting pictures, the microscope image is overlaid with the mask that was created from the DAPI signal (cells/areas outlined in yellow; Fig. 6). Green lines indicate the signals that were counted as the brightest 5% of the signal in step 3.If the threshold is too low, many signals will not be counted (and therefore many fluorescent areas will not be encircled by green lines). If the threshold is too high, too much background will be counted as signal (negative areas will be encircled by green lines). Testing several different thresholds for each staining will help to find the perfect fit. It is also important to consider testing thresholds for all different time points of each staining of the experiment.
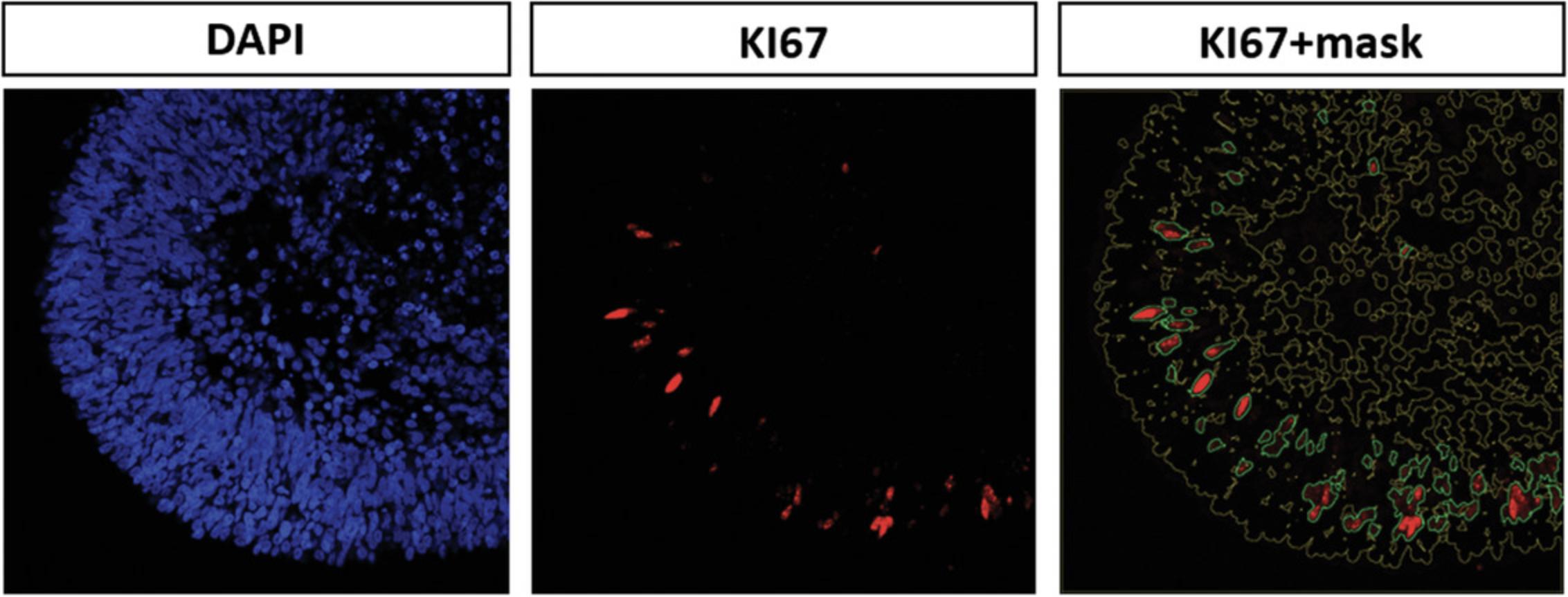
5.To adjust the pixel range for the particle size (defining the size of stained areas to be taken into account), proceed as described for the fluorescence signal threshold in step 3 (line 163 of the macro; here: 500 to infinity; Fig. 7). This step helps to discriminate between background, staining artifacts, and the actual fluorescence signal. Try different particle-size thresholds to find the one that fits for your analyses. If needed, adjust particle-size threshold for all channels separately.
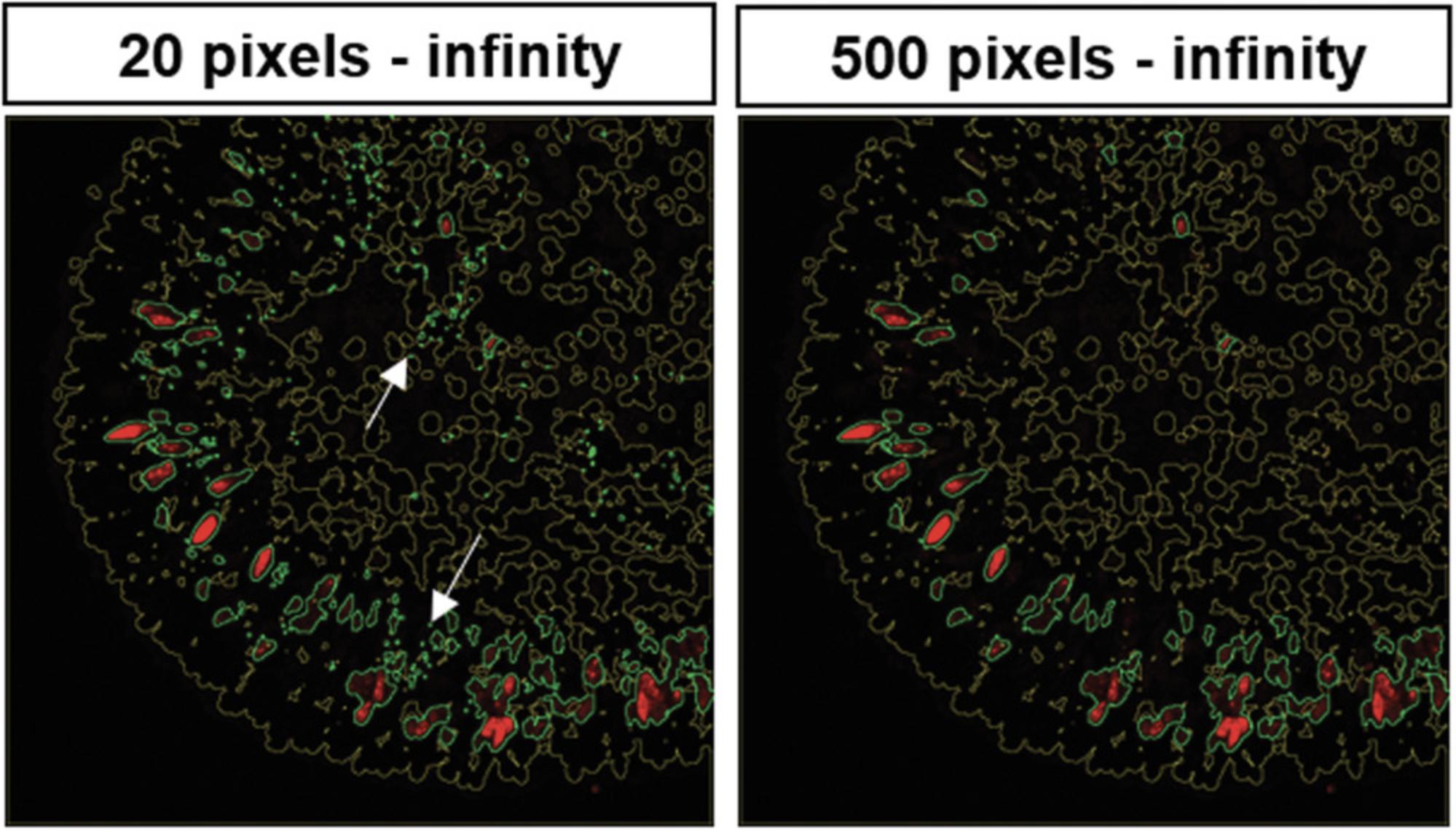
6.Before running the macro, create a folder for the results.
7.Run the macro with the adjusted thresholds for all pictures to be analyzed and select the destination folder.
8.A summary of the analyses will be saved as a text file in the results folder. Copy the data to Excel or any statistics program of choice.
9.One approach to calculating the percentage of immunofluorescence-positive cells among all nucleated cells is to use the area values of the DAPI signal and the area values of the fluorescent signal for calculation. In order to do this, the mean value of the DAPI signal of all 36 pictures is calculated. This value represents the average number of nucleated cells or the average area of nucleated cells.
10.Calculate the mean value of the fluroescent signal for each set of the four images per organoid. This will result in nine values (three organoids, three differentiations).
11.To calculate the percentage of cells positive for the fluorescent signal, each of the nine mean values for the fluorescent signal (calculated in step 10) is divided by the mean DAPI value (calculated in step 9) and the result is multiplied by 100. This set of nine percentage values can be used for statistics.
12.For quantitation of cells grown on cell culture slides, it would also be possible to directly use the number of counted fluorescence signals, which are listed in the results table (as absolute number of stained cells). In this case, cells need to be very evenly distributed in a single layer and should not be too close together. It is also necessary to make sure that there is only one fluorescent signal per nucleated cell (to be checked when adjusting the thresholds).
REAGENTS AND SOLUTIONS
Table 8 lists recipes for media and solutions used in this article.
Ingredient | Final conc. | Comment | |
---|---|---|---|
Blocking buffer 1a | |||
Donkey serum | Merck Millipore, S30-100ML | 5% | v/v |
Bovine serum albumin (BSA) | Sigma-Aldrich, A9418-10G | 3% | w/v |
Tris-buffered saline (TBS) | Prepare from Pierce 20× TBS buffer (ThermoFisher Scientific, 28358) | 1× | – |
Triton X-100 | Sigma-Aldrich, T9284-100ML | 0.2% | v/v |
Blocking buffer 2a | |||
Bovine serum albumin (BSA) | Sigma-Aldrich, A9418-10G | 2% | w/v |
Tris-buffered saline (TBS) | Prepare from Pierce 20× TBS buffer (ThermoFisher Scientific, 28358) | 1× | – |
Triton X-100 | Sigma-Aldrich, T9284-100ML | 0.2% | v/v |
M0 medium (day 0)b | |||
AggreWell medium | StemCell Technologies, 5893 | – | – |
Y-27632 ROCK inhibitor | Selleckchem, S1049 | 20 µM | – |
IWR1-endo | Selleckchem, S7086 | 3 µM | – |
SB-431542 | Selleckchem, S1067 | 10 µM | – |
LDN-193189 | Selleckchem, S2618 | 100 nM | – |
M1 medium (day 1 to day 15)c | |||
Hams F12, GlutaMAXTM Supplement | Gibco, 31765-027 | 45% | v/v |
IMDM, GlutaMAXTM Supplement | Gibco, 31980-022 | 45% | v/v |
KnockOut Serum Replacement (KSR) | ThermoFisher Scientific, 10828010 | 10% | v/v |
Chemically defined lipid concentrate | Gibco, 11905-031 | 1× | – |
Monothioglycerol | Sigma-Aldrich, M6145-25ML | 450 µM | – |
Penicillin-streptomycin | ThermoFisher Scientific, 15140122 | 100 U/ml penicillin; 100 µg/ml streptomycin | – |
IWR1-endo | Selleckchem, S7086 | 3 µM | Add briefly before use only on d1 |
SB-431542 | Selleckchem, S1067 | 10 µM | Add briefly before use only on d1 |
LDN-193189 | Selleckchem, S2618 | 100 nM | Add briefly before use only on d1 |
BMP4d | R&D Systems, 314-BP/CF | 55 ng/ml | Add briefly before use only on d6 |
M2 medium (day 15 to day 21)c | |||
DMEM/F12-GlutaMAXTM Supplement | ThermoFisher Scientific, 31331-093 | – | – |
N2 Supplement, 100× | ThermoFisher Scientific, 17502-148 | 1× | – |
Penicillin-streptomycin | ThermoFisher Scientific, 15140122 | 100 U/ml penicillin; 100 µg/ml streptomycin | – |
CHIR99021 | Selleckchem, S1263 | 3 µM | Add briefly before use |
SU5402 | Selleckchem, SML0443-5MG | 5 µM | Add briefly before use |
M3 medium (long-term medium)c | |||
DMEM/F12-GlutaMAXTM Supplement | ThermoFisher Scientific, 31331-093 | – | – |
Fetal bovine serum (FBS) | Gibco, 10270106 | 10% | v/v |
N2 Supplement, 100× | ThermoFisher Scientific, 17502-048 | 1× | – |
Penicillin-streptomycin | ThermoFisher Scientific, 15140122 | 100 U/ml penicillin; 100 µg/ml streptomycin | – |
Taurine | Sigma-Aldrich, T8691-25G | 0.1 mM | – |
Amphotericin B | Thermo Scientific, 15290018 | 0.25 µg/ml | – |
Retinoic acide | Sigma-Aldrich, R2625-50 mg | 0.5 µMe | Add briefly before use; last addition on d119 |
Paraformaldehyde (PFA), 4%a | |||
16% Formaldehyde | ThermoFisher Scientific, 28906 | 4% | v/v; dilute in D-PBS (ThermoFisher Scientific, 14190-169) |
Sucrose, 30%f | |||
Sucrose | 30% | w/v; dilute in D-PBS (ThermoFisher Scientific, 14190-169) | |
TBS-Tg | |||
Pierce 20× TBS Buffer | ThermoFisher Scientific, 28358 | 1× | – |
Triton X-100 | Sigma-Aldrich, T9284-100ML | 0.05% | v/v |
- a Store 1-ml aliquots at −20°C and use within 6 months.
- b Prepare fresh and use up at d0.
- c Store at 4°C and use within 2 weeks—before feeding the organoids keep at room temperature for 20-30 min.
- d Store aliquots of stock solution (50 µg/ml) at −20°C and use within 3 months.
- e Store aliquots of stock solution (100 µM) at −80°C and use within 3 months.
- f Store at 4°C and use within 4 weeks.
- g Store at room temperature and use within 4 weeks.
COMMENTARY
Background Information
The retina is a complex tissue consisting of seven different cells types that are arranged in certain layers. Retinal development starts from cells of the neuroectoderm. An optic vesicle evaginates form the rostral diencephalon and subsequently invaginates, building a double-walled optic cup (Fig. 8; Eiraku, Adachi, & Sasai, 2012). The outer wall of this optic cup forms the retinal pigment epithelium (RPE), whereas the inner wall forms the neural retina. In the early eye field, markers like RX, SIX3, SIX6, LHX2, and PAX6 are detected; later, these progenitor cells differentiate into the different neuronal cells (ganglion, horizontal, amacrine, photoreceptor, and bipolar cells) and glia cells (Müller glia cells) of the retina (Heavner & Pevny, 2012; Meyer et al., 2009). These different retinal cell types arise in a distinct order: the early-born cell types are ganglion, horizontal, and amacrine cells, as well as cone photoreceptors, whereas late-born cell types are rod photoreceptors, bipolar cells, and Müller glia cells. After their emergence, they migrate to their specific positions in the retina—photoreceptors form the outer nuclear layer; horizontal, bipolar, amacrine, and Müller glia cells form the inner nuclear layer; and the ganglion cells build the ganglion cell layer (Fig. 4; Centanin & Wittbrodt, 2014; Kapatai et al., 2013]).
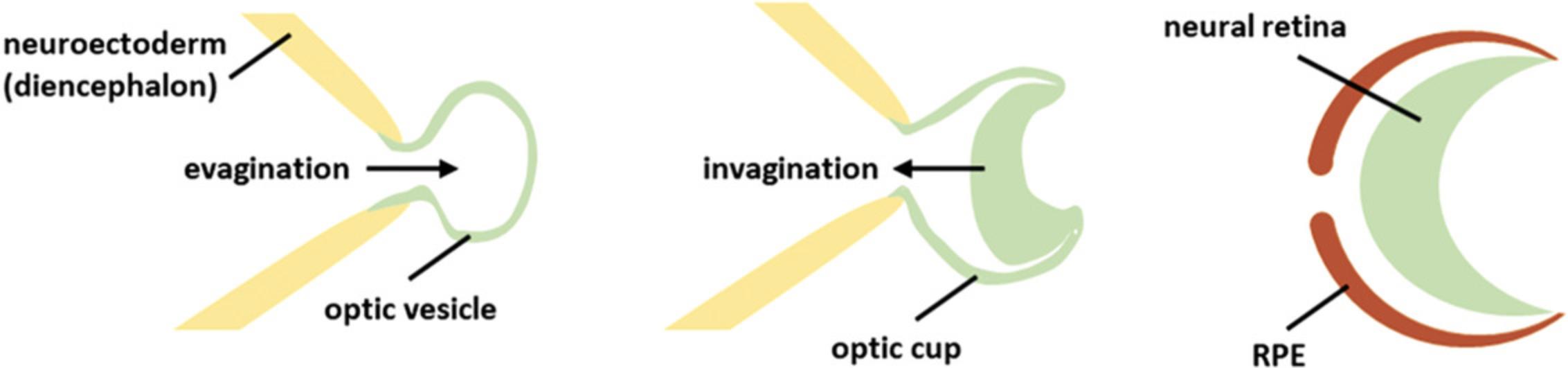
Organoid technology now makes it possible to study this process by modeling retinogenesis. Different protocols have been established, all based on the self-organizing nature of pluripotent stem cells. Nakano et al. (2012) published the first 3D differentiation protocol for retinal organoids from human embryonic stem cells. In this protocol, Matrigel was used, which is a complex protein mixture consisting of up to 1851 different proteins. The main component is laminin, which promotes formation of a rigid neuroepithelial structure (Eiraku & Sasai, 2012; Hughes, Postovit, & Lajoie, 2010). In 2012, Eiraku and Sasai showed that Matrigel induced the eye field in the generation of retinal organoids (Eiraku & Sasai, 2011, 2012). However, Matrigel shows lot-to-lot differences in protein composition, and therefore influences reproducibility. This problem was overcome with the protocol of Kuwahara et al. (2015), where the use of Matrigel is omitted. Instead, bone morphogenetic protein–4 (BMP4) is used to induce eye field formation. Moreover, in contrast to the Nakano protocol, no manual manipulation like excision of the neural retina from floating aggregates is necessary. Similar to what happens in vivo, retinal organoids develop all seven retinal cell types in a distinct order. However, when the latest-born cell types, the bipolar and Müller glia cells, arise, the earliest-born ganglion cells have already vanished (Capowski et al., 2019; Browne et al., 2017; Zhong et al., 2014). This is possibly due to malnutrition of the inner part of the organoid as the neural retina becomes thicker during differentiation and vascularization is missing, or due to apoptosis because no functional neuronal connections are formed. The layering in retinal organoids is close to the in vivo retina morphology. However, ectopic photoreceptors can be detected in the inner nuclear layer of the retina, which could be due to the lack of contributions from other missing structures (Capowski et al., 2019; Hallam et al., 2018; Phillips et al., 2018). Nevertheless, these stem cell–based retinal models are the best in vitro models for human retinogenesis at this time. 3D culturing in combination with patient-derived iPSCs or CRISPR/Cas technology enables models of genetic diseases, which are promising in terms of drug testing (Crespo et al., 2017; Deng et al., 2018; Jin, Okamoto, Xiang, & Takahashi, 2012; Parfitt et al., 2016; Tucker et al., 2013). Furthermore, organoids have the potential for transplantation therapies because, e.g., retinal organoids can serve as a source of certain retinal cell types to treat retinal degeneration (Gagliardi et al., 2018; Gonzalez-Cordero et al., 2017; Mandai et al., 2017; McLelland et al., 2018). Even personalized therapy might be possible, as the required retinal cell type can be generated from patient-specific iPSCs where gene correction was performed using CRISPR/Cas technology, eliminating rejection responses.
Critical Parameters and Troubleshooting
3D retina differentiation should only be set up with starting cells of good quality. Colonies should not show any signs of differentiation and should not be more than 70%-80% confluent. As a matter of course, the mycoplasma test at d0 should be negative. Quality of starting cell population is critical.
Supplements should be aliquotted into ready-to-use aliquots to avoid repeated thawing and freezing, and strict attention should be paid to shelf life. The medium should only be warmed at room temperature and never at 37°C. In addition, due to its undefined nature, which could hinder consistent differentiation, FBS should be tested before use. Differentiation up to d61 and immunostainings at d35 and d61 should be performed to determine whether the batch can be used for differentiation or not. To avoid repeated testing, purchase of a larger quantity of FBS from the tested batch should be considered.
Whether your differentiation is going in the right direction can be judged by light microscopy. If you see a bright outer layer that becomes thicker with time, the differentiation is correct (Fig. 2). On d19 and d21, you should see some loops forming. Thereafter, the layer starts thickening. If organoids or rather the outer bright layer do not grow, or other unusual characteristics appear, one should consider stopping that run of differentiation (see Supporting Information Figure S1). Of course, immunostaining for specific markers at that point should be considered to support the decision of ending the differentiation. Usually, if immunostaining at d35 stains positive for RX, VSX2, CRX, BRN3, and at d61 stains positive for CRX, PROX1, and AP2α, the differentiation is good and can be continued (Fig. 4).
Another point to consider is that different cell lines can behave differently, resulting in poor results (Bar-Nur, Russ, Efrat, & Benvenisty, 2011; Hiler et al., 2015; Mellough et al., 2019). In these cases, the differentiation protocol may be adjusted to the specific cell line (e.g., try different seeding densities). We have used the hESC line H9 for this protocol and have obtained stable and reproducible results.
Table 9 lists possible problems that can occur with the differentiation, as well as their possible causes and solutions.
Observation | Possible cause | Remarks |
---|---|---|
No organoid formed on d1 | Not enough cells | Make sure counting is correct and seed 12,000 cells per well |
Medium composition wrong |
Aliquot supplements in ready-to-use aliquots, do not refreeze Make sure Y-27632 ROCK inhibitor was added |
|
Cells of poor quality | No signs of differentiation should be visible, confluency not more than 70%-80% | |
No bright retinal layer visible at d12 | Media composition wrong; final concentration of small molecules wrong |
Aliquot supplements in ready-to-use aliquots, do not refreeze. Make sure the end concentration of all supplements used is correct. |
Organoids form atypical characteristics, e.g., cysts | Media composition wrong; final concentration of small molecules wrong |
Aliquot supplements in ready-to-use aliquots, do not refreeze Make sure the end concentration of all supplements used is correct Make sure that all supplements are added at the correct time point Make sure FBS batch was tested before |
Organoids lost by aspiration | Pump speed too high | Adjust the pump speed |
Too much autofluorescence | Wash not performed properly |
Be sure to perform all washing steps TrueBlack is an autofluorescence quencher that is used in tissue sections for immunofluorescence staining |
Understanding Results
Using this protocol, you should obtain retinal organoids with all seven retinal cell types that arise in their natural order and are organized in the known retinal layers, i.e., the ganglion cell layer, inner nuclear layer, and outer nuclear layer. At d1, an organoid is formed that develops a bright outer layer which is clearly visible at d12 (Figs. 1 and 2). This bright layer is the neural retina, and becomes thicker during differentiation (Fig. 2). The first-born retinal cell type is ganglion cells (d35, Fig. 4). These cells, however, disappear during differentiation as the retinal layer thickens and the supply of medium becomes poorer. At d61, horizontal cells, amacrine cells, and cone photoreceptors should be present. The rod photoreceptors arise a bit later and are present at d96. The bipolar cells and Müller glia cells are the late-born retinal cell types and will not be present before d126. It could be that you observe organoids falling apart into several small organoids (Fig. 3). All of these smaller organoids should still have a bright outer layer and stain positive for retinal markers.
Time Considerations
The 3D differentiation protocol needs at least 22 weeks and will result in a fully stratified neural retina—although at this time point ganglion cells will be missing for the aforementioned reason. If ganglion cells are the point of interest, following the protocol for 5 weeks is sufficient. Embedding the organoids will take 1 day, followed by 1 day for sectioning and 2 days for immunostaining.
Acknowledgments
We would like to thank Jennifer Aparicio and David Cobrinik (both affiliated with The Vision Center, Department of Surgery, Children's Hospital Los Angeles, Los Angeles, California, United States) for helpful discussions.
The monoclonal antibody AP2α, developed by T.J. Williams (University of Colorado, Denver) was obtained from the Developmental Studies Hybridoma Bank, created by the NICHD of the NIH, and maintained at The University of Iowa, Department of Biology, Iowa City, Iowa.
Open-access funding was enabled and organized by Projekt DEAL. This work was funded by the Deutsche Forschungsgemeinschaft (STE 1987/6-1) and partly by the Ernst und Berta Grimmke-Stiftung (9/18). Use of hESCs for this work has been reviewed and approved by the Central Ethical Review Board for Stem Cell Research at the Robert Koch Institut, Berlin, Germany (Az.3.04.02/0101) and the Ethical Review Board of the University Duisburg-Essen (16-7215-BO).
Supporting Information
Filename | Description |
---|---|
cpsc120-sup-0001-FigureS1.tif18.7 MB | Supporting Information Figure S1: Examples of unusual morphological characteristics during differentiation. The quality of organoid differentiation can be judged by light microscopy. d19: The bright outer layer (arrow) should be present and loops (arrowheads) should start to form. If the bright outer rim is absent (A), differentiation is not successful. d21: Formation of cysts (blue box) without a bright outer layer (B) or immoderate growth with formation of big loops (C) are atypical characteristics. x-mark: atypical/unusual characteristics; checkmark: typical characteristics. Scale bar: 500 µm. |
cpsc120-sup-0002-macro.docx18.2 KB | Supplementary Information Macro S1: Macro used for cell quantitation in Fiji. |
Please note: The publisher is not responsible for the content or functionality of any supporting information supplied by the authors. Any queries (other than missing content) should be directed to the corresponding author for the article.
Literature Cited
- Bar-Nur, O., Russ, H. A., Efrat, S., & Benvenisty, N. (2011). Epigenetic memory and preferential lineage-specific differentiation in induced pluripotent stem cells derived from human pancreatic islet beta cells. Cell Stem Cell , 9(1), 17–23. doi: 10.1016/j.stem.2011.06.007.
- Brocher, J. (2014). Qualitative and quantitative evaluation of two new histogram limiting binarization algorithms. International Journal of Image Processing (IJIP) , 8(2), 30–48.
- Brocher, J. (2015). The BioVoxxel Image Processing and Analysis Toolbox. EuBIAS Conference, 2015, Jan 5, Institut Curie, Paris, France.
- Browne, A. W., Arnesano, C., Harutyunyan, N., Khuu, T., Martinez, J. C., Pollack, H. A., … Cobrinik, D. (2017). Structural and functional characterization of human stem-cell-derived retinal organoids by live imaging. Investigative Ophthalmology & Visual Science, 58(9), 3311–3318. doi: 10.1167/iovs.16-20796.
- Capowski, E. E., Samimi, K., Mayerl, S. J., Phillips, M. J., Pinilla, I., Howden, S. E., … Gamm, D. M. (2019). Reproducibility and staging of 3D human retinal organoids across multiple pluripotent stem cell lines. Development (Cambridge, England) , 146(1), dev171686. doi: 10.1242/dev.171686.
- Centanin, L., & Wittbrodt, J. (2014). Retinal neurogenesis. Development , 141(2), 241–244. doi: 10.1242/dev.083642.
- Crespo, M., Vilar, E., Tsai, S. Y., Chang, K., Amin, S., Srinivasan, T., … Chen, S. (2017). Colonic organoids derived from human induced pluripotent stem cells for modeling colorectal cancer and drug testing. Nature Medicine , 23(7), 878–884. doi: 10.1038/nm.4355.
- Deng, W. L., Gao, M. L., Lei, X. L., Lv, J. N., Zhao, H., He, K. W., … Jin, Z. B. (2018). Gene correction reverses ciliopathy and photoreceptor loss in iPSC-derived retinal organoids from retinitis pigmentosa patients. Stem Cell Reports , 10(6), 2005. doi: 10.1016/j.stemcr.2018.05.012.
- Eiraku, M., & Sasai, Y. (2011). Mouse embryonic stem cell culture for generation of three-dimensional retinal and cortical tissues. Nature Protocols , 7(1), 69–79. doi: 10.1038/nprot.2011.429.
- Eiraku, M., & Sasai, Y. (2012). Self-formation of layered neural structures in three-dimensional culture of ES cells. Current Opinion in Neurobiology , 22(5), 768–777. doi: 10.1016/j.conb.2012.02.005.
- Eiraku, M., Adachi, T., & Sasai, Y. (2012). Relaxation-expansion model for self-driven retinal morphogenesis: A hypothesis from the perspective of biosystems dynamics at the multi-cellular level. Bioessays , 34(1), 17–25. doi: 10.1002/bies.201100070.
- Fischer, A. H., Jacobson, K. A., Rose, J., & Zeller, R. (2008). Cryosectioning tissues. CSH Protocols , 2008, pdb prot4991. doi: 10.1101/pdb.prot4991.
- Gagliardi, G., Ben M'Barek, K., Chaffiol, A., Slembrouck-Brec, A., Conart, J. B., Nanteau, C., … Goureau, O. (2018). Characterization and transplantation of CD73-positive photoreceptors isolated from human iPSC-derived retinal organoids. Stem Cell Reports , 11(3), 665–680. doi: 10.1016/j.stemcr.2018.07.005.
- Gonzalez-Cordero, A., Kruczek, K., Naeem, A., Fernando, M., Kloc, M., Ribeiro, J., … Ali, R. R. (2017). Recapitulation of human retinal development from human pluripotent stem cells generates transplantable populations of cone photoreceptors. Stem Cell Reports , 9(3), 820–837. doi: 10.1016/j.stemcr.2017.07.022.
- Hallam, D., Hilgen, G., Dorgau, B., Zhu, L., Yu, M., Bojic, S., … Lako, M. (2018). Human-induced pluripotent stem cells generate light responsive retinal organoids with variable and nutrient-dependent efficiency. Stem Cells , 36(10), 1535–1551. doi: 10.1002/stem.2883.
- Heavner, W., & Pevny, L. (2012). Eye development and retinogenesis. Cold Spring Harbor Perspectives in Biology , 4(12), a008391. doi: 10.1101/cshperspect.a008391.
- Hiler, D., Chen, X., Hazen, J., Kupriyanov, S., Carroll, P. A., Qu, C., … Dyer, M. A. (2015). Quantification of retinogenesis in 3D cultures reveals epigenetic memory and higher efficiency in iPSCs derived from rod photoreceptors. Cell Stem Cell , 17(1), 101–115. doi: 10.1016/j.stem.2015.05.015.
- Hira, V. V. V., de Jong, A. L., Ferro, K., Khurshed, M., Molenaar, R. J., & Van Noorden, C. J. F. (2019). Comparison of different methodologies and cryostat versus paraffin sections for chromogenic immunohistochemistry. Acta Histochemica , 121(2), 125–134. doi: 10.1016/j.acthis.2018.10.011.
- Hughes, C. S., Postovit, L. M., & Lajoie, G. A. (2010). Matrigel: A complex protein mixture required for optimal growth of cell culture. Proteomics , 10(9), 1886–1890. doi: 10.1002/pmic.200900758.
- Jin, Z. B., Okamoto, S., Xiang, P., & Takahashi, M. (2012). Integration-free induced pluripotent stem cells derived from retinitis pigmentosa patient for disease modeling. Stem Cells Translational Medicine , 1(6), 503–509. doi: 10.5966/sctm.2012-0005.
- Kapatai, G., Brundler, M. A., Jenkinson, H., Kearns, P., Parulekar, M., Peet, A. C., & McConville, C. M. (2013). Gene expression profiling identifies different sub-types of retinoblastoma. British Journal of Cancer , 109(2), 512–525. doi: 10.1038/bjc.2013.283.
- Kuwahara, A., Ozone, C., Nakano, T., Saito, K., Eiraku, M., & Sasai, Y. (2015). Generation of a ciliary margin-like stem cell niche from self-organizing human retinal tissue. Nature Communications , 6, 6286. doi: 10.1038/ncomms7286.
- Llonch, S., Carido, M., & Ader, M. (2018). Organoid technology for retinal repair. Developmental Biology , 433(2), 132–143. doi: 10.1016/j.ydbio.2017.09.028.
- Mandai, M., Fujii, M., Hashiguchi, T., Sunagawa, G. A., Ito, S. I., Sun, J., … Takahashi, M. (2017). iPSC-derived retina transplants improve vision in rd1 end-stage retinal-degeneration mice. Stem Cell Reports , 8(4), 1112–1113. doi: 10.1016/j.stemcr.2017.03.024.
- McLelland, B. T., Lin, B., Mathur, A., Aramant, R. B., Thomas, B. B., Nistor, G., … Seiler, M. J. (2018). Transplanted hESC-derived retina organoid sheets differentiate, integrate, and improve visual function in retinal degenerate rats. Investigative Ophthalmology & Visual Science, 59(6), 2586–2603. doi: 10.1167/iovs.17-23646.
- Mellough, C. B., Collin, J., Queen, R., Hilgen, G., Dorgau, B., Zerti, D., … Lako, M. (2019). Systematic comparison of retinal organoid differentiation from human pluripotent stem cells reveals stage specific, cell line, and methodological differences. Stem Cells Translational Medicine , 8(7), 694–706. doi: 10.1002/sctm.18-0267.
- Meyer, J. S., Shearer, R. L., Capowski, E. E., Wright, L. S., Wallace, K. A., McMillan, E. L., … Gamm, D. M. (2009). Modeling early retinal development with human embryonic and induced pluripotent stem cells. Proceedings of the National Academy of Sciences of the United States of America , 106(39), 16698–16703. doi: 10.1073/pnas.0905245106.
- Nakano, T., Ando, S., Takata, N., Kawada, M., Muguruma, K., Sekiguchi, K., … Sasai, Y. (2012). Self-formation of optic cups and storable stratified neural retina from human ESCs. Cell Stem Cell , 10(6), 771–785. doi: 10.1016/j.stem.2012.05.009.
- Parfitt, D. A., Lane, A., Ramsden, C. M., Carr, A. J., Munro, P. M., Jovanovic, K., … Cheetham, M. E. (2016). Identification and correction of mechanisms underlying inherited blindness in human iPSC-derived optic cups. Cell Stem Cell , 18(6), 769–781. doi: 10.1016/j.stem.2016.03.021.
- Phelan, K., & May, K. M. (2017). Mammalian cell tissue culture techniques. Current Protocols in Molecular Biology , 117, A.3F.1–A.3F.23. doi: 10.1002/cpmb.31.
- Phillips, M. J., Capowski, E. E., Petersen, A., Jansen, A. D., Barlow, K., Edwards, K. L., & Gamm, D. M. (2018). Generation of a rod-specific NRL reporter line in human pluripotent stem cells. Scientific Reports , 8(1), 2370. doi: 10.1038/s41598-018-20813-3.
- Schindelin, J., Arganda-Carreras, I., Frise, E., Kaynig, V., Longair, M., Pietzsch, T., … Cardona, A. (2012). Fiji: An open-source platform for biological-image analysis. Nature Methods , 9(7), 676–682. doi: 10.1038/nmeth.2019.
- Tucker, B. A., Mullins, R. F., Streb, L. M., Anfinson, K., Eyestone, M. E., Kaalberg, E., … Stone, E. M. (2013). Patient-specific iPSC-derived photoreceptor precursor cells as a means to investigate retinitis pigmentosa. Elife , 2, e00824. doi: 10.7554/eLife.00824.
- Zhong, X., Gutierrez, C., Xue, T., Hampton, C., Vergara, M. N., Cao, L. H., … Canto-Soler, M. V. (2014). Generation of three-dimensional retinal tissue with functional photoreceptors from human iPSCs. Nature Communications , 5, 4047. doi: 10.1038/ncomms5047.
Internet Resources
Fiji website.
Biovoxxel website.
Citing Literature
Number of times cited according to CrossRef: 9
- Kotoe Kawai, Margaret T. Ho, Yui Ueno, Dhana Abdo, Chang Xue, Hidenori Nonaka, Hiroyuki Nishida, Yoichi Honma, Valerie A. Wallace, Molly S. Shoichet, Hyaluronan improves photoreceptor differentiation and maturation in human retinal organoids, Acta Biomaterialia, 10.1016/j.actbio.2024.05.001, 181 , (117-132), (2024).
- Sudhiksha Maramraju, Andrew Kowalczewski, Anirudh Kaza, Xiyuan Liu, Jathin Pranav Singaraju, Mark V. Albert, Zhen Ma, Huaxiao Yang, AI‐organoid integrated systems for biomedical studies and applications, Bioengineering & Translational MedicineBioengineering & Translational MedicineBioengineering & Translational Medicine, 10.1002/btm2.10641, 9 , 2, (2024).
- Davinia Beaver, Ioannis Jason Limnios, A treatment within sight: challenges in the development of stem cell-derived photoreceptor therapies for retinal degenerative diseases, Frontiers in Transplantation, 10.3389/frtra.2023.1130086, 2 , (2023).
- Xihao Sun, Zekai Cui, Yuqin Liang, Chunwen Duan, Hon Fai Chan, Shengru Mao, Jianing Gu, Chengcheng Ding, Xu Yang, Qing Wang, Shibo Tang, Jiansu Chen, One-stop assembly of adherent 3D retinal organoids from hiPSCs based on 3D-printed derived PDMS microwell platform, Biofabrication, 10.1088/1758-5090/acc761, 15 , 3, (035005), (2023).
- Jiahui Kang, Jing Gong, Cao Yang, Xi Lin, Lijuan Yan, Yu Gong, Haiwei Xu, Application of Human Stem Cell Derived Retinal Organoids in the Exploration of the Mechanisms of Early Retinal Development, Stem Cell Reviews and Reports, 10.1007/s12015-023-10553-x, 19 , 6, (1755-1772), (2023).
- Deniz Kanber, Julia Woestefeld, Hannah Döpper, Morgane Bozet, Alexandra Brenzel, Janine Altmüller, Fabian Kilpert, Dietmar Lohmann, Claudia Pommerenke, Laura Steenpass, RB1-Negative Retinal Organoids Display Proliferation of Cone Photoreceptors and Loss of Retinal Differentiation, Cancers, 10.3390/cancers14092166, 14 , 9, (2166), (2022).
- Ellie Wagstaff, Andrea Heredero Berzal, Camiel Boon, Peter Quinn, Anneloor ten Asbroek, Arthur Bergen, The Role of Small Molecules and Their Effect on the Molecular Mechanisms of Early Retinal Organoid Development, International Journal of Molecular Sciences, 10.3390/ijms22137081, 22 , 13, (7081), (2021).
- Yu. V. Markitantova, V. N. Simirskii, The Role of the Purinergic Signaling System in the Control of Histogenesis, Homeostasis, and Pathogenesis of the Vertebrate Retina, Russian Journal of Developmental Biology, 10.1134/S1062360421060084, 52 , 6, (430-448), (2021).
- Daniel Besser, Advancing Stem Cell Technologies and Applications: A Special Collection from the PluriCore Network in the German Stem Cell Network (GSCN), Current Protocols in Stem Cell Biology, 10.1002/cpsc.129, 55 , 1, (2020).